- Food Microbiology Laboratory, Department of Food Technology, University of North Bengal, Siliguri, India
Fermented foods are functional foods with better nutritional and technological characteristics that prove numerous health benefits to the host as they harbor diverse group of microorganisms in them. There has been increased consumption of fermented vegetables, cereal based foods, dairy products, meat and fish products and rice based foods and beverages throughout the globe. Diseases like obesity, cardiovascular diseases and diabetes are the chief metabolic disorders that have become a leading concern of public health in the world. According to the World Health Organization statistics, approximately 422 million people, around the globe, suffer from diabetes, and about 2.6 million deaths, worldwide, have been caused due to raised cholesterol levels in humans. Although there is no doubt that low-fat and low-carbohydrate diets are an effective means of lowering blood cholesterol and managing blood sugar levels under experimental conditions, however, they appear to be less effective, owing to poor compliance, which can be linked to the diets’ low palatability and acceptability by consumers. Therefore, there is a need for novel strategies to mitigate the effects of raised blood glucose and blood cholesterol levels, also keeping in mind the consumer acceptability. Probiotic lactic acid bacterial supplements have gathered much attention in the prevention of diet induced metabolic diseases. Lactobacillus is the largest genus falling under the group of Lactic acid bacteria which includes approximately 300 species of bacteria and innumerable strains. Further, they have a long history of use in food fermentation and has also procured the “generally recognized as safe” status. This genus is accredited as probiotics due to their countless health-promoting effects on the host. They have been suggested to facilitate positive contribution to the nutritional, physiological, microbiological and immunological effect on the host. This paper focuses on the in vitro and in vivo studies of Lactobacillus probiotics reported from fermented vegetable and milk products in the treatment of obesity, diabetes and cardiovascular diseases.
Introduction
In recent times, people’s inclination towards unhealthy lifestyle, intake of calorie-rich diet, low intake of fruits and vegetables have led to a drastic increase in obesity-related metabolic disorders such as hypertension, fatty liver disease, type 2 diabetes, and hyperlipidemia (Gomes et al., 2014; Albano et al., 2018; Kim et al., 2019; Chen et al., 2020). It is noteworthy that diabetes and raised total cholesterol is a prominent cause of disease prevalent among the people throughout the globe (Townsend et al., 2016; Ghatani et al., 2022). The World Health Organization (WHO) delineates IschemicHeart Disease (IHD), stroke and Diabetes among the top 10 global cause of death in 2019 (WHO, 2022a). The major contributory factor for the global prevalence of cardiovascular disease (CVD) is hyperlipidemia (Guo et al., 2016). The danger of heart attack in people with hyperlipidemia is three times higher than in those with normal blood lipid profiles (Albano et al., 2018). As per the prediction of World Health Organization (WHO), cardiovascular disease shall become a prime reason for death by the year 2030 (Tang et al., 2022). Also, the WHO reports from the year 1980–2014 suggests that the prevalence of diabetes have risen from 108 to 422 million (WHO, 2022b). A 5% upsurge in premature death due to diabetes between the years 2000–2016 has also been recorded (WHO, 2022a).
Hyperlipidemia denotes a lipid metabolic disorder in which the blood contains abnormally elevated levels of triglycerides (TG), total cholesterol (TC), low-density lipoprotein cholesterol (LDL-C), very low-density lipoprotein cholesterol (VLDL-C); and declined levels of high-density lipoprotein cholesterol (HDL-C) (Chen et al., 2014). According to “The third Report of the Expert Panel on Detection, Evaluation, and Treatment of High Blood Cholesterol in Adults”, optimum levels of LDL-C, total cholesterol, triglycerides are <100 mg/dl, <200 mg/dl and <150 mg/dl, respectively, while the favorable level of HDL-C is not below 40 mg/dl (Ali et al., 2022). The concentration of serum LDL has determined to bring forth utmost adverse cardio-metabolic effects, chiefly through the elevation of atherosclerosis (Skrypnik et al., 2018). Similarly, an elevated level of serum triglycerides (TG) is also related to cardiovascular risks. 1 mmol/L rise in serum TG concentration is accountable for 32% increased cardiovascular risks in males and 76% increased cardiovascular risks in females (Skrypnik et al., 2018). Reports have also suggested the relation of Hyperlipidemia to the activation of inflammation by the increased production of infammatory cytokines (e.g., tumor necrosis factor alpha and interleukin 6). Cytokines can in-turn play vital roles in the pathophysiology of atherosclerosis (Reamtong et al., 2021).
Diabetes, often known as diabetes mellitus, is characterized by persistently high blood glucose levels inside the body. Diabetes has caused 6.7 million of deaths in 2021, despite of the pandemic COVID-19. According to projections, 783 million adults will have diabetes by 2045, accounting for about 12% of the world’s adult population (Algonaiman et al., 2022). It is classified into two categories: type 1 diabetes, in which the pancreas fails to produce the hormone insulin. Insulin resistance is thought to be the cause of Type 2 Diabetes, in which cells fail to respond properly to insulin. Type 2 diabetes affects more than 90% of people and is considered among the most common diseases. Another kind of diabetes is gestational diabetes mellitus (GDM), which occurs after or during pregnancy and is marked by the emergence of glucose intolerance (Malchoff, 1991; Cabello-Olmo et al., 2019). It can also be caused by a combination of poor insulin production and sensitivity, as well as hormonal imbalance during pregnancy (Gardner and Shoback, 2017). Thus, it is a complex disease that accounts for roughly 3.5 percent of non-communicable disease mortality (Gomes et al., 2014). Numerous metagenomic studies have shown that dysregulation in the gut microbiome (GM) has a direct influence on the development of T2DM by affecting gut permeability, inflammation, the immune system, and energy metabolism. As a result, modulation of the GM by bioactive substances has recently gained popularity in recent years in the search for suitable bioactive components or formulations with preventative or therapeutic potential for T2DM (Sharma et al., 2022). T2DM patients are at a much greater risk of life-threatening complications including cardiovascular disease, eye problems, and kidney damage (Wang et al., 2022). In fact, patients with hepatocellular disease are more susceptible to developing diabetes (Wang et al., 2022). Despite this there is no satisfactory cure has been discovered. But only option is to keep blood sugar levels as normal as possible, which requires the use of medicines. Nevertheless, lifestyle changes including a balanced diet, regular exercise and trying to maintain a normal body weight have now been reported as first-line monitoring for type 2 diabetes (Shen et al., 2016; Algonaiman et al., 2022).
Although various cholesterol-lowering drugs like statins and sugar-lowering drugs like sulfonylureas, have emerged, yet the long-term intake of drugs is not considered optimal because of the expense and the severe side effects associated with the drug therapy (Krentz and Bailey, 2005; Guo et al., 2016; Lu et al., 2017; Cabello-Olmo et al., 2019). Therefore, numerous non-pharmacological outlook towards lowering cholesterol and sugar levels have been mapped out and researched, dietary approaches like the use of probiotics being one among them (Guo et al., 2016). Various clinical research suggested that the consumption of fermented food containing probiotics have a significant correlation to bettering human health (Mallappa et al., 2022). It was observed that long-term yoghurt ingestion has been linked to a lower risk of cardiovascular disease (CVD), type 2 diabetes (T2D), and mortality risk (Marco et al., 2017; Baruah et al., 2022). It was also observed that Kimchi, a traditional Korean fermented vegetable food, has been linked to anti-obesity and anti-diabetic activity (An et al., 2013; Baruah et al., 2022). This review focuses on the in vitro and in vivo studies of Lactobacillus isolated from fermented vegetable and milk products in the treatment of hyperlipidemia and hyperglycemia.
Methodology
The articles included in the review were referred from Google scholar, PubMed and World Health Organization database. The period of the search was from the year 1991–2022.
Probiotics
Probiotics are the living microorganisms, whose intake (in adequate amount) has been reported to have numerous beneficial effects on the host in a variety of diseases, including oral diseases, gastrointestinal diseases, allergic diseases, prevention of cancer and autoimmune diseases, etc. (Aponte et al., 2020; Liang et al., 2021). They take part in promoting the host defense mechanisms and also help in stabilizing the gut microbiota (Hamouda et al., 2022). Furthermore, probiotics also have a high potential for survival in the intestine (acidic pH, enzymes, biliary salts, etc.) (Plaza-Diaz et al., 2019). Probiotics are regarded as the panacea of the 21st century due to their therapeutic potential in curing various forms of illnesses (Aponte et al., 2020). In comparison to the traditional therapies, the bio-therapeutic role of probiotics has escalated their importance in food and medicine due to several competitive superiority over the traditional ones (Aponte et al., 2020). It is well known that diet has a direct influence on the composition of the gut microbiome, which in consequence affects one’s well-being. It is believed that the intake of probiotic containing food may positively affect one’s health (Raghuvanshi et al., 2019).
Numerous research shows a strong link between probiotic supplementation and chronic metabolic illnesses such as hyperglycemia, hyperlipidemia, and hypertension (Liang et al., 2021). Reports have suggested that there is a good association between probiotic consumption and metabolic profile in diabetic individuals (Everard et al., 2013). Also, the hypolipidemic activity of the probiotic lactic acid bacterial members is one of their most promising characteristics (Albano et al., 2018). The mechanisms involved in mitigating the effects of hyperglycemia and hyperlipidemia has been outlined in Figures 1, 2, respectively. Among the most commonly used probiotics, Lactobacillus spp. Are popular helpingin mitigating hyperlipidemia (Chen et al., 2020). Various strains of Lactobacillus present in the traditional fermented foods indicate remarkable protective outcomes on hyperlipidemia (Chen et al., 2020). Several clinical and pre-clinical trials have underlined the importance of probiotic Lactobacillus spp. In the prevention and treatment of hyperlipidemia and hyperglycemia. Furthermore, Lactobacillus has been considered as GRAS with approved implication of safeness (Liu et al., 2018).
