- 1Department of Plant Science, University of Saskatchewan, Saskatoon, SK, Canada
- 2Swift Current Research and Development Centre, Agriculture & Agri-Food Canada, Swift Current, SK, Canada
Intercropping with different crop species and different spatial patterns is suggested to lead to increased competition with weeds and reduced weed abundance and biomass. In this study, our objective was to explore the ability of multi-species annual forage crop mixtures to control weeds while providing productive forage. We utilized field and greenhouse trials to evaluate the impact of different crop mixtures and row spacing on weed control in the semi-arid Brown soil region of southwestern Saskatchewan, Canada. Seven different mixtures of up to eight annual forage crops were grown with row spacing of 15 or 30 cm in a replicated field trial. Weed abundance and biomass were significantly affected by crop species mixtures. Crop mixtures that contained radish and barley generally had higher weed suppression. Row spacing did not significantly impact weed abundance or biomass across the treatments. Results were similar over both years in spite of drastically different precipitation conditions. Forage production was significantly different between cropping mixtures in July in both years. The barley-radish mixture had the highest crop biomass in July, and this early crop production was linked to weed suppression because crop biomass had a significant effect on weed abundance and biomass in July, but not August. A greenhouse experiment was used to further evaluate the crops (i.e., barley and radish) that demonstrated the highest weed suppressive activity in the field trial. Crop identity and row spacing were both significant factors affecting weed and crop biomass production. Radish exhibited stronger control of common lamb's quarters (Chenopodium album) compared to barley, but the binary mixture of the two species produced the highest crop biomass and equivalent weed control compared to the radish monoculture. This research suggests that cropping with multiple species (particularly forage radish) may be an effective way to control weeds in semiarid environments.
Introduction
The shift toward more sustainable agricultural practices due to concerns over biodiversity loss, environmental pollution, and greenhouse gas emissions has promoted reduced reliance on agrochemicals (Gomiero et al., 2011). This is particularly relevant for weed control in annual cropping systems due to the extensive use of herbicides. Diversification of cropping patterns and crop species selection may provide one way to lower reliance on chemical inputs. Multiple species cropping (polyculture) is the spatial or temporal intermixing of growing multiple crop species selected based on their contributions to the system (e.g., legumes included for nitrogen fixation) or complementarity with other species [e.g., varying plant architecture and resource use Anil et al., 1998] on the same land base in the same year. Multiple cropping systems can increase productivity through resource partitioning and other ecosystem benefits may accompany these diverse systems including increased soil health and water use efficiency (Anil et al., 1998; Bedoussac and Justes, 2011). In many cases, polycultures have also been associated with weed suppression via increased competition or allelopathic potential (Liebman and Dyck, 1993; Mohler, 2001; Szumigalski and Van Acker, 2005).
Important factors that influence weed dynamics in multiple cropping or intercropping systems are planting density, row spacing, and selection of crop species. From an agronomic perspective, it has long been suggested that increased crop density can reduce the competitive ability of weeds, although this is not always correlated with narrower row spacing (Mohler, 2001). In general, narrower row spacing of crops should increase the interception of light by crop plants, and row intercropping with species of varying canopy structure can increase light use efficiency and increase the competitive pressure on weeds (Anil et al., 1998). Seeding functionally diverse crop mixtures in different densities and arrangements can increase the competitive ability and resource utilization of the crop, both of which can reduce weed abundance (Lowry and Smith, 2018). In a meta-analysis, Verret et al. (2017) found that intercropping with a companion crop (i.e., a crop not harvested as a cash crop) resulted in significantly lower weed biomass and often higher crop yield. For example, several studies have shown that intercropping mixtures had better weed suppression than either crop planted alone (Izaurralde et al., 1993; Hauggaard-Nelson et al., 2001; Nelson et al., 2012; Wang et al., 2012) or had comparable weed suppression to the better of the crops planted alone (Poggio, 2005; Deveikyte et al., 2009; Begna et al., 2011). However, the opposite has also been found, for example, intercropped mixtures of maize and climbing bean didn't show any improvement in weed control over the monoculture maize and in some cases decreased crop yield (Nurk et al., 2017). Most intercropping studies focus on binary mixtures, and little is known about weed dynamics in annual intercropping with three or more crops, particularly in temperate forage-based systems.
In this study, we explored the potential for weed control through the combination of two aspects of intercropping—crop diversity and variable row spacing—in annual forage crops in the semiarid prairie region of southwestern Saskatchewan, Canada. Annual forage crops are currently being evaluated for their potential as a low-input addition to standard crop rotations in Saskatchewan, and maximizing low-input weed control in these crops will enhance the benefit of this system for producers. Our hypotheses were: (1) higher crop species diversity will be better at suppressing weeds, and (2) narrower crop row spacing will reduce weed abundance and biomass. The hypotheses were tested in two field trials followed by two greenhouse trials.
Materials and Methods
Site
The field trials were located on a Brown Chernozem soil at the Swift Current Research and Development Center, southeast of Swift Current, Saskatchewan (50°16′N lat., 107°43′W long., 824 m elev.). The land for the 2015 study was seeded to oats in 2013 and chem fallowed in 2014, and the land for the 2016 study was seeded to wheat in 2014 and chem fallowed in 2015. Both sites were tilled and harrow packed prior to seeding.
