- 1Grassland Science, Georg-August-University of Göttingen, Göttingen, Germany
- 2Departamento de Nutrición Animal Dr. Fernando Pérez-Gil Romo, Instituto Nacional de Ciencias Médicas y Nutrición Salvador Zubirán (INCMNSZ), Mexico City, Mexico
- 3Departamento de Ciencias Pecuarias, Facultad de Estudios Superiores de Cuautitlán, National Autonomous University of Mexico, Mexico City, Mexico
- 4Grassland and Fodder Sciences, University of Rostock, Rostock, Germany
- 5Centre for Biodiversity and Sustainable Land Use, Göttingen, Germany
Increasing sward phytodiversity has been suggested as having potential to increase primary production of grasslands, but whether any such gains are converted into secondary production, through improved performance of grazing livestock, remains uncertain. Animal production by cattle and sheep can also be enhanced by mixed-grazing. To our knowledge, this effect has never been studied in relation to differences in sward phytodiversity. Therefore, a rotational grazing experiment was conducted over 5 years (2007–2011) on permanent grassland in Germany using sheep and cattle in mono- (single-species) or mixed-grazing of swards differing in plant species richness. Herbicides against dicotyledonous plant species were used to create different sward types: species-poor, grass-dominated swards in contrast to untreated “diverse” control swards. We found no differences in herbage production between the sward types. However, compared to the grass-dominated sward, the diverse sward showed greater concentrations of crude protein and lower contents of acid detergent fiber in the herbage dry-matter. Lamb live weight gains were slightly greater on the diverse-swards (P < 0.05), but calf performance was unaffected by sward type. Mixed-grazing increased daily average live weight gains of suckler cows (g cow−1 d−1) (P < 0.05) as well as area-related daily live weight gains (kg ha−1 d−1) and total live weight gains (kg ha−1) during the complete grazing season (P < 0.001). This indicates advantages of combining livestock species, attributed to complementary pasture use. We suggest that mixed-grazing of cattle and sheep on phytodiverse swards is an effective and sustainable means to enhance ecological and agronomic traits such as livestock production and plant species conservation. Lamb production especially showed benefits under mixed-grazing, with a 17% increase in live weight gain. Compared to the grass-dominated sward, diverse swards resulted in an average 12% increase of live weight gains (across grazing systems and livestock species).
Introduction
Grasslands in temperate regions of the world are an important resource, and often the main supply, of feed for ruminant livestock (O'Mara, 2012). Applications of mineral and organic fertilizers have been widely used on grasslands over many decades, on grazed pastures as well as mown meadow swards, to optimize primary (herbage mass), and secondary (livestock performance) productivity, but their use has contributed to a decline of biodiversity (Hopkins and Wilkins, 2006). Therefore, attention needs to be focused on other grassland management options to enable the integration of production with biodiversity objectives in agricultural systems (Isselstein et al., 2005). There is an on-going debate on how plant diversity can affect ecosystem services, including grassland productivity, under varying conditions (e.g., Hector et al., 2010; Wrage et al., 2011; Seither et al., 2012; Petersen et al., 2013; Isbell et al., 2015; Carlsson et al., 2017). Many experiments on sown grasslands have indicated positive relationships with species richness (e.g., Hector et al., 1999; Bullock et al., 2001; Tilman et al., 2001) or evenness (Kirwan et al., 2007) on herbage dry matter (DM) yield as measured under cutting. This relationship might apply not only to cut swards as found in experimental plots, but also to grazed grasslands (Sanderson et al., 2005; Isbell and Wilsey, 2011). For instance, Grace et al. (2018b) found greater biomass production of botanically diverse sown swards compared with grass-dominated swards under sheep grazing, despite lower nitrogen input. In contrast, other authors did not find species richness to be a predictor of primary production, either on mown (Kahmen et al., 2005) or on grazed sites (Rusch and Oesterheld, 1997; Tracy and Faulkner, 2006). Phytodiversity has also been shown to affect forage quality, although reports are inconsistent (Bullock et al., 2001; Tracy and Faulkner, 2006). For example, White et al. (2004) found a negative effect, while other studies (Hofmann and Isselstein, 2005; Isselstein, 2005; Seip et al., 2012; Petersen et al., 2013) have suggested that the herbage of more diverse-swards contains higher concentrations of crude protein, particularly in late season.
It would be of considerable interest to establish whether suggested benefits, as reported in terms of increased primary production associated with higher levels of phytodiversity, might also be reflected in improved performance by grazing livestock. When Totty et al. (2013) investigated dairy milk production from sown mixtures differing in species diversity, they found that the five-species mixture resulted in increased total milk yield but not in terms of yield of milk solids, when compared with a species-poor ryegrass-white clover sward. In a similar experiment, Soder et al. (2006) found no increase in milk solids production, and Tracy and Faulkner (2006) reported that cow-calf performance was also not influenced by the complexity of sown grassland mixtures. In an experiment with grazing sheep, complex mixtures resulted in better live weight gain than from simple swards, and the improved performance was attributed to higher rates of herbage utilization (Grace et al., 2018a,b).
The choice of the grazing system in terms of mixed-grazing vs. single-species grazing (subsequently referred to as mono-grazing) can also affect the agronomic output over a range of grazing species (Forteau et al., 2019; Sharpe, 2019). Mixed-grazing of cattle and sheep has been found to enhance animal performance (Nolan and Conolly, 1989; Abaye et al., 1994; Marley et al., 2006; Fraser et al., 2007, 2013, 2014). Herbivore species differ in how they defoliate, the sward, and in excreta deposition and trampling (Rook et al., 2004; Wrage et al., 2011), and can thereby affect pasture productivity (Boswell, 1977; White and Knight, 2007) and quality (Abaye et al., 1994). Sheep graze selectively for preferred plants, but their excreta are more evenly distributed than cattle excreta (Monteath et al., 1977; Abaye et al., 1997; Seither et al., 2012). Cattle and sheep both tend to avoid grazing in close proximity to dung patches of their own species; this can lead to a considerable amount of residual herbage on grazed swards, with direct implications for overall grazing efficiency (Nolan and Conolly, 1989; Murphy et al., 1995). As a result, complementary mixed-grazing of cattle and sheep has been advocated for obtaining a more even utilization of pastures, as sheep graze closer to cattle dung patches (Forbes and Hodgson, 1985), and as cattle are less selective at the single- plant -scale they in turn will and also graze the more mature forage that is avoided by sheep (Dumont et al., 1995). The positive effects of complementary grazing might be most distinct in diverse swards offering the greatest opportunities to utilize dietary differences among livestock species and, thus, lower the competition for vegetation resources (Forbes and Hodgson, 1985; Fraser et al., 2007). Mixed-grazing has generally been found to have a positive effect on utilization by sheep, particularly on lamb performance (Abaye et al., 1994; Marley et al., 2006; Wright et al., 2006; Fraser et al., 2007, 2013). Cattle reacted to mono- as opposed to mixed-grazing as well (Nolan and Conolly, 1989). However, the general amplitude seems lower for cattle than for sheep in such a mixed-grazing system (Abaye et al., 1994; Kitessa and Nicol, 2001; Wright et al., 2006).
Few studies have investigated how sward diversity and botanical composition influence secondary production in grassland (see review by Soder et al., 2007). Such studies that have been reported have usually been limited in scope, for instance in comparing either the effects of simple mixtures on mixed-grazing or of diverse mixtures on single-species of grazing livestock. To our knowledge, the relationship between the phytodiversity of permanent grassland and mixed-grazing has not been studied over long-term periods. Grassland offers great potential for biodiversity conservation, subject to it being managed in a suitable way (Pavlu et al., 2006). There are, however, ongoing threats of grassland abandonment in many marginal areas in regions of Europe (Pakeman et al., 2018), and mixed-grazing of diverse swards offers potential opportunities for a more sustainable maintenance of ecosystem services of grasslands, including both biodiversity conservation and utilized agricultural production. Therefore, in this study, we designed a two-factorial experiment with two different sward types (“diverse-” and “grass-dominated”) and three grazing treatments (cattle and sheep in mono- and mixed-grazing) analyzed over five successive years.
The underlying hypotheses of this study were:
i) Primary productivity on diverse swards is higher than on grass-dominated swards
ii) Diverse swards offer forage of higher quality than grass-dominated swards
iii) Phytodiversity results in greater secondary performance than on grass-dominated swards
iv) Mixed-grazing is beneficial for livestock performance.
Materials and Methods
Experimental Site and Weather Conditions
The present study was based on a grazing experiment conducted between spring 2007 and autumn 2011 at the experimental farm Relliehausen situated in the Solling Uplands of Lower Saxony, Germany (51°46′47″ N, 9°42′11″ E; altitude 184–209 m above sea-level). The site has a mean air temperature of 8.2°C and an annual precipitation sum of 879 mm (1961–1990). The experimental farm is located in a marginal to hilly upland area in Central Germany. The grassland in this region is managed over a gradient of intensity, which includes sheep and beef or dairy production (Klimek et al., 2008). The conditions of the experiment may be considered as representative of such situations under a temperate climate. The dominant soil type is a pelosol with a silty/clay-loam texture (for details see Jerrentrup et al., 2015). The plant association of the vegetation was classified as moderately species-rich Lolio-Cynosuretum. Proportions of the three most abundant species, Dactylis glomerata, Lolium perenne, and Taraxacum sect. Ruderale were 22, 15, and 13%, respectively. In the years before the experiment, the site had been manured regularly prior to cutting, and managed as cut and carry pasture, with varying proportions of cattle and sheep grazing for more than 16 years. Soil nutrient status of phosphorus and potassium were low and heterogeneously distributed over the sward (Seither et al., 2012; Jerrentrup et al., 2015). No fertilization was applied over the duration of the experiment. Weather conditions are presented in Table 1.