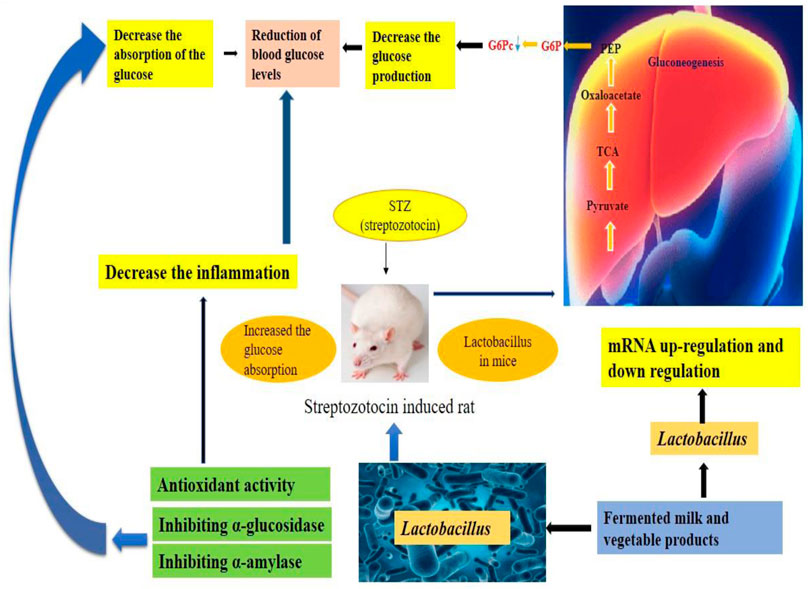
FIGURE 2. Schematic representation of the mechanism involved in mitigating hypoglycemia by probiotics.
In recent years, the intake of fermented foods containing probiotics has unfolded as a prominent dietary strategy for boosting the human health. Generally, LAB from numerous genera Lactobacillus, Streptococcus, and Leuconostoc, are the most common reported bacteria found in fermented foods (Rezac et al., 2018).
Fermented foods
Currently, the interest in fermented foods has re-newed due to its associated health benefits and its role as a carrier of probiotics (Lavefve et al., 2019). Fermented foods are defined as foods or beverages developed by the process of fermentation (Dimidi et al., 2019). Fermentation is a process by which the microorganisms bring about the preservation and enzymatic transformation of raw material into an organoleptically and nutritionally improved food (Sharma and Sarkar, 2015). A myriad of fermented food exists, depending on the variety of raw materials used, the fermentation procedures employed, the variety of parameters involved in fermentation and the microorganisms involved in fermentation (Anal, 2019). Numerous raw materials have undergone fermentation, which includes fish and meat, dairy products and plant-based foods (vegetables, cereals, fruits, soybeans and other legumes) (Dimidi et al., 2019). Currently, about 5,000 varieties of fermented foods and beverages are consumed worldwide (Marco et al., 2021). Also, much research has been carried out in the dairy-based fermented food, while, the fermented plant-based foods are underexplored, except for some like kimchi, sauerkraut, etc (Wuyts et al., 2020).
Fermented foods are a promising source of the underexplored microorganisms (Wuyts et al., 2020). Generally, most fermented foods contain a minimum of 106 microbial cells per Gram, however, the microbial concentration vary depending on several variables like the product’s origin, age and the time of consumption (Dimidi et al., 2019). Lactic acid bacteria and yeasts are the natural microbial population of vegetable fermentations (Medina-Pradas et al., 2017). Numerous Gram-positive and Gram-negative bacterial community, yeasts and molds compete for dominance during the vegetable-fermentation process but, usually the Lactic acid bacterial members predominate due to their potential of producing lactic acid, which lowers the pH and thereby inhibits the survival of other competing groups. Few naturally present species of LAB involved in different vegetable fermentations are; Leuconostoc mesenteroides, Pediococcus pentosaceus, Pediococcus acidilactici, Lactobacillus brevis, Lactobacillus plantarum and Lactobacillus pentosus (Medina-Pradas et al., 2017).
Fermented vegetables
Fermented vegetables include fruits (edible part of a tree/plant) and vegetables (root/plant cultivated for food) (Medina-Pradas et al., 2017). While fruits are regarded as acidic foods, vegetables fall in the category of low acidic food. Nevertheless, the microorganisms involved in vegetable fermentation contribute to the acidity of the fermented product, irrespective of the raw material (Medina-Pradas et al., 2017). Fresh vegetables and fruits are liable to be spoilt by microorganisms and in few cases by pathogenic microorganisms contracted during their cultivation and harvest, thereby decreasing their shelf-life (Di Cagno et al., 2013; Di Cagno et al., 2015). Although various techniques like pasteurization, cooking, etc. may guarantee their safety yet, their long-term storage and escape from the unwanted change in the physical and chemical composition depends on the fermentation process (Di Cagno et al., 2013; Di Cagno et al., 2015). Lactic acid fermentation is one among the fermentation techniques to ensure safe fermented vegetables and fruits with extended shelf-life (Di Cagno et al., 2013; Di Cagno et al., 2015). Lactic acid-fermented vegetables like Sauerkraut, Kimchi and fermented cucumbers are the most studied owing to their commercial significance (Di Cagno et al., 2013; Di Cagno et al., 2015).
Lactobacillus spp. have multiple contributions in the fermentation process (Peng et al., 2018). They improve the nutritional aspect of the fermented vegetable by bringing about reduction in the nitrite and other harmful substance built-up and also inhibit the growth of harmful microorganisms (Peng et al., 2018). In addition to this, Lactobacillus contributes to the palatability of the fermented food (Peng et al., 2018). They are crucial microorganisms having major physiological functions in animals and human beings (Peng et al., 2018). Lactobacillus is a highly salt tolerant genera (Peng et al., 2018). It can ferment the sugars present in the vegetables to produce acid thereby creating an acidic environment that inhibits the growth of other spoilage bacteria (Peng et al., 2018). Besides this, the metabolic by-products of Lactobacillus such as hydrogen peroxide, diacetyl, nisin, carbon dioxide, bacteriocin, etc. also add to their anti-microbial property (Peng et al., 2018). Further, the vegetables also contain particular chemicals like isothiocyanates and allyldisulphide that selectively favor Lactobacillus over the other microorganisms (Peng et al., 2018). Even though Lactobacillus spp. are found in lesser number in the plant’s native microbiotic community, yet they predominate the vegetable fermentation process (Peng et al., 2018). Also, they represent the microbes having health-promoting effects (Peng et al., 2018). Apart from being a source of health-boosting compounds, plants also contain numerous anti-nutritional factors (Peng et al., 2018). However, the combined effects of plant enzymes and the metabolic activity of Lactobacillus increase the bioavailability and activity of phytochemicals, which in-turn results in a significant increase in the number of functional microbial metabolites, exerting beneficial health-promoting effects (Peng et al., 2018).
Currently, vegetable-based fermented foods gain much focus not only because of its health benefits, industrial applications, prebiotic property and as a source of probiotics/synbiotics, but also due to its use as a non-dairy alternative (Table 1) (Medina-Pradas et al., 2017; Wuyts et al., 2020). Unlike the dairy-based food products, vegetable-based fermented foods are favorable for the lactose-intolerant people, vegans, and people allergic to milk (Wuyts et al., 2020). Vegetable-based fermented foods may also find application in various industries. The food industry can thereby exploit these microbial strains to be used as starter cultures and their productions as enzymes, food additives and antibiotics, etc. For instance, the exopolysaccharides secreted may be used to enhance the texture of the food produced in the food industries. The pharmaceutical industry on the other hand can use these exopolysaccharides in reducing the viscosity of the blood (Wuyts et al., 2020). Thus, plant-based fermented foods contributes significantly to the human well-being by providing nourishment, vitamins, minerals, nutrients, etc. (Sivamaruthi et al., 2018). In anticipation, the future of fermented vegetable products are promising.
Fermented dairy products
Fermentation is a traditional method of food preservation that enhances the organoleptic, nutritional, and health-promoting qualities of foods while also improving their safety (Macori and Cotter, 2019). Fermented dairy products are made by fermenting milk with different kinds of microorganisms which generally are regarded as safe (GRAS). During the fermentation process beneficial substances as well as bacterium-derived metabolites are produced. Fermented dairy products are an excellent matrix for the incorporation of additives and/or nutrients, which addsnutrition to the final product, thereby making them actual functional foods that are intriguing in a balanced diet (García-Burgos et al., 2020). Fermented dairy products are gaining popularity among consumers due to their associated health benefits and nutritional benefits (Table 2). The impact of fermented dairy products on the intestinal microbiota is related to the improvement of gut system leading to a healthy lifestyle and longer life expectancy (Chen et al., 2019). It was observed that Lactobacillus brevis and Lactobacillus pentosusisolated from the Iranian butter reduce the cholesterol (Ostadzadeh et al., 2022). Lactobacillus paracasei and Lactobacillus plantarum isolated from the traditional fermented dairy product of Tibet can prevent and alleviate T2D (Zhao et al., 2022). Specific lactic acid bacterial strains may be able to remove toxic or anti-nutritional components from fermented milks, and also alleviate the symptoms of lactose intolerance and galactose buildup (Shiby and Mishra, 2013). Many fermented dairy products, including yoghurt, kumys, skyr, sweetened probiotic milk, and kefir, which is the best studied fermented dairy product, include probiotics and prebiotics (Bourrie et al., 2016). Lactobacillus, Lactococcus, Leuconostoc, Pediococcus, Bacillus, Propionibacterium, and Bifidobacterium are among the bacteria found in fermented dairy products (Ghosh et al., 2019).
