- 1USC 1339, Centre d'Etudes Biologiques de Chizé, INRAE, Villiers-en-Bois, France
- 2UMR7372 Centre d'Études Biologiques de Chizé, CNRS & La Rochelle Université, Beauvoir-sur-Niort, France
- 3LTSER Zone Atelier “Plaine & Val de Sèvre”, CNRS, Villiers-en-Bois, France
- 4UMR ECOSYS, Platform Biochem-Env, INRAE, AgroParisTech, Université Paris-Saclay, Versailles, France
- 5Université de Lorraine, INRAE, UMR Laboratoire Agronomie et Environnement, Vandœuvre les Nancy, France
The current challenge in agriculture is to move from intensively managed to multifunctional agricultural landscapes that can simultaneously provide multiple ecological functions (multifunctionality), thus ensuring the delivery of ecosystem services important for human well-being. There is evidence that biodiversity is the main driver of multiple ecosystem functions. However, how biodiversity, and which components of biodiversity are the sources of multifunctionality, remain elusive. In the present study, we explore the role of weed richness and weed abundance as possible sources of ecosystem multifunctionality of an intensive agricultural landscape. Weeds are a key component of the arable field ecosystem trophic network by supporting various ecological functions while being a possible threat for production. We combine empirical data on ten ecosystem functions related to pollination, pest control and soil fertility, and measured across 184 fields cultivated with winter cereal, oilseed rape or hays in the Long Term Socio-Ecological Research site Zone Atelier Plaine & Val de Sèvre. We found that weed diversity was a strong contributor to multifunctionality in all crop types, especially when using the threshold-based approach. The effects of weed diversity were less pronounced for individual ecological functions except for weed seed predation and urease activity. As weeds may have dual effects on yields, we also explored the relationship between ecosystem multifunctionality and yield considering weed abundance. We however found a neutral relationship between yield and ecosystem multifunctionality. These results suggest that field management that maintains high levels of weed diversity can enhance multifunctionality and most ecological functions. Understanding how to maintain weed diversity in agricultural landscapes can therefore help to design sustainable management favoring the delivery of multiple services while maintaining food production. The next challenge will therefore be to assess the relative contribution of management practices, landscape features and weed diversity on ecosystem multifunctionality and yield.
Introduction
Knowledge of how biodiversity contributes to ecosystem functioning at multiple scales is critical to conserving, managing and restoring multifunctional landscapes, especially because the capacity of ecosystem to maintain multiple processes has been related to stability of ecosystems (Huang et al., 2019). Biodiversity is one of the main drivers of ecosystem functioning, along with land use or soil conditions (Lavorel et al., 2011; Balvanera et al., 2014). In general, a positive asymptotic relationship between biodiversity and single ecosystem function has been found (Isbell et al., 2011; Liang et al., 2016), suggesting that a tiny fraction of the species pool is necessary to support an individual function (Slade et al., 2019). However, to maintain the entire set of functions and services simultaneously, far more species are required (Isbell et al., 2011; Sasaki et al., 2019). There is evidence that multidiversity, i.e., the diversity of a variety of taxa, enhances multifunctionality (Wang et al., 2019). Indeed, different species enhance ecosystem functioning during different years, at different places, for different functions and under different environmental contexts (Isbell et al., 2011). However, the source of multifunctionality, i.e., how biodiversity, and which components of biodiversity are the sources of multifunctionality, remain elusive.
Weeds are a key component of the trophic network in arable fields, supporting various functions such as pollination, pest control or soil fertility (Marshall et al., 2003; Nicholls and Altieri, 2013; Bretagnolle and Gaba, 2015). Multifunctionality is here defined as ecosystem function multifunctionality (Manning et al., 2018), which has been widely studied in grasslands (Sasaki et al., 2019; Wang et al., 2019), and more recently in other ecosystems, such as forests (Zavaleta et al., 2010; Xie et al., 2018; Huang et al., 2019), soils (Valencia et al., 2018), and farmland habitats (Rallings et al., 2019; see Hölting et al., 2019 for a review). Agricultural land represents ~40% of the Earth's land surface and therefore a better understanding of the drivers of multifunctionality as well as the relationship between multifunctionality and crop production may provide fill knowledge gaps to develop sustainable management strategies.
Species richness has been the main metric analyzed in regard to the role of biodiversity in multifunctionality (Huang et al., 2019). But in addition to species richness within communities, the functional properties of the most locally abundant species may drive ecosystem functioning following the mass–ratio hypothesis (Grime, 1998), or at least may provide a limited number of functions (Gamfeldt et al., 2008; Isbell et al., 2011). Locally rare species, alternatively, have been shown to be important diversity component for preserving high levels of ecosystem multifunctionality (Soliveres et al., 2016). Therefore, whether locally abundant species, locally rare species or both are required to maintain multifunctionality is still unclear. In addition, multifunctionality may be quantified by various methods [review in (Hölting et al., 2019)]: the most common consists in aggregating the multiple assessed functions into a single metric [>80% of studies reviewed in (Hölting et al., 2019)], most often by using the threshold approach (Gamfeldt et al., 2008) though the averaging approach accounts for 30% of studies.
In the present study, for the first time to our knowledge, we explore the role of weed diversity as a possible source of multifunctionality in an intensive agricultural landscape. To do so, we examine the relationship between weed diversity and ecosystem multifunctionality in 184 production fields cultivated with an arable crop or a hay in the Long Term Socio-Ecological Research site (LTSER) Zone Atelier Plaine & Val de Sèvre in South-West France. To understand how weed diversity may affect ecosystem multifunctionality, we explore the relationships between weed diversity and ecosystem multifunctionality using two different components of weed diversity (weed richness and weed total abundance) and two approaches to measure ecosystem multifunctionality (i.e., the averaging approach and the threshold approach). We also examine to which extent ecosystem multifunctionality is related to either locally abundant weed species or locally rare ones. In each field, biodiversity and various ecological functions [pollination, pest control (weed seed and aphid predation) and soil fertility (total organic carbon and enzyme activities involved in carbon, nitrogen, sulfur and phosphorus cycles)] were estimated to assess the effects of weed species diversity on ecosystem functioning. Finally, as crop production is the main service in agricultural landscapes, we investigate the relationship between crop production and ecosystem multifunctionality. Because a higher ecosystem multifunctionality suggests the provision of more ecological functions related to pest control, pollination and soil activities, we expect higher productivity in fields with higher ecosystem multifunctionality.
Materials and Methods
Study Site
The study site area, LTSER “Zone Atelier Plaine & Val de Sèvre” [hereafter ZA PVS; (Bretagnolle et al., 2018b)], covers 435 km2 of agricultural land in the south of the city of Niort, in the Deux-Sèvres department in the Nouvelle-Aquitaine Region, France. The most common crops are winter cereal (33.8%), maize (9.6%), sunflower (10.4%), winter oilseed rape (8.3%), and haylands (13.5%, including mainly forage temporary crops such as alfalfa and to a lesser extent permanent grasslands). The field size is 4.5 ha in average.
Biodiversity Surveys
Biodiversity (weeds, ground beetles and bees) was surveyed in 184 fields (i.e., the scale of management decisions in farmland) including 78 winter cereals (winter wheat, winter barley) and 45 winter oilseed rape fields, and 61 haylands (i.e., temporary grasslands used as forage crops). For convenience and because most of haylands are temporary crops (generally 2–5 years, mainly alfalfa), we refer as crop types when referring to these three types of cultivated plants. All fields sown with winter cereals and winter oilseed rape were tilled before sowing. Weed species identities and occurrences were recorded in 80, 0.25 m2 quadrat positioned in the core field along two transects of 10 quadrats after spring herbicide application (mid-April-end of May). Weed abundance was computed as the sum of each species occurrence in the 80 0.25 m2 quadrats in each field. Ground beetles (carabid) diversity and abundance were assessed using two pitfall traps placed in the margin (first crop row) and two others at 10 and 25 m from the edge. Bee diversity was monitored in the same fields using colored six pan traps per field left for 4 days in the fields. Figure 1 depicts the survey design. Details on all protocols are available in Bretagnolle et al. (2018a).
Estimation of Ecological Functions
Pollination by insects was measured using two oilseed rape phytometer plants (Brassica napus sp.), one placed in the field edge and one in the field core. Phytometers were grown in an insect-proof greenhouse in the lab. At flowering, they were left for 4 days in fields and then placed again in the insect-proof greenhouse in the lab. For each phytometer, we assessed the fruiting success of the flowers that were opened during the 4 days in the fields. Here, we used the average fruiting success per field to estimate pollination (Perrot et al., 2018).
