- School of Agriculture and Food Sciences, The University of Queensland, Brisbane, QLD, Australia
Increases in human populations and the emerging challenges of climate change mean that the world's agricultural systems will need to produce more food in an environment that is increasingly variable and where the quality of our natural resource base is declining. One central measure of an agricultural system's capacity to do this is its ability to preserve soil organic carbon (SOC), due to the pivotal role that this plays in maintaining soil physical, chemical, and biological properties and ultimately yield. This narrative review examines the literature published worldwide over the last 30 years to assess the impact of one widely applied agricultural management system, conservation agriculture (CA), on its ability to maintain SOC and the subsequent impacts on soil physical, chemical and biological properties, and yield. While the effects of CA on SOC worldwide are variable, with both increases and decreases observed, in regions where soil and climatic conditions are favorable for biomass production and where the system does not negatively impact yield, then CA can lead to higher amounts of SOC relative to conventionally managed systems, particularly in the surface of the soil profile. Where greater SOC occurs, these are also often accompanied by improvements in soil structure, water infiltration and soil water storage, plant nutrient availability, microbial biomass and diversity, and yield. However, where CA is used in certain environments (e.g., cold, wet environments with poorly drained soils) or where the CA system has not been well-adapted to local conditions, taking into account the specific agronomic, social, and environmental challenges present, then it may not be a successful system of management. Farmers require access to a range of tools and resources to allow them to identify if the principles of CA are likely to lead be appropriate for their situation and well-designed, locally adapted systems to successfully overcome the agronomic, social and economic challenges that can be associated with its use.
Introduction
The world population is expected to increase from 7.7 billion in 2019 to 9.7 billion in 2050 (United Nations, 2019). In order to feed these extra people, it will obviously be necessary to boost world food production, particularly in developing countries where the greatest rates of population growth currently occur (United Nations, 2019). This must occur in a world where the opportunity to expand the area utilized for agriculture is limited and our ability to increase production on existing agricultural land is threatened by land degradation, water resource scarcity, and increases in the climate variability and extreme events associated with climate change. Thus, in order to meet the world's demand for food, agricultural systems worldwide need to evolve to produce more food, with greater sustainability.
While it is not without challenges, one agricultural system that has been promoted as capable of achieving the sustainable intensification required to meet world food demand is conservation agriculture (CA) (Kassam et al., 2009; Lal, 2015a). Conservation agriculture is defined as a system that combines minimum or no tillage (NT) with permanent soil cover (that leaves at least 30% of the soil covered between harvest and planting) and diversified crop species that include legumes (FAO, 2019). Other companion practices, such as integrated pest and nutrient management, are also often incorporated into the CA system on a site specific basis to help ensure its success (Lal, 2015a; Thierfelder et al., 2018). Overall, CA is a farming system designed to enhance the sustainability of agricultural production by conserving and protecting soil, water and biological resources so that external inputs can be kept to a minimum (Figure 1). The characteristics of CA can vary regionally and can include small landholder systems that use direct planting with hand tools, through to large scale mechanized systems that use tractor mounted direct seeders (Lal, 2015a).
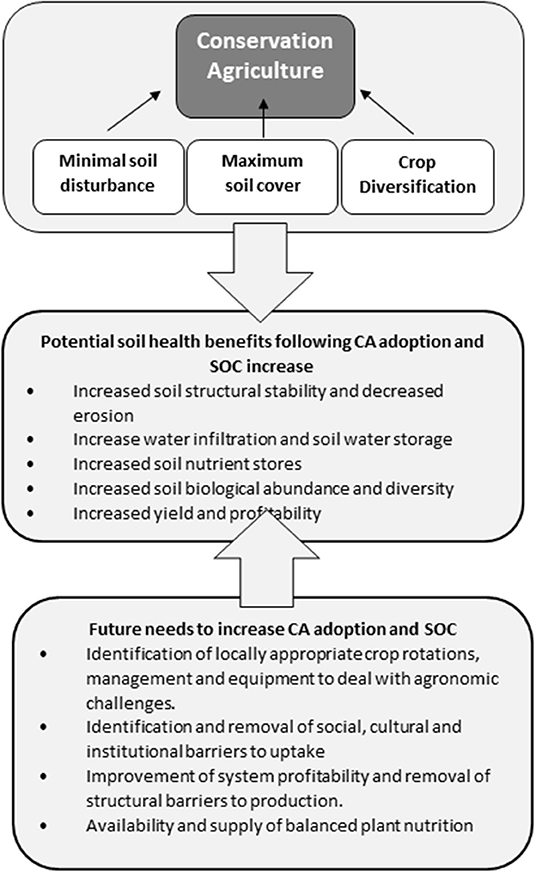
Figure 1. Summary of the benefits of increased soil organic carbon (SOC) under conservation agriculture (CA) and the future needs to increase adoption.
Conservation agriculture and its components have been associated with many benefits including greater soil water storage (Verhulst et al., 2011; Lampurlanés et al., 2016; Page et al., 2019), improved soil quality (Jat et al., 2019; Somasundaram et al., 2019), decreased erosion (Montgomery, 2007), and in some instances, greater yield and net farm income (Thierfelder et al., 2015; Pradhan et al., 2018; Page et al., 2019). These benefits have led to the identification of CA as an important tool to help ensure future food production and help buffer agricultural productivity against extreme climate events, such as drought and heat waves, which are likely to increase in frequency under climate change (FAO, 2019). One of the key drivers of the improvements observed under CA is the greater soil organic matter (SOM), particularly at the surface of the profile, and the associated improvements in soil structural stability, fertility, and biological diversity relative to conventional agricultural systems (Lal, 2015a).
This paper will provide a comprehensive summary of our knowledge regarding the effect of CA and its components (i.e., NT, residue retention and crop diversification) on SOM by conducting a narrative review of the peer reviewed research published worldwide over the past 30 years. The situations under which gains, no change or even losses of SOM have been observed when using CA systems will be examined and discussed. The subsequent changes in soil physical, chemical and biological properties stemming from changes in to SOM will also be explored and the ultimate impact of this on yield and yield stability in different environments and soil types examined. Some of the agronomic, social and economic challenges associated with the adoption of CA will be explored and potential opportunities and strategies required to increase the adoption of CA discussed.
Effect of CA on SOC
The amount of organic matter present in a soil is the net difference between organic matter inputs (biomass) and losses (erosion, decomposition, leaching). The extent and direction that CA affects SOM (typically measured via SOC) is thus a function of how it impacts these inputs and losses. In comparison to conventional agricultural systems, CA makes modifications to tillage practices, residue management and crop/nutrient management. Each of these modifications can influence SOC, as discussed below.
Tillage Management
It has been well-established that tilling the soil leads to losses of SOC as cultivation breaks up the soil and exposes organic matter previously protected within soil macroaggregates to microbial decay (Beare et al., 1994; Six et al., 2000). Cultivation also incorporates and fragments plant material, increasing its vulnerability to microbial attack (Hendrix et al., 1986; Beare et al., 1993). Decreasing the amount of tillage or introducing no-till (NT) thus has the potential to decrease the amount of SOC lost from the profile by decreasing the turnover rate of macroaggregates, increasing the physical protection of particulate organic material, and reducing soil to residue contact. For example, in a Brazilian Acrisol under cereal cropping, turnover times of 17 vs. 36 years were observed in conventional and CA, respectively, due to the reduced disturbance in the NT CA system (Bayer et al., 2006a).
However, it should be noted that in some instances tillage has also been observed to increase SOC stores relative to CA systems (Chan et al., 1992; Christopher et al., 2009; Blanco-Canqui et al., 2011; Zhang et al., 2014). In areas where full inversion tillage is carried out, residues may be buried in a region where poor soil aeration can limit decomposition (relative to the soil surface), particularly under cool, moist climatic conditions (Gregorich et al., 2005; Christopher et al., 2009).
Residue Management
Crop residues can be defined as biomass remaining on the soil's surface after harvest. In some systems, linear increases in SOC stocks can be observed with increasing rates of residue addition (Duiker and Lal, 2002; Virto et al., 2012; Liu et al., 2014). Thus, in CA systems, which emphasize the retention of residues on the soil surface, greater residue input can potentially lead to greater SOC storage. However, where residue production is low e.g., due to low soil fertility or the presence of soil constraints, there can be insufficient residue retention under CA to positively impact SOC stores (Virto et al., 2012; Palm et al., 2014). Indeed, in some situations CA can even lead to a reduction in plant growth (e.g., due to lower soil temperatures, compaction or greater disease/weed/pest pressure) and this can also lead to decreases in residue inputs and lower or no SOC sequestration (Yang et al., 2013). In areas with low fertility, integrated nutrient management is essential to ensure a buildup of SOC and the success of CA systems. Low fertility results in low crop yields, which leads to reduced organic matter input and hence, lower SOC (Lal, 2015b). This cycle can only be broken by the addition of nutrients to the soil system via external fertilizer input (organic or inorganic) and/or the incorporation of legumes into cropping rotations.
Crop Rotation
Different crops may have different effects on the quantity, quality, and periodicity of C inputs and can modify the soil in different ways (e.g., rates of water extraction, nutrient use), which can influence mineralisation rates and the growth of subsequent crops (Huggins et al., 2007). Thus, differences in crop rotation between CA and conventional agricultural systems also have the potential to impact SOC values. The elimination of monocultures and incorporation of plant species into rotations that return greater amounts of residue to the soil are often associated with greater SOC stock in CA systems (Huggins et al., 2007; dos Santos et al., 2011; Conceição et al., 2013). In some systems, root input, in particular, has been found to be important. For example, in one study conducted on a Brazillian Ferrosol of the long-term (17 years) contribution of cover crop or forage based NT rotations to SOC stocks, SOC stocks showed a close relationship with the root additions of different plant species (dos Santos et al., 2011). Where legumes are included, these can also add additional N to the system, which can enhance soil fertility and subsequent crop biomass production (Hansen et al., 2012; Mbuthia et al., 2015; Raphael et al., 2016; Veloso et al., 2018). The maintenance of residue cover can also decrease processes that can limit the growth and biomass production of the main crop, such as erosion, nutrient leaching, and weeds, pests and diseases (Tittonell et al., 2012; Gabriel et al., 2013; Veloso et al., 2018; Williams et al., 2018).
Net Effects of CA on SOC
The exact magnitude of the difference in SOC between CA and conventional agricultural systems varies greatly and is influenced by many factors, including climate (Ogle et al., 2005, 2019), soil type (Liang et al., 2002; Nyamangara et al., 2014), baseline SOC (VandenBygaart et al., 2003; Steinbach and Alvarez, 2006), crop management (VandenBygaart et al., 2003), time since management change (González-Sánchez et al., 2012), and sampling depth and methodology (Olson, 2013). This means that estimates of the magnitude of the change following conversion to CA vary widely (Table 1). For example, estimates ranging from −0.15 Mg/ha/year in areas such as the midwestern USA (Christopher et al., 2009) to +0.93 Mg/ha/years in tropical Brazil (Bernoux et al., 2006) have been recorded. However, it is clear that in regions where soil and climatic conditions are favorable for biomass production and where negative yield impacts are not observed, then CA systems will often have higher amounts of SOC relative to conventionally managed systems, particularly in the surface of the soil profile. Although, the large range in the sequestration rates observed indicates that the magnitude of change is likely to be highly site specific. It should also be noted that most of the literature regarding the effect of CA on soil properties has focused on NT and residue retention v conventional tillage and residue removal. Fewer studies have also considered the impact of species diversification and the inclusion of practices such as cover crops and intercropping on SOC change. Where these are carried out they are often found to lead to greater increases in SOC than NT and stubble retention alone, and the effect of management changes on soil properties would thus often be greater when all elements of the CA system, which may have interactive and synergic effects, are incorporated together (Blanco-Canqui and Ruis, 2018).
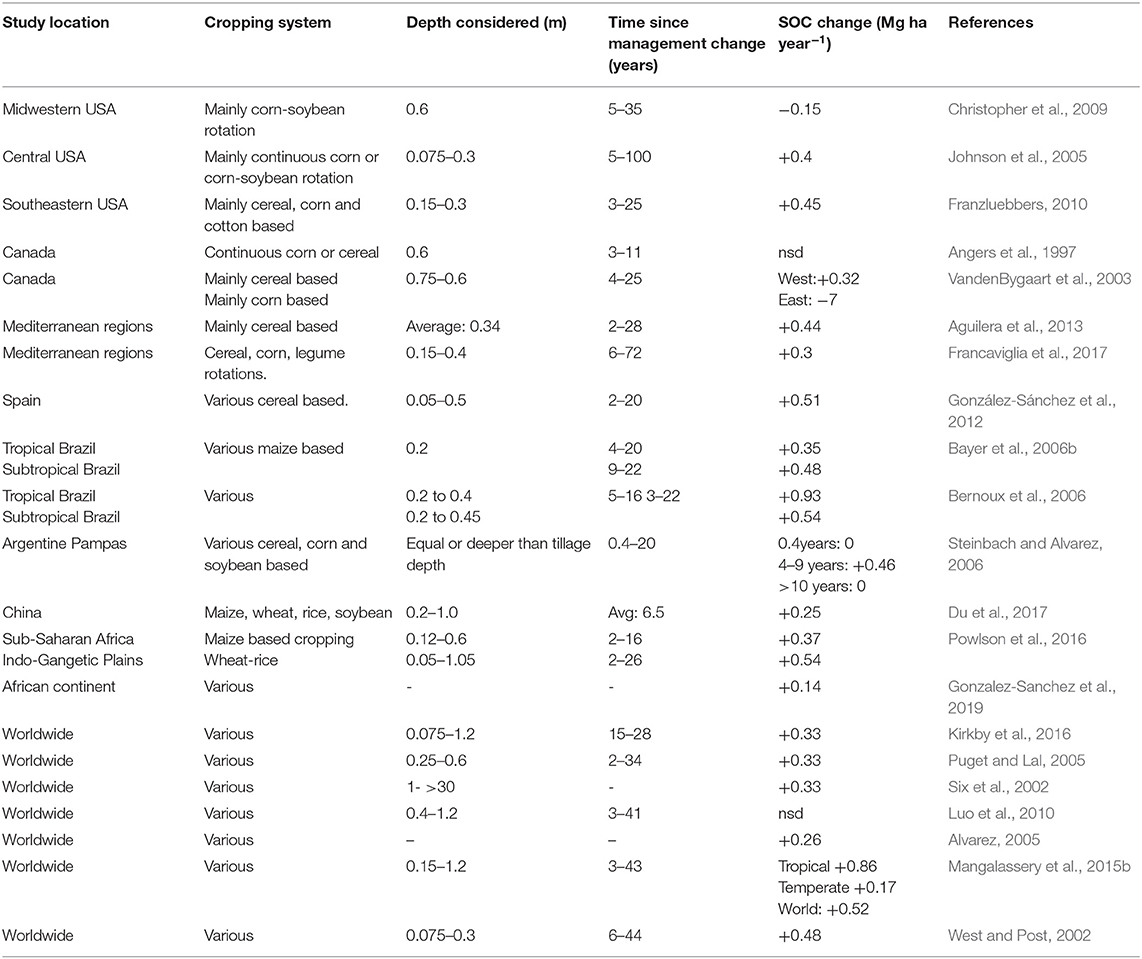
Table 1. Worldwide estimates of SOC change following the incorporation of CA practices (NT + residue retention with or without crop diversification, as indicated by the cropping system).
