- 1UNESP, Department of Soil Science, College of Agricultural Science, Botucatu, Brazil
- 2NIOO-KNAW, Department of Microbial Ecology, Netherlands Institute of Ecology, Wageningen, Netherlands
- 3UNESP, Department of Soil Science, College of Agricultural Science, Botucatu, Brazil
- 4USDA, Agricultural Research Service, NCSU Campus, Raleigh, NC, United States
- 5IAC, Soils and Environmental Resources Center, Instituto Agronômico de Campinas, Campinas, Brazil
Intercropping forage grasses with upland rice is an alternative cropping system to improve agroecosystem diversification and could potentially enhance sustainability in tropical regions. However, nitrogen (N) immobilization and nutrient competition between rice and forage grasses could reduce rice grain yield and decrease overall productivity. Therefore, fertilizer N requirements of upland rice intercropped with forage grasses needs to be better defined. Field experiments were carried out during three growing seasons on a Typic Haplorthox soil in São Paulo state of Brazil. The experimental design was a randomized block design with a 3 × 4 factorial scheme with four replications. Treatments were cropping system [monocropped rice (Oryza sativa L.), rice intercropped with palisadegrass (Urochloa brizantha), and rice intercropped with guineagrass (Megathyrsus maximus) and sidedress N application rate (0, 40, 80, and 120 kg N ha−1). Intercropped grasses were sown between upland rice rows 30 days after rice emergence. On average, intercropping of rice with palisadegrass or guineagrass decreased rice shoot dry matter and grain yield by 11% and milled rice productivity by 10% compared with monocropped rice. Grain yield, grain protein, and milled productivity of rice increased as N application rate increased. Forage dry matter production (first and second cut) and crude protein (second cut) were greatest in the rice + palisadegrass intercropping system. Production of both forage grasses increased with up to 80 kg N ha−1 in the first cut and increased linearly with N in the second cut. Intercropping of rice with palisadegrass or guineagrass with 80 kg N ha−1 application resulted in the greatest land equivalent ratio (1.96 and 1.55, respectively). Relative N yield was greatest at 120 kg N ha−1 (220 and 173%, respectively). Although rice monocropping had greatest grain yield, intercropping systems with forage grasses were more favorable from both economic and environmental perspectives by enhancing plant diversification, nutrient cycling with forage grasses, land use production per unit area, and profitability throughout the year.
Introduction
Rice (Oryza sativa L.) is a staple food consumed by more than half of the world's population (Emerick and Ronald, 2019) and one of the three most important cereal crops globally (Africa Rice Center - AfricaRice, 2019; Yadav et al., 2019). Rice cultivation is particularly prominent in Asia, Africa, and the Americas (CGIAR Science Council, 2006). The Cerrado of Brazil is the world's largest producer of upland rice (Silva et al., 2020), a region where soils are acidic and of low fertility (Allen et al., 2007). Low-level technology use by farmers and summer dry spells greatly restricts plant development and upland rice yields (Nascente et al., 2013).
A common agricultural practice in tropical regions is to cultivate upland rice in degraded pastures for two growing seasons before returning to pasture (Kluthcouski et al., 2000). Upland rice tolerates high soil acidity and exchangeable aluminum, which are frequent characteristics of degraded soils (Fageria, 1998). However, cropping systems based on conservation management with crop rotation, intercropping, integrated crop-livestock system (ICLS), and no-tillage system (NTS) are recommended to reduce soil degradation. These systems provide great efficiency in preserving natural resources and sustaining high agricultural production in tropical regions (Borghi et al., 2014; Crusciol et al., 2015; Moraes et al., 2019).
Intercropping upland rice with tropical forage grasses may be an excellent option to improve crop diversity and soil quality, particularly in tropical soils with low fertility and dry winters (Allen et al., 2007; Wood and Mendelsohn, 2014). In many regions of the world, including the tropics, ICLS may be a suitable alternative to improve food production and decrease poverty (Food and Agriculture Organization of the United Nations (FAO), 2017). Because ICLS reduces the need to cultivate new agricultural areas, these systems are considered more sustainable (Surve and Arvadia, 2011) and promote crop production diversity in the same area (Crusciol et al., 2014; Mateus et al., 2016).
Studies of intercropping systems have assessed yields of corn (Borghi et al., 2013a; Crusciol et al., 2013), sorghum (Crusciol et al., 2011; Borghi et al., 2013b), and soybean (Crusciol et al., 2012, 2014) in the summer season (Surve and Arvadia, 2011) and biomass production of tropical grass during the off-season (Pariz et al., 2017; Mateus et al., 2020). These studies aim to develop better management practices for increasing plant development and decreasing competition between intercropped species, thereby increasing yields (Crusciol et al., 2014; Pariz et al., 2016; Moraes et al., 2019; Mateus et al., 2020). However, information on N management of upland rice when intercropped with forage grasses in tropical regions is scarce.
Nitrogen is the most important nutrient impacting development and yield of rice, and its dynamics in the soil-plant system vary according to soil conditions and fertilizer management strategy (Fageria et al., 2011; Nascente et al., 2013). Increasing N use efficiency in agroecosystems is an ongoing goal to improve agricultural sustainability, promote high revenue per area, and increase upland rice yield (Nascente et al., 2013). In ICLS, cash crops and forage grasses are intercropped in the summer season, followed by forage grass production with animal grazing in the off-season (Crusciol et al., 2014, 2016; Franzluebbers and Stuedemann, 2014; Moraes et al., 2019). Successive grass-only cultivation can compromise the sustainability of ICLS due to soil N depletion through crop N removal (Garcia et al., 2016). Low N fertilizer recovery efficiency is common in grass crops. Optimal N fertilizer application rate varies according to soil conditions, crop technological level, and type of crop rotation with or without leguminous crops that fix atmospheric N (Borghi et al., 2014; Crusciol et al., 2016). Therefore, improved N fertilizer recommendations in intercropped systems, particularly those involving upland rice, are needed.
We hypothesized that intercropping systems can (i) increase biomass production of rice in the summer season and forage grasses in the off-season in tropical regions and, as a consequence, (ii) improve land-use efficiency compared with monocropped systems. Beyond that, (iii) better management of N fertilizer rate in intercropping systems could reduce the competition between intercropped forage grass and upland rice and improve the whole system of food production. Thus, the first objective was to compare the feasibility of production between monocropped rice and rice intercropped with the two most commonly used forage species (palisadegrass and guineagrass). Response variables included production and quality of rice, pasture production, land-use efficiency, and estimated meat production during three growing seasons. The second objective was to determine the most appropriate sidedress N rate for monocropped rice and rice intercropped with tropical grasses relative to food production, land use, competition factors, and economic aspects of the systems.
Materials and Methods
Site Description
Field experiments were conducted during 2011–2012, 2012–2013, and 2014–2015 growing seasons in Botucatu, State of São Paulo, southeastern Brazil (48° 26′ W, 22° 51′ S; elevation of 740 m above sea level). The soil is classified as a clayey, kaolinitic, thermic Typic Haplothox (United States Department of Agriculture (USDA), 2014) with 630, 90, and 280 g kg−1 of clay, silt, and sand, respectively. At the beginning of the experiment, soil (0–0.2 m depth) was sampled to evaluate chemical characteristics (Supplementary Table 1) according to the methods of van Raij et al. (2001). The climate is Cwa, humid subtropical zone, with dry winters and hot summer, according to the Köppen climate classification system. Temperature and rainfall during the experimental period are reported in Supplementary Figure 1. Long-term (1956–2016) mean annual minimum and maximum temperatures are 15 and 26°C, respectively, with mean annual precipitation of 1,359 mm (Unicamp, 2016).
Each field experiment was performed in a new field previously cultivated with corn (Zea mays L.). Management history was NTS for 6 years. Prior to the 2011–2012 growing season, the field had previous crop history going back in time of corn, fallow/soybean [Glycine max (L.) Merr.], black oat (Avena strigosa)/common bean (Phaseolus vulgaris L.), black oat/soybean, palisadegrass (Urochloa brizantha)/corn, palisadegrass/corn, and black oat/soybean. Prior to the 2012–2013 growing season, previous crop history was corn, castor bean (Ricinus communis L.)/common bean, forage grass/corn, forage grass/corn, forage grass/corn, black oat/soybean, and black oat/corn. Prior to the 2013–2014 growing season, previous crop history was corn, black oat/corn, black oat/soybean, black oat/corn, black oat/soybean, black oat/corn, and black oat/soybean.
Experimental Design and Treatments
The experimental design was a randomized complete block with a 3 × 4 factorial scheme and four replications implemented each of the three growing seasons. The three cropping systems were: monocropped rice, rice intercropped with palisadegrass, and rice intercropped with guineagrass (Supplementary Figure 2). Four sidedress N application rates were: 0, 40, 80, and 120 kg N ha−1, applied as ammonium nitrate. Each plot was 3.4 × 20 m. Buffer area of 0.5–0.7 m along each plot edge was not sampled.
Crop Management
The upland rice cultivar BRS Monarca was sown on Nov. 21 (2011–12), Nov. 21 (2012–13), and Nov. 22 (2014–15) at a depth of 3 cm and row spacing of 0.34 m at a density of 200 viable seeds m−2 using no-till seeding (Semeato, Model Personale Drill 13, Passo Fundo, RS, Brazil). For all treatments, the basic fertilization in the sowing furrow consisted of 20 kg N ha−1 as urea, 50 kg P2O5 ha−1 as triple superphosphate, and 50 kg K2O ha−1 as potassium chloride (Cantarella et al., 1997). Sidedress N fertilization rates were applied at tillering growth stage of upland rice (Supplementary Figure 2). Upland rice was cultivated according to crop needs.
At the time of sidedress N application, intercropping systems were sown with palisadegrass (Urochloa brizantha cv. Marandu) and guineagrass (Megathyrsus maximum) at densities of 15.3 and 15.9 kg ha−1 seed (34% viable seeds), respectively, with the same no-till drill in between all rows of rice. At the same time, replicated same-size plots of palisadegrass and guineagrass were sown solely as controls to calculate an intercropping competition factor.
Sampling and Analyses of Rice and Tropical Forage Grasses
Upland rice leaf samples were collected for nutrient concentration analysis when 50% of the panicles in each plot were at flowering stage. The selection was randomized by collecting 20 flag leaves of plants per plot (Cantarella et al., 1997). Leaf samples were dried by forced-air circulation at 65°C for 72 h and ground to pass a 0.85-mm stainless-steel sieve. Samples were digested with sulfuric acid for N determination and with a nitro-perchloric solution for P, K, Ca, Mg, and S determinations. Concentrations of N, P, and S were determined from digested solutions by semi-micro-Kjeldahl distillation, colorimetry, and turbidimetry methods, respectively. Concentrations of K, Ca, and Mg were determined by atomic absorption spectrophotometry (Malavolta et al., 1997).
