- 1Department of Poultry Science, University of Georgia, Athens, GA, United States
- 2Department of Animal Science, University of São Paulo, Piracicaba, Brazil
The objective of this study was to evaluate the effects of dietary autolyzed yeast (AY; Saccharomyces cerevisiae) supplementation on growth performance, immune system, and intestinal bacterial count in broiler chickens. A total of 1,260 1-day-old male Ross AP95 chicks were placed in a completely randomized design (4 treatments, 7 replicates each, and 45 birds/replicate). The treatments were: basal diet—negative control (NC); basal diet supplemented with 55 ppm of zinc bacitracin—positive control (PC); NC + 0.2% of AY; NC + 0.4% of AY. The diets were formulated based on corn-soybean meal with 5% inclusion of wheat bran and 5% of poultry by-product meal. At 7 days of age, all birds were eye drop-vaccinated with live vaccine against coccidiosis. At 8 and 21 days of age, one chicken per pen was then euthanized by cervical dislocation to collect ileal and cecal contents for enumeration of Enterococcus sp., Escherichia coli and Lactobacillus sp. Yet, ileal samples were collected to analyze the gene expression of Claudin-1, IL-1β, IL-4, TLR-4, and MUC-2 through real time PCR. On d 21, it was observed that the inclusion of 0.2% of AY improved FCR (P < 0.05) when compared to the NC treatment. In the overall experimental period, the inclusion of zinc bacitracin and 0.4% of AY improved FCR (P < 0.05) compared to the NC group. On d 8, supplementation of 0.2% of AY increased Enterococcus and both concentrations of AY reduced Lactobacillus in the ileal digesta compared to birds supplemented with zinc bacitracin. On d 21, 0.2% of AY reduced E. coli in the cecal digesta. On d 8, AY supplementation downregulated the expression of TLR-4 vs. the PC group (P = 0.04). On d 21, supplementation of AY upregulated the expression of IL-1β (P < 0.05) vs. the NC group. Supplementation of AY improved the growth performance of broiler chickens vaccinated against coccidiosis, partially explained by the modulation of the intestinal microbiota and immune-system.
Introduction
The search for antimicrobial replacements in the diets of broiler chickens is of paramount importance due to the recent restrictions in the use of antimicrobial growth promoters which is driven primarily by the increased incidence of antimicrobial resistance. In animal production systems, the restriction means only using antibiotic when necessary to protect the health of animals or humans (Hoelzer et al., 2018), and under a veterinarian prescription. However, according to the report “UK 5 Years Antimicrobial Resistance Strategy 2013–2018” there is scientific evidences showing that the increase of clinical issues related to “superbugs” and antimicrobial resistance in humans is a result of the use of antimicrobial in humans, and not in animals. Regardless of this fact, prevention of subclinical and clinical diseases in poultry must rely on non-antibiotic alternatives and good management conditions (Hoelzer et al., 2018).
The use of prebiotics, such as whole yeast (Saccharomyces cerevisiae), as well as carbohydrates isolated from its cellular wall (mannan-oligosaccharides-MOS, fructooligosaccharides-FOS, and β-glucans) has been the focus of several studies in poultry (Shanmugasundaram and Selvaraj, 2012; Shao et al., 2013; Shang et al., 2015, 2018; Adhikari et al., 2018). Nucleotides, isolated from the cytoplasm of yeast, have also been tested in broiler chickens (Uauy, 1994; Carver and Walker, 1995; Mateo et al., 2004). Autolyzed yeast is the product of cellular degradation, activated by its own enzymes, that solubilize cellular components (Reed and Nagodawithana, 1991; Sommer, 1996) and can be used in animal nutrition as well. Therefore, the use of autolyzed yeast in the diets of broiler chickens would supply the cellular components and the cell wall carbohydrates.
The composition of autolyzed yeast varies depending on the process used for its production. Usually, autolyzed yeast of Saccharomyces cerevisiae contains 29–64% of β-glucans, 31% mannan-oligosaccharides, 13% of protein, and 9% of lipids (Jaehrig et al., 2008). The benefits of supplementing yeast in diets of ruminants relies on the increase of cellulolytic bacteria in the rumen, increasing the energy extracted from the diets, and consequently the performance of the animals (Wallace, 1994; Newbold et al., 1995). In broiler chickens, the effects of prebiotics partially rely on blocking receptor sites of pathogen adhesion, immunomodulation, production of antimicrobial molecules by the intestinal microbiota, and shifts in the intestinal microbial structure (Shang et al., 2015, 2018; Adhikari et al., 2018; Suresh et al., 2018). For instance, supplementation of FOS to diets of broilers reduced the amount of potential pathogenic microorganisms, such as Helicobacter and Desulfovibrio, in the ileal mucosa, and increased beneficial members of the family Lachnospiraceae (Shang et al., 2018). Additionally, FOS and MOS leads to proliferation of beneficial groups of bacteria, such as Lactobacillus and Bifidobacterium, and reduction of Salmonella and Escherichia coli (Sims et al., 2004; Brümmer et al., 2010). Gram-negative bacteria, such as Salmonella and E. coli, bind to MOS through the fimbriae instead of binding to epithelial cells, which carries the bacteria with the excreta (Adhikari and Kim, 2017; Adhikari et al., 2018).