Biological pest control was quantified in each field using two types of predation cards: cards with weed seeds (Viola arvensis) and cards with aphids (Acyrthosiphon pisum Harris.). Fourteen cards were placed during 4 days in the first 10 m in each field along two transects. Predation rates were estimated by dividing the number of seeds that were predated over the number of seeds on the cards.
Soil cores were sampled to quantify soil carbon and soil enzyme activities, between the end of March and early April 2016. Five soil cores were collected in the topsoil layers (0–15 or 0–30 cm depending on the soil depth, i.e., the total soil depth never exceeds 30 cm in the study area). Position of cores was random in the field. The core samples were pooled per field and stored at ambient temperature until the analyses were performed. Soil samples were air-dried for estimation of total organic carbon (C) content that was measured by dry combustion. Fresh soils were used to measure oil enzyme activities involved in carbon (C), nitrogen (N), sulfur (S), and phosphorus (P) cycles. Arylamidase, β-glucosidase, urease, arylsulfatase and phosphatase were quantified by the platform Biochem-Env (Cheviron et al., 2018) using colorimetric methods according to the ISO (ISO, 2018) standard, with a slight modification for urease. All measurements were performed at the soil pH, in an unbuffered soil water solution (Lessard et al., 2013), except arylamidase, performed in Tris 100 mM pH 7.5 (Acosta-Martínez and Tabatabai, 2000).
Yields and the amount and type of fertilizers applied during the cropping season were collected by means of farmers' interviews. Data were collected for 100 fields, because some farmers did not accept face-to-face interviews. From these surveys, we derived the amount of N input. The quantity of inorganic nitrogen used was directly calculated from the fertilizer composition and the quantity applied, and the quantity of nitrogen mineralized in organic fertilizers was calculated using the method described by Jeuffroy and Recous (1999).
The general statistics of biodiversity, ecological functions and agricultural practices are given in Supplementary Material, Table S1.
Statistical Analysis
We first compared, among crop types, the magnitude of each individual ecological function, the number of functions achieved above each threshold, and ecosystem multifunctionality (EF-multifunctionality; Manning et al., 2018) using ANOVA. We used EF multifunctionality (also referred to averaging approach) which summarizes ecosystem multifunctionality and reflects the change in the average level of a bundle of ecosystem functions (Barnes et al., 2014). EF-multifunctionality per field is the sum of the standardized values of all the ecosystem functions. To avoid an overweight of the bee richness, soil carbon content and enzyme activities, values of these functions were standardized to range between 0 and 1. Pollination and pest control estimates, being rates, already vary between 0 and 1, and were thus not standardized. We also evaluated whether multiple functions are simultaneously performing at high levels using the threshold approach (Byrnes et al., 2014). This approach consists in computing the number of functions achieved above a given threshold. We selected six thresholds which corresponds to the percentages (i.e., 20, 30, 40, 50, 60, and 70%) of the maximum observed value of each function (Byrnes et al., 2014). If the number of functions greater than a threshold is always lower than the total number of functions, this highlights a trade-off between functions i.e., one function is always maximized when another is minimized. For all tests, we checked for the prerequisites of homogeneity of variances using Bartlett test, normality using Shapiro test, and applied Kruskall–Wallis rank test when necessary. We used Tukey's post hoc tests to assess differences among crop types.
We then tested whether weeds (richness or abundance) would promote each individual ecological function, the number of functions achieved above each threshold, and EF-multifunctionality. We used ordinary least squares regression to determine how the various weed metrics influenced the dependent variables (each individual function, the number of functions achieved above each threshold, and EF-multifunctionality). We also included crop type and its interaction with weed richness or abundance. For pest control (aphid and seed predation rates), we also included ground beetle diversity and abundance as explanatory variables with, respectively, weed diversity and weed abundance. Two models per explanatory variable were built and compared to identify whether weed richness or abundance better explained each function, the number of functions achieved above each threshold and EF-multifunctionality. A model selection procedure based on Akaïke criterion (AIC) was performed to select the model that best explained the data (Burnham and Anderson, 2002).
To examine how EF-multifunctionality and each ecological functions were related to abundant vs. rare weed species, we built ten competing models (five with the number of abundant species and five with the number of rare species) and quantified the percentage of variance explained by the number of locally abundant species or of locally rare ones. The number of abundant species was arbitrary defined as the number of species over quantile 50, 60, 70, 80, and 90% of the distribution of the plant abundance per field whereas the number of rare species was in symmetry the number of species lower than quantile 50, 40, 30, 20, and 10% of that distribution. Models include crop type and its interaction with either the number of abundant species or the number of rare species.
Finally, we examined the relationship between EF-multifunctionality and productivity, i.e., crop yield in cereal and oilseed rape fields and aboveground biomass production in grasslands. We built a complete linear model with EF-multifunctionality, crop type, field area, weed abundance and the amount of N input (kg/ha) as covariate. We considered the amount of N input because it is usually positively related to productivity, and use field area to account for the discrepancy in the spatial coverage between productivity (measured at field scale) and ecosystem function (part of the field). To account for potential interactive effect between EF-multifunctionality on one hand and weed abundance, crop type and field area on the other hand, we included these three two-way interactions. Finally, because weed assemblies and the amount of N input vary among crops, we included the interaction between weed abundance and crop type, and between the mount of N input and crop type. Among the fields surveyed, 21 fields (8 winter cereals and 13 haylands) were managed as organic farming. Yields and weed abundances were standardized per crop type and farming system using z-scores. This transformation does not constrain the variability found in the raw data and allows focusing on each effect independently of the crop and farming system effects.
Analyses and plots were performed using packages MASS (Venables and Ripley, 2002) and ggplot2 (Wickham, 2009) on R v. 3.5.1 (R Core Team, 2018). R code are available in Supplementary Text.
Results
Multifunctionality in Haylands and Annual Crops
EF-multifunctionality was higher in haylands [average 4.79 (sd ± 1.13)] than in annual crops (winter cereal: 4.42 ± 0.83 and oilseed rape: 4.08 ± 1.03; Figure 2A), although not significantly. No significant differences in bee diversity and oilseed rape fruiting success (a proxy of insect pollination) were found among crop types (Figures 2D,E). In contrast, pest control was significantly higher in haylands (weed seed predation rate: 0.82 ± 0.13 and aphid predation rate: 0.73 ± 0.22), and to a lesser extent in winter cereal (0.61 ± 0.21 and 0.64 ± 0.2) than in oilseed rape (0.56 ± 0.22 and 0.45 ± 0.18) (Figures 2B,C). A similar pattern was observed when considering belowground ecological functions: soil carbon and soil enzyme activities related to C, N, P, and S cycles were significantly higher in haylands compared to annual crop fields and no differences were observed among annual crops (Figures 2F–K).
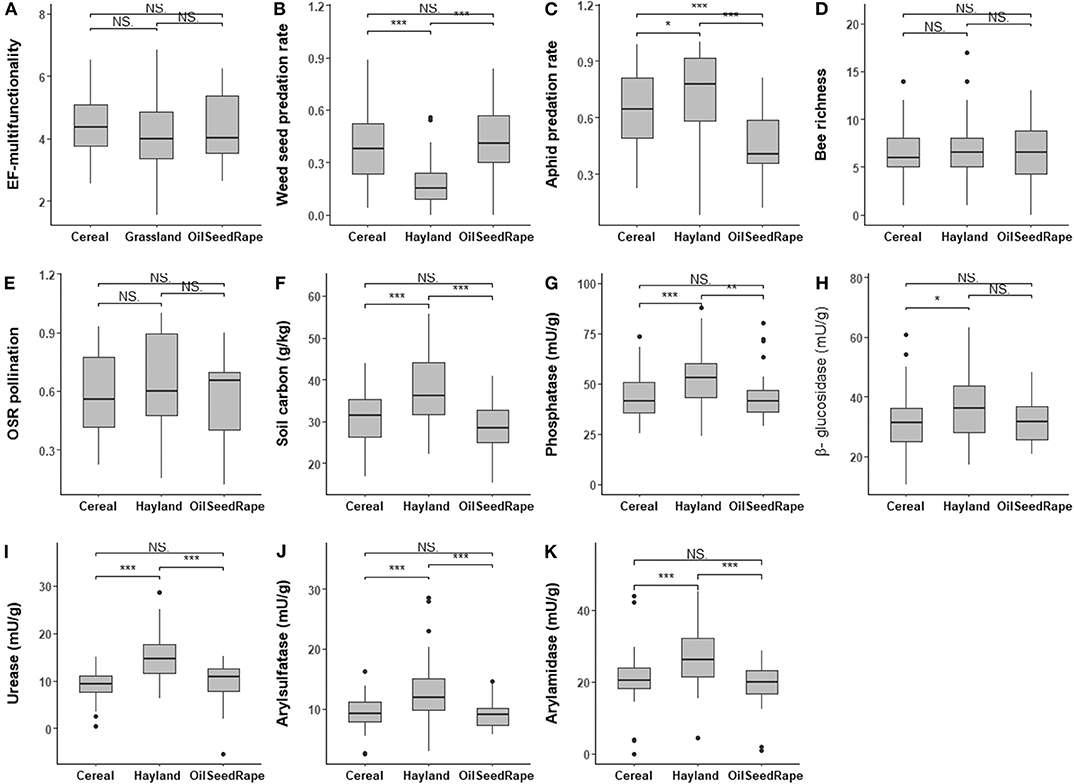
Figure 2. Among crop type variation of EF-multifunctionality (A) and each ecological function (B–K). Soil enzyme activities are expressed in mU.g−1 dry soil, representing nanomoles of product released per minute and per g of equivalent dry soil. Significant differences are indicated by *(P < 0.05), **(P < 0.01), and ***(P < 0.001). NS indicates a non-significant effect.
