- 1School of Biological Sciences, Washington State University, Pullman, WA, United States
- 2Department of Entomology, Washington State University, Pullman, WA, United States
- 3Department of Crop and Soil Sciences, Washington State University, Pullman, WA, United States
- 4Tree Fruit Research and Extensions Center, Washington State University, Wenatchee, WA, United States
- 5Department of Entomology, University of Georgia, Athens, GA, United States
- 6Centre for Tropical Environmental Sustainability Science, James Cook University, Cairns, QLD, Australia
To promote food security and sustainability, ecologically intensive farming systems should reliably produce adequate yields of high-quality food, enhance the environment, be profitable, and promote social wellbeing. Yet, while many studies address the mean effects of ecologically intensive farming systems on sustainability metrics, few have considered variability. This represents a knowledge gap because producers depend on reliable provisioning of yields, profits, and environmental services to enhance the sustainability of their production systems over time. Further, stable crop yields are necessary to ensure reliable access to nutritious foods. Here we address this by conducting a global meta-analysis to assess the average magnitude and variability of seven sustainability metrics in organic compared to conventional systems. Specifically, we explored the effects of these systems on (i) biotic abundance, (ii) biotic richness, (iii) soil organic carbon, (iv) soil carbon stocks, (v) crop yield, (vi) total production costs, and (vii) profitability. Organic farms promoted biotic abundance, biotic richness, soil carbon, and profitability, but conventional farms produced higher yields. Compared to conventional farms, organic farms had lower variability in abundance and richness but greater yield variability. Organic farms thus provided a “win-win” (high means and low variability) for environmental sustainability, while conventional farms provided a “win-win” for production by promoting high crop yields with low variability. Despite lower yields, and greater yield variability, organic systems had similar costs to conventional systems and were more profitable due to organic premiums. Our results suggest certification guidelines for organic farms successfully promote reliable environmental benefits, but greater reliance on ecological processes may reduce predictability of crop production.
Introduction
Organic agriculture is an ecologically intensive production system expanding worldwide as demand for sustainability increases (Eyhorn et al., 2019; Willer et al., 2019). Although organic farms produce lower yields than comparable conventional farms (Seufert et al., 2012; Ponisio et al., 2015), they are more profitable, more friendly to pollinators and the environment, and deliver equally or more nutritious foods with fewer pesticide residues (Kennedy et al., 2013; Tuck et al., 2014; Reganold and Wachter, 2016; Kovács-Hostyánszki et al., 2017; Seufert and Ramankutty, 2017). These findings are based on comparison studies (Mäeder et al., 2002; Crowder et al., 2010; Reganold et al., 2010; Forrest et al., 2015) and meta-analyses (Crowder et al., 2010, 2012; Gattinger et al., 2012; Seufert et al., 2012; Tuck et al., 2014; Crowder and Reganold, 2015; Ponisio et al., 2015; Lichtenberg et al., 2017; Lori et al., 2017) of the mean effects of organic and conventional farming systems on sustainability metrics such as biodiversity, yield, soil quality, and profitability; they did not consider variability. In contrast, few studies have considered variability of any sustainability metric over time (Pimentel et al., 2005; Smith et al., 2007).
Not taking variability of sustainability metrics into account when comparing production systems is a key knowledge gap. Producers depend on reliable provisioning of yields, profits, and environmental services to ensure production system sustainability over time. Moreover, reduced yield variability is necessary to ensure reliable food access for consumers (Schmidhuber and Tubiello, 2007; Müller et al., 2018; Mehrabi and Ramankutty, 2019). Low variability allows producers to achieve consistent production and avoid unprofitable years, while also ensuring that consumers have reliable access to nutritious and sufficient food. When producers are able to generate consistent crop yields, food prices are also less volatile and global trade markets are more stable (Müller et al., 2018). Decreased variability in ecosystem services can also prevent food shortages (Mehrabi and Ramankutty, 2019). Systems that promote low variability in ecosystem services may also be more resilient to changing climatic conditions, including an increased frequency of drought and extreme weather events (Schmidhuber and Tubiello, 2007).
We hypothesize that organic farms will have lower yields as well as greater variability in yield over time compared to conventional farms (Knapp and van der Heijden, 2018). This is because organic farms are more reliant on ecosystem services for production of high crop yields, whereas conventional farms can rely more heavily on external inputs (Reganold and Wachter, 2016). Specifically, conventional producers may be more readily able to react to pest outbreaks or low soil nutrient availability by applying a broad range of pesticides and synthetic fertilizers. In contrast, organic producers may be more strongly affected by changing environmental conditions that affect ecosystem service providers, such as soil microbes, pollinators, and natural enemy species (Mäeder et al., 2002; Crowder et al., 2010; Kennedy et al., 2013; Karp et al., 2018). If organic farms experience greater variability in yields, organic farmers may experience greater variability in profits. At the same time, we hypothesize that organic farms, when compared to their conventional counterparts, will have lower variability in environmental sustainability because they have a narrower range of control methods and management options (Reganold and Wachter, 2016). However, these hypotheses have never been tested. There also have been few attempts to identify “win-win” scenarios where organic or conventional farming systems optimize high productivity and low variability of various sustainability metrics.
We addressed these knowledge gaps by conducting a global meta-analysis assessing the mean and variability of seven sustainability metrics from paired comparison studies of organic and conventional systems. Our meta-analysis spanned 61 crop types on 6 continents (Figure 1; Figure S1) to assess seven sustainability metrics: (i) biotic abundance, (ii) biotic richness, (iii) soil organic carbon, (iv) soil carbon stocks, (v) crop yield, (vi) total production costs, and (vii) profitability. We also assessed how each metric varied for annual vs. perennial crops, study type (experimental station vs. farm), certification body (United States vs. European Union), and crop type. Overall, our study shows that the variability of ecosystem health metrics and farm production differs between organic and conventional systems and reveals areas where ecological intensive production systems need to improve to better address United Nations sustainable development goals (UN SDG; FAO et al., 2018).
