- 1World Vegetable Center, Shanhua, Taiwan
- 2Bioversity International, Costa Rica Office, Turrialba, Costa Rica
- 3Catholic Relief Services, Baltimore, MD, United States
- 4Sustainable Impact Platform, International Rice Research Institute, Los Baños, Philippines
On-farm diversification is a promising strategy for farmers to adapt to climate change. However, few recommendations exist on how to diversify farm systems in ways that best fit the agroecological and socioeconomic challenges farmers face. Farmers' ability to adopt diversification strategies is often stymied by their aversion to risk, loss of local knowledge, and limited access to agronomic and market information, this is especially the case for smallholders. We outline seven steps on how practitioners and researchers in agricultural development can work with farmers in decision-making about on-farm diversification of cropping, pasture, and agroforestry systems while taking into account these constraints. These seven steps are relevant for all types of farmers but particularly for smallholders in tropical and subtropical regions. It is these farmers who are usually most vulnerable to climate change and who are, subsequently, often the target of climate-smart agriculture (CSA) interventions. Networks of agricultural innovation provide an enabling environment for on-farm diversification. These networks connect farmers and farmer organizations with local, national, or international private companies, public organizations, non-governmental organizations (NGOs), and research institutes. These actors can work with farmers to develop diversified production systems incorporating both high-value crops and traditional food production systems. These diversified farm systems with both food and cash crops act as a safety net in the event of price fluctuations or other disruptions to crop value chains. In this way, farmers can adapt their farm systems to climate change in ways that provide greater food security and improved income.
Introduction
On-farm diversification is a promising strategy for farmers to adapt to climate change while also contributing to diverse food production, healthier diets, and a better use of agricultural biodiversity (Vermeulen et al., 2012; Waha et al., 2018; Willett et al., 2019). However, few recommendations exist for farmers, practitioners, and researchers on how to diversify farm systems in ways which best fit the agroecological and socioeconomic challenges that farmers face.
In this paper, we outline seven steps on how to work with farmers in decision-making about on-farm diversification of cropping, pasture, and agroforestry systems (Figure 1). Existing tools to select agroecological practices and plant species for on-farm diversification (Altieri et al., 2015; de Sousa et al., 2019) or to economically optimize crop portfolios (Werners et al., 2011; Knoke et al., 2015) cover different considerations in decision-making on on-farm diversification strategies. These tools are not always linked to farmers' goals and constraints, which are embedded in a range of social, economic, ecological, cultural, and political relationships, and which determine the decisions farmers make about farm management and livelihood options (Gardner and Lewis, 1996; Shiferaw et al., 2009). This paper offers a practical and comprehensive framework, which takes into account these different issues in decision-making about on-farm diversification, and which brings together agroecological, agrobotanical, social, and economic considerations and recommendations.
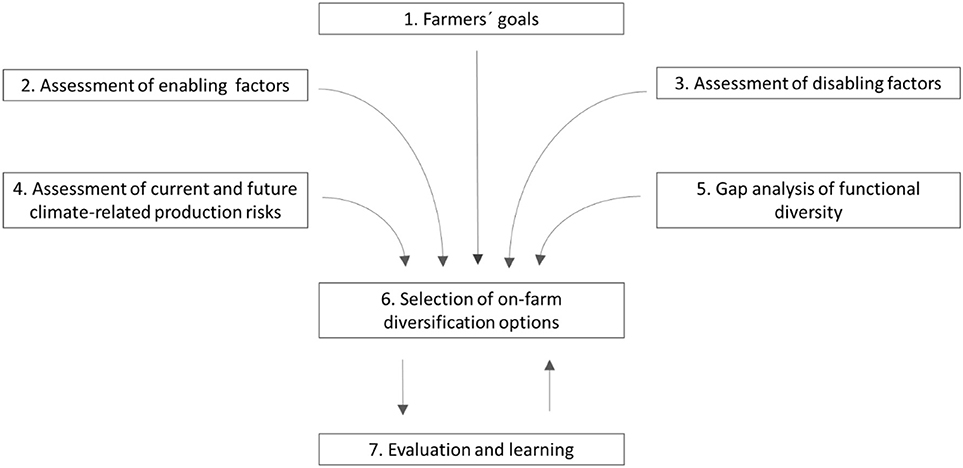
Figure 1. Decision-making framework to develop, select, evaluate, and implement on-farm diversification strategies for climate change adaptation. We propose seven steps for practitioners and researchers to work with farmers in decision-making. Steps 1–5 help farmers and other actors to collect information to select on-farm diversification options in step 6. After the selection of on-farm diversification options, farmers can evaluate them in step 7 and implement or adjust them or replace them by other on-farm diversification options. This is reflected in a feedback loop between step 6 and 7. The arrows indicate which steps influence other steps in the decision-making framework.
This decision-making framework is intended for practitioners and researchers in agricultural development. The framework can be used to establish a dialogue with individual farmers or farmer groups to develop on-farm diversification strategies with the use of participatory research approaches, which have proved to be successful approaches in the selection and adoption of new agricultural technologies (Carberry et al., 2002; Grothmann and Patt, 2005; Urwin and Jordan, 2008).
In this framework, we first discuss enabling and disabling factors, which warrant consideration when developing on-farm diversification strategies. Second, we propose straightforward tools and techniques, which can help farmers to select on-farm diversification options. Finally, we explain how researchers, practitioners, and farmers can apply participatory approaches to evaluate on-farm diversification options.
The seven steps are useful for all types of farmer but are particularly relevant to smallholder farmers. Smallholders are often more vulnerable to climate change compared with large-scale farmers and usually face higher risks when adopting new technologies because of lower resource endowments. Smallholder farmers are the main target of interventions, which are collectively known as climate-smart agriculture (CSA). CSA contributes to an increase in global food security and broader development, secondly enhances farmers' ability to adapt to climate change, and finally mitigates greenhouse gas emissions (Lipper et al., 2014). On-farm diversification is a component of CSA, and not only contributes to the realization of the Sustainable Development Goal (SDG) 13: Climate Action but also of other SDGs, including SDG 1: No Poverty; SDG 2: Zero Hunger; SDG 12 Responsible Consumption and Production; and SDG 15 Life on Land.
Approach
The seven steps resulted from the authors' discussions on existing concepts and tools from literature on climate change adaptation and on-farm diversification. These concepts have been presented separately in literature. By connecting these concepts, we establish a practical framework for decision-making to diversify farm systems for climate change adaptation. We focus on tropical and subtropical regions where most smallholders live and work, and on cropping, pasture, and agroforestry systems as principal components of farm systems in these regions. Many examples of crops and traditional production systems in this paper come from Central America and Mexico where each of the authors has over 12 years' work experience complemented by extensive experience from Sub-Saharan Africa, South Asia, and South-East Asia. The seven-step decision-making process is applicable to other tropical and subtropical regions.
Step 1. Defining farmers' goals: Any initiative to work with farmers starts with understanding the goals of the different farm household members, and identifying how on-farm diversification can contribute to these goals (Allen et al., 2011).
Step 2. Assessment of enabling factors: Enabling factors determine the feasibility and potential of on-farm diversification options. Farmers are more willing to select, evaluate, and implement new diversification strategies in the context of an enabling environment consisting of support from farmer organizations and private and public extension services, and access to credit, insurance, and markets.
Step 3. Assessment of disabling factors: Successful adoption of on-farm diversification strategies depends on the extent to which farmers have the possibility and are willing to invest in labor, financial capital, and learning new skills.
Step 4. Assessment of current and future climate-related production risks: On-farm diversification strategies can be tailored to local conditions when farmers, practitioners, and researchers identify the principal climate stresses for current and future agricultural production in their locations (Vermeulen et al., 2013).
Step 5. Gap analysis of functional diversity in farm systems: Farmers and other actors can identify the need for diversifying their farm systems with new crop functional types, such as cereals with C4 photosynthesis (Lavorel and Garnier, 2002) or the need for new management practices, such as the establishment of shade trees to make farm systems more resilient against climate changes (Altieri et al., 2015).
Step 6. Selection of on-farm diversification options: Farmers choose crops on the basis of multiple criteria considering their goals, enabling and disabling factors, climate-related production risks, and gaps in functional diversity (Coe et al., 2014).
Step 7. Evaluation and learning: These activities are part of adaptive management. Farmers continuously evaluate and improve on-farm diversification strategies in dialogue with other farmers, practitioners, and researchers (Allen et al., 2011).
Step 1. Farmers' Goals
Any initiative to work with farmers starts with understanding farmers' goals and identifying how diversification of their farm systems contributes to these goals. Often profit-maximizing approaches, such as Modern Portfolio Theory (MPT) are used to determine the optimal number and type of crops or land-use systems to manage production risks for a certain expected return on investment under climate change (Figge, 2004; Werners and Incerti, 2007; Werners et al., 2011). Farmers, especially smallholders, often perceive benefits from on-farm diversification in ways which profit-maximizing approaches do not necessarily capture. When diversifying their farm systems, farmers often define multiple goals, for example, they consider cereals for food security; pulses and vegetables for nutrition; cash crops for increasing income; off-seasons crops and forages for animal production to stabilize income; and finally intercropping and field scattering to reduce production risks (Schroth and Ruf, 2014). Different household members, such as women and men, may have different goals (van de Fliert and Braun, 2002; Chaudhury et al., 2013). Participatory approaches have proved effective in enabling practitioners and researchers to understand the goals of different members of farm households (Mazón et al., 2016; Dumont et al., 2017). Understanding farmers' goals is thus the basis of working with farmers in developing, selecting, evaluating, and implementing on-farm diversification strategies.
Step 2. Enabling Factors
Extension
A particular challenge is that our proposal to work in a participatory way with farmers comes at a time when public extension services have been severely eroded in much of the developing world (Umali-Deininger, 1997; Hellin, 2012). Private extension has increased but there has been a tendency to focus efforts on better-off farmers leaving those in marginal areas with limited services (Hellin, 2012). There are, however, growing examples of innovative extension approaches which include both the public and private sector (Chapman and Tripp, 2003). The transformation from specialized to diversified farm systems can be fostered by agricultural innovation systems (Schut et al., 2014). In the absence of extension and agricultural innovation systems, farmers would need to rely largely on neighboring farmers, farmer organizations, and local knowledge to adapt their farm systems to climate change.
Farmer Organization
The organization of farmers in associations, farmer-to-farmer movements, or other types of social organization can be an effective way to scale practices to diversify farm systems because these organizations are conduits for the dissemination of knowledge and information (Shiferaw et al., 2009; Mier et al., 2018), and allow to establish safety nets for farmers through formal and informal insurance programs (Tucker et al., 2010; Bacon et al., 2014) (Table 1, Examples 1 and 2). Capacity development on good governance and finance makes farmer organizations more competent, efficient, and transparent, and diminishes dependence on external authorities or donors. With these skills, farmer organizations can reduce the risks on “elite capture,” secondly they can access credit from banks and social investors to invest in on-farm diversification, and finally they can connect to networks of agricultural innovation to access markets and external support (Table 1, Example 2). Farmer organizations are thus in principle good partners in selecting, developing, evaluating, and implementing on-farm diversification strategies.
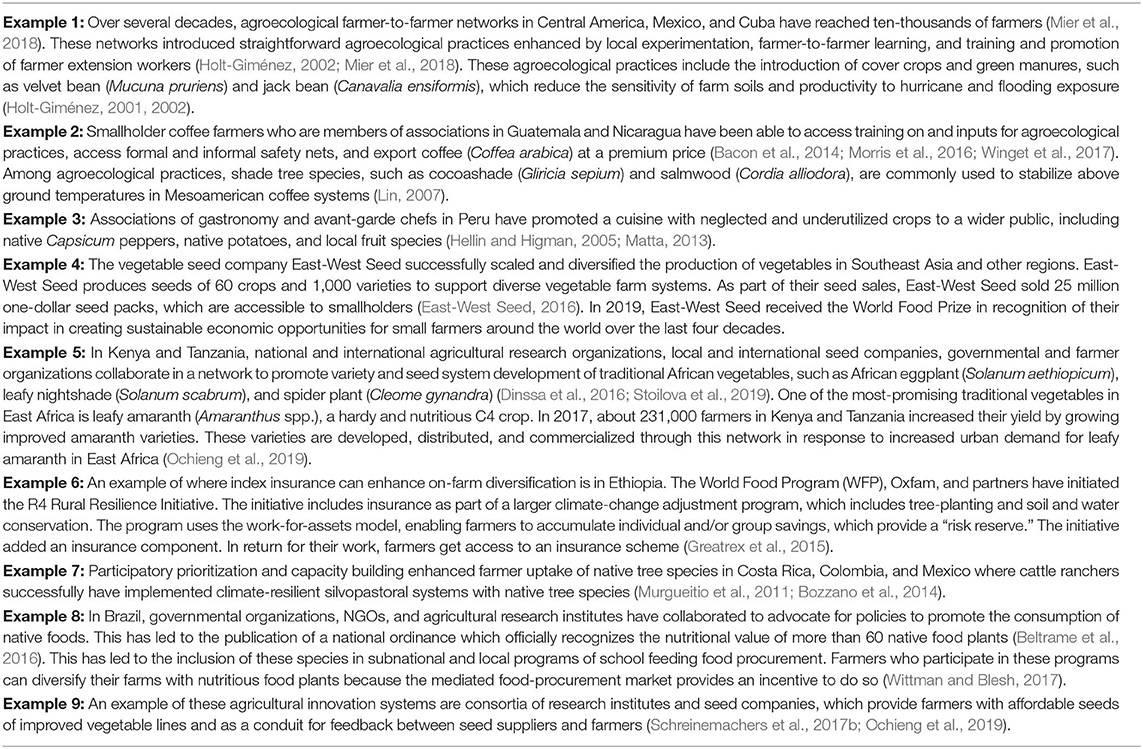
Table 1. Examples of successful societal, public and private initiatives to support farmers in diversifying their farm systems.
Local Knowledge and Neglected and Underutilized Crops
At least 7,000 food plant species have been documented and these provide a rich basket of crop choices (Padulosi et al., 1999). Many are neglected and underutilized (National Research Council, 1989; Clement, 1999). These species could become important for food security under changing climate conditions because they have evolved during a long history of human selection and fluctuating climate conditions (Mercer and Perales, 2010; Padulosi et al., 2011). Some examples of promising species for diversification and climate change adaptation are provided in Table 2.
