- Department of Plant Agriculture, University of Guelph, Guelph, ON, Canada
Common bean (Phaseolus vulgaris L.) is the most important food legume crop worldwide. Canadian beans, especially large seeded cultivars of Andean origin, have relatively narrow genetic diversities. Establishing crops with mixtures of cultivars instead of pure lines is a simple, cost effective way to increase genetic diversity in the field. A number of studies have demonstrated the benefits of mixture cropping over monocropping in controlling disease, increasing water use efficiency, and increasing yield stability. The objective of this study was to determine the effects of increasing in-field diversity, by using mixtures of bean cultivars instead of monocultures, on productivity. The feasibility of growing bean cultivar mixtures in southern Ontario environments was confirmed with a small pilot study that was conducted with four bean cultivars and restricted number of mixtures at two locations in 2017. Mixture performance experiments were performed with seven diverse bean genotypes at two Ontario locations [Woodstock and Elora (two planting dates) research stations] as pure stands and all possible binary mixtures (planted in alternate rows or as completely random mixtures) in 2018. Conventional plot-based above ground crop data were collected. Mixing efficiencies were calculated from the yield data using a relative yield of the mixture (RYM) index. Diallel analysis was used to identify general mixing ability of cultivars and specific mixing abilities of mixtures. Significant differences among seven bean cultivars and their mixtures were identified in all three environments for all analyzed traits. The results indicated multiple benefits of planting mixtures compared to monocultures A number of mixtures overyielded component cultivars grown in pure stands; they had higher yields, RYM index values >1 and positive specific mixing abilities (for yield) in both types of biblends. The research has the potential to provide a theoretical basis for the use of precision agriculture tools to plant fields with mixtures instead of monocultures. It could lead to greater in-field diversity in the crop and in the above and below ground ecosystems that might provide greater buffering capacity and resiliency to the cropping system as well as increased ecosystem services.
Introduction
Coupled with effective inputs and improved management practices, plant cultivars that were improved during the green revolution doubled world grain production in 40 years (between 1960 and 2000) and averted predicted mass starvation and world order changes during a period of rapid global population growth (Khush, 2001; Tilman et al., 2002). Similar concerns exist about feeding a projected population of over nine billion people by year 2050 (Cardinale et al., 2012). However, there is increasing public interest in addressing food security concerns with crop production practices that increase diversity at all levels, including the soil microbiome, the crop cultivars, and the production systems that are utilized as well as reducing the use of inputs such as fertilizers and agrochemicals (Østergård et al., 2009; Bommarco et al., 2013; Gaba et al., 2014; Schipanski et al., 2016). A number of studies have demonstrated that mixed cropping systems have benefits over monocultures in reducing environmental impacts and in increasing above and below ground biodiversity, field productivity and yield stability, soil quality, weed/disease/pest control, energy use efficiency and profitability (Jolliffe and Wanjau, 1999; Malézieux et al., 2009).
Intraspecific mixtures (intracropping), which is simultaneous cultivation of two or more cultivars of the same species, can increase the in-field genetic diversity of a crop (Tooker and Frank, 2012; Vidala et al., 2017; Reiss and Drinkwater, 2018). The efficiency of these mixtures may be enhanced by blending high-yielding cultivars varying in their reactions to environmental stressors (Kiær et al., 2012). A meta-analysis of grain yields in cereals based on 26 published studies (documenting 246 instances of wheat and barley cultivar mixtures located in temperate regions) confirmed that yields in mixtures are higher than yields in pure stands. Mixing small grain cultivars is practiced in a number of regions in the world and management associated with these mixtures (mixing seeds, harvesting, and marketing) does not interfere with production (Mundt, 2002). In addition, the results obtained by Horner et al. (2019) from field pea mixtures, suggested that cultivar diversification increased yields and altered root bacterial and fungal communities and promoted their interactions.
Various spatio-temporal arrangements of crops can be utilized to perform intercropping, including planting mechanical mixtures of cultivars, or planting alternate rows, or strips in a field or planting them in relay. Each intercropping system is characterized by specific interactions between mixture components, with the highest frequency occurring in fields established with mixed crops. The selection of the mixing system depends largely on socioeconomic conditions and access to input, machinery and labor (Fageria, 1992).
Data from intercropping can be evaluated in a number of ways, including univariate and multivariate analyses of observed variables and usually, more than one method of analysis is necessary (Mead and Riley, 1981; Wijesinha et al., 1982; Mead, 1990; Federer, 1993). Diallel analysis was used to test for mixing ability analogous to genetic analysis of combining ability and select the best performing components for various intercrops. For example, Gallandt et al. (2001) used diallel analysis to evaluate pure stands and 15 biblends of six winter wheat cultivars grown in 33 environments in eastern Washington (USA). They identified a 1.5% yield advantage for mixtures compared to the average of their components grown in pure stands. In addition, significant correlations between yields of the mixtures and their components in pure stands suggested that mixture performance can be predicted from information from pure stands.
In addition to the raw data, numerous indices (which evaluate intercropping systems from agronomic, economic, ecological, and nutritional viewpoints) have been developed to assess yield potential, plant competition, and economic feasibility of various mixtures. Weigelt and Jolliffe (2003) reviewed over 50 indices commonly used in evaluating intercropping efficiencies. Indices such the relative yield of the mixture (RYM) calculates mixing efficiency by dividing yield response of the entire mixture by the average value of the mixture components evaluated in pure stands (Trenbath, 1974; Wilson, 1988). In mixtures with RYM = 1.0, there is no mixing (intercropping) effect; value of RYM <1.0 indicates mixing disadvantage (some type of competition among mixture components); and, mixtures having RYM >1.0 are more efficient than the monocultures (facilitation, complementarity, or avoidance of competition between the mixture components) (Williams and McCarthy, 2001).
Since intercropping performance can be affected by numerous factors, including crop selection (number and species), plant density, and competition among mixing components, the selection of the right index is critical in evaluating and interpreting the potential interactions and advantages of intercropping (Weigelt and Jolliffe, 2003; Bedoussac and Justes, 2011). Considering that there is no perfect index (Williams and McCarthy, 2001), the use of several indices, especially when more than one aspect of competition is being studied, could be beneficial.
The common (dry) bean (Phaseolus vulgaris L.) is the most important legume crop consumed directly by humans. Similar to other major crops, domestication and migration reduced the genetic diversity in common bean, particularly in the Andean compared to the Mesoamerican gene pool (Bitocchi et al., 2013). Similarly, a pedigree-based estimate of genetic diversity of Canadian bean cultivars indicated that the large seeded beans of Andean origin (such as kidney and cranberry) were most limited in their genetic diversity (Navabi et al., 2014).
A cost-effective way to increase in crop genetic diversity is to grow mixtures of cultivars, which is a common practice in many regions of Africa and South America. For example, in Eastern Africa, small holder farmers are planting common bean cultivar mixtures composed of two to 30 components varying in seed color, shape and size (Smithson and Lenne, 1996). Similarly, farmers in many regions of Brazil, are sowing mixtures of several bean lines (multilines) to ensure greater yield stability (Botelho et al., 2008). It was shown that the mixtures of bean cultivars can reduce the development and spreading of anthracnose (Ntahimpera et al., 1996; Prasad et al., 2016) and bean fly infestations (Ssekandi et al., 2016) in fields. Federer et al. (1982) compared the yields of 28 dry bean biblends in equal proportion to evaluate the mixing effect of the cultivars. Some cultivars had higher specific mixing effects than others, but the results were not consistent over environments. Similarly, Riley et al. (1993) identified inconsistent responses for seed yield among common bean biblends across five different mixing ratios. This might be partially due to a narrow genetic diversity resulting in combinations of components from the same gene pool.
Southern Ontario is a major bean growing region in Canada (http://www.pulsecanada.com/producers-industry/about-pulses/growing-regions/) but there is no information on the performance of bean cultivar mixtures for this area. Therefore, the main objective of this study was to determine the agronomic (including crop yield) effects of increasing crop diversity in Ontario production systems by using common bean cultivars bred for this region in mixtures instead of monocultures. The work also tested the effects of the mixture type, namely, random mixing, or planting components in alternating rows on the performance of the mixture. In addition, the potential of predicting mixture performance from information measured from its components evaluated in pure stands, was tested. To meet these objectives, a number of diverse, high yielding common bean genotypes were evaluated for their performance in pure stands and binary mixtures, using a replacement design at a single density, in replicated trials in two Ontario locations in 2017 and 2018.
Materials and Methods
Genotype Selection and Mixture Formation
The 2017 mixture study was initiated with four diverse, high yielding genotypes from the University of Guelph bean breeding program, including small seeded (Mesoamerican) white bean cultivars Lighthouse (Khanal et al., 2017) and Rexeter (Smith et al., 2012a), a black bean breeding line ACUG 15-B4 (registered as OAC Vortex in 2019), and a large seeded (Andean) light red kidney bean OAC Inferno (Smith et al., 2012b). In 2018, three additional cultivars were added, including a navy bean cultivar Bolt (Khanal et al., 2016b), a dark red kidney bean cultivar Dynasty (Khanal et al., 2016a), and a cranberry bean cultivar Red Rider (Park et al., 2009). Therefore, seven diverse genotypes [four Mesoamerican (small seeded: Lighthouse, Rexeter, Bolt and OAC Vortex) and three Andean (large seeded: OAC Inferno, Dynasty and Red Rider) gene pool] were evaluated in this study in 2018. The genotypes varied in growth habit, seed size, maturity, and resistance to three major common bean diseases {CBB [common bacterial blight caused by gram-negative bacteria Xanthomonas axonopodis pv. phaseoli (Xap)], Ant [Anthracnose caused by seed-borne fungus Colletotrichum lindemuthianum(Sacc. & Magnus) Brosi & Cav.] and BCMV (Bean common mosaic virus, a member of the genus Potyvirus) an aphid-transmitted seed borne viral disease}. These genotypes (except new cultivar OAC Vortex) are checks in the trials performed by the Ontario Pulse Crop Committee (Table 1).
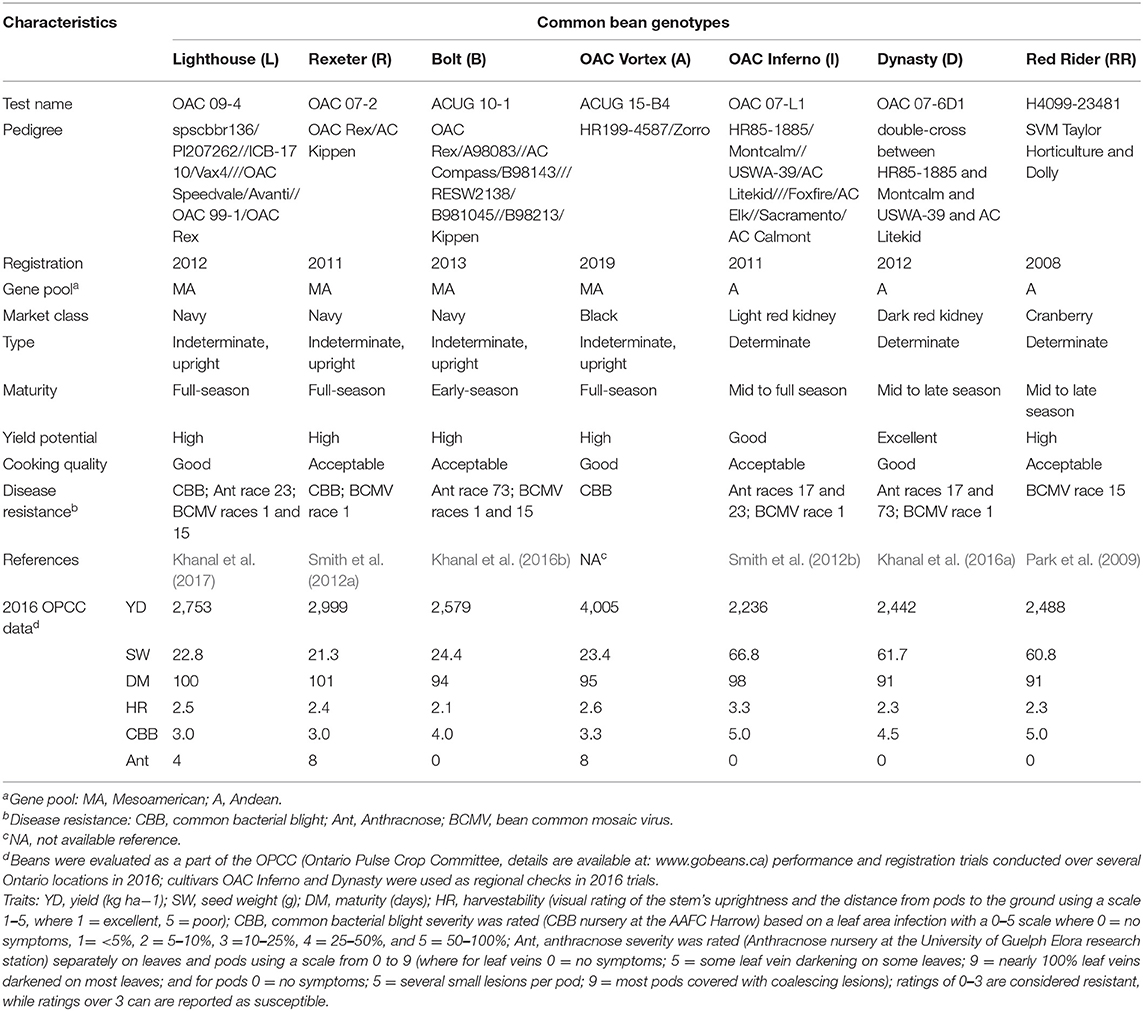
Table 1. Characteristics of seven common bean genotypes selected for the mixture study conducted at two Ontario locations in 2017 and 2018.
The genotypes were evaluated in pure stands and two types of binary mixtures [alternate row mixture (ARM) and same row (completely random) mixture (SRM), as described in Essah and Stoskopf, 2002]. An equal proportion replacement (substitutive) design at a single density was used as a mixing technique (Jolliffe, 2000). The aim of the work in 2017 was to test the feasibility of mixing bean cultivars in Ontario environments and only a limited number of mixtures were formed. In all genotypic mixtures, the white bean cultivar Lighthouse was mixed with one of the other three genotypes [Rexeter, OAC Vortex (ACUG 15-B4) or OAC Inferno] in a 1:1 seed number ratio. In 2018, binary mixtures were formed for all possible combinations of seven cultivars (Lighthouse, Rexeter, OAC Vortex, OAC Inferno, Bolt, Dynasty, and Red Rider). The mixtures and pure stands had the same density (n) and each mixture component had a n/2 density (Figure 1).
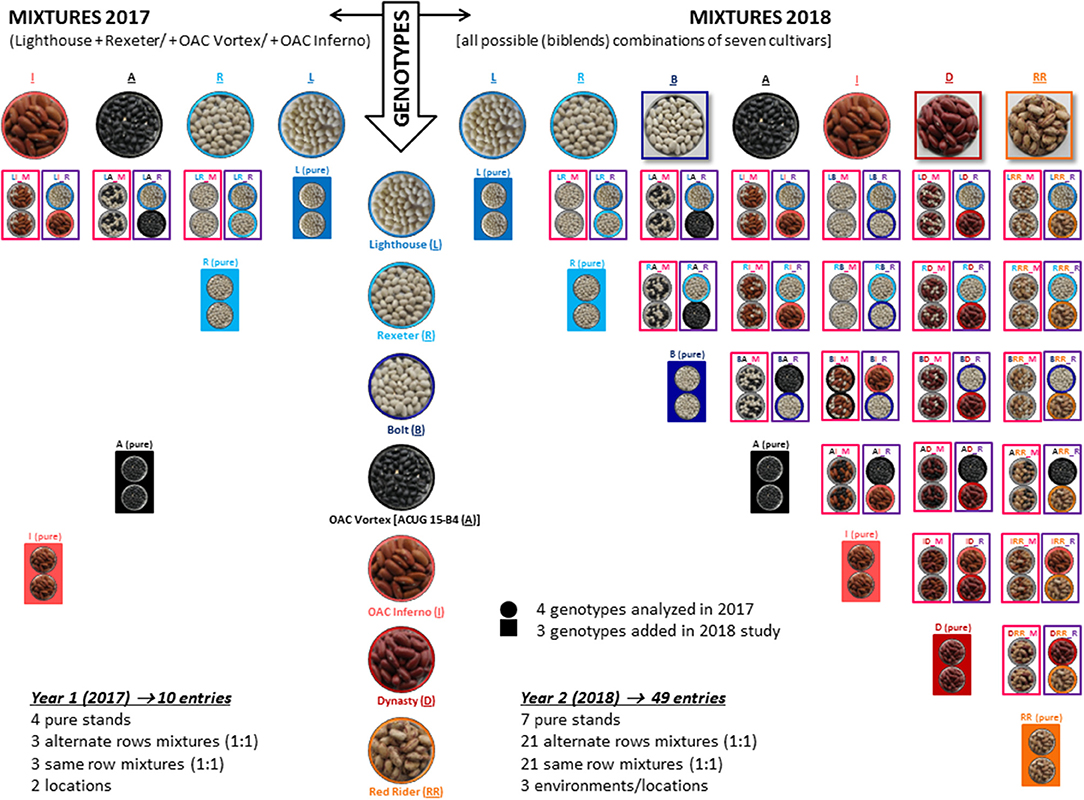
Figure 1. Formation or binary mixtures among seven common bean cultivars evaluated in three Ontario environments/locations over 2 years. All mixtures were formed by mixing two genotypes in a 1:1 seed number ratio. In 2017 (left), mixtures were formed with four cultivars [Lighthouse (white), Rexeter (white), OAC Vortex (black), and OAC Inferno (light red kidney)]; Lighthouse was mixed with one of the remaining three genotypes. In 2018 (right), binary mixtures were formed in all possible combination of seven cultivars [Lighthouse, Rexeter, Bolt (white), OAC Vortex, OAC Inferno, Dynasty (dark red kidney), and Red Rider (cranberry)]. Genotypes were evaluated in pure stands and two types of binary mixtures [alternate rows mixture (ARM) and same row (complete random) mixture (SRM)]. An equal proportion replacement (substitutive) design at a single density was used as a mixing technique (Jolliffe, 2000). Mixture names are composed of three letters: the first letter indicates cultivar component 1 [L (Lighthouse), R (Rexeter), B (Bolt), A (OAC Vortex, work initiated with the test name ACUG 15-B4), I (OAC Inferno), D (Dynasty), and RR (Red Rider)], the second letter indicates cultivar component 2 and the third letter represents mixture type [M stands for the same row mixture (SRM) and R is for the alternate row mixture (ARM)]. Mixtures and pure stands had the same density (n) and each mixture component had an n/2 density (for details Figure S1).
