- 1Department of Biomedical Sciences and Veterinary Public Health, Swedish University of Agricultural Sciences, Uppsala, Sweden
- 2Division of Food Control, National Food Agency, Uppsala, Sweden
The United Nations sustainable development goals include eradication of hunger. To feed 10 billion persons 2050, we need to get the trade-offs right between sustainability, food security, food safety, and make better use of food already produced. The hierarchy of strategies for reducing food losses and waste are in descending order source reduction, reusing or reprocessing surplus foods, recycle food as feed for animals, recover the energy as biofuels, nutrients as compost, or raw materials for industry, while as last resorts one may consider recovering the energy by incineration or dumping as garbage in landfills. This paper will explore the trade-offs inherent when aiming at triple goals of sustainability, food security, and safety looking at these strategies for reducing food losses and waste and resource footprints. Intensification of food production and circular food systems could be parts of these solutions to future food security. In this regard could our future trade-offs be informed by the experiences from the use of antimicrobials to intensify food production and from the outbreak of bovine spongiform encephalopathy (BSE) in terms of circular food production? There is no trade-off between intensification of food production aided by antimicrobials and the public health risks from antimicrobial resistance due to the zoo-technical use of antimicrobials. A sustainable future requires control of antimicrobial resistance. If one avoids that cycles of nutrients become cycles of pathogens and/or hazards, circular food production systems will a major contribution to the future sustainable food security. Source reduction i.e., limiting food losses and waste appears to the strategy most promising for achieving sustainability. By using artificial intelligence and intelligent packaging major progress is possible, with the added benefit of better control of food fraud. A changed diet—eating more plant-based foods and not eating animal protein produced by edible feedstuffs, and source reduction of the food lost or wasted should enable us to feed at least an additional billion persons. Solutions to sustainability and food security should integrate food safety considerations from the start.
Introduction
Food safety and security are two complementing elements of our sustainable future. This paper will argue that in the long run the aims of food safety and security must be aligned to achieve sustainability, and the trade-offs between these three goals must be managed carefully and based on evidence. Hence, we need novel solutions for our future food security and sustainability without compromising food safety to achieve the United Nations sustainable development goals (SDG) including eradication of hunger and poverty, clean water, sustainable land use, responsible production and consumption, mitigating climate change, and sustainable life on land and water. Several approaches are possible for achieving sustainability and food security, such as limiting food losses and waste, eating more plant based foods or recycling foodstuffs. The trade-offs between food safety and security are fraught with challenges, e.g., when constructing circular food production systems where nutrients are recycled, one could also get a cycle accumulating pathogens.
The tools and strategies used to achieve food security must align with food safety, and public health as well as sustainability. Food chains are complex and not transparent, hence we believe a One Health approach is needed to assess trade-offs and achieving sustainability (Boqvist et al., 2018). In addition, the control of food frauds are an emerging issue requiring attention. Getting the trade-offs right, between the security, safety, and sustainability of food production, will require careful balancing between multiple concerns and challenges. In this balancing exercise could previous failures inform us—for example antimicrobials used for intensifying animal production, and the failed circular food and feed system based on meat and bone meal (MBM) that caused the mad cow disease epidemic (Bovine Spongiform Encephalopathy, BSE)?
This paper explores and discusses some of the trade-offs between sustainability, food security and food safety. The discussion will follow the outline and food recovery hierarchy suggested by the US Environmental Protection Agency (Figure 1), but focus on the most promising options at the top of the hierarchy.
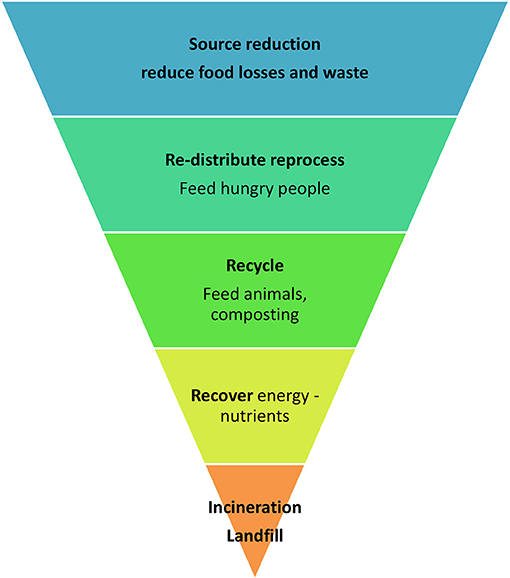
Figure 1. The food recovery hierarchy. From upper most desirable source reduction to the least desirable at the bottom—landfill.
Sustainable Food Security a Political Imperative and Challenge
Sustainable food security will require: (a) availability of food or sufficient food production, (b) access to food and ability to purchase food, (c) sufficiency in terms of nutrition including energy, proteins and micronutrients as well as safety, and (d) the stability and foreseeability of these conditions (Helland and Sörbö, 2014).
Threats to food security include limited supplies of nutritious and safe foods or when the consumers' food purchasing powers is limited (Bazerghi et al., 2016). Food insecurity affects low-income groups most with increased risks of hunger and malnutrition The International Monetary Fund (Arezki and Brückner, 2011) found in low-income countries a correlation between rising food prices and social unrest. Social unrest and wars result in consequent food insecurity and famines. However, the question whether initial food insecurity are a driver for and triggers unrest is complicated (Helland and Sörbö, 2014). It is raising food prices together with failing political institutions, social safety nets, demographic pressures and presence of other grievances, that is associated with social unrest. The Arab spring 2011 is one example of where spiking food prices was one of the drivers for social unrest (Johnstone and Mazo, 2011). Hence, food insecurity is a serious threat to public health, social sustainability, and political stability This challenge is magnified as the world population is foreseen to increase to nearly 10 billion 2050 (United Nations, 2019). This 10 billion people will eat a diet richer in animal protein (Sundström et al., 2014). The main reason is that 3 billion people will shift toward a diet richer in meat, fish, poultry, and dairy foods. Hence, feeding 10 billion people in a sustainable way will probably require disruptive changes of the food supply chains during the next 20 years (AT Kearney, 2019). Moreover, the reduction of food losses and food waste is part of the solution.
Another challenge is the diversion of edible crops (e.g., corn, sugar cane) to biofuel production. These diverted crops to biofuel could have provided food for 400 million people (Helland and Sörbö, 2014). Moreover, these diversions makes the prices of biofuel crops more correlated to oil prices than to supply and demand of food. Another concern is the increasing speculation in food prices, as hedge funds become greater players in the markets for food commodities as wheat, oilseeds, and corn. Increasing the variability of food prices poses a risk to food security for low-income groups.
A rapid urbanization has resulted in that more than half of the global population is living in cities, which is another challenge to food security. As urban consumers are dependent on being able to purchase foods, the food security of low-income urban populations are susceptible to increases in food prices or unpredictable variations of food prices, in particular of staple foods in countries with incomplete socioeconomic safety nets. Moreover, relying on imports may not be the solution as some countries impose export restrictions of foods in times of limited food supplies. Hence, solutions to long-term food security becomes national aims that might explain the drives for developing novel sources of food and animal feedstuffs and resilient food chains. Several reports have addressed these challenges.
The EAT Lancet Commission on healthy diets from sustainable food production (Willett et al., 2019) suggested several dietary changes to enable feeding a world with 10 billion people in a resilient way. In brief, the recommendation was to double the consumption of fruits, vegetables, nuts, and legumes and to halve the consumption of red meat and sugar. A diet rich in plant-based foods and with fewer animal source foods confers both health and environmental benefits. Another example is the AT Kearney (AT Kearney, 2019) report on the disruptions from novel meat alternatives on the global food production systems. The report suggested that meat will be replaced by vegan or vegetarian meat replacements, insect based meat replacements, and/or cultured meat i.e., meat produced in bioreactors. Animal proteins such as milk, eggs and products thereof will be easier to replace, as the structures and biochemistry are simpler. The report suggests that in the future animal proteins and energy i.e., meat, milk and eggs, should be produced using feed sources not suitable for human consumption e.g., pastures, grass, hay, or by-products.
Food safety and food security are by necessity complementing aims for achieving freedom from hunger. One tenet is that unsafe food do not solve food security problems. However, measures to ensure safety and quality of food can sometimes reduce the amount of food available, and thereby amplify food scarcities. For example, consumers interpret best before dates as food being poisonous thereafter dates thus increasing food waste and threatening food security. Massive and not targeted food recalls are other examples of food waste due to safety concerns.
Environmental sustainability is part to a varying degree of all the 17 sustainable development goals (SDG—https://sustainabledevelopment.un.org). One key message though is that environmental sustainability is a part of social and economic sustainability as well as food security and safety. The pursuit of one goal could be to the detriment of other goals. Hence, we need to balance these goals and manage the trade-offs.
Food Losses and Waste vs. Food Security
FAO (FAO, 2011) defined food loss as lost supplies along the food chain between the producer and the market while food waste is discarding safe and nutritious foods. Around one third of the food produced is lost. The foodstuffs lost and wasted represent around 28% of the world agricultural area and 8% of global greenhouse emissions. Moreover, reducing food waste and losses will represent a major business opportunity valued at more than 400 billion USD (Unilever Reducing food loss and waste, 2019) according to Unilever, a leading global food company.
