- 1Department of Population Health, Poultry Diagnostic and Research Center, University of Georgia, Athens, GA, United States
- 2Center for Food Safety, College of Agricultural and Environmental Sciences, University of Georgia, Griffin, GA, United States
- 3Department of Veterinary Medicine, Universidade Federal do Parana, Curitiba, Brazil
- 4Department of Population Health, University of Georgia, Athens, GA, United States
- 5Department of Biomedical Sciences and Pathobiology, Virginia-Maryland College of Veterinary Medicine, Virginia Tech, Blacksburg, VA, United States
The role of invasive Salmonella in contamination of ground poultry is poorly defined. Salmonella harborage sites were determined in experimentally infected chickens and turkeys. Bioluminescent-tagged Salmonella were used to follow their spread in bone, meat, and skin following infection. Immunohistochemistry and culture were used to localize Salmonella. Chicken neck skin was positive for S. Heidelberg and S. Typhimurium throughout the experimental period, and the bacteria were localized on the stratum corneum of the epidermis and feather follicles. S. Heidelberg and S. Typhimurium were intermittently detected in drumstick muscle of chickens, with Salmonella primarily localized in connective tissues and lymphatics. Twenty percent of the drumstick muscles were culture positive for Salmonella in chickens at 42 days of age. Blood and tibiotarsus bone were culture positive for S. Heidelberg and S. Typhimurium during the first 2 weeks of infection. Salmonella levels in neck skin and muscle were <102 CFU/g in chickens at 42 days of age. Multiple S. Heidelberg isolates associated with foodborne outbreaks were used to infect chickens and turkeys to determine whether some strains attained high abundance in the muscle of infected birds. No chicken drumsticks or thighs were Salmonella positive by bioluminescence in chickens at 42 days of age (n = 210). In turkeys, all drumstick muscles (n = 132) and tibiotarsus bone (n = 93) were negative for S. Heidelberg. Thirty percent of the breast skins (n = 93) were culture positive for S. Heidelberg when turkey hens were 11 weeks old. S. Heidelberg were primarily localized on the stratum corneum of the epidermis in turkeys. Exclusion of skin from ground poultry products may be the best option for reducing Salmonella contamination in ground chicken and ground turkey.
Introduction
It is estimated that Salmonella causes 1 million illnesses and 380 deaths per year in the U.S and the cost associated with foodborne salmonellosis is estimated in excess of $2.7 billion annually (CDC., 2014b). S. Typhimurium and S. Heidelberg are among the top 10 Salmonella serovars associated with human cases. Poultry products are frequently implicated as the source of human Salmonella infections. Multidrug resistant S. Heidelberg have been implicated in several multistate foodborne outbreaks linked to contaminated chicken and ground turkey products in recent years (CDC., 2011, 2014a). A ground turkey outbreak, associated with S. Heidelberg, caused a total of 136 cases, with one reported death and resulted in a recall of ~36 million pounds of ground turkey products (CDC., 2011). Healthy asymptomatic birds are known to carry Salmonella and cross-contamination between carcasses occurs in different steps of the processing line (Rigney et al., 2004; Nde et al., 2007; Erol et al., 2013). Although intensive measures have been implemented to reduce surface contamination in poultry carcasses during processing, the United States Department of Agriculture Food Safety and Inspection Service reported 18% prevalence of Salmonella contamination in ground chicken for 2013 (USDA-FSIS, 2014).
In birds, Salmonella infection and disease are dependent on several factors including age, immune status of the host, genetic susceptibility, environmental factors and stress (Barrow et al., 1987; Gast, 2013). Later in infection in birds, Salmonella colonizes the ceca where it is shed in feces for several weeks to months (Phillips and Opitz, 1995; Gast and Holt, 1998; Gast, 2013). Salmonella is transmitted to birds and other animals via the fecal-oral route. Following passage through the crop and stomach, Salmonella moves through the intestinal mucin layer where it attaches to epithelial cells and invades. After transcytosis through the epithelial cell, Salmonella are taken up by sub-epithelial dendritic cells and macrophages and transported systemically via the bloodstream and lymphatic system to different sites, including the liver, spleen and bone marrow (Chappell et al., 2009; Mastroeni and Grant, 2011). Free Salmonella cells in the blood are generally opsonized by the complement and lysed. Therefore, systemic spread of Salmonella is dependent on infected macrophages and dendritic cells (Swart and Hensel, 2012). Salmonella enhanced survival in phagocytes is provided by genes located on pathogenicity island 2; a type III secretion system that inhibits lysosome fusion and modulates major histocompatibility complex (MHC) and cytokine expression (Ochman et al., 1996; Cheminay et al., 2005).
The role of systemic infection and its impact on the prevalence and numbers of Salmonella in ground poultry components are poorly understood. There is limited published information on the presence of internalized Salmonella in ground poultry tissues. Ground poultry contains bone-in and boneless parts, such as drumsticks, thighs, neck and wings and recent studies have reported the potential of internalized Salmonella in bone as one possible source for contamination in these products (Wu et al., 2014; Cui et al., 2015). In these studies, Salmonella prevalence was reported at 0.8 and 9.3% in chicken and turkey bone samples, respectively (Wu et al., 2014; Cui et al., 2015).
Published studies evaluating Salmonella infection and invasiveness have relied primarily on standard microbiological methods for detection or enumeration. Bioluminescence imaging is a tool that can be used in real-time to reveal the presence of bacterial cells in living animals, tissues and environment, enabling tracking of the pathogen over time. Bacteria tagged with chromosomal integration of the Photorrhabdus luminescens lux operon can constitutively produce visible light (Engebrecht and Silverman, 1984; Meighen and Dunlap, 1993). The bioluminescent marker has been used to tag and trace important foodborne pathogens including Campylobacter jejuni, Campylobacter coli, Salmonella Typhimurium, and Escherichia coli O157:H7 (Ritchie et al., 2003; Burns-Guydish et al., 2005; Kassem et al., 2010). Two studies have reported the use of bioluminescence imaging to evaluate survival of Campylobacter species in chicken litter and Salmonella attachment to skin samples (Howe et al., 2010; Kassem et al., 2010). A recent study reported the use of bioluminescence imaging to assess the efficacy of medium chain fatty acid feed supplementation for reducing intestinal colonization of lux-tagged S. Typhimurium in turkey poults (Evans et al., 2017).
In this study, bioluminescence imaging, culture, and immunohistochemistry were used to localize Salmonella isolates in skin, skeletal muscle, and bone of chickens and turkeys in order to reveal the contribution of these sites to contamination of ground poultry meat.
Materials and Methods
Bacterial Strains
The constitutively bioluminescent S. Typhimurium SL1344 Tn5-lux was obtained from Dr. Christopher H. Contag (Stanford University, Stanford, CA). Mini-Tn5-luxCDABE was also introduced into S. Heidelberg SH380 and 14 additional S. Heidelberg poultry/foodborne outbreak isolates (representing multiple PFGE strains) by generalized transduction with P22HTint transducing phage (Burns-Guydish et al., 2005). The transposon was engineered to constitutively express the kanamycin resistance gene and the luxCDABE operon, the latter making the transduced Salmonella cells strongly luminescent regardless of growth conditions (Burns-Guydish et al., 2005). Salmonella strains were streaked on MacConkey agar with kanamycin (50 μg/mL) and incubated at 37°C for 24 h. For each strain, a single bioluminescent colony was collected and grown static in 5 mL Luria-Bertani broth at 37°C for 16 h, after which the bacterial cell density was estimated from the optical density (OD)600 for the cell suspension. Following overnight incubation, bacterial cells were pelleted (5,000 × g, 10 min) and the cell pellet was suspended in equal volume of buffered saline gelatin (BSG) (Burns-Guydish et al., 2005). The final bacterial count was confirmed by plating 10-fold serial dilutions from the inoculum on MacConkey agar with kanamycin (50 μg/mL). After 24 h incubation at 37°C, colony forming units (CFU) were determined.