Impacts of Increased SOC on Soil Physical, Chemical, and Biological Properties
Where CA leads to greater SOC, this will often have a positive impact on soil physical, chemical, and biological properties that can interact to affect crop production, as discussed below and summarized in Figure 1.
Physical
Aggregate Stability
Soil organic matter is very important for aggregate stability and thus the maintenance of good soil structure. In soils that are depleted of organic matter, aggregates are more likely to slake into smaller sub-units during wetting, resulting in a soil structure that is more likely to erode and which can limit water infiltration and the emergence of seedlings (Tisdall and Oades, 1982; Blanco-Canqui and Ruis, 2018). Where CA increases SOC, associated improvements in soil aggregate stability are typically observed (Blanco-Canqui and Ruis, 2018; Li et al., 2019b). Greater fungal populations and the persistence of root networks in the absence of tillage in particular have a positive effect (Wang et al., 2010; Spurgeon et al., 2013). As a reflection of this, a recent worldwide meta-analysis observed that, on average, the number of water stable aggregates in NT systems are 31% greater compared to conventionally tilled systems without residue retention (Li et al., 2019b). These improvements are important as they lead to greater rates of soil water infiltration, provide increased resistance to wind and water erosion (in combination with residue retention), improve the physical protection of organic matter and provide greater abundance of habitats to support microbial activity (Helgason et al., 2010; Spurgeon et al., 2013; Blanco-Canqui and Ruis, 2018; Li et al., 2019b).
Soil Water
Improved aggregate stability, combined with the residue retention in CA systems, is often observed to have a significant positive impact on soil water storage (for examples see Table 2). These increases typically occur due to a combination of greater rates of infiltration and decreased soil water evaporation (Li et al., 2019b). Increases in infiltration are commonly attributed to the improved aggregate stability in the surface of the profile (where improvements in SOC are highest) and the greater number and continuity of macropores available to rapidly transmit water into the soil profile in the absence of tillage (Blanco-Canqui and Ruis, 2018; Li et al., 2019b). The presence of crop residues can also help protect the surface of the soil from raindrop impact and prevent the formation of surface seals, which can decrease infiltration rates (McGarry et al., 2000). They also shade the soil and decrease wind speeds at the soil surface, decreasing water loss from evaporation (O'Leary and Connor, 1997a; Nielsen et al., 2005; Lampurlanés and Cantero-Martínez, 2006).
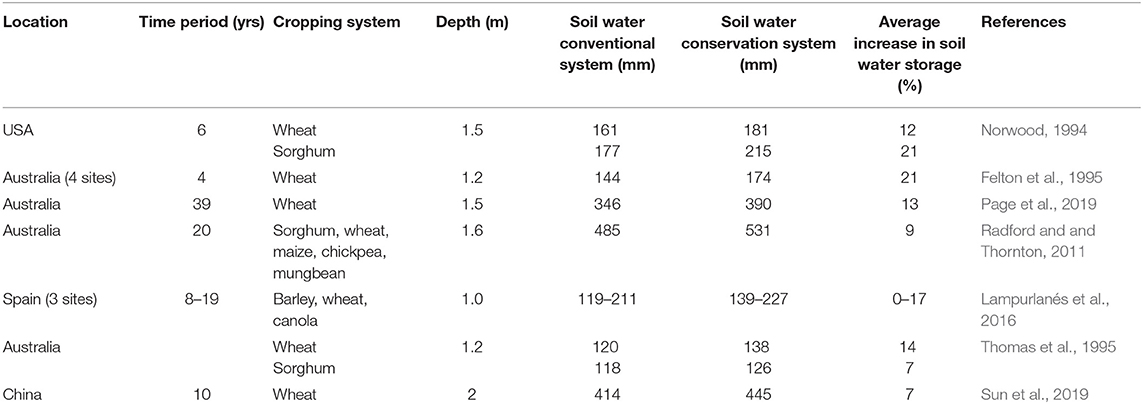
Table 2. Examples of the increase in soil water observed at planting in conventional agricultural systems compared to those incorporating CA practices (NT + residue retention with or without crop diversification, as indicated by the cropping system).
Where the practice of CA does not lead to sufficient rates of residue retention, as may occur where soil fertility or weed, pest or disease issues limit plant growth, and/or where residue is removed for animals/ fuel, surface sealing may still occur and lead to decreased infiltration (Baudron et al., 2012). In these situations, conventionally tilled soils can lead to greater infiltration and soil water storage than CA as cultivation is able to loosen the soil surface and destroy crusts (Hamblin, 1984; Jones et al., 1994). In wetter and cooler regions, the greater retention of soil moisture under CA can also lead to waterlogging, with associated negative effects on crop growth and yield (Rusinamhodzi et al., 2011; Soane et al., 2012).
Bulk Density
Bulk density can be used as a measure of a soil's compaction and indicates the effect a soil is likely to have on seedling emergence, root growth and thus crop production (Blanco-Canqui and Ruis, 2018). It can also indicate properties such as porosity and likely water infiltration (Blanco-Canqui and Ruis, 2018). Bulk density, has been observed to increase (Li et al., 2007; Soane et al., 2012; Mbuthia et al., 2015; Blanco-Canqui and Ruis, 2018; Somasundaram et al., 2019), decrease (Hansen et al., 2012; Mrabet et al., 2012; Blanco-Canqui and Ruis, 2018), and be no different under CA systems (Dalal et al., 2011; Zhang et al., 2014; Blanco-Canqui and Ruis, 2018), with differences between studies largely due to soil type, duration of the study and time of sampling. However, a recent global meta-analysis found that NT with residue retention, on average increased bulk density by 1.4% (Li et al., 2019b).
Where greater bulk densities are observed, these are generally attributed to reduced soil disturbance and subsequent soil settling in the absence of tillage, and the repeated trafficking of the soil by agricultural machinery (Blanco-Canqui and Ruis, 2018). However, this is not necessarily associated with decreased crop growth due to the maintenance of stable macropores in CA soils, which can provide pathways for root growth and water infiltration (Mrabet et al., 2012; Soane et al., 2012). Initially greater bulk densities may also decline over time as SOC improves (Blanco-Canqui and Ruis, 2018). However, in some instances, increases in bulk density do limit production, this may occur because the greater soil strength under compacted conditions limits root growth and thus water and nutrient uptake (Braim et al., 1992), and/or because it can lead to decreased air permeability and a reduction in oxygen concentrations for plants during wetting events (Rusinamhodzi et al., 2011; Nyagumbo et al., 2016). Where decreases in bulk density are observed, these are generally associated with increased addition of organic residue, and associated increases in soil faunal activities (Stagnari et al., 2009; Hansen et al., 2012; Blanco-Canqui and Ruis, 2018).
Chemical
Soil pH
The larger SOC at the surface of the profile in CA systems, is commonly associated with greater acidity relative to conventionally tilled systems (Dalal, 1989; Franzluebbers and Hons, 1996; Limousin and Tessier, 2007; Vieira et al., 2009; Mrabet et al., 2012; Sithole and Magwaza, 2019). This is typically associated with the accumulation of plant residues and organic acids at the soil surface (Dalal, 1989; Heenan and Taylor, 1995; Franzluebbers and Hons, 1996) and greater rates of nitrogen mineralization combined with the leaching of nitrate-nitrogen (Heenan and Taylor, 1995). Greater rates of root exudation due to the accumulation of roots in the surface of the soil profile can also contribute to greater rates of acidification (Limousin and Tessier, 2007).
The magnitude of any change in pH will depend on the buffering capacity of the soil, the magnitude of the change in SOM concentrations, climate, and nitrogen management. For example, in semi-arid environments in the absence of fertilizer and lime application, changes can be relatively small, and range from 0 to ~0.1–0.3 pH units following ~10+ years of CA management (Page et al., 2013). However, in legume-based systems and systems with mineral N fertilizer addition, declines in pH can be much higher. For example, in a CA system from southern Brazil, changes in pH were found to range from 0.4 to 1.5 units, with greater decreases observed in treatments with legume-based crop rotations and mineral N fertilization (Vieira et al., 2009).
Cation Exchange Capacity
Soil cation exchange capacity (CEC) impacts soil fertility, soil structural stability, and soil pH buffer capacity (McBride, 1994). While CEC is largely an inherent soil characteristic dependent on mineralogy and clay content, it can also be influenced by changes to SOM and pH (McBride, 1994). As such, there is potential for the CA to influence CEC. The magnitude and direction of the changes are variable, with increases (Chan et al., 1992; Pankhurst et al., 2002a; Duiker and Beegle, 2006; Sa et al., 2009), decreases (Loch and Coughlan, 1984; Lal, 1999; Duiker and Beegle, 2006; Limousin and Tessier, 2007; Thomas et al., 2007; Sithole and Magwaza, 2019), and no change observed (Bravo et al., 2007; Qin et al., 2010; Williams et al., 2018). Where CEC is greater, it is generally associated with a higher organic matter content, which increases the amount of negative charge (Chan et al., 1992; Pankhurst et al., 2002a; Duiker and Beegle, 2006; Sa et al., 2009). Lower CEC may be observed in soils where a decrease in pH has occurred, and resulted in a decrease in pH-dependent cation exchange sites (Limousin and Tessier, 2007; Thomas et al., 2007; Sithole and Magwaza, 2019).
Plant Nutrients
Where improvements in SOC are observed in CA systems, this can have a significant effect on plant nutrient availability due to both changes to the quantity of nutrients available, and their distribution in the soil profile. In situations where CA successfully leads to greater residue addition and thus input of nutrient containing organic material into the soil, this can lead to higher plant nutrient stores, with greater nitrogen (N) (Pankhurst et al., 2002a; Li et al., 2007; Thomas et al., 2007; González-Chávez et al., 2010; Page et al., 2019; Pheap et al., 2019; Sithole and Magwaza, 2019),phosphorus (Ismail et al., 1994; Bravo et al., 2007; Qin et al., 2010; Zhao et al., 2017; Sithole and Magwaza, 2019), calcium (Chan et al., 1992), magnesium (Chan et al., 1992), potassium (Duiker and Beegle, 2006; Bravo et al., 2007; Zhao et al., 2017; Sithole and Magwaza, 2019), manganese (Rhoton, 2000) and zinc (Rhoton, 2000; Pankhurst et al., 2002a) concentrations all observed CA systems in response to increases in organic matter.
However, while in many instances increases in the amount of nutrients stored in the soil will lead to greater plant nutrient availability (Pheap et al., 2019), this may not always be the case. In the case of N, for example, while total stores of N may be higher under CA, the amount of plant available N can decrease, particularly soon after CA is implemented (Thompson, 1992; Thomas et al., 1995; O'Leary and Connor, 1997b) and applications of N fertilizer may be required to maintain yield (Rusinamhodzi et al., 2011; Mrabet et al., 2012; Sithole and Magwaza, 2019). This can occur due to slower rates of N mineralisation due to reduced soil-stubble mixing, and/or greater rates of immobilization due to the presence of crop residues with high C:N ratios (Thompson, 1992; O'Leary and Connor, 1997b). Where immobilization is responsible for decreases in N availability, a gradual improvement in N supply may be observed over time as a new steady state between C and N supply is attained (Tessier et al., 1990; Mrabet et al., 2012; Soane et al., 2012).
The absence of soil mixing in CA systems, especially those using complete NT, can also lead to the stratification of immobile nutrients at the surface of the soil profile (Mrabet et al., 2012; Dang et al., 2015). This can be a problem in more arid regions, where drying at the surface may prevent plant roots from accessing nutrients from surface layers (Mrabet et al., 2012; Dang et al., 2015). Where CA systems lead to higher soil bulk density and lower air-filled porosity under wet conditions, losses of N due to greater denitrification can also be observed (Doran et al., 1998; Rochette, 2008). Similarly, greater water infiltration can be accompanied by increased leaching and the movement of soluble nutrients below plant rooting zones (Radford et al., 1995; Turpin et al., 1998).