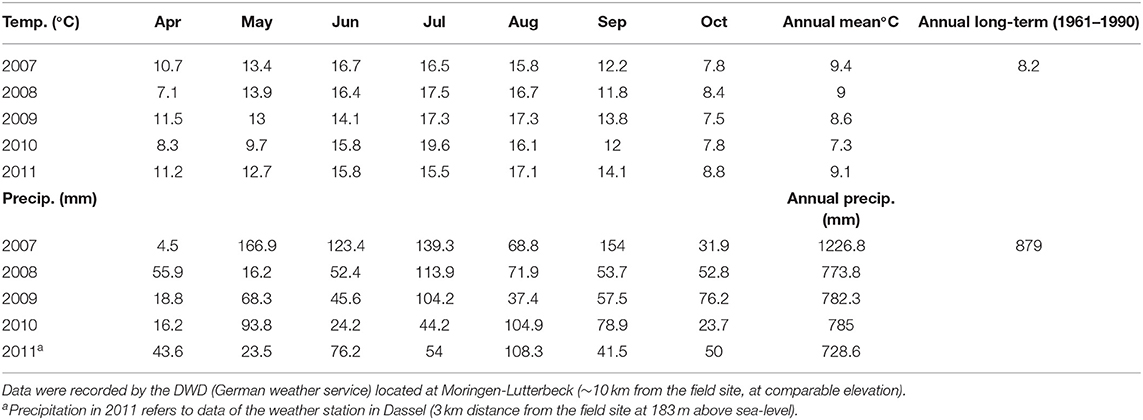
Table 1. Annual and monthly mean temperatures (°C) and precipitation sums (precip., mm) between April and October for the grazing seasons 2007 until 2011 compared to long-term averages (1961–1990).
Experimental Setup and Grazing Management
The experimental plots, i.e., paddocks, were laid out as a two-factorial (sward type and grazing-system) randomized block design with three replications. The plot size was 100 × 50 m resulting in 18 plots in total (two sward types × three grazing systems × three blocks).
The sward type was manipulated prior to the experiment in 2006 by applying herbicides against dicotyledonous plants {Starane Ranger and Duplosan KV with the active components fluroxypy [4-amino-3,5-dichloro-6-fluoro-2-pyridyloxyacetic acid] or mecocrop-P [(R)-2-(4-chloro-otolyloxy) propionic acid], respectively, at a rate of 3L ha−1 Starane and Duplosan KV}. In response to the herbicide application, grass-dominated (gd) paddocks were obtained, to provide a contrast to the diverse (div) paddocks with greater species richness and greater abundances of forbs and legumes (Table 2). To maintain the sward differentiation, a there was a second herbicide application in autumn 2009, according to Petersen et al. (2012). Records of single plant species numbers and proportions of plants and functional groups (grasses, legumes, forbs) were quantified annually in spring in each paddock from permanently installed subplots of 1 m2 size. On this basis, species richness and evenness were calculated (Magurran, 2004).
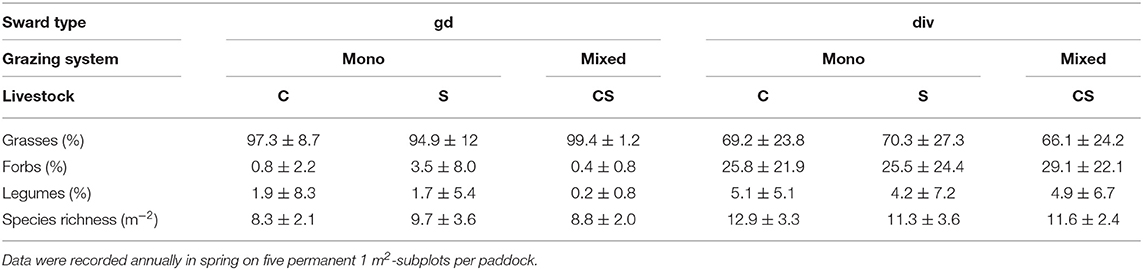
Table 2. Proportions of plant functional groups and species richness as affected by sward-type (gd, grass-dominated; div, diverse sward) and grazing system (mono-grazing with cattle (C) and sheep (S) vs. mixed-grazing of cattle and sheep (CS) averaged over years 2007–2011 (means ± sd).
In each year of the experiment the grazing period lasted from May to September and was divided into three grazing cycles (GC) with an interruption of 6 weeks for animal mating between GC 2 and GC 3. Suckler cows (German Simmental breed, lactation no. 2 ± 0.6) with calves (average age of 22 ± 4 days and 64 ± 4 kg average weight at the beginning of the grazing season); and ewes (German Blackheaded Mutton and Leine Sheep, lactation no. 3 ± 0.3) with lambs (average age of 132 ± 18 days and 33 ± 4 kg average weight at the beginning of the grazing season) were assigned equally to paddocks on a live weight (LW) basis. The stocking density declined from 3,000 kg LW ha−1 or 6 livestock units (LU) ha−1 (one LU represents 500 kg LW) during GC 1 and GC 2, to 2,000 kg LW ha−1 or 4 LUs ha−1 during GC 3 to account for reduced herbage growth rates in autumn. The total number of animals, as affected by grazing system and GC, is given in Table 3. Livestock adapted to grazing the area around the experimental paddocks prior to the start of the study were selected from the herd of the experimental farm. Paddocks were either stocked by cattle (C) or sheep (S) in mono-species grazing, or by cattle and sheep in mixed-grazing (CS). Stocking density in the mixed-grazing treatment was spread evenly between cattle and sheep. Each individual animal was consistently allocated within the same treatment group of individual animals during one year, and within a study year, each grazing treatment group stayed on the same sward type. During the interruption prior to GC 3, all individuals were kept within a large group grazing around the study plots. Before GC 3 animals were reallocated to the same treatment group as in GC 1 and GC 2; however, in a reduced number of animals per group. Blocks were grazed successively in a rotational system, beginning from the first block as exemplified in Figure 1. There were no interruptions of grazing between blocks within GC. In each block grazed, every treatment (sward type × grazing system) was covered by a total number of six herds (C, S, and CS for each sward type, i.e., gd- and div-swards) (Figure 1) so that treatment replications were grazed one after another but each treatment simultaneously within one block. Averaged over 5 years, the GC per block lasted between 8.8 ± 1.7, 5.5 ± 1.9, and 12.3 ± 0.9 grazing days for GC 1, GC 2, and GC 3, respectively. Periods for regrowth varied between 34 ± 6 and 93 ± 11 days between GC 1 and GC 2 or GC 2 and GC 3, respectively.
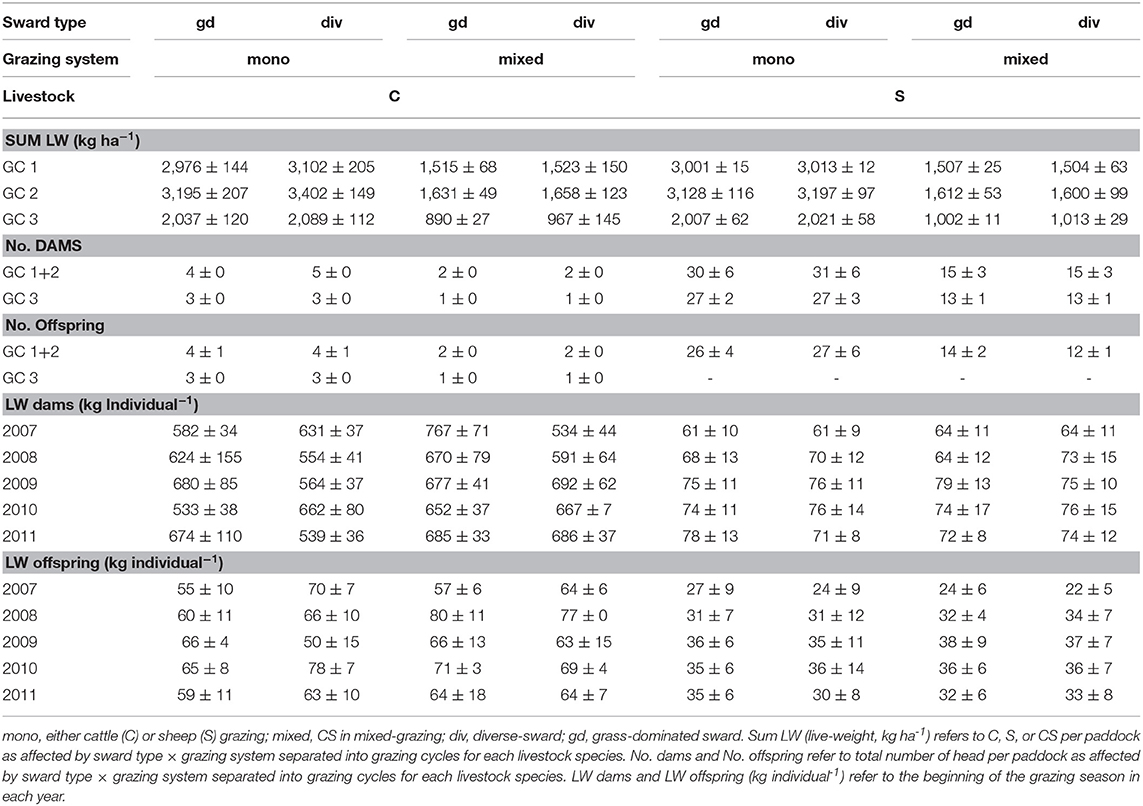
Table 3. Livestock characteristics during the grazing seasons 2007 until 2011 as affected by sward-type, grazing system and gracing cycle (GC) for each livestock species.
