- 1Poultry Production and Product Safety Research Unit, Agricultural Research Service (USDA), Fayetteville, AR, United States
- 2Department of Animal and Food Sciences, University of Delaware, Newark, DE, United States
- 3Institute of Agriculture, University of Tennessee, Knoxville, TN, United States
- 4Genetics and Sustainable Agricultural Research, Agricultural Research Service (USDA), Mississippi State, MS, United States
- 5Virginia Tech, Blacksburg, VA, United States
- 6Eastern Shore AREC, Virginia Tech, Painter, VA, United States
- 7Biosystems and Agricultural Engineering, Oklahoma State University, Stillwater, OK, United States
- 8National Laboratory for Agriculture and the Environment, Agricultural Research Service (USDA), Ames, IA, United States
Ammonia (NH3), dust and odor emissions from animal feeding operations (AFOs) can cause atmospheric pollution and disputes with neighbors. The objectives of this study were to: (1) re-design the ARS Air Scrubber to improve NH3 removal efficiency, (2) conduct full-scale testing of the scrubber under controlled conditions, (3) evaluate the efficacy of various acids for scrubbing NH3, and (4) determine the effects of air flow rate and NH3 concentration on scrubber performance. A full-scale prototype was constructed and a series of experiments were conducted under various conditions. Acid salts, such as aluminum sulfate (alum), sodium bisulfate, potassium bisulfate, ferric chloride and ferric sulfate were found to work as well as strong acids (hydrochloric, phosphoric and sulfuric) for capturing NH3. The efficiency of the scrubber for capturing NH3 decreased as the ventilation rate increased from over 90% at flow rates of 5,097 m3 h−1 to around 55% at 16,141 m3 h−1. However, the mass of N captured by the scrubber tripled as flow rates increased from 5,097 to 16,141 m3 h−1. Similarly, there was a slight reduction in NH3 removal efficiency as inflow NH3 levels were increased from 10 to 75 μL L−1, whereas the mass of N captured increased from 25 g N h−1 to around 200 g N h−1. This technology could result in the capture of a significant amount of the N lost from AFOs, while simultaneously reducing emissions of dust and odors, which would improve the social and environmental sustainability of poultry and swine production.
Introduction
During the past 20 years there has been increased scrutiny of atmospheric emissions from AFOs in the U.S. since the National Academy of Science reported that there is a urgent need to collect emission data from these facilities (National Academy of Science, 2002). One of the pollutants of greatest concern is NH3, with poultry manure reportedly contributing 27% of the total NH3 emission in the U.S. (U.S. EPA, 2004).
Moore et al. (2011) evaluated NH3 emissions from a typical broiler farm with four tunnel ventilated houses in NW Arkansas. They found that NH3 emissions during the flock, between flocks, during storage and following land application were 28.3, 9.09, 0.18, and 7.91 g NH3 bird−1, respectively. The total NH3 emission was 45.5 g bird−1, with the majority (82%) of the emissions occurring while the litter was still in the barn. Hence, technologies that reduce in-house NH3 should be utilized, when possible. Moore et al. (2011) found the total NH3 emissions for a typical broiler farm with four houses reported was 15,571 kg N yr−1, which was higher than the amount of N that remained in the house as litter or cake (14,464 kg N yr−1). This not only represents a huge waste of an important natural resource since nitrogen (N) is generally the number one element limiting crop production, it also results in substantial air and water pollution.
Environmental problems linked to NH3 emissions include soil acidification, formation of fine particulate matter, and N deposition into aquatic and terrestrial ecosystems (Hutchinson and Viets, 1969; van Breemen et al., 1982; Schroder, 1985; Sutton and Fowler, 2002; Behera and Sharma, 2010; Updyke et al., 2012). When NH3 is deposited via wet or dry fallout, it is converted in the soil to nitrate () via nitrification reactions, generating acidity in the process (van Breemen et al., 1982). In Holland it was estimated that 45% of the total acid deposition was caused by NH3 in 1989 and 85% of the total ammonia emission originated from livestock farming (Groot Koerkamp et al., 1998). Ammonia has also been shown to react with NOx and SO2 to form the dominant fraction of particulate matter less than 2.5 μm (PM2.5) in the atmosphere (Behera and Sharma, 2010; Updyke et al., 2012). Inhalation of such fine particulate matter can be very detrimental to human health (WHO, 2006). Ammonia deposition can also cause species changes in terrestrial environments (Sheppard et al., 2011).
One technology that has been used to control NH3 emissions from animal rearing facilities in Europe is the use of acid scrubbers (Melse and Ogink, 2005; Melse et al., 2006, 2009a, 2012; Van der Heyden et al., 2015). With acid scrubbers exhaust air from animal barns is passed through a reactor that has been filled with an inert packing material that has a large porosity or void volume (Melse and Ogink, 2005). Acidified water is then sprayed on top of the reactor. Exhaust air from the animal barn enters the scrubber either horizontally (cross-current scrubber) or upwards (counter-current scrubbers). Mass transfer of ammonia and other compounds occurs from the gas to liquid phase, which is dependent on the concentration gradient, contact time of gas and liquid phase, and the size of the contact area between the gas and water phase (Melse and Ogink, 2005).