Biological
Soil Microbiology
Where additional SOC is present in CA systems, this can provide an energy source for soil microorganisms and lead to a greater microbial biomass relative to conventional agricultural systems (Dou et al., 2008; Helgason et al., 2009, 2010; González-Chávez et al., 2010; Mangalassery et al., 2015a). Where increases in SOC and residue retention create a more favorable environment for the microbial populations due to improvements in soil aggregation, soil moisture and/or more favorable soil temperature, this can also improve microbial abundance (Kladivko, 2001; Lupwayi et al., 2001; Govaerts et al., 2007a; Zhang et al., 2018). Examples of the changes in microbial abundance between conventional and conservation agriculture systems can be seen in Table 3.
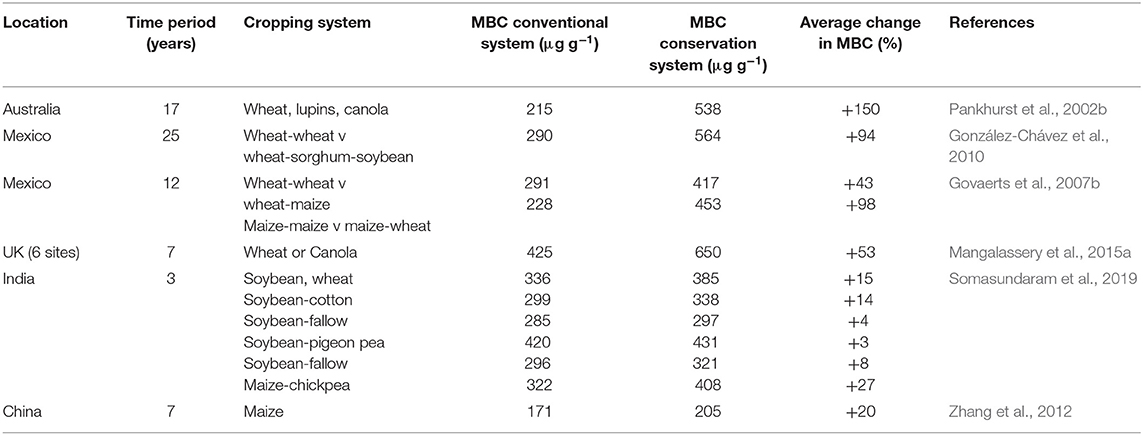
Table 3. Examples of the increase in soil microbial biomass carbon (MBC) in conventional agricultural systems compared to those incorporating CA practices (NT + residue retention with or without crop diversification, as indicated by the cropping system).
Conservation agriculture can also be associated with an improvement in the diversity of both fungal and bacterial populations, especially in the presence of more diversified crop rotations (Lupwayi et al., 2001; González-Chávez et al., 2010; Wang et al., 2010, 2016; Yang et al., 2012). Fungal populations, in particular, are often observed in greater abundance in CA systems incorporating NT due to the absence of tillage, particularly at the surface of the profile (Kladivko, 2001; Helgason et al., 2010; Spurgeon et al., 2013; Zhang et al., 2018). A greater abundance and diversity of microbes can have many important implications for crop production as a more microbially diverse soil is more likely to contain organisms that promote plant growth and suppress disease (Peters et al., 2003; van Bruggen et al., 2006; Govaerts et al., 2008). For example, increases in the populations of arbuscular mycorrhizal fungi have been observed under NT corn grown on sandy loam soils in Canada (Kabir et al., 1997) and cotton fields on silt loam soils in the US (Mbuthia et al., 2015), which may improve P nutrition. The US study also found key enzymes associated with N and P cycling to be greater under CA, corresponding to greater nutrient availability (Mbuthia et al., 2015).
Macro-Fauna
Soil macro-fauna can be significantly influenced by CA systems. Macro-fauna, such as earthworms, termites and beetles, that burrow through the soil and/or break up plant residues are important for the creation of soil macroporosity (and thus water infiltration and hydraulic conductivity), and for helping mix organic material into the soil to aid nutrient cycling and aggregate formation (Kladivko, 2001; Spurgeon et al., 2013). The tillage operations in conventional agricultural systems can affect macrofauna by directly killing or injuring them, by bringing them closer to the soil surface and exposing them to adverse environmental conditions and predators, and by destroying their food sources, burrows and tunnels (Kladivko, 2001; Briones and Schmidt, 2017). The population of macro-fauna are thus commonly greater, both in terms of abundance and biomass, under CA systems and this increase in abundance becomes larger with the longer duration of the CA system (Kladivko, 2001; Stagnari et al., 2009; Soane et al., 2012; Spurgeon et al., 2013; Briones and Schmidt, 2017). For example, one worldwide meta-analyses comparing conventional agricultural systems with CA observed greater abundance and biomass of earthworms with reduced disturbance (average increases of >100%), with greater impacts in warm temperate zones with fine textured soils, and in soils with higher clay contents (>35%) and low pH (<5.5) (Briones and Schmidt, 2017).
Diseases
While much of the soil's microbiology has positive implications for plant growth, numerous diseases also exist, and disease prevalence can both increase and decrease under CA systems. For example, greater disease prevalence can occur due to the retention of residues, which provide some pathogens with a refuge to survive the period between harvest and planting when host plants are absent (Roper and Gupta, 1995; Bockus and Shroyer, 1998). Reduced soil disturbance, greater soil moisture and lower soil temperatures can also create an environment more favorable for many plant pathogens (Bockus and Shroyer, 1998). Pathogens commonly observed to increase in the absence of tillage and/or residue removal include Gaeumannomyces graminis var tritici (take all) (Pankhurst et al., 1995a; Roget et al., 1996), Fusarium pseudograminearum (head blight, scab or crown rot)(Wildermuth et al., 1997a,b), Pyrenophora tritici-repentis (tan or yellow spot) (Marley and Littler, 1989; Bockus and Shroyer, 1998), Pythium spp. (Pythium seed and root rot) (Pankhurst et al., 1995a), Rhizoctonia solani (Rhizoctonia root rot, bare patch, purple patch) (Cook and Haglund, 1991; Pankhurst et al., 1995a; Paulitz et al., 2010), and Pratylenchus spp. (root lesion nematode) (Pankhurst et al., 1995b; Rahman et al., 2007).
However, when reduced tillage and residue retention are incorporated effectively within a CA system that also includes diversification of cropping rotations, increases in disease prevalence are much less. Indeed, the general improvements in biological diversity under CA can increase the abundance of micro-organisms that suppress disease (Peters et al., 2003; van Bruggen et al., 2006; Govaerts et al., 2008). For example, the prevalence of take-all can decrease due to increases in the prevalence of antagonistic Pseudomonas spp. (Paulitz et al., 2010). The greater soil moisture often observed under CA may also lead to decreased plant moisture stress and reduced disease severity, even in instances where disease abundance is greater (Wildermuth et al., 1997a; Paulitz et al., 2010).
Impact on Yield, Yield Stability and Farm Profitability
Yield
Where improvements in soil properties are observed under a system of CA management, there are many instances where this has been associated with yield increases (Table 4). However, no change and decreases in yield have also been observed (Table 4). A recent global meta-analysis of the effect of CA practices on yield concluded that CA (NT+residue retention+crop diversification) on average worldwide leads to yield reductions of 2.5% (Pittelkow et al., 2015). Where only partial implementation of CA occurs greater yield loss can be expected (e.g., −9.9% for NT alone, −5.2% for NT+residue retention). However, yield increases were observed under certain circumstances, for example, gains of 7.3% were observed under rainfed agriculture in dry climates, and the yield declines observed overall tended to decrease the longer CA had been implemented.
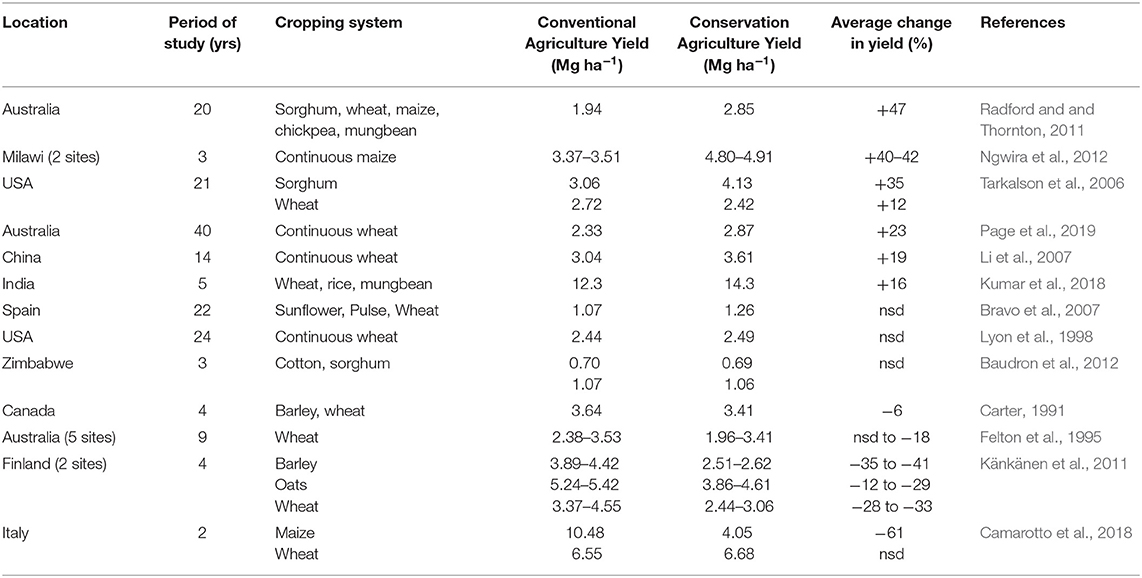
Table 4. Examples of the change in yield observed between conventional agricultural systems and those incorporating CA practices (NT + residue retention with or without crop diversification, as indicated by the cropping system).
The yield gains frequently observed in dry climates are commonly attributed to the improvements in soil water storage under CA (Halvorson et al., 2000; Hemmat and Eskandari, 2004, 2006; Li et al., 2007; Radford and and Thornton, 2011; Araya et al., 2012; Soane et al., 2012; Steward et al., 2018; Garcia-Palacios et al., 2019; Page et al., 2019). The possibility for early planting is also greater in CA systems due to the reduced land preparation requirements, which may result in more efficient use of rainfall and reduced risk of crop failure due to terminal heat stress (Kumar et al., 2018; Devkota et al., 2019). In hot regions, the lower soil temperatures under CA systems due to residue retention can also help improve plant growth and yield (Zhang et al., 2014; Steward et al., 2018). Improvements in soil fertility and/or reductions in pests/diseases as a result of the inclusion of legumes and diversified species into cropping rotations have also been reported to contribute to yield increases in some instances (Rusinamhodzi et al., 2011; Ngwira et al., 2012; Nyagumbo et al., 2016; Kaye and Quemada, 2017).
Where yield decreases are observed, these are commonly associated with greater waterlogging in response to the greater water infiltration and lower evaporation in CA systems—with higher rainfall areas and poorly drained soils the most significantly affected (Rusinamhodzi et al., 2011; Nyagumbo et al., 2016; Lal, 2018; Steward et al., 2018). Decreased soil temperatures can also delay plant maturity, particularly in cooler, high latitude regions, and negatively affect yield (Hatfield et al., 2001; Wang et al., 2007; Rusinamhodzi et al., 2011; Soane et al., 2012; Zhang et al., 2014; Lal, 2015b). Consequently, in cold, wet environments with poorly drained soils, CA is often unlikely to lead to yield increases. Other instances of yield loss have been associated with:
Inadequate control of weeds, pests and/or diseases in the absence of tillage (Soane et al., 2012; Dang et al., 2015; Li et al., 2019a);
Soil structural issues, such as compaction or surface sealing, due to the absence of tillage and inadequate residue cover/biomass production, particularly in drier regions with infertile soils (Rusinamhodzi et al., 2011; Baudron et al., 2012; Kienzler et al., 2012; Vanlauwe et al., 2014; Giller et al., 2015; Thierfelder et al., 2015); and
Lack of access to effective seeding equipment, required inputs (herbicides, fertilizers), and knowledge regarding how best to manage CA systems (nutrition, weeds, pest and diseases) (Rusinamhodzi et al., 2011; Kienzler et al., 2012; Thierfelder et al., 2015).
The poor yields observed due to these reasons can often be improved by better customizing the CA system to a particular region or by removing fertility constraints to plant growth to improve residue cover. The yield loss observed in the early years of CA adoption before improvements in SOC and associated structural/fertility improvements occur may also often reverse over time as soil properties and nutrient availability improve and farmers/researchers gain greater skills in managing the CA system (Araya et al., 2012; Soane et al., 2012; Brouder and Gomez-Macpherson, 2014; Pittelkow et al., 2015; Vastola et al., 2017; Steward et al., 2018).
Yield Stability
It is often stated that the CA system can help agriculture adapt to increasing climate variability and the occurrence of extreme events (Kassam et al., 2009; Williams et al., 2018). The erosion protection, reduction in soil temperatures and improved infiltration rates, for example, can help deal with more intense rainstorms, and increased daily temperature ranges and frequency of drought (Kassam et al., 2009). In particular, the yield advantage often observed in CA in dry years is likely to provide an advantage in environments where rainfall is, or is becoming, more variable (Vastola et al., 2017). In agreement with this, some analyses have found that CA practices, and in particular the incorporation of cover crops, are associated with increased crop yield stability (Williams et al., 2018; Page et al., 2019; Anderson et al., 2020). Although, other analyses have found that there is no difference in the yield stability of CA vs. conventional agricultural systems (Rusinamhodzi et al., 2011; Daigh et al., 2018; Knapp and van der Heijden, 2018).