At time of rice leaf sampling, shoots of plants from 1.0 m rows of the two central rows were cut at soil level for the determination of shoot dry matter (DM) of rice. Shoots were dried by forced-air circulation at 65°C for 72 h, weighed, and data extrapolated to Mg ha−1. The following parameters were evaluated 85 days after rice emergence: number of panicles per m2 (by counting the number of panicles in an area of 2.0 m in the two rows from the usable area of each plot), total number of spikelets per panicles (by counting the number of spikelets in 20 panicles in the useable area), spikelet fertility (calculated using the following function: number of grain-bearing spikelets/total number of spikelets per panicle × 100), and 1,000-grain weight (evaluated by randomly collecting and weighing four samples of 1,000 grains from each plot adjusted to a moisture content of 130 g kg−1). Plants were manually harvested and panicles were dried in the sun for 2 days and later subjected to mechanical threshing using a research plot thresher. Rice grain yield was determined from unhulled grain weight, correcting moisture content to 130 g kg−1, and converting to Mg ha−1. Nitrogen-use efficiency (NUE) was defined as the increase in grain yield per unit of N applied (Fageria et al., 2005), which was determined by dividing the difference between the grain yield (kg ha−1) in each N treatment and the grain yield of the control (no N application) by each N rate (kg ha−1).
For industrial quality and milled rice productivity, 100-g sample of rice was collected from each plot and processed for 1 min in a proof mill for milling yield determination. Polished grains were weighed and calculated as percentage of total grain weight. Polished grains were placed in a grain sorting machine for 30 s to determine broken and unbroken kernels (i.e., milling fraction). Milling fraction was unbroken kernel yield divided by total grain weight. Milled rice productivity was calculated as: total grain yield × milling fraction (expressed as kg ha−1). Grain protein (g kg−1) was calculated from total N concentration of grain samples from Kjeldahl digestion multiplied by 5.95.
Palisadegrass and guineagrass DM production was evaluated at 60 days (first cut) and 150 days (second cut) after upland rice harvest. All forage from 2 m2 per plot was cut with a mechanical rotary mower at 0.25 m from the soil surface. Forage was dried by forced air circulation at 65°C for 72 h, weighed, and data extrapolated to Mg ha−1. Crude protein of first and second cut was calculated from total N concentration of forage samples from Kjeldahl digestion multiplied by 6.25 (Malavolta et al., 1997).
Statistical Analyses
All data were initially tested for normality using the Shapiro-Wilk test from the UNIVARIATE procedure of SAS v. 9.4 (SAS Inst. Inc., Cary, NC); all data were distributed normally (W ≥ 0.90). Data were then analyzed using the MIXED procedure and the Satterthwaite approximation. Cropping system, sidedress N application rate, and resultant interactions were considered fixed effects. Block was a random variable. Growing season and its interaction with cropping system and sidedress N application rate were not significant at P < 0.05 for any of the dependent variables. Therefore, data were combined across growing seasons. Results were reported as least square means and separated using the probability of differences option (PDIFF).
Regressions of variables on the four rates of N fertilizer were tested across the replications of growing seasons. All data were fit to the non-linear models of quadratic function and, when the non-linear equation resulted an unrealistically outcome, a linear regression was fitted to data. Effects were considered significant at P < 0.05. Error bars are presented as standard error (SE) and the regressions were calculated using the SigmaPlot v. 14.0 (Systat Inc., San Jose, CA).
Economic Evaluation and Estimated Meat Production
Cost per hectare to produce each crop was calculated for each treatment (CONAB, 2018). The only differences among treatments were forage seed cost and sidedress N application cost. Return value of rice grain production was calculated using prices in US$.
Although we did not have livestock graze the palisadegrass and guineagrass after rice harvest, meat production was estimated using the Large Ruminant Nutrition System (LRNS; http://nutritionmodels.tamu.edu/lrns.html) model based on the Cornell Net Carbohydrate and Protein System (CNCPS) v. 5, according to Fox et al. (2004). To predict energy and protein requirements, performance and dry matter intake by 450 kg Nellore bull cattle in a continuous grazing system were used and assumed to produce 52% carcass yield with 22% Body Fat Grading System. Forage nutritive values of palisadegrass and guineagrass in the intercropping system with specific sidedress N application rate were used to predict performance of each treatment.
Dry matter intake was assumed as 9.9–10.0 kg DM day−1. Due to the high forage crude protein (CP) concentration (9.5–14.4%), average daily gain (ADG) was based on metabolizable energy and protein to estimate meat production. Dry matter herbage allowance was double the amount of DM intake by individual cattle, considering a grazing efficiency of 60%, according to Braga (1983).
Animal grazing time was considered to be 150 days, assuming 112 days for rice production, 60 days forage accumulation period prior to stocking, and 43 days after animal grazing for biomass regrowth and desiccation to allow sufficient surface residue accumulation for effective ICLS management. Stocking rate was estimated from forage DM production, time available for grazing, DM intake by individual cattle, and grazing efficiency. Stocking rate was multiplied by ADG, time of animal grazing, and carcass yield (52%) to estimate total meat production per hectare averaged across the three growing seasons during off-season (150 days per year). Meat was produced in the fall-winter period, after the rice crop was harvested.
Gross revenue per hectare was calculate by the formula: (price per kg × rice yield) + (price per kg × estimated meat production). Net return per ha was calculated by the formula: (gross revenue ha−1 minus cost ha−1). Unit values were from the Brazilian national average over the last 5 years and we converted these values to US dollars (Agrolink, 2020). Unit values were $0.19 kg−1 for rice grain, $3.00 kg−1 for meat, $8.05 kg−1 for N fertilizer, and $89.37 and $90.40 kg−1 for palisadegrass and guineagrass seeds, respectively.
Intercropping Competition Factors
Competition effects between rice and forage crops were calculated by land equivalent ratio (LER), relative crowing (K), and aggressivity (A). The LER was calculated according Mead and Willey (1980) by the following formula:
where Y is aboveground biomass, and the suffixes 1 and 2 denote crop 1 (rice) and crop 2 (palisadegrass or guineagrass) and vice versa, respectively. The Y1, 2 was the aboveground biomass of rice when grown in intercropping with grasses; Y1, 1 was the yield of rice when grown monocropped; Y2, 1 was the aboveground biomass of palisadegrass or guineagrass when grown in intercropping with rice; and Y2, 2 was the aboveground biomass of palisadegrass or guineagrass when grown monocropped (Baumann et al., 2001; Biabani et al., 2008).
Aggressivity (A) is used to determine the competitive relationship between two crops in a mixture (Takim, 2012) and was calculated by the following formula (Agegnehu et al., 2006):
The K is a measure of the competition experienced by crop 1 (rice) when grown in intercropping with crop 2 (palisadegrass or guineagrass) and vice versa. The calculation was according Agegnehu et al. (2006) as the following formula:
where Z1, 2 is the sown proportion of rice, and Z2, 1 is the sown proportion of the forage species. The plant density of each species used was from the day of rice harvest.
Results
Leaf Nutrient Concentrations, Agronomic Characteristics, Yield, and Grain Quality of Upland Rice
Monocropped rice had similar N concentration as rice intercropped with palisadegrass and greater leaf P and K concentrations as with rice in the intercropping systems (Supplementary Table 2); N and P concentrations were lowest in rice intercropped with guineagrass. In the first year (2011–2012), greatest P and K concentrations were observed, while Ca and Mg concentrations were greatest in the third year (2014–2015). Across all cropping systems, sidedress N application rate significantly increased leaf N concentration, but with diminishing effect at higher N rates (Figure 1A).
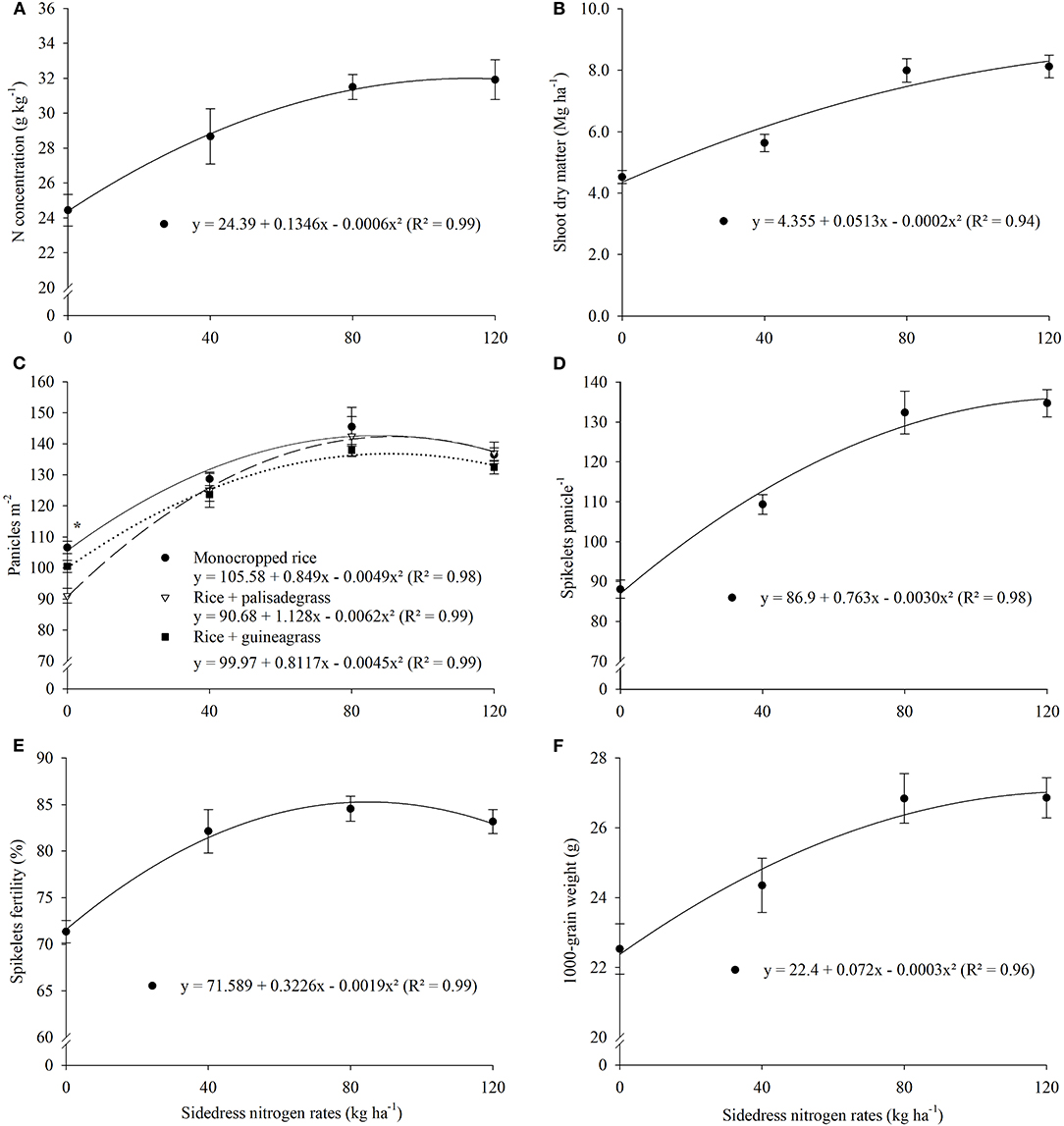
Figure 1. Rice leaf nitrogen concentration (A), shoot dry matter (B), cropping system × sidedress nitrogen rates interaction effect on panicles m−2 (C), spikelets panicle−1 (D), spikelets fertility (E), and 1,000-grain weight (F) as affected by sidedress nitrogen rate. Each data point is the mean of 36 replicates (three cropping systems, four blocks, and three growing seasons) and associated error bar is ± one SE. *P ≤ 0.05.