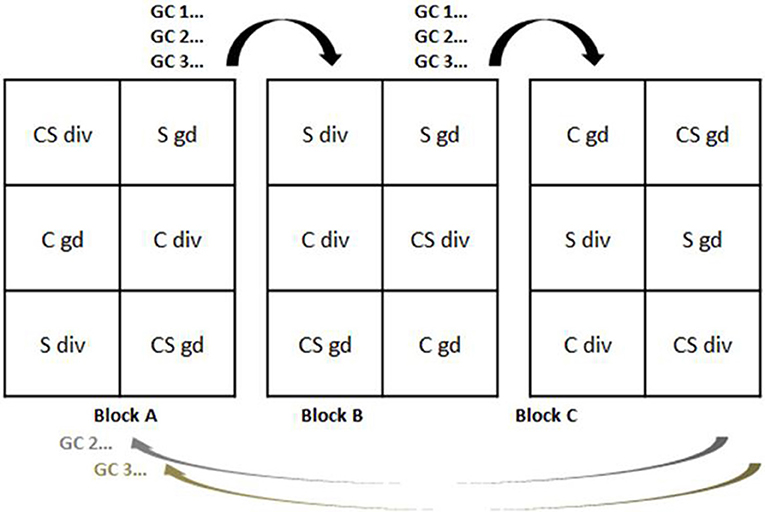
Figure 1. Conceptual figure of the grazing management during each grazing season from 2007 until 2011. C, cattle; S, sheep; CS, mixed-grazing of cattle and sheep; div, diverse-sward; gd: grass-sward; GC 1-3, number of grazing cycle. Each paddock consisted of 0.5 ha area. During each grazing cycle, blocks were grazed successively with a total of six herds each arranged within the factorial combination of sward type × grazing system. Blocks were grazed successively without interruption. Each individual animal stayed within the same group of animals allocated on the same sward type within each year.
Sward Measurements and Forage Quality
Prior and subsequent to each GC, the compressed sward height (CSH) was recorded at 50 randomly assigned sampling points per paddock, performed in a zigzag walk using a Rising Plate Meter (RPM, aluminum model, d = 0.3 m and 0.2 kg) (Castle, 1976). The average pre-grazing CSH (preCSH) was 30 cm height. To derive the herbage mass (HM), CSH measurements were calibrated regularly by cutting herbage samples close to ground level from below the RPM with known CSH. These samples were weighed and dried in a forced-air oven at 60°C for 48 h. The annually produced herbage mass (HM, kg DM ha−1) in 2010 and 2011 was then calculated based on these calibrations between CSH and biomass for each paddock and year with a coefficient of determination of 0.77 ± 0.11. For the present study, we used HM and forage quality data from the experimental years 2010 and 2011, whereas data on sward productivity and forage quality during the preceding experimental years (2007 until 2009) is given in Seither et al. (2012). The primary productivity was estimated from the pre- and postgrazing HM as sum of the pregrazing HM of GC 1 (pregraze HM1) and the difference between pre- and postgrazing HM from GC 2 (HM2) and GC 3 (HM3) according to the following equation:
Annual herbage accumulation = pregraze HM1 + (pregraze HM2–postgraze HM2) + (pregraze HM3 – postgraze HM3).
Vegetation regrowth during the stocking period was not quantified in this study. Therefore, the actual annual primary productivity was larger than calculated.
Concentrations of crude protein (CP), acid detergent fiber (ADF), gas formation (Gb), and crude lipids (CL) were quantified in the cut herbage samples used for calibration of HM, taken prior and subsequent to each GC and estimated using near infrared reflectance spectroscopy (NIRS). Spectra were evaluated with a calibration dataset of samples from different grasslands in Germany (Tillmann, 2010). Mean H-values (Mahalanobis distances) of 1.79 ± 0.68 indicated a sufficient coverage by the calibration set, and data with H-values above 3.0 were removed from the analysis (Petersen et al., 2013). The standard errors of calibration of CP, ADF, and CL were 0.69, 1.19, and 0.28, respectively. The Crude ash concentration (CA) was determined by burning subsamples in a muffle furnace (550°C, 12 h). These parameters were used to calculate the concentration of metabolizable energy (ME) according to GfE (2008) with the following equation:
ME (MJ kg−1) = 7.81 + 0.07559 Gb−0.00384 CA + 0.00565 CP + 0.01898 CL−0.00831 ADF.
Livestock Performance
Between 2007 and 2011, livestock weights were recorded prior to and subsequent to each GC. In addition, during GC 1, livestock were weighed prior to moving among blocks. On the basis of these measurements, average daily LW-gains (animal-ADG, g d−1) and area-related daily LW-gains (area-ADG, kg ha−1 d−1) were calculated with average values of total ADG measured between GCs divided by the number of grazing days separately for each livestock species. Additionally, area-related total LW-gain (kg ha−1) for each livestock species was calculated from recordings at the beginning and the end of the grazing season. During GC 3, no ADG-parameters were calculated due to reduced livestock numbers during that cycle. Therefore, final live weights of cattle refer to records after GC 1 plus GC 2. Moreover, individual ADGs of sheep were calculated exclusively for GC 1 due to the availability of precise scales during that GC. No weight recordings were obtained in 2009 after GC 2. Consequently, data of 2007 plus 2008 and 2010 plus 2011 are presented in this study as parameters for secondary productivity.
Statistical Analysis
Statistical analysis was conducted using the statistic software “R 2.14.2” (R Development Core Team, 2012). Model assumptions were checked visually, while transformations and variance modeling was performed where necessary (indicated in the results-part of this paper). Sward productivity parameters, forage quality and area-related livestock performance (area-ADG, kg ha−1 d−1 and total LW-gain, kg ha−1) were analyzed for effects of sward type and grazing system. Individual average daily livestock performance (animal-ADG, g d−1) was evaluated for effects of sward type and grazing system within one livestock species.
An analysis of covariance (ANCOVA) was conducted for sward productivity (t DM ha−1) with sward type, grazing system (mono- vs. mixed-grazing) and their interaction as fixed effects and block as categorical covariate separately for each year 2010 and 2011. For the post-grazing compressed sward height (postCSH, cm), a mixed model with sward type, grazing system and their interaction as fixed effects and the pre-grazing CSH as covariate was calculated. For forage quality (CP, ADF, and ME), statistical models included sward type, grazing system and interactions as fixed factors, and the GC was included as a covariate. The random structure accounted for repeated measurements of paddocks nested within blocks. Calculations were conducted separately for 2010 and 2011. A correlation analysis was conducted to quantify the relationships between species richness, evenness, and legume percentage as predictors on primary productivity (t DM ha−1) and forage quality (CP, ADF, ME) separately for 2010 and 2011. For each predictor (species richness, evenness and legume percentage) a separate model was generated. The model included year, the predictor and the interaction of year × predictor as fixed effects, while blocks and temporally repeated measurements were included in the random term. The replications of quality-data sampled within a treatment were pooled for this analysis.
The individual ADGs (animal-ADG, g d−1) of cattle and sheep were analyzed in mixed models using year, sward type, grazing system and their interactions as fixed effects separately for each species. The repeatedly measured ADG of individual animals was included within the random design and the GC was used as covariate. This model included the experimental years of 2007, 2008, 2010, and 2011. Additionally, an identical model of individual ADGs was performed for single years by excluding the factor year. For the analysis of daily area-ADG (kg ha−1 d−1) as well as total LW gain (kg ha−1) a mixed model was calculated, which accounted for similarities within years in the random term and included sward type, grazing system and their interactions as fixed factors. Comparison between treatment means for significant differences followed by post-hoc Tukey HSD tests.
Results
Primary Productivity and Forage Quality
In 2010 and 2011, primary productivity (t DM ha−1) was not affected by sward type or grazing system. However, postCSH was affected significantly by grazing system in 2011 (P ≤ 0.05) with a lower sward height in div-swards compared to gd-swards (Table 4). In contrast, CP was affected significantly by the interaction of sward type × grazing system in 2010 (P ≤ 0.05) as well as each by sward type (P ≤ 0.05) and grazing system (P ≤ 0.05) in 2011. Compared to gd-swards, div-swards reached greater CP concentrations (2010: P ≤ 0.01, 2011: P ≤ 0.05). The ADF concentration was significantly affected by the interaction of sward type × grazing system in 2010 (P ≤ 0.05), while in 2011 only sward type had a significant effect (P ≤ 0.05) with the div-swards reaching slightly lesser ADF contents compared to gd-swards (2011: P ≤ 0.05). For ME, ANOVA revealed a significant interaction of sward type × grazing system in 2010 (P ≤ 0.05).
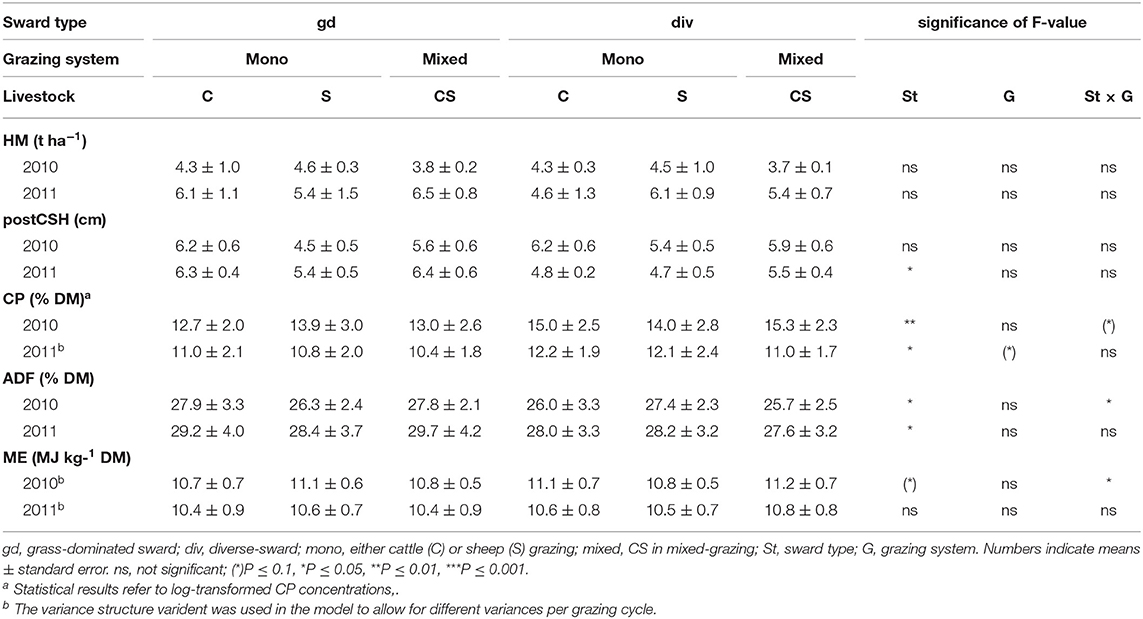
Table 4. Herbage mass (HM, t DM ha−1), postCSH (cm), and concentrations of CP (% DM), ADF (% DM), and energy (MJ ME kg−1 DM) as affected by sward type × grazing system for the experimental years 2010 and 2011.