Profitability
Where CA leads to similar or greater yields, profitability is generally improved due to reduced costs of land preparation and labor, and reduced water requirements (in irrigated systems) (Ngwira et al., 2012; Jat et al., 2014; Kumar et al., 2018; Devkota et al., 2019). Savings in fertilizer use, and in some instances reduced herbicide and insecticide costs, can also increase profitability (Kassam et al., 2009; LaCanne and Lundgren, 2018). Indeed, in some instances, even when yield reductions are observed, CA systems can still be more profitable than conventional agricultural systems due to reduced input costs (Vastola et al., 2017; LaCanne and Lundgren, 2018). Examples of improvements in the profitability of CA vs. conventional agricultural systems can be seen in Table 5.
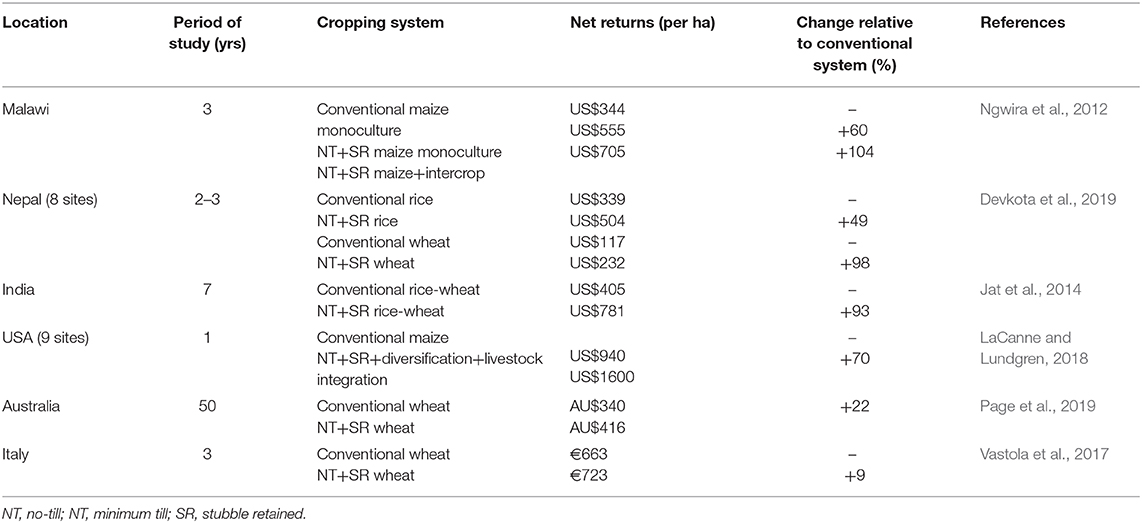
Table 5. Examples of the improvements in profitability reported between conventional agricultural systems and those incorporating CA practices (NT + residue retention with or without crop diversification, as indicated by the cropping system).
However, increases in profitability are not a universally observed trend. Where markets for alternative crop rotations are absent, or offer a reduced price compared to the main cash crop, losses in net farm income can be observed despite input cost savings (Giller et al., 2015; TerAvest et al., 2019). Indeed, lack of access to seed, suitable markets, transportation facilities and/or suitable storage facilities can sometimes be a significant barrier to the profitable use of diversified crop rotations, which are often an important part of the success of CA systems (Andersson and D'Souza, 2014; Ares et al., 2015; Carlisle, 2016; Brown et al., 2017; Dhar et al., 2018). In small holder operations some input costs, such as labor requirements, may also not be monetized, making the cost reductions that can be realized with CA small (Giller et al., 2015). Indeed, particularly in the early stages of CA system adoption before soil fertility increases and weeds, pests and diseases are effectively controlled, input costs may actually increase as new tools (e.g., planting equipment), additional fertilizers, and herbicides are required (Andersson and D'Souza, 2014; Dhar et al., 2018). In smallholder operations, CA can also often be associated with greater labor requirements in instances where hand weeding is required due to the lack of herbicide availability (Giller et al., 2015). Opportunity costs can also be significant, particularly for residue use when these can also be profitably used as stock feed, or baled for sale to other producers (Andersson and D'Souza, 2014; Ares et al., 2015; Beuchelt et al., 2015).
Future Needs
The system of CA agriculture can have clear advantages over conventional agricultural systems of management. In particular, its ability to help reduce input costs, improve soil physical, chemical and biological properties and increase yields is highly valued and has the potential to allow sustainable intensification in many instances (Lyon et al., 2004; Triplett and Dick, 2008; Verhulst et al., 2010; Zarea, 2010). However, a number of aspects of the CA system mean that its implementation is not without significant challenges and a number of approaches are required to increase worldwide adoption, as summarized in Figure 1. In particular, this will require that CA systems are well-adapted to individual regions and environments, taking into account the specific agronomic, social and economic challenges present. Where the system is not suitable for a region (e.g., cool, moist environments with high clay content soils), or in situations where the inputs required to ensure the success of CA systems (e.g., fertilizer, seeding equipment) are unavailable, CA may not be a successful system of management. Farmers require access to a range of tools and resources to allow them to identify if the principles of CA are likely to be appropriate for their operation and successfully overcome some of the challenges that can be associated with its use.
Agronomic Challenges
The successful control of weeds, pests and diseases in the absence of tillage, the stratification of immobile nutrients at the surface of the profile, poor plant establishment due to inadequate seeding equipment, decreased N availability, and the development of soil structural issues, such as surface crust and compaction, can all be significant constraints to crop production in CA systems (Dang et al., 2015). In addition, in areas where low yields are the norm, it can be difficult to build up SOC and residue cover to improve soil fertility, prevent erosion and suppress weeds (Andersson and D'Souza, 2014.). In many instances these agronomic challenges can be overcome through better adaptation of the principles of CA to local conditions. For example:
The development of locally appropriate seeding equipment and the identification of locally adapted crop rotations/cover crops can help deal with the problems of plant establishment and weed, pest and disease pressures in the absence of tillage (Verhulst et al., 2010; Thierfelder et al., 2018).
Appropriate application of fertilizers and/or better incorporation of legumes into cropping systems can help deal with problems of N availability and allow greater plant biomass production to increase residue cover and improve soil fertility (Verhulst et al., 2010).
The incorporation of cover crops can maintain soil cover during periods when the main crop is not growing and add additional organic material to the soil where crop residue production is low (Fageria et al., 2005; Tittonell et al., 2012; Veloso et al., 2018; Williams et al., 2018).
The use of strategic tillage, which is the practice of occasionally cultivating NT soils, can help with weed, pest and disease management, nutrient stratification, and compaction in continuous long-term NT systems (Kirkegaard et al., 2014; Dang et al., 2015, 2018).
However, in order to successfully identify practices appropriate for different geographical regions and cropping systems, adequate research is required to develop effective and locally adapted approaches. In particular, the identification of locally appropriate crop rotations is important, as is greater research into strategies such as strategic tillage to understand the pros and cons of this type of approach and optimum frequency and timing of tillage for a range of soils and agro-climatic regions.
Social Challenges
The successful implementation of CA systems must also take into account not just technical and agronomic issues, but social, cultural and institutional factors (Lal, 2015b). For example, in many farming communities, some of the principles of CA agriculture, particularly the use of NT, may run counter to many established land management traditions that have worked for generations and which have often created cultural values and rural traditions (Gonzalez-Sanchez et al., 2016; Tekle, 2016). Practices such as NT may be viewed as a “lazy” and ineffective way of farming, and farmers who initially adopt CA can risk backlash from their community (Zhang et al., 2014; Tekle, 2016). In some situations, it can even foster social conflict due to competing demands for crop residues (e.g., from stock grazing) (Knapp and van der Heijden, 2018). Overcoming the mindset in farming communities that tillage is required for successful agricultural production and addressing issues around residue use is thus required to increase the uptake of CA systems (Bhan and Behera, 2014; Gonzalez-Sanchez et al., 2016; Tekle, 2016).
Similarly, farmer access to information regarding the benefits of CA and how to implement it effectively is an important precursor for adoption and continued uptake over time (Llewellyn et al., 2012; Abdulai, 2016; Carlisle, 2016). Indeed, the complexity of CA systems and the need to understand how to use agronomic techniques to manage weed/ pest/ diseases and ensure sufficient crop nutrient availability mean that the implementation of CA without sufficient education around the management of its challenges is unlikely to result in long-term success. Effective extension and the development of partnerships between farmers, researchers and the private sector (e.g., seed companies, machinery suppliers) to refine CA systems and demonstrate their benefits is required to facilitate knowledge dissemination, increase CA uptake and reducing dis-adoption rates over time (Dauphin, 2003; Bellotti and Rochecouste, 2014; Bhan and Behera, 2014; de Freitas and Landers, 2014; Ares et al., 2015).
Economic Challenges
Conservation agriculture also needs to be demonstrated to farmers as a profitable way of farming before widespread adoption will occur. This may require a degree of structural change to address issues such as the lack of markets for produce (particularly for diversified crop rotations) and the inability to economically obtain required inputs such as seed, fertilizer, herbicide, and locally adapted machinery (Corbeels et al., 2014; Carlisle, 2016; Tekle, 2016; Brown et al., 2017). The adequate availability of fertilizers can be particularly important in areas with inherently low soil fertility where the production of sufficient quantities of crop residue for soil protection is difficult (Giller et al., 2015; Thierfelder et al., 2018). The presence of protectionist mechanisms, such as subsidies for the production of certain commodities, can also hinder adoption as these do not encourage the reduction of production costs and can act to decrease the profitability of CA practices (Carvalho and Lourenco, 2014; Tekle, 2016).
Additional assistance may also be required for poorer farmers who are less likely to adopt CA due to the initial investment required around establishment and the risk associated with decreased yields early in the adoption process (Abdulai, 2016; Brown et al., 2017; Ding, 2018; Harper et al., 2018). Policy initiatives to subsidize or incentivize NT, for example by increasing access to credit and the farm machinery required for NT operations (e.g., rental schemes, custom hire) can increase adoption (Bhan and Behera, 2014; Abdulai, 2016; Carlisle, 2016). However, ongoing support and the removal of larger institutional barriers to adoption are essential for farmers to continue with CA once initial incentives have been removed (Andersson and D'Souza, 2014; Brown et al., 2017; Chinse et al., 2019). Indeed, until institutional and/or social barriers are able to be overcome, CA is unlikely to be a successful system of sustainable intensification.
Conclusion
It is clear that when CA systems are well-designed and adapted to local conditions, they can improve the SOC content of many soils compared to conventional agricultural systems and that this can lead to significant improvements in soil physical, chemical, and biological properties and productive capacity. However, to increase the adoption of CA worldwide, it is critical that the system be adapted to specific climates, soil types and communities, particularly considering the farmer's investment capacity, and the availability of resources (Corbeels et al., 2014; Pannell et al., 2014; Giller et al., 2015). This may require some flexibility in approach to adapt agronomic management practices to local circumstances (Giller et al., 2015). The most effective systems are likely to be those that take into account the production objectives and constraints of farmers in a given region, and consider not only the technical aspects of CA, but also the socio-economic factors that make CA cost-effective and attractive for farmers (Tittonell et al., 2012; Giller et al., 2015). Given the initial yield loss that may be observed under CA before SOC increases and farmers learn how to effectively manage CA systems, some institutional support is essential to assist farmers with its adoption. However, given the need to reverse the trend of agriculturally induced soil degradation to ensure the long-term sustainability of agroecosystems, this is investment is likely to accrue significant long-term advantages for both individual farmers and society at large.
Author Contributions
KP, YD, and RD contributed to the design, writing, and editing of the manuscript.
Conflict of Interest
The authors declare that the research was conducted in the absence of any commercial or financial relationships that could be construed as a potential conflict of interest.
