- Technology Transfer Centre, Fondazione Edmund Mach, San Michele all'Adige, Italy
Sustainable livestock effluent management is becoming an increasingly important issue in mountain areas, with particular regard to the agro-environmental performance of forage production and the social acceptability of organic fertilizer application in mixed urban-rural contexts. The present paper proposes a GIS-based methodological approach to the management and planning of digestate spreading on hay meadows, based on the integration of geo-morphological, agro-botanical and spatio-functional data about cultivated agricultural land. The proposed methodology was tested in a case-study Community of the Italian Alps, with seven dairy farms operating an anaerobic digestion plant. Nitrogen production by cattle was quantified and compared to sustainable nitrogen requirements of cut meadows, computed at the single-plot level through agro-botanical typization of swards. Subsequently, spreading restrictions provided by national and local regulations were spatially implemented. Farm-tailored nitrogen balances and digestate spreading plans were designed to help livestock farms adjust effluent spreading patterns according to meadow type and surface runoff risk. Findings are transferrable to other mountain regions based on cattle farming and grassland management.
Introduction
The economy of alpine areas is based on tourism and livestock farming, which ensures the attractiveness of rural territories by providing high-quality traditional dairy products and maintaining typical, bio-diverse cultivated landscapes (Bätzing, 2005; Tappeiner et al., 2008; Tasser et al., 2013).
One of the main critical issues related to the co-existence of animal husbandry and other human activities, tourism included, is the odor impact of livestock breeding and effluent spreading (Lohr, 1996; Miner et al., 2000; Schauberger et al., 2001; Copeland, 2007). National and local legislation provide spreading restrictions in order to minimize the odor emissions of organic fertilization, but issues persist.
Anaerobic digestion of livestock effluents represents, in some areas, a viable solution. Scientific and practical evidence demonstrates that anaerobic digestion significantly reduces the odor potential of livestock effluents, with measured reduction values up to 80% compared to untreated slurry (Powers et al., 1999; Immovilli et al., 2008; Hjorth et al., 2009; Riva et al., 2016).
Considering the small size of livestock farms in alpine areas (Streifeneder, 2009), anaerobic digestion plants have to be planned, constructed and run by several farms together, grouped in cooperatives or enterprise networks (Blome-Drees et al., 2016). This is necessary not only to take advantage of economies of scale, but also to tackle odor issues at a larger level.
In addition, creating multiple-farm cooperation in livestock effluent management represents a chance to address the sustainable use of organic fertilizers on alpine meadows. The polarization of agricultural use between valley floors (intensification) and slopy areas (extensification or abandonment) has been generating the progressive degradation of the environmental and agronomic quality of hay meadows in mountain areas, provoking questions about the disequilibrium between nutrient inputs and outputs (Cernusca et al., 1996; Gubert, 2008; Penati et al., 2008; Zimmermann et al., 2010; Scotton et al., 2014). Collecting and processing all livestock effluents of an area in a common anaerobic digestion plant offers the opportunity to comprehensively plan nutrient restitution to agricultural land, thus ensuring a balanced organic fertilization and a progressive improvement of the agro-environmental performance of cut meadows (Holm-Nielsen et al., 2009).
Alpine meadows represent not only an important element of biological diversity and landscape quality (Dietl and Jourquera, 2004), but they are the main forage source for alpine farms, binding milk production to the terroir (Rubino, 2006). Therefore, improving meadow quality means increasing forage self-sufficiency at the farm scale and strengthening the bond between typical cheese production and the agro-environment of origin (Penati et al., 2013; Battaglini et al., 2014).
The overall objective of the present study is to provide livestock farms operating in alpine environments with innovative tools for a sustainable use of livestock effluents, in particular digestate, in order to maintain both meadow biodiversity and productivity. The new methodological approach proposed, based on GIS tools, was developed for an anaerobic digestion plant in Trentino (Eastern Italian Alps) but it is transferrable to any other alpine context with rural economies built on livestock farming. The expected outcome is the definition of farm-tailored nitrogen balances and digestate spreading plans, indicating spatial and temporal patterns of digestate use at the plot scale.
Materials and Methods
Framework
The area of interest of the present study is the Community of Predazzo, in the Autonomous Province of Trento (Eastern Italian Alps). A cooperative of seven dairy farmers plans the construction of an anaerobic digestion plant, in order to improve the management of animal waste in terms of odor impact of effluent spreading and sustainable nutrient supply to cut meadows. As required by national and local legislation, the anaerobic digestion plant has to be provided with an Agronomic Utilization Plan (AUP) of digestate, certifying the equilibrium between nitrogen produced by livestock and nitrogen needed by cultivated land. The design of the AUP offers the chance to propose a new, transferrable approach for the sustainable management of livestock effluents in an alpine context, where cut meadows represent the dominant type of agricultural land use.
Figure 1 represents the methodological scheme adopted and the expected outputs. Data collected through direct on-farm survey, regarding in particular herd size and composition, were used to calculate nitrogen production, net of any losses from excretion to the field. On the other hand, spatialized data about cut meadow plots were used to estimate nitrogen requirements of cultivated land, in order to produce farm-scale nitrogen balances and digestate spreading plans with cartographic implementation. Further detail is provided in the following sections.
A similar approach was proposed by Peratoner et al. (2010) for the calculation of forage balances at the regional scale. Combining the extent of grassland areas, subdivided according to meadow type and altitude ranges, with their average yield potential, with livestock population and with mean daily demand of different livestock classes, Peratoner et al. (2010) were able to compute a forage balance and a forage self-supply rate for South Tyrol. The present study goes a step further, providing new methodological tools which allow to tackle agro-environmental issues of livestock farming (such as nutrient management) both at the regional and at the single-farm level.
Data Collection Through Farm Surveys
Initial data about herd size, herd composition, housing system, current effluent management and summer grazing of cattle were collected through farm surveys, in order to quantify housing-related nitrogen produced by each farm on a yearly basis. Nitrogen not related to housing, produced during summer grazing, was calculated taking into account the number of days spent by cattle on alpine pastures and was subtracted from the yearly amount, as it is not involved in organic fertilization of hay meadows through effluent spreading. Grazing on hay meadows is not a common practice and was therefore not considered.