Weed Abundance Rather Than Weed Diversity Sustains Ecological Functioning
Weed richness and abundance significantly varied among crop types with highest richness and abundance in hay (21.5 ± 9.8 species and 308 ± 128 plants/m2) than in winter cereal (12.3 ± 9.75 species and 153 ± 176 plants/m2) and in oilseed rape (16.6 ± 4.26 species and 153 ± 83 plants/m2) fields, a difference that could not be accounted for by sown species in hay (e.g., alfalfa, ray-grass, Festuca spp.) that were withdrawn from analyses. The most common species were Epilobium tetragonum, Cirsium arvense and Poa pratensis in winter cereal, Epilobium tetragonum, Lolium multiflorum and Trifolium pratense in oilseed rape and Dactylis glomerata, Poa trivialis and Rubus fruticosus in haylands. The threshold-based approach to multifunctionality revealed strong positive effects of weed abundance whatever the crop type (Figure 3A), especially for thresholds higher than quantile 30 (Figure 3B; Table 1B). Weed abundance was also a strong contributor to aboveground EF-multifunctionality (i.e., considering pest control, bee diversity, and pollination success; Table 1B). EF-multifunctionality tended to increase with both weed abundance and richness in all crop types (Figure 3A; Table 1). The effects of weed richness on multifunctionality thresholds and aboveground EF-multifunctionality were less pronounced than those of weed abundance (Supplementary Table S1) and varied among crop types being positive in winter cereal and oilseed rape and slightly negative in hay (Supplementary Figure 1).
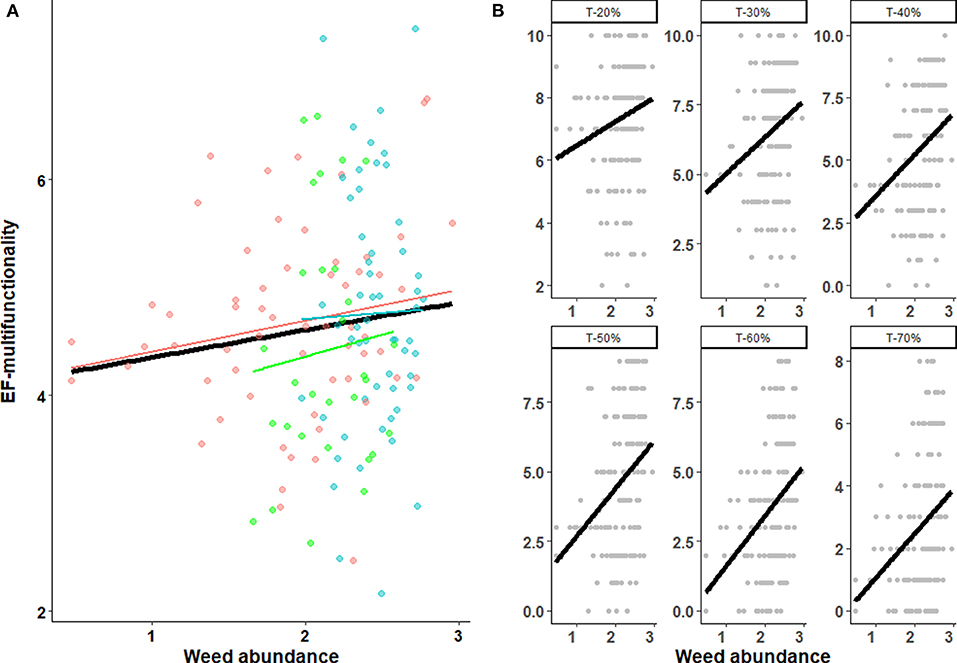
Figure 3. Weed abundance effects on EF-multifunctionality (A) and across functional thresholds (B) measured as the number of functions achieved in each field above thresholds (T), where T is the quantile-based ranking of each function across all fields. EF-multifunctionality was computed using the averaging approach. Lines represent the predicted relationship from the statistical models and shade areas the 95% confidence interval. Colors indicate the different crop type (cereals in red, oilseed rape in green and haylands in blue). Weed abundance is log-transformed.
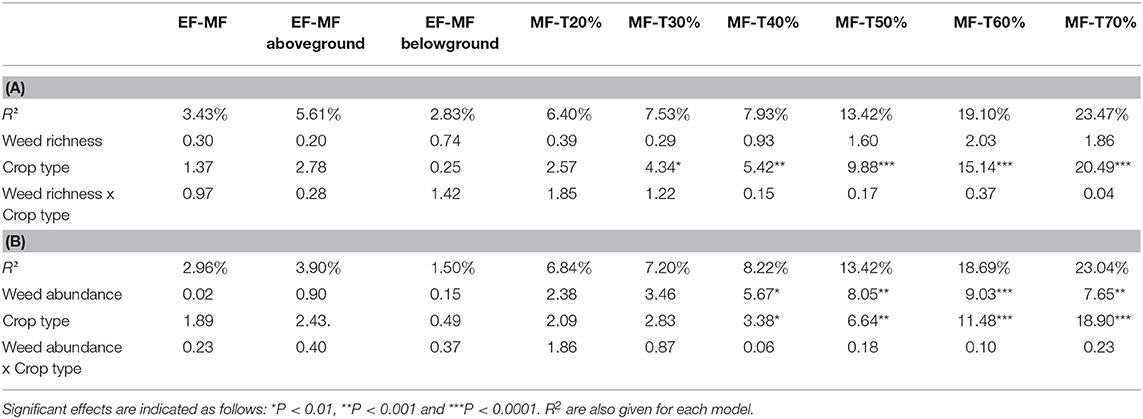
Table 1. F-Statistics for the effects of weed richness (A) or weed abundance (B), crop type and their interaction on ecosystem multifunctionality computed as the sum of all standardized ecological functions (EF-MF), aboveground ecological functions (EF-MF aboveground), belowground ecological functions (EF-MF belowground) and using a threshold approach (with threshold from 20 to 70%).
When considering each individual function, only slight differences in the goodness-of-fits were found between weed abundance or weed richness except for weed predation rate and urease activity (Supplementary Table S2). Weed abundance strongly and positively influenced aphid predation rate and soil enzyme activities (arylsulfatase, β-glucosidase, and urease), while weed richness affected weed seed predation rate and arylamidase activity (Table 2). Weed diversity had no significant effects on bee diversity, oilseed rape fruiting success, and phosphatase activities. However, relationships between weed abundance or richness and each individual function varied strongly among crop types and between functions (Figure 4). In haylands, weed diversity (either abundance or richness) had a negative effect on soil carbon, and most enzyme activities, and a positive one on aphid and weed seed predation rates. In winter cereal fields, weed richness had a significant negative effect on weed seed predation and soil carbon. Finally, in oilseed rape, weed diversity generally had a positive effect on weed seed predation rate, soil carbon and functions related to soil activity, and a negative one on aphid predation (Figure 4).