Among agronomic characteristics, shoot dry matter (DM), panicles per m2, spikelets per panicle, spikelet fertility, 1,000-grain weight, and grain yield of upland rice were influenced by treatment (Table 1). Agronomic characteristics were greatest in monocropped rice. Greater DM, panicles per m2, spikelets per panicle, and grain yields with monocropped rice than rice intercropped with forage grasses was most dramatic in the first growing season. In addition, these agronomic characteristics responded positively to increasing N rate (Table 1).
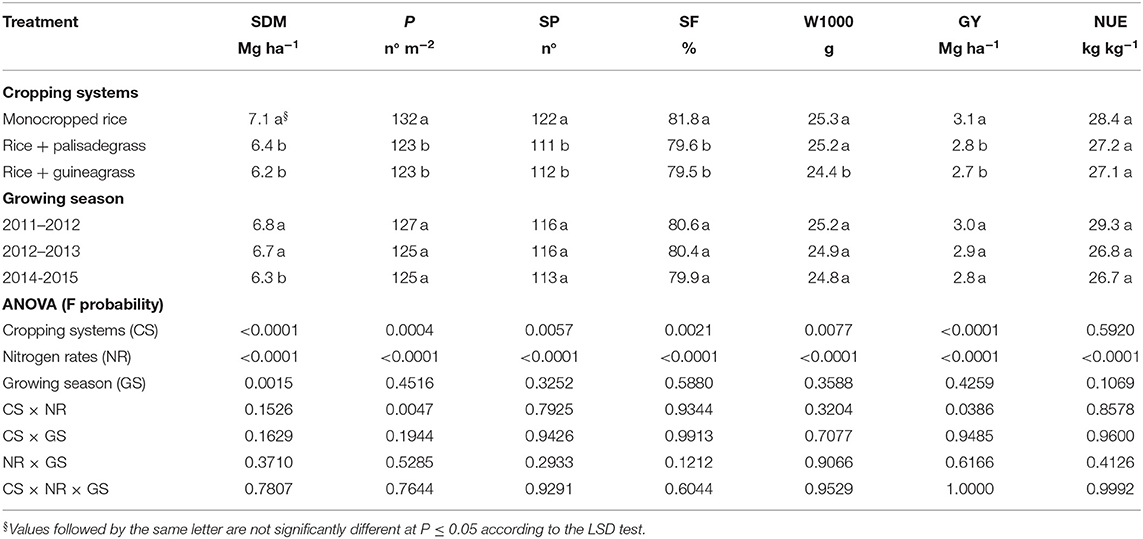
Table 1. Shoot dry matter (SDM), panicles per m2 (P), spikelets per panicle (SP), spikelet fertility (SF), 1,000-grain weight (W1000), grain yield (GY), and N-use efficiency (NUE) of upland rice as affected by cropping systems, sidedress nitrogen rates, and growing season.
A significant cropping system × N rate interaction occurred for panicles per m2 (Figure 1C) and grain yield of upland rice (Figure 2A), with the highest values in the monocropped system. Shoot DM (7.6 Mg ha−1) was greatest at 128 kg N ha−1 (Figure 1B), spikelets per panicle (135) at 127 kg N ha−1 (Figure 1D), spikelet fertility (85%) at 85 kg N ha−1 (Figure 1E), 1,000-grain weight (22.8 g) at 120 kg N ha−1 (Figure 1F), and NUE (32 kg kg−1) at 68 kg N ha−1 (Figure 2B). The effects of sidedress N rate on grain yield did not differ among cropping systems, with monocropped rice (4.0 Mg ha−1) achieving a cost-to-value threshold of 15 kg gain per kg N at 86 kg N ha−1, rice + palisadegrass (3.7 Mg ha−1) at 87 kg N ha−1, and rice + guineagrass (3.5 Mg ha−1) at 83 kg N ha−1 (Figure 2A).
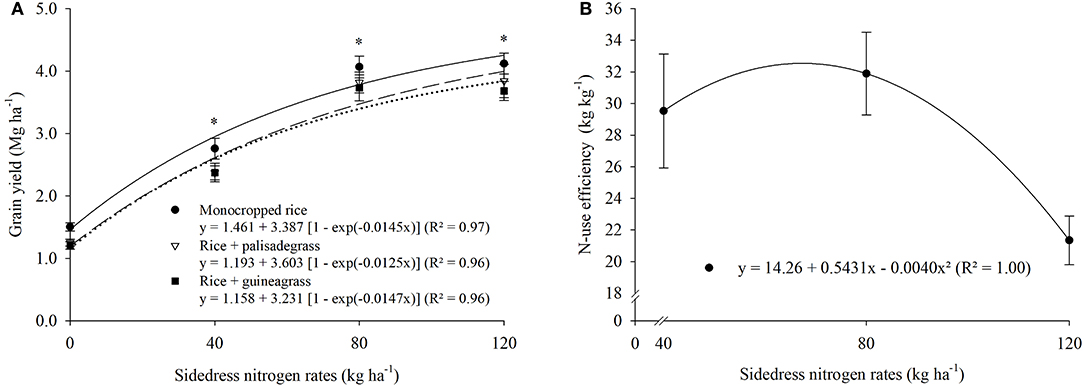
Figure 2. Cropping system × sidedress nitrogen rates interaction effect on grain yield of upland rice (A), and N-use efficiency as affected by sidedress nitrogen rate (B). Each data point is the mean of 36 replicates (three cropping systems, four blocks, and three growing seasons) and associated error bar is ± one SE. *P ≤ 0.05.
Milled rice productivity was influenced by treatment (Table 2), with greatest yields for the monocropped system. Milling yield, head rice yield, grain protein, and milled rice productivity were influenced by sidedress N application rate (Table 2). Industrial quality of upland rice increased with sidedress N rate; milling yield (72%) was greatest at 117 kg N ha−1, head rice yield (62%) was greatest at 96 kg N ha−1 (Figure 3A), grain protein (11.6%) was greatest at 145 kg N ha−1 (Figure 3B), and milled rice productivity (3.5 Mg ha−1) was greatest at 165 kg N ha−1 (Figure 3C).
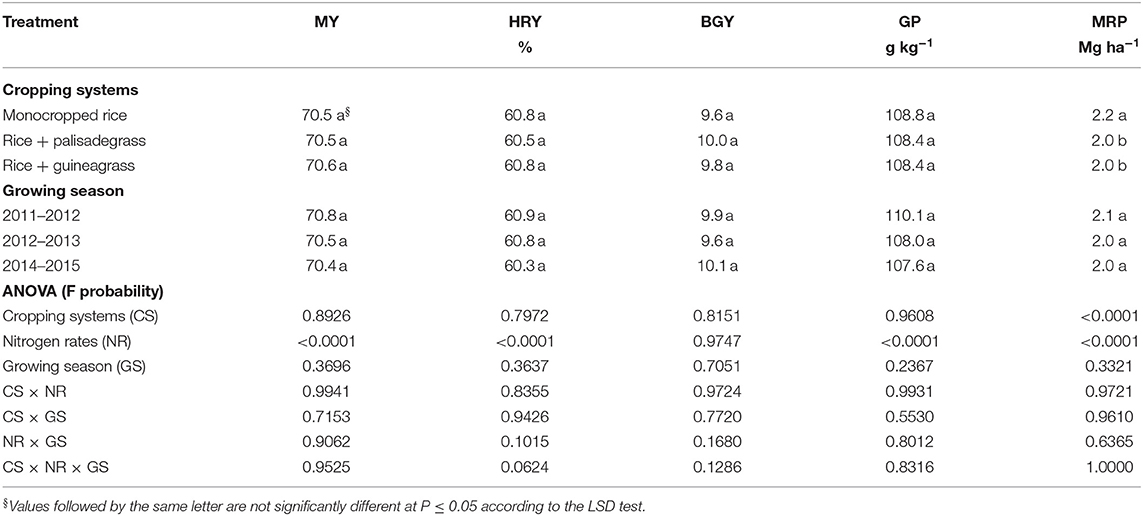
Table 2. Milling yield (MY), head rice yield (HRY), broken-grain yield (BGY), grain protein (GP), and milled rice productivity (MRP) of upland rice as affected by cropping systems, sidedress nitrogen rates, and growing season.
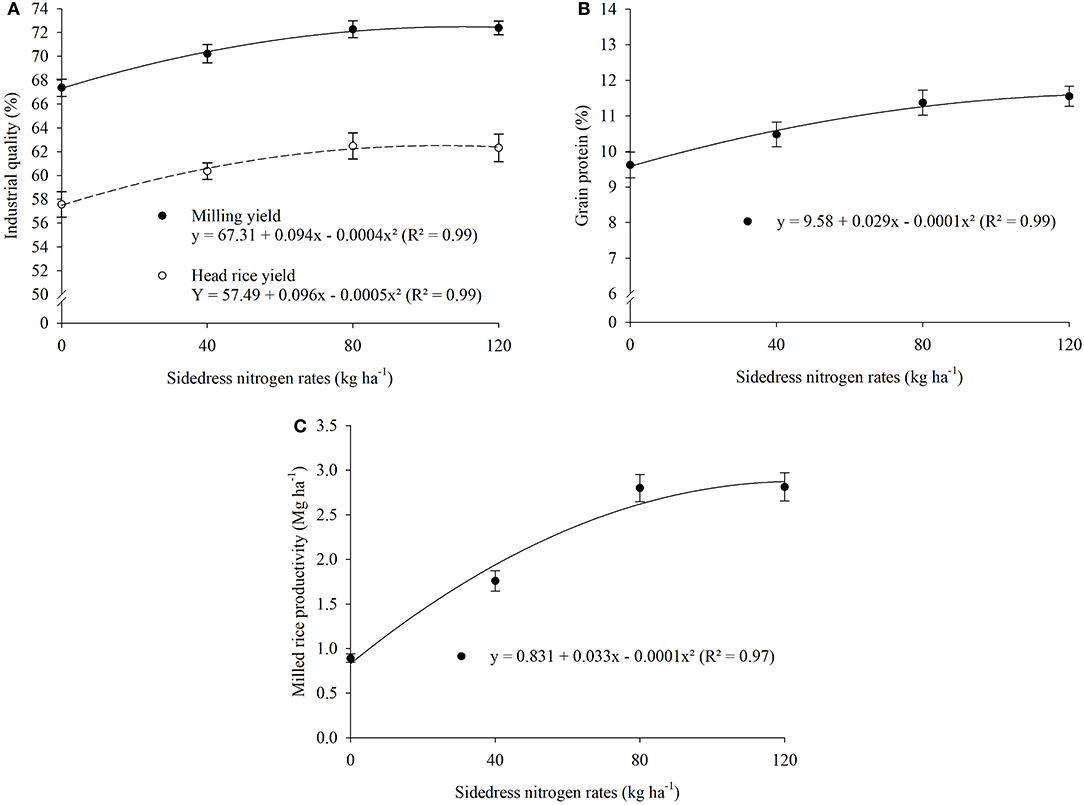
Figure 3. Industrial quality (A), grain protein (B), and milled rice productivity (C) as affected by sidedress nitrogen rate. Each data point is the mean of 36 replicates (three cropping systems, four blocks, and three growing seasons) and associated error bar is ± one SE.