Standard field nitrogen values provided by Italian national legislation, specifically the Ministerial Decree April 7, 2006 (MIPAAF, 2006), were applied, as reported in Table 1. Field nitrogen refers to the nitrogen reaching the field, net of any losses occurring from excretion to the meadow. Nitrogen efficiency related to spreading patterns was not considered at this stage but was included in the nitrogen balancing step.
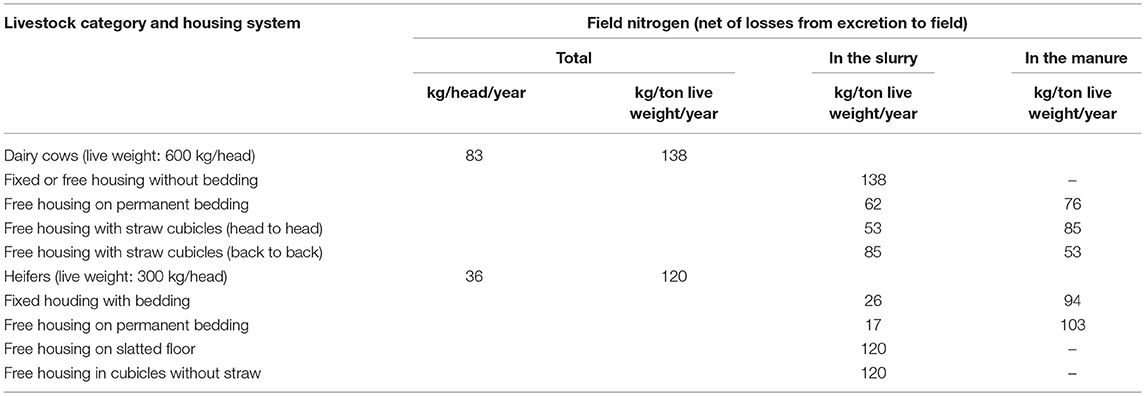
Table 1. Standard values of fild nitrogen according to the Ministerial Decree April 7, 2006 for dairy cows and heifers, depending on housing system (MIPAAF, 2006).
GIS-Based Data Management Framework
Dealing with spatial patterns of digestate spreading requires a common data framework with spatial reference, in which existing information layers, i.e., slope maps, infrastructure maps, cadastral maps, can be integrated with newly processed or collected data, i.e., farm meadow plots, areas with spreading restrictions, agro-botanical meadow types. Such framework was created for the area of interest using a commercial GIS-application, acquiring existing data from the Geo-Cartographic Portal of the Autonomous Province of Trento (PAT, 2015).
Digitalization of Meadow Plots
One of the preliminary steps was to convert existing alfa-numeric data regarding cut meadows into a spatial layer, which contains the polygons of cadastral plots cultivated by each farm, net of non-productive areas such as trees, roads and buildings, with information about the user and net cultivated surface. This layer represents the base for further data collection and processing. Alpha-numeric data about cut meadows plots, net cultivated surfaces and meadow users were acquired from the Provincial Agency for Payments in Agriculture of the Autonomous Province of Trento (APPAG, 2015). Apart from alpine pastures, which are grazed during the summer months, cut meadows for hay production represent the only type of agricultural land use in the area.
Agro-Botanical Typization of Meadow Plots
Digitalized meadow plots were characterized on-site from an agro-botanical point of view, using the typology of Trentino's hay meadows published by Scotton et al. (2012). The typology is based on a comprehensive characterization of terrain morphology, climatic conditions, geological and biological features as well as management practices. Agro-botanical surveys were extensively conducted on 500 hectares cut meadows at the beginning of the vegetation season and cartographically implemented.
The agro-botanical classification proposed by Scotton et al. (2012) has a high degree of complexity (44 botanic units and 17 botanic types) and requires some simplification for farm-oriented knowledge transfer. As proposed by La Notte et al. (2014), surveyed meadow plots were consequently grouped into three main categories, namely (a) valley floor meadows, classified by geo-morphologic parameters such as altitude and slope, (b) species-rich meadows, identified through agro-botanical surveys, and (c) slope meadows, not in (a) and (b). The three types of meadows have distinctive geo-spatial, botanical and management patterns (Gubert, 2015): valley floor meadows are located around the villages in the valley bottom, have low slope steepness and are intensively fertilized and cut (eutrophic), with poor botanical diversity; slope meadows are located at higher altitudes in steeper areas, have a less intensive cutting and fertilization regime (mesotrophic), with intermediate botanical diversity; species-rich meadows are located in marginal areas, with bad accessibility and low mechanizability, have very low utilization intensity (oligotrophic) and are characterized by an outstanding botanical value.
Nitrogen Balance of Meadow Types
The three types of meadow identified have different forage productivity and fertilization requirements (Gubert, 2015). In order to determine necessary nitrogen input through organic fertilization, and consequently necessary digestate volumes, a nitrogen balance was calculated for each meadow type, using the nitrogen balance formula proposed by MIPAAF (2006).
where:
Y, expected forage production, calculated using on-site productivity data provided by Scotton et al. (2012);
B, nitrogen removal coefficient, calculated using on-site forage quality data provided by Scotton et al. (2012);
Nc, nitrogen availability from previous crops, not applicable for permanent grassland;
Nf, nitrogen availability from fertilization of the previous year, not applicable for digestate;
An, net natural nitrogen inputs from dry and wet deposition and from the mineralization of the organic matter;
Kc, nitrogen efficiency coefficient for mineral fertilization, set equal to 1 as suggested by MIPAAF (2006);
Fc, nitrogen input from mineral fertilization, set equal to 0 as fertilization occurs only through digestate;
K0, nitrogen efficiency coefficient for organic fertilization, calculated according to MIPAAF (2006) depending on soil type and spreading period;
F0, nitrogen input from organic fertilization, which represents the “unknown” of the formula and corresponds to the sustainable amount of nitrogen that can be spread on field with organic fertilizers to cover nitrogen outputs, net of other nitrogen inputs.
Excretions during grazing on hay meadows was not considered, as hay meadows are not subject to grazing. Nitrogen input from mineral fertilization was set equal to zero, as mineral nitrogen is not used by farms on hay meadows due to unfavorable cost-benefit ratio.
As mentioned above, expected forage production (Y) and nitrogen removal coefficient (B) were calculated using forage quantity and quality data available for the area of interest, collected on 10 different sampling sites by Scotton et al. (2012). This allows to adapt calculation to the site-specific production potential of hay meadows. Nitrogen input from organic fertilization (F0) was calculated for each meadow type and represents the sustainable yearly amount of nitrogen to be spread through digestate on cut meadows per unit of area (ha).