Ethics Statement
The University of Georgia's, Institutional Animal Care and Use Committee approved all animal care protocols described in this work.
Animal Studies
In experiment 1, 1-day-old, specific-pathogen-free (SPF) white leghorn chickens were housed in biosafety level 2 (BSL-2) wire-bottom Horsfall isolator units at the Poultry Diagnostic and Research Center, College of Veterinary Medicine, University of Georgia (Athens, GA). Water and an un-medicated corn-soy diet were provided ad libitum. Vaccinations were not performed. One hundred and twenty-one, 1-day-old SPF chicks were randomly divided into two treatment groups and one control group. Fifty-four chicks were randomly placed in 4 Horsfall isolator units with 13–14 chicks per unit, per group, and orally administered 1 × 108 CFU/0.1 ml of Salmonella Heidelberg SH380-lux or S. Typhimurium SL1344-lux. Thirteen chicks were placed in one Horsfall isolator unit and sham-inoculated with 0.1 ml of BSG. The chicks received the inoculum right before their placement in isolators. Oral inoculations were performed using a pipette tip carefully placed in the mouth of the birds. Birds were evaluated twice a day for clinical signs and mortality. Five birds from each treatment group and one bird from the sham-inoculated group were euthanized by carbon dioxide and exsanguinated on days 2, 5, 7, 14, 21, 28, 35, and 42 post-administration. Blood samples were aseptically collected through the femoral vein and placed into heparinized tubes. Samples of neck skin were also aseptically collected for bacteriology and immunohistochemistry. The body of the chickens was then disinfected by spraying with 70% alcohol. Samples of skeletal muscle (drumstick) with lymphatics adjacent to the posterior tibial vein, bone (tibiotarsus), liver, spleen and ceca were aseptically removed from sham-inoculated and treated birds for bioluminescence imaging, culture and Salmonella immunohistochemistry. The tibiotarsus samples were disinfected in 70% alcohol prior to sectioning with sterile tools.
In experiment 2, the ability of multiple Salmonella Heidelberg-lux isolates (n = 15) to seed drumsticks and thighs at 42 days post-oral administration was evaluated using bioluminescence imaging. Two hundred and seventy one-days-old, SPF chickens were housed in BSL-2 colony houses at the Poultry Diagnostic and Research Center, College of Veterinary Medicine, University of Georgia (Athens, GA). Chickens were placed on pine shavings litter and brooded following standard temperature regimens. Water and an un-medicated corn-soy diet were provided ad libitum. Vaccinations were not performed. The chickens were divided into 3 groups (groups A, B, and C) with 90 birds each and orally inoculated with a pooled, Salmonella cocktail containing five S. Heidelberg isolates at 1 day of age (oral dose: 4 × 108 CFU/0.1 ml). Fifteen isolates were used to make three cocktails. Oral inoculations were performed using a pipette tip carefully placed in the mouth of the birds. Birds were evaluated twice a day for clinical signs and mortality. Ten birds in groups A and C and five birds in group B were euthanized at 5 weeks post-inoculation and all remaining birds were euthanized at 6 weeks of age. Legs with thigh and drumstick were examined from 210 birds for bioluminescence. This sample size is expected to detect 1% prevalence with 95% confidence.
In experiment 3, 1-day-old turkey hens (Nicholas) were obtained from a commercial breeder company and placed in four colony houses at the Poultry Diagnostic and Research Center, College of Veterinary Medicine, University of Georgia (Athens, GA). Parent flocks had been vaccinated at 15 and 25 weeks of age with an autogenous Salmonella Hadar, killed-vaccine. The progeny received a single dose of gentamicin at the hatchery. Fecal samples and chick paper were tested prior to inoculation and confirmed that the day-old birds were Salmonella-free. One hundred and thirty-three, 1-day-old turkey poults were reared in 3 colony houses (stocking density ranged from 1.2 to 1.8 ft2/bird until week 7; and from 1.9 to 2.1 ft2/bird between weeks 7 and 11). Turkey poults were placed on pine shavings litter and brooded following standard temperature regimens. All birds were given access to water and feed containing coccidiostat (Amprolium 125 ppm) ad libitum. Vaccinations were not performed. One-day-old turkey poults were orally inoculated with a 0.1 mL inoculum containing 4.4 × 107 CFU of the pooled, bioluminescent Salmonella strains (SH380-lux, SH038-lux, SH682-lux, SH198-lux, and SH-890-lux). Twenty, uninfected control birds were placed in a fourth colony house and orally inoculated with 0.1 mL sterile BSG. Oral inoculations were performed using a pipette tip carefully placed in the mouth of the birds. The turkeys were evaluated twice a day for clinical signs and mortality. Thirty-nine birds from the Salmonella Heidelberg-challenged group and six birds from the control group were euthanized by carbon dioxide followed by cervical dislocation between 6 and 7 weeks of age to reduce bird density in the colony houses. The legs of the turkeys were disinfected by dipping the half lower part of the carcass in a 0.08% sodium hypochlorite solution for 5 min. Drumstick muscle samples with lymphatics were aseptically collected for bioluminescence imaging and Salmonella culture. At 11 weeks of age, all remaining 93 birds from the Salmonella Heidelberg-challenged group and 14 birds from the control group were euthanized by carbon dioxide followed by jugular exsanguination. Feathered breast skin was aseptically collected for Salmonella culture and immunohistochemistry and the half lower part of the carcass (legs and hip) was disinfected by soaking it in a 0.08% sodium hypochlorite solution for 5 min. Drumstick muscle samples were aseptically collected for bioluminescence imaging, immunohistochemistry and Salmonella culture. Drumsticks were aseptically collected from the opposite leg and dissected to remove muscle and cartilage from the bone (tibiotarsus). Decontamination of the tibiotarsus surface with 70% ethanol solution for 5 min was performed prior to bacteriology in bone marrow samples. Cecal droppings present on litter were collected from all 4 colony houses from weeks 2 to 10 post-inoculation for estimation of Salmonella prevalence and abundance. All animal experiments were conducted under strict adherence to Institutional Animal Care and Use Committee (IACUC) guidelines at the University of Georgia.
Salmonella Detection and Enumeration by Culture
In experiment 1, all tissues were cultured; in experiment 2, only luminescent tissues were cultured. Tissues were weighed, placed in sterile Nasco Whirl-PAK bags (Nasco; Fort Atkinson, WI), and an equal volume to weight of BSG was added to each sample. Tissues were homogenized with a stomacher (Tekmar Co.; Cincinnati, OH) for 1 min. Tissue homogenate was serially diluted 10-fold in BSG and plated on MacConkey agar plates with kanamycin (50 μg/ml). Plates were incubated for 24 h at 37°C, lactose-negative colonies were enumerated, and CFU/g tissue was determined. Bacterial enumeration was not performed for bone samples. Samples of blood, tibiotarsus, neck skin, skeletal muscle, and ceca were also enriched in tetrathionate brilliant green (TBG) broth for 24 h at 41°C and plated on MacConkey agar plates with kanamycin. Growth of lactose-negative colonies on MacConkey agar was recorded as positive for Salmonella. Isolation of lux-tagged Salmonella was confirmed upon visual inspection of plates for bioluminescent colonies.