Normally the pH of the water in acid scrubbers is kept between 2 and 4 with the addition of acid. In Holland, acid scrubbers typically use sulfuric acid (Melse and Ogink, 2005), which results in the production of a solution that contains (NH4)2SO4 (ammonium sulfate). Ammonia removal efficiencies reported in several studies of full-scale acid scrubbers in Holland varied from 90 to 99% (Vrielink et al., 1997; Hol and Satter, 1998; Hol et al., 1999; Verdoes and Zonderland, 1999; Wever and Groot Koerkamp, 1999, as cited by Melse and Ogink, 2005; Melse et al., 2012; Van der Heyden et al., 2015). Acid scrubbers also remove odors and dust from exhaust air, with Dutch scrubbers showing an odor removal efficiency of 29–34% (Melse and Ogink, 2005) and a PM-10 (particulate matter less than 10 μm, also called thoracic particles) removal efficiency ranging from 62 to 93% (Melse et al., 2009a).
Currently NH3 scrubbers are not used on poultry or swine barns in the USA. Although the European scrubbers have exceptionally high NH3, dust and odor removal, they are very expensive; often as much as $250,000 per house for the initial investment. Melse et al. (2009b) reported that acid scrubbers were not considered Best Available Technique (BAT) by the European Union, mainly because of the extremely high cost to build and run them, along with the amount of energy and acid needed, which were not considered sustainable. However, the recent BAT reference document for the intensive rearing of poultry or pigs does list acid scrubbers (Joint Research Centre, 2015). Ogink et al. (2008) indicated that acid scrubbers are mainly used in pig housings in Europe and stated that only a few examples of scrubbers on poultry houses were known. The high dust content of air in poultry houses leads to clogging in the packing of the scrubber, causing high pressure drops and an increase in energy use (Ogink et al., 2008).
During the past decade scientists with USDA/ARS in Fayetteville, AR have conducted scrubber research with the goal of developing an inexpensive (< $10,000) acid scrubber that can handle heavy dust loadings, which are typical in air exhausted from broiler houses, without clogging (Angus et al., 2006; Moore et al., 2013). Another goal of this work was to develop a scrubber that is both simple and safe, so that any poultry or swine producer would be able to use the technology without outside technical assistance or hazardous material training. To achieve this goal, Moore (2007, 2014) developed and patented the use of acid salts, such as alum (Al2(SO4)314H2O), potassium bisulfate (KHSO4) and sodium bisulfate (NaHSO4) which can be used as a replacement for strong acids, such as sulfuric acid, to acidify the water. Although one advantage of using acid salts for acid scrubbers is safety, another big advantage is their availability on poultry farms. At present, billions of broiler chickens are grown each year in the USA on litter that has been treated with acid salts, like alum (sold under the tradename Al+Clear) or sodium bisulfate (sold under the tradename PLT) in order to control NH3 levels inside the houses (Moore et al., 1999, 2000; Blake and Hess, 2001; Moore, 2011).
Rather than having an expensive, computer-controlled acid delivery system, the grower would simply use a pH meter or litmus paper every day or two to determine whether the scrubber needs acid and manually add dry acid. Between flocks of birds the scrubbers would be pumped out and the contents would be directly applied to crops on the farm. Martin et al. (2018) found that yields of forages fertilized with scrubber solutions obtained from acid salts like KHSO4 or NaHSO4 were higher than that observed when strong acids were used in the scrubber or when ammonium nitrate was applied at an equivalent N rate (112 kg N ha−1).
The first version of this scrubber was a simple single stage unit that was constructed from wood (Angus et al., 2006). The only moving part in that version was a pump used to circulate the acid. There were multiple problems with this version; (1) high quantities of dust, which have high concentrations of calcium carbonate, would neutralize the acid needed to capture NH3, (2) during cold weather, the contents of the reservoir would freeze, (3) feathers in the exhaust air would clog the pump, and (4) within a year the scrubber begin to leak.
The objectives of this study were to: (1) re-design the ARS Air Scrubber to improve NH3 removal efficiency, (2) conduct full-scale testing of the scrubber under controlled conditions at various ventilation rates, (3) evaluate the practicality and efficacy of various acids for scrubbing NH3 and (4) determine the effects of air flow rate and NH3 concentration entering the scrubber on scrubber performance.
Materials and Methods
Scrubber Construction
A full-scale NH3 scrubber was used in this research. The re-designed scrubber was comprised of two separate scrubbers; the first scrubber uses water to remove particulate matter (dust, bacteria, etc.) from the air, and the second scrubber uses a dilute acid solution to capture NH3 and VOCs (Figures 1, 2). The dimensions of each box are approximately 1.5 × 1.5 × 1.5 m. The re-designed scrubber had shells made out of fiberglass. Each scrubber is equipped with a 360 L reservoir. A simple float switch is used to control the water level in both scrubbers. Both scrubbers are also equipped with acid tolerant heaters in the re-designed scrubber, which are made by Vulcan Industries, which have a 75 cm long heating element, which are totally immersed in the reservoirs. These heaters are programmed to turn on at 3.3°C to keep the contents in the reservoirs from freezing.
The dust scrubber can hold up to 8 rows of wooden slats at 45° angles (Figure 2). Wooden slats are used because wood is much cheaper than plastic or fiberglass and experience has shown that wooden slats have a long lifetime. An ½ HP submersible pump is used to recirculate water in the dust scrubber (Little Giant, 6EN-CIA-SFS). Water from the pump is delivered into three 3.5 cm (OD) PVC pipes which form water curtains at the top of the dust scrubber (Figure 2). The pipes have a series of holes (0.1 cm diameter) from which the water is delivered. The design of this scrubber allows it to be utilized for several flocks of broilers without clogging and if clogs occur, then a long handled brush can be passed through the PVC pipe to remove clogs. The dust scrubber is also equipped with a removable screen above the reservoir to catch feathers and other large particulate matter, which should be cleaned after each flock.
