- 1Department of Plant Biology and Ecology, University of the Basque Country UPV/EHU, Bilbao, Spain
- 2Department of Conservation of Natural Resources, NEIKER-Tecnalia, Derio, Spain
Nowadays, soil functionality and productivity are severely impaired due, in great part, to the fact that most of the land is being intensively used for food production and urbanization purposes, with a consequent rise in the generation of wastes and consumption/degradation of fertile soils. These issues can be jointly addressed by an integrated and sustainable management of the soil resource carried out in the framework of two recent paradigms: circular economy and phytomanagement. Within the fields of resource conservation and regenerative (urban) land management, at least the three following fundamental aspects can be contemplated: (i) the valorization and safe recovery of organic wastes (e.g., composts, green and animal manures, and biosolids) as soil amendments (from residue to resource); (ii) the re-development and revegetation of degraded soils characterized by low organic matter content, deficient physical structure and depressed biological status (from bare to vegetated soil); and, finally, (iii) the promotion of soil health in order to support soil ecological processes, functions and concomitant ecosystem services (integration of ecocentric and anthropocentric perspectives). Here, we discuss the benefits (knowns), potential risks (known unknowns) and future/affordable uncertainties (unknowns) resulting from the application of organic amendments (OAs) to soil. Traditionally, most studies have used physical-chemical parameters to assess soil health/quality, whereas less attention has been paid to soil biological criteria. Thus, we highlight the relevance of soil biological properties as key drivers of ecological restoration and suitable indicators of soil health. Notwithstanding, special attention should be paid to “amendments-plants-microorganisms” interactions in different soils and field conditions. Finally, the risk of introduction of emerging contaminants, including for instance microplastics and antibiotic resistance genes (ARGs), through the application of OAs to soil, is currently a matter of much concern that must urgently be addressed if we are to continue with such practice.
Phytomanagement Strategies for the “Soft re-use” of Soils: Promising Approach Into the Circular Economy Paradigm
According to global demographic projections, by the year 2050 the world's population will reach, at least, nine billion people. This fact, together with the current scenarios of accelerated urbanization and climate change, points out to a major challenge for our present society: how to increase food and energy production whilst preserving ecosystem health. Fortunately, there is increasing recognition that the protection of soil health is critical to the stability of the environmental, economic and social spheres (Jones et al., 2012; Glæsner et al., 2014). In particular, we must learn how to sustainably manage the soil resource so that it keeps on providing an essential suite of ecosystem functions (e.g., provisioning, regulating, habitat, information) and services (e.g., food production, organic matter decomposition, nutrient cycling, water regulation, control of pests and diseases, cultural heritage, etc.). Hereinafter, we will use the term “soil health” to refer to the continued capacity of soil to function as a vital living system and the closely connected term “soil quality” to indicate the fitness of soil for a specific land-use, such as plant growth (Gómez-Sagasti et al., 2012; Laishram et al., 2012).
The so-called “Green Revolution” entailed the modernization of traditional agriculture by introducing mechanization, irrigation, genetically improved high-yield grain varieties, and synthetic agrochemicals, in order to increase crop productivity. However, the overuse of water and soil resources and the indiscriminate inputs of agrochemicals have undoubtedly taken a toll on the health of agricultural soils. Within this context, the regenerative and resource-conserving agriculture pursues the integration of high crop production with the sustainable management of the soil resource (i.e., the protection of its physical, chemical, and biological properties), while minimizing the use of external and non-renewable inputs.
Understood as a strategic non-renewable resource at human scale, soils constitute dynamic, complex, and multi-functional ecosystems that lie at the interface between the atmosphere, hydrosphere, lithosphere and biosphere. Erosion, compaction, sealing, salinization, contamination, loss of soil of biodiversity, floods, landslides, and decline of soil organic matter (SOM) have been identified as the main threats that lead to soil degradation (Food and Agriculture Organization of the United Nations (FAO), 2015; Karlen and Rice, 2015). It comes, therefore, at no surprise that the European Union recognizes soil degradation as a “serious problem” and emphasizes the urgent need for a more holistic approach to soil protection (Paleari, 2017). In 2006, the European Commission presented the “Thematic Strategy for Soil Protection” (COM(2012)46), which included a proposal for a Soil Framework Directive and Impact Assessment. However, in 2014, such proposal was rejected. Without exclusive soil legislation, nowadays, the legal protection of soils is integrated into the already developed European Directives (e.g., EU Directive on Environmental Liability 2004/35/CE). There is a tight linkage among specific soil threats, soil multi-functionality and ecosystem services (Bünemann et al., 2018), but this perspective has rarely been implemented for the assessment of soil health/quality.
In recent years, soil health/quality is severely threatened by the unwavering changes of land-use and depletion of SOM (Vimal et al., 2017). In fact, approximately 45% of European topsoils (0–30 cm) are characterized by low SOM content (<3.5%) (European Commission, 2006; Martínez-Blanco et al., 2013), and principally soils of Mediterranean regions are very susceptible to its loss, where nearly 75% of soils has a low (≤2%) or very low (≤1%) SOM content (Schreuder and De Visser, 2014), being most of them considered degraded soils. Degraded soils are those often arisen from abandonment of rural areas and agricultural practices (Benayas et al., 2007; Rodrigo-Comino et al., 2017), as well as from urban vacant and derelict lands usually located within the city limits (Németh and Langhorst, 2014; Kumar and Hundal, 2016). As these areas are frequently used as dumpsites, their soils are usually characterized by poor soil structure, low SOM (García et al., 2017) and, oftentimes by low contaminant levels. While recent attention has been largely focused on the reclamation of urban brownfields (i.e., highly contaminated and industrialized sites), few researches render attention to the vast potential of urban vacant and derelict sites as a resource for re-development and achievement of economic and social benefits (Kim et al., 2018) by, for instance, the application of phytomanagement practices.
At the same time as the scarcity of fertile soil is sharpening, the fast economic and demographic growth is leading to a massive generation of organic wastes derived from human activities, with their subsequent environmental impacts and management costs. Consequently, wastes in general and organic ones in particular are increasingly being viewed as potential resources. In fact, considering contemporary societal demands and increasing cost of agrochemicals (Thangarajan et al., 2013), a sustainable restoration of functionality and fertility of such degraded soils can be achieved through the application of organic amendments (OAs) derived from properly treated organic wastes such as composts, crops residues, animal manures, and biosolids (Aparna et al., 2014; Reeve et al., 2016).
The reutilization of organic wastes as soil conditioners/energy suppliers is an old but increasingly popular practice that not only helps to reduce agrochemicals dependence, but also represents an ecologically-sound, economically-attractive and socially-acceptable alternative to landfill disposal and incineration and, likewise, contributes toward the objectives of the EU's “Zero Waste” Policy, “End-Of-Waste” Policy, and “Circular Economy Strategy” (Saveyn and Eder, 2014). To combat soil degradation in a sustainable way, the Circular Economy (CE) concept has recently gained momentum in the debates on waste and resource management. Unlike open lineal models of production and consumption (“production-use-disposal”), CE promotes resource closed-loops, meaning that large volumes of finite resources (metals and minerals, among others), and surplus organic wastes are captured and reused, reducing the need for extraction of raw materials (Preston, 2012). In Europe, efforts are underway to comprehensive legislative breakthroughs along with actions for waste reduction, management, and recycling (Zuin, 2016), with a view to provide a more holistic approach of sustainability (Geissdoerfer et al., 2017). Although the application of OAs to soil has a long history as an economically-feasible and effective practice (Vimal et al., 2017), its known (eutrophication, presence of ecotoxic contaminants and pathogens, etc.) and emerging risks [microplastics and dissemination of plasmid-containing antibiotic resistance genes (ARGs), etc.], together with the OA-plant-microorganism tripartite interactions, need to be further investigated.
In this environmental scenario, the management and sustainable restoration of urban vacant lands and other degraded areas often require a different approach from that applied to natural or agricultural soils (De Lucia et al., 2013). In this regard, phytomanagement has emerged as a new paradigm that allows the re-use and re-development of degraded and/or contaminated sites. Phytomanagement is often touted as a suite of management strategies, based on gentle remediation options, which, alongside risk management, place realization of other benefits (including economic and social benefits) at the core of site design (Cundy et al., 2016). In phytomanagement, plant-based systems are used as a “holding strategy” while concurrently seeking for ecological (e.g., restoration of ecosystem functions and services), economical (e.g., biomass and energy generation, site value uplift of surroundings) and social benefits (e.g., urban climate management, ecosystem services, amenity, and leisure; Cundy et al., 2016). Phytomanagement usually encompasses a combination of biomass and energy crops (and their associated microorganisms), and the application of OAs for the recovery of underutilized lands (e.g., degraded and/or contaminated soils). Thus, phytomanagement generates a synchronous improvement of soil biological functions and ecosystem services (i.e., crop performance) through an effective recovery of land profitability.
Thus, the main goal of this review is to outline the potential profits and drawbacks conferred by recently used OAs for soil restoration as a routine phytomanagement practice. This work is organized as follows: first, a brief and current (2013–2018) literature review, that explores the positives and negatives impacts of the application of different OAs on the soil physico-chemical and biological properties, is presented, whereby the most relevant endpoint effects in plant and associated microorganisms are summarized. Later, greater attention is paid to emerging contaminants associated with organic amendments (concretely to microplastics and antibiotic-resistance genes) and their surrounding uncertainties.
Why Are Organic Amendments Recognized as Suitable Tools for Soil Restoration and Remediation?
Soil organic matter (SOM), composed of a mixture of animal, plant and microbial residues at different mineralization and humification stages, is often considered the most determinant natural soil component inasmuch as it governs, in the long-term, the physical, chemical, and biological dimensions of soil health (Hijbeek et al., 2017). There is widespread recognition that SOM prevents erosion by improving/stabilizing bulk structure, porosity, and water holding capacity, provides essential nutrients for (micro)biota (that, in turn, convert them to plant-available/absorbable forms; Reeve et al., 2016), stimulates soil microbial biomass and activity (Larkin, 2015), allows high biodiversity (Turmel et al., 2015), increases natural suppressiveness against soil-borne pathogens, and reduces pollutant ecotoxicity (Scotti et al., 2015). Correspondingly, healthy soils with a high organic matter content are expected to have more robust soil communities which may result in more resilient soils (Larkin, 2015). In this situation, plants receive better nutrition and produce better defense responses, and are in general less susceptible to infection by phytopathogens (Larkin, 2015). In this light, the restoration and maintenance of SOM content at an adequate level is a critical objective to safeguard soil ecosystem services (Scotti et al., 2015; Lal, 2016), as evidenced by some European policy documents (European Commission, 2011a,b) and international food security and climate objectives (UNFCCC, 2015).
Conservation of SOM can be improved by minimizing tillage, maintaining active crop growth (shorter fallow periods), and controlling erosion (Larkin, 2015). Organic matter can be added to soil through (i) in-field sources like crop residues, rotations and cover crops; and (ii) off-field sources such as composts, manures and biosolids (Larkin, 2015). Conversely, these latter practices need to be short-term balanced not only with economic objectives (i.e., profit maximization, labor use efficiency or minimization of gross margin variation; Hijbeek et al., 2018), but also with social and environmental objectives (i.e., soil health and nutritional security), all of them mutually interdependent (Geissdoerfer et al., 2017).
Overall, the basic benefits of the direct addition of organic matter to soil through OAs rely on: (i) the increase of the soil organic carbon (SOC) stock; (ii) the facilitation of growth and diversity of soil microbial communities; and (iii) the enhancement of available nutrients (N, P, K, Ca, and Mg), often due to the slightly acidic pH and also elevated soil cation exchange capacity (CEC), which in turn allows the chelation, supply and storage of essential nutrients, thereby affecting plant nutrient balance (Scotti et al., 2015; Reeve et al., 2016; Alvarenga et al., 2017). Even so, it must be noticed that, depending on the type and nature of the amendment and receiving soil, OAs could also raise soil pH and, therefore, decrease the bioavailability of both essential and ecotoxic metals, by shifting them to fractions of low availability associated with OM, carbonates or metal oxides (Bernal et al., 2007; Clemente et al., 2015). Organic amendment application is also reported to decrease soil crusting and bulk density, both essential properties of a healthy soil (Zhao et al., 2009; Thangarajan et al., 2013). Among other properties, OAs can maximize a stable C stock recovery and, at the same time, provide a continuous release of mineral nutrients, thus satisfying crop requirements (Scotti et al., 2015). Even though a large body of empirical studies carried out in different agricultural systems have demonstrated the benefits of OAs as substitutes of chemical fertilizers, a number of studies have also evidenced potential environmental risks associated to their improperly application/overreliance.