Field Trials
Field trials were carried out in the summers of 2015 and 2016. Eight annual forage crops were selected that are known to grow well in the local environment, and that were also being tested in long-term multi-species cropping trials. The species represent four functional groups: cool season grasses—barley (Hordeum vulgare “AC Metcalfe”), oats (Avena sativa “Common No. 1”), triticale (Triticosecale “Bunker”); a warm season grass—corn (Zea mays “Roundup Ready Corn 2”); legumes—forage pea (Pisum sativum “CDC Leroy”), hairy vetch (Vicia villosa “Common No. 1”); and brassicas—radish (Raphanus sativus “Common No. 1”), turnip (Brassica rapa subsp. rapa “Purple Top”). These crops were used in seven different mixtures (see Table 1) seeded in two different row spacings: species planted together in rows 30 cm apart, or planted in alternating rows 15 cm apart. Total crop densities were the same in all plots regardless of row spacing.
The experimental design was a full factorial randomized complete block with the seven cropping treatments, two row spacing treatments, and four blocks. Each block had 14 plots measuring 6 m long by 1.8 m wide. These proportions matched the dimensions of the self-propelled hydrostatic seeder used, which seeded six rows with 30 cm between rows. The 15 cm spaced treatments were seeded in a second pass (a second pass without seeding was included on the 30 cm plots). Crop density was 50 live seeds per linear meter at the 15 cm spacing and 100 live seeds per meter at 30 cm, resulting in the same amount of live seeds per unit area. In the mixtures, the component crops were seeded in equal proportions. A seeding depth of 2 cm was selected as an intermediate depth suitable for all species. Plots were seeded on June 4, 2015 and June 3, 2016. The later seeding dates are a reflection of dates selected for the provision of late-season grazing material as well as environmental conditions at the time of seeding (e.g., extremely wet spring in 2016). Data collection from each plot included crop biomass (clipped at 5 cm from a 0.25 m2 quadrat, and dried at 60°C) and weed count and identification (within a 0.25 m2 quadrat). Sampling dates were July 22 and August 20 in 2015, and July 19 and August 22 in 2016. In 2016 weed biomass was also assessed by clipping, drying and weighing all weeds in the 0.25 m2 quadrat at the time of crop biomass sampling.
Greenhouse Trials
Following the 2015 field trial, the crop mixture with the best weed control (barley-radish mixture) was selected for further studies in the greenhouse. As radish was not grown as a monoculture in the field trial, we wanted to investigate the relative impact of both crops on weed control. The greenhouse trial was conducted twice, in 2016 and 2018. In the greenhouse, 24 plastic bins (53 cm long × 39 cm wide × 18 cm high) were filled with a soil mix of 1 part field soil, 1 part silica sand, and 1 part potting mix (Sunshine Mix #4, Sun Gro Horticulture). The cropping treatments were barley monoculture, radish monoculture, and barley-radish mixture (grown in the same rows). In each bin, two crop rows were seeded, with a spacing treatment of either 15 cm apart or 30 cm apart. Crops were seeded at the same rate as the field trials (100 live seeds per linear meter) resulting in two rows of 50 plants per bin. In the very middle of the bin between the cropping rows, Chenopodium album (common lamb's quarters) was seeded and thinned back to 20 plants after emergence. C. album was selected because it is a common agricultural weed in Western Canada that was frequently present in our field trials. An additional control treatment of C. album seeded alone was included. Three replicates of each treatment were seeded (barley at 15 cm, barley at 30 cm, radish at 15 cm, radish at 30 cm, barley and radish at 15 cm, barley and radish at 30 cm, and weeds alone) resulting in a total of 28 bins. The greenhouse conditions were set for a 14 h day length, with a day temperature of 20°C and a night temperature of 15°C. Plants were watered consistently as needed (based on measuring soil moisture levels), and fertilizer (20-20-20) was applied twice in the middle of each trial, when plants showed signs of nutrient stress. Pots were rotated on the bench every 2 weeks to reduce spatial effects. Plants were harvested 8 weeks after seeding. Measurements included crop biomass, weed height, weed shoot dry weight, and weed root dry weight.
Statistical Analysis
All statistical analyses (field and greenhouse) were carried out in R v. 3.3.1 (R Core Team, 2016), using the packages Lme4 (Bates et al., 2015) and lmerTest (Kuznetsova et al., 2014). A mixed model fit via lmer was used with row spacing and crop mixture as fixed effects and block as a random effect. Multiple comparisons were made using the function difflsmeans. In both the field and greenhouse trials, preliminary analyses indicated that there were significant year by treatment effects, so in both studies the 2 years were analyzed independently. Generalized linear models (GLM) were used to determine if there was an effect of crop biomass production on weed biomass and abundance.
Results and Discussion
Field Trials
Weed abundance was significantly affected by crop mixtures in both July and August in 2015 and in July 2016 (Tables 2, 3). Total weed abundance was much lower in 2016 than 2015 and the barley-radish mixture had the lowest weed abundance overall. The four species mix (triticale-corn-peas-radish) also had relatively low weed populations over both site-years, and the eight species mix had low weed abundance in 2015. Treatments that included radish generally had fewer weeds than the other cropping combinations (Table 3). The forage radish used in this study has several factors that give it strong competitive ability: it is quick to germinate, grows rapidly, and has large above-ground biomass. Radish has the potential for allelopathy (e.g., Norsworthy, 2003), but it is likely the rapid canopy development of radish that plays the biggest role in weed suppression (Lawley et al., 2012). Barley has also been recognized as a good weed suppressant, both in monoculture and in mixture. In a study by Nelson et al. (2012), weed suppression was highest in treatments containing barley seeded alone and in intercrops. In 2016 we also measured weed biomass, which showed the same patterns as weed abundance (Tables 2, 4).