Correlation analysis showed a positive relationship between species richness and CP concentration in both experimental years (Figure 2D). A trend for slightly negative effects of species richness on sward productivity and ADF was found, whereas ME remained unaffected (Figures 2A,G,J). There was no effect of evenness on productivity, CP, ADF or ME (Figures 2B,E,H,K). Proportions of legumes in the sward had no effect on sward productivity, while CP (P ≤ 0.01) and ME (P ≤ 0.01) increased and ADF (P ≤ 0.01) decreased with greater legume proportions in the sward (Figures 2C,F,I,L).
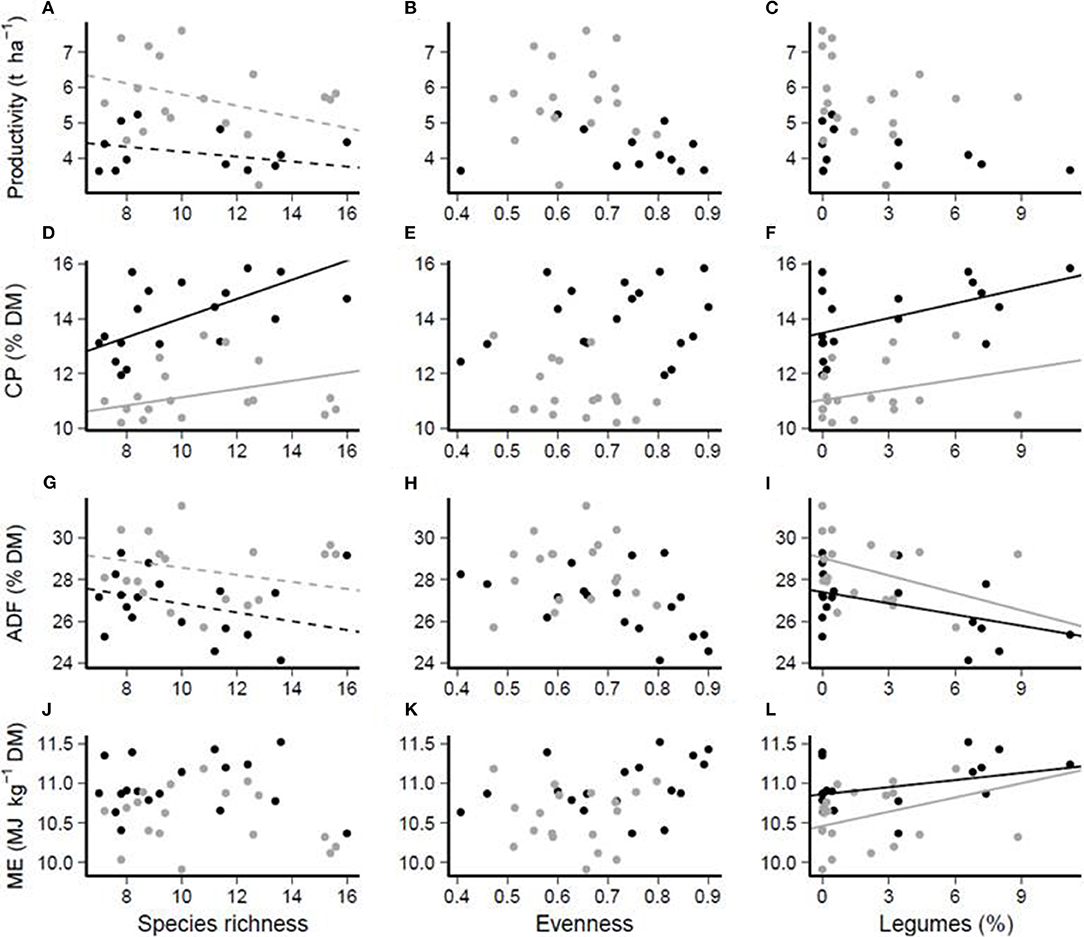
Figure 2. (A–L) Functional relationship between species richness (first column), evenness (second column), or legume proportion (%, third column) and productivity (first row), CP (crude protein, second row, % DM), ADF (acid detergent fiber, third row, % DM), or ME (metabolizable energy, fourth row, MJ ME kg−1 DM) concentrations, respectively. Black dots: year 2010, gray dots: year 2011. Solid lines indicate a significant relationship (P < 0.05) and dashed lines a trend (P < 0.1). Interactions between year and predictor were in no case significant.
Secondary Productivity
For animal-ADG (g d−1) of calves, a significant interaction between sward type × grazing system in 2007 (P ≤ 0.05) and an effect of sward type in 2010 (P ≤ 0.001) was found, with significantly greater animal-ADG on div-swards compared to gd-swards. Animal-ADGs of calves differed significantly among years (P ≤ 0.01) (Figure 3).
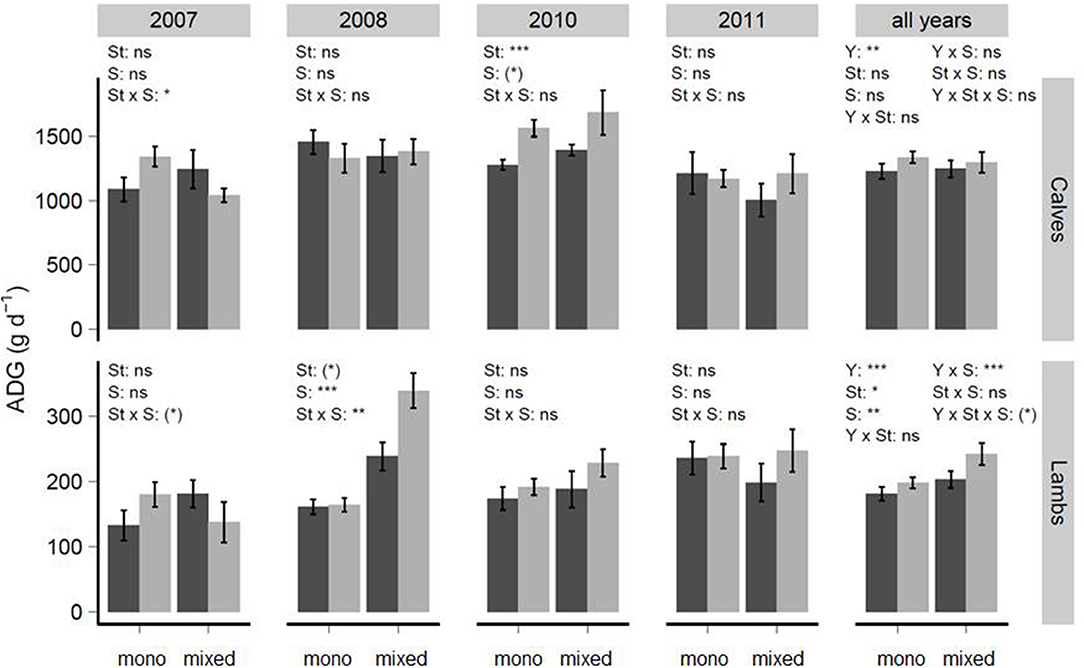
Figure 3. Animals' average daily gains (animal-ADG, g d−1) of calves and lambs (means ± se) in relation to year, sward type (St) and grazing system (S, mono- or mixed-grazing). The dark gray bars highlight grass-dominated swards, while light gray bars show diverse-swards. In statistical analyses of lamb animal-ADGs, the varIdent-structure was used to model heterogeneous variances within treatments (2008), grazing cycles and sward type (2010) or years (all years-model). ns, not significant, *P < 0.1, *P < 0.05, **P < 0.01, ***P < 0.001.
The animal-ADG (g d−1) of lambs was significantly affected by the interaction of sward type × grazing system in 2008 (P ≤ 0.01) with a larger animal-ADG in div-swards and mixed-grazing. Among years, the animal-ADG of lambs was greater on div-swards compared to gd-swards. Including year as factor into the model revealed a significant interaction between year × grazing system (P < 0.001).
Sward type affected cow animal-ADG (g d−1) in 2010 (P < 0.001). In 2010 and 2011, grazing system had a significant effect (P < 0.05), with a greater animal-ADG in mixed- compared to mono-grazing (Figure 4). Including year into the model resulted in a significant effect of the year (P < 0.001) and grazing system (P < 0.05) with greater animal-ADGs (g d−1) in mixed-grazing compared to mono-grazing (Figure 4). The interaction of sward type × grazing system affected animal-ADGs (g d−1) of ewes in 2007 (P < 0.001) and 2010 (P < 0.05). The results for 2011, however, showed a significant effect of grazing system (P < 0.05) and greater animal-ADGs in mixed- compared to mono-grazing was found (Figure 4). The model including year, showed a significant threefold interaction between year × sward type × grazing system (P < 0.001) (Figure 4).