Mixture names are composed of three letters: the first letter indicates cultivar component 1 [L (Lighthouse), R (Rexeter), B (Bolt), A (OAC Vortex, work initiated with the test name ACUG 15-B4), I (OAC Inferno), D (Dynasty) and RR (Red Rider)], the second letter indicates cultivar component 2 and the third letter represents mixture type [M stands for the same row mixture (SRM) and R is for the alternate row mixture (ARM)].
Field Evaluation
Field experiments were conducted at University of Guelph research stations at Elora [ERS; 2,680 CHU (Crop Heat Unit; Brown, 1993), London loam soil] and Woodstock (WRS; 2,890 CHU, Guelph loam soil; Voisey, 1971), Ontario in 2017 and 2018. In both years, the previous crop was winter wheat at the WRS. At the ERS in both years the first planting followed alfalfa and the second (2018) planting followed barley.
In 2017, the trials with 10 entries (four pure stands, three SRMs, and three ARMs) were carried out using a randomized complete block design (RCBD) with four replications. The rows were 6 m long and between rows spaces were 0.36 m. Each entry was planted in two adjacent 4-row plots (88 seeds row−1) with a distance of 0.50 m between plots. Experiments were machine planted on June 6, 2017 at the WRS and on June 7, 2017 at the ERS. At both locations, fertilizer [200 kg ha−1 of 5-20-20 (10 kg ha−1 of N, 40 kg ha−1 of P and 40 kg ha−1 of K)] and herbicides [ppi tank mix of Pursuit® (BASF-Canada) at 200 ml ha−1 (active ingredient Imazethapyr at 48 g ha−1) and 1.75 l ha−1 of Dual II Magnum® (active ingredient S-Metolachlor Group 2 at 1.6 kg ha−1, Syngenta-Canada)] were applied before planting. No other chemicals were applied to control anthracnose, white mold and/or bacterial blights in the fields. Plots were combine harvested on September 19, 2017 at WRS and on September 29, 2017 at ERS.
In 2018, the trials with 49 entries (seven pure stands, 21 SRMs, and 21 ARMs) were planted as a square (7 × 7) lattice design with four replications. The experiments were machine planted on June 5, 2018 at the WRS and on June 7, 2018 for the first planting and on June 12, 2018 for the second planting at the ERS. The row spacing, seed density, fertilizer, and herbicide applications were same as in the 2017 experiments. The plots were combine harvested on September 18, 2018 at WRS and on October 10, 2018 planting 1 and October 23, 2018 planting 2 at ERS, respectively.
Data Collection
In all mixtures, both genotypic components were harvested and analyzed together. Plot-based crop data were collected for the conventional above ground traits. Flowering was determined as the number of days from planting to 50% of plants in a plot with at least one fully opened flower. Maturity was reported as the number of days from planting to 95% of the pods in a plot being dry and ready to harvest. Plant height (cm) was measured from the soil surface to the tip of a main stem at the mid-pod filling stage. Seed weight (g) was computed as a weight of 100 seeds adjusted to 18% moisture content. Yield was a measure of a plot seed weight, expressed in kg ha−1, and adjusted to 18% moisture content. Harvestability was determined at maturity using a scale of 1 (erect, suitable for direct combining harvest) to 5 (prostrate, unsuitable for machine harvesting). Relative leaf chlorophyll content was quantified as greenness of the plants using a portable non-destructive SPAD (Soil Plant Analysis Development) chlorophyll meter (SPAD-502, Minolta, Japan) on eight, fully expanded, young leaves in each plot, and expressed as SPAD values. In 2017 the SPAD readings were done at a mid-pod filling stage [63 days after planting (DAP) on four randomly selected plants (two leaves/one reading)] (Monje and Bugbee, 1992). In 2018, the SPAD readings were collected at full flowering [43/44 DAP on eight randomly selected plants (one reading, one leaf, one plant row−1)]. Anthracnose disease reaction was evaluated under natural field infection using a 0–9 scale (Pastor-Corrales et al., 1995), where entries with ratings of 0–3 were considered resistant and ratings 4–9 were marked as susceptible. No anthracnose symptoms were identified in 2018.
Mixing efficiency was calculated from the yield data using indices applicable for the mixtures in which separate crop responses are not available (Figure S1). A relative yield of the mixture (RYM) index was calculated with the formula RYM = YM/[(YP1+YP2)/2] (Trenbath, 1974; Wilson, 1988). In the formula, YP1 and YP2 are yields of pure stands of cultivar component 1 (P1) and component 2 (P2), respectively; YM is a yield of the cultivar mixture (combined response of two mixture cultivar components). RYM measures weather the yield of the mixture is greater than the mean of the two monocultures (considers the proportion at which the species were grown). The values of RYM >1.0 indicate greater yield of the mixture compared to the mean of the two monocultures.
Additionally, mixtures were compared to the average of the two cultivar components (mid-parent) as well as a better performing cultivar component (better parent) when evaluated in a pure stands. Mid-component (parent) Superiority (MS, or relative measure of mixing effect) was calculated using the formula MS = {[YM - (YP1 + YP2)/2]/(YP1 + YP2)/2} × 100 (Kiær et al., 2012; Wang et al., 2016). The same results can be obtained from the RYM values using the formula: YI = (RYM - 1) × 100 (Williams and McCarthy, 2001), as the percentages of the overall yield increase or decrease (YI). Better-component (parent) Superiority (BS, or maximal mixing effect or overyield) was calculated using the formula BS = [(YM - YBP)/YBP] × 100 (Trenbath, 1974; Kiær et al., 2012; Wang et al., 2016). In the formula, BP indicates a better performing (higher yielding) cultivar component evaluated in a pure stand. The advantage of mixing bean cultivars was also calculated by comparing yield (kg ha−1) of the mixture with the difference between the mixture yield and yield of the cultivar component evaluated as monocrops (midcomponent, better yielding component, and lower yielding component).
Data Analysis
Crop data were analyzed as RCBD (2017) and lattice design (2018) using the generalized linear mixed models (Glimmix) procedure in SAS (Statistical Analysis System) v.9.4 software (SAS Institute, 2013). Treatments [cropping systems (genotypes)], locations, and interactions were considered as fixed effects. Replications (block and iblock) were evaluated as random effects using “random” and “covtest” statements. When necessary, the “nobound” option was used to remove boundary constraints on covariances (0), which allows their estimates to be negative. A few outliers were removed, and data were re-analyzed. If significant treatment differences were detected, pairwise multiple comparisons (LSmeans) were made using a Tukey HSD test. In addition, treatments [cropping system: SRM (same row mixture), ARM (alternate rows mixture) and PS (pure stand)] were compared using the “LSMestimate” statement. Significance was declared at p < 0.05. Residual analysis diagnostic plots were used to check for assumptions for Gaussian distribution (normality, homogeneity of error variances) and to identify the most suitable solutions if violations were identified (Kozak and Piepho, 2017).
Although, generally considered as a random effect in statistical analysis (as it is difficult to repeat location effects year after year), locations were considered as a fixed effect in the current study. At University of Guelph, research stations at Elora and Woodstock are routinely used for field experiments. There is 210 CHU difference between these locations; in general, if planted in the same time, beans grown at WRS will mature ~1 week earlier. However, in order to apply the results over wider bean production area instead of limiting the results to Elora and Woodstock in 2017 or 2018, the average of the locations was also presented.
After identifying significant genotype effects, diallel analysis was performed for the seven measured traits. The analysis was done using the Plant Breeding Tools (PBTools) software (IRRI, 2014) based on the Griffing model 2 [pure stands (parents) and mixtures (F1s)] (Griffing, 1956) method 1 (fixed effects). To determine the relative importance of the General Mixing Ability [GMA of pure lines, similar to the General Combining Ability (GCA) of parents in genetic studies] and Specific Mixing Ability [SMA of binary mixtures, similar to the Specific Combining Ability (SCA) of F1s in genetic studies] effects, the GMA/SMA (GCA/SCA) variance ratios were calculated as 2MSGMA/2MSGMA + MSSMA (Baker, 1978). A ratio closer to unity (1) indicates higher importance of the GMA effects.
The relationships among traits (raw data) at the experiment as well as treatment levels (cropping system) were analyzed by Pearson's correlation in SAS. Significant relationships among a number of traits suggested that multivariate (principal component, PCA) analysis (LSmeans) would be a suitable method for data reduction. After calculation (princomp in SAS), trait eigenvalues, and cultivar scores were exported to Microsoft Excel to produce Genotype x Traits (GT) biplots where angles between variable vectors indicate the level of association between them.
Soil Analysis and Weather Conditions at ERS and WRS in 2017 and 2018 Bean Growing Seasons
Daily weather data for two experimental years (2017 and 2018) at two locations (ERS and WRS) for the bean growing season (May to October) were collected at the nearest weather stations (available at: http://climate.weather.gc.ca/climateData/dailydata_e.html).
Prior to planting in 2018, a standard soil sample probe (Oakfield Classic Soil Probe) was used to randomly collect 10–16 soil samples at 0–9 inch (22.86 cm) depths within each replication to create a bulk sample. After hand mixing, representative samples were sent for analysis to the SGS Agri-Food Laboratories Inc., Guelph, ON.
Results
Soil Properties and Environmental Conditions
The plot soils were analyzed before planting the field trials in 2018. The soils at the three fields had different physico-chemical properties (Table S1). The field at the first planting at the ERS (1ERS18) had highest content of N (both nitrate and ammonium), K, Mg, and Ca as well as cation exchange capacity (CEC). On the other hand, soil at the WRS (WRS18) had highest content of P, Zn, Mn, Cu, and Fe. All of the other parameters had very similar values for the three fields.
The beans were in the field 105 days at the WRS and 114 days at the ERS in the 2017 pilot study. In 2018, beans were in the field 105 days at the WRS and 125 days at the ERS first planting and 133 days at the ERS second planting, respectively. During the bean growing season (June - September/October), the weather conditions (daily temperature and precipitation) at ERS varied considerably in two experimental years (Figure S2). However, due to the incomplete weather data for the WRS site direct comparison between the two sites was not possible for the whole bean growing seasons. As expected, in both years the warmer location was WRS resulting in earlier maturity. This site also received more rainfall in 2017 (more complete data). It received 259.8 mm precipitation (July 89.1 mm, August 108.2 mm) compared to 154.8 mm (July 32.4 mm, August 44.7 mm) rain at ERS for the periods with data collected for both sites, Although more fragmentary, 2018 data shown that WRS received more rainfall for overlapping periods in June (23.6 mm WRS and 0.8 mm ERS) and August (43.1 mm WRS and 26.2 mm ERS) (Figure S2).
Feasibility of Growing Bean Cultivar Mixtures
In 2017, significant interactions between genotypes and locations were identified for flowering, maturity, harvestability, and seed weight. The presence of the interactions for some traits implies that different patterns of responses occurred for the pure stands and the mixtures at the two locations. Significant differences among four genotypes and their mixtures were identified in both locations for the majority of the analyzed traits, except anthracnose, and yield-based indices (Table S2). Similarly, the location effect was significant for most of the traits, except for harvestability, SPAD, and yield-based indices. Beans evaluated at the WRS matured earlier and had higher yields compared to the mixtures grown at the ERS (Figure 2).
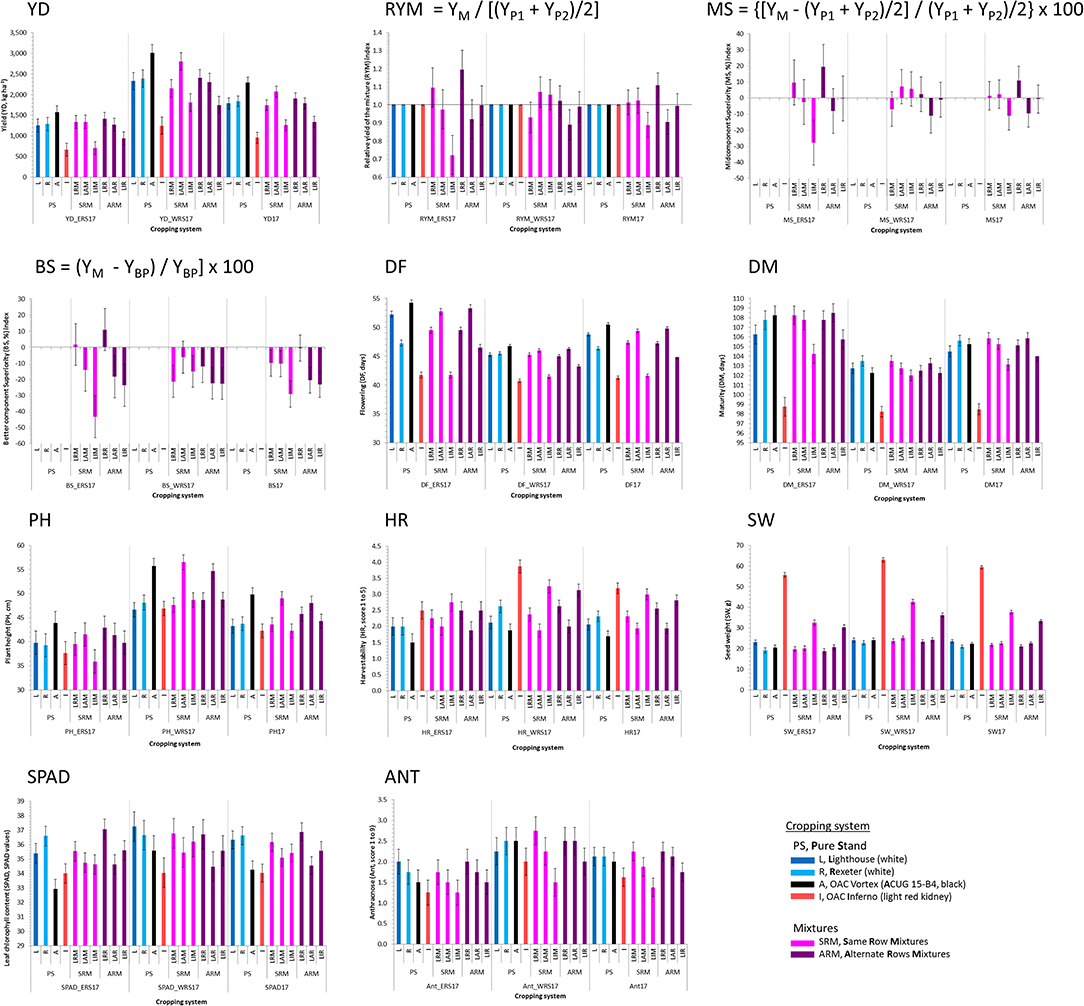
Figure 2. Trait means and standard errors of the four common bean genotypes evaluated in pure stands and binary mixtures [same row mixture (SRM) and alternate rows mixture (ARM)] at two Ontario locations [Elora (ERS) and Woodstock research stations (WRS)] in 2017 (pilot) study. Traits: Yield (YD, kg ha−1), three yield-based indices [relative yield of the mixture (RYM), midcomponent superiority (MS), and better component superiority (BS)] and seven other traits [days to flowering (DF, days), days to maturity (DM, days), plant height (PH, cm), harvestability (HR, scale 1–5), seed weight (SW, g), and leaf chlorophyll content (SPAD, SPAD values).
The yields of the bean mixtures were mostly similar to the averages of their component cultivars but, they were not always consistent at the two locations. The values of the RYMs varied between locations indicating inconsistent relative mixing efficiencies of the bean biblends. A consistent yield advantage of the mixture, in terms of RYMs, was observed for mixtures between two white bean cultivars Lighthouse and Rexeter. Although statistically not significant, yield increases of 2.3% (WRS) and 19.5% (ERS) in LRR (ARM biblend) and 9.7% (ERS) in LRM (SRM) mixtures that were measured compared to monocrops of Lighthouse and Rexeter (Figure 2), indicated that it would be advantageous to grow mixtures compared to the pure lines. The yield and RYM values of the SRMs were higher compared to ARMs at WRS in 2017, however, the differences between mixture types (SRM vs. ARM) for these measures were not significant (Table S3).