As to net natural nitrogen inputs (An), dry and wet deposition as well as mineralization of the organic matter were considered. For nitrogen deposition, the standard value of 20 kg nitrogen per hectare and year proposed by MIPAAF (2006) was adopted. Rihm and Achermann (2016) report for Switzerland an average total nitrogen deposition of 16.3 kg per hectare and year, with values between 20 and 30 kg in the Southern Alps. Asel (2015) proposes for Austria a value of 20 kg nitrogen per hectare and year in nitrogen balancing on permanent grasslands. Net nitrogen from mineralization of organic matter was set equal to zero, as a permanent meadow subject to adequate cutting and organic fertilization reaches, on the long run, an equilibrium between nitrogen release and immobilization in the organic matter of the soil (T'Mannetje and Jarvins, 1990). Theiss (1989) and Kasper et al. (2015) show that permanent hay meadows in alpine environments, when fertilized with organic fertilizers, may even become net accumulators of organic nitrogen.
Cartographic Implementation of Spreading Restrictions
The net meadow area of a plot does not always correspond to the area on which effluent spreading is allowed. National and local legislation define spreading restrictions for livestock effluents, in order to minimize the impact of organic fertilization on other human activities (i.e., mobility, housing, public infrastructures) and on the environment (i.e., contamination of surface and groundwater through leaching and surface run-off). These restrictions reduce the net meadow area on which each farm is allowed to spread organic fertilizers.
Spreading restrictions for liquid effluents1 are summarized in Table 2. In order to identify and measure areas excluded from effluent application, spreading restrictions were spatialized using available layers from the Geo-Cartographic Portal of the Autonomous Province of Trento (PAT, 2015) and, where applicable, implementing the necessary buffer zones. Collected agro-botanical data were used for the localization of humid grasslands. Consequently, the meadow plot shapefile was intersected with the spreading restriction shapefile to highlight meadow areas where spreading is not allowed.
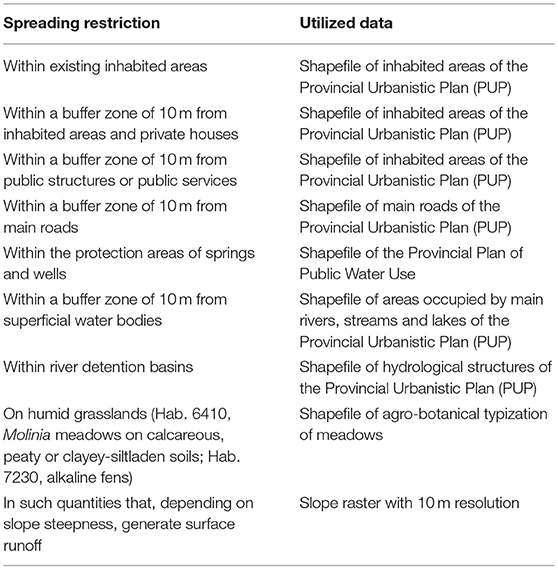
Table 2. Criteria adopted for the spatial definition of areas with spreading restrictions for liquid livestock effluents.
As to spreading limitations related to slope steepness, Italian national legislation sets a slope threshold of 10%, above which no spreading of liquid livestock effluents is allowed, except for specific local circumstances. In the alpine context of interest, around 70% of cut meadows have a slope steepness higher than 10%. For this reason, local legislation derogates to national legislation introducing the concept of “no runoff”: the spreading of liquid livestock effluents is not allowed if it generates surface runoff, depending on quantity spread and on slope steepness.
For the sake of the present study, a slope threshold of 40% was proposed. It was demonstrated that on meadows with slope steepness between 10 and 40% no surface runoff is expected from liquid digestate spreading, at given spreading volumes per operation and in absence of natural precipitation close to spreading.
For the calculation of the runoff potential of digestate spreading, the Runoff Curve Number method proposed by the USDA Soil Conservation Service was applied (USDA-SCS, 1985). This is an empirical hydrological method which allows to estimate surface runoff of a water precipitation depending on soil texture and soil cover. The spreading of liquid digestate was considered as comparable to a water precipitation, since the produced digestate is expected to have a very low dry-matter content. Representative effluent samples collected in Predazzo and digested at the Edmund Mach Foundation showed a total solids content around 5.5%. Comparable data on total solids content is provided by Landesanstalt für Landwirtschaft (2006) and Hjorth et al. (2010) for slurry-based digestates. In case of higher dry-matter contents, solid/liquid separation represents a viable solution to providing a clarified effluent fraction with water-like runoff behavior (Landesanstalt für Landwirtschaft, 2006).
The Runoff Curve Number method requires, in first instance, the definition of the soil hydrological group (A, B, C or D), which basically depends on soil texture. In the area of interest, soil texture data was available from Scotton et al. (2012) for 12 different meadow sites, with slope steepness between 10 and 40%. According to the USDA-SCS classification, all considered soils could be ascribed to the hydrological group B: soils in this group have moderately low runoff potential when thoroughly wet as water transmission through the soil is unimpeded, have typically loamy sand or sandy loam textures and the saturated hydraulic conductivity in the least transmissive layer between the surface and 50 centimeters ranges from 10.0 to 40.0 micrometers per second (USDA-SCS, 1985).
The soil hydrological group, together with soil cover (cut meadow), determine a table-based, non-dimensional Curve Number (CN) of 58. This value was corrected for available soil moisture by the Antecedent Moisture Condition (AMC) factor, which was taken at the highest level (moist soil) for precautionary reasons. Given an AMC factor of 1.3, the corrected CN was equal to 75.4.
CN is correlated to the potential maximum retention after runoff begins (S), in inches, according to the formula:
At a CN value of 75.4, S was equal to 3.3 inches. On its turn, S is correlated to runoff (Q), in inches, according to the formula:
where P is the precipitation (in inches) and Ia the initial abstraction (in inches), or the amount of water before runoff, such as infiltration, or rainfall interception by vegetation. For a precipitation lower than or equal to the initial abstraction, surface runoff can be considered equal to zero.