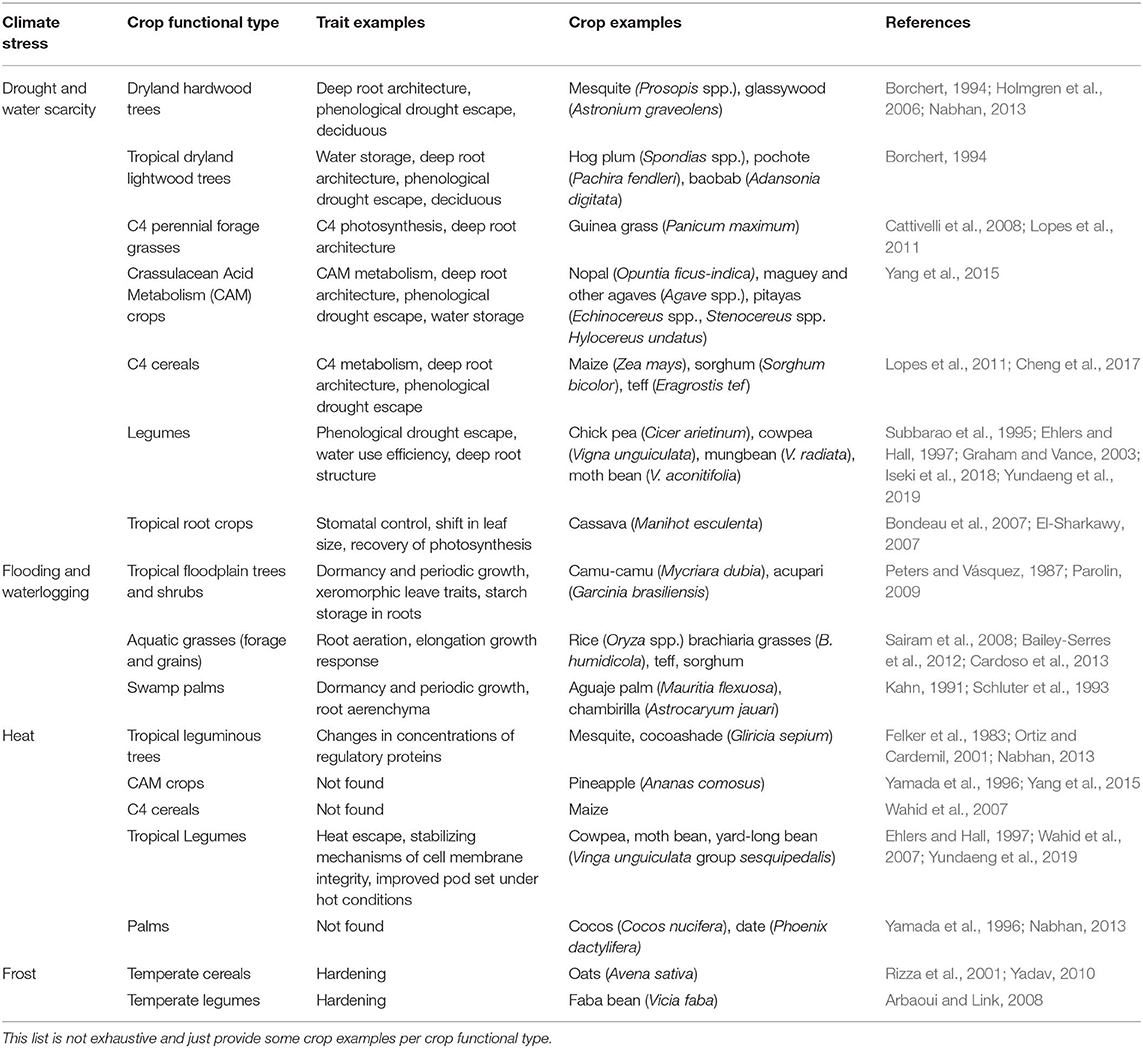
Table 2. Crop functional types and crop examples to diversify in response to various climate stresses.
Farmers in traditional communities have commonly diversified their farm systems with these crops to manage production risks related to unpredictable weather cycles (Winterhalder et al., 1999; Matsuda, 2013; Altieri et al., 2015). Much of the local knowledge associated with growing neglected and underutilized crops is at risk of extirpation due to changing diets, reduced interest by young people in agriculture, and shifts in production systems under climate change (Padulosi et al., 2011; Khoury et al., 2014). With this loss, farmers have fewer diversification options. This makes them more vulnerable to climate change. Finally, the decline of production and consumption of these neglected and underutilized crops leads to the disappearance of local varieties whose traits for adaptation to climate stresses are not only important to local farmers but also for research and breeding by the global agricultural research community (Table 3, Example 1).
The promotion of these neglected and underutilized crops is complex and requires actions at both the supply side to incite farmers to continue using these crops and demand side to persuade consumers to incorporate these crops in their diets. Here we name three approaches to provide incentives to farmers' use of neglected and underutilized crops to diversify farm systems. First, within each community, commonly a few farmers are knowledge hubs on the management of these neglected and underutilized crops (Altieri and Merrick, 1987; Sthapit et al., 2013). These persons are custodian or lighthouse farmers who merit recognition in society and who can be encouraged to share their knowledge with other farmers as well as with practitioners and researchers. Second, empowerment of women in agriculture increases the options for on-farm diversification because both men and women maintain exclusive and complementary knowledge about crops and farm management (Padulosi et al., 2011). Because female-headed farm systems are not necessary more diverse than male-headed ones (Saenz and Thompson, 2017), it is important to understand the complementary impacts of women and men's choices on the diversification of farm systems (Farnworth et al., 2016). Finally, the identification and development of niche markets and new uses of neglected and underutilized crops can stimulate their production and the maintenance of local knowledge (Table 1, Example 3). There are, hence, several strategies to maintain and use local knowledge on neglected and underutilized crops to promote diversified farm systems.
Getting the Right Variety
Farmers often struggle to find planting material of crops with high potential for on-farm diversification even though appropriate varieties are often available at agricultural institutions or maintained by neighboring farmers (Jarvis et al., 2011). Due to weak formal and informal seed systems, farmers are not always able to access germplasm of appropriate varieties and diversify their farm systems. Farmers can access more varied germplasm when they are better connected to public and private germplasm suppliers (Coomes et al., 2015; Stoilova et al., 2019) and when these suppliers strengthen their germplasm production capacity (Schreinemachers et al., 2017a). The desired type of seed system differs between crop groups and should be defined per crop and region (Louwaars and de Boef, 2012). For example, public-private networks of research institutes and local, national, and international seed companies have proven to be successful to scale the supply of affordable and high-quality vegetable seeds (Schreinemachers et al., 2017a) (Table 1, Examples 4 and 5). Aside from fostering farmers' access to commercial and public germplasm in formal seed systems, farmer communities across the world successfully establish networks to conserve, use, and exchange germplasm of local varieties and associated knowledge (Coomes et al., 2015; Vernooy et al., 2017). The promotion of promising crops to diversify farm systems requires an assessment of the existing formal and informal seed systems to strengthen, where necessary, germplasm quality and supply in collaboration with farmer organizations, NGOs, breeders, genebanks, and private and public suppliers of planting material.
Insurance
Risk aversion on the part of farmers, especially smallholders, is an obstacle to the adoption of new crops, varieties, and novel management practices (Lee, 2005). Weather shocks, such as drought, can trap farm households in poverty because the risk of the shocks limits farmers' willingness and capacity to invest in on-farm diversification strategies (Dick et al., 2011; Carter et al., 2016). For example, fire risk in drought-prone areas limits farmers to diversify farm systems with tree species (Jacobi et al., 2017). As a complement to on-farm diversification, agricultural insurance against yield loss mitigates the risks farmers face and encourages them to diversify their farm systems (Bobojonov et al., 2013).
One approach gaining much attention is index insurance. With index insurance, payouts are based on an index, such as the total seasonal rainfall or average crop yield for a larger area. This index reduces the costs of insuring individual farmers (Bell et al., 2013). Furthermore, the insurance is based on a reliable and independently verifiable index and can be reinsured, allowing insurance companies to transfer part of their risk to international markets (Binswanger-Mkhize, 2012). Index insurance can be bundled with climate-adapted germplasm or cropping systems to encourage farmers to invest in crop productivity (Bobojonov et al., 2013) (Table 1, Example 6).
Index insurance, however, is not a perfect predictor of an individual loss. The difference between the farmers' actual losses and the expected payout is known as basis risk; it may result in a farmer suffering a yield loss, but not receiving a payout, or in a payout without the farmer experiencing any loss (Dick et al., 2011; Miranda and Farrin, 2012).
Whole-farm insurances could be another promising insurance measure to provide farmers an incentive to diversify farm systems (Hart et al., 2006; Turvey, 2012). What whole-farm and index insurances have in common is that combining agricultural insurance with on-farm diversification benefits both farmers and insurance providers. Diversified farm systems can stabilize income and productivity and reduce the risks and corresponding premia of insurance. A recommendation is to develop policies and incentives for innovative insurance services, which support and promote on-farm diversification.
Markets
High-value crops, such as fruit and vegetable species, have been identified as promising crops to diversify farm systems and to increase farmers' net income (Joshi et al., 2004; Pingali, 2007; Birthal et al., 2015). Vegetable species are of special interest because in general they have short rotation cycles and can provide quick and year-round returns (Schreinemachers et al., 2018). Market access may, however, be limited to large-scale farmers as smallholders often lack capital to make investments to convert a semi- or fully-subsistence farm system into a commercial farm system (Pingali, 2007; Eakin et al., 2012). Many high-value crops, such as leafy vegetables, are perishable and this often requires additional investments in post-harvesting and transportation. Finally, smallholders can be particularly vulnerable to fluctuating market prices (Eakin, 2003; Carletto et al., 2010). Linking farmers, especially smallholders, to markets therefore requires support by governments, food processors, and distributors to strengthen post-harvesting facilities, distribution channels, stable production supply, and insurance.
Farmers tend to focus on one or a few crops to meet quality demands. However, a sole focus on one or two high-value cash crops in a farm system can be a risk for food security and livelihoods for individual farm households as well as for local economies (Immink and Alarcon, 1991; Chakrabarti and Kundu, 2009) (Table 3, Examples 2 and 3). Rather than focusing solely on one or two cash crops, farmers may therefore opt to manage several crops and varieties with different production and price risks, to meet food and nutrition security goals, and increase net income (Table 4, Example 1).
Step 3. Disabling Factors
Scale Effects
Scale effects leading to crop and farm specialization may be stronger drivers than those leading to on-farm diversification. Such specialization can occur in the case of commodities where there is a demand for large quantities and where sophisticated and product-specific technical packages drive monocultures. Such can be the case for oil palm (Elaeis guineensis), sugarcane (Saccharum officinarum), and soybean (Glycine max). Indeed, for several decades, research and development efforts in the agricultural sector of many countries support technologies, which reinforce scale effects and favor specialization (Griffon, 2006; Pingali, 2012). Agricultural subsidies in countries, such as Mexico, Bolivia, and Zambia support large-scale monocultures rather than diversified production systems (Eakin and Wehbe, 2009; Jacobi et al., 2017; Saenz and Thompson, 2017).
With more research investment and policy support, scalable and economically-feasible diversification practices can be developed. So far, scaling of species mixtures has been successful for pasture and cover crops because these mixtures increase productivity without extra management costs (Bybee-Finley et al., 2018) (Table 4, Example 2). The wide-scale introduction of high-quality seed of vegetable crops to smallholder farmers in Southeast Asia during the last decades is a successful example on how to scale diversification of farm systems with high-value crops (Schreinemachers et al., 2018) (Table 1, Example 4).
Labor Constraints
Any on-farm diversification option should save labor and/or increase and/or stabilize net income to make it an attractive option for climate change adaptation (Lee, 2005). Labor saving is urgent because climate change is predicted to reduce farming labor capacity in tropical regions by up to 50–80% in peak months of heat stress (Dunne et al., 2013; Myers et al., 2017). Diversification with cover crops and shade trees can reduce the labor costs of weed control (Raintree and Warner, 1986; Holt-Giménez, 2006; Liebman and Dyck, 2007) or fertilizer input in the case of cocoa agroforestry systems (Armengot et al., 2016). However, often diversified farm systems require more labor compared with less complex systems (Bacon et al., 2012). This has been the case for diversified rice systems and cocoa systems (Pingali, 1992; Armengot et al., 2016). The introduction of high-value crops, such as fruit and vegetable species could be an alternative diversification strategy to increase or stabilize net income (Joshi et al., 2004). Finally, diversification strategies, which improve on-farm climate conditions, such as the establishment of shade trees can eventually improve labor conditions because while they may require a large initial labor input this tails off substantially after tree establishment.
Farm Size and Land Ownership
Although farm size is thought to be a constraint for diversification, we did not find a clear correlation between farm size and on-farm diversification. As part of a systematic literature review, which included 13 detailed studies, six reported that on-farm diversification increases with farm size; four studies reported no effect; and three studies reported that on-farm diversification reduces with farm size (Table S1). There is thus scant evidence that farm size is an enabling factor or constraint for on-farm diversification. Our recommendations to diversify farms are therefore relevant for different farm sizes.
We found only a few studies, which consider land ownership as a factor in diversification (Lawin and Tamini, 2017; Asante et al., 2018). These studies showed no relationship between land ownership and diversified farms. More research is needed to understand better if there is any relation between these two variables.
Step 4. Current and Future Climate-Related Production Risks
Farmer perceptions of weather cycles and climate change are a good starting point for identifying climate risks. Their knowledge may need to be combined with formal predictions to reduce bias from their recent experiences and to reflect long-term climate trends. Once climate risks are identified, crops, varieties, and management practices can be selected to manage these risks.
Climate models with projections in climate change under different economic and climatic scenarios allow for predictions of climate change impact on crop production for the next decades (Lobell et al., 2008; Baca et al., 2014; de Sousa et al., 2019). The main purpose of these models is to reduce uncertainty in decision-making rather than to give precise predictions (Vermeulen et al., 2013). These models are relevant for planting decisions for both annual and perennial commodities, such as soybean and coffee (Coffea spp.), for which a whole infrastructure needs to be maintained or put in place. Even in the case of the introduction of non-commodities, time may be required to develop seed systems and to develop the capacity of farmers who are interested in growing these crops.