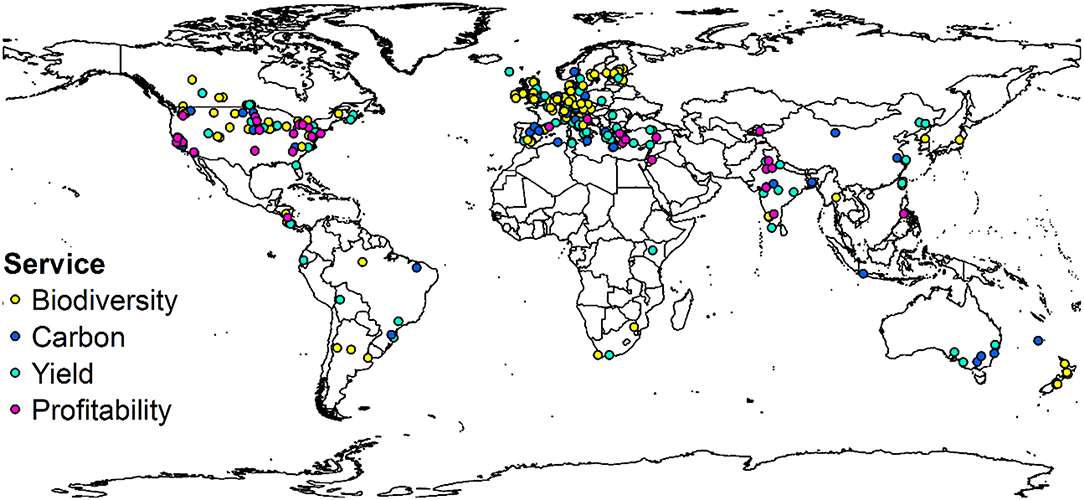
Figure 1. Study locations for seven sustainability metrics: biodiversity (abundance and richness), soil carbon (organic carbon and carbon stock), crop yield, and profitability (benefit/cost ratios and production costs).
Methods
Data Generation
We leveraged data from prior meta-analyses that reported means and standard deviations for paired organic and conventional systems related to the seven metrics of sustainability (see Supplementary Methods and Supplementary Data 1). We gathered estimates from the meta-data, which included one metric of mean and standard deviation averaged across replicates and years for each crop and/or organism measured in each study. Our variation metric thus represents a combination of spatial-temporal variability, hereafter variability. We gathered additional data from each study on country, continent, annual or perennial production system, certification body (United States or European Union), study type (experimental station or farm), and crop type (Tables S1–S5; Supplementary Data 2). For biotic abundance and biotic richness, we report estimates by organismal (arthropod, microbe, plant, vertebrate) and functional group (decomposer, natural enemy, herbivore, pollinator, producer) when available (Table S2). Organismal groups represent distinct taxonomic groups without regard to function, while functional group classifications represent the function of organisms on ecosystem services in agroecosystems. The final dataset had between 30 and 290 data points for each of the seven sustainability metrics (Figure 1; Figure S1; Tables S6, S7).
Meta-Analysis
For each sustainability metric, we used two variability measures: (1) standard deviation, which indicates absolute variability and (2) coefficient of variation, which indicates relative variability (i.e., standardized by means) (Knapp and van der Heijden, 2018) (Figure S2). Because the coefficient of variation is unitless and is scaled by the mean, it does a better job than standard deviation of accounting for differences in the means of the sustainability metrics between organic and conventional systems. We thus focus our results and discussion on the coefficient of variation, but we present the standard deviation in the Supplemental Materials for clarity and transparency. We calculated the coefficient of variation for all services by dividing the system (organic or conventional) standard deviation by the system mean.
To compare effects of farming system on means and variability for each sustainability metric, we used the log-response ratio as an effect size metric (Hedges et al., 1999). We used the log-response ratio rather than a weighted effect size for three reasons following Cardinale et al. (2006) and Crowder et al. (2010, 2012). First, weighted effect sizes could not be calculated for studies that did not report variability. Second, our biotic abundance and biotic richness studies classified organisms at varying levels of biotic resolution. Studies classified at a courser resolution had less variability, and a weighted metric would give these studies greater weight. Finally, studies conducted on experimental stations often relied on small-plots with extensive replication, while studies conducted on farms often were conducted at larger spatial scales but had decreased replication. Using weighted metrics would thus give small-plot studies on experimental stations considerably greater weight than on-farm studies which often are more representative of real-world farming situations (Crowder and Reganold, 2015).
Once log response-ratio effect sizes were calculated, we used one-sample t-tests (Crowder and Reganold, 2015; Lichtenberg et al., 2017) to determine whether the mean effect sizes for each sustainability metric, and associated variability, differed between conventional and organic sites. In parallel with the t-tests, we calculated 90% confidence intervals for each metric examined by adding and subtracting from the mean the standard error multiplied by the critical t-value at an α of 0.10 for a two-tailed test. We described effect sizes that were significant using an α of 0.10 to capture effects that may be ecologically important in accordance with a recent policy statement by the American Statistical Association, which notes that reliance on an arbitrary alpha value of 0.05 can lead to erroneous conclusions (Wasserstein and Lazar, 2016).
Evaluating Effects of Covariates on Mean and Variability of Sustainability Metrics
Our meta-analysis included a wide range of studies, and the context of these studies might affect the mean and variability of each of the seven sustainability metrics. To evaluate context-dependency, we considered effects of three key factors on each response variable. First, we assessed perenniality of crops given that perennial crops may have lower temporal variability than annual crops due to turnover of fields from year to year. Second, we considered whether studies were conducted on experimental stations or farms. Studies on experimental stations may not have sufficient time for organic treatments to manifest and may show greater differences than farm studies. Moreover, studies from experimental stations may not be as affected by economic considerations compared to farms where producer's livelihoods are at stake. Third, organic certification standards differ between the United States (US) and the European Union (EU), so we also evaluated the effects of certification scheme to determine if variation in these standards has affected sustainability of organic farming in the unique regions. Each comparison used a different subset of the data (Tables S8–S12). For example, when comparing US and EU certification schemes we excluded studies conducted in other regions.