Lactobacillus
The genus Lactobacillus is a large heterogeneous group of Gram-positive, nonsporulating, facultative anaerobic bacteria which includes, Lactobacillus plantarum, Lactobacillus fermentum, Lactobacillus paracasei, L. acidophilus, L. rhamnosus, L. bulgaricus, L. casei, and L. reuteri. This genus plays a vital role in food fermentation and can also be found in the gastrointestinal (GI) system of humans and animals in variable amounts (Mu et al., 2018). The genuswas proposed by Beijerinck in 1901 and the genus is classified in the phylum Firmicutes, class Bacilli, order Lactobacillales, family Lactobacillaceae (Schleifer, 2015). Lactobacilli were first classified based on phenotypic characteristics such as ideal growth temperature, sugar utilization, and the range of metabolites generated (Zheng et al., 2020). In the 20th century, genotypic and chemotaxonomic criteria such as DNA-DNA hybridization, GC content, and peptidoglycan chemical structure were studied to identify novel bacterial taxa. Since 1983, similarity of 16S rRNA genes have been utilized in bacterial taxonomy for classification and nomenclature (Zheng et al., 2020).
In the LPSN database (The List of Prokaryotic Names with Standing in Nomenclature, http://www.bacterio.net), the genus Lactobacillus currently has around 200 species. Lactobacilli can be found in a range of places, including milk and plant surfaces, as well as human and animal gastrointestinal tracts. Lactobacilli have been consumed safely by humans for centuries in fermented foods and beverages, as well as more recently in probiotic products (Zielińska, et al., 2019).
One of most effective research fields of lactic acid bacteriaincludesLactobacillus, which possess antibacterial activity, anti-inflammatory, ACE-inhibitory, antioxidant, antidiarrheal, antiviral, immune-modulatory, hypercholesterolemic, anti-diabetic, and anti-cancer activities (Minj et al., 2021). Lactobacillus strains are commonly ingested as probiotics. One of the most promising areas of current research and applications is the use of Lactobacillus spp. For the production of functional meals. Individual bacterial species have distinct biological activities that differ from strain to strain and could be used in the development of alternative and therapeutic food products (Minj et al., 2021).
Habitats of Lactobacillus
Lactobacilli occupy nutrient-rich habitats such as fermented foods and animal feed, the environment, which includes the surface of plants, soil, and the bodies of invertebrate and vertebrate animals, and the environment (Duar et al., 2017) (Figure 3).
In-vitro and in-vivo studies of the effects of lactobacillus species on hypolipidemia and hypoglycemia
In-vivo studies including animal and human models have been in trend in recent times. Numerous studies on animal models (rats, hamsters, guinea pigs, mice and pigs) have been conducted owing to their similarities with humans in terms of lipid and bile metabolism, regulation of hepatic cholesterol enzymes and plasma lipoprotein distribution. Further, the model animals used possess almost similar nutrient requirements, digestive anatomy and physiology and metabolic processes with humans, making them beneficial experimental representation for research purposes (Table 3). However, the in-vitro experiments has its own benefit as it provides a clue to whether the proposed in-vivo experiment may lead a fruitful result or not (Ooi and Liong, 2010).
The effects of lactobacillus species on hypolipidemia
Gao et al. (2011) evaluated the antioxidant and hypolipidemic activity of LAB isolated from pickled Chinese cabbage. Out of the 28 LAB strains isolated, two strains namely, L. plantarum and L. brevis, having high tolerance to acid and bile salt were further screened for the antioxidant and hypolipidemic activity (Gao et al., 2011). Numerous studies have shown a link between an increase in free radicals and lipid peroxidation, LDL-C and blood glucose (Tanaka et al., 2002). Free radicals can trigger a cascade of detrimental metabolic reactions, resulting in a variety of illnesses such as cancer, diabetes, neurological disease, and cardiovascular disease. Also, Intracellular diffusion of free radicals is possible. This diffusion of free radicals leads to mitochondrial enzyme damage and DNA breaks, impairment of cellular function (Bonnefont-Rousselot et al., 2000). SOD and glutathione peroxidase (GSH-px) being an anti-oxidant (scavenger of free radicals) has the potency to control oxidation reactions in the body. In this study, when the SOD and glutathione peroxidase (GSH-px) concentration in the tissues of kidney and liver of the experimental rats were studied, Gao et al. (2011) observed the increase in SOD and GSH-px in L. plantarum and L. brevis fed animal groups when compared with the control groups. Thus, the results indicated that both the strains could become potent agents for anti-oxidation and hypocholesterolemia (Gao et al., 2011).
In an invivo evaluation conducted by Costabile et al. (2017) the cholesterol-lowering ability of Lactobacillusplantarum ECGC 13110402, a dairy isolate possessing a high bile salt hydrolase activity was assessed in normal to mildly hpercholesterolaemic individuals. Males and females of age 18–50 years; BMI 18.5–29.9 kg/m2; TC ranging from 200 to 300 mg/dl were selected and randomly grouped into active group and placebo control group. The active group consumed 2 × 109 CFU of encapsulated Lactobacillusplantarum ECGC 13110402 twice daily. This daily intake of the probiotic bacteria led to a statistically significant lowering of the LDL-C (13.9%, p = 0.030) in the volunteers during a period of 0–12 weeks when compared to the placebo group. Also, the TC levels in the volunteers fed with the probiotic also decreased to a significant value (36.7%, p = 0.045). In contrast, an increase in HDL-C (14.7%, p = 0.007) was observed in a period of 6–12 weeks. Thus, this study suggests that Lactobacillusplantarum ECGC 13110402 can be opted as a suitable candidate to be used in mitigating hypercholesterolaemia (Costabile et al., 2017).
Probiotic characteristics of LAB isolated from fermented yak milk products, which includes Chhurpi, Shyow and Thara/Khachu that are indigenous to the Dukpa and the Bhutia community of the Sikkim Himalayan regions were assessed by Ghatani and Tamang (2017). Out of the 170 LAB isolates screened for their probiotic properties, 70 isolates were found to lower cholesterol levels in-vitro. Moreover, six isolates were identified as the best strains for possessing cholesterol-reducing potential. Their cholesterol-reducing potential in terms of percentage were as follows; L. plantarum YD5S (85%) and YD9S (70%), L. pentosus YD8S (70%), L. paraplantarum YD11S (55%), Enterococcuslactis YHC20 (65%) and Enterococcusfaecium YY1 (65%) showed the best cholesterol-reducing potential. This finding highlights the cholesterol-reducing ability of the Lactic acid bacteria, especially the Lactobacillus species (Ghatani and Tamang, 2017).
In an in-vitro study conducted by Albano et al. (2018) on the activity of Lactic Acid bacterial members isolated from traditional Italian cheeses resulted a significant lowering of the cholesterol in broth. L. casei VC199, L. paracasei SE160 and VC213, L. plantarum VS.166 and VS.513, Enterococcus faecium VC223, and E. lactis BT161 resulted in a 42%–55% reduction of the cholesterol level in broth. When these strains were subjected to cheese manufacturing process, all the strains showed a decreased cholesterol content in the cheeses. However, Lb. Paracasei VC213 and E. lactis BT161 showed the best result i.e., a cholesterol reduction percent of upto 23%. Further, the sensory properties of the cheese were unaffected by these cultures (Albano et al., 2018).
Wa et al. (2019) evaluated the mechanism by which the single probiotic- and combined probiotic-fermented milk plays role in lipid metabolism. Numerous studies have revealed that farnesoid X receptor (FXR), 5′-AMP-activated protein kinase (AMPK) and liver X receptors (LXRs) play vital role in the metabolism of lipids thus, this study investigated the gene transcriptions modulated by the FXR, AMPK and LXRs. In comparison to Sprague Dawley rats fed with high fat diet, the group of High-fat rats supplemented with a single probiotic (L. rhamnosus LV108) fermented milk and those fed with combined probiotics (L. rhamnosus LV108, L. casei grx12 and L. fermentum grx08) fermented milk showed significant reduction in the levels of serum TC and TG. Also, an increase in bile acids in the liver and the small intestine of the probiotic fed high-fat rats were observed. Further, the quantitative PCR (qPCR) investigation revealed the up-regulation of transcription of LXR axis genes in the liver and small intestines of high-fat rats fed with probiotic-fermented milk, thereby suggesting the pathway of cholesterol balance. Although, the comparison of the upregulation of FXR gene transcription of probiotic fed high-fat rats were not significantly compared with the high-fat control rats but, a significant low upregulation of FXR genes in high-fat rats fed with mixed-probiotics fermented milk was observed. This observation suggested that the probiotics may not lower the TC via the FXR axis. Also, no significant differences in the transcription of AMPK genes of the high-fat rats and those groups fed with probiotics were noticed, which also suggested that probiotics may not directly activate AMPK. However, the conclusion drawn by Wa et al. (2019) through their findings suggested that the lipid-metabolism-related gene modulation of single probiotics was more efficacious in comparison to the combined probiotics (Wa et al., 2019).