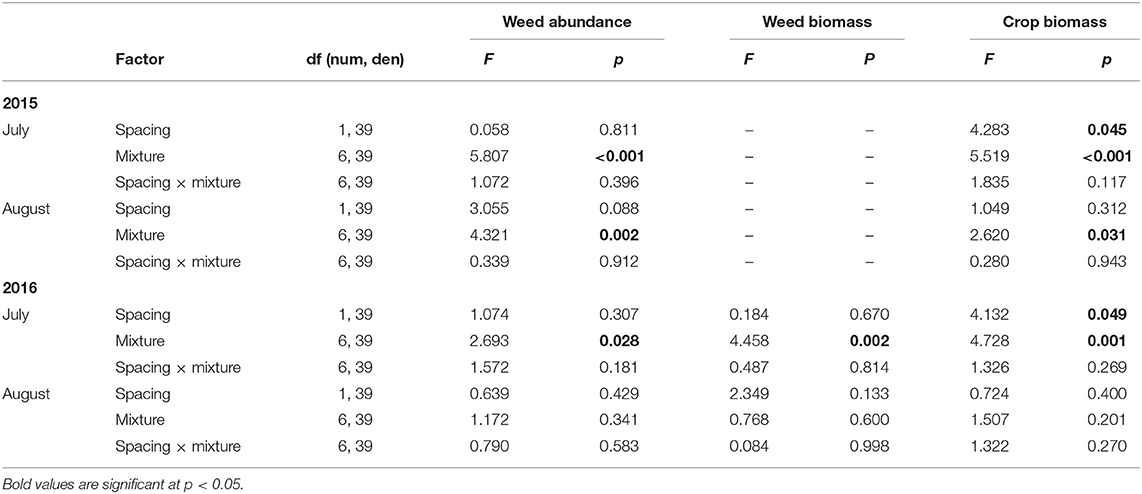
Table 2. Mixed model results of weed abundance (2015, 2016), weed biomass (2106), and crop biomass (2015, 2016) with crop spacing and crop mixture from the field trials.
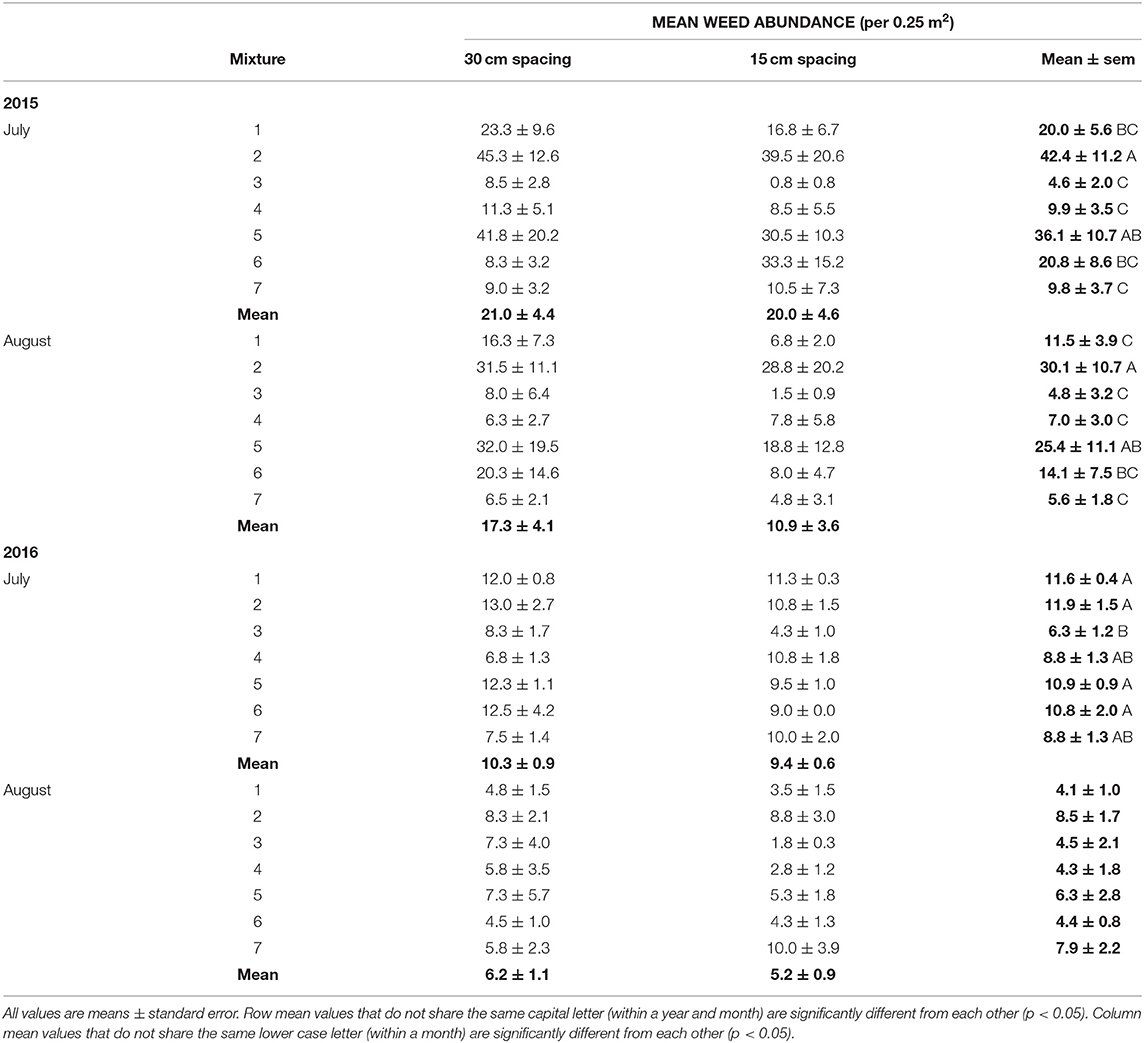
Table 3. Mean weed abundance by crop mixture treatment and row spacing (see Table 1 for list of cropping treatments) in July and August, from the 2015 and 2016 field trials.