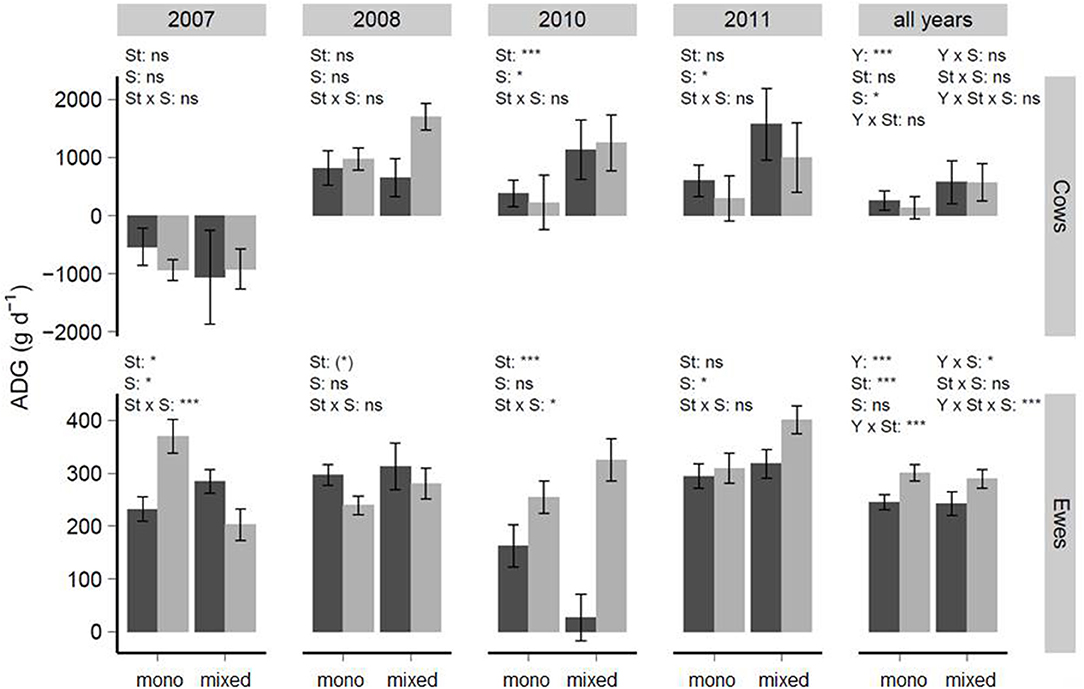
Figure 4. Average daily animal-ADG (animal-ADG, g d−1) of suckler cows and ewes (means ± se) in relation to year, sward type (St) and grazing system (S, mono-or mixed-grazing). The dark gray bars highlight grass-dominated swards, while light gray bars show diverse-swards. In statistical analyses of suckler cow animal-ADGs, the varIdent-structure was used to model heterogeneous variances between sward types (2007, 2010) or years (all years-model). Concerning ewes, the same variance modeling was used to account for differing variances between grazing cycle (2011) and years (all years-model). ns, not significant, P < 0.1, *P < 0.05, **P < 0.01, ***P < 0.001.
The grazing system had a significant effect for both total LW-gain (kg ha−1) (P < 0.01) and daily area-ADG (kg ha−1 d−1) (P < 0.001). For cattle, total LW-gain and daily area-ADG were less under mono-grazing compared to mixed-grazing as well when compared with sheep-grazing treatments (P < 0.05). No differences were found between the sheep-grazing treatments (Table 5).
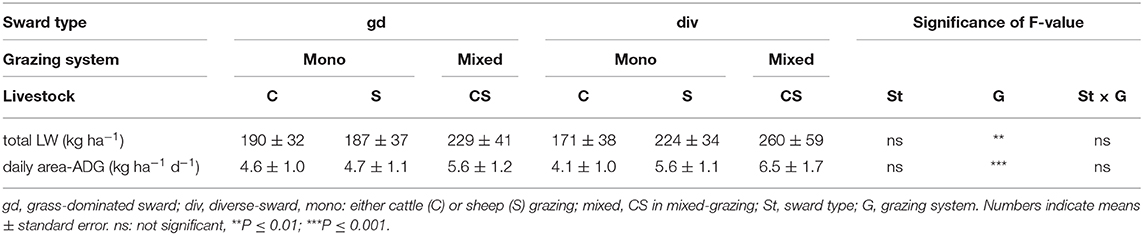
Table 5. Secondary productivity expressed as area-related total live weight (LW, kg ha−1) and daily area-related LW gains (area-ADG, kg ha−1 d−1) as affected by sward type × grazing system separated for each livestock species and grazing system averaged over years (2007, 2008, 2010, and 2011).
Discussion
Effects of Sward Type and Phytodiversity on Productivity
Primary Productivity of Swards
Biodiversity has traditionally been seen as a response variable rather than as a predictor of productivity (Kahmen et al., 2005). Phytodiversity experiments based on sown swards have provided new insights and in some cases shown positive responses of primary productivity to increased species richness (e.g., Hector et al., 1999; Tilman et al., 2001; Isbell et al., 2015) even despite lower fertilizer input (Grace et al., 2018b). This relationship has mainly been attributed to niche complementarity due to interspecific differences in resource use (Yachi and Loreau, 2007; Brophy et al., 2017).
Results of the present study are not in line with this hypothesis as no influence of sward type on primary productivity was found to support our first hypothesis. Moreover, species richness and primary productivity were slightly negatively correlated. The forage intake on the diverse-swards, however, was increased in 2011 as revealed by lower postCSH (Table 4). This was probably attributed to differences in grazing preference (Grace et al., 2018a). Our findings support an observational study by Kahmen et al. (2005) who investigated semi-natural grasslands in central Germany, in which species richness and aboveground productivity were not related. In that study, species composition was a better predictor of sward productivity. A pronounced effect of species richness on productivity was observed in studies with a limited number of (up to five) plant species (Roy, 2001; Wrage et al., 2011). On permanent grassland the range of species is typically much larger, which was also the case for the swards of the present study. Consequently, even species-poor grassland ecosystems can show a certain level of complementary resource use (Wrage et al., 2011; Petersen et al., 2013), and this may also explain the absence of a relationship between species richness and productivity in the present study.
Evenness, as an index of phytodiversity, was used by Kirwan et al. (2007) to predict primary productivity and showed a positive relationship, which was not in line with the present results. Several studies deriving positive relationships between biodiversity indicators (e.g., evenness) and primary productivity, have investigated newly established swards with productive legumes under moderately high external input (Kirwan et al., 2007; Brophy et al., 2017). The establishment of mixtures that included high yielding and N-fixing legumes (e.g., Medicago sativa or Trifolium repens) most likely contribute to positive phytodiversity effects on primary productivity during the initial years (Herrmann et al., 2008; Hector et al., 2010; Bouton, 2012). Legume proportions of only 5% were insufficient to improve the yield levels (Table 2). In the present study, the botanically simple sward treatment was achieved by using herbicides against dicotyledonous species. These herbicide applications resulted in a reduced level of species richness and an almost complete reduction of the proportion of biomass contributed by dicotyledons. Therefore, species richness and abundance of forbs and legumes were confounded per se and effects of species richness and occurrence of dicotyledonous plants cannot be disentangled. In addition, the heterogenous distribution between functional groups may also cause a lack of biodiversity effect (Jerrentrup et al., 2015). Nonetheless, experimental approaches based on species removal, as used in our study, provide an important link between controlled experiments with an artificially created vegetation composition and observational studies at natural sites that are subject to less controllable conditions (Diaz et al., 2003).
As part of normal agricultural practice, many farmers use herbicides to remove species regarded as weeds, or of low value, in order to extend the life of a sown sward and improve production of grasses and overall forage quality (Fuhlendorf et al., 2009; Petersen et al., 2013). However, Petersen et al. (2013) did not detect any consistent effect of herbicide application on the herbage yield in an experiment on cut grassland that had vegetation similar to the swards of the present study. Seither et al. (2012) also found no effects of sward manipulation on primary productivity in the initial years of the present study. This was also confirmed for belowground biomass (Rose et al., 2013) and is in line with the present results. Besides provision of forage for livestock, extensive management of diverse grasslands also contributes to the conservation of many species (Everwand et al., 2014; Pakeman et al., 2018). In addition, mixed low-intensity grazing was shown to offer good opportunities for butterflies (Fraser et al., 2014) and vertebrates (Evans et al., 2015) and this should be taken into account when evaluating the performance, in terms of ecosystem services, of such systems.
Forage Quality
As per our second hypothesis, div-swards containing dicotyledons (legumes and forbs) contributed to an increased herbage quality (Figures 2D,F,I,L). This may be attributed to their slower rate of maturation and greater digestibility, relative to grasses, throughout the year (Bruinenberg et al., 2002; White et al., 2004; Petersen et al., 2013). In addition, CP concentrations were higher on diverse swards (Figure 2D), in line with previous studies (Hofmann and Isselstein, 2005; Seip et al., 2012; Seither et al., 2012). The positive effect of species richness can be attributed to either a legume effect or a functional group effect. The significance of the relationship between CP concentration and species richness was stronger than that with legume percentage (Figures 2D,F). Consequently, the increased forage quality was not caused solely by legumes in this study. The identity and agricultural value of forbs might, therefore, have positively influenced forage quality (Sanderson et al., 2003; Hopkins and Holz, 2006; Grace et al., 2018b), which is emphasized by larger forb concentrations in the div-swards (Jerrentrup et al., 2015).
Growth of Livestock
The effect of phytodiversity on secondary productivity was less clear than expected in relation to our third hypothesis. There was no clear effect of sward type on lamb performance with respect to individual years. However, when averaged over years, lamb growth rates were 12%-higher in div-swards compared to gd-swards, and this was also confirmed for ewes (Figures 3, 4). Sheep benefit from grazing diverse swards with a greater array of plant species due to their preference for highly digestible and protein-rich plant material (Illius and Gordon, 1987; Rook et al., 2004). The share of forbs in the div-sward increased until 2011 (Jerrentrup et al., 2015). On average, 26.8 and 1.6% forbs were found in div- and gd-swards of the present study, respectively (Table 2). Legumes and forbs have the potential to increase sheep performance, and this could explain the differences found between sward types. For instance, Hutton et al. (2011) found increased live weights of ewes and lambs on a sward that contained chicory (Cichorium intybus), plantain (Plantago lanceolata), white (Trifolium repens), and red clover (Trifolium pratense) compared with a sward dominated by perennial ryegrass (Lolium perenne). In addition, certain forbs contribute positively to animal health and consequently to secondary performance; for instance, in a study by Tariq et al. (2008) yarrow (Achillea millefolium) reduced parasitic infections in sheep. The greater animal-ADG of lambs on div-swards in 2008 was indicated by the interaction of sward type × grazing system. This may possibly be attributed to a lower abundance of parasites in the mixed-grazing treatment, in conjunction with 20% forbs in the sward in that year (Jerrentrup et al., 2015; Grace et al., 2018a).