Given the wide array of OAs, we briefly summarize the specific influences of four major categories of OAs defined here (i.e., composts, crop residues and green manures, animal manures, and biosolids) on the physical, chemical, and/or biological properties of soils (Table 1). This classification results from the modification of that proposed by Goss et al. (2013). In this latter work, six categories of OAs were established: (1) composts; (2) food residues and wastes; (3) green manure and crop residues; (4) animal manures; (5) municipal biosolids; and (6) wastes from manufacturing processes. We have grouped them to four broader categories: (1) and (2) categories have been merged into what we called “composts,” since food residues and wastes are frequently composted for land application; and (5) and (6) categories have been clustered into what we called “biosolids” due the similarity of their final sludge treatments.
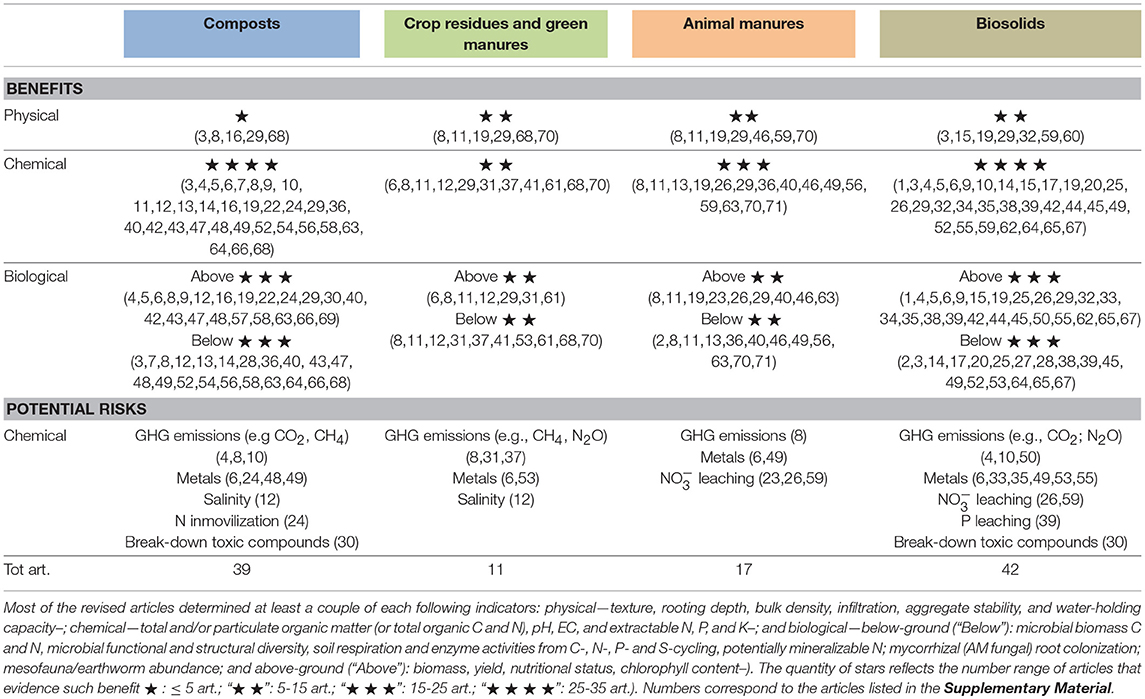
Table 1. Potential benefits and risks on the soil health dimensions (physical, chemical, and biological) resulted from the currently used organic amendments (2013–2018).
Composting represents the most common option to recover material from organic wastes (Cesaro et al., 2015). Overall, composts consist of stable mixtures of largely decayed organo-mineral material produced from food scraps, leaves, cattle manures or sewage sludges (also referred to as “biosolid composts”). The regulations and standards on compost quality vary considerably across European Member States, mainly due to differences in soil policies, as pointed out by Cesaro et al. (2015) and Bernal et al. (2017). Almost 50% of the total amount of compost produced in Europe is applied to agricultural soils (Saveyn and Eder, 2014). Beyond increasing the SOC content, compost amendments have been shown to increase soil microbial biomass and activity and modify the structural and functional diversity of the native microbial communities, which often are indirectly involved in the long-term suppression of soil-borne plant diseases such as those caused by Pythium, Phytophthora, and Fusarium spp. (Larkin, 2015; Scotti et al., 2015). Nevertheless, the high electrical conductivity (EC) or high C/N ratios of some compost may contribute to soil salinity or can enhance nitrogen immobilization, respectively (Scotti et al., 2015). Additionally, mainly in composted biosolids, heavy metals can also be incorporated to the soil system.
Despite of the plethora of research carried out for decades on composting, nowadays, an increased interest in its use as a sustainable alternative for the biocontrol of phytopathogens, as compared to the traditional use of synthetic fungicides (Meghvansi and Varma, 2015), has emerged. The increase in soil microbial biomass and activity provoked by compost application can lead to a general disease suppression (Larkin, 2015). Generally, microbiostasis (inhibition of growth, reproduction and multiplication of pathogens), hyperparasitism/predation and induction of systemic resistance in host plants are the principal mechanisms responsible for the development of soil suppressiveness (i.e., the capacity to reduce disease incidence caused by soil-borne phytopathogens; (Meghvansi and Varma, 2015). Supporting this, previous meta-analytical studies evidenced that just over half of composts resulted in significant disease suppression, with effects varying for different pathogens (Bonanomi et al., 2007; Termorshuizen et al., 2007). However, the variation and complex interactions between the (i) quality, maturity and decomposition time of organic matter, (ii) microbial community composition, (iii) phytosystem, and (iv) saprophytic ability of pathogen populations can lead to inconsistent results and hinder the identification of specific indicators of disease suppression (Bonanomi et al., 2010; Larkin, 2015). In this sense, it is noteworthy that microbiological properties (e.g., microbial biomass and enzymatic activities), rather than chemical ones, result better indicators of suppressiveness (Bonanomi et al., 2010).
Regarding crop residues and green manures (e.g., stalks and stubble, leaves, and seed pods), when incorporated by tillage or retained on soil surface, they (i) enhance assimilable nitrogen pool by the incorporation of reactive nitrogen compounds; (ii) retain soil moisture; (iii) deliver sizeable input of carbonaceous material, immobilizing some inorganic N present in the soil; and (iv) dramatically affect soil microbial communities and, subsequently, the development of disease-suppressive soils (Thangarajan et al., 2013; Larkin, 2015; Turmel et al., 2015).
Animal manures (mix of excrement and urine from domestic animals) and biosolids (treated and stabilized solid organic residual by-products derived from agro-industrial and municipal wastewater treatment process) have been recognized as valuable “nutrient suppliers” owing to their content of plant essential macro- and microelements, form soil aggregates, reduce bulk density, and increase porosity and water infiltration/percolation rate (Thangarajan et al., 2013; Larkin, 2015). Potential side-effects of animal manures and biosolids have been reported, such as: (i) high concentrations of N- and N- and elevated CO2 and CH4 emission from aerobic and anaerobic decomposition, which lead to greenhouse gas (GHG) and leachate emissions; (ii) input of metal(oid)s, like Pb, As, Cu, and Zn, organic contaminants, antibiotics and pathogenic microorganisms; and finally; (iii) OA application also could incur in an excessive input of nutrients (e.g., N and P run off; Martínez-Blanco et al., 2013; Thangarajan et al., 2013; Alvarenga et al., 2015; Scotti et al., 2015; García et al., 2017).
The intensity and durability of the above mentioned benefits and risks are regionally variable and site-specific (physico-chemical and biological soil properties) as they depend on (i) climatic and socioeconomic factors (Turmel et al., 2015); (ii) type and features of OA (stability, maturity and intrinsic biochemical quality); (iii) application design (dose, method and frequency); and (iv) cropping system. The combination of any (or all) these factors explains variable observations and prevents the retrieving of universal conclusions about OA application, making a meta-analytical approach imperative. Without claiming to be exhaustive in excess, Table 1 gathers compelling evidences of above-mentioned benefits and potential risks on soil-plant system. We conducted a literature search in Google Scholar (scholar.google.com) database using the keywords “organic,” “amendment,” “soil,” “plant,” and “health” (most recent search: June 6, 2018). Only original articles published in the last 5 years (2013–2018) were considered. For each identified research article, firstly we evaluated the title and abstract to determine whether physical or chemical and plant and/or microbial parameters were determined. Then, those articles that fitted all these criteria were examined in detail (total number of 71 articles; Supplementary Material).
Most of the studies carried out in the last 5 years were mainly field studies (with an average duration of 1.9 years) focused on the use of composts and biosolids (mainly biosolid composts) in agricultural soils, with the main objective of studying their effects on chemical properties and crop production. A large body of the studies reported the responses of chemical, plant, and microbial parameters (81.7, 70.4, and 60.6%, respectively) and only 18.3% of the examined studies measure physical properties. Although the influence of the native microbial communities of the rhizosphere on plant growth and crop yield is widely recognized, plant-microorganism interactions are still burdened by uncertainty, and this is certainly reflected in the low number of studies (only 32.4% of the papers) that collect data on both plant and microbial properties. We also noticed that none of the revised articles compiles information about the potential disturbances of microbial populations of OA on the biomass, activity, and diversity of the native soil microbiota.
It is generally assumed that the intrinsic microbial communities of the OA surrender to native microbial communities in the receiving soil with a minimal impact on them (Saison et al., 2006; Bastida et al., 2008). This is probably due to the short survival time of amendment-borne microorganisms (with exception of pathogenics) following land application (Holm et al., 2010). However, changes occurring in soil microbial community are usually associated to the chemical characteristics provided by the OA whereas the impact of OA-borne microorganisms (bacteria and fungi) are often overlooked, as recognized Tian et al. (2015), García et al. (2017), and Ren et al. (2017). Indeed, OAs can introduce changes in the biomass, activity and composition of the native microbial communities due to competition for available substrates and/or antagonisms (Ondoño et al., 2014) and the degree of contaminant removal (Ros et al., 2006; Masciandaro et al., 2013). In this regards, Galende et al. (2014b) observed that native microbial communities of Zn-Pb mine soil were not substantially affected by the following amendments: sheep manure, poultry litter, cow slurry, and paper mill sludge mixed with poultry litter. This could be related to the nature of the harsh environments of mine soils showing high levels of metals (Zn, Pb, and Cd), which would allow only the survival of native extremophile microorganisms. Tian et al. (2015) and Lloret et al. (2016) also concluded that the microorganisms associated to pig manure based compost and sewage sludge, respectively, did not significantly displace the native microbiota of agricultural soils. In contrast, Anastasi et al. (2009), Purnomo et al. (2010), and Lin et al. (2016) ascribe to compost-borne bacteria and fungi a relevant role in governing the biodegradation of organic compounds in soils.
The assessment and monitoring of environmental benefits and risks associated to organic management of degraded lands can be routinely carried out by a set of simple but robust indicators and minimum data set (see also Table 1). The most commonly used and accepted indicators that have meaning to both scientists and land managers include: (i) physical properties as texture, rooting depth, bulk density, infiltration, aggregate stability, and water-holding capacity; (ii) chemical properties as total and/or particulate organic matter (or total organic C and N), pH, EC, and extractable N, P, and K; and (iii) biological properties as microbial biomass C and N, microbial functional and structural diversity, soil respiration and enzyme activities, potentially mineralizable N, earthworm populations and plant bioassays (for more details see Larkin, 2015). In any case, usually the objective is to increase crop production and health.
Most of the studies on OA effects on plants focus on the analysis of total biomass production. However, for further understanding of soil health-plant interaction, it is necessary to explore other indicators of the potential phytotoxic effects and physiological status of plants. Notwithstanding, Table 1 evidence the knowledge gap regarding the short/long-term phytotoxicity and physiological status (e.g., net photosynthesis, transpiration, plant water potential, photochemical efficiency of PSII, pigment profiles and secondary metabolite content, etc.) of crops grown in organically managed soils. Seed and/or root elongation plant bioassays performed by direct exposure to soil matrix or indirectly to its leachates are highly recommended ecotoxicological tests for soil phytotoxic assessment, being also helpful for amendment screening and selection programs. Moreover, bioassays using rapid-cycling plants like dicotyledonous Cucumis sativus (cucumber) and Lactuca sativa (lettuce) (OPPTS 850.4100, 1996; OPPTS 850.4200, 1996; OECD Guidelines for Testing of Chemicals No. 208, 2006; ISO 11269-1, 2012; ISO 11269-2, 2012), allow measurement of multiple endpoints at biochemical (e.g., antioxidant content) and morphological level (e.g., survival and root elongation; Alvarenga et al., 2017).
Meanwhile, soil microbial properties (i.e., biomass, activity, functional, and structural diversity) have attracted great interest as quick, integrative, and sensitive indicators of soil perturbations (Epelde et al., 2010; Burges et al., 2015; Garaiyurrebaso et al., 2017) and can serve to identify the magnitude of the improvements brought about by management interventions (Aparna et al., 2014). These microbial indicators provide more direct insight into soil health/quality than traditional physical and chemical indicators alone (Ge et al., 2013). Extensive researches showed the ecological relevance of microbial communities of rhizosphere in the orchestration of energy and nutrient cycling (specially, fate and rate of mineralization of macronutrients), thereby acting as both nutrient source and sink (Thangarajan et al., 2013). Native microorganisms also directly influence plant health by altering physiological and development processes, facilitating nutrient and water uptake, and helping plants tolerate biotic and abiotic stress (Philippot et al., 2013; Reeve et al., 2016). Thus, progresses in the knowledge of ecological status of the microbial community within the soil are necessary to determine the long-term effects of changing soil quality (Su et al., 2014; García et al., 2017).