The acid scrubber uses a 1/3 HP magnetically driven pump, which can withstand extremely acidic conditions (IWAKI MK-100RCT), which can deliver acid at a rate of up to 53 L/min. The new design initially included plastic cool cell material (Polypropylene Humipacking made by GEA), which was enclosed in a stainless steel frame on the exhaust end of the scrubber. Although this Humipacking is an effective medium for acid scrubbing (it is used in many European scrubbers), it was found to clog with dust within 3–4 weeks when the scrubber was attached to fans on commercial broiler houses and is no longer being used. This occurred even though the dust scrubber was functioning, indicating small amounts of dust can clog that material.
The experiments reported in this paper were conducted in a USDA/ARS machine shop located at the poultry farm on the main University of Arkansas Agricultural Experiment Station in Fayetteville, AR. The dust and acid scrubbers were connected to a ChoretimeTM 122 cm variable speed poultry house fan via a plenum. This fan has a frequency driver that allows for variable flow rates and is capable of producing air flows up to 33,980 m3 h−1 (20,000 cfm) at 0 Pa.
Airflow measurements in this study were made with a Fan Assessment Numeration System (FANS unit), which was attached to the variable speed fan. The FANS unit measures cumulative outflow of air from the fan throughout the cross-sectional area by measuring air velocities on a transect throughout the fan (Gates et al., 2001, 2004). The FANS unit used for this research was constructed by the University of Kentucky and calibrated by the BESS Laboratory (Dept. of Agri. and Biol. Eng., Univ. of Illinois, Champaign, IL). Static pressure was measured with a Setra 2601MS1 differential pressure sensor.
Effect of Slat Angle on Static Pressure and Air Flow
As part of the scrubber redesign, an experiment was conducted to determine the effect of the angle of the slats in the scrubber on static pressure and air flow. One row of 11 wooden slats (10.2 cm wide and 152.4 cm long) was placed in the dust scrubber only. The slats were all attached to a hinge in which the angle of the slats could simultaneously be varied from 0 to 90°. Air flow and static pressure was determined as described above at angles of 0°, 10°, 20°, 30°, 40°, 50°, 60°, 70°, 80°, and 90° with the variable speed fan set at 40 and 60 Hz.
Effect of Acid Type on Ammonia Scrubbing Efficiency
Ammonia removal efficiencies were measured with various acids at 60 and 40 Hz, which corresponded to air flows of roughly 13,592 and 8,495 m3 h−1 (8,000 and 5,000 cfm). During this experiment there were a total of five slats in the dust scrubber, while the acid scrubber was equipped with three slats and two cool cells. Anhydrous NH3 was metered out into the air in front of the fan using a distribution manifold with five openings at a sufficient rate to result in 25 μL L−1 NH3 entering the scrubber. Air flow rates during each run were measured with the FANS unit as before and pressure drop was measured using the Setra 2601. Ammonia gas concentrations entering and exiting the scrubber were measured every 5 min during the 2 h trials using a photoaccustic multigas analyzer (Innova 1412; Ballerup, Denmark). A tank of 25 μL NH3 L−1 calibration gas (balance air) was used to test the Innova 1412 before and after each trial.
Three 2-h trials were conducted with solutions of the following chemicals at both 40 and 60 Hz: alum, aluminum chloride (AlCl3), calcium chloride (CaCl2), ferric sulfate (Fe2(SO4)3), ferric chloride (FeCl3), sodium bisulfate, potassium bisulfate (KHSO4), sulfuric acid (H2SO4), hydrochloric acid (HCl), phosphoric acid (H3PO4), nitric acid (HNO3) and water. The amount of acid added using strong acids or dry acids was equivalent to two liters of concentrated sulfuric acid (acid equivalent determined by titration with sodium hydroxide). The amount of strong acids added (in ml) to the acid scrubber for H2SO4, HCl, HNO3, and H3PO4 was 2,000, 5,946, 4,613 and 1,622 ml. This amount of acid was generally sufficient to run 4 of the 6 trials needed for each chemical; after the fourth trial the acid scrubber was cleaned out and fresh acid was added. The amount of dry acid (in grams) added for the same four trials with Al2(SO4)314H2O, Fe2(SO4)3, NaHSO4 and KHSO4 was 7,137, 6,744, 8,652, and 9,812. Although calcium chloride is a neutral salt, we wanted to investigate its effect; 3,999 g was used. The amount of acid added for liquid AlCl3 and liquid FeCl3 was 1,164 and 1,200 ml, respectively.
Scrubbing efficiency was calculated by measuring the concentration of NH3 in the scrubber's inflow and exhaust air using the Innova 1412. In order to measure the mass accumulation of NH3+NH4-N in the scrubber solution, 20 ml aliquots of the scrubber solution were taken at time 0, 1, and 2 h from the acid scrubber and analyzed for ammonium colorimetrically with an auto-analyzer using the salicylate-nitroprusside technique (Method 351.2; U.S. EPA, 1979). The pH of these samples was also determined.