Forage Dry Matter Production and Crude Protein and Economics
An advantage of intercropping systems compared with monocropping was the production of forage in the off-season. Palisadegrass intercropped with rice provided greater forage DM production, estimated animal stocking rate (SR), and estimated meat production in both cuts compared with guineagrass intercropped with rice (Table 3). However, guineagrass had greatest CP content of forage in the second cut.
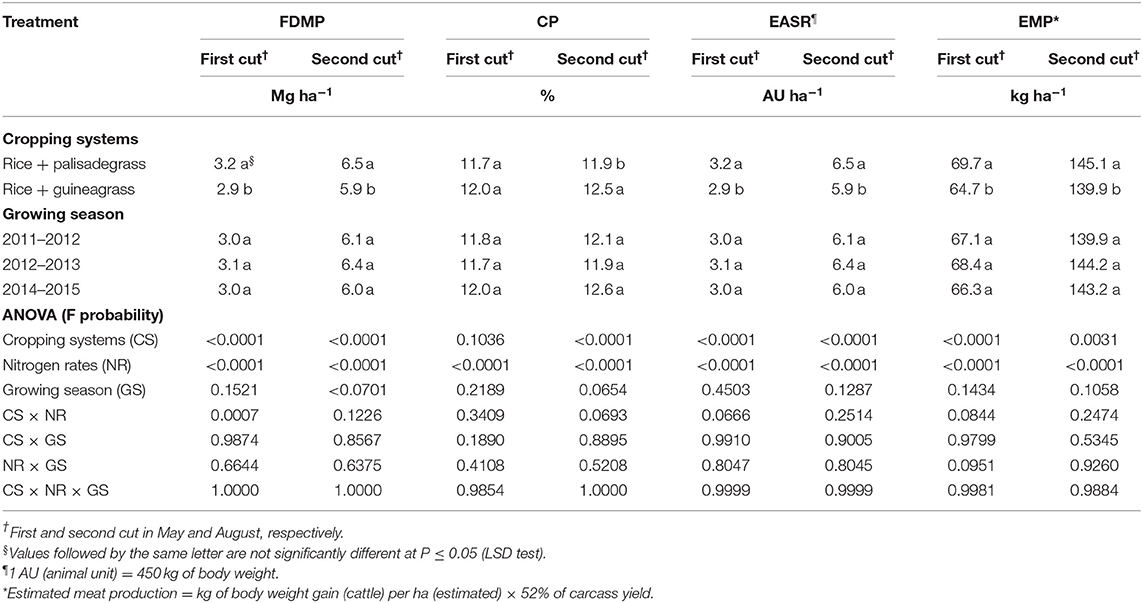
Table 3. Forage dry matter production (FDMP), forage crude protein concentration (CP), estimated animal stocking rate (EASR), and estimated meat production (EMP) in the fall-winter as affected by cropping systems, sidedress nitrogen rates and growing season.
Forage DM production, CP, SR, and meat production were positively influenced by increasing N rates (Tables 3, 4, Figures 4, 5). There was a significant cropping system × N rate interaction for forge DM production in the first cut (Figure 4A), with best results in the rice + palisadegrass intercropping system (5.15 Mg ha−1) receiving 185 kg N ha−1, followed by the rice + guineagrass intercropping system (4.6 Mg ha−1) receiving 175 kg N ha−1. In the second cut, forage DM production continued to increase with increasing N rates applied to the rice crop, demonstrating the positive carryover effect of N on forage production in the off-season (Figure 4B). Crude protein was greatest during both first and second cuts at 120 kg N ha−1 (Figure 4C).
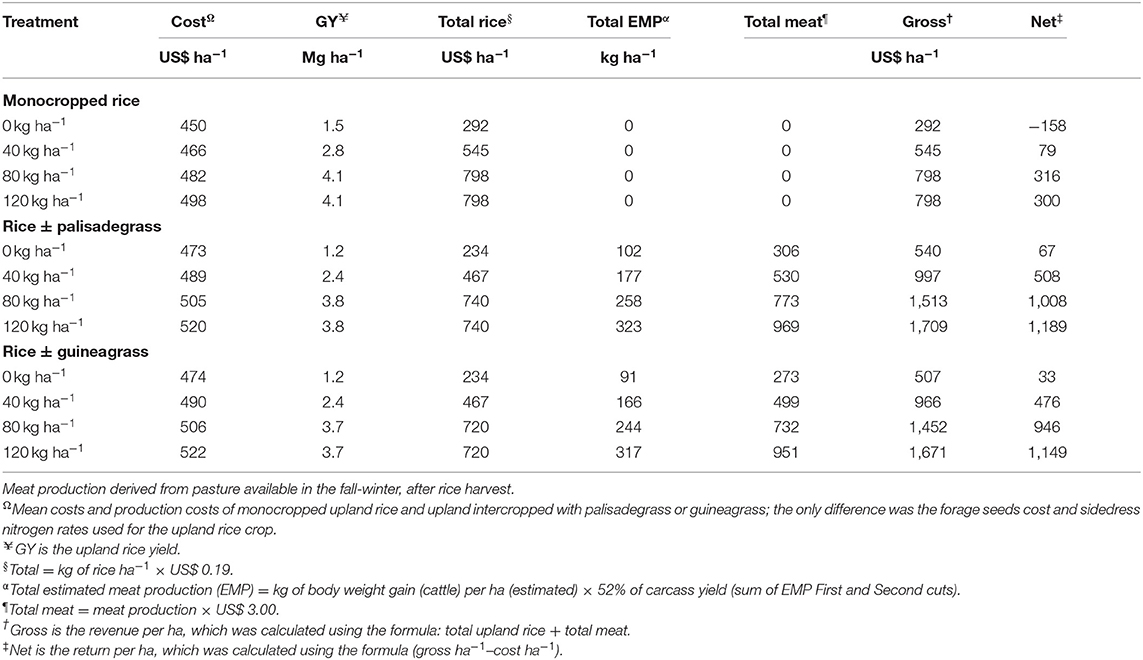
Table 4. Economic evaluation of monocropped upland rice, upland rice intercropped with palisadegrass and upland rice intercropped with guineagrass as a function of sidedress nitrogen rates for upland rice (average of three growing seasons).
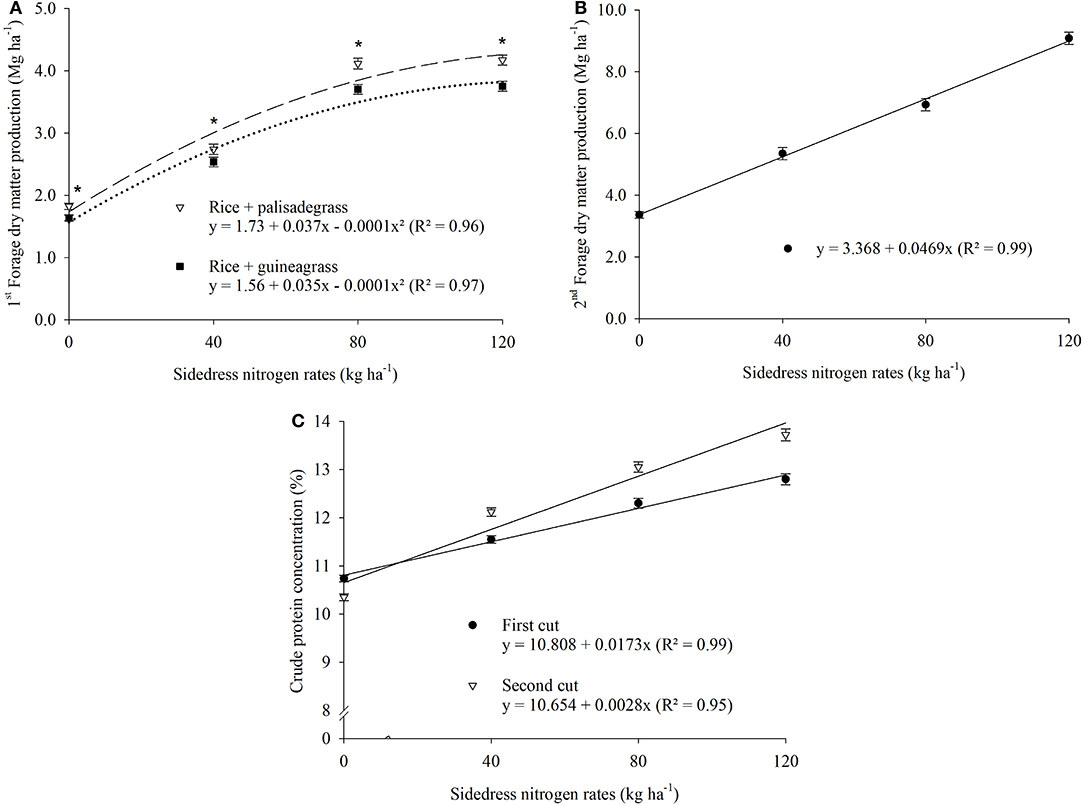
Figure 4. Crop system × sidedress nitrogen rates interaction effect on forage dry matter production in the first cut (A), forage dry matter production in second cut (B), and crude protein concentration at second cut as affected by sidedress nitrogen rate (C). Each data point is the mean of 24 replicates (two cropping systems, four blocks, and three growing seasons) and associated error bar is ± one SE. *P ≤ 0.05.
