- School of GeoSciences, and Moray House School of Education, University of Edinburgh, Edinburgh, United Kingdom
The global food system contributes approximately one-quarter of greenhouse gas (GHG) emissions, with these dominated by the livestock sector. The projected increase in livestock demand is likely to undermine efforts to keep global average warming below a 2°C target. A carbon tax is often proposed as the preferred demand-side mechanism for reduced meat consumption. Previous studies, however, suggest that while this could prove successful in reducing net global emissions, it may worsen nutritional standards in lowest-income nations. An alternative market mechanism which may simultaneously reduce GHG emissions and improve health at all income levels is a reduction in the price of meat substitute products (meat-free proteins with particular nutritional and aesthetic similarities to meat). Using a combined ecological and health modeling approach, we project the associated GHG savings and health benefits associated with a stepwise reduction in the price of meat substitute products. Utilizing food demand elasticities, we quantify the substitution of meat commodities across a range of social acceptability scenarios. Our results show that meat substitute products—integrated within a “flexitarian” approach (primarily vegetarian but occasionally eating meat and fish)—have a large potential for reducing GHG emissions (up to 583 MtCO2e per year) and improving nutritional outcomes (up to 52,700 premature deaths avoided per year). However, this capacity is strongly dependent on a combination of price reductions and improved social acceptability of this product group; therefore both will be essential.
Introduction
Agriculture and food production are estimated to contribute approximately 25–30% of global greenhouse gas (GHG) emissions, dominated by the livestock sector which accounts for an estimated 14.5% globally (World Watch Institute, 2009; IPCC, 2014). Meat consumption shows strong coupling to economic growth (Fiala, 2008); as a result, the combination of continued population growth and economic development means global meat consumption is projected to increase by 75–80% by 2050 (Wellesley et al., 2015). Without a significant reduction in the GHG-intensity (quantity of greenhouse gases emitted per unit of meat produced) of livestock production, it's likely that this level of meat consumption will undermine efforts to keep average global warming below 2°C [as targeted within the UN Paris Agreement (UNFCCC, 2015)] in the twenty first century (UNFCCC, 2015). GHG emissions mitigation within the livestock, and broader agriculture sector, will therefore be essential in meeting global climate targets.
Meat consumption can have both positive and negative implications for nutrition. Whilst animal-based products generally have a higher GHG emissions-intensity relative to plant-based food commodities, moderate consumption of meat and dairy products can have a significant positive impact on human health and nutrition, providing high-quality protein, complete amino acid composition, and a vital source of micronutrients such as iron, calcium, zinc and vitamin B12 (Wu et al., 2014). This is particularly important in developing nations where per capita meat intake is often lower than in developed nations, and where dietary composition is typically dominated by micronutrient-poor cereals and starchy roots (Gómez and Ricketts, 2013). Even small amounts of meat supplementation to the diets of low-income households has been shown to have nutritional benefits and reduce severe malnourishment indicators such as childhood stunting (Rivera et al., 2003).
In contrast, the overconsumption of meat within the typical Western diet can have severe negative health implications, including increased risk of chronic diseases such as cardiovascular disease, stroke and some forms of cancer (also known as “non-communicable diseases”; NCDs) (Walker et al., 2005). In the Global Burden of Disease (GBD) study, red and processed meat in particular are known and quantified risk factors for a number of NCDs (Forouzanfar et al., 2016), analysis which forms a critical component of the World Health Organization's (WHO) action plan on combating NCDs (WHO, 2013).
Being able to simultaneously address the second United Nations Sustainable Development Goal - SDG2 (zero hunger and malnutrition) and SDG13 (urgent action to combat climate change) will require a convergence of meat consumption trends: a sustainable increase in intakes within developing nations, and a significant decrease in per capita consumption within the typical high-income diet. The reduced risk of non-communicable diseases (NCDs) as a result of reduced red and processed meat consumption in particular will also be important in meeting global targets on NCDs as part of SDG3 (good health and wellbeing) (United Nations, 2017). The ability to simultaneously enhance global GHG mitigation efforts and improve human nutritional health would be a notable win-win scenario for society; all the more significant due to the timescale over which the SDGs are to be achieved (2015–2030).
While a number of demand management policies and market-based levers have been suggested to reduce average meat intakes, a carbon taxation on food commodities is often recommended as the preferable approach (Cuevas and Haines, 2016). Since animal-based products tend to have a higher carbon footprint than plant-based alternatives, they would experience a proportionally higher price increase under a carbon taxation scheme. This holds potential in driving consumers toward alternatives with a lower carbon-intensity.
Results of the first (and only, to date) global assessment of the GHG mitigation and health impacts of emissions pricing of food commodities indicate that levying GHG taxes could have a synergistic positive impact on human health and emissions reduction across most countries—particularly high and middle-income nations (Springmann et al., 2016c). The key potential drawback to such a tax-based mechanism is a negative nutritional impact on low-income nations and households; even in regionally-optimized tax scenarios, emissions pricing would result in an increase in the prevalence of underweight individuals (Springmann et al., 2016c). These negative impacts may be more concerning still if overall dietary quality, including high-quality protein, fat and micronutrient intake, were assessed—a food commodity tax may push low-income groups further toward a low-cost cereal-dominant diet (Gómez-Galera et al., 2010; Gómez and Ricketts, 2013). Therefore, while a food carbon tax would result in promising GHG reductions and provide a net human health benefit globally, the negative consequences for low-income groups and regions cannot be ignored if SDG2 is also to be addressed.
An alternative market mechanism which may support both improved nutrition and GHG mitigation at all income levels, is a significant decrease in the market price of meat substitute products. Meat substitutes (also termed “meat analogs” or “meat-free alternatives”) are products which share aesthetic and chemical qualities (such as texture, taste and appearance) with certain meat products (Joshi and Kumar, 2016). The meat substitute market has grown strongly in recent years, largely due to increasing awareness of the purported health and ecological benefits of reduced meat consumption (MINTEL, 2014). Despite continued growth, the overall market share of meat substitutes is small, with only 3–5% of the meat market in Europe (MINTEL, 2013). There are a number of social challenges to the uptake of meat substitute products, however, one of the largest barriers is their often high price relative to the meat products they are intended to replace (Apostolidis and McLeay, 2016). This provides no economic incentive for substitution, relying on social motivations alone—as a result meat substitutes typically occupy a niche, premium segment of the market (Ritchie et al., 2017).