The current information on the effect of OAs on soil microbial properties indicates that microbial biomass, usually inferred by induced respiration, microbial biomass C (MBC) and phospholipid fatty acid (PFLA) measurements, and soil enzymes whose activities are related to the biochemical cycles of N (urease and protease), S (arylsulphatase), P (alkaline-phosphatase), and C (β-glucosidase) are highly influenced by the presence of exogenous organic matter. Functional and structural microbial diversity, despite of revealing a great sensitivity to changing soil conditions, is more difficult to assess (Hartmann et al., 2015). At present, some of the primary publications deal with soil microbial diversity (e.g., Bastida et al., 2015, 2016; Bonanomi et al., 2016; Daquiado et al., 2016; Fernández et al., 2016; Cesarano et al., 2017; Tian et al., 2017) in part because of multiple “omic” approaches (metagenomic, metatranscriptomic, metaproteomic, and metabolomic) that, in combination with more traditional methods like Biolog microplates and denaturing gradient gel electrophoresis (DGGE), have come to exceed the uncertainties surrounding dynamics of microbial community as well as taxon level. The incorporation to soil of vermicompost (Wu et al., 2013; Song et al., 2015), green residues (Kim et al., 2013; Aslam et al., 2014; Tejada and Benítez, 2014); animal manures (Bowles et al., 2014; Ninh et al., 2015; Blanchet et al., 2016), and biosolid-based composts (Di Bene et al., 2013; Xue and Huang, 2013; Bonanomi et al., 2014) increases significantly plant growth and crop yield, which is principally attributed to an enhance of biomass, activity and diversity of the microbial rhizosphere community. However, changes in microbial properties vary considerably in accordance with soil type (mineral composition and texture), nature, dose and application frequency of the OA and duration of the study (Ninh et al., 2015; Reardon and Wuest, 2016; Zornoza et al., 2016). The complex interaction networks in microbial communities and technical constraints make difficult full understand of the response of microbial communities toward OAs (Hartmann et al., 2015).
Using a “win-to-win” Approach: Organic Wastes as Amendments for Aided Phytostabilization Strategy in Contaminated Soils
Many peri-urban vacant lands at former industrial sites tend to have increased load of organic and inorganic pollutants relative to more distant rural areas (Basta et al., 2016; Beniston et al., 2016). Among pollutants, metals are especially critical as they are characterized by high stability and lack of biodegradability (Zhou et al., 2017). Even worse, some metals are ecotoxic and exhibit long-term persistence in soil, strongly affecting the structure and functioning of ecosystems and allowing their migration and incorporation into the trophic web until, finally, endanger human health (Deng et al., 2015; Venegas et al., 2015; García et al., 2017). As a consequence, and being the public awareness a driving force, researchers and authorities worldwide have paid attention to remediation of metal contaminated soils not only for environmental and human health concerns (Madejón et al., 2018), just as much for the potential implications to international (food) trade (Park et al., 2011).
Soil management of these polluted areas faces dual challenges of minimizing contaminant exposure to soil inhabitants (including human) and restoring and maintaining soil functioning/health by rapid development of thriving soil microbial community and plant assemblies (Basta et al., 2016; Obrycki et al., 2017). Research and demonstration studies have evidenced that the application of organic soil amendments have been particularly effective to achieve both management goals (Basta et al., 2016; Zhou et al., 2017), which also possess the acceptance of public and regulators (Obrycki et al., 2017). According to Kästner and Miltner (2016), the use of OAs is certainly justified for polluted soils in urban areas, where the economic pressure for their reuse is high enough to make even cost-intensive remediation strategies economically feasible. Moreover, the use of OAs to restore urban and polluted areas run in harmony with European pollution prevention and control proposals (García et al., 2017).
Over last years, phytoremediation technologies such as “aided phytostabilization” have been successfully implemented as a phytomanagement strategy, succeeding mitigate the environmental impact of metal-contaminated soils and displaying great potential to be applied to extended areas (Venegas et al., 2015). Aided phytostabilization is defined as an environmentally friendly in situ phytoremediation strategy based on the combination of metal-tolerant plants (phytostabilization) and organic or inorganic amendments (chemical stabilization; Garaiyurrebaso et al., 2017), which simultaneously reduce soil metal mobility/bioavailability, thereby reducing leaching and their transfer through trophic web, improving soils microbial properties and facilitating plant establishment (revegetation; Gómez-Sagasti et al., 2012; Clemente et al., 2015). In the field of restoration of mining impacted soils and metalliferous mine tailings, the application of aided phytostabilization programs are particularly encouraged (Galende et al., 2014a; Pardo et al., 2014; Madejón et al., 2018), in order to avoid the burdensome resource investments that involved soil remediation (Brown and Chaney, 2016). Comprehensive overviews of the effects of aided phytostabilization experiences on physical, chemical and biological properties of mining soils are summarized in Clemente et al. (2015), Brown and Chaney (2016), and more recently, in Sharma and Nagpal (2017).
The metal stabilization and immobilization processes, including adsorption, precipitation and/or complexation (Sharma and Nagpal, 2017), depend upon the particular metal and soil type involved, degree of humification of the organic matter, content of metals and salts and the effects of organic matter on the redox potential and soil pH (Clemente et al., 2005; Pérez-Esteban et al., 2014). However, although metals are immobilized within soil and become less bioavailable, the amended sites require long-term and regular monitoring and runoff control measures to ensure that the optimal stabilizing conditions of metals remain unchanged (Basta et al., 2016; Garaiyurrebaso et al., 2017; Khalid et al., 2017).
The effectiveness of aided phytostabilization of polluted soils has been validated in both laboratory and field scale experiments. Increasingly, measures of microbial biomass, activity, and diversity are being used to evaluate the reductions in metal bioavailability and the restoration of soil functioning in amended soils in both in microcosm and field studies (Gómez-Sagasti et al., 2012; Pardo et al., 2014; Brown and Chaney, 2016). Animal manure, biosolids and their respective composts are often recommended OAs for metal immobilization (Khalid et al., 2017). For example, Carlson et al. (2015) reported that biosolids from sewage sludges increased soil enzyme activities and fungal biomass within the first year after amending degraded industrial soil. Similarly, Basta et al. (2016) evidenced that biosolid amendments increased soil enzymatic activities and grass diversity in urban degraded soil. Zhou et al. (2017) observed significant reduction of metal available fraction while microbial biomass was increased in urban agricultural soil amended with red mud and compost. More information about the efficacy of amendments was reviewed by García et al. (2017). Concerning fungal community, Montiel-Rozas et al. (2016) indicated that biosolid composts promoted a more phylogenetically diverse arbuscular mycorrhizal (AM) in metal polluted soils. However, when colonizing plant species were changed and experiment was carried out in a longer term, exogenous organic matter addition did not influence AM fungal parameters or metal plant uptake (Montiel-Rozas et al., 2017). Additionally, OAs such as green and biosolid composts can also stimulate the degradation and/or mineralization of other frequent soil co-pollutants like organic pollutants because they play a role in supplementing nutrients and carbon source, as was evidenced by Masciandaro et al. (2013), Bastida et al. (2016), and Lacalle et al. (2018).
Contaminants of Emerging Concern in Soils Amended With Organic Wastes and the Road Ahead
Ever since “Silent Spring” (1962) by Rachel Carson and “The Closing Circle: Nature, Man, and Technology” (1971) by Barry Commoner were published in full Green Revolution, the popular and political concerns toward side-effects of contaminants in the environment have been increased, and with it the legislation and directive proposals about approval and use of chemicals (Kästner and Miltner, 2016). Traditionally, regular application of biosolids and animal manures to agricultural soils has been considered as a major contamination source of metal(loid)s (Park et al., 2011; Clemente et al., 2015), organic contaminants (Semblante et al., 2015) and most prevalent pathogenic microorganisms such as Campylobacter, Escherichia coli, Salmonella, and Yersinia (García et al., 2017). The identification, characterization, and prioritization of potentially hazardous chemical and biological agents of these organic wastes before their use as soil amendments is an important requirement, if not mandatory, in the framework of waste management and environmental risk assessment. Nonetheless, to date only the 29.6% of the studies take into account the potential risks of exogenous organic matter application (Table 1).
In accordance with this, the existing EU legislation covering waste management, fundamentally comprised of Waste Framework Directive 2008/98/EC, Hazardous Waste Directive 94/31/EC (further specified by the Decision 2000/532/EC), Integrated Pollution Prevention and Control Directive 96/61/EC and Directive on the Landfill of Waste 99/31/EC and complemented by Sewage Sludge Directive 86/278/EEC and Animal Waste Directive 90/667/EEC, among others, seeks to encourage prevention mechanisms and the establishment of indicative maximum (limit) concentrations (values) for each class of contaminant present in wastes to ensure their safe use as OAs. Thereupon, the advances in the sewage and wastewater treatment technologies and improvements in feed utilization in animal and poultry industries, have successfully reduced the metal content of biosolids and animal manures (Park et al., 2011; Khalid et al., 2017). Furthermore, the humification of organic matter may also decrease the bioavailability of metal and organic contaminants through the formation of pollutant-humic complexes (Clemente and Bernal, 2006; Masciandaro et al., 2013). The risk of pathogens embedded in manures can be significantly mitigated through composting, which mostly comprise efficient hygienization, although it may not entirely prevent pathogenic regrowth (Masciandaro et al., 2013; García et al., 2017). More specific criteria or guidelines with reference to compost' assessment for its use as soil amendment in different European countries are provided by Saveyn and Eder (2014) and Cesaro et al. (2015).
Unlike metals and pathogens, much less is known about the fate and behavior in terrestrial environments of micro(nano)plastics and ARGs in some organic wastes, including composts, livestock manures, and biosolids. As such, these micro- and nano-sized agents should be considered global emerging contaminants that need to be immediately addressed in the current regulatory framework for wastes. As shown below, their increasingly occurrence in soils and the uncertainties connected to their potential exposure pathways and unpredictable ecotoxicological effects render them worthy of research attention in recent years.
Immersed as we are in the golden age of the “plastic era” due the accessibility and spread of fabrication technology and versatility for applications, petroleum-based plastics can be found in each and every industry (e.g., packaging, building and construction, aeronautics, automotive, electronics, agriculture and healthcare) and, hence, they have become essential for the daily life. Within Europe, 60 million tons of plastics were produced in 2016 (accounting for 18% of world production) to cover an estimated total demand of 50 million tons (PlasticsEurope, 2017). Official data also shown that only the 45% of produced plastics in 2016 (c.a. 27 million tons) were then collected after use, of which around 31% entered to recycling stream (PlasticsEurope, 2017). Although the relevance of plastics for economies and social welfare are unquestionable, the overproduction and inadequate waste management elevated plastics into a major environmental threat. Furthermore, the most commonly used polymers (e.g., polyethylene, polypropylene, polyvinyl chloride) are not readily biodegradable, they are subjected to weathering and fragmenting into microplastics and remain in the environment for hundreds of years (United Nations Environment Programme (UNEP), 2015; Horton et al., 2017). Thus, European Commission identified “plastics” as a key priority area and, profit of that, is the recently adopted European Commission (2018).
Microplastic (plastic particles smaller than 5 mm) can occur in the environment either as primary (manufactured at a microscopic size and intentionally added to personal care products, known as microbeads) or indirectly as secondary microplastics (resulting from the disintegration of larger-sized plastic debris and litter by the effect of UV radiation or mechanic abrasion such as those generated during use of tire and synthetic garments, plastic mulching or along the production, supply and end-of-life stage of plastics; Duis and Coors, 2016). Even though agricultural and urban lands are expected to represent major entry points and reservoirs for microplastics in terrestrial ecosystems, possibly comprehensively larger than the marine basins, very few data is available regarding the sources, pathways, and possible accumulation of microplastics in different soil compartments (Duis and Coors, 2016; Nizzetto et al., 2016; Horton et al., 2017; Rillig et al., 2017; de Souza Machado et al., 2018; Hurley and Nizzetto, 2018; Mai et al., 2018). This is in part due to analytical difficulties. Commonly employed methods for analyzing microplastics often involve filtering, flotation, visual selection by microscope followed by confirmation methods or pyrolysis gas chromatography-mass spectrometry that are suitable for aquatic environments but present particular limitations for terrestrial and other continental environments (de Souza Machado et al., 2017). Efforts are currently undertaken to establish effective and standardized analytical procedures, but the detection of microplastic from heterogeneous, organic-rich soil matrices is not yet possible (Hurley and Nizzetto, 2018). In line with European Strategy for Plastics and REACH procedures for restricting potentially hazardous substances to the environment or human health, European Commission requested scientific evidences to European Chemical Agency for taking regulatory measures to reduce the use of intentionally-added microplastics to products.