Effect of Air Flow Rate and Inlet Ammonia Concentration on Scrubber Performance
In this experiment the effects of varying air flow rate and NH3 concentration entering the scrubber was evaluated. The number of slats in the dust scrubber was kept at 5 and the number of slats in the acid scrubber was kept at 3, but only one cool cell was used. Sodium bisulfate was used as the acid in the acid scrubber at the same levels as above. Ammonia reduction was evaluated at four fan speeds (10, 20, 40, and 60 Hz) which corresponded to air flow rates of 2,131, 4,557, 10,367, and 15,981 m3/h. Three 2-h trials were conducted at each fan speed. Anhydrous NH3 was metered out in front of the fan at sufficient quantities to result in inflow NH3 levels of 25 μL L−1. Ammonia concentrations at the inflow and outflow of the scrubber were measured every 5 min as before. Likewise, the concentration of NH3+NH4 in the scrubber reservoirs, air flow and pressure drop were measured as described above.
The effect of inflow NH3 concentration on scrubber performance was evaluated using the identical scrubber configuration as the air flow experiment. The NH3 levels evaluated in this study were 10, 25, 50, and 75 μL L−1. The desired inflow NH3 levels were maintained by varying the amount of anhydrous NH3, as before. Three 2-h trials were conducted at each of the four NH3 levels at two fan speeds (10,367, and 15,981 m3/h). Inflow and outflow NH3 levels were measured with the Innova 1,412 every 5 min. Concentrations of NH3+NH4 in the reservoirs, air flow, and pressure drop were measured as described earlier.
Statistical analyses of the data were performed using the PROC GLM of SAS Institute (1990). Significant differences among treatment means were determined using Duncan's new multiple-range test at the P < 0.05 level.
Results and Discussion
Effect of Slat Angle on Static Pressure and Air Flow
Preliminary research on the effect of slat angle on static pressure (SP) showed the SP increased exponentially as the slat angle increased (Figure 3). The greatest increase in SP occurred when the angle was greater than 45°. Air flow was reduced as slat angle increased, with the greatest reduction observed at angles greater than 45°. At 60 Hz air flow varied from 34,362 to 17,367 m3 h−1 when slat angles varied from 0 to 90°, whereas at 40 Hz air flow varied from 2,250 to 11,004 m3 h−1 when angles from 0 to 90°. Therefore it was decided to minimize pressure drop while maximizing the possibility of particles colliding with a wet surface that 45° the best angle would be the best angle to use.
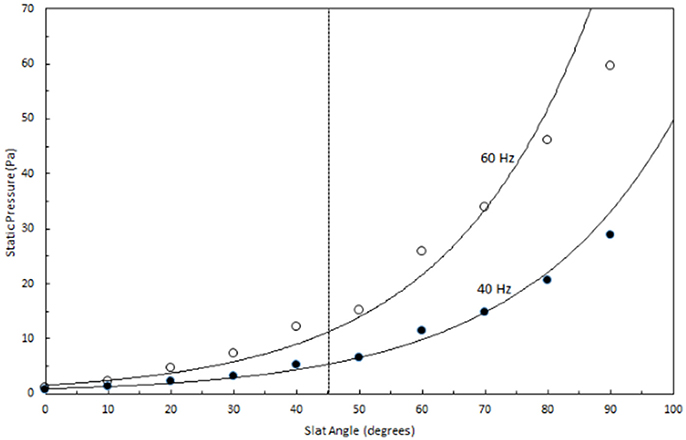
Figure 3. Effect of slat angle on static pressure when one row of slats was placed in the dust scrubber only.
Effect of Various Acids and Water on Ammonia Removal
Ammonia concentrations in the intake and exhaust of the scrubber when NaHSO4 was used are shown in Figure 4. Typically NH3 removal efficiency ((NH3 inflow—NH3 outflow)/NH3 inflow) was slightly better when the variable speed fan was set on 40 Hz (72%), compared to 60 Hz (70%), due to lower air flow rates at 40 Hz (10,367 m3 h−1) than at 60 Hz (15,981 m3 h−1).
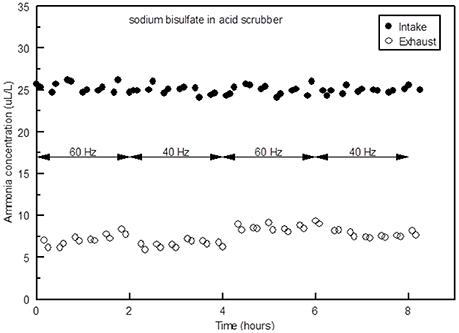
Figure 4. Ammonia concentrations in the intake and exhaust of the scrubber at fans speeds of 40 and 60 Hz with NaHSO4 in the acid scrubber.
Ammonia removal efficiency for tap water was much lower than NaHSO4 or the other acids, as would be expected, although during the first 2 h there was surprisingly good NH3 removal (Figure 5). These data indicate that the scrubber could be operated without acid, if the residence time of the water in the chemical scrubber was kept to around an hour or less. In other words, a system could be devised in which freshwater was constantly trickled into the scrubber before being discharged into an irrigation reservoir. If the NH3 concentration of the air exhausted from the poultry barn was around 25 ppm (average annual concentration found by Moore et al., 2011), then the N concentration of that water would be increased to 50–100 mg N/L, which could help meet the N requirements of any crop being irrigated. A system using water like this may be the most sustainable scrubbing system since water is much cheaper than acid. However, to be cost-effective it would only be feasible on farms which are already growing crops that are irrigated. Other issues may also arise. In Arkansas most liquid waste management systems associated with AFO's fall under Regulation 5, which requires a permit for the operation (VanDevender, 2008). If this is the case, it is unlikely poultry producers would be willing to utilize the system, due to an increased burden of reporting requirements. However, it is unclear whether such as system would cause a broiler farm to all under this regulation.