Financial incentives have been highlighted as one of the prime mechanisms which may be utilized to drive a larger transition toward meat substitutes (Apostolidis and McLeay, 2016). These could involve financial interventions such as subsidization, however, a more sustainable scenario would be of a natural reduction of meat substitute production and retail costs through technological innovation and efficiencies of scale. A range of meat substitute products are at the stage of technological development, with the commercialization of a growing number of products in turn helping to create a more competitive market. As a result, the unit cost of these products is likely to decrease with time. For example, a proposed process innovation in the production of mycoprotein (the base protein component of the global branded leader Quorn™) is expected to halve current production costs (Ritchie et al., 2017), potentially making the unit cost to consumers lower than meat alternatives.
Meat substitutes have the potential to simultaneously address SDG2 (zero hunger) and SDG6 (clean water and sanitation) since they not only have a lower (and declining) carbon-intensity relative to most meat products, but can also offer significant nutritional benefits. Plant-based proteins typically provide high-quality protein with high digestibility and amino acid scoring and a range of key micronutrients, but with a lower caloric and fat content relative to animal-based proteins (Denny et al., 2008). The nutritional suitability of meat substitutes relative to meat products is further addressed within the present study's discussion. A low-cost supply of sustainable protein could therefore help to avoid the drawback of a carbon taxation scheme: it would carry similar nutritional benefits for reduction in NCD risk factors and obesity in meat-intensive diets, but would also help to address protein and micronutrient malnutrition in low-income nations.
While the sustainability and nutritional benefits of meat substitute products are often highlighted at an individual dietary level (Denny et al., 2008; Smetana et al., 2015), no comprehensive analysis has been conducted on the overall scale of these benefits at the national, regional and international level.
Here we present what we believe is the first analysis of the magnitude of GHG mitigation and human health benefits which could be achieved through price reductions within the meat substitute sector. This combined ecological and health analysis approach aligns with the emergent and shifting field of shifting from public health toward “planetary health” which attempts to recognize the interdependencies and of benefits of human health alongside reductions in environmental impact (Horton et al., 2014; Whitmee et al., 2015). A combined ecological and health approach has been widely used in dietary-climate impact analyses within the field (Tilman and Clark, 2014; Springmann et al., 2016a,c).
This analysis is based on a combination of meat price elasticity relationships with a range of social acceptability scenarios. Our assessment is based on the meat substitute product mycoprotein, which is currently sold solely under the brand name Quorn™. We have selected mycoprotein for several reasons: Quorn™ is currently the global branded leader in the meat analog sector; in-depth life-cycle assessments (LCA) of the product are publicly available (Finnigan, 2010; Smetana et al., 2015); and significant price reductions are deemed to be technologically realistic (Ritchie et al., 2017). However, the methodology and concept utilized in this paper could be readily applied to any similar meat substitute product.
Methods
Quantifying Meat Substitution Rates
Two key variables were adjusted in this analysis: level of price reduction in meat substitutes, and level of social acceptability. In this analysis, mycoprotein in the form of bulk Quorn™ products was selected as choice of meat substitute; Quorn™ global branded leader in the meat analog sector; and has in-depth life-cycle assessments (LCA) publicly available (Finnigan, 2010; Smetana et al., 2015). Mycoprotein sales are currently limited to markets within Europe, the United States of America (USA), Australia, New Zealand and South Africa. While the expansion of meat substitute markets into additional high- to middle-income countries is likely, there has been little discussion of their potential emergence within developing nations. Social attitudes and acceptance of these types of products within developing nations is therefore insufficiently understood. For this reason, and in addition to poor data availability, the present study has focused on the quantification of meat substitution within higher-income markets. However, the potential for meat substitute products within lower-income and transitioning economies is addressed within our discussion, and in our view, deserves more attention. Our assessment considers potential impacts across 40 high-income countries which are either existing markets for mycoprotein, or are likely to be in the near future (see Table A1 in Supplementary Material). Countries analyzed in this study were either OECD countries, or those considered within the OECD-FAO Agricultural Outlook as “developed” (OECD, 2016). Scenarios are focused on the year 2020—a near-term date by which time lower-cost meat substitute products may realistically become commercialized.
Our analysis considers the price-induced changes in consumption which would occur through incremental price reductions of meat substitutes from present-day prices. This is modeled based on cross-price elasticities, which measure the change in demand for one “good,” based on a price change in another (Cornelsen et al., 2014).
The level of meat substitution, and resultant changes in consumption were modeled using economic demand elasticity methods. Cross-price elasticities were utilized from one of the most up-to-date assessments of demand variations with price, income and product category (Lusk and Tonsor, 2016). Credible data on the cross-price elasticity of demand for meat substitute products is scarce. In this assessment, we have therefore utilized cross-price elasticities of meat products relative to a price reduction in chicken—which is the lowest cost, and often considered lowest quality, of meat products in the regions considered (Lusk and Tonsor, 2016). We note that this introduces an important assumption with limitations. As we address in our discussion, approaches to dietary change may vary depending on consumer segment. Consumer acceptance of meat substitute products—particularly with regards to comparability to lower-impact meat products, such as poultry—deserves further attention. The demand elasticity assessment used in this study was based on choices made by 12,255 US consumers across low, middle and high-income levels. In our “perfect” substitution scenario (scenario 1), cross-price elasticities have been applied based on change in demand relative to a price reduction in chicken (with chicken being the cheapest form of meat). To map the effect that meat substitutes would have on chicken demand, we have applied cross-price elasticities of high-quality chicken with lower-quality, cheaper chicken cuts.
It cannot be assumed that consumers would respond to a price reduction in meat substitutes in the same way that they would to the same reduction in chicken. To account for this uncertainty, we have modeled five scenarios which reflect differing levels of social acceptance of mycoprotein as a viable meat substitute. Scenario 1 is based on a high level of social acceptability, and assumes a perfect reflection of chicken cross-price elasticities (i.e., that consumers respond to price reductions in meat substitutes in exactly the same way as they do to chicken). Scenarios 2 and 3 are based on a medium and low level of social acceptability, respectively. Results are based on a respective 50 and 25% change in substitution relative to scenario 1 (perfect substitution). Scenarios 4 and 5 are ambitious, but have been included to cover the possibility of increased social preference relative to chicken—they assume a 125 and 150% change in substitution relative to scenario 1 results, respectively. These scenarios would reflect the case where meat substitutes became increasingly preferred over meat as a result of increased health, nutrition and sustainability concerns. Achieving such a drastic change in consumer preference would require significant governmental and advisory input. Note that the results of this study report the additional price-induced changes in consumption, which will allow for market penetration to the average consumer; it is acknowledged that in scenarios 1–3, meat substitutes will already hold a share—albeit small—of the market.