The addition of biosolids collected from municipal wastewater treatments as soil amendments have been recently estimated as one of the largest sources of microplastics to the soils (Nizzetto et al., 2016; Hurley and Nizzetto, 2018). Nizzetto et al. (2016) even estimate that annually between 125 and 850 tons microplastics per million inhabitants are added to European agricultural soils either through direct application of sewage sludge or as processed biosolids. As approximately 99% of microplastics in the wastewater stream are likely to be retained in sludge, there is a possibility that, even after treatment prior land-spreading, they could contain significant amounts of microplastics (Mahon et al., 2017). In this sense, several studies (Carr et al., 2016; Mahon et al., 2017; Mintenig et al., 2017; Talvitie et al., 2017; Ziajahromi et al., 2017; Lares et al., 2018) pointed out the urgent need for advanced final-stage wastewater treatment technologies more efficient in the removal of microplastics from wastewater effluents and sludges.
In essence, microplastics could be ingested and accumulate by biota leading to starvation (physical damage), could interact and concentrate persistent organic contaminants (Rocha-Santos and Duarte, 2015) and/or adsorb metals more than by soil particles with equal mass (Hodson et al., 2017), due to their large surface area-to-volume ratio (chemical damage). Microplastic-associated ecotoxicity could be also caused by the leaching of endocrine-disrupting additives added during manufacturing processes of plastics (Andrady, 2015). The subsequent transfer of such contaminants and additives from microplastics to marine organisms has been confirmed under experimental conditions (Taylor et al., 2016; Galloway et al., 2017). However, there are no experimental evidences so far to our knowledge on the transfer and bioaccumulation of micro(nano)plastics in the terrestrial biotic food web (Mai et al., 2018; Ng et al., 2018).
Ecological impacts of microplastics on invertebrates, vertebrates, birds, and mammals of freshwater and marine environments are currently the subject of intense field and laboratory research (Anbumani and Kakkar, 2018 and references therein). Early investigations evidenced that soil micro- and mesofauna, such as microbial community (Wang et al., 2016; de Souza Machado et al., 2018; Rillig and Bonkowski, 2018); collembolan (Maaß et al., 2017; Zhu et al., 2018), earthworms (Huerta Lwanga et al., 2016; Cao et al., 2017; Rillig et al., 2017; Rodriguez-Seijo et al., 2017), and terrestrial birds (Zhao et al., 2016) might be also susceptible to the ecotoxicological effects of microplastics. It should be emphasized, however, that the exposure concentrations used in most of the studies are higher than those found in land scenario (Ng et al., 2018). When considering nanoplastics (<100 nm), it has been observed that yeasts (Miyazaki et al., 2014), filamentous fungi (Nomura et al., 2016), and mammals (Mutua et al., 2011) show toxicity symptoms once they are exposed to nanobeads. Uptake of microplastics by plants is not expected, but it has been reported that nanoplastics could enter into plants due to their comparable density to micronutrients (Bandmann et al., 2012). However, no study has been carried out so far on translocation and distribution of nanoplastics in plant organs (Ng et al., 2018). Ecotoxicity of nano- and microplastics in soils due to application of OAs required further attention in future studies.
Simultaneously to the spreading of microplastics, the selective pressure exerted by overuse of antibiotics for decades has caused an unprecedented prevalence and proliferation of clinically relevant antibiotic-resistant bacteria (ARB) and genes (ARGs) in natural environments. According to World Health Organization (WHO) (2014, 2015) and UnitedNations (UN) (2016), antibiotic resistance is one of the greatest threats to global public health. In response to the potential loss of efficacy of clinically relevant antibiotics, last year European One Health Action Plan against Antimicrobial Resistance was published in order to establish the road ahead to mitigate social and economic impacts of this emerging risk.
Long-term amended soils with biosolids resulted from the treatment of domestic sewages, hospital wastewaters, and drainage from livestock feeding operations are hotspots for ARB and ARGs (Mao et al., 2015; Goulas et al., 2018; Urra et al., 2018). In fact, Su et al. (2014) and Chen et al. (2016) revealed more than 130 unique ARGs and mobile genetic elements (MEGs) in sewage sludges and animal manures that confer resistance to most used antibiotics groups such as β-lactams and tetracyclines. When soils are amended with manure, bacteria carrying ARGs are also introduced into the soil and persist for a long time, as demonstrated the studies conducted by Joy et al. (2013), Fahrenfeld et al. (2014), Udikovic-Kolic et al. (2014), Ross and Topp (2015), Wang et al. (2015), Peng et al. (2017), and Tien et al. (2017). Chen et al. (2017) even detect a total of 240 unique ARGs via a high-throughput quantitative PCR (qPCR) targeting almost all major classes of ARGs.
Potential risks associated to ARGs that threaten the future of antibiotic therapy include the horizontal gene transfer by MGEs between bacteria and pathogens residing in soils (Garbisu et al., 2018), which in turn tilt the balance of selection toward increased survival and proliferation of resistant bacteria, and the vertical dissemination along the food chain via plant-uptake. Actually, conjugative plasmid-mediated horizontal gene transfer is considered the most important process in the dissemination of multidrug resistance genes (Garbisu et al., 2017). Resistance plasmids often carry integrons. It is well documented that integrons (genetic units that act as natural cloning systems and expression vectors), and concretely the Class 1 integrons, are probably the most prevalent vehicles of ARGs cassettes in wastewater treatment plants (Di et al., 2016), and land applied sewage sludges (Burch et al., 2014; Zhang et al., 2017) and manures (Sandberg and LaPara, 2016).
The consumption of plants and vegetables, particularly those that are eaten raw or are subjected to minimal processing, by humans or animals represents a potential route of exposure to ARB and, subsequently, a potential pathway for ARGs transmission toward food web. Recent studies evidenced the dissemination of ARGs to plants through endophytic system. When abundance of ARGs in both amended soil and harvested vegetables were analyzed, Marti et al. (2013, 2014) detected ARGs on tomatoes, peppers, cucumbers, carrots, radishes, and lettuces grown in soils amended with pig manures, including root endophytes, leaf endophytes, and phyllosphere microorganisms. Strikingly, numerous antibiotic-resistance determinants were also detected in those vegetables grown in un-manured soil. Nonetheless, their results reinforce the advisability of pre-treating biosolids and manures and/or the establishment of off-set times between amending and harvesting vegetables for human consumption. Similar results were reported by Wang et al. (2015) in lettuce and endive grown in manure-amended soils under controlled conditions. Yang et al. (2014) also identified antibiotic-resistant endophytic bacteria with high resistance to cephalexin in celery, Chinese cabbages, and cucumbers collected from greenhouse fields fertilized with chicken manure. Zhu et al. (2017) detected a total of 134 ARGs in the phyllosphere and leaf endophyte of lettuces under conventional and organic farming using high-throughput qPCR and 16sRNA Illumina sequencing technology. They observed lower diversity of both phyllosphere and leaf endophytic bacteria in organically-produced lettuces than in conventionally-produced ones. Furthermore, qPCR analysis revealed ARGs and MGEs on various vegetables (lettuce, carrots, radish, and tomatoes) exposed to biosolids (Rahube et al., 2014).
In addition, ARGs are often, if not always, found in bacteria with other genes promoting resistance to other potentially ecotoxic chemicals such as metals and biocides (Singer et al., 2016). Co-resistance phenomenon is of great importance in the case of the agricultural use of biosolids, since the presence of metals in both biosolid and biosolid-amended soil may select for ARB (Gullberg et al., 2014; Bondarczuk et al., 2016). In a long-term (24 years) field experiment carried out to study the impact of digested sewage sludge on agricultural soil quality, Urra et al. (2018) investigated the presence of ARGs and MEGs. Using high-throughput RT-qPCR, the authors revealed an increase of the abundance of ARGs and MGEs with sludge application as well as their correlation with soil Cu and Zn concentrations. That suggests co-selection mechanisms for metals and antibiotics and a need to properly treat sewage sludge prior to its application. To assess long-term effects of different animal manures and paper sludge on the presence of MGEs and ARGs in metal contaminated mine soil, Garbisu et al. (2018) analyzed metabolic traits and metal resistance of putative transconjugants. Their results confirmed the presence of putative conjugative or mobilisable plasmids and concluded that the bacteria in the mine soils had gene-mobilizing capacity and, therefore, potential for the dissemination of antibiotic and metal resistance genes. This is yet further proof of natural soil resistome. For additional comprehensive information about ARGs, see the works of Thanner et al. (2016), Hashmi et al. (2017), Jagtap (2017), and Xie et al. (2018).
In addition, surveillance of ARGs in environmental samples and compilation of evidence-based information about the significance and magnitude of the potential impacts of ARGs in health and food security contexts at international level must also be encouraged (Berendonk et al., 2015; Petrillo and Ogunseitan, 2018). The current lack of an standardize characterization and quantification procedure of ARGs applicable to environmental samples due the highly diverse and abundant soil microbiome (Ju et al., 2016) is the reason why there are scarce the detailed dose-response data useful for ecological risk assessment of ARGs introduced after the amendment of soils with organic wastes. Culturing techniques together with qPCR and more recently shotgun metagenomics have been applied to quantify ARGs in both amendments and terrestrial environments. Nonetheless, the number of targeted resistance phenotypes and genotypes, DNA extraction methods, narrow availability of primer sets, amplification bias, false-negative results due to inhibition in PCR and false-positive results due to non-specific amplifications often limit the suitability of mentioned molecular techniques (Berendonk et al., 2015; Li et al., 2015). Methods applied for revealing the diversity and the abundance of ARB and ARG in wastes and in soil samples have been discussed by Rizzo et al. (2013), Luby et al. (2016), Manaia et al. (2016), and Christou et al. (2017). In spite of the increasingly number of studies, an accurate risk assessment of amendment-borne ARGs is still needed.
Conclusions and Perspectives
The changes in land-uses and land-covers have aggravated the soil degradation, with an attendant reduction in ecosystem's capacity to provide goods and services. However, degraded soils, and particularly those in urban location, should be considered as opportunity for soft re-uses after the improvement of ecological, economic and social values by application of phytomanagement. In order to restore soil functioning (soil health) and soil fitness for crop production (soil quality), phytomanagement programs usually include the use of organic wastes as soil conditioners. Thus, phytomanagement of degraded soils is understood as a restoration strategy within Circular Economy paradigm, wherein resiliency of resources (soils and wastes) is promoted.
The large number of studies reviewed here contributes to the better understanding of the potential benefits OAs, mostly regarding the improvement of soil microbial properties. However, at the moment, few are the studies that explore simultaneously both microbial and crop eco-physiological status in degraded soils amended with different OAs. A key outcome of this review is to encourage future research on ecotoxicological impacts of OA-borne microplastics and ARGs, which usually persist beyond composting and wastewater treatment processes and are challenging analytical tasks. This will be critical to implement effective environmental risk assessment procedures and compile/synthetize validated information to include them into the waste management policy and regulatory framework.
Author Contributions
MG-S has contributed significantly to the conception and development of the idea, writing and revision of the manuscript. AH, UA, CG, and JB have been actively involved in the conception, revision and supervision of the manuscript.
Funding
This work has been funded by the following projects: AGL2015-64481-C2-1 and AGL2016-76592-R (MINECO/FEDER), PhytoSUDOE SOE1/P5/E0189; FEDER funds; and IT1018-16 (Basque Government).
Conflict of Interest Statement
The authors declare that the research was conducted in the absence of any commercial or financial relationships that could be construed as a potential conflict of interest.
Supplementary Material
The Supplementary Material for this article can be found online at: https://www.frontiersin.org/articles/10.3389/fsufs.2018.00068/full#supplementary-material
References
Alvarenga, P., Mourinha, C., Farto, M., Santos, T., Palma, P., Sengo, J., et al. (2015). Sewage sludge, compost and other representative organic wastes as agricultural soil amendments: benefits versus limiting factors. Waste Manag. 40, 44–52. doi: 10.1016/j.wasman.2015.01.027
Alvarenga, P., Palma, P., Mourinha, C., Farto, M., Dôres, J., Patanita, M., et al. (2017). Recycling organic wastes to agricultural land as a way to improve its quality: a field study to evaluate benefits and risks. Waste Manag. 61, 582–592. doi: 10.1016/j.wasman.2017.01.004
Anastasi, A., Coppola, T., Prigione, V., and Varese, G. C. (2009). Pyrene degradation and detoxification in soil by a consortium of basidiomycetes isolated from compost: role of laccases and peroxidases. J. Hazard. Mater. 165, 1229–1233. doi: 10.1016/j.jhazmat.2008.10.032
Anbumani, S., and Kakkar, P. (2018). Ecotoxicological effects of microplastics on biota: a review. Environ. Sci. Pollut. Res. Int. 25, 14373–14396. doi: 10.1007/s11356-018-1999-x
Andrady, A. L. (2015). “Persistence of plastic litter in the oceans,” in Marine Anthropogenic Litter, eds M. Bergmann, L. Gutow, and M. Klages (Cham: Springer), 57–72.