In experiment 3, tissues were placed in sterile Nasco Whirl-PAK bags (Nasco; Fort Atkinson, WI) and 10 mL of tetrathionate brilliant green (TBG) broth with iodine was added to each sample. Muscle with lymphatics and skin samples were homogenized with a stomacher (Stomacher80 Seward; England) for 1 min. Bone samples were gently mixed for ~1 min. Tissue homogenates were then incubated at 42°C for 24 h. A loopful of TBG enrichment (1 μL) was streaked onto MacConkey agar plates with kanamycin (50 μg/mL). Cecal droppings were placed in sterile 50 mL, conical centrifuge tubes (Thermo Scientific; Rochester, NY), weighed, and an equal volume to weight of BSG was added to each cecal sample. Samples were vortexed thoroughly, serially diluted 10-fold in BSG (final dilution 10−7) for enumeration on MacConkey agar plates with kanamycin (50 μg/mL). Plates were incubated at 37°C for 24 h and bioluminescent Salmonella were detected and enumerated (CFU/g cecal droppings) by bioluminescence imaging. Enrichment was performed to detect low levels of Salmonella in cecal samples. Briefly, 10 mL of TBG broth with iodine were added to cecal samples followed by incubation at 37°C for 24 h. A loopful of the TBG enrichment was streaked onto MacConkey agar plates with kanamycin (50 μg/mL). Plates were incubated at 37°C for 24 h and bioluminescent Salmonella were detected by bioluminescence imaging.
Bioluminescence Imaging
The presence of bioluminescent Salmonella in tissues was monitored using an IVIS Lumina XR imaging system (Perkin Elmer; Greenville, SC) as previously described (Burns-Guydish et al., 2005; Özkaya et al., 2012). The IVIS Lumina XR imaging system uses a sensitive charge-coupled device (CCD) camera for detecting luminescence in infected animals or tissues. Three to five-minutes images of light (emitted photons) transmitted through the tissues were taken in the dark, and a pseudocolor representation of light intensity (red, most intense; blue, least intense) was overlaid over the grayscale image of the tissue surface. Regions of interest, of the same circular circumference, were drawn around tissues examined and the luminescence signal intensity in photons/sec/cm2/sr was measured by the Living Imaging Software (Perkin Elmer; Greenville, SC). In order to determine the range of detection for the instrument, different concentrations of Salmonella-lux (101 to 107 CFU/g) were injected into drumsticks of chickens. Tissues from uninfected chickens yielded a mean background luminescence of log10 2.35 (Range: log10 2.09–2.82). In addition, because the cecum exhibits the greatest range of Salmonella abundance (102-109 CFU/g) log10 Salmonella numbers were plotted against luminescence.
Immunohistochemistry
Tissue samples were placed in 10% buffered formalin, embedded in paraffin, and sectioned at 4 μm. Tissue sections were deparaffinized in xylene and hydrated in decreasing alcohol solutions. Antigen retrieval was performed in citrate buffer at pH 6.0 with the use of a steamer. Sections were washed with distilled water and the endogenous peroxidase activity was blocked using a 3% H2O2 (or Bloxall) solution for 10 min. After 10 min incubation, the sections were washed with PBS and incubated for 1 h at room temperature with the primary antibody Rabbit anti-Salmonella O serogroup B (BD; Franklin Lakes, NJ) at 1:500 antibody concentrations. The sections were washed with PBS to remove any unbound primary antibody and then treated with Protein Block solution (Dako Agilent Pathology Solutions; Carpinteria, CA) for 10 min. After 10 min incubation, the sections were washed with PBS and then incubated for 1 h with the secondary antibody Rabbit-on-Farma horseradish peroxidase polymer (Biocare Medical; Concord, CA). After washing steps, the slides were stained for 10 min with 3,3′-diaminobenzidine (Vector Labs; Burlingame, CA) and counterstained with Mayer's hematoxylin. Sections of intestine from chickens infected with S. Typhimurium from a previous study were used as positive control samples. Sections of intestine incubated with rabbit antiserum instead of primary antibodies were used as negative controls. The slides were examined using a bright field microscope.
Statistical Analyses
Bacterial numbers were logarithmically transformed before statistical analysis. Before log-transformation, the constant 1 was attributed to culture-negative samples and the constant 50 was attributed to samples positive for Salmonella only upon enrichment. The Mann-Whitney test was performed to determine significant differences in log10-tranformed CFU data for tissues in chickens infected with either lux-tagged S. Heidelberg or S. Typhimurium strains (p < 0.05). Linear regression was used to determine correlation between log10 transformed data for Salmonella abundance (CFU/g) vs. luminescence (Figure 3).
Results
Identification of Harborage Sites in Salmonella-Infected Chickens
Culture and bioluminescence revealed that Salmonella colonized the ceca for the 42-days sampling period following oral administration. Salmonella abundance in the ceca was highest during the first week (108-109 CFU/g) (Figure 1) and decreased 2–4 log10 on subsequent weeks until day 42, when cecal abundances were <102 (S. Typhimurium) and 104 (S. Heidelberg) CFU/gram ceca (Table 1). Neck skin, tibiotarsus, drumstick muscle and blood were also screened for Salmonella by culture and bioluminescence imaging. Neck skins were consistently positive for Salmonella for the 42-days sampling period; 2/5 (S. Heidelberg) and 1/5 (S. Typhimurium) skin samples were culture positive by enrichment (Salmonella abundance <102 CFU/g) at day 42. Mean Salmonella abundance was highest within neck skin in 3-days-old birds, at log10 3.5 S. Heidelberg (range: log10 <2.0–4.4) and log10 4.5 S. Typhimurium (range: log10 2.0–6.2). Salmonella was detected in internal tissues (muscle, bone, blood, liver and spleen) during the first 2 weeks of infection, then intermittently in bone and muscle at later times. Salmonella abundance in muscle tissue was low, often present at levels <102 CFU/g and detected only by enrichment.
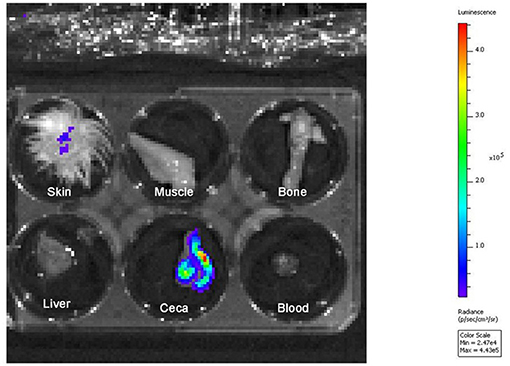
Figure 1. Bioluminescence imaging in tissues of a 2-days-old chicken experimentally infected with S. Typhimurium-lux. Pseudocolor overlays represent the amount of bioluminescence produced by S. Typhimurium-lux in skin and ceca. The color scale indicates the amount of bioluminescence (surface radiance: p/s/cm2/sr) detected during imaging.