Yi et al. (2020) evaluated the lipid reduction effects and mechanism of action of L. fermentum CQPC04, isolated from traditional naturally fermented vegetable, Sichuan pickle. In this in-vivo study conducted in 6-week-old C57BL/6J mice, the Lactobacillus fermentum CQPC04 showed reduction in abnormal weight gain and abnormal visceral index of mice caused by a high-fat diet. Lactobacillusfermentum CQPC04 could efficiently lower TG, TC, LDL-C, AST, ALT, and AKP (alkaline phosphatase) levels, while increasing the HDL-C levels in the serum of high-fat diet fed mice. Since abnormal levels of ALT, AST, AKP are markers of liver damage, their reduction in this finding suggested that Lactobacillusfermentum CQPC04 could prevent liver damage and as a result prevent obesity and obesity-related disorders. Further, this Lactobacillus strain could also decrease the levels of inflammatory cytokines, like IL-6 (interleukin-6), IL-1β (interleukin-1 beta), TNF-α (tumor necrosis factor alpha), and IFN-γ (interferon gamma) in the serum of high-fat mice (Yi et al., 2020).
Mu et al. (2020) studied the in-vivo lipid-reducing property of L. plantarum KFY02 isolated from naturally fermented yogurt on C57BL/6J mice. They observed the reduction in the levels of AKP, ALT, AST, TG, TC and LDL-C in the serum of high-fat-induced C57BL/6J mice. Further, qPCR experiments revealed that L. plantarum KFY02 could significantly upregulate the mRNA expression of lipid-accumulation regulated enzymes like cholesterol 7α-hydroxylase (CYP7A1), lipoprotein lipase (LPL), peroxisome proliferative-activating receptor α (PPAR-α), carnitinepalmitoyltransferase 1A (CPT1A) in the liver and epididymal adipose tissues, while downregulating the mRNA expression of adipocyte differentiation factors like CCAAT enhancer-binding proteins (C/EBP-α) and peroxisome proliferative-activating receptor γ (PPAR-γ). These results indicated that L. plantarum KFY02 possessed a significant regulatory potential for reducing hyperlipidemia and obesity (Mu et al., 2020).
In an investigation carried out by Kim et al. (2021), four strains of LAB (Pediococcuspentosaceus SMFM2017-GK1, Lactobacillusfermentum SMFM2017-NK3, L. plantarum SMFM2017-NK2, and L. fermentum SMFM2017-NK4), isolated from Kimchi were selected and administered to differently grouped obesity-induced SPF C57BL/6J mice. The findings revealed that the total cholesterol (TC), triglycerides (TG), alanine aminotransferase (ALT) and aspartate transaminase (AST) levels in the serum of LFNK4 group (obesity-induced mice treated with L. fermentum SMFM2017-NK4 were lower than that in the control group (obesity-induced mice treated with phosphate buffer saline) as well as in those groups fed with probiotic strains other than L. fermentum SMFM2017-NK4. Furthermore, on the analysis of the lipid-metabolism associated genes (FAS and Cpt-2), low levels of FAS and high levels of carnitinepalmitoyltransferase (Cpt)-2 in the liver of LFNK4 group when compared with the control group was observed. Also, the levels of expression of anti-oxidant protein Superoxide dismutase (SOD) enzyme -2, CAT, and GPx-1 in the liver were found to be higher in the LFNK4 group, comparatively. This investigation thus indicated the superiority and potency of L. fermentum SMFM2017-NK4 over the other groups in regulating the lipid balance in the mice models (Kim et al., 2021).
L. paracasei HII01, isolated from northern Thai pickles were assessed by Chaiyasut et al. (2021) for their effect on lipid- and carbohydrate-metabolism, inflammation, oxidative stress and digestion. For this purpose 52 hypercholesterolemic Thai human volunteers were randomly grouped into a placebo group and a group treated with L. paracasei HII01 (1.25 × 1010 CFU/ml/day). The outcome of the results revealed a significant reduction in the TC, TG, lipopolysaccharide (LPS) and tumor necrosis factor (TNF)-α of the Lactobacillus treated group when compared to the placebo group. Comparatively, the total antioxidant capacity (TAC), propionic acid, lactic acid, interleukin (IL-10), interferon (IFN-γ) and HDL-C also increased in the lactobacillus treated group. Thus, this double-blind placebo experiment thus explored the hypolipidemic potential of L. paracasei HII01 (Chaiyasut et al., 2021).
The hypocholesterolemic property of L. johnsonii BFE6154, procured from Maasai traditional fermented milk was studied using high-fat high-cholesterol (HFHC) fed C57BL/6 J mice model. In comparison to the mice fed only with HFHC (control), those that were also supplemented with 5 × 109 CFU/day of L. johnsonii BFE6154 apart from receiving a HFHC diet, gained weight slowly upto 2 weeks. Unlike the control group no change in the weight of adipose tissue was observed in those groups supplemented with BFE6154. Moreover, lipid profile tests exhibited that the supplementation of BFE6154 could lower the TC and LDL-C levels while increasing the levels of HDL-C. For further understanding the molecular mechanism involved in the lowering of cholesterol levels via the administration of BFE6154 was analyzed. The results revealed that the BFE6154 supplementation significantly upregulated the gene expression of ATP-binding cassette transporter (ABC) G5, which pumps the cholesterol from the enterocytes into the lumen of the intestine. Also, a decrease in the Intestinal Niemann-Pick C1-Like1 (NPC1L1) with BFE6154 supplementation suggested a suppression of cholesterol absorption in the intestine mediated by NPC1L1. Furthermore, increased cholesterol excretion in the feces of BFE6154 supplemented mice unlike to those of the HFHC control mice, confirmed the Lipid regulating potency of BFE6154 (Yoon et al., 2021).
The effects of lactobacillus species on hypoglycemia
In an investigation carried out by Yadav et al. (2007)probiotic dahi containing Lactobacillus acidophilus and Lactobacillus casei supplemented diet significantly delayed the progression of high fructose-induced glucose intolerance, hyperglycemia, hyperinsulinemia, dyslipidemia, and oxidative stress in rats. White male Wistar rats aged 6–8 weeks (150–160 g body weight) were divided into three groups: a normal control group (NCG) supplemented with standard diet and normal drinking water, a high fructose-fed control group (HFCG) that supplemented with standard diet and 21% fructose solution along with drinking water, and a dahi- and high fructose-treated group (DHFG) that treated with standard diet supplemented with 15% dahi and 21% fructose (Yadav et al., 2007). The liver glycogen level was higher in the HFCG group in the current study due to the high load of fructose mobilized to the liver (Yadav et al., 2007). In general, glycogen in the liver is beneficial; however, presence of excess sugars may result in high levels of LDL and VLDL, which may contribute to the development of diabetic dyslipidemia. The DHFG group accumulated less glycogen than the HFCG group. Lactobacillus casei remarkably delayed the progression of high fructose-induced glucose intolerance, hyperglycemia, hyperinsulinemia (Yadav et al., 2007).
Experiment was conducted by Yadav et al. (2008)where Male Wistar rats aged 6–8 weeks and weighing 91 g were housed in a cage in a small animal house. The effect of oral administration and the impact of dahi comprising probiotic Lactobacillus acidophilus NCDC14 and Lactobacilluscasei NCDC19 (73 × 108 cfu/g) on the progression of streptozotocin (STZ)-induced diabetes in rats (15 g/day/rat) was examined for 28 days (Yadav et al., 2008). Furthermore, probiotic dahi significantly reduced STZ-induced oxidative damage in pancreatic tissues by hindering lipid peroxidation and nitric oxide formation while maintaining antioxidant pools such as glutathione content and superoxide dismutase, catalase, and glutathione peroxidase activities (Yadav et al., 2008). The findings suggest that combining probiotic L. acidophilus and L. casei with dahi cultures increased dahi’s ability to suppress STZ-induced diabetes in rats by inhibiting insulin depletion, preserving diabetic dyslipidemia, and inhibiting lipid peroxidation and nitrite formation which may strengthen the antioxidant system of β-cells and slow the reduction of insulin and rise in blood glucose levels (Yadav et al., 2008).
Study revealed by Hsieh et al. (2013) Lactobacilli Lr 263 (2 × 109 CFU/rat) was orally administered to Male sprague–Dawley by an oral gavage on a daily basis for 14 weeks. This study found a reduction in PPAR-mRNA expression in the adipose tissue of High fat diet rats. Lr263 administration, on the other hand, restored the PPAR-mRNA expression that had been reduced by high fructose treatment. Furthermore, GLUT1 and GLUT4 mRNA expression in HFD rats’ adipose tissue was lower than in the control group (Hsieh et al., 2013). While GLUT1 mRNA expression remained unchanged, Lr263 treatment significantly increased GLUT4 mRNA expression. These findings suggest that Lr263 may improve insulin resistance, enhance glycemic control, and maintain glucose homeostasis in adipose tissue by increasing PPAR- γ. Lr263 administration also significantly reduced adipose tissue TNF- and IL-6 production in HFD rats, implying that Lr263 improved insulin resistance by lowering oxidative stress and pro-inflammatory cytokine production and GLUT4 expression (Hsieh et al., 2013).