The initial abstraction Ia was calculated, according to USDA-SCS (1985), with the formula Ia = 0.2*S. However, several studies show that this ratio is too high when compared to field measurements (Jiang, 2001; Hawkins et al., 2002; Mishra and Singh, 2004; Baltas et al., 2007). Therefore, a more appropriate initial abstraction ratio, namely Ia = 0.05*S, was proposed according to Hawkins et al. (2002). A lower initial abstraction ratio determines a lower threshold precipitation amount for runoff and, accordingly, a lower maximum amount of digestate that can be spread on hay meadows. As a consequence, more surface is necessary to spread a given amount of digestate. This choice was supported by the Regional Agency for Environmental Protection as a precautionary measure to minimize the risk of surface-runoff.
Applying the latter formula for the calculation of initial abstraction, the threshold precipitation amount for runoff (P = Ia) on the considered soils was equal to 0.16 inches, corresponding to 4.06 millimeters. This means that the amount of liquid digestate per spreading operation that can be distributed on meadows with a slope steepness between 10 and 40% without any risk of surface runoff is equivalent to 4.06 millimeters, or approximately 41 cubic meters per hectare.
The slope threshold of 40% was integrated in the GIS data framework to compute, together with the other spatialized spreading restrictions, the net meadow surface available for spreading at the single-plot as well as at the farm level.
Farm Nitrogen Balance and Digestate Spreading Plans
The above described methodological steps allow to define, for each meadow plot, the meadow type, the corresponding yearly sustainable nitrogen input from organic fertilization, the net meadow surface available for spreading and, ultimately, the quantity of effluent which could be spread to cover actual nitrogen requirements on a yearly basis. Additional information about runoff-related threshold volumes of effluents was used to generate an agronomically viable and environmentally sustainable spreading plan, consisting of different volumes and frequencies of slurry application during the growing season depending on meadow type. Spreading plans could be spatially implemented both at the plot and at the farm scale. The comparison between nitrogen produced by cattle and actual nitrogen requirements of cultivated meadows allowed to quantify, for each farm, occurring nitrogen excess / deficit, thus highlighting potentially critical situations.
Results and Discussion
Farm Survey Findings
The seven farms surveyed breed dairy cows predominantly in free housing with slurry-based effluent management. To different extents, all farms bring their cattle on high-altitude pastures during the summer months, thus reducing housing-related nitrogen loads and effluent volumes by approximately 1/3. The declared total number of dairy cows was equal to 517 units, while the number of young cattle under 24 months was equal to 224 units. Nitrogen reaching the field was equal to 39,557 kg per year, corresponding to 9,922 cubic meters effluent volume. Total effluent stock capacity was equal to 13,368 cubic meters. This data was used not only for calculating nitrogen balances at the farm scale, but also for dimensioning the anaerobic digestion plant and its stock volumes.
The use of standard field nitrogen values proposed by national legislation presents two main limits. On the one hand, nitrogen excretions are calculated for intensive dairy farms of plain areas and may be over-estimated when applied to more extensive farming systems in mountain areas. For instance, the Region Valle d'Aosta in the North-Western Italian Alps has introduced lower nitrogen excretion values for local breeds compared with non-local ones (Francesia et al., 2008; Regione Autonoma Valle d'Aosta, 2017), whereas Steinwidder (2009) has calculated nitrogen excretion depending on milk productivity and protein intake. On the other hand, nitrogen losses occurring from excretion to the field are standardized and cannot be differentiated depending on effluent management practices. Adaptation of standard field nitrogen values to specific situations and to alpine conditions can therefore improve overall accuracy of calculation.
Digitalization and Agro-Botanical Typization of Meadow Plots
Total meadow surface cultivated by the seven farms, net of non-productive areas, was equal to 210.36 hectares, with a total number of 2,505 cadastral plots (840 square meters per plot on average). Spatial distribution of meadow plots on a single-farm basis confirm a high degree of land fragmentation and dispersion, as already assessed and measured by Bittante (2011) in over 1,000 dairy farms of the Province of Trento. Land parcelization is a peculiar trait of the Southern Alps and is one of the most evident weaknesses of mountain agriculture in these areas (Bätzing, 1992).
Due to the high degree of land parcelization and of land turn-over between farms, agro-botanical surveys were conducted independent of existing utilization patterns on all meadow surfaces of the Community of Predazzo, including agricultural land cultivated by farms not joining the anaerobic digestion project. At an aggregate level, 20 different agro-botanical types of meadows were recorded on approximately 500 hectares, ranging from oligotrophic, species-rich Nardus stricta grasslands to over-fertilized, almost mono-specific lowland Agropyron repens meadows. Slope areas were dominated by swards of the Centaure-transalpinae-Triseteum flavescentis community, while valley bottoms were predominantly characterized by more productive meadows of the Centaureo carniolicae-Arrhenaterum elatioris community. To date, the case study of Predazzo represents the vastest area of cartographic implementation of Scotton et al. (2012) agro-botanical typology in the region.
As previously described, the agro-botanical classification of cut meadows proposed by Scotton et al. (2012) is fairly complex. However, it allows to identify both meadows with outstanding ecological value, because of species-diversity or rarity, and meadows with agronomic problems related to utilization intensity, showing distrophic botanical compositions (e.g., high weed coverage). This information was used to produce farm-tailored “health-maps” of hay meadows, with specific management recommendations for the maintenance of species-rich swards and the agronomic improvement of degraded surfaces.
For the sake of transferability to farmers, the meadow plots managed by the seven farms were subsequently classified in three macro-categories. This classification allowed to cartographically identify 73.37 hectares valley floor meadows (34.9%), 95.63 hectares slope meadows (45.5%) and 41.36 hectares species-rich meadows (19.6%). As expected, considering the geo-morphological and pedological traits of the area, meadows with low intensification potential (slope meadows and species-rich meadows) represented the majority of cultivated land. Figure 2 shows a cartographic detail of agro-botanical meadow typization in a sample area of Predazzo.