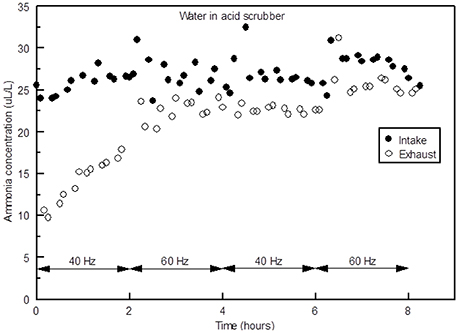
Figure 5. Ammonia concentrations in the intake and exhaust of the scrubber at fans speeds of 40 and 60 Hz with tap water in the acid scrubber.
The concentration of NH3 in the scrubber after an 8 h period with NaHSO4 in the acid scrubber was over 2,000 mg N/L, whereas when water was used in the acid scrubber the concentration was lower by an order of magnitude (Figure 6). This difference in NH3 removal is due to the difference in pH. The pH of tap water started out around 8.4 and increased to over 9.5 after 1 h, whereas when NaHSO4 was added the pH started out at around 1.1 and increased to around 2.5 after hours (Figure 7). When the pH of a scrubbing solution is acidic, NH3 in solution is converted to , which can build up in solution, whereas under very alkaline pH conditions the ammoniacal N stays in the NH3 form and the amount in solution is limited by the solubility of NH3 in water.
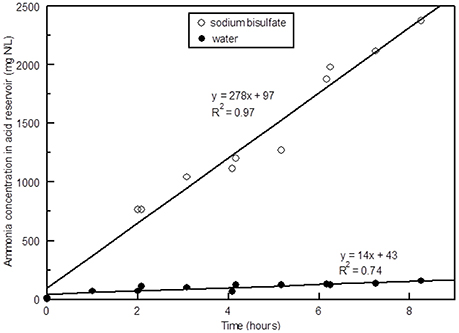
Figure 6. Ammonia (plus ammonium) concentration in the acid scrubber vs. time with NaHSO4 vs. tap water.
The effectiveness of the various compounds tested is shown in Table 1. The two acid salts of Fe (Fe2(SO4)3 and FeCl3) resulted in slightly better reductions in NH3 levels in the exhaust that the other acids. Iron compounds resulted in the formation of iron oxides or hydroxides on the scrubber, which may have increased the surface area. Likewise, the Al compounds formed aluminum oxides or hydroxides on scrubber surfaces. Potassium bisulfate worked as well as the Fe and Al compounds at high air flow rates maintained at 60 Hz (15,981 m3 h−1) and as well as the Al compounds and FeCl3 at 10,367 m3 h−1 (40 Hz). Potassium bisulfate performed slightly better than NaHSO4 at 15,981 m3 h−1 (60 Hz). Potassium bisulfate also performed better than HCl, HNO3, and H3PO4.
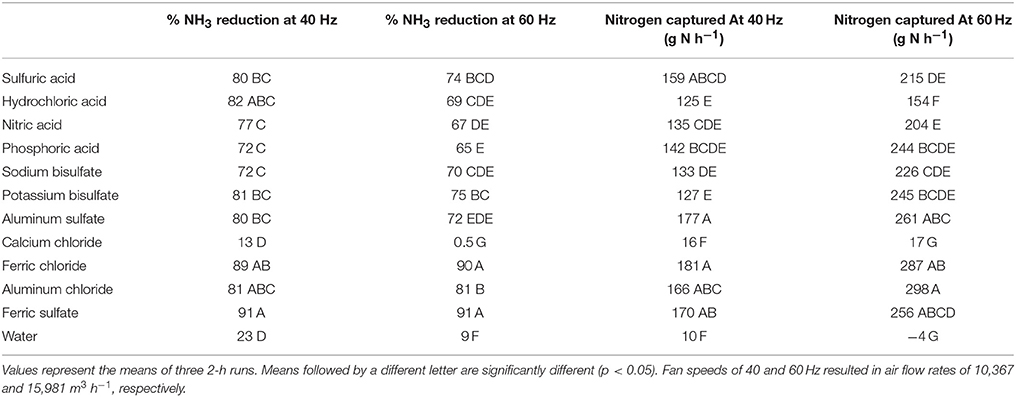
Table 1. Relative NH3 reduction efficiency and rate of N captured for strong acids, acid salts, calcium chloride and water at two ventilation rates.
The amount of N captured in the scrubber with various compounds is shown Table 1. At 15,981 m3 h−1 (60 Hz) KHSO4 performed as well as all of the strong acids and most of the acid salts, with the exception of AlCl3, whereas at 10,367 m3 h−1 (40 Hz) the Al and Fe compounds outperformed KHSO4. Although AlCl3 performed well with respect to capturing NH3 it formed a gelatinous white mass in the scrubber reservoirs. This did not occur with alum.
