- 1Department of Soil Science, Federal University of Ceara, Fortaleza, Brazil
- 2Research Group Applied to Agrochemicals and Environment, Orihuela Polytechnic School, Miguel Hernandez University, Orihuela, Spain
- 3Coastal Plains Soil, Water and Plant Research Center (USDA-ARS), Florence, SC, United States
- 4Department of Biology Molecular and Biochemical Engineering, Pablo de Olavide University, Sevilla, Spain
Anaerobic digestion of agro-industrial and livestock waste generates considerable digestate volumes that are important sources of nitrogen (N). However, on some occasions, the high concentrations of N present in the digestates may represent an obstacle to its use locally as fertilizer, since it can cause an environmental impact (European Community, 2000). This study analyzes the efficiency of gas-permeable membranes (GPM) in the recovery of the ammoniacal nitrogen (NH4+) present in the swine manure (SM, Control) and three digestates generated from the anaerobic co-digestion of mixtures of SM, fruit and vegetable sludge (FVS) from the vegetable industry (peppers and artichokes), and by-products of the tomato processing industry (TW) (skins and seeds), which were mixed at three different proportions [TW + FVS]: [SM]. Their NHx+ content was 2,240 mg L−1 for the SM and 4,670–5,370 for the digestate mixtures. Throughout the duration of the Necovery experiment (96 h), the percentages of NH4+ removal and recovery achieved were consistent among treatments, approximatelly 78 and 96%, respectively. The recovery of NH4+ for the digestate mixtures seems to be dependent on the treatment time, since the potential of N recovery was higher when the treatment time increased. However, for the control experiment (SM), with lower N concentration, the maximum N recovery was obtained at the experimental time established, without increasing the treatment time.
Introduction
Livestock production is a sector that contributes significantly to global greenhouse gas (GHG) emissions, with annual values estimated at 7.1 Gton of the CO2 equivalent, accounting for 14.5% of all anthropogenic emissions (Food and Agriculture Organization of the United Nations (FAO), 2013). In the specific case of pig production, this activity has the potential to emit about 4.87 kg of CO2 equivalent per kg of carcass produced (Philippe and Nicks, 2015). The accumulation of swine manures (SM) is responsible for the emission of various gases into the atmosphere. Among them, ammonia (NH3) stands out for its high release (86.8 μg NH3 s−1 m−2) and high environmental impact potential (Dai et al., 2015).
Anaerobic co-digestion (ACoD) has been used as a technology to minimize the negative effects of the accumulation of SM and other organic residues on rural areas, generating biogas (renewable energy) and digestate, which can be used as agricultural fertilizer (Mata-Alvarez et al., 2014; Akhiar et al., 2017). The AcoD has been used as a way to improve the efficiency of biogas production systems that use a single manure substrate. Molinuevo-Salces et al. (2013) indicated that AcoD of SM with vegetable processing residues increases methane production by 219%, while De Vries et al. (2012) the AcoD of SM with fruits and vegetable wastes (FVW) (skin, seeds and slurry from fruit washing) can increase bioenergy production by 568%. Furthermore, Muscolo et al. (2017) confirmed that the agronomic properties improved (organic matter, nutrient balance) in the soils where digestates were applied coming from the AcoD of the mixture of SM, olive waste and citrus pulp compared with chemical fertilizers.
However, high concentrations of nitrogen (N) in the AcoD effluents (digestate) can complicate its management and cause negative impacts to the environment due to its application in local agricultural areas (European Community, 2000). Some technologies have been used to reduce the excess N content in livestock wastewater, promoting its recovery in the mineral form () and allowing its reuse as agricultural fertilizer in areas far from the livestock concentration with greater needs for the N nutrient. Dube et al. (2016) and Garcia-Gonzalez et al. (2016) used a new process utilizing gas-permeable membranes (GPM) in low pressure systems to capture the N present in the digestate from the anaerobic mono-digestion of SM and store it in a concentrated form in an acid solution of 0.5 M H2SO4.
The new process using GPM consists of submerging the gas membranes in the organic liquid residue causing the NH3(g) to penetrate into its pores and is captured by an acidic solution circulating in the membrane interior. The combination of the NH3(g) with H+ ions from H2SO4 inside the membrane generates a concentrated solution with potential to be used as a nitrogen fertilizer (Figure 1; Garcia-Gonzalez and Vanotti, 2015; García-González et al., 2015; Vanotti and Szogi, 2015).