On the other hand, FOS and MOS are cited in the literature as feed additives able to improve gut health by their action on the immune-response in the gut-associated lymphoid tissue (GALT). Part of this action is mediated directly by the interaction between prebiotics and immune cells as well as due to the effect of prebiotics on the microbiota (Adhikari and Kim, 2017). MOS can modulate the intestinal immune system by the stimulation of the mucosal immunity as they may act as non-pathogenic microbial antigens (Davis et al., 2004), and act as adjuvants due to the presence of mannose receptors on the epithelial surface, and therefore involved in the antigenic recognition (Engering et al., 1997; Ferket et al., 2002). Adhikari et al. (2018) conducted a broad study looking at the effects of FOS in layers challenged with Salmonella Enteritidis and observed that FOS reduced Salmonella in the fecal excreta and lowered the colonization in the ovaries. Yet, FOS upregulated TLR-4, and increased IgA positive cells in the ileal mucosa (Adhikari et al., 2018).
Therefore, it was hypothesized that AY supplementation would improve growth performance of broiler chickens by modulating the immune system of broiler chickens vaccinated against coccidiosis and modulating specific populations of bacteria in the gut. The aim of this study was to investigate the effects of supplementing diets with AY of Saccharomyces cerevisiae on the performance, intestinal microbiota, immune cells profile, and expression of intestinal immune-related genes of broiler chickens vaccinated against coccidiosis.
Materials and Methods
Birds, Housing, and Feeding
All the procedures used in this study were approved by the institutional animal care and use committee of the Luiz de Queiroz College of Agriculture, University of Sao Paulo. One-day-old male Ross 308 AP95 (1,260 birds) were used in the experiment. Chicks were weighed by pen for equal weight distribution and placed into 28 pens (45 birds/pen and 7 replicates/treatment), from 1 to 35 days, in a completely randomized design. The treatments were: basal diet—negative control (NC); basal diet supplemented with 55 ppm of zinc bacitracin—positive control (PC); NC + 0.2% of AY (SINERGIS®, Aleris, Jundiai, SP, Brazil); NC + 0.4% of AY. The nutritional program consisted of 3 diets: pre-starter (1–7 days), starter (7–21 days), and grower (21–35 days). For each phase a basal diet was formulated with corn, soybean meal, and the inclusion of 5% poultry by-products meal and 5% wheat bran (Table 1). The latter 2 ingredients were used with the purpose of imposing a challenge to the intestinal health. Ingredient composition and nutritional specifications followed Brazilian Tables (2011). Chickens had ad libitum access to water and feed in mash form during the entire experimental period.
On d 7, all the birds were eye-drop vaccinated with a coccidiosis vaccine (Bio-Coccivet R/Biovet) containing 7 Eimeria species: E. acervulina; E. brunetti; E. maxima; E. necatrix; E. praecox; E. tenella; and E. mitis using the dose recommended by the manufacturer. Chickens and feed were weighed weekly to determine body weight gain (BW gain), feed intake (FI), and feed conversion ratio (FCR).
Sample Collection
At 8 and 21 days of age, one bird per pen (7 per treatment) was selected for blood collection and was then euthanized by cervical dislocation to collect ileal and cecal digesta for microbial analysis. Additionally, a sample of ileum was collected for gene expression analysis.
Bacterial Enumeration of the Ileal and Cecal Digesta
Samples of the contents of the proximal ileum and cecum were aseptically collected and stored into 15 mL tubes and kept under 4°C for 24 h until analysis. One gram of sample was used and submitted to serial dilutions with saline solution (0.85%) and plated on a medium specific for each bacterial group. The plates were then incubated at 37°C for 24 h. Enterococcus sp., E. coli, and Lactobacillus sp. were enumerated using Mueller-Hinton, Levine EMB, and MRS agar, respectively.
Flow Cytometry Analysis
Blood (2 mL) was collected from one bird per pen on d 8 and 21 using heparinized syringes (Blausiegel, SP, Brazil), and placed into heparinized tubes (Becton, Dickinson and Company, NJ, USA). The samples were then stored at 4°C until analysis. The antibodies were acquired from Southern Biotechnology Associates (USA). The mouse anti-chicken CD45 antibody was used to separate the leukocytes from the total blood. The antibodies used to identify the superficial molecules are described in Table 2. Blood samples were treated according to Stabel et al. (2000) with a slight modification. In short, 50 μL of blood was incubated for 30 min at 37°C with the specific antibody and fixed with paraformaldehyde for 30 min at 4°C, and then put into the cytometry tubes. The flow cytometry analysis was done using FACSCalibur® flow (Becton, Dickinson and Company, NJ, USA).
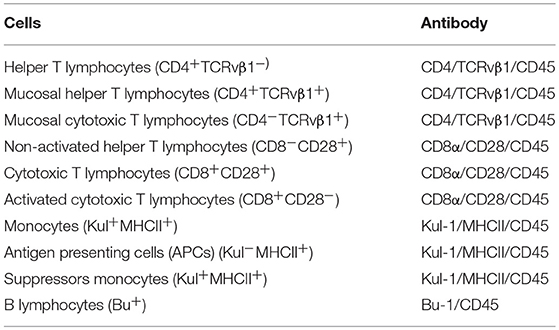
Table 2. Specific monoclonal antibodies used in the flow cytometry analysis with their target cell population.