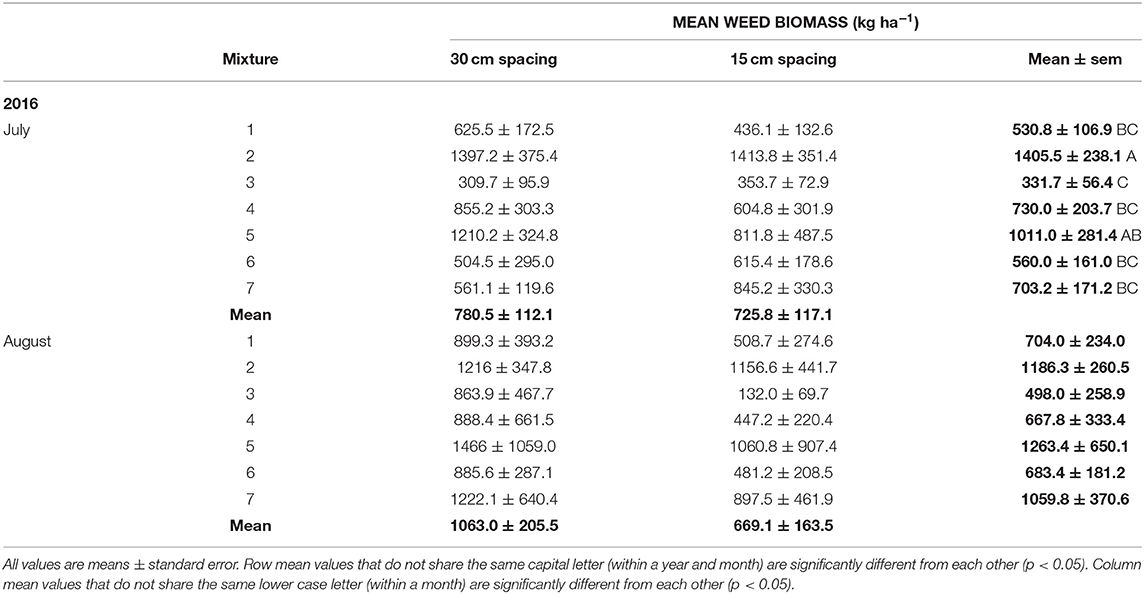
Table 4. Mean weed biomass by crop mixture treatment and row spacing (see Table 1 for list of cropping treatments) in July and August, from the 2016 field trial.
The effect of row spacing on weed abundance was not statistically significant (Tables 2, 3), although the lowest weed abundance overall was attributed to the 15 cm row spacing in the barley-radish intercrop. One factor that might lead to the high variability in results is the spatial heterogeneity of weed growth. Each field site had an uneven distribution of weed diversity likely related to weed dispersal and the existing seed bank. This resulted in certain site areas that had greater abundance of weeds, or the presence of large aggressive weeds which contributed considerably to the weed biomass measures in 2016, regardless of crop mixture and spacing. Other factors that may contribute to the lack of crop spacing effects include nutrient management, the relative heights of the crops, and the timing of weed and crop emergence (Mohler, 2001). Our seeding dates were later than those recommended for our region, and thus the emergence timing of the weeds and crops was similar. The close emergence timing meant that many weeds were established by the time the crop canopy was fully expanded. The different crop species also are likely to have variable rates of seedling recruitment (Szumigalski and Van Acker, 2005).
Crop biomass in 2015 significantly differed between species combinations in both July and August, but was only affected by row spacing in July (Tables 2, 5). At the July sampling date in both 2015 and 2016, the barley-radish intercrop had the highest biomass production at both crop row spacings. This early strong biomass production is a good indicator of the success of this mixture at controlling weeds. The GLMs supported this, showing a significant effect (p < 0.05) of crop biomass production on weed abundance and weed biomass in July. Crop spacing was not significant in August of both years. The barley monoculture and oats-peas mixture had the highest late season (August) crop biomass in 2015, while no significant differences among the crop mixtures were detected in 2016 (Table 5). The oats-peas mixture had the highest weed abundance and biomass each year (only significant at p < 0.05 in 2015), suggesting that late season crop growth has little impact on overall weed suppression. This is also supported by the GLMs, which found no significant effect (p > 0.05) of August crop biomass production on weed abundance or biomass.