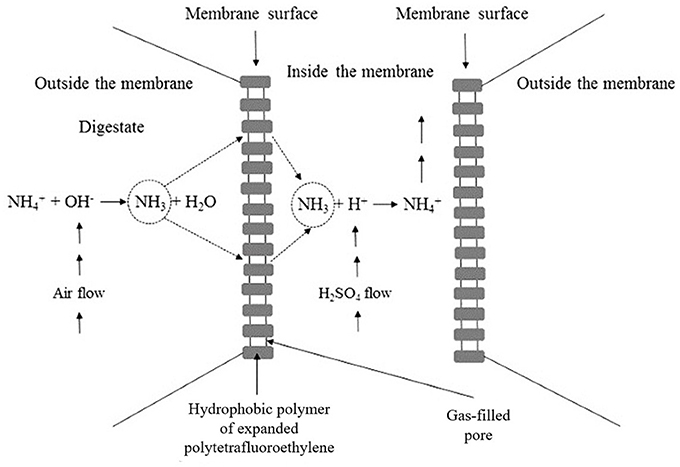
Figure 1. Illustration of the cross section of the hydrophobic membrane with the principal chemical reactions that occurred during the operation of the system.
To carry out this process, it is necessary to increase the pH of the wastewater to be treated to favor the transformation of into NH3(g) (Equation 1) and enable its passage through the gas-permeable hydrophobic membrane where an acidic solution circulates (Figure 1). This solution dissolves the ammonia in the form of ammonium, which is the predominant species at pH values below 7.
Previous studies have shown that the efficiency of the removal and recovery of depends on the design and type of system used. Thus, different authors have reported better results using hydrophobic GPM submerged in the effluent, since this sytem can conduct the gaseous species toward the acidic stripping solution placed on the other side of the membrane (Lauterböck et al., 2013; Boehler et al., 2015; Vanotti and Szogi, 2015).
In addition, the dynamics of the chemical and biochemical reactions that may occur in the wastewater environment have an impact on the efficiency of the process. The recovery of is reduced when there is microbial activity capable of (1) oxidizing the ammonium to nitrites (Equation 2) and nitrates (Equation 3), and (2) reducing the carbonate alkalinity (Equation 2). Moreover, the ammonium recovery through the gas-permeable membrane (GPM) is affected by the pH of the environment, and the environments could be affected by the presence and concentration of the different forms of inorganic carbon (CO2/HCO3−/); among which, the bicarbonate ion is the dominant species in livesock wastewater and the transformations of both the CO2 (Equation 4) and the (Equation 5) are shifted to the right.
Additionally, a reaction (Equation 6) between the present and the H+ of the balance of Equation (4) may occur, and also a reaction (Equation 7) between the CO2 present and the OH− of the balance (Equation 5).
Taking into account all of the above, the presence of carbonates favors the basic environment (according to Equation 5); whilst nitrification processes reduce the concentration of ammonium and the pH (according to Equations 2–4, Magrí et al., 2012).
García-González et al. (2015) and Vanotti and Szogi (2015) have proposed the use of nitrification inhibitors and the application of low-rate aeration to increase the pH without the need of adding alkaline chemical agents, in this way reducing the economic and environmental costs involved in the treatments of effluents rich in ammonium. During low-rate aeration, the CO2 that is in equilibrium in the solution is stripped (Equation 7) and the reaction (Equation 8) is shifted to the formation of OH−, increasing the pH and reducing the bicarbonate in the environment. All these factors cause an increase in the formation of gaseous NH3(g) (Equation 1), which accelerates its uptake by the membrane (Figure 1).
According to Equation (9), the recovery of NH3(g) through the membrane causes an increase of acidity in the environment being treated and a decrease of the bicarbonate (Equations 1, 8) and, therefore, it is necessary that the operating conditions allow to continually raise the pH levels in order to maintain recovery efficiency (Garcia-Gonzalez and Vanotti, 2015).
The aeration effect is double, on the one hand, it promotes the increase in pH values and, on the other hand, promotes the increase in the concentration of the free ammonium which favors the passage of NH3(g) through the membrane (Vanotti et al., 2017). In the studies conducted by Dube et al. (2016), the GPM system with low-rate aeration promoted removals of 97–99% of the initial contents in the digestate in 5 days of operation, obtaining an accumulation of 11,900 mg of -N L−1 in the acid solution. In the studies conducted by Daguerre-Martini et al. (2018), the GPM system with low-rate aeration removed 65–85% of the initial contents in swine manure in 4 days of operation, obtaining concentration of -N in the acid tank of 37,400 mg L−1.
Successful N recovery using GPM has already been performed on different organic wastes such as poultry litter (Rothrock et al., 2010, 2013), swine manure (Garcia-Gonzalez and Vanotti, 2015; García-González et al., 2015) and digestate from mono-digestion of swine manure (Dube et al., 2016; Garcia-Gonzalez et al., 2016; Vanotti et al., 2017) with recovery above 90% of the total content of this form of the nutrient in the residues. However, recovery of N in the digestate from systems operating in ACoD has not yet been performed.
In this study, a low pressure GPM system operated under low-rate aeration was evaluated to recover the ammonia in the digestates generated by an AcoD pilot system, aiming for the production of N fertilizer concentrates. SM, FVS, and tomato waste (TW) mixtures were used as co-substrates in three different mixing ratios for AcoD, which gave rise to three different digestates to evaluate N recovery. The recovery of N in raw SM was also performed and served as a control treatment.