Food loss and waste represent also the lost labor, capital, water, energy, land and other resources that went into producing the food and thereby threatening sustainability. The links between sustainability, food insecurity, and food waste are important. More than one third of the food produced is lost or wasted along the production chain (Lipinski et al., 2013). These lost and wasted foodstuffs amount to 24% of the energy content of the food produced, illustrating the huge potential of improved food security. By eliminating global food waste and loss, one could feed more than one billion additional persons. Less food lost or wasted would lead to more efficient land use and better water resource management with positive impacts on climate change, livelihoods, and sustainability. In conclusion reducing food losses and waste is a well-reasoned political objective. For example, the European Parliament (European Parliament, 2017) adopted a resolution to reduce food waste in the European Union by 30 and 50% in 2025 and 2030, respectively. The two approaches foreseen in its resolution were to make food donations easier and make “best before” and “use by” labels less confusing. The world's fisheries and fish farming can illustrate some of these food loss challenges. Fisheries and fish farming contribute to nearly 20% of human protein consumption (Moffitt and Cajas-Cano, 2014). However, the food losses are considerable, as 20 to 30% of the catch is lost at sea (FAO, 2012), while another 10–15% of the catch is diverted as feed for fish farming resulting in protein and energy losses.
Of the total harvest of cereals and vegetables, nearly half (46%) is used as feed to animals while only around one third is used to feed people. The feed conversion from plant to animal-based food means that most of the edible energy and proteins are lost in the conversion. For example, 10 kilograms of edible grains feed to cattle, produces only 1 kg of edible beef. The possibilities for increasing conventional meat, milk and egg production based on cereals are constrained by lack of additional arable land and water, as well as difficulties in intensifying agriculture and animal production (AT Kearney, 2019). The restrictions posed by land use, biodiversity, fresh water use, greenhouse gas emissions as well as nitrogen and phosphorus cycling determine the sustainability and resilience of food systems. In this regard, it appears that doubling of animal food production will require expansion of cereal and vegetable production beyond sustainable levels (AT Kearney, 2019).
Causes of Food Losses and Waste
Food losses might be the result of pre-harvest failures. For example, in a US study of pre-harvest losses of vegetables, more than half of the vegetable crops were not harvested (Johnson et al., 2018) indicating a huge potential for increased food production. The edible and wholesome vegetables (cucumbers, zucchini, bell pepper, cabbage, eggplant, yellow squash) available for recovery amounted to 8,840 kg per hectare.
Food losses occurs also at harvest and post-harvest due to problems in processing, handling, packing, transportation, and retail. Some of the underlying causes of food loss include the inadequacies of infrastructure, cold chains, skewed incentives or lack of legal frameworks—waiting for customs clearance. Tomatoes crushed during transport because of improper packaging is one example of food loss. Food waste, on the other hand, refers to the discarding of food that is safe and nutritious for human consumption for example binning tomato cans if the labels on the can is incomplete or the labels was peeled off. The reasons for food loss and waste include:
• Failure and consequent spoilage in the agricultural process, harvest, storage, processing, packaging, and marketing;
• Abnormal reduction of quality such as bruising of packages, fruits or vegetables;
• Quality flaws—e.g., fresh produce that deviates from what is considered optimal in terms of shape, size, and color such as curved cucumbers, wrong sized apples being discarded during sorting operations;
• Foods that are close to, at or beyond the “best-before” dates are discarded by retailers and consumers;
• Large quantities of wholesome edible food are often left over and discarded from households and catering establishments; and
• Producer or retailer recall of foods.
These recalled foods might present a risk for a minority of the population for example by containing allergens e.g., nuts. The recalled foods will be safe for the majority of the population not allergic to nuts. Unspecific food recalls increase food waste. In the United States, it was found that out of 382 recalls 42% was due to undeclared allergens (milk, nuts, eggs, soy, and wheat), 18% due to presence of food borne pathogens (e.g., Salmonella spp., Listeria spp. and E. coli), and 7% due to presence of foreign material (plastic and metal pieces; Maberry, 2019).
Assessment of Different Options for Reducing Food Loss and Waste
The United States Environmental Protection Agency (EPA) (2019) has described a hierarchy of options for reducing food losses and waste (Figure 1), that aligns well with the goals of sustainability. Mourad (2016) compared the approaches of France and United States to food waste prevention. Her main finding was the three competing hierarchies of solutions to surplus food production based on environmental, economic, and social justifications. Implementing strong food waste prevention strategies such as changing the acceptance criteria for fresh produce (ugly cucumbers) instead of weak prevention strategies (developing new best practices of food business operators) could achieve more in terms of long-term sustainability. While the aims of reducing food losses and waste are highly desirable, food safety should be included in this pursuit. The trade-offs, judgmental decisions taken, should be evidence based and transparently justified.
Source Reduction—Reduce Waste and Losses
Food Security an Insurance Question?
We need novel ways of looking at the food security and sustainability. One consequence of such a shift in perspective is that the food policy should focus on achieving zero hunger and good nutrition, and not on food production. Changing the perspective could open new ways of thinking about food and sustainability. Should food security be thought of as an insurance question—how much to pay in premium to ensure that we do not suffer famine, get right nutrition, and avoid food borne illnesses in the future? The term nega-watt triggered a lot new thinking in the energy field (Lovins, 1990). The most resource friendly watt was the one not wasted or lost, hence investments in saving energy was just as efficient as investments in additional production capacity. Could one use the same thinking e.g., nega-foods for food security and sustainability? An investment in reducing the amount of foods lost or wasted (source reduction) will be just as valuable as an investment in additional food production capacity. Hence, source reduction by reducing food losses and waste from farm to fork will be necessary part of the future solution to feed 10 billion people sustainably.
Source Reduction—Using Novel IT and AI Solutions
To achieve sustainability we must eliminate food losses and waste. This proposition aligns well with the goals for reducing resource and environmental footprints. On the other hand, the consumers wish to purchase their wanted food whenever is convenient. Food businesses have difficulties in getting their inventory policies right—when to reorder foods and the appropriate stock levels to be maintained. In other words, food businesses, and retailers have a dynamic resource allocation problem—the concurrent challenges of avoiding food waste and stock-outs. Food waste and stock-outs occur mainly due to inaccurate forecasting of sales with the consequent incorrect ordering of products (Arunraj and Ahrens, 2015). The factors correlated with the demand for foodstuffs in retail stores include price, weather, season, events or festivals, promotions, or discounts in the store or competing stores, products characteristics (shelf life), and number of customer visits. Moreover, the time series of sales in food retail have high volatility and skewness varying over time, thus violating several assumptions of the standard statistical models.
Could greater use of predictive models, machine learning, neural networks and expert systems (artificial intelligence or AI) enable the food business operators, to predict the demand for food? Could fine-tuning the supply operations and demand forecasts, minimize stock-outs, and food waste? By using seasonal autoregressive, forecasting models with integrated moving averages with external variables model one could forecast reasonably the daily sales of perishable foods (Arunraj and Ahrens, 2015). The forecast accuracies would improve if two or more models with different analytical approaches are used. For example by combining moving averages with back propagation neural networks, one could predict better the demand for fresh food in convenience stores (Chen-Yuan et al., 2010). Furthermore, food supplies could be better predicted by using big data approaches such as data based on remote sensing—metrology, images, genetic information, as well as laboratory results and historical production data (Gounden et al., 2015). In this regard, Bayesian networks would be a more transparent method enabling better understanding and insights of the food supply chain compared with machine learning approaches. One major concern is that food safety and quality considerations should be included into these promising big data models, to obtain the full benefits.
There are complementary and competing visions for safe food in the big data era (Nychas et al., 2016; Ropodi et al., 2016). Multidisciplinary approaches should give the food industry better tools for ensuring food quality and safety (Ropodi et al., 2016). Possible methods for real time analyses of these huge data sets include complicated and not very transparent algorithms such as machine learning and computational intelligence (Ropodi et al., 2016). The lack of transparency means that interpretation of the results and giving evidence-based recommendations are difficult. Moreover, the naïve application of forecasting of food supply and demand, as well as monitoring the food chain from farm to fork while ignoring food safety concerns, could be a recipe for disaster. Hence, the big data strategies should include food safety considerations. The benefits could be reduction of food waste, ensuring food safety through a more efficient control of the processes, and reassuring trust between consumers and the food industry. Moreover, a move from invasive or destructive testing toward non-invasive automated monitoring based on sensors would be beneficial. These sensors are easily implemented on-site, and will monitor production in real-time. The massive amounts of high-throughput, analytical, and imaging metadata collected with these instruments will provide a holistic view of the spoilage and decaying processes of the various food products across diverse storage conditions (temperature and packaging) thereby better predicting food supply. The online availability of this growing knowledge could provide continuous benefits for the food industry. Big data analyses promise a way forward to achieve the better sustainability by reducing food loss and waste while also ensuring food safety and quality.
Source Reduction by Intelligent Labeling
Shelf Lives “Best Before Date” and “Use by Date”
The shelf life of foodstuffs is labeled either as “best before dates” or “use by dates.” The “best before date” is a food quality management tool. The food business operator guarantees that foods consumed before the best before date, is of good quality provided the storage instructions are followed e.g., cold, dark storage. After the best before date the food is safe but the quality may decline. The consumer might deem the food as unsuitable and unsafe, and consequently throw the food in the waste bin. This is due to consumers confusing the “best before dates” with “use by dates” intepreting both as “poisonous thereafter dates.”