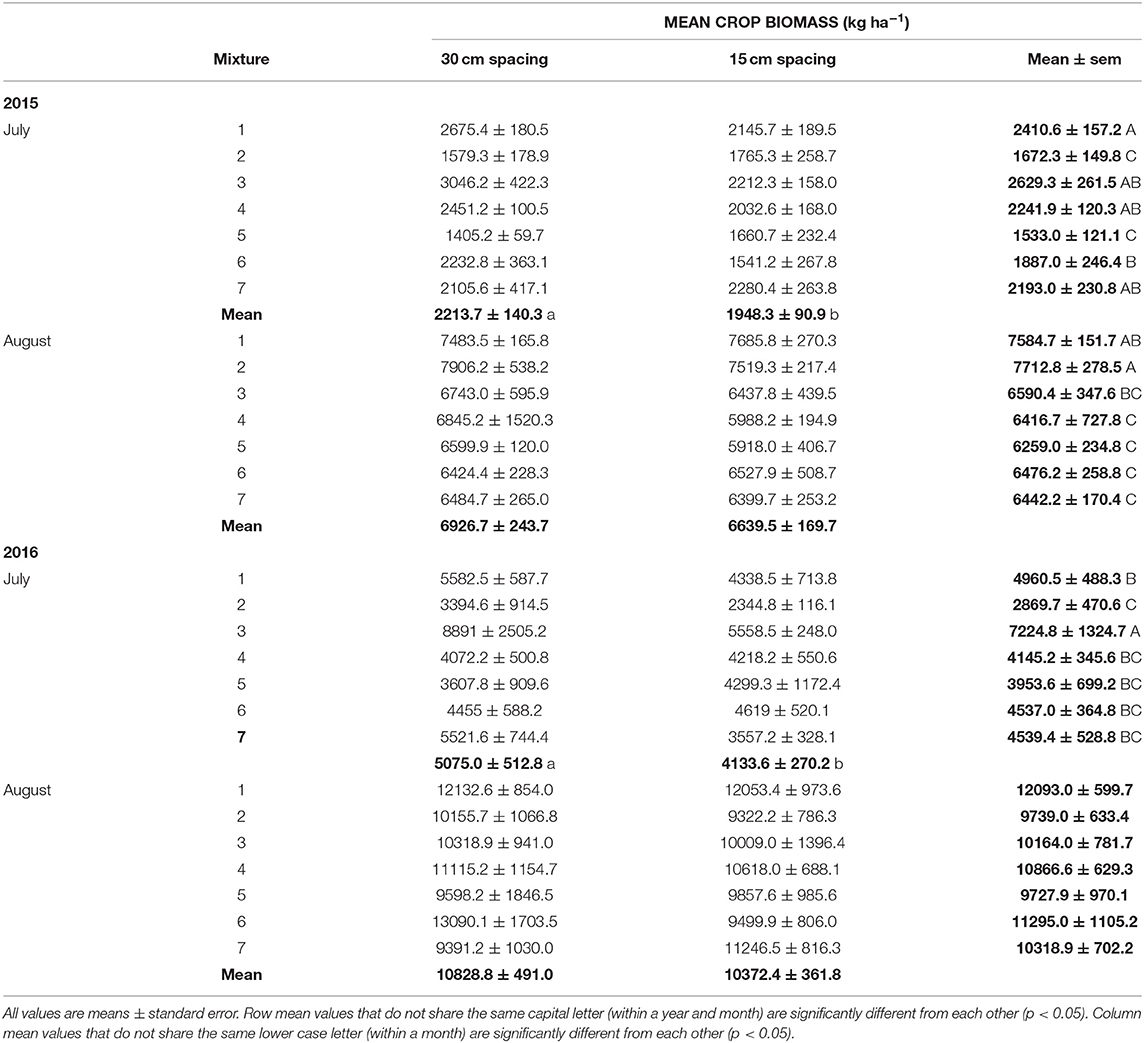
Table 5. Mean crop biomass by crop mixture treatment and row spacing (see Table 1 for list of cropping treatments) in July and August, from the 2015 and 2016 field trials.
Increased crop diversity is suggested to lead to higher productivity and yield (e.g., intercropping vs. monocultures; Bedoussac et al., 2015). This trend was not observed in our study. In 2015, the monoculture and two species mixtures had higher biomass production than the four and eight species mixtures, and in 2016 there were no significant differences in biomass between any of the cropping treatments (Table 5). However, the productivity of the two species mixtures (barley-radish, oats-peas) were comparable to the barley monoculture. This shows that the inclusion of certain crops (i.e., radish and barley) in mixtures can improve weed control, but not at the expense of reduced forage production. Further, more diverse mixtures can provide improved forage quality (Mischkolz et al., 2013), enhancing the advantages of multi-species crops.
During the growing season, monthly total precipitation was considerably different in 2015 and 2016 (Supplemental Figure 1). The spring of 2015 was particularly dry in southwestern Saskatchewan, experiencing one of the lowest levels of precipitation on record from May to mid-July, while the same period in 2016 was one of the wettest on record (Supplemental Figure 1). We expected that these contrasting conditions would have considerably altered the results of the spacing component. In particular, the dry field conditions in 2015 may have impacted the establishment and growth of some crop species (e.g., legumes) which would have reduced canopy closure in the narrower row spacing. Additionally, water availability may have been a more limiting factor for weed growth than light, reducing the importance of row spacing in a dry year relative to a wet year. However, even under the heavy precipitation of spring 2016, the lack of relationship between weed abundance and crop spacing was the same. We did see much lower weed abundance in 2016 than 2015, but this could also be a result of the different site locations. Olsen et al. (2012) found that drought conditions decreased the effect of crop spacing pattern on weed biomass production relative to non-drought conditions. When we assessed weed biomass in 2016, we did observe consistently reduced weed biomass in the 15 cm row spacing treatments at the August sampling date, which was supported by visual observations of stunted and senescing weeds that did not have nearly the seed set of weeds found in the 30 cm row spacing. If annual weeds are present but out-competed to the point of reduced fitness, then there is still a long-term benefit to these seeding patterns.