From an agronomic point of view, KHSO4 would probably be the most sustainable acid salt for this purpose, since its use would result in the formation of an ammonium potassium sulfate fertilizer in the scrubber. In a study evaluating the fertilizer effectiveness of various scrubber solutions obtained from ARS Air Scrubbers attached to fans on a commercial broiler farm, Martin et al. (2018) found forage yields were higher when fertilized with scrubber solutions from KHSO4 and NaHSO4 than other scrubber solutions or ammonium nitrate applied at an equivalent N rate (112 kg N ha−1). However, at present KHSO4 is not produced in North America on an industrial scale, therefore the price is much higher than the other acids.
Effects of Air Flow and Ammonia Concentration on Scrubber Efficiency
As ventilation increased from 2,000 to 10,000 m3 h−1, static pressure increased from 0 to 30 Pa (Figure 8). This is similar to the 27.5 Pa backpressure reported by Manuzon et al. (2007) for a three stage wet scrubber. As mentioned earlier, the scrubber described in the current study was designed for use on minimum vents, which typically operate at 10,194 m3 h−1 or less. Under such ventilation rates, the static pressure should always be less than 37.4 Pa (0.15” of water), which is far better than pressure drop (74.7 Pa) that is allowed by the USDA/NRCS Conservation Practice Standard 371 for air scrubbers (USDA/NRCS, 2010).
The efficiency of the scrubber was over 90% when air flow rates were 4,000 m3 h−1 or less with an intake NH3 concentration of 25 μL L−1, but dropped linearly as the flow rate increased (Figure 9). At 10,000 m3 h−1 the average NH3 reduction was around 70%. Although the efficiency decreased as the flow rate increased, the amount of N captured increased from less than 20 g N h−1 at lowest flow rate to near 60 g N h−1 at the highest (Figure 10).
There was a slight reduction in NH3 removal efficiency when the intake NH3 concentration was increased from 10 to 75 μL L−1 (Figure 11). The scrubbing efficiencies reported here are similar to the two stage scrubber tested by Manuzon et al. (2007) that had a 64–70% efficiency at NH3 concentrations of 100 and 30 μL L−1, respectively.
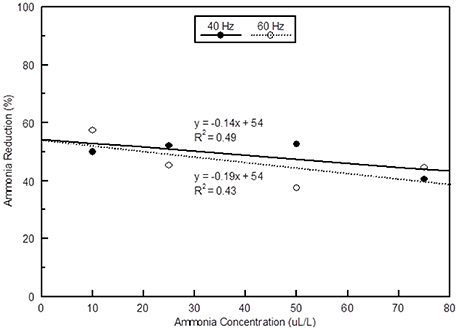
Figure 11. The effect of NH3 concentration on the relative amount of NH3 reduction at two ventilation rates.
The mass of N captured increased linearly from around 25 g N h−1 at 10 μL L−1 to approximately 200 g N h−1 at 75 μL L−1 (Figure 12). As mentioned earlier, Moore et al. (2011) found average NH3 levels in poultry houses were much higher during cooler months (42.5 μL L−1) than in the summer (11.1 μL L−1), because during warmer periods of the year the ventilation in the houses is greatly increased to relieve heat stress from the birds. These data indicate that these scrubbers will capture the greatest amount of N during the cooler months of the year when NH3 concentrations are highest.
Although the ARS Air Scrubber is not nearly as effective as European scrubbers, which have NH3 removal efficiencies of up to 95%, they can be manufactured for a small fraction of the cost and they will operate under very dusty conditions. However, these scrubbers were designed for minimum vent fans on broiler house, in order to capture the most N per dollar spent (Melse et al., 2006); therefore when birds are older and tunnel ventilation is being used, only a small percentage of the total air volume will be scrubbed.
Conclusions
A full-scale prototype of the ARS Air Scrubber was constructed and a series of experiments were conducted to evaluate its ability to capture NH3. Research on the effects of ventilation rate and NH3 concentration indicated that as either variable was increased, the efficiency of NH3 removal decreased somewhat, whereas the mass of N captured increased dramatically. As NH3 concentrations increased from 10 to 75 μL L−1 the amount of N captured increased by almost an order of magnitude (25–200 g N h−1), indicating it is most efficient when NH3 levels are high, which is typical during cooler months. This technology could potentially capture a significant fraction of N currently lost from poultry and swine facilities as NH3, which may improve the sustainability of these operations.
Author Contributions
All authors listed have made a substantial, direct and intellectual contribution to the work, and approved it for publication.
Funding
Funding for this research was provided by USDA/ARS.
Disclaimer
Mention of a trade name, proprietary product, or specific equipment does not constitute a guarantee or warranty by the USDA and does not imply its approval to the exclusion of other products that may be suitable.
Conflict of Interest Statement
The authors declare that the research was conducted in the absence of any commercial or financial relationships that could be construed as a potential conflict of interest.
References
Angus, A. J., Hodge, I. D., McNally, S., and Sutton, M. A. (2006). “Evaluation and management of ammonia emissions from poultry litter,” in Workshop on Agricultural Air Quality: State of the Science, eds V. P. Aneja, W. H. Schlesinger, R. Knighton, G. Jennings, D. Niyogi, W. Gilliam and C. S. Duke (Potomac, MA; Raleigh, NC: North Carolina State University), 304–310.
Behera, S. N., and Sharma, M. (2010). Investigating the potential role of ammonia in ion chemistry of fine particulate matter formation for an urban environment. Sci. Total Environ. 408, 3569–3575. doi: 10.1016/j.scitotenv.2010.04.017
Blake, J. P., and Hess, J. B. (2001). Sodium Bisulfate (PLT) as a Litter Amendment. ACES Publications. ANR-1208. Auburn, AL: Alabama A&M and Auburn Universities.