The “use by date” is a food safety management tool, indicating the food is safe to eat until the' use by date', provided the food is stored in compliance to the food business operators' instructions. The food business operator guarantees the food safety until the “use by date.” Thereafter consumers should discard the food. The foodstuff must comply with the end-product microbiological safety criteria on the last “use by date.” Hence, these criteria will determine the shelf life of a foodstuff. In the European Union (EU), the microbiological safety criteria are legally binding [Commission Regulation (EC) No 2073/2005]. For example, the number of listeria should be <100 c.f.u., per gram during the whole shelf life, for ready-to-eat foods able to support the growth of L. monocytogenes. However complicating the discussion, there are great differences in susceptibility between healthy and immuno-compromised groups (Rocourt et al., 2003) as listeriosis is primarily a disease of those with impaired or altered immune function (e.g., pregnant women and their fetuses, the elderly, cancer patients, or patients being immunosuppressed). Persons having organ transplants, being pregnant, and persons undergoing dialysis are nearly 3,000, 1,000, and 500 times more likely to catch listeriosis than the normal person, respectively (FAO/WHO, 2001). Further increasing the disease burden is the fact that one out of seven listeriosis cases is pregnant women (Desai and Smith, 2017). In the future, could one differentiate the shelf lives for consumers by use of intelligent packaging and sensors? Could those susceptible to listeriosis download an app on their smartphone and scan the label on the food that could indicate whether eating the food presents a risk. This app could potentially reduce the amount of discarded foods and food waste.
Intelligent Labeling and Sensors
Intelligent packaging and use of sensors have great potential for reducing food waste and thereby improving food security (Newsome et al., 2014; Poyatos-Racinero et al., 2018). There are four families of sensors—for freshness, food package integrity usually gas sensors, time-temperature indicators (TTI), and identification tags such as radio-frequency identification (RFID; Poyatos-Racinero et al., 2018).
The TTIs' are helpful for detecting temperature abuse along the distribution chain, and could permit dynamic dating of a foodstuff's shelf life. One could foresee replacing the “use by dates” by e.g., a TTI indicator turning red if the foodstuff is not suitable for human consumption or yellow if the food quality declines. The freshness indicators monitor the freshness by reacting to metabolites generated by the foodstuff. Such indicators include organic acids, pH, biogenic amines, ammonia, and/or carbon dioxide. The freshness indicators need calibration for each foodstuff. Those indicators suitable for freshness of leafy greens may not be suitable for fish products. The gas sensors can check the integrity of the food packaging, leakages of protective atmospheres, or changes in gas metabolites (Ghani et al., 2016). To make sensor a commercially viable proposition, they need to be low cost, reusable, reversible, and long lasting. Probably more difficult could be to educate consumers on the meaning of the sensor outputs.
The RFID tags are helpful for tracing and tracking a food product as it provides real time information on the food product identity and the food chain. Moreover, the RFID tags could provide data for analyzing the causes of food waste and losses. An additional benefit could be in controlling food frauds. Food frauds and fraudulent inputs in the food production has emerged as an issue in recent years (Manning and Soon, 2016). In foods subject to fraud, RFID tags coupled with block-chains could verify the origin and fate of the food. Block-chains are tamper and fraud resistant and could thus facilitate safe trade and reduce paperwork (Ahmed and Broek, 2017). The trace-back when investigating outbreaks will be faster and more precise when using block-chains. Thus, one could target food recalls precisely. An important element of food security is the ability of governments to mitigate famines by importing foods from other areas. Trust in the safety of foods is vital and use of block-chains could facilitate trust and speed up the procedures.
Could one develop an alternative to large unspecific food recalls due to allergens, by sending allergic consumers or those with specific food preferences (i.e., vegetarians or avoiding pork) information through mobile phone apps enabling avoiding certain foods by scanning the food labels? This would allowing consumers to check the foods in real time on the spot. The food businesses could then update these smartphone apps instead of recalling the foods. This should also be simpler and enable more rapid spread of critical information. Those not allergic or having particular food preferences could eat the food and consequently less food is wasted.
Source Reduction—Reduce Food Losses by Intensification of Food Production
The intensification of food production must align with the requirements for long-term sustainability (Rockström et al., 2017). The long-term sustainable agriculture has to operate within its environmental boundaries to remain sustainable. The key considerations of this approach include the ecological dimensions, resource footprints and resilience, the social dimension of food security, and improving livelihoods of the global food production systems. Agriculture and aquaculture food production should change from being a driver of global climate and environmental change to be the basis for global sustainability. In particular, food security resilience or the ability to deal with shocks and stress in food production and distribution without increasing the risks of hunger, malnutrition or food borne diseases, is critical. The double challenges of shrinking arable land and increasing global population will necessitate a smart intensification of food production (AT Kearney, 2019). For example, more than half of the edible vegetable production is lost in the fields (Johnson et al., 2018). The losses are caused by failures of the harvest process, failures to comply with supermarket specifications (wrong shape of cucumbers), or uneven ripening of the crops. This indicates a straightforward way of increasing the amount of supplied foods and improved food security with limited downside risks including food safety. Moreover, the sustainability would improve as the doubling of the amounts of plant foods available to consumers would require the same resource footprints i.e., inputs in terms of fertilizer, labor, irrigation water, and chemicals. Another approach could be shifting to novel animal proteins derived from insects fed food waste and by-products that could replace traditional meat products. A third approach to improve resilience is going down the food chain by eating the cereals today intended as animal feedstuffs. The sustainability and food security would improve if animal production were based on feeds not available for human consumption i.e., pastures and grasslands. This would mean that pastoral production of beef, mutton and milk could be a very important contribution to the supply of high quality proteins that ensures future food security. The intensification of animal production derived from better animal nutrition, health and disease prevention could be a very important contribution to sustainable development in low and medium income countries (McDermott et al., 2010).
Antimicrobials in Food Production—A Case of Unsustainable Intensification
Antimicrobials has been used to intensify animal production by improving feed conversion and growth of the animals i.e., zoo-technical use. This approach was linked to large industrial animal production systems in which antimicrobials was an input to the production (Aarestrup and Wegner, 1999). When Sweden joined the EU in 1995, one of the controversies was the Swedish ban on zoo-technical use of antimicrobials. The Swedish Commission on Antimicrobial Feed Additives (Commission on Antimicrobial Feed Additives. Antimicrobial feed additives. SOU, 1997) concluded that whilst the use of antimicrobials could intensify animal production and thereby decrease footprints; these gains could not match the negative impacts of the consequent antimicrobial resistances (AMR). Animal production and welfare will be more sustainably maintained by implementing good husbandry, biosecurity, and preventive medicine than by using antimicrobials. More than 20 years later, the EU has banned the use of antimicrobials for growth promotion and prophylaxis when the Regulation (EU) 2019/4 came into force. In plant production (Vidaver, 2002) the antimicrobials used are streptomycin and tetracycline, mainly as spray treatments of orchards. It appears that resistance to streptomycin has become widespread among bacterial plant pathogens, illustrating the generic problem of using antimicrobials as productivity enhancers (Vidaver, 2002). Hence, we need alternative options to antimicrobials in plant production including biocontrol agents, disinfectants, and resistant plants.
Food as Vehicle for Spreading Resistant Bacteria
Hence, today AMR is a silent pandemic (Jasovský et al., 2016) for which the use of antimicrobials in food production is a major driver globally. Detection of antimicrobial substances in foodstuffs is uncommon while the findings of bacteria with genes for AMR are frequent events. For example, in Sweden, 1395 samples were taken in 2016 from animal foods (meat, dairy, egg, fish, and honey) and only one sample was positive for antimicrobial substances [National Food Agency (NFA), 2018]. Moreover, the import controls did not detect antimicrobial residues when sampling 3,693 consignments of foodstuffs originating outside EU. In contrast, between 10 and 55% of the broiler carcasses were contaminated with extended spectrum beta-laktamase producing enterobacteria (ESBL) during the monitoring of broilers at slaughter between 2010 and 2018 (Swedres-Svarm, 2018).
The Biological Hazards panels of the European Food Safety Authority (EFSA) published two opinions in 2008 and 2013 [EFSA BIOHAZ Panel (EFSA Panel on Biological Hazards), 2008, 2013] on AMR as a food borne hazard. The opinion from 2008 was a self-task opinion—a tool for EFSA scientific panels to warn about food safety risks. Could food could as a vehicle for human exposure to AMR bacteria and could one rank the identified risks and control options were the terms of reference. The second opinion was on carbapenems i.e., broad-spectrum β-lactam antimicrobials used for the treatment of serious infections in humans and the presence of resistance in animals. Both opinions noted that foodborne pathogens and commensals display diverse ranges of resistance to antimicrobial agents of human and veterinary importance. Spread of resistance amongst bacteria in foods will influence public health. For example, the bacteria resistant to fluoroquinolones as well as 3rd and 4th generation cephalosporin being found in a variety of foods and in animals in primary production. The major source of human exposure to fluoroquinolone resistance via food was poultry, whereas for cephalosporin resistance the sources were poultry, pork and beef.