Materials and Methods
Substrates for Anaerobic Co-digestion (AcoD)
TW mainly constituted by skins and seeds, was collected from a tomato processing industry to obtain tomato cans. FVS was obtained from three fruit and vegetable processing plants in Murcia (Spain): a canning company and two companies producing frozen artichoke and pepper. SM was collected from a receiving pit in a pig farm located in Alicante (Spain). Immediately after sampling, SM and FVS were preserved at 4°C, while TW was preserved at −20°C. The main characteristics of the feedstocks are presented in Table 1.
AcoD Process
A cylindrical anaerobic reactor with a working volume of 300 L was used. The reactor was kept under agitation (8–10 rpm) and constant temperature (35 ± 1°C) and was operated in a semi-continuous mode three times a week with the manual addition of 10 kg of substrate mixture (fresh weight), with 6% TS, through the reactor feed intake (30 kg wk−1 or 4.3 kg d−1), followed by the removal of 10 kg of digestate, through the sample opening of the reactor, to maintain a constant working weight of 245 kg (fresh weight). Our contribution is enclosed in a complex experiment (Journal of Cleaner Production, 156: 757–765, 2017) that included in a 4 month period of optimization of biogas production using these wastes. We sampled the digestate at the end of stage III to obtain a pseudo-steady-state inside the reactor (Figure 2).
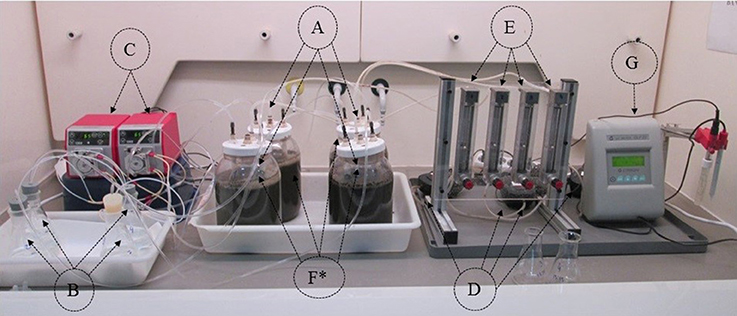
Figure 2. Components of the ammonia recovery system (4 batch units). (A) N removal vessels with the wastewater. (B) Acid solution tanks. (C) Pumps for acid recirculation. (D) Air pumps. (E) Airflow meters and valves. (F) Tubular membranes submerged in the wastewater. (G) pH meter. *Duplicate reactors.
TW and FVS were initially homogenized and then mixed in different proportions to SM. Three mixing percentage ratios [TW + FVS]:[SM] were established: 7/3; 5/5; and 4/6. Each mixture gave rise to a specific digestate as described below: Digestate 1: 7/3; Digestate 2: 5/5; and Digestate 3: 4/6. The reactor was fed with each mixture for 15 days. After this period, the digestate for each mixture was collected, chemically analyzed and immediately taken to the NH3 recovery system.
Ammonia Recovery System
The elements that constituted the NH3 recovery system follow the diagram of Dube et al. (2016) and are identified in Figure 2 (showing four identical units). Specifically, it consisted of the following parts: (A) 2 L wastewater reaction vessels with an effective volume of 1.5 L SM or digestate; (B) glass vessel (Erlenmeyer) containing 200 mL of 1N H2SO4 solution; (C) peristaltic pump (Watson-Marlow 120S) used to continuously recirculate the H2SO4 through the membrane lumen; (D) aeration pump (Sera Precision Air 110 Plus: 1.8 L min−1); (E) airflow meter; (F) tubular GPM made of expanded polytetrafluoroethylene (ePTFE) that were microporous and hydrophobic (Phillips Scientific Inc., Rock Hill, SC), and (G) pH meter for pH monitoring during the experiment. Characteristics of the membrane are provided by Dube et al. (2016).
The experiment was conducted under laboratory conditions and under controlled temperature (25 ± 1°C) with a total duration of 4 days (93 h). During this period, SM and digestate samples were taken daily for and pH determinations both at the beginning (9 a.m.) and end (8 p.m.) of the day.
The process of ammonia recovery consisted of submerging the tubular membrane in the wastewater and circulating the acidic solution through the inside using the peristaltic pumps. The hydrophobic material of the membrane prevents both the acid solution from flowing out of it and the wastewater to penetrate into the membrane lumen. The manure was aerated continuously in order to raise its pH and promote transformation into gaseous NH3. The NH3(g) formed penetrates in the membrane gas pores and is immediately captured by the H2SO4 solution circulating inside. Finally, a concentrated acid solution was formed which returned to the acid reservoirs (Figure 1).