For comparisons of how these covariates affected the means and variability for each of the seven sustainability metrics, we calculated 90% confidence intervals for each metric and used a linear mixed model with study as a random effect. In some cases the random effect described sufficiently small data that reliable model fits could not be made, and therefore we used Welch's t-tests for these comparisons. Comparisons focused on the mean, standard deviation, and coefficient of variation of each sustainability metric for each covariates. To further evaluate context dependency, we calculated 90% confidence intervals from a t-distribution for each crop type (cereals, fruits, oil crops, pulses, root crops, vegetables, or forage) (Table S13), for means and coefficient of variation of each of the seven focal metrics.
Results and Discussion
Effects of Organic Agriculture on Mean Sustainability Metrics
Compared to conventional sites, organic sites had greater (i.e., log response ratio > 0) mean biotic abundance, biotic richness, soil organic carbon, carbon stocks, and profitability (Figure 2A; Table 1), with stronger effects (i.e., higher log mean response ratios) observed for abundance, richness, and profitability compared to soil metrics (Table 1). Organic sites had lower yield than conventional sites but had similar total production costs (Figure 2A; Table 1); the reduced yield effect was similar in magnitude to the positive effects of organic farming on abundance, richness, and profitability (Table 1). For biotic abundance and richness, organic sites had higher mean effect sizes for all organismal and functional groups, except microbial richness, herbivore abundance, and decomposer richness, which were similar for both management systems (Figures 3A,B; Figure S3; Tables S14, S15). Overall, these results are in line with previous research showing that sustainability metrics other than crop yield, including greater profitability, are promoted by organic farming systems compared to conventional systems (e.g., Crowder et al., 2010, 2012; Gattinger et al., 2012; Kennedy et al., 2013; Tuck et al., 2014; Crowder and Reganold, 2015; Reganold and Wachter, 2016; Lichtenberg et al., 2017).
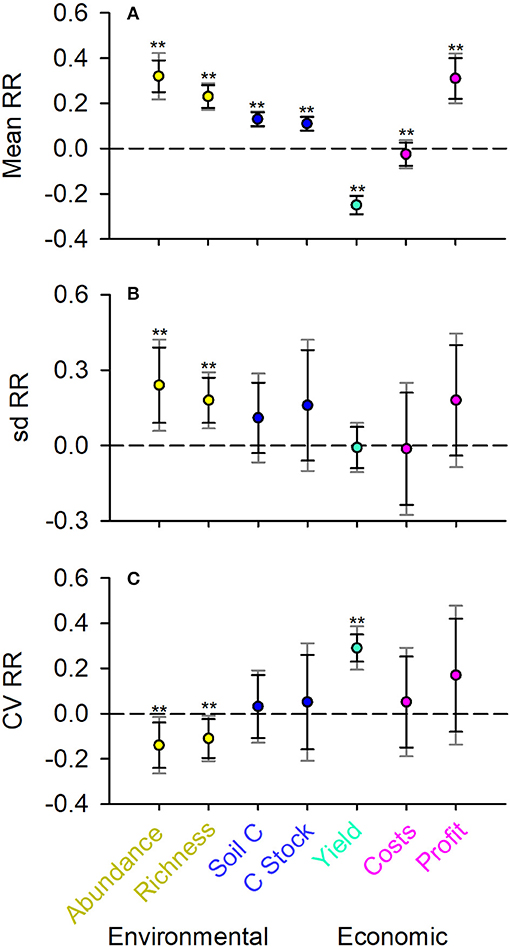
Figure 2. (A) Mean response ratio, (B) standard deviation response ratio, and (C) coefficient of variation response ratio of organic compared to conventional farming systems for seven sustainability metrics. All values shown are means ± 90% CI (black) and ± 95% CI (gray). **Indicates 90% and 95% CIs did not overlap 0.

Table 1. Mean response ratio, standard deviation response ratio, and coefficient of variation response ratio mean and 90% confidence interval (CI) for 7 sustainability metrics.
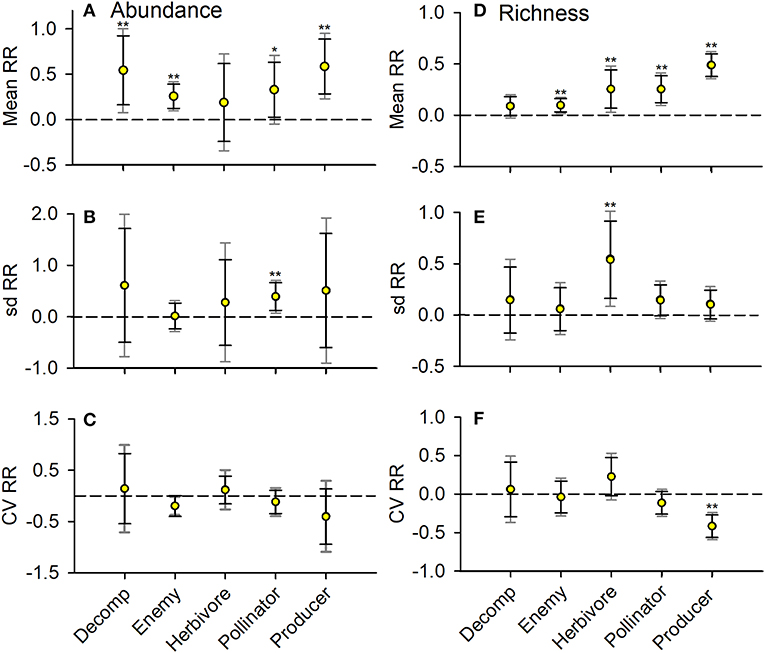
Figure 3. Functional group (decomp = decomposer, enemy = natural enemy, herbivore, pollinator, producer) (A) abundance mean response ratio, (B) abundance standard deviation response ratio, (C) abundance coefficient of variation response ratio, (D) richness mean response ratio, (E) richness standard deviation response ratio, and (F) richness coefficient of variation response ratio. All values shown are means ± 90% CI (black) and ± 95% CI (gray). *Indicates 90% CI did not overlap 0; **indicates 95% CI did not overlap 0.