Performance of Bean Cultivar Mixtures
In 2018, significant interactions between genotypes and locations were identified for all analyzed traits. This confirmed results of the initial mixture experiments conducted in 2017 indicating different patterns of responses for the pure stands and the mixtures over the three environments. Similarly, a significant location effect was identified for most of the analyzed traits except for yield and plant height. Significant differences among seven bean cultivars and mixtures were identified for all analyzed traits, except for the plant height at the WRS (Table S4).
Yield and Yield-Based Indices
Beans evaluated at the WRS matured earlier and had higher yields compared to the mixtures grown in two plantings at the ERS. As expected, small seeded (Mesoamerican) beans yielded on average 1,058 kg ha−1 more compared to the large seeded (Andean) genotypes (Table S5). Among the seven genotypes evaluated in pure stands, the highest mean yield value (averaged over the three environments) was obtained for the small seeded black bean cultivar OAC Vortex (4,787 kg ha−1) and the lowest (2,760 kg ha−1) was in the dark red kidney cultivar Dynasty (Figure 3). On average, the SRM biblends yielded slightly higher compared to the ARMs, but the difference (on average, 10 kg ha−1) was not significant. However, within each type of mixture, Mesoamerican (M) biblends were significantly higher yielding compared to both Andean (A) and between gene pool (Mesoamerican + Andean, MA) mixtures (Table S5). The highest mean yield value (averaged over three environments) for the SRMs was found in the biblend of two small seeded genotypes Rexeter and OAC Vortex (RAM, 4,548 kg ha−1). The lowest yield mean value for the SRMs was found in a biblend of two large seeded cultivars Dynasty and Red Rider (DRRM, 2,851 kg ha−1). In ARMs, the highest yielding was a biblend of two small seeded genotypes OAC Vortex and Bolt (BAR, 4,107 kg ha−1). The lowest yielding ARM was a mixture between two large seeded cultivars, Dynasty and OAC Inferno (2,908 kg ha−1). In general, between gene pool mixtures (both SRMs and ARMs) had intermediate yield values that were closer to the values obtained for the pure small seeded beans. Similar yield trends were observed in separate environments/locations (Table S6).
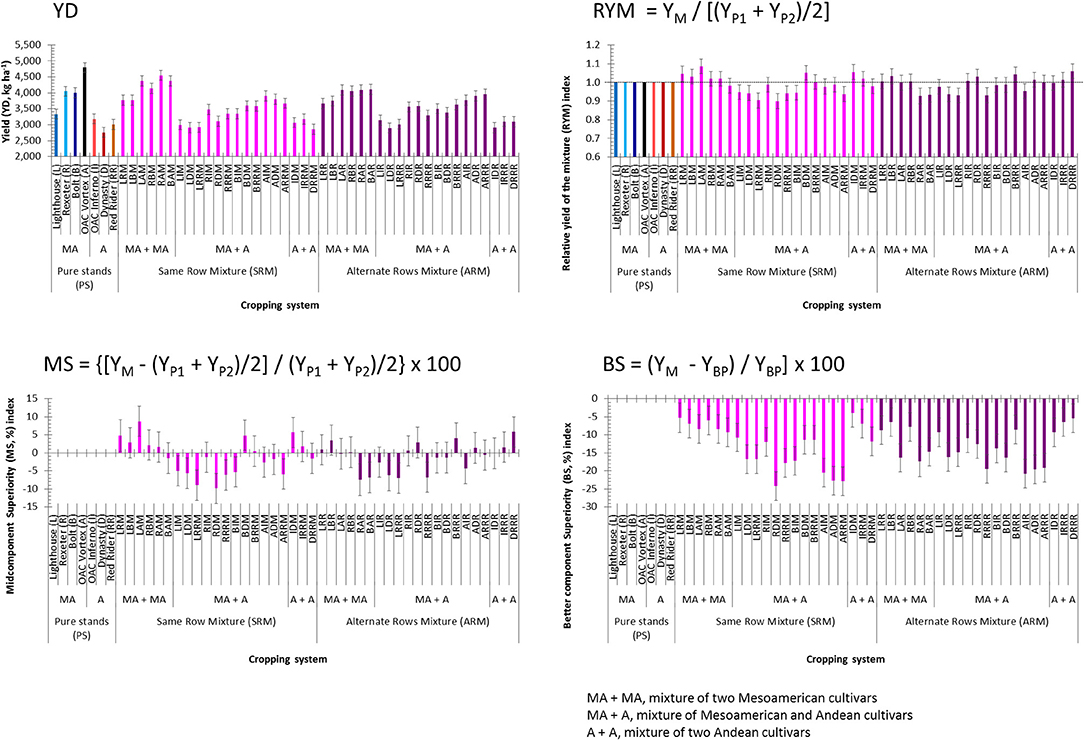
Figure 3. Trait means and standard errors of the seven common bean genotypes evaluated in pure stands and binary mixtures [same row mixture (SRM) and alternate rows mixture (ARM)] averaged over three Ontario environments/locations in 2018. Traits: yield (YD, kg ha−1) and three yield-based indices [relative yield of the mixture (RYM), midcomponent superiority (MS) and better component superiority (BS)]. In each histogram, cropping system [seven cultivars in pure stands, 21 SRMs (same row mixtures) and 21 ARMs (alternate row mixtures)] and gene pool [MA, Mesoamerican; A, Andean; MA + MA, mixture of two Mesoamerican cultivars; MA + A, mixture of Mesoamerican and Andean cultivars; A + A, mixture of two Andean cultivars].
For the yield-based indices, significant differences between SRM and ARM type of the bean biblends were identified only for the BS (better component superiority) index (Table S5). Averaged over three environments, the values of the RYM (relative yield of the mixture) index higher than unity and with positive MS (midcomponent superiority) indices were identified for five out of six Mesoamerican SRM mixtures (Figure 3). The highest RYM was observed in biblend of two small seeded cultivars OAC Vortex and Lighthouse (LAM, 1.087) indicating better performance (8.7% higher yield) in the mixture compared to the cultivar components evaluated in pure stands. Two out of three Andean SRM biblends outperformed monocropped cultivars. The IDM mixture had a 5.6% better yield compared to the cultivars OAC Inferno and Dynasty grown in pure stands. In ARM biblends, three out of six Mesoamerican (LRR, LBR, and RBR) and two out of three Andean (IRRR and DRRR) combinations had higher yields than their component cultivars evaluated as monocrops. In addition, two SRMs (BDM and BRRM) and four ARMs (RIR, RDR, BRRR and ADR) overyielded monocropped cultivar components (Figure 3). However, all mixtures had negative values for the BS index, indicating that none of the biblends outperformed the better cultivar component in the mixture, for both the SRM and ARM formats (Figure 3). Similar trends for all three indices were observed in separate environments (Table S6).
Other Characteristics
Significant differences between Mesoamerican and Andean genotypes in pure stands were identified for all additional traits. On average, Mesoamerican genotypes flowered (+6.5 days) and matured (+4.2 days) later, were taller (+4.9 cm), but had better harvestability (−0.434), lighter seeds (−41.4 g), and higher leaf chlorophyll contents (+2.7 SPAD values) compared to the Andean genotypes evaluated in pure stands (Table S5). The cultivar Lighthouse had the longest flowering (46.4 days) and maturity (100.7 days) but the highest SPAD reading (39.0 SPAD values). The black bean cultivar OAC Vortex was the tallest (60.4 cm) while the white cultivar Rexeter had the lightest seeds (21.1 g). The best (lowest) harvestability score was recorded in cultivar Bolt (1.5). The Andean cultivar Dynasty had the earliest flowering time (37.8 days) and the lowest SPAD reading (34.2 SPAD values) while the cranberry cultivar Red Rider was the shortest plant (48.8 cm) and had the earliest maturity (90.1 days, same as the small seeded cultivar Bolt) (Figure 4).
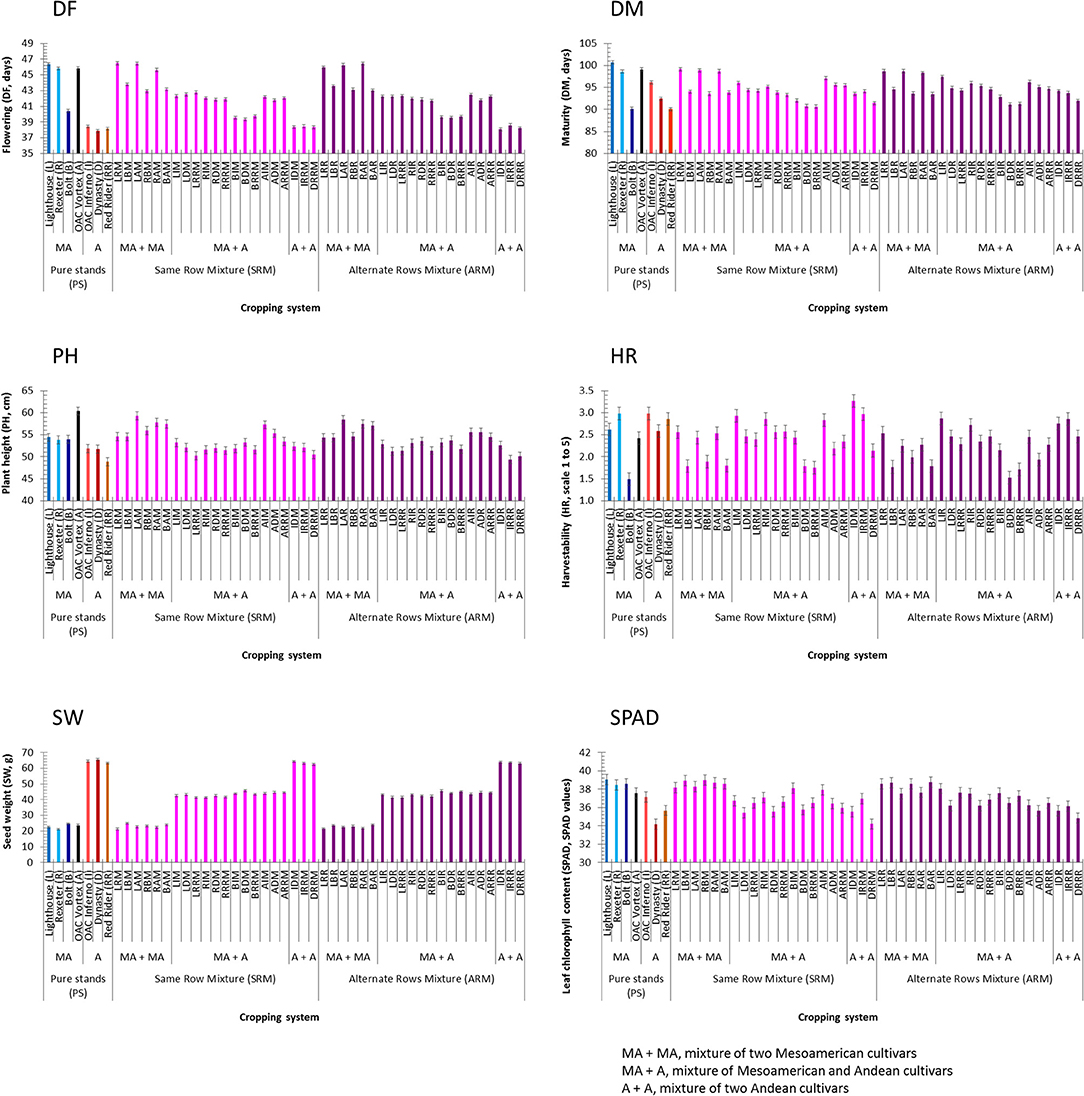
Figure 4. Trait means and standard errors of the seven bean genotypes evaluated in pure stands and binary mixtures [same row mixture (SRM) and alternate rows mixture (ARM)] averaged over three Ontario environments/locations in 2018. Traits: days to flowering (DF, days), days to maturity (DM, days), plant height (PH, cm), harvestability (HR, scale 1 to 5), seed weight (SW, g), leaf chlorophyll content (SPAD, SPAD values)]. In each histogram, cropping system [seven cultivars in pure stands, 21 SRMs (same row mixtures) and 21 ARMs (alternate row mixtures)] and gene pool [MA, Mesoamerican; A, Andean; MA + MA, mixture of two Mesoamerican cultivars; MA + A, mixture of Mesoamerican and Andean cultivars; A + A, mixture of two Andean cultivars].
For the mixtures, the SRMs matured earlier (−0.25) and had poorer harvestability scores (+0.12) compared to the ARMs. However, there were no significant differences between the two biblend types for flowering, plant height, seed weight, and SPAD (Table S5). In the SRM biblends, the highest values for flowering (46.5 days, LRM), maturity (99.1 days, LRM), plant height (59.4 cm, LAM), harvestability (3.27 score, BAM), and SPAD reading (39.0 SPAD values, BAM) but the lowest for seed weight (21.3 g, LRM) were observed in Mesoamerican mixtures. On the other hand, the lowest values for flowering (38.3 days, DRRM) and SPAD reading (34.2 SPAD values, DRRM) but the highest value for seed weight (64.3 g, IDM) were recorded in Andean SRMs. Maturity (90.6 days) and harvestability (1.75 score, lower is better) had the lowest values in the BRRM while the LRRM biblend had the shortest plant (50.2 cm), both between gene pool (Mesoamerican + Andean) biblends (Figure 4).
In the ARM biblends, the highest values for flowering (46.45 days, RAR), maturity (98.7 days, LRR and LAR), plant height (58.4 cm, LAR) and SPAD reading (38.7 SPAD values, BAR) but lowest value for seed weight (21.5 g, LRR) were identified in Mesoamerican mixtures. The highest value for seed weight (63.9 g) but the lowest values for flowering (38.1 days, IDR), plant height (49.4 cm, IRRR), and for SPAD reading (34.8 SPAD values, DRRR) were observed in Andean ARMs. The lowest value for maturity (91.1 days) was found in the BDR (Mesoamerican + Andean) mixture. Both, the lowest and the highest values for harvestability [1.53 score (BDR) and 2.86 score (LIR)] were observed in between gene pool (Mesoamerican + Andean) mixtures (Figure 4). Similar trends in these traits were identified when environments were analyzed separately (Table S6).
Relationships Among the Traits
Exploratory correlation analysis with raw data identified significant relationships among the evaluated traits (not shown). The majority of the correlations were confirmed using LSmeans (N = 49) averaged over the three environments (Table 2) or for each environment/location separately (Table S7). Significant positive associations were identified between the three environments for all analyzed traits except the RYM index (Table S7). Some traits were associated with a number of other traits. For example, yield was significantly positively correlated with flowering, plant height and SPAD reading but in an inverse relationship with seed weight. Flowering was positively correlated with yield, maturity, plant height and SPAD reading but negatively correlated with seed weight (Table 2, Table S7). The SPAD reading was significantly positively associated with yield and flowering but was negatively related with seed weight. In contrast, the RYM index was not significantly correlated with any of the analyzed traits.
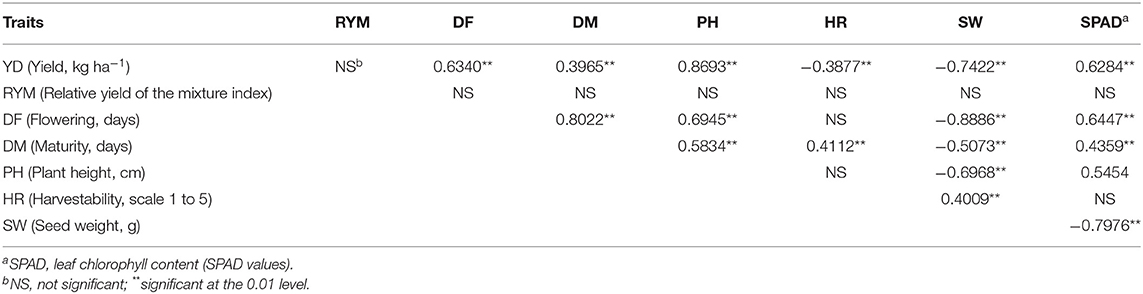
Table 2. Correlations among analyzed traits averaged over three Ontario environments in 2018 (LSmeans, N = 49).
These correlations suggested that there are complex relationships among the traits and a principal component analysis (PCA), based on the correlation matrix, was used to reduce the dimensionality of the data. Using the Kaiser criterion (Kaiser, 1958), 10 principal components (PC) were identified that had eigenvalues >1 and cumulatively explained 96.3% [48.92% (PC1) to 1.44% (PC10)] of the variation for evaluated traits (Table 3, Figure S3). The first two PCs explained 65.8% of the variation. Averaged over three environments, PC1 had large positive associations with yield, flowering, plant height, and SPAD and was negatively associated with seed weight. PC2 had large positive associations with maturity and harvestability (Figure 5).