The peristaltic pumps circulated the acid solution constantly at a rate of 5.8 L day−1 throughout the experimental period. The air flow was constantly applied at a rate of 0.24 L−1 air L−1 residue min−1. The membranes had a length of 60 cm with an outer diameter of 10.25 mm and a wall thickness of 0.75 mm. The pores open to gas passage had a mean diameter of 2.5 μm and had a bubble point of 210 kPa. At the beginning of the experiment, 10 mg L−1 of the n-allylthiourea 98% (nitrification inhibitor) was added to each vessel to avoid transformation of to NO3− according to Equations (2) and (3) (Vanotti and Szogi, 2015).
The buffer capacity of the liquid was measured according to the TAC method of McGhee (1968) by titration with 0.1N H2SO4 to pH 5.0 and expressed in terms of mg CaCO3 L−1. The pH of digestate was measured directly with a Consort C860 multiparameter analyzer. Total Solids (TS), Volatile Solids (VS) and ammonium were determined at the beginning and end of the experiment according to American Public Health Association/American (1998) 2540B-2540E-4500B and C methods, respectively.
Results and Discussion
The chemical characterizations of SM and digestates before and after treatment are presented in Table 2. For both the SM and the digestates, an increase in pH and a reduction of values of TS, VS, and buffer capacity were observed. The increase of pH values and the reduction of carbonate buffer capacity and concentration were expected and indicated a correct functioning of the system.
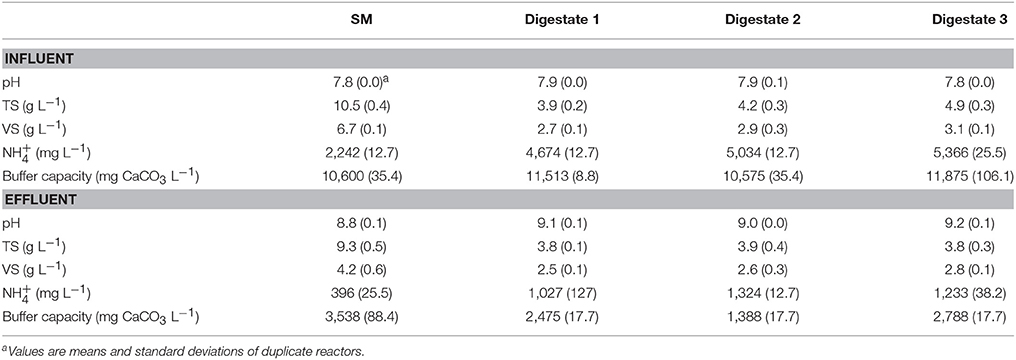
Table 2. Chemical characterization of the swine manure (SM) and the digestates at the start and end of the N recovery experiment.
In the specific case of the reduction of the buffer capacity TAC in the residues, this characteristic is the result of the application of the aeration that consumes HCO3− in order to achieve the generation of OH− and subsequent increase of the pH of the residues according to Equations (7) and (8). As a consequence of the increase in pH values, an active transformation to NH3(g) occurs in the residue according to Equation (9). The NH3(g) formed can then be recovered by the membrane submerged in the residue. A lower loss of the NH3(g) to the environment contributes to a higher NH3(g) recovery efficiency of the system.
In our case, the recovery efficiencies were high (about 96%) in the four treatments and the unaccounted N fraction was generally low (around 4%). This means that almost all the that was removed from the manure and digestates with the GPM has been recovered in the acidic solution (Table 3). The efficiency of this system is affected by the concentration of and the carbonate alkalinity in the wastewater. Thus, raising such parameters means a higher removal and efficiency in the recovery of (Garcia-Gonzalez et al., 2009; Garcia-Gonzalez and Vanotti, 2015; Daguerre-Martini et al., 2018), which is consistent with the results obtained in this study.
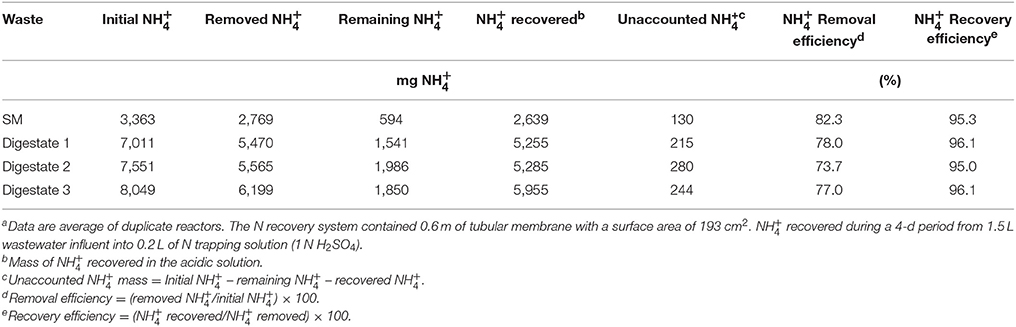
Table 3. Mass balance for the recovery from swine manure (SM) and co-digested effluents using gas-permeable membranesa.