The five social acceptability/preference scenarios are summarized below:
Scenario 1 = high social acceptability = perfect substitution (i.e., consumers respond to a price decrease in meat substitutes in the same way as they would with chicken);
Scenario 2 = medium social acceptability = cross-price elasticities are assumed to be 50% of those utilized in scenario 1 (i.e., consumers are only half as responsive to a change in price);
Scenario 3 = low social acceptability = cross-price elasticities are assumed to be 25% of those utilized in scenario 1 (i.e., consumers are only one-quarter as responsive to a change in price);
Scenario 4 = social preference = cross price elasticities are assumed to be 125% of those utilized in scenario 1 (i.e., consumers are 25% more responsive to a change in price due to positive social attitudes);
Scenario 5 = high social preference = cross price elasticities are assumed to be 150% of those utilized in scenario 1 (i.e., consumers are 50% more responsive to a change in price due to positive social attitudes).
Price reductions are mapped in five percent increments from a 5 to 75% reduction in the average 2015 market price of standard Quorn™ products ($US8.52 per kg) relative to chicken (US$7.52 per kg) from World Bank data. This range of price reductions and consumer acceptability scenarios attempt to provide quantification across the large spectrum of possible scenarios. This allows for assessment of the lower and upper range of possibilities. A price reduction of 75% relative to current prices represents a highly ambitious scenario, although technological innovation with potential to reduce price by at least 50% has been deemed feasible (Ritchie et al., 2017). As is shown in results of scenarios 1–3, standard market prices of Quorn™ products are 10% more expensive than chicken per unit, meaning no substitution effect occurs until these products reach price-parity. This relative price reduction is then multiplied by cross-price elasticity values to attain changes in consumption of each of the meat commodities (beef, pigmeat, chicken, and sheep). To calculate total reductions in consumption, business-as-usual (BAU) baseline emissions were first calculated based on projected per capita meat intake (by commodity) (OECD, 2016) and UN Population Division projections in 2020 (United Nations, 2015) for each country included in this study (see Table A1 in Supplementary Material). Reductions in meat consumption were then calculated based on changes in consumption relative to BAU levels.
Here we assume a substitution of Quorn™ mycoprotein for meat products on an equal mass basis (for example, a 100 g substitution of mycoprotein for 100 g of meat). A number of assessments have found higher satiety benefits of mycoprotein relative to meat products served in similar meal contexts (Turnbull et al., 1993; Denny et al., 2008; Finnigan et al., 2016). Mycoprotein may therefore be consumed in lower quantities relative to meats as a substitute. However, due to insufficient evidence on the magnitude of such differences we have assumed a conservative approach of perfect substitution by mass.
Quantifying Greenhouse Gas (GHG) Reductions
To convert changes in meat consumption to changes in GHG emissions, we used commodity-specific GHG intensities (kilograms of carbon-dioxide equivalents of GHG emissions emitted to produce 1 kg of product; kgCO2e/kg product). We adopted average FAO livestock emission factors measured and reported across the full value chain, from farm-gate to retail sale (see Table A3 in Supplementary Material) (Gerber et al., 2013). The GHG intensity of livestock systems varies, both in terms of the type and intensity of farming system, and the geographical context. Here we adopted global average FAO figures, however we acknowledge that actual intensities introduce variability—both above and below this average depending on context. The assessed GHG intensity of mycoprotein in the form of Quorn™ products was adopted from full life-cycle analysis (LCA) assessments—these evaluations extend beyond the footprint of the mycoprotein base product to the total GHG intensity of production of the final marketable product (Finnigan, 2010; Smetana et al., 2015). GHG emissions savings were calculated based on baseline emissions levels which would be expected from BAU 2020 meat consumption intakes vs. emissions which would result with meat substitution included.
Quantifying Health Impacts
Our health analysis replicated the standard methods utilized in Springmann et al.'s (2016c) assessment of mitigation potential and health impacts from emissions pricing (Springmann et al., 2016c). This methodology utilizes a global comparative risk assessment framework (Springmann et al., 2016b). In this analysis, we included only health risk factors directly related to meat, and red meat consumption, in addition to weight-related risks as a result of changes in body mass index (BMI). This utilized population attributable fractions (PAFs) which measure the number of negative health cases which would resultant in any given scenario vs. those in a baseline/BAU condition (Lim et al., 2012). Relative risk factors for coronary heart disease (CHD), stroke and cancer in relation to diet- and weight-related factors were derived from pooled meta-analyses (World Cancer Research Fund, 2007; Prospective Studies Collaboration et al., 2009; Micha et al., 2010; Chen et al., 2013) (see Appendix 1 in Supplementary Material for extended methods). Mortality rates were assumed based on data from the Global Burden of Disease project, which measures the prevalence of deaths by cause across 20 age groups (Lozano et al., 2012). To quantify the overall health implications, these have been reported as number of premature deaths avoided.
Results
Meat Substitution Rates
Our results show a strong sensitivity to both key variables analyzed in this study: the magnitude of price reduction and level of social acceptance of meat substitutes. The change in consumption of the different meat commodities (beef, pigmeat, poultry, and sheep) relative to projected 2020 levels, for each of the scenarios is represented in Figures 1A–E. Note that in scenarios 1–3, where the social acceptability or preference for meat substitutes is equal or less than that of chicken, no substitution effect occurs until prices decrease by more than 10%; this represents the level at which chicken and Quorn™ mycoprotein reach price parity. Under these scenarios, we therefore assume that consumers would still prefer to substitute other forms of meat with chicken rather than mycoprotein. In scenarios 4 and 5, we assume that consumers actually prefer mycoprotein over chicken and would therefore continue to substitute, even if mycoprotein was more expensive.