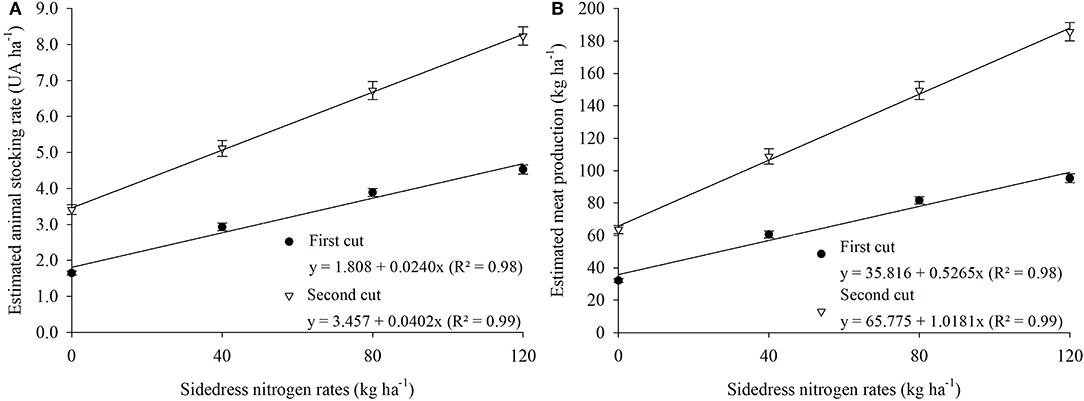
Figure 5. Estimated animal stocking rate (A), and estimated meat production as affected by sidedress nitrogen rate (B). Average of three growing seasons. Stocking rate and meat production in the fall-winter period, after rice harvest. Each data point is the mean of 24 replicates (two cropping systems, four blocks, and three growing seasons) and associated error bar is ± one SE.
The rice + palisadegrass and rice + guineagrass treatments with 120 kg ha−1 of applied sidedress N resulted in the highest net profits (US$ 1,189 and 1,149 ha−1, respectively) (Table 4). Monocropped rice without sidedress N application rate resulted in the lowest net profit (US$ −158 ha−1).
Intercropping Competition
Aboveground biomass of monocropped rice was greater than that of rice intercropped with palisade or guineagrass in all growing seasons (Supplementary Table 3). However, intercropping of rice with tropical forages increased total aboveground biomass in both intercropping systems. In general, the greater the N fertilizer rate, the greater the aboveground biomass. Total aboveground biomass was greater for rice intercropped with guineagrass than for rice intercropped with palisadegrass. The plant density of rice and forage increased with increasing N rate, demonstrating the efficiency of this nutrient in promoting plant development and crop establishment. Total N content of rice intercropped with palisadegrass or guineagrass was greater than that of monocropped rice (Supplementary Table 3). Among the N rates, 120 kg N ha−1 resulted in the greatest total N uptake.
Land equivalent ratio (LER) and relative N yield (RNY) of rice and forages were calculated from aboveground biomass and N content measured on the day of rice harvest (Table 5). All intercropping systems resulted in LER >1. Across growing seasons, LER and RNY were greater for rice intercropped with palisadegrass than for rice intercropped with guineagrass, and differences increased with increasing N rate. Compared with the control treatment (0 kg N ha−1), sidedressing rice intercropped with palisadegrass increased total biomass by 31% at 80 kg N ha−1 and by 59% at 120 kg ha−1.
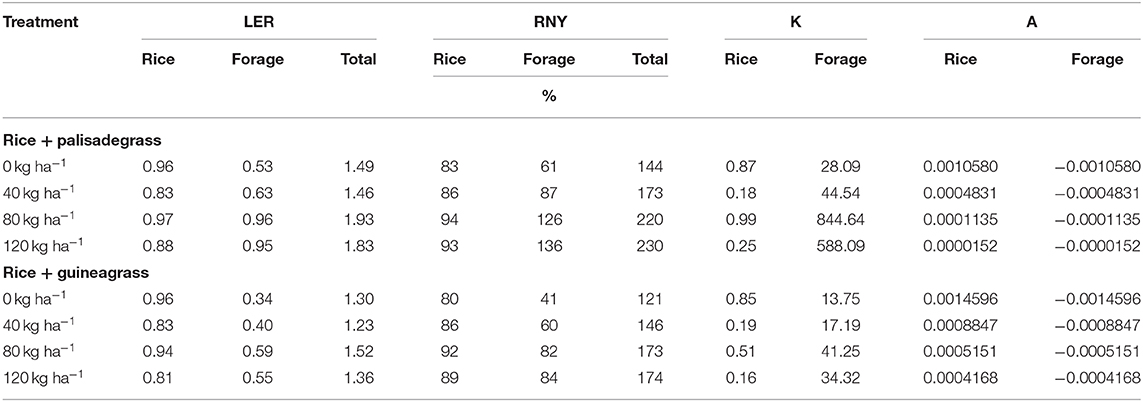
Table 5. Land equivalent ratio (LER), relative nitrogen yield (RNY), relative crowding coefficient (K) and aggressivity (A) of upland rice, palisadegrass, and guineagrass intercropped as a function of sidedress nitrogen rates for upland rice crop.
The relative crowding coefficient (K) showed that intercropping competition between plants was similar in the two intercropping systems in the control treatment and at 40 kg N ha−1 (Table 5). In the treatments with 80 kg N ha−1, the K value of rice intercropped with guineagrass was lower than that of rice intercropped with palisadegrass. Low values of aggressivity (A) were observed for forage crops independent of N rate, indicating low interspecific competition of either forage grasses (Table 5).
Discussion
Upland Rice
Nutritional status of upland rice was influenced by cropping system and sidedress N rate. Availability of N for upland rice was lowest when intercropped with guineagrass (28 g kg−1), indicating competition between rice and the forage for N. However, leaf N concentration was within the range considered ideal for rice (27–35 g kg−1) according to Cantarella et al. (1997). Guineagrass has high N demand when intercropped with grain crops, because of high soil fertility requirement (Pires, 2006). Regardless of type of intercropping, leaf N concentration increased with sidedress N application up to 112 kg N ha−1. Results of a previous study led to a sidedress recommendation of 40–60 kg N ha−1 (depending on the expected response to sidedress N fertilization) for monocropped upland rice (Cantarella et al., 1997). Our results suggest that N fertilizer recommendations might need to be greater than published in fertilization tables, but such changes in recommendation may need to be defined by soil N availability indices.
Across cropping systems, rice leaf P concentration varied from 2.3 to 2.6 g kg−1, and rice leaf K concentration varied from 19 to 20 g kg−1. These values were considered within the adequate range for rice, i.e., 1.8–3.0 g kg−1 for P and 13–30 g kg−1 for K according to Cantarella et al. (1997). Intercropping of palisadegrass and guineagrass with upland rice reduced rice leaf P and K concentrations due to competition for these nutrients between rice and the forage species.
Shoot DM, panicles per m2, spikelets per panicle, spikelet fertility, 1,000-grain weight and grain yield of upland rice were lower in intercropped systems than in rice monoculture (Table 1). Rice grain yield varied from 2.7 to 3.1 Mg ha−1 among treatments. According to Crusciol et al. (2011) and Nascente et al. (2013), it is possible to achieve upland rice yields of 4.0–5.0 Mg ha−1 under well-distributed rainfall conditions using NTS for monocropped rice in tropical regions. Our results showed a small interspecific competition between intercropped plants. Therefore, forages reduced rice vegetative growth and grain yield in these intercropping systems.
Rice does not have a strong ability to compete with other plants, especially aggressive forage grasses (Fischer et al., 2001). Plant competition is one of the most yield-limiting constraints in upland rice production and can reduce rice yield by 50%. Tropical forage grasses can reduce plant development of cash crops, resulting in low crop yields. Intercropping may also lead to interspecific competition and may decrease crop yields when plants are not adequately managed (Baldé et al., 2011; Pariz et al., 2016, 2017). In addition, rice and forage grasses have different photosynthetic pathways, i.e., rice is C3 (Karki et al., 2013) and these tropical grasses are C4 (Silva et al., 2015). C4 species are more efficient in converting energy intercepted by the canopy into biomass production (Zhu et al., 2010), resulting in greater competition compared with C3 species under tropical conditions (Atkinson et al., 2016). Therefore, forage species likely reduced the availability of water, nutrients, and solar radiation for rice even when sown 30 days after rice emergence, resulting in reduced plant development and grain yield of upland rice. Studies have highlighted the challenges of food production in intercropping systems with grasses and the need to seek alternatives to reduce competition for resources among plants grown simultaneously (Costa et al., 2012; Mateus et al., 2020). One alternative to reduce the negative effects of competition on yields of upland rice intercropped with palisadegrass is the application of a low rate of herbicide (cyhalofop-butyl), with the aim of reducing forage growth at sowing while simultaneously increasing food production in the system (Carvalho et al., 2010). Another alternative to reduce competition and increase rice yields is N application as fertilizer since N is essential for rice plant growth (Basuchaudhuri, 2016) and its cultivation in NTS usually develops slowly in the early stages as a result of N immobilized by microorganisms that decompose straw (Rosolem et al., 2017). Our study showed that a rate of ~115 kg N ha−1 increased production components, and the highest NUE was obtained at a rate of 68 kg N ha−1. NUE reduction in rates above 68 kg N ha−1 indicates that rice plants were not able to absorb the N applied in excess, because their absorption mechanisms could be saturated (Fageria, 2014).
There was an effect of the cropping system × N rate interaction on the number of panicles per m2 such that in the absence of N application, the number of panicles per m2 was greatest for monocropped rice. N application increases the number of rice tillers and ensures their survival to become producers of panicles (Gitti et al., 2012). With reduced N supply, intercropping of rice with palisadegrass or guineagrass intensified the competition for N between species, reducing the development of rice. For monocropped and intercropped rice, the number of panicles per m2 was greatest at 89 kg N ha−1.
At all N rates, grain yield of rice was greater with monocropping than with intercropping of forage grasses. This result shows the potential for interference from forages intercropped with rice due to competition for N. According to Atkinson et al. (2016), species with C4 photosynthetic metabolism (palisadegrass and guineagrass) have faster growth than C3 species (rice). For all cropping systems, rice yields were optimized with N rate of 85 kg N ha−1. The rate of increase in all systems was 32 kg grain kg−1 N applied. With no N fertilizer application, rice yield was greater when monocropped than in the intercropping systems, and this difference increased as the rate of N fertilizer application increased. In the intercropping systems, forage grasses shaded rice plants due to vigorous growth of the grasses, and the consequent lower solar radiation on the rice leaves reduced plant development and photosynthetic processes during grain filling (Meirelles et al., 2019).
Milled rice productivity was 12% greater when monocropped than when intercropped with palisadegrass or guineagrass, reflecting this negative effect of competition. Industrial quality of rice grains, as assessed by milled and whole grain yields, achieved maximum production at an average of 106 kg N ha−1. At this rate, milled rice productivity was 24 kg grain kg−1 N. Lower levels of N in the plant reduce the industrial quality of rice grains (Portugal et al., 2020). Low N availability causes chalkiness of grain (Zhou et al., 2015) due to grain opacity caused by the arrangement between starch and protein granules in the cells, resulting in a large percentage of broken grains (Marchezan et al., 1992). As N availability improves, starch and protein accumulation in rice grains increase. This accumulation results in densely compacted starch granules interspersed with protein bodies (Zhou et al., 2015), which increases grain resistance to breakage (Silva et al., 2013). Rice quality and actual productivity influence the market price of rice and consequently revenue in the growing area (Salassi et al., 2013).