The benefits of organic farming for mean biotic abundance, mean biotic richness, and mean profitability were robust to variation in each of the covariates we evaluated (annual vs. perennial crops, experimental station vs. farm, and US vs. EU certification guidelines), while mean yield was lower for organic systems across all these comparisons (Figure 4; Tables S12–S21). Alternatively, mean soil organic carbon and mean carbon stocks each benefited more from organic farming in the US than in the EU and carbon stock benefited more from organic farming in annual compared to perennial crops as demonstrated by a significant effect of each covariate on the response ratio (Figure 4; Tables S12–S21).
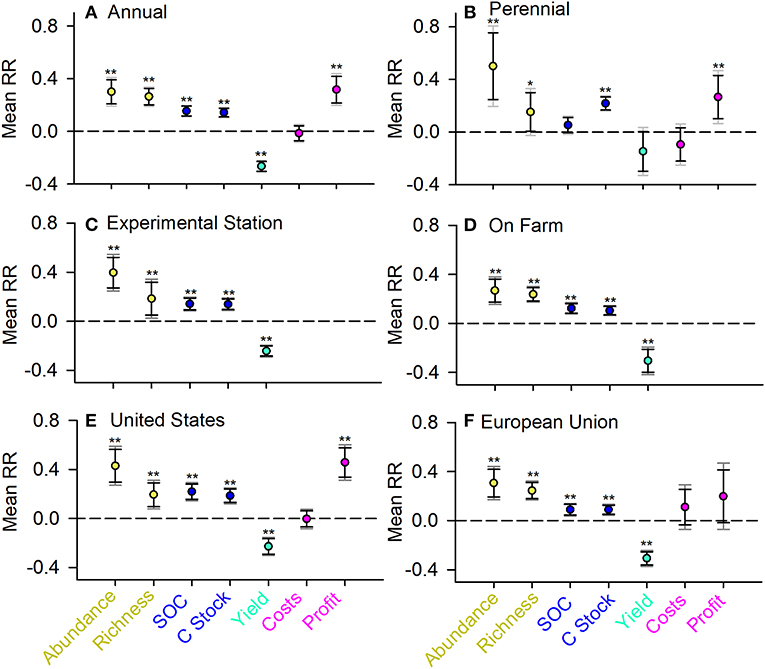
Figure 4. Response ratio for mean sustainability (± 90% CI black; 95% CI gray) for (A) annual crop types, (B) perennial crop types, (C) on experimental stations, (D) on farms, (E) studies in the United States, and (F) studies in the European Union. *Indicates 90% CI did not overlap 0; **indicates 95% CI did not overlap 0.
Effects of Organic Agriculture on Variability of Sustainability Metrics
Compared to conventional sites, organic sites had lower variability (i.e., CV response ratio < 0) in biotic abundance and richness (Figure 2C; Table 1) but higher yield variability (Figure 2C; Table 1), although the magnitude of effects on yield variability (i.e., log variability response ratios) were more than twice as large as the magnitude of effects on biotic communities (Table 1). In contrast, organic and conventional sites exhibited similar variability in soil organic carbon, carbon stocks, total production costs, and profitability (Figure 2C; Table 1). Standard deviations in biotic abundance and richness were generally greater on organic farms, but the magnitude of this difference was small in comparison to the large difference in mean biotic abundance and richness in the two farming systems (Figure 2B; Figure S4). Similarly, organic farming significantly reduced mean yield, but not standard deviation, leading to a higher coefficient of variation (Figure 2; Figure S4).
Our evaluation of covariates suggests our findings are quite robust. Of the 19 comparisons we made for effects of covariates (annual vs. perennial crops, experimental station vs. farm, and US vs. EU certification guidelines) on coefficient of variation response ratios for the sustainability metrics, only two were significant at α = 0.10 (Figure 5; Tables S17, S19, S21). Further breaking down variability of abundance and richness by organismal type or functional groups indicated that organic farming had significantly lower variability in plant and producer richness, whereas other organismal groups had similar variability between systems (Figures 3C–F; Figure S3; Tables S14, S15).
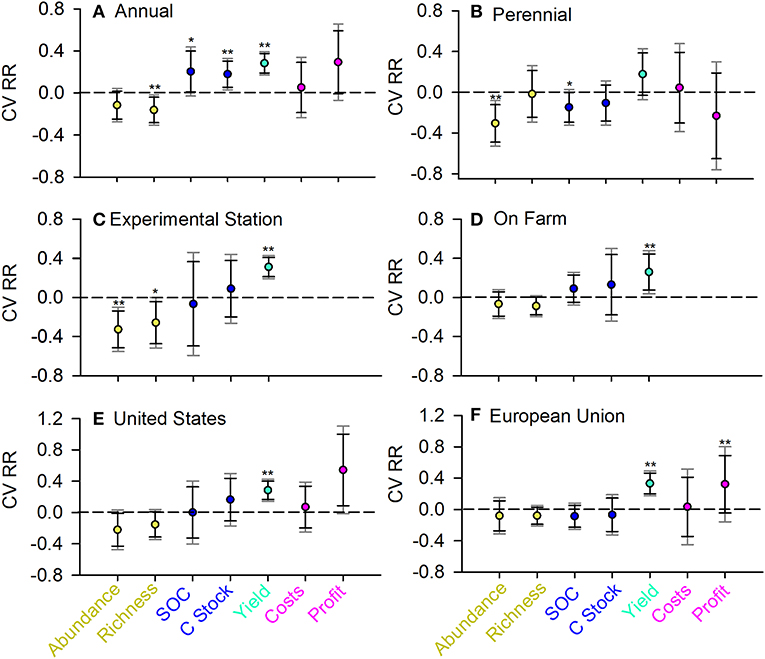
Figure 5. Response ratio for coefficient of variation of sustainability (± 90% CI black; 95% CI gray) for (A) annual crop types, (B) perennial crop types, (C) on experimental stations, (D) on farms, (E) studies in the United States, and (F) studies in the European Union. *Indicates 90% CI did not overlap 0; **indicates 95% CI did not overlap 0.