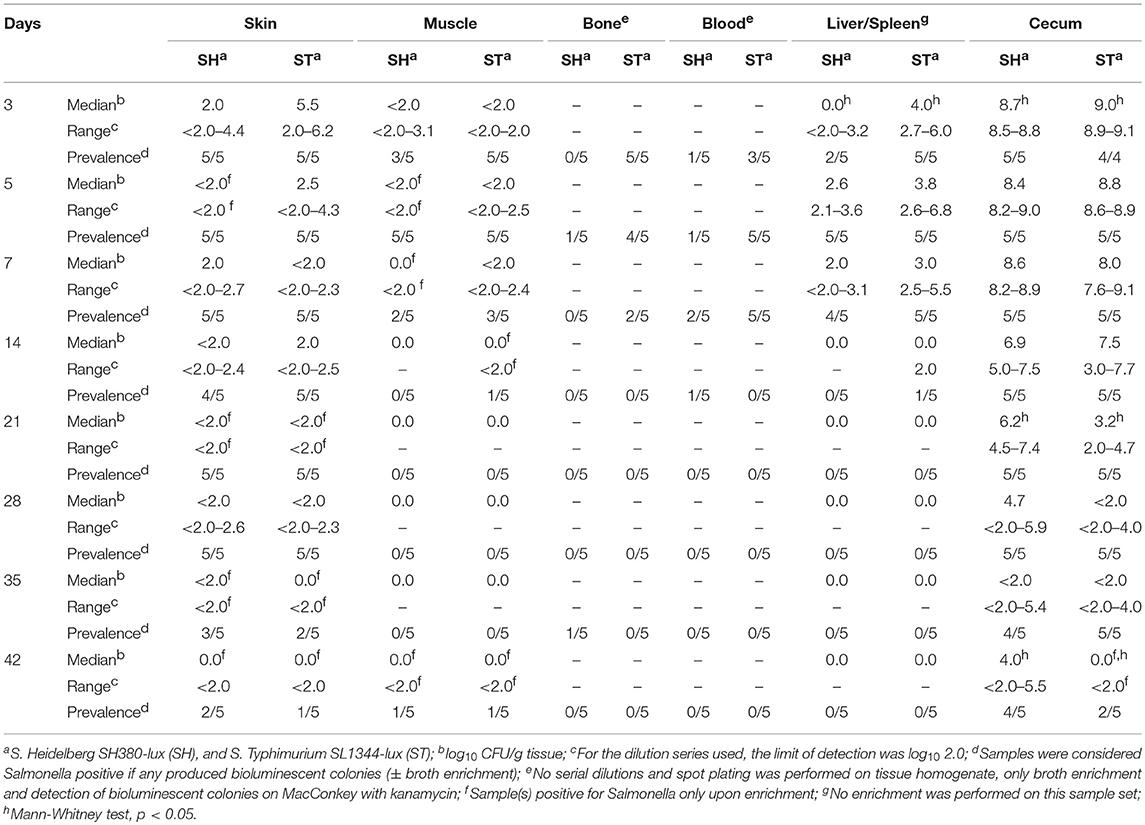
Table 1. Salmonella prevalence and abundance in chickens infected with S. Heidelberg or S. Typhimurium.
Significant differences in the abundance of Salmonella serotypes were observed in the ceca in a few time points; S. Typhimurium had higher counts in the ceca on day 3, and lower counts on days 21 and 42, compared to S. Heidelberg.
Among tissues that are included in ground meat, neck skin exhibited the highest prevalence for Salmonella. Immunohistochemistry revealed small to moderate numbers of Salmonella O serogroup B-positive bacterial cells individually or in clusters in the stratum corneum of the epidermis; mainly within skin folds, and occasionally present in the stratum corneum of the feather follicles (Figures 2A,B; Supplementary Table 1). Salmonella O serogroup B-positive bacterial cells were also seen in the dermis during the first 2 weeks of infection, most frequently observed in the connective tissue, and infrequently in the lymphatics. Small clusters of bacterial cells were also observed in skeletal muscle samples throughout the study. The bacteria were most frequently observed in the connective tissue of endomysium and perimysium, admixed with collagen fibers. Occasionally a few Salmonella cells were found in the cytoplasm of cells resembling fibroblasts (Figure 2C; Supplementary Table 1), present in muscle tissue, during the first week. There were also small numbers of Salmonella O serogroup B-positive bacterial cells within the lymphatics, free in the lumen (Figure 2D; Supplementary Table 1) or in the cytoplasm of cells resembling monocytes (Figure 2E) in some samples at different time points including at 42 days of age. Salmonella were not detected in microscopic lymphoid nodules. A few bone samples had small numbers of Salmonella O serogroup B-positive cells free in the bone marrow (Figure 2F; Supplementary Table 1) and Salmonella were occasionally observed in the lumen of physeal vessels during the first 2 weeks post-inoculation. Liver and spleen samples were positive for Salmonella by immunostaining throughout the study period, and staining was predominantly observed within the cytoplasm of Kupffer cells and macrophages in the paratyphoid nodules (data not shown). All samples from sham-inoculated chickens were negative for Salmonella by immunohistochemistry and culture.
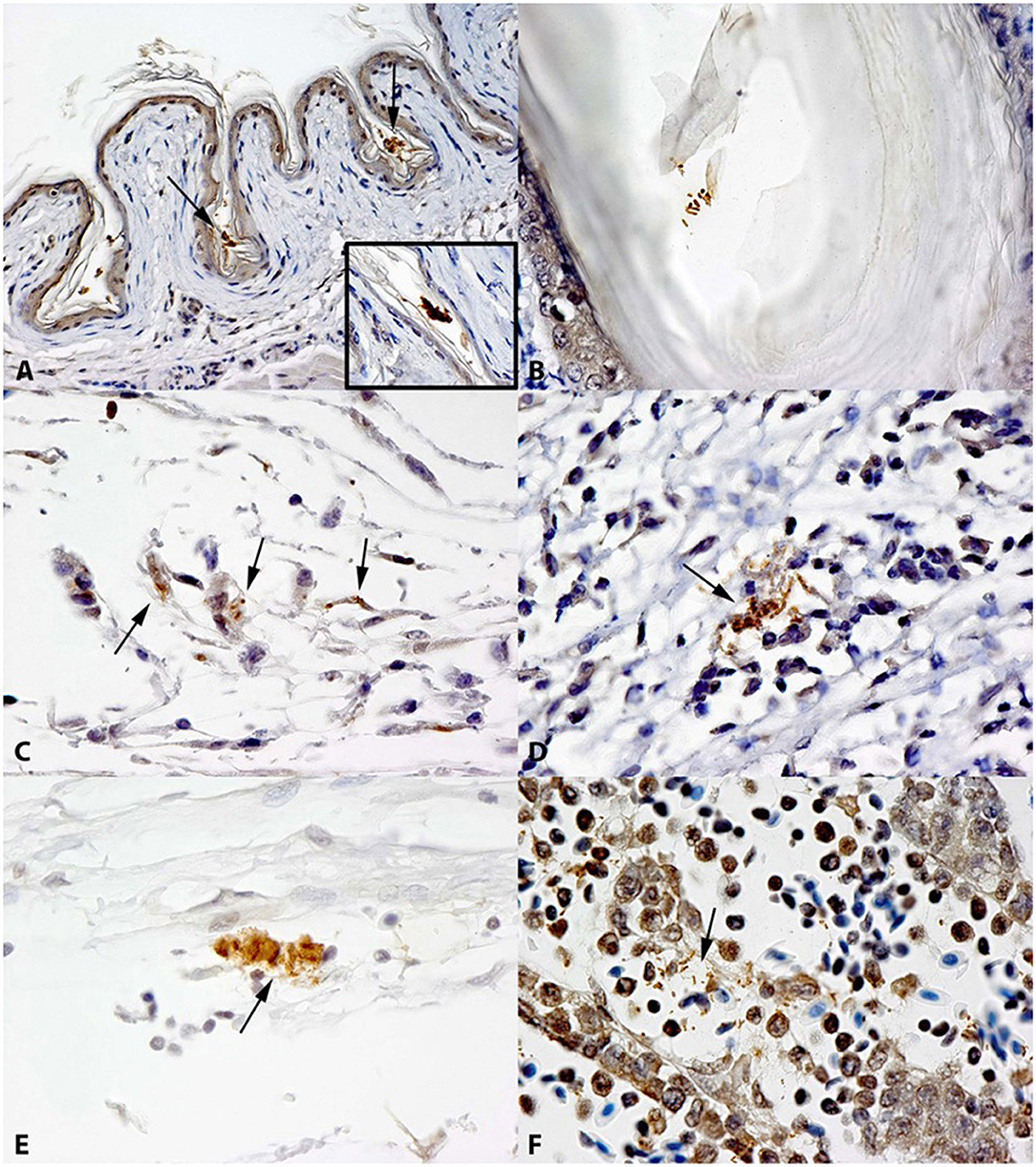
Figure 2. Immunohistochemical localization of Salmonella in tissues of experimentally infected chickens. (A) Clusters of Salmonella O serogroup B-positive bacteria on epidermal keratin (arrows) in a chicken infected with S. Heidelberg 380-lux. (B) Salmonella O serogroup B-positive bacteria on the stratum corneum of the feather follicle in a chicken infected with S. Heidelberg 380-lux. (C) Salmonella O serogroup B-positive bacteria in the cytoplasm of cells resembling fibroblasts (arrows) in the muscle from a chicken infected with S. Typhimurium SL1344-lux. (D) Salmonella O serogroup B-positive bacteria in the lumen of a lymphatic vessel (arrow) in the muscle of a chicken infected with S. Typhimurium SL1344-lux. (E) Salmonella O serogroup B-positive bacteria in the cytoplasm of cells resembling monocytes (arrow) in lymphatic vessel in the muscle of a chicken infected with S. Heidelberg 380-lux. (F) Salmonella O serogroup B-positive bacteria in the bone marrow (arrow) in a chicken infected with S. Typhimurium SL1344-lux.