Climate models, which use historic climate trends, help to predict trends in climate stress for shorter time spans compared with the decadal predictions of climate models on the basis of projections in climate change. To be effective, the results of these models have to be communicated clearly to farmers (Pulwarty and Sivakumar, 2014). The Famine Early Warning Systems Network (FEWS NET), for example, provides rainfall predictions for the next 10–365 days on the basis of high-resolution rainfall and hydrological models (Senay et al., 2015). These predictions allow farmers and other actors in the value chain to anticipate and adjust cropping systems to water scarcity or surplus. High-quality modeling in combination with good communication is thus essential to provide farmers meaningful information about current and future climate risks.
Step 5. Gap Analysis of Functional Diversity in Farm Systems
By filling functional gaps in farm systems, farmers can stabilize and even increase primary productivity of their farm systems under climate change. This occurs via two distinct but linked agroecological mechanisms. First, diversification with crops and varieties, each with a differential response to climate stresses, stabilizes primary productivity in agroecosystems under climate variability. The second mechanism is related to diversification of crops and management practices to foster ecological functions. Ecological functions increase and stabilize primary productivity in farm systems and include climate regulation, water storage, nutrient cycling, and pest regulation. By understanding these two agroecological mechanisms and translating that knowledge into practical recommendations for decision-making, farmers can make informed choices about adapting their farm systems to climate change.
Crop Choices for Differential Responses to Climate Stresses
Spatial diversification stabilizes primary productivity of farm systems under climate variability when crops with differential responses to climate stresses are grown in polycultures or in separate fields. These crops expand together the physiological range to produce a minimum yield under different climate conditions. In addition to physiological range expansion, positive plant interactions and niche complementary further increase and stabilize agricultural productivity (Brookfield, 2001; Malézieux et al., 2009).
When considering polycultures to diversify farm systems in a specific area, local knowledge on crops and management practices provide a rich source of possibilities for rotations, intercropping, and agroforestry systems (Eakin, 2005; Hellin and Dixon, 2008; Isakson, 2009) (Table 4, Example 3). Traditional polyculture systems can fall into disuse because of labor constraints, poor markets, and erosion of local knowledge. It is therefore important to address these economic and cultural constraints in order to maintain and improve traditional systems, and introduce new systems as well.
Crop functional types help to differentiate between crops, which, because of their physiological differences, tolerate different types and different levels of climate stress (Table 2). For polycultures, farmers ideally choose crops, which besides their differentiated tolerance to climate stresses, have complementary traits to reduce competition for similar resources, such as different rooting depths, complementary nutrient requirements, and differential light interception patterns (Brooker et al., 2015). In this way, farmers can minimize competition for light, water, and nutrients between crops, and avoid production and income loss.
The upper temperature ranges for the production of many crops is below 40°C while temperature conditions above 40°C become more prevalent in tropical growing areas (Farooq et al., 2017). Only a limited amount of crops can adapt to temperatures above 40°C, either through short growth seasons or by coping with high temperatures during sensitive development stages, such as pollen development, fruit setting, and grain filling (Wahid et al., 2007; Barnabás et al., 2008). Table 2 gives a few examples of the crops which are reported to be strong candidates for agricultural production under hot conditions. In contrast, low temperatures can cause production risks in mountain areas in tropical and subtropical regions (Table 4, Example 4).
Plant production is principally limited by lack or excess of water. Drought and flooding events have occurred with greater frequency over the past 50 years and the trend is predicted to continue (Lobell et al., 2008). Despite the vulnerability of many plant species to drier conditions (McCord et al., 2015), a wide range of species is adapted to dry conditions in rain-fed systems. Table 2 includes a few examples of species, which are reported to be strong candidates for on-farm diversification of rain-fed systems under increasing drought conditions.
C4-metabolism crops, such as maize (Zea mays) and sorghum (Sorghum spp.) have in general a high water-use efficiency and are better in tolerating water stress compared with C3-metabolism crops, such as wheat (Tritricum spp.) and sunflower (Helianthus annuus) because of their more efficient photosynthetic apparatus (Zhang and Kirkham, 1995; Nayyar and Gupta, 2006). This makes C4 crops potential candidates for production under dry and hot conditions, although several C4 crops may be susceptible to water stress because of the wide diversity in C4 plant evolution (Ghannoum, 2009). Crassulacean Acid Metabolism (CAM) crops use significantly less water and can grow in higher temperatures compared with C3 and C4 crops. Some CAM crops, such as pineapple (Ananas comosus) are commercial crops. The majority of CAM crops, however, are neglected or underutilized (Mizrahi et al., 2007; Yang et al., 2015).
Tree planting is a common on-farm diversification strategy to improve microclimates after their establishment (Bryan et al., 2009; Meldrum et al., 2018). Native tree species may be preferred candidates for diversification (Table 1, Example 7; Table 2). Since most tree species are wild or at an incipient stages of domestication, some exotic tree species can become invasive, such as the American species Prosopis juliflora in African countries (Richardson, 1998), or can be highly competitive for water, such as Eucalyptus spp. and may outcompete understory crops under drought-stress conditions (Saxena, 1991; German et al., 2006). Native food tree species provide also a reliable food source for farmer households in lean months (Graefe et al., 2012; Bacon et al., 2014) (Table 4, Example 5). Despite their potential importance for food and nutrition security, there is generally a lack of focus on these tree species in people's diets under climate seasonality and inter-annual variability (Rowland et al., 2015).
As periods of drought become longer and more frequent, farmers may need to replace water-competitive shade trees with species, which are less water demanding. The pruning of tree species reduces water stress and allows farmers to manage shade (Bayala et al., 2002) while also providing mulch to conserve soils and retain soil moisture (Hellin et al., 1999).
With respect to water excess, food tree species from tropical floodplains and swamps, such as many palm species, tolerate long periods of waterlogging (Table 2). In a similar line, sugarcane and perennial forage grasses, such as Brachiaria spp., can withstand waterlogging conditions (Cardoso et al., 2013; Gomathi et al., 2014). As with tree species, native forage grasses may be preferred because of the risk that exotic ones become invasive (DiTomaso, 2000).
Many traits related to stress tolerance can be found at variety level. Major advances have been made in breeding to increase drought tolerance of main cereal crops, such as maize (Cairns et al., 2013). Nevertheless, farmers may still want to diversify with drought-tolerant minor cereals and legumes (Table 2). For some cereals, such as maize, landraces could be good choices in strategies of on-farm diversification because they contain high levels of genetic variation, which enable landraces to evolve under the interplay of human selection and climate change (Mercer and Perales, 2010; Vigouroux et al., 2011). Evaluation of these landraces in different environments helps shed light on their potential for climate change adaptation and in breeding strategies in a similar way to the search for climate-adapted durum wheat landraces (Ceccarelli, 2015; Mengistu et al., 2016).
Even though breeders use advanced technologies, such as genomic selection and editing to develop varieties with multiple traits to tolerate climate stresses (Tester and Langridge, 2010; Mousavi-Derazmahalleh et al., 2019), it remains a challenge to stack these traits in single varieties (Mercer and Perales, 2010). Alternatively, a traditional approach is to grow multiple varieties of the same crop to respond to multiple stresses (Jarvis et al., 2008; Matsuda, 2013; Salazar-Barrientos et al., 2016). Farmers can thus diversify their farm systems by growing both multiple crops and varietal mixtures. In the same line, livestock and feed producers may prefer pasturelands, which are both rich in grass species and rich in genotypes because these pasturelands are more productive and recover better after extreme events, compared with less diverse ones in the same biotope (MacDougall et al., 2013; Prieto et al., 2015).
Crop Choices and Management Practices to Foster Ecological Functions
Diversification of farm systems in space and time can foster ecological functions, such as climate regulation, water storage, nutrient cycling, and pest regulation. Farmers may find it useful to use a straightforward checklist of management practices, which foster ecological functions to improve their farm systems (Table 5).
Microclimates can be regulated by tree shade, which buffers against high temperatures above ground and in some cases prevent frost damage (Barradas and Fanjul, 1986; Caramori et al., 1996) (Table 1, Example 2). Forage tree and shrub species, which are planted along field borders, provide a wind-break to maintain moisture levels in agriculture fields (Holt-Giménez, 2002), and are a source of animal fodder in times of drought (Kort, 1988; Tamang et al., 2010). Tree species can therefore be selected for multiple goals in farm systems including for food or fodder production and to maintain ecological functions.
On-farm diversification with cover crops and green manures can improve and conserve soil by building up organic matter, adding nitrogen, improving soil structure, and reducing soil erosion (Cong et al., 2014). As a consequence, soil fertility, infiltration, water holding capacity, and soil moisture can increase, and with that the crops' ability to cope with drought (Erenstein, 2003; Waraich et al., 2011). However, under humid conditions and on poorly drained soils, mulching can cause waterlogging resulting in lower yields (Giller et al., 2009). Some cover crops are competitive for water, and if intercropped, they can reduce the yields of the main crop under water limiting conditions. Therefore, selection of soil-improving intercrops or relay crops, which are water efficient, is important in drought-prone environments. Alternative management options in semi-arid regions include external biomass input from hedgerows or woodlots and establishment of rotation schemes with cover crops.
Crop residue incorporation is an important practice to improve soil quality (Turmel et al., 2015). In mixed cropping and livestock systems, especially in semi-arid areas, trade-offs exist between using residues for fodder or soil cover (Giller et al., 2009). In many areas, however, farmers require these residues for animal feed and in some cases they earn more from selling the residues for feed than they can from the maize they grow (Beuchelt et al., 2015). If farmers leave at least a portion of their residues in their fields, then they provide soil cover and build organic matter (Turmel et al., 2015). Alternative biomass-producing crops and sources of forages and soil cover can be introduced in intercropping, agroforestry, or silvopastoral systems to address these needs.
Holt-Giménez (2002) showed how diversification of Nicaraguan farm systems with agroecological practices, such as soil cover, windbreaks, crop rotation, and alley cropping, protected farmers' fields during extreme weather events compared with farmers' conventional practices (Table 1, Example 1). This evidence suggests that diversification enables farm systems to recover more quickly from extreme weather events compared with uniform farm systems.
Diversification across multiple spatial scales beyond the farm level is thought to further stabilize micro and mesoclimates and make farm systems more resilient against extreme weather events (Kremen et al., 2012). Forest patches surrounding cropping systems and pasturelands may control rainfall distributions and regulate temperatures at meso-level, but more evidence is needed (Teuling et al., 2010; Lawrence and Vandecar, 2015). Preliminary evidence show that farm systems in a diversified landscape indeed recover more quickly from extreme weather events compared with farm systems in uniform landscapes but the finding are not yet conclusive (Philpott et al., 2008; Gil et al., 2017). Monitoring farm systems in areas with extreme weather events will help to collect more data to understand further how diversification at multiple spatial scales makes farms more resilient against extreme weather events.
Caution is needed when introducing a new crop into a farm system since it can be a host of new crop diseases (Marshall, 1977; Anderson et al., 2004). Often, however, it is only a question of time until a pest or disease arrives because of globalized food export and import, and shifting distributions of pest and diseases due to climate change (Shaw and Osborne, 2011; Bebber et al., 2013). On-farm diversification is therefore a good preparation for when these pests or diseases arrive. First, crop diversification may reduce the risk of pest and disease outbreaks related to monoculture host plants (Rosenzweig et al., 2001). Some pests and pathogens, however, use a wide range of host plants, which limits the potential of crop diversification for preventing these outbreaks (Ratnadass et al., 2011). Second, heterogeneity in vegetation and crops obstruct pest movement and provide habitats for natural pest enemies (Avelino et al., 2012). Finally, a wide range of plant species, which repel or attract pests, is available to farmers. By understanding which climate stresses these plant species tolerate, they can be selected for pest control under changing climate conditions (Table 4, Example 6).
Step 6. Selection of On-Farm Diversification Options
To support on-farm diversification, all the relevant information mentioned in steps 1 to 5 can be combined in a decision model, which captures multiple criteria (Figure 2). For many crops no exact information about markets and optimal growing conditions exist. Alternatively, ranking and scaling by a group of persons already provides robust estimates and comparisons (Hubbard, 2014; van Etten et al., 2016). These straightforward scoring approaches help determine which crops, varieties, and management practices are more appropriate for farmers' goals, such as income stability, food security, and/or nutrition; which crops and varieties require more or less labor, and so on. Selected diversification options can be further evaluated on-farm to test how well they fit farmers' realities, goals, and aspirations. The selection of these options can be done in focus-group discussions in farmer communities with farmers, practitioners, and researchers, and by interviewing key persons from farmer communities, as well external actors, which could support farmers in access to markets, germplasm, climate information, credit, or insurance (Schattman et al., 2015; Morris et al., 2016).
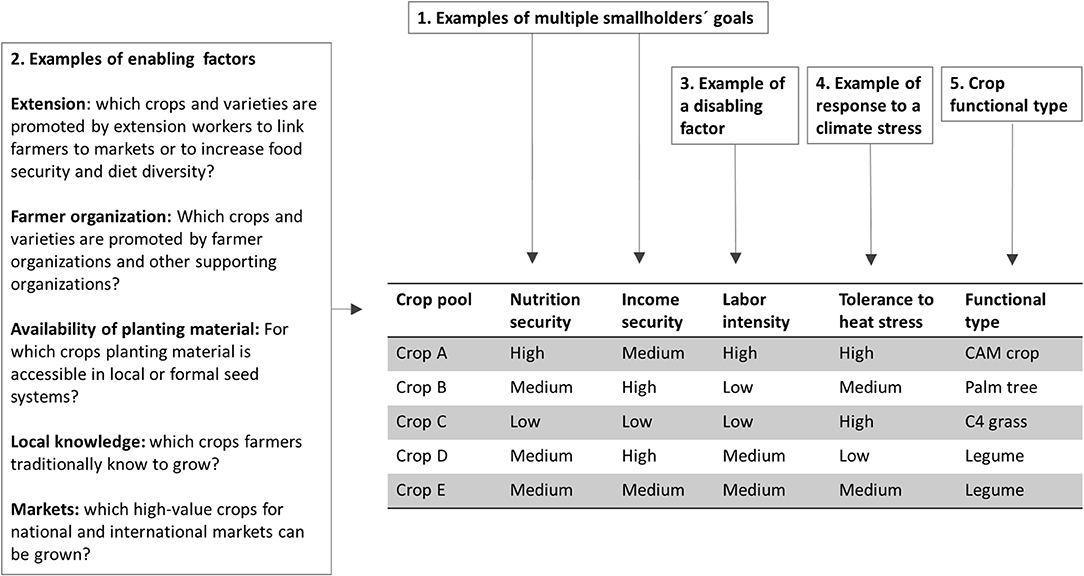
Figure 2. Decision model to select crops and management practices for on-farm diversification. The existing enabling factors as defined in step 2 determine the availability of crop choices. Crops can be chosen using multiple criteria in function of the farmers' goals defined in step 1; disabling factors defined in step 3; climate stresses defined in step 4; and a gap analysis of functional diversity in step 5.