Gene Expression Analysis
Ileal samples from one bird per pen were collected to analyze the expression of IL-1β, IL-4, TLR-4, Claudin-1, and MUC2 genes. The sample preparation for real time PCR was done in accordance with Horn et al. (2014). RNA was isolated using TRIzol® reagent (Invitrogen, Carlsbad, CA, USA). The quantity and quality of RNA was assessed using spectrophotometry. The complementary DNA (cDNA) was synthesized from 5 μL of total RNA using oligodT primers and reverse transcriptase (Invitrogen, China). The cDNA was then diluted in distilled water and stored at −20°C for further analysis. The primers used are shown in Table 3. The PCR analysis was performed with a total solution of 25 μL, containing 5 μL of cDNA, 12.5 μL of SYBR Green PCR Master Mix (Roche, Switzerland), 2.5 μL of each primer, and 3 μL of molecular water. The PCR reaction consisted of 95°C for 10 m, followed by 40 cycles of 95°C for 15 s, and 55, 48, 50, 51, and 52°C for IL-1β, IL-4, TLR-4, Claudin-1 e MUC-2, respectively. The relative expression was calculated according to Pfaffl (2001). B-actin was used as the endogenous control gene.
Statistical Analysis
The data were tested for normality and homogeneity of variances using PROC UNIVARIATE and Bartlett test of SAS (2012). The bacterial enumeration data were transformed to log10 and converted from CFU/μL to CFU/mL. The flow cytometry data were submitted to inverse or logarithmic transformation when necessary. For performance data, the pen was considered as the experimental unit, and for bacterial count, gene expression, and flow cytometry analysis the single birds from each pen was considered the experimental unit. The model included the effects of the four dietary treatments. All the data were submitted to one-way ANOVA (P ≤ 0.05) and in case of significance the means were separated using Tukey's test using SAS (2012).
Results and Discussion
In the present study, it was evaluated the effects of supplementing AY as an alternative to zinc bacitracin in diets of broiler chickens and their effects on the growth performance, immune system and some specific groups of intestinal bacteria. In order to impose a higher sanitary challenge, the diets were formulated with 5% inclusion of poultry by-products meal and wheat bran, and vaccination against coccidiosis. The cumulative performance data is shown in Table 4. No differences among treatments was observed in the first 2 weeks of the trial. From d 1 to 21, however, it was observed that the FCR was improved in chickens supplemented with 0.2% of AY when compared to the birds in the negative control group (P = 0.02). Additionally, in the period from 1 to 35 days it was observed that the FCR was better in chickens supplemented with 0.4% of AY or zinc bacitracin compared to birds in the negative control treatment (P = 0.02). No differences were observed for BW gain or FI among treatments. From d 21 to 35 (Table 4), it was observed that BW gain of chickens supplemented with 0.4% of AY was better (P = 0.03) than birds supplemented with 0.2% of AY. Yet, FCR of birds fed 0.4% of AY was better (P = 0.04) than birds supplemented with 0.2% of AY or the unsupplemented birds (negative control).
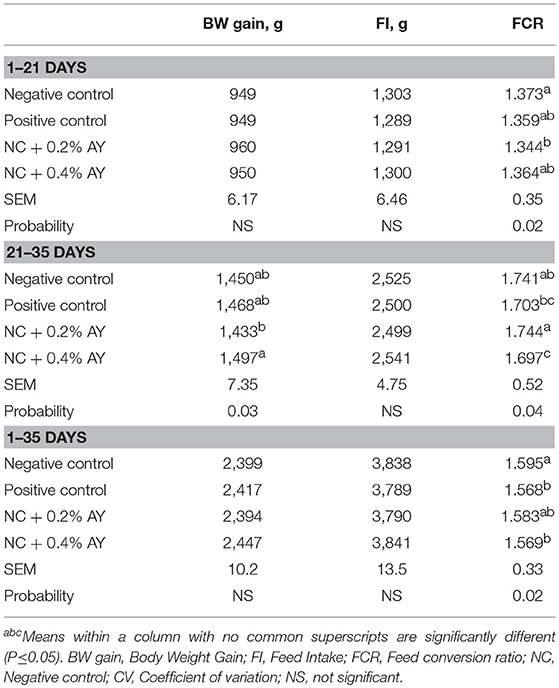
Table 4. Growth performance of broiler chickens supplemented with zinc bacitracin or autolyzed yeast of Saccharomyces cerevisiae.
Studies have demonstrated the potential use of yeast cell components in the diets of broiler chickens (Zhang et al., 2005; Gao et al., 2008; Shang et al., 2015; Alizadeh et al., 2016). Gao et al. (2008) supplemented AY to broiler chickens and did not find difference in the growth performance from d 1 to 21. However, in the grower period (21–42 days) and in the cumulative period (1–42 days), chickens supplemented with AY had better BW gain and FCR compared to non-supplemented birds. The findings in the present study agree with Gao et al. (2008) as the supplementation of AY improved the growth performance in the grower phase and consequently from 1 to 35 days. The effect of AY in improving performance in the grower period only, suggests that the components of yeast (MOS, β-glucans, and others) have their effects augmented during intense immune activation or extended challenge exposition. Another explanation may be related to the maturation of the intestinal microbiota in older birds which would result in higher metabolic activity and higher production of short-chain fatty acids (SCFA) stimulated by prebiotics. Production of SCFA has been cited as one of the mechanisms of action by which prebiotics improve growth performance (Chapman et al., 1994), as it may be used as energy source by the tissues and increase partition of nutrients in the body of an animal (Lu et al., 2012; Ajuwon, 2015).