References
Abdulai, A. N. (2016). Impact of conservation agriculture technology on household welfare in Zambia. Agric. Econ. 47, 729–741. doi: 10.1111/agec.12269
Aguilera, E., Lassaletta, L., Gattinger, A., and Gimeno, B. S. (2013). Managing soil carbon for climate change mitigation and adaptation in Mediterranean cropping systems: A meta-analysis. Agric. Ecosyst. Environ. 168, 25–36. doi: 10.1016/j.agee.2013.02.003
Alvarez, R. (2005). A review of nitrogen fertilizer and conservation tillage effects on soil organic carbon storage. Soil Use Manage. 21, 38–52. doi: 10.1079/SUM2005291
Anderson, A. E., Hammac, W. A., Stott, D. E., and Tyner, W. E. (2020). An analysis of yield variation under soil conservation practices. J. Soil. Water. Conserv. 75, 103–111. doi: 10.2489/jswc.75.1.103
Andersson, J. A., and D'Souza, S. (2014). From adoption claims to understanding farmers and contexts: a literature review of Conservation Agriculture (CA) adoption among smallholder farmers in southern Africa. Agr. Ecosyst. Environ. 187, 116–132. doi: 10.1016/j.agee.2013.08.008
Angers, D. A., Bolinder, M. A., Carter, M. R., Gregorich, E. G., Drury, C. F., Liang, B. C., et al. (1997). Impact of tillage practices on organic carbon and nitrogen storage in cool, humid soils of eastern Canada. Soil Till. Res. 41, 191–201. doi: 10.1016/S0167-1987(96)01100-2
Araya, T., Cornelis, W. M., Nyssen, J., Govaerts, B., Getnet, F., Bauer, H., et al. (2012). Medium-term effects of conservation agriculture based cropping systems for sustainable soil and water management and crop productivity in the Ethiopian highlands. Field Crops Res. 132, 53–62. doi: 10.1016/j.fcr.2011.12.009
Ares, A., Thierfelder, C., Reyes, M., Eash, N. S., and Himmelstein, J. (2015). “Global perspectives on conservation agriculture for small households,” in Conservation Agriculture in Subsistence Farming: 22 Case Studies from South Asia and Beyond, eds C. Chan and J. Fantle-Lepczyk (Oxfordshire: CAB International), 22–54. doi: 10.1079/9781780644233.0022
Baudron, F., Tittonell, P., Corbeels, M., Letourmy, P., and Giller, K. E. (2012). Comparative performance of conservation agriculture and current smallholder farming practices in semi-arid Zimbabwe. Field Crops Res. 132, 117–128. doi: 10.1016/j.fcr.2011.09.008
Bayer, C., Lovato, T., Dieckow, J., Zanatta, J. A., and Mielniczuk, J. (2006a). A method for estimating coefficients of soil organic matter dynamics based on long-term experiments. Soil Tillage Res. 91, 217–226. doi: 10.1016/j.still.2005.12.006
Bayer, C., Martin-Neto, L., Mielniczuk, J., Pavinato, A., and Dieckow, J. (2006b). Carbon sequestration in two Brazilian Cerrado soils under no-till. Soil Till. Res. 86, 237–245. doi: 10.1016/j.still.2005.02.023
Beare, M. H., Coleman, D. C., Pohlad, B. R., and Wright, D. H. (1993). Residue placement and fungicide effects on fungal communities in conventional and no-tillage soils. Soil Sci. Soc. Am J. 57, 392–399. doi: 10.2136/sssaj1993.03615995005700020018x
Beare, M. H., Hendrix, P. F., Cabrera, M. L., and Coleman, D. C. (1994). Aggregate-protected and unprotected organic matter pools in conventional- and no-tillage soils. Soil Sci. Soc. Am J. 58, 787–795. doi: 10.2136/sssaj1994.03615995005800030021x
Bellotti, B., and Rochecouste, J. F. (2014). The development of conservation agriculture in Australia—Farmers as innovators. Int. Soil Wat. Cons. Res. 2, 21–34. doi: 10.1016/S2095-6339(15)30011-3
Bernoux, M., Cerri, C. C., Cerri, C. E. P., Siqueira-Neto, M., Metay, A., Perrin, A. S., et al. (2006). Cropping systems, carbon sequestration and erosion in Brazil, a review. Agron. Sustain. Develop. 26, 1–8. doi: 10.1051/agro:2005055
Beuchelt, T. D., Villa, C. T. C., Gohring, L., Rodriguez, V. M. H., Hellin, J., Sonder, K., et al. (2015). Social and income trade-offs of conservation agriculture practices on crop residue use in Mexico's central highlands. Agr. Syst. 134, 61–75. doi: 10.1016/j.agsy.2014.09.003
Bhan, S., and Behera, U. K. (2014). Conservation agriculture in India – Problems, prospects and policy issues. Int. Soil Wat. Cons. Res. 2, 1–12. doi: 10.1016/S2095-6339(15)30053-8
Blanco-Canqui, H., and Ruis, S. J. (2018). No-tillage and soil physical environment. Geoderma 326, 164–200. doi: 10.1016/j.geoderma.2018.03.011
Blanco-Canqui, H., Schlegel, A. J., and Heer, W. F. (2011). Soil-profile distribution of carbon and associated properties in no-till along a precipitation gradient in the central Great Plains. Agric. Ecosyst. Environ. 144, 107–116. doi: 10.1016/j.agee.2011.07.004
Bockus, W. W., and Shroyer, J. P. (1998). The impact of reduced tillage on soilborne plant pathogens. Annu. Rev. Phytopathol. 36, 485–500. doi: 10.1146/annurev.phyto.36.1.485
Braim, M. A., Chaney, K., and Hodgson, D. R. (1992). Effects of simplified cultivation on the growth and yield of spring barley on a sandy loam soil. 2. Soil physical properties and root growth; root: shoot relationships, inflow rates of nitrogen; water use. Soil Tillage Res. 22, 173–187. doi: 10.1016/0167-1987(92)90030-F
Bravo, C. A., Giraldez, J. V., Ordonez, R., Gonzalez, P., and Torres, F. P. (2007). Long-term influence of conservation tillage on chemical properties of surface horizon and legume crops yield in a vertisol of southern Spain. Soil Sci. 172, 141–148. doi: 10.1097/ss.0b013e31802db198
Briones, M. J. I., and Schmidt, O. (2017). Conventional tillage decreases the abundance and biomass of earthworms and alters their community structure in a global meta-analysis. Glob. Chang. Biol. 23, 4396–4419. doi: 10.1111/gcb.13744
Brouder, S. M., and Gomez-Macpherson, H. (2014). The impact of conservation agriculture on smallholder agricultural yields: A scoping review of the evidence. Agric. Ecosyst. Environ. 187, 11–32. doi: 10.1016/j.agee.2013.08.010
Brown, B., Nuberg, I., and Llewellyn, R. (2017). Negative evaluation of conservation agriculture: perspectives from African smallholder farmers. Int. J. Agr. Sustain. 15, 467–481. doi: 10.1080/14735903.2017.1336051
Camarotto, C., Dal Ferro, N., Piccoli, I., Polese, R., Furlan, L., Chiarini, F., et al. (2018). Conservation agriculture and cover crop practices to regulate water, carbon and nitrogen cycles in the low-lying Venetian plain. Catena 167, 236–249. doi: 10.1016/j.catena.2018.05.006
Carlisle, L. (2016). Factors influencing farmer adoption of soil health practices in the United States: a narrative review. Agroecol. Sust. Food Syst. 40, 583–613. doi: 10.1080/21683565.2016.1156596
Carter, M. R. (1991). Evaluation of shallow tillage for spring cereals on a fine sandy loam. 1. Growth and yield components, N accumulation and tillage economics. Soil Tillage Res. 21, 23–35. doi: 10.1016/0167-1987(91)90003-G
Carvalho, M., and Lourenco, E. (2014). Conservation agriculture - a portuguese case study. J. Agron. Crop Sci. 200, 317–324. doi: 10.1111/jac.12065
Chan, K. Y., Roberts, W. P., and Heenan, D. P. (1992). Organic carbon and associated properties of a red earth after 10 years rotation under different stubble and tillage practices. Aust. J. Soil Res. 30, 71–83. doi: 10.1071/SR9920071
Chinse, E., Dougill, N., and Stringer, L. (2019). Why do smallholder farmers dis-adopt conservation agriculture? Insights from Malawi. Land Degrad. Dev. 30, 533–543. doi: 10.1002/ldr.3190
Christopher, S. F., Lal, R., and Mishra, U. (2009). Regional study of no-till effects on carbon sequestration in the Midwestern United States. Soil Sci. Soc. Am. J. 73, 207–216. doi: 10.2136/sssaj2007.0336
Conceição, P. C., Dieckow, J., and Bayer, C. (2013). Combined role of no-tillage and cropping systems in soil carbon stocks and stabilization. Soil Till. Res. 129, 40–47. doi: 10.1016/j.still.2013.01.006
Cook, R. J., and Haglund, W. A. (1991). Wheat yield depression associated with conservation tillage caused by root pathogens in the soil not phytotoxins from the straw. Soil Biol. Biochem. 23, 1125–1132. doi: 10.1016/0038-0717(91)90024-E
Corbeels, M., de Graaff, J., Ndah, T. H., Penot, E., Baudron, F., Naudin, K., et al. (2014). Understanding the impact and adoption of conservation agriculture in Africa: A multi-scale analysis. Agric. Ecos. Environ. 187, 155–170. doi: 10.1016/j.agee.2013.10.011
Daigh, A. L. M., Dick, W. A., Helmers, M. J., Lal, R., Lauer, J. G., Nafziger, E., et al. (2018). Yields and yield stability of no-till and chisel-plow fields in the Midwestern US Corn Belt. Field Crops Res. 218, 243–253. doi: 10.1016/j.fcr.2017.04.002
Dalal, R. C. (1989). Long-term effects of no-tillage, crop residue, and nitrogen applications on properties of a vertisol. Soil Sci. Society Am. J. 53, 1511–1515. doi: 10.2136/sssaj1989.03615995005300050035x
Dalal, R. C., Wang, W., Allen, D. E., Reeves, S., and Menzies, N. W. (2011). Soil nitrogen and nitrogen-use efficiency under long-term no-till practice. Soil Sci. Society Am. J. 75, 2251–2261. doi: 10.2136/sssaj2010.0398
Dang, Y. P., Balzer, A., Crawford, M., Rincon-Florez, V., Liu, H., Melland, A. R., et al. (2018). Strategic tillage in conservation agricultural systems of north-eastern Australia: why, where, when and how? Enviro. Sci. Pollut. Res. 25, 1000–1015. doi: 10.1007/s11356-017-8937-1
Dang, Y. P., Seymour, N. P., Walker, S. R., Bell, M. J., and Freebairn, D. M. (2015). Strategic tillage in no-till farming systems in Australia's northern grains-growing regions: I. Drivers and implementation. Soil Till. Res. 152, 104–114. doi: 10.1016/j.still.2015.03.009
Dauphin, F. (2003). “Investing in conservation agriculture,” in Cons. Agric, ed L. Garcia Torres (Dordrecht: Kluwer Academic Publishers), 445–456. doi: 10.1007/978-94-017-1143-2_54
de Freitas, P. L., and Landers, J. N. (2014). The transformation of agriculture in Brazil through development and adoption of zero tillage conservation agriculture. Int. Soil Wat. Cons. Res. 2, 35–46. doi: 10.1016/S2095-6339(15)30012-5
Devkota, M., Devkota, K. P., Acharya, S., and McDonald, A. J. (2019). Increasing profitability, yields and yield stability through sustainable crop establishment practices in the rice-wheat systems of Nepal. Agric. Syst. 173, 414–423. doi: 10.1016/j.agsy.2019.03.022
Dhar, A. R., Islam, M. M., Jannat, A., and Ahmed, J. U. (2018). Adoption prospects and implication problems of practicing conservation agriculture in Bangladesh: a socioeconomic diagnosis. Soil Till. Res. 176, 77–84. doi: 10.1016/j.still.2017.11.003
Ding, Y. (2018). The role of government policies in the adoption of conservation tillage in China: a theoretical model. IOP Conf. Ser. Earth Environ. Sci. 108, 1–8. doi: 10.1088/1755-1315/108/4/042012
Doran, J. W., Elliott, E. T., and Paustian, K. (1998). Soil microbial activity, nitrogen cycling, and long-term changes in organic carbon pools as related to fallow tillage management. Soil Tillage Res. 49, 3–18. doi: 10.1016/S0167-1987(98)00150-0
dos Santos, N. Z., Dieckow, J., Bayer, C., Molin, R., Favaretto, N., Pauletti, V., et al. (2011). Forages, cover crops and related shoot and root additions in no-till rotations to C sequestration in a subtropical Ferralsol. Soil Till. Res. 111, 208–218. doi: 10.1016/j.still.2010.10.006
Dou, F., Wright, A. L., and Hons, F. M. (2008). Sensitivity of labile soil organic carbon to tillage in wheat-based cropping systems. Soil Sci. Soc. Am. J. 72, 1445–1453. doi: 10.2136/sssaj2007.0230
Du, Z., Angers, D. A., Ren, T., Zhang, Q., and Li, G. (2017). The effect of no-till on organic C storage in Chinese soils should not be overemphasized: A meta-analysis. Agric. Ecosyst. Environ. 236, 1–11. doi: 10.1016/j.agee.2016.11.007
Duiker, S. W., and Beegle, D. B. (2006). Soil fertility distributions in long-term no-till, chisel/disk and moldboard plow/disk systems. Soil Tillage Res. 88, 30–41. doi: 10.1016/j.still.2005.04.004
Duiker, S. W., and Lal, R. (2002). “Mulch rate and tillage effects on carbon sequestration and CO2 flux in an Alfisol in central Ohio,” in Agricultural Practices and Policies for Carbon Sequestration in Soil, eds J. M. Kimble, R. Lal, and R. F. Follet (Boca Raton, FL: Lewis Publishers), 53–61. doi: 10.1201/9781420032291.pt2
Fageria, N. K., Baligar, V. C., and Bailey, B. A. (2005). Role of cover crops in improving soil and row crop productivity. Commun. Soil Sci. Plant Anal. 36, 2733–2757. doi: 10.1080/00103620500303939
FAO (2019). Conservation Agriculture. Available online at: http://www.fao.org/conservation-agriculture/overview/what-is-conservation-agriculture/en/ (accessed August 2019).