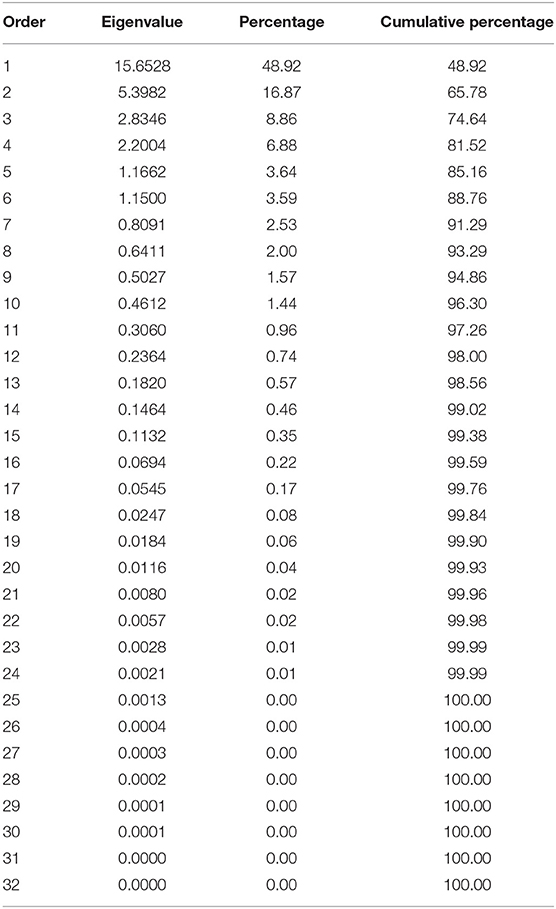
Table 3. Eigenvalues of the correlation matrix from the principal component analysis of the traits evaluated in pure stands and binary mixtures of seven common bean cultivars at three Ontario environments/locations in 2018.
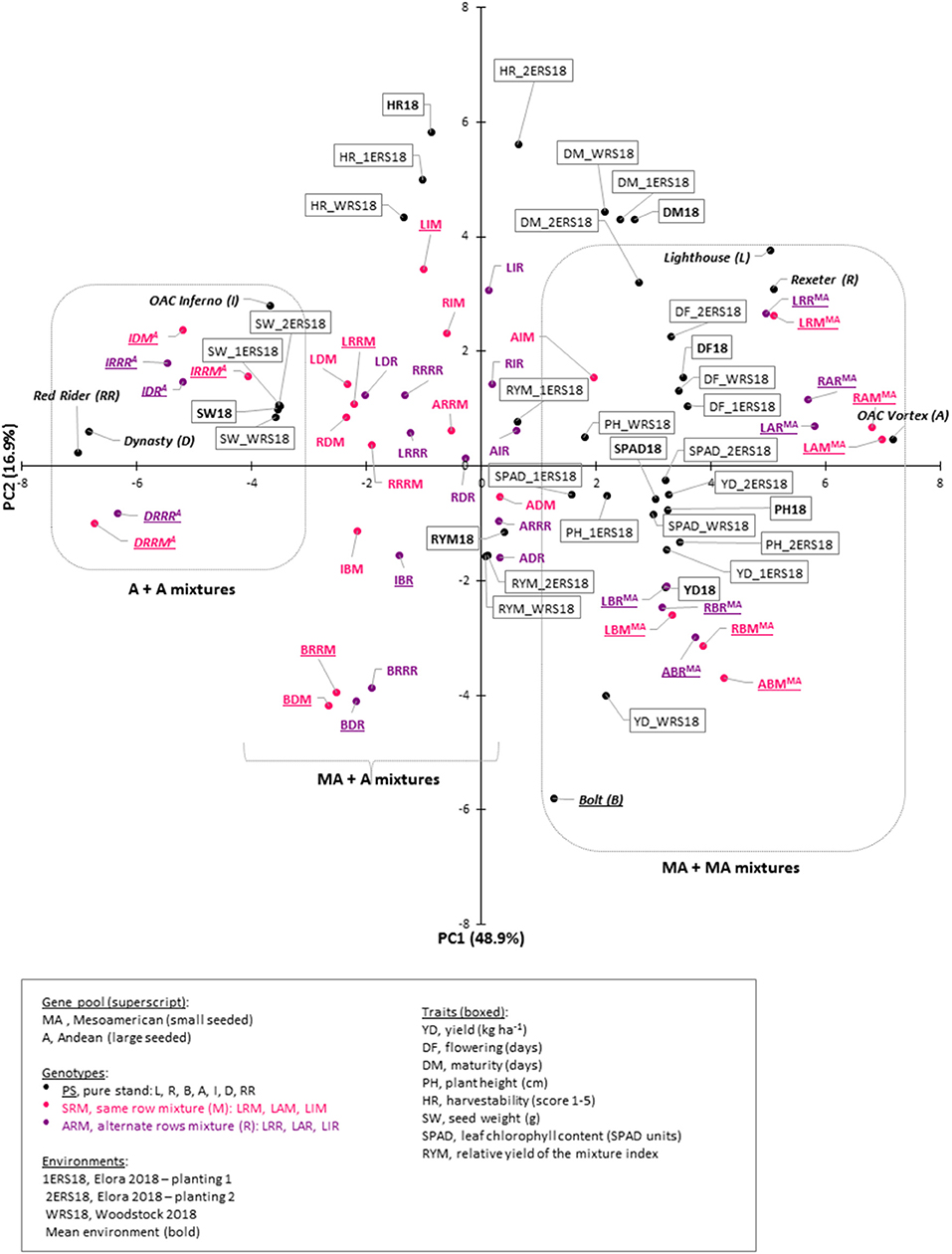
Figure 5. Genotype-trait (GT) biplot analysis for seven common bean genotypes and their mixtures in three Ontario environments/locations in 2018. Cumulatively, first two principal components (PC) explained 65.8% variability. Genotypes separation into Andean (A, left), Mesoamerican (MA, right) and combined (MA + A, middle) is indicated. Traits: yield (YD, kg ha−1), relative yield of the mixture (RYM) index, flowering (DF, days), maturity (DM, days), plant height (PH, cm), harvestability (HR, scale 1 to 5), seed weight (SW, g) and leaf chlorophyll content (SPAD, SPAD values). Environments/locations: mean environment [trait symbol with a year (e.g. YD18)], Woodstock research station (WRS18), Elora research station planting 1 (1ERS18) and planting 2 (2ERS18). Additional information (scree plot, eigenvectors and genotype scores) can be found in Figure S3.
Significant associations among traits, analyzed in different locations, were indicated by their groupings in a genotype-trait (GT) biplot. For example, all four seed weight measurements (mean environment, WRS18, 1ERS18, and 2ERS18) clustered together in a quadrant opposite to the negatively associated yield trait. In contrast, yield evaluated at WRS was positioned further from the yield values measured at the ERS (two plantings). Significant negative correlations between yield and harvestability at WRS, ERS first planting and environmental average are reflected by their positions in different quadrants. However, there was no associations observed between these traits at ERS, second planting (Figure 5).
In the GT biplot, the genotypes were divided into three distinct clusters. Three large seeded Andean cultivars and their mixtures (three SRMs and three ARMs) clustered together (left side of the plot). Similarly, the four small seeded Mesoamerican genotypes and their mixtures (six SRMs and six ARMs) grouped together (right side). Interestingly, mixtures with both types [composed of between gene pool (Mesoamerican + Andean) genotypes] grouped close to each other and in a third cluster that was placed in the middle between the two within gene pool groups (mixture of two Mesoamerican or two Andean) cultivars (Figure 5).
Yield Advantage of Bean Cultivar Mixtures
The results of the current work indicated a yield advantage for some of the bean cultivar mixtures (Figure 6). Significant positive correlations were identified between the yields of the mixtures and yields of the midcomponent (average of two cultivar components evaluated in pure stands) averaged over three environments (r = 0.9373; p < 0.0001; N = 42; Figure 6A) as well as in each environment/location evaluated separately (Figure S4A).
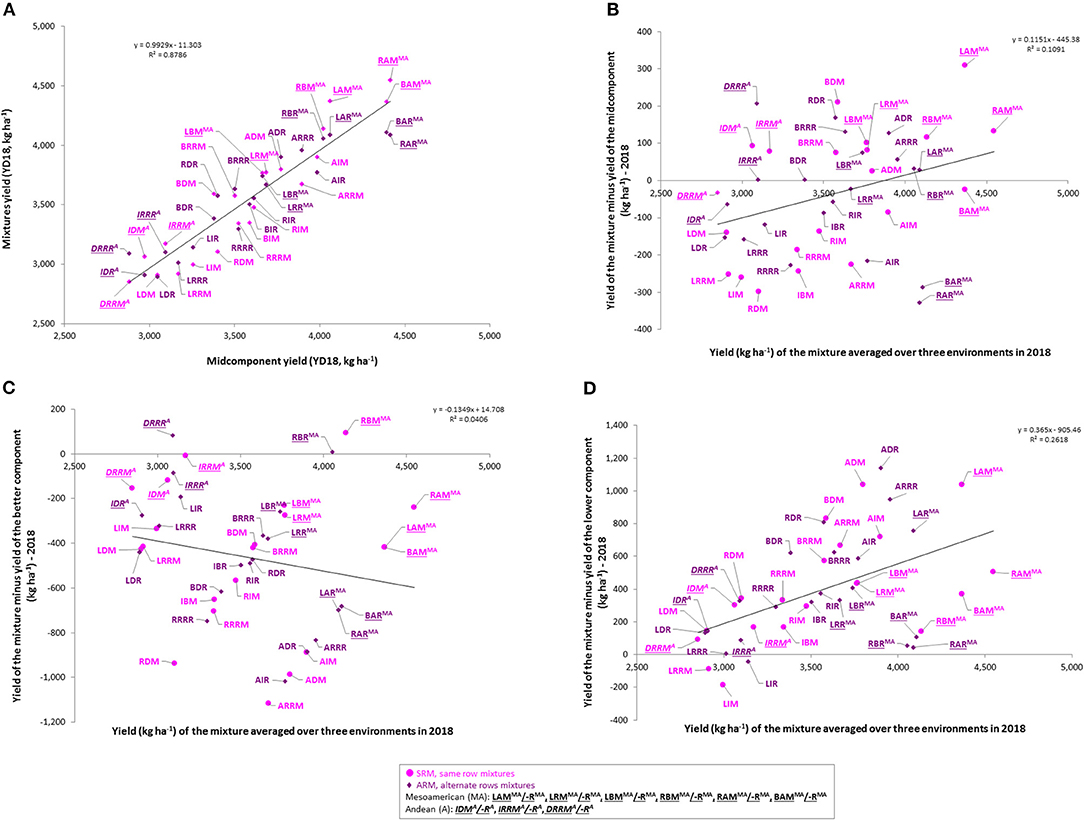
Figure 6. Yield advantage of the common bean cultivar mixtures averaged over three Ontario environments/locations in 2018. (A) Yield of the mixtures compared to the yield of the midcomponent (mean of two cultivar components grown in pure stands); (B) Yield of the mixture vs. yield of the mixture minus yield of the midcomponent; (C) Yield of the mixture vs. yield of the mixture minus yield of the better component; (D) Yield of the mixture vs. yield of the mixture minus yield of the lower component. Mixture names consist of three letters: first letter indicates cultivar component 1 (L, Lighthouse; R, Rexeter; B, Bolt; A, OAC Vortex; I, OAC Inferno, D, Dynasty; RR, Red Rider), second letter indicated cultivar component 2 and the third letter indicates mixture type [M, same row mixture (SRM, pink); R, alternate rows mixture (ARM, purple)]. Mixtures of two Andean (A + A) or two Mesoamerican (MA + MA) cultivars are indicates by a superscript (MA or A) with their names [e.g. same row mixture between two Mesoamerican cultivars, Lighthouse and Rexeter (LRMMA) or alternate rows mixture between two Andean cultivars, OAC Inferno and Dynasty (IDRA)].
When the mixture yield superiority over the yield of the midcomponent (yield of the mixture minus yield of the average of the two components in pure stands) was regressed over the yield of the mixture, a significant linear relationship was observed averaged over the three environments (Figure 6B) as well as in each environment/location separately, except at the ERS for the first planting (Figure S4B).
However, when the mixture yield superiority over the yield of the better component (yield of the mixture minus yield of the better component in a pure stand) was regressed over the yield of the mixture, no significant linear relationships was observed (Figure 6C, Figure S4C). Averaged over the three environments, only three mixtures (RBM, RBR, and DRRR) yielded more compared to the better yielding cultivar component, (Figure 6C).
The advantage of growing bean cultivars in mixtures instead of monocultures, was also assessed in comparison to the yield of the lower yielding component. When the mixture yield superiority over the yield of the lower yielding component (yield of the mixture minus yield of the lower yielding components in pure stands) was regressed over the yield of the mixture, a significant linear relationship was observed (Figures 6D, 4D). In general, mixtures yielded more than the lower yielding cultivar component [ranging from 5.4 kg ha−1 (LRRR) to 1141.0 kg ha−1 (ADR) in the mean environment]. Averaged over the three environments, only three between gene pool mixtures [LIM (−185.4 kg ha−1), LRRM LIM (−90.1 kg ha−1) and LIR LIM (−41.6 kg ha−1)] yielded lower than the low-yielding cultivar component (Figure 6D).
Identification of the Best Performing Mixtures
Mixture Selection Based on the Yield and RYM Index
To identify the best performing mixture, yield and RYM index were plotted against each other [similar to (Nyikako et al., 2014)] for each environment/location and averaged over the three environments (Figure 7). In general, mixtures between higher yielding Mesoamerican genotypes placed in the two top quadrants while mixtures between lower yielding Andean genotypes were always in the bottom two quadrants.
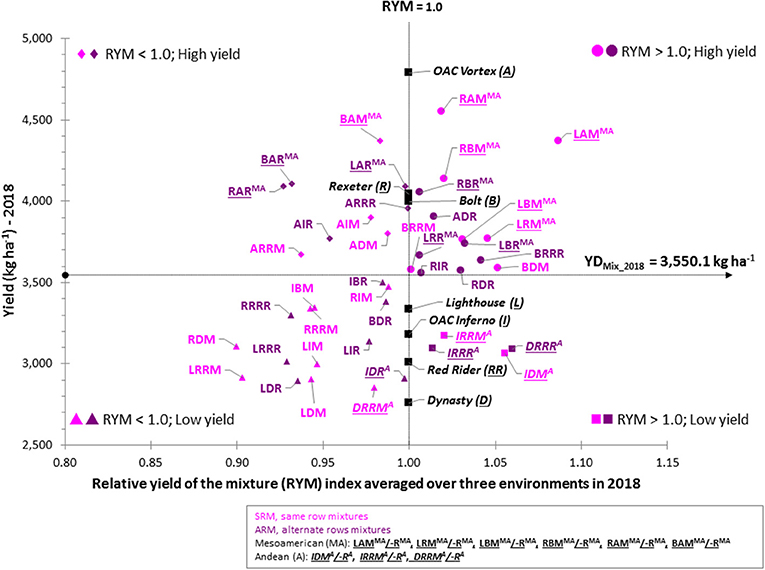
Figure 7. Comparison of the yield and relative yield of the mixture (RYM) index in common bean averaged over three Ontario environments/locations in 2018. In each scatter plot, horizontal line indicates the mean value of the yield; vertical line indicates RYM value of 1 and connects cultivars in pure stands. Plot is divided into four quadrants: top right, mixtures having RYM > 1 and high yield (best performers); bottom right, mixtures having RYM > 1 but yield lower than the average; top left are mixtures with RYM < 1 and high yield; bottom left are mixtures with RYM < 1 and low yield (worst performers).
The best performing mixtures (high yield and RYM >1) were identified in upper right quadrant. Based on the three-environment average data, 14 mixtures [seven SRM (RAM, LAM, RBM, LRM, LBM, BDM, and BRRM) and seven ARM (ADR, BRRR, RDR, RIR, RBR, LRR, and LBR)] had combined high yields [ranging from 3,555.8 kg ha−1 (RIR) to 4,547.7 kg ha−1 (RAM)] with RYM values >1 [ranging from 1.002 (BRRM) to 1.087 (LAM)]. Eight mixtures were biblends between Mesoamerican genotypes and six were between gene pool mixtures (Figure 7). Mixtures between white bean cultivars Lighthouse and Rexeter, Lighthouse, and Bolt, Rexeter and Bolt as well as Bolt (white) and Red Rider (cranberry) were good performers in both SRM and ARM. The mixtures LAM, LBM, and BRRR had the most stable performances across all environments (they consistently placed in the top right quadrant in all three environments/locations as well as averaged over the three environments).
Four out of the six Andean mixtures had good RYM values [ranging from 1.014 (IRRR) to 1.060 (DRRR)] but had low yields, averaged over the three environments (Figure 7). However, their performances were inconsistent among environments (Figure S5). Only the DRRR mixture [ARM biblends between Dynasty (dark red kidney) and Red Rider (cranberry)] consistently placed in the bottom right quadrant [yield lower than the average of the mixtures but RYM index over one (from 1.008 at the ERS first planting to 1.128 at the ERS second planting].
Although the majority of between gene pool biblends were placed on the left side quadrants with high (top) or low (bottom) yields and values of the RYM index below one, some of these mixtures had both high yields and good RYMs. For example, ARM biblend between small seeded white cultivar Bolt and large seeded cranberry cultivar Red Rider (BRRR) had both high yields and high RYMs [range from 1.032 (WRS) to 1.054 (ERS, planting 2)] in all three environments (Figure S5) as well as in the three environment average (Figure 7). This mixture also performed well as the SRM biblend having high values for yield and RYM at WRS and three-environment average.
Mixture Selection Based on Diallel Analysis
The performances of the bean SRM and ARM mixtures were further evaluated in separate diallel analyses and significant differences among seven bean cultivars and their mixtures for all measured traits were identified. This confirmed the Glimmix results that there was variability among the genotypes for the tested traits in three Ontario environments. The total genotypic variation of the data was further partitioned into general mixing ability (GMA) and specific mixing ability (SMA) of pure lines and their binary mixtures, respectively. Mean squares (MS) for GMA were greater in magnitude than the SMA MS and GMA/SMA (Baker's) ratios were close to unity, which indicated that additive effects were more important than dominance in the expression of these traits (Table 4).