Aparna, K., Pasha, M. A., Rao, D. L. N., and Krishnaraj, P. U. (2014). Organic amendments as ecosystem engineers: microbial, biochemical and genomic evidence of soil health improvement in a tropical arid zone field site. Ecol. Eng. 71, 268–277. doi: 10.1016/j.ecoleng.2014.07.016
Aslam, A. A., Sattar, M. A., Nazmul Islam, M., and Inubushi, K. (2014). Integrated effects of organic, inorganic and biological amendments on methane emission, soil quality and rice productivity in irrigated paddy ecosystem of Bangladesh: field study of two consecutive rice growing seasons. Plant Soil 378, 239–252. doi: 10.1007/s11104-014-2023-y
Bandmann, V., Müller, J. D., Köhler, T., and Homann, U. (2012). Uptake of fluorescent nano beads into BY2-cells involves clathrin-dependent and clathrin-independent endocytosis. FEBS Lett. 586, 3626–3632. doi: 10.1016/j.febslet.2012.08.008
Basta, N. T., Busalacchi, D. M., Hundal, L. S., Kumar, K., Dick, R. P., Lanno, R. P., et al. (2016). Restoring ecosystem function in degraded urban soil using biosolids, biosolids blend, and compost. J. Environ. Qual. 45, 74–83. doi: 10.2134/jeq2015.01.0009
Bastida, F., Jehmlich, N., Lima, K., Morris, B. E. L., Richnow, H. H., Hernández, T., et al. (2016). The ecological and physiological responses of the microbial community from a semiarid soil to hydrocarbon contamination and its bioremediation using compost amendment. J. Proteomics 135, 162–169. doi: 10.1016/j.jprot.2015.07.023
Bastida, F., Kandeler, E., Moreno, J. L., Ros, M., García, C., and Hernández, T. (2008). Application of fresh and composted organic wastes modifies structure, size and activity of soil microbial community under semiarid climate. Appl. Soil Ecol. 40, 318–329. doi: 10.1016/j.apsoil.2008.05.007
Bastida, F., Selevsek, N., Torres, I. F., Hernández, T., and García, C. (2015). Soil restoration with organic amendments: linking cellular functionality and ecosystem processes. Sci. Rep. 5:15550. doi: 10.1038/srep15550
Benayas, J. R., Martins, A., Nicolau, J. M., and Schulz, J. J. (2007). Abandonment of agricultural land: an overview of drivers and consequences. CAB Rev. 2, 1–14. doi: 10.1079/PAVSNNR20072057
Beniston, J. W., Lal, R., and Mercer, K. L. (2016). Assessing and managing soil quality for urban agriculture in a degraded vacant lot soil. Land Degrad. Dev. 27, 996–1006. doi: 10.1002/ldr.2342
Berendonk, T. U., Manaia, C. M., Merlin, C., Fatta-Kassinos, D., Cytryn, E., Walsh, F., et al. (2015). Tackling antibiotic resistance: the environmental framework. Nat. Rev. Microbiol. 13, 310–317. doi: 10.1038/nrmicro3439
Bernal, M. P., Clemente, R., and Walker, D. J. (2007). “The role of organic amendments in the bioremediation of heavy metal-polluted soils,” in Environmental Research at the Leading Edge, ed R. W. Gore (New York, NY: Nova Science Publishers Inc.), 1–57.
Bernal, M. P., Sommer, S. G., Chadwick, D., Qing, C., Guoxue, L., and Michel, F. C. Jr. (2017). “Current approaches and future trends in compost quality criteria for agronomic, environmental, and human health benefits,” in Advances in Agronomy, Vol. 144, ed D. L. Sparks (Cambridge, MA: Elsevier Academic Press), 143–233.
Blanchet, G., Gavazov, K., Bragazza, L., and Sinaj, S. (2016). Responses of soil properties and crop yields to different inorganic and organic amendments in a Swiss conventional farming system. Agric. Ecosyst. Environ. 230, 116–126. doi: 10.1016/j.agee.2016.05.032
Bonanomi, G., Antignani, V., Capodilupo, M., and Scala, F. (2010). Identifying the characteristics of organic soil amendments that suppress soilborne plant diseases. Soil Biol. Biochem. 42, 136–144. doi: 10.1016/j.soilbio.2009.10.012
Bonanomi, G., Antignani, V., Pane, C., and Scala, F. (2007). Suppression of soilborne fungal diseases with organic amendments. J. Plant Pathol. 89, 311–340. Available online at: https://www.jstor.org/stable/41998409
Bonanomi, G., D'Ascoli, R., Scotti, R., Gaglione, S. A., González Cáceres, M., Sultana, S., et al. (2014). Soil quality recovery and crop yield enhancement by combined application of compost and wood to vegetables grown under plastic tunnels. Agric. Ecosyst. Environ. 192, 1–7. doi: 10.1016/j.agee.2014.03.029
Bonanomi, G., De Filippis, F., Cesarano, G., La Storia, A., Ercolini, D., and Scala, F. (2016). Organic farming induces changes in soil microbiota that affect agro-ecosystem functions. Soil Biol. Biochem. 103, 327–336. doi: 10.1016/j.soilbio.2016.09.005
Bondarczuk, K., Markowicz, A., and Piotrowska-Seget, Z. (2016). The urgent need for risk assessment on the antibiotic resistance spread via sewage sludge land application. Environ. Int. 87, 49–55. doi: 10.1016/j.envint.2015.11.011
Bowles, T. M., Acosta-Martinez, V., Calderon, F., and Jackson, L. E. (2014). Soil enzyme activities, microbial communities, and carbon and nitrogen availability in organic agroecosystems across an intensively-managed agricultural landscape. Soil. Biol. Biochem. 68, 252–262. doi: 10.1016/j.soilbio.2013.10.004
Brown, S. L., and Chaney, R. L. (2016). Use of amendments to restore ecosystem function to metal mining-impacted sites: tools to evaluate efficacy. Curr. Rep. 2, 91–102. doi: 10.1007/s40726-016-0029-1
Bünemann, E. K., Bongiorno, G., Baic, Z., Creamer, R. E., De Deyn, G., de Goede, R., et al. (2018). Soil quality-A critical review. Soil Biol. Biochem. 120, 105–125. doi: 10.1016/j.soilbio.2018.01.030
Burch, T. R., Sadowsky, M. J., and LaPara, T. M. (2014). Fate of antibiotic resistance genes and class 1 integrons in soil microcosms following the application of treated residual municipal wastewater solids. Environ. Sci. Technol. 48, 5620–5627. doi: 10.1021/es501098g
Burges, A., Epelde, L., and Garbisu, C. (2015). Impact of repeated single-metal and multi-metal pollution events on soil quality. Chemosphere 120, 8–15. doi: 10.1016/j.chemosphere.2014.05.037
Cao, D., Wang, X., Luo, X., Liu, G., and Zheng, H. (2017). Effects of polystyrene microplastics on the fitness of earthworms in an agricultural soil. IOP Conf. Ser. Earth Environ. Sci. 61:012148. doi: 10.1088/1755-1315/61/1/012148
Carlson, J., Saxena, J., Basta, N., Hundal, L., Busalacchi, D., and Dick, R. P. (2015). Application of organic amendments to restore degraded soil: effects on soil microbial properties. Environ. Monit. Assess. 187:109. doi: 10.1007/s10661-015-4293-0
Carr, S. A., Liu, J., and Tesoro, A. G. (2016). Transport and fate of microplastic particles in wastewater treatment plants. Water Res. 91, 174–182. doi: 10.1016/j.watres.2016.01.002
Cesarano, G., De Filippis, F., La Storia, A., Scala, F., and Bonanomi, G. (2017). Organic amendment type and application frequency affect crop yields, soil fertility and microbiome composition. Appl. Soil Ecol. 120, 254–264. doi: 10.1016/j.apsoil.2017.08.017
Cesaro, A., Belgiorno, V., and Guida, M. (2015). Compost from organic solid waste: quality assessment and European regulations for its sustainable use. Resour. Conserv. Recycl. 94, 72–79. doi: 10.1016/j.resconrec.2014.11.003
Chen, Q., An, X., Li, H., Su, J., Ma, Y., and Zhu, Y. G. (2016). Long-term field application of sewage sludge increases the abundance of antibiotic resistance genes in soil. Environ. Int. 92, 1–10. doi: 10.1016/j.envint.2016.03.026
Chen, Q. L., An, X. L., Li, H., Zhu, Y. G., Su, J. Q., and Cui, L. (2017). Do manure-borne or indigenous soil microorganisms influence the spread of antibiotic resistance genes in manured soil? Soil Biol. Biochem. 114, 229–237. doi: 10.1016/j.soilbio.2017.07.022
Christou, A., Agüera, A., Bayona, J. M., Cytryn, E., Fotopoulos, V., Lambropoulou, D., et al. (2017). The potential implications of reclaimed wastewater reuse for irrigation on the agricultural environment: the knowns and unknowns of the fate of antibiotics and antibiotic resistant bacteria and resistance genes-A review. Water Res. 123, 448–467. doi: 10.1016/j.watres.2017.07.004
Clemente, R., and Bernal, M. P (2006) Fractionation of heavy metals distribution of organic carbon in two contaminated soils amended with humic substances. Chemosphere 64, 1264–1273 doi: 10.1016/j.chemosphere.2005.12.058
Clemente, R., Pardo, T., Madejón, P., Madejón, E., and Bernal, M. P. (2015). Food byproducts as amendments in trace elements contaminated soils. Food Res. Int. 73, 176–189. doi: 10.1016/j.foodres.2015.03.040
Clemente, R., Walker, D. J., and Bernal, M. P (2005) Uptake of heavy metals As by Brassica juncea grown in a contaminated soil in Aznalcóllar (Spain): the effect of soil amendments. Environ. Pollut. 138, 46–58 doi: 10.1016/j.envpol.2005.02.019
Cundy, A. B., Bardos, R. P., Puschenreiter, M., Mench, M., Bert, V., Friesl-Hanl, W., et al. (2016). Brownfields to green fields: realising wider benefits from practical contaminant phytomanagement strategies. J. Environ. Manage. 184, 67–77. doi: 10.1016/j.jenvman.2016.03.028
Daquiado, A. R., Kuppusamy, S., Kim, S. Y., Kim, J. H., Yoon, Y. E., Kim, P. J., et al. (2016). Pyrosequencing analysis of bacterial community diversity in long-term fertilized paddy field soil. Appl. Soil Ecol. 108, 84–91. doi: 10.1016/j.apsoil.2016.08.006
De Lucia, B., Cristiano, G., Vecchietti, L., and Bruno, L. (2013). Effect of different rates of composted organic amendment on urban soil properties, growth and nutrient status of three Mediterranean native hedge species. Urban For. Urban Green. 12, 537–545. doi: 10.1016/j.ufug.2013.07.008
de Souza Machado, A. A., Kloas, W., Zarfl, C., Hempel, S., and Rillig, M. C. (2017). Microplastics as an emerging threat to terrestrial ecosystems. Glob. Chang. Biol. 24, 1405–1416. doi: 10.1111/gcb.14020
de Souza Machado, A. A. S., Lau, C. W., Till, J., Kloas, W., Lehmann, A., Becker, R., et al. (2018). Impacts of microplastics on the soil biophysical environment. Environ. Sci. Technol. 52, 9656–9665. doi: 10.1021/acs.est.8b02212
Deng, L., Zeng, G., Fan, C., Lu, L., Chen, X., Chen, M., et al. (2015). Response of rhizosphere microbial community structure and diversity to heavy metal co-pollution in arable soil. Appl. Microbiol. Biotechnol. 99, 8259–8269. doi: 10.1007/s00253-015-6662-6
Di Bene, C., Pellegrino, E., Debolini, M., Silvestri, N., and Bonari, E. (2013). Short-and long-term effects of olive mill wastewater land spreading on soil chemical and biological properties. Soil Biol. Biochem. 56, 21–30. doi: 10.1016/j.soilbio.2012.02.019
Di, A., Eckert, E. M., Urso, S. D., Bertoni, R., Gillan, D. C., Wattiez, R., et al. (2016). Cooccurrence of integrase 1, antibiotic and heavy metal resistance genes in municipal wastewater treatment plants. Water Res. 94, 208–214. doi: 10.1016/j.watres.2016.02.049
Duis, K., and Coors, A. (2016). Microplastics in the aquatic and terrestrial environment: sources (with a specific focus on personal care products), fate and effects. Environ. Sci. Eur. 28:2. doi: 10.1186/s12302-015-0069-y
Epelde, L., Becerril, J. M., Kowalchuk, G. A., Deng, Y., Zhou, J., and Garbisu, C. (2010). Impact of metal pollution and Thlaspi caerulescens growth on soil microbial communities. Appl. Environ. Microbiol. 76, 7843–7853. doi: 10.1128/AEM.01045-10
European Commission (2011a). Commission Staff Working Paper. Analysis Associated with the Roadmap to a Resource Efficient Europe Part I. Brussels.
