- Department of Food Science, University of Massachusetts, Amherst, MA, United States
Human noroviruses are the leading cause of foodborne illness globally. Numerous challenges in control of these viruses exist due to multiple viral characteristics: the ability to environmentally persist for over a month; relatively low infectious dose; lack of extremely effective, non-corrosive inactivation agents; and considerable inhibitory effects of food matrices and organic loads observed with common use of promising inactivation agents. Although a major breakthrough in the field, recent in vitro human norovirus cultivation systems have been limited in their ability to be widely utilized for identification of promising inactivation agents. Thus, cultivable human norovirus surrogates remain the most readily utilizable models for studying the effect of various inactivation agents on viral infectivity. The purpose of this review is to highlight recent developments and reports related to norovirus surrogate inactivation with a special focus on the various degrees of food matrix-associated inhibition for different inactivation agents.
Introduction
Human norovirus is the leading cause of foodborne illness globally, accounting for 684 million foodborne illnesses and 212,000 foodborne deaths annually (Kirk et al., 2015). It is a single stranded positive sense RNA, non-enveloped virus of the Caliciviridae family. The viruses have an icosahedral capsid composed of 180 copies of a major capsid protein, VP1, with a few copies of a minor capsid protein, VP2 (Moore et al., 2015). One considerable challenge to their control is their general resistance to many commonly used inactivation agents (Hirneisen et al., 2010). Further, study and identification of norovirus inactivation has been complicated by the historical lack of an in vitro cultivation system for the virus. Two in vitro cultivation models have been reported (Jones et al., 2014; Ettayebi et al., 2016), however their availability and ability to be routinely utilized in the study of viral inactivation is presently limited. Because of this, a number of morphologically similar and cultivable virus surrogates have been used to evaluate the effectiveness of various chemical disinfectants and physical processes. Among them, feline calicivirus (FCV) (Hoover and Kahn, 1975) and murine norovirus (MNV) (Karst et al., 2003) have been most widely used as surrogates for norovirus for inactivation studies (Hirneisen et al., 2010). Feline calicivirus was first reported and chosen as a surrogate because it is in the same family as human norovirus, cultivable, and shared similarities with human norovirus in primary sequence (Jiang et al., 1993). However, FCV causes respiratory illness in cats, hence it may not be as easily translated to human noroviruses with the study of certain disinfectants (Cannon et al., 2006; Pesavento et al., 2008; Richards, 2012; Cromeans et al., 2014). MNV was subsequently reported, and is more genetically related to human norovirus than FCV, as it is in the Norovirus genus (Wobus et al., 2006). But some disadvantages of MNV include the fact it utilizes a different putative receptor than human norovirus and does not display intense diarrhea and vomiting in mice despite being shed fecally (Cannon et al., 2006; Taube et al., 2009; Karst et al., 2014). More recently, Tulane virus (Farkas et al., 2008) has been utilized and belongs to a newly proposed genus, Recovirus. Similar to human norovirus, TV binds to histo-blood group antigens and causes diarrhea in rhesus macaques (Farkas et al., 2008; Zhang et al., 2015). Additionally, other surrogates such as bacteriophage MS2 (Lacombe et al., 2017) and echovirus (Schiff et al., 1984) have also been used to study inactivation. In addition to viral surrogates, infectious human norovirus from human fecal specimens may also be used in combination with RT-qPCR. However, there are a number of challenges in relating reduction of RT-qPCR signal to reduction of infectious virus observed, which will be discussed below and has been reviewed elsewhere (Knight et al., 2013; Moore et al., 2015; Manuel et al., 2018).
Although surrogates provide valuable information related to reduction in viral infectivity, a number of issues related to the ability to extrapolate the results of surrogate studies to human norovirus exist (Richards, 2012). Specifically, notable differences in susceptibility of different surrogates to different types of inactivation treatments has been a setback, as one specific surrogate that is the most resistant to a wide variety of inactivation treatments has not been identified. Similarly, translation of inactivation observed using human norovirus in fecal specimens has been challenging, as RT-qPCR results tend to underestimate the degree of inactivation of virus because signal is still obtained from un-encapsidated RNA, RNA in damaged/non-functional capsids, and RNA with fatal mutations (Knight et al., 2013; Manuel et al., 2018).
Although effective against many vegetative bacteria, most commercially available, and commonly used disinfectants have not displayed ideal efficacy against noroviruses and their surrogates (Hirneisen et al., 2010). Although bleach has been demonstrated to be effective against noroviruses including human noroviruses (Cromeans et al., 2014; Costantini et al., 2018), its utilization is limited by its unwanted effects on fabrics and stainless steel. Another major challenge related to foodborne virus inactivation has been to identify disinfectants that are more resistant to the effects of organic load on inactivation than current agents. Although frequently discussed, to the authors' knowledge no review exists with a focus on summarizing and compiling the effects of organic load on inactivation. While still a recalcitrant issue, a number of studies reporting novel inactivation agents for norovirus have been reported since the last comprehensive reviews of foodborne viral inactivation (Hirneisen et al., 2010; Li et al., 2012; DiCaprio et al., 2013). The purpose of this review is to present recent reports of novel inactivation agents with a focus on the effects of organic load for studies that utilize norovirus surrogates.
Gaseous and Vapor-Based Viral Inactivation Strategies
There are various forms of gaseous or vapor treatments employed against microbes in the food industry. Ozone treatment has been reported and evaluated as a novel alternative treatment to traditional methods like chlorine treatment. One advantage of ozone treatment is its limited effects on the environment, as ozone treatments rapidly dissociate into oxygen and do not produce the toxic byproducts that are generated from disinfectants like chlorine (Hirneisen et al., 2011). Ozone is a strong oxidizer that has been shown to inactivate a wide range of foodborne pathogens (Sharma and Demirci, 2002). It can act as a disinfectant both in the gaseous state as well as dispersed in water. Gaseous ozone is produced by corona discharge from compressed purified oxygen using an ozone generator. The ozone generated is then pumped to the desired location under controlled conditions (Wang et al., 2015). Although there have been a lot of studies conducted on assessing the effects of ozone on bacterially contaminated produce, there is a lack of research on the inactivation of viruses by ozone on fresh produce. Hirneisen et al. (2011) investigated the effects of aqueous ozone (not in gaseous form) applied against FCV and MNV on green onions and lettuce and in sterile water. Not surprisingly, the produce matrix was one of the largest variables in the efficacy of the treatment. For instance, to achieve 2-3 log inactivation of FCV with 6.25 ppm ozone, 5–10 min was required with produce samples while only 1 min was required in water. Overall, treatment with 6.25 ppm of ozone for viruses showed promise in buffer (>4 log reduction after 5 min treatment) and to some degree on produce (2–3.8 log reduction after 10 min of 6.25 ppm) (Table 1). MNV and FCV showed comparable susceptibility to the treatment on produce samples (Hirneisen et al., 2011). Further, Lim et al. (2010a) investigated the effects of pH and temperature on ozone inactivation of MNV. Interestingly, pH did not appear to have a significant effect on inactivation, but cooler temperatures tended to result in higher reduction of MNV (5 vs. 20°C), although the effect was not statistically significant (Lim et al., 2010a) (Table 1).
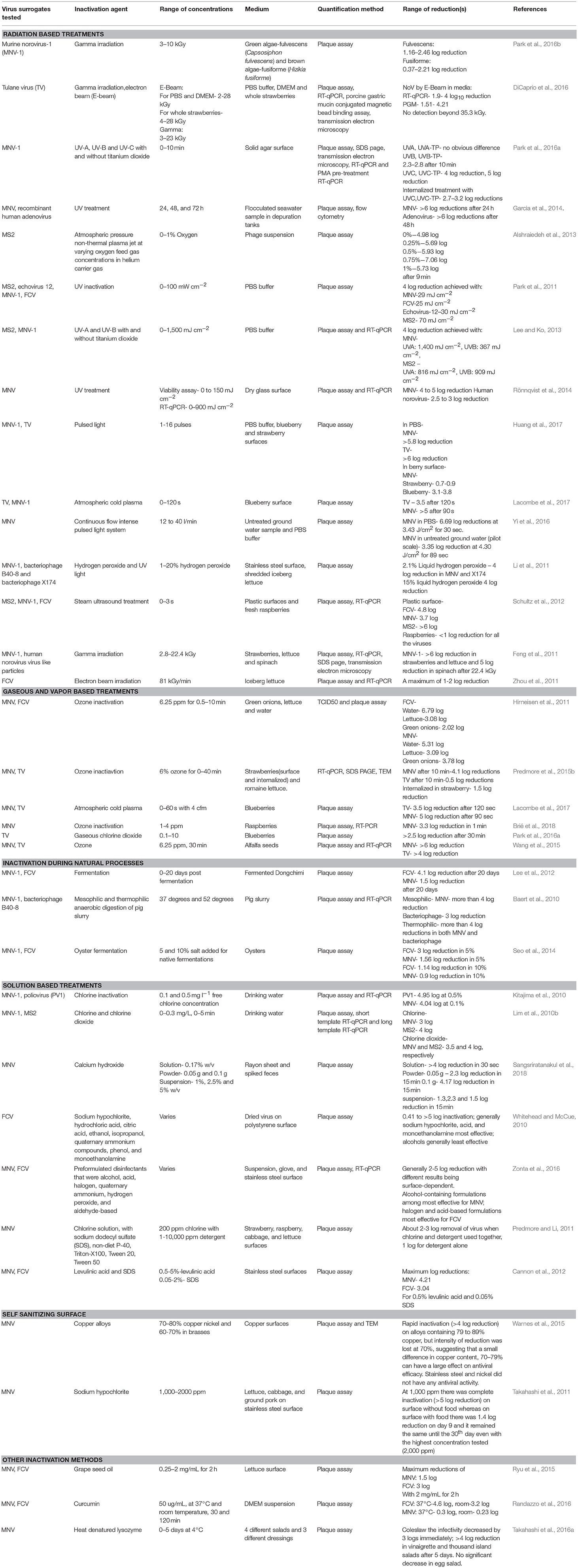
Table 1. Inactivation of human norovirus surrogates in multiple different applications with different inactivation agents.
In an interesting study by Predmore et al. (2015b), the ability of gaseous ozone to inactivate MNV and TV both internalized and deposited on the surface of strawberries and lettuce was investigated. After 40 min of treatment, there was a significantly lower log reduction internally (~1.5 log and 1 log for MNV and TV) than externally (3.3 log and >6 log for MNV and TV). This suggests that although gaseous treatments have potential to inactivate virus internalized in produce, one or more unknown factors limit its efficacy. In another study of gaseous ozone on produce, Brié et al. (2018) report promising inactivation of MNV on raspberries (>3 log reduction in 1 min with 3 ppm gaseous ozone); however, hepatitis A virus was also investigated and little inactivation (maximum of <1 log reduction) was observed. Interestingly, higher inactivation of MNV was observed on raspberries (>3.3 log) than in PBS (1.1 log) for the same treatment; suggesting that the raspberry matrix may enhance inactivation of MNV (Brié et al., 2018) (Table 2). It is possible other components of the raspberries (organic acids, discussed below) could have enhanced inactivation; however, it could be possible that raspberry inactivation results may have been confounded by reduced elution efficiency from the berry as MNV may have bound carbohydrates on the raspberry surface. Given the interesting results observed, further investigation into the mechanisms of enhanced inactivation on raspberries observed would be of value. Overall, the results observed for ozone suggest that ozone inactivation of viruses is variable and depends largely on factors such as the nature of the matrix and virus being studied (Brié et al., 2018).