Crop options are available for different agroecological zones. In all these zones, legumes and trees are common functional types to diversify farm systems for climate change adaptation (Tables 2, 4). Some studies suggest that a low optimum number of on-farm diversification options for semi-arid agroecological zones (Waha et al., 2018). Therefore, it would be important to maximize the functional diversity in semi-arid regions within a few crops (see Table 2).
Many crops, which are hardy and can tolerate climate stresses, are neglected and underutilized (Table 2). The reality is that most of these crops have limited market opportunities. A selection of the crops with most potential for both climate change adaptation and markets, and targeted and long-term efforts to strengthen both supply of and demand for these selected crops, can help to support farmers to diversify their farms with these crops (Table 1, Examples 3 and 8).
Among high-value crops, vegetable species are commercially interesting for smallholder farmers and easy to incorporate in farm systems. However, we found little research on climate stress tolerance in vegetable species compared with species from other crop groups. Further research is needed to evaluate the response of vegetable species to different climate stresses because these are potentially interesting crops for diversification.
Step 7. Evaluation and Learning
Participatory evaluation is a cost-effective way to evaluate crops, varieties, and management practices despite high transaction costs in communication and information exchange (Almekinders et al., 2007; Thomas et al., 2007). For on-farm testing of new crops, varieties, and management practices, home gardens are convenient because farmers traditionally use these places for experimentation (Williams, 2004; Galluzzi et al., 2010). After evaluation, farmers can decide if they wish adopt these new options and how best to incorporate them in their farm systems.
For uptake and scaling of diversification measures within communities, it is often advantageous to work initially with the most innovative female and male farmers, such as custodian or lighthouse farmers. They are often the most eager to experiment with diversification options and can subsequently inspire others (Hellin and Dixon, 2008). Researchers and practitioners can foster knowledge exchange between farmers by supporting farmer networks. Women and other vulnerable groups in many countries, would need to be involved in these activities to prevent increase in inequality as a consequence of differential access to information and learning opportunities (Tompkins and Adger, 2004).
Agricultural innovation systems are another form to share knowledge and to encourage learning about on-farm diversification options among farmers, and other private, public, and societal actors in value chains (Schut et al., 2014) (Table 1, Example 9). Feedback and information exchange on crop and variety performance between germplasm suppliers, farmers, and other actors improves site-specific crop and variety recommendations and enhances farmers' access to high-quality germplasm (van Etten et al., 2019).
Discussion
In this paper, we propose seven steps to work with farmers in making choices about the development, selection, evaluation, and implementation of on-farm diversification strategies for climate change adaptation. These steps are based on existing concepts on climate change adaptation, which are often recommended separately. Complementary to existing tools, which recommend agroecological practices (Altieri et al., 2015), select species (de Sousa et al., 2019), or economically optimize crop portfolios (Werners et al., 2011), this decision-making framework brings together agroecological, agrobotanical, social, and economic considerations and recommendations from different disciplines, and links these to farmers' goals and constraints. The framework, coupled with extensive field experience from Latin America, Sub-Saharan Africa, and Asia, offers a practical and comprehensive tool for researchers and practitioners to establish a dialogue with farm households or with farmer groups to develop on-farm diversification strategies.
We argue that the four most essential elements for selection of appropriate on-farm diversification options are: step 1 on understanding farmers' goals, which is the basis of any adaptation plan; step 2 on identifying enabling factors to identify opportunities to support farmers with financial and technical support; step 5 on assessing gaps in functional diversity in farm systems, which need to be filled to adapt farm systems to climate change; and step 6 on the selection of on-farm diversification options to fill these gaps. These four steps would be the minimum needed to work with farmers in the development and selection of viable on-farm diversification options for climate change adaptation.
Practitioners, policy-makers, and farmer organizations who aim to incite farmers to diversify their farm systems in a specific territory, can use the framework as a check box and follow the steps in this framework on the basis of their existing knowledge and with support of local and international research organizations and networks. For example, the CGIAR Research Program on Climate Change, Agriculture and Food Security (CCAFS) provides a toolbox to select climate-smart options (https://csa.guide/). Agroecological networks, such as the Community Agroecological Network (CAN), have established guidelines to carry out participatory action research (Méndez et al., 2017).
The framework counts in the lessons learned from successful cases on scaling agroecological practices (Mier et al., 2018). These cases stress the importance to foster farmer organization and external support as two key enabling factors, and to select effective and straightforward agroecological practices. One of the most compelling examples of scaling agroecological practices are agroecological farmer-to-farmer networks in Central America, Mexico, and Cuba (Table 1, Example 1). These networks show the importance of horizontal learning from farmer-to-farmer and through the establishment of dialogues between farmers and other actors (Holt-Giménez, 2006; Morris et al., 2016). Therefore, in addition to the four essential steps mentioned above, step 7 in our decision-framework on evaluation and learning is another important step in the diversification of farm systems.
The framework identifies insurance policies and market access as two additional enabling factors for on-farm diversification, in recognition of farmers' needs for enabling institutional environments to incentivize on-farm changes in crop and land management. Network structures for agricultural innovation for sustainable agriculture link farmer organizations to markets and insurance providers (Schut et al., 2014). We are not aware of successful policies to link insurance products to on-farm diversification, and we recommend policy-makers and practitioners to pilot these combinations.
The framework stresses the importance of understanding the goals of different farm household members and their diverse livelihood options and preferences. This provides the basis on which to establish a dialogue on diversifying farm systems, and allows to consider gender in the selection of diversification strategies. We stress this, because this may not always happen, resulting in a focus on profit-maximization in projects biased to narrow economic objectives or to poor linkage between recommended agroecological practices and the objectives of the different members of farm households.
To ensure that recommended practices align with farmers' economic objectives, we recommend practitioners and researchers to work with farmers in estimating the production costs and economic benefits of their existing farm systems in comparison with more diversified systems. Farmers are likely to determine the optimum extent of on-farm diversification by the balance between the labor input and other management costs associated with diversifying their farm systems, and the benefits from increased and more stable productivity leading to enhanced income and food security as a result of on-farm diversification.
Since labor constraints increase with climate change, it will be important to consider these increased labor costs in cost-benefit analysis and the implementation of diversification strategies. Recommended practices to diversify farm systems under climate change should therefore minimize extra labor, more so because of growing labor-scarcity due to rural-urban migration (Bacud et al., 2019). This fits well to the existing lesson in scaling agroecology to promote effective and straightforward agroecological practices (Holt-Giménez, 2001). When these practices minimize extra labor, then this will help to the successful implementation of diversification measures.
On-farm diversification strategies contribute effectively to CSA and SDG policies, which many governments aim to promote to enhance food security, climate change adaptation, and sustainable development (Lipper et al., 2014; Totin et al., 2018; Willett et al., 2019). On-farm diversification contributes less to climate change mitigation, which is another important component of CSA and SDG 13 on Climate Action. Although several on-farm diversification strategies, such as agroforestry or growing cover crops already address mitigation by sequestering carbon, this is not their primary goal when adapting farm systems to the adverse effects of global climate change. On-farm diversification in integrated CSA strategies should therefore be evaluated for their mitigation potential and when necessary combined with other mitigation strategies.
In some cases, on-farm diversification will not be sufficient to reduce the vulnerability of farmers to climate change (Harvey et al., 2014); on-farm diversification options simply do not save sufficient labor or sufficiently increase or stabilize net income. In these cases, off-farm diversification, such as seasonal labor in the non-agricultural sectors or a permanent exit from agriculture, may be a better option for farmers to adapt to climate change (Hansen et al., 2019).
Conclusions
On-farm diversification is a key component of a range of climate change adaptation and mitigations practices and technologies known collectively as CSA. Poorer farmers are particularly vulnerable to climate change and it is, hence, even more imperative that diversification options address the resources available to them and their aspirations. Increasing resources are being directed at CSA and we suggest following the seven steps presented in this paper as an approach to working with farmers for appropriate on-farm diversification as part of climate change adaptation and mitigation efforts. The seven steps provide a framework to identify appropriate diversification options in the context of farmers' agroecological and socio-economic conditions: (step 1) defining farmers' goals; (step 2) assessment of enabling factors; (step 3) assessment of disabling factors; (step 4) assessment of current and future climate-related production risks; (step 5) gap analysis of functional diversity; (step 6) selection of on-farm diversification options; and finally (step 7) evaluation and learning.
Governments often have few economic resources to put in force an agenda for CSA and, hence, network structures for agricultural innovation are vital for sustainable agriculture under climate change. Scale effects often favor monocultures. There are, however, several examples how food and feed demand in combination with adequate germplasm supply enables large numbers of farmers to diverse their farm systems and access markets. A successful example is diversified horticultural systems with high-value fruit and vegetable species for urban markets. Networks of agricultural innovation enable farmers to adopt diversification options by connecting with local, national, and international private companies, farmer organizations, public and private extension services, NGOs, as well as research institutes.
The key is to work with farmers in a participatory way and to prioritize their constraints, aspirations, and opportunities for on-farm diversification. A failure to do so, risks stymieing CSA efforts and ultimately perpetuating the vulnerability of those farmers who are often the target group of CSA. This would also result in CSA falling well short of its potential to contribute meaningfully to several of the SDGs including 13: Climate Action; SDG 1: No Poverty; SDG 2: Zero Hunger; and SDG 15 Life on Land.
Author Contributions
All authors listed have made a substantial, direct and intellectual contribution to the work, and approved it for publication.
Funding
This study was supported by Hivos, the Central American research platform on Production and Conservation in Partnership (PCP) and the CGIAR Research Programs Humidtropics, and Climate Change, Agriculture and Food Security (CCAFS), with support from CGIAR Fund and Donors. Funding for the World Vegetable Center's general research activities was provided by core donors: Republic of China (Taiwan), UK aid from the UK government, United States Agency for International Development (USAID), Australian Centre for International Agricultural Research (ACIAR), the Federal Ministry for Economic Cooperation and Development of Germany, Thailand, Philippines, Korea, and Japan.
Conflict of Interest
The authors declare that the research was conducted in the absence of any commercial or financial relationships that could be construed as a potential conflict of interest.
Acknowledgments
We thank Abigail Fallot from CIRAD, the editor, and the two reviewers for valuable comments in the development of this paper.
Supplementary Material
The Supplementary Material for this article can be found online at: https://www.frontiersin.org/articles/10.3389/fsufs.2020.00032/full#supplementary-material
References
Allen, C. R., Fontaine, J. J., Pope, K. L., and Garmestani, A. S. (2011). Adaptive management for a turbulent future. J. Environ. Manage. 92, 1339–1345. doi: 10.1016/j.jenvman.2010.11.019
Almekinders, C. J. M., Thiele, G., and Danial, D. L. (2007). Can cultivars from participatory plant breeding improve seed provision to small-scale farmers? Euphytica 153, 363–372. doi: 10.1007/s10681-006-9201-9
Altieri, M. A., and Merrick, L. C. (1987). In situ conservation of crop genetic resources through maintenance of traditional farming systems. Econ. Bot. 41, 86–96. doi: 10.1007/BF02859354
Altieri, M. A., Nicholls, C. I., Henao, A., and Lana, M. A. (2015). Agroecology and the design of climate change-resilient farming systems. Agron. Sustain. Dev. 35, 869–90. doi: 10.1007/s13593-015-0285-2
Anderson, P. K., Cunningham, A. A., Patel, N. G., Morales, F. J., Epstein, P. R., Daszak, P., et al. (2004). Emerging infectious diseases of plants: pathogen pollution, climate change and agrotechnology drivers. Trends Ecol. Evol. 19, 535–544. doi: 10.1016/j.tree.2004.07.021
Arbaoui, M., and Link, W. (2008). Effect of hardening on frost tolerance and fatty acid composition of leaves and stems of a set of faba bean (Vicia faba L.) genotypes. Euphytica 162, 211–219. doi: 10.1007/s10681-007-9521-4
Armengot, L., Barbieri, P., Andres, C., Milz, J., and Schneider, M. (2016). Cacao agroforestry systems have higher return on labor compared to full-sun monocultures. Agron. Sustain. Dev. 36:70. doi: 10.1007/s13593-016-0406-6
Asante, B. O., Villano, R. A., Patrick, I. W., and Battese, G. E. (2018). Determinants of farm diversification in integrated crop–livestock farming systems in Ghana. Renew. Agric. Food Syst. 33, 131–149. doi: 10.1017/S1742170516000545
Avelino, J., Cristancho, M., Georgiou, S., Pablo, I., Aguilar, L., Bornemann, G., et al. (2015). The coffee rust crises in Colombia and Central America (2008–2013): impacts, plausible causes and proposed solutions. Food Secur. 7, 303–321. doi: 10.1007/s12571-015-0446-9
Avelino, J., Romero-Gurdián, A., Cruz-Cuellar, H. F., and Declerck, F. A. J. (2012). Landscape context and scale differentially impact coffee leaf rust, coffee berry borer, and coffee root-knot nematodes. Ecol. Appl. 22, 584–596. doi: 10.1890/11-0869.1
Baca, M., Laderach, P., Haggar, J., Schroth, G, and Ovalle, O. (2014). An integrated framework for assessing vulnerability to climate change and developing adaptation strategies for coffee growing families in Mesoamerica. PLoS ONE. 9:e88463. doi: 10.1371/journal.pone.0088463
Bacon, C. M., Getz, C., Kraus, S., Montenegro, M., and Holland, K. (2012). The social dimensions of sustainability and change in diversified farming systems. Ecol Soc 17:41. doi: 10.5751/ES-05226-170441
Bacon, C. M., Sundstrom, W. A., Flores Gómez, M. E., Méndez, V. E., Santos, R., Goldoftas, B., et al. (2014). Explaining the “hungry farmer paradox”: smallholders and fair trade cooperatives navigate seasonality and change in Nicaragua's corn and coffee markets. Glob. Environ. Chang. 25, 133–149. doi: 10.1016/j.gloenvcha.2014.02.005
Bacud, E. S., Puskur, R., Nhat, T., Sander, B. O., and Luis, J. (2019). Rural outmigration—feminization—agricultural production nexus : case of Vietnam. Migr. Dev. 9, 1–25. doi: 10.1080/21632324.2019.1679962
Bailey-Serres, J., Lee, S. C., and Brinton, E. (2012). Waterproofing crops: effective flooding survival strategies. Plant Physiol. 160, 1698–1709. doi: 10.1104/pp.112.208173
Barnabás, B., Jäger, K., and Fehér, A. (2008). The effect of drought and heat stress on reproductive processes in cereals. Plant Cell Environ. 31:11–38. doi: 10.1111/j.1365-3040.2007.01727.x
Barradas, V. L., and Fanjul, L. (1986). Microclimatic characterization of shaded and opengrown coffee (Coffea arabica L.) plantations in Mexico. Agric. For. Meteorol. 38, 101–112. doi: 10.1016/0168-1923(86)90052-3
Bayala, J., Teklehaimanot, Z., and Ouedraogo, S. J. (2002). Millet production under pruned tree crowns in a parkland system in Burkina Faso. Agrofor. Syst. 54, 203–214. doi: 10.1023/A:1016058906682
Bebber, D. P., Ramotowski, M. A. T., and Gurr, S. J. (2013). Crop pests and pathogens move polewards in a warming world. Nat. Clim. Chang. 3, 985–988. doi: 10.1038/nclimate1990
Bell, A. R., Osgood, D. E., Cook, B. I., Anchukaitis, K. J., Mccarney, G. R., Greene, A. M., et al. (2013). Paleoclimate histories improve access and sustainability in index insurance programs. Glob. Environ. Chang. 23, 774–781. doi: 10.1016/j.gloenvcha.2013.03.003
Beltrame, D. M. O., Oliveira, C. N. S., Borelli, T., Hunter, D., Neves, C., De Andrade, R., et al. (2016). Diversifying institutional food procurement–opportunities and barriers for integrating biodiversity for food and nutrition in Brazil. Raizes 36, 55–69.