Forage Characteristics and Estimated Meat Production
The optimal temperature range for palisadegrass and guineagrass development is 30–35°C. Temperatures of 10–15°C that occur during the winter greatly reduce the growth of these forage grasses (Costa et al., 2005). In addition, during the off-season in the Brazilian Cerrado (dry winters), rainfall is limited, further reducing the development of these forage grasses, mainly in June to July, the period corresponding to the 1st cut. Forage DM production of the 1st cut was greater for palisadegrass than for guineagrass, regardless of the N rate applied. For both forage grasses, greatest DM production was obtained with an application rate of 120 kg N ha−1 to rice, resulting in forage production of 4.7 and 4.3 Mg ha−1 for palisadegrass and guineagrass, respectively. Forage DM production of both grasses during the 2nd cut responded linearly to rice N fertilizer application. In general, greater stocking rate and meat production were obtained with greater forage DM production.
Tropical forage grasses have strong potential for regrowth and provide high availability of biomass (pasture) in the off-season (Costa et al., 2015), mainly in crop systems with N application. Forage DM production can be used as a parameter for hay production potential or as grazed pasture in the off-season (Pariz et al., 2009) to increase the sustainability of agricultural activities. In addition, rice monocropping in the summer season is usually followed by a fallow period in the dry season, which increases the number of weeds in agricultural areas (Nascente et al., 2013). In tropical regions, forage availability is usually lower in the off-season due to the dry winter weather conditions (Borghi et al., 2013a). Therefore, rice intercropped with palisade or guineagrass can be a good option for the diversification of farm activities year-round. Intercropping systems provide the possibility of grazing during the off-season period, when climatic conditions are unfavorable for the development of most crops. Sowing of tropical perennial grasses after rice harvest usually does not provide enough fodder in the off-season due to the short time period and slow plant development for the establishment of fodder under dry winter conditions, making it a risky option.
Intercropped forage crops accumulated significant DM, even after 6 months without rain, which is characteristic of these regions (Cerrado or African Savannas). Forage residue accumulation can favor the success of a NTS, leading to greater nutrient cycling from the large biomass production and establishment of deep root systems (Pacheco et al., 2011; Momesso et al., 2019). Forage roots can absorb nutrients otherwise lost by leaching and return them to the surface of the soil (Mateus et al., 2020). In addition, mineralization of plant and animal residues releases nutrients into the soil that can be absorbed by crops in succession (Carvalho et al., 2010; Pariz et al., 2016, 2017; Moraes et al., 2019).
Among cycled nutrients, N provides the greatest returns to the crop system and can be reused by crops in succession (Rosolem et al., 2017; Momesso et al., 2019). Thus, high mulch cover production by intercropped systems of grain crops with forage grasses is key for the successful maintenance of NTS in the tropics, as well as a very important strategy for enhancing the early establishment and successful production of forage for grazing by animals in the off-season (Barth Neto et al., 2014; Mateus et al., 2016; Pariz et al., 2017).
The benefits of intercropping and N fertilization for increased forage production and nutrient cycling are strongly reflected in the quality of the pasture established in succession. Crude protein was the same in the two forage grasses in the 1st cut, but greater in guineagrass than in palisadegrass in the 2nd cut. Crude protein was also greater in the 2nd cut than in the 1st cut. Both forages responded strongly to the previous application of N to rice. In general, CP exceeded the minimum of 70 g kg−1 (7%) considered necessary by van Soest (1994) to maintain the population of microorganisms in the rumen.
Palisadegrass growth was initially greater than guineagrass growth during the 1st cut (Pariz et al., 2017), but with greater N application guineagrass responded with greater growth during the 2nd cut (Mateus et al., 2016), resulting in equivalent overall DM production. The rate of N fertilizer needed to achieve optimum forage production potential is 80 kg N ha−1. Recommended N fertilizer rates for monocropped upland rice have been established (Cantarella et al., 1997). However, there is no N fertilizer recommendation for intercropping systems, especially in NTS (Nascente et al., 2013; Arf et al., 2018). We can infer that the current recommended N fertilizer rate may be insufficient to optimize grain yield of rice and biomass and CP in intercropped forage grasses.
Greater forage production and quality can increase meat and milk production in the dry winter (Crusciol et al., 2012). The amount of N required by crops varies according to the environmental conditions and characteristics of the plants used in rotation, with greater needs for N in crop systems that include only grasses (Crusciol et al., 2012; Garcia et al., 2016; Mateus et al., 2020). Thus, our results provide a better understanding of the use of N in upland rice intercropping systems to support greater N fertilization efficiency and more sustainable agricultural systems.
Revenue
Intercropped systems can be considered a sustainable manner of food production to improve quality of pastures and animal carrying capacity. Our results demonstrated that intercropping of forages with rice using NTS is a feasible option for increasing sustainability in tropical areas and can result in higher revenues for farmers due to the productive, economic, and environmental benefits of these systems. Furthermore, these systems can increase global food production in the same area (Carvalho et al., 2010; Herrero et al., 2010; Franzluebbers and Stuedemann, 2014; Food and Agriculture Organization of the United Nations (FAO), 2017; Moraes et al., 2019). Therefore, our data indicated that rice intercropped with palisadegrass or guineagrass is a promising approach for farmers, especially in the tropical regions of South America, Africa, and parts of Asia, where additional opportunities to produce food are needed.
Except for monocropped rice without sidedress N rate, all treatments resulted in positive net profit, particularly the rice + palisadegrass and rice + guineagrass treatments, because in addition to rice yield in the summer/autumn, farmers could use forage DM production of palisadegrass and guineagrass for animal fodder in the winter/spring. In all treatments with low N sidedress rate (0 or 40 kg N ha−1), net profits were negative when considering rice production only. However, when considering intercropping, one could produce 102–323 kg ha−1 meat during the fall-winter season, with net profits of US$ 33–1,196 ha−1, depending on the rate of sidedress N application to rice. Greater N rates result in greater net profits as a function of greater forage DM production, which could effectively add an extra US$ 125–889 to the production system. In addition, the need for soil mulch would be satisfied in planning for the next crop.
Similar to those of rice yield, economic results highlight the need for sufficient sidedress N application rates for the rice + palisadegrass (80–120 kg ha−1 N) and rice + guineagrass (120 kg ha−1 N) treatments to achieve greatest net revenue (>US$ 1,000 ha−1).
Intercropping Competition Factors
Intercropping changes the N dynamics and nutrient use in the system. Our study showed positive effects of N application in intercropping systems, resulting in greater yield per land area. Increases in total LER and RNY were observed when tropical forages were intercropped with rice, indicating that forage is a suitable option for agricultural systems. Crop performance in intercropping systems is measured by LER, which describes the relative land use per unit area compared with monocropping (Biabani et al., 2008). Values of LER <1 indicate that the species is disadvantageous in the system. RNY may have low nutrient cycling and imposes greater competition per area of crops. However, intercropping systems such as rice + tropical grasses are also beneficial due to straw production and soil coverage throughout the year in NTS. Palisadegrass exhibited greater growth rate than guineagrass when intercropped with rice, indicating that this grass is a viable option in intercropping systems. Systems that improve land use are important for the sustainability of the environment and food production, especially in regions with poor farmers (Costa et al., 2015; Mateus et al., 2020). In addition, the RNY values indicated a high percentage of N cycling in the intercropping systems with N fertilization. Palisadegrass and guineagrass increase nutrient cycling in agricultural systems (Pariz et al., 2017) due to their deep root systems and nutrient uptake in the soil profile (Rosolem et al., 2017). Our study highlights that, although monocropped rice produced the greatest grain yield, intercropping systems of rice, and forage increased total agricultural yields as well as enhanced land use and N cycling.
Competition between rice and forages was evident. Based on the high K values for the forage grasses, tropical forages are more competitive than upland rice due to the plant characteristics of these grasses (Zarochentseva, 2012; Rosolem et al., 2017). Pariz et al. (2017) and Mateus et al. (2016) reported similar high competition between forage grasses and maize and sorghum in intercropping systems, respectively. However, the aggressivity of upland rice was higher than that of the forage in our study, as the rice was sown before the forage (Namuco et al., 2009). In addition, aboveground biomass and plant density of crops were affected by environmental factors, such as climatic conditions and N fertilizer. A shorter time of co-existence in intercropping can favor the growth of crops by reducing interspecific competition (Crusciol et al., 2014). Although there was competition between grain and forage species, intercropping systems provided advantages, including soil coverage, high land use per area and food for cattle as pasture due to forage production. This study contributes to sustainable agricultural production and provides a foundation for subsequent research on the production of rice in intercropping with forage and the adequate rate of N fertilizer application in these tropical systems.
Conclusions
In this study, intercropping systems with upland rice and forage grasses showed considerable improvements in productivity, economic, and environmental outcomes over three growing seasons. Monocropping upland rice provided a superior rice performance, with high agronomic characteristics and yields; however, intercropping with forage grasses was superior in total food production due to increased diversification of production, land use per unit area, nutrient cycling, and profitability throughout the year. Both forages were viable for cultivation in intercropping system, production of forage dry matter, crude protein, and estimated meat. For upland rice monoculture or intercropped with perennial grasses, the recommendation of 85 kg N ha−1 was sufficient to optimize grain production. However, our study showed that with crop-livestock integration, food production (grains and meat), and economic return responded positively with the application of 120 kg N ha−1. Additional studies with rates >120 kg N ha−1 and different management of N fertilizer in the intercropped rice should be considered, as well as long-term studies to understand the changes in soil nutrient cycling capacity that might develop in these systems. In addition, intercropping of upland rice and forage grasses enhanced land equivalency ratio at an application rate of 80 kg N ha−1 and relative N yield at an application rate of 120 kg N ha−1. From an overall perspective considering grain yields, land use, nutrient cycling, and profitability, intercropping systems were effective to improve diversity and food production in the same area with a potential to decrease poverty in developing countries.
Data Availability Statement
The raw data supporting the conclusions of this article will be made available by the authors, without undue reservation.
Author Contributions
CACC, CC, and HC: design of experiment. CASC, LM, and CHMC: obtain and process the data. CACC, JP, JB, CP, AC, and AF: analysis of data. CACC, JP, LM, JB, and NC: wrote the paper with the contribution of all co-authors. All authors confirm being contributors of this work and have approved it for publication.
Conflict of Interest
The authors declare that the research was conducted in the absence of any commercial or financial relationships that could be construed as a potential conflict of interest.
Acknowledgments
The authors would like to thank the Coordination for the Improvement of Higher Level Personnel (CAPES/PROAP). The first, ninth and eleventh authors would like to thank the National Council for Scientific and Technological Development (CNPq) for an award for excellence in research.