Organic certification restricts organic farms to using a narrower set of agrochemical inputs than conventional farms (Reganold and Wachter, 2016). This may discourage disturbance and may result in lower variability in environmental health and impacts (Schrama et al., 2018). Our results reflect this, as organic sites had lower variability in biotic abundance and richness. This suggests that organic certification schemes effectively limit negative environmental effects of agriculture on communities, which benefits beneficial natural enemy populations (Crowder et al., 2010; Porcel et al., 2018) and pollinators (Kennedy et al., 2013). Because organic certification standards exclude most chemical fertilizers and pesticides, organic farmers have to plan ahead, not having instant solutions to the problems that come up. Organic systems are also more information intensive, requiring farmers to learn how to manage longer rotations, grow different crops together, and come up with cultural and mechanical strategies for battling weeds and disease (Reganold and Wachter, 2016; Seufert and Ramankutty, 2017). This in turn forces heavier reliance on ecosystem service providers, such as biological control of pests, to produce adequate yields (Karp et al., 2018). While organic fields have a greater richness of these ecosystem service providers (Crowder et al., 2010; Kennedy et al., 2013; Tuck et al., 2014; Lichtenberg et al., 2017), the effects of organic management do not persist at larger scales (Schneider et al., 2014), potentially denying organic farmers any spillover benefits.
Conventional farms, in contrast, may be capable of more readily adapting to changing environmental pressures by having a greater variety of pesticide and fertilizer options, leading to a decrease in yield variability. This is similar to studies showing that greater dependency of a crop on ecological services, like pollination or biological control, can be positively correlated with greater yield variability (Garibaldi et al., 2011; Martin et al., 2013). Recent theoretical work also suggests that increased yield of crops dependent on ecosystem service providers may often be correlated with higher variability (Montoya et al., 2019). These factors are reflected in the lower yield variability on conventional farms in our dataset. However, although yields were more variable on organic compared with conventional farms, we observed similar variability in costs and profitability for the two systems (Figure 2; Table 1). This suggests that geographical or temporal fluctuations in crop prices or organic premiums may allow organic farmers to achieve relatively consistent profits regardless of differences in crop yield. While organic farms often have higher labor costs, they have reduced variable costs, which likely contributes to the overall lack of differences in cost variability across the two systems (Crowder and Reganold, 2015). Moreover, price premiums on organic goods are likely the primary reason that organic farms produce greater overall profits than conventional farms despite lower overall yields and greater yield variability (Crowder and Reganold, 2015).
Soil organic carbon had higher means on organic farms but similar variability across the two systems. In addition to land use, climate, elevation, soil type, and soil thickness are the most influential factors affecting soil carbon in agroecosystems (Teng et al., 2017). This is important because these factors are independent of cropping system, which may contribute to the similar variability we observed between organic and conventional sites. Additionally, practices such as tillage and biomass harvest affect variability of soil carbon within both organic and conventional systems (West and Post, 2002), and alter communities of beneficial soil arthropods (Patterson et al., 2019). We did observe greater effects of farming system (organic vs. conventional) on soil organic carbon in annual vs. perennial crops, such that differences in tillage for organic and conventional systems may have important effects on soil health (Figure 5).
Relationships Between Mean and Variability of Sustainability Metrics
We assessed whether the mean and variability of each sustainability metric were related. If particular systems produce high means and low variability, this reflects a “win-win” scenario that would benefit producers. Importantly, a plot of each mean sustainability metric response ratio by its coefficient of variation response ratio showed a similar pattern across crop types: for each metric examined, the higher the mean, the lower the coefficient of variation (Figure 6; Figure S5; Tables S22–S27). Thus, cropping systems with the highest yields tended to have the least variable yields (Figure 6C), and systems with high levels of richness had the least variable richness (Figure 6B). These results suggest that practices which lead to greater mean sustainability are also associated with less variation in the sustainability metric, and vice versa. Organic agriculture produced a “win-win” for biological communities by promoting high diversity with low variability, while conventional agriculture produced a “win-win” for production by promoting high yields with low variability. Organic and conventional agriculture generally had similar costs and profitability for crop types examined (Figures 6D,E).