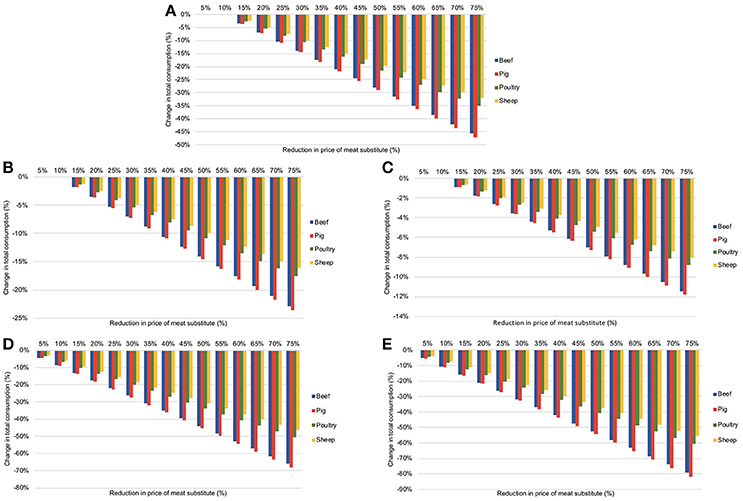
Figure 1. Changes in consumption of meat commodities through substitution with mycoprotein. Percentage changes in consumption of meat commodities as a result of substitution with mycoprotein, or alternative meat substitute products, across a range of price reduction assumptions in (A) scenario 1 (high acceptability); (B) scenario 2 (medium acceptability); (C) scenario 3 (low acceptability); (D) scenario 4 (social preference); and (E) scenario 5 (high social preference).
Under all scenarios we see that the composition of substitution between the various meat commodities remains constant, with the percentage change in pigmeat consistently highest, followed by beef, poultry and sheep meat. The magnitude of this substitution effect, however, shows significant variability. In the case of perfect substitution (scenario 1), our maximum consideration of a 75% decrease in price would result in approximately 40–45% reduction in beef and pigmeat consumption. At a more realistic target of a 50% reduction in price, substitution would result in a 20–30% reduction across all individual meat commodities. Our results show that the social acceptability variable is highly significant: at low levels of social acceptability (scenario 3), even if meat substitute prices were to decline by 75%, substitution would be low at less than 12%. In contrast, if there was strong consumer preference toward meat substitutes, this substitution effect could be as high as 60–80% for all individual commodities.
Greenhouse Gas (GHG) Reductions From Meat Substitution
Table 1 and Figure 2 detail the resultant GHG emissions savings which would occur under each of the scenarios and price decline assumptions. In line with our results of changes in meat consumption, in scenarios 1–3, no additional GHG savings would occur as a result of a price decrease below 10%. Annual GHG reductions show large variability across the five scenarios, ranging from a low of 48 MtCO2e at 75% price reduction in scenario 3 to 583 MtCO2e in scenario 5. For reference, we estimate (based on average GHG intensities, national per capita meat consumption and population figures in 2020) baseline emissions from meat commodities in countries included in this study to be 950 MtCO2e.

Table 1. GHG emissions saving by percentage price reduction in mycoprotein across five social acceptability scenarios.
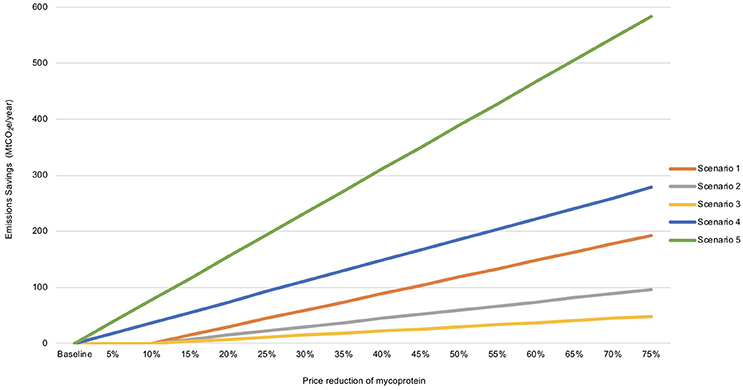
Figure 2. Greenhouse gas emissions savings as a result of meat commodities substitution with mycoprotein. Total greenhouse gas reductions, measured in MtCO2e, across the countries modeled in this study by level of price reduction and social acceptability scenarios.
To illustrate how GHG savings are distributed geographically and across the commodity types, this breakdown has been shown in Figures 3A,B for Scenario 1. This breakdown by region highlights that GHG reductions would be dominated by the USA and EU28 markets—this is promising given that these are the markets in which mycoprotein sales are currently highest. When summarized by meat commodity, we observe that GHG savings are dominated by beef substitution, accounting for approximately the same as pigmeat and poultry combined. Emissions savings potential from sheep substitution is relatively small. Note that GHG emissions resulting from mycoprotein production (in the form of Quorn™) are here shown as negative savings; how these emissions may evolve with time is further discussed in section Discussion.
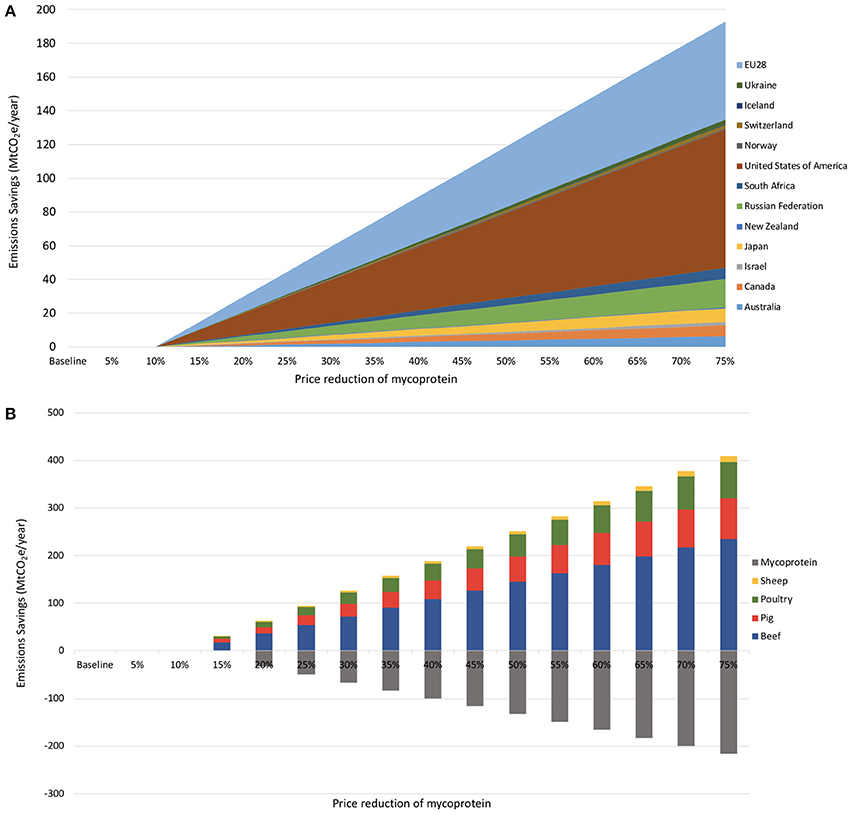
Figure 3. Greenhouse gas emissions savings in scenario 1, broken down by country/region and meat commodity. Annual GHG emissions savings (MtCO2e), represented as a breakdown by (A) country or region; and (B) type of meat commodity substituted. Note that emissions associated with the production of mycoprotein have been shown as negative savings.