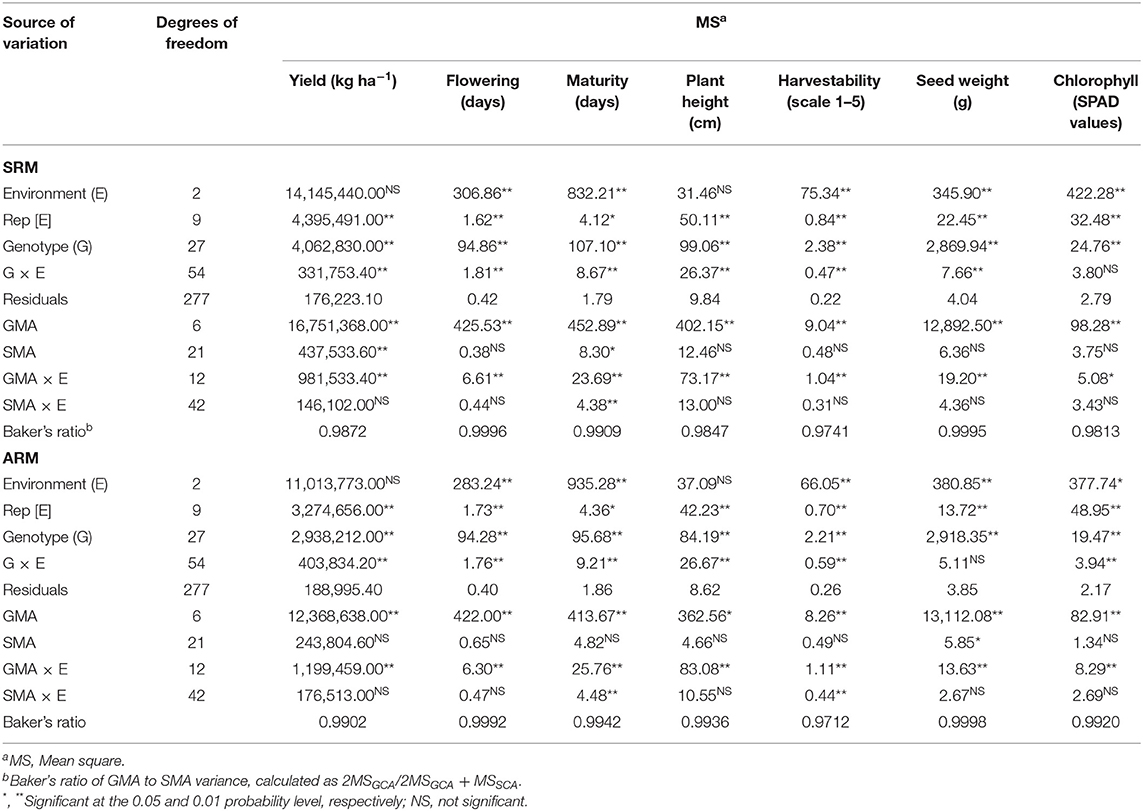
Table 4. Analysis of variance for general mixing ability (GMA) and specific mixing ability (SMA) of the bean same row (SRM) and alternate rows (ARM) binary mixtures evaluated in three Ontario environments/locations in 2018.
Significant GMA effects were observed for all seven measured traits. SMA had significant effects only for yield and maturity in SRM and seed weight in ARM biblends. Individual GMA and SMA analyses were also conducted for each environment/location (Table S8). Significant genotype × environment interactions (G × E) were observed for the majority of the traits, except for the SPAD in SRM and seed weight in the ARM biblends.
Both positive and negative estimates of GMA effects were observed among cultivar components of the mixtures (pure stands) for all analyzed traits (Tables 5, 6). For example, the highest positive GMA effect on yield was observed in a small seeded black cultivar OAC Vortex, which also had the highest value for this trait. Similarly, the large seeded kidney bean cultivar Dynasty had a negative GMA value for the trait and had the lowest yield. Therefore, cultivar OAC Vortex was the best general mixer with the maximum positive GMA effects on yield (673.5 in SRM and 564.6 in ARM) but the poorest general mixer for plant height, having the highest positive GMA effect (if short plants are desirable) on this trait, averaged over three environments (Tables 5, 6). On the other hand, with the maximum negative values of the GMA effects on yield (−400.2 in SRM and −345.3 in ARM biblends), cultivar Dynasty was the poorest general mixer for this trait, and also for SPAD, but increased seed weight (+12.3 in SRM) and reduced flowering (−2.0).
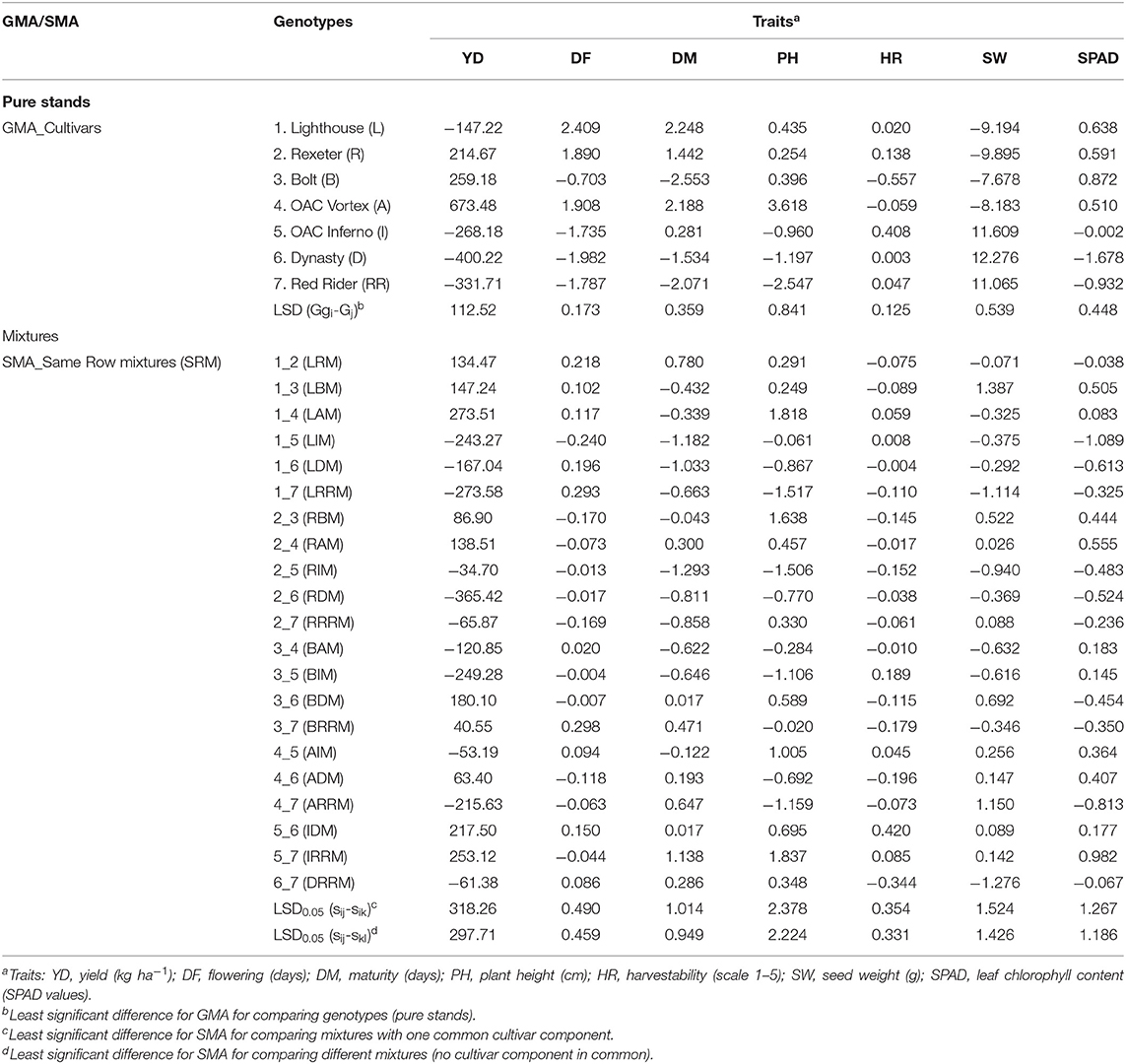
Table 5. Estimated general mixing ability (GMA) for traits analyzed in seven bean genotypes and specific mixing ability (SMA) of their same row binary mixtures (SRM) averaged over three Ontario environments/locations in 2018.
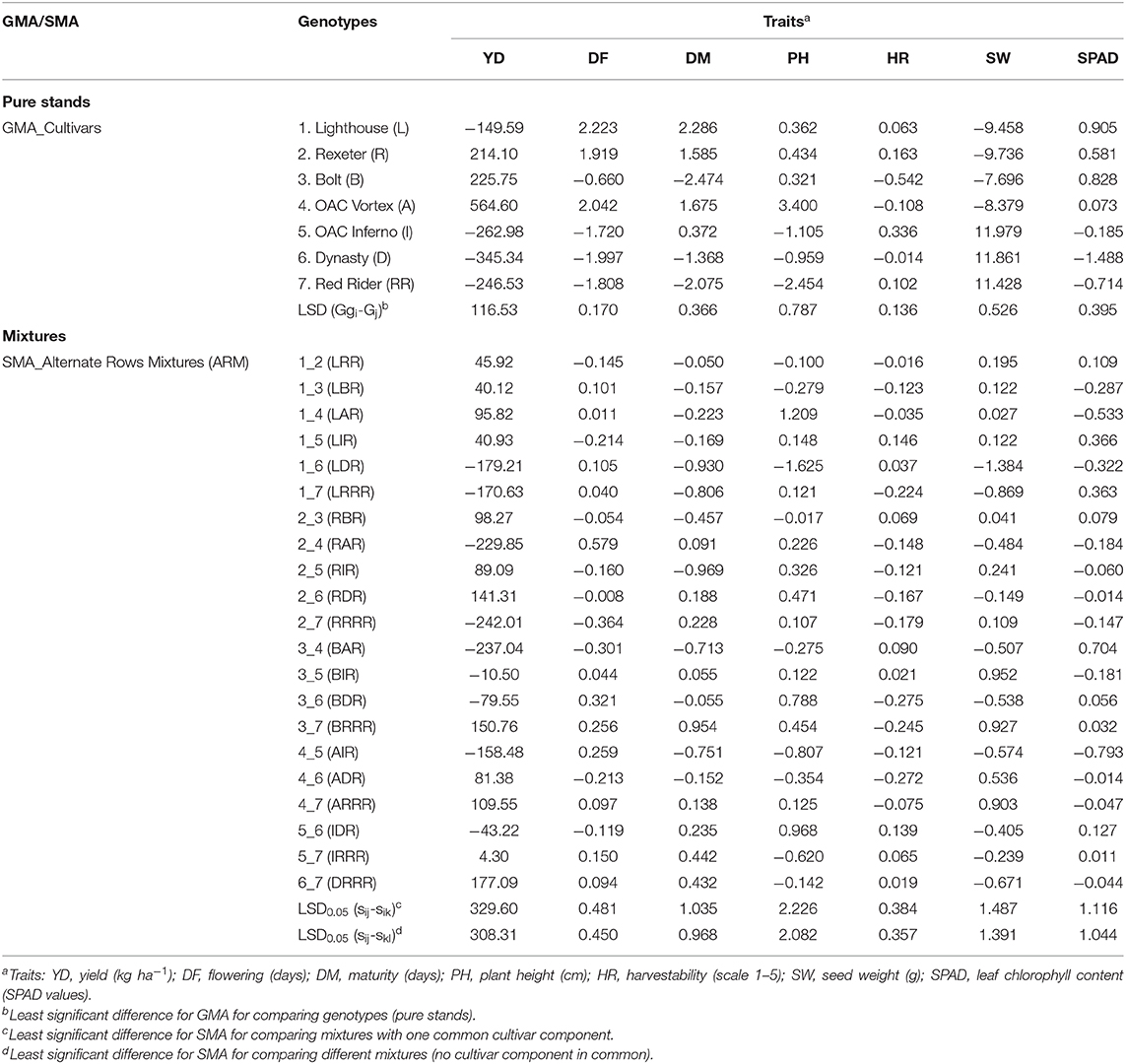
Table 6. Estimated general mixing ability (GMA) for traits analyzed in seven bean genotypes and specific mixing ability (SMA) of their same row binary mixtures (ARM) averaged over three Ontario environments/locations in 2018.
In the SRM biblends, 10 mixtures had positive SMA values for yield (Table 5). The most positive SMA effect was observed in the low (Lighthouse, GMA = −147.2) × high (OAC Vortex, GMA = 673.5) LAM mixture (SMA = 273.5) and the most negative SMA effect for yield was identified in the high (Rexeter, GMA = 214.7) × low (Dynasty, GMA = −400.2) RDM mixture (SMA = −365.4). The most positive SMA effect for flowering was observed in the low × low BRRM mixture (SMA = 0.298), for the maturity in the high (OAC Inferno, GMA = 0.281) × low (Red Rider, GMA = −2.071)] IRRM mixture (SMA = 1.138), for plant height in the low × low IRRM mixture (SMA = 1.837), for harvestability in the high × high IDM mixture (SMA = 0.420), for seed weight was found in the low × low LBM mixture (SMA = 1.387) and for the SPAD readings in the low × low IRRM mixture (SMA = 0.982). The most negative SMA effect for flowering was found in the high × low LIM biblend (SMA = −0.240), for maturity in the high × high RIM biblend (SMA = −1.2930), for plant height in the high × low LRRM biblend (SMA = −1.517), for harvestability in the high × high DRRM biblend (SMA = −0.344), for seed weight in the high × high DRRM biblend (SMA = −1.276)and for the SPAD readings in the high × low LIM biblend (SMA = −1.089) (Table 5). Similar trends in SRM biblends were observed when analyses were performed separately for each environment/location (Table S9).
In the ARM biblends, 12 mixtures had positive SMA values for yield (Table 6). The most positive SMA effects was observed in the low (Dynasty, GMA = −345.3) × low (Red Rider, GMA = −246.5) DRRR mixture (SMA = 177.1) and the most negative SMA effects for yield was found in the high × low RRRR mixture (SMA = −242.0). The most positive SMA effect for flowering was found in the high × high RAR mixture (SMA = 0.579), for maturity in the low × low BRRR mixture (SMA = 0.954), for plant height was observed in the high × high LAR mixture (SMA = 1.209), for harvestability in the high × high LIR mixture (SMA = 0.146), for seed weight in the low × high BIR mixture (SMA = 0.952) and for SPAD in the high × high BAR biblend (SMA = 0.704). The most negative SMA effect for flowering was observed in the high × low RRRR biblend (SMA = −0.364), for maturity in the high × high RIR biblend (SMA = −0.969), for plant height in the high × low LDR biblend (SMA = −1.625), for harvestability in the low × low BDR biblend (SMA = −0.275), seed weight in the low × high LDR biblend (SMA = −1.384) and for SPAD in the high × low AIR mixture (SMA = −0.793) (Table 6). Similar trends for the ARM biblends were observed when the analyses were performed separately for each environment/location (Table S10).
Best Performing (Yielding) Mixtures
Significant association was identified between values of seven measured traits and their mixing abilities (GMA of cultivar components and SMA of biblends) in both types of the mixtures (Figure 8, Figure S6). For yield, correlation was higher in SRM (r = 0.682) compared to the ARM (r = 0.598) biblends.
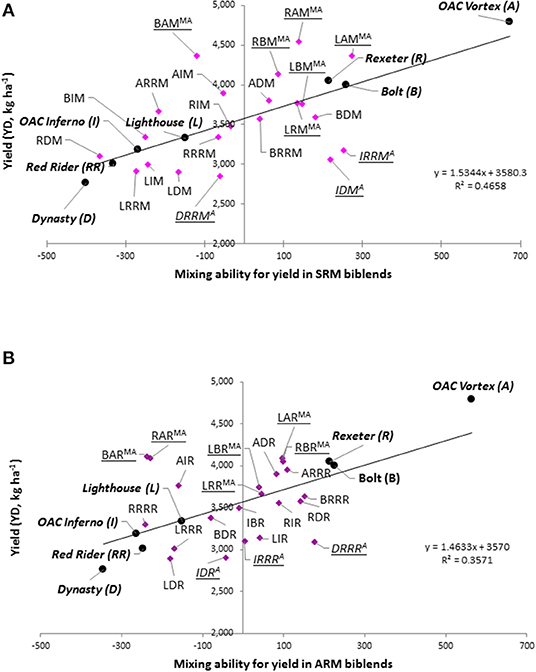
Figure 8. Relationships between yield and mixing abilities [General Mixing Abilities (GMA) and Specific Mixing Abilities (SMA)] in common bean cultivar mixtures. (A) Same row mixtures (SRM); (B) Alternate rows mixtures (ARM).
When evaluated in pure stands, three Mesoamerican genotypes (Rexeter, Bolt, and OAC Vortex) were higher yielding compared to the average of all seven genotypes (3,587 kg ha−1) and had positive general mixing ability (GMA). In contrast, Lighthouse and all three Andean cultivars (OAC Inferno, Dynasty, and Red Rider) had negative GMA effects and had lower yields compared to the average yield of all seven genotypes (Figure 9).