Supplementary Material
The Supplementary Material for this article can be found online at: https://www.frontiersin.org/articles/10.3389/fsufs.2020.00129/full#supplementary-material
References
Africa Rice Center - AfricaRice (2019). Africa Rice Center (Africa Rice) Annual Report 2018: Sustainable Rice Production in the Face of Climate Emergency. Abidjan, Côte d'Ivoire, 28.
Agegnehu, G., Ghizaw, A., and Sinebo, W. (2006). Yield performance and land-use efficiency of barley and fava bean mixed cropping in Ethiopian highlands. Eur. J. Agron. 25, 202–207. doi: 10.1016/j.eja.2006.05.002
Agrolink (2020). Quotations to Farming. Avaialble online at: www.agrolink.com.br. (accessed 1 February, 2020).
Allen, V. G., Baker, M. T., Segarra, E., and Brown, C. P. (2007). Integrated irrigated crop–livestock system in dry climates. Agron. J. 99, 346–360. doi: 10.2134/agronj2006.0148
Arf, O., Portugal, J. R., Buzetti, S., Rodrigues, R. A. F., and Sá, M. E. (2018). Crop rotation green manure and nitrogen fertilizers in upland rice under no-tillage. Pesq. Agropec. Trop. 48, 153–162. doi: 10.1590/1983-40632018v4851446
Atkinson, R. R. L., Mockford, E. J., Bennett, C., Christin, P. A., Spriggs, E. L., Freckleton, R. P., et al. (2016). C4 photosynthesis boosts growth by altering physiology, allocation and size. Nat. Plant 2:16038. doi: 10.1038/nplants.2016.38
Baldé, A. B., Scopel, E., Affholder, F., Corbeels, M., Silva, F. A. M., Xavier, J. H. V., et al. (2011). Agronomic performance of no-tillage relay intercropping with maize under smallholder conditions in Central Brazil. Field Crop. Res. 124, 240–251. doi: 10.1016/j.fcr.2011.06.017
Barth Neto, A., Savian, J. V., Schons, R. M. T., Bonnet, O. J. F., Canto, M. W., Moraes, A., et al. (2014). Italian ryegrass establishment by self-seeding in integrated crop-livestock systems: effects of grazing management and crop rotation strategies. Eur. J. Agron. 53, 67–73. doi: 10.1016/j.eja.2013.11.001
Basuchaudhuri, P. (2016). Nitrogen Metabolism in Rice. Boca Raton, FL; New York, NY: CRC Press, p. 1-29. doi: 10.1201/b19974
Baumann, D. T., Lammert, B., and Kropff, M. J. (2001). Competition and crop performance in a leek-celery intercropping system. Crop. Sci. 41, 764–774. doi: 10.2135/cropsci2001.413764x
Biabani, A., Hashemi, M., and Herbert, S. J. (2008). Agronomic performance of two intercropped soybean cultivars. Int. J. Plant Prod. 2, 215–222. doi: 10.22069/IJPP.2012.614
Borghi, E., Crusciol, C. A. C., Mateus, G. P., Nascente, A. S., and Martins, P. O. (2013a). Intercropping time of corn and palisadegrass or guineagrass affecting grain yield and forage production. Crop Sci. 53, 629–636. doi: 10.2135/cropsci2012.08.0469
Borghi, E., Crusciol, C. A. C., Nascente, A. S., Souza, V. V., Martins, P. O., Mateus, G. P., et al. (2013b). Sorghum grain yield, forage biomass production and revenue as affected by intercropping time. Eur. J. Agron. 51, 130–139. doi: 10.1016/j.eja.2013.08.006
Borghi, E., Crusciol, C. A. C., Trivelin, P. C. O., Nascente, A. S., Costa, C., and Mateus, G. P. (2014). Nitrogen fertilization (15NH4NO3) of palisadegrass and residual effect on subsequent no-tillage maize. R. Bras. Ci. Solo 38, 1457–1468. doi: 10.1590/S0100-06832014000500011
Cantarella, H., van Raij, B., and Camargo, C. E. O. (1997). “Cereals,” in Lime and fertilizer recommendations for the State of São 428 Pãulo. 2nd Edn, eds B. Raij, H. van Cantarella, J. A. Quaggio, and A. C. M. Furlani (Campinas: Tech. Bull. 100. Inst. Agronomico), 43–50.
Carvalho, P. C. F., Anghinoni, I., Moraes, A., Souza, E. D., Sulc, R. M., Lang, C. R., et al. (2010). Managing grazing animals to achieve nutrient cycling and soil improvement in no-till integrated systems. Nutr. Cycling Agroecosyst. 88, 259–273. doi: 10.1007/s10705-010-9360-x
CGIAR Science Council (2006). IRRI's Upland Rice Research Follow-up Review to the 6th IRRI EPMR. Rome: Science Council Secretariat.
CONAB (2018). Cost of Agricultural Production. Available online at: http://www.conab.gov.br/conab/Main.php?MagID=3&MagNo=39 (accessed 22 August, 2019).
Costa, K. A. P., Rosa, B., Oliveira, I. P., Custodio, D. P., and Silva, D. C. (2005). Effect of seasonal climate condition on the dry matter production and bromatological composition of Brachiaria brizantha cv. Marandu. Cienc. Anim. Bras. 6, 187–193. doi: 10.1590/1089-6891v17i332914
Costa, N. R., Andreotti, M., Gameiro, R. A., Pariz, C. M., Buzetti, S., and Lopes, K. S. M. (2012). Nitrogen fertilization in the intercropping of corn with two Brachiaria species in a no tillage system. (In Portuguese, with English abstract.). Pesqui. Agropecu. Bras. 47, 1038–1047. doi: 10.1590/S0100-204X2012000800003
Costa, N. R., Andreotti, M., Lopes, K. S. M., Yokobatake, K. L., Ferreira, J. P., Pariz, C. M., et al. (2015). Soil properties and carbon accumulation in an integrated crop-livestock system under no-tillage. Rev. Bras. Cienc. Solo 39, 852–863. doi: 10.1590/01000683rbcs20140269
Crusciol, C. A. C., Marques, R. R., Carmeis Filho, A. C. A., Soratto, R. P., Costa, C. H. M., Ferrari Neto, J., et al. (2016). Annual crop rotation of tropical pastures with no-till soil as affected by lime surface application. Eur. J. Agron. 80, 88–104. doi: 10.1016/j.eja.2016.07.002
Crusciol, C. A. C., Mateus, G. P., Nascente, A. S., Martins, P. O., Borghi, E., and Pariz, C. M. (2012). An innovative crop-forage intercrop system: early cycle soybean cultivars and palisade grass. Agron. J. 104, 1085–1095. doi: 10.2134/agronj2012.0002
Crusciol, C. A. C., Mateus, G. P., Pariz, C. M., Borghi, E., Costa, C., and Silveira, J. P. F. (2011). Nutrition and sorghum hybrids yield with contrasting cycles intercropped with Marandu grass. Pesq. Agropec. Bras. 46, 1234–1240. doi: 10.1590/S0100-204X2011001000017
Crusciol, C. A. C., Nascente, A. S., Borghi, E., Soratto, R. P., and Martins, P. O. (2015). Improving soil fertility and crop yield in a tropical region with palisadegrass cover crops. Agron. J. 107, 2271–2280. doi: 10.2134/agronj14.0603
Crusciol, C. A. C., Nascente, A. S., Mateus, G. P., Borghi, E., Leles, E. P., and Santos, N. C. B. (2013). Effect of intercropping on yields of corn with different relative maturities and palisadegrass. Agron. J. 105, 599–606. doi: 10.2134/agronj2012.0426
Crusciol, C. A. C., Nascente, A. S., Mateus, G. P., Pariz, C. M., Martins, P. O., and Borghi, E. (2014). Intercropping soybean and palisade grass for enhanced land use efficiency and revenue in a no till system. Eur. J. Agron. 58, 53–62. doi: 10.1016/j.eja.2014.05.001
Emerick, K., and Ronald, P. C. (2019). Sub1 rice: engineering rice for climate change. Cold Spring Harbor Perspect. Biol. 11:a034637. doi: 10.1101/cshperspect.a034637
Fageria, N. K. (1998). “Manejo da Calagem e Adubação do Arroz,” in Tecnologias Para Arroz de Terras Altas. Santo Antonio de Goiás: EMBRAPA-CNPAF, eds F. Breseghello and L. F. Stone (Embrapa Arroz e Feijao), 67–78.
Fageria, N. K., Baligar, V. C., and Bailey, B. A. (2005). Role of cover crops in improving soil and row crop productivity. Commun. Soil Sci. Plan. 36, 2733–2757. doi: 10.1080/00103620500303939
Fageria, N. K., Moreira, A., and Coelho, A. M. (2011). Yield and yield components of upland rice as influenced by nitrogen sources. J Plant Nutr. 34, 361–370. doi: 10.1080/01904167.2011.536878
Fischer, A. J., Ramirez, H. V., Gibson, K. D., and Pinheiro, B. D. S. (2001). Competitiveness of semidwarf upland rice cultivars against palisadegrass (Brachiaria palisadegrass) and signalgrass (B. decumbens). Agron J. 93, 967–973. doi: 10.2134/agronj2001.935967x
Food Agriculture Organization of the United Nations (FAO) (2017). Integrated Crop-Livestock Systems (ICLS). Available online at: http://www.fao.org/agriculture/crops/core-themes/theme/spi/scpi-home/managing-ecosystems/integrated-crop-livestock-systems/en/ (accessed 1 November, 2018).
Fox, D. G., Tedeschi, L. O., Tylutki, T. P., Russell, J. B., van Amburgh, M. E., Chase, L. E., et al. (2004). The Cornell Net Carbohydrate and Protein System model for evaluating herd nutrition and nutrient excretion. Anim. Feed. Sci. Technol. 112:29–78. doi: 10.1016/j.anifeedsci.2003.10.006
Franzluebbers, A. J., and Stuedemann, J. A. (2014). Crop and cattle production responses to tillage and cover crop management in an integrated crop–livestock systemin the southeastern USA. Eur. J. Agron. 57, 62–70. doi: 10.1016/j.eja.2013.05.009
Garcia, C. M. P., Costa, C., Andreotti, M., Meirelles, P. R. L., Pariz, C. M., Freitas, L. A., et al. (2016). Wet and dry maize yield under intercrop culivation with marandu grass and/or dwarf pigeon pea and nutritional value of the marandu grass in succession. Austral. J. Crop. Sci. 10, 1564–1571. doi: 10.21475/ajcs.2016.10.11.PNE183
Gitti, D. C., Arf, O., Portugal, J. R., Corsini, D. C. D. C., Rodrigues, R. A. F., and Kaneko, F. H. (2012). Coberturas vegetais, doses de nitrogênio e inoculação de sementes com Azospirillum brasilense em arroz de terras altas no sistema plantio direto. Bragantia 71, 509-517. doi: 10.1590/S0006-87052013005000002
Herrero, M., Thornton, P. K., Notenbaert, A. M., Wood, S., Msangi, S., Freeman, H. A., et al. (2010). Smart investments in sustainable food production: revisiting mixed crop-livestock systems. Science 327, 822–825. doi: 10.1126/science.1183725
Karki, S., Rizal, G., and Quick, W. P. (2013). Improvement of photosynthesis in rice (Oryza sativa L.) by inserting the C4 pathway. Rice 6:28. doi: 10.1186/1939-8433-6-28
Kluthcouski, J., Fancelli, A. L., Dourado-Neto, D., Ribeiro, C. M., and Ferraro, L. A. (2000). Yield of soybean, corn, common bean and rice under no-tillage management. Sci. Agric. 57, 97–104. doi: 10.1590/S0103-90162000000100016
Malavolta, E., Vitti, G. C., and Oliveira, S. A. (1997). Evaluation of Nutritional Status of Plants: Principles and Applications. Piracicaba: Potafós.