In general, no effect of sward type was found for animal-ADGs of calves. An exception was for 2010, when calves showed faster growth on the div-swards. This is consistent with results from an earlier grazing study that used the same breed (Giebelhausen et al., 2007). Milk yield of the cow represents the primary nutrient source for the suckled calf during the first month of life, and determines the growth rate (McGee et al., 2017). With respect to unaffected LW-gains of suckler cows by sward type in the present study, and under the premise of relatively constant lactation curves, only small responses to the vegetation structure may, therefore, be expected in calf growth rates. The year 2010, which was the exception in showing a sward-type effect on the calves (Figure 3), might be due to a higher milk yield of the cows on the div-swards compared to the gd-swards. Our findings, therefore, support the study of Fuhlendorf et al. (2009), who found no advantage in using herbicides to enhance grazing cattle daily live weight gains. The lower animal-ADG of cows in 2007 may be associated with 39% more precipitation in that year, relative to the long-term average, as Schütz et al. (2010), also found that excessive rain reduced the dry matter intake of cows.
Effect of Livestock Species and Grazing System
Primary Productivity and Forage Quality
In the present experiment, mixed-grazing had no impact on vegetation composition (Jerrentrup et al., 2015). Primary productivity was found to be more enhanced by grazing of sheep compared to cattle in previous studies (Boswell, 1977; Murphy et al., 1995; White and Knight, 2007). The present results, however, do not indicate an advantage of sheep over cattle with respect to total plant biomass and postCSH (Table 4). This is in accordance with earlier observations on the same experiment reported by Seither et al. (2012), who found no difference in primary productivity in response to grazing species. In that study, however, pre- and postgrazing forage quality (CP and ADF) differed as a consequence of sheep grazing due to the higher quality requirements and selectivity. Pre-grazing forage quality, however, was not affected by grazing species in the present study. This may be attributed to little variation of vegetation composition in response to the grazing species (Jerrentrup et al., 2015).
Consequences for Livestock Growth
Averaged over all experimental years of the present study, mixed- compared to mono-grazing increased animal-ADG of lambs by 17%. This finding supports our fourth hypothesis. Many authors have reported positive responses in sheep live weight gain to mixed-grazing (Abaye et al., 1994; Marley et al., 2006; Wright et al., 2006; Fraser et al., 2007, 2013, 2014; d'Alexis et al., 2013). Under mixed-grazing, better utilization of the pasture herbage (Forbes and Hodgson, 1985; Esmail, 1991) and reduction of intestinal parasite burdens have been suggested (Esmail, 1991; Marley et al., 2006; Fraser et al., 2013). Positive responses of cattle to mixed-grazing have seldom been reported (d'Alexis et al., 2013), and some studies have even indicated negative effects on cattle weights (Olson et al., 1999; Kitessa and Nicol, 2001). However, cattle might also profit from mixed-grazing since the quality of the herbage in the vicinity of dung patches increases as consequence of sheep removing herbage and thereby avoiding senescence (Nolan and Conolly, 1989).
The return of plant nutrients from excreta stimulates subsequent growth and quality of forage around dung and urine patches (MacDiarmid and Watkin, 1971; Scheile et al., 2018). However, cattle, and to a lesser extent sheep, avoid grazing around their own dung patches, which reduces herbage utilization (Scheile et al., 2018). The return of ingested N in urinary patches of cattle, however, may contain up to 99% of their previously incorporated N (Haynes and Williams, 1993). Consequently, urine patches on swards may contain greater nutrient concentrations (Forbes and Hodgson, 1985; Scheile et al., 2018). Sheep are known to graze relatively close to cattle dung patches and are consequently able to benefit from residual forage with more nutrients (Forbes and Hodgson, 1985; Murphy et al., 1995).
In the present study, an interaction between sward type and grazing system was found for the ADF concentration in the year 2010 (Table 4). In that year, herbage from sheep paddocks contained more ADF on div-swards but less on gd-swards. In comparison with cattle paddocks, sheep paddocks contained less ADF in the gd-swards and more ADF in div-swards. Sampled forage from cattle paddocks was characterized by having less ADF than that from sheep paddocks on div-swards; this was due potentially to less selective foraging by cattle and greater selectivity toward forbs by sheep. In addition, Seither et al. (2012) observed an increasing proportion of Dactylis glomerata in sheep paddocks of the present experiment. This explains the significant interaction of sward type × grazing system for the ME concentration in 2010. However, greater ADF intake in the herbage by cattle, relative to sheep, may diminish the response of cows towards different sward types. In the div-sward, a slightly positive impact of mixed-grazing on overall suckler cow performance was observed. Despite dietary overlap between both livestock species, cattle diets usually contain more reproductive vegetation and dead herbage (Dumont et al., 1995; Armstrong et al., 1997; Celaya et al., 2008). Consequently, cattle utilize forage left by sheep in the sward (Illius and Gordon, 1987).
Assuming an average surface area distribution of excretal patches of 30–40%, leading to forage loss in cattle pastures (White et al., 2001), the additional productivity benefit of mixed-grazing is highlighted. In the present study, mixed-grazing increased the area-related total LW by 20% compared to mono-grazing (13–37% ± 7.9) (Table 5), which is in accordance with previous studies (Olson et al., 1999; d'Alexis et al., 2013). In the present study, the benefit of mixed-grazing consequently ranges below the potential when considering the share of excretal patches, which may be attributed to stocking rate adaptations during the last grazing cycle or a greater surface area of dung patches. Moreover, calf growth rates were not affected by mixed-grazing. Calves are more selective and prefer forage of higher digestibility than mature cattle (Cazcarra and Petit, 1995). Consequently, calves may have a larger dietary overlap with sheep and show greater competition in mixed-grazing.
Conclusions
Sustainable grassland production seeks to maximize ecological and agronomic benefits. Long-term observations of management decisions on target variables are required due to variable climatic influences. The present study highlights both animal and grassland productivity on hilly and marginal regions of grassland production over a long-term period. As investigated here, less intensive systems contribute to an improved relationship between ecology and agronomy. With diverse swards and mixed-grazing, plant biodiversity was enhanced without adverse effects on productivity parameters. Consequently, ecological and agronomic goals were combined efficiently. Moreover, the present study indicated improved forage quality in response to increased species richness. Mixed-grazing of cattle and sheep on diverse permanent grassland represents a promising option for increasing resource-use efficiency and biodiversity of extensive grassland-based meat production.
Data Availability Statement
The datasets generated for this study are available on request to the corresponding author.
Ethics Statement
The animal study was reviewed and approved by Tierschutzbeauftragte der Universität Göttingen. No harmful treatment was conducted for the livestock. Written informed consent was not required from the owners for the participation of the animals owned by the conductor of this study.
Author Contributions
JJ initiated this manuscript and conducted data analysis. MK revised the manuscript. JJ, MS, and MC-H conducted most of the data sampling and experimental work. NW-M and JI designed and initiated the study, assisted with data analysis, and revision of the manuscript.
Funding
The research leading to these results has received funding from the European Community's Seventh Framework Programme (FP7/2007-2013) under the grant agreement no. FP7-244983 (MULTISWARD).
Conflict of Interest
The authors declare that the research was conducted in the absence of any commercial or financial relationships that could be construed as a potential conflict of interest.
Acknowledgments
We gratefully thank our technician Barbara Hohlmann for her help concerning all aspects of the experiment as well as Knut Salzmann and Arne Oppermann from the experimental farm in Relliehausen for animal handling. Furthermore, we thank Manfred Kayser for statistical advice and valuable comments on the manuscript. Thank goes to A. Hopkins for linguistic revision. This work is part of a Ph.D. programme leading to a Ph.D.-thesis (Jerrentrup, 2013). We acknowledge support by the Open Access Publication Funds of the Göttingen University.
References
Abaye, A., Allen, V., and Fontenot, J. (1994). Influence of grazing cattle and sheep together and separately on animal performance and forage quality. J. Anim. Sci. 72, 1013–1022. doi: 10.2527/1994.7241013x
Abaye, A. O., Allen, V. G., and Fontenot, J. P. (1997). Grazing sheep and cattle together or separately: effect on soils and plants. Agron. J. 89:380. doi: 10.2134/agronj1997.00021962008900030003x
Armstrong, R. H., Grant, S., Common, T. G., and Beattie, M. M. (1997). Controlled grazing studies on Nardus grassland: effects of between-tussock sward height and species of grazer on diet selection and intake. Grass Forage Sci. 52, 219–231. doi: 10.1111/j.1365-2494.1997.tb02352.x
Boswell, C. C. (1977). Comparisons of cattle grazing and sheep grazing. Proc. NZ Grassland Assoc. 38, 56–65.