Greenhouse Trials
To better understand what factors contributed to weed control in the best cropping mixture of barley and radish, we grew these crops in the greenhouse to isolate the effect of row spacing and crop combination on weed control from environmental variation. In the 2016 greenhouse trial, crop spacing and crop mixture significantly affected C. album shoot and root biomass, as well as height (Table 6). In 2018, there was a significant effect of crop spacing and mixture on weed shoot biomass, but not root biomass, and weed height was only affected by mixture (and not spacing). In both years, all cropping and spacing treatments significantly lowered the shoot weight, root weight, and height of C. album compared to the control treatment of weeds grown alone (Tables 6, 7). In both trials, the radish monoculture and barley-radish mix reduced weed shoot biomass and height significantly more than barley alone. In all cropping treatments, the 15 cm row spacing reduced weed shoot biomass and height more than at 30 cm spacing, although the effect was not always significant. Finally, the barley-radish mixture had the highest crop biomass in both trials.
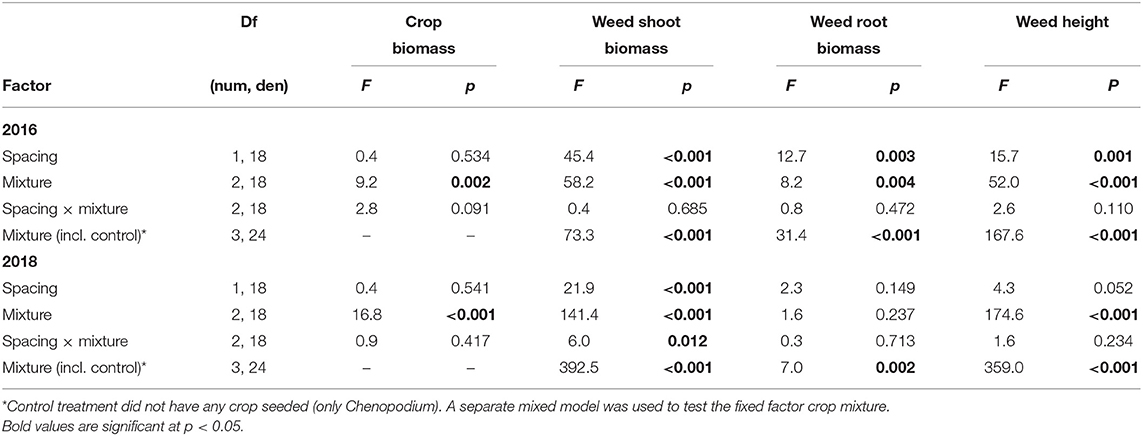
Table 6. Greenhouse experiments (2016 and 2018) mixed model results of crop biomass, weed shoot biomass, weed root biomass, and weed height with crop mixture and row spacing as fixed factors.
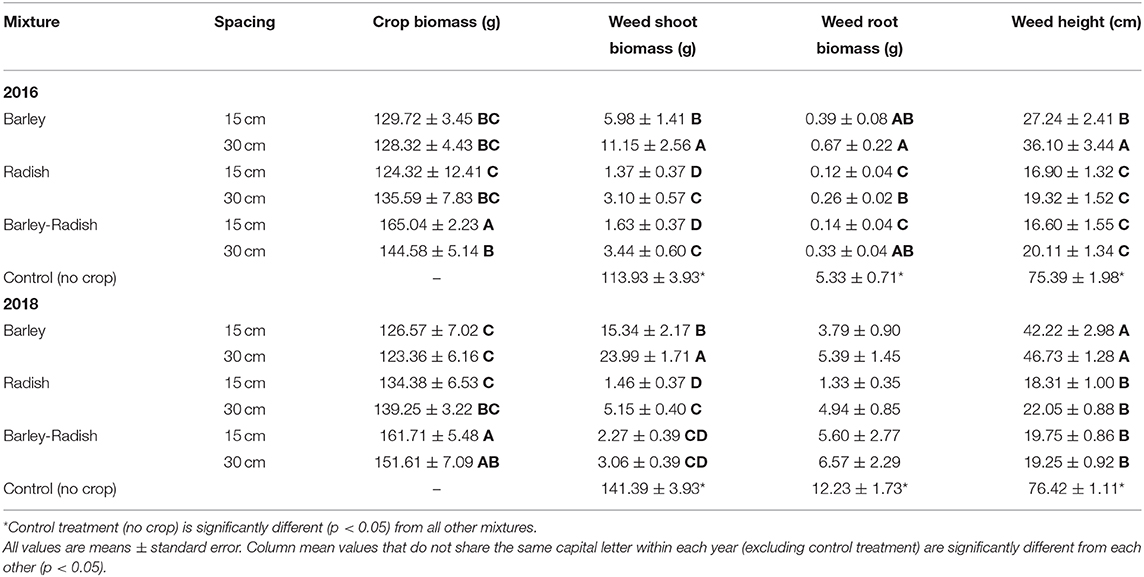
Table 7. Mean crop biomass, weed shoot biomass, weed root biomass, and weed height with crop mixture and row spacing for the 2016 and 2018 greenhouse experiments.