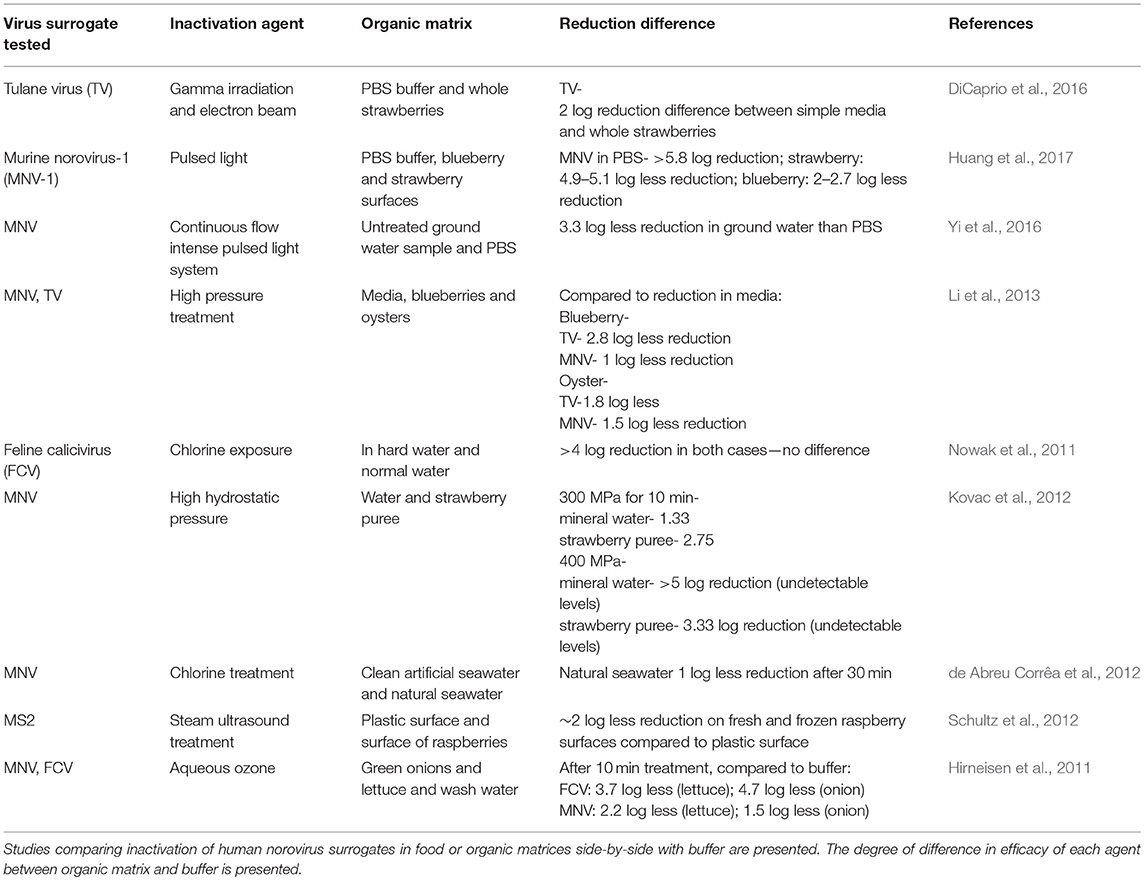
Table 2. Effect of organic load and food matrices on inactivation of human norovirus surrogates subjected to various treatments.
Another gaseous treatment for viral inactivation is atmospheric cold plasma. It is an emerging non-thermal technology that is chemical- and water-free. Typically, the cold plasma can be generated at atmospheric pressure using corona discharge, dielectric barrier discharge, radio frequency plasma or gliding arc discharge (Aboubakr et al., 2015). Atmospheric plasma had shown promise in inactivation of bacteria, yeasts, molds, and biofilms that are generally very difficult to inactivate (Niemira, 2012). Alshraiedeh et al. (2013) studied the effect of atmospheric pressure non-thermal plasma on bacteriophage MS2 with different percentages of oxygen gas added in the generation of the plasma (0–1% oxygen with the rest being helium carrier gas). Overall, the most reduction was observed for treatments with 0.75% oxygen, as nearly a 4 log (3.90) reduction was observed after 5 min, and ~7 log reduction was observed after 9 min. The higher reduction with less oxygen (0.75% > 1% oxygen) was suspected to be due to the increasing oxygen concentration influencing the plasma production itself (Alshraiedeh et al., 2013). Another study by Aboubakr et al. (2015) reported >6 log reduction of FCV when plasma generated with 1% oxygen was applied for 90 s, a reduction that is considerably larger than many other norovirus inactivation methods. Lacombe et al. (2017) further investigated the ability of cold plasma to non-thermally inactivate TV and MNV on the surface of blueberries (keeping temperature-time treatments below that required to kill enteric viruses), which achieved a maximum reduction of about 3.5 log and >5 log PFU/g reduction of TV and MNV on blueberries with a maximum treatment time of 2 min when ambient air was introduced (Lacombe et al., 2017) (Table 1). Although it has demonstrated considerable promise as a norovirus control agent, further study of atmospheric cold plasma is warranted to further characterize and elucidate any discrepancy in results between different surrogates and on different matrices.
Radiation-based Treatments
Irradiation has become a standard process used to sterilize many consumer and medical products, including some application in the food industry (Tauxe, 2001; Predmore et al., 2015a). Radiation-based techniques for treatment of foods have numerous advantages such as the generally high efficacy against bacteria and maintenance of the treated food quality. The safety of consuming irradiated foods has been evaluated in large scale trials in animals with no ill effects observed, and, in particular, no teratogenic effects (Samuel, 2007). The most extensively investigated radiation-based treatments include gamma radiation, electron beam and X-rays. High energy X-rays are produced when an electron beam is applied to metal foil. Like gamma rays, a beam of X-rays can penetrate foods to a much greater depth than electron beams (Tauxe, 2001). Gamma radiation is commonly produced using cobalt 60 and cesium 137, with rays that are generated during the isotopic negative beta decay of radioactive isotopes. Gamma radiation also has the powerful advantage of being completely able penetrate through the food, thereby killing both microbes on the surface as well as those internalized without substantially affecting the quality of the treated food (De Roda Husman et al., 2004). Electron beams are produced by passing electrons through high voltage electrostatic fields prior to application to the target food or surface. Unlike gamma radiation, electron beam radiation has shown more promise to be utilized for inactivation of surfaces, as it is not capable of completely penetrating foods. The efficacy of both electron beam and gamma radiation in both buffer and on whole strawberries was evaluated for TV by DiCaprio et al. (2016). Overall, electron beam treatment did not appear as effective as gamma irradiation. For electron beam inactivation, a fairly high dose of exposure (about 11 kGy) was required to obtain a 4 log reduction in buffer (PBS/DMEM), with an even higher amount required on strawberries (~15 kGy) (Table 2). A lower dose of gamma radiation was required to achieve the same level of inactivation in buffer (~9 kGy) (DiCaprio et al., 2016). Park et al. (2016b) studied the effect of gamma irradiation on reduction of MNV inoculated onto edible green and brown algae, fulvescens (Capsosiphon fulvescens), and fusiforme (Hizikia fusiforme). Generally, minor reductions of viral titer were observed, as there was 1.2–2.5 log reduction in fulvescens and 0.4–2.2 log reduction in fusiforme after electron beam treatments of 3 to 10 kGy. Additionally, the sensory characteristics such as the color and appearance of the seaweed were analyzed, with no significant difference noted. Similar results were observed for electron bean reduction of FCV on lettuce, with only a maximum of 2 log reduction observed (Zhou et al., 2011). The FDA has approved a maximum dose of 4 kGy of gamma irradiation for treatment of fresh produce, which has been effective for bacteria but appears not to be as effective for norovirus surrogates, including novel surrogates like vesicular stomatitis virus (Feng et al., 2011) (Table 1).
UV light treatment has historically been widely utilized by water treatment plants, has also been used for seawater disinfection in depuration tanks (Garcia et al., 2014). Depuration is a process where specific organisms (in this case bivalve mollusks) are placed into a large area or tank of clean water to allow for expulsion and diffusion/removal of any contaminants that may have been present on the organism (in this case viruses) to be removed. This is particularly relevant, as molluscan shellfish are a leading food implicated in human norovirus illness. Garcia et al. (2014) investigated the ability of UV to reduce MNV and recombinant human adenovirus artificially seeded into depuration water. Water samples were taken after the treatment and prior to further chemical disinfection (without residual free chlorine). More reduction of MNV was observed than adenovirus after 24 and 48 h of treatment, however the treatment was quite effective overall. There was a 4 log reduction of adenovirus and MNV had reached undetectable limits (>6 log reduction) after 24 h, with adenovirus being reduced to the same levels after 48 h (Garcia et al., 2014). Park et al. (2016a) investigated the effects of different variables of UV wavelength and photocatalysis on inactivation of MNV. The experiment was conducted on a solidified agar matrix (SAM) used to replicate the matrix of a blueberry. There are multiple types of UV treatment based on the wavelength of the radiation chosen (UV-A, UV-B, UV-C). Additionally, differences in efficacy of UV lamps had been reported for lamps coated and not coated with TiO2. Both of these variables and their effects on reduction of MNV were investigated. UV-C treatment caused a significantly higher reduction of virus than UV-A and UV-B. Additionally, application of TiO2 further enhanced viral inactivation. The mechanism of inactivation was also investigated, and the UV treatment was found to inactivate virus by causing damage to both the capsid protein and genomic RNA (Park et al., 2016a). Similar observations are reported by Lee and Ko (2013) when applying different UV wavelengths to inactivate MS2 with and without TiO2, with UV-A showing no significant reduction without enhancement of inactivation by including TiO2. In the case of UV-B, a 4 log reduction was obtained even without the presence of TiO2. UV-C was not investigated (Lee and Ko, 2013). As with other inactivation methods, differences in the susceptibility of different surrogates has also been observed for UV treatment. Park et al. (2011) compared the UV inactivation of FCV, echovirus 12, and bacteriophage MS2 in suspension. The order of UV resistance from highest to lowest was MS2>echovirus 12>MNV>FCV. The UV dose required to obtain 4 log reduction was 70, 30, 29, and 25 mJ cm−2 for MS2, echovirus 12, MNV, and FCV, respectively. Interestingly, viral association with host cells was found to have a protective effect against UV, as cell-associated echovirus 12 was three times more resistant than free virus (Park et al., 2011). Rönnqvist et al. (2014) also obtained promising results for reduction of MNV on dry glass, observing over a 4 log reduction at 60 mJ/cm2. (Rönnqvist et al., 2014) (Table 1). Because UV treatment alone in some cases has not traditionally achieved the desired levels of viral reduction, Li et al. (2011) investigated the potential use of vapor and liquid hydrogen peroxide in addition to UV treatment for reduction of MNV and bacteriophages X174 and B40-8. The results obtained suggested that liquid hydrogen peroxide was more effective than the vapor when used without UV treatment, as a 4 log reduction of virus on stainless steel disks was observed at 2% liquid hydrogen peroxide and <1 log reduction was observed for vapor hydrogen peroxide treatments for MNV and bacteriophage, respectively. However, when used in conjunction with UV there was faster inactivation, as a 4 log reduction of X174 and B40-8 and 3 log reduction for MNV in the case of vapor hydrogen peroxide with UV treatment in about half the time. Additionally, the ability of the combined hydrogen peroxide-UV treatments to reduce virus in shredded iceberg lettuce was also investigated, with similar trends related to liquid vs. vapor hydrogen peroxide and with added efficacy for UV-hydrogen peroxide used in combination (Table 1). However, the overall degree of inactivation of MNV and bacteriophages reduced, with 2 log and 1 log maximum reductions observed, respectively (Li et al., 2011) (Table 2).