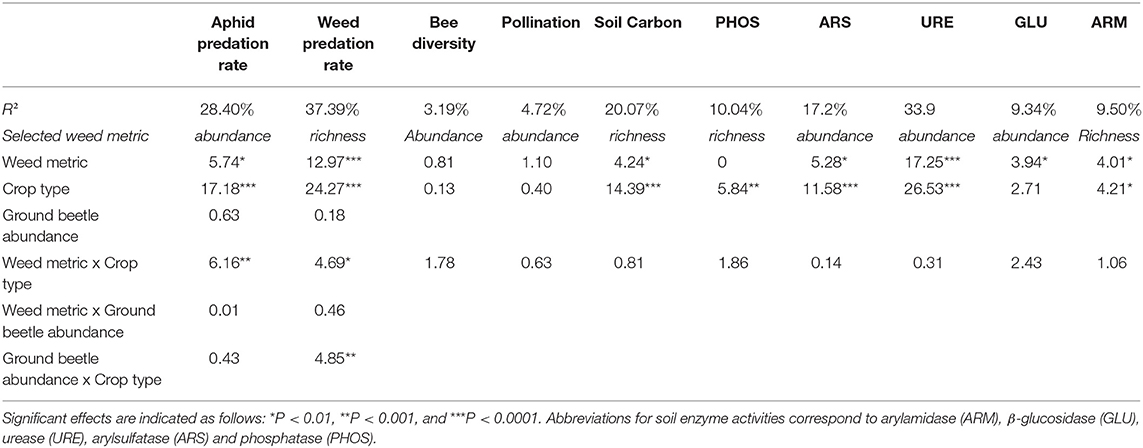
Table 2. F-Statistics for the effects of weeds (abundance or richness) and crop type on each individual function.
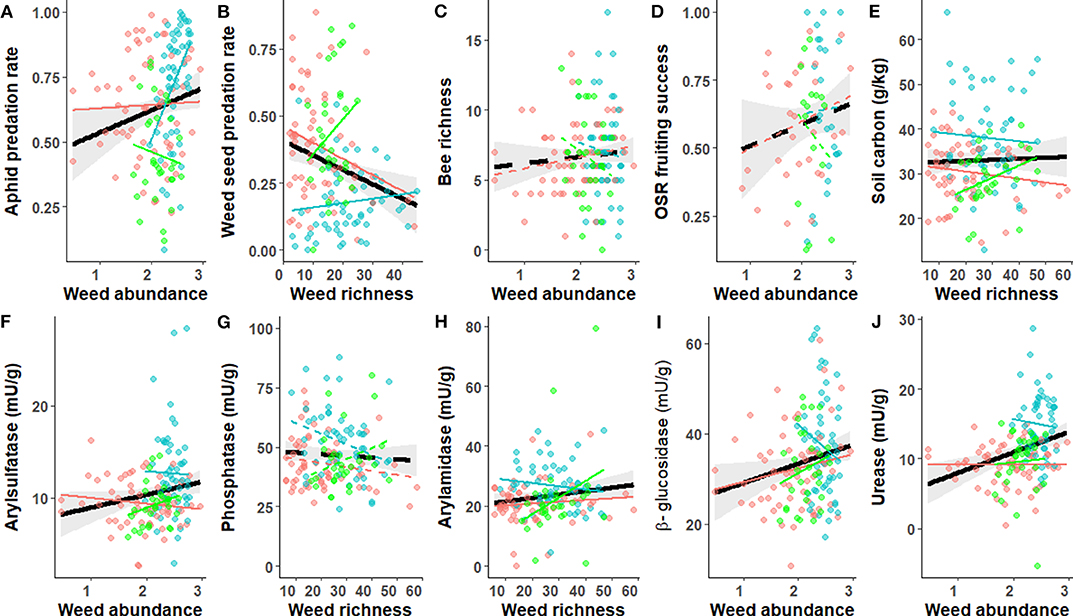
Figure 4. Weed abundance or richness effect on each ecological function in winter cereals (red), oilseed rape (green) and haylands (blue). Ecological functions are: aphid predation rate (A), weed seed predation rate (B), bee richness (C), OSR fruiting success (D), soil carbon (E), arylsulfatase (F), phosphatase (G), arylamidase (H), β-glucosidase (I) and urease (J). Lines represent the predicted relationship from the statistical models. The black line shows the relationship across crop types and shade area the 95% confidence interval. Dotted lines indicate non-significant relationships. Weed abundance is log-transformed. Soil enzyme activities are expressed in mU.g−1 dry soil, representing nanomoles of product released per minute and per g of equivalent dry soil.
The Contribution of Rare Weed Species to Multifunctionality
The relative contributions of rare vs. abundant species to multifunctionality or the single functions, varied with the quantile considered to classify the species. The highest explanatory power of EF- multifunctionality variance (i.e., % of variance explained) was observed when the number of rare and abundant species was defined based on respective quantiles 40 and 60% (Supplementary Figure 2). While we observed a higher contribution of rare weed species to EF-multifunctionality (35.2% of variance explained) than of abundant ones (30.1%; Figure 5). This suggests the importance of the threshold used to define species groups on the results. Among the 4.15 (±3.78) rare weed species, the most frequent species were Medicago lupulina, Galium aparine and Vicia sativa in winter cereal, Galium aparine, Helminthotheca echioides, Geranium rotundifolium in oilseed rape and Anthriscus caucalis, Achillea millefolium, and Geranium pusillum in haylands. The provision of aboveground ecological functions was also related to the rare weed species, although the difference was relatively low. An exception was observed for bee diversity which was strongly explained by abundant weed species. Surprisingly, whatever the threshold for defining rare and abundant species, neither abundant nor rare weed species had high explanatory power for belowground ecological functions (soil carbon content and the enzyme activities); the differences was mostly explained by crop types.
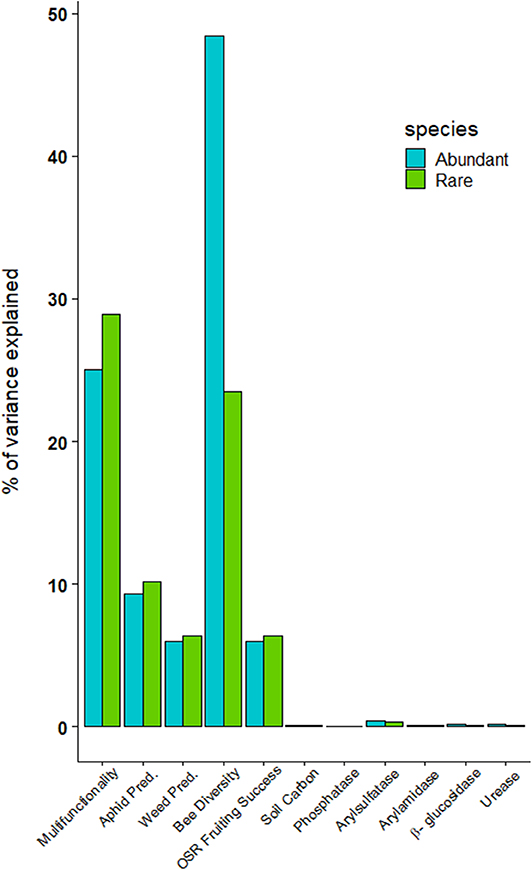
Figure 5. Percentage of variance on EF-multifunctionality and each ecological functions explained by the number of locally abundant (Blue) and rare (Green) weed species which were defined using the number of species with an plant abundance <60% quantile and lower than 40% quantile of the weed abundance distribution.
Neutral Relationship Between Productivity and Multifunctionality
Contrary to our expectation, productivity (yield in annual crops and biomass production in haylands) was not higher in fields with high EF-multifunctionality. Rather, we observed a neutral relationship between EF-multifunctionality and productivity (Figure 6A; Table 3). The amount of N, crop type and field area did not affect productivity either. But most importantly, we did not find any significant effect of weed abundance on productivity (Figure 6B; Table 3). Overall, the variables selected for the productivity model (Table 3) resulted in a model with a low explanatory power (R2 = 22.07%, P = 0.098).