Effect of Aeration on pH Value
The dynamics of the pH values of SM and of the different digestates during the experimental period are presented in Figure 3. All the residues presented an increase of the pH with time. The initial pH of the SM was 7.83 and after 51 h of aeration reached the maximum pH value (8.93), increasing by 1.10 pH units. After this period and with continued aeration up to the end of the experiment at 93 h, the SM pH at the end of the experiment was 8.8. García-González et al. (2015) and Dube et al. (2016) reported similar results in experiments of recovery of ammonia from swine manure and swine manure derived anaerobic digestate, respectively.
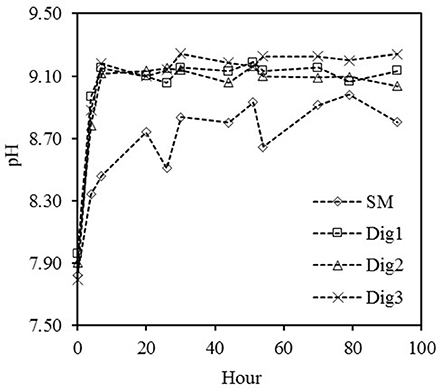
Figure 3. Increases in the pH of the swine manure (SM) and in the three co-digested effluents (Dig 1, 2, and 3) as a result of the low-rate aeration during the batch experiment.
In the three digestates, the pH increase during the treatment was similar. The digestates presented initial pH values of 7.8–7.9 (Table 2). In digestate 1 and 2, the maximum pH value (9.19 and 9.17) was observed after 51 h of treatment and in digestate 3, the maximum pH value (9.25) was observed at 30 h of treatment (Figure 3). Aeration provided an increase of 1.23, 1.26, and 1.45 pH units for digestates 1, 2, and 3 respectively. After 93 h of treatment with active N removal, the aeration of the manure still maintained a high pH (9.14, 9.04, and 9.24, respectively). Therefore, the modified wastewater environment promoted NH3 formation.
In our case, as the experiment evolves, the increase in pH is smoothed in all four tests, as a result of acidification by the NH3 crossing the membrane and being removed from the wastewater environment; the balance moves toward the formation of H+ (Dube et al., 2016). The consumption of TAC varied between the SM and the digestate treatments due to the differences in amounts of N removed from the wastewater by the GPM system. In the case of SM, 7062 mg CaCO3 L−1 was consumed, while 9038, 9187, and 9087 mg CaCO3 L−1 were consumed in digestates 1, 2, and 3, respectively.
The use of aeration in the waste with the objective of raising the pH about 1 unit was proposed by Vanotti and Szogi (2015) to accelerate membrane uptake of the N without chemicals. In experiment with swine manure wastewater, García-González et al. (2015) determined that the increase of pH with the aeration approach is equivalent to the addition of 2.14 g NaOH L−1 to the wastewater without aeration.
Removal of N From Digestates and Recovery in the Acidic Solution
Data in Figure 4 shows the decrease of in SM and the digestates during the experiment and the corresponding concentrations of being accumulated in the acid solution over time. The initial concentration of in SM was 2242 mg L−1 and after 4 days of treatment, it decreased to 396 mg L−1. This represents a removal efficiency of of 82.3% (Table 3). The digestates 1, 2, and 3 initially contained 4674, 5034, and 5366 mg L−1 and after 4 days of treatment, it decreased to 1027, 1324, and 1233 mg L−1 respectively, with corresponding removal efficiencies of N of 78, 73.7, and 77%.
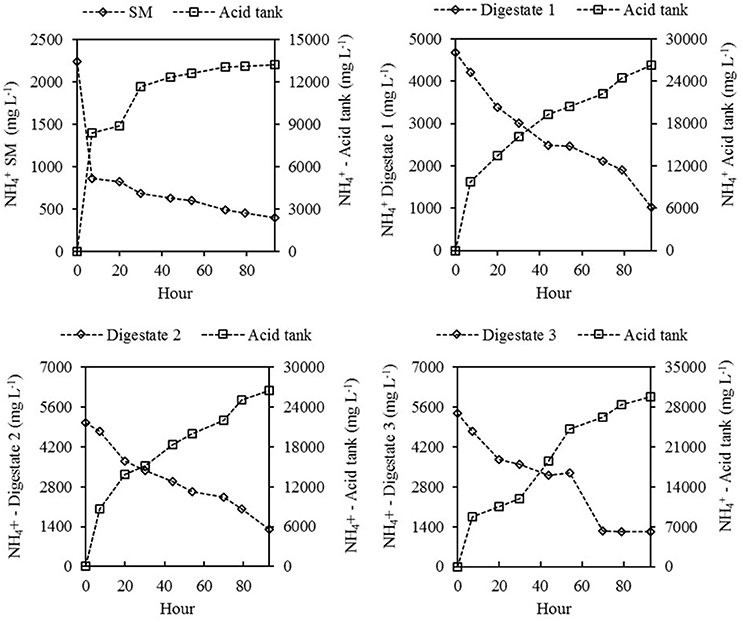
Figure 4. Decrease of concentration in the swine manure (SM) and the three digestates (Left axis) and corresponding increase of concentration in the acidic solutions (Right axis) during the N recovery experiment.