Beuchelt, T. D., Camacho Villa, C. T., Göhring, L., Rodríguez, V. M. H., Hellin, J., Sonde, K., et al. (2015). Social and income trade-offs of conservation agriculture practices on crop residue use in Mexico's central highlands. Agric. Syst. 134, 61–75. doi: 10.1016/j.agsy.2014.09.003
Binswanger-Mkhize, H. (2012). Is there too much hype about index-based agricultural insurance? J. Dev. Stud. 48, 187–200. doi: 10.1080/00220388.2011.625411
Birthal, P. S., Roy, D., and Negi, D. S. (2015). Assessing the impact of crop diversification on farm poverty in India. World Dev. 72, 70–92. doi: 10.1016/j.worlddev.2015.02.015
Bobojonov, I., Aw-Hassan, A., and Sommer, R. (2013). Index-based insurance for climate risk management and rural development in Syria. Clim. Dev. 6, 166–178. doi: 10.1080/17565529.2013.844676
Bondeau, A., Smith, P. C., Schaphoff, S., Zaehle, S., Lucht, W., Cramer, W., et al. (2007). Modelling the role of agriculture for the 20th century global terrestrial carbon balance. Glob. Chang. Biol. 13, 679–706. doi: 10.1111/j.1365-2486.2006.01305.x
Borchert, R. (1994). Soil and stem water storage determine phenology and ditribution of tropical dry forest trees. Ecology 75, 1437–1449. doi: 10.2307/1937467
Bozzano, M., Jalonen, R., Thomas, E., Loo, J., Boshier, D., Gallo, L., et al. (2014). Genetic Considerations in Ecosystem Restoration Using Native Tree Species. State of the World's Forest Genetic Resources–Thematic Study. Rome: FAO and Bioversity International.
Brooker, R. W., Bennett, A. E., Cong, W.-F., et al. (2015). Improving intercropping: a synthesis of research in agronomy, plant physiology and ecology. New Phytol. 206, 107–117. doi: 10.1111/nph.13132
Brookfield, H. (2001). Exploring Agrodiversity. New York, NY: Columbia University Press. doi: 10.7312/broo10232
Bryan, E., Deressa, T. T., Gbetibouo, G. A., and Ringler, C. (2009). Adaptation to climate change in Ethiopia and South Africa: options and constraints. Environ. Sci. Policy. 12, 413–426. doi: 10.1016/j.envsci.2008.11.002
Bybee-Finley, K., Ryan, M., Bybee-Finley, K. A., and Ryan, M. R. (2018). Advancing intercropping research and practices in industrialized agricultural landscapes. Agriculture 8:80. doi: 10.3390/agriculture8060080
Cairns, J. E., Crossa, J., Zaidi, P. H., Grudloyma, P., Sanchez, C., Araus, J. L., et al. (2013). Identification of drought, heat, and combined drought and heat tolerant donors in maize. Crop Sci. 53, 1335–1346. doi: 10.2135/cropsci2012.09.0545
Caramori, P. H., Androcioli Filho, A., and Leal, A. C. (1996). Coffee shade with Mimosa scabrella Benth. for frost protection in southern Brazil. Agrofor. Syst. 33, 205–214. doi: 10.1007/BF00055423
Carberry, P. S., Hochman, Z., McCown, R. L., Dalgliesh, N. P., Foale, M. A., Poulton, P. L., et al. (2002). The FARMSCAPE approach to decision support: farmers', advisers', researchers' monitoring, simulation, communication and performance evaluation. Agric. Syst. 74, 141–177. doi: 10.1016/S0308-521X(02)00025-2
Cardoso, J. A., Rincon, J., Jimenez, J. d. l. C., Noguera, D., and Rao, I. M. (2013). Morpho-anatomical adaptations to waterlogging by germplasm accessions in a tropical forage grass. AoB Plants 5:plt047. doi: 10.1093/aobpla/plt047
Carletto, C., Kirk, A., Winters, P. C., and Davis, B. (2010). Globalization and smallholders: the adoption, diffusion, and welfare impact of non-traditional export crops in Guatemala. World Dev. 38, 814–827. doi: 10.1016/j.worlddev.2010.02.017
Carter, M. R., Cheng, L., and Sarris, A. (2016). Where and how index insurance can boost the adoption of improved agricultural technologies. J. Dev. Econ. 118, 59–71. doi: 10.1016/j.jdeveco.2015.08.008
Cattivelli, L., Rizza, F., Badeck, F.-W., Mazzucotelli, E., Mastrangelo, A. M., Franciaa, E., et al. (2008). Drought tolerance improvement in crop plants: an integrated view from breeding to genomics. F. Crop Res. 105, 1–14. doi: 10.1016/j.fcr.2007.07.004
Ceccarelli, S. (2015). Efficiency of plant breeding. Crop Sci. 55:87. doi: 10.2135/cropsci2014.02.0158
Chakrabarti, S., and Kundu, A. (2009). Rural non-farm economy: a note on the impact of crop-diversification and land-conversion in India. Econ. Polit. Weekly 44, 69–75. doi: 10.2307/40278811
Chapman, R., and Tripp, R. (2003). Changing Incentives for Agricultural Extension–A Review of Privatised Extension in Practice. London: Agricultural Research and Extension Network Paper.
Chaudhury, M., Vervoort, J., Kristjanson, P., Ericksen, P., and Ainslie, A. (2013). Participatory scenarios as a tool to link science and policy on food security under climate change in East Africa. Reg. Environ. Chang. 13, 389–398. doi: 10.1007/s10113-012-0350-1
Cheng, A., Mayes, S., Dalle, G., Demissew, S., and Massawe, F. (2017). Diversifying crops for food and nutrition security–a case of teff. Biol. Rev. 92, 188–198. doi: 10.1111/brv.12225
Clement, C. R. (1999). 1492 and the loss of Amazonian crop genetic resources. I. The relation between domestication and human population decline. Econ. Botany 53, 188–202. doi: 10.1007/BF02866498
Coe, R., Sinclair, F., and Barrios, E. (2014). Scaling up agroforestry requires research “in” rather than “for” development. Curr. Opin. Environ. Sustain. 6, 73–77. doi: 10.1016/j.cosust.2013.10.013
Cong, W.-F., van Ruijven, J., Mommer, L., De Deyn, G. B., Berendse, F., and Hoffland, E. (2014). Plant species richness promotes soil carbon and nitrogen stocks in grasslands without legumes. J. Ecol. 102, 1163–1170. doi: 10.1111/1365-2745.12280
Coomes, O. T., McGuire, S. J., Garine, E., Caillon, S., McKey, D., Demeulenaere, E., et al. (2015). Farmer seed networks make a limited contribution to agriculture? Four common misconceptions. Food Policy 56, 41–50. doi: 10.1016/j.foodpol.2015.07.008
de Sousa, K., van Zonneveld, M., Holmgren, M., Kindt, R., and Ordonez, J. C. (2019). The future of coffee and cocoa agroforestry in a warmer Mesoamerica. Sci. Rep. 9:8828. doi: 10.1038/s41598-019-45491-7
Dick, W., Stoppa, A., and Anderson, J. (2011). Weather Index-Based Insurance in Agricultural Development: A Technical Guide. Rome, Italy: International Fund for Agricultural Development (IFAD).
Dinssa, F. F., Hanson, P., Dubois, T., Tenkouano, A., Stoilova, T., Hughes, J. d'A., et al. (2016). AVRDC–the World Vegetable Center's women-oriented improvement and development strategy for traditional African vegetables in sub-Saharan Africa. Eur. J. Hortic. Sci. 81, 91–105. doi: 10.17660/eJHS.2016/81.2.3
DiTomaso, J. M. (2000). Invasive weeds in rangelands: species, impacts, and management. Weed Sci. 48, 255–265. doi: 10.1614/0043-1745(2000)048[0255:IWIRSI]2.0.CO;2
Dumont, E. S., Bonhomme, S., Pagella, T. F., and Sinclair, F. L. (2017). Structured stakeholder engagement leads to development of more diverse and inclusive agroforestry options. Exp. Agric. 55, 1–23. doi: 10.1017/S0014479716000788
Dunne, J., Stouffer, R., and John, J. (2013). Reductions in labour capacity from heat stress under climate warming. Nat. Clim. Chang. 3, 563–566. doi: 10.1038/nclimate1827
Eakin, H. (2003). The social vulnerability of irrigated vegetable farming households in Central Puebla. J. Environ. Dev. 12, 414–429. doi: 10.1177/1070496503257733
Eakin, H. (2005). Institutional change, climate risk, and rural vulnerability: cases from central Mexico. World Dev. 33, 1923–1938. doi: 10.1016/j.worlddev.2005.06.005
Eakin, H., Benessaiah, K., Barrera, J. F., Bello, G. M. C., and Morales, H. (2012). Livelihoods and landscapes at the threshold of change: disaster and resilience in a Chiapas coffee community. Glob. Environ. Chang. 12, 475–488. doi: 10.1007/s10113-011-0263-4
Eakin, H. C., and Wehbe, M. B. (2009). Linking local vulnerability to system sustainability in a resilience framework: two cases from Latin America. Clim Change 93, 355–377. doi: 10.1007/s10584-008-9514-x
Ehlers, J. D., and Hall, A. E. (1997). Cowpea (Vigna unguiculata L. Walp.). F. Crop. Res. 53, 187–204. doi: 10.1016/S0378-4290(97)00031-2
El-Sharkawy, M. A. (2007). Physiological characteristics of cassava tolerance to prolonged drought in the tropics: implications for breeding cultivars adapted to seasonally dry and semiarid environments. Br. J. Plant Physiol. 19, 257–286. doi: 10.1590/S1677-04202007000400003
Erenstein, O. (2003). Smallholder conservation farming in the tropics and sub-tropics: a guide to the development and dissemination of mulching with crop residues and cover crops. Agric. Ecosyst. Environ. 100, 17–37. doi: 10.1016/S0167-8809(03)00150-6
Espitia Rangel, E., Villaseñor Mir, H. E., Huerta Espino, J., Salmerón Zamora, J. J., González Iñiguez, R. M., Osorio Alcalá, L., et al. (2007). Obsidiana, variedad de avena para la producción de grano y forraje en México. Agric. Técnica en México 33, 95–98.
Farnworth, C. R., Baudron, F., Andersson, J. A., Misiko, M., Badstue, L. B., Stirling, C. M., et al. (2016). Gender and conservation agriculture in East and Southern Africa: towards a research agenda. Int. J. Agric. Sustain. 14:142–165. doi: 10.1080/14735903.2015.1065602
Farooq, M., Nadeem, F., Gogoi, N., Ullah, A., Alghamdi, S. S., Nayyar, H., et al. (2017). Heat stress in grain legumes during reproductive and grain-filling phases. Crop. Pasture Sci. 68, 985–1005. doi: 10.1071/CP17012
Felker, P., Cannell, G. H., Clark, P. R., Osborn, J. F., and Nash, P. (1983). Biomass production of Prosopis species (mesquite), Leucaena, and other leguminous trees grown under heat/drought stress. For. Sci. 29: 592–606.
Figge, F. (2004). Applying portfolio theory to biodiversity. Biodivers. Conserv. 13, 827–849. doi: 10.1023/B:BIOC.0000011729.93889.34
Fresco, L., and Westphal, E. (1988). A hierarchical classification of farm systems. Exp. Agric. 24, 399–419. doi: 10.1017/S0014479700100146
Gallopín, G. C. (2006). Linkages between vulnerability, resilience, and adaptive capacity. Glob. Environ. Chang. 16, 293–303. doi: 10.1016/j.gloenvcha.2006.02.004
Galluzzi, G., Eyzaguirre, P., and Negri, V. (2010). Home gardens: neglected hotspots of agro-biodiversity and cultural diversity. Biodiversity Conserv. 19, 3635–3654. doi: 10.1007/s10531-010-9919-5
Gardner, K., and Lewis, D. (1996). Anthropology, Development and the Post-Modern Challenge. London, UK, Sterling, VA, USA: Pluto Press.