Bouton, J. H. (2012). Breeding lucerne for persistence. Crop Pasture Sci. 63, 95–106. doi: 10.1071/CP12009
Brophy, C., Finn, J. A., Lüscher, A., Suter, M., Kirwan, L., Sebastià, M.-T., et al. (2017). Major shifts in species' relative abundance in grassland mixtures alongside positive effects of species diversity in yield: a continental-scale experiment. J. Ecol. 105, 1210–1222. doi: 10.1111/1365-2745.12754
Bruinenberg, M. H., Valk, H., Korevaar, H., and Struik, P. C. (2002). Factors affecting digestibility of temperate forages from seminatural grasslands: a review. Grass Forage Sci. 57, 292–301. doi: 10.1046/j.1365-2494.2002.00327.x
Bullock, J. M., Pywell, R. F., Burke, M. J., and Walker, K. J. (2001). Restoration of biodiversity enhances agricultural production. Ecol. Lett. 4, 185–189. doi: 10.1046/j.1461-0248.2001.00215.x
Carlsson, M., Merten, M., Kayser, M., Isselstein, J., and Wrage-Mönnig, N. (2017). Drought stress resistance and resilience of permanent grasslands are shaped by functional group composition and N fertilization. Agric. Ecosyst. Env. 236, 52–60. doi: 10.1016/j.agee.2016.11.009
Castle, M.E. (1976). A simple disc instrument for estimating herbage yield. Grass Forage Sci. 31, 37–40. doi: 10.1111/j.1365-2494.1976.tb01113.x
Cazcarra, R.F., and Petit, M. (1995). The influence of animal age and sward height on the herbage intake and grazing behaviour of Charolais cattle. Anim. Sci. 61, 497–506. doi: 10.1017/S1357729800014065
Celaya, R., Benavides, R., García, U., Ferreira, L. M. M., Ferre, I., Martínez, A., et al. (2008). Grazing behaviour and performance of lactating suckler cows, ewes and goats on partially improved heathlands. ANM 2:1818. doi: 10.1017/S1751731108003224
d'Alexis, S., Sauvant, D., and Boval, M. (2013). Mixed-grazing systems of sheep and cattle to improve liveweight gain: a quantitative review. J. Agric. Sci. 152, 1–12. doi: 10.1017/S0021859613000622
Diaz, S., Symstad, A. J., Stuart Chapin, F., Wardle, D. A., and Huenneke, L. F. (2003). Functional diversity revealed by removal experiments. Trends Ecol. Evol. 18, 140–146. doi: 10.1016/S0169-5347(03)00007-7
Dumont, B., Petit, M., and D'hour, P. (1995). Choice of sheep and cattle between vegetative and reproductive cocksfoot patches. Appl. Anim. Behav. Sci. 43, 1–15. doi: 10.1016/0168-1591(95)00553-5
Evans, D. M., Villar, N., Littlewood, N. A., Pakeman, R. J., Evans, S. A., Dennis, P., et al. (2015). The cascading impacts of livestock grazing in upland ecosystems: a 10-year experiment. Ecosphere 6:42. doi: 10.1890/ES14-00316.1
Everwand, G., Rösch, V., Tscharntke, T., and Scherber, C. (2014). Disentangling direct and indirect effects of experimental grassland management and plant functional-group manipulation on plant and leafhopper diversity. BMC Ecol. 14:1. doi: 10.1186/1472-6785-14-1
Forbes, T.D.A., and Hodgson, J. (1985). The reaction of grazing sheep and cattle to the presence of dung from the same or the other species. Grass Forage Sci. 40, 177–182. doi: 10.1111/j.1365-2494.1985.tb01735.x
Forteau, L., Dumont, B., Sallé, G., Bigot, G., and Fleurance, G. (2019). Horses grazing with cattle have reduced strongyle egg count due to the dilution effect and increased reliance on macrocyclic lactones in mixed farms. Animal 4, 1–7. doi: 10.1017/S1751731119002738
Fraser, M., Davies, D., Vale, J., Hirst, W., and Wright, I. (2007). Effects on animal performance and sward composition of mixed and sequential grazing of permanent pasture by cattle and sheep. Livest. Sci. 110, 251–266. doi: 10.1016/j.livsci.2006.11.006
Fraser, M., Vale, J., and Dhanoa, M. (2013). Alternative upland grazing systems: impacts on livestock performance and sward characteristics. Agric. Ecosyst. Environ. 175, 8–20. doi: 10.1016/j.agee.2013.05.002
Fraser, M. D., Moorby, J. M., Vale, J. E., Evans, D. M., and Kaltenboeck, B. (2014). Mixed-grazing systems benefit both upland biodiversity and livestock production. PLoS ONE. 9:e89054. doi: 10.1371/journal.pone.0089054
Fuhlendorf, S. D., Engle, D. M., O'Meilia, C. M., Weir, J. R., and Cummings, D. C. (2009). Does herbicide weed control increase livestock production on non-equilibrium rangeland? Agric. Ecosyst. Environ. 132, 1–6. doi: 10.1016/j.agee.2009.02.015
GfE (2008). New equations for predicting metabolisable energy of grass and maize products for ruminants. Proc. Soc. Nutr. Physiol. 17, 191–198.
Giebelhausen, H., Priebe, R., Lepetit, D., and Richter, K. (2007). Untersuchungen zur entwicklung der pflanzenbestände sowie der lebendmasse von kälbern auf mutterkuhweiden. Mitteil. Arbeitsg. Grünl. Futter. 8, 237–241.
Grace, C., Boland, T. M., Sheridan, H., Lott, S., Brennan, E., Fritch, R., et al. (2018b). The effect of increasing pasture species on herbage production, chemical composition and utilization under intensive sheep grazing. Grass Forage Sci. 73, 852–864. doi: 10.1111/gfs.12379
Grace, C., Lynch, M. B., Sheridan, H., Lott, S., Fritch, R., and Boland, T. M. (2018a). Grazing multispecies swards improves ewe and lamb performance. Animal 13, 1–9. doi: 10.1017/S1751731118003245
Haynes, R. J., and Williams, P. H. (1993). Nutrient cycling Iand soil fertility in the grazed pasture ecosystem. Advanc. Agron. 49, 119–199. doi: 10.1016/S0065-2113(08)60794-4
Hector, A., Hautier, Y., Saner, P., Wacker, L., Baggchi, R., Joshi, J., et al. (2010). General stabilizing effects of plant diversity on grassland productivity through population asynchrony and overyielding. Ecology 91, 2213–2220. doi: 10.1890/09-1162.1
Hector, A., Schmid, B., Beierkuhnlein, C., Caldeira, M. C., Diemer, M., Dimitrakopoulos, P. G., et al. (1999). Plant diversity and productivity experiments in european grasslands. Science 286, 1123–1127. doi: 10.1126/science.286.5442.1123
Herrmann, D., Boller, B., Studer, B., Widmer, F., and Kölliker, R. (2008). Improving persistence in red clover: insights from QTL analysis and comparative phenotypic evaluation. Crop Sci. 48, 269–277. doi: 10.2135/cropsci2007.03.0143
Hofmann, M., and Isselstein, J. (2005). Species enrichment in an agriculturally improved grassland and its effects on botanical composition, yield and forage quality. Grass Forage Sci. 60, 136–145. doi: 10.1111/j.1365-2494.2005.00460.x
Hopkins, A., and Holz, B. (2006). Grassland for agriculture and nature conservation: production, quality and multi-functionality. Agron. Res. 4, 3–20.
Hopkins, A., and Wilkins, R. J. (2006). Temperate grassland: key developments in the last century and future perspectives. J. Agric. Sci. 144:503. doi: 10.1017/S0021859606006496
Hutton, P., Kenyon, P., Bedi, M., Kemp, P., Stafford, K., West, D., et al. (2011). A herb and legume sward mix increased ewe milk production and ewe and lamb live weight gain to weaning compared to a ryegrass dominant sward. Anim. Feed Sci. Technol. 164, 1–7. doi: 10.1016/j.anifeedsci.2010.11.014
Illius, A., and Gordon, I. (1987). The allometry of food intake in grazing ruminants. J. Anim. Ecol. 56, 989–999. doi: 10.2307/4961
Isbell, F., Craven, D., Connolly, J., Loreau, M., Schmid, B., Beierkuhnlein, C., et al. (2015). Biodiversity increases the resistance of ecosystem productivity to climate extremes. Nature 526, 574–577. doi: 10.1038/nature15374
Isbell, F., and Wilsey, B.J. (2011). Increasing native, but not exotic, biodiversity increases aboveground productivity in ungrazed and intensely grazed grasslands. Oecologia 165, 771–781. doi: 10.1007/s00442-010-1877-9
Isselstein, J. (2005). “Enhancing grassland biodiversity and its consequences for grassland management and utilisation,” in Grassland: A Global Resource, ed D. A. McGilloway (Wageningen: Wageningen Academic Publishers), 305–320.
Isselstein, J., Jeangros, B., and Pavlu, V. (2005). Agronomic aspects of biodiversity targeted management of temperate grasslands in Europe – A review. Agron. Res. 3, 139–151.
Jerrentrup, J. S. (2013). Effects of Management on Biodiversity and Productivity of Grazed Grassland. Göttingen: Dissertation University of Göttingen.
Jerrentrup, J. S., Seither, M., Petersen, U., and Isselstein, J. (2015). Little grazer species effect on the vegetation in a rotational grazing system. Agric. Ecosyst. Environ. 202, 243–250. doi: 10.1016/j.agee.2015.01.007
Kahmen, A., Perner, J., Audorff, V., Weisser, W., and Buchmann, N. (2005). Effects of plant diversity, community composition and environmental parameters on productivity in montane European grasslands. Oecologia 142, 606–615. doi: 10.1007/s00442-004-1749-2
Kirwan, L., Lüscher, A., Sebastià, M. T., Finn, J. A., Collins, R. P., Porqueddu, C., et al. (2007). Evenness drives consistent diversity effects in intensive grassland systems across 28 European sites. J. Ecol. 95, 530–539. doi: 10.1111/j.1365-2745.2007.01225.x
Kitessa, S., and Nicol, A. (2001). The effect of continuous or rotational stocking on the intake and live-weight gain of cattle co-grazing with sheep on temperate pastures live-weig pastures. Anim. Sci. 72, 199–208. doi: 10.1017/S1357729800055697
Klimek, S., Marini, L., Hofmann, M., and Isselstein, J. (2008). Additive partitioning of plant diversity with respect to grassland management regime, fertilization and abiotic factors. Basic Appl. Ecol. 9, 626–634. doi: 10.1016/j.baae.2007.11.005
MacDiarmid, B. N., and Watkin, B. R. (1971). The cattle dung patch. 1. Effect of dung patches on yield and botanical composition of surrounding and underlying pasture. Grass Forage Sci. 26, 239–246. doi: 10.1111/j.1365-2494.1971.tb00670.x
Marley, C., Fraser, M., Davies, D., Rees, M., Vale, J., and Forbes, A. (2006). The effect of mixed or sequential grazing of cattle and sheep on the faecal egg counts and growth rates of weaned lambs when treated with anthelmintics. Vet. Parasitol. 142, 134–141. doi: 10.1016/j.vetpar.2006.06.030
McGee, M., Kavanagh, S., and Kennedy, E. (2017). TEAGASC beef manual – section 6. Calf Nutr. 36, 213–216.