Fahrenfeld, N., Knowlton, K., Krometis, L. A., Hession, W. C., Xia, K., Lipscomb, E., et al. (2014). Effect of manure application on abundance of antibiotic resistance genes and their attenuation rates in soil: field-scale mass balance approach. Environ. Sci. Technol. 48, 2643–2650. doi: 10.1021/es404988k
Fernández, A. L., Sheaffer, C. C., Wyse, D. L., Staley, C., Gould, T. J., and Sadowsky, M. J. (2016). Structure of bacterial communities in soil following cover crop and organic fertilizer incorporation. Appl. Microbiol. Biotechnol. 100, 9331–9341. doi: 10.1007/s00253-016-7736-9
Food and Agriculture Organization of the United Nations (FAO) (2015). Status of the World's Soil Resources (SWSR)-Main Report Prepared by the Intergovernmental Technical Panel on Soils (ITPS). Rome, 648.
Galende, M. A., Becerril, J. M., Barrutia, O., Artetxe, U., Garbisu, C., and Hernández, A. (2014a). Field assessment of the effectiveness of organic amendments for aided phytostabilization of a Pb-Zn contaminated mine soil. J. Geochem Explor. 145, 181–189. doi: 10.1016/j.gexplo.2014.06.006
Galende, M. A., Becerril, J. M., Gómez-Sagasti, M. T., Barrutia, O., Epelde, L., Garbisu, C., et al. (2014b). Chemical stabilization of metal-contaminated mine soil: early short-term soil-amendment interactions and their effects on biological and chemical parameters. Water Air Soil Pollut. 225:1863. doi: 10.1007/s11270-013-1863-z
Galloway, T. S., Cole, M., and Lewis, C. (2017). Interactions of microplastic debris throughout the marine ecosystem. Nat. Ecol. Evol. 1:0116. doi: 10.1038/s41559-017-0116
Garaiyurrebaso, O., Garbisu, C., Blanco, F., Lanzén, A., Martín, I., Epelde, L., et al. (2017). Long-term effects of aided phytostabilisation on microbial communities of metal-contaminated mine soil. FEMS Microbiol. Ecol. 93:fiw252. doi: 10.1093/femsec/fiw252.
Garbisu, C., Garaiyurrebaso, O., Epelde, L., Grohmann, E., and Alkorta, I. (2017). Plasmid-mediated bioaugmentation for the bioremediation of contaminated soils. Front. Microbiol. 8:1966. doi: 10.3389/fmicb.2017.01966
Garbisu, C., Garaiyurrebaso, O., Lanzén, A., Álvarez-Rodríguez, I., Arana, L., Blanco, F., et al. (2018). Mobile genetic elements and antibiotic resistance in mine soil amended with organic wastes. Sci. Total Environ. 621, 725–733. doi: 10.1016/j.scitotenv.2017.11.221
García, C., Hernández, T., Coll, M. D., and Ondoño, S. (2017). Organic amendments for soil restoration in arid and semiarid areas: a review. AIMS Environ. Sci. 4, 640–676. doi: 10.3934/environsci.2017.5.640
Ge, T., Chen, X., Yuan, H., Li, B., Zhu, H., Peng, P., et al. (2013). Microbial biomass, activity, and community structure in horticultural soils under conventional and organic management strategies. Eur. J. Soil Biol. 58, 122–128. doi: 10.1016/j.ejsobi.2013.07.005
Geissdoerfer, M., Savaget, P., Bocken, N. M., and Hultink, E. J. (2017). The Circular Economy–A new sustainability paradigm? J. Clean. Prod. 143, 757–768. doi: 10.1016/j.jclepro.2016.12.048
Glæsner, N., Helming, K., and de Vries, W. (2014). Do current European policies prevent soil threats and support soil functions? Sustainability 6, 9538–9563. doi: 10.3390/su6129538
Gómez-Sagasti, M. T., Alkorta, I., Becerril, J. M., Epelde, L., Anza, M., and Garbisu, C. (2012). Microbial monitoring of the recovery of soil quality during heavy metal phytoremediation. Water Air Soil Pollut. 223, 3249–3262. doi: 10.1007/s11270-012-1106-8
Goss, M. J., Tubeileh, A., and Goorahoo, D. (2013). “A review of the use of organic amendments and the risk to human health,” in Advances in Agronomy, Vol. 120, ed D. L. Sparks (Cambridge, MA: Elsevier Academic Press), 275–379.
Goulas, A., Livoreil, B., Grall, N., Benoit, P., Couderc-Obert, C., Dagot, C., et al. (2018). What are the effective solutions to control the dissemination of antibiotic resistance in the environment? A systematic review protocol. Environ. Evid. 7:3. doi: 10.1186/s13750-018-0118-2
Gullberg, E., Albrecht, L. M., Karlsson, C., Sandegren, L., and Andersson, D. I. (2014). Selection of a multidrug resistance plasmid by sublethal levels of antibiotics and heavy metals. MBio 5:e01918–14. doi: 10.1128/mBio.01918-14
Hartmann, M., Frey, B., Mayer, J., Mäder, P., and Widmer, F. (2015). Distinct soil microbial diversity under long-term organic and conventional farming. ISME J. 9:1177. doi: 10.1038/ismej.2014.210
Hashmi, M. Z., Mahmood, A., Kattel, D. B., Khan, S., Hasnain, A., and Ahmed, Z. (2017). “Antibiotics and antibiotic resistance genes (ARGs) in soil: occurrence, fate, and effects,” in Xenobiotics in the Soil Environment Monitoring, Toxicity and Management, eds M. Z. Hashmi, V. Kumar, and A. Varma (Cham: Springer), 41–54.
Hijbeek, R., Pronk, A. A., van Ittersum, M. K., ten Berge, H. F. M., Bijttebier, J., and Verhagen, A. (2018). What drives farmers to increase soil organic matter? Insights from the Netherlands. Soil Use Manag. 34, 85–100. doi: 10.1111/sum.12401
Hijbeek, R., van Ittersum, M. K., ten Berge, H. F., Gort, G., Spiegel, H., and Whitmore, A. P. (2017). Do organic inputs matter-a meta-analysis of additional yield effects for arable crops in Europe. Plant Soil 411, 293–303. doi: 10.1007/s11104-016-3031-x
Hodson, M. E., Duffus-Hodson, C. A., Clark, A., Prendergast-Miller, M. T., and Thorpe, K. L. (2017). Plastic bag derived-microplastics as a vector for metal exposure in terrestrial invertebrates. Environ. Sci. Technol. 51, 4714–4721. doi: 10.1021/acs.est.7b00635
Holm, P. E., Jensen, L. S., and McLaughlin, M. J. (2010). “Utilization of biologically treated organic waste on land,” in Solid Waste Technology and Management, ed T. H. Christensen (Copenhagen: John Wiley & Sons), 665–682.
Horton, A. A., Walton, A., Spurgeon, D. J., Lahive, E., and Svendsen, C. (2017). Microplastics in freshwater and terrestrial environments: evaluating the current understanding to identify the knowledge gaps and future research priorities. Sci. Total Environ. 586, 127–141. doi: 10.1016/j.scitotenv.2017.01.190
Huerta Lwanga, E., Gertsen, H., Gooren, H., Peters, P., Salánki, T., van der Ploeg, M., et al. (2016). Microplastics in the terrestrial ecosystem: implications for Lumbricus terrestris (Oligochaeta, Lumbricidae). Environ. Sci. Technol. 50, 2685–2691. doi: 10.1021/acs.est.5b05478
Hurley, R. R., and Nizzetto, L. (2018). Fate and occurrence of micro (nano) plastics in soils: knowledge gaps and possible risks. Curr. Opin. Environ. Sci. Health 1, 6–11. doi: 10.1016/j.coesh.2017.10.006
ISO 11269-1 (2012). Soil Quality - Determination of the Effects of Pollutants on Soil Flora – Part 1: Method for the Measurement of Inhibition of Root Growth.
ISO 11269-2 (2012). Soil Quality - Determination of the Effects of Pollutants on Soil Flora - Part 2: Effects of Contaminated Soil on the Emergence and Early Growth of Higher Plants.
Jagtap, U. B. (2017). “Antibiotics in the soil: sources, environmental issues, and bioremediation,” in Antibiotics and Antibiotics Resistance Genes in Soils, eds M. Z. Hashmi, V. Strezov, and A. Varma (Cham: Springer), 381–389.
Jones, A., Panagos, P., Barcelo, S., Bouraoui, F., Bosco, C., Dewitte, O., et al. (2012). The State of Soil in Europe - A Contribution of the JRC to the European Environment Agency's Environment State and Outlook Report - SOER 2010. No. EUR 25186 EN in EUR - Scientific and Technical Research Series. Publications Office of the European Union.
Joy, S. R., Bartelt-Hunt, S. L., Snow, D. D., Gilley, J. E., Woodbury, B. L., Parker, D. B., et al. (2013). Fate and transport of antimicrobials and antimicrobial resistance genes in soil and runoff following land application of swine manure slurry. Environ. Sci. Technol. 47, 12081–12088. doi: 10.1021/es4026358
Ju, F., Li, B., Ma, L., Wang, Y., Huang, D., and Zhang, T. (2016). Antibiotic resistance genes and human bacterial pathogens: co-occurrence, removal, and enrichment in municipal sewage sludge digesters. Water Res. 91, 1–10. doi: 10.1016/j.watres.2015.11.071
Karlen, D. L., and Rice, C. W. (2015). Soil degradation: will humankind ever learn? Sustainability 7, 12490–12501. doi: 10.3390/su70912490
Kästner, M., and Miltner, A. (2016). Application of compost for effective bioremediation of organic contaminants and pollutants in soil. Appl. Microbiol. Biotechnol. 100, 3433–3449. doi: 10.1007/s00253-016-7378-y
Khalid, S., Shahid, M., Niazi, N. K., Murtaza, B., Bibi, I., and Dumat, C. (2017). A comparison of technologies for remediation of heavy metal contaminated soils. J. Geochem. Explor. 182, 247–268. doi: 10.1016/j.gexplo.2016.11.021
Kim, G., Miller, P. A., and Nowakc, D. J. (2018). Urban vacant land typology: a tool for managing urban vacant land. Sustain. Cities Soc. 36, 144–156. doi: 10.1016/j.scs.2017.09.014
Kim, S. Y., Chang, H. L., Gutierrez, J., and Kim, P. (2013). Contribution of winter cover crop amendments on global warming potential in rice paddy soil during cultivation. Plant Soil 366, 273–286. doi: 10.1007/s11104-012-1403-4
Kumar, K., and Hundal, L. S. (2016). Soil in the city: sustainably improving urban soils. J. Environ. Qual. 45, 2–8. doi: 10.2134/jeq2015.11.0589
Lacalle, R. G., Gómez-Sagasti, M. T., Artetxe, U., Garbisu, C., and Becerril, J. M. (2018). Brassica napus has a key role in the recovery of the health of soils contaminated with metals and diesel by rhizoremediation. Sci. Total Environ. 618, 347–356. doi: 10.1016/j.scitotenv.2017.10.334
Laishram, J., Saxena, K. G., Maikhuri, R. K., and Rao, K. S. (2012). Soil quality and soil health: a review. Int. J. Ecol. Environ. 38, 19–37.
Lal, R. (2016). Soil health and carbon management. Food Energy Secur. 5, 212–222. doi: 10.1002/fes3.96
Lares, M., Ncibi, M. C., Sillanpää, M., and Sillanpää, M. (2018). Occurrence, identification and removal of microplastic particles and fibers in conventional activated sludge process and advanced MBR technology. Water Res. 133, 236–246. doi: 10.1016/j.watres.2018.01.049
Larkin, R. P. (2015). Soil health paradigms and implications for disease management. Annu. Rev. Phytopathol. 53, 199–221. doi: 10.1146/annurev-phyto-080614-120357
Li, B., Yang, Y., Ma, L., Ju, F., Guo, F., Tiedje, J. M., et al. (2015). Metagenomic and network analysis reveal wide distribution and co-occurrence of environmental antibiotic resistance genes. ISME J. 9:2490. doi: 10.1038/ismej.2015.59
Lin, Z., Bai, J., Zhen, Z., Lao, S., Li, W., Wu, Z., et al. (2016). Enhancing pentachlorophenol degradation by vermicomposting associated bioremediation. Ecol. Eng. 87, 288–294. doi: 10.1016/j.ecoleng.2015.12.004
Lloret, E., Pascual, J. A., Brodie, E. L., Bouskill, N. J., Insam, H., Juárez, M. F. D., et al. (2016). Sewage sludge addition modifies soil microbial communities and plant performance depending on the sludge stabilization process. Appl. Soil Ecol. 101, 37–46. doi: 10.1016/j.apsoil.2016.01.002
Luby, E., Ibekwe, A. M., Zilles, J., and Pruden, A. (2016). Molecular methods for assessment of antibiotic resistance in agricultural ecosystems: prospects and challenges. J. Environ. Qual. 45, 441–453. doi: 10.2134/jeq2015.07.0367
Maaß, S., Daphi, D., Lehmann, A., and Rillig, M. C. (2017). Transport of microplastics by two collembolan species. Environ. Poll. 225, 456–459. doi: 10.1016/j.envpol.2017.03.009
Madejón, P., Domínguez, M. T., Madejón, E., Cabrera, F., Marañón, T., and Murillo, J. M. (2018). Soil-plant relationships and contamination by trace elements: a review of twenty years of experimentation and monitoring after the Aznalcóllar (SW Spain) mine accident. Sci. Total Environ. 625, 50–63. doi: 10.1016/j.scitotenv.2017.12.277
Mahon, A. M., O'connell, B., Healy, M. G., O'connor, I., Officer, R., Nash, R., et al. (2017). Microplastics in sewage sludge: effects of treatment. Environ. Sci. Technol. 51, 810–818. doi: 10.1021/acs.est.6b04048
Mai, L., Bao, L. J., Wong, C. S., and Zeng, E. Y. (2018). “Microplastics in the terrestrial environment,” in Microplastic Contamination in Aquatic Environments, ed E. Zeng (Amsterdam: Elsevier), 365–378.