Future Perspectives on Control of AMR in Food
To be sustainable the intensification of food production systems (Rockström et al., 2017) must incorporate the control of AMR. The EFSA opinion [EFSA BIOHAZ Panel (EFSA Panel on Biological Hazards), 2008] suggested monitoring and restrictions of antimicrobial use in food animals, and a focus on pre-harvest control. This aligns well with the US Food and Drug Administration's (FDA) action plan on antimicrobial drug steward ship (FDA/CVM, 2018). FDA concluded that medically important antimicrobial drugs should not be used for zootechnical purposes in food animals or as over the counter (OTC) drugs. Moreover, the Food and Agriculture Organization's (FAO) action plan on FAO (2016) suggested improving (a) global awareness of risk factors for AMR emergence and spread, (b) improve monitoring of antimicrobial use and AMR, (c) strengthen the governance related to AMR in foodstuffs and agriculture, and (d) promote good practices and prudent use. WHO (2015) noted that a One Health approach and political will are prerequisites for tackling the challenge of AMR. In the future, one should reserve the remaining efficient antimicrobials for treating human infections. These insights have major implications for veterinary medicine and animal science including:
• Antimicrobial drugs should not substitute for substandard rearing facilities and animal welfare,
• Great need for improved preventive medicine,
• Better vaccines to protect against infections,
• Food production systems with better biosecurity and animal health and welfare,
• Animal breeding program aimed at robustness and resilience; and
• The development of separate veterinary and human antimicrobial substances based on different biological mechanisms.
Redistribute OR Reprocess Food
One can redistributed food through food banks and food donation programs. These are both examples of urban mining (Schneider, 2013). These measures may be important to make the food available to disadvantaged socioeconomic groups. Typically, the donated foods are closer to the end of their shelf lives, which in the case of “use-by dates” could indicate an increased risk for food borne disease or “best before dates” of possible decreased quality.
Food Donations—Liability Concerns
Could liability concerns impair the willingness to donate or redistribute foods? For example, Austrian supermarkets discard around 10% of the bread (Lebersorger and Schneider, 2014). Of these discarded breads, only 7 % is donated to food banks i.e., <1% of total bread production. One reason for this is the concerns of food business operators about their liability when donating perishable foods that might be associated with foodborne disease. To ease such liability concerns, USA and Italy have implemented Good Samaritan Laws. These laws protect donors from liability when donating to non-profit organization as well as from civil and criminal liability if a product, donated in good faith, later causes harm to one of the needy beneficiaries (Braun, 2010). To encourage food donations and use of food banks, one will need legislation on how to donate food without incurring liabilities.
Food Donations—Resilience and Novel IT Solutions
Food donation programs and food banks will help to mitigate food insecurities (EFSA AHAW Panel, 2006). If these programs are well-designed and run they will prevent starvation and thereby enhance welfare for socioeconomic disadvantaged groups. This will improve the sustainability, resilience, and stability of a society. The typical donations to food banks are non-perishable foods such as canned, frozen, and dry foodstuffs. Food banks could also be in the form of rescue programs such as community shelters, soup kitchens, and food pantries where perishable and non-perishable foods are distributed. Food banks are important for easing acute food deprivation and risk of hunger (Bazerghi et al., 2016). On the other hand, food banks may have limited ability to improve the nutritional status of the recipients due to the limited supply of nutrient-dense and perishable foods such as dairy, vegetables, and fruits. Nevertheless, the contributions to food security will be considerable if the food banks address their clients' nutritional needs and provisions of perishable foods are available.
Moreover, food banks have usually limited economic resources for transportation and storage of foods. The biggest challenge for food banks are predicting their food supplies, which impair their ability to transport, store, and distribute donated foods cost efficiently (Brock and Davis, 2015). Moreover, to ensure a wholesome diet for their clients the food banks often need to purchase complementary foods. Novel IT and artificial intelligence solutions such as multilayer perceptron neural networks (MLP-NN) appear best suited to predict the dynamics of food supplies. This is another example how novel solutions like artificial intelligence could help limiting the food waste.
Food Donations and Food Safety
Compared with conventional food chains, food donation chains are often less structured and with incomplete cold chains (De Boeck et al., 2017). Another concern is the frequent lack of food hygiene training of those working with food donations. The donated perishable foods are mostly ready to eat or ready to be reheated meals. When analyzing 72 samples from perishable foodstuffs taken from social groceries in Belgium, increased numbers of listeria monocytogenes (log 3.5 CFU/g) and enterobacter (6.7 CFU/g) were found in ready-to-eat cooked meat (Wooldridge et al., 2006). It highlights the need for sufficient cooling capacity if food banks intend to accept perishable foods. Another concern is that people eating donated foods are often more susceptible to catch food borne diseases due to other co-morbidities or health conditions, indicating the need for careful trade-offs between food safety and mitigating food insecurity. In conclusion, for food donation programs to work food safety is a prerequisite.
Reprocessing Foodstuffs for Human Consumption
Reprocessing often means that foodstuffs reaching their “best before dates” or “use by dates” are reheated or frozen. For example, salmon filets and beef pieces are minced into salmon or beef patties, and thereafter fried for another shelf life. Another option is freezing foods just before their best before or use by dates. A third option is to use food leftovers as raw materials for next meals or reheat the leftovers. A study of Swedish supermarkets (Lagerberg Fogelberg et al., 2011) found that for the supermarket having its own chef making warm lunches or dinner portions was a paying proposition. The amounts of food waste were reduced as foodstuffs nearing the “best before date” or with any visible signs linked to lesser consumer acceptance, were processed into warm lunches, pates or pies, and ready to eat sandwiches. In addition, customers got better service, and the supermarkets got another business opportunity. That foods intended for the bin, were processed into meals to be sold appeared to improve the profits. The supermarkets reported moreover, that employing a chef enhanced the hygiene standards amongst the other employees.
Reheating already prepared dishes improve food supply but includes a food safety risk. The classic example is the growth of toxin producing bacteria linked to the slow cooling of and thereafter reheating of pea soup (Nyberg and Lindqvist, 2017). For example in Sweden around 20% of the reported cases of food borne diseases were associated with toxin producing bacteria—Staphylococcus aureus, Clostridium perfringens, and Bacillus cereus (Lindqvist, 2019), while the European Food Safety Authority (EFSA) reported that a similar proportion of the food borne outbreaks in EU during 2018 were linked to bacterial toxins−18.5% (EFSA (European Food Safety Authority), 2019). Staphyloccus aureus food poisoning is associated with cross contamination and thereafter temperature abuse of foodstuffs kept between 5° and 60°C (Hennekinne et al., 2012). The latter two species are spore forming and the spores survive cooking or similar heat treatments. For example, C. perfringens foodborne intoxications are linked to slowly cooling and reheating dishes with meat such as pea soup (Andersson et al., 1995; Nyberg and Lindqvist, 2017). In a similar way, insufficiently heated (typically not hot enough water baths) rice dishes are linked to B. cereus food poisoning (Andersson et al., 1995). In the dairy industry B. cereus is linked to quality and spoilage problems in the dairy industry contributing to food losses since it survives pasteurization (Andersson et al., 1995). While reprocessing food is beneficial from food security and sustainability perspectives, one must avoid negative trade-offs with food safety. Hence, training of the food business operators involved in food reprocessing is a strong recommendation.
Recycle
Reprocess Wasted and Lost Food to Feed Animals
Can we manage the trade-offs when developing circular food production systems based on reprocessing foodstuffs to animal feed? One conclusion is that the management of these trade-offs should be evidence based and balance carefully the costs, risks and benefits to food safety, security, and sustainability. The worry is that the recycling of nutrients could result in the recycling of biological and chemical hazards. For example, EFSA AHAW Panel (2006) concluded on the use of dairy byproducts such as raw milk, white water, or unpasteurized dairy products as feed for animals could present risks for transmission of biological hazards. The EFSA opinion identified 24 hazards as animal pathogens including four zoonoses. Animal pathogens such as Foot and Mouth Disease virus (FMDV) present a threat to animal health and thereby to food security, while zoonoses like Q-fever, brucellosis and salmonellosis, in addition present a threat to food safety and public health. Hence, in reprocessing foods there should be treatment steps that eliminates the relevant pathogens. For example, feeding wasted or lost foodstuffs as e.g., scraps to pigs is an old way of taking care of the nutrients to maintain and improve the food supply and sustainability (Salemdeeb et al., 2017). Historically, pigs were living storages of food for people when food spoilage were the big problem. On the other hand, feeding pigs scraps could spread diseases such as Classical Swine Fever, African Swine Fever, Swine Vesicular Disease, and, Foot and Mouth Disease (Wooldridge et al., 2006).
For lost or wasted plant foodstuffs, recycling, and reprocessing food as animal feed should be easier as fewer of the concerns about transmitting animal disease or zoonotic pathogens apply. The BSE epidemic caused the tightened controls of feed producers reprocessing plant food in the European Union and feeding scraps to food animals was prohibited. All feed producers processing food waste of plant origin to animal feed must be officially supervised. Thus, feeding the neighbor's pig some pieces of bread is a regulated activity. One side effect of these prohibitions has been the limits on circular and thereby more sustainable animal production systems. Consequently, of nearly 100 million tons of food waste only 3 million tons are recycled as animal feed (Salemdeeb et al., 2017). There are several benefits from substituting feedstuffs based on cereals or soybeans with plant based food scraps in terms of resource footprints, sustainability, and amounts of heavy metals entering the food chains. Moreover, the costs to farmers of feeding their animals recycled foods are more predictable than of feedstuffs where prices determined by a volatile market. In conclusion, getting this trade-off right could unlock substantial benefits and profits to consumers and farmers, respectively.
Recycle Through Insects
A novel way would be to feed insects e.g., house crickets (Acheta domesticus) with wasted or lost foodstuffs with a view of eating the insects or feeding them to animals. However, following food safety and quality concerns were identified (Fernandez-Cassi et al., 2019):
• High total counts of aerobic bacteria meaning that spoilage will be rapid unless there is a heat treatment step soon after harvest;
• The presence of spore-forming bacteria after heat treatment with the implication that insect based foods with slow cooling after heat treatment could have high numbers of these bacteria;
• The accumulation of cadmium and other heavy metals necessitating controls of the feed given to insects; and
• The possible increase of allergenic reactions due to exposure to insects and insect derived products, this could increase risk of product recalls.