German, L. A., Kidane, B., and Shemdoe, R. (2006). Social and environmental trade-offs in tree species selection: a methodology for identifying niche incompatibilities in agroforestry. Environ. Dev. Sustain. 8, 535–552. doi: 10.1007/s10668-006-9054-3
Ghannoum, O. (2009). C4 photosynthesis and water stress. Ann. Bot. 103, 635–644. doi: 10.1093/aob/mcn093
Gil, J. D. B., Cohn, A. S., Duncan, J., Newton, P., and Vermeulen, S. (2017). The resilience of integrated agricultural systems to climate change. Wiley Interdiscip. Rev. Clim. Change 8:e461. doi: 10.1002/wcc.461
Gilbert, M., and Holbrook, N. (2011). Limitations to Crop Diversification for Enhancing the Resilience of Rain-Fed Subsistence Agriculture to Drought. Available online at: http://www.hks.harvard.edu/centers/cid/publications/faculty-working-papers/cid-working-paper-no.-228 (accessed August 8, 2015).
Giller, K. E., Witter, E., Corbeels, M., and Tittonell, P. (2009). Conservation agriculture and smallholder farming in Africa: the heretics' view. F Crop. Res. 114, 23–34. doi: 10.1016/j.fcr.2009.06.017
Gliessman, S. R. (2014). Agroecology—The Ecology of Sustainable Food Systems. 3rd Edn. Boca Raton, FL: CRC Press/Taylor and Francis.
Goland, C. (1993). Agricultural risk management through diversity: field scattering in Cuyo Cuyo, Peru. Cult. Agric. 13, 8–13. doi: 10.1525/cuag.1993.13.45-46.8
Gomathi, R., Gururaja Rao, P. N., Chandran, K., and Selvi, A. (2014). Adaptive responses of sugarcane to waterlogging stress: an over view. Sugar Tech 17, 325–338. doi: 10.1007/s12355-014-0319-0
Graefe, S., Dufour, D., van Zonneveld, M., Rodriguez, F., and Gonzalez, A. (2012). Peach palm (Bactris gasipaes) in tropical Latin America: implications for biodiversity conservation, natural resource management and human nutrition. Biodiversity Conserv. 22, 269–300. doi: 10.1007/s10531-012-0402-3
Graham, P. H., and Vance, C. P. (2003). Legumes: importance and constraints to greater use. Plant Physiol. 131, 872–877. doi: 10.1104/pp.017004
Greatrex, H., Hansen, J., and Garvin, S. (2015). Scaling up Index Insurance for Smallholder Farmers: Recent Evidence and Insights. Copenhagen. Available online at: https://hdl.handle.net/10568/53101
Grothmann, T., and Patt, A. (2005). Adaptive capacity and human cognition: the process of individual adaptation to climate change. Glob. Environ. Change 15, 199–213. doi: 10.1016/j.gloenvcha.2005.01.002
Hansen, J. W., Hellin, J., Rosenstock, T., Fisher, E., Cairns, J., Stirling, C. L., et al. (2019). Climate risk management and rural poverty reduction. Agric. Syst. 172, 28–46. doi: 10.1016/j.agsy.2018.01.019
Hart, C. E., Hayes, D. J., and Babcock, B. A. (2006). Insuring eggs in baskets: should the government insure individual risks? Can. J. Agric. Econ. 54, 121–137. doi: 10.1111/j.1744-7976.2006.00041.x
Harvey, C. A., Rakotobe, Z. L., Rao, N. S., Dave, R., Razafimahatratra, H., Rabarijohn, R. H., et al. (2014). Extreme vulnerability of smallholder farmers to agricultural risks and climate change in Madagascar. Phil. Trans. R Soc. 369:20130089. doi: 10.1098/rstb.2013.0089
Hellin, J. (2012). Agricultural extension, collective action and innovation systems: lessons on network brokering from Peru and Mexico. J. Agric. Educ. Ext. 18, 141–159. doi: 10.1080/1389224X.2012.655967
Hellin, J., Cox, R., and López-Ridaura, S. (2017). Maize diversity, market access, and poverty reduction in the Western highlands of Guatemala. Mt. Res. Dev. 37, 188–197. doi: 10.1659/MRD-JOURNAL-D-16-00065.1
Hellin, J., and Dixon, J. (2008). Operationalising participatory research and farmer-to-farmer extension : the Kamayoq in Peru. Dev. Pract. 18, 627–632. doi: 10.1080/09614520802181889
Hellin, J., and Higman, S. (2005). Crop diversity and livelihood security in the Andes. Dev. Pract. 15, 165–174. doi: 10.1080/09614520500041344
Hellin, J., Welchez, L. A., and Cherrett, I. (1999). The Quezungual system: an indigenous agroforestry system from western Honduras. Agrofor. Syst. 46, 229–237. doi: 10.1023/A:1006217201200
Holmgren, M., Stapp, P., Dickman, C. R., Gracia, C. A., Graham, S., Gutierrez, J., et al. (2006). Extreme climatic events shape arid and semiarid ecosystems. Front. Ecol. Environ. 4, 87–95. doi: 10.1890/1540-9295(2006)004[0087:ECESAA]2.0.CO;2
Holt-Giménez, E. (2002). Measuring farmers' agroecological resistance after Hurricane Mitch in Nicaragua: a case study in participatory, sustainable land management impact monitoring. Agric. Ecosyst. Environ. 93, 87–105. doi: 10.1016/S0167-8809(02)00006-3
Holt-Giménez, E. (2006). Campesino a campesino: voices from Latin America' s farmer to farmer movement for sustainaible agriculture voices from Latin america' s farmer to farmer. New York, NY: Food first books.
Hubbard, D. W. (2014). “Preference and attitudes: The softer side of measurement,” in How to Measure Anything: Finding the Value of Intangibles in Business (Hoboken, NJ: John Wiley & Sons, Inc.), 203–220. Available online at: https://books.google.com/books?hl=en&lr=&id=EAPXAgAAQBAJ&oi=fnd&pg=PA175&dq=hubbard+how+to+measure+anything&ots=Cqcet1CqC7&sig=95gsOZI3RuRzHwu3eqdHOOtnQPs (accessed August 8, 2015).
Immink, M. D. C., and Alarcon, J. A. (1991). Household food security, nutrition, and crop diversification among smallholder farmers in the highlands of Guatemala. Ecol. Food Nutr. 25, 287–305. doi: 10.1080/03670244.1991.9991177
Isakson, S. R. (2009). No hay ganancia en la milpa: the agrarian question, food sovereignty, and the on-farm conservation of agrobiodiversity in the Guatemalan highlands. J. Peasant Stud. 36, 725–759. doi: 10.1080/03066150903353876
Iseki, K., Takahashi, Y., Muto, C., Naito, K., and Tomooka, N. (2018). Diversity of drought tolerance in the genus Vigna. Front. Plant Sci. 9:729. doi: 10.3389/fpls.2018.00729
Jacobi, J., Rist, S., and Altieri, M. A. (2017). Incentives and disincentives for diversified agroforestry systems from different actors' perspectives in Bolivia. Int. J. Agric. Sustain. 15, 365–379. doi: 10.1080/14735903.2017.1332140
Jarvis, D. I., Brown, A. H. D., Cuong, P. H., Latournerie-Moreno, L., Gyawali, S., Tanto, T., et al. (2008). A global perspective of the richness and evenness of traditional crop-variety diversity maintained by farming communities. Proc. Natl. Acad. Sci. USA. 105, 5326–5331. doi: 10.1073/pnas.0803431105
Jarvis, D. I., Hodgkin, T., Sthapit, B. R., Fadda, C., and Lopez-Noriega, I. (2011). An heuristic framework for identifying multiple ways of supporting the conservation and use of traditional crop varieties within the agricultural production system. Crit. Rev. Plant Sci. 30, 125–176. doi: 10.1080/07352689.2011.554358
Joshi, P. K., Gulati, A., Birthal, P. S., and Tewari, L. (2004). Agriculture diversification in South Asia: patterns, determinants and policy implications. Econ. Polit. Wkly. 39, 2457–2467.
Kahn, F. (1991). Palms as key swamp forest resources in Amazonia. For. Ecol. Manage. 38, 133–142. doi: 10.1016/0378-1127(91)90139-M
Khoury, C. K., Bjorkman, A. D., Dempewolf, H., Ramirez-Villegas, J., Guarino, L., Jarvis, A., et al. (2014). Increasing homogeneity in global food supplies and the implications for food security. Proc. Natl. Acad. Sci. U.S.A. 111, 4001–4006. doi: 10.1073/pnas.1313490111
Knoke, T., Paul, C., Härtl, F., Castro, L. M., Calvas, B., and Hildebrandt, P. (2015). Optimizing agricultural land-use portfolios with scarce data–a non-stochastic model. Ecol. Econ. 120, 250–259. doi: 10.1016/j.ecolecon.2015.10.021
Kort, J. (1988). Benefits of windbreaks to field and forage crops. Agric. Ecosyst. Environ. 22–23, 165–90. doi: 10.1016/0167-8809(88)90017-5
Kremen, C., Iles, A., and Bacon, C. (2012). Diversified farming systems: an agroecological, systems-based alternative to modern industrial agriculture. Ecol. Soc. 17:44. doi: 10.5751/ES-05103-170444
Lavorel, S., and Garnier, E. (2002). Predicting changes in community composition and ecosystem functioning from plant traits: revisting the Holy Grail. Funct. Ecol. 16, 545–556. doi: 10.1046/j.1365-2435.2002.00664.x
Lawin, K. G., and Tamini, L. D. (2017). “Risk preferences and crop diversification amongst smallholder farmers in Burkina Faso,” in Canadian Agricultural Economics Society (Montreal, QC: Canadian Agricultural Economics Society), 1–27. Available online at: https://ideas.repec.org/p/ags/caes17/258058.html (accessed March 16, 2019).
Lawrence, D., and Vandecar, K. (2015). Effects of tropical deforestation on climate and agriculture. Nat. Clim. Change 5, 27–36. doi: 10.1038/nclimate2430
Lee, D. (2005). Agricultural sustainability and technology adoption: issues and policies for developing countries. Am. J. Agric. Econ. 87, 1325–1334. doi: 10.1111/j.1467-8276.2005.00826.x
Liebman, M., and Dyck, E. (2007). Crop rotation and intercropping strategies for weed management. Ecol. Appl. 3, 92–122. doi: 10.2307/1941795
Lin, B. B. (2007). Agroforestry management as an adaptive strategy against potential microclimate extremes in coffee agriculture. Agric. For. Meteorol. 144, 85–94. doi: 10.1016/j.agrformet.2006.12.009
Lipper, L., Thornton, P., Campbell, B. M., Baedeker, T., Braimoh, A., Bwalya, M., et al. (2014). Climate-smart agriculture for food security. Nat. Clim. Change 4, 1068–1072. doi: 10.1038/nclimate2437
Lobell, D. B., Burke, M. B., Tebaldi, C., Mastrandrea, M. D., Falcon, W., and Naylor, R. L. (2008). Prioritizing climate change adaptation needs for food security in 2030. Science 319, 607–610. doi: 10.1126/science.1152339
Lopes, M. S., Araus, J. L., van Heerden, P. D., and Foyer, C. H. (2011). Enhancing drought tolerance in C4 crops. J. Exp. Bot. 62, 3135–3153. doi: 10.1093/jxb/err105
Louwaars, N. P., and de Boef, W. S. (2012). Integrated seed sector development in Africa: a conceptual framework for creating coherence between practices, programs, and policies. J. Crop. Improv. 26, 39–59. doi: 10.1080/15427528.2011.611277
Lowder, S. K., Skoet, J., and Raney, T. (2016). The number, size, and distribution of farms, smallholder farms, and family farms worldwide. World Dev. 87, 16–29. doi: 10.1016/j.worlddev.2015.10.041
MacDougall, A. S., McCann, K. S., Gellner, G., and Turkington, R. (2013). Diversity loss with persistent human disturbance increases vulnerability to ecosystem collapse. Nature 494, 86–89. doi: 10.1038/nature11869
Malézieux, E., Crozat, Y., Dupraz, C., Laurans, M., Makowski, D., Ozier-Lafontaine, H., et al. (2009). Mixing plant species in cropping systems: concepts, tools and models. A review. Agron. Sustain. Dev. 29, 43–62. doi: 10.1051/agro:2007057
Maqbool, A., Shafiq, S., and Lake, L. (2010). Radiant frost tolerance in pulse crops—a review. Euphytica 172, 1–12. doi: 10.1007/s10681-009-0031-4
Marshall, D. R. (1977). The advantages and hazards of genetic homogeneity. Ann. N. Y. Acad. Sci. 287, 1–20. doi: 10.1111/j.1749-6632.1977.tb34226.x
Matsuda, M. (2013). Upland farming systems coping with uncertain rainfall in the central dry zone of Myanmar: how stable is indigenous multiple cropping under semi-arid conditions? Hum. Ecol. 41, 927–936. doi: 10.1007/s10745-013-9604-x
Matta, R. (2013). Valuing native eating: the modern roots of Peruvian food heritage. Anthropol. Food S8. doi: 10.4000/aof.7361
Mazón, N., Peralta, E., Murillo, Á. R, Rivera, M., Guzman, A., Nicklin, C., et al. (2016). It's not just the technology, it's the surrounding system: how researchers in Ecuador found ways to make themselves useful to farmers through quinoa and lupin seed systems. Exp. Agric. 55, 107–124. doi: 10.1017/S0014479716000442
McCord, P. F., Cox, M., Schmitt-Harsh, M., and Evans, T. (2015). Crop diversification as a smallholder livelihood strategy within semi-arid agricultural systems near Mount Kenya. Land use policy 42:738–750. doi: 10.1016/j.landusepol.2014.10.012
Meldrum, G., Mijatović, D., Rojas, W., Flores, J., Pinto, M., Mamani, G., et al. (2018). Climate change and crop diversity: farmers' perceptions and adaptation on the Bolivian Altiplano. Environ. Dev. Sustain. 20, 703–730. doi: 10.1007/s10668-016-9906-4
Méndez, V., Caswell, M., Gliessman, S., and Cohen, R. (2017). Integrating agroecology and participatory action research (PAR): lessons from Central America. Sustainability 9:705. doi: 10.3390/su9050705
Mengistu, D. K., Kidane, Y. G., Catellani, M., Frascaroli, E., Fadda, C., Pè, M. E., et al. (2016). High-density molecular characterization and association mapping in Ethiopian durum wheat landraces reveals high diversity and potential for wheat breeding. Plant Biotechnol. J. 14, 1800–1812. doi: 10.1111/pbi.12538
Mercer, K. L., and Perales, H. R. (2010). Evolutionary response of landraces to climate change in centers of crop diversity. Evol. Appl. 3, 480–493. doi: 10.1111/j.1752-4571.2010.00137.x
Midega, C. A. O., Pittchar, J. O., Pickett, J. A., Hailu, G., and Khan, Z. (2018). A climate-adapted push-pull system effectively controls fall armyworm, Spodoptera frugiperda (J. E. Smith), in maize in East Africa. Crop Prot. 105, 10–15. doi: 10.1016/j.cropro.2017.11.003
Mier, M., Giménez Cacho, T., Giraldo, O. F., Aldasoro, M., Morales, H., Ferguson, G. B., et al. (2018). Bringing agroecology to scale: key drivers and emblematic cases. Agroecol. Sustain. Food Syst. 42, 637–665. doi: 10.1080/21683565.2018.1443313
Miranda, M. J., and Farrin, K. (2012). Index insurance for developing countries. Appl. Econ. Perspect Policy 34, 391–427. doi: 10.1093/aepp/pps031
Mizrahi, Y., Raveh, E., and Yossov, E. (2007). “New fruit crops with high water use efficiency,” in Issues in New Crops and New Uses. eds J. Janick and A. Whipkey (Alexandria, VA: ASHS Press), 216–222.