Health Impacts of Meat Substitution
Table 2 details the projected health impacts of substitution in terms of number of premature deaths avoided, by scenario and level of price reduction in mycoprotein. In a perfect substitution case (scenario 1), the number of avoided deaths ranges from zero at < 10% price reduction, to approximately 38,300 at 75%. At this upper price reduction limit, the number of deaths avoided ranges from a low of approximately 8,500 in scenario 3, to 52,700 in scenario 5. In all scenarios, diet-related factors (as opposed to weight management) were responsible for >85% of the number of deaths avoided. Figure 4 illustrates the breakdown of number of deaths avoided by region in scenario 1; trends reflect those seen in GHG emissions reductions with strong dominance of health improvements in the USA and EU28 nations.

Table 2. Number of deaths avoided as a result of meat substitution with mycoprotein across the range of price reduction and social acceptability scenarios.
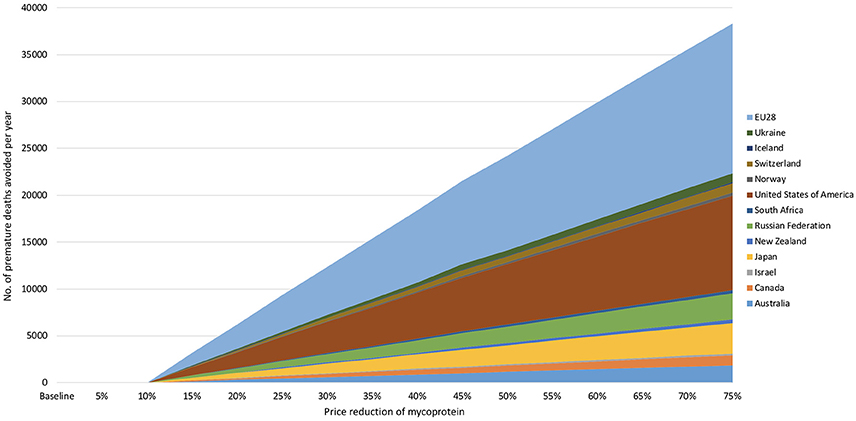
Figure 4. Number of premature deaths avoided in scenario 1, by country/region. Health benefits, quantified as the number of premature deaths avoided per year in scenario 1, as a breakdown by country or region.
Discussion
This study has attempted to provide the first quantification of GHG mitigation and human health benefits of meat substitutes across a range of economic and social acceptability scenarios, and has done so for the year 2020. It is worth noting that, with time, we would expect the potential for net GHG mitigation to increase for a given level of meat substitution based on reduced emission intensity of mycoprotein production. Quorn™ mycoprotein products have a footprint of approximately 5.6 kgCO2ekg−1, which is significantly lower than beef and sheep products but comparable to the global average for poultry of 5.7 kgCO2ekg−1 and only marginally better than pigmeat at 6.1 kgCO2ekg−1. So while the substitution of poultry and pigmeat could be significant, the embedded emissions in mycoprotein production typically offset any expected GHG savings.
Although currently comparable in GHG intensity to poultry, there is potential to substantially reduce the GHG footprint of meat substitutes through further technological innovations and efficiency improvements. Even in the unlikely case that improvements in process design and efficiency were not realized—since a large component of mycoprotein's industrial footprint lies in energy consumption (Smetana et al., 2015), its GHG-intensity should decline by default through progress in energy decarbonization. This is in contrast to livestock production where emissions are dominated by non-CO2 gases (Gerber et al., 2013), and where farming systems have already been heavily intensified in high-income countries (Robinson et al., 2011).
Our results have highlighted the sensitivity of the potential impact of meat substitutes to both economic and social acceptability factors. Our analysis suggests that meat substitutes are unlikely to gain a substantial share of the meat market unless their relative price declines significantly—this is apparent even in scenarios where social acceptability is high. A transformative shift in meat substitute production and its economic structure is therefore likely to be necessary if this group of food products is to make notable contributions to GHG mitigation and human health improvements. Equally crucial is a shift in public perceptions and attitudes toward this group of products; our results show that substitution effects would be marginal, even if relative prices were to decrease by 75%, in scenarios with poor social acceptability. Improving public acceptability of, and preferences for, meat substitute products—whether via sustainability or nutritional justifications—will also be essential. The scale of required shift in dietary habits should not be underestimated; although red meat consumption in particular has declined in numerous high-income countries in recent years, the rate of such change has been slow (Ritchie et al., 2018).
Improved understanding of the role of different marketing and public engagement strategies will be crucial if such shifts in overall eating habits are to be achieved. Assessments of consumer receptivity to meat reduction and substitution campaigns have shown that different segments of society respond positively to different approaches (Verain et al., 2015). Some consumer segments prefer an approach of smaller, more consistent reductions in meat consumption (i.e., reductions in serving size); some for the replacement of more resource-intensive meats, such as beef, for lower-impact poultry meat; whilst others take the approach of meat elimination for a given period of the week (e.g., “meatless days”) (de Boer et al., 2014). The role of meat substitutes may therefore be stronger in consumer segments with an interest in complete elimination of meat for certain meals or periods of time. Improved understanding of such differences will be crucial in determining the role of meat substitute products vs. other sustainable choices such as substitution with lower-impact meats.
The substitution of meat with meat-free substitutes holds established nutritional and health benefits. Such a dietary transition strongly follows nutritional guidance of the World Cancer Research Fund to “eat mostly foods of plant origin” and “limit intake of red meat and avoid processed meat” (World Cancer Research Fund, 2007); the International Agency for Research on Cancer (IARC) (Bouvard et al., 2015); and most national dietary guidelines which recommend to limit red and processed meat intake (American Dietetic Association, 2009; Food and Nutrition Board, 2011; Van Dooren et al., 2014; Fischer and Garnett, 2016; FAO, 2017). Such guidelines are primarily based on the increased risk factor of red, and particularly, processed meat for a range of cancers (World Cancer Research Fund, 2007; Bouvard et al., 2015). Mycoprotein in particular, as a meat substitute holds several additional nutritional benefits. These include a shown reduction in blood cholesterol with frequent consumption; improved satiety relative to chicken prepared within comparable test meals; and reduction in glycaemic response (the rate of change in blood glucose) (Turnbull et al., 1993; Denny et al., 2008; Finnigan et al., 2016).