Another radiation-based method is pulsed light treatment, which has been shown to be effective against a broad spectrum of bacteria, viruses, and spores, and is approved by the U.S. Food and Drug Administration for use on food and food contact surfaces (Rowan et al., 1999). Pulsed light inactivation of TV and MNV was recently assessed in PBS and on blueberries and strawberries by Huang et al. (2017). TV displayed higher resistance to treatment than MNV, with maximum reductions of 4.9 log and 5.8 log reduction achieved after 8 pulses for TV and MNV, respectively in PBS. As with other studies, higher inactivation of MNV was seen in PBS than on the surface of the berries when treated with 6 pulses of pulsed light, with 1.5 less log reduction in blueberries and 4 log less reduction in strawberries (Huang et al., 2017) (Table 2). Steam ultrasound is another alternative technology for disinfection of microorganisms on food and food contact surfaces. This technology combines pressurized steam and high-power ultrasound to efficiently remove the boundary air present on the surface of the treated sample by intensifying the air into oscillation. This allows the steam to penetrate into the microstructures and cavities on the surfaces of the treated sample more easily, resulting in a reduction of microorganisms within seconds. Schultz et al. (2012) tested this method against MS2, FCV, and MNV on the surface of raspberries as well as plastic. The highest reduction of >8 log was observed for MS2 on plastic surface after 3 s of treatment. Similarly promising reductions on plastic surfaces were observed for FCV and MNV: 4.5 log and 4 log reductions, respectively. However, a reduction of about 1 log of the MS2 was observed on the surface of the raspberries. Although displaying a promising degree of inactivation on the plastic surface, the treatment was not effective on the surface of the raspberries while also damaging the appearance of the berry (Schultz et al., 2012).
Solution-based Treatments
Chlorine Inactivation
Chlorine is a commonly used solution-based disinfectant in the food industry as it is affordable, and generally broadly effective as a biocide. Chlorine in water is used in many forms amongst which hypochlorous acid has been found to be effective against numerous microorganisms (Robson et al., 2007; Wang et al., 2007; Rahman et al., 2010). The main disadvantage in using chlorine-based disinfectants is their ability to form potentially toxic byproducts upon reacting with various matrix-based substances. As it does not react with matrix-based substances to form halogenated byproducts, chlorine dioxide has been widely investigated as a solution-based disinfectant. Lim et al. (2010b) investigated the ability of chlorine and chlorine dioxide to inactivate MNV and MS2 at two different temperatures (5 and 20°C) in oxygen demand free buffer. In the case of chlorine, it took 2 min to obtain a maximum 2 log reduction in MNV at 5°C, and 1 min to obtain a maximum 2.5 log reduction at 20°C. For MS2 it took 4 min at 5°C and 1.5 min at 20°C to attain a maximum 4 log reduction. For chlorine dioxide, a 3.5 log reduction was observed for MNV after 1 min at 5°C and 0.5 min at 20°C. For MS2, it took 2 min at 5°C and 0.7 min at 20°C to observe the same reduction (Lim et al., 2010b) (Table 1). In addition to MNV and MS2, poliovirus has been used as a cultivable non-enveloped enteric virus used in the study of inactivation. In a study investigating the ability of chlorine to inactivate non-enveloped viruses in water, Kitajima et al. (2010) inoculated water with poliovirus and MNV prior to chlorination and studied the resulting viral reduction using plaque assay. Two free chlorine concentrations of 0.5 mg l−1 and 0.1 mg l−1 were used. At 0.1 mg l−1, there was more inactivation with MNV (4 log reduction) than poliovirus (3.8 log reduction) after 120 min. Whereas, in the case of 0.5 mg l−1 there was 0.5 more log reduction of poliovirus than MNV after 30 min. In an interesting study by de Abreu Corrêa et al. (2012) chlorine inactivation of MNV in relatively clean artificial seawater and natural seawater was investigated. More reduction in viral titer in the artificial seawater was observed, suggesting that the presence of various other matrix-associated components in natural seawater reduced the ability of chlorine to inactivate MNV (de Abreu Corrêa et al., 2012) (Table 2). Sodium hypochlorite (bleach) is another form of chlorine that is a recommended disinfectant for human norovirus. In an interesting study by Takahashi et al. (2011), the degree to which food residue aids MNV persistence and reduces the efficacy of sodium hypochlorite on stainless steel surfaces was investigated. The food samples used were lettuce, cabbage and ground pork which were applied to stainless steel coupons. In positive control stainless steel coupons without any food residue, complete inactivation with 1,000 ppm (6.2 log MPN/ml) was observed after 30 days whereas in the steel surfaces with food residues, a maximum 1.4 log MPN/ml reduction was observed with a higher concentration of bleach (2,000 ppm). These drastic differences in viral reduction underscore the importance of proper cleaning protocols prior to disinfection in food processing and preparation settings.
Calcium Hydroxide
In addition to chlorine-based treatments, calcium hydroxide is another alkaline microbial inactivation agent, and is available in powder, solution and suspension forms. It has also been demonstrated to inactivate bacteria even in the presence of organic compounds. Sangsriratanakul et al. (2018) investigated the degree to which the food grade calcium hydroxide obtained in powder, solution, and suspension forms could inactivate MNV in solution and in the presence of organic material using TCID50. Calcium hydroxide in a solution form (0.17%) was highly effective with and without organic load, obtaining over a 4 log reduction in 30 s, including the inclusion of 5% fetal bovine serum. Similarly, the 0.17% solution was effective on rayon sheets with 5% fetal bovine serum, achieving over a 4 log reduction in 5 min. For suspension assay, 1, 2.5, and 5% suspensions of calcium hydroxide were applied to MNV spiked into mouse feces, and there were less dramatic reductions of 1.3, 2.3, and 1.5 log reductions within 15 min, respectively. Calcium hydroxide applied directly in powdered form 10 or 20% (weight/weight) to feces and litter resulted in >3 log reduction in 30 min. These results are promising given the ability of calcium hydroxide to reduce virus in high organic load samples; however, the extremely alkaline nature of calcium hydroxide (pH 13 at 0.17% solution) may limit its practical application (Sangsriratanakul et al., 2018).
Common Household Sanitizers
Investigation of the effectiveness of common sanitizers added to products that are used on an everyday basis on norovirus surrogates has also been conducted. In an interesting study by Whitehead and McCue (2010), common household disinfectants including alcohol, acid, quaternary ammonium compounds, and phenols both alone and in various combinations as formulated in common disinfectants were tested on FCV with an initial titer of 4 log dried on polystyrene surfaces for 1 min. The dried virus was treated with the active test substance in both aerosol and trigger spray forms and virus enumerated using TCID50. Sodium hypochlorite at a concentration of 1,000 ppm demonstrated >3 log reduction, similarly organic and inorganic acid at a pH of 2 and alkali at pH of 12 demonstrated a >3 log reduction. However, the results for organic acids should be taken with caution given FCV's higher susceptibility to low pH. Alternatively, purified alcohol and quaternary compound solutions and phenol and pine oil at common disinfectant use concentrations were not as effective. Both ethanol and isopropanol at 60% concentration and alkyl dimethyl benzyl ammonium chloride at 0.3% active at pH 8.0 provided a 1 log reduction, while a phenol-based disinfectant at 500 ppm active ingredient demonstrated a 2 log reduction. pH was found to be an important factor in the efficacy of some treatments. An alkali, monoethaloamine, was not considerably effective at a pH of 10.8, but was at pH 12 (Whitehead and McCue, 2010). In a similar survey study by Zonta et al. (2016), the antiviral efficacy of seven different disinfectant formulations (alcohol, halogens, peracetic acid/hydrogen peroxide, quaternary ammonium compounds/alcohol/aldehyde, and alcohol/biguanide) when applied to MNV and FCV in suspension and on stainless steel and gloves was investigated. A number of treatments showed promising reductions for both surrogates, with nearly all treatments exceeding a 3 log reduction. These generally higher numbers of complete formulations of disinfectants observed by Zonta et al. (2016) compared to those reductions observed with the active ingredient by Whitehead and McCue (2010) could be due to the longer contact times studied by Zonta et al. (2016) (5–15 min vs. 1 min) or could also suggest that disinfectant formulation/combination can enhance viral inactivation. In an interesting study by Predmore and Li (2011), the addition of surfactants to a chlorine solution (200 ppm chlorine) demonstrated significantly higher removal and reduction of MNV from different produce samples. Interestingly, the most inactivation tended to be observed on either gloves or in suspension while the least inactivation was observed on stainless steel surfaces for MNV and FCV; however, determination of the potential mechanism for this was beyond the scope of the study and could be an avenue of future investigation. Additionally, the mechanisms and effects of disinfectant combination and formulation on enhancing inactivation could also be of future study.
Levulinic acid with sodium dodecyl sulfate (SDS) has been demonstrated to be effective against bacterial foodborne pathogens, including in the presence of samples with high organic load. Cannon et al. (2012) investigated the effectiveness of levulinic acid and SDS both alone and in combination against MNV and FCV in suspension and on stainless steel surfaces at pH 2-5. Generally, lower reduction for both MNV and FCV was observed when levulinic acid and SDS were used individually (<0.5 log reduction). However, the combination of 0.5% levulinic acid with 0.5% SDS inactivated both surrogates by 3 to 4.21 log PFU/ml after 1 min of exposure in suspension for MNV and FCV, respectively, suggesting a synergistic effect. There was significantly more inactivation at lower pH for the combination for both MNV and FCV. Overall this study suggested that addition of detergents to organic acids can produce a synergistic effect and enhance viral inactivation (Cannon et al., 2012). In another study investigating the antiviral ability of organic acids and alcohols, Rabenau et al. (2014) tested the efficacy of five disinfectants against a number of non-enveloped model viruses on stainless steel surfaces and in suspension, specifically a model adenovirus (Adenovirus 5) and different animal parvoviruses (bovine, murine, canine, and porcine). Five compounds were investigated: glutaraldehyde (125–2,500 ppm), peracetic acid (50–1,500 ppm), ethanol (40–60%), 1-propanol (10–60%) and 2-propanol (20–60%). Interestingly, the general stability of porcine parvovirus to these disinfectants was similar to previous reports for MNV. Specifically, the concentrations of 1,500 ppm for PAA and 2,500 ppm for GDA to achieve a 4 log inactivation within 5 min previously observed for MNV (Magulski et al., 2009) were similar to the 4.27-5.61 log reduction for 1,500 ppm peracetic acid and 3.65–3.8 log reduction for 2,500 ppm glutaraldehyde observed. Further, the alcohols tested had generally limited activity against porcine parvovirus, suggesting it should be investigated further as a potential cultivable surrogate for human noroviruses (Rabenau et al., 2014).