Regarding the bacteria enumeration analysis (Table 5), it was observed that the supplementation of 0.4% of AY decreased the number of Enterococcus in the ileum of chickens at d 8 vs. birds receiving 0.2% of AY, and both groups fed AY had decreased Lactobacillus vs. birds fed zinc bacitracin. In the cecum, it was observed that birds fed 0.2% of AY had lower E. coli count compared to birds fed zinc bacitracin. On d 21, however, the only difference was observed in the ileum wherein zinc bacitracin supplementation reduced Enterococcus compared to non-supplemented birds (negative control). Prebiotics cannot be digested or absorbed by the GIT but rather used as food source by the beneficial bacteria such as Lactobacillus and Bifidobacterium in the lower intestine (Adhikari and Kim, 2017). Therefore, one can presume that Lactobacillus counts would increase with dietary supplementation of prebiotics; however, the AY used herein led to a decrease in the counts of Lactobacillus in the ileum. Even though this result may suggest that AY may have reduced Lactobacillus species that are linked with poor performance in chickens (Torok et al., 2011), one should keep in mind that enumeration techniques only take into consideration the bacterial group under study. Next-generation sequencing analysis would be more reliable to study the changes in the intestinal microbial populations of chickens supplemented with different feed additives and under challenge conditions.
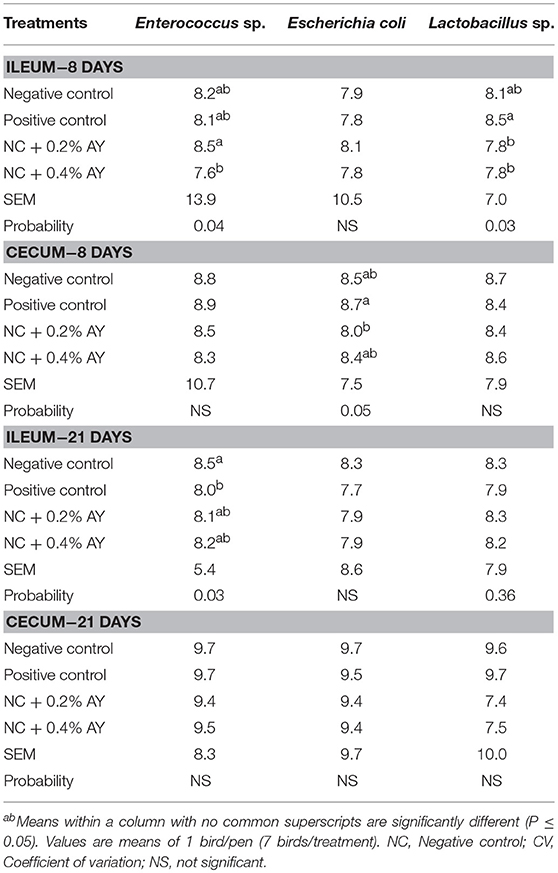
Table 5. Bacterial enumeration (log10 CFU/g) from the ileal and cecal digesta of broiler chickens supplemented with zinc bacitracin or autolyzed yeast of Saccharomyces cerevisiae.
There are numerous GIT bacteria that can metabolize prebiotics (Ricke, 2018). Due to their chemical nature, prebiotics with FOS are more likely to be fermented by the bacteria in the gut, while MOS can reduce pathogen colonization by binding to the flagella of the microorganism such as E. coli and Salmonella (Ricke, 2018). This action would reduce their attachment to the epithelial cells of the intestine (Adhikari et al., 2018) and therefore, promote their elimination through excreta. Adhikari et al. (2018) showed that FOS supplemented to layers challenged with Salmonella Enteritidis reduced Salmonella shedding and lowered ovary colonization by the bacteria. As FOS are fermented by beneficial bacteria in the intestine the production of SCFA and lactate increases (Ricke, 2018). A hypothesis has been proposed that lactic acid bacteria can tolerate lower pH values, but pathogens such as E. coli cannot (Russell, 1992).
In order to stimulate the immune system, the chickens were vaccinated against coccidiosis on d 7, besides being fed a diet with 5% inclusion of poultry by-product meal and wheat bran. This challenge model has been used before by our group (Bortoluzzi et al., 2014). The development of immunity was not evaluated in this previous study, even though the model was beneficial in causing impairment in the growth performance of the birds. In the present study, flow cytometry analysis and expression of immune-related genes was conducted to assist in the understanding of the mechanisms of action performed by AY. No statistical difference was observed in the flow cytometry analysis (Table 6). However, on d 8 a trend (P = 0.12) toward lower number of B lymphocytes with supplementation of 0.2% of AY, and lower number (P = 0.16) of helper T cells with both concentrations of AY was verified. On d 21, a trend (P = 0.19) toward decreased number of activated T cytotoxic lymphocytes was observed in chickens supplemented with both concentrations of AY. The tendency of lower number of these cells in the blood due to the supplementation of AY may be a reflect of their movement to the lamina propria of the intestine (Beirão et al., 2012) and an improved local immunity.