Does High Salmonella Abundance in Muscle Tissue Contribute to Contamination of Ground Chicken?
Bioluminescence imaging was used to assess bacterial numbers in tissues during natural infection. Tissues from uninfected chickens were used to set baseline detection for the bioimaging system and Salmonella numbers were plotted against luminescence, log10, for the cecum. Bioluminescence imaging was considered positive in samples with signal intensity above log10 2.82 luminesce. The ceca were chosen for analysis because this tissue had the greatest range of Salmonella abundance (102-109 CFU/g). There was a non-linear relationship between CFU and luminescence in ceca (r2 = 0.805) (Figure 3). Bioluminescence imaging was able to reliably detect Salmonella concentration >107 Salmonella cells/g in injected drumsticks (Supplementary Figure 1) and ceca, with 97.1% sensitivity detected in ceca. Sensitivity of bioluminescence imaging for Salmonella concentrations in ceca between 106-107/g and between 105-106/g was 40 and 28.6%, respectively. In experiment 2, bioluminescence imaging was used to assess the prevalence of high Salmonella abundance in muscle of birds administered a cocktail of S. Heidelberg isolates cultured from commercial poultry or from actual foodborne outbreaks. Because of strain variability in infection dynamics, we sought to determine whether the outbreak isolates were more likely to reach high levels in the muscle of birds. At 42 days of age, chicken legs with drumstick and thigh were screened for bioluminescence. None of the 210 samples examined produced any signal above log10 2.82 luminesce, the threshold for detecting bioluminescent Salmonella. This data indicates that the prevalence of high pathogen levels in the muscle is <1% based on a power estimate.
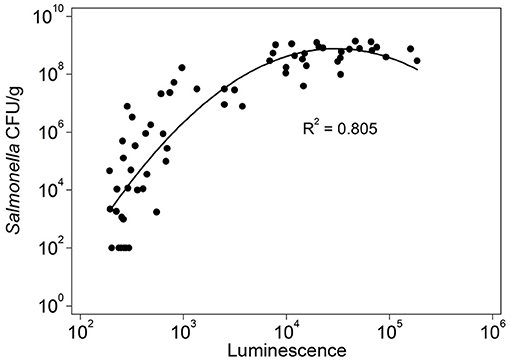
Figure 3. Correlation between luminescence and Salmonella cell numbers in the cecum of chicken experimentally infected with S. Heidelberg-lux or S. Typhimurium-lux strains. Salmonella cell numbers (log10) in the cecum was plotted against luminescence (log10) detected using the IVIS bioluminescence imaging system. Background luminescence was set at the upper range of signal detected (luminescence log10 2.82) in tissues from un-inoculated controls. A positive correlation (r2 = 0.805) was observed between luminescence (log10 photons/sec/cm2/sr) and cell density (log10 CFU/g).
Identification of Harborage Sites in Salmonella-Infected Turkeys
All muscle samples collected between 6 and 7 weeks of age and at 11 weeks of age (n = 132) were negative for S. Heidelberg by bioluminescence imaging and culture. Similarly, all tibiotarsus bone samples (n = 93) were Salmonella negative at 11 weeks of age. Only breast skin samples were S. Heidelberg positive, with 30.1% prevalence (Table 2). Immunohistochemistry revealed Salmonella O serogroup B-positive bacterial cells localized on epidermal keratin in all 28 skin samples which were positive by bacteriology (Figure 4B). Bacteria cells were mostly organized in clusters. High numbers of Salmonella cells were observed entrapped within skin folds (Figure 4C). Salmonella O serogroup B-positive bacterial cells were also observed within feather follicles in 10.7% of skin samples culture positive for Salmonella (Figure 4D). Interestingly, Salmonella O serogroup B-positive bacterial cells were also observed within epidermal ulcers on breast skin samples (breast button) in two birds at 11 weeks of age; these bacteria cells were surrounded by necrotic material within a serocellular crust (Figure 4E). In one of these two birds, Salmonella O serogroup B-positive bacterial cells were also observed within a dermal blood vessel, as free cells and in the cytoplasm of monocytes (Figure 4F).
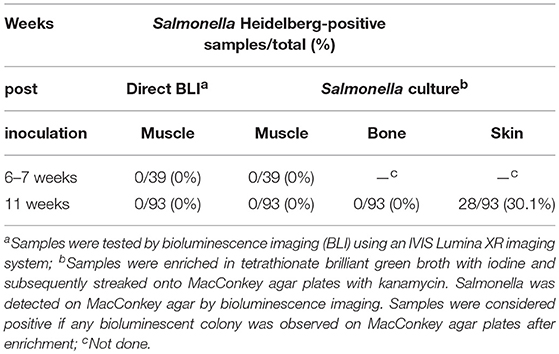
Table 2. Salmonella prevalence in muscle, bone, and skin of turkeys infected with multiple S. Heidelberg isolates.