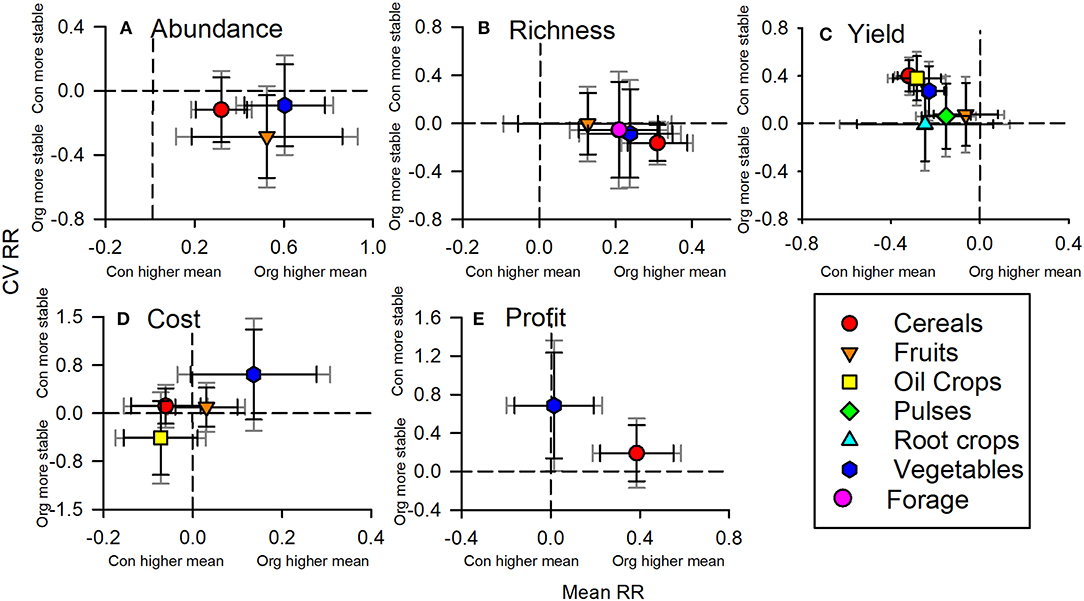
Figure 6. Mean response ratio (± 90% CI black; 95% CI gray) vs. coefficient of variation response ratio (± 90% CI black; 95% CI gray) for seven FAO crop types (cereals = red, fruits = orange, oil crops = yellow, pulses = green, root crops = teal, vegetables = blue, and forage crops = pink) for (A) biotic abundance, (B) biotic richness, (C) crop yield, (D) total production costs, and (E) profitability.
Within crop types, there appeared to be a trade-off between yield and biotic richness (Figure 6; Figure S5), which conforms with previous studies (Gabriel et al., 2013). Crop types with high mean and low coefficient of variation for organic system yields (Figure 6C) tended to have low mean and high coefficient of variation in organic system species richness compared to conventional systems (Figure 6B). Cereals had the greatest increase in richness from organic methods and the greatest decrease in yield, for example. In contrast, fruit crops had the smallest increase in richness but also the lowest decrease in yield; vegetable and forage crops had an intermediate biotic richness and yield tradeoff (Figure 6; Figure S5). This may be related to the varying degrees of pollinator dependence by crop type- fruits tend to be highly reliant on pollination while cereals crops are not (Andersson et al., 2012). Different specific management methods used in different crop systems may explain why the magnitude of differences in the ecological, production, and economic outcomes of organic vs. conventional farming depended on crop types. Identifying which specific practices are associated with these outcomes would likely lead to improvements in the magnitude and stability of sustainability metrics across the spectrum of crop types.
Conclusion and Future Directions
Our results identify an apparent trade-off between variability in environmental impacts and agricultural production, such that efforts to reliably curb environmental impacts through organic certification may come at a cost of lower and more variable yields. However, despite the lower yields and greater yield variability on organic farms, organic farms were more profitable, and had similar costs compared than conventional farms. This is likely due to the organic premiums received, which can vary with market conditions and mitigate effects of lower yields (Crowder and Reganold, 2015). Despite the relatively high profits of organic farming systems, a critical hurdle for organic farming, and other types of ecologically intensive farming systems, is to identify methods to improve the stability of ecosystem service provisioning that enhances and supports consistently high yields. This effort will benefit from experimental research on agricultural systems that focuses on not just means but also variation in yields and biological conservation in agroecosystems. If it is not possible to identify methods to reduce variability in food production by stabilizing ecosystem services, we will need to strike a balance between the stability of food production and the ecological functions provided by agroecosystems.
An important caveat associated with our results is that the vast majority of research to date has been conducted in developed countries, mainly in the European Union and the United States (Figure 1; Figure S1). While variability in agricultural yields in these countries may impact global market volatility and commodity prices, agricultural policies in these developed countries can help buffer the effects of yield volatility on greater market impacts (Thompson et al., 2018). In contrast, food security is at greatest risk in less-developed countries, where we have the least data. Commercial management practices in developed countries may be able to tolerate higher variability of services because they have a wider array of tools to manage and control changing field conditions, but the negative effects of inputs like synthetic fertilizer and pesticides suggest this management may come at a cost of ecosystem stability. This relationship between yield and the stability of other sustainability metrics may play out differently in less-developed countries where low-income farmers cannot keep up the inputs necessary to maintain yields in conventional or organic systems and fall into a poverty gap (Tittonell and Giller, 2013).
A key to accomplishing the United Nations Food and Agricultural Organization's sustainable development goals is to both reduce the environmental impacts of agriculture, as well as to improve food security by reducing yield variability in food-insecure regions (FAO et al., 2018). Our results highlight a need to better understand (i) the ways in which these goals are linked and (ii) trade-offs between variability in environmental impacts and yields that may exist. If the trade-offs we have identified are ubiquitous in both developed and less-developed regions, new methods of improving the long-term sustainability of ecosystem service provisioning are needed to avoid trade-offs between food security and ecosystem stability.
Data Availability Statement
All datasets generated for this study are included in the manuscript/Supplementary Files.
Author Contributions
All authors contributed conception and design of the study. OS, AC, and JT organized the database. OS and TN performed the statistical analyses. OS, AC, CR, AD, RO, TN, and DC wrote sections of the manuscript. All authors contributed to manuscript revision, read, and approved the submitted version.
Funding
This work was supported by the USDA National Institute of Food and Agriculture, Hatch project 1014754.
Conflict of Interest
The authors declare that the research was conducted in the absence of any commercial or financial relationships that could be construed as a potential conflict of interest.
Acknowledgments
We thank W. Ford for critical edits on the manuscript.