Manaia, C. M., Macedo, G., Fatta-Kassinos, D., and Nunes, O. C. (2016). Antibiotic resistance in urban aquatic environments: can it be controlled? Appl. Microbiol. Biotechnol. 100, 1543–1557. doi: 10.1007/s00253-015-7202-0
Mao, D., Yu, S., Rysz, M., Luo, Y., Yang, F., Li, F., et al. (2015). Prevalence and proliferation of antibiotic resistance genes in two municipal wastewater treatment plants. Water Res. 85, 458–466. doi: 10.1016/j.watres.2015.09.010
Marti, R., Scott, A., Tien, Y. C., Murray, R., Sabourin, L., Zhang, Y., et al. (2013). The impact of manure fertilization on the abundance of antibiotic-resistant bacteria and frequency of detection of antibiotic resistance genes in soil, and on vegetables at harvest. Appl. Environ. Microbiol. 79, 5701–5709. doi: 10.1128/AEM.01682-13
Marti, R., Tien, Y. C., Murray, R., Scott, A., Sabourin, L., and Topp, E. (2014). Safely coupling livestock and crop production systems: how rapidly do antibiotic resistance genes dissipate in soil following a commercial application of swine or dairy manure? Appl. Environ. Microbiol. 80, 3258–3265. doi: 10.1128/AEM.00231-14
Martínez-Blanco, J., Lazcano, C., Christensen, T. H., Muñoz, P., Rieradevall, J., Møller, J., et al. (2013). Compost benefits for agriculture evaluated by life cycle assessment. A review. Agron. Sustain. Dev. 33, 721–732. doi: 10.1007/s13593-013-0148-7
Masciandaro, G., Macci, C., Peruzzi, E., Ceccanti, B., and Doni, S. (2013). Organic matter-microorganism-plant in soil bioremediation: a synergic approach. Rev. Environ. Sci. Biotechnol. 12, 399–419. doi: 10.1007/s11157-013-9313-3
Meghvansi, M. K., and Varma, A. (eds.). (2015). Organic Amendments and Soil Suppressiveness in Plant Disease Management. London: Springer.
Mintenig, S. M., Int-Veen, I., Löder, M. G., Primpke, S., and Gerdts, G. (2017). Identification of microplastic in effluents of waste water treatment plants using focal plane array-based micro-Fourier-transform infrared imaging. Water Res. 108, 365–372. doi: 10.1016/j.watres.2016.11.015
Miyazaki, J., Kuriyama, Y., Miyamoto, A., Tokumoto, H., Konishi, Y., and Nomura, T. (2014). Adhesion and internalization of functionalized polystyrene latex nanoparticles toward the yeast Saccharomyces cerevisiae. Adv. Powder Technol. 25, 1394–1397. doi: 10.1016/j.apt.2014.06.014
Montiel-Rozas, M. M., López-García, Á., Kjøller, R., Madejón, E., and Rosendahl, S. (2016). Organic amendments increase phylogenetic diversity of arbuscular mycorrhizal fungi in acid soil contaminated by trace elements. Mycorrhiza 26, 575–585. doi: 10.1007/s00572-016-0694-3
Montiel-Rozas, M. M., López-García, Á., Madejón, P., and Madejón, E. (2017). Native soil organic matter as a decisive factor to determine the arbuscular mycorrhizal fungal community structure in contaminated soils. Biol. Fertil. Soils 53, 327–338. doi: 10.1007/s00374-017-1181-5
Mutua, P. M., Gicheru, M. M., Makanya, A. N., and Kiama, S. G. (2011). Comparative quantitative and qualitative attributes of the surface respiratory macrophages in the domestic duck and the rabbit. Int. J. Morphol. 29, 353–362. doi: 10.4067/S0717-95022011000200008
Németh, J., and Langhorst, J. (2014). Rethinking urban transformation: temporary uses for vacant land. Cities 40, 143–150. doi: 10.1016/j.cities.2013.04.007
Ng, E. L., Lwanga, E. H., Eldridge, S. M., Johnston, P., Hu, H. W., Geissen, V., et al. (2018). An overview of microplastic and nanoplastic pollution in agroecosystems. Sci. Total Environ. 627, 1377–1388. doi: 10.1016/j.scitotenv.2018.01.341
Ninh, H. T., Grandy, A. S., Wickings, K., Snapp, S. S., Kirk, W., and Hao, J. (2015). Organic amendment effects on potato productivity and quality are related to soil microbial activity. Plant Soil 386, 223–236. doi: 10.1007/s11104-014-2223-5
Nizzetto, L., Futter, M., and Langaas, S. (2016). Are agricultural soils dumps for microplastics of urban origin? Environ. Sci. Technol. 50, 10777–10779. doi: 10.1021/acs.est.6b04140
Nomura, T., Tani, S., Yamamoto, M., Nakagawa, T., Toyoda, S., Fujisawa, E., et al. (2016). Cytotoxicity and colloidal behavior of polystyrene latex nanoparticles toward filamentous fungi in isotonic solutions. Chemosphere 149, 84–90. doi: 10.1016/j.chemosphere.2016.01.091
Obrycki, J. F., Basta, N. T., and Culman, S. W. (2017). Management options for contaminated urban soils to reduce public exposure and maintain soil health. J. Environ. Qual. 46, 420–430. doi: 10.2134/jeq,2016.07.0275
OECD Guidelines for Testing of Chemicals No. 208 (2006). Terrestrial Plant Test: Seedling Emergence and Seedling Growth Test.
Ondoño, S., Bastida, F., and Moreno, J. L (2014) Microbiological biochemical properties of artificial substrates: a preliminary study of its application as Technosols or as a basis in Green Roof Systems. Ecol. Eng. 70, 189–199. doi: 10.1016/j.ecoleng.2014.05.003
OPPTS 850.4200 (1996). Ecological Effect Guideline. Seedling Germination/Root elongation Toxicity Tests. US EPA.
Paleari, S. (2017). Is the European union protecting soil? A critical analysis of community environmental policy and law. Land Use Policy 64, 163–173. doi: 10.1016/j.landusepol.2017.02.007
Pardo, T., Clemente, R., Epelde, L., Garbisu, C., and Bernal, M. P. (2014). Evaluation of the phytostabilisation efficiency in a trace elements contaminated soil using soil health indicators. J. Hazard. Mater. 268, 68–76. doi: 10.1016/j.jhazmat.2014.01.003
Park, J. H., Lamb, D., Paneerselvam, P., Choppala, G., Bolan, N., and Chung, J. W. (2011). Role of organic amendments on enhanced bioremediation of heavy metal(loid) contaminated soils. J. Hazard. Mater. 185, 549–574. doi: 10.1016/j.jhazmat.2010.09.082
Peng, S., Feng, Y., Wang, Y., Guo, X., Chu, H., and Lin, X. (2017). Prevalence of antibiotic resistance genes in soils after continually applied with different manure for 30 years. J. Hazard. Mater. 340, 16–25. doi: 10.1016/j.jhazmat.2017.06.059
Pérez-Esteban, J., Escolástico, C., Moliner, A., Masaguer, A., and Ruiz-Fernández, J. (2014). Phytostabilization of metals in mine soils using Brassica juncea in combination with organic amendments. Plant Soil 377, 97–109. doi: 10.1007/s11104-013-1629-9
Petrillo, J. E., and Ogunseitan, O. A. (2018). Emerging issues in the environmental context of antibiotic-resistance. Environ Int. 116, 39–42. doi: 10.1016/j.envint.2018.03.049
Philippot, L., Raaijmakers, J. M., Lemanceau, P., and van der Putten, W. H. (2013). Going back to the roots: the microbial ecology of the rhizosphere. Nat. Rev. Microbiol. 11, 789–799. doi: 10.1038/nrmicro3109
PlasticsEurope (2017). Available online at: https://www.plasticseurope.org/en/resources/publications (Accessed August 20, 2018).
Preston, F. (2012). A Global Redesign?: Shaping the Circular Economy. Briefing Paper. London: Chatham House.
Purnomo, A. S., Koyama, F., Mori, T., and Kondo, R. (2010). DDT degradation potential of cattle manure compost. Chemosphere 80, 619–624. doi: 10.1016/j.chemosphere.2010.04.059
Rahube, T. O., Marti, R., Scott, A., Tien, Y. C., Murray, R., Sabourin, L., et al. (2014). Impact of fertilizing with raw or anaerobically-digested sewage sludge on the abundance of antibiotic-resistant coliforms, antibiotic resistance genes and pathogenic bacteria in soil, and on vegetables at harvest. Appl. Environ. Microbiol. 80, 6898–6907. doi: 10.1128/AEM.02389-14
Reardon, C. L., and Wuest, S. B. (2016). Soil amendments yield persisting effects on the microbial communities-a 7-year study. Appl. Soil Ecol. 101, 107–116. doi: 10.1016/j.apsoil.2015.12.013
Reeve, J. R., Hoagland, L. A., Villalba, J. J., Carr, P. M., Atucha, A., Cambardella, C., et al. (2016). “Organic farming, soil health, and food quality: considering possible links,” in Advances in Agronomy, Vol. 137, ed. D. L. Sparks (Cambridge, MA: Elsevier Academic Press), 319–367.
Ren, X., Zeng, G., Tang, L., Wang, J., Wan, J., Wang, J., et al. (2017). The potential impact on the biodegradation of organic pollutants from composting technology for soil remediation. Waste Manag. 72, 138–149. doi: 10.1016/j.wasman.2017.11.032
Rillig, M. C., and Bonkowski, M. (2018). Microplastic and soil protists: a call for research. Environ. Poll. 241, 1128–1131. doi: 10.1016/j.envpol.2018.04.147
Rillig, M. C., Ingraffia, R., and de Souza Machado, A. A. (2017). Microplastic incorporation into soil in agroecosystems. Front. Plant Sci. 8:1805. doi: 10.3389/fpls.2017.01805
Rizzo, L., Manaia, C., Merlin, C., Schwartz, T., Dagot, C., Ploy, M. C., et al. (2013). Urban wastewater treatment plants as hotspots for antibiotic resistant bacteria and genes spread into the environment: a review. Sci. Total Environ. 447, 345–360. doi: 10.1016/j.scitotenv.2013.01.032
Rocha-Santos, T., and Duarte, A. C. (2015). A critical overview of the analytical approaches to the occurrence, the fate and the behavior of microplastics in the environment. Trends Analyt. Chem. 65, 47–53. doi: 10.1016/j.trac.2014.10.011
Rodrigo-Comino, J., Martínez-Hernández, C., Iserloh, T., and Cerd,à, A. (2017). The contrasted impact of land abandonment on soil erosion in Mediterranean agriculture fields. Pedosphere 28, 617–631. doi: 10.1016/S1002-0160(17)60441-7
Rodriguez-Seijo, A., Lourenço, J., Rocha-Santos, T. A. P., da Costa, J., Duarte, A. C., Vala, H., et al. (2017). Histopathological and molecular effects of microplastics in Eisenia andrei Bouché. Environ. Pollut. 220, 495–503. doi: 10.1016/j.envpol.2016.09.092
Ros, M., Pascual, J. A., Garcia, C., Hernandez, M. T., and Insam, H (2006) Hydrolase activities, microbial biomass bacterial community in a soil after long-term amendment with different composts. Soil Biol. Biochem. 38, 3443–3452. doi: 10.1016/j.soilbio.2006.05.017
Ross, J., and Topp, E. (2015). Abundance of antibiotic resistance genes in bacteriophage following soil fertilization with dairy manure or municipal biosolids, and evidence for potential transduction. Appl. Environ. Microbiol. 81, 7905–7913. doi: 10.1128/AEM.02363-15
Saison, C., Degrange, V., Oliver, R., Millard, P., Commeaux, C., Montange, D., et al. (2006). Alteration and resilience of the soil microbial community following compost amendment: effects of compost level and compost-borne microbial community. Environ. Microbiol. 8, 247–257. doi: 10.1111/j.1462-2920.2005.00892.x
Sandberg, K. D., and LaPara, T. M. (2016). The fate of antibiotic resistance genes and class 1 integrons following the application of swine and dairy manure to soils. FEMS Microbiol. Ecol. 92:fiw001. doi: 10.1093/femsec/fiw001
Saveyn, H., and Eder, P. (2014). End-of-Waste Criteria for Biodegradable Waste Subjected to Biological Treatment (Compost & Digestate): Technical Proposals. Publications Office of the European Union.