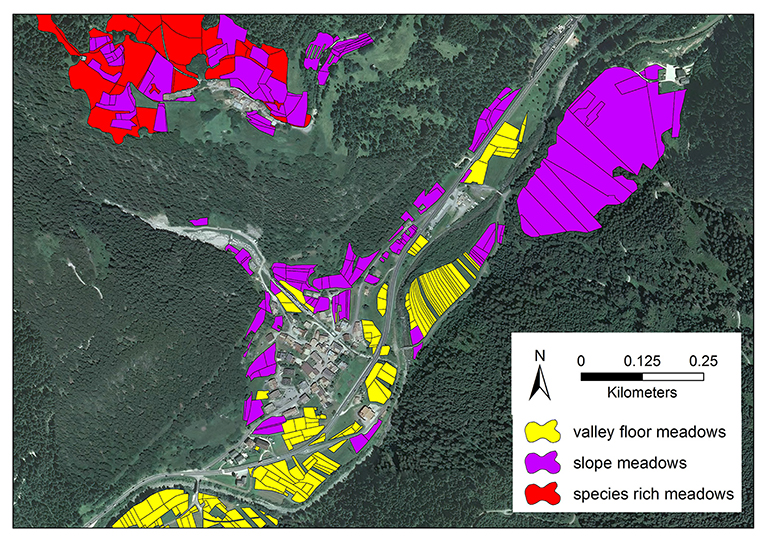
Figure 2. Cartographic detail of agro-botanical typization of cut meadows in a sample area of Predazzo.
Nitrogen Balance of Meadow Types
For each meadow type, a nitrogen balance was computed according to Equation (1). Table 3 reports the values used in the calculation and the resulting sustainable nitrogen input from organic fertilization (F0). Nitrogen exports, calculated multiplying expected meadow productivity by unitary nitrogen content of forage, is in line with average values reported by Scotton et al. (2012) for the entire Province of Trento. Comparable values are described by Buchgraber and Gindl (2004) in Austria and Dietl and Lehmann (2006) in Switzerland for meadows with similar production potentials.

Table 3. Values used in the calculation of the sustainable nitrogen input from organic fertilization (F0) for the three meadow types according to formula (1).
One of the key factors determining the sustainable nitrogen input from organic fertilization is the nitrogen efficiency coefficient (K0), calculated according to a table provided by MIPAAF (2006) depending on soil texture and spreading period. The resulting K0 value was equal to 50%, corresponding to the minimum level indicated by MIPAAF (2006) for liquid bovine effluents. This means that, on average, for every kg nitrogen spread on meadows through slurry or digestate, only 0.5 kg are actually absorbed by plants, while the remaining 0.5 kg are lost through leaching and volatilization (Stanley, 2014). A mean nitrogen efficiency value of 50% for bovine slurry is reported by Webb et al. (2010) in many nitrates action programmes of European Union Member States. More recent Italian legislation has introduced a minimum nitrogen efficiency coefficient for digestate of 60%, starting from the assumption that anaerobic digestion increases nitrogen availability to plants in digestate when compared to untreated effluents (MIPAAF, 2016). As the present study was carried in 2015, a K0 value of 50% was considered, as indicated by MIPAAF (2006). Different nitrogen efficiency coefficients, related either to legal thresholds or to different types of effluents, do not affect the proposed method.
Cartographic Implementation of Spreading Restrictions
The effluent spreading restrictions reported in Table 2 were cartographically implemented to identify and measure the portions of meadow plots subject to limitations in organic fertilization. Figure 3 shows a cartographic detail of this implementation in a sample area. All areas excluded from effluent application were subtracted from net meadow surface to compute the agricultural surface on which spreading is allowed. On average, 16% of net meadow surface was found to be excluded from spreading. Slope meadow represented the category most affected by spreading restrictions due to higher average slope steepness (Gubert, 2015). A simulation with a slope-threshold of 10% for effluent spreading, as proposed by MIPAAF (2006), resulted in the exclusion of 85% of net meadow area from effluent application. This confirms the need to take the specificity of mountain areas into consideration when planning normative tools for the agro-environmental management of livestock effluents.
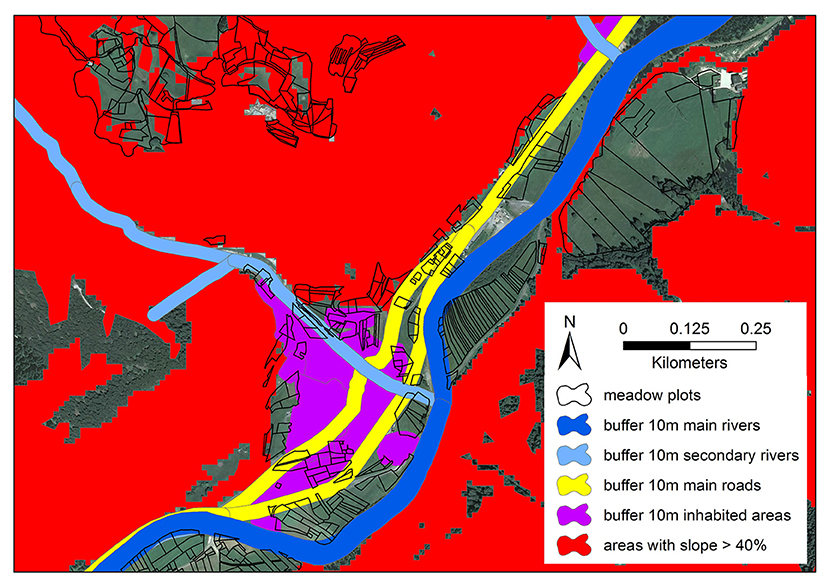
Figure 3. Cartographic detail of spatial implementation of effluent spreading restrictions in a sample area of Predazzo.
Effluent spreading restrictions maps were elaborated in detail for each farm, in cooperation with the local Agency for Environmental Protection (APPA), in order to reduce the environmental impact of effluent spreading and to increase its social acceptance. The information layers created for this purpose might be potentially integrated in an open-access web-GIS application, similar to the Wisconsin Manure Management Advisory System, which helps farmers and others who apply nutrients to identify suitable cropland areas for spreading (MMAS, 2014).
Farm Nitrogen Balance and Digestate Spreading Plans
Information about field nitrogen production, sustainable nitrogen requirements of each meadow class and net meadow surface available for spreading were used to compute a nitrogen balance at the single-farm scale. Table 4 reports an example for one of the seven farms involved, with a good balance between nitrogen produced by cattle and nitrogen required by meadows. Some farms showed a positive nitrogen balance, with more nitrogen produced than required, and some others a negative balance, with less nitrogen produced than required. In sum, the total nitrogen balance of the seven farms was well-balanced.