Meat substitution therefore holds a number of potential health benefits, particularly in higher-income countries (as analyzed in this study) where consumption of meat is typically well above dietary guidelines (Smil, 2002; Westhoek et al., 2014). However, it's important to consider any nutritional deficiencies which could arise with such dietary transition. In Table A2 (Supplementary Material) we provide nutritional composition comparison between mycoprotein and different meats across a number of essential nutrients. On a per unit mass basis, Quorn™ mycoprotein is a low-calorie, low-fat, high-fiber source of protein. Protein quality and completeness or amino acid profile is an important concern, particularly in consideration of lower-income markets where intake of highly digestible and complete proteins are often low (WHO/FAO/UNU Expert Consultation, 2007; Swaminathan et al., 2012). The amino acid, lysine, is most typically the limiting amino acid in plant proteins (WHO/FAO/UNU Expert Consultation, 2007). Mycoprotein is a highly complete protein source with a protein digestibility corrected amino acid score (PDCAAS) score of 0.99 (higher than that of 0.92 for beef) (Edwards and Cummings, 2010), and has a complete amino acid profile—including that of lysine with 8.3 g per 100 g protein (Finnigan et al., 2016). Mycoprotein has a low total and saturated fat content; it also contains no cholesterol (Finnigan et al., 2016). The presence and balance of essential fatty acids are an important nutritional consideration for meat and potential replacement products (Wood et al., 2004). Mycoprotein contains both of the essential polyunsaturated fatty acids (linoleic acid of the n-6 series and α-Linolenic acid of the n-3 series); extended randomized control studies have shown essential fatty acid contents of a meat and mycoprotein-substitution diet to be closely balanced (Denny et al., 2008).
In terms of micronutrient profile, mycoprotein is higher in zinc relative to meat products, lower in iron and vitamin B12 relative to red meats, although comparable to that of poultry (Denny et al., 2008). In diets which are typically low in iron and vitamin B12, substitution must therefore be considered within overall nutritional balance to prevent specific micronutrient deficiency. One key advantage of the industrial production of meat substitutes (over poultry, for example) is the potential to integrate with micronutrient fortification. This holds potential in being able to offset nutritional deficiencies. Furthermore, if such products were to break into developing markets where micronutrient deficiency is widespread, fortification with other micronutrients could provide an important nutritional contribution (Allen et al., 2006; Miller and Welch, 2013).
The high-quality protein profile of mycoprotein, combined with the potential for micronutrient fortification means it holds significant nutritional potential within lower income markets. However, breakthrough and high uptake in such markets will further require significant price reductions. The availability of industrially-produced meat substitutes in developing nations is negligible to non-existent, and therefore excluded from this study. Global increases in meat consumption (and sectoral GHG emissions) are projected to be driven primarily through increased intakes within lower-income and transitioning economies (Vinnari and Tapio, 2009). If substitutes entered these markets below the price of meat commodities, this projected rate of increased meat consumption may be curbed.
Meat substitutes therefore hold significant potential for GHG mitigation and improved nutrition across all income levels. Indeed, this may be an effective mechanism by which SDG2 and SDG6 could be approached simultaneously through to 2030. To do so, significant progress will have to be achieved in technological innovation and efficiency—to realize lower cost production, and in improving consumer acceptability of meat substitute products.
Author Contributions
HR conceptualized the research, developed the methodology and carried out the analysis. All authors contributed to writing the paper.
Funding
This research was funded by the UK Natural Environment Research Council (NERC, grant number: NE/L002558/1) under its E3 Doctoral Training Programme (DTP).
Conflict of Interest Statement
The authors declare that the research was conducted in the absence of any commercial or financial relationships that could be construed as a potential conflict of interest.
Supplementary Material
The Supplementary Material for this article can be found online at: https://www.frontiersin.org/articles/10.3389/fsufs.2018.00016/full#supplementary-material
References
Allen, L., Benoist, B., de Dary, O., and Hurrell, R. (2006). Guidelines on Food Fortification With Micronutrients. World Health Organization.
American Dietetic Association (2009). Position of the American Dietetic Association: Vegetarian Diets. J. Am. Diet. Assoc. 109, 1266–1282. doi: 10.1016/j.jada.2009.05.027
Apostolidis, C., and McLeay, F. (2016). Should we stop meating like this? Reducing meat consumption through substitution. Food Policy 65, 74–89. doi: 10.1016/j.foodpol.2016.11.002
Bouvard, V., Loomis, D., Guyton, K. Z., Grosse, Y., Ghissassi, F. E., Benbrahim-Tallaa, L., et al. (2015). Carcinogenicity of consumption of red and processed meat. Lancet Oncol. 16, 1599–1600. doi: 10.1016/S1470-2045(15)00444-1
Chen, G. C., Lv, D. B., Pang, Z., and Liu, Q. F. (2013). Red and processed meat consumption and risk of stroke: a meta-analysis of prospective cohort studies. Eur. J. Clin. Nutr. 67, 91–95. doi: 10.1038/ejcn.2012.180
Cornelsen, L., Green, R., Turne, R., Dangour, A. D., Shankar, B., Mazzocchi, M., et al. (2014). What happens to patterns of food consumption when food prices change? Evidence from a systematic review and meta-analysis of food price elasticities globally. Health Econ. 24, 1548–1559. doi: 10.1002/hec.3107
Cuevas, S., and Haines, A. (2016). Health benefits of a carbon tax. Lancet 387, 7–9. doi: 10.1016/S0140-6736(15)00994-0
de Boer, J., Schösler, H., and Aiking, H. (2014). “Meatless days” or “less but better”? Exploring strategies to adapt Western meat consumption to health and sustainability challenges. Appetite 76, 120–128. doi: 10.1016/j.appet.2014.02.002
Denny, A., Aisbitt, B., and Lunn, J. (2008). Mycoprotein and health. Nutr. Bull. 33, 298–310. doi: 10.1111/j.1467-3010.2008.00730.x
Edwards, D. G., and Cummings, J. H. (2010). The protein quality of mycoprotein. Proc. Nutr. Soc. 69:69. doi: 10.1017/S0029665110001400
FAO (2001). Food Balance Sheets Handbook. FAOstats. Rome. Available online at: http://faostat3.fao.org/download/FB/*/E
FAO (n.d.). FAOstat Database. Available online at: http://www.fao.org/faostat/en/#data/FBS (accessed January 6, 2017).