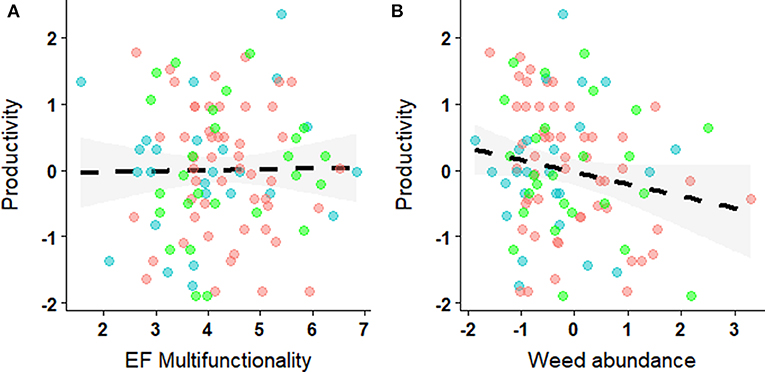
Figure 6. Relationship between productivity (standardized) and (A) EF-multifunctionality or (B) weed abundance. Colors indicate the different crop type (cereals in red, oilseed rape in green and haylands in blue) and the shade area the 95% confidence interval.
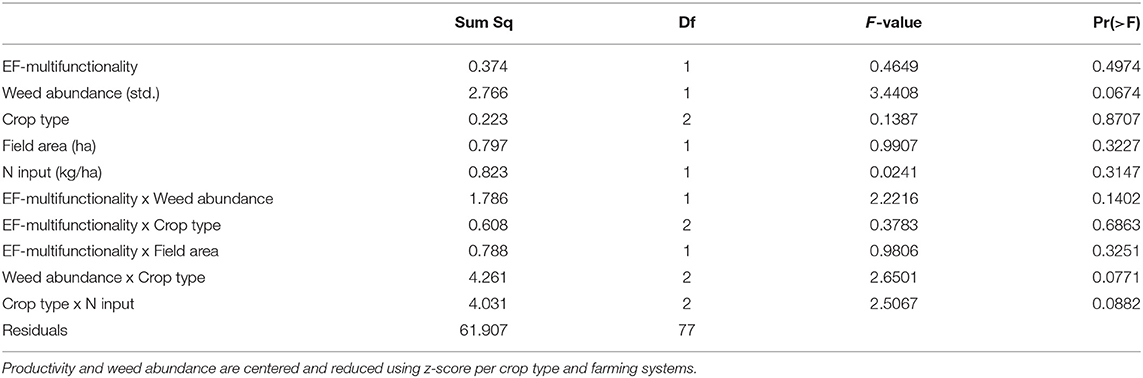
Table 3. Statistics for the effects of EF-multifunctionality, crop type, amount of N input, weed abundance, field area and the interaction between EF-multifunctionality and crop type EF-multifunctionality and weed abundance, EF-multifunctionality and field area, as well as the interaction between weed abundance and field area and crop type and N input on productivity (yields in cereal and oilseed rape fields and aboveground biomass production in haylands).
Discussion
In this study, we quantified the role of weeds as a potential source of multifunctionality in real farming conditions in an intensive agricultural landscape. The relationship between weed abundance or diversity, and ecosystem multifunctionality was evaluated in three crop types, cereal, oilseed rape, and hay. We considered above- and below-ground functions related to regulation services. The magnitude of ecosystem multifunctionality and of most individual ecosystem functions were higher in haylands than in annual crop fields. We found that weed diversity was a strong contributor of ecosystem multifunctionality especially when considering the simultaneous delivery of functions (threshold approach) and when considering only aboveground ecological functions. Weed diversity had also an effect in the provision of most the individual ecosystem functions under study here, with the strongest effect observed on weed seed predation and urease activity. Interestingly, across crop types, rare species had a higher contribution to ecosystem multifunctionality than abundant weed species at a given threshold (i.e., % quantile) used to defined the species groups. Finally, we found that contrary to our expectation, fields with higher ecosystem multifunctionality did not show higher productivity in terms of yield or biomass. Rather, we found a neutral relationship between productivity and ecosystem multifunctionality.
Our finding that biodiversity correlates with ecosystem multifunctionality extends past work showing a positive relationship between plant diversity and ecosystem multifunctionality, especially in experimental studies where such positive relationship has been repeatedly found (Wardle, 2016). However, in naturally assembled communities, as the ones studied here, there is much less evidence and the picture is less clear (Allan et al., 2015; Soliveres et al., 2016; Pennekamp et al., 2018). Our results show that the positive relationship between weed diversity and ecosystem multifunctionality was even stronger when considering aboveground ecological functions. High weed diversity contributed to the regulation of pests by increasing weed seed and aphid predation rates. Fields with high weed diversity may shelter more pest natural enemies. Indeed, predator and parasitoïd species richness are generally positively related to plant species diversity (Scherber et al., 2010; Leles et al., 2017; Schuldt et al., 2019). More pest natural enemies may enhance pest regulation by improving predator (parasitoïd)-prey interactions (Letourneau et al., 2009). Such increase may also result from a higher pest mortality, or through a synergistic interaction between predators in their ability to suppress pest populations, with a mortality greater than the summed mortality caused by each natural enemy species on its own (Barbosa and Castellanos, 2005). Evidence of synergistic interaction has indeed been found between foliar-foraging and ground-foraging pest natural enemies in the suppression of pea aphid populations in alfalfa (Losey and Denno, 1998). In our study, we only sampled ground beetles and found that their richness and abundance increased with weed diversity (Supplementary Figure 3). Ground beetles have been suggested to be natural enemies of both aphids (Firlej et al., 2013) and weed seeds (Bohan et al., 2011), the consumption rate of the latter being variable with both ground beetle and weed species (Gaba et al., 2019). Determining whether the increase of weed diversity promotes a higher diversity of pest natural enemies and whether this higher diversity results in synergistic or additive effects among these natural enemies should be investigated in future research through a trophic network approach allowing the quantification of the intensity of predation rate per guild of natural enemies.
Surprisingly, although a high percentage of variance in bee richness was explained by rare or abundance weeds, neither a higher weed richness nor a higher weed abundance promoted bee richness or improved fruiting success of OSR phytometers. A positive relationship was expected between weed diversity and bee richness, since weeds are important resource for insect pollinators (Rollin et al., 2013; Requier et al., 2015). Higher plant species richness should enhance pollinator richness because of plant species specific pollinator preferences and a greater temporal and spatial availability of pollen and nectar resources (Potts et al., 2003; Fontaine et al., 2006; Ebeling et al., 2008). Higher weed abundance should also increase bee richness by providing a higher resource abundance and increasing bee attractiveness (Papanikolaou et al., 2017). Rather we found neutral relationships between weed richness or abundance and the number of bee species and OSR fruiting success whatever the crop type. In oilseed rape fields, bee monitoring was performed during oilseed rape flowering period (from April to May 2016). Oilseed rape is a mass-flowering crop offering a resource bloom during its flowering period (Requier et al., 2015), and weed floral resource may be less attractive for bees at this period. A different mechanism may result in neutral relationship in cereals and hay fields: the increase in the number of species of plants may not be sufficient to attract bees because such crops do not provide floral resource either in quantity, quality, or both. In our study, we however found that the number of abundant weed species explained a high proportion of bee diversity (>50, Figure 5). Weeds differ in their melliferous potential (Baude et al., 2016; Ion et al., 2019). Consequently, a functional approach using traits related to pollination (e.g., flower resource), pest control (e.g., seed energetic content) and soil activities functions [root traits; but see Gaba et al. (2017) for other examples] may be useful to go deeper in the relationships. This more comprehensive and quantitative assessment would require to compile existing databases on traits because there is currently a lack of data for many functional traits especially for rare species (but see Bourgeois et al., 2019).
Similarly, to bee richness, we failed to find a positive relationship between weed richness or abundance, and fruiting success of OSR phytometers. Such relation would have suggested an indirect positive effect of weed richness or abundance through an increase of bee richness. Actually very few studies investigated the relationship between plant diversity and pollination success [only 8 according to van der Plas (2019)], but all found a neutral relationship, as here. (van der Plas, 2019) suggests that this absence of relationship may reflect a more important effect of pollinator diversity for pollination success than the one of plant diversity. We found a negative relationship between bee richness and OSR fruiting success (Supplementary Figure 4). In our study area, a previous study revealed that honeybee and Lasioglossum sp. abundances increased OSR fruiting success in oilseed rape fields (Perrot et al., 2018). The use of phytometers here to estimate OSR fruiting success may explained this discrepancy. Honeybees are assumed to be strong competitor, and exploitative competition between honeybees and wild bees was recently evidenced (Henry and Rodet, 2018), though relationship between honeybees and wild bees were found to vary from being positive, negative or neutral, depending on the plant species (Nielsen et al., 2012). Such relationships further affect pollinator community structure, resulting in a distortion in plant-pollinator interactions (Diekötter et al., 2010). In our study, we could not explore the link between weed diversity, OSR fruiting success, bee richness and honeybee abundance because bee richness was estimated using pan traps which poorly estimate honeybee abundance (Westphal et al., 2008). Further research therefore needs the use of complementary methods, for instance pan traps and sweep nets (Westphal et al., 2008), to assess bee diversity and hence disentangle the complex interactions between weeds, bees, and pollination in agricultural landscapes [but see (Bretagnolle and Gaba, 2015) for a conceptual framework].