The peculiarity of the nitrogen balance developed in the present study is that the sustainable input from organic fertilizers was “constructed” starting from the actual surfaces available for spreading and their agro-botanical characterization, with on-site collected data about forage quantity and quality of hay meadows. This means that spatial distribution patterns of cultivated land as well as site-specific production potentials were taken into account when computing the nitrogen balance, as already suggested by Scotton et al. (2012). Transferability to other areas of the Province of Trento is ensured by data about meadow types and productivity provided by Scotton et al. (2012). For other alpine regions, transferability may be limited by the lack of comprehensive, site-specific information. However, simplification of meadow types as proposed by La Notte et al. (2014), integrated with literature data about production potentials of hay meadows in the region of interest, still allows method implementation, even if with a larger degree of approximation. Peratoner et al. (2010), for instance, have computed forage balances for South Tyrol starting from average productivity data of different types of meadows in the region.
The second important output of the study is the definition of farm-tailored digestate spreading plans, which consider farm-specific meadow type composition and spatial distribution. The dataset developed allowed to quantify, for each meadow plot managed by a farm, not only the total amount of nitrogen—and consequently of digestate—to be spread during a vegetation season, but also the sustainable digestate volume per operation according to actual vegetation requirements and runoff risk potential. Table 5 summarizes the digestate spreading recommendations elaborated for the three macro-categories of hay meadows. Given the total effluent volume to be spread per hectare and year, spreading was distributed during the vegetation period according to Buchgraber and Gindl (2004) (decreasing effluent volumes from spring to autumn), ensuring at the same time the absence of surface runoff (spreading volume lower than 41 cubic meters per hectare and operation). Proposed spreading recommendations can be finally spatialized at the plot level, to deliver seasonal spreading plans for each farm.
Conclusions
The methodological approach proposed in the present study allows to tackle the issue of animal effluent spreading in mountain areas, with a specific focus on the environmental and agronomic sustainability of digestate use on alpine hay meadows. Data collected on-site were spatialized and integrated with existing geographic information layers, in order to develop new management and planning tools which are transferable to livestock farmers and help them adjust effluent spreading patterns according to the actual nutrient requirements of cut meadows as well as to the potential risk of surface runoff.
The case-study of Predazzo (Trentino, Eastern Italian Alps) allowed to test a new methodological approach, delivering usable results for the agronomic utilization of digestate in seven dairy farms in an alpine context. The main outcomes are a) farm-scale nitrogen balances, calibrated on size and agro-botanical type of hay meadows managed by the farm, b) farm-tailored digestate spreading plans, providing sustainable spatio-temporal patterns of organic fertilization on agricultural land. The methodological procedure as well as findings about nutrient balancing of alpine meadows and effluent-related surface runoff are transferrable to other mountain regions based on livestock farming and grassland management, and are also applicable for farms without anaerobic digestion to optimize effluent and nutrient management on hay meadows in general.
To date, the present study represents the first implementation of GIS tools for the management of livestock effluents in Trentino's mountain areas. Besides methodological aspects, one of the most important innovation elements is the spatial scale, which enables to deliver agro-botanical and management information about hay meadows at the single-plot level. Further developments regard the implementation at a larger geographic scale (i.e., district or region) and the integration of computed geo-referenced data in existing regional cartographic portals and web-GIS applications. Validation as well as monitoring of results will occur in the next 3 years during practical implementation, in order to verify the quality and effectiveness of the proposed method.
Ethics Statement
The authors declare that: no ethics approval was required for the survey as per institutional and national guidelines; they obtained a letter of engagement from the cooperative of farmers, signed by the president of the cooperative after having defined and agreed with them the content of the activity; oral informed consent was obtained from all research participants. They also consented by virtue of survey completion.
Author Contributions
SS conceived and supervised the present study in cooperation with the Cooperative Biodigester Predazzo, involving AP, FG, and LG in the development and implementation phases. SS and LG conducted on-farm surveys and related data elaboration, effluent sampling and laboratory analysis, biodigester design and dimensioning. FG developed the GIS-framework and performed related computations and AP helped supervise the project. FG took the lead in writing the manuscript. All authors provided critical feedback and helped shape the research, analysis and manuscript.
Conflict of Interest Statement
The authors declare that the research was conducted in the absence of any commercial or financial relationships that could be construed as a potential conflict of interest.
Acknowledgments
The authors are particularly grateful to the Cooperative Biodigester Predazzo, specifically to Dr. Alberto Bucci and Franco Morandini, for the fundamental contribution to the outcome of the present study.
Footnotes
1. ^National and local legislation define spatial spreading limitations also for solid livestock effluents. These limitations were not considered in this study because (a) digestate is spread as liquid effluent, (b) in the case of solid/liquid separation of digestate, the solid fraction is subject to less strict limitations than the liquid fraction.
2. ^According to the Ministerial Decree April 7, one adult cattle unit corresponds to 600 kg live weight, producing 83 kg field nitrogen per year.
References
APPAG, (2015). Provincial Agency for Payments in Agriculture of the Autonomous Province of Trento. Available online at: http://www.appag.provincia.tn.it/ (Accessed December 10, 2017).
Asel, A. (2015). Auswirkungen unterschiedlicher Klima- und Standortbedingungen auf Ertrag, Futterqualität sowie Nährstoffbilanzen von Dauergrünland in Österreich. Degree thesis, University of Natural Resources and Life Sciences, Vienna.
Baltas, E. A., Dervos, N. A., and Mimikou, M. A. (2007). Determination of the SCS initial abtraction ratio in an experimental watershed in Greece. Hydrol. Earth Syst. Sci. 11, 1825–1829. doi: 10.5194/hess-11-1825-2007
Battaglini, L., Bovolenta, S., Gusmeroli, F., Salvador, S., and Sturaro, E. (2014). Environmental sustainability of alpine livestock farms. Ital. J. Anim. Sci. 13, 431–443. doi: 10.4081/ijas.2014.3155
Bätzing, W. (1992). Naturräumliche, agrargeschichtliche, demographische und soziokulturelle Unterschiede zwischen Nord und Südalpen. CIPRA Schriften 9, 115–129.
Bätzing, W. (2005). Le Alpi: Una Regione Unica al Centro dell'Europa. Torino: Bollati Boringhieri Editore.
Bittante, G. (2011). Cowability: Adaptability of Dairy Cattle Reared in Alpine Territory. Final Research Project Report, Padova: University of Padova.
Blome-Drees, J., Bøggild, N., Degens, P., Michels, J., Schimmele, C., and Werner, J. (2016). “Potenziale und Hemmnisse von unternehmeriscen Aktivitäten in der Rechtsform der Genossenschaft,” in: Neue Kölner Genossenschaftswissenschaft, eds F. Schulz-Nieswandt, J. Blome-Drees, and I. Schmale (Berlin: LIT Verlag), Band 12.