Fiala, N. (2008). Meeting the demand: an estimation of potential future greenhouse gas emissions from meat production. Ecol. Econ. 67, 412–419. doi: 10.1016/j.ecolecon.2007.12.021
Finnigan, T. J. (2010). Food 2030 Life Cycle Analysis and The Role of Quon Foods withing the New Fundamentals of Food Policy. Available online at: http://www.mycoprotein.org/assets/timfinniganfood2030.pdf
Finnigan, T., Needham, L., and Abbott, C. (2016). Mycoprotein: A Healthy New Protein With a Low Environmental Impact. Sustainable Protein Sources. London: Elsevier Inc.
Fischer, C. G., and Garnett, T. (2016). Plates, Pyramids, and Planets: Developments in National Healthy and Sustainable Dietary Guidelines: A State of Play Assessment. Food and Agriculture Organization of the United Nations (FAO).
Food and Nutrition Board (2011). Dietary Reference Intakes (DRIs): Recommended Dietary Allowances and Adequate Intakes, Vitamins. Washington, DC: Institute of Medicine, National Academies.
Forouzanfar, M. H., Afshin, A., Alexander, L. T., Anderson, H. R., Bhutta, Z. A., Biryukov, S., et al. (2016). Global, regional, and national comparative risk assessment of 79 behavioural, environmental and occupational, and metabolic risks or clusters of risks, 1990-2015: a systematic analysis for the Global Burden of Disease Study 2015. Lancet 388, 1659–1724. doi: 10.1016/S0140-6736(16)31679-8
Gerber, P., Steinfeld, H., Henderson, B., Mottet, A., Opio, C., Dijkman, J., et al. (2013). Tackling Climate Change through Livestock: A Global Assessment of Emissions and Mitigation Opportunities. Rome: Food and Agriculture Organization of the United Nations (FAO).
Gómez, M. I., and Ricketts, K. D. (2013). Food value chain transformations in developing countries: selected hypotheses on nutritional implications. Food Policy 42, 139–150. doi: 10.1016/j.foodpol.2013.06.010
Gómez-Galera, S., Rojas, E., Sudhakar, D., Zhu, C., Pelacho, A. M., Capell, T., et al. (2010). Critical evaluation of strategies for mineral fortification of staple food crops. Transgenic Res. 19, 165–180. doi: 10.1007/s11248-009-9311-y
Horton, R., Beaglehole, R., Bonita, R., Raeburn, J., McKee, M., and Wall, S. (2014). From public to planetary health: a manifesto. Lancet 383:847. doi: 10.1016/S0140-6736(14)60409-8
IPCC (2014). Summary for policymakers. Climate Change 2014: Mitigation of Climate Change. Contribution of Working Group III to the Fifth Assessment Report of the Intergovernmental Panel on Climate Change. Geneva: IPCC.
Joshi, V. K., and Kumar, S. (2016). Meat Analogues: plant based alternatives to meat products- A review. Int. J. Food Ferment. Technol. 5, 107–119. doi: 10.5958/2277-9396.2016.00001.5
Lim, S. S., Vos, T., Flaxman, A. D., Danaei, G., Shibuya, K., Adair-Rohani, H., et al. (2012). A comparative risk assessment of burden of disease and injury attributable to 67 risk factors and risk factor clusters in 21 regions, 1990-2010: A systematic analysis for the Global Burden of Disease Study 2010. Lancet 380, 2224–2260. doi: 10.1016/S0140-6736(12)61766-8
Lozano, R., Naghavi, M., Foreman, K., Lim, S., Shibuya, K., Aboyans, V., et al. (2012). Global and regional mortality from 235 causes of death for 20 age groups in 1990 and 2010: a systematic analysis for the Global Burden of Disease Study 2010. Lancet 380, 2095–2128. doi: 10.1016/S0140-6736(12)61728-0
Lusk, J. L., and Tonsor, G. T. (2016). How meat demand elasticities vary with price, income, and product category. Appl. Econ. Perspect. Policy 38, 1–39. doi: 10.1093/aepp/ppv050
Micha, R., Wallace, S. K., and Mozaffarian, D. (2010). Red and processed meat consumption and risk of incident coronary heart disease, stroke, and diabetes mellitus a systematic review and meta-analysis. Circulation 121, 2271–2283. doi: 10.1161/CIRCULATIONAHA.109.924977
Miller, D. D., and Welch, R. M. (2013). Food system strategies for preventing micronutrient malnutrition. Food Policy 42, 115–128. doi: 10.1016/j.foodpol.2013.06.008
OECD (2016). OECD-FAO Agricultural Outlook 2016-2025. Available online at: http://www.oecd-ilibrary.org/agriculture-and-food/data/oecd-agriculture-statistics_agr-data-en (accessed January 23, 2017).
Prospective Studies Collaboration, Whitlock, G., Lewington, S., Sherliker, P., Clarke, R., Emberson, J., et al. (2009). Body-mass index and cause-specific mortality in 900 000 adults: collaborative analyses of 57 prospective studies. Lancet 373, 1083–1096. doi: 10.1016/S0140-6736(09)60318-4
Ritchie, H., Laird, J., and Ritchie, D. (2017). 3f bio: halving the cost of mycoprotein through integrated fermentation processes. Indust. Biotechnol. 13, 29–31. doi: 10.1089/ind.2017.29065.hri
Ritchie, H., Reay, D. S., and Higgins, P. (2018). The impact of global dietary guidelines on climate change. Glob. Environ. Change 49, 46–55. doi: 10.1016/j.gloenvcha.2018.02.005
Rivera, J. A., Hotz, C., Gonzalez-Cossio, T., Neufeld, L., and Garcia-Guerra, A. (2003). Animal source foods to improve micronutrient nutrition and human function in developing countries: the impact of dietary intervention on the cognitive development of kenyan school children. J. Nutr. 133, 3965S−3971S.
Robinson, T. P., Thornton, P. K., Franceschini, G., Kruska, R. L., Chiozza, F., Notenbaert, A., et al. (2011). Global Livestock Production Systems. Rome: FAO and ILRI.