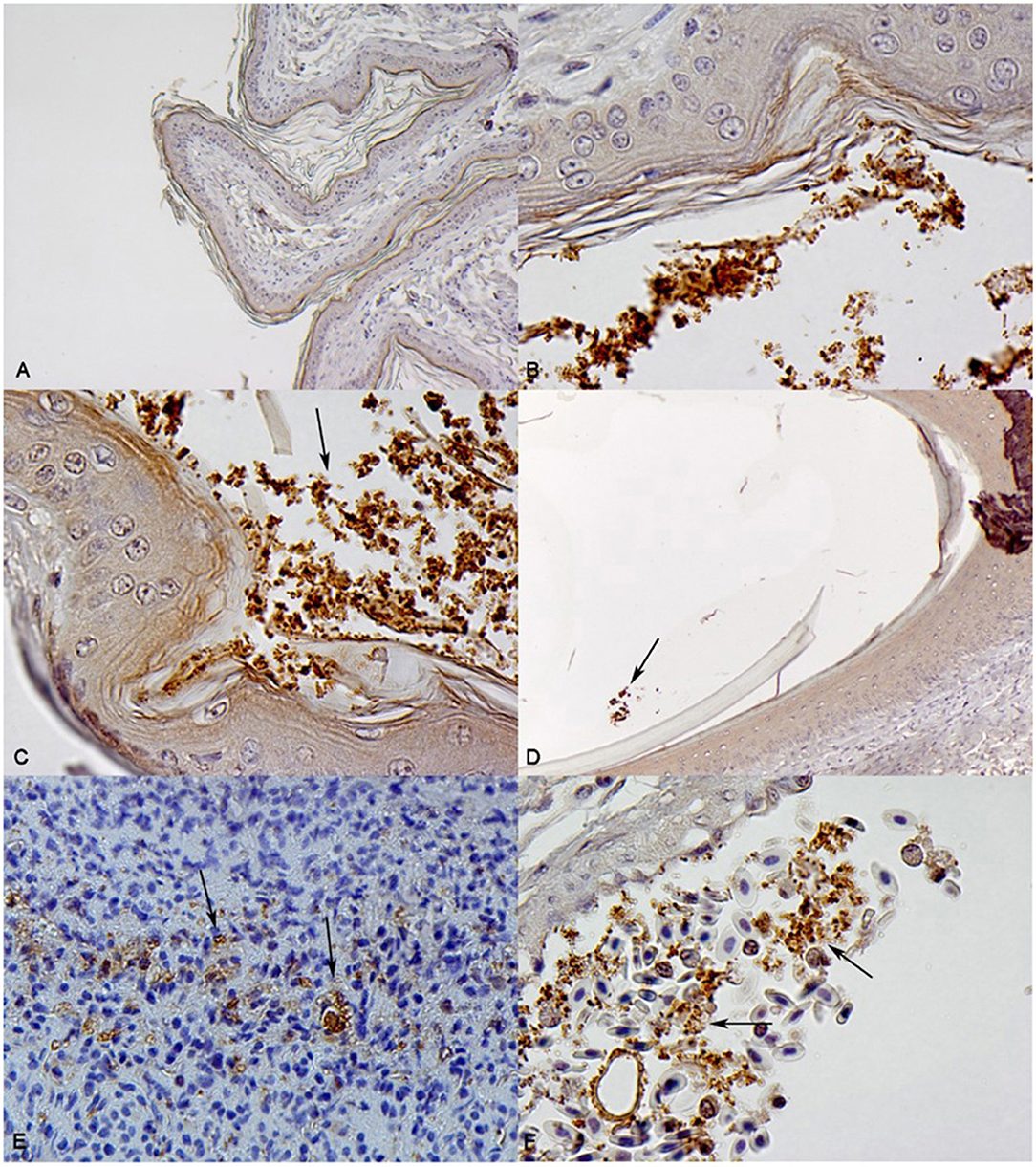
Figure 4. Immunohistochemical localization of Salmonella Heidelberg in skin samples from 11-weeks-old turkeys orally inoculated with S. Heidelberg-lux strains at day of age. (A) Sham-inoculated group, integument and epidermal keratin. Absence of Salmonella O serogroup B-positive bacteria (B) Salmonella O serogroup B-positive bacteria cells in clusters on epidermal keratin. (C) Salmonella O serogroup B-positive bacteria cells in clusters on epidermal keratin and within a fold in the skin (arrow). (D) Salmonella O serogroup B-positive bacteria cells within a feather follicle (arrow). (E) Salmonella O serogroup B-positive bacteria (arrows) within serocellular crust (scab) of an ulcerated skin sample. (F) Salmonella O serogroup B-positive bacteria cells within a blood vessel, free or in cytoplasm of monocyte-like cells (arrows).
The prevalence of S. Heidelberg in cecal droppings was 100% between weeks 2 and 4 post-inoculation. S. Heidelberg abundance in cecal droppings was highest in week 3 post-inoculation (4.4 log10 CFU/g). Salmonella counts subsequently decreased thereafter, with mean Salmonella counts of 1.3 log10 CFU/g at 10 weeks of age (Figure 5).
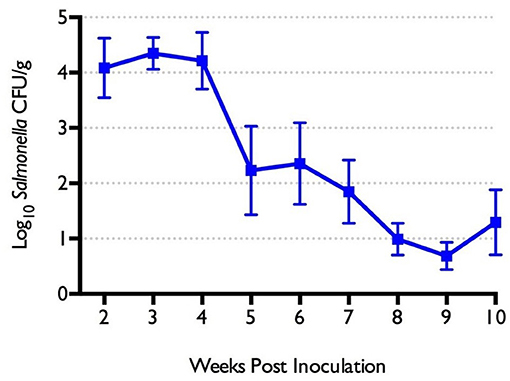
Figure 5. Salmonella cecal shedding (log10 CFU/g) in turkeys experimentally infected with S. Heidelberg-lux at 1 day of age.
Discussion
Bone-in and boneless meat from poultry parts, such as drumsticks and thighs, generally go into making ground poultry meat (FSIS, 2013). Skin in natural proportions is often included for its fat content (FSIS, 2013); however the amount of bone in the final ground product is regulated and only 130 mg calcium per 100 g of product is allowed (FSIS, 2015). For this reason, we examined Salmonella prevalence and abundance in poultry muscle, bone, and skin from experimentally infected chickens and turkeys. Chicken skins and ceca were consistently positive for S. Typhimurium and S. Heidelberg in infected birds up to 42 days. This indicates that fecal Salmonella shedding resulted in contamination of chicken skin. Twenty percent of skin samples were culture positive at 42 days which is similar to prevalence reported from processed, chicken carcasses (Wu et al., 2014). Muscle was also culture positive for Salmonella with low numbers (<102 CFU/g) in one out of five SPF chickens at 42 days of age, with a few Salmonella cells detected within connective tissue and in the lumen of lymphatics by immunohistochemistry. Thus, it appears that high Salmonella prevalence in ground poultry is likely due to one of two possible scenarios: 1) high Salmonella prevalence but few bacteria in component(s) (skin, meat, or bone) that go into ground product; or 2) low Salmonella prevalence but high cell numbers in contaminated component(s). In this study, chicken skin contained low numbers of Salmonella but at high prevalence. Moreover, Salmonella levels in muscle and skin were low (<102 CFU/g). The latter scenario is less likely as high Salmonella levels in skeletal muscle of infected chickens occurred at a low prevalence. Furthermore, high Salmonella Heidelberg prevalence in skin of infected turkeys, rather than internalized in muscle and bone, would more significantly contribute to contamination of ground turkey as 30.1% of the turkey breast skin samples were culture-positive in 11-weeks-old birds, while all muscle and bone samples were culture-negative.
In this study, bioluminescence imaging, culture, and immunohistochemistry were used to identify specific tissues sites colonized by Salmonella. Bioluminescence imaging allowed visualization of Salmonella, but the sensitivity of this method was best for high levels (>106 CFU/g). Culture using enrichment remains the most sensitive method to monitor Salmonella infection and spread in vertebrates. Immunohistochemistry was effective for identifying specific sites of Salmonella localization and in chicken and turkey skin, Salmonella cells were organized in clusters on the epidermal keratin and were occasionally present in the lumen of feather follicles. Studies of skin that was inoculated with Salmonella have reported bacterial cells present on the flat areas and ridges of the skin, and entrapped in feather follicles (Kim et al., 1996; Lee et al., 2014). This study is the first that describes Salmonella cells within the lumen of feather follicles of colonized poultry. Salmonella were often observed as aggregate of cells in skin tissue, a cellular arrangement characteristic of curli-producing bacteria (Maurer et al., 1998). Curli are aggregative, flexible fimbriae (Maurer et al., 1998) that bind fibronectin; a host protein prevalent in many tissues including skin (Olsén et al., 1993). Salmonella optimally express curli when grown at ambient room temperature (Maurer et al., 1998) or starved for nutrients in the chicken cecum (Cheng et al., 2015). Flagella may also be involved in attachment of Salmonella on chicken skin surfaces (Salehi et al., 2017). Expression of these adhesive structures depends on the genetic background of the isolate as well as environmental factors, such as temperature, oxygen, and nutrient availability (Collinson et al., 1993; Maurer et al., 1998; Gerstel and Römling, 2001). Ambient temperature may contribute to thin aggregative fimbriae formation and keratinized epithelium may select for cells expressing these adhesins resulting in bacterial aggregates on poultry skin (Collinson et al., 1993; Maurer et al., 1998; Gerstel and Römling, 2001).
USDA-approved chemicals for the processing plant can reduce Salmonella loads in scalding and chilling baths, but they are not as efficient in eliminating Salmonella entrapped into the skin (Lillard, 1989; Yang et al., 2001; Lee et al., 2014). Unfortunately, Salmonella cells lodged in crevices and within feather follicles are protected from rinses and chemical treatments (Lillard, 1989; Kim et al., 1996; Lee et al., 2014). Similar observations have been made if combination of sonification and chemical treatments is used on poultry carcasses (Lillard, 1993; Lee et al., 2014). Entrapped Salmonella cells can be released once skin is ground and this may contribute to contamination of ground poultry when ground skin is mixed with other ground components. It may explain why Salmonella prevalence is significantly higher in ground turkey (19.9%) than on raw turkey carcasses (1.7%) (USDA-FSIS, 2015).