Supplementary Material
The Supplementary Material for this article can be found online at: https://www.frontiersin.org/articles/10.3389/fsufs.2019.00082/full#supplementary-material
References
Andersson, G. K., Rundlöf, M., and Smith, H. G. (2012). Organic farming improves pollination success in strawberries. PLoS ONE 7:e31599. doi: 10.1371/journal.pone.0031599
Cardinale, B. J., Srivastava, D. S., Dummet, J. E., Wright, J. P., Downing, A. L., Sankaran, M., et al. (2006). Effects of biodiversity on the functioning of trophic groups and ecosystems. Nature 443, 989–992. doi: 10.1038/nature05202
Crowder, D. W., Northfield, T. D., Gomulkiewicz, R., and Snyder, W. E. (2012). Conserving and promoting evenness: organic farming and fire-based wildland management as case studies. Ecology 93, 2001–2007. doi: 10.1890/12-0110.1
Crowder, D. W., Northfield, T. D., Strand, M. R., and Snyder, W. E. (2010). Organic agriculture promotes evenness and natural pest control. Nature 466, 109–112. doi: 10.1038/nature09183
Crowder, D. W., and Reganold, J. P. (2015). Financial competitiveness of organic agriculture on a global scale. Proc Natl Acad Sci U.S.A. 112, 7611–7616. doi: 10.1073/pnas.1423674112
Eyhorn, F., Muller, A., Reganold, J. P., Frison, E., Herren, H. R., Luttikholt, L., et al. (2019). Sustainability in global agriculture driven by organic farming. Nature 2, 253–255. doi: 10.1038/s41893-019-0266-6
FAO, IFAD, UNICEFF, WFP, and WHO. (2018). The State of Food Security and Nutrition in the World 2018: Building Climate Resilience for Food Security and Nutrition. Rome: Food and Agriculture Organization of the United Nations.
Forrest, J. R. K., Thorp, R. W., Kremen, C., and Williams, N. M. (2015). Contrasting patterns in species and functional-trait diversity of bees in an agricultural landscape. J Appl Ecol. 52, 706–715. doi: 10.1111/1365-2664.12433
Gabriel, D., Sait, S. M., Kunin, W. E., and Benton, T. G. (2013). Food production vs. biodiversity: comparing organic and conventional agriculture. J Appl Ecol. 50, 355–364. doi: 10.1111/1365-2664.12035
Garibaldi, L. A., Aizen, M. A., Klein, A. M., Cunningham, S. A., and Harder, L. D. (2011). Global growth and stability of agricultural yield decrease with pollinator dependence. Proc Natl Acad Sci U S A. 108, 5909–5914. doi: 10.1073/pnas.1012431108
Gattinger, A., Muller, A., Haeni, M., Skinner, C., Fleissbach, A., Buchmann, N., et al. (2012). Enhanced top soil carbon stocks under organic farming. Proc Natl Acad Sci U S A. 109, 18226–18231. doi: 10.1073/pnas.1209429109
Hedges, L. V., Gurevitch, J., and Curtis, P. S. (1999). The meta-analysis of response ratios in experimental ecology. Ecology 80, 1150–1156. doi: 10.1890/0012-9658(1999)080[1150:TMAORR]2.0.CO;2
Karp, D. S., Chaplin-Kramer, R., Meehan, T. D., Martin, E. A., DeClerk, F., Grab, H., et al. (2018). Crop pests and predators exhibit inconsistent responses to surrounding landscape composition. Proc Natl Acad Sci U S A. 115, E7863–E7870. doi: 10.1073/pnas.1800042115
Kennedy, C. M., Lonsdorf, E., Neel, M. C., Williams, N. M., Ricketts, T. H., Winfree, R., et al. (2013). A. global quantitative synthesis of local and landscape effects on wild bee pollinators in agroecosystems. Ecol Lett. 16, 584–599. doi: 10.1111/ele.12082
Knapp, S., and van der Heijden, M. G. A. (2018). A. global meta-analysis of yield stability in organic and conservation agriculture. Nat Commun. 9, 1–9. doi: 10.1038/s41467-018-05956-1
Kovács-Hostyánszki, A., Espíndola, A., Vanbergen, A. J., Settele, J., Kremen, C., and Dicks, L. V. (2017). Ecological intensification to mitigate impacts of conventional intensive land use on pollinators and pollination. Ecol Lett. 20, 673–689. doi: 10.1111/ele.12762
Lichtenberg, E. M., Kennedy, C. M., Kremen, C., Batáry, P., Berendse, F., Bommarco, R., et al. (2017). A. global synthesis of the effects of diversified farming systems on arthropod diversity within fields and across agricultural landscapes. Glob Chang Biol. 23, 4946–4957. doi: 10.1111/gcb.13714
Lori, M., Symnaczik, S., Mäder, P., De Deyn, G., and Gattinger, A. (2017). Organic farming enhances soil microbial abundance and activity – a meta-analysis and meta-regression. PLoS ONE 12:e0180442. doi: 10.1371/journal.pone.0180442
Mäeder, P., Fliessbach, A., Dubois, D., Gunst, L., Fried, P., and Niggli, U. (2002). Soil fertility and biodiversity in organic farming. Science 296, 1694–1697. doi: 10.1126/science.1071148
Martin, E. A., Reineking, B., Seo, B., and Steffan-Dewenter, I. (2013). Natural enemy interactions constrain pest control in complex agricultural landscapes. Proc Nat Acad Sci U.S.A. 110, 5534–5539. doi: 10.1073/pnas.1215725110
Mehrabi, Z., and Ramankutty, N. (2019). Synchronized failure of global crop production. Nat Ecol Evol. 3, 780–786. doi: 10.1038/s41559-019-0862-x
Montoya, D., Haegeman, B., Gaba, S., de Mazancourt, C., Bretagnolle, V., and Loreau, M. (2019). Trade-offs in the provisioning and stability of ecosystem services in agroecosystems. Ecol Appl. 29, 1–12. doi: 10.1002/eap.1853
Müller, C., Elliott, J., Pugh, T. A. M., Ruane, A. C., Ciais, P., Balkovic, J., et al. (2018). Global patterns of crop yield stability under additional nutrient and water inputs. PLoS ONE 13:e0198748. doi: 10.1371/journal.pone.0198748