Morris, K., Méndez, V., van Zonneveld, M., and Gerlicz, A. (2016). Agroecology and climate change resilience: in smallholder coffee agroecosystems of Central America. Burlington. Available online at: https://cgspace.cgiar.org/handle/10568/78410 (accessed March 11, 2018).
Mousavi-Derazmahalleh, M., Bayer, P. E., Hane, J. K., Valliyodan, B., Nguyen, H. T., Nelson, M. N., et al. (2019). Adapting legume crops to climate change using genomic approaches. Plant Cell Environ. 42, 6–19. doi: 10.1111/pce.13203
Murgueitio, E., Calle, Z., Uribe, F., Calle, A., and Solorio, B. (2011). Native trees and shrubs for the productive rehabilitation of tropical cattle ranching lands. For. Ecol. Manage 261, 1654–1663. doi: 10.1016/j.foreco.2010.09.027
Myers, S. S., Smith, M. R., Guth, S., Golden, C. D., Vaitla, B., Mueller, N. D., et al. (2017). Climate change and global food systems: potential impacts on food security and undernutrition. Annu. Rev. Public Health 38, 259–277. doi: 10.1146/annurev-publhealth-031816-044356
Nabhan, G. (2013). Growing Food in a Hotter, Drier Land: Lessons from Desert Farmers on Adapting to Climate Uncertainty. White River Junction: Chelsea Green Publishing.
National Research Council (1989). Lost Crops of the Incas: Little known plants of the Andes Promise for Worldwide Cultivation. Washington, DC: National Academy Press.
Nayyar, H., and Gupta, D. (2006). Differential sensitivity of C3 and C4 plants to water deficit stress: association with oxidative stress and antioxidants. Environ. Exp. Bot. 58, 106–113. doi: 10.1016/j.envexpbot.2005.06.021
Ochieng, J., Schreinemachers, P., Ogada, M., Dinssa, F. F., Barnos, W., Mndigafg, H., et al. (2019). Adoption of improved amaranth varieties and good agricultural practices in East Africa. Land Use Policy 83, 187–194. doi: 10.1016/j.landusepol.2019.02.002
Ortiz, C., and Cardemil, L. (2001). Heat-shock responses in two leguminous plants: a comparative study. J. Exp. Bot. 52, 1711–1719. doi: 10.1093/jxb/52.361.1711
Padulosi, S., Eyzaquirre, P., and Hodgkin, T. (1999). “Challenges and strategies in promoting conservation and use of neglected and underutilized crop species.,” in Perspectives on New Crops and New Uses. ed J. Janick (Alexandria, VA: ASHS Press).
Padulosi, S., Heywood, V., Hunter, D., and Jarvis, A. (2011). “Underutilized species and climate change: current status and outlook,” in Crop adaptation to Climate Change, eds S. S. Yadav, R. J. Redden, J. L. Hatfield, H. Lotze-Campen, and A. Hall (Oxford: Wiley-Blackwell), 507–521. doi: 10.1002/9780470960929.ch35
Padulosi, S., Hodgkin, T., Williams, J., International, N. H.-C., and Wallingford, U. (2002). “Underutilized crops: trends, challenges and opportunities in the 21st century, managing plant genetic diversity,” in Managing Plant Genetic Diversity, eds J. M. M. Engels, V. R. Rao, A. H. D. Brown, and M. T. Jackson (Wallingford: CAB International), 323–338. doi: 10.1079/9780851995229.0323
Parolin, P. (2009). Submerged in darkness: adaptations to prolonged submergence by woody species of the Amazonian floodplains. Ann. Bot. 103, 359–376. doi: 10.1093/aob/mcn216
Peters, C., and Vásquez, A. (1987). Estudios ecológicos de camu-camu (Myrciaria dubia). I. Producción de frutos en poblaciones naturales. Acta Amaz. 17, 161–188. doi: 10.1590/1809-43921987171174
Philpott, S., Lin, B., Jha, S., and Brines, S. (2008). A multi-scale assessment of hurricane impacts on agricultural landscapes based on land use and topographic features. Agric. Ecosyst. Environ. 128, 12–20. doi: 10.1016/j.agee.2008.04.016
Pingali, P. (1992). Diversifying Asian Rice-Farming Systems: A deterministic Paradigm. Manila. Available online at: http://citeseerx.ist.psu.edu/viewdoc/download?doi=10.1.1.898.1828&rep=rep1&type=pdf#page=117 (accessed March 16, 2019).
Pingali, P. (2007). Westernization of Asian diets and the transformation of food systems: implications for research and policy. Food Policy 32, 281–298. doi: 10.1016/j.foodpol.2006.08.001
Pingali, P. (2012). Green revolution: impacts, limits, and the path ahead. Proc. Natl. Acad. Sci. U. S. A. 109, 12302–12308. doi: 10.1073/pnas.0912953109
Prieto, I., Violle, C., Barre, P., Durand, J. L., Ghesquiere, M., and Litrico, I. (2015). Complementary effects of species and genetic diversity on productivity and stability of sown grasslands. Nat. Plants 1:15033. doi: 10.1038/nplants.2015.33
Pulwarty, R. S., and Sivakumar, M. V. K. (2014). Information systems in a changing climate: early warnings and drought risk management. Weather Clim. Extrem. 3, 14–21. doi: 10.1016/j.wace.2014.03.005
Raintree, J. B., and Warner, K. (1986). Agroforestry pathways for the intensification of shifting cultivation. Agrofor. Syst. 4, 39–54. doi: 10.1007/BF01834701
Ratnadass, A., Fernandes, P., Avelino, J., and Habib, R. (2011). Plant species diversity for sustainable management of crop pests and diseases in agroecosystems: a review. Agron. Sustain. Dev. 32, 273–303. doi: 10.1007/s13593-011-0022-4
Richardson, D. M. (1998). Forestry trees as invasive aliens. Conserv. Biol. 12, 18–26. doi: 10.1046/j.1523-1739.1998.96392.x
Rizza, F., Pagani, D., Stanca, A. M., and Cattivelli, L. (2001). Use of chlorophyll fluorescence to evaluate the cold acclimation and freezing tolerance of winter and spring oats. Plant Breed. 120, 389–396. doi: 10.1046/j.1439-0523.2001.00635.x
Rosenzweig, C., Iglesius, A., Epstein, P. R., and Chivian, E. (2001). Climate change and extreme weather events–implications for food production, plant diseases, and pests. Glob. Change Hum. Heal. 2, 90–104. doi: 10.1023/A:1015086831467
Rowland, D., Blackie, R., Powell, B., Houria, D., Barbara, V., Ickowitz, A., et al. (2015). Direct contributions of dry forests to nutrition: a review. Int. For. Rev. 17, 45–53. doi: 10.1505/146554815815834804
Saenz, M., and Thompson, E. (2017). Gender and policy roles in farm household diversification in Zambia. World Dev. 89, 152–169. doi: 10.1016/j.worlddev.2016.08.007
Sairam, R. K., Kumutha, D., Ezhilmathi, K., Deshmukh, P. S., and Srivastava, G. C. (2008). Physiology and biochemistry of waterlogging tolerance in plants. Biol. Plant 52, 401–412. doi: 10.1007/s10535-008-0084-6
Salazar-Barrientos, L. de L., Magaña-Magaña, M. Á., Aguilar-Jiménez, A. N., and Ricalde-Pérez, M. F. (2016). Factores socioeconómicos asociados al aprovechamiento de la agrobiodiversidad de la milpa en Yucatán. Ecosistemas y Recur. Agropecu. 3, 391–400.
Saxena, N. C. (1991). Crop losses and their economic implications due to growing of Eucalyptus on field bunds–a pilot study. Agrofor. Syst. 16, 231–245. doi: 10.1007/BF00119320
Schattman, R., Mendez, E., Westdijk, K., Caswell, M., Conner, D., Koliba, C. J., et al. (2015). “Vermont agricultural resilience in a changing environment: a transdisciplinary and participatory action reseach process,” in Archeology, Ecosystems, and Sustainability, ed N. Benkeblia (Boca Raton, FL: CRC Press/Taylor and Francis), 325–346. doi: 10.1201/b17775-17
Schluter, U. B., Furch, B., and Joly, C. A. (1993). Physiological and anatomical adaptations by young Astrocaryum Jauari Mart (Arecaceae) in periodically inundated biotopes of central Amazonia. Biotropica 25, 384–396. doi: 10.2307/2388862
Schreinemachers, P., Rao, K. P. C., Easdown, W., Hanson, P., and Kumar, S. (2017a). The contribution of international vegetable breeding to private seed companies in India. Genet. Resour. Crop Evol. 64, 1037–1049. doi: 10.1007/s10722-016-0423-y
Schreinemachers, P., Sequeros, T., and Lukumay, P. J. (2017b). International research on vegetable improvement in East and Southern Africa: adoption, impact, and returns. Agric. Econ. 48, 707–717. doi: 10.1111/agec.12368
Schreinemachers, P., Simmons, E. B., and Wopereis, M. C. S. (2018). Tapping the economic and nutritional power of vegetables. Glob. Food Sec. 16, 36–45. doi: 10.1016/j.gfs.2017.09.005
Schroth, G., and Ruf, F. (2014). Farmer strategies for tree crop diversification in the humid tropics. A review. Agron. Sustain. Dev. 1, 139–154. doi: 10.1007/s13593-013-0175-4
Schultze-Kraft, R., Rao, I. M., Peters, M., Clements, R. J., Bai, C., Liu, G., et al. (2018). Tropical forage legumes for environmental benefits: an overview. Trop. Grasslands-Forrajes Trop. 6:1. doi: 10.17138/TGFT(6)1-14
Schut, M., Klerkx, L., Rodenburg, J., Kayeke, J., Ast, A., Bastiaans, L., et al. (2014). RAAIS: Rapid Appraisal of Agricultural Innovation Systems (part I). A diagnostic tool for integrated analysis of complex problems and innovation capacity. Agric. Syst. 132, 1–11. doi: 10.1016/j.agsy.2014.08.009
Senay, G. B., Velpuri, N. M., Bohms, S., Budde, M. E., Young, C. J., Rowland, J., et al. (2015). “Drought monitoring and assessment: remote sensing and modeling approaches for the famine early warning systems network,” in Hydro-Meteorological Hazards, Risks, and Disasters, eds. J. F. Shroder, P. Paron, and G. Di Baldassarre (Amsterdam: Elsevier), 233–262. doi: 10.1016/B978-0-12-394846-5.00009-6
Shaw, M. W., and Osborne, T. M. (2011). Geographic distribution of plant pathogens in response to climate change. Plant Pathol. 60, 31–43. doi: 10.1111/j.1365-3059.2010.02407.x
Shiferaw, B. A., Okello, J., and Reddy, R. V. (2009). Adoption and adaptation of natural resource management innovations in smallholder agriculture: reflections on key lessons and best practices. Environ. Dev. Sustain. 11, 601–619. doi: 10.1007/s10668-007-9132-1
Somarriba, E. (1992). Revisiting the past: an essay on agroforestry definition. Agrofor. Syst. 19, 233–240. doi: 10.1007/BF00118781
Spielman, D. J., Ekboir, J., Davis, K., and Ochieng, C. M. O. (2008). An innovation systems perspective on strengthening agricultural education and training in sub-Saharan Africa. Agric. Syst. 98, 1–9. doi: 10.1016/j.agsy.2008.03.004
Sthapit, B., Lamers, H., and Rao, R. (2013). Custodian Farmers of Agricultural Biodiversity: Selected Profiles from South and South East Asia. New Delhi: Bioversity International.