High Pressure Processing
High pressure processing (HPP) has been an alternative non-thermal inactivation method growing in popularity commercially for specific foods and has been well-studied for viruses in foods (Kingsley, 2013; Lou et al., 2015a). In a study by Sánchez et al. (2011), the effectiveness of HPP against MNV and norovirus GII.4 in solution at different temperatures were investigated. Specifically, the effect of HPP at 200–500 MPa after 15 min treatment at two temperatures (25 and 45°C) was tested. For MNV, HPP was able to inactivate at least 6.5 log of infectious viruses at a pressure of 450 MPa independently of the temperature applied when analyzed by TCID50. However, when 10 mM CaCl2 was added, <1 log reduction was observed, suggesting that certain matrix-based components or foods treated with HPP may require more intense treatment. MNV genomes were detected even after 15 min treatment at 500 MPa, with <2.5 log10 reduction—demonstrating that RT-qPCR underestimates the extent of viral inactivation as mentioned above (Sánchez et al., 2011; Manuel et al., 2018). Thus, the observation by the authors that HPP did not reduce GII.4 genomes by more than 1 log10 for any treatment may be potentially misleading. On the other hand, a subsequent study by Lou et al. (2012) did support the observation that GII.4 norovirus may be more resistant than MNV, as the effectiveness of HPP against GII.4 VLPs as a surrogate was analyzed. Interestingly, the study found that treatment that was reported to completely inactivate MNV and FCV (500–600 MPa for 2 min) did not have a considerable effect on the reduction of VLP HBGA (receptor) binding ability. However, when the pressure was increased above 800 MPa complete reduction of VLP integrity was observed in 15 min or less (Lou et al., 2012). Interestingly, further human norovirus work for which infectivity was determined using a gnotobiotic pig model demonstrated the efficacy of HPP with treatments as low as 350 MPa for 2 min so long as treatments were at 0°C (Lou et al., 2015b).
Further HPP work on noroviruses evaluated the effectiveness of HPP on virus in different food matrices beyond those investigated previously (Hirneisen et al., 2010; Kingsley, 2013; Lou et al., 2015a). Huang et al. (2014) investigated the degree to which the effectiveness of HPP on MNV in strawberries could be enhanced using different additional treatment factors. Reducing the treatment temperature generally ended up enhancing MNV inactivation, as reducing the temperature from 20 to 0°C increased viral reduction by nearly 4 log10 when a 350 MPa 2 min treatment was applied (Huang et al., 2014), similar to the observations for human norovirus strains (Lou et al., 2015b, 2016). Additionally, introducing water to the samples prior to treatment also significantly enhanced reduction, achieving additional 1-2 log reduction over the “dry” sample. Cycling pressure treatments did not appear to contribute considerable additional reduction (Huang et al., 2014). Sido et al. (2017) further investigated the effect including water in HPP treatment has on inactivation of MNV in green onions at different temperatures. As observed by Huang et al. (2014), inclusion of water significantly enhanced MNV inactivation after treatment at 350 MPa for 2 min at 4°C (>4 log reduction compared to 1.5 log reduction). However, no effect was observed at 20°C (both about 1.5 log reduction). As has been observed in other reports, decreasing temperature resulted in higher reductions of MNV, as 30 MPa treatment for 2 min at 1°C resulted in a higher reduction than similar treatments at 4°C and 10°C (Sido et al., 2017) (Table 1).
HPP was tested in addition to other treatment strategies to reduce MNV, hepatitis A, and adenovirus 41 in an interesting study by Hirneisen and Kniel (2013). In it, the ability of UV (240 mJ/s cm2), ozone (6.25 ppm, 10 min), calcium hypochlorite (150 ppm, 4°C), and HPP (500 MPa, 5 min, 20°C) to inactivate viruses both internalized and on the surface of green onions was evaluated. Not surprisingly, lower reductions were observed for internalized viruses compared to surface-associated viruses. Of the treatments, the HPP treatment achieved the highest reductions relative to the other three treatments. For instance, for MNV, reductions of >4.7 log and >6.4 log virus were observed for internalized and surface viruses, respectively. The next most effective treatment was ozone, with 1.5 and 2.5 log reductions for internalized and surface viruses, respectively (Hirneisen and Kniel, 2013). This could suggest that the physical nature of the inactivation caused by HPP also makes it less effected by organic load than many chemical-based treatments and allows for comparably better efficacy for internalized pathogens. Another study by Hirneisen et al. (2014) compared the ability of HPP to inactivate different picornaviruses (hepatitis A virus and Aichi virus) and caliciviruses (MNV and FCV) in salsa. Samples were treated at 9°C with 250–500 MPa for 1-10 min in salsa. FCV and hepatitis A were both rapidly inactivated, as >5 log reduction was observed in <1 min at 400 MPa; whereas MNV and Aichi virus were more resilient. For MNV, 5 min of 400 MPa treatment was needed to achieve a 4 log reduction, while <1 log reduction was observed after 10 min with Aichi virus (Table 1). As seen above for MNV, a similar generally similar results between buffer and salsa for Aichi virus reduction were observed (Hirneisen et al., 2014) (Table 2).
HPP has also been used in conjunction with the development of alternative molecular infectivity estimation methods for noroviruses due to the inability of RT-qPCR to reflect the infectious virus as discussed above. Li and Chen (2015) investigated the ability of a porcine gastric mucin binding assay followed by RT-qPCR to estimate the inactivation of MNV and TV in buffer by HPP compared to inactivation observed using plaque assay. MNV and TV were treated for 2 min at 250–550 MPa in both neutral and acidic buffer (pH 4) at refrigeration and room temperatures. Generally, the PGM-RT-qPCR method displayed similar reduction to plaque assays for the first 2 log of viral inactivation but then began to underestimate the degree of inactivation at higher inactivation levels. Overall, TV was more susceptible to HPP treatment than MNV, generally displaying 2 log more reduction than MNV for a given treatment under all temperature conditions. Further, reduction of pH at room temperature (21°C) decreased the efficacy of HPP treatment for both viruses (Li and Chen, 2015), making consideration of the food being treated important in application of HPP despite the technology's previously demonstrated general resistance to organic load-related effects. Similar trends were observed for HPP treatment of TV in buffer in a follow-up study by Li et al. (2017), which additionally evaluated the ability of direct RT-qPCR and propidium monoazide (PMA) pretreatment followed by RT-qPCR to estimate infectivity. Both RT-qPCR and PMA/RT-qPCR methods tended to further underestimate TV reductions relative to PGM-RT-qPCR and plaque assay methods for HPP treatment at refrigeration and room temperatures. A similar trend for the methods was observed with heat treatment in another study (Li et al., 2017). Interested readers are referred to a recent review on in vitro molecular infectivity methods (Manuel et al., 2018).
Thermal Inactivation
Numerous studies have been conducted evaluating the thermal susceptibility and inactivation kinetics of human norovirus surrogates in foods, as understanding the degree to which cooking can reduce the risk of foodborne norovirus transmission is of importance. As thermal inactivation of human norovirus surrogates has been excellently reviewed relatively recently (Bertrand et al., 2012; Bozkurt et al., 2015; Knight et al., 2016; Messens et al., 2017; Peng et al., 2017), only a few more recent selected studies will be discussed.
One interesting study by Takahashi et al. (2016b) examined the persistence and thermal stability of MNV in breads and dough in response to a large outbreak associated with bread in Japan in 2014. Not surprisingly, MNV was able to persist for at least 5 days with little reduction on both the crust and inside all three types of breads investigated: butter-rich rolls, French bread, and white bread. Further, the ability of MNV to survive on the surface of toasted white bread was investigated, with results suggesting that mere warming/slight toasting may not be sufficient to reduce >4 log of virus—though it should be noted that generally contamination at that high of a level of virus is uncommon. The ability of MNV to survive in dough when baked (130–190°C, 0–12 min) was tested, with some virus persisting in lightly baked/under-baked breads. Once the internal temperature of the bread reached around 70°C (~2–9 min of baking) generally >5.0 log of MNV was reduced (Takahashi et al., 2016b). The thermal inactivation kinetics of MNV and TV in strawberries (specifically strawberry puree), a food more commonly associated with norovirus outbreaks, was investigated by Bartsch et al. (2019). Generally, MNV was slightly more resistant to heat than TV, usually with 0.5–1 log less reduction at more intense heat treatments; though the difference was not statistically significant. Overall, treatment of the puree at 80°C for 8 s was sufficient to remove all virus tested for both viruses (>7 log); with over a 4 log reduction achieved after 4 s. Higher TV inactivation (~1 log more reduction at 60 vs. 63°C) in buffer was observed in another study primarily focused on evaluating molecular assays for estimating infectivity of heat and HPP treated viruses (Li et al., 2017), suggesting that some potential degree of strawberry matrix-associated protection of virus against heat treatment could occur. However, considerably more study of different treatments and additional controls on the internal temperature and its distribution in the food matrix would need to be conducted to confirm this. In another study in oysters, a similar phenomenon of a protective effect of oyster matrices against heat treatment of MNV and TV was observed (Araud et al., 2016), but as with the strawberry puree, the mechanism of the protective effect was beyond the scope of the study. One potential mechanism of a protective effect could be viral binding to carbohydrates present in the strawberry and oyster matrices, as such binding has been shown to protect the virus in the case of binding to bacterial cell surface carbohydrates (Li et al., 2015). Future study into the mechanisms of the potential protective effects of different food matrices—especially those associated with norovirus outbreaks—would likely be of value.