Smetana, S., Mathys, A., Knoch, A., and Heinz, V. (2015). Meat alternatives – life cycle assessment of most known meat substitutes. Int. J. Life Cycle Assessm. 2050, 1254–1267. doi: 10.1007/s11367-015-0931-6
Smil, V. (2002). Worldwide transformation of diets, burdens of meat production and opportunities for novel food proteins. Enzyme Microb. Technol. 30, 305–311. doi: 10.1016/S0141-0229(01)00504-X
Springmann, M., Godfray, H. C., Rayner, M., and Scarborough, P. (2016a). Analysis and valuation of the health and climate change cobenefits of dietary change. Proc. Natl. Acad. Sci. U.S.A. 113, 4146–4151. doi: 10.1073/pnas.1523119113
Springmann, M., Mason-D'Croz, D., Robinson, S., Garnett, T., Godfray, H. C. J., Gollin, D., et al. (2016b). Global and regional health impacts of future food production under climate change: a modelling study. Lancet 6736, 491–504. doi: 10.1016/S0140-6736(15)01156-3
Springmann, M., Mason-D'Croz, D., Robinson, S., Wiebe, K., Godfray, H. C. J., Rayner, M., et al. (2016c). Mitigation potential and global health impacts from emissions pricing of food commodities. Nat. Climate Change 7, 1–54. doi: 10.1038/nclimate3155
Swaminathan, S., Vaz, M., and Kurpad, A. V. (2012). Protein intakes in India. Brit. J. Nutr. 108, S50–S58. doi: 10.1017/S0007114512002413
Tilman, D., and Clark, M. (2014). Global diets link environmental sustainability and human health. Nature 515, 518–522. doi: 10.1038/nature13959
Turnbull, W. H., Walton, J., and Leeds, A. R. (1993). Acute effects of mycoprotein on subsequent energy intake and appetite variables. Amer. J. Clin. Nutr. 58, 507–512. doi: 10.1093/ajcn/58.4.507
UNFCCC (2015). Adoption of the Paris Agreement. Available online at: http://unfccc.int/resource/docs/2015/cop21/eng/l09r01.pdf
United Nations (2015). UN Population Prospects. Available online at: http://esa.un.org/unpd/wpp/ (accessed February 6, 2016).
United Nations (2017). Global Indicator Framework for the Sustainable Development Goals and Targets of the 2030 Agenda for Sustainable Development. Work of the Statistical Commission pertaining to the 2030 Agenda for Sustainable Development (A/RES/71/313).
Van Dooren, C., Marinussen, M., Blonk, H., Aiking, H., and Vellinga, P. (2014). Exploring dietary guidelines based on ecological and nutritional values: a comparison of six dietary patterns. Food Policy 44, 36–46. doi: 10.1016/j.foodpol.2013.11.002
Verain, M. C. D., Dagevos, H., and Antonides, G. (2015). Sustainable food consumption. Product choice or curtailment? Appetite 91, 375–384. doi: 10.1016/j.appet.2015.04.055
Vinnari, M., and Tapio, P. (2009). Future images of meat consumption in 2030. Futures 41, 269–278. doi: 10.1016/j.futures.2008.11.014
Walker, P., Rhubart-Berg, P., McKenzie, S., Kelling, K., and Lawrence, R. S. (2005). Public health implications of meat production and consumption. Public Health Nutr. 8, 348–356. doi: 10.1079/PHN2005727
Wellesley, L., Happer, C., and Froggatt, A. (2015). Changing Climate, Changing Diets Pathways to Lower Meat Consumption. Chatham House Report, 64. Available online at: http://www.itv.com/news/2015-11-24/taxing-the-sale-of-meat-would-be-less-unpopular-than-many-governments-believe-report-says/
Westhoek, H., Peter, J., Rood, T., Wagner, S., Marco, A., De Murphy-bokern, D., et al. (2014). Food choices, health and environment: effects of cutting Europe's meat and dairy intake. Glob. Environ. Change 26, 196–205. doi: 10.1016/j.gloenvcha.2014.02.004
Whitmee, S., Haines, A., Beyrer, C., Boltz, F., Capon, A. G., de Souza Dias, B. F., et al. (2015). Safeguarding human health in the Anthropocene epoch: report of the Rockefeller Foundation-Lancet Commission on planetary health. Lancet 386, 1973–2028. doi: 10.1016/S0140-6736(15)60901-1
WHO (2013). Global Action Plan for the Prevention and Control of Noncommunicable Diseases 2013-2020. Geneva: World Health Organization.
WHO/FAO/UNU Expert Consultation (2007). Protein and Amino Acid Requirements in Human Nutrition. Geneva: World Health Organization Technical Report Series.
Wood, J. D., Richardson, R. I., Nute, G. R., Fisher, A. V., Campo, M. M., Kasapidou, E., et al. (2004). Effects of fatty acids on meat quality: a review. Meat Sci. 66, 21–32. doi: 10.1016/S0309-1740(03)00022-6
World Cancer Research Fund (2007). Food, Nutrition, Physical Activity, and the Prevention of Cancer: A Global Perspective. Washington, DC: Cancer Research.
World Watch Institute (2009). Livestock and Climate Change. What if the Key Actors in Climate Change Are Cows, Pigs and Chickens? Available online at: http://www.worldwatch.org/node/6294
Keywords: dietary change, greenhouse gas emissions, health, meat substitutes, sustainable protein, Flexitarian
Citation: Ritchie H, Reay DS and Higgins P (2018) Potential of Meat Substitutes for Climate Change Mitigation and Improved Human Health in High-Income Markets. Front. Sustain. Food Syst. 2:16. doi: 10.3389/fsufs.2018.00016
Received: 30 January 2018; Accepted: 23 April 2018;
Published: 15 May 2018.
Edited by:
Mauro Serafini, Università di Teramo, ItalyReviewed by:
Penelope Anne Field, University of Otago, New ZealandArturo Anadón, Complutense University of Madrid, Spain
Copyright © 2018 Ritchie, Reay and Higgins. This is an open-access article distributed under the terms of the Creative Commons Attribution License (CC BY). The use, distribution or reproduction in other forums is permitted, provided the original author(s) and the copyright owner are credited and that the original publication in this journal is cited, in accordance with accepted academic practice. No use, distribution or reproduction is permitted which does not comply with these terms.
*Correspondence: Hannah Ritchie, aGFubmFoLnJpdGNoaWVAZWQuYWMudWs=