Stoilova, T., van Zonneveld, M., Roothaert, R., and Schreinemachers, P. (2019). Connecting genebanks to farmers in East Africa through the distribution of vegetable seed kits. Plant Genet. Resour. Charact. Util. 17, 1–4. doi: 10.1017/S1479262119000017
Subbarao, G. V., Johansen, C., Slinkard, A. E., et al. (1995). Strategies for improving drought resistance in grain legumes. CRC. Crit. Rev. Plant Sci. 14:469–523. doi: 10.1080/07352689509701933
Tamang, B., Andreu, M. G., and Rockwood, D. L. (2010). Microclimate patterns on the leeside of single-row tree windbreaks during different weather conditions in Florida farms: implications for improved crop production. Agrofor. Syst. 79, 111–122. doi: 10.1007/s10457-010-9280-4
Tester, M., and Langridge, P. (2010). Breeding technologies to increase crop production in a changing world. Science 327, 818–822. doi: 10.1126/science.1183700
Teuling, A. J., Seneviratne, S. I., Stöckli, R., Reichstein, M., Moors, E., Ciais, P., et al. (2010). Contrasting response of European forest and grassland energy exchange to heatwaves. Nat. Geosci. 3, 722–727. doi: 10.1038/ngeo950
Thomas, D. S. G., Twyman, C., Osbahr, H., and Hewitson, B. C. (2007). Adaptation to climate change and variability: Farmer responses to intra-seasonal preciptation trends in South Africa. Clim. Change 83, 301–322. doi: 10.1007/s10584-006-9205-4
Tompkins, E. L., and Adger, W. N. (2004). Does adaptive management of natural resources enhance resilience to climate change? Ecol. Soc. 9, 1–14. doi: 10.5751/ES-00667-090210
Totin, E., Segnon, A., Schut, M., Affognon, H., Zougmore, R., Rosenstock, T., et al. (2018). Institutional perspectives of climate-smart agriculture: a systematic literature review. Sustainability 10:1990. doi: 10.3390/su10061990
Trouche, G., Hocdé, H., Aguirre Acuña, S., Martínez Sánchez, F., and Gutiérrez Palacios, N. (2006). Dinámicas campesinas y fitomejoramiento participativo: el caso de los sorgos blancos en la región norte de Nicaragua. Agron. Mesoam. 17:407. doi: 10.15517/am.v17i3.5175
Tucker, C. M., Eakin, H., and Castellanos, E. J. (2010). Perceptions of risk and adaptation: coffee producers, market shocks, and extreme weather in Central America and Mexico. Glob. Environ. Change 20, 23–32. doi: 10.1016/j.gloenvcha.2009.07.006
Turmel, M.-S., Speratti, A., Baudron, F., Verhulst, N., Govaerts, B., et al. (2015). Crop residue management and soil health: a systems analysis. Agric. Syst. 134, 6–16. doi: 10.1016/j.agsy.2014.05.009
Turvey, C. G. (2012). Whole farm income insurance. J. Risk Insur. 79, 515–540. doi: 10.1111/j.1539-6975.2011.01426.x
Umali-Deininger, D. (1997). Public and private agricultural extension: partners or rivals? World Bank Res. Obs. 12, 203–224. doi: 10.1093/wbro/12.2.203
Urwin, K., and Jordan, A. (2008). Does public policy support or undermine climate change adaptation? Exploring policy interplay across different scales of governance. Glob. Environ. Change 18, 180–191. doi: 10.1016/j.gloenvcha.2007.08.002
van de Fliert, E., and Braun, A. R. (2002). Conceptualizing integrative, farmer participatory research for sustainable agriculture : from opportunities to impact. Agric. Hum. Values 19, 25–38. doi: 10.1023/A:1015081030682
van Etten, J., Beza, E., Calderer, L., van Duijvebdijk, K., Fadda, C., Fantahun, B., et al. (2016). First experiences with a novel farmer citizen science approach: crowdsourcing participatory variety selection through on-farm triadic comparisons of technologies (tricot). Exp. Agric. 55, 1–22. doi: 10.1017/S0014479716000739
van Etten, J., de Sousa, K., Aguilar, A., Aguirrez, M. B., Coto, A., Dell'Acqua, M., et al. (2019). Crop variety management for climate adaptation supported by citizen science. Proc. Natl. Acad. Sci. U. S. A. 116, 4194–4199. doi: 10.1073/pnas.1813720116
Vermeulen, S., Challinor, A. J., Thornton, P. K., Eriyagama, N., Kinyangi, J., Laderach, P., et al. (2013). Addressing uncertainty in adaptation planning for agriculture. Proc. Natl. Acad. Sci. U. S. A. 110, 8357–8362. doi: 10.1073/pnas.1219441110
Vermeulen, S. J., Aggarwal, P. K., Ainslie, A., Angelone, C., Campbell, B. M. S., Andrew, J., et al. (2012). Options for support to agriculture and food security under climate change. Environ. Sci. Policy 15, 136–144. doi: 10.1016/j.envsci.2011.09.003
Vernooy, R., Sthapit, B., Otieno, G., Shrestha, P., and Gupta, A. (2017). The roles of community seed banks in climate change adaption. Dev. Pract. 27, 316–327. doi: 10.1080/09614524.2017.1294653
Vigouroux, Y., Mariac, C., de Mita, S., Pham, J. L., Gérard, B., Kapran, I., et al. (2011). Selection for earlier flowering crop associated with climatic variations in the Sahel. PLoS ONE 6:e19563. doi: 10.1371/journal.pone.0019563
Vohman, E., and Monro, A. K. (2011). Healthy kids, healthy forests: restoring mayanut agroecosystems for a healthy and prosperous future for neotropical communities. in Contribution of Ecosystem Restoration to the Objectives of the CBD and a Healthy Planet for All People, eds. J. Grekin and J. Mulongoy (Montreal, QC: Secretariat of the Convention on Biological Diversity), 104–106.
Waha, K., van Wijk, M. T., Fritz, S., See, L., Thornton, P. K., Wichern, J., et al. (2018). Agricultural diversification as an important strategy for achieving food security in Africa. Glob. Change Biol. 24, 3390–3400. doi: 10.1111/gcb.14158
Wahid, A., Gelani, S., Ashraf, M., and Foolad, M. R. (2007). Heat tolerance in plants: an overview. Environ. Exp. Bot. 61, 199–223. doi: 10.1016/j.envexpbot.2007.05.011
Waraich, E., Ahmad, R., Saifullah, U., Ashraf, M. Y., Ehsanullah, et al. (2011). Role of mineral nutrition in alleviation of drought stress in plants. Aust. J. Crop Sci. 5, 764–777.
Warburton, H., and Martin, A. (1999). Local people's knowledge in natural resources research. Socio-economic methodologies for natural resources research. Chatham.
Webb, M., Chary, A., de Vries, T. T., Davis, S., and Dykstra, M. (2016). Exploring mechanisms of food insecurity in indigenous agricultural communities in Guatemala: a mixed methods study. BMC Nutr. 2:55. doi: 10.1186/s40795-016-0091-5
Werners, S., Erdélyi, É., and Supit, I. (2011). “Use of modern portfolio theory to evaluate diversification of agricultural land use as an adaptation to climate risks in the Tisza river basin,” in Climate Change Adaptation in Developed Nations, eds. J. D. Ford and L. Berrang-Ford (Dordrecht: Springer Netherlands), 371–383. doi: 10.1007/978-94-007-0567-8_27
Werners, S., and Incerti, F. (2007). “Diversification of agricultural crops to adapt to climate change in the Guadiana River Basin,” in Proceedings from the International Conference on Climate Change, Hong Kong.
Willett, W., Rockström, J., Loken, B., Springmann, M., Lang, T., Vermeulen, S., et al. (2019). Food in the Anthropocene: the EAT–Lancet commission on healthy diets from sustainable food systems. Lancet 393, 447–492. doi: 10.1016/S0140-6736(18)31788-4
Williams, D. (2004). “The conservation and evolution of landraces of peanuts and peppers,” in Home Gardens and Agrobiodiversity, eds P. B. Eyzaguirre, and O. Linares (Washington, DC: Smithsonian Institution), 256–265.
Winget, M., Lamb, N. H., and Wheatley, K. K. (2017). Root capital measuring the effects of impact investing on impoverished rural communities. J. Case Stud. 35:19.
Winterhalder, B., Lu, F., and Tucker, B. (1999). Risk-senstive adaptive tactics: models and evidence from subsistence studies in biology and anthropology. J. Archaeol. Res. 7, 301–348. doi: 10.1007/BF02446047
Wittman, H., and Blesh, J. (2017). Food sovereignty and fome zero: connecting public food procurement programmes to sustainable rural development in Brazil. J. Agrar. Change 17, 81–105. doi: 10.1111/joac.12131
Yadav, S. K. (2010). Cold stress tolerance mechanisms in plants. A review. Agron. Sustain. Dev. 30, 515–527. doi: 10.1051/agro/2009050
Yamada, M., Hidaka, T., and Fukamachi, H. (1996). Heat tolerance in leaves of tropical fruit crops as measured by chlorophyll fluorescence. Sci. Hortic. 67, 39–48. doi: 10.1016/S0304-4238(96)00931-4
Yang, X., Cushman, J. C., Borland, A. M., Edwards, E. J., Wullschleger, S. D., Tuskan, G. A., et al. (2015). A roadmap for research on crassulacean acid metabolism (CAM) to enhance sustainable food and bioenergy production in a hotter, drier world. New Phytol. 207, 491–504. doi: 10.1111/nph.13393
Yundaeng, C., Somta, P., Amkul, K., Kongjaimun, A., Kaga, A., Tomooka, N., et al. (2019). Construction of genetic linkage map and genome dissection of domestication-related traits of moth bean (Vigna aconitifolia), a legume crop of arid areas. Mol. Genet. Genom. 294, 621–635. doi: 10.1007/s00438-019-01536-0
Zhang, J., and Kirkham, M. B. (1995). Water relations of water-stressed, split-root C4 (Sorghum bicolor; Poaceae) and C3 (Helianthus annuus; Asteraceae) plants. Am. J. Bot. 82, 1220–1229. doi: 10.1002/j.1537-2197.1995.tb12655.x
Glossary
Agricultural innovation system or network: A network of actors including researchers, input suppliers, extension agents, farmers, traders, processors, and other actors who are engaged in the creation and use of knowledge relevant to agricultural production and marketing (Spielman et al., 2008).
Agroecosystem: A site or integrated region of agricultural production understood as an ecosystem with organisms, such as crop plant individuals, populations of crops, communities of polycultures, and ecosystems as farms or watersheds (Gliessman, 2014).
On-farm diversification refers to the incorporation of species, plant varieties or breeds, and management practices and land-use systems in farm systems in space and time through a range of spatial practices, such as polycultures, agroforestry systems, field scattering, and hedgerows; and temporal diversification through crop rotations (Somarriba, 1992; Vandermeer, 1992; Goland, 1993; Brookfield, 2001; Liebman and Dyck, 2007; Kremen et al., 2012).
Crop functional type: Practical ecological approach to group crops with similar traits and responses to changes in environmental factors (Lavorel and Garnier, 2002; Bondeau et al., 2007; Gilbert and Holbrook, 2011).
Farm system: A decision-making unit comprising the farm household, cropping, agroforestry, and/or livestock systems, which transforms land, capital, and labor into useful products, which can be consumed or sold (adjusted from Fresco and Westphal, 1988).
Germplasm: Living tissue from which new plants can be grown, such as seeds, meristem, or pollen.
Index insurance: Payouts are based on an index (such as the total seasonal rainfall or average crop yield for a larger area) and this reduces the costs of insuring farmers (Bell et al., 2013).
Local knowledge: A collection of certainties and experiences, which relate to a system of concepts, beliefs, and perceptions, which people hold about their environment. This includes the way people observe and measure their surroundings, how they solve problems and validate new information. It includes the processes whereby knowledge is generated, stored, applied, and transmitted to others (Warburton and Martin, 1999).
Modern Portfolio Theory: Optimization technique to determine optimal number and type of crops or land-use systems to manage production risks for specific expected returns on investment under climate change. In MPT, risks are defined as the variance in returns to expected production or gross margin across years.
Polyculture: Multiple cropping systems, such as intercropping systems and multistrata systems.
Resilience: The amount of change a system can undergo and still remain within the same domain of attraction (Gallopín, 2006). This is related to the extent that farmers can adapt their farming systems to climate change (Eakin et al., 2012).
Smallholders: Farmers who own small-based plots of land on which they grow subsistence crops and one or two cash crops and generally rely principally on family labor. Smallholders generally have <2 ha of land in production but farm-size is context-specific. In the western highlands of Guatemala many farm households have access to land well below 2 ha (Hellin et al., 2017) while in parts of Brazil a smallholder farmer may own up to 50 ha. Smallholders often have limited marketing, storage, and processing capacity. The average annual income for commercial smallholder production in generally below 5,000 USD/year (Lowder et al., 2016).
Neglected and underutilized crops: Neglected crops may be globally distributed, but tend to occupy special niches in the local ecology and in production and consumption systems. While these crops continue to be maintained by socio-cultural preferences and use practices, they remain inadequately characterized and neglected by research and conservation. Many underutilized crops were once more widely grown but have fallen into disuse for a variety of agronomic, genetic, economic and cultural factors. Farmers and consumers are using these crops less because these crops are in some way not competitive with other crops in the same agricultural environment (Padulosi et al., 2002). These crops include food and forage tree species and any other agricultural plant species; they are also known as minor, orphan, underexploited, underdeveloped, lost, new, novel, promising, alternative, local, traditional, or niche crops.
Whole farm insurance: A single insurance, which covers the covariate risk of jointly produced farm crop and livestock enterprises (Turvey, 2012).
Keywords: on-farm diversification, agroecosystem diversification, climate-smart agriculture, climate variability, crop diversification, diversified farming systems, participatory research, risk management
Citation: van Zonneveld M, Turmel M-S and Hellin J (2020) Decision-Making to Diversify Farm Systems for Climate Change Adaptation. Front. Sustain. Food Syst. 4:32. doi: 10.3389/fsufs.2020.00032
Received: 12 September 2019; Accepted: 02 March 2020;
Published: 07 April 2020.
Edited by:
Timothy Bowles, University of California, Berkeley, United StatesReviewed by:
Paul Wilson, University of Nottingham, United KingdomDavid Rose, University of Reading, United Kingdom
Copyright © 2020 van Zonneveld, Turmel and Hellin. This is an open-access article distributed under the terms of the Creative Commons Attribution License (CC BY). The use, distribution or reproduction in other forums is permitted, provided the original author(s) and the copyright owner(s) are credited and that the original publication in this journal is cited, in accordance with accepted academic practice. No use, distribution or reproduction is permitted which does not comply with these terms.
*Correspondence: Maarten van Zonneveld, bWFhcnRlbi52YW56b25uZXZlbGQmI3gwMDA0MDt3b3JsZHZlZy5vcmc=