Inactivation During Natural Processes
There are many natural processes in the production of food products that have the potential to protect the food from spoilage while producing sensory and nutritional benefits. Traditionally, these foods have been produced without consideration of their antiviral effect. For example, fermentation is one such process in which favorable bacteria are grown that suppresses the growth of unfavorable microbes though competitive exclusion and the production of antimicrobial compounds. This has led to investigation of the effects of fermentation on viral inactivation. Lee et al. (2012) evaluated the antiviral effect of fermentation of Dongchimi, a type of kimchi, on MNV and FCV. The Dongchimi was initially fermented in a traditional manner at room temperature overnight, stored at 4°C, and spiked with MNV and FCV. Along with viral titer, the levels of lactic acid bacteria, pH, and acidity were also tested over the course of 20 days. In the case of FCV, there was about a 1.5 log reduction after 10 days, followed by a more intense decrease to get over a 4 log reduction by the end of the 20th day. Comparatively, there was less inactivation in the case of MNV, with a maximum reduction of 1.5 log after 20 days. Increases in lactic acid bacteria and acidity in both samples proceeded as would be expected for the fermentation. Overall, FCV was much more susceptible than MNV, which is consistent with previous reports demonstrating the high acid sensitivity of FCV (Lee et al., 2012). In another interesting study involving kimchi, Bae et al. (2018) tested the potential of black raspberry extract to enhance reduction of MNV and FCV in the development of kimchi over the period of 50 days. FCV titers were completely removed (>5 log) after 30 days, likely due to both the acidity and extract; while MNV titers were reduced by a maximum of 2.1 log after 50 days. Interestingly, the black raspberry extract seemed to have a more pronounced effect on enhancing FCV inactivation beyond the reduction observed with the kimchi fermentation alone than was observed for MNV, where inclusion of the extract had little additional effect (Bae et al., 2018).
Molluscan shellfish are among one of the most common foods associated with human norovirus outbreaks (Hall et al., 2014). There have been many cases of human norovirus outbreaks due to the consumption of raw oysters; however, they have not been as associated with fermented oysters, a popular Korean dish. Thus, Seo et al. (2014) conducted a study to determine if the fermentation process potentially has an antiviral effect on FCV and MNV. Oysters spiked with virus and two spontaneous fermentations based upon the addition of 5 and 10% NaCl were conducted at 18°C. The viral levels, pH, enzymatic activity, acidity, and levels of lactic acid bacteria were measured until the 15th day post fermentation. In both the fermentations, there was more reduction for FCV than MNV after 15 days. There was a 3 log reduction for FCV and a 1.6 log reduction for MNV in the 5% NaCl fermentation, and a 1.1 log reduction of FCV and 0.9 log reduction for MNV in the 10% NaCl fermentation. The higher reduction observed in the 5% fermentation compared to the 10% also corresponded to higher levels of lactic acid bacteria, acidity, enzymatic activity, and lower pH for the 5% fermentation compared to the 10%. The specific composition of the lactic acid bacteria communities in the two fermentations was not measured nor the degree of bacteriocin production (Seo et al., 2014), some of which have shown effectiveness against viruses (Al Kassaa et al., 2014)
Potential differences in the effect of temperature on viral reduction during fermentation were investigated by Baert et al. (2010), who investigated the inactivation of MNV and Bacteroides fragilis phage B40-8 in mesophilic (37°C) and thermophilic (52°C) anaerobic digestion of pig slurry, which is commonly used as a fertilizer in agricultural and livestock production and thus a potential source of viral contamination of produce. B. fragilis phage B40-8 had been reported to be more prevalent in sewage and is more resistant than other surrogate phages and has been used as an alternative model for viral persistence in sewage. At the mesophilic conditions, 4 and 3 log reductions of MNV and phage B40-8 were observed after 13 min, respectively. Overall, more reduction under thermophilic conditions was observed, with 4 and 2.5 log reductions for MNV and B40-8 after 7 min, respectively. In order to study the effect of live bacteria on inactivation, the experiment was also conducted with autoclaved slurry. In both thermophilic and mesophilic digestion, in MNV the level of inactivation was similar to the previous experiments, suggesting live bacteria do not play a significant role in MNV reduction. However, there was more reduction observed for B40-8, suggesting that phage replication in the slurry that was not autoclaved may have occurred, confounding those results (Baert et al., 2010). These applications demonstrate a potential protective, antiviral effect of fermentations in some conditions to reduce levels of norovirus surrogates. Future work to potentially identify the specific components and/or mechanisms involved in these reductions may be useful in the development of novel disinfectant formulations.
Self-Sanitizing Surfaces
The use of antimicrobial surfaces in high-risk environments may help to prevent the spread of many infectious agents, including noroviruses, that are capable of retaining infectivity on surfaces for prolonged periods of time. Copper and copper alloys have been shown to be effective at rapidly killing a range of bacterial, fungal, and viral pathogens in laboratory studies under various temperatures and humidity conditions. This led to investigation of their effectiveness against human norovirus and its surrogates. An initial study by Warnes and Keevil (2013) found a range of copper alloy surfaces (60–100% copper) were effective at inactivating MNV in both wet and dry contamination conditions. Specifically, a larger volume of MNV that would take longer to dry was applied to the surface to represent wet contamination conditions, whereas a very small volume (1 μl) that would very rapidly dry was applied to simulate dry touch contamination. Dry touch conditions resulted in the most rapid reduction, with a 4 log reduction of virus in <5 min for pure copper compared to a similar reduction on the wet contamination surface taking a little over 20 min. Further, Warnes and Keevil (2013) also investigated the relative role of different ionic forms of copper on inactivation of MNV; finding that Cu+1 was extremely effective while Cu+2 had some but less dramatic effect. Additionally, inclusion of molecules capable of quenching reactive oxygen species generated with Fenton chemistry did not prevent viral reduction, suggesting that copper inactivation of MNV did not involve Fenton generation of reactive oxygen species (Warnes and Keevil, 2013). A follow-up study by Warnes et al. (2015) investigated the ability of a larger range of copper alloys to inactivate MNV. MNV reduction was measured with plaque assay and the morphology of the virus studied by using TEM. MNV-1 was significantly inactivated on alloys containing 79 to 89% copper (~4 log reduction in 80 min), but a considerable amount of antiviral efficacy was lost at 70% copper and below (<2 log reduction over 120 min), suggesting that a small difference in copper content, 70 to 79% can have a large effect on antiviral efficacy. Further, alloys containing zinc (copper brasses) were generally more effective than copper-nickel alloys, suggesting the inclusion of zinc in a copper-based alloy may have a synergistic effect for viral reduction. In both studies, stainless steel did not have any antiviral activity (Warnes and Keevil, 2013; Warnes et al., 2015).
Other Inactivation Agents
Some components found in foods like plant antimicrobials have been investigated as potential food-grade inactivation agents for noroviruses. These natural disinfectants have been reviewed relatively recently (Ryu et al., 2015), but there have been some subsequent reports of the use of essential oils for inactivation of human norovirus surrogates. One such plant antimicrobial compound is curcumin. Curcumin is identified as an excellent natural photosensitizer, as the exposure of curcumin to light causes the generation of reactive oxygen species that can damage organic molecules. Randazzo et al. (2016) tested the antiviral efficacy of different concentrations (5–50 μg/ml) of curcumin subjected to photoactivation by intense LED blue light against FCV and MNV in suspension with media. Additionally, the experiment was conducted at two different temperatures, room temperature and 37°C. A promising degree of reduction was observed for FCV at 37°C (>4 log reduction), with less reduction observed at room temperature (1.7–3.3 log reduction). Although promising results were observed for FCV, there was little inactivation against MNV (0.6–0.9 log reduction), suggesting that additional treatment or more optimization of this method for viral inactivation may be warranted (Randazzo et al., 2016). In another study, Gilling et al. (2014a) investigated the antiviral properties of oregano oil and its active ingredient, carvacrol, against MNV in buffer and on surfaces. Overall, carvacrol was much more effective at inactivating MNV, as the tested concentration of oregano oil (4%) produced about a 1 log reduction, while 0.5% carvacrol produced up to a 3.87 log reduction after 1 h of treatment and over 4.5 log reduction after 24 h at room temperature. This is interesting, as carvacrol is the major component of oregano oil, sometimes comprising as much as 85% of oregano oil—thus the disproportionally higher inactivation observed with carvacrol suggests other components in oregano oil could act in a way to reduce carvacrol's antiviral efficacy. Additional use of cell binding, RNase protection, and electron microscopy suggested that carvacrol acts to disrupt/destroy the viral capsid, with capsids expanding in size substantially after treatment prior to degradation (Gilling et al., 2014a).
Another study by Gilling et al. (2014b) investigated the efficacy of multiple other essential oils and one active ingredient (allspice oil, lemongrass oil, and citral) in buffer, all with modest reductions observed (<1 log-2 log) after 6 h of treatment, and more substantial reductions (2–3 log) after 24 h. Interestingly, the oils seemed to have different inactivation mechanisms. Allspice oil appeared to directly damage the capsid and genome of the virus with capsids enlarging to over twice their size, while lemongrass oil and its active component citral appeared to indirectly inactivate MNV by coating the viral capsid and preventing effective binding and uncoating of the virus in host cells (Gilling et al., 2014b). In a subsequent study on antiviral efficacy of lemongrass oil, Kim et al. (2017) investigated the ability of lemongrass essential oil to inactivate MNV both in suspension and therapeutically in vivo in a mouse model. Using plaque assay, a maximum reduction in plaque formation of <1 log (75%) was observed. Next, GC-MS was further used to identify individual components responsible for viral reduction. Of them, 32 were selected for screening and citral was still found to be the most effective, achieving a similar maximum reduction (75.5%) as the oil. For the in vivo studies, pretreatment of MNV with lemongrass oil for 72 h at 4°C prior to oral administration resulted in significant reduction in viral RNA shedding over seven days, and a somewhat similar reduction in viral shedding of lemongrass oil-treated virus to that observed in vitro (~1 log) (Kim et al., 2017) (Table 1).
Another biological component with previously reported antimicrobial activity is heat denatured lysozyme. Lysozyme is an enzyme that hydrolyses the cell walls of gram-positive bacteria, specifically targeting peptidoglycans. Although it had been tested and used for control of bacteria, its effectiveness for noroviruses had not been tested, likely because of the lack of a viral lipid envelope. Takahashi et al. (2016a) tested the ability of heat denatured lysozyme to inactivate MNV in 4 types of salads (coleslaw, thousand island salad, vinaigrette salad, and egg salad) stored for 5 days at 4°C. The addition of the lysozyme reduced persistence of MNV >4 log for vinaigrette and thousand island salads, by a little <3 log for coleslaw, and by 0.3 log in egg salad. Interestingly, this trend was related to the acidity of the salads, as egg salad (pH 6.27) and coleslaw (pH 4.33) were the two least acidic of the salads tested (Takahashi et al., 2016a). In a recent, subsequent study, the application of heat denatured lysozyme to reduce levels of HAV and MNV on the surface of fresh berries was investigated (Takahashi et al., 2018). Specifically, Takahashi et al. (2018) demonstrated the ability of heat denatured lysozyme to inactivate HAV in addition to MNV in solution, and then tested its ability to reduce infectious virus on the surface of blueberries and mixed berries (strawberry and raspberry). Exposure of a 1% solution of heat denatured lysozyme to the berry surface for 1 min resulted in reductions comparable to those observed for 100 ppm sodium hypochlorite for HAV (~2–3 log) and MNV (~2–2.5 log) relative to a distilled water control (Takahashi et al., 2018) (Table 1). These findings are interesting, as heat denatured lysozyme lacks enzymatic activity. Further work identifying the antinoroviral mechanism of heat denatured lysozyme and its higher efficacy at lower pH would be valuable for this promising edible inactivation agent.