Chickens and turkeys can shed Salmonella asymptomatically for a long period of time because of intestinal colonization (i.e., 10 weeks in turkeys in this study) raising the risk of dissemination in the processing plant. Evans et al. (2015) demonstrated a linear relationship between the percentage of Salmonella-positive live-haul trailers entering the processing plant and the percentage of Salmonella-positive ground turkey samples. During transport, skin and feathers can be easily contaminated with Salmonella present in fecal material. Effective pre-harvest measures to minimize Salmonella prevalence in poultry may reduce cross contamination of poultry carcasses during processing. However, exclusion of skin from the product may be the best option for post-harvest reduction of Salmonella in ground chicken and ground turkey.
Conclusions
High prevalence of Salmonella in skin of infected poultry significantly contributes to contamination of ground chicken and ground turkey. Exclusion of skin from ground poultry components may be the best option for reducing Salmonella contamination in ground poultry products.
Author Contributions
C-SR, JM, MF, and ML designed and performed the experiments. C-SR, JM, and MF analyzed the data and wrote the manuscript. LP, LS, KJ, and AV provided technical assistance. RB provided statistical guidance. RB and ML contributed to the writing, discussion, and editing of this manuscript.
Funding
This work was funded by the North American Meat Institute Foundation and the State of Georgia, Veterinary Medical Experiment Station. Any opinions, findings, and conclusions or recommendations expressed in this material are those of the author(s) and do not necessarily reflect the views of the Veterinary Medical Experiment Station and the North American Meat Institute Foundation.
Conflict of Interest Statement
The authors declare that the research was conducted in the absence of any commercial or financial relationships that could be construed as a potential conflict of interest.
Acknowledgments
We would like to thank Dr. Biao He for allowing us to use his IVIS Lumina XR imaging system in experimental trial 1. We also thank Adrian Pickar, Alliyah Byrd, and Nimita Fifadara for their technical assistance. This work, presented here, was part of C-SR MS thesis, Salmonella harborage sites in infected poultry and influence of coccidiosis on the course of Salmonella infection (2018), the University of Georgia.
Supplementary Material
The Supplementary Material for this article can be found online at: https://www.frontiersin.org/articles/10.3389/fsufs.2019.00002/full#supplementary-material
References
Barrow, P. A., Huggins, M. B., Lovell, M. A., and Simpson, J. M. (1987). Observations on the pathogenesis of experimental Salmonella typhimurium infection in chickens. Res. Vet. Sci. 42, 194–199. doi: 10.1016/S0034-5288(18)30685-4
Burns-Guydish, S. M., Olomu, I. N., Zhao, H., Wong, R. J., Stevenson, D. K., and Contag, C. H. (2005). Monitoring age-related susceptibility of young mice to oral Salmonella enterica serovar Typhimurium infection using an in vivo murine model. Pediatr. Res. 58, 153–158. doi: 10.1203/01.PDR.0000157725.44213.C4
CDC. (2011). Multistate Outbreak of Human Salmonella Heidelberg Infections Linked to Ground Turkey. Atlanta, GA. Available online at: http://www.cdc.gov/salmonella/2011/ground-turkey-11-10-2011.html (accessed June 21, 2016).
CDC. (2014a). Multistate Outbreak of Multidrug-Resistant Salmonella Heidelberg Infections Linked to Foster Farms Brand Chicken. Atlanta, GA. Available online at: http://www.cdc.gov/salmonella/heidelberg-10-13/index.html (accessed June 21, 2014).
CDC. (2014b). Salmonella. Atlanta, GA. Available online at: http://www.cdc.gov/salmonella/ (accessed June 21, 2016).
Chappell, L., Kaiser, P., Barrow, P., Jones, M. A., Johnston, C., and Wigley, P. (2009). The immunobiology of avian systemic salmonellosis. Vet. Immunol. Immunopathol. 128, 53–59. doi: 10.1016/j.vetimm.2008.10.295
Cheminay, C., Möhlenbrink, A., and Hensel, M. (2005). Intracellular Salmonella inhibit antigen presentation by dendritic cells. J. Immunol. 174, 2892–2899. doi: 10.4049/jimmunol.174.5.2892
Cheng, Y., Pedroso, A. A., Porwollik, S., Mcclelland, M., Lee, M. D., Kwan, T., et al. (2015). rpoS-Regulated core genes involved in the competitive fitness of Salmonella enterica Serovar Kentucky in the intestines of chickens. Appl. Environ. Microbiol. 81, 502–514. doi: 10.1128/AEM.03219-14
Collinson, S. K., Doig, P. C., Doran, J. L., Clouthier, S., Trust, T. J., and Kay, W. W. (1993). Thin, aggregative fimbriae mediate binding of Salmonella enteritidis to fibronectin. J. Bacteriol. 175, 12–18. doi: 10.1128/jb.175.1.12-18.1993
Cui, Y., Guran, H. S., Harrison, M. A., Hofacre, C. L., and Alali, W. Q. (2015). Salmonella levels in turkey neck skins, drumstick bones, and spleens in relation to ground turkey. J. Food Prot. 78, 1945–1953. doi: 10.4315/0362-028X.JFP-15-240
Engebrecht, J., and Silverman, M. (1984). Identification of genes and gene products necessary for bacterial bioluminescence. Proc. Natl. Acad. Sci. U.S.A. 81, 4154–4158. doi: 10.1073/pnas.81.13.4154
Erol, I., Goncuoglu, M., Ayaz, N. D., Ellerbroek, L., Ormanci, F. S., and Kangal, O. I. (2013). Serotype distribution of Salmonella isolates from turkey ground meat and meat parts. Biomed Res. Int. 2013, 281591. doi: 10.1155/2013/281591
Evans, N. P., Collins, D. A., Pierson, F. W., Mahsoub, H. M., Sriranganathan, N., Persia, M. E., et al. (2017). Investigation of medium chain fatty acid feed supplementation for reducing Salmonella typhimurium colonization in Turkey poults. Foodborne Pathog. Dis. 14, 531–536. doi: 10.1089/fpd.2016.2273
Evans, N. P., Evans, R. D., Regalado, J., Sullivan, J. F., Dutta, V., Elvinger, F., et al. (2015). Preharvest Salmonella detection for evaluation of fresh ground poultry product contamination. J. Food Prot. 78, 1266–1271. doi: 10.4315/0362-028X.JFP-14-509
FSIS (2013). Ground Poultry and Food Safety, on United States Department of Agriculture. Available online at: http://www.fsis.usda.gov/wps/portal/fsis/topics/food-safety-education/get-answers/food-safety-fact-sheets/poultry-preparation/ground-poultry-and-food-safety/CT_Index (accessed June 8, 2016).
FSIS (2015). Meat and Poultry Labeling Terms, on United States Department of Agriculture. Available online at: http://www.fsis.usda.gov/wps/portal/fsis/topics/food-safety-education/get-answers/food-safety-fact-sheets/food-labeling/meat-and-poultry-labeling-terms (accessed June 8, 2016).
Gast, R. K. (2013). “Paratyphoid infections,” in Diseases of Poultry, 13th Edn., eds D. E. Swayne, J. R. Glisson, L. R. Mcdougald, L. K. Nolan, D. L. Suarez, and V. Nair (Ames, IA: Wiley-Blackwell), 693–706.