Schreuder, R., and De Visser, C. (2014). EIP AGRI focus group protein crops: final report. European Innovation Partnership for Agricultural Productivity and Sustainability (EIP AGRI). Brussels. Available online at: https://ec.europa.eu/eip/agriculture/en/publications/eip-agri-focus-group-protein-crops-final-report (Accessed May 21, 2018).
Scotti, R., Bonanomi, G., Scelza, R., Zoina, A., and Rao, M. A. (2015). Organic amendments as sustainable tool to recovery fertility in intensive agricultural systems. J. Soil Sci. Plant Nutr. 15, 333–352. doi: 10.4067/S0718-95162015005000031
Semblante, G. U., Hai, F. I., Huang, X., Ball, A. S., Price, W. E., and Nghiem, L. D. (2015). Trace organic contaminants in biosolids: impact of conventional wastewater and sludge processing technologies and emerging alternatives. J. Hazard. Mater. 300, 1–17. doi: 10.1016/j.jhazmat.2015.06.037
Sharma, A., and Nagpal, A. K. (2017). Soil amendments: a tool to reduce heavy metal uptake in crops for production of safe food. Rev. Environ. Sci. Biotechnol. 7, 187–203. doi: 10.1007/s11157-017-9451-0
Singer, A. C., Shaw, H., Rhodes, V., and Hart, A. (2016). Review of antimicrobial resistance in the environment and its relevance to environmental regulators. Front. Microbiol. 7:1728. doi: 10.3389/fmicb.2016.01728
Song, X., Liu, M., Wu, D., Griffiths, B., Jiao, J., Li, H., et al. (2015). Interaction matters: synergy between vermicompost and PGPR agents improves soil quality, crop quality and crop yield in the field. Appl. Soil Ecol. 89, 25–34. doi: 10.1016/j.apsoil.2015.01.005
Su, J. Q., Wei, B., Xu, C. Y., Qiao, M., and Zhu, Y. G. (2014). Functional metagenomic characterization of antibiotic resistance genes in agricultural soils from China. Environ. Int. 65, 9–15. doi: 10.1016/j.envint.2013.12.010
Talvitie, J., Mikola, A., Koistinen, A., and Setälä, O. (2017). Solutions to microplastic pollution-removal of microplastics from wastewater effluent with advanced wastewater treatment technologies. Water Res. 123, 401–407. doi: 10.1016/j.watres.2017.07.005
Taylor, M. L., Gwinnett, C., Robinson, L. F., and Woodall, L. C. (2016). Plastic microfibre ingestion by deep-sea organisms. Sci. Rep. 6:33997. doi: 10.1038/srep33997
Tejada, M., and Benítez, C. (2014). Effects of crushed maize straw residues on soil biological properties and soil restoration. Land Degrad. Dev. 25, 501–509. doi: 10.1002/ldr.2316
Termorshuizen, A. J., van Rijn, E., van der Gaag, D. J., Alabouvette, C., Chen, Y., Lagerlöf, J., et al. (2007). Suppressiveness of 18 composts against 7 pathosystems: variability in pathogen response. Soil Biol. Biochem. 38, 2461–2477. doi: 10.1016/j.soilbio.2006.03.002
Thangarajan, R., Bolan, N. S., Tian, G., Naidu, R., and Kunhikrishnan, A. (2013). Role of organic amendment application on greenhouse gas emission from soil. Sci. Total Environ. 465, 72–96. doi: 10.1016/j.scitotenv.2013.01.031
Thanner, S., Drissner, D., and Walsh, F. (2016). Antimicrobial resistance in agriculture. MBio 7:e02227-15. doi: 10.1128/mBio.02227-15
Tian, J., Lou, Y., Gao, Y., Fang, H., Liu, S., Xu, M., et al. (2017). Response of soil organic matter fractions and composition of microbial community to long-term organic and mineral fertilization. Biol. Fertil. Soils 53, 523–532. doi: 10.1007/s00374-017-1189-x
Tian, W., Wang, L., Li, Y., Zhuang, K., Li, G., Zhang, J., et al. (2015). Responses of microbial activity, abundance, and community in wheat soil after three years of heavy fertilization with manure-based compost and inorganic nitrogen. Agric. Ecosyst. Environ. 213, 219–227. doi: 10.1016/j.agee.2015.08.009
Tien, Y. C., Li, B., Zhang, T., Scott, A., Murray, R., Sabourin, L., et al. (2017). Impact of dairy manure pre-application treatment on manure composition, soil dynamics of antibiotic resistance genes, and abundance of antibiotic-resistance genes on vegetables at harvest. Sci. Total Environ. 581, 32–39. doi: 10.1016/j.scitotenv.2016.12.138
Turmel, M. S., Speratti, A., Baudron, F., Verhulst, N., and Govaerts, B. (2015). Crop residue management and soil health: a systems analysis. Agric. Syst. 134, 6–16. doi: 10.1016/j.agsy.2014.05.009
Udikovic-Kolic, N., Wichmann, F., Broderick, N. A., and Handelsman, J. (2014). Bloom of resident antibiotic-resistant bacteria in soil following manure fertilization. Proc. Natl. Acad. Sci. U.S.A. 111, 15202–15207. doi: 10.1073/pnas.1409836111
UNFCCC (2015). “Join the 4/1000 Initiative. Soils for Food Security and Climate. Lima- Paris Action Agenda.
United Nations (UN) (2016). Draft Political Declaration of the High-Level Meeting of the General Assembly on Antimicrobial Resistance. Available online at: https://www.un.org/pga/71/wp-content/uploads/sites/40/2016/09/DGACM_GAEAD_ESCAB-AMR-Draft-Political-Declaration-1616108E.pdf (Accessed August 21, 2018).
United Nations Environment Programme (UNEP) (2015) Biodegradable Plastics and Marine Litter: Misconceptions Concerns and Impacts on Marine Environments. Nairobi: United Nations Publications.
Urra, J., Alkorta, I., Mijangos, I., Epelde, L., and Garbisu, C. (2018). Application of sewage sludge to agricultural soil increases the abundance of antibiotic resistance genes without altering the composition of prokaryotic communities. Sci. Total Environ. 647, 1410–1420. doi: 10.1016/j.scitotenv.2018.08.092
Venegas, A., Rigol, A., and Vidal, M. (2015). Viability of organic wastes and biochars as amendments for the remediation of heavy metal-contaminated soils. Chemosphere 119, 190–198. doi: 10.1016/j.chemosphere.2014.06.009
Vimal, S. R., Singh, J. S., Arora, N. K., and Singh, S. (2017). Soil-plant-microbe interactions in stressed agriculture management: a review. Pedosphere 27, 177–192. doi: 10.1016/S1002-0160(17)60309-6
Wang, F. H., Qiao, M., Chen, Z., Su, J. Q., and Zhu, Y. G. (2015). Antibiotic resistance genes in manure-amended soil and vegetables at harvest. J. Hazard. Mater. 299, 215–221. doi: 10.1016/j.jhazmat.2015.05.028
Wang, J., Lv, S., Zhang, M., Chen, G., Zhu, T., Zhang, S., et al. (2016). Effects of plastic film residues on occurrence of phthalates and microbial activity in soils. Chemosphere 151, 171–177. doi: 10.1016/j.chemosphere.2016.02.076
World Health Organization (WHO) (2014). Antimicrobial Resistance: Global Report on Surveillance. Available online at: http://www.who.int/drugresistance/documents/surveillancereport/en/ (Accessed August 21, 2018).
World Health Organization (WHO) (2015). Global Action Plan on Antimicrobial Resistance. Available online at: http://apps.who.int/iris/handle/10665/193736 (Accessed August 21, 2018).
Wu, Y., Li, Y., Zheng, C., Zhang, Y., and Sun, Z. (2013). Organic amendment application influence soil organism abundance in saline alkali soil. Eur. J. Soil Biol. 54, 32–40. doi: 10.1016/j.ejsobi.2012.10.006
Xie, W. Y., Shen, Q., and Zhao, F. J. (2018). Antibiotics and antibiotic resistance from animal manures to soil: a review. Eur J Soil Sci. 69, 181–195. doi: 10.1111/ejss.12494.
Xue, D., and Huang, X. (2013). The impact of sewage sludge compost on tree peony growth and soil microbiological, and biochemical properties. Chemosphere 93:583. doi: 10.1016/j.chemosphere.2013.05.065
Yang, Q., Ren, S., Niu, T., Guo, Y., Qi, S., Han, X., et al. (2014). Distribution of antibiotic-resistant bacteria in chicken manure and manure-fertilized vegetables. Environ. Sci. Pollut. Res. Int. 21, 1231–1241. doi: 10.1007/s11356-013-1994-1
Zhang, H., Li, X., Yang, Q., Sun, L., Yang, X., Zhou, M., et al. (2017). Plant growth, antibiotic uptake, and prevalence of antibiotic resistance in an endophytic system of pakchoi under antibiotic exposure. Int. J. Environ. Res. Public Health 14:1336. doi: 10.3390/ijerph14111336
Zhao, S., Zhu, L., and Li, D. (2016). Microscopic anthropogenic litter in terrestrial birds from Shanghai, China: not only plastics but also natural fibers. Sci. Total Environ. 550, 1110–1115. doi: 10.1016/j.scitotenv.2016.01.112
Zhao, Y., Wang, P., Li, J., Chen, Y., Ying, X., and Liu, S. (2009). The effects of two organic manures on soil properties and crop yields on a temperate calcareous soil under a wheat-maize cropping system. Eur. J. Agron. 31, 36–42. doi: 10.1016/j.eja.2009.03.001
Zhou, R., Liu, X., Luo, L., Zhou, Y., Wei, J., Chen, A., et al. (2017). Remediation of Cu, Pb, Zn and Cd-contaminated agricultural soil using a combined red mud and compost amendment. Int. Biodeterior. Biodegr. 118, 73–81. doi: 10.1016/j.ibiod.2017.01.023
Zhu, B., Chen, Q., Chen, S., and Zhu, Y. G. (2017). Does organically produced lettuce harbor higher abundance of antibiotic resistance genes than conventionally produced? Environ. Int. 98, 152–159. doi: 10.1016/j.envint.2016.11.001
Zhu, D., Chen, Q. L., An, X. L., Yang, X. R., Christie, P., Ke, X., et al. (2018). Exposure of soil collembolans to microplastics perturbs their gut microbiota and alters their isotopic composition. Soil Biol. Biochem. 116, 302–310. doi: 10.1016/j.soilbio.2017.10.027
Ziajahromi, S., Neale, P., Rintoul, L., and Leusch, F. (2017). Wastewater treatment plants as a pathway for microplastics: development of a new approach to sample wastewater-based microplastics. Water Res. 112, 93–99. doi: 10.1016/j.watres.2017.01.042
Zornoza, R., Acosta, J. A., Faz, A., and Bååth, E. (2016). Microbial growth and community structure in acid mine soils after addition of different amendments for soil reclamation. Geoderma 272, 64–72. doi: 10.1016/j.geoderma.2016.03.007
Keywords: circular economy, degraded soils, contamination, microplastics, antibiotic-resistance genes, soil health, soil quality
Citation: Gómez-Sagasti MT, Hernández A, Artetxe U, Garbisu C and Becerril JM (2018) How Valuable Are Organic Amendments as Tools for the Phytomanagement of Degraded Soils? The Knowns, Known Unknowns, and Unknowns. Front. Sustain. Food Syst. 2:68. doi: 10.3389/fsufs.2018.00068
Received: 27 June 2018; Accepted: 24 September 2018;
Published: 16 October 2018.
Edited by:
Paula Alvarenga, Instituto Superior de Agronomia (ISA), PortugalReviewed by:
Engracia Madejon, Institute of Natural Resources and Agrobiology of Seville (CSIC), SpainRaul Zornoza, Universidad Politécnica de Cartagena, Spain
Copyright © 2018 Gómez-Sagasti, Hernández, Artetxe, Garbisu and Becerril. This is an open-access article distributed under the terms of the Creative Commons Attribution License (CC BY). The use, distribution or reproduction in other forums is permitted, provided the original author(s) and the copyright owner(s) are credited and that the original publication in this journal is cited, in accordance with accepted academic practice. No use, distribution or reproduction is permitted which does not comply with these terms.
*Correspondence: María T. Gómez-Sagasti, bWFyaWF0ZXJlc2EuZ29tZXpAZWh1LmV1cw==