Buchgraber, K., and Gindl, G. (2004). Zeitgemässe Grünlandbewirtschaftung. Graz: Leopold Stocker Verlag.
Cernusca, A., Tappeiner, U., Bahn, M., Bayfield, N., Chemini, C., Filat, F., et al. (1996). ECOMONT: ecological effects of land use changes on European terrestrial mountain ecosystems. Pirineos 147–148, 145–172. doi: 10.3989/pirineos.1996.v147-148.141
Copeland, C. (2007). “Air quality issues and animal agriculture: a primer,” in: Animal Agriculture Research Progress, ed K. B. Tolenhoff (New York, NY: Nova Science Publishers), 1–26.
Dietl, W., and Jourquera, M. (2004). Wiesen- und Alpenpflanzen. Leopoldsdorf: Österreichischer Agrarverlag.
Dietl, W. (2006). Ökologischer Wiesenbau: Nachhaltige Bewirtschaftung von Wiesen und Weiden. Leopoldsdorf: Österreichischer Agrarverlag.
Francesia, C., Madormo, F., Tarello, C., and Vernetti-Prot, L. (2008). Sostenibilità del Sistema Zootecnico Valdostano: Aspetti Economici, Sociali ed Ambientali. Aosta: Institut Agricole Regional.
Gubert, F. (2008). Structural Change in Agriculture and Landscape Change: Three Case-Study Communities in Trentino's Mountain Areas. Master thesis, University of Applied Sciences, Vienna
Gubert, F. (2015). Zonazione delle aree prative del Trentino: sintesi metodologica e caratterizzazione geo-statistica. Rapporto Centro di Trasferimento Tecnologico Fondazione Edmund Mach (San Michele all'Adige: Fondazione Edmund Mach), 100–102.
Hawkins, R. H., Jiang, R., Woodward, D. E., Hjelmfelt, A. T., and Van Mullem, J. A. (2002). “Runoff curve number method: examination of the initial abstraction ratio,” in Proceedings of the Second Federal Interagency Hydrologic Modeling Conference, Las Vegas, NV.
Hjorth, M., Christensens, K. V., Christensens, M. L., and Sommer, S. G. (2010). Solid-liquid separation of animal slurry in theory and practice: a review. Agron. Sustain. Dev. 30, 153–180. doi: 10.1051/agro/2009010
Hjorth, M., Nielsen, A. M., Nyord, Y., Hansen, M. N., Nisse, P., and Sommer, S. G. (2009). Nutrient value, odour emission and energy production of manure as influenced by anaerobic digestion and separation. Agron. Sustain. Dev. 29, 329–338. doi: 10.1051/agro:2008047
Holm-Nielsen, J. B., Al Seadi, T., and Oleskowicz-Popiel, P. (2009). The future of anaerobic digestion and biogas utilization. Bioresour. Technol. 100, 5478–5484. doi: 10.1016/j.biortech.2008.12.046
Immovilli, A., Valli, L., and Fabbri, C. (2008). La digestione anaerobica riduce gli odori dei liquami. L'Informatore Agrario 43, 66–69. Available online at: http://www.crpa.it/media/documents/crpa_www/Settori/Ambiente/Download/Archivio-2008/43066.pdf
Jiang, R. (2001). Investigation of Runoff Curve Number Initial Abstraction Ratio. MS Thesis, Watershed Management, University of Arizona, 120 pp.
Kasper, M., Freyer, B., Hülsbergen, K. J., Schmid, H., and Friedel, J. K. (2015). Humus balances of different farm production systems in main production areas in Austria. J. Plant Nut. Soil Sci. 178, 25–34. doi: 10.1002/jpln.201400111
La Notte, A., Scolozzi, R., Molfetta, P., Gubert, F., Molignoni, R., Franchi, R., et al. (2014). An ecosystem service-based approach to design agro-environment-climate payments for the rural development programme 2014-2020: the case of the Autonomous Province of Trento. Ann. Bot. 4, 91–96. doi: 10.4462/annbotrm-11646
Landesanstalt für Landwirtschaft LfL (2006). Biogastechnologie zur Umweltverträglichen Flüssigmistverwertung und Energiegewinnung in Wasserschutzgebieten. Freising: Institut für Landtechnik, Bauwesen und Umwelttechnik
Lohr, L. (1996). Perception of rural air quality: what will the neighbours think? J. Agribus. 14, 109–128.
Miner, J. R., Humenik, F. J., and Overcash, M. R. (2000). Managing Livestock Wastes to Preserve Environmental Quality. Ames, IA: Iowa State University Press.
MIPAAF (2006). Ministerial Decree April 7, 2006 “Criteri e Norme Tecniche Generali per la Disciplina Regionale dell'Utilizzazione Agronomica Degli Effluenti di Allevamento, di cui all'articolo 38 del D. Lgs. 11 Maggio 1999, n. 152” Official Gazette of the Italian Republic, Mai 12, 2006, n. 109. Rome: Italian Republic.
MIPAAF (2016). Ministerial Decree February 25, 2016 n. 5046 “Criteri e Norme Tecniche Generali per la Disciplina Regionale dell'utilizzazione Agronomica Degli Effluenti di Allevamento e delle Acque Reflue di cui all'art. 113 del Decreto legislativo 3 aprile 2006 n. 152, Nonché per la Produzione e l'Utilizzazione Agronomica del Digestato.” Rome: Italian Republic.
Mishra, S. K., and Singh, V. P. (2004). Long-term hydrological simulation based on the soil conservation service curve number. J. Hydrol. Process. 18, 1291–1313. doi: 10.1002/hyp.1344
MMAS (2014) Manure Management Advisory System. Department of Agriculture, Trade and Consumer Protection, Winsconsin, Available online at: http://www.manureadvisorysystem.wi.gov/app/ (Accessed December 09, 2017).
PAT (2015). Geo-Cartographic Portal and Catalogue of the Autonomous Province of Trento. Available online at: http://www.territorio.provincia.tn.it/portal/server.pt/community/sgc_-_geocatalogo/862/sgc_-_geocatalogo/32157 (Accessed December 08, 2017).