Gates, R. S., Simmons, J. D., Casey, K. D., Greis, T. J., Xin, H., and Barnett, E. F. (2001). Fan Assessment Numeration System (FANS) Design and Calibrations Specifications. ASAE paper No. 024124, ASAE, St. Joseph, MI.
Gates, R. S., Casey, K. D., Zin, H., Wheeler, E. F., and Simmons, J. D. (2004). Fan assessment and numeration system (FANS) design and calibration specifics. Trans. ASAE 47, 1709–1715. doi: 10.13031/2013.17613
Groot Koerkamp, P. W. G., Metz, J. H. M., Uenk, G. H., Phillips, V. R., Holden, M. R., and Wathes, R. W. (1998). Concentrations and emissions of livestock buildings in Northern Europe. J. Agri. Eng. Res. 70, 79–95. doi: 10.1006/jaer.1998.0275
Hol, J. M. G., Wever, A. C., and Groot Koerkamp, P. W. G. (1999). Acid Scrubbing of Exhaust Air from Broilers (in Dutch). Report P 99–23, Wageningen: DLO Instituut voor.
Hol, J. M. G., and Satter, I. H. G. (1998). Acid Scrubbing of Exhaust Air from Free-range Layer Breeders (in Dutch). Report 98–1002. Wageningen: DLO Instituut voor Milieu- en Agritechniek (IMAG-DLO).
Hutchinson, G. L., and Viets, F. G. Jr. (1969). Nitrogen enrichment of surface water by absorption of ammonia volatilized from cattle feedlots. Science 166, 514–515. doi: 10.1126/science.166.3904.514
Joint Research Centre (2015). Best Available Techniques (BAT) Reference Document for the Intensive Rearing of Poultry or Pigs. Industrial Emissions Directive 2010/75/EU. Available online at: http://eippcb.jrc.ec.europa.eu/reference/BREF/IRPP_Final_Draft_082015_bw.pdf
Manuzon, R. B., Zhao, L. Y., Keener, H. M., and Darr, M. J. (2007). A prototype acid spray scrubber for absorbing ammonia emissions from exhaust fans of animal buildings. Trans. ASABE 50, 1395–1407. doi: 10.13031/2013.23628
Martin, J. W., Moore, P. A. Jr., Li, H., Ashworth, A. J., and Miles, D. M. (2018). Effects of land-applied ammonia scrubber solutions on yield, nitrogen uptake, soil test phosphorus, and phosphorus runoff. J. Environ. Qual. 47, 263–269. doi: 10.2134/jeq2017.09.0383
Melse, R. W., van Wagenberg, A. V., and Mosquera, J. (2006). Size reduction of ammonia scrubbers for pig and poultry houses: use of conditional bypass vent at high air loading rates. Biosyst. Eng. 95, 69–82. doi: 10.1016/j.biosystemseng.2006.05.006
Melse, R. W., Hofschreuder, P., and Ogink, N. W. M. (2012). Removal of particulate matter (PM10) by air scrubbers at livestock facilities: results of an on-farm monitoring program. Trans. ASABE 55, 689–698. doi: 10.13031/2013.41378
Melse, R. W., and Ogink, N. W. M. (2005). Air scrubbing techniques for ammonia and odor reduction at livestock operations: review of on-farm research in the Netherlands. Trans. ASAE 48, 2303–2313. doi: 10.13031/2013.20094
Melse, R. W., Ogink, N. W. M., and Rulkens, W. H. (2009a). Air treatment techniques for abatement of emissions from intensive livestock production. Open Agric. J. 3, 6–12. doi: 10.2174/1874331500903010006
Melse, R. W., Ogink, N. W. M., and Rulkens, W. H. (2009b). Overview of European and Netherlands' regulations on airborne emissions from intensive livestock production with a focus on the application of air scrubbers. Biosyst. Eng. 104, 289–298. doi: 10.1016/j.biosystemseng.2009.07.009
Moore, P. A. Jr. (2007). Method and Device for Scrubbing Ammonia From Air Exhausted From Animal Rearing Facilities. U.S. Patent 7,194,979, Fayetteville, AR (Accessed March 27, 2007).
Moore, P. A. Jr. (2014). Animal Containment Facility Ventilation System. U.S. Patent 8,663,551, Fayetteville, AR (Accessed March 4, 2014).
Moore, P. A. Jr. (2011). “Improving the sustainability of animal agriculture by treating manure with alum,” in Environmental Chemistry of Animal Manure, ed Z. He (Hauppauge, NY: Nova Science Publishers, Inc.), 349–381.