In an in-vivo study conducted by Li et al. (2014) where Male Wistar rats were randomly divided into four groups as follows: untreated diabetes mellitus (DM), DM treated with 109 CFU/mL L plantarum NCU116 (NCU), DM treated with fermented carrot juice with L. plantarum NCU116 (FCJ, 109 CFU/ml), and DM treated with non-fermented carrot juice (NFCJ). The effect of fermented carrot juice with Lactobacillus plantarum NCU116 on high-fat and low-dose STZ-induced type 2 diabetes in rats was investigated. For 5 weeks, treatments of DM plus L. plantarum NCU116 (NCU) and DM plus fermented carrot juice with L. plantarum NCU116 (FCJ) were found to favorably control blood glucose, hormones, and lipid metabolism in diabetic rats, with an increase in short-chain fatty acid (SCFA) in the colon (Li et al., 2014). Furthermore, NCU and FCJ improved the antioxidant potential and morphological characteristics of the pancreas and kidney and also upregulated the mRNA of the low-density lipoprotein (LDL) receptor, cholesterol 7-hydroxylase (CYP7A1), glucose transporter-4 (GLUT-4), peroxisome proliferator-activated receptor-α (PPAR-α), and peroxisome proliferator-activated receptor-γ (PPAR-γ). These findings show that L. plantarum NCU116 and fermented carrot juice have the potential to alleviate type 2 diabetes in rats for the initial time (Li et al., 2014).
Lactobacillus is being used in fruit and vegetable fermentation to boost nutritional content, sensory properties, and lifespan. L. plantarum NCU116, isolated from Chinese sauerkraut brine, has been effectively used to boost carrot juice’s anti-diabetic properties (Li et al., 2014). Momordicacharantia also known as bitter melon, is a Cucurbitaceae plant that is green in colour and extremely bitter. It is said to have anti-diabetic properties and has long been used in traditional medicine to alleviate diabetic illnesses. A prior analysis revealed that fermenting Momordicacharantia with L. plantarum improved the anti-diabetic activity in vitro, but further in vivo research is needed (Mazlan et al., 2015).
In a study Male C57BL/6J mice (3 weeks old, inbred-specific, pathogen-free) were grouped as follows; L. plantarum X1(X1) group: mice with T2DM and oral administration of 0.25 ml 2 × 109 L. plantarum X1; L. plantarum CCFM30 (CCFM30) group: mice with T2DM and oral administration of 0.25 ml 2 × 109 L. plantarum CCFM30. In vitro, the cell-free supernatant of Lactobacillus plantarum X1 demonstrated potential anti-diabetic activity by inhibiting -glucosidase activity (Li et al., 2016). L. plantarum X1 was found to improve hyperglycemia, glucose tolerance, insulin resistance, hormone levels, and lipid metabolism when taken orally. In type 2 diabetic mice, L. plantarum X1 partially increased antioxidant capacity and improved cytokine secretion. Furthermore, L. plantarum X1 significantly increased the levels of butyric acid and recovered the levels of acetic acid in the faeces of diabetic mice (Li et al., 2016). Furthermore, oral administration of L. plantarum X1 significantly decreased TNF- and IL-6 levels while increasing IL-10 in T2DM mice, similar to the effect of pioglitazone (diabetes drug). TNF- and IL-6 levels decreased, while IL-10 levels increased, possibly due to SCFA production regulation from oral supplementation with L. plantarum X1. These findings showed that L. plantarum X1 had the potential to alleviate type 2 diabetes and the hypoglycemic ability was closely related to modifications in short-chain fatty acids and gut microbiota (Li et al., 2016).
In a study the mRNA expression levels of phosphatidylinositol-3-kinase (PI3K), protein kinase B (Akt), glycogen synthesis kinase-3 (GSK-3) and glycogen synthesis (GS) in the livers of mice were evaluated using RT-qPCR to further investigate the molecular mechanism of L. casei CCFM419 in alleviating insulin resistance. Three-week old male C57BL/6J mice were grouped into four groups according to body weight as follows: normal control (NC) Diabetic control (DC) group, pioglitazone (P) group, and L. casei CCFM419 (LC) group. The mRNA expression of PI3K and GS was clearly down-regulated in diabetic mice, whereas L. casei CCFM419 intake efficiently increased the expression of the two genes comparative to the DC (Diabetic control) group (Li et al., 2017). Simultaneously, a statistically significant decrease in GSK3 mRNA expression was observed after L. casei CCFM419 treatment compared to the DC (Diabetic control) group. There was no discernible difference in AKT mRNA expression between the four groups (Li et al., 2017).
Among hundreds of LABs isolated from fermented foods, researchers suggested that L. plantarum Ln4 isolates obtained from napa cabbage kimchi has the best ability to reduce diet-induced body weight and insulin resistance. The beneficial effect of the strain on obesity and insulin resistance leads to the recovery of the associated proteins that regulate glucose and lipid metabolism. Administration of Ln4 can alter the levels of mRNA that associated with the glucose and lipid metabolism, as well as the insulin-responsive signaling pathway in in-vitro studies. As a result, Ln4 has the potential to be developed as a therapeutic probiotic for obesity and type 2 diabetes (Lee et al., 2018).
In a study the probiotics L. rhamnosus MTCC: 5957 and L. rhamnosus MTCC: 5897 were isolated from domestic curd and showing bile acid tolerance, hydrophobicity, adhesion, and immunomodulatory capabilities. Probiotic Fermented Milk (PFM) made with Lactobacillus rhamnosus MTCC: 5957, Lactobacillus rhamnosus MTCC: 5897, and their combination improved the health of diabetic male albino rats of Wistar strain (8 weeks old). Enhanced non-enzymatic glycation is one plausible hypothesis connecting high blood sugar and diabetic vascular complications. During diabetes, excess glucose in blood reacts with haemoglobin to form high levels of HbA1c compared to the normal rats, indicating poor glycemic control. According to this research diabetic rats treated with probiotics had significantly lower levels HbA1c which may be the result of improved glucose metabolism. Furthermore, it was also observed that the actions of reactive oxygen scavenging enzymes such as CAT, SOD, and GPx were disrupted in the liver and kidneys of diabetic rats, resulting in the generation of free radicals. Moreover, these free radicals caused lipid peroxidation, which was demonstrated by a significant increase in TBARS concentrations in the liver and kidney of untreated diabetic rats. The findings of this study demonstrated that probiotics improved the hepatic and renal antioxidative enzyme profiles, which can help to reduce the complications of type 1 diabetes over time. Probiotic fermented milks have indeed reported as dietary sources of natural antioxidants that increase the body’s intrinsic antioxidant properties. In diabetic rats, feeding these PFM reduced blood glucose and HbA1c levels while increasing body weight, serum insulin, and HDL-C levels. (Yadav et al., 2018).
Lipid metabolism, oxidative stress, and glucose metabolism were all improved after the administration of oral dose of L. paracasei TD062, which had high -glucosidase inhibitory action. In term of hypoglycemic processes it was observed that in diabetic mice produced by a combination of high-glucose-fat diets and STZ, genes involved in gluconeogenesis and the PI3K/Akt pathway were altered. These results suggested that L. paracasei TD062 might be useful in treating of T2DM (Dang et al., 2018; Feng et al., 2018). L. paracasei isolates have been tested in vitro for their -glucosidase inhibiting properties. The anti-diabetic efficacy of L. paracasei TD062, which had a significant -glucosidase inhibitory activity (31.9%) could persist in simulated gastrointestinal fluids (Dang et al., 2018).
L.brevis strains KLDS 1.0727 and KLDS 1.0373, isolated from dairy products proved to survive in vitro digesting models and had a high level of virulence against foodborne pathogenic microorganisms. Furthermore, the KLDS 1.0727 and KLDS 1.0373 strains produced sufficient GABA, demonstrating an excellent physiological effect in an in vivo testing and considerable impact on reducing blood glucose and insulin levels (Abdelazez et al., 2018).
Fermented foods, including Chinese sauerkraut (Dandong, China), pickled cabbage (Nanjing, China), kipper (Guiyang, China), milk curd (XilinGol League, China), and kefir yogurt (Tibet, China), are the local fermented foods of China. The findings revealed that L. plantarum EPS may effectively lower -amylase activity and could be used as a possible functional food for hyperglycemia treatment (Huang et al., 2020). α-Amylase could efficiently hydrolyze α-1,4-O-glycosidic bonds in starch and produce glucose so, inhibition of α-amylase activity could reduce the blood glucose level. Insulin promotes glucose utilization and consumption in hepatocytes by causing GLUT-4 vesicles to translocate to the plasma membrane, and GLUT-4 translocation is involved in the PI3K/Akt pathway. As previously stated, the most common cause of T2DM is insulin resistance, which results from a failure in the translocation of GLUT-4 vesicles to the plasma membrane. Increases plasma membrane GLUT-4 expression and increases glucose consumption in an insulin-independent manner by upregulating the activity of the PI3K/Akt signalling pathway (Huang et al., 2020). Lactobacillus plantarum EPS inhibited -amylase activity while increasing GLUT-4, Akt-2, and AMPK gene expression in insulin-resistant HepG2 cells in in-vitro study. However, this experiments were conducted in vitro, it is unknown whether it is applicable in vivo or whether this EPS can regulate other metabolic pathways (Huang et al., 2020).
The most common species is pomegranate, or Punica granatum, which originally belonged to the Punicaceae family. Punica is a small genus of deciduous fruits bearing shrubs and it is a nutrient-dense fruit that is high in phytochemicals. The findings were intriguing, especially the preparation technique for improving nutrient value of P. granatum juice by using L. casei as probiotics. The synthesis of quercetin-3-glucoside was linked with suppression of DPPH radicals and DPP4 by L. casei fermented juice (Mustafa et al., 2020). In the therapeutic mechanism of diabetes treatment, fermented P. granatum juice is expected to be an essential source of phytonutrients. The bacterial strain’s capacity to use fruit sugars for fast development without the need for food supplements. P. granatum juice with L. casei might be used for candidates who are allergic to medicine (Mustafa et al., 2020).