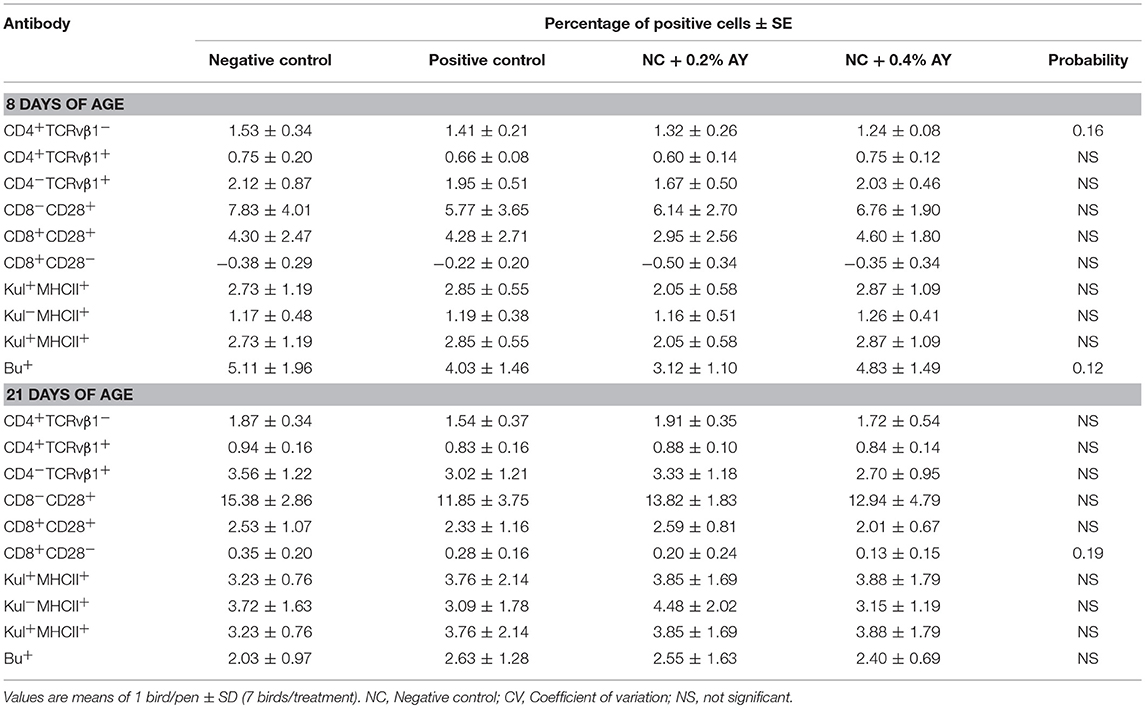
Table 6. Percentages of identified subpopulations of circulating blood cells of broiler chickens supplemented with zinc bacitracin or autolyzed yeast of Saccharomyces cerevisiae.
In fact, MOS and β-glucans can increase the movement of cytotoxic T lymphocytes to the intestine which will be the main group of cells responsible for the last phase of coccidia oocysts replication in the intestine. Shanmugasundaram and Selvaraj (2012) looked at the effects of prebiotics supplementation in broiler chickens in the absence of challenge and verified that the total T CD4+ and T CD8+ cells in the cecal tonsils did not change according to the treatments. On the other hand, Gao et al. (2009) challenged broilers with E. tenella and observed that the supplementation with AY increased T CD4+ and T CD8+ cells in the peripheral blood, and intraepithelial lymphocytes and IgA in the cecal tonsils. Therefore, supplementation of prebiotics shows to be beneficial in modulating the intestinal immune-system and helping the animal to cope with an infection.
Although histology and mechanisms of migration of cells from blood to the intestine were not evaluated in the present study, the changes in the cellular profile in the lamina propria of the intestine can lead to changes in the production of cytokines and other molecules. Studies have confirmed that yeast products can stimulate the innate immune-system by modulating the expression of Toll Like Receptors (TLR) and cytokines (Yitbarek et al., 2013). In the present study, there was an effect on the expression of TLR-4 (P = 0.04) on d 8, wherein the supplementation of zinc bacitracin upregulated its expression in 4-folds when compared to the other treatments (Figure 1). Also, the expression of MUC2 tended to be higher (P = 0.09) in birds supplemented with 0.4% of AY compared to the other groups. The expression of Claudin-1, IL-1β, and IL-4 did not differ among treatments on day 8. Similar to the finding observed herein, Munyaka et al. (2012) reported that the supplementation of MOS downregulated the expression of TLR-4 in the ileum of broilers. Yeast cell products may act downregulating the TLR-4 which will avoid the overstimulation of the immune system through the production of pro-inflammatory cytokines as shown by Munyaka et al. (2012). Yet, Tan et al. (2014) have demonstrated that the anti-inflammatory effect of some nutrients relies on downregulating the expression of TLR-4.