It is probable that greater N removals would have been possible with a longer treatment time, since substantial amounts of CaCO3 (buffer capacity) still remained in the four effluents after 4 days of treatment (3,538, 2,475, 1,388, and 2,788 mg CaCO3 L−1 in SM, and digestates 1–3, Table 2), with the potential to be transformed into OH− and maintain high pH values, according to equation 8.
In the case of recovery in the SM acid tank, a maximum accumulation of 13,195 mg L−1 was observed (Figure 5). This accumulation represented a recovery efficiency of 95.3% (Table 3). This means that nearly all of the that was removed from the liquid (2,769 mg) passed through the membrane and was captured by the acidic solution, forming the mineral nitrogen fertilizer. Only a small amount of the (130 mg) was unaccounted and probably lost in the air. In the case of recovery in the digestates acid tanks, higher concentration recoveries were obtained (26,275, 26,425, and 29,775 mg L−1, Figure 5), representing recovery efficiencies >95% (Table 3). In quantitative terms, with 4 days of treatment using GPM technology and low-rate aeration, it was possible to form 5,255, 5,285, and 5,955 mg of as fertilizer salt from co-digested effluents 1, 2, and 3 with concentrations of about 2.6–3% . The lower recovery observed in SM was due to the lower initial content. Compared with the SM, the digestates were almost double the initial content and doubled the recovery of in the acidic solution. The ammonia fluxes (N recovery per unit of membrane area) were: 2.66 mg N per cm2 of membrane per day for SM (initial = 2,242 mg L−1), and 5.29, 5.32, and 6.00 mg N cm−2 d−1 for digestates 1, 2, and 3 (initial = 4,674, 5,034, and 5,366 mg L−1). With even higher effluent concentations (initial = 6,350 mg L−1), Daguerre-Martini et al. (2018) obtained higher ammonia fluxes (8.9 mg N cm−2 d−1). Therefore, the transport efficiency of the GPM is positively affected by the initial concentration of in the effluent.
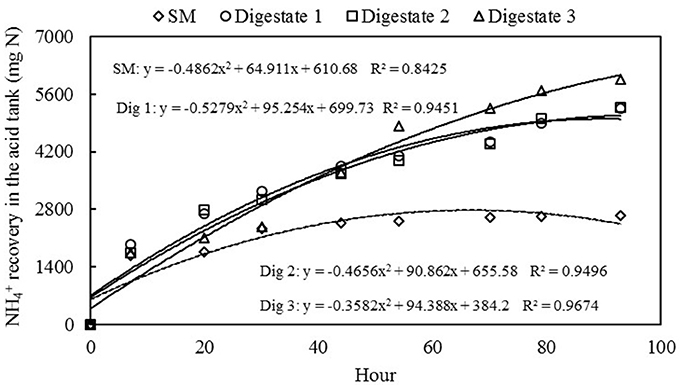
Figure 5. Evolution of mass recovered in the acidic solution obtained from the swine manure (SM) and three digestates during the experimental period.
The control of the pH of the acid solution used in NH3(g) recovery must be rigorous, maintained at between 1 and 2 units, so as to guarantee the total recovery of NH3(g) with an acid trapping system (Lahav et al., 2008). As the acidic solution recovers NH3(g), its pH increases substantially, reducing its ability to form . The recommendation is to add concentrated acid to the acid tank to an end-point pH of 1 with a pH controller when pH in the acidic solution reaches 2.
The recovery of by the acid solution of the four treatments followed a second order curve (Figure 5). In the case of SM, recovery showed a higher recovery of N in the first hours of the experiment and decreasing rates afterwards. In the case of the digestate mixtures, the recovery kinetics indicated that the potential of the N recovery process was higher with an increase in treatment time, whereas in the case of the control experiment (SM) with lower N concentration, the maximum N recovery was observed within the experimental time imposed.
In the recovery system used in this study, all effluents presented consistent values of removal (average 78%) and recovery (average 96%). These results indicate that it is possible to use GPM with digestates from biogas plants operating in the ACoD system for production of nitrogen fertilizer solutions. This combination of AcoD and GPM will generate renewable energy and nitrogen fertilizer () from the same waste feedstock. Furthermore, the reduction of the N content in the digestate may facilitate its use for other subsequent treatments such as the chemical precipitation of phosphate minerals in the form of newberyite (MgHPO4), which can also be used as agricultural fertilizer (Vanotti et al., 2017).