Perhaps more important were the data gaps leading to high levels of uncertainty when considering trade-offs. These data gaps include lack of knowledge on the farming conditions of the insects, the breeding pyramids, and the impact of thermal processing of the products prior to consumption. For example, what could be the impact of mycotoxin-producing fungi, a likely proposition since the crickets are foreseen reared in hot and humid conditions? Could heat-treating the insects imply risks from chemical hazards such as heterocyclic aromatic amines or acrylamide? Hence when introducing novel foods that are very promising from sustainability and food security perspectives, we need sufficient evidence for managing the food safety risks.
Circular Food Production—Aquaculture and Aquaponics
Circular food systems are very efficient in terms of environmental and resource footprints. Circular systems means that most of the nutrients are recycled, but unless there sufficient hurdles such as species barriers this cycle of nutrients can become a cycle of pathogens or chemical hazards. This means that the biosecurity and possible hurdles of circular food production systems should receive special attention. One example of a circular food production system is the combination of aquaculture and aquaponics (Monsees et al., 2017). Aquaculture has environmental benefits compared with animal production on land such as smaller water footprints and better feed-conversion approaching 1 kg of feed to 1 kg of fish meat. A combined aquaponics and aquaculture based food production system could further minimize the environmental footprints since the waste produced by the fish are fertilizing plants. However, circular food productions systems have their own challenges. The fish metabolism produces ammonia that in the bio-filters are converted to nitrates. This conversion of ammonia to nitrates requires the pH to be kept above 7 that subjects the fish suboptimal rearing conditions with consequent health and welfare risks. Thereafter by adding acids to the water after the bio-filter, the pH is lowered to 5–6 to optimize the uptake of nutrients by the plants when used for irrigation. Hence, for fish welfare and health reasons decoupling the aquaponics and aquaculture systems could be beneficial. Moreover, the yields from separately optimized aquaponics and aquaculture production systems appear to be higher than for coupled systems.
Circular Food Systems—Benefits and Risks
Circular food systems had the potential to reduce food waste and losses and thereby improve the sustainability of the food systems and food security (Jurgilevich et al., 2016). Today the sustainability of our food security is challenged since between 30 and 50% of the food is lost or wasted in different stages of the food system, with consequent higher consumption of animal foodstuffs and larger environmental footprints. Circular food production systems will imply smaller environmental and resource footprints and the recycling of nutrients, by-products and food waste, resulting in less food waste, and losses. An additional benefit is the improved transparency possible from shorter and local food chains. Combining local and seasonal elements in supply chains could improve the balance of food supply and demand and reduce needs for storage and transportation. On the other hand, there are risks when making the transition from a linear extractive food production system to a circular and recycling one. Slaughterhouses produce a lot of food waste for example offal, specified risk material (SRM), condemned parts of carcasses or condemned whole carcasses. Approval or condemnation of carcasses is necessary food safety procedure, but too frequent condemnation contributes to additional food waste (Arzoomand et al., 2019). One of the reasons for this excess condemnation is the lack of training of the meat inspector and slaughterhouse staff. Previously, the waste and by-products from slaughterhouses were rendered into meat and bone meal (MBM) to recover the high quality nutrients for animal feed. Could such a cycle of nutrients also could become a cycle of pathogens?
BSE Outbreak and the Risks From Circular Food Systems
One example of a failed circular food production system was the outbreak of mad cow disease (Bovine Spongiform Encephalopathy—BSE; Ducrot et al., 2008). BSE is one of the diseases called Transmissible Spongiform Encephalopathies (TSEs) affecting the brain and nervous system of humans and animals, all caused by abnormal forms of proteins (prions). BSE is a zoonotic disease in cattle causing variant Creutzfeldt-Jakob's disease (vCJD) in people (Bruce et al., 1997). In the European Union, between 1996 and 2013, 226 cases of VCJD were detected of which 177 was detected in in UK (European Center for Disease Control, 2019). In cattle from 1987 to 2014, 190,182 cases of BSE were detected of which 184,637 cases in UK (European Commission, 2015). One BSE infected cow could infect 15–20 other cows through the rendering, MBM and ruminant feed cycle (De Koeijer et al., 2004), thus illustrating the disease transmission potential of circular food production systems when they fail. Could lessons learned from the BSE and vCJD outbreak inform the design of future circular food production systems? It is worth remembering that the rendering of offal from slaughter to protein and energy concentrates intended for feeding to animals was good husbandry practice until 1988 (ARC (Agricultural Research Council), 1980). These practices included feeding calves and dairy cows with ruminant meat and bone meal (MBM) in milk replacers and in concentrate feedstuffs. When the rendering temperature, time and pressure were reduced and the ether extraction of fats were discontinued, the circulation of the prions became possible. The reasons for these changes in rendering process were: increased demands for high nutrient feedstuffs due to increasing yields in dairy production, cost pressures—feeding the cheapest protein feed ingredients, the aims of reducing animal waste and by-products and reducing the environmental footprints, and food security and self-sufficiency (Ducrot et al., 2008).
According to the UK BSE Inquiry (The inquiry into BSE Variant CJD of the United Kingdom, 2002) the first cases were noted in December 1984, while the official recognition of the new cattle disease as BSE was 2 years later. In 1988 the results epidemiological and pathological studies (Wilesmith et al., 1988) established that ruminant MBM was a risk factor for BSE. Consequently, feeding cattle with ruminant MBM was prohibited in the summer of 1988. In addition, BSE became notifiable, and a stamping out policy for cattle showing clinical symptoms were introduced. In 1989 Specified Bovine Offal (SBO) e.g., the brains and spinal cords, eyes but later extended to distal ileum and spleen) were prohibited from human consumption, and pet manufactures ceased voluntary to use of SBO. In retrospect, it appeared that the number of BSE infected cows decreased for each annual cohort borne 1988 and thereafter indicating the efficiency of the control measures (Ducrot et al., 2008). The BSE outbreak became a crisis in 1996 when BSE was designated as a zoonosis (Bruce et al., 1997). The BSE epidemic in UK peaked in 1992/93 4 years after control measures were implemented as a consequence of the incubation period of BSE being 4–6 years (Doherr, 2007).
Due to this delayed peak, the rest of European Union (EU) member states considered from 1988 to 1993 that BSE was a UK problem. The consequence was the EU member states delayed implementing control measures in their food chains. During these years, UK exported ruminant MBM to the rest of Europe as protein ingredients for poultry and pig feed production (Ducrot et al., 2008) as well as calves and heifers. These exports were the drivers for the next wave of BSE that emerged in several EU countries (Doherr, 2007). BSE cases were diagnosed in Ireland 1989, in Switzerland 1990 and in France 1991. However, it was first in 1994 that EU imposed a mammalian MBM ban to cattle all over EU. EU extended this ban in 2001 to a complete ban of MBM fed to food animals in EU. The BSE geographical risk assessments predicted that countries having imported cattle and MBM from UK was at higher risk in particular if their rendering and feeding practices enabled the BSE to circulate (European Commission, 2003; Salman et al., 2012). The public health concerns were elevated as modeling studies predicted up to 1 million persons in UK were incubating vCJD (Cousens et al., 1997; Ghani et al., 1998). These modeling results were revisited later (Smith et al., 2010) by studying the prevalence of abnormal prion protein in appendixes, indicating a prevalence of 1 carrier out of 2,000 persons, or around 30.000 carriers in total in UK, in contrast to the 177 vCJD cases reported to date (Gill et al., 2013).
Take Home Lessons BSE Epidemic
In retrospect, while BSE epidemic was a huge outbreak in cattle and tragedy for society, the actual public health impact was smaller than for many other food borne diseases. Another lesson was that dealing with the fear of a huge disease outbreak and resultant loss of trust, might be just as difficult as dealing with an actual outbreak. A particular challenge was that when BSE emerged as a cattle disease, the causes for this emergence emerged around 5 years earlier. Furthermore, the control measures implemented would give results after lag period of 5 years. Explaining this lag between control actions and seeing results to decision makers and the public was difficult. Moreover, the ability BSE to spread amongst cattle and thereafter to other species indicating the zoonotic risks was first grasped when thinking in One Health perspectives. Another insight was that the good functioning of the internal market for food in the EU is contingent on the consumers' trust in the management of food safety risks, and that the loss of public confidence can be difficult to repair. Hence, the public confidence in the food safety is critical for sustainable and resilient food production systems. When designing circular food production systems one priority must be avoiding cycles of biological and chemical hazards. One surprise to remember was the higher persistence of prions (BSE pathogen) in the food and feed chains compared with the pathogens thought to be present in 1980s.
Recover as Biofuel and Nutrients
Here the strategy is to recover the energy and use as fertilizers the nutrients from food losses and wastes as well as byproducts from animals and vegetable food production. These recover strategies are less favorable in terms of food security and sustainability than the previously discussed strategies. On the other hand, as complements they could be valuable. Even here there are trade-offs between food safety, sustainability and food security.