Penati, C., Sandrucci, A., Tamburini, A., Bava, L., and Timini, M. (2008). Bilanci aziendali dell'azoto e del fosforo di un campione di allevamenti bovini della bassa Valtellina e Valchiavenna. Quaderni SoZooAlp 5, 226–236. Available online at: http://www.sozooalp.it/Quaderno-5.70.0.html
Penati, C., Tamburini, A., Bava, L., Zucali, M., and Sandrucci, A. (2013). Environmental impact of cow milk production in the Italian Alps using life cycle assessment. Ital. J. Anim. Sci. 12, 584–592. doi: 10.4081/ijas.2013.e96
Peratoner, G., Kasal, A., and Plitzner, C. (2010). Stima del bilancio foraggero per l'Alto Adige. Quaderni SoZooAlp 6, 111–122. Available online at: http://www.sozooalp.it/Quaderno-6.71.0.html
Powers, W. J., Van Horn, H. H., Wilkie, A. C., Wilcox, C. J., and Nordstedt, R. A. (1999). Effects of anaerobic digestion and additives to effluent or cattle feed on odor and odorant concentrations. J. Anim. Sci. 77, 1412–1421. doi: 10.2527/1999.7761412x
Regione Autonoma Valle d'Aosta RAVA (2017). Programma di Sviluppo Rurale della Regione Valle d'Aosta 2014–2020. Available online at: http://www.regione.vda.it/agricoltura/PSR_2014_2020/default_i.aspx (Accessed July 27, 2018).
Rihm, B., and Achermann, B. (2016). “Critical loads of nitrogen and their exceedances,” in Swiss Contribution to the Effects-Oriented Work Under the Convention on Long-Range Transboundary Air Pollution (UNECE), ed Federal Office for the Environment, Bern: Environmental Studies, Available online at: https://www.bafu.admin.ch/bafu/en/home/topics/air/publications-studies/publications/Critical-Loads-of-Nitrogen-and-their-Exceedances.html (Accessed August 02, 2018). no. 1642, 78 pages.
Riva, C., Orzi, V., Carozzi, M., Acutis, M., Boccasile, G., Lonati, S., et al. (2016). Short-term experiments in using digestate products as substitutes for mineral (N) fertilizer: agronomic performance, odours, and ammonia emission impacts. Sci.Total Environ. 547, 206–214. doi: 10.1016/j.scitotenv.2015.12.156
Rubino, R. (2006). Livestock Farming Systems: Product Quality Based on Local Resources Leading to Improved Sustainability. Wageningen: Wageningen Academic Publishers. doi: 10.3920/978-90-8686-565-9
Schauberger, G., Piringer, M., and Petz, E. (2001). Separation distance to avoid odour nuisance due to livestock calculated by the Austrian odour dispersion model (AODM). Agri. Ecosyst. Environ. 87, 13–28. doi: 10.1016/S0167-8809(00)00299-1
Scotton, M., Pecile, A., and Franchi, R. (2012). I tipi di prato Permanente in Trentino: Tipologia Agro-Ecologica della Praticoltura con Finalità Zootecniche, Paesaggistiche e Ambientali. San Michele all'Adige: Fondazione Edmund Mach.
Scotton, M., Sicher, L., and Kasal, A. (2014). Semi-natural grasslands of the Non valley (Eastern Italian Alps): agronomic and environmental value of traditional and new Alpine hay-meadow types. Agri. Ecosyst. Environ. 197, 243–254. doi: 10.1016/j.agee.2014.08.003
Stanley, T. J. L. (2014). Cattle Slurry on Grassland: Application Methods and Nitrogen unse Efficiency. Master's Thesis, Wageningen Univeristy, Wageningen
Steinwidder, A. (2009). Einfluss der Proteinversorgung auf Futteraufnahme, Milchleistung, Pansen- und Blutparameter sowie N-Ausscheidung von Milchkühen.– Lehr- und Forschungszentrum für Landwirtschaft, LFZ Raumberg-Gumpenstein. Stuttgart: Verlag Eugen Ulmer, 2009. Züchtungskunde 81, 106–124.
Streifeneder, T. F. (2009). Die Agrarstrukturen in den Alpen und ihre Entwicklung unter Berücksichtigung ihrer Bestimmungsgründe. Dissertation, Ludwig-Maximilian-Universität, München.
Tappeiner, U., Bordsorf, A., and Tasser, E. (2008). Mapping the Alps: Society, Economy, Environment. Heidelberg: Spektrum Verlag.
Tasser, E., Aigner, S., Egger, G., and Tappeiner, U. (2013). ArgeAlp: Atlante delle Malghe. Bolzano: Athesia Editore.
Theiss, H. (1989). Zur Dynamik der Nitrat- und Wasservorräte verschiedener Bodenschichten in Abhängigkeit von Hauptbestandesbildner, Narbendichte und N-Düngung. Dissertation, Universität Gießen, Gießen.
T'Mannetje, L., and Jarvins, S. (1990). “Nitrogen flows and losses in grasslands,” in Proceedings 13th EGF-General Meeting, Vol. I, Banska-Bystrica, 114–131.
Webb, J., Sørensen, P., Velthof, G., Amon, B., Pinto, M., Rodhe, L., et al. (2010). Study on Variation of Manure N Efficiency Throughout Europe. Didcot: AEA Technology Plc.
Keywords: livestock effluents, alpine environment, organic fertilization, cut meadows, digestate, anaerobic digestion
Citation: Gubert F, Silvestri S, Pecile A and Grandi L (2018) A GIS-Based Approach for the Sustainable Management of Livestock Effluents on Alpine Meadows. Front. Sustain. Food Syst. 2:55. doi: 10.3389/fsufs.2018.00055
Received: 29 January 2018; Accepted: 14 August 2018;
Published: 03 September 2018.
Edited by:
Tom Misselbrook, Rothamsted Research (BBSRC), United KingdomReviewed by:
Laura Zavattaro, Università degli Studi di Torino, ItalyHarald Menzi, Federal Office for the Environment, Switzerland
Copyright © 2018 Gubert, Silvestri, Pecile and Grandi. This is an open-access article distributed under the terms of the Creative Commons Attribution License (CC BY). The use, distribution or reproduction in other forums is permitted, provided the original author(s) and the copyright owner(s) are credited and that the original publication in this journal is cited, in accordance with accepted academic practice. No use, distribution or reproduction is permitted which does not comply with these terms.
*Correspondence: Silvia Silvestri, silvia.silvestri@fmach.it