α-Glucosidase is an enymethat helps in carbohydrate metabolism found in the brush border membrane. Inhibiting α-glucosidase activity has been shown to prevent T2D by lowering glucose absorption and blood glucose levels. A study was conducted to find a strain with remarkable hypoglycemic ability and the effects of LAB strains on anti-glucosidase activity was studied. It was observed that the rate of α-glucosidase inhibition varied when compared to the control from 19.8% to 72.3%. The -glucosidase inhibition rates of L. gasseriMG4524, L. rhamnosus MG4502, and L. reuteri MG5149 were determined. Inhibition rates were found to be 63.8 1.1%, 63.4 4.7%, and 60.3 1.2% (Jeong et al., 2021).
L. paracasei NL41, which was isolated from a traditional Chinese dairy product in the Xinjiang area of China had the ability to alter the main species of gut microbiome that were linked to production of SCFA (short chain fatty acid) and have anti-inflammation mechanisms. As a consequence it was suggested that the an-tidiabetic mechanism of L. paracasei NL41 was partly linked to the maintenance of the gut microbiomeecosystem, leading to an improvement in gut barrier function, reducing LPS permeation and inhibiting inflammation, and thus alleviating insulin resistance property (Zeng et al., 2021).
In another study isolated from dairy product were selected based on their potential probiotic properties that improved glucose and lipid profiles. L. fermentum MCC2760 applied to Wistar rats (female) were reduced pro-inflammatory cytokines in the liver, gut, MAT (Methionine adenosyltransferases), and muscle tissue, and improved gut barrier and expressions of GLUT4 (Glucose transporter type 4), GLP1 (Glucagon-like peptide 1) and adiponectin in HFD and type 2 diabetic rats.L. fermentum MCC2760can elevated TNF-, IL-1, and IL-6 expression levels in the tissues of the HFD control group and diabetic control group. In both studies, however, groups treated with L. fermentum showed a decrease in TNF- while increasing the expression of the anti-inflammatory marker IL-10. (Archer et al., 2021).
Conclusion
Since the ancient period, fermented foods have been a part of the human diet and are consumed in a variety of forms worldwide. Fermented milk products like dahi can be consumed as a side-dish along with the main food course, while fermented vegetable products may be consumed in the form of pickles or curry in order to enhance flavor in the meals and also add to a healthy diet. Fermented foods are a rich source of microbial repository. Though numerous researches have explored the microbial community of the fermented foods, yet innumerable untapped microorganisms still exist in the fermented foods. Currently, fermented foods have gained much interest due to its associated health benefits and its role as a carrier of probiotics. One of the most promising areas of current research and applications is the use of probiotic Lactobacillus in the formulation of various functional meals owing to its health benefits. Lactobacillus possess various effects namely; antibacterial, anti-inflammatory, ACE-inhibitory, antioxidant, antidiarrheal, antiviral, immunomodulatory, hypoglycemic, hypolipidemic and anti-cancer activities. Apart from these health benefits, they are considered as safe probiotics. However, bacterial species have distinct biological activities that differ from strain to strain and could be used in the development of alternative and therapeutic food products. The ever-increasing risk of cardiovascular diseases and diabetes is an alarming threat to the mankind. Though numerous pharmacological measures have been taken to curb the risk associated with hyperlipidemia and hyperglycemia yet, these drugs are either unsafe for long-term use or are very costly. Thus, non-pharmacological, dietary measures that prove to be a safer alternative to the expensive pharmacological means maybe adopted, probiotic Lactobacillus supplementation being one among the dietary means. Various studies have already highlighted the benefits of Lactobacillus species in regulating the lipid and sugar levels, yet there is scope for novel findings. Also, it is necessary to tap the lesser-known traditional fermented foods to explore their microbial community and their related health benefits. Future research on fermented foods should focus on the microbial community and metabolites of fermented foods using metagenomics, whole genome sequencing (WGS), and metabolomic techniques, with a focus on probiotic markers, as well as large-scale human trials to determine the holistic view of the therapeutic effects of the microorganism present in fermented foods.
Author contributions
KG has framed the hypothesis, corrected and finalized the manuscript. ST and PC has written and edited the manuscript.
Acknowledgments
The authors acknowledge computational and infrastructural support provided by the Department of Food Technology and the Library, University of North Bengal. The authors also acknowledge the Department of Science and Technology (DST)-Science and Engineering Research Board (SERB), Government of India, Project No. EEQ/2019/000738.
Conflict of interest
The authors declare that the research was conducted in the absence of any commercial or financial relationships that could be construed as a potential conflict of interest.
Publisher’s note
All claims expressed in this article are solely those of the authors and do not necessarily represent those of their affiliated organizations, or those of the publisher, the editors and the reviewers. Any product that may be evaluated in this article, or claim that may be made by its manufacturer, is not guaranteed or endorsed by the publisher.
References
Abdelazez, A., Abdelmotaal, H., Evivie, S. E., Melak, S., Jia, F. F., Khoso, M. H., et al. (2018). Screening potential probiotic characteristics of Lactobacillusbrevis strains in vitro and intervention effect on type I diabetes in vivo. Biomed. Res. Int. 2018, 7356173. doi:10.1155/2018/7356173
Abouloifa, H., Gaamouche, S., Rokni, Y., Hasnaoui, I., Bellaouchi, R., Ghabbour, N., et al. (2021). Antifungal activity of probiotic Lactobacillusstrains isolated from natural fermented green olives and their application as food bio-preservative. Biol. Control 152, 104450. doi:10.1016/j.biocontrol.2020.104450
Ai, C., Ma, N., Zhang, Q., Wang, G., Liu, X., Tian, F., et al. (2016). Immunomodulatory effects of different lactic acid bacteria on allergic response and its relationship with in vitro properties. PLoS One 11 (10), e0164697. doi:10.1371/journal.pone.0164697
Albano, C., Morandi, S., Silvetti, T., Casiraghi, M. C., Manini, F., and Brasca, M. (2018). Lactic acid bacteria with cholesterol-lowering properties for dairy applications: In vitro and in situ activity. J. Dairy Sci. 101 (12), 10807–10818. doi:10.3168/jds.2018-15096
Algonaiman, R., Alharbi, H. F., and Barakat, H. (2022). Antidiabetic and hypolipidemic efficiency of Lactobacillus plantarum fermented oat (Avena sativa) extract in streptozotocin-induced diabetes in rats. Fermentation 8 (6), 267. doi:10.3390/fermentation8060267
Ali, S. M., Salem, F. E., Aboulwafa, M. M., and Shawky, R. M. (2022). Hypolipidemic activity of lactic acid bacteria: Adjunct therapy for potential probiotics. Plos one 17 (6), e0269953. doi:10.1371/journal.pone.0269953
An, S. Y., Lee, M. S., Jeon, J. Y., Ha, E. S., Kim, T. H., Yoon, J. Y., et al. (2013). Beneficial effects of fresh and fermented kimchi in prediabetic individuals. Ann. Nutr. Metab. 63 (1-2), 111–119. doi:10.1159/000353583
Anal, A. K. (2019). Quality ingredients and safety concerns for traditional fermented foods and beverages from asia: A review. Fermentation 5 (1), 8. doi:10.3390/fermentation5010008
Aponte, M., Murru, N., and Shoukat, M. (2020). Therapeutic, prophylactic, and functional use of probiotics: A current perspective. Front. Microbiol. 11, 562048. doi:10.3389/fmicb.2020.562048
Archer, A. C., Muthukumar, S. P., and Halami, P. M. (2021). Lactobacillusfermentum MCC2759 and MCC2760 alleviate inflammation and intestinal function in high-fat diet-fed and streptozotocin-induced diabetic rats. Probiotics Antimicrob. Proteins 13 (4), 1068–1080. doi:10.1007/s12602-021-09744-0
Baruah, R., Ray, M., and Halami, P. M. (2022). Preventive and therapeutic aspects of fermented foods. J. Appl. Microbiol. 132 (5), 3476–3489. doi:10.1111/jam.15444
Bin Masalam, M. S., Bahieldin, A., Alharbi, M. G., Al-Masaudi, S., Al-Jaouni, S. K., Harakeh, S. M., et al. (2018). Isolation, molecular characterization and probiotic potential of lactic acid bacteria in Saudi raw and fermented milk. Evid. Based. Complement. Altern. Med. 2018, 7970463. doi:10.1155/2018/7970463
Bonnefont-Rousselot, D., Bastard, J. P., Jaudon, M. C., and &Delattre, J. (2000). Consequences of the diabetic status on the oxidant/antioxidant balance. Diabetes Metab. 26 (3), 163–176. PMID: 10880889.