Monteath, M. A., Johnstone, P. D., and Boswell, C. C. (1977). Effects of animals on pasture production. New Zeal. J. Agr. Res. 20, 23–30. doi: 10.1080/00288233.1977.10427297
Murphy, W., Mena Barreto, A., and Silman, J. P. (1995). Sward dynamics of a smooth-stalked meadowgrass dominant white clover sward rotationally grazed by cattle and/or sheep. Grass Forage Sci. 50, 183–190. doi: 10.1111/j.1365-2494.1995.tb02312.x
Nolan, T., and Conolly, J. (1989). Mixed v. mono-grazing by steers and sheep. Anim. Prod. 48, 519–533. doi: 10.1017/S0003356100004049
Olson, K., Wiedmeier, R., Bowns, J., and Hurst, R. (1999). Livestock response to Multispecies and deferred-rotation grazing on forested rangeland. J. Range Manage. 52, 462–470. doi: 10.2307/4003773
O'Mara, F. P. (2012). The role of grasslands in food security and climate change. Ann. Bot. 110, 1263–1270. doi: 10.1093/aob/mcs209
Pakeman, R. J., Fielding, D. A., Everts, L., and Littlewood, N. A. (2018). Long-term impacts of changed grazing regimes on the vegetation of heterogeneous upland grasslands. J. App. Ecol. 56, 1794–1805. doi: 10.1111/1365-2664.13420
Pavlu, V., Hejcman, M., Pavlu, L., Gaisler, J., and NeŽerková, P. (2006). Effect of continuous grazing on forage quality, quantity and animal performance. Agric. Eco. Env. 113, 349–355. doi: 10.1016/j.agee.2005.10.010
Petersen, U., Wrage, N., Köhler, L., Leuschner, C., and Isselstein, J. (2012). Manipulating the species composition of permanent grasslands—A new approach to biodiversity experiments. Basic Appl. Ecol. 13, 1–9. doi: 10.1016/j.baae.2011.10.003
Petersen, U., Wrage-Mönnig, N., and Isselstein, J. (2013). Effects of herbicide application to control sward composition in different management variants. Int. J. Biodivers. Sci. Ecosy. Serv. Manage. 9, 155–165. doi: 10.1080/21513732.2012.760488
Rook, A., Dumont, B., Isselstein, J., Osoro, K., WallisDeVries, M., Parente, G., et al. (2004). Matching type of livestock to desired biodiversity outcomes in pastures – a review. Biol. Conserv. 119, 137–150. doi: 10.1016/j.biocon.2003.11.010
Rose, L., Hertel, D., and Leuschner, C. (2013). Livestock-type effects on biomass and nitrogen partitioning in temperate pastures with different functional-group abundance. Grass Forage Sci. 68, 386–394. doi: 10.1111/gfs.12001
Roy, J. (2001). “How does biodiversity control primary productivity,” in Terrestrial Global Productivity, eds J. Roy, B. Saugier, and H. A. Mooney (San Diego, CA: Academic Press), 169–186. doi: 10.1016/B978-012505290-0/50009-0
Rusch, G. M., and Oesterheld, M. (1997). Relationship between productivity, and species and functional group diversity in grazed and non-grazed Pampas grassland. Oikos 78, 519–526. doi: 10.2307/3545613
Sanderson, M. A., Labreveux, M., Hall, M. H., and Elwinger, G. F. (2003). Nutritive value of chicory and english plantain forage. Crop Sci. 43, 1797–1804. doi: 10.2135/cropsci2003.1797
Sanderson, M. A., Soder, K. J., Muller, L. D., Klement, K. D., Skinner, R. H., and Goslee, S. C. (2005). Forage mixture productivity and botanical composition in pastures grazed by dairy cattle. Agron. J. 97:1465. doi: 10.2134/agronj2005.0032
Scheile, T., Isselstein, J., and Tonn, B. (2018). Herbage biomass and uptake under low-input grazing as affected by cattle and sheep excrement patches. Nutr. Cycl. Agroecosyst. 112, 277–289. doi: 10.1007/s10705-018-9945-3
Schütz, K. E., Clark, K. V., Cox, N. R., Matthews, L. R., and Tucker, C. B. (2010). Responses to short-term exposure to simulated raind and wind by dairy cattle: time budgets, shelter use, body temperature and feed intake. Anim. Welfare 19, 375–383.
Seip, K., Breves, G., Isselstein, J., Daş, G., and Abel, H. (2012). Ruminal fermentation characteristics and microbial nitrogen assimilation in sheep fed differently composed grass silages. Arch. Anim. Nutr. 66, 215–226. doi: 10.1080/1745039X.2012.676815
Seither, M., Wrage, N., and Isselstein, J. (2012). Sward composition and grazer species effects on nutritive value and herbage accumulation. Agron. J. 104:497. doi: 10.2134/agronj2011.0322
Sharpe, P. (2019). Mixed species grazing. Horse Past. Manage. 2019, 157–175. doi: 10.1016/B978-0-12-812919-7.00010-X
Soder, K., Sanderson, M., Stack, J., and Muller, L. (2006). Intake and performance of lactating cows grazing diverse forage mixtures. J. Dairy Sci. 89, 2158–2167. doi: 10.3168/jds.S0022-0302(06)72286-X
Soder, K. J., Rook, A. J., Sanderson, M. A., and Goslee, S. C. (2007). Interaction of plant species diversity on grazing behavior and performance of livestock grazing temperate region pastures. Crop Sci. 47:416. doi: 10.2135/cropsci2006.01.0061
Tariq, K., Chishti, M., Ahmad, F., and Shawl, A. (2008). Anthelmintic efficacy of Achillea millifolium against gastrointestinal nematodes of sheep: in vitro and in vivo studies. J. Helminthol. 82, 135–41. doi: 10.1017/S0022149X08912360
Tillmann, P. (2010). Anwendung der Nahinfrarotspektroskopie (NIRS) an Grünlandproben. VDLUFASchriftenreihe 66, 145–150. doi: 10.1007/978-3-642-02680-5_2
Tilman, D., Reich, P. B., Knops, J., Wedin, D., Mielke, T., and Lehman, C. (2001). Diversity and productivity in a long-term grassland experiment. Science 294, 843–845. doi: 10.1126/science.1060391
Totty, V., Greenwood, S., Bryant, R., and Edwards, G. (2013). Nitrogen partitioning and milk production of dairy cows grazing simple and diverse pastures. J. Dairy Sci. 96, 141–149. doi: 10.3168/jds.2012-5504
Tracy, B. F., and Faulkner, D. B. (2006). Pasture and cattle responses in rotationally stocked grazing systems sown with differing levels of species richness. Crop Sci. 46:2062. doi: 10.2135/cropsci2005.12.0473
White, S. L., Sheffield, R. E., Washburn, S. P., King, L. D., and Green, J.-T. Jr. (2001). Spatial and time distribution of dairy cattle excreta in an intensive pasture system. J. Env. Qual. 30, 2180–2187. doi: 10.2134/jeq2001.2180
White, T. A., Barker, D. J., and Moore, K. J. (2004). Vegetation diversity, growth, quality and decomposition in managed grasslands. Agric. Ecosys. Env. 101, 73–84. doi: 10.1016/S0167-8809(03)00169-5
White, T. A., and Knight, T. L. (2007). Pasture yield and composition under different land uses in north otago rolling downlands. Proc. N. Z. Grassland Assoc. 69, 65–71.
Wrage, N., Strodthoff, J., Cuchillo, H. M., Isselstein, J., and Kayser, M. (2011). Phytodiversity of temperate permanent grasslands: ecosystem services for agriculture and livestock management for diversity conservation. Biodivers. Conserv. 20, 3317–3339. doi: 10.1007/s10531-011-0145-6
Wright, I. A., Jones, J. R., Davies, D. A., Davidson, G. R., and Vale, J. E. (2006). The effect of sward surface height on the response to mixed-grazing by cattle and sheep. Anim Sci. 82, 271–276. doi: 10.1079/ASC200517
Keywords: species-rich grasslands, multispecies-grazing, biodiversity, livestock production, forage-quality
Citation: Jerrentrup JS, Komainda M, Seither M, Cuchillo-Hilario M, Wrage-Mönnig N and Isselstein J (2020) Diverse Swards and Mixed-Grazing of Cattle and Sheep for Improved Productivity. Front. Sustain. Food Syst. 3:125. doi: 10.3389/fsufs.2019.00125
Received: 11 September 2019; Accepted: 23 December 2019;
Published: 21 January 2020.
Edited by:
David Crowder, Washington State University, United StatesReviewed by:
Emma Louise Burns, Australian National University, AustraliaTommy Boland, University College Dublin, Ireland
Copyright © 2020 Jerrentrup, Komainda, Seither, Cuchillo-Hilario, Wrage-Mönnig and Isselstein. This is an open-access article distributed under the terms of the Creative Commons Attribution License (CC BY). The use, distribution or reproduction in other forums is permitted, provided the original author(s) and the copyright owner(s) are credited and that the original publication in this journal is cited, in accordance with accepted academic practice. No use, distribution or reproduction is permitted which does not comply with these terms.
*Correspondence: Martin Komainda, martin.komainda@uni-goettingen.de
†Present address: Jana Sabrina Jerrentrup, DBU Naturerbe GmbH, Osnabrück, Germany