Conclusions
The GPM system with low-rate aeration was efficient to recover the present in the digestates from biogas plants operating in anaerobic co-digestion systems. The physico-chemical characteristics of the digestates (high concentrations of and buffer capacity and low percentage of total solids) created a favorable environment for the recovery of using the technology. The percentage of removal was consistent among co-digested effluents, about 78%. Also the percentage of recovery, about 96%. The ammonia fluxes (N recovery per unit of membrane area) were 5.3–6.0 mg N cm−2 d−1 for digestate effluents containing 4,700–5,400 mg L−1. In quantitative terms, with 4 days of treatment using GPM technology and low-rate aeration, it was possible to form liquid fertilizer salt solution from the co-digested effluents with concentrations of about 26,000–30,000 mg L−1. This means that, with the operating conditions imposed, the joint treatment of the waste using AcoD and N recovery doesn't affect, adversely, the efficacy of this type of technology in terms of depuration and ammoniacal nitrogen recovery in the environment.
Author Contributions
JO developed the experiments of ammonia capture and monitored all the batches and replications. Also contributed to data collection and data results summary. SD-M produced the experimental set-up, including all mechanical and engineering tests for fluxes, pumps, membranes. Also maintained the set-up and analyzed gaseous measurements. MV supervised the set-up and the general design of batch experiments, collaborated in discussion and conclusions. JS-T developed the chemical analyses and the monitoring parameters during the experiments. AR collaborated at engineering scale, mass fluxes calculations and analysis of raw data. MP-M analyzed the results at statistical and reproducibility scales. MB collaborated in discussion of the results and documentation. RM coordinated all the research, collaborated in discussion and conclusions.
Conflict of Interest Statement
The authors declare that the research was conducted in the absence of any commercial or financial relationships that could be construed as a potential conflict of interest.
Acknowledgments
Cooperation of University Miguel Hernandez with USDA-ARS Project 6082-13630-001-00D Improvement of Soil Management Practices and Manure Treatment/Handling Systems of the Southern Coastal Plains is acknowledged. Mention of trade names or commercial products in this article is solely for the purpose of providing specific information and does not imply recommendation or endorsement by the USDA.
References
Akhiar, A., Battimelli, A., Torrijos, M., and Carrere, H. (2017). Comprehensive characterization of the liquid fraction of digestates from full-scale anaerobic co-digestion. Waste Manage. 59, 118–128. doi: 10.1016/j.wasman.2016.11.005
American Public Health Association/American (1998). Standard Methods for the Examination of Water and Wastewater, 19th edn. Washington, DC: American Public Health Association/American Water Works Association/Water Environment Federation (APHA).
Boehler, M. A., Heisele, A., Siegrist, A., and Grömping, M. (2015). (NH4)2SO4 recovery from liquid side streams. Environ. Sci. Pollut. Res. Int. 22, 7295–7305. doi: 10.1007/s11356-014-3392-8
Daguerre-Martini, S., Vanotti, M. B., Rodriguez-Pastor, M., Rosal, A., and Moral, R. (2018). Nitrogen recovery from wastewater using gas-permeable membranes: impact of inorganic carbon content and natural organic matter. Water Res. 137, 201–210. doi: 10.1016/j.watres.2018.03.013
Dai, X. R., Saha, C. K., Ni, J. Q., Heber, A. J., Vidal, V. B., and Dunn, J. (2015). Characteristics of pollutant gas releases from swine, dairy, beef, and layer manure, and municipal wastewater. Water Res. 76, 110–119. doi: 10.1016/j.watres.2015.02.050
De Vries, J. W., Vinken, T. M. W. J., Hamelin, L., and Boer, I. J. M. (2012). Comparing environmental consequences of anaerobic mono and co-digestion of pig manure to produce bio-energy – A life cycle perspective. Bioresour. Technol. 125, 239–248. doi: 10.1016/j.biortech.2012.08.124
Dube, P. J., Vanotti, M. B., Szogi, A. A., and García-González, M. C. (2016). Enhancing recovery of ammonia from swine manure anaerobic digester effluent using gas-permeable membrane technology. Waste Manage. 49, 372–377. doi: 10.1016/j.wasman.2015.12.011
Food and Agriculture Organization of the United Nations (FAO) (2013). Tackling Climate Change Though Livestock: A Global Assessment of Emissions and Mitigation Opportunities. Rome: Food and Agriculture Organization of the United Nations.