Conclusion
As has been reported in the past, this review highlights the variable success of different disinfectants against human norovirus surrogates, especially with a focus on the degree of food matrix inhibition on their efficacy. As has been reported, sodium hypochlorite remains one of the most effective inactivation agents, despite the numerous other advances reviewed here. Additionally, pulsed light irradiation, HPP, non-thermal atmospheric cold plasma, calcium hydroxide, and heat denatured lysozyme all showed promising degrees of viral inactivation; however, all have limitations in their application and gaps in knowledge that warrant further study. There were also promising results obtained with inactivation by utilizing gaseous/vapor treatments such as ozone if the appropriate dose is utilized under the appropriate conditions; especially regarding efficacy in potentially internalized viral contamination of foods. Amongst all the surrogates used, Aichi virus, hepatitis A, MNV, and TV generally showed higher resistance to most inactivation treatments studied compared to other surrogates like FCV and phages; however the former two were studied for one or two inactivation agents. Regardless of surrogate used, the suspected mechanism of inactivation of the method studied should be taken into consideration and multiple surrogates used when inactivation study is conducted. Despite the advances in inactivation reported here, future work is still needed to further identify promising non-corrosive inactivation agents for noroviruses that are still effective in the presence of organic loads. Quantification and compilation in the degree to which different disinfectants are resistant to organic loads is important for identifying agents that may be of more practical value in an applied setting. In sum, numerous advances in disinfectant development have been reported using norovirus surrogates for which infectivity assays are available, while future work identifying and developing promising agents more tolerant of organic load is still needed.
Author Contributions
PK generated initial draft and edited. H-YH aided in the construction of the initial draft. MDM conceived, edited, revised, and submitted the manuscript.
Funding
We thank the University of Massachusetts Amherst for providing funding for the generation of this manuscript.
Conflict of Interest Statement
The authors declare that the research was conducted in the absence of any commercial or financial relationships that could be construed as a potential conflict of interest.
Acknowledgments
The authors greatly acknowledge the support of the University of Massachusetts Amherst. The authors would also like to thank J. Valdez for his help with organizational insight into the paper.
References
Aboubakr, H. A., Williams, P., Gangal, U., Youssef, M. M., El-Sohaimy, S. A. A., Bruggeman, P. J., et al. (2015). Virucidal effect of cold atmospheric gaseous plasma on feline calicivirus, a surrogate for human norovirus. Appl. Environ. Microbiol. 81, 3612–3622. doi: 10.1128/AEM.00054-15
Al Kassaa, I., Hober, D., Hamze, M., Chihib, N. E., and Drider, D. (2014). Antiviral potential of lactic acid bacteria and their bacteriocins. Prob Antimicrob. Proteins 6, 177–185. doi: 10.1007/s12602-014-9162-6
Alshraiedeh, N. H., Alkawareek, M. Y., Gorman, S. P., Graham, W. G., and Gilmore, B. F. (2013). Atmospheric pressure, nonthermal plasma inactivation of MS2 bacteriophage: effect of oxygen concentration on virucidal activity. J. Appl. Microbiol. 115, 1420–1426. doi: 10.1111/jam.12331
Araud, E., DiCaprio, E., Ma, Y., Lou, F., Gao, Y., Kingsley, D., et al. (2016). Thermal inactivation of enteric viruses and bioaccumulation of enteric foodborne viruses in live oysters (Crassostrea virginica). Appl. Environ. Microbiol. 82, 2086–2099. doi: 10.1128/AEM.03573-15
Bae, G., Kim, J., Kim, H., Hyeon, J., Bi, D., Hyun, K., et al. (2018). Inactivation of norovirus surrogates by kimchi fermentation in the presence of black raspberry. Food Control 91, 390–396. doi: 10.1016/j.foodcont.2018.04.025
Baert, L., De Gusseme, B., Boon, N., Verstraete, W., Debevere, J., and Uyttendaele, M. (2010). Inactivation of murine norovirus 1 and Bacteroides fragilis phage B40-8 by mesophilic and thermophilic anaerobic digestion of pig slurry. Appl. Environ. Microbiol. 76, 2013–2017. doi: 10.1128/AEM.01657-09
Bartsch, C., Plaza-Rodriguez, C., Trojnar, E., Filter, M., and Johne, R. (2019). Predictive models for thermal inactivation of human norovirus and surrogates in strawberry puree. Food Control 96, 87–97. doi: 10.1016/j.foodcont.2018.08.031
Bertrand, I., Schijven, J. F., Sánchez, G., Wyn-Jones, P., Ottoson, J., Morin, T., et al. (2012). The impact of temperature on the inactivation of enteric viruses in food and water: a review. J. Appl. Microbiol. 112, 1059–1074. doi: 10.1111/j.1365-2672.2012.05267.x
Bozkurt, H., D'Souza, D. H., and Davidson, P. M. (2015). Thermal Inactivation of foodborne enteric viruses and their viral surrogates in foods. J. Food Prot. 78, 1597–1617. doi: 10.4315/0362-028X.JFP-14-487
Brié, A., Boudaud, N., Mssihid, A., Loutreul, J., Bertrand, I., and Gantzer, C. (2018). Inactivation of murine norovirus and hepatitis A virus on fresh raspberries by gaseous ozone treatment. Food Microbiol. 70, 1–6. doi: 10.1016/j.fm.2017.08.010
Cannon, J. L., Aydin, A., Mann, A. N., Bolton, S. L., Zhao, T., and Doyle, M. P. (2012). Efficacy of a levulinic acid plus sodium dodecyl sulfate–based sanitizer on inactivation of human norovirus surrogates. J. Food Prot. 75, 1532–1535. doi: 10.4315/0362-028X.11-572
Cannon, J. L., Papafragkou, E., Park, G. W., Osborne, J., Jaykus, L.-A., and Vinjé, J. (2006). Surrogates for the study of norovirus stability and inactivation in the environment: aA comparison of murine norovirus and feline calicivirus. J. Food Prot. 69, 2761–5. doi: 10.4315/0362-028X-69.11.2761
Costantini, V., Morantz, E. K., Browne, H., Ettayebi, K., Zeng, X.-L., Atmar, R. L., et al. (2018). Human norovirus replication in human intestinal enteroids as model to evaluate virus inactivation. Emerg. Infect. Dis. 24, 1453–1464. doi: 10.3201/eid2408.180126
Cromeans, T., Park, G. W., Costantini, V., Lee, D., Wang, Q., Farkas, T., et al. (2014). Comprehensive comparison of cultivable norovirus surrogates in response to different inactivation and disinfection treatments. Appl. Environ. Microbiol. 80, 5743–5751. doi: 10.1128/AEM.01532-14
de Abreu Corrêa, A., Carratala, A., Barardi, C. R. M., Calvo, M., Girones, R., and Bofill-Mas, S. (2012). Comparative inactivation of murine norovirus, human adenovirus, and human JC polyomavirus by chlorine in seawater. Appl. Environ. Microbiol. 78, 6450–6457. doi: 10.1128/AEM.01059-12
De Roda Husman, A. M., Bijkerk, P., Lodder, W., Van Den Berg, H., Pribil, W., Cabaj, A., et al. (2004). Calicivirus inactivation by nonionizing (253.7-nanometer-wavelength [UV]) and ionizing (gamma) radiation. Appl. Environ. Microbiol. 70, 5089–5093. doi: 10.1128/AEM.70.9.5089-5093.2004
DiCaprio, E., Ma, Y., Hughes, J., and Li, J. (2013). Epidemiology, prevention, and control of the number one foodborne illness. Human norovirus. Infect. Dis. Clin. North Am. 27, 651–674. doi: 10.1016/j.idc.2013.05.009
DiCaprio, E., Phantkankum, N., Culbertson, D., Ma, Y., Hughes, J. H., Kingsley, D., et al. (2016). Inactivation of human norovirus and Tulane virus in simple media and fresh whole strawberries by ionizing radiation. Int. J. Food Microbiol. 232, 43–51. doi: 10.1016/j.ijfoodmicro.2016.05.013
Ettayebi, K., Crawford, S. E., Murakami, K., Broughman, J. R., Karandikar, U., Tenge, V. R., et al. (2016). Replication of human noroviruses in stem cell–derived human enteroids. Science 353, 1387–1393. doi: 10.1126/science.aaf5211
Farkas, T., Sestak, K., Wei, C., and Jiang, X. (2008). Characterization of a rhesus monkey calicivirus representing a new genus of Caliciviridae. J. Virol. 82, 5408–5416. doi: 10.1128/JVI.00070-08
Feng, K., Divers, E., Ma, Y. M., and Li, J. R. (2011). Inactivation of a human norovirus surrogate, human norovirus virus-like particles, and vesicular stomatitis virus by gamma irradiation. Appl. Environ. Microbiol. 77, 3507–3517. doi: 10.1128/aem.00081-1
Garcia, L. A. T., Nascimento, M. A., and Barardi, C. R. M. (2014). Effect of UV light on the inactivation of recombinant human adenovirus and murine norovirus seeded in seawater in shellfish depuration tanks. Food Environ. Virol. 7, 67–75. doi: 10.1007/s12560-014-9177-x
Gilling, D. H., Kitajima, M., Torrey, J. R., and Bright, K. R. (2014a). Antiviral efficacy and mechanisms of action of oregano essential oil and its primary component carvacrol against murine norovirus. J. Appl. Microbiol. 116, 1149–1163. doi: 10.1111/jam.12453
Gilling, D. H., Kitajima, M., Torrey, J. R., and Bright, K. R. (2014b). Mechanisms of antiviral action of plant antimicrobials against murine norovirus. Appl. Environ. Microbiol. 80, 4898–4910. doi: 10.1128/AEM.00402-14
Hall, A. J., Wikswo, M. E., Pringle, K., Gould, L. H., and Parashar, U. D. (2014). Vital signs: foodborne norovirus outbreaks - United States, 2009-2012. MMWR. Morb. Mortal. Wkly. Rep. 63, 491–495.
Hirneisen, K. A., Markland, S. M., and Kniel, K. E. (2011). Ozone inactivation of norovirus surrogates on fresh produce. J. Food Prot. 74, 836–839. doi: 10.4315/0362-028X.JFP-10-438
Hirneisen, K., Reith, J. L., Wei, J., Hoover, D. G., Hicks, D. T., Pivarnik, L. F., et al. (2014). Comparison of pressure inactivation of caliciviruses and picornaviruses in a model food system. Innov. Food Sci. Emerg. Technol. 26, 102–107. doi: 10.1016/j.ifset.2014.10.003
Hirneisen, K. A., Black, E. P., Cascarino, J. L., Fino, V. R., Hoover, D. G., and Kniel, K. E. (2010). Viral inactivation in foods: a review of traditional and novel food-processing technologies. Compr. Rev. Food Sci. Food Saf. 9, 3–20. doi: 10.1111/j.1541-4337.2009.00092.x
Hirneisen, K. A., and Kniel, K. E. (2013). Inactivation of internalized and surface contaminated enteric viruses in green onions. Int. J. Food Microbiol. 166, 201–206. doi: 10.1016/j.ijfoodmicro.2013.07.013
Hoover, E. A., and Kahn, D. E. (1975). Experimentally induced feline calicivirus infection: clinical signs and lesions. J. Am. Vet. Med. Assoc. 166, 463–468.