The storage of organic carbon in soils represents a key function of soils that is critical in mitigating climate change effects (Paustian et al., 2016). Positive relationships between plant diversity and organic soil carbon stocks have been shown in experimental studies (Cardinale et al., 2012) and in most of natural communities (Gamfeldt et al., 2013; Maestre et al., 2016) although some studies found negative or neutral relationships (van der Plas, 2019). In our study, aboveground ecological functions appeared to be affected by the composition and structure of weed assemblies, but not by individual species as locally abundance and rare species poorly explained their variations. Weed richness and abundance equally explained the variation in soil carbon contents in the three crop types. Higher soil carbon content in more rich and abundant weed communities may influence soil microbial activity. This is supported by the positive relationships between weed abundance and the activity of β-glucosidase and urease, those enzymes being involved in C (β-glucosidase) and N (urease) cycles. In contrast, the activity of phosphatase (an enzyme involved in P cycle) was not affected by weed diversity. These results suggest that different mechanisms affect soil enzyme activities. Higher activity of β-glucosidase in abundant weed communities suggests greater availability of cellulose for soil microorganisms in high abundance weed communities. These communities may produce more root biomass resulting in a higher root exudation (Paterson et al., 2007) which is known to affect the activity and composition of soil microbial community (Shahzad et al., 2015) resulting in an increase of soil carbon uptake (You et al., 2014). Weed abundance may also increase enzyme activities by providing a greater amount of enzyme substrate in soil (Geisseler and Horwath, 2009).
A high number of studies has investigated the relationship between multifunctionality and productivity (i.e., biomass production and yield), mostly in experimental conditions (Wardle, 2016). While positive relationships are often expected, we were not able to detect such positive effect of ecosystem multifunctionality and productivity whatever the crop type. In contrast, our analysis revealed a neutral relationship between ecosystem multifunctionality and productivity. Various parameters affect cereal and OSR yield and biomass production in haylands. Although we included nitrogen input, we may have missed other important variables such as soil properties (i.e., organic matter) or pest control management. Indeed, in this study site, a recent study showed that agrochemical applications overall accounted for about 24% of the variance of the OSR yield (Catarino et al., 2019). The neutral relationship may also arise from a compensation between benefits to yield provided by ecological functions sustained by weeds such as pest control, and competition between crop and weeds resulting in yield loss. Indeed, although we detected a negative relationship between weed abundance on productivity, this was not significant. Ecosystem multifunctionality was assessed using the averaging approach. Similar results were obtained with the threshold approach (data not shown). In both approaches, ecological functions had similar weights on productivity. However, it is likely that the different ecological functions benefit differentially to productivity (both in strength and type of relation e.g., linear, saturated). An assessment of ecosystem multifunctionality in which ecological functions are weighted according to their benefit to productivity may give a better picture of the relation [but see Allan et al. (2015) for an example in hay]. However, this requires a deeper understanding of the relationship between each ecological functions and productivity, and of the interactive effects of a suite of ecological functions on productivity. Exploring the effects of specific ecological functions while considering the others (i.e., taking account the complex interaction), for instance using structural equation modeling, could be relevant way to assess the effect of ecosystem multifunctionality on productivity. This would also allow taking account environmental factors (e.g., soil properties) and management practices.
Finally, our study emphasizes the critical role of haylands, and presumably grasslands in general, for maintaining ecosystem multifunctionality in agricultural landscape. Ecosystem multifunctionality was higher in haylands than in winter cereal and oilseed rape fields. Except for bee richness and fruiting success of OSR phytometers, the amount of ecological functions was greater in haylands. Haylands showed higher plant diversity than arable crop fields, and are less intensively managed fields, with no tillage and a low agrochemical inputs. There is strong evidence that intensive management has substantially altered biodiversity and affected ecosystem. The next challenge will be to assess the relative contribution of management practices and plant diversity (both being closely related) on ecosystem multifunctionality and productivity, to understand how diversifying agroecosystems by introducing meadows in landscapes and in crop rotation sequences could efficiently improve their functioning and sustain both the provisioning of ecological functions and yields.
Data Availability Statement
The datasets generated for this study are available on request to the corresponding author.
Author Contributions
SG and VB designed the study. NC and SP conducted the soil and enzyme activities analyses. TP and J-LG performed the phytometers pollination experiments. SG prepared the data and conducted the statistical analysis and wrote the first draft of the manuscript. All authors substantially contributed to revisions.
Funding
This paper was produced with the support of CESAB-FRB as part of the activities of the DISCO-WEED Working Group. The project was also supported by the French Ministry of Ecology (project 2017-2020 Pollinisateurs), the INRAE MP ECOSERV (BIOSERV project) and the ANR IMAGHO project (ANR-18-CE32-0002). Biochem-Env is a service of the Investment d'Avenir infrastructure AnaEE-France, overseen by the French National Research Agency (ANR) (ANR-11-INBS-0001).
Conflict of Interest
The authors declare that the research was conducted in the absence of any commercial or financial relationships that could be construed as a potential conflict of interest.
Acknowledgments
We would like to express our thanks to all field workers for their help with weed surveys, insect identification, pollination experiment and soil sampling. We sincerely thank the farmers of the LTSER Zone Atelier Plaine & Val de Sèvre for their involvement in our research programs. The authors are also grateful to C. Mougin and F. Martin for comments on the final version of the manuscript. We also wish to thank the editor and the two reviewers for valuable comments throughout the reviewing process.
Supplementary Material
The Supplementary Material for this article can be found online at: https://www.frontiersin.org/articles/10.3389/fsufs.2020.00071/full#supplementary-material
References
Acosta-Martínez, V., and Tabatabai, M. A. (2000). Arylamidase activity of soils. Soil Sci. Soc. Am. J. 64, 215–221. doi: 10.2136/sssaj2000.641215x
Allan, E., Manning, P., Alt, F., Binkenstein, J., Blaser, S., Blüthgen, N., et al. (2015). Land use intensification alters ecosystem multifunctionality via loss of biodiversity and changes to functional composition. Ecol. Lett. 18, 834–843. doi: 10.1111/ele.12469
Balvanera, P., Siddique, I., Dee, L., Paquette, A., Isbell, F., Gonzalez, A., et al. (2014). Linking biodiversity and ecosystem services: current uncertainties and the necessary next steps. Bioscience. 64, 49–57. doi: 10.1093/biosci/bit003
Barbosa, P., and Castellanos, I. (2005). Ecology of Predator-Prey Interactions. New York, NY: Oxford University Press.