Felton, W. L., Marcellos, H., and Martin, R. J. (1995). A comparison of three fallow management strategies for the long-term productivity of wheat in northern New South Wales. Aust. J. Exp. Agric. 35, 915–921. doi: 10.1071/EA9950915
Francaviglia, R., Di Bene, C., Farina, R., and Salvati, L. (2017). Soil organic carbon sequestration and tillage systems in the Mediterranean Basin: a data mining approach. Nut. Cycling Agroecos. 107, 125–137. doi: 10.1007/s10705-016-9820-z
Franzluebbers, A. J. (2010). Achieving soil organic carbon sequestration with conservation agricultural systems in the Southeastern United States. Soil Sci. Soc. Am. J. 74, 347–357. doi: 10.2136/sssaj2009.0079
Franzluebbers, A. J., and Hons, F. M. (1996). Soil-profile distribution of primary and secondary plant-available nutrients under conventional and no tillage. Soil Tillage Res. 39, 229–239. doi: 10.1016/S0167-1987(96)01056-2
Gabriel, J. L., Garrido, A., and Quemada, M. (2013). Cover crops effect on farm benefits and nitrate leaching: Linking economic and environmental analysis. Agric. Syst. 121, 23–32. doi: 10.1016/j.agsy.2013.06.004
Garcia-Palacios, P., Alarcon, M. R., Tenorio, J. L., and Moreno, S. S. (2019). Ecological intensification of agriculture in drylands. J. Arid Environ. 167, 101–105. doi: 10.1016/j.jaridenv.2019.04.014
Giller, K. E., Andersson, J. A., Corbeels, M., Kirkegaard, J., Mortensen, D., Erenstein, O., et al. (2015). Beyond conservation agriculture. Front. Plant Sci. 6:870. doi: 10.3389/fpls.2015.00870
González-Chávez, M. D. C. A., Aitkenhead-Peterson, J. A., Gentry, T. J., Zuberer, D., Hons, F., and Loeppert, R. (2010). Soil microbial community, C, N, and P responses to long-term tillage and crop rotation. Soil Tillage Res. 106, 285–293. doi: 10.1016/j.still.2009.11.008
Gonzalez-Sanchez, E. J., Kassam, A., Basch, G., Streit, B., Holgado-Cabrera, A., and Trivino-Tarradas, P. (2016). Conservation Agriculture and its contribution to the achievement of agri-environmental and economic challenges in Europe. Aims Agric. Food 1, 387–408. doi: 10.3934/agrfood.2016.4.387
González-Sánchez, E. J., Ordóñez-Fernández, R., Carbonell-Bojollo, R., Veroz-González, O., and Gil-Ribes, J. A. (2012). Meta-analysis on atmospheric carbon capture in Spain through the use of conservation agriculture. Soil Till. Res. 122, 52–60. doi: 10.1016/j.still.2012.03.001
Gonzalez-Sanchez, E. J., Veroz-Gonzalez, O., Conway, G., Moreno-Garcia, M., Kassam, A., Mkomwa, S., et al. (2019). Meta-analysis on carbon sequestration through Conservation Agriculture in Africa. Soil Tillage Res. 190, 22–30. doi: 10.1016/j.still.2019.02.020
Govaerts, B., Fuentes, M., Mezzalama, M., Nicol, J. M., Deckers, J., Etchevers, J. D., et al. (2007a). Infiltration, soil moisture, root rot and nematode populations after 12 years of different tillage, residue and crop rotation managements. Soil Tillage Res. 94, 209–219. doi: 10.1016/j.still.2006.07.013
Govaerts, B., Mezzalama, M., Sayre, K. D., Crossa, J., Lichter, K., Troch, V., et al. (2008). Long-term consequences of tillage, residue management, and crop rotation on selected soil micro-flora groups in the subtropical highlands. Appl. Soil Ecol. 38, 197–210. doi: 10.1016/j.apsoil.2007.10.009
Govaerts, B., Mezzalama, M., Unno, Y., Sayre, K. D., Luna-Guido, M., Vanherck, K., et al. (2007b). Influence of tillage, residue management, and crop rotation on soil microbial biomass and catabolic diversity. Appl. Soil Ecol. 37, 18–30. doi: 10.1016/j.apsoil.2007.03.006
Gregorich, E. G., Rochette, P., VandenBygaart, A. J., and Angers, D. A. (2005). Greenhouse gas contributions of agricultural soils and potential mitigation practices in Eastern Canada. Soil Till. Res. 83, 53–72. doi: 10.1016/j.still.2005.02.009
Halvorson, A. D., Black, A. L., Krupinsky, J. M., Merrill, S. D., Wienhold, B. J., and Tanaka, D. L. (2000). Spring wheat response to tillage and nitrogen fertilization in rotation with sunflower and winter wheat. Agron. J. 92, 136–144. doi: 10.2134/agronj2000.921136x
Hamblin, A. P. (1984). The effect of tillage on soil surface properties and the water balance of a Xeralfic Alfisol. Soil Tillage Res. 4, 534–559. doi: 10.1016/0167-1987(84)90004-7
Hansen, N. C., Allen, B. L., Baumhardt, R. L., and Lyon, D. J. (2012). Research achievements and adoption of no-till, dryland cropping in the semi-arid U.S. Great Plains. Field Crops Res. 132, 196–203. doi: 10.1016/j.fcr.2012.02.021
Harper, J. K., Roth, G. W., Garalejic, B., and Skrbic, N. (2018). Programs to promote adoption of conservation tillage: a Serbian case study. Land Use Pol. 78, 295–302. doi: 10.1016/j.landusepol.2018.06.028
Hatfield, J. L., Sauer, T. J., and Prueger, J. H. (2001). Managing soils to achieve greater water use efficiency. Agron. J. 93, 271–280. doi: 10.2134/agronj2001.932271x
Heenan, D. P., and Taylor, A. C. (1995). Soil pH decline in relation to rotation, tillage, stubble retention and nitrogen fertilizer in S.E. Australia. Soil Use Manag. 11, 4–9. doi: 10.1111/j.1475-2743.1995.tb00487.x
Helgason, B. L., Walley, F. L., and Germida, J. J. (2009). Fungal and bacterial abundance in long-term no-till and intensive-till soils of the northern Great Plains. Soil Sci. Soc. Am. J. 73, 120–127. doi: 10.2136/sssaj2007.0392
Helgason, B. L., Walley, F. L., and Germida, J. J. (2010). No-till soil management increases microbial biomass and alters community profiles in soil aggregates. Appl. Soil Ecol. 46, 390–397. doi: 10.1016/j.apsoil.2010.10.002
Hemmat, A., and Eskandari, I. (2004). Tillage system effects upon productivity of a dryland winter wheat–chickpea rotation in the northwest region of Iran. Soil Tillage Res. 78, 69–81. doi: 10.1016/j.still.2004.02.013
Hemmat, A., and Eskandari, I. (2006). Dryland winter wheat response to conesrvation tillage in a continuous cropping system in northwestern Iran. Soil Tillage Res. 86, 99–109. doi: 10.1016/j.still.2005.02.003
Hendrix, P. F., Parmelee, R. W., Crossley, D. A Jr, Coleman, D. C., Odum, E. P., and Groffman, P. M. (1986). Detritus food webs in conventional and no-tillage agroecosystems. Bioscience 36, 374–380. doi: 10.2307/1310259
Huggins, D. R., Allmaras, R. R., Clapp, C. E., Lamb, J. A., and Randall, G. W. (2007). Corn-Soybean Sequence and Tillage Effects on Soil Carbon Dynamics and Storage. Soil Sci. Soc. Am. J. 71, 145–154. doi: 10.2136/sssaj2005.0231
Ismail, I., Blevins, R. L., and Frye, W. W. (1994). Long-term no-tillage effects on soil properties and continuous corn yields. Soil Sci. Soc. Am J. 58, 193–198. doi: 10.2136/sssaj1994.03615995005800010028x
Jat, H. S., Datta, A., Choudhary, M., Sharma, P. C., Yadav, A. K., Choudhary, V., et al. (2019). Climate Smart Agriculture practices improve soil organic carbon pools, biological properties and crop productivity in cereal-based systems of North-West India. CATENA 181:104059. doi: 10.1016/j.catena.2019.05.005
Jat, R. K., Sapkota, T. B., Singh, R. G., Jat, M. L., Kumar, M., and Gupta, R. K. (2014). Seven years of conservation agriculture in a rice–wheat rotation of Eastern Gangetic Plains of South Asia: Yield trends and economic profitability. Field Crops Res. 164, 199–210. doi: 10.1016/j.fcr.2014.04.015
Johnson, J. M. F., Reicosky, D. C., Allmaras, R. R., Sauer, T. J., Venterea, R. T., and Dell, C. J. (2005). Greenhouse gas contributions and mitigation potential of agriculture in the central USA. Soil Till. Res. 83, 73–94. doi: 10.1016/j.still.2005.02.010
Jones, O. R., Hauser, V. L., and Popham, T. W. (1994). No-tillage effects on infiltration, runoff, and water conservation on dryland. Trans. ASAE. 37, 473–479. doi: 10.13031/2013.28099
Kabir, Z., O'Halloran, I. P., Fyles, J. W., and Hamel, C. (1997). Seasonal changes of arbuscular mycorrhizal fungi as affected by tillage practices and fertilization: Hyphal density and mycorrhizal root colonization. Plant Soil 192, 285–293. doi: 10.1023/A:1004205828485
Känkänen, H., Alakukku, L., Salo, Y., and Pitkänen, T. (2011). Growth and yield of spring cereals during transition to zero tillage on clay soils. Eur. J. Agron. 34, 35–45. doi: 10.1016/j.eja.2010.10.002
Kassam, A., Friedrich, T., Shaxson, F., and Pretty, J. (2009). The spread of Conservation Agriculture: justification, sustainability and uptake. Inter. J. Agr. Sust. 7, 292–320. doi: 10.3763/ijas.2009.0477
Kaye, J. P., and Quemada, M. (2017). Using cover crops to mitigate and adapt to climate change. A review. Agron. Sustain. Dev. 37:4. doi: 10.1007/s13593-016-0410-x
Kienzler, K. M., Lamers, J. P. A., McDonald, A., Mirzabaev, A., Ibragimov, N., Egamberdiev, O., et al. (2012). Conservation agriculture in Central Asia—What do we know and where do we go from here? Field Crops Res. 132, 95–105. doi: 10.1016/j.fcr.2011.12.008
Kirkby, C. A., Richardson, A. E., Wade, L. J., Conyers, M., and Kirkegaard, J. A. (2016). Inorganic nutrients increase humification efficiency and C-Sequestration in an Annually cropped soil. PLoS ONE 11:e0153698. doi: 10.1371/journal.pone.0153698
Kirkegaard, J. A., Conyers, M. K., Hunt, J. R., Kirkby, C. A., Watt, M., and Rebetzke, G. J. (2014). Sense and nonsense in conservation agriculture: Principles, pragmatism and productivity in Australian mixed farming systems. Agric. Ecos. Environ. 187, 133–145. doi: 10.1016/j.agee.2013.08.011
Kladivko, E. J. (2001). Tillage systems and soil ecology. Soil Tillage Res. 61, 61–76. doi: 10.1016/S0167-1987(01)00179-9
Knapp, S., and van der Heijden, M. G. A. (2018). A global meta-analysis of yield stability in organic and conservation agriculture. Nat. Commun. 9:3632. doi: 10.1038/s41467-018-05956-1
Kumar, V., Jat, H. S., Sharma, P. C., Balwinder, S., Gathala, M. K., Malik, R. K., et al. (2018). Can productivity and profitability be enhanced in intensively managed cereal systems while reducing the environmental footprint of production? Assessing sustainable intensification options in the breadbasket of India. Agric. Ecosyst. Environ. 252, 132–147. doi: 10.1016/j.agee.2017.10.006
LaCanne, C. E., and Lundgren, J. G. (2018). Regenerative agriculture: merging farming and natural resource conservation profitably. Peerj 6:e4428. doi: 10.7717/peerj.4428
Lal, R. (1999). Long-term tillage and wheel traffic effects on soil quality for two central Ohio soils. J. Sust. Agric. 14, 67–84. doi: 10.1300/J064v14n04_07
Lal, R. (2015a). Sequestering carbon and increasing productivity by conservation agriculture. JSWC. 70, 55A−62A. doi: 10.2489/jswc.70.3.55A
Lal, R. (2015b). A system approach to conservation agriculture. JSWC. 70:82A. doi: 10.2489/jswc.70.4.82A
Lal, R. (2018). Sustainable intensification of China's agroecosystems by conservation agriculture. Inter. Soil Water Conserv. Res. 6, 1–12. doi: 10.1016/j.iswcr.2017.11.001
Lampurlanés, J., and Cantero-Martínez, C. (2006). Hydraulic conductivity, residue cover and soil surface roughness under different tillage systems in semiarid conditions. Soil Tillage Res. 85, 13–26. doi: 10.1016/j.still.2004.11.006
Lampurlanés, J., Plaza-Bonilla, D., Álvaro-Fuentes, J., and Cantero-Martínez, C. (2016). Long-term analysis of soil water conservation and crop yield under different tillage systems in Mediterranean rainfed conditions. Field Crops Res. 189, 59–67. doi: 10.1016/j.fcr.2016.02.010
Li, H., Gao, H., Wu, H., Li, W., Wang, X., and He, J. (2007). Effects of 15 years of conservation tillage on soil structure and productivity of wheat cultivation in northern China. Aust. J. Agric. Res. 45, 344–350. doi: 10.1071/SR07003
Li, J., Huang, L., Zhang, J., Coulter, J. A., Li, L., and Gan, Y. (2019a). Diversifying crop rotation improves system robustness. Agric. Ecosyst. Environ. 39:38. doi: 10.1007/s13593-019-0584-0
Li, Y., Li, Z., Cui, S., Jagadamma, S., and Zhang, Q. P. (2019b). Residue retention and minimum tillage improve physical environment of the soil in croplands: a global meta-analysis. Soil Tillage Res. 194:104292. doi: 10.1016/j.still.2019.06.009
Liang, B. C., McConkey, B. G., Campbell, C. A., Johnston, A. M., and Moulin, A. P. (2002). “Short-term crop rotation and tillage effects on soil organic carbon on the Canadian prarires,” in Agricultural Practices and Policies for Carbon Sequestration in Soil, eds J. M. Kimble, R. Lal, and R. F. Follet (Boca Raton, FL: Lewis Publishers), 287–293. doi: 10.1201/9781420032291.ch27
Limousin, G., and Tessier, D. (2007). Effects of no-tillage on chemical gradients and topsoil acidification. Soil Tillage Res. 92, 167–174. doi: 10.1016/j.still.2006.02.003
Liu, C., Lu, M., Cui, J., Li, B., and Fang, C. (2014). Effects of straw carbon input on carbon dynamics in agricultural soils: a meta-analysis. Global Change Bio. 20, 1366–1381. doi: 10.1111/gcb.12517
Llewellyn, R. S., D'Emden, F. H., and Kuehne, G. (2012). Extensive use of no-tillage in grain growing regions of Australia. Field Crop Res. 132, 204–212. doi: 10.1016/j.fcr.2012.03.013
Loch, R. J., and Coughlan, K. J. (1984). Effects of zero tillage and stubble retention on some properties of a cracking clay Aust. J. Soil Res. 22, 91–98. doi: 10.1071/SR9840091
Luo, Z., Wang, E., and Sun, O. J. (2010). Can no-tillage stimulate carbon sequestration in agricultural soils? A meta-analysis of paired experiments. Agric. Ecosyst. Environ. 139, 244–231. doi: 10.1016/j.agee.2010.08.006
Lupwayi, N. Z., Arshad, M. A., Rice, W. A., and Clayton, G. W. (2001). Bacterial diversity in water-stable aggregates of soils under conventional and zero tillage management. Appl. Soil Ecol. 16, 251–261. doi: 10.1016/S0929-1393(00)00123-2
Lyon, D., Bruce, S., Vyn, T., and Peterson, G. (2004). “Achievements and future challenges in conservation tillage,” in New Directions for a Diverse Planet. Proceedings of the 4th International Crop Science Congress (Brisbane: Published on CDROM). Available online at: www.cropscience.org.au