Recover Biogas and Nutrients From Manure
One complementary approach is the recycling of manure from animal production (Leibler et al., 2017) for industrial uses to recover the energy and recover the nutrients as fertilizers. Here it is a possible win-win situation for sustainability and food security, but with risks for food safety. The amounts of manure from animal production such as poultry and pig farms that are often concentrated on small areas. Hence, there might be risks of releases of pathogens such as the avian flu virus. The avian flu virus might survive up to 600 days in manure (Graiver et al., 2009) creating a potential hot spot for transmission or later re-emergence of the disease if the manure is left untreated. The food waste residues after biogas production could be valuable as fertilizers and lowering the resource footprint of food production. Composting, anaerobic digestion, and ammonia treatment are three methods for recovering nutrients and energy from bio-wastes and manure (Albihn and Vinnerås, 2007), with different advantages and disadvantages. For example, anaerobic digestion might produce biogas and degrade organic pollutants, while on the downside there is the need for expensive high tech equipment. The selection of treatment methods should be on case-by-case basis, but the key parameters for controlling pathogens in producing fertilizers were the time temperature profiles and ammonia content.
Recover Energy Through Biofuel Production
Biofuels include biogas, bioethanol, biodiesel and biobuthanol (Tabatabaei et al., 2015). The choice of substrates for biofuel production should be as wide as possible such as agricultural and food industry losses and waste, household waste, and solids from municipal wastewater. For example, EFSA reviewed the biosecurity of making biodiesel from animal by-products such as fish oil, animal fats derived from offal [EFSA BIOHAZ Panel (EFSA Panel on Biological Hazards), 2015]. Even fats from sewage cleaning facilities, cooking and frying oils were possible substrates. The current biosecurity requirement for the biodiesel process is to reduce the infectivity of the BSE agent by at least one million times (log 6) in order to use all risk categories of offal as substrate. The sustainability gains originates from smaller climate footprints as biodiesel could substitute for kerosene to airplanes and for diesel to trucks and agricultural machines. Another caveat for sustainability is that the substrates for biofuel production should not be possible to use as human food or animal feeds. In this regard, the emerging linkages between the oil and food prices (Al-Maadid et al., 2017) are a concern. Consequently, the prices of global staple foods such as cereals and sugar would go up if oil price increased. This could be a major concern to social sustainability and food security as food prices become more volatile when edible foodstuffs are diverted into biofuel production to substitute for oil.
Incineration and Landfills
Incineration and landfills are the least desirable strategies for dealing with food waste and losses. However, incineration has some advantages from a sustainability perspective. In developing countries with energy poverty and where food waste is dumped in unsanitary landfills, incineration to produce electricity and heat is an alternative that could improve sustainability (Unaegbu and Baker, 2019). The food lost or wasted is already available as fuel and can replace oil used to produce energy needed locally. The benefits are from converting food waste into an energy and electricity resource available locally. This would improve the resilience and make cold chains a viable proposition in development settings. Another example from Sweden is that if farmers or feed mills have spoiled cereals due to mold, one could incinerate the grains to recover the energy (SOU, 2007). Benefits include avoiding for sure those moldy grains enter the food or feed chains and green energy the foods already lost due to molds. Hence, incineration could cut oil consumption and carbon footprints. Hence, one could think of incineration as starting point in development settings and a safety valve for more advanced sustainable food systems.
Landfills are unpopular, as most people do not want them nearby. Landfills appear to be the least sustainable system for taking care of food waste () having the largest environmental and resource footprints. Moreover, the biosecurity and food safety risks are important as landfills where food waste is dumped, tend be populated by vermin. For example in Finland, an outbreak of trichinellosis could be linked invasion of rats from an improperly closed dump nearby (Oivanen et al., 2000).
Insights and Conclusions
Feeding 10 billion people in 2050 sustainably will require changes of our food chains. Changing of our food demand to more plant based diets could help as half of the world's cereal production ends up as animal feed while only around one third is for human nutrition (Willett et al., 2019). The future diets might align with recommendations of the EAT-Lancet report (AT Kearney, 2019), but with local adaptions. For example, the beef, mutton, and milk produced from pastoral farming systems will remain. The ecosystem benefits of open landscapes should give further incentives for pastoral farming practices. Moreover, seafood produced through farming and in circular systems could supply high quality proteins wherever this production is feasible (Oivanen et al., 2000). Another major source of food supply could emerge if the 30% of food produced that is now lost or wasted, could become available for human consumption. Source reduction and reprocessing of foods appears to be the best options to eliminate food waste or loss. One example of sustainable intensification and source reduction could be intensifying the harvest of vegetable crops to double the output with the same footprint. This will require consumers and food businesses to adapt their quality requirements and specifications (Johnson et al., 2018). If the global food systems could change in this direction, the global food security will improve and be more resilient. Using modern IT technology offers the best promise of more efficient source reduction, reprocessing, and recycling of food.
It is however, vital to get the trade-offs right between food safety, food security and economic, social and environmental sustainability. These trade-offs should be evidence and risk based. Good intentions will not compensate for failures as shown by the failed use of antimicrobials to achieve food security through intensifying food production. A veterinary medicine and food value chain not requiring antimicrobials is therefore a necessary aim for research and innovation. The transition of animal production from intensive cereal based farming to more extensive pastoral farming will probably imply changes to veterinary medicine. For example, nutritional supplements and control of parasites may become bigger concerns than diseases related to rapid growth. In conclusion, the trade-offs and subsequent decisions regarding food safety, food security, and sustainability are not trivial and should be evidence based.
Author Contributions
IV designed, searched and collated references, assessed evidence in particular on source reduction, and wrote the paper. NA contributed on the issue of circular food system in reference to the slaughter and recycling of offal and the BSE epidemic, assessing evidence, and the writing of the paper. SB contributed on the design of the paper, the issues of circular food production systems, antimicrobial resistance and sustainable intensification, and the writing of the paper.
Conflict of Interest
The authors declare that the research was conducted in the absence of any commercial or financial relationships that could be construed as a potential conflict of interest.
The reviewer RK-T and handling editor declared their shared affiliation at the time of the review.
References
Aarestrup, F. M., and Wegner, H. C. (1999). The effects of antibiotic usage in food animals on the development of antimicrobial resistance of importance for humans in Campylobacter and Escherichia coli. Microbes Infect. 1, 639–644. doi: 10.1016/s1286-4579(99)80064-1
Ahmed, S., and Broek, N. T. (2017). Food supply: block-chain could boost food security. Nature 550:43. doi: 10.1038/550043e
Albihn, A., and Vinnerås, B. (2007). Biosecurity and arable use of manure and biowaste - treatment alternatives. Livestock Sci. 112, 232–239. doi: 10.1016/j.livsci.2007.09.015
Al-Maadid, A., Caporale, G. M., Spagnolo, F., and Spagnolo, N. (2017). Spillovers between food and energy prices and structural breaks. Int. Econ. 150, 1–18. doi: 10.1016/j.inteco.2016.06.005
Andersson, A., Ronner, U., and Granum, P. E. (1995). What problems does the food industry have with the spore-forming pathogens Bacillus cereus and Clostridium perfringens? Int. J. Food Microbiol. 28, 145–155.
ARC (Agricultural Research Council) (1980). The Nutrient Requirements of Ruminant Livestock. Slough: Commonwealth Agricultural Bureaux.
Arezki, R., and Brückner, M. (2011). Food Prices and Political Instability. IMF working paper. WP/11/62. IMF (Washington, DC). Available online at: https://www.imf.org/en/Publications/WP/Issues/2016/12/31/Food-Prices-and-Political-Instability-24716 (accessed July 2, 2019).
Arunraj, N. S., and Ahrens, D. (2015). A hybrid seasonal autoregressive integrated moving average and quantile regression for daily food sales forecasting. Int. J. Prod. Econ. 170, 321–335. doi: 10.1016/j.ijpe.2015.09.039
Arzoomand, N., Vågsholm, I., Niskanen, R., Johansson, A., and Comin, A. (2019). Flexible distribution of tasks in meat inspection – a pilot study. Food Control 102, 166–172. doi: 10.1016/j.foodcont.2019.03.010
AT Kearney. (2019). How Will Cultured Meat and Meat Alternatives Disrupt the Agricultural and Food Industry?Dusseldorf; AT Kearney Studie zur Zukunft des Fleischmarkts bis 2040. Available online at: https://www.atkearney.com/retail/article/?/a/how-will-cultured-meat-and-meat-alternatives-disrupt-the-agricultural-and-food-industry (accessed July 5, 2019)
Bazerghi, C., McKay, F. H., and Dunn, M. (2016). The role of food banks in addressing food insecurity: a systematic review. J. Community Health 41, 732–40. doi: 10.1007/s10900-015-0147-5
Boqvist, S., Söderqvist, K., and Vågsholm, I. (2018). Food safety challenges and one health within Europe. Acta Vet. Scand. 60:1. doi: 10.1186/s13028-017-0355-3
Braun, J. (2010). Food insecurity, hunger and malnutrition: necessary policy and technology changes. N. Biotechnol. 27, 449–452. doi: 10.1016/j.nbt.2010.08.006
Brock, L. G., and Davis, L. B. (2015). Estimating available supermarket commodities for food bank collection in the absence of information. Expert Syst. Appl. 42, 3450–3461. doi: 10.1016/j.eswa.2014.11.068
Bruce, M. E., Will, R. G., Ironside, J., and McConnell, I. (1997). Transmissions to mice indicate that 'new variant' CJD is caused by the BSE agent. Nature 389, 498–501. doi: 10.1038/39057
Chen-Yuan, C., Wan-I, L., Hui-Ming, K., Cheng-Wu, C., and Kung-Hsing, C. (2010). The study of a forecasting sales model for fresh food. Expert Syst. Appl. 37, 7696–7702. doi: 10.1016/j.eswa.2010.04.072
Commission on Antimicrobial Feed Additives. Antimicrobial feed additives. SOU (1997). Swedish Government, Ministry of Enterprise and Innovation, Stockholm, Sweden. Available online at: https://www.government.se/legal-documents/1997/01/sou-1997132/ (accessed July 4, 2019).