Barnes, A. D., Jochum, M., Mumme, S., Haneda, N. F., Farajallah, A., Widarto, T. H., et al. (2014). Consequences of tropical land use for multitrophic biodiversity and ecosystem functioning. Nat. Comm. 5, 1–7. doi: 10.1038/ncomms6351
Baude, M., Kunin, W. E., Boatman, N. D., Conyers, S., Davies, N., Gillespie, M. A. K., et al. (2016). Historical nectar assessment reveals the fall and rise of floral resources in Britain. Nature 530, 85–88. doi: 10.1038/nature16532
Bohan, D. A., Boursault, A., Brooks, D. R., and Petit, S. (2011). National-scale regulation of the weed seedbank by carabid predators. J. Appl. Ecol. 48, 888–898. doi: 10.1111/j.1365-2664.2011.02008.x
Bourgeois, B., Munoz, F., Fried, G., Mahaut, L., Armengot, L., Denelle, P., et al. (2019). What makes a weed a weed? A large scale evaluation of arable weeds through a functional lens. Am. J. Botany 106, 90–100. doi: 10.1002/ajb2.1213
Bretagnolle, V., Berthet, E., Gross, N., Gauffre, B., Plumejeaud, C., Houte, S., et al. (2018a). Towards sustainable and multifunctional agriculture in farmland landscapes: lessons from the integrative approach of a French LTSER platform. Sci. Total Environ. 627, 822–834. doi: 10.1016/j.scitotenv.2018.01.142
Bretagnolle, V., Berthet, E., Gross, N., Gauffre, B., Plumejeaud, C., Houte, S., et al. (2018b). Description of long-term monitoring of farmland biodiversity in a LTSER. Data Brief 19, 1310–1313. doi: 10.1016/j.dib.2018.05.028
Bretagnolle, V., and Gaba, S. (2015). Weeds for bees? a review. Agron. Sustain. Dev. 35, 891–909. doi: 10.1007/s13593-015-0302-5
Burnham, K. P., and Anderson, D. R. (2002). “Model selection and multimodel inference,” in A Practical Information-Theoretic Approach, 2nd Edn. eds. D. Anderson, K. P. Burnham (Springer-Verlag, Heidelberg) doi: 10.1016/j.ecolmodel.2003.11.004
Byrnes, J. E. K., Gamfeldt, L., Isbell, F., Lefcheck, J. S., Griffin, J. N., Hector, A., et al. (2014). Investigating the relationship between biodiversity and ecosystem multifunctionality: challenges and solutions. Methods Ecol. Evol. 5, 111–124. doi: 10.1111/2041-210X.12143
Cardinale, B. J., Duffy, J. E., Gonzalez, A., Hooper, D. U., Perrings, C., Venail, P., et al. (2012). Biodiversity loss and its impact on humanity. Nature 486, 59–67. doi: 10.1038/nature11148
Catarino, R., Bretagnolle, V., Perrot, T., Vialloux, F., and Gaba, S. (2019). Bee pollination outperforms pesticides for oilseed crop production and profitability. Proc. R. Soc. B. 286:20191550. doi: 10.1098/rspb.2019.1550
Cheviron, N., Grondin, V., and Mougin, C. (2018). Biochem-Env: a platform of biochemistry for research in environmental and agricultural sciences. Environ. Sci. Pollut. Res. 25, 6154–6157. doi: 10.1007/s11356-017-8973-x
Diekötter, T., Kadoya, T., Peter, F., Wolters, V., and Jauker, F. (2010). Oilseed rape crops distort plant-pollinator interactions. J. Appl. Ecol. 47, 209–214. doi: 10.1111/j.1365-2664.2009.01759.x
Ebeling, A., Klein, A. M., Schumacher, J., Weisser, W. W., and Tscharntke, T. (2008). How does plant richness affect pollinator richness and temporal stability of flower visits? Oikos 117, 1808–1815. doi: 10.1111/j.1600-0706.2008.16819.x
Firlej, A., Doyon, J., Harwood, J. D., and Brodeur, J. (2013). A multi-approach study to delineate interactions between carabid beetles and soybean aphids. Environ. Entomol. 42, 89–96. doi: 10.1603/en11303
Fontaine, C., Dajoz, I., Meriguet, J., and Loreau, M. (2006). Functional diversity of plant-pollinator interaction webs enhances the persistence of plant communities. PLoS Biol. 4, 0129–0135. doi: 10.1371/journal.pbio.0040001
Gaba, S., Deroulers, P., Bretagnolle, F., and Bretagnolle, V. (2019). Lipid content drives weed seed consumption by ground beetles (Coleopterea, Carabidae) within the smallest seeds. Weed Res. 59, 170–179. doi: 10.1111/wre.12354
Gaba, S., Perronne, R., Fried, G., Gardarin, A., Bretagnolle, F., Biju-Duval, L., et al. (2017). Response and effect traits of arable weeds in agro-ecosystems: a review of current knowledge. Weed Res. 57, 123–147. doi: 10.1111/wre.12245
Gamfeldt, L., Hillebrand, H., and Jonsson, P. R. (2008). Multiple functions increase the importance of biodiversity for overall ecosystem functioning. Ecology 89, 1223–1231. doi: 10.1890/07-1861.1
Gamfeldt, L., Snäll, T., Bagchi, R., Jonsson, M., Gustafsson, L., Kjellander, P., et al. (2013). Higher levels of multiple ecosystem services are found in forests with more tree species. Nat. Comm. 4, 1–8. doi: 10.1038/ncomms2328
Geisseler, D., and Horwath, W. R. (2009). Short-term dynamics of soil carbon, microbial biomass, and soil enzyme activities as compared to longer-term effects of tillage in irrigated row crops. Biol. Fertil. Soils 46, 65–72. doi: 10.1007/s00374-009-0400-0
Grime, J. P. (1998). Benefits of plant diversity to ecosystems: immediate, filter and founder effects. J. Ecol. 86, 902–910. doi: 10.1046/j.1365-2745.1998.00306.x
Henry, M., and Rodet, G. (2018). Controlling the impact of the managed honeybee on wild bees in protected areas. Sci. Rep. 8:9308. doi: 10.1038/s41598-018-27591-y
Hölting, L., Beckmann, M., Volk, M., and Cord, A. F. (2019). Multifunctionality assessments – More than assessing multiple ecosystem functions and services? A quantitative literature review. Ecol. Indic. 103, 226–235. doi: 10.1016/j.ecolind.2019.04.009
Huang, X., Su, J., Li, S., Liu, W., and Lang, X. (2019). Functional diversity drives ecosystem multifunctionality in a pinus yunnanensis natural secondary forest. Sci. Rep. 9:6979. doi: 10.1038/s41598-019-43475-1
Ion, N., Odoux, J.-F., and Vaissière, B. E. (2019). Melliferous potential of weedy herbaceous plants in crop fields of romania from 1949 to 2012. J. Apic. Sci. 62, 149–165. doi: 10.2478/jas-2018-0017
Isbell, F., Calcagno, V., Hector, A., Connolly, J., Harpole, W. S., Reich, P. B., et al. (2011). High plant diversity is needed to maintain ecosystem services. Nature 477, 199–202. doi: 10.1038/nature10282
ISO (2018). Soil Quality — Measurement of Enzyme Activity Patterns in Soil Samples Using Fluorogenic Substrates in Micro-Well Plates. (ISO), 13.
Jeuffroy, M. H., and Recous, S. (1999). Azodyn: a simple model simulating the date of nitrogen deficiency for decision support in wheat fertilization. Eur. J. Agron. 10, 129–144. doi: 10.1016/S1161-0301(98)00059-8
Lavorel, S., Grigulis, K., Lamarque, P., Colace, M. P., Garden, D., Girel, J., et al. (2011). Using plant functional traits to understand the landscape distribution of multiple ecosystem services. J. Ecol. 99, 135–147. doi: 10.1111/j.1365-2745.2010.01753.x
Leles, B., Xiao, X., Pasion, B. O., Nakamura, A., and Tomlinson, K. W. (2017). Does plant diversity increase top–down control of herbivorous insects in tropical forest? Oikos 126, 1142–1149. doi: 10.1111/oik.03562
Lessard, I., Renella, G., Sauvé, S., and Deschênes, L. (2013). Metal toxicity assessment in soils using enzymatic activity: can water be used as a surrogate buffer? Soil Biol. Biochem. 57, 256–263. doi: 10.1016/j.soilbio.2012.09.009
Letourneau, D. K., Jedlicka, J. A., Bothwell, S. G., and Moreno, C. R. (2009). Effects of natural enemy biodiversity on the suppression of arthropod herbivores in terrestrial ecosystems. Annu. Rev. Ecol. Evol. Syst. 40, 573–592. doi: 10.1146/annurev.ecolsys.110308.120320
Liang, J., Crowther, T. W., Picard, N., Wiser, S., Zhou, M., Alberti, G., et al. (2016). Positive biodiversity-productivity relationship predominant in global forests. Science 354:aaf8957. doi: 10.1126/science.aaf8957
Losey, J. E., and Denno, R. F. (1998). International association for ecology interspecific variation in the escape responses of aphids : effect on risk of predation from foliar-foraging and ground-foraging predators published by : springer in cooperation with international association for ecol. Oecologia 115, 245–252.