Moore, P. A. Jr., Miles, D. M., Burns, R., Berg, K., and Choi, I. H. (2011). Ammonia emission factors from broiler litter in barns, in storage, and after land application. J. Environ. Qual. 40, 1395–1404. doi: 10.2134/jeq2009.0383
Moore, P.A., Maguire, R., Reiter, M., Ogejo, J., Burns, R., Li, H., et al. (2013). Development of an Acid Scrubber for Reducing Ammonia Emissions From Animal Rearing Facilities. Available online at: http://www.extension.org/pages/67663/development-of-an-acid-scrubber-for-reducing ammonia-emissions-from-animal-rearing-facilities#VAyY35hOzEY
Moore, P. A. Jr., Daniel, T. C., and Edwards, D. R. (1999). Reducing phosphorus runoff and improving poultry production with alum. Poult. Sci. 78, 692–698. doi: 10.1093/ps/78.5.692
Moore, P. A. Jr., Daniel, T. C., and Edwards, D. R. (2000). Reducing phosphorus runoff and inhibiting ammonia loss from poultry manure with aluminum sulfate. J. Environ. Qual. 29, 37–49. doi: 10.2134/jeq2000.00472425002900010006x
National Academy of Science (2002). The Scientific Basis for Estimating Emissions From Animal Feeding Operations: Interim Report. Available online at: https://www3.epa.gov/ttnchie1/ap42/ch09/draft/interimanimalfeed.pdf
Ogink, N. W. M., Melse, R. W., and Mosquera, J. (2008). “Multi-pollutant and one-stage scrubbers for removal of ammonia, odor, and particulate matter from animal house exhaust air,” in Proceedings of Livestock Environment VIII (Iguassu Falls: ASABE Publications Num. 701P.0408). 269–275.
Schroder, H. (1985). Nitrogen losses from Danish agriculture-trends and consequences. Agri. Ecosyst. Environ. 14:279–289. doi: 10.1016/0167-8809(85)90042-8
Sheppard, L. J., Leith, I. D., Mizunuma, T., Cape, J. N., Crossley, A., and Fowler, S. (2011). Dry deposition of ammonia gas drives species change faster than wet deposition of ammonium ions: evidence from a long-term field manipulation. Global Change. Biol. 17, 3589–3607. doi: 10.1111/j.1365-2486.2011.02478.x
Sutton, M. A., and Fowler, D. (2002). Introduction: fluxes and impacts of atmospheric ammonia on national, landscape and farm scales. Environ. Pollut. 119, 7–8. doi: 10.1016/S0269-7491(01)00145-2
USDA/NRCS (2010). Air Filtration and Scrubbing. Code 371. Natural Resources Conservation Service Conservation Practice Standard. Available online at: https://www.nrcs.usda.gov/Internet/FSE_DOCUMENTS/nrcs143_026146.pdf
Updyke, K. M., Nguyen, T. B., and Nizkorodov, S. A. (2012). Formation of brown carbon via reactions of ammonia with secondary organic aerosols from biogenic and anthropogenic precursors. Atmos. Environ. 63, 22–31. doi: 10.1016/j.atmosenv.2012.09.012
U.S. EPA (1979). Methods for Chemical Analysis of Water and Wastes. USEPA Report 600/4-79-020. Washington, DC: U.S. EPA.
U.S. EPA (2004). National Emission Inventory – Ammonia Emissions from Animal Husbandry Operations. Washington, DC: U.S. EPA.
van Breemen, N., Burrough, P. A., Velthorst, E. J., van Dobben, H. F., de Wit, T., and Reijinders, T. B. (1982). Soil acidification from atmospheric ammonium sulphate in forest canopy throughfall. Nature 299, 548–550. doi: 10.1038/299548a0
Van der Heyden, C., Demeyer, P., and Volcke, E. I. P. (2015). Mitigating emissions from pig and poultry housing facilties through air scrubbers and biofilers: state-of-the-art and perspectives. Biosys. Eng. 134, 74–93. doi: 10.1016/j.biosystemseng.2015.04.002
VanDevender, K. (2008). Regulation No. 5: Liquid Animal Waste Management Systems. University of Arkansas Cooperative Extension Service FSA3004. Available online at: https://www.uaex.edu/publications/PDF/FSA-3004.pdf
Verdoes, N., and Zonderland, J. J. (1999). The Effect of a Chemical Air Scrubber on Ammonia Emission From a Growing-Finishing Pig House (in Dutch, With English Summary). Report P 4.39, Praktijkonderzoek Varkenshouderij, Rosmalen.
Vrielink, M. G. M., Verdoes, N., and Van Gastel, J. P. B. F. (1997). Reducing the Ammonia Emission With a Chemical Air Scrubber (in Dutch, With English Summary). Report P 1.178, Praktijkonderzoek Varkenshouderij, Rosmalen.
Keywords: ammonia, scrubber, poultry, emissions, air pollution, nitrogen, recycling
Citation: Moore PA Jr, Li H, Burns R, Miles D, Maguire R, Ogejo J, Reiter MS, Buser MD and Trabue S (2018) Development and Testing of the ARS Air Scrubber: A Device for Reducing Ammonia Emissions from Animal Rearing Facilities Front. Sustain. Food Syst. 2:23. doi: 10.3389/fsufs.2018.00023
Received: 28 March 2018; Accepted: 29 May 2018;
Published: 15 June 2018.
Edited by:
Maria Cruz Garcia Gonzalez, Instituto Tecnológico Agrario de Castilla y León, SpainReviewed by:
Xavier Flotats, Universitat Politecnica de Catalunya, SpainClinton D. Church, Pasture Systems and Watershed Management Research Unit (USDA-ARS), United States
Copyright © 2018 Moore, Li, Burns, Miles, Maguire, Ogejo, Reiter, Buser and Trabue. This is an open-access article distributed under the terms of the Creative Commons Attribution License (CC BY). The use, distribution or reproduction in other forums is permitted, provided the original author(s) and the copyright owner are credited and that the original publication in this journal is cited, in accordance with accepted academic practice. No use, distribution or reproduction is permitted which does not comply with these terms.
*Correspondence: Philip A. Moore Jr., philip.moore@ars.usda.gov