Huang, R., Li, X., Huang, Y., and Chen, H. (2014). Strategies to enhance high pressure inactivation of murine norovirus in strawberry puree and on strawberries. Int. J. Food Microbiol. 185, 1–6. doi: 10.1016/j.ijfoodmicro.2014.05.007
Huang, Y., Ye, M., Cao, X., and Chen, H. (2017). Pulsed light inactivation of murine norovirus, Tulane virus, Escherichia coli O157:H7 and Salmonella in suspension and on berry surfaces. Food Microbiol. 61, 1–4. doi: 10.1016/j.fm.2016.08.001
Jiang, X., Wang, M., Wang, K., and Estes, M. K. (1993). Sequence and genomic organization of norwalk virus. Virology 195, 51–61. doi: 10.1006/viro.1993.1345
Jones, M. K., Watanabe, M., Zhu, S., Graves, C. L., Keyes, L. R., Grau, K. R., et al. (2014). Enteric bacteria promote human and mouse norovirus infection of B cells. Science 346, 755–759. doi: 10.1126/science.1257147
Karst, S. M., Wobus, C. E., Goodfellow, I. G., Green, K. Y., and Virgin, H. W. (2014). Advances in norovirus biology. Cell Host Microbe 15, 668–680. doi: 10.1016/j.chom.2014.05.015
Karst, S. M., Wobus, C. E., Lay, M., Davidson, J., and Virgin, H. W. (2003). STAT-1 dependent innate immunity to a Norwalk-like virus. Science 299, 1575–1578. doi: 10.1126/science.1077905
Kim, Y. W., You, H. J., Lee, S., Kim, B., Kim, D. K., Choi, J.-B., et al. (2017). Inactivation of norovirus by lemongrass essential oil using a norovirus surrogate system. J. Food Prot. 80, 1293–1302. doi: 10.4315/0362-028X.JFP-16-162
Kingsley, D. H. (2013). High pressure processing and its application to the challenge of virus-contaminated foods. Food Environ. Virol. 5, 1–12. doi: 10.1007/s12560-012-9094-9
Kirk, M. D., Pires, S. M., Black, R. E., Caipo, M., Crump, J. A., Devleesschauwer, B., et al. (2015). World Health Organization estimates of the global and regional disease burden of 11 foodborne bacterial, protozoal, and viral diseases, 2010: a data synthesis. PLoS Med. 12:e1001920. doi: 10.1371/journal.pmed.1001920
Kitajima, M., Tohya, Y., Matsubara, K., Haramoto, E., Utagawa, E., and Katayama, H. (2010). Chlorine inactivation of human norovirus, murine norovirus and poliovirus in drinking water. Lett. Appl. Microbiol. 51, 119–121. doi: 10.1111/j.1472-765X.2010.02869.x
Knight, A., Haines, J., Stals, A., Li, D., Uyttendaele, M., Knight, A., et al. (2016). A systematic review of human norovirus survival reveals a greater persistence of human norovirus RT-qPCR signals compared to those of cultivable surrogate viruses. Int. J. Food Microbiol. 216, 40–49. doi: 10.1016/j.ijfoodmicro.2015.08.015
Knight, A., Li, D., Uyttendaele, M., and Jaykus, L.-A. (2013). A critical review of methods for detecting human noroviruses and predicting their infectivity. Crit. Rev. Microbiol. 39, 295–309. doi: 10.3109/1040841X.2012.709820
Kovac, K., Diez-Valcarce, M., Raspor, P., Hernández, M., and Rodríguez-Lázaro, D. (2012). Effect of high hydrostatic pressure processing on norovirus infectivity and genome stability in strawberry puree and mineral water. Int. J. Food Microbiol. 152, 35–39. doi: 10.1016/j.ijfoodmicro.2011.10.005
Lacombe, A., Niemira, B. A., Gurtler, J. B., Sites, J., Boyd, G., Kingsley, D. H., et al. (2017). Nonthermal inactivation of norovirus surrogates on blueberries using atmospheric cold plasma. Food Microbiol. 63, 1–5. doi: 10.1016/j.fm.2016.10.030
Lee, J. E., and Ko, G. P. (2013). Norovirus and MS2 inactivation kinetics of UV-A and UV-B with and without TiO2. Water Res. 47, 5607–5613. doi: 10.1016/j.watres.2013.06.035
Lee, M. H., Yoo, S. H., Ha, S. D., and Choi, C. (2012). Inactivation of feline calicivirus and murine norovirus during Dongchimi fermentation. Food Microbiol. 31, 210–214. doi: 10.1016/j.fm.2012.04.002
Li, D., Baert, L., De Jonghe, M., Van Coillie, E., Ryckeboer, J., Devlieghere, F., et al. (2011). Inactivation of murine norovirus 1, coliphage phiX174, and Bacteroides fragilis phage B40-8 on surfaces and fresh-cut iceberg lettuce by hydrogen peroxide and UV light. Appl. Environ. Microbiol. 77, 1399–1404. doi: 10.1128/AEM.02131-10
Li, D., Breiman, A., le Pendu, J., and Uyttendaele, M. (2015). Binding to histo-blood group antigen-expressing bacteria protects human norovirus from acute heat stress. Front. Microbiol. 6:659. doi: 10.3389/fmicb.2015.00659
Li, J., Predmore, A., Divers, E., and Lou, F. (2012). New interventions against human norovirus: progress, opportunities, and challenges. Annu. Rev. Food Sci. Technol. 3, 331–352. doi: 10.1146/annurev-food-022811-101234
Li, X., and Chen, H. (2015). Evaluation of the porcine gastric mucin binding assay for high-pressure-inactivation studies using murine norovirus and tulane virus. Appl. Environ. Microbiol. 81, 515–521. doi: 10.1128/AEM.02971-14
Li, X., Huang, R., and Chen, H. (2017). Evaluation of assays to quantify infectious human norovirus for heat and high-pressure inactivation studies using tulane virus. Food Environ. Virol. 9, 314–325. doi: 10.1007/s12560-017-9288-2
Li, X., Ye, M., Neetoo, H., Golovan, S., and Chen, H. (2013). Pressure inactivation of Tulane virus, a candidate surrogate for human norovirus and its potential application in food industry. Int. J. Food Microbiol. 162, 37–42. doi: 10.1016/j.ijfoodmicro.2012.12.016
Lim, M. Y., Kim, J.-M., Lee, J. E., and Ko, G. (2010a). Characterization of ozone disinfection of murine norovirus. Appl. Environ. Microbiol. 76, 1120–1124. doi: 10.1128/AEM.01955-09
Lim, M. Y., Kim, J. M., and Ko, G. (2010b). Disinfection kinetics of murine norovirus using chlorine and chlorine dioxide. Water Res. 44, 3243–3251. doi: 10.1016/j.watres.2010.03.003
Lou, F., DiCaprio, E., Li, X., Dai, X., Ma, Y., Hughes, J., et al. (2016). Variable high-pressure-processing sensitivities for genogroup II human noroviruses. Appl. Environ. Microbiol. 82, 6037–6045. doi: 10.1128/AEM.01575-16
Lou, F., Huang, P., Neetoo, H., Gurtler, J. B., Niemira, B. A, Chen, H., et al. (2012). High-pressure inactivation of human norovirus virus-like particles provides evidence that the capsid of human norovirus is highly pressure resistant. Appl. Environ. Microbiol. 78, 5320–5327. doi: 10.1128/AEM.00532-12
Lou, F., Neetoo, H., Chen, H., and Li, J. (2015a). High hydrostatic pressure processing: a promising nonthermal technology to inactivate viruses in high-risk foods. Annu. Rev. Food Sci. Technol. 6, 389–409. doi: 10.1146/annurev-food-072514-104609
Lou, F., Ye, M., Ma, Y., Li, X., DiCaprio, E., Chen, H., et al. (2015b). A gnotobiotic pig model for determining human norovirus inactivation by high-pressure processing. Appl. Environ. Microbiol. 81, 6679–6687. doi: 10.1128/AEM.01566-15
Magulski, T., Paulmann, D., Bischoff, B., Becker, B., Steinmann, E., Steinmann, J., et al. (2009). Inactivation of murine norovirus by chemical biocides on stainless steel. BMC Infect. Dis. 9, 3–9. doi: 10.1186/1471-2334-9-107
Manuel, C. S., Moore, M. D., and Jaykus, L.-A. (2018). Predicting human norovirus infectivity: recent advances and continued challenges. Food Microbiol. 76, 337–345. doi: 10.1016/j.fm.2018.06.015
Messens, W., Fernandez-Escamez, P. S., Lees, D., Lindqvist, R., O'Mahony, M., Suffredini, E., et al. (2017). Thermal processing of live bivalve molluscs for controlling viruses: on the need for a risk-based design. Crit. Rev. Food Sci. Nutr. 8398, 0–0. doi: 10.1080/10408398.2017.1338246
Moore, M. D., Goulter, R. M., and Jaykus, L.-A. (2015). Human Norovirus as a foodborne pathogen: challenges and developments. Annu. Rev. Food Sci. Technol. 6, 411–433. doi: 10.1146/annurev-food-022814-015643
Niemira, B. A. (2012). Cold plasma decontamination of foods. Annu. Rev. Food Sci. Technol. 3, 125–142. doi: 10.1146/annurev-food-022811-101132
Nowak, P., Topping, J. R., Bellamy, K., Fotheringham, V., Gray, J. J., Golding, J. P., et al. (2011). Virolysis of feline calicivirus and human GII.4 norovirus following chlorine exposure under standardized light soil disinfection conditions. J. Food Prot. 74, 2113–2118. doi: 10.4315/0362-028X.JFP-11-087
Park, D., Shahbaz, H. M., Kim, S. H., Lee, M., Lee, W., Oh, J. W., et al. (2016a). Inactivation efficiency and mechanism of UV-TiO2 photocatalysis against murine norovirus using a solidified agar matrix. Int. J. Food Microbiol. 238, 256–264. doi: 10.1016/j.ijfoodmicro.2016.09.025
Park, G. W., Linden, K. G., and Sobsey, M. D. (2011). Inactivation of murine norovirus, feline calicivirus and echovirus 12 as surrogates for human norovirus (NoV) and coliphage (F+) MS2 by ultraviolet light (254 nm) and the effect of cell association on UV inactivation. Lett. Appl. Microbiol. 52, 162–167. doi: 10.1111/j.1472-765X.2010.02982.x
Park, S. Y., Kang, S., and Ha, S. D. (2016b). Inactivation of murine norovirus-1 in the edible seaweeds Capsosiphon fulvescens and Hizikia fusiforme using gamma radiation. Food Microbiol. 56, 80–86. doi: 10.1016/j.fm.2015.12.006
Peng, J., Tang, J., Barrett, D. M., Sablani, S. S., Anderson, N., and Powers, J. R. (2017). Thermal pasteurization of ready-to-eat foods and vegetables: critical factors for process design and effects on quality. Crit. Rev. Food Sci. Nutr. 57, 2970–2995. doi: 10.1080/10408398.2015.1082126
Pesavento, P. A., Chang, K. O., and Parker, J. S. L. (2008). Molecular virology of feline calicivirus. Vet. Clin. North Am. 38, 775–786. doi: 10.1016/j.cvsm.2008.03.002
Predmore, A., and Li, J. (2011). Enhanced removal of a human norovirus surrogate from fresh vegetables and fruits by a combination of surfactants and sanitizers. Appl. Environ. Microbiol. 77, 4829–4838. doi: 10.1128/AEM.00174-11
Predmore, A., Sanglay, G., Li, J., and Lee, K. (2015b). Control of human norovirus surrogates in fresh foods by gaseous ozone and a proposed mechanism of inactivation. Food Microbiol. 50, 118–125. doi: 10.1016/j.fm.2015.04.004
Predmore, A., Sanglay, G. C., DiCaprio, E., Li, J., Uribe, R. M., and Lee, K. (2015a). Electron beam inactivation of Tulane virus on fresh produce, and mechanism of inactivation of human norovirus surrogates by electron beam irradiation. Int. J. Food Microbiol. 198, 28–36. doi: 10.1016/j.ijfoodmicro.2014.12.024
Rabenau, H. F., Steinmann, J., Rapp, I., Schwebke, I., and Eggers, M. (2014). Evaluation of a virucidal quantitative carrier test for surface disinfectants. PLoS ONE 9:e86128. doi: 10.1371/journal.pone.0086128
Rahman, S. M. E., Ding, T., and Oh, D. H. (2010). Effectiveness of low concentration electrolyzed water to inactivate foodborne pathogens under different environmental conditions. Int. J. Food Microbiol. 139, 147–153. doi: 10.1016/j.ijfoodmicro.2010.03.020
Randazzo, W., Aznar, R., and Sánchez, G. (2016). Curcumin-mediated photodynamic inactivation of norovirus surrogates. Food Environ. Virol. 8, 244–250. doi: 10.1007/s12560-016-9255-3
Richards, G. P. (2012). Critical review of norovirus surrogates in food safety research: rationale for considering volunteer studies. Food Environ. Virol. 4, 6–13. doi: 10.1007/s12560-011-9072-7
Robson, M. C., Payne, W. G., Ko, F., Mentis, M., Donati, G., Shafii, S. M., et al. (2007). Hypochlorous acid as a potential wound care agent Part II. Stabilized hypochlorous acid: its role in decreasing tissue bacterial bioburden and overcoming the inhibition of infection on wound healing. J. Burns Wounds 6, 80–90.