Lyon, D. J., Stroup, W. W., and Brown, R. E. (1998). Crop production and soil water storage in long-term winter wheat–fallow tillage experiments. Soil Tillage Res. 49, 19–27. doi: 10.1016/S0167-1987(98)00151-2
Mangalassery, S., Mooney, S. J., Sparkes, D. L., Fraser, W. T., and Sjögersten, S. (2015a). Impacts of zero tillage on soil enzyme activities, microbial characteristics and organic matter functional chemistry in temperate soils. Eur. J. Soil Biol. 68, 9–17. doi: 10.1016/j.ejsobi.2015.03.001
Mangalassery, S., SjÖGersten, S., Sparkes, D. L., and Mooney, S. J. (2015b). Examining the potential for climate change mitigation from zero tillage. J. Agric. Sci. 153, 1151–1173. doi: 10.1017/S0021859614001002
Marley, J. M., and Littler, J. W. (1989). Winter cereal production on the Darling Downs-an 11 year study of fallowing practices. Aust. J. Exp. Agric. 29, 807–827. doi: 10.1071/EA9890807
Mbuthia, L. W., Acosta-Martínez, V., DeBruyn, J., Schaeffer, S., Tyler, D., Odoi, E., et al. (2015). Long term tillage, cover crop, and fertilization effects on microbial community structure, activity: Implications for soil quality. Soil Biol. Biochem. 89, 24–34. doi: 10.1016/j.soilbio.2015.06.016
McGarry, D., Bridge, B. J., and Radford, B. J. (2000). Contrasting soil physical properties after zero and traditional tillage of an alluvial soil in the semi-arid subtropics. Soil Tillage Res. 53, 105–115. doi: 10.1016/S0167-1987(99)00091-4
Montgomery, D. R. (2007). Soil erosion and agricultural sustainability. Proc. Natl. Acad. Sci. U.S.A. 104, 13268–13272. doi: 10.1073/pnas.0611508104
Mrabet, R., Moussadek, R., Fadlaoui, A., and van Ranst, E. (2012). Conservation agriculture in dry areas of Morocco. Field Crops Res. 132, 84–94. doi: 10.1016/j.fcr.2011.11.017
Ngwira, A. R., Aune, J. B., and Mkwinda, S. (2012). On-farm evaluation of yield and economic benefit of short term maize legume intercropping systems under conservation agriculture in Malawi. Field Crops Res. 132, 149–157. doi: 10.1016/j.fcr.2011.12.014
Nielsen, R. G. H., Unger, P. W., and Millar, G. (2005). Efficient water use in dryland cropping systems in the Great Plains. Agron. J. 97, 364–372. doi: 10.2134/agronj2005.0364
Norwood, C. (1994). Profile water distribution and grain yield as affected by cropping system and tillage. Agron. J. 86, 558–563. doi: 10.2134/agronj1994.00021962008600030019x
Nyagumbo, I., Mkuhlani, S., Pisa, C., Kamalongo, D., Dias, D., and Mekuria, M. (2016). Maize yield effects of conservation agriculture based maize-legume cropping systems in contrasting agro-ecologies of Malawi and Mozambique. NCA 105, 275–290. doi: 10.1007/s10705-015-9733-2
Nyamangara, J., Marondedze, A., Masvaya, E. N., Mawodza, T., Nyawasha, R., Nyengerai, K., et al. (2014). Influence of basinbased conservation agriculture on selected soil quality parameters under smallholder farming in Zimbabwe. Soil Use Manage. 30, 550–559. doi: 10.1111/sum.12149
Ogle, S., Alsaker, C., Baldock, J., Bernoux, M., Breidt, F., McConkey, B. G., et al. (2019). Climate and soil characteristics determine where No-Till management can store carbon in soils and mitigate greenhouse gas emissions. Sci. Rep. 9:11665. doi: 10.1038/s41598-019-47861-7
Ogle, S. M., Breidt, F. J., and Paustian, K. (2005). Agricultural management impacts on soil organic carbon storage under moist and dry climatic conditions of temperate and tropical regions. Biogeochem. 72, 87–121. doi: 10.1007/s10533-004-0360-2
O'Leary, G. J., and Connor, D. J. (1997a). Stubble retention and tillage in a semi-arid environment: 1. Soil water accumulation during fallow. Field Crops Res. 52, 209–219. doi: 10.1016/S0378-4290(97)00034-8
O'Leary, G. J., and Connor, D. J. (1997b). Stubble retention and tillage in a semi-arid environment: 2. Soil mineral nitrogen accumulation during fallow. Field Crops Res. 52, 221–229. doi: 10.1016/S0378-4290(97)00035-X
Olson, K. R. (2013). Soil organic carbon sequestration, storage, retention and loss in U.S. croplands: Issues paper for protocol development. Geoderma 195–196, 201–206. doi: 10.1016/j.geoderma.2012.12.004
Page, K. L., Dang, Y. P., Dalal, R. C., Reeves, S., Thomas, G., Wang, W., et al. (2019). Changes in soil water storage with no-tillage and crop residue retention on a Vertisol: Impact on productivity and profitability over a 50 year period. Soil Tillage Res. 194:104319. doi: 10.1016/j.still.2019.104319
Page, K. L., Dang, Y., and Dalal, R. C. (2013). Impacts of conservation tillage on soil quality, including soil-borne crop diseases, with a focus on semi-arid grain cropping systems. Aust. Plant Path. 42, 363–377. doi: 10.1007/s13313-013-0198-y
Palm, C., Blanco-Canqui, H., DeClerck, F., Gatere, L., and Grace, P. (2014). Conservation agriculture and ecosystem services: an overview. Agric. Ecosyst. Environ. 187, 87–105. doi: 10.1016/j.agee.2013.10.010
Pankhurst, C. E., Hawke, B. G., McDonald, H. J., Kirby, C. A., Buckerfield, J. C., Michelsen, P., et al. (1995a). Evaluation of soil biological properties as potential bioindicators of soil health. Aust. J. Exp. Agric. 35, 1015–1028. doi: 10.1071/EA9951015
Pankhurst, C. E., Hawke, B. G., McDonald, H. J., Kirby, C. A., Buckerfield, J. C., Michelsen, P., et al. (1995b). Root-lesion nematode (Pratylenchus thornei) limits response of wheat but not barley to stored soil moisture in the Hermitage long-term tillage experiment. Aust. J. Exp. Agric. 35, 1049–1055. doi: 10.1071/EA9951049
Pankhurst, C. E., Kirby, J. M., Hawke, B. G., and Harch, B. D. (2002a). Impact of a change in tillage and crop residue management practice on soil chemical and microbiological properties in a cereal-producing red duplex soil in NSW, Australia. Biol. Fertil. Soils 35, 189–196. doi: 10.1007/s00374-002-0459-3
Pankhurst, C. E., McDonald, H. J., Hawke, B. G., and Kirkby, C. A. (2002b). Effect of tillage and stubble management on chemical and microbiological properties and the development of suppression towards cereal root disease in soils from two sites in NSW, Australia. Soil Biol. Biochem. 34, 833–840. doi: 10.1016/S0038-0717(02)00014-7
Pannell, D. J., Llewellyn, R. S., and Corbeels, M. (2014). The farm-level economics of conservation agriculture for resource-poor farmers. Agric. Ecosyst. Environ. 187, 52–64. doi: 10.1016/j.agee.2013.10.014
Paulitz, T. C., Schroeder, K. L., and Schillinger, W. F. (2010). Soilborne pathogens of cereals in an irrigated cropping System: Effects of tillage, residue management, and crop rotation. Plant Dis. 94, 61–68. doi: 10.1094/PDIS-94-1-0061
Peters, R. D., Sturz, A. V., Carter, M. R., and Sanderson, J. B. (2003). Developing disease-suppressive soils through crop rotation and tillage management practices. Soil Tillage Res. 72, 181–192. doi: 10.1016/S0167-1987(03)00087-4
Pheap, S., Lefevre, C., Thoumazeau, A., Leng, V., Boulakia, S., Koy, R., et al. (2019). Multi-functional assessment of soil health under Conservation Agriculture in Cambodia. Soil Tillage Res. 194:104349. doi: 10.1016/j.still.2019.104349
Pittelkow, C. M., Liang, X., Linquist, B. A., van Groenigen, K. J., Lee, J., Lundy, M. E., et al. (2015). Productivity limits and potentials of the principles of conservation agriculture. Nature 517, 365–368. doi: 10.1038/nature13809
Powlson, D. S., Stirling, C. M., Thierfelder, C., White, R. P., and Jat, M. L. (2016). Does conservation agriculture deliver climate change mitigation through soil carbon sequestration in tropical agro-ecosystems? Agric. Ecosyst. Environ. 220, 164–174. doi: 10.1016/j.agee.2016.01.005
Pradhan, A., Chan, C., Roul, P. K., Halbrendt, J., and Sipes, B. (2018). Potential of conservation agriculture (CA) for climate change adaptation and food security under rainfed uplands of India: a transdisciplinary approach. Agric. Syst. 163, 27–35. doi: 10.1016/j.agsy.2017.01.002
Puget, P., and Lal, R. (2005). Soil organic carbon and nitrogen in a Mollisol in central Ohio as affected by tillage and land use. Soil Till. Res. 80, 201–213. doi: 10.1016/j.still.2004.03.018
Qin, S. P., He, X. H., Hu, C. S., Zhang, Y. M., and Dong, W. X. (2010). Responses of soil chemical and microbial indicators to conservational tillage versus traditional tillage in the North China Plain. Eur. J. Soil Biol. 46, 243–247. doi: 10.1016/j.ejsobi.2010.04.006
Radford, B. J., Key, A. J., Robertson, L. N., and Thomas, G. A. (1995). Conservation tillage increases soil water storage, soil animal populations, grain yield, and response to fertiliser in the semi-arid subtropics. Aust. J. Exp. Agric. 35, 223–232. doi: 10.1071/EA9950223
Radford, B. J., and Thornton, C. M. (2011). Effects of 27 years of reduced tillage practices on soil properties and crop performance in the semi-arid subtropics of Australia. Inter. J. Energy Environ. Econom. 19, 565–588.
Rahman, L., Chan, K. Y., and Heenan, D. P. (2007). Impact of tillage, stubble management and crop rotation on nematode populations in a long-term field experiment. Soil Tillage Res. 95, 110–119. doi: 10.1016/j.still.2006.11.008
Raphael, J. P. A., Calonego, J. C., Milori, D. M. B. P., and Rosolem, C. A. (2016). Soil organic matter in crop rotations under no-till. Soil Tillage Res. 155, 45–53. doi: 10.1016/j.still.2015.07.020
Rhoton, F. E. (2000). Influence of time on soil response to no-till practices. Soil Sci. Soc. Am. J. 64, 700–709. doi: 10.2136/sssaj2000.642700x
Rochette, P. (2008). No-till only increases N2O emissions in poorly-aerated soils. Soil Till. Res. 101, 97–100. doi: 10.1016/j.still.2008.07.011
Roget, D. K., Neate, S. M., and Rovira, A. D. (1996). Effect of sowing point design and tillage practice on the incidence of rhizoctonia root rot, take-all and cereal cyst nematode in wheat and barley. Aust. J. Exp. Agric. 36, 683–693. doi: 10.1071/EA9960683
Roper, M. M., and Gupta, V. V. S. R. (1995). Management practices and soil biota. Aust. J. Soil Res. 33, 321–339. doi: 10.1071/SR9950321
Rusinamhodzi, L., Corbeels, M., van Wijk, M. T., Rufino, M. C., Nyamangara, J., and Giller, K. E. (2011). A meta-analysis of long-term effects of conservation agriculture on maize grain yield under rain-fed conditions. Agron. Sustain. Dev. 31:657. doi: 10.1007/s13593-011-0040-2
Sa, J. C. D., Cerri, C. C., Lal, R., Dick, W. A., Piccolo, M. D., and Feigl, B. E. (2009). Soil organic carbon and fertility interactions affected by a tillage chronosequence in a Brazilian Oxisol. Soil Tillage Res. 104, 56–64. doi: 10.1016/j.still.2008.11.007
Sithole, N. J., and Magwaza, L. S. (2019). Long-term changes of soil chemical characteristics and maize yield in no-till conservation agriculture in a semi-arid environment of South Africa. Soil Tillage Res. 194:104317. doi: 10.1016/j.still.2019.104317
Six, J., Elliott, E. T., and Paustian, K. (2000). Soil macroaggregate turnover and microaggregate formation: a mechanism for C sequestration under no-tillage agriculture. Soil Bio. Biochem. 32, 2099–2103. doi: 10.1016/S0038-0717(00)00179-6
Six, J., Feller, C., Denef, K., Ogle, S., De Moraes Sa, J. C., and Albrecht, A. (2002). Soil organic matter, biota and aggregation in temperate and tropical soils - Effects of no-tillage. Agronomie 22, 755–775. doi: 10.1051/agro:2002043
Soane, B. D., Ball, B. C., Arvidsson, J., Basch, G., Moreno, F., and Roger-Estrade, J. (2012). No-till in northern, western and south-western Europe: a review of problems and opportunities for crop production and the environment. Soil Tillage Res. 118, 66–87. doi: 10.1016/j.still.2011.10.015
Somasundaram, J., Salikram, M., Sinha, N. K., Mohanty, M., Chaudhary, R. S., Dalal, R. C., et al. (2019). Conservation agriculture effects on soil properties and crop productivity in a semiarid region of India. Soil Res. 57, 187–199. doi: 10.1071/SR18145
Spurgeon, D. J., Keith, A. M., Schmidt, O., Lammertsma, D. R., and Faber, J. H. (2013). Land-use and land-management change: relationships with earthworm and fungi communities and soil structural properties. BMC Ecol. 13:46. doi: 10.1186/1472-6785-13-46
Stagnari, F., Ramazzotti, S., and Pisante, M. (2009). “Conservation agriculture: a different approach for crop production through sustainable soil and water management: a review,” in Organic Farming, Pest Control and Remediation of Soil Pollutants, ed E. Lichtfouse (Dordrecht: Springer Science and Business Media), 55–83. doi: 10.1007/978-1-4020-9654-9_5
Steinbach, H. S., and Alvarez, R. (2006). Changes in soil organic carbon contents and nitrous oxide emissions after introduction of No-Till in pampean agroecosystems. J. Environ. Qual. 35, 3–13. doi: 10.2134/jeq2005.0050
Steward, P. R., Dougill, A. J., Thierfelder, C., Pittelkow, C. M., Stringer, L. C., Kudzala, M., et al. (2018). The adaptive capacity of maize-based conservation agriculture systems to climate stress in tropical and subtropical environments: a meta-regression of yields. Agric. Ecosyst. Environ. 251, 194–202. doi: 10.1016/j.agee.2017.09.019
Sun, L., Wang, R., Li, J., Wang, Q., Lyu, W., Wang, X., et al. (2019). Reasonable fertilization improves the conservation tillage benefit for soil water use and yield of rain-fed winter wheat: a case study from the Loess Plateau, China. Field Crops Res. 242:107589. doi: 10.1016/j.fcr.2019.107589
Tarkalson, D. D., Hergert, G. W., and Cassman, K. G. (2006). Long-term effects of tillage on soil chemcial properties and grain yields of a dryland winter wheat-sorghum/corn-fallow roration in the great plains. Agron. J. 98, 26–33. doi: 10.2134/agronj2004.0240
Tekle, A. T. (2016). Adaptation and Constraints of Conservation Agriculture. J. Bio. Agric. Healthcare 6, 1–14.