Cousens, S., Vynnycky, E., Zeidler, M., Will, R., and Smith, P. (1997). Predicting the CJD epidemic in humans. Nature 385, 197–198.
De Boeck, E., Jacxsens, L., Goubert, H., and Uyttendaele, M. (2017). Ensuring food safety in food donations: case study of the Belgian donation/acceptation chain. Food Res. Int. 100, 137–149. doi: 10.1016/j.foodres.2017.08.046
De Koeijer, A., Heesterbeek, H., Schreuder, B., Oberthür, R., Wilesmith, J., van Roermund, H., et al. (2004). Quantifying BSE control by calculating the basic reproduction ratio R0 for the infection among cattle. J. Math. Biol. 48, 1–22. doi: 10.1007/s00285-003-0206-x
Desai, R. W., and Smith, M. A. (2017). Pregnancy-related listeriosis. Birth Defects Res. 109, 324–335. doi: 10.1002/bdr2.1012
Doherr, M. G. (2007). Brief review on the epidemiology of transmissible spongiform encephalopathies (TSE). Vaccine 25, 5619–5624. doi: 10.1016/j.vaccine.2006.10.059
Ducrot, C., Arnold, M., Koeijer, A., Heim, D., and Calavas, D. (2008). Review on the epidemiology and dynamics of BSE epidemics. Vet. Res. 39:15. doi: 10.1051/vetres:2007053
EFSA (European Food Safety Authority) (2019). Scientific report on the European Union One Health 2018 Zoonoses Report. EFSA J. 17:5926. doi: 10.2903/j.efsa.2019.5926
EFSA AHAW Panel, 2006###EFSA AHAW Panel. (2006). Opinion of the scientific panel on animal health and welfare on a request from the commission related to “the animal health risks of feeding animals with ready-to-use dairy products without further treatment”. EFSA J. 347, 1–21. doi: 10.2903/j.efsa.2006.347
EFSA BIOHAZ Panel (EFSA Panel on Biological Hazards) (2008). Scientific opinion of the panel on biological hazards on a request from the European Food Safety Authority on foodborne antimicrobial resistance as a biological hazard. EFSA J. 765, 1–87. doi: 10.2903/j.efsa.2008.765
EFSA BIOHAZ Panel (EFSA Panel on Biological Hazards) (2015). Scientific opinion on a continuous multiple-step catalytic hydro-treatment for the processing of rendered animal fat (Category 1). EFSA J. 13:4307. doi: 10.2903/j.efsa.2015.4307
EFSA BIOHAZ Panel (EFSA Panel on Biological Hazards) (2013). Scientific opinion on Carbapenem resistance in food animal ecosystems. EFSA J. 11:3501. doi: 10.2903/j.efsa.2013.3501
Environmental Protection Agency (EPA) (2019). Sustainable Management Food. Available online at: https://www.epa.gov/sustainable-management-food (accessed March 1, 2019).
European Center for Disease Control (2019). Facts About Creutzfelt Jacob's Disease. Available online at: https://ecdc.europa.eu/en/vcjd/facts (accessed April 10, 2019).
European Commission (2015). Transmissible Spongiform Encephalopathies (TSEs) in the EU in 2014. Available online at: https://ec.europa.eu/food/sites/food/files/safety/docs/biosafety_food-borne-disease_tse_ms-annual-report_2014.pdf (accessed April 10, 2019).
European Commission, Scientific Steering Committee. (2003). The Scientific Steering Committee (SSC): A Short History of Six Busy Years. Available online at: https://ec.europa.eu/food/sites/food/files/safety/docs/biosafety_food-borne-disease_tse_ssc-history.pdf (accessed July 10, 2019).
European Parliament (2017). Cutting Food Waste. Press Release 20170509IPR73930. Available online at: http://www.europarl.europa.eu/news/en/press-room/20170509IPR73930/cutting-food-waste (accessed March 1, 2019).
FAO (2016). The FAO Action Plan on Antimicrobial Resistance 2016-2020. Rome: Food and Agriculture Organization of the United Nations. Available online at: http://www.fao.org/3/a-i5996e.pdf (accessed Oct 14, 2019).
FAO/WHO (2001). Joint FAO/WHO Expert Consultation on Risk Assessment of Microbiological Hazards in Foods. Risk Characterization of Salmonella in Eggs and Broilers and Listeria monocytogenes in Ready-to-Eat Foods. FAO Food and Nutrition Paper No. 72. Rome: Food and Agriculture Organization of the United Nations.
FDA/CVM (2018). Supporting Antimicrobial Stewardship in Veterinary Settings – Goals for Fiscal years 2019-2023. Rockville, MD: Center for Veterinary Medicine Food and Drug Administration. Available online at: https://www.fda.gov/animal-veterinary/judicious-use-antimicrobials/fdas-cvm-key-initiatives-antimicrobial-stewardship (accessed October 11, 2019).
Fernandez-Cassi, X., Supeanu, A., Vaga, M., Jansson, A., Boqvist, S., and Vagsholm, I. (2019). The house cricket (Acheta domesticus) as a novel food: a risk profile. J. Insects Food Feed 5, 137–157. doi: 10.3920/JIFF2018.0021
Ghani, A., Ferguson, N., Donnelly, C., Hagenaars, T., and Anderson, R. (1998). Estimation of the number of people incubating variant CJD. Lancet 352, 1353–1354.
Ghani, M., Cozzolino, C. A., Castelli, G., and Farris, S. (2016). An overview of the intelligent packaging technologies in the food sector. Trends Food Sci. Technol. 51, 1–11. doi: 10.1016/j.tifs.2016.02.008
Gill, O. N., Spencer, Y., Richard-Loendt, A., Kelly, C., Dabaghian, R., Boyes, L., et al. (2013). Prevalent abnormal prion protein in human appendixes after bovine spongiform encephalopathy epizootic: large scale survey. BMJ 347:f5675. doi: 10.1136/bmj.f5675
Gounden, C., Irvine, J. M., and Wood, R. J. (2015). Promoting food security through improved analytics. Procedia Eng. 107, 335–336. doi: 10.1016/j.proeng.2015.06.089
Graiver, D. A., Topliff, C. L., Kelling, C. L., and Bartelt-Hunt, S. L. (2009). Survival of the avian influenza virus (H6N2) after land disposal. Environ. Sci. Technol. 43. 4063–4067. doi: 10.1021/es900370x
Helland, J., and Sörbö, G. M. (2014). Food Security and Social Conflict. CMI Report 2014:1. Bergen: Christian Michelssen Institute.
Hennekinne, J. A., De Buyser, M. L., and Dragacci, S. (2012). Staphylococcus aureus and its food poisoning toxins: characterization and outbreak investigation. FEMS Microbiol. Rev. 36, 815–836. doi: 10.1111/j.1574-6976.2011.00311.x
Jasovský, D., Littmann, J., Zorzet, A., and Cars, O. (2016). Antimicrobial resistance-a threat to the world's sustainable development. Ups. J. Med. Sci. 121, 159–164. doi: 10.1080/03009734.2016.1195900
Johnson, L. K., Dunning, R. K., Bloom, J. D., Gunter, C. C., Boyette, M. D., and Creamer, N. G. (2018). Estimating on-farm food loss at the field level: a methodology and applied case study on a North Carolina farm. Resour. Conserv. Recy. 37, 243–250. doi: 10.1016/j.resconrec.2018.05.017
Johnstone, S., and Mazo, J. (2011). Global Warming and the Arab Spring. J. Surviv. 53, 11–17. doi: 10.1080/00396338.2011.571006
Jurgilevich, A., Birge, T., Kentala-Lehtonen, J., Korhonen-Kurki, K., Pietikäinen, J., Saikku, L., et al. (2016). Transition towards circular economy in the food system. Sustainability 8:69. doi: 10.3390/su8010069
Lagerberg Fogelberg, C., Vågsholm, I., and Birgersson, A. (2011). Från Förlust Till Vinst - Såhär Minskar vi Matsvinnet i Butik. (In Swedish, English summary). Available online at: https://www.slu.se/globalassets/ew/org/inst/energy-technology/oldies/nj-energi-och-teknik/lagerbergfogelberg-vagsholm-birgersson-2011-fran-forlust-till-vinst—sahar-minskar-vi-matsvinnet-i-butik.pdf (accessed July 5, 2019).
Lebersorger, S., and Schneider, F. (2014). Food loss rates at the food retail, influencing factors and reasons as a basis for waste prevention measures. Waste Manag. 34, 1911–1919. doi: 10.1016/j.wasman.2014.06.013
Leibler, J. H., Dalton, K., Pekosz, A., Gray, G. C., and Silbergeld, E. K. (2017). Epizootics in industrial livestock production: preventable gaps in biosecurity and biocontainment. Zoonoses Public Health 64, 137–145. doi: 10.1111/zph.12292
Lindqvist, R. (2019). L 2019 nr 02: Sjukdomsbördan av Inhemska Fall av Matförgiftning (Disease Burden for Domestically Aquired Cases of Food Borne Diseases). Livsmedelsverkets Rapportserie. Uppsala. In Swedish with English summary Available online at: https://www.livsmedelsverket.se/bestall-ladda-ner-material/sok-publikationer/artiklar/2019/l-2019-nr-02-sjukdomsbordan-av-inhemska-fall-av-matforgiftning-smittskyddsunderlag-del-1-livsmedelsverkets-rapportserie (accessed July 4, 2019).