Gast, R. K., and Holt, P. S. (1998). Persistence of Salmonella enteritidis from one day of age until maturity in experimentally infected layer chickens. Poult. Sci. 77, 1759–1762. doi: 10.1093/ps/77.12.1759
Gerstel, U., and Römling, U. (2001). Oxygen tension and nutrient starvation are major signals that regulate agfD promoter activity and expression of the multicellular morphotype in Salmonella typhimurium. Environ. Microbiol. 3, 638–648. doi: 10.1046/j.1462-2920.2001.00235.x
Howe, K., Karsi, A., Germon, P., Wills, R. W., Lawrence, M. L., and Bailey, R. H. (2010). Development of stable reporter system cloning luxCDABE genes into chromosome of Salmonella enterica serotypes using Tn7 transposon. BMC Microbiol. 10:197. doi: 10.1186/1471-2180-10-197
Kassem, I. I., Sanad, Y., Gangaiah, D., Lilburn, M., Lejeune, J., and Rajashekara, G. (2010). Use of bioluminescence imaging to monitor Campylobacter survival in chicken litter. J. Appl. Microbiol. 109, 1988–1997. doi: 10.1111/j.1365-2672.2010.04828.x
Kim, K. Y., Frank, J. F., and Craven, S. E. (1996). Three-dimensional visualization of Salmonella attachment to poultry skin using confocal scanning laser microscopy. Lett. Appl. Microbiol. 22, 280–282. doi: 10.1111/j.1472-765X.1996.tb01161.x
Lee, N. Y., Park, S. Y., Kang, I. S., and Ha, S. D. (2014). The evaluation of combined chemical and physical treatments on the reduction of resident microorganisms and Salmonella typhimurium attached to chicken skin. Poult. Sci. 93, 208–215. doi: 10.3382/ps.2013-03536
Lillard, H. S. (1989). Factors affecting the persistence of Salmonella during the processing of poultry. J. Food Prot. 52, 829–832. doi: 10.4315/0362-028X-52.11.829
Lillard, H. S. (1993). Bactericidal effect of chlorine on attached Salmonellae with and without sonification. J. Food Prot. 56, 716–717. doi: 10.4315/0362-028X-56.8.716
Mastroeni, P., and Grant, A. J. (2011). Spread of Salmonella enterica in the body during systemic infection: unravelling host and pathogen determinants. Expert Rev. Mol. Med. 13, e12. doi: 10.1017/S1462399411001840
Maurer, J. J., Brown, T. P., Steffens, W. L., and Thayer, S. G. (1998). The occurrence of ambient temperature-regulated adhesins, curli, and the temperature-sensitive hemagglutinin tsh among avian Escherichia coli. Avian Dis. 42, 106–118. doi: 10.2307/1592582
Meighen, E. A., and Dunlap, P. V. (1993). Physiological, biochemical and genetic control of bacterial bioluminescence. Adv. Microb. Physiol. 34, 1–67. doi: 10.1016/S0065-2911(08)60027-2
Nde, C. W., Mcevoy, J. M., Sherwood, J. S., and Logue, C. M. (2007). Cross contamination of turkey carcasses by Salmonella species during defeathering. Poult. Sci. 86, 162–167. doi: 10.1093/ps/86.1.162
Ochman, H., Soncini, F. C., Solomon, F., and Groisman, E. A. (1996). Identification of a pathogenicity island required for Salmonella survival in host cells. Proc. Natl. Acad. Sci. U.S.A. 93, 7800–7804. doi: 10.1073/pnas.93.15.7800
Olsén, A., Arnqvist, A., Hammar, M., and Normark, S. (1993). Environmental regulation of curli production in Escherichia coli. Infect. Agents Dis. 2, 272–274.
Özkaya, H., Akcan, A. B., Aydemir, G., Aydinöz, S., Razia, Y., Gammon, S. T., et al. (2012). Salmonella typhimurium infections in BALB/c mice: a comparison of tissue bioluminescence, tissue cultures and mice clinical scores. New Microbiol. 35, 53–59.
Phillips, R. A., and Opitz, H. M. (1995). Pathogenicity and persistence of Salmonella enteritidis and egg contamination in normal and infectious bursal disease virus-infected leghorn chicks. Avian Dis. 39, 778–787. doi: 10.2307/1592414
Rigney, C. P., Salamone, B. P., Anandaraman, N., Rose, B. E., Umholtz, R. L., Ferris, K. E., et al. (2004). Salmonella serotypes in selected classes of food animal carcasses and raw ground products, January 1998 through December 2000. J. Am. Vet. Med. Assoc. 224, 524–530. doi: 10.2460/javma.2004.224.524
Ritchie, J. M., Campbell, G. R., Shepherd, J., Beaton, Y., Jones, D., Killham, K., et al. (2003). A stable bioluminescent construct of Escherichia coli O157:H7 for hazard assessments of long-term survival in the environment. Appl. Environ. Microbiol. 69, 3359–3367. doi: 10.1128/AEM.69.6.3359-3367.2003
Salehi, S., Howe, K., Lawrence, M. L., Brooks, J. P., Bailey, R. H., and Karsi, A. (2017). Salmonella enterica Serovar Kentucky flagella are required for broiler skin adhesion and Caco-2 cell invasion. Appl. Environ. Microbiol. 83:e02115-16. doi: 10.1128/AEM.02115-16
Swart, A. L., and Hensel, M. (2012). Interactions of Salmonella enterica with dendritic cells. Virulence 3, 660–667. doi: 10.4161/viru.22761
USDA-FSIS (2014). Progress Report on Salmonella and Campylobacter Testing of Raw Meat and Poultry Products, CY 1998–2013. Available online at: https://www.fsis.usda.gov/wps/wcm/connect/885647f4-2568-48bf-ae5c-4a0d8279f435/Progress-Report-Salmonella-Campylobacter-CY2013.pdf?MOD=AJPERES (Accessed January 22, 2019).
USDA-FSIS (2015). Progress Report on Salmonella and Campylobacter Testing of Raw Meat and Poultry Products, CY, 1998–2014. Available online at: https://www.fsis.usda.gov/wps/portal/fsis/topics/regulatory-compliance/!ut/p/a0/04_Sj9CPykssy0xPLMnMz0vMAfGjzOINAg3MDC2dDbwMDIHQ08842MTDy8_YwMhYvyDbUREAhrzf7A!!/?1dmy¤t=true&urile=wcm%3Apath%3A%2Ffsis-content%2Finternet%2Fmain%2Ftopics%2Fdata-collection-and-reports%2Fmicrobiology%2Fannual-progress-reports (Accessed January 22, 2019).
Wu, D., Alali, W. Q., Harrison, M. A., and Hofacre, C. L. (2014). Prevalence of salmonella in neck skin and bone of chickens. J. Food Prot. 77, 1193–1197. doi: 10.4315/0362-028X.JFP-14-006
Keywords: Salmonella, Heidelberg, Typhimurium, chicken, turkey, bioluminescence imaging, immunohistochemistry
Citation: Rimet C-S, Maurer JJ, Pickler L, Stabler L, Johnson KK, Berghaus RD, Villegas AM, Lee M and França M (2019) Salmonella Harborage Sites in Infected Poultry That May Contribute to Contamination of Ground Meat. Front. Sustain. Food Syst. 3:2. doi: 10.3389/fsufs.2019.00002
Received: 06 November 2018; Accepted: 14 January 2019;
Published: 04 February 2019.
Edited by:
Steven C. Ricke, University of Arkansas, United StatesReviewed by:
Agnes Kilonzo-Nthenge, Tennessee State University, United StatesWalid Alali, Kuwait University, Kuwait
Copyright © 2019 Rimet, Maurer, Pickler, Stabler, Johnson, Berghaus, Villegas, Lee and França. This is an open-access article distributed under the terms of the Creative Commons Attribution License (CC BY). The use, distribution or reproduction in other forums is permitted, provided the original author(s) and the copyright owner(s) are credited and that the original publication in this journal is cited, in accordance with accepted academic practice. No use, distribution or reproduction is permitted which does not comply with these terms.
*Correspondence: Monique França, bWZyYW5jYUB1Z2EuZWR1