TerAvest, D., Wandschneider, P. R., Thierfelder, C., and Reganold, J. P. (2019). Diversifying conservation agriculture and conventional tillage cropping systems to improve the wellbeing of smallholder farmers in Malawi. Agric. Syst. 171, 23–35. doi: 10.1016/j.agsy.2019.01.004
Tessier, S., Peru, M., Dyck, F. B., Zentner, F. P., and Campbell, C. A. (1990). Conservation tillage for spring wheat production in semi-arid Saskatchewan. Soil Tillage Res. 18, 73–89. doi: 10.1016/0167-1987(90)90094-T
Thierfelder, C., Baudron, F., Setimela, P., Nyagumbo, I., Mupangwa, W., Mhlanga, B., et al. (2018). Complementary practices supporting conservation agriculture in southern Africa. A review. Agron. Sustain. Dev. 38:16. doi: 10.1007/s13593-018-0492-8
Thierfelder, C., Matemba-Mutasa, R., and Rusinamhodzi, L. (2015). Yield response of maize (Zea mays L.) to conservation agriculture cropping system in Southern Africa. Soil Tillage Res. 146, 230–242. doi: 10.1016/j.still.2014.10.015
Thomas, G. A., Dalal, R. C., and Standley, J. (2007). No-till effects on organic matter, pH, cation exchange capacity and nutrient distribution in a Luvisol in the semi-arid subtropics. Soil Tillage Res. 94, 295–304. doi: 10.1016/j.still.2006.08.005
Thomas, G. A., Gibson, G., Nielsen, R. G. H., Martin, W. D., and Radford, B. J. (1995). Effects of tillge, stubble, gypsum, and nitrogen fertiliser on cereal cropping on a red-brown earth in south-west Queensland. Aust. J. Exp. Agric. 35, 997–1008. doi: 10.1071/EA9950997
Thompson, J. P. (1992). Soil biotic and biochemical factors in a long-term tillage and stubble management experiment on a vertisol. 2. Nitrogen deficiency with zero tillage and stubble retention. Soil Tillage Res. 22, 339–361. doi: 10.1016/0167-1987(92)90048-G
Tisdall, J. M., and Oades, J. M. (1982). Organic matter and water-stable aggregates in soils. J. Soil Sci. 33, 141–163. doi: 10.1111/j.1365-2389.1982.tb01755.x
Tittonell, P., Scopel, E., Andrieu, N., Posthumus, H., Mapfumo, P., Corbeels, M., et al. (2012). Agroecology-based aggradation-conservation agriculture (ABACO): Targeting innovations to combat soil degradation and food insecurity in semi-arid Africa. Field Crops Res. 132, 168–174. doi: 10.1016/j.fcr.2011.12.011
Triplett, G. B., and Dick, W. A. (2008). No-Tillage Crop Production: a revolution in agriculture! Agron. J. 100 (Suppl. 3.), S-153–S-165. doi: 10.2134/agronj2007.0005c
Turpin, J. E., Thompson, J. P., Waring, S. A., and MacKenzie, J. (1998). Nitrate and chloride leaching in Vertosols for different tillage and stubble practices in fallow-grain cropping. Soil Res. 36, 31–44. doi: 10.1071/S97037
United Nations, D.o.E.a.S.A., Population Division. (2019). World Population Prospects 2019: Highlights. (New York, NY: UN Department of Economic and Social affairs).
van Bruggen, A. H. C., Semenov, A. M., van Diepeningen, A. D., de Vos, O. J., and Blok, W. J. (2006). Relation between soil health, wave-like fluctuations in microbial populations, and soil-borne plant disease management. Eur. J. Plant Pathol. 115, 105–122. doi: 10.1007/s10658-005-1250-8
VandenBygaart, A. J., Gregorich, E. G., and Angers, D. A. (2003). Influence of agricultural management on soil organic carbon: A compendium and assessment of Canadian studies. Can. J. Soil Sci. 83, 363–380. doi: 10.4141/S03-009
Vanlauwe, B., Wendt, J., Giller, K. E., Corbeels, M., Gerard, B., and Nolte, C. (2014). A fourth principle is required to define Conservation Agriculture in sub-Saharan Africa: the appropriate use of fertilizer to enhance crop productivity. Field Crops Res. 155, 10–13. doi: 10.1016/j.fcr.2013.10.002
Vastola, A., Zdruli, P., D'Amico, M., Pappalardo, G., Viccaro, M., Di Napoli, F., et al. (2017). A comparative multidimensional evaluation of conservation agriculture systems: a case study from a Mediterranean area of Southern Italy. Land Use Policy 68, 326–333. doi: 10.1016/j.landusepol.2017.07.034
Veloso, M. G., Angers, D. A., Tiecher, T., Giacomini, S., Dieckow, J., and Bayer, C. (2018). High carbon storage in a previously degraded subtropical soil under no-tillage with legume cover crops. Agric. Ecosyst. Environ. 268, 15–23. doi: 10.1016/j.agee.2018.08.024
Verhulst, N., Govaerts, B., Verachtert, E., Castellanos-Navarrete, A., Mezzalama, M., Wall, P., et al. (2010). “Conservation agriculture, improving soil quality for sustainable production systems,” in Advances in Soil Science: Food Security and Soil Quality. eds R. Lal and B. A. Stewart (Boca Raton, FL: CRC Press), 137–208. doi: 10.1201/EBK1439800577-7
Verhulst, N., Nelissen, V., Jespers, N., Haven, H., Sayre, K. D., Raes, D., et al. (2011). Soil water content, maize yield and its stability as affected by tillage and crop residue management in rainfed semi-arid highlands. Plant Soil 344, 73–85. doi: 10.1007/s11104-011-0728-8
Vieira, F. C. B., Bayer, C., Zanatta, J., and Ernani, P. R. (2009). Organic matter kept Al toxicity low in a subtropical no-tillage soil under long-term (21-year) legume-based crop systems and N fertilisation. Aust. J. Soil Res. 47, 707–714. doi: 10.1071/SR08273
Virto, I., Barr,é, P., Burlot, A., and Chenu, C. (2012). Carbon input differences as the main factor explaining the variability in soil organic C storage in no-tilled compared to inversion tilled agrosystems. Biogeochem. 108, 17–26. doi: 10.1007/s10533-011-9600-4
Wang, X. B., Cai, D. X., Hoogmoed, W. B., Oenema, O., and Perdok, U. D. (2007). Developments in conservation tillage in rainfed regions of North China. Soil Tillage Res. 93, 239–250. doi: 10.1016/j.still.2006.05.005
Wang, Y., Xu, J., Shen, J. H., Luo, Y. M., Scheu, S., and Ke, X. (2010). Tillage, residue burning and crop rotation alter soil fungal community and water-stable aggregation in arable fields. Soil Tillage Res. 107, 71–79. doi: 10.1016/j.still.2010.02.008
Wang, Z., Chen, Q., Liu, L., Wen, X., and Liao, Y. (2016). Responses of soil fungi to 5-year conservation tillage treatments in the drylands of northern China. Appl. Soil Ecol. 101, 132–140. doi: 10.1016/j.apsoil.2016.02.002
West, T. O., and Post, W. M. (2002). Soil organic carbon sequestration rates by tillage and crop rotation: a global data analysis. Soil Sci. Soc. Am. J. 66, 1930–1946. doi: 10.2136/sssaj2002.1930
Wildermuth, G. B., Thomas, G. A., Radford, B. J., McNamara, R. B., and Kelly, A. (1997a). Crown rot and common root rot in wheat grown under different tillage and stubble treatments in southern Queensland, Australia. Soil Tillage Res. 44, 211–224. doi: 10.1016/S0167-1987(97)00054-8
Wildermuth, G. B., Thompson, J. P., and Robertson, L. N. (1997b). “Biological change: diseases, insects and beneficial organisms,” in Sustainble Crop Production in the Sub-Tropics: An Australian Perspective. eds A. L. Clarke and P. B. Wylie (Brisbane: Queensland Department of Primary Industries), 112–130.
Williams, A., Jordan, N. R., Smith, R. G., Hunter, M. C., Kammerer, M., Kane, D. A., et al. (2018). A regionally-adapted implementation of conservation agriculture delivers rapid improvements to soil properties associated with crop yield stability. Sci. Rep. 8:8467. doi: 10.1038/s41598-018-26896-2
Yang, A. N., Hu, J. L., Lin, X. G., Zhu, A. N., Wang, J. H., Dai, J., et al. (2012). Arbuscular mycorrhizal fungal community structure and diversity in response to 3-year conservation tillage management in a sandy loam soil in North China. J. Soils Sediments 12, 835–843. doi: 10.1007/s11368-012-0518-9
Yang, X., Drury, C. F., and Wander, M. M. (2013). A wide view of no-tillage practices and soil organic carbon sequestration. ACTA. 63, 523–530. doi: 10.1080/09064710.2013.816363
Zarea, M. J. (2010). Conservation Tillage and Sustainable Agriculture in Semi-Arid Dryland Farming. New York, NY: Springer, 195–238. doi: 10.1007/978-90-481-9513-8_7
Zhang, B., He, H., Ding, X., Zhang, X., Zhang, X., Yang, X., et al. (2012). Soil microbial community dynamics over a maize (Zea mays L.) growing season under conventional- and no-tillage practices in a rainfed agroecosystem. Soil Tillage Res. 124:153. doi: 10.1016/j.still.2012.05.011
Zhang, H., Lal, R., Zhao, X., Xue, J., and Chen, F. (2014). Opportunities and challenges of soil carbon sequestration by conservation agriculture in China. Adv. Agron. 124, 1–36. doi: 10.1016/B978-0-12-800138-7.00001-2
Zhang, X., Xin, X., Zhu, A., Yang, W., Zhang, J., Ding, S., et al. (2018). Linking macroaggregation to soil microbial community and organic carbon accumulation under different tillage and residue managements. Soil Tillage Res. 178, 99–107. doi: 10.1016/j.still.2017.12.020
Keywords: conservation agriculture, no-till, soil organic carbon, yield, soil physical, chemical and biological properties
Citation: Page KL, Dang YP and Dalal RC (2020) The Ability of Conservation Agriculture to Conserve Soil Organic Carbon and the Subsequent Impact on Soil Physical, Chemical, and Biological Properties and Yield. Front. Sustain. Food Syst. 4:31. doi: 10.3389/fsufs.2020.00031
Received: 04 December 2019; Accepted: 27 February 2020;
Published: 18 March 2020.
Edited by:
Somasundaram Jayaraman, Indian Institute of Soil Science (ICAR), IndiaReviewed by:
Maria Silveira, University of Florida Health, United StatesJules Bayala, World Agroforestry Centre, Kenya
Copyright © 2020 Page, Dang and Dalal. This is an open-access article distributed under the terms of the Creative Commons Attribution License (CC BY). The use, distribution or reproduction in other forums is permitted, provided the original author(s) and the copyright owner(s) are credited and that the original publication in this journal is cited, in accordance with accepted academic practice. No use, distribution or reproduction is permitted which does not comply with these terms.
*Correspondence: Kathryn Louise Page, a2F0aHJ5bi5wYWdlJiN4MDAwNDA7dXEuZWR1LmF1