Lipinski, B., Hanson, C., Lomax, J., Kitinoja, L., Waite, R., and Searchinger, T. (2013). Reducing Food Loss and Waste. Working Paper, Installment 2 of Creating a Sustainable Food Future. Washington, DC: World Resources Institute. Available online at: http://www.worldresourcesreport.org (accessed March 1, 2019).
Maberry, T. (2019). A Look Back at 2018 Food Recalls. Food Safety Magazine, E-Newsletter, February 19. Available online at: https://www.foodsafetymagazine.com/enewsletter/a-look-back-at-2018-food-recalls-outbreaks/ (accessed July 4, 2019).
Manning, L., and Soon, J. M. (2016). Food safety, food fraud, and food defense: a fast evolving literature. J. Food Sci. 81, 823–834. doi: 10.1111/1750-3841.13256
McDermott, J. J., Staal, S. J., Freeman, H. A., and Van de Steeg, J. A. (2010). Sustaining intensification of smallholder livestock systems in the tropics. Livestock Sci. 130, 95–109. doi: 10.1016/j.livsci.2010.02.014
Moffitt, C. M., and Cajas-Cano, L. (2014). Blue Growth: The 2014 FAO state of world fisheries and Aquaculture. J. Fish. 39, 552–553. doi: 10.1080/03632415.2014.966265
Monsees, H., Kloas, W., and Wuertz, S. (2017). Decoupled systems on trial: eliminating bottlenecks to improve aquaponic processes. PLoS ONE 12:e0183056. doi: 10.1371/journal.pone.0183056
Mourad, M. (2016). Recycling, recovering and preventing “food waste”: competing solutions for food systems sustainability in the United States and France. J. Clean. Prod. 126, 461–477. doi: 10.1016/j.jclepro.2016.03.084
National Food Agency (NFA) (2018). Kontroll av Restsubstanser i Levande Djur och Animaliska Livsmedel (Results From the Swedish Monitoring of Residues in Animals and Animal Products in 2016). Uppsala: Livsmedelsverket. (in Swedish with English summary) Available online at: https://www.livsmedelsverket.se/globalassets/publikationsdatabas/rapporter/2018/restsubstanser-kontrollen-2016-livsmedelsverket-nr-6-2018.pdf (accessed July 9, 2019).
Newsome, R., Balestrini, C. G., Baum, M. B., Corby, J., Fisher, W., Goodburn, K., et al. (2014). Applications and perceptions of date labeling of food. Compr. Rev. Food Sci. Food Technol. 13, 744–769. doi: 10.1111/1541-4337.12086
Nyberg, K., and Lindqvist, R. (2017). Tillväxt av Bakterier Under Avsvalning, Förvaring och Upptining (Bacterial Growth During Cooling, Storage and Thawing). Livsmedelsverkets Rapportserie nr 2 del 2/2017. Uppsala: National Food Administration.
Nychas, G. J. E., Panagou, E., and Mohareb, F. R. (2016). Novel approaches for food safety management and communication. Curr. Opin. Food Sci. 12, 13–20. doi: 10.1016/j.cofs.2016.06.005
Oivanen, L., Mikkonen, T., and Sukura, A. (2000). An outbreak of trichinellosis in farmed wild boar in Finland. APMIS 108, 814–818. doi: 10.1111/j.1600-0463.2000.tb00003.x
Poyatos-Racinero, E., Ros-Lis, J. V., Vivancos, J. L., and Martinez-Manes, R. (2018). Recent advances on intelligent packaging as tools to reduce food waste. J. Clean. Prod. 172, 3398–3409. doi: 10.1016/j.jclepro.2017.11.075
Rockström, J., Williams, J., Daily, G., Noble, A., Matthews, N., Gordon, L., et al. (2017). Sustainable intensification of agriculture for human prosperity and global sustainability. Ambio 46, 4–17. doi: 10.1007/s13280-016-0793-6
Rocourt, J., BenEmbarek, P., Toyofuku, H., and Schlundt, J. (2003). Quantitative risk assessment of Listeria monocytogenes in ready-to-eat foods: the FAO/WHO approach. FEMS Immunol. Med. Microbiol. 35, 263–267. doi: 10.1016/S0928-8244(02)00468-6
Ropodi, A. I., Panagou, E. Z., and Nychas, G. J. E. (2016). Data mining derived from food analyses using non-invasive/non-destructive analytical techniques; determination of food authenticity, quality & safety in tandem with computer science disciplines. Trends Food Sci. Technol. 50, 11–25. doi: 10.1016/j.tifs.2016.01.011
Salemdeeb, R., zu Ermgassen, E. K., Kim, M. H., Balmford, A., and Al-Tabbaa, A. (2017). Environmental and health impacts of using food waste as animal feed: a comparative analysis of food waste management options. J. Clean. Prod. 140, 871–880. doi: 10.1016/j.jclepro.2016.05.049
Salman, M., Silano, V., Heim, D., and Kreysa, J. (2012). Geographical BSE risk assessment and its impact on disease detection and dissemination. Prev.Vet. Med. 105, 255–264. doi: 10.1016/j.prevetmed.2012.01.006
Schneider, F. (2013). The evolution of food donation with respect to waste prevention. Waste Manag. 33, 755–763. doi: 10.1016/j.wasman.2012.10.025
Smith, M. D., Roheim, C. A., Crowder, L. B., Halpern, B. S., Turnipseed, M., Anderson, J. L., et al. (2010). Economics. Sustainability global seafood. Science 327, 784–786. doi: 10.1126/science.1185345
SOU (2007). Betankandet om Jordbruket som Bioenergiproducent (Report on Agriculture as Supplier of Bioenergy). (In Swedish). Available online at: http://www.regeringen.se/sb/d/8963/a/81974 (accessed Jan 16, 2020).
Sundström, J. F., Albihn, A., Boqvist, S., Ljungvall, K., Marstorp, H., Martiin, C., et al. (2014). Future threats to agricultural food production posed by environmental degradation, climate change, and animal and plant diseases – a risk analysis in three economic and climate settings. Food Secur. 6, 201–215. doi: 10.1007/s12571-014-0331-y
Swedres-Svarm (2018). Consumption of Antibiotics and Occurrence of Antibiotic Resistance in Sweden Solna. Uppsala: Public Health Agency of Sweden and National Veterinary Institute.
Tabatabaei, M., Karimi, K., Kumar, R., and Horváth, I. S. (2015). Renewable energy and alternative fuel technologies. Biomed Res. Int. 2015:245935. doi: 10.1155/2015/245935
The inquiry into BSE Variant CJD of the United Kingdom (2002). Available online at: http://webarchive.nationalarchives.gov.uk/20090505194948/http://www.bseinquiry.gov.uk/ (accessed July 10, 2019).
Unaegbu, EU, and Baker, K. (2019). “Assessing the potential for energy from waste plants to tackle energy poverty and earn carbon credits for Nigeria”. Int. J. Energy Policy Manag. 4, 8–16.
Unilever Reducing food loss waste (2019). Available online at: https://www.unilever.com/sustainable-living/reducing-environmental-impact/waste-and-packaging/reducing-food-loss-and-waste/ (accessed March 1, 2019).
United Nations (2019). Revision of World Population Prospects. Available online at: https://population.un.org/wpp/ (accessed June 26, 2019).
Vidaver, A. K. (2002). Uses of antimicrobials in plant agriculture. Clin. Infect. Dis. 34(Suppl. 3), S107–10. doi: 10.1086/340247
WHO (2015). Global Action Plan on Antimicrobial Resistance. Geneva: World Health Organization. Available online at: https://apps.who.int/iris/bitstream/handle/10665/193736/9789241509763_eng.pdf?sequence=1 (accessed Oct 14, 2019).
Wilesmith, J. W., Wells, G. A., Cranwell, M. P., and Ryan, J. B. (1988). Bovine spongiform encephalopathy: epidemiological studies. Vet. Rec. 123, 638–644.
Willett, W., Rockström, J., Loken, B., Springmann, M., Lang, T., Vermeulen, S., et al. (2019). Food in the Anthropocene: the EAT-Lancet Commission on healthy diets from sustainable food systems. Lancet 393, 447–492. doi: 10.1016/S0140-6736(18)31788-4
Keywords: source reduction, circular food systems, food loss and waste, antimicrobial resistance, BSE
Citation: Vågsholm I, Arzoomand NS and Boqvist S (2020) Food Security, Safety, and Sustainability—Getting the Trade-Offs Right. Front. Sustain. Food Syst. 4:16. doi: 10.3389/fsufs.2020.00016
Received: 25 July 2019; Accepted: 04 February 2020;
Published: 21 February 2020.
Edited by:
Janne Mikael Lundén, University of Helsinki, FinlandReviewed by:
Séamus Fanning, University College Dublin, IrelandRiikka Keto-Timonen, University of Helsinki, Finland
Copyright © 2020 Vågsholm, Arzoomand and Boqvist. This is an open-access article distributed under the terms of the Creative Commons Attribution License (CC BY). The use, distribution or reproduction in other forums is permitted, provided the original author(s) and the copyright owner(s) are credited and that the original publication in this journal is cited, in accordance with accepted academic practice. No use, distribution or reproduction is permitted which does not comply with these terms.
*Correspondence: Ivar Vågsholm, ivar.vagsholm@slu.se