Bourrie, B. C., Willing, B. P., and Cotter, P. D. (2016). The microbiota and health promoting characteristics of the fermented beverage kefir. Front. Microbiol. 7, 647–302X. doi:10.3389/fmicb.2016.00647
Cabello-Olmo, M., Oneca, M., Torre, P., Sainz, N., Moreno-Aliaga, M. J., Guruceaga, E., et al. (2019). A fermented food product containing lactic acid bacteria protects ZDF rats from the development of type 2 diabetes. Nutrients 11 (10), 2530. doi:10.3390/nu11102530
Chaiyasut, C., Tirawat, Y., Sivamaruthi, B. S., Kesika, P., Thangaleela, S., Khongtan, S., et al. (2021). Effect of lactobacillusparacasei HII01 supplementation on total cholesterol, and on the parameters of lipid and carbohydrate metabolism, oxidative stress, inflammation and digestion in Thai hypercholesterolemic subjects. Appl. Sci. (Basel). 11 (10), 4333. doi:10.3390/app11104333
Chen, H., Miao, H., Feng, Y. L., Zhao, Y. Y., and Lin, R. C. (2014). Metabolomics in dyslipidemia. Adv. Clin. Chem. 66, 101–119. doi:10.1016/b978-0-12-801401-1.00004-9
Chen, M., Guo, W. L., Li, Q. Y., Xu, J. X., Cao, Y. J., Liu, B., et al. (2020). The protective mechanism of Lactobacillusplantarum FZU3013 against non-alcoholic fatty liver associated with hyperlipidemia in mice fed a high-fat diet. Food Funct. 11 (4), 3316–3331. doi:10.1039/C9FO03003D
Chen, M., Ye, X., Shen, D., and Ma, C. (2019). Modulatory effects of gut microbiota on constipation: The commercial beverage yakult shapes stool consistency. J. Neurogastroenterol. Motil. 25 (3), 475–477. doi:10.5056/jnm19048
Costabile, A., Buttarazzi, I., Kolida, S., Quercia, S., Baldini, J., Swann, J. R., et al. (2017). An in vivo assessment of the cholesterol-lowering efficacy of Lactobacillusplantarum ECGC 13110402 in normal to mildly hypercholesterolaemic adults. PloS one 12, e0187964. doi:10.1371/journal.pone.0187964
Dang, F., Jiang, Y., Pan, R., Zhou, Y., Wu, S., Wang, R., et al. (2018). Administration of Lactobacillusparacasei ameliorates type 2 diabetes in mice. Food Funct. 9 (7), 3630–3639. doi:10.1039/C8FO00081F
Di Cagno, R., Coda, R., De Angelis, M., and Gobbetti, M. (2013). Exploitation of vegetables and fruits through lactic acid fermentation. Food Microbiol. 33 (1), 1–10. doi:10.1016/j.fm.2012.09.003
Di Cagno, R., Filannino, P., and Gobbetti, M. (2015). “Fermented foods: Fermented vegetables and other products,” in Encyclopedia of food and health. Editors B. Caballero, P. Finglas, and F. Toldrá (Cambridge, Massachusetts, Oxford: Academic Press), 668–674. doi:10.1016/B978-0-12-384947-2.00284-1
Dimidi, E., Cox, S. R., Rossi, M., and Whelan, K. (2019). Fermented foods: Definitions and characteristics, impact on the gut microbiota and effects on gastrointestinal health and disease. Nutrients 11 (8), 1806. doi:10.3390/nu11081806
Duar, R. M., Lin, X. B., Zheng, J., Martino, M. E., Grenier, T., Pérez-Muñoz, M. E., et al. (2017). Lifestyles in transition: Evolution and natural history of the genus lactobacillus. FEMS Microbiol. Rev. 41 (1), S27–S48. doi:10.1093/femsre/fux030
Everard, A., and Cani, P. D. (2013). Diabetes, obesity and gut microbiota. Best Pract. Res. Clin. Gastroenterol. 27 (1), 73–83. doi:10.1016/j.bpg.2013.03.007
Feng, J., Jiang, Y., Li, M., Zhao, S., Zhang, Y., Li, X., et al. (2018). Diversity and evolution of Lactobacilluscasei group isolated from fermented dairy products in Tibet. Arch. Microbiol. 200 (7), 1111–1121. doi:10.1007/s00203-018-1528-9
Gao, D., Zhu, G., Gao, Z., Liu, Z., Wang, L., and Guo, W. (2011). Antioxidative and hypolipidemic effects of lactic acid bacteria from pickled Chinese cabbage. J. Med. Plants Res. 5 (8), 1439–1446. doi:10.5897/JMPR.9000235
García-Burgos, M., Moreno-Fernández, J., Alférez, M. J., Díaz-Castro, J., and López-Aliaga, I. (2020). New perspectives in fermented dairy products and their health relevance. J. Funct. Foods 72, 104059. doi:10.1016/j.jff.2020.104059
Gardner, D. G., and Shoback, D. M. (2017). Greenspan's basic and clinical endocrinology. New York, NY, United States: McGraw-Hill Education.
Ghatani, K., and Tamang, B. (2017). Assessment of probiotic characteristics of lactic acid bacteria isolated from fermented yak milk products of Sikkim, India: Chhurpi, Shyow, and Khachu. Food Biotechnol. 31 (3), 210–232. doi:10.1080/08905436.2017.1335212
Ghatani, K., Thapa, S., Sha, S. P., Sarkar, S., Modak, D., and Bhattacharjee, S. (2022). Revealing probiotic potential of Enterococcus strains isolated from traditionally fermented Chhurpi and healthy human gut. Front. Microbiol. 13, 909987. doi:10.3389/fmicb.2022.909987
Ghosh, T., Beniwal, A., Semwal, A., and Navani, N. K. (2019). Mechanistic insights into probiotic properties of lactic acid bacteria associated with ethnic fermented dairy products. Front. Microbiol. 10, 502. doi:10.3389/fmicb.2019.00502
Gomes, A. C., Bueno, A. A., de Souza, R. G. M., and Mota, J. F. (2014). Gut microbiota, probiotics and diabetes. Nutr. J. 13 (1), 60–13. doi:10.1186/1475-2891-13-60
Guo, L., Li, T., Tang, Y., Yang, L., and Huo, G. (2016). Probiotic properties of Enterococcus strains isolated from traditional naturally fermented cream in China. Microb. Biotechnol. 9 (6), 737–745. doi:10.1111/1751-7915.12306
Hamouda, R. A., Hamza, H. A., Salem, M. L., Kamal, S., Alhasani, R. H., Alsharif, I., et al. (2022). Synergistic hypolipidemic and immunomodulatory activity of lactobacillus and spirulinaplatensis. Fermentation 8 (5), 220. doi:10.3390/fermentation8050220
Hsieh, F. C., Lee, C. L., Chai, C. Y., Chen, W. T., Lu, Y. C., and Wu, C. S. (2013). Oral administration of Lactobacillusreuteri GMNL-263 improves insulin resistance and ameliorates hepatic steatosis in high fructose-fed rats. Nutr. Metab. 10 (1), 35–14. doi:10.1186/1743-7075-10-35
Huang, Z., Lin, F., Zhu, X., Zhang, C., Jiang, M., and Lu, Z. (2020). An exopolysaccharide from Lactobacillusplantarum H31 in pickled cabbage inhibits pancreas α-amylase and regulating metabolic markers in HepG2 cells by AMPK/PI3K/Akt pathway. Int. J. Biol. Macromol. 143, 775–784. doi:10.1016/j.ijbiomac.2019.09.137
Jawan, R., Kasimin, M. E., Jalal, S. N., Faik, A. M., Abbasiliasi, S., and Ariff, A. (2019). Isolation, characterisation and in vitro evaluation of bacteriocins-producing lactic acid bacteria from fermented products of Northern Borneo for their beneficial roles in food industry. J. Phys. Conf. Ser. 1358, 012020. doi:10.1088/1742-6596/1358/1/012020
Jeong, Y., Kim, H., Lee, J. Y., Won, G., Choi, S. I., Kim, G. H., et al. (2021). The antioxidant, anti-diabetic, and anti-adipogenesis potential and probiotic properties of lactic acid bacteria isolated from human and fermented foods. Fermentation 7 (3), 123. doi:10.3390/fermentation7030123
Kim, D., Choi, Y., Kim, S., Ha, J., Oh, H., Lee, Y., et al. (2021). Lactobacillusfermentum SMFM2017-NK4 isolated from Kimchi can prevent obesity by inhibiting fat accumulation. Foods 10 (4), 772. doi:10.3390/foods10040772
Kim, J., Lee, H., An, J., Song, Y., Lee, C. K., Kim, K., et al. (2019). Alterations in gut microbiota by statin therapy and possible intermediate effects on hyperglycemia and hyperlipidemia. Front. Microbiol. 10, 1947. doi:10.3389/fmicb.2019.01947
Krentz, A. J., and Bailey, C. J. (2005). Oral antidiabetic agents: Current role in type 2 diabetes mellitus. Drugs 65 (3), 385–411. doi:10.2165/00003495-200565030-00005
Kumari, V. B, C., Huligere, S. S., Bajpe, S. N., Sreenivasa, M. Y., &Ramu, R., Silina, E., et al. (2022). Evaluation of probiotic and antidiabetic attributes of Lactobacillus strains isolated from fermented beetroot. Front. Microbiol. 13, 911243. doi:10.3389/fmicb.2022.911243
Lavefve, L., Marasini, D., and Carbonero, F. (2019). Microbial ecology of fermented vegetables and non-alcoholic drinks and current knowledge on their impact on human health. Adv. Food Nutr. Res. 87, 147–185. doi:10.1016/bs.afnr.2018.09.001
Lee, E., Jung, S. R., Lee, S. Y., Lee, N. K., Paik, H. D., and Lim, S. I. (2018). Lactobacillusplantarum strain Ln4 attenuates diet-induced obesity, insulin resistance, and changes in hepatic mRNA levels associated with glucose and lipid metabolism. Nutrients 10 (5), 643. doi:10.3390/nu10050643
Li, C., Ding, Q., Nie, S. P., Zhang, Y. S., Xiong, T., and Xie, M. Y. (2014). Carrot juice fermented with Lactobacillusplantarum NCU116 ameliorates type 2 diabetes in rats. J. Agric. Food Chem. 62 (49), 11884–11891. doi:10.1021/jf503681r
Li, X., Wang, E., Yin, B., Fang, D., Chen, P., Wang, G., et al. (2017). Effects of Lactobacilluscasei CCFM419 on insulin resistance and gut microbiota in type 2 diabetic mice. Benef. Microbes 8 (3), 421–432. doi:10.3920/BM2016.0167
Li, X., Wang, N., Yin, B., Fang, D., Zhao, J., Zhang, H., et al. (2016). Lactobacillusplantarum X1 with α-glucosidase inhibitory activity ameliorates type 2 diabetes in mice. RSC Adv. 6 (68), 63536–63547. doi:10.1039/C6RA10858J
Liang, T., Wu, L., Xi, Y., Li, Y., Xie, X., Fun, C., et al. (2021). Probiotics supplementation improves hyperglycemia, hypercholesterolemia, and hypertension in type 2 diabetes mellitus: an update of meta-analysis. Crit. Rev. Food Sci. Nutr. 61 (10), 1670–1688. doi:10.1080/10408398.2020.1764488
Liu, Y. W., Liong, M. T., and Tsai, Y. C. (2018). New perspectives of Lactobacillusplantarum as a probiotic: The gut-heart-brain axis. J. Microbiol. 56 (9), 601–613. doi:10.1007/s12275-018-8079-2
Lu, C., Sun, T., Li, Y., Zhang, D., Zhou, J., and Su, X. (2017). Modulation of the gut microbiota by krill oil in mice fed a high-sugar high-fat diet. Front. Microbiol. 8, 905. doi:10.3389/fmicb.2017.00905
Macori, G., and Cotter, P. D. (2018). Novel insights into the microbiology of fermented dairy foods. Curr. Opin. Biotechnol. 49, 172–178. doi:10.1016/j.copbio.2017.09.002
Malchoff, C. D. (1991). Diagnosis and classification of diabetes mellitus. Conn. Med. 55 (11), 625–629.