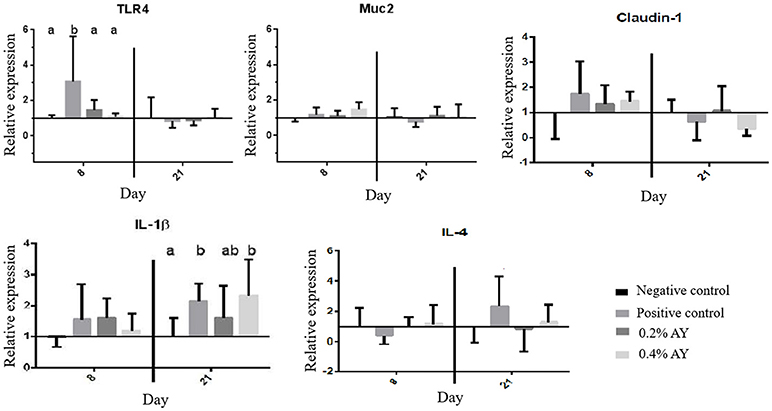
Figure 1. Relative gene expression of TLR-4, MUC2, Claudin-1, IL-1β, and IL-4 in the ileum of broiler chickens supplemented with zinc bacitracin or autolyzed yeast of Saccharomyces cerevisiae. Values are means ± SEM (1 bird/pen; 7 birds/treatment). abMeans with different superscripts in a column differ significantly (P ≤ 0.05).
On d 21, supplementation of zinc bacitracin and 0.4% of AY upregulated (P = 0.01) the expression of IL-1β compared to the negative control group, and the supplementation of zinc bacitracin tended to upregulate the expression of IL-4 compared to the other treatments. No differences were observed for the expression of TLR-4, MUC2, and Claudin-1. The upregulation of IL-1β by AY may be responsible for the intestinal recruitment of lymphocytes as it can be correlated with the lower T cytotoxic cells in the blood. Nevertheless, previous studies have shown that products derived from yeast cell wall downregulate the transcription of pro-inflammatory cytokines such as IL-1β, IFN-γ, and IL-12 (Shanmugasundaram and Selvaraj, 2012; Alizadeh et al., 2016).
Conclusion
Supplementation of 0.4% of AY of Saccharomyces cerevisiae in the diets of broiler chickens improved FCR in the grower phase, and consequently in the overall experimental period to the same level as zinc bacitracin. Additionally, both concentrations of AY distinctly modulated the counts of specific microbial groups without significantly changing the total number of immune cell in blood as measured by flow cytometry analysis.
The non-activation of transcription of TLR-4 by AY and upregulation of IL-1β by the supplementation of 0.4% of AY show that AY molecules work through different pathways, but in general have beneficial effects on the host.
Antimicrobial growth promoters have multiple mechanisms of action, and nutritionists have been looking for replacements of these molecules; however, it is unlikely that only one product will bring the desirable effects as a whole to poultry flocks. Therefore, further studies must test different combinations of feed additives and nutrients to help the poultry industry to face its current challenges.
Author Contributions
CB, JB, and JM were responsible for the experimental design. JM performed the animal trial and laboratory analysis. RP, NF, and JR helped with the animal trial. CB wrote the manuscript. All the authors read and approved the last version of the manuscript.
Funding
The study was financially supported by Aleris Brazil.
Conflict of Interest Statement
The authors declare that the research was conducted in the absence of any commercial or financial relationships that could be construed as a potential conflict of interest.
References
Adhikari, P. A., Cosby, D. E., Cox, N. A., Franca, M. S. S., Williams, M., Gogal, R. M. Jr, et al. (2018). Effect of dietary fructooligosaccharides supplementation on internal organs Salmonella colonization, immune response, ileal morphology, and ileal immunohistochemistry inlaying hens challenged with Salmonella Enteritidis. Poult. Sci. 97, 2525–2533. doi: 10.3382/ps/pey101
Adhikari, P. A., and Kim, W.K. (2017). Overview of prebiotics and probiotics: focus on performance, gut health and immunity–a review. Ann. Anim. Sci. 4, 949–966. doi: 10.1515/aoas-2016-0092
Ajuwon, K.M. (2015). Toward a better understanding of mechanisms of probiotics and prebiotics action in poultry species. J. Appl. Poult. Res. 25, 277–283. doi: 10.3382/japr/pfv074
Alizadeh, M., Rodriguez-Lecompte, J. C., Rogiewicz, A., Patterson, R., and Slominski, B. A. (2016). Effect of yeast-derived products and distillers dried grains with solubles (DDGS) on growth performance, gut morphology, and gene expression of pattern recognition receptors and cytokines in broiler chickens. Poult. Sci. 95, 507–517. doi: 10.3382/ps/pev362
Beirão, B. C., Fávaro, C., Nakao, L. S., Caron, L. F., Zanata, S. M., and Mercadante, A. F. (2012). Flow cytometric immune profiling of specific-pathogen-free chickens before and after infectious challenges. Vet. Immunol. Immunopathol. 145, 32–41. doi: 10.1016/j.vetimm.2011.10.004
Bortoluzzi, C., Menten, J. F. M., Romano, G. G., Pereira, R., and Napty, G.S. (2014). Effect of hops beta-acids (Humulus lupulus) on the performance and intestinal health of broiler chickens. J. Appl. Poult. Res. 23, 437–443. doi: 10.3382/japr.2013-00926
Brazilian Tables (2011). To Poultry and Swine: Feed Composition and Nutritional Requirements, 3th Edn. Viçosa: Horacio Santiago Rostagno.