Garcia-Gonzalez, M. C., and Vanotti, M. B. (2015). Recovery of ammonia from swine manure using gas-permeable membranes: effects of waste strength and pH. Waste Manage. 38, 455–461. doi: 10.1016/j.wasman.2015.01.021
Garcia-Gonzalez, M. C., Szogi, A. A., Vanotti, M. B., Chastain, J. P., and Millner, P. D. (2009). Enhanced solid-liquid separation of dairy manure with natural flocculants. Bioresour. Technol. 100, 5417–5423. doi: 10.1016/j.biortech.2008.11.012
García-González, M. C., Vanotti, M. B., and Szogi, A. A. (2015). Recovery of ammonia from swine manure using gas-permeable membranes: effect of aeration. J. Environ. Manage. 152, 19–26. doi: 10.1016/j.jenvman.2015.01.013
Garcia-Gonzalez, M. C., Vanotti, M. B., and Szogi, A. A. (2016). Recovery of ammonia from anaerobically digested manure using gas-permeable membranes. Sci. Agric. 73, 434–438. doi: 10.1590/0103-9016-2015-0159
Lahav, O., Mor, T., Heber, A. J., Molchanov, S., Ramirez, J. C., Li, C., et al. (2008). A new approach for minimizing ammonia emissions from poultry houses. Water Air Soil Pollut. 191, 183–197. doi: 10.1007/s11270-008-9616-0
Lauterböck, B., Moder, K., Germ, T., and Fuchs, W. (2013). Impact of characteristic membrane parameters on the transfer rate of ammonia in membrane contactor application. Sep Purif. Technol. 116, 327–334. doi: 10.1016/j.seppur.2013.06.010
Magrí, A., Vanotti, M. B., Szogi, A. A., and Cantrell, K. B. (2012). Partial nitritation of swine wastewater in view of its coupling with the anammox process. J. Environ. Qual. 41, 1989–2000. doi: 10.2134/jeq2012.0092
Mata-Alvarez, J., Dosta, J., Romero-Guiza, M. S., Fonoll, X., Peces, M., and Astals, S. (2014). A critical review on anaerobic co-digestion achievements between 2010 and 2013. Renew. Sust. Ener. Rev. 36, 412–427. doi: 10.1016/j.rser.2014.04.039
McGhee, T. J. (1968). A method for approximation of the volatile acid concentrations in anaerobic digesters. Water Sewage Works 115, 162–166.
Molinuevo-Salces, B., Gómez, X., Morán, A., and García-González, M. C. (2013). Anaerobic co-digestion of livestock and vegetable processing wastes: fibre degradation and digestate stability. Waste Manage. 33, 1332–1338. doi: 10.1016/j.wasman.2013.02.021
Muscolo, A., Settineri, G., Papalia, T., Attina, E., Basile, C., and Panuccio, M. R. (2017). Anaerobic co-digestion of recalcitrant agricultural wastes: characterizing of biochemical parameters of digestate and its impacts on soil ecosystem. Sci. Total Environ. 586, 746–752. doi: 10.1016/j.scitotenv.2017.02.051
Philippe, F. X., and Nicks, B. (2015). Review on greenhouse gas emissions from pig houses: production of carbon dioxide, methane and nitrous oxide by animals and manure. Agric. Ecosyst. Environ. 199, 10–25. doi: 10.1016/j.agee.2014.08.015
Rothrock, J. M. J., Szogi, A. A., and Vanotti, M. B. (2010). Recovery of ammonia from poultry litter using gas-permeable membranes. Am. Soc. Agric. Biol. Eng. 53, 1267–1275.
Rothrock, M. J., Szögi, A. A., and Vanotti, M. B. (2013). Recovery of ammonia from poultry litter using flat gas permeable membranes. Waste Manage. 33, 1531–1538. doi: 10.1016/j.wasman.2013.03.011
Vanotti, M. B., and Szogi, A. A. (2015). Systems and Methods for Reducing Ammonia Emissions from Liquid Effluents and for Recovering the Ammonia. U.S. Patent No. 9005333.
Keywords: ammonium, anaerobic digestion, nitrogen recovery, semipermeable membrane, sludge, vegetable sludge
Citation: Oliveira Filho JdS, Daguerre-Martini S, Vanotti MB, Saez-Tovar J, Rosal A, Perez-Murcia MD, Bustamante MA and Moral R (2018) Recovery of Ammonia in Raw and Co-digested Swine Manure Using Gas-Permeable Membrane Technology. Front. Sustain. Food Syst. 2:30. doi: 10.3389/fsufs.2018.00030
Received: 15 March 2018; Accepted: 04 June 2018;
Published: 25 June 2018.
Edited by:
José Luis Garcia-Morales, University of Cádiz, SpainReviewed by:
Anders Peter S. Adamsen, SEGES Agriculture & Food FmbA, DenmarkM. Angeles De La Rubia, Universidad Autonoma de Madrid, Spain
Copyright © 2018 Oliveira Filho, Daguerre-Martini, Vanotti, Saez-Tovar, Rosal, Perez-Murcia, Bustamante and Moral. This is an open-access article distributed under the terms of the Creative Commons Attribution License (CC BY). The use, distribution or reproduction in other forums is permitted, provided the original author(s) and the copyright owner are credited and that the original publication in this journal is cited, in accordance with accepted academic practice. No use, distribution or reproduction is permitted which does not comply with these terms.
*Correspondence: Raul Moral, cmF1bC5tb3JhbEB1bWguZXM=