The greenhouse trials were different from the field trials in that row spacing had a significant effect on weed growth. This could be due to the growth of plants in a more restricted space, where competition for resources was accentuated by the limited space in a bin. Even though seeding density was the same in the greenhouse and the field, in the field there was a greater surrounding area for roots to grow into or draw resources from. In addition, the greenhouse trials only observed one weed species, C. album, which could be more susceptible to the increased competition for light at the 15 cm spacing than some of other weed species which we observed in the field. Other weed species in the field may have been more aggressive in their growth rate and form, which would have contributed more to weed biomass (e.g., large weeds such as redroot pigweed, Amaranthus retroflexus) or abundance (e.g., small prostrate weeds such as Portulaca oleracea).
The use of radish as a cover crop has previously been highlighted as a mechanism for weed control (e.g., Haramoto and Gallandt, 2004; Kruidhof et al., 2008; Lawley et al., 2011, 2012). In our greenhouse study, while the narrower row spacing had a significant effect on C. album shoot biomass, it is not clear which mechanism was most responsible for this—aboveground competition for light, or belowground competition for resources and/or the presence of allelopathic root compounds. Kruidhof et al. (2008) found that an autumn fodder radish cover crop severely decreased the abundance of C. album in the field, citing the main mechanism of control as light interception, although interestingly, seeding density did not impact weed suppression. Lawley et al. (2012) found that in-crop competition for light had the greatest impact on weed control, while the alellopathic potential of forage radish could not be documented in multiple field and controlled environment studies.
Conclusions
Weed abundance in the field was associated with the selection of different cropping mixtures, while cropping pattern with closer row spacing was less important in the field but was significant in the greenhouse trials. The selection of specific crops appears to provide significant weed control, in particular forage radish grown alone and in mixture with barley. The ability to maintain strong weed control when the two crops are grown together is important in a forage context, where having the two crops together would provide forage with a more balanced nutritional profile compared to radish alone. This study provides incentive for improving species selection in forage intercropping trials and testing spatial arrangements that might provide greater weed suppression.
Data Availability Statement
The datasets generated for this study are available on request to the corresponding author.
Author Contributions
JB, EL, and MPS designed the field experiment. JB conducted the field work. JB, EL, and MS designed the greenhouse experiment. JB and MS conducted the greenhouse work. JB, LB, and EL conducted statistical analyses. JB and LB wrote the manuscript, with contributions and editing from all authors.
Funding
Funding support was received from the Saskatchewan Agriculture Development Fund and Western Grains Research Foundation (project #20140057).
Conflict of Interest
The authors declare that the research was conducted in the absence of any commercial or financial relationships that could be construed as a potential conflict of interest.
Acknowledgments
We would like to thank Tyson Wiens, Perry Coward, Russ Muri, and Elaine Walliser for field and greenhouse assistance and Lei Ren and reviewers for comments on an earlier draft of this manuscript.
Supplementary Material
The Supplementary Material for this article can be found online at: https://www.frontiersin.org/articles/10.3389/fsufs.2020.00092/full#supplementary-material
References
Anil, L., Park, J., Phipps, R. H., and Miller, F. A. (1998). Temperate intercropping of cereals for forage: a review of the potential for growth and utilization with particular reference to the UK. Grass. Forage. Sci. 53, 301–317. doi: 10.1046/j.1365-2494.1998.00144.x
Bates, D., Maechler, M., Bolker, B., and Walker, S. (2015). Fitting linear mixed-effects models using lme4. J. Stat. Softw. 67, 1–48. doi: 10.18637/jss.v067.i01
Bedoussac, L., Journet, E. P., Hauggaard-Nielsen, H., Naudin, C., Corre-Hellou, G., Jensen, E. S., et al. (2015). Ecological principles underlying the increase of productivity achieved by cereal-grain legume intercrops in organic farming. A review. Agron. Sustain. Dev. 35, 911–935. doi: 10.1007/s13593-014-0277-7
Bedoussac, L., and Justes, E. (2011). A comparison of commonly used indices for evaluating species interactions and intercrop efficiency: application to durum wheat-winter pea intercrops. Field. Crop. Res. 124, 25–36. doi: 10.1016/j.fcr.2011.05.025
Begna, S. H., Fielding, D. J., Tsegaye, T., Van Veldhuizen, R., Angadi, S., and Smith, D. L. (2011). Intercropping of oat and field pea in alaska: an alternative approach to quality forage production and weed control. Acta Agric. Scand. Sect. B 61, 235–244. doi: 10.1080/09064711003745508
Deveikyte, I., Kadziuliene, Z., and Sarunaite, L. (2009). Weed suppression ability of spring cereal crops and peas in pure and mixed stands. Agron. Res. 7, 239–244.