Brümmer, M., Jansen Van Rensburg, C., and Moran, C.A. (2010). Saccharomyces cerevisiae cell wall products: the effects on gut morphology and performance of broiler chickens. S. Afr. J. Anim. Sci. 40, 14–21. doi: 10.4314/sajas.v40i1.54125
Carver, J.D., and Walker, W.A. (1995). The role of nucleotides in human nutrition. J. Nutr. Biochem. 6, 58–72. doi: 10.1016/0955-2863(94)00019-I
Chapman, M. A., Grahn, M. F., Boyle, M. F., Hutton, M. A., Rogers, J., and Williams, N. S. (1994). Butyrate oxidation is impaired in the colonic mucosa of sufferers of quiescent ulcerative colitis. Gut 35, 73–76. doi: 10.1136/gut.35.1.73
Cox, C. M., Sumners, L. H., Kim, S., Mcelroy, A. P., Bedford, M. R., and Dalloul, R. A. (2010). Immune responses to dietary β-glucan in broiler chicks during an Eimeria challenge. Poult. Sci. 89, 2597–2607. doi: 10.3382/ps.2010-00987
Davis, M. E., Maxwell, C. V., Erf, G. F., Brown, D. C., and Wistuba, T. J. (2004). Dietary supplementation with phosphorylated mannans improves growth response and modulates immune function of weanling pigs. J. Anim. Sci. 82, 1882–1891. doi: 10.2527/2004.8261882x
Engering, A. J., Cella, M., Fluitsma, D., Brockhaus, M., Hoefsmit, E. C., Lanzavecchia, A., et al. (1997). The mannose receptor functions as a high capacity and broad specificity antigen receptor in human dendritic cells. Eur. J. Immunol. 27, 2417–2425. doi: 10.1002/eji.1830270941
Ferket, P. R., Parks, C. W., and Grimes, J. L. (2002). “Benefits of dietary antibiotic and mannoligosaccharide supplementation for poultry,” in Multi-State Poultry Feeding and Nutrition Conference, 2002. Proceeding of Multi-State Poultry Feeding and Nutrition Conference. (Indianapolis, IN) 1–22.
Gao, J., Zhang, H. J., Wu, S. G., Yu, S. H., Yoon, I., Moore, D., et al. (2009). Effect of Saccharomyces cerevisiae fermentation product on immune functions of broilers challenged with Eimeria tenella. Poult. Sci. 88, 2141–2151. doi: 10.3382/ps.2009-00151
Gao, J., Zhang, H. J., Yu, S. H., Wu, S. G., Yoon, I., Quigley, J., et al. (2008). Effects of yeast culture in broiler diets on performance and immunomodulatory functions. Poult. Sci. 87, 377–1384. doi: 10.3382/ps.2007-00418
Hoelzer, K., Bielke, L., Blake, D. P., Cox, E., Cutting, S. M., Devriendt, B., et al. (2018). Vaccines as alternatives to antibiotics for food producing animals. Part 1: challenges and needs. Vet. Res. 49:64. doi: 10.1186/s13567-018-0560-8
Horn, N., Ruch, F., Miller, G., Ajuwon, K. M., and Adeola, O. (2014). Impact of acute water and feed deprivation events on growth performance, intestinal characteristics, and serum stress markers in weaned pigs. J. Anim. Sci. 92, 4407–4416. doi: 10.2527/jas.2014-7673
Jaehrig, S. C., Rohn, S., Kroh, L. W., Wildenauer, F. X., Lisdat, F., Fleischer, L. G., et al. (2008). Antioxidative activity of (1 → 3), (1 → 6)-β-D-glucan from Saccharomyces cerevisiae grown on different media. Food Sci. Technol. 41, 868–877. doi: 10.1016/j.lwt.2007.06.004
Lu, H., Su, S., and Ajuwon, K. M. (2012). Butyrate supplementation to gestating sows and piglets induces muscle and adipose tissue oxidative genes and improves growth performance. J. Anim. Sci. 90(Suppl. 4), 430–432. doi: 10.2527/jas.53817
Mateo, E. D., Dave, R. I., and Stein, H. H. (2004). Effect of supplemental nucleosides for newly weaned pigs. J. Anim. Sci. 82(Suppl. 2):71.
Munyaka, P. M., Echeverry, H., Yitbarek, A., Camelo-Jaimes, G., Sharif, S., Guenter, W., et al. (2012). Local and systemic innate immunity in broiler chickens supplemented with yeast-derived carbohydrates. Poult. Sci. 91, 2164–2172. doi: 10.3382/ps.2012-02306
Newbold, C. J., Wallace, R. J., Chen, X. B., and McIntosh, F. M. (1995). Different strains of Saccharomyces cerevisiae differ in their effects on ruminal bacterial numbers in vitro and in sheep. J. Anim. Sci. 73, 1811–1818. doi: 10.2527/1995.7361811x
Pfaffl, M. W. (2001). A new mathematical model for relative quantification in real-time RT-PCR. Nucleics Acids Res. 29, 2003–2007. doi: 10.1093/nar/29.9.e45
Reed, G., and Nagodawithana, T. W. (1991). Yeast Technology, 2nd Edn. New York, NY:Van Nostrand Reinhold.
Ricke, S. C. (2018). Impact of prebiotics on poultry production and food safety. Yale J. Biol. Med. 91, 151–159.