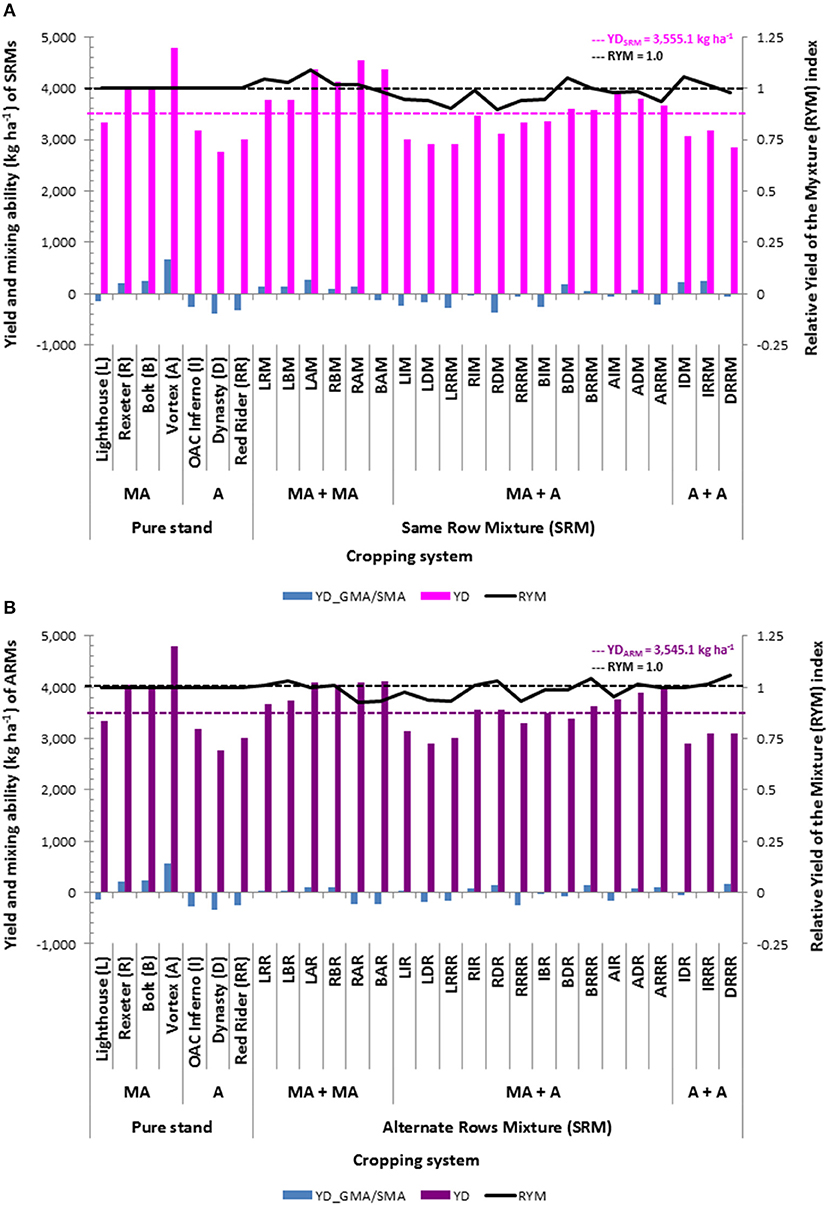
Figure 9. Selection of the best mixture of common bean cultivars based on the yield, relative yield of the mixture (RYM) index and mixing ability. (A) Same row mixtures (SRM); (B) Alternate rows mixtures (ARM).
A combination of high yield, RYM index >1 (RYM >1) and positive SMA was used to identify mixtures that outperformed (in yield) cultivar components when evaluated in pure stands. All 12 biblends formed among the four Mesoamerican genotypes had higher yields compared to the average yield of all biblends [3,555.1 kg ha−1(SRMs) and 3,545.1 kg ha−1(ARMs)]. In addition, five SRMs (LRM, LBM, LAM, RBM, and RAM) and four ARMs (LRR, LBR, LAR, and RBR), respectively, had positive SMA effects and values of the RYMs >1 (Figure 9). Although high yielding, three mixtures [BAM (SRM), RAR (ARM), and BAR (ARM)] showed negative SMA effects and RYM index values below one and one ARM biblend (LAR) had positive SMA effects but did not outperform cultivar components in pure stands (RYM <1). Pure Andean biblends (three SRMs and three ARMs) were lower yielding compared to the mixture averages. However, two SRMs (IDM and IRRM) and two ARMs (IRRR and DRRR) performed better than their cultivar components in pure stands (RYM >1) and showed positive SMA effects. Yields of the between gene pool biblends were variable; only five SRMs (BDM, BRRM, AIM, ADM, and ARRM) and six ARMs (RIR, RDR, BRRR, AIR, ADR, and ARRR) had yields higher than the averages of the two mixture types. Among them only two of the SRMs (BDM and BRRM) and four of the ARMs (RIR, RDR, BRRR, and ADR) biblends had positive SMA effects and RYMs >1.
Based on these three parameters (above average yield, RYM >1 and positive SMA effects) seven SRMs (LRM, LBM, LAM, RBM, RAM, BDM, and BRRM) and seven ARMs (LRR, LBR, RBR, RIR, RDR, BRRR, and ADR) could be selected as better performers compared to their cultivar components evaluated in pure stands. Among them, four biblends (LR, LB, RB, and BRR) were good performers both as SRM and ARM. The best SRM was biblend between the two Mesoamerican cultivars, white Lighthouse and black OAC Vortex (LAM), having the highest positive SMA effect (273.5), second highest yield among the SRMs (4,368.9 kg ha−1) and a potential yield increase of 8.7% based on the RYM index (1.087). Among the ARMs, the best performer was a between gene pool BRRR mixture (white Bolt and cranberry Red Rider) with the SMA highest in ARM biblends (150.8), high yield (3,633.5 kg ha−1) and a potential yield increase of 4.2% (RYM = 1.042).
Discussion
A common criticism of modern field crop production is that it relies on highly related cultivars propagated in monocultures that are selected for performance under ideal conditions but are poised for collapse under stress. A study of Canadian beans revealed that many of the small seeded Mesoamerican cultivars have common parents, and large seeded (Andean) cultivars, have relatively narrow genetic diversity (Navabi et al., 2014). The use of cultivar mixtures has been suggested as a means of increasing genetic diversity in the production field, and achieving better disease control, increasing of crop productivity and, resiliency to stress and disease resistance (Browning and Frey, 1969; Wortmann et al., 1996; Kristoffersen et al., 2020). Increased plant diversity may also increase ecosystem functioning (Loreau et al., 2001; Letourneau et al., 2011; Bybee-Finley and Ryan, 2018). However, although widely used in the tropics and some temperate regions, intracropping is not a common agricultural practice in developed countries. Currently, the most successful use of the cultivar mixtures is for disease control in small cereals (Bowden et al., 2001; Tratwal and Bocianowski, 2018; Giunta et al., 2020). The aim of the current study was to determine the agronomic (including crop yield) and ecosystem effects of increasing bean crop diversity by using cultivar mixtures instead of monocultures in cropping systems in Ontario environments.
Genotype Selection and Mixture Formation
To maximize the diversity of the mixtures in the current study, genotypes that originated from both Mesoamerican and Andean gene pools differing in their architecture, phenological traits, and resistance to several bean diseases were selected as mixing components. All cultivars had high yield potentials, and this was their first use in mixture studies.
Although widely used in intercropping, the replacement series design confounds the effects of the plant size, plant density, and species proportion (Connolly, 1986; Snaydon, 1991; Banik, 1996; Jolliffe, 2000; Connolly et al., 2001a,b; Bybee-Finley and Ryan, 2018). In this study, a “fixed-ratio mixture design” (Federer, 1993) was used where both cultivars were considered as main crops. In all mixtures, two cultivar components (same number of seeds) were jointly evaluated relative to their response in pure stands. This gave a single and accurate performance result for each mixture. However, it is possible that improved productivity for one cultivar may have resulted in a yield reduction of the other component, but this assessment was not available with the current study design. In particular, differences in plant size and architecture between Mesoamerican and Andean cultivar (Kelly, 2001) components could have affected the performance of the MA mixtures, both negatively (through shading for example), and positively (through nitrogen fixation).
The current work was restricted to two cultivar mixtures with a constant single (1:1) density, which allowed the use of diallel analysis to test mixing ability of cultivar components as well as performance of the mixtures. Kiær et al. (2009) found significant increases in the relative mixing effects with the number and diversity of the component cultivars in a meta-analysis of grain yields in cereal mixtures. However, Frey and Maldonado (1967) did not find an association between relative yield and the number of cultivars in oat mixtures, analyzed over two planting dates; in their study mixtures containing two or five genotypes were equally effective.
Reasons to Choose a Cultivar Mixture Over the Monoculture in Industrialized Agriculture
Potential Buffering Effect
One of the advantages of intercropping systems is a buffering effect against various biotic and abiotic factors (Finckh et al., 2000). In the current study, environmental buffering was reflected in the finding that significant SMA × E effects were observed only for a few traits for the mixtures [maturity (SRM and ARM) and harvestability (ARM)], compared to the cultivars in pure stands for which significant environmental effects were observed for all of the traits. A graphic illustration of the buffering potential was observed in some plots at the ERS (2017) which were flooded shortly after the planting and resulted in reduced establishment in the rows with the black bean cultivar OAC Vortex but did not affect the rows seeded with the navy bean Lighthouse. During the season the Lighthouse plants filled in the gaps in the mixed plots so that by the end of the season there was canopy closure (data not shown) and overall yields in the mixture plots were similar to the two parent means. This type of compensatory effect is similar to the yield protection seen in mixtures of bean cultivars grown in Palmira, Colombia (Panse et al., 1989) where it was observed that mixtures had a greater potential to compensate for plant establishment losses caused by inferior seed quality of individual genotypes. Panse et al. (1989) concluded that intergenotypic compensation was a major determinant for a positive mixture effect on yield. However, further analysis including yield, field germination, and number of plants at harvesting for each cultivar component in the mixtures used in the current study would be necessary for a proper assessment of potential buffering (compensatory) effect in mixtures of Lighthouse and OAC Vortex as well as bean cultivar mixtures in general.
Better Disease Resistance - Could Not Be Determined
Anthracnose is a seed-borne fungal disease that can significantly reduce yield and quality in all bean market classes. Ontario is characterized by frequent warm and humid summers that make ideal conditions for a rapid fungal spread. This could result in anthracnose outbreaks and Ontario bean fields are regularly monitored for disease symptoms (OMAFRA, 2018; Conner et al., 2020) and treated with fungicides to prevent outbreaks.
In the current study, bean genotypes were scored for the anthracnose symptoms under the field conditions. However, potential advantage of bean cultivar mixtures over monocrops in resistance to anthracnose could not be assessed in the present study. There was no significant difference between mixtures and pure stands in 2017 and no disease symptoms were detected in 2018. To obtain necessary environmental conditions for fungal development, a future work should include screening bean cultivar mixtures in anthracnose disease nursery.
Yield Advantage Was Identified in Some Cultivar Mixtures
Both positive and negative effects of mixing bean cultivars on yield were observed in the current study. Similar findings were reported for the mixtures in other crops. For example, working with cowpea cultivar mixtures, Okonya and Maass (2014) noticed that mixtures grown in marginal environments were more stable than a single cultivar but not all mixtures yielded more than a single cultivar. A significant positive correlation was identified between yield of the mixtures and yield of the midcomponent. These findings are similar to Gallandt et al. (2001) and suggested that mixture yield could be predicted from the average yield of the two cultivar components grown in pure stands.
In general, the two types of common bean cultivar mixtures used in the current study, namely the same row mixtures SRMs) and the alternate row mixtures (ARMs) were not significantly different (average of SRMs vs. average of ARMs) for the traits that were evaluated. However, some individual mixtures were significantly different in the SRM vs. the ARM configuration. There were also differences between Mesoamerican and Andean (M vs. A) mixtures, probably due to different plant architecture and root systems (Singh et al., 2019; Strock et al., 2019). The lowest yield in the ARM configuration was identified in mixture between the two large seeded colored kidney bean cultivars Dynasty and OAC Inferno.
In general, between gene pool mixtures (both SRMs and ARMs) had intermediate yield values that were closer to the small seeded beans. This agrees with findings of Essah and Stoskopf (2002) who observed that mixtures of four diverse barley cultivars (evaluated as ARMs and SRMs at ERS over two years) yielded similar to the midvalue. Only two of the 12 gene pool biblends overyielded the monocrops, by 13 and 14%, respectively.
Similar to Diaz et al. (2018), significant positive correlations among the three environments/locations for all analyzed traits (except the RYM index) were identified in the current study. In general, the strongest associations for the majority of the traits were observed between the two plantings at the ERS, illustrating the importance of the fit of genotypes, singly or in mixtures, to their environment.
Some trait associations were specific for the plant materials and environments used in this study but, some confirmed previously identified correlations. For example, in a number of studies, earliness is negatively associated with productivity (Kamfwa et al., 2015; Polania et al., 2016; Diaz et al., 2017). Similarly, in the current study, yield was significantly positively correlated with flowering and maturity, except at the WRS. At this location, yield was negatively associated with maturity. Similar contrary findings between environments were reported in a number of previous studies (Asfaw et al., 2017; Polania et al., 2017; Diaz et al., 2018) emphasizing the importance of environmental effects. In addition, a positive correlation between yield and SPAD confirmed relationships between these traits identified previously in bean (Guler and Ozcelik, 2007).
SPAD readings are used as a measurement of relative chlorophyll content in leaves, which relates to photosynthetic capacity. Higher SPAD values are often associated with a higher photosynthetic rate and potentially higher yield.
In the current study, yield was negatively correlated with seed weight. In some studies, positive correlations were identified in various environmental conditions (Mukeshimana et al., 2014; Polania et al., 2016; Assefa et al., 2017; Diaz et al., 2018) and in the others, both positive and negative correlations were identified (Asfaw et al., 2017).
Based on the RYM Index, Some Mixtures Outperformed Monocrops
To evaluate the bean cultivar mixtures, a combined crop response was of interest and use of the RYM index was appropriate to determine mixing efficiencies of particular combinations. There were some synergistic effects of intracropping on total productivity, as the total yield and RYM index of some bean mixtures at the 50:50 seed rate exceeded the average yields of the cultivars grown in pure stands. No particular influence of environment on the RYM index was noted. It appeared that specific mixtures had RYM indices that were optimal for specific environments.
In mixtures consisting of components with very similar resource utilization patterns, competition rather than cooperation can be expected. In this case, mixtures generally yield less than the higher yielding monocrop (Rao and Willey, 1980). The bean cultivars that were chosen for the study had similar maturities to ensure that they could be harvested together but that choice may also have resulted in competition among the components. However, not all mixtures had RYM values >1.0. Averaged over three environments/locations, the RYMs ranged from 0.900 (RDM) to 1.087 (LAM) in the SRMs and from 0.927 (RAR) to 1.060 (DRRR) in the ARMs. Also, in the present study the RYM index also showed a positive relationship with the sum of the yields of the two cultivar components in the mixture, indicating that the nature of the interaction between components was mainly facilitation, rather than competition (Li et al., 1999; Loreau and Hector, 2001).
It may be possible to design mixtures with non-competitive growth patterns. For example, Mesoamerican and Andean genotypes have different root distribution patterns (Strock et al., 2019). Henry et al. (2010) evaluated three bean multilines each composed of two bean RILs with contrasting root architectures in seven environments in Honduras, in soils with varying phosphorus levels and moisture availabilities. Production of the multilines was not associated with a yield penalty, even though variation in belowground competition among bean genotypes was identified.
Diallel Analysis Enabled Identification of Bean Cultivar(s) Having the Best Mixing Abilities
Higher values of General Mixing Abilities (GMA) MS compared to Specific Mixing Abilities (SMA) MS and GMA/SMA ratios closer to unity, indicated the importance of additive effects in the expression of traits evaluated in the current study (Griffing, 1956). The finding that significant GMA effects were observed for all seven measured traits indicated that significant differences existed among the cultivar components of the mixtures and suggests that there was at least one (or more) cultivar(s) that demonstrated superior mean performances in the mixture combinations. OAC Vortex, Bolt and Rexeter were genotypes that were frequently members of mixtures with high yields, whereas, Dynasty and Red Rider had poor GMA values for yield.
SMA was significant only for yield and maturity in SRM and ARM biblends. The lack of significant SMA effects for most traits suggests that there is a lack of complementary phenotypic variability among the genotypes for the traits that were evaluated. When diallel mating is used in a breeding program, at least one parent should have good general combining ability and the best combination must be the one presenting the highest SCA (Griffing, 1956). In the current study, mixtures with high SMA for the analyzed traits were obtained from mixing cultivar components with various types of GMA effects (high × high, high × low, and low × low general mixers) and were different in SRM and ARM biblends. For example, OAC Inferno and Red Rider, which had a poor GMA values, combined in a mixture with the highest SMA. The results indicate that good mixture combinations will need to be determined experimentally, on a case-by case basis.
The existence of significant mixture × E interactions (observed for majority of the traits, except for the SPAD in SRM and seed weight in ARM biblends) suggested that the mixtures responded differently in different environments. In the current study, it probably resulted from the significant GMA × E (all traits). In general, small seeded Mesoamerican beans (Lighthouse, Rexeter, Bolt, and OAC Vortex) had positive GMA values for most of the traits except seed weight. These genotypes generally contributed positively to the yield and SPAD readings of the mixtures but had negative effects on other traits resulting in delays in flowering and lengthening the time to maturity. In contrast, the large seeded Andean cultivars (OAC Inferno, Dynasty, and Red Rider) had negative effects on the yields and SPAD readings of the mixtures and their inclusion as mixture components resulted in reductions in days to flowering and maturity, as well as increases in seed weight and harvestability. Therefore, between gene pool mixtures combined the potential for higher yield and SPAD readings and reduced harvestability from Mesoamerican component with positive effects of the Andean component to increase seed weight and phenological traits (flowering and maturity).