Patterson, E. S. P., Sanderson, R. A., and Eyre, M. D. (2019). Soil tillage reduces arthropod biodiversity and has lag effects within organic and conventional crop rotations. J Appl Entomol. 143, 430–440. doi: 10.1111/jen.12603
Pimentel, D., Hepperly, P., Hanson, J., Douds, D., and Seidel, R. (2005). Environmental, energetic, and economic comparisons of organic and conventional farming systems. Bioscience 55, 573–582. doi: 10.1641/0006-3568(2005)055[0573:EEAECO]2.0.CO;2
Ponisio, L. C., M'Gonigle, L. K., Mace, K. C., Palomino, J., de Valpine, P., and Kremen, C. (2015). Diversification practices reduce organic to conventional yield gap. Proc R Soc B Biol Sci. 282:20141396. doi: 10.1098/rspb.2014.1396
Porcel, M., Andersson, G. K. S., Pålsson, J., and Tasin, M. (2018). Organic management in apple orchards: higher impacts on biological control than on pollination. J Appl Ecol. 55, 2779–2789. doi: 10.1111/1365-2664.13247
Reganold, J. P., Andrews, P. K., Reeve, J. R., Carpenter-Boggs, L., Schadt, C. W., Alldredge, J. R., et al. (2010). Fruit and soil quality of organic and conventional strawberry agroecosystems. PLoS ONE 5:e12346. doi: 10.1371/annotation/1eefd0a4-77af-4f48-98c3-2c5696ca9e7a
Reganold, J. P., and Wachter, J. M. (2016). Organic agriculture in the twenty-first century. Nat Plants. 2, 15–221. doi: 10.1038/nplants.2015.221
Schmidhuber, J., and Tubiello, F. N. (2007). Global food security under climate change. Proc Nat Acad Sci U.S.A. 104, 19703–19708. doi: 10.1073/pnas.0701976104
Schneider, M. K., Lüscher, G., Jeanneret, P., Arndorfer, M., Ammari, Y., Bailey, D., et al. (2014). Gains to species diversity in organically farmed fields are not propagated at the farm level. Nat Commun. 5, 1–9. doi: 10.1038/ncomms5151
Schrama, M., Haan, J. J., de Kroonen, M., Verstegen, H., and van der Putten, W. H. (2018). Crop yield gap and stability in organic and conventional farming systems. Agric Ecosyst Environ. 256, 123–130. doi: 10.1016/j.agee.2017.12.023
Seufert, V., and Ramankutty, N. (2017). Many shades of gray—the context-dependent performance of organic agriculture. Sci Adv. 3:e1602638. doi: 10.1126/sciadv.1602638
Seufert, V., Ramankutty, N., and Foley, J. A. (2012). Comparing the yields of organic and conventional agriculture. Nature 485, 229–232. doi: 10.1038/nature11069
Smith, R. G., Menalled, F. D., and Robertson, G. P. (2007). Temporal yield variability under conventional and alternative management systems. Agron J. 99, 1629–1634. doi: 10.2134/agronj2007.0096
Teng, M., Zeng, L., Xiao, W., Huang, Z., Zhou, Z., Yan, Z., et al. (2017). Spatial variability of soil organic carbon in Three Gorges Reservoir area, China. Sci Total Environ. 599–600, 1308–1316. doi: 10.1016/j.scitotenv.2017.05.085
Thompson, W., Lu, Y., Gerlt, S., Yang, X., Cambell, J. E., Kuepper, L. M., et al. (2018). Automatic responses of crop stocks and policies buffer climate change effects on crop markets and price volatility. Ecol Econ. 152, 98–105. doi: 10.1016/j.ecolecon.2018.04.015
Tittonell, P., and Giller, K. E. (2013). When yield gaps are poverty traps: the paradigm of ecological intensification in African smallholder agriculture. Field Crops Res. 143, 76–90. doi: 10.1016/j.fcr.2012.10.007
Tuck, S. L., Winqvist, C., Mota, F., Ahnström, J., Turnbull, L. A., and Bengtsson, J. (2014). Land-use intensity and the effects of organic farming on biodiversity: a hierarchical meta-analysis. J Appl Ecol. 51, 746–755. doi: 10.1111/1365-2664.12219
Wasserstein, R. L., and Lazar, N. A. (2016). The ASA's statement on p-values: context, process, and purpose. Am Stat. 70, 129–133. doi: 10.1080/00031305.2016.1154108
West, T. O., and Post, W. M. (2002). Soil organic carbon sequestration rates by tillage and crop rotation: a global data analysis. Soil Sci Soc Am J. 66, 1930–1946. doi: 10.2136/sssaj2002.1930
Keywords: agroecosystems, biodiversity, conventional agriculture, meta-analysis, profitability, soil carbon, stability, sustainability
Citation: Smith OM, Cohen AL, Rieser CJ, Davis AG, Taylor JM, Adesanya AW, Jones MS, Meier AR, Reganold JP, Orpet RJ, Northfield TD and Crowder DW (2019) Organic Farming Provides Reliable Environmental Benefits but Increases Variability in Crop Yields: A Global Meta-Analysis. Front. Sustain. Food Syst. 3:82. doi: 10.3389/fsufs.2019.00082
Received: 31 May 2019; Accepted: 16 September 2019;
Published: 27 September 2019.
Edited by:
Stacy Michelle Philpott, University of California, Santa Cruz, United StatesReviewed by:
Adam J. Vanbergen, INRA UMR1347 Agroécologie, FranceLauren C. Ponisio, University of California, Riverside, United States
Copyright © 2019 Smith, Cohen, Rieser, Davis, Taylor, Adesanya, Jones, Meier, Reganold, Orpet, Northfield and Crowder. This is an open-access article distributed under the terms of the Creative Commons Attribution License (CC BY). The use, distribution or reproduction in other forums is permitted, provided the original author(s) and the copyright owner(s) are credited and that the original publication in this journal is cited, in accordance with accepted academic practice. No use, distribution or reproduction is permitted which does not comply with these terms.
*Correspondence: Olivia M. Smith, olivia.m.smith@wsu.edu