Maestre, F. T., Eldridge, D. J., Soliveres, S., Kéfi, S., Delgado-Baquerizo, M., Bowker, M. A., et al. (2016). Structure and functioning of dryland ecosystems in a changing world. Annu. Rev. Ecol. Evol. Syst. 47, 215–237. doi: 10.1146/annurev-ecolsys-121415-032311
Manning, P., Van Der Plas, F., Soliveres, S., Allan, E., Maestre, F. T., Mace, G., et al. (2018). Redefining ecosystem multifunctionality. Nat. Ecol. Evol. 2, 427–436. doi: 10.1038/s41559-017-0461-7
Marshall, E. J. P., Brown, V. K., Boatman, N. D., Lutman, P. J. W., Squire, G. R., and Ward, L. K. (2003). The role of weeds in supporting biological diversity within crop fields. Weed Res. 43, 77–89. doi: 10.1046/j.1365-3180.2003.00326.x
Nicholls, C. I., and Altieri, M. A. (2013). Plant biodiversity enhances bees and other insect pollinators in agroecosystems. A review. Agron. Sustain. Dev. 33, 257–274. doi: 10.1007/s13593-012-0092-y
Nielsen, A., Dauber, J., Kunin, W. E., Lamborn, E., Jauker, B., Moora, M., et al. (2012). Pollinator community responses to the spatial population structure of wild plants : a pan-European approach. Basic Appl. Ecol. 13, 489–499. doi: 10.1016/j.baae.2012.08.008
Papanikolaou, A. D., Kuhn, I., Frenzel, M., Kuhlmann, M., Poschlod, P., Potts, S. G., et al. (2017). Wild bee and floral diversity co-vary in response to the direct and indirect impacts of land use. Ecosphere 8:e02008. doi: 10.1002/ecs2.2008
Paterson, E., Gebbing, T., Abel, C., Sim, A., and Telfer, G. (2007). Rhizodeposition shapes rhizosphere microbial community structure in organic soil. New Phytol. 173, 600–610. doi: 10.1111/j.1469-8137.2006.01931.x
Paustian, K., Lehmann, J., Ogle, S., Reay, D., Robertson, G. P., and Smith, P. (2016). Climate-smart soils. Nature 532, 49–57. doi: 10.1038/nature17174
Pennekamp, F., Pontarp, M., Tabi, A., Altermatt, F., Alther, R., Choffat, Y., et al. (2018). Biodiversity increases and decreases ecosystem stability. Nature 563, 109–112. doi: 10.1038/s41586-018-0627-8
Perrot, T., Gaba, S., Roncoroni, M., Gautier, J. L., and Bretagnolle, V. (2018). Bees increase oilseed rape yield under real field conditions. Agric. Ecosyst. Environ. 266, 39–48. doi: 10.1016/j.agee.2018.07.020
Potts, S. G., Vulliamy, B., Dafni, A., Ne 'eman, G., and Willmer, P. (2003). Linking bees and flowers: how do floral communities structure pollinator communities? Ecology 84, 2628–2642. doi: 10.1890/02-0136
R Core Team (2018). R software: Version 3.5.1. R Foundation for Statistical Computing. doi: 10.1007/978-3-540-74686-7
Rallings, A. M., Smukler, S. M., Gergel, S. E., and Mullinix, K. (2019). Towards multifunctional land use in an agricultural landscape: a trade-off and synergy analysis in the lower fraser Valley, Canada. Landsc. Urban Plan. 184, 88–100. doi: 10.1016/j.landurbplan.2018.12.013
Requier, F., Odoux, J. F., Tamic, T., Moreau, N., Henry, M., Decourtye, A., et al. (2015). Honey bee diet in intensive farmland habitats reveals an unexpectedly high flower richness and a major role of weeds. Ecol. Appl. 25, 881–890. doi: 10.1890/14-1011.1
Rollin, O., Bretagnolle, V., Decourtye, A., Aptel, J., Michel, N., Vaissière, B. E., et al. (2013). Differences of floral resource use between honey bees and wild bees in an intensive farming system. Agric. Ecosyst. Environ. 179, 78–76. doi: 10.1016/j.agee.2013.07.007
Sasaki, T., Lu, X., Hirota, M., and Bai, Y. (2019). Species asynchrony and response diversity determine multifunctional stability of natural grasslands. J. Ecol. 107, 1862–1875. doi: 10.1111/1365-2745.13151
Scherber, C., Eisenhauer, N., Weisser, W. W., Schmid, B., Voigt, W., Fischer, M., et al. (2010). Bottom-up effects of plant diversity on multitrophic interactions in a biodiversity experiment. Nature 468, 553–556. doi: 10.1038/nature09492
Schuldt, A., Ebeling, A., Kunz, M., Staab, M., Guimarães-Steinicke, C., Bachmann, D., et al. (2019). Multiple plant diversity components drive consumer communities across ecosystems. Nat. Commun. 10:1460. doi: 10.1038/s41467-019-09448-8
Shahzad, T., Chenu, C., Genet, P., Barot, S., Perveen, N., Mougin, C., and Fontaine, S. (2015). Contribution of exudates, arbuscular mycorrhizal fungi and litter depositions to the rhizosphere priming effect induced by grassland species. Soil Biol. Biochem. 80, 146–155. doi: 10.1016/j.soilbio.2014.09.023
Slade, E. M., Bagchi, R., Keller, N., and Philipson, C. D. (2019). When do more species maximize more ecosystem services? Trends Plant Sci. 24, 790–793. doi: 10.1016/j.tplants.2019.06.014
Soliveres, S., Manning, P., Prati, D., Gossner, M. M., Alt, F., Arndt, H., et al. (2016). Locally rare species influence grassland ecosystem multifunctionality. Philos. Trans. R. Soc. B Biol. Sci. 371:20150269. doi: 10.1098/rstb.2015.0269
Valencia, E., Gross, N., Quero, J. L., Carmona, C. P., Ochoa, V., Gozalo, B., et al. (2018). Cascading effects from plants to soil microorganisms explain how plant species richness and simulated climate change affect soil multi functionality. Glob. Change Biol. 24, 5642–5654. doi: 10.1111/gcb.14440
van der Plas, F. (2019). Biodiversity and ecosystem functioning in naturally assembled communities. Biol. Rev. 94, 1220–1245. doi: 10.1111/brv.12499
Venables, W. N., and Ripley, B. D., (eds.). (2002). Modern Applied Statistics with S Fourth ed. New York, NY: Springer. doi: 10.2307/2685660
Wang, L., Delgado-Baquerizo, M., Wang, D., Isbell, F., Liu, J., Feng, C., et al. (2019). Diversifying livestock promotes multidiversity and multifunctionality in managed grasslands. Proc. Natl. Acad. Sci. U.S.A. 116, 6187–6192. doi: 10.1073/pnas.1807354116
Wardle, D. A. (2016). Do experiments exploring plant diversity-ecosystem functioning relationships inform how biodiversity loss impacts natural ecosystems? J. Veg. Sci. 27, 646–653. doi: 10.1111/jvs.12399
Westphal, C., Bommarco, R., Carré, G., Lamborn, E., Morison, M., Petanidou, T., et al. (2008). Measuring bee diversity in different European habitats and biogeographic regions. Ecol. Monogr. 78, 653–671. doi: 10.1890/07-1292.1
Wickham, H. (2009). “Getting started with qplot,” in ggplot2 (Houston, TX: Springer). doi: 10.1007/978-0-387-98141-3_2
Xie, H., Wang, G. G., and Yu, M. (2018). Ecosystem multifunctionality is highly related to the shelterbelt structure and plant species diversity in mixed shelterbelts of eastern China. Glob. Ecol. Conserv. 16:e00470. doi: 10.1016/j.gecco.2018.e00470
You, Y., Wang, J., Huang, X., Tang, Z., Liu, S., and Sun, O. J. (2014). Relating microbial community structure to functioning in forest soil organic carbon transformation and turnover. Ecol. Evol. 4, 633–647. doi: 10.1002/ece3.969
Keywords: bee richness, carabids, haylands, pest control, pollination, soil organic carbon, soil enzyme activity, yield
Citation: Gaba S, Cheviron N, Perrot T, Piutti S, Gautier J-L and Bretagnolle V (2020) Weeds Enhance Multifunctionality in Arable Lands in South-West of France. Front. Sustain. Food Syst. 4:71. doi: 10.3389/fsufs.2020.00071
Received: 02 October 2019; Accepted: 27 April 2020;
Published: 28 May 2020.
Edited by:
Lisa Schulte Moore, Iowa State University, United StatesReviewed by:
Liming Ye, Ghent University, BelgiumIlias Travlos, Agricultural University of Athens, Greece
Copyright © 2020 Gaba, Cheviron, Perrot, Piutti, Gautier and Bretagnolle. This is an open-access article distributed under the terms of the Creative Commons Attribution License (CC BY). The use, distribution or reproduction in other forums is permitted, provided the original author(s) and the copyright owner(s) are credited and that the original publication in this journal is cited, in accordance with accepted academic practice. No use, distribution or reproduction is permitted which does not comply with these terms.
*Correspondence: Sabrina Gaba, c2FicmluYS5nYWJhQGlucmFlLmZy