Gomiero, T., Pimentel, D., and Paoletti, M. G. (2011). Is there a need for a more sustainable agriculture? Crit. Rev. Plant. Sci. 30, 6–23. doi: 10.1080/07352689.2011.553515
Haramoto, E. R., and Gallandt, E. R. (2004). Brassica cover cropping for weed management: a review. Renew. Agr. Food Syst. 19, 187–198. doi: 10.1079/RAFS200490
Hauggaard-Nelson, H., Ambus, P., and Jensen, E. S. (2001). Interspecific competition, N use and interference with weeds in pea-barley intercropping. Field Crop. Res. 70, 101–109. doi: 10.1016/S0378-4290(01)00126-5
Izaurralde, R., Juma, N., McGill, W., Chanasyk, D., Pawluk, S., and Dudas, M. (1993). Performance of conventional and alternative cropping systems in cryoboreal subhumid central Alberta. J. Agric. Sci. 120, 33–42. doi: 10.1017/S0021859600073561
Kruidhof, H. M., Bastiaans, L., and Kropff, M. J. (2008). Ecological weed management by cover cropping: effects on weed growth in autumn and weed establishment in spring. Weed. Res. 48, 492–502. doi: 10.1111/j.1365-3180.2008.00665.x
Kuznetsova, A., Brockhoff, P. B., and Christensen, R. H. B. (2014). lmerTest: Tests in Linear Mixed Effects Models. R package version 2.0-20. Available online at: http://CRAN.R-project.org/package=lmerTest
Lawley, Y. E., Teasdale, J. R., and Weil, R. R. (2012). The mechanism for weed suppression by a forage radish cover crop. Agron. J. 104, 205–214 doi: 10.2134/agronj2011.0128
Lawley, Y. E., Weil, R. R., and Teasdale, J. R. (2011). Forage radish cover crop suppresses winter annual weeds in fall and before corn planting. Agron. J. 103, 137–144. doi: 10.2134/agronj2010.0187
Liebman, M., and Dyck, E. (1993). Crop rotation and intercropping strategies for weed management. Ecol. Appl. 3, 92–122 doi: 10.2307/1941795
Lowry, C. J., and Smith, R. G. (2018). “Weed control through crop plant manipulations,” in Non-Chemical Weed Control, eds K. Jabran and B. S. Chauhan (London: Elsevier), 73–96. doi: 10.1016/B978-0-12-809881-3.00005-X
Mischkolz, J. M., Schellenberg, M. P., and Lamb, E. G. (2013). Early productivity and crude protein content of establishing forage swards composed of combinations of native grass and legume species in mixed-grassland ecoregions. Can. J. Plant. Sci. 93, 445–454 doi: 10.4141/cjps2012-261
Mohler, C. (2001). “Enhancing the competitive ability of crops,” in Ecological Management of Agricultural Weeds, eds M. Liebman, C. L. Mohler, and C. P. Staver (Cambridge: Cambridge University Press Pages), 269–321.
Nelson, A. G., Pswarayi, A., Quideau, S., Frick, B., and Spaner, D. (2012). Yield and weed suppression of crop mixtures in organic and conventional systems of the western canadian prairie. Agron. J. 104, 756–762. doi: 10.2134/agronj2011.0374
Norsworthy, J. K. (2003). Allelopathic potential of wild radish (Raphanus raphanistrum). Weed Technol. 17, 307–313. doi: 10.1614/0890-037X(2003)017[0307:APOWRR]2.0.CO;2
Nurk, L., Graß, R., Pekrun, C., and Wachendorf, M. (2017). Effect of sowing method and weed control on the performance of maize (Zea mays L.) intercropped with climbing beans (Phaseolus vulgaris L.). Agriculture 7:51. doi: 10.3390/agriculture7070051
Olsen, J. M., Griepentrog, H., Nielsen, J., and Weiner, J. (2012). How important are crop spatial pattern and density of weed suppression by spring wheat? Weed Sci. 60, 501–509. doi: 10.1614/WS-D-11-00172.1
Poggio, S. L. (2005). Structure of weed communities occurring in monoculture and intercropping of field pea and barley. Agric. Ecosyst. Environ. 109, 48–58. doi: 10.1016/j.agee.2005.02.019
R Core Team (2016). R: A Language and Environment for Statistical Computing. Vienna: R Foundation for Statistical Computing. Avaialble online at: http://www.R-project.org/
Szumigalski, A., and Van Acker, R. (2005). Weed suppression and crop production in annual intercrops. Weed Sci. 53, 813–825. doi: 10.1614/WS-05-014R.1
Verret, V., Gardarin, A., Pelzer, E., Médiène, S., Makowski, D., and Valantin-Morison, M. (2017). Can legume companion plants control weeds without decreasing crop yield? A meta-analysis. Field Crop. Res. 204, 158–168. doi: 10.1016/j.fcr.2017.01.010
Keywords: weed suppression, multi-species forage mixtures, polyculture, row spacing, intercropping
Citation: Bainard JD, Serajchi M, Bainard LD, Schellenberg MP and Lamb EG (2020) Impact of Diverse Annual Forage Mixtures on Weed Control in a Semiarid Environment. Front. Sustain. Food Syst. 4:92. doi: 10.3389/fsufs.2020.00092
Received: 16 February 2020; Accepted: 20 May 2020;
Published: 14 July 2020.
Edited by:
José Laércio Favarin, University of São Paulo, BrazilReviewed by:
Rafael Munhoz Pedroso, University of São Paulo, BrazilRakesh K. Upadhyay, United States Department of Agriculture (USDA), United States
Copyright © 2020 Her Majesty the Queen in Right of Canada, as represented by the Minister of Agriculture and Agri-Food Canada. This is an open-access article distributed under the terms of the Creative Commons Attribution License (CC BY). The use, distribution or reproduction in other forums is permitted, provided the original author(s) and the copyright owner(s) are credited and that the original publication in this journal is cited, in accordance with accepted academic practice. No use, distribution or reproduction is permitted which does not comply with these terms.
*Correspondence: Jillian D. Bainard, jillian.bainard@canada.ca