Marchezan, E., Daria, G. J. A., and Torres, S. (1992). Grain chalkness in three paddy rice cultivars. (In Portuguese, with English abstract). Scien. Agric. 49, 87–91. doi: 10.1590/S0103-90161992000400012
Mateus, G. P., Crusciol, C. A. C., Pariz, C. M., Borghi, E., Costa, C., Martello, J. M., et al. (2016). Sidedress nitrogen application rates to sorghum intercropped with tropical perennial grasses. Agron. J. 108:433–447. doi: 10.2134/agronj2015.0236
Mateus, G. P., Crusciol, C. A. C., Pariz, C. M., Costa, N. R., Borghi, E., Costa, C., et al. (2020). Corn intercropped with tropical perennial grasses as affected by sidedress nitrogen application rates. Nutr. Cycl. Agroecosyst. 1, 1–22. doi: 10.1007/s10705-019-10040-1
Mead, R., and Willey, R. W. (1980). The concepts of a ‘Land equivalent ratio' and advantages in yields from intercropping. Exp. Agric. 16, 217–228. doi: 10.1017/S0014479700010978
Meirelles, F. C., Arf, O., Garcia, N. F. S., Takasu, A. T., Buzo, F. S., Corsini, D. C. D. C., et al. (2019). Performance of upland rice cultivars at different sowing times: an alternative for crop rotation in low altitude Cerrado region, Brazil. Austr. J. Crop Sci. 13, 242–250. doi: 10.21475/ajcs.19.13.02.p1312
Momesso, L., Crusciol, C. A. C., Soratto, R. P., Vyn, T. J., Tanaka, K. S., Costa, C. H. M., et al. (2019). Impacts of nitrogen management on no-till maize production following forage cover crops. Agron. J. 111, 639–649. doi: 10.2134/agronj2018.03.0201
Moraes, A., Carvalho, P. C. F., Crusciol, C. A. C., Lang, C. R., Pariz, C. M., Deiss, L., et al. (2019). Integrated crop-livestock systems as a solution facing the destruction of Pampa and Cerrado biomes in South America by intensive monoculture systems. Agroecosyst. Div. 1, 257–273. doi: 10.1016/B978-0-12-811050-8.00016-9
Namuco, O. S., Cairns, J. E., and Johnson, D. E. (2009). Investigating early vigour in upland rice (Oryza sativa L.): Part I. seedling growth and grain yield in competition with weeds. Field Crop. Res. 113, 197–206. doi: 10.1016/j.fcr.2009.05.008
Nascente, A. S., Crusciol, C. A. C., and Cobucci, T. (2013). The no-tillage system and cover crops—alternatives to increase upland rice yields. Europ. J. Agron. 45, 134–131. doi: 10.1016/j.eja.2012.09.004
Pacheco, L. P., Leandro, W. M., Machado, P. L. O. A., Assis, R. L., Cobucci, T., Madari, B. E., et al. (2011). Biomass production and nutrient accumulation and release by cover crops in the off-season. Pesquis. Agropec. Bras. 46, 17–25. doi: 10.1590/S0100-204X2011000100003
Pariz, C. M., Andreotti, M., Tarsitano, M. A. A., Bergamaschine, A. F., Buzetti, S., and Chioderolli, C. A. (2009). Technical and economical performance of corn intercropped with Panicum and Brachiaria forage in crop-livestock integration system. Pesq. Agropec. Trop. 39, 360–370.
Pariz, C. M., Costa, C., Crusciol, C. A. C., Meirelles, P. R. L., Castilhos, A. M., Andreotti, M., et al. (2016). Production and soil responses to intercropping of forage grasses with corn and soybean silage. Agron. J. 108, 1–13. doi: 10.2134/agronj2016.02.0082
Pariz, C. M., Costa, C., Crusciol, C. A. C., Meirelles, P. R. L., Castilhos, A. M., Andreotti, M., et al. (2017). Production, nutrient cycling and soil compaction to grazing of grass companion cropping with corn and soybean. Nutr. Cycl. Agroecosyst. 108, 35–54. doi: 10.1007/s10705-016-9821-y
Portugal, J. R., Arf, O., Buzetti, S., Portugal, A. R. P., Garcia, N. F. S., Meirelles, F. C., et al. (2020). Do cover crops improve the productivity and industrial quality of upland rice? Agron. J. 112, 327–343. doi: 10.1002/agj2.20028
Raij, B., Andrade, J. C., Cantarella, H., and Quaggio, J. A. (2001). Análise Química Para Avaliação da Fertilidade de Solos Tropicais. São Paulo: Inst. Agron. De Campinas.
Rosolem, C. A., Ritz, K., Cantarella, H., Galdos, M. V., Hawkesford, M. J., Whalley, W. R., et al. (2017). Enhanced plant rooting and crop system management for improved N use efficiency. Adv. Agron. 146, 205–239. doi: 10.1016/bs.agron.2017.07.002
Salassi, M. E., Deliberto, M. A., Linscombe, S. D., Wilson, C. E. Jr., Walker, T. W., McCauley, G. N., et al. (2013). Impact of harvest lodging on rough rice milling yield and market price. Agron. J. 105, 1860–1867. doi: 10.2134/agronj2013.0238
Silva, E. C., Muraoka, T., Bastos, A. V. S., Franzin, V. I., Buzetti, S., Soares, F. A. L., et al. (2020). Biomass and nutrient accumulation by cover crops and upland rice grown in succession under no-tillage system as affected by nitrogen fertilizer rate. J. Crop Sci. Biotechnol. 23, 117–126. doi: 10.1007/s12892-019-0288-0
Silva, L. P., Alves, B. M., Silva, L. S., Pocojeski, E., Kaminski, T. A., and Roberto, B. S. (2013). Adubação nitrogenada sobre rendimento industrial e composição dos grãos de arroz irrigado. Ciênc. Rural 43, 1128–1133. doi: 10.1590/S0103-84782013005000055
Silva, S. C., Sabrissia, A. F., and Pereira, L. E. T. (2015). Ecophysiology of C4 forage grasses—understanding plant growth for optimising their use and management. Agriculture 5, 598–625. doi: 10.3390/agriculture5030598
Surve, V. H., and Arvadia, M. K. (2011). Performance of fodder sorghum (Sorghum bicolour L.), maize (Zea mays L.) and cowpea (Vigna unguiculata (L.) Walp.) under sole and intercropping systems. Int. J. Agric. Res. 2, 28–31.
Takim, F. O. (2012). Advantages of maize-cowpea intercropping over sole cropping through competition indices. J. Agric. Biodivers. Res. 1, 53–59.
Unicamp (2016) Centro de Pesquisas Meteorologicas e Climaticas Aplicadas a Agricultura. Clima dos Municípios Paulistas. Botucatu: Cepagri Meteorologia-UNICAMP. Available online at: https://www.cpa.unicamp.br/outras-informacoes/clima-dos-municipios-paulistas (accessed November 12, 2019).
United States Department of Agriculture (USDA) (2014). Keys to Soil Taxonomy, 12 Edn. Washington, DC, 372.
van Soest, P. J. (1994). Nutritional Ecology of the Ruminant. 2nd edn. New York, NY: Cornell Univ. Press. doi: 10.7591/9781501732355
Wood, S. A., and Mendelsohn, R. O. (2014). The impact of climate change on agricultural net revenue: a case study in the Fouta Djallon, West Africa. Environ. Dev. Econ. 20, 20–36. doi: 10.1017/S1355770X14000084
Yadav, G. S. A., Lal, R., Babu, S., Datta, M., Meena, R. S., Patil, S. B., et al. (2019). Impact of no-till and mulching on soil carbon sequestration under rice (Oryza sativa L.)-rapeseed (Brassica campestris L. var. rapeseed) cropping system in hilly agro-ecosystem of the Eastern Himalayas, India. Agric. Ecosyst. Environ. 275, 81–92. doi: 10.1016/j.agee.2019.02.001
Zarochentseva, O. (2012). Adaptation of Methodology Calculation Relative Crowding Coefficient for Evaluation Competition of Tree Species in Polyculture. Chernivtsi: Yuriy Fedkovich Chernivtsi Natl. Univ.
Zhou, L., Liang, S., Ponce, K., Marundon, S., Ye, G., and Zhao, X. (2015). Factors affecting head rice yield and chalkiness in indica rice. Field Crop. Res. 172, 1–10. doi: 10.1016/j.fcr.2014.12.004
Keywords: Oryza sativa L., Urochloa brizantha, Megathyrsus maximus, intercropping crops, sustainable agroecosystem
Citation: Crusciol CAC, Portugal JR, Momesso L, Bossolani JW, Pariz CM, Castilhos AM, Costa NR, Costa CHM, Costa C, Franzluebbers AJ and Cantarella H (2020) Overcoming Competition From Intercropped Forages on Upland Rice With Optimized Nitrogen Input to Food Production in Tropical Region. Front. Sustain. Food Syst. 4:129. doi: 10.3389/fsufs.2020.00129
Received: 26 March 2020; Accepted: 23 July 2020;
Published: 03 September 2020.
Edited by:
Paulo Mazzafera, Campinas State University, BrazilReviewed by:
Mohamed M. Hanafi, Putra Malaysia University, MalaysiaAdônis Moreira, Brazilian Agricultural Research Corporation (EMBRAPA), Brazil
Copyright © 2020 Crusciol, Portugal, Momesso, Bossolani, Pariz, Castilhos, Costa, Costa, Costa, Franzluebbers and Cantarella. This is an open-access article distributed under the terms of the Creative Commons Attribution License (CC BY). The use, distribution or reproduction in other forums is permitted, provided the original author(s) and the copyright owner(s) are credited and that the original publication in this journal is cited, in accordance with accepted academic practice. No use, distribution or reproduction is permitted which does not comply with these terms.
*Correspondence: Carlos A. C. Crusciol, Y2FybG9zLmNydXNjaW9sQHVuZXNwLmJy