Selection of the Best Performing Mixture
This work confirmed that the selection of the best performing mixtures needs to be carried out using several approaches including direct mixture performance (trait-based), or index- or diallel-based selection. Different superior mixtures will be identified, based on the selection method used, and all of them have some limitations.
Based on the performance (yield) a small seeded black cultivar OAC Vortex was the best performer. This cultivar was the highest yielding genotype among all monocrops and mixtures. Mixtures of two high yielding genotypes usually cannot overyield the monocrops. In addition, trait expression (values) could potentially be different in mixtures and pure stands due to their plasticity (Barot et al., 2017).
Among 50+ indices (Weigelt and Jolliffe, 2003) available for the assessment of the intercropping, that compare mixture yield or biomass data with component cultivars evaluated in pure stands, only few were applicable to the current study. Since no information from individual components of the mixtures was collected, it was not possible to use indices such as LER, competition, or economic evaluation. The economic evaluation of the bean mixtures would be interesting, especially for the mixtures between gene pools, since the large seeded beans have the higher market price (http://www.omafra.gov.on.ca/english/stats/crops/index.html). However, careful use of the index-based selection is recommended. It is a ratio-based index (Jasienski and Bazzaz, 1999) and high RYM values (RYM >1) can also be obtained blending low yielding genotypes.
In general, diallel analysis of mixture yield data complemented well the index evaluation approach used in the current study. All three indices (RYM, MS, and BS) used were derived from the measured yield data. High yield is the major selection criteria in the most bean breeding programs but good architecture, appropriate phenological characteristics and disease resistance should also be considered in the selection. Although information regarding additional traits can be obtained by diallel analysis (as in the current study), the disadvantage is in its restricted use for two-component mixtures (analogous to its use for bi-parental crosses) and requires that all genotype combinations are tested. A benefit of utilizing a diallel analysis to determine GMA, is that the information can be used to select pairs of cultivars as the best mixture components. There is also possibility to predict performance of more complex mixtures by applying analysis of mixing ability from the two-component mixtures to three-, four-, and five-component mixtures as Lopez and Mundt (2000) have shown in wheat.
The selection of the best performing mixture should be performed with at least two criteria, including an index or diallel analysis in combination with trait (yield) values. In the current study, based on the yield (above average), RYM index (RYM >), and mixing ability (positive SMA effect) averaged over three locations, different best performing mixtures were identified in the two mixture types. For the SRMs the LAM mixture (Mesoamerican, white Lighthouse, and black OAC Vortex) was the best. Among the ARM biblends the BRRR mixture (Mesoamerican, white Bolt and Andean, cranberry Red Rider) was identified as the best performer.
In addition to the high yield, significant other objectives of the University of Guelph bean breeding program, are early maturing genotypes with a good architecture (good harvestability) and resistance to various diseases, especially anthracnose and common bacterial blight, for the Ontario environment. Based on the seven traits that were measured in the current study, as mixture components, cultivars should have positive effects (GMA) on yield, and some market classes seed weight and photosynthetic efficiency (SPAD). These would be complemented by cultivars that have negative effects (GMA) on flowering (early), maturity (early), and harvestability (smaller values are associated with better standability). Information about the performance of cultivars for these attributes in mixture would allow bean breeders to select appropriate cultivars to combine in bean cultivar mixtures with a high commercial potential.
In order to maximize crop yields in mixtures, Hamblin and Zimmermann (1986) suggested that breeding should focus on selection in a mixed cropping system instead of selecting individual components. This approach would require a whole new level of testing for interactions in cultivar and agrochemical trials and would require different approaches to breeding crops. Litrico and Violle (2015) introduced a new system of breeding “from ideotypes to ideomixes,” while Gaudio et al. (2019) reviewed various approaches for modeling mixtures in annual crops.
Adoption of the Bean Cultivar Mixture Cropping System
To justify the study and to ensure a balanced view of this research topic, questions such as how the cultivar mixtures may address production constraints and/or help bean growers achieve high yield potential need also to be discussed. Although the importance of cultivar mixtures to increase and stabilize yield, reduce disease pressure and enhance diversity have been reported (Wolfe, 1985; Creissen et al., 2016), increased cost to growers and seed cleaning/processing plants could also be anticipated. Some of the agronomic considerations and potential disadvantages of cultivar mixtures have been discussed previously (Castro, 2001; Barot et al., 2017). One of the reasons that bean growers have not embraced mixtures for crop production is seed uniformity demanded by marketers, processors (canning), and consumers. Common bean seeds also undergo extensive cleaning, which in some years result in seed coat breakage or splitting.
The current work tested bean biblends formed by mixing two cultivar components based on the same number of seeds and evaluated as SRM and ARM biblends. A number of mixtures had high and stable yields over three Ontario environments/locations. However, the adaptation of intracropping practices to modern agriculture may have some difficulties including potential incompatibility with mechanization (Reiss and Drinkwater, 2018; Snyder et al., 2020).
Planting
Step one (same for both mixture types) would include seed counting (additional step for grower). SRM would require mechanical mixing of selected cultivar components (additional step) prior to planting. In ARM biblends, each component is planted in a separate row, which may require planter adjustment as an additional step.
In-Field Management
In general, no additional cost for fertilizers, weed management, and pest/disease control would be required. Moreover, mixture cropping could reduce use of the nitrogen fertilizer if one of the components is a highly efficient nitrogen fixer. As a part of the pilot study, plants were dug, and roots were examined for the nodule formation. Lighthouse had a greater number of viable (pink) nodules compared to OAC Inferno (data not shown) probably associated with the better nitrogen fixing ability.
Harvesting
Basically there is no additional cost associated with the harvesting. In the current study, cultivar components were selected to differ up to 10 days in maturity and the harvesting was done when the later component in the mixture was mature. This may however prolong harvest and potentially reduce yield of the earlier component due to possibility of pod shattering.
Processing
There might be some additional cost associated with the processing (more seed splits with large seeded beans; need to separate the components). However, some mixtures can be marketed as a specialty crops (small seeded white/black or large seeded kidneys). In addition, there is no need to sort bean mixtures of the same market class; most are actually combined during the processing and marketing. In particular, the three mixtures between white beans (Lighthouse/Rexeter, Lighthouse/Bolt, and Rexeter/Bolt) that had excellent and stable yields could be immediately utilized by growers. The mixtures between Lighthouse/Bolt and Rexeter/Bolt could also potentially provide crop resistance to a broader range of diseases, since Lighthouse and Rexeter are resistant to common bacterial blight and Bolt is resistant to anthracnose (https://www.gobeans.ca/head2head.php).
Farmer's Acceptance and Mixture Marketing
To be accepted by bean growers, the advantages of mixture cropping over the monocropping should be significant, stable and without additional production cost. Yield increase up to 8% obtained for some mixtures in the current study is the first step toward implementing growing common bean mixture in Ontario environments. However, additional questions would be mixture advertising and marketing.
In conclusion, the study represents the first field evaluation of bean cultivar mixtures in Ontario environments. Initial, one year two location study results indicated that it is feasible to grow bean mixtures instead of monocultures. Results of the second year confirmed some advantages (plot-based) of growing beans in mixtures instead of monoculture in southern Ontario environments.
The research has the potential to provide a theoretical basis for the use of precision agriculture tools to plant fields with mixtures instead of monocultures. It could lead to greater in-field diversity in the crop and in the above and below ground ecosystems that might provide greater buffering capacity and resiliency to the cropping system. General adoption of the use of cultivar mixtures, instead of pure lines, would transform cropping system practices and supporting research. For the latter, it might require different approaches to breeding crops and a whole new level of testing for interactions in cultivar and agrochemical trials and require different approaches to breeding crops.
The experiment needs to be expanded and repeated over sites and years in order to estimate the stability of the positive interactions in mixtures, to define those factors that are responsible for variation in yield, understand the mechanisms that produce advantages in intercropping systems as well as to identify a broad range of good cultivar combinations. More research is needed to understand competition/complementarity between cultivar components in the mixtures. Direct comparison of mixing effects on ecosystem parameters such as soil microbial diversity is also needed. Additional testing the performance of bean mixtures within the same market classes (e.g., within whites, blacks or kidneys); these types of mixtures might find immediate acceptance from Ontario bean producers; they could be marketed as mixtures with a uniform appearance but with enhanced resilience to disease or other environmental challenges. Finally, to be accepted by Ontario bean producers/processors/consumers, bean mixture cropping system requires testing over several years/locations under more conditions relevant to the Ontario farming systems, preferably in large field settings with bean growers' co-operation.
Data Availability Statement
All datasets presented in this study are included in the article/Supplementary Material.
Author Contributions
KP designed the project. KP, TS, and YR conceived and planned the work. TS and LS set up experiments and performed field work. Crop data were collected by LS and YR. YR analyzed the data and wrote first draft of the manuscript. KP revised and proofread the manuscript. All authors contributed to the article and approved the submitted version.
Conflict of Interest
The authors declare that the research was conducted in the absence of any commercial or financial relationships that could be construed as a potential conflict of interest.
Acknowledgments
This project was a part of the Food From Thought research program at the University of Guelph. The research was undertaken in part thanks to generous funding from the Canada First Research Excellence Fund. Current study was also funded by the Ontario Bean Growers, Agriculture and Agri-Food Canada, the Ontario Ministry for Food and Rural Affairs and the Ontario Ministry for Research and Innovation. Special thanks to Dr. Michelle Edwards (University of Guelph, Ontario Agricultural College) for statistical (SAS) consulting and numerous summer students for assisting in field works.
Supplementary Material
The Supplementary Material for this article can be found online at: https://www.frontiersin.org/articles/10.3389/fsufs.2020.00126/full#supplementary-material
References
Asfaw, A., Ambachew, D., Shah, T., and Blair, M. W. (2017). Trait associations in diversity panels of the two common bean (Phaseolus vulgaris L.) gene pools grown under well-watered and water-stress conditions. Front. Plant Sci. 8:733. doi: 10.3389/fpls.2017.00733
Assefa, T., Rao, I. M., Cannon, S. B., Wu, J., Gutema, Z., Blair, M., et al. (2017). Improving adaptation to drought stress in white pea bean (Phaseolus vulgaris L.): Genotypic effects on grain yield, yield components and pod harvest index. Plant Breed. 136, 548–561. doi: 10.1111/pbr.12496
Baker, R. J. (1978). Issues in diallel analysis. Crop Sci. 18, 533–536. doi: 10.2135/cropsci1978.0011183X001800040001x
Banik, P. (1996). Evaluation of wheat + legume intercropping under 1:1 and 2:1 row replacement series system. J. Agron. Crop Sci. 176, 289–294. doi: 10.1111/j.1439-037X.1996.tb00473.x
Barot, S., Allard, V., Cantarel, A., Enjalbert, J., Gauffreteau, A., Goldringer, I., et al. (2017). Designing mixtures of varieties for multifunctional agriculture with the help of ecology. a review. Agron. Sustain. Dev. 37:13. doi: 10.1007/s13593-017-0418-x
Bedoussac, L., and Justes, E. (2011). A comparison of commonly used indices for evaluating species interactions and intercrop efficiency: application to durum wheat-winter pea intercrops. Field Crops Res. 124, 25–36. doi: 10.1016/j.fcr.2011.05.025
Bitocchi, E., Bellucci, E., Giardini, A., Rau, D., Rodriguez, M., Biagetti, E., et al. (2013). Molecular analysis of the parallel domestication of the common bean (Phaseolus vulgaris) in Mesoamerica and the Andes. New Phytol. 197, 300–313. doi: 10.1111/j.1469-8137.2012.04377.x
Bommarco, R., Kleijn, D., and Potts, S. G. (2013). Ecological intensification: harnessing ecosystem services for food security. Trends Ecol. Evol. 28, 230–238. doi: 10.1016/j.tree.2012.10.012
Botelho, F. B. S., Ramalho, M. A. P., Abreu, A. F. B., and Rezende, B. A. (2008). Estimates of compensation and stability parameters in common bean lines aiming at multilines. Crop Breed. Appl. Biotechnol. 8, 339–345. doi: 10.12702/1984-7033.v08n04a12
Bowden, R., Shoyer, J., Roozeboom, K., Claasen, M., Evans, P., Gordon, B., et al. (2001). Performance of wheat variety blends in Kansas. Kansas State University. Agric. Extension Bull. 128. Available online at: www.oznet.ksu.edu/library/crpsl2/SRL128.pdf
Brown, D. M. (1993). Crop Heat Units for Corn and Other Warm-Season Crops in Ontario. Toronto, ON: Ontario Ministry of Agriculture and Food.
Browning, J. A., and Frey, K. J. (1969). Multiline cultivars as a means of disease control. Ann. Rev. Phytopathol. 7, 355–382. doi: 10.1146/annurev.py.07.090169.002035
Bybee-Finley, K. A., and Ryan, M. R. (2018). Advancing intercropping research and practices in industrialized agricultural landscapes. Agriculture 8:80. doi: 10.3390/agriculture8060080
Cardinale, B. J., Duffy, J. E., Gonzalez, A., Hooper, D. U., Perrings, C., Venail, P., et al. (2012). Biodiversity loss and its impact on humanity. Nature 486, 59–67. doi: 10.1038/nature11148
Conner, R. L., Boland, G. J., Gillard, C. L., Chen, Y., Shan, X., McLaren, D. L., et al. (2020). Identification of anthracnose races in Manitoba and Ontario from 2005 to 2015 and their reactions on Ontario dry bean cultivars. Can. J. Plant Sci. 100, 40–55. doi: 10.1139/cjps-2019-0003
Connolly, J. (1986). On difficulties with replacement-series methodology in mixture experiments. J. Appl. Ecol. 23, 125–137. doi: 10.2307/2403086
Connolly, J., Goma, H.C., and Rahim, K. (2001a). The information content of indicators in intercropping research. Agric. Ecosyst. Environ. 87, 191–207. doi: 10.1016/S0167-8809(01)00278-X
Connolly, J., Wayne, P. W., and Bazzaz, F. A. (2001b). Interspecific competition in plants: how well do current methods answer fundamental questions? Amer. Natural. 157, 107–125. doi: 10.1086/318631
Creissen, H. E., Jorgensen, T. H., and Brown, J. K. M. (2016). Increased yield stability of field-grown winter barley (Hordeum vulgare L.) varietal mixtures through ecological processes. Crop Protect. 85, 1–8. doi: 10.1016/j.cropro.2016.03.001
Diaz, L. M., Ricaurte, J., Cajiao, C., Galeano, C. H., Rao, I., Beebe, S., et al. (2017). Phenotypic evaluation and QTL analysis of yield and symbiotic nitrogen fixation in a common bean population grown with two levels of phosphorus supply. Mol. Breed. 37:76. doi: 10.1007/s11032-017-0673-1
Diaz, L. M., Ricaurte, J., Tovar, E., Cajiao, C., Terân, H., Grajales, M., et al. (2018). QTL analysis for tolerance to abiotic stresses in common bean (Phaseolus vulgaris L.) population. PLoS ONE 13:e0202342. doi: 10.1371/journal.pone.0202342
Essah, S. Y. C., and Stoskopf, N. C. (2002). Mixture performance of phenotypically contrasting barley cultivars. Can. J. Plant Sci. 82, 1–6. doi: 10.4141/P01-043
Fageria, N. K. (1992). “Multiple-cropping systems and crop yields,” in Maximizing Crop Yields, ed. G. Stotzky (New York, NY: Marcel Dekker, Inc.), 81–104.
Federer, W.T. (1993). Statistical Design and Analysis for Intercropping Experiments, Vo. 1: Two crops. Springer Series in Statistics, New York, NY: Springer-Verlag. doi: 10.1007/978-1-4613-9305-4
Federer, W.T., Connigale, J.C, Rutger, J.N., and Wijesinha, A. (1982). Statistical analyses of yields from uniblends and biblends of eight dry bean cultivars. Crop Sci. 22, 111–115. doi: 10.2135/cropsci1982.0011183X002200010026x
Finckh, M. R., Gacek, E. S., Goyeau, H., Lannou, C., Merz, U., Mundt, C. C., et al. (2000). Cereal variety and species mixtures in practice, with emphasis on disease resistance. Agronomie 20, 813–837. doi: 10.1051/agro:2000177
Frey, K. J., and Maldonado, U. (1967). Relative productivity of homogeneous and heterogeneous oat cultivars in optimum and suboptimum environments. Crop Sci. 7, 532–535. doi: 10.2135/cropsci1967.0011183X000700050037x
Gaba, S., Fried, G., Kazakou, E., Chauvel, B., and Navas, M.-L. (2014). Agroecological weed control using a functional approach: a review of cropping systems diversity. Agron. Sustain. Dev. 34, 103–119. doi: 10.1007/s13593-013-0166-5
Gallandt, E.R., Dofing, S. M., Reisenauer, P. E., and Donaldson, E. (2001). Diallel analysis of cultivar mixtures in winter wheat. Crop Sci. 41, 792–796. doi: 10.2135/cropsci2001.413792x
Gaudio, N., Escobar-Gutiérrez, A. J., Casadebaig, P., Evers, J. B., Gérard, F., Louarn, G., et al. (2019). Current knowledge and future research opportunities for modeling annual crop mixtures. a review. Agron. Sustain. Develop. 39:20. doi: 10.1007/s13593-019-0562-6
Giunta, F., Cadeddu, F., Mureddu, F., Virdis, A., and Motzo, R. (2020). Triticale cultivar mixtures: productivity, resource use and resource use efficiency in a Mediterranean environment. Eur. J. Agron. 115:126019. doi: 10.1016/j.eja.2020.126019
Griffing, G. (1956). Concept of general and specific combining ability in relation to diallel crossing systems. Aust. J. Biol. Sci. 9, 463–493. doi: 10.1071/BI9560463
Guler, S., and Ozcelik, H. (2007). Relationship between leaf chlorophyll and yield related characters of dry bean (Phaseolus vulgaris L.). Asian J. Plant Sci. 6, 700–703. doi: 10.3923/ajps.2007.700.703
Hamblin, J., and Zimmermann, M. J. (1986). “Breeding common bean for yield in mixtures,” in Plant Breeding Reviews. ed. J. Janick (Westport: The AVI), 245–272.