Rönnqvist, M., Mikkelä, A., Tuominen, P., Salo, S., and Maunula, L. (2014). Ultraviolet light inactivation of murine norovirus and human norovirus GII: PCR may overestimate the persistence of noroviruses even when combined with Pre-PCR treatments. Food Environ. Virol. 6, 48–57. doi: 10.1007/s12560-013-9128-y
Rowan, N. J., MacGregor, S. J., Anderson, J. G., Fouracre, R. A., McIlvaney, L., and Farish, O. (1999). Pulsed-light inactivation of food-related microorganisms. Appl. Environ. Microbiol. 65, 1312–1315.
Ryu, S., You, H. J., Kim, Y. W., Lee, A., Ko, G. P., Lee, S. J., et al. (2015). Inactivation of norovirus and surrogates by natural phytochemicals and bioactive substances. Mol. Nutr. Food Res. 59, 65–74. doi: 10.1002/mnfr.201400549
Samuel, J. E. (2007). An historical review of food irradiation1. J. Food Saf. 5, 161–189. doi: 10.1111/j.1745-4565.1983.tb00469.x
Sánchez, G., Aznar, R., Martinez, A., and Rodrigo, D. (2011). Inactivation of human and murine norovirus by high-pressure processing. Foodborne Pathog. Dis. 8:249. doi: 10.1089/fpd.2010.0667
Sangsriratanakul, N., Toyofuku, C., Suzuki, M., Komura, M., Yamada, M., Alam, M. S., et al. (2018). Virucidal efficacy of food additive grade calcium hydroxide against surrogate of human norovirus. J. Virol. Methods 251, 83–87. doi: 10.1016/j.jviromet.2017.10.017
Schiff, G. M., Stefanovic, G. M., Young, E. C., Sander, D. S., Pennekamp, J. K., and Ward, R. L. (1984). Studies of echovirus-12 in volunteers: determination of minimal infectious dose and the effect of previous infection on infectious dose. J. Infect. Dis. 150, 858–866. doi: 10.1093/infdis/150.6.858
Schultz, A. C., Uhrbrand, K., Nørrung, B., and Dalsgaard, A. (2012). Inactivation of norovirus surrogates on surfaces and raspberries by steam-ultrasound treatment. J. Food Prot. 75, 376–381. doi: 10.4315/0362-028X.JFP-11-271
Seo, D. J., Lee, M. H., Seo, J., Ha, S. D., and Choi, C. (2014). Inactivation of murine norovirus and feline calicivirus during oyster fermentation. Food Microbiol. 44, 81–86. doi: 10.1016/j.fm.2014.05.016
Sharma, R. R., and Demirci, A. L. (2002). Inactivation of Escherichia coli on inoculated alfalfa seeds with ozonated water under pressure. J. food Saf. 22, 107–119. doi: 10.1111/j.1745-4565.2002.tb00334.x
Sido, R. F., Huang, R., Liu, C., and Chen, H. (2017). High hydrostatic pressure inactivation of murine norovirus and human noroviruses on green onions and in salsa. Int. J. Food Microbiol. 242, 1–6. doi: 10.1016/j.ijfoodmicro.2016.11.003
Takahashi, H., Ohuchi, A., Miya, S., Izawa, Y., and Kimura, B. (2011). Effect of food residues on norovirus survival on stainless steel surfaces. PLoS ONE 6:e21951. doi: 10.1371/journal.pone.0021951
Takahashi, H., Tsuchiya, T., Takahashi, M., Nakazawa, M., and Watanabe, T. (2016a). Viability of murine norovirus in salads and dressings and its inactivation using heat-denatured lysozyme. Int. J. Food Microbiol. 233, 29–33. doi: 10.1016/j.ijfoodmicro.2016.06.006
Takahashi, M., Okakura, Y., Takahashi, H., Imamura, M., Takeuchi, A., Shidara, H., et al. (2018). Heat-denatured lysozyme could be a novel disinfectant for reducing hepatitis A virus and murine norovirus on berry fruit. Int. J. Food Microbiol. 266, 104–108. doi: 10.1016/j.ijfoodmicro.2017.11.017
Takahashi, M., Takahashi, H., Kuda, T., and Kimura, B. (2016b). Viability and heat resistance of murine norovirus on bread. Int. J. Food Microbiol. 216, 127–131. doi: 10.1016/j.ijfoodmicro.2015.09.018
Taube, S., Perry, J. W., Yetming, K., Patel, S. P., Auble, H., Shu, L., et al. (2009). Ganglioside-linked terminal sialic acid moieties on murine macrophages function as attachment receptors for murine noroviruses. J. Virol. 83, 4092–4101. doi: 10.1128/JVI.02245-08
Tauxe, R. V. (2001). Food safety and irradiation: protecting the public from foodborne infections. Emerging Infect. Dis. 7, 516–521. doi: 10.3201/eid0707.017706
Wang, L., Bassiri, M., Najafi, R., Najafi, K., Yang, J., Khosrovi, B., et al. (2007). Hypochlorous acid as a potential wound care agent. J. Burns Wounds 6:e5.
Wang, Q., Markland, S. M., and Kniel, K. E. (2015). Inactivation of human norovirus and its surrogates on alfalfa seeds by aqueous ozone. J. Food Prot. 78, 1586–1591. doi: 10.4315/0362-028X.JFP-15-029
Warnes, S. L., and Keevil, C. W. (2013). Inactivation of norovirus on dry copper alloy surfaces. PLoS ONE 8:e75017. doi: 10.1371/journal.pone.0075017
Warnes, S. L., Summersgill, E. N., and Keevil, C. W. (2015). Inactivation of murine norovirus on a range of copper alloy surfaces is accompanied by loss of capsid integrity. Appl. Environ. Microbiol. 81, 1085–1091. doi: 10.1128/AEM.03280-14
Whitehead, K., and McCue, K. A. (2010). Virucidal efficacy of disinfectant actives against feline calicivirus, a surrogate for norovirus, in a short contact time. Am. J. Infect. Control 38, 26–30. doi: 10.1016/j.ajic.2009.03.015
Wobus, C. E., Thackray, L. B., and Virgin, H. W. (2006). Murine norovirus: a model system to study norovirus biology and pathogenesis. J. Virol. 80, 5104–5112. doi: 10.1128/JVI.02346-05
Yi, J. Y., Lee, N. H., and Chung, M. S. (2016). Inactivation of bacteria and murine norovirus in untreated groundwater using a pilot-scale continuous-flow intense pulsed light (IPL) system. LWT Food Sci. Technol. 66, 108–113. doi: 10.1016/j.lwt.2015.10.027
Zhang, D., Huang, P., Zou, L., Lowary, T. L., Tan, M., and Jiang, X. (2015). Tulane virus recognizes the A Type 3 and B histo-blood group antigens. J. Virol. 89, 1419–1427. doi: 10.1128/JVI.02595-14
Zhou, F., Harmon, K. M., Yoon, K.-J., Olson, D. G., and Dickson, J. S. (2011). Inactivation of feline calicivirus as a surrogate for norovirus on lettuce by electron beam irradiation. J. Food Prot. 74, 1500–1503. doi: 10.4315/0362-028X.JFP-11-121
Keywords: norovirus, surrogate, murine norovirus, inactivation, Tulane virus, organic load
Citation: Kamarasu P, Hsu H-Y and Moore MD (2018) Research Progress in Viral Inactivation Utilizing Human Norovirus Surrogates. Front. Sustain. Food Syst. 2:89. doi: 10.3389/fsufs.2018.00089
Received: 27 September 2018; Accepted: 11 December 2018;
Published: 21 December 2018.
Edited by:
Kristen E. Gibson, University of Arkansas, United StatesReviewed by:
Erin DiCaprio, University of California, Davis, United StatesMarilyn Erickson, University of Georgia, United States
Copyright © 2018 Kamarasu, Hsu and Moore. This is an open-access article distributed under the terms of the Creative Commons Attribution License (CC BY). The use, distribution or reproduction in other forums is permitted, provided the original author(s) and the copyright owner(s) are credited and that the original publication in this journal is cited, in accordance with accepted academic practice. No use, distribution or reproduction is permitted which does not comply with these terms.
*Correspondence: Matthew D. Moore, bWRtb29yZUB1bWFzcy5lZHU=