Russell, J. B. (1992). Another explanation for the toxicity of fermentation acids at low pH: anion accumulation versus uncoupling. J. Appl. Bacteriol. 73, 363–370. doi: 10.1111/j.1365-2672.1992.tb04990.x
Shang, Y., Kumar, S., Thippareddi, H., and Kim, W. K. (2018). Effect of dietary fructooligosaccharide (FOS) supplementation on ileal microbiota in broiler chickens. Poult. Sci. 97, 3622–3634. doi: 10.3382/ps/pey131
Shang, Y., Regassa, A., Kim, J. H., and Kim, W. K. (2015). The effect of dietary fructooligosaccharides supplementation on growth performance, intestinal morphology, and immune response in broiler chickens challenged with Salmonella Enteritidis lipopolysaccharides. Poult. Sci. 94, 2887–2897. doi: 10.3382/ps/pev275
Shanmugasundaram, R., and Selvaraj, R. K. (2012). Effect of killed whole yeast cell prebiotic supplementation on broiler performance and intestinal immune cell parameters. Poult. Sci. 91, 107–111. doi: 10.3382/ps.2011-01732
Shao, Y., Guo, Y., and Wang, Z. (2013). β-1,3/1,6-Glucan alleviated intestinal mucosal barrier impairment of broiler chickens challenged with Salmonella enterica serovar typhimurium. Poult. Sci. 92, 1764–1773. doi: 10.3382/ps.2013-03029
Sims, M. D., Dawson, K. A., Newman, K. E., Spring, P., and Hoogell, D. M. (2004). Effects of dietary mannan oligosaccharide, bacitracin methylene disalicylate, or both on the live performance and intestinal microbiology of turkeys. Poult. Sci. 83, 1148–1154. doi: 10.1093/ps/83.7.1148
Sommer, R. (1996). “Yeast extract: production, properties and components,” in 9th International Symposium of Yeast. Procedings of 9th International Symposium of Yeast (Sydney, NSW).
Stabel, T. J., Bolin, S. R., Pesch, B. A., and Rahner, T. E. (2000). A simple and rapid flow cytometric method for detection of porcine cell surface markers. J. Immunol. Methods. 245, 147–152. doi: 10.1016/S0022-1759(00)00289-1
Suresh, G., Das, R. K., Kaur Brar, S., Rouissi, T., Ramirez, A. A., Chorfi, Y., et al. (2018). Alternatives to antibiotics in poultry feed: molecular perspectives. Crit. Rev. Microbiol. 44, 318–335. doi: 10.1080/1040841X.2017.1373062
Tan, J., Liu, S., Guo, Y., Applegate, T. J., and Eicher, S. D. (2014). Dietary L-arginine supplementation attenuates lipopolysaccharide induced inflammatory response in broiler chickens. Br. J. Nutr. 111, 1394–1404. doi: 10.1017/S0007114513003863
Torok, V. A., Hughes, R. J., Mikkelsen, L. L., Perez-Maldonado, R., Balding, K., McAlpine, R., et al. (2011). Identification and characterization of potential performance-related gut microbiota in broilers chickens across various feeding trials. App. Environ. Microbiol. 77, 5868–5878. doi: 10.1128/AEM.00165-11
Uauy, R. (1994). Nonimmune system responses to dietary nucleotides. J. Nutr. 124, 1575–1585. doi: 10.1093/jn/124.suppl_1.157S
Wallace, R. J. (1994). Ruminal microbiology, biotechnology and ruminant nutrition: progress and problems. J. Anim. Sci. 72, 2992–3003. doi: 10.2527/1994.72112992x
Yitbarek, A., Rodriguez-Lecompte, J. C., Echeverry, H. M., Munyaka, P., Barjesteh, N., Sharif, S., et al. (2013). Performance, histomorphology, and Toll-like receptor, chemokine, and cytokine profile locally and systemically in broiler chickens fed diets supplemented with yeast-derived macromolecules. Poult. Sci. 92, 2299–2310. doi: 10.3382/ps.2013-03141
Keywords: autolyzed yeast, broilers, coccidiosis, intestinal immunity, Saccharomyces cerevisiae
Citation: Bortoluzzi C, Barbosa JGM, Pereira R, Fagundes NS, Rafael JM and Menten JFM (2018) Autolyzed Yeast (Saccharomyces cerevisiae) Supplementation Improves Performance While Modulating the Intestinal Immune-System and Microbiology of Broiler Chickens. Front. Sustain. Food Syst. 2:85. doi: 10.3389/fsufs.2018.00085
Received: 10 September 2018; Accepted: 21 November 2018;
Published: 05 December 2018.
Edited by:
Michael J. Rothrock, United States Department of Agriculture, United StatesReviewed by:
Elizabeth Santin, Universidade Federal do Paraná, BrazilRicardo Mitsuo Hayashi, Universidade Federal do Paraná, Brazil
Copyright © 2018 Bortoluzzi, Barbosa, Pereira, Fagundes, Rafael and Menten. This is an open-access article distributed under the terms of the Creative Commons Attribution License (CC BY). The use, distribution or reproduction in other forums is permitted, provided the original author(s) and the copyright owner(s) are credited and that the original publication in this journal is cited, in accordance with accepted academic practice. No use, distribution or reproduction is permitted which does not comply with these terms.
*Correspondence: José Fernando Machado Menten, amZtZW50ZW5AdXNwLmJy
†These authors have contributed equally for this publication