Henry, A., Rosas, J. C., Beaver, J. S., and Lynch, J. P. (2010). Multiple stress response and belowground competition in multilines of common bean (Phaseolus vulgaris L.). Field Crops Res. 117, 209–218. doi: 10.1016/j.fcr.2010.03.004
Horner, A., Browett, S. S., and Antwis, R.E. (2019). Mixed-cropping between field pea varieties alters root bacterial and fungal communities. Sci Rep. 9:16953. doi: 10.1038/s41598-019-53342-8
IRRI (2014). PBTools, version 1.4. Biometrics and Breeding Informatics, PBGB Division, International Rice Research Institute. Los Baños, CA.
Jasienski, M., and Bazzaz, F. A. (1999). The fallacy of ratios and the testability of models in biology. Oikos 84, 321–326. doi: 10.2307/3546729
Jolliffe, P. A. (2000). The replacement series. J. Ecol. 88, 371–385. doi: 10.1046/j.1365-2745.2000.00470.x
Jolliffe, P. A., and Wanjau, F. M. (1999). Competition and productivity in crop mixtures: some properties of productive intercrops. J. Agric. Sci. 132, 425–435. doi: 10.1017/S0021859699006450
Kaiser, H. F. (1958). The varimax criterion for analytic rotation in factor analysis. Psychometrika 23, 187–200. doi: 10.1007/BF02289233
Kamfwa, K., Cichy, K. A., and Kelly, J. D. (2015). Genome-wide association study of agronomic traits in common bean. Plant Genome 8, 1–12. doi: 10.3835/plantgenome2014.09.0059
Kelly, J. D. (2001). Remaking bean plant architecture for efficient production. Advan. Agron. 71, 109–143. doi: 10.1016/S0065-2113(01)71013-9
Khanal, R., Rupert, T., Navabi, A., Smith, T. H., Michaels, T. T., Burt, A. J., et al. (2016b). Bolt common bean. Can. J. Plant Sci. 96, 218–221. doi: 10.1139/cjps-2015-0180
Khanal, R., Smith, T. H., Michaels, T. E., and Pauls, K. P. (2016a). Dynasty kidney bean. Can. J. Plant Sci. 96, 215–217. doi: 10.1139/cjps-2015-0194
Khanal, R., Smith, T. H., Michaels, T. E., and Pauls, K. P. (2017). Lighthouse common bean. Can. J. Plant Sci. 97, 165–168. doi: 10.1139/CJPS-2016-0073
Khush, G. S. (2001). Green revolution: the way forward. Nat. Rev. Genet. 2, 815–822. doi: 10.1038/35093585
Kiær, L.P., Skovgaard, I.M., and Østergard, H. (2009). Grain yield increase in cereal variety mixtures: a meta-analysis of field trials. Field Crops Res. 114, 361–373. doi: 10.1016/j.fcr.2009.09.006
Kiær, L. P., Skovgaard, I. M., and Østergard, H. (2012). Effects of inter-varietal diversity, biotic stresses and environmental productivity on grain yield of spring barley variety mixtures. Euphytica 185, 123–138. doi: 10.1007/s10681-012-0640-1
Kozak, M., and Piepho, H.-P. (2017). What's normal anyway? Residual plots are more telling than significance tests when checking ANOVA assumptions. J. Agron. Crop Sci. 204, 86–98. doi: 10.1111/jac.12220
Kristoffersen, R., Jørgensen, L. N., Eriksen, L. B., Nielsen, G. C., and Kiær, L. P. (2020). Control of Septoria tritici blotch by winter wheat cultivar mixtures: meta-analysis of 19 years of cultivar trials. Field Crops Res. 249:107696. doi: 10.1016/j.fcr.2019.107696
Letourneau, D. K., Armbrecht, I., Rivera, B. S., Lerma, J. M., Carmona, E. J., Daza, M. C., et al. (2011). Does plant diversity benefit agroecosystems? A synthetic review. Ecol. Appl. 21, 9–21. doi: 10.1890/09-2026.1
Li, L., Yang, S., Li, X., Zhang, F., and Christie, F. (1999). Interspecific complementary and competitive interactions between intercropped maize and faba bean. Plant Soil 212, 105–114. doi: 10.1023/A:1004656205144
Litrico, I., and Violle, C. (2015). Diversity in plant breeding: a new conceptual framework. Trends Plant Sci. 20, 604–613. doi: 10.1016/j.tplants.2015.07.007
Lopez, C. G., and Mundt, C. C. (2000). Using mixing ability analysis from two-way cultivar mixtures to predict the performance of cultivars in complex mixtures. Field Crops Res. 68, 121–132. doi: 10.1016/S0378-4290(00)00114-3
Loreau, M., and Hector, A. (2001). Partitioning selection and complementarity in biodiversity experiments. Nature 412, 72–76. doi: 10.1038/35083573
Loreau, M., Naeem, S., Inchausti, P., Bengtsson, J., Grime, J. P., Hector, A., et al. (2001). Biodiversity and ecosystem functioning: current knowledge and future challenges. Science 294, 804–808. doi: 10.1126/science.1064088
Malézieux, E., Crozat, Y., Dupraz, C, Laurans, M., Makowski, D., Ozier-Lafontaine, H., et al. (2009). Mixing plant species in cropping systems: concepts, tools and models. a review. Agron. Sustain. Dev. 29, 43–62. doi: 10.1051/agro:2007057
Mead, R. (1990). A Review of Methodology for the Analysis of Intercropping Experiments. Series: CIMMYT Training Working Document 6. Mexico: CIMMYT.
Mead, R., and Riley, J. (1981). A review of statistical ideas relevant to intercropping research. J. R. Statist. Soc. A 144, 462–509. doi: 10.2307/2981827
Monje, O. A., and Bugbee, B. (1992). Inherent limitations of non-destructive chlorophyll meters: a comparison of two types of meters. Hort. Sci. 27, 69–71. doi: 10.21273/HORTSCI.27.1.69
Mukeshimana, G., Butare, L., Cregan, P. B., Blair, M. W., and Kelly, J. D. (2014). Quantitative trait loci associated with drought tolerance in common bean. Crop Sci. 54, 923–938. doi: 10.2135/cropsci2013.06.0427
Mundt, C. C. (2002). Use of multiline cultivars and cultivar mixtures for disease management. Annu. Rev. Phytopathol. 40, 381–410. doi: 10.1146/annurev.phyto.40.011402.113723
Navabi, A., Balasubramanian, P., Pauls, K. P., Bett, K., and Hou, H. (2014). Genetic diversity of the Canadian dry bean varieties release since 1930: a pedigree analysis. Crop Sci. 54, 993–1003. doi: 10.2135/cropsci2013.04.0210
Ntahimpera, N., Dillard, H. R., Cobb, A. C., and Seem, R. C. (1996). Anthracnose development in mixtures of resistant and susceptible dry bean cultivars. Phytopathology 86, 668–673. doi: 10.1094/Phyto-86-668
Nyikako, J., Schierholt, A., Kessel, B., and Becker, H.C. (2014). Genetic variation in nitrogen uptake and utilization efficiency in a segregating DH population of winter oilseed rape. Euphytica 199, 3–11. doi: 10.1007/s10681-014-1201-6
Okonya, J. S., and Maass, B. L. (2014). Potential of cowpea variety mixtures to increase yield stability in subsistence agriculture: preliminary results. Inter. J. Agron., 1–7. doi: 10.1155/2014/515629
OMAFRA (2018). Soil fertility Handbook, Publication 611. Available online at: http://www.omafra.gov.on.ca/english/crops/pub611/pub611.pdf (accessed April 28, 2020).
Østergård, H., Finckh, M. R., Fontaine, L., Goldringer, I., Hoad, S. P., Kristensen, L., et al. (2009). Time for a shift in crop production: embracing complexity through diversity at all levels. J. Sci. Food Agric. 89, 1439–1445. doi: 10.1002/jsfa.3615
Panse, A., Davis, J. H. C, and Fischbeck, G. (1989). Compensation-induced yield gains in mixtures of common bean (Phaseolus vulgaris L.). J. Agron. Crop Sci. 162, 347–353. doi: 10.1111/j.1439-037X.1989.tb00727.x
Park, S. J., Rupert, T., Yu, K., and Navabi, A. (2009). Red rider common bean. Can. J. Plant Sci. 89, 925–927. doi: 10.4141/CJPS09016
Pastor-Corrales, M. A., Otoya, M. M., Molina, A., and Singh, S. P. (1995). Resistance to Colletotrichum lindemuthianum isolates from Middle America and Andean South America in different common bean races. Plant Dis. 79, 63–67. doi: 10.1094/PD-79-0063
Polania, J., Poschenrieder, C., Beebe, S., and Rao, I. M. (2016). Effective use of water and increased dry matter partitioned to grain contribute to yield of common bean improved for drought resistance. Front. Plant Sci. 7:660. doi: 10.3389/fpls.2016.00660
Polania, J., Rao, I., Cajiao, C., Grajales, M., Rivera, M., Velasquez, C., et al. (2017). Shoot and root traits contribute to drought resistance in recombinant inbred lines of MD 23-24 x SEA 5 of common bean. Front. Plant Sci. 8:296. doi: 10.3389/fpls.2017.00296
Prasad, R. C., Paudel, M. N., Ghimire, N. H., and Joshi, B. K. (2016). Cultivar mixtures in bean reduced disease infestation and increased grain yield under mountain environment of Nepal. Agron. J. Nepal. 4, 128–135. doi: 10.3126/ajn.v4i0.15535
Rao, M. R., and Willey, R. W. (1980). Evaluation of yield stability in intercropping: Studies on sorghum/pigeonpea. Exp. Agric. 16, 105–116. doi: 10.1017/S0014479700010796
Reiss, E. R., and Drinkwater, L. E. (2018). Cultivar mixtures: a meta-analysis of the effect of intraspecific diversity of crop yield. Ecol. Appl. 28, 62–77. doi: 10.1002/eap.1629
Riley, R. H., Brick, M. A., Conniff, K. L., Wood, D. R., Pearson, C. H., and Chapman, P. L. (1993). Response of seed yield and yield components in mixtures of dry edible bean. Field Crops Res. 33, 283–292. doi: 10.1016/0378-4290(93)90086-3
Schipanski, M. E., Macdonald, G. K., Rosenzweig, S., Chappell, M. J., Bennett, E. M., Bezner Kerr, R., et al. (2016). Realizing resilient food systems. BioSci 66, 600–610. doi: 10.1093/biosci/biw052
Singh, J., Gezan, S. A., and Vallejos, C. E. (2019). Developmental pleiotropy shaped the roots of the domesticated common bean (Phaseolus vulgaris). Plant Physiol. 180, 1467–1479. doi: 10.1104/pp.18.01509
Smith, T. H., Michaels, T. E., Navabi, A., and Pauls, K. P. (2012a). Rexeter common bean. Can. J. Plant Sci. 92, 351–353. doi: 10.4141/cjps2011-184
Smith, T. H., Michaels, T. E., Navabi, A., and Pauls, K. P. (2012b). OAC Inferno common bean. Can. J. Plant Sci. 92, 589–592. doi: 10.4141/cjps2011-186
Smithson, J. B., and Lenne, J. M. (1996). Varietal mixtures: a viable strategy for sustainable productivity in subsistence agriculture. Ann. Appl. Biol. 128, 127–158. doi: 10.1111/j.1744-7348.1996.tb07096.x
Snaydon, R. W. (1991). Replacement or additive designs for competition studies? J. Appl. Ecol. 28, 930–946. doi: 10.2307/2404218
Snyder, L. D., Gómez, M. I., and Power, A. G. (2020). Crop varietal mixtures as a strategy to support insect pest control, yield, economic, and nutritional services. Front. Sustain. Food Syst. 4:60. doi: 10.3389/fsufs.2020.00060
Ssekandi, W., Mulumba, J. W., Colangelo, P., Nankya, R., Fadda, C., Karungi, J., et al. (2016). The use of common bean (Phaseolus vulgaris) traditional varieties and their mixtures with commercial varieties to manage bean fly (Ophiomyia spp.) infestations in Uganda. J. Pest Sci. 89, 45–57. doi: 10.1007/s10340-015-0678-7
Strock, C. F., Burridge, L., Massas, JA. S. F., Beaver, J., Beebe, S., Camilo, S. A., et al. (2019). Seedling root architecture and its relationship with seed yield across diverse environments in Phaseolus vulgaris. Field Crops Res. 237, 53–64. doi: 10.1016/j.fcr.2019.04.012
Tilman, D., Cassman, K. G., Matson, P. A., Naylor, R., and Polasky, S. (2002). Agricultural sustainability and intensive production practices. Nature 418, 671–677. doi: 10.1038/nature01014
Tooker, J. F., and Frank, S. D. (2012). Genotypically diverse cultivar mixtures for insect pest management and increased crop yields. J. Appl. Ecol. 49, 974–958. doi: 10.1111/j.1365-2664.2012.02173.x
Tratwal, A., and Bocianowski, J. (2018). Cultivar mixtures as part of integrated protection of spring barley. J. Plant Dis. Prot. 125, 41–50. doi: 10.1007/s41348-017-0139-z
Trenbath, B. R. (1974). Biomass productivity of mixtures. Advances Agron. 26, 177–210. doi: 10.1016/S0065-2113(08)60871-8
Vidala, T., Boixela, A.-L., Duranda, B., de Vallavieille-Popeb, C., Hubera, L., and Saint-Jean, S. (2017). Reduction of fungal disease spread in cultivar mixtures: impact of canopy architecture on rain-splash dispersal and on crop microclimate. Agric. Forest Meteorol. 246, 154–161. doi: 10.1016/j.agrformet.2017.06.014
Voisey, P. W. (1971). The Ottawa texture measuring system. J. Can. Inst. Food Sci. Technol. 4, 91–103. doi: 10.1016/S0008-3860(71)74189-0
Wang, Y., Zhang, Y., Ji, W., Yu, P., Wang, B., Li, J., et al. (2016). Cultivar mixture cropping increased water use efficiency in winter wheat under limited irrigation conditions. PLoS ONE 11:e0158439. doi: 10.1371/journal.pone.0158439
Weigelt, A., and Jolliffe, P. (2003). Indices of plant competition. J. Ecol. 91, 707–720. doi: 10.1046/j.1365-2745.2003.00805.x
Wijesinha, A., Federer, W. T., Carvalho, J. R., and De Aquino Portes, T. (1982). Some statistical analyses for a maize and beans intercropping experiment. Crop Sci. 22, 660–666. doi: 10.2135/cropsci1982.0011183X002200030057x
Williams, A. C., and McCarthy, B. C. (2001). A new index of interspecific competition for replacement and additive designs. Ecol. Res. 16, 29–40. doi: 10.1046/j.1440-1703.2001.00368.x
Wilson, J.B. (1988). Shoot competition and root competition. J. Appl. Ecol. 25, 279–296. doi: 10.2307/2403626
Wolfe, M. S. (1985). The current status and prospects of multiline cultivars and variety mixtures for disease resistance. Ann. Rev. Phytopathol. 23, 251–273. doi: 10.1146/annurev.py.23.090185.001343
Keywords: common bean, cultivar mixtures, diversity, yield, mixing efficiency
Citation: Reinprecht Y, Schram L, Smith TH and Pauls KP (2020) Enhancing In-crop Diversity in Common Bean by Planting Cultivar Mixtures and Its Effect on Productivity. Front. Sustain. Food Syst. 4:126. doi: 10.3389/fsufs.2020.00126
Received: 15 May 2020; Accepted: 22 July 2020;
Published: 02 September 2020.
Edited by:
Johann G. Zaller, University of Natural Resources and Life Sciences Vienna, AustriaReviewed by:
Suprasanna Penna, Bhabha Atomic Research Centre (BARC), IndiaMaria Conceição Caldeira, School of Agriculture, University of Lisbon, Portugal
Copyright © 2020 Reinprecht, Schram, Smith and Pauls. This is an open-access article distributed under the terms of the Creative Commons Attribution License (CC BY). The use, distribution or reproduction in other forums is permitted, provided the original author(s) and the copyright owner(s) are credited and that the original publication in this journal is cited, in accordance with accepted academic practice. No use, distribution or reproduction is permitted which does not comply with these terms.
*Correspondence: Yarmilla Reinprecht, eXJlaW5wcmVAdW9ndWVscGguY2E=