Malki, A. A., Yoon, S. H., Firoz, A., Ali, H. M., Park, Y. H., Hor, Y. Y., et al. (2022). Characterization of new probiotic isolates from fermented ajwa dates of madinah and their anti-inflammatory potential. Appl. Sci. 12 (10), 5082. doi:10.3390/app12105082
Mallappa, R. H., Balasubramaniam, C., Amarlapudi, M. R., Kelkar, S., Adewumi, G. A., Kadyan, S., et al. (2022). “Role of probiotics in the prevention and management of diabetes and obesity,” in Probiotics in the prevention and management of human diseases (Academic Press), 321–336. doi:10.1016/B978-0-12-823733-5.00006-4
Marco, M. L., Heeney, D., Binda, S., Cifelli, C. J., Cotter, P. D., Foligné, B., et al. (2017). Health benefits of fermented foods: Microbiota and beyond. Curr. Opin. Biotechnol. 44, 94–102. doi:10.1016/j.copbio.2016.11.010
Marco, M. L., Sanders, M. E., Gänzle, M., Arrieta, M. C., Cotter, P. D., De Vuyst, L., et al. (2021). The international scientific association for probiotics and prebiotics (ISAPP) consensus statement on fermented foods. Nat. Rev. Gastroenterol. Hepatol. 18, 196–208. doi:10.1038/s41575-020-00390-5
Marquez, A., Andrada, E., Russo, M., Bolondi, M. L., Fabersani, E., Medina, R., et al. (2022). Characterization of autochthonous lactobacilli from goat dairy products with probiotic potential for metabolic diseases. Heliyon 8, e10462. doi:10.1016/j.heliyon.2022.e10462
Mazlan, F. A., Annuar, M. S. M., and Sharifuddin, Y. (2015). Biotransformation of Momordicacharantia fresh juice by Lactobacillus plantarum BET003 and its putative anti-diabetic potential. PeerJ 3, e1376. doi:10.7717/peerj.1376
Medina-Pradas, E., Pérez-Díaz, I. M., Garrido-Fernández, A., and Arroyo-López, F. N. (2017). Review of vegetable fermentations with particular emphasis on processing modifications, microbial ecology, and spoilage. Food Microbiol., 211–236. doi:10.1016/B978-0-08-100502-6.00012-1
Minj, J., Chandra, P., Paul, C., and Sharma, R. K. (2021). Bio-functional properties of probiotic lactobacillus: Current applications and research perspectives. Crit. Rev. Food Sci. Nutr. 61 (13), 2207–2224. doi:10.1080/10408398.2020.1774496
Mohamad, N. I., Manan, M. A., Mohd, M. I., and Abdullah Sani, N. (2022). Antibacterial activity and organic acids formation by Lactobacillus sp. Originated from pickled guava and papaya. J. Sustain. Sci. Manag. 17 (2), 286–295. doi:10.46754/jssm.2022.02.020
Motey, G. A., Owusu-Kwarteng, J., Obiri-Danso, K., Ofori, L. A., Ellis, W. O., and Jespersen, L. (2021). In vitro properties of potential probiotic lactic acid bacteria originating from Ghanaian indigenous fermented milk products. World J. Microbiol. Biotechnol. 37 (3), 52–13. doi:10.1007/s11274-021-03013-6
Mu, J., Zhang, J., Zhou, X., Zalan, Z., Hegyi, F., Takács, K., et al. (2020). Effect of Lactobacillus plantarum KFY02 isolated from naturally fermented yogurt on the weight loss in mice with high-fat diet-induced obesity via PPAR-α/γ signaling pathway. J. Funct. Foods 75, 104264. doi:10.1016/j.jff.2020.104264
Mu, Q., Tavella, V. J., and &Luo, X. M. (2018). Role of Lactobacillus reuteri in human health and diseases. Front. Microbiol. 9, 757. doi:10.3389/fmicb.2018.00757
Mustafa, S. M., Chua, L. S., El-Enshasy, H. A., Abd Majid, F. A., and &Hanapi, S. Z. (2020). Kinetic profile and anti-diabetic potential of fermented Punicagranatum juice using Lactobacillus casei. Process Biochem. 92, 224–231. doi:10.1016/j.procbio.2020.01.014
Nawangsih, E. N., Tugi, R. J. S., and Fasihah, I. S. (2022). Effect of soyghurtLactobacillus acidohillus on blood glucose levels in alloxan-induced diabetic rats. Kme., 46–56. doi:10.18502/kme.v2i2.11067
Ooi, L. G., and Liong, M. T. (2010). Cholesterol-lowering effects of probiotics and prebiotics: A review of in vivo and in vitro findings. Int. J. Mol. Sci. 11 (6), 2499–2522. doi:10.3390/ijms11062499
Ostadzadeh, M., HabibiNajafi, M. B., and Ehsani, M. R. (2022). Lactic acid bacteria isolated from traditional Iranian butter with probiotic and cholesterol‐lowering properties: In vitro and in situ activity. Food Sci. Nutr. doi:10.1002/fsn3.3066
Park, H. D., and Rhee, C. H. (2001). Antimutagenic activity of Lactobacillus plantarum KLAB21 isolated from kimchi Korean fermented vegetables. Biotechnol. Lett. 23 (19), 1583–1589. doi:10.1023/A:1011921427581
Peng, Q., Jiang, S., Chen, J., Ma, C., Huo, D., Shao, Y., et al. (2018). Unique microbial diversity and metabolic pathway features of fermented vegetables from Hainan, China. Front. Microbiol. 9, 399. doi:10.3389/fmicb.2018.00399
Plaza-Diaz, J., Ruiz-Ojeda, F. J., Gil-Campos, M., and Gil, A. (2019). Mechanisms of action of probiotics. Adv. Nutr. 10 (1), S49–S66. doi:10.1093/advances/nmy063
Raghuvanshi, R., Grayson, A. G., Schena, I., Amanze, O., Suwintono, K., and Quinn, R. A. (2019). Microbial transformations of organically fermented foods. Metabolites 9 (8), 165. doi:10.3390/metabo9080165
Reamtong, O., Thiangtrongjit, T., Kosoltanapiwat, N., Panbangred, W., and Prangthip, P. (2021). Potential benefits of L. acidophilus in dyslipidemic rats. Sci. Rep. 11 (1), 1–12. doi:10.1038/s41598-021-85427-8
Rezac, S., Kok, C. R., Heermann, M., and &Hutkins, R. (2018). Fermented foods as a dietary source of live organisms. Front. Microbiol. 9, 1785. doi:10.3389/fmicb.2018.01785
Rodríguez-Sánchez, S., Ramos, I. M., Seseña, S., Poveda, J. M., and &Palop, M. L. (2021). Potential of Lactobacillus strains for health-promotion and flavouring of fermented dairy foods. LWT 143, 111102. doi:10.1016/j.lwt.2021.111102
Schleifer, K. H. (2015). Leuconostocaceae fam. nov. Bergey's Manual of Systematics of Archaea and Bacteria, 1. doi:10.1002/9781118960608.fbm00127
Sharma, A., and Sarkar, P. K. (2015). Microbial diversity in ethno-fermented foods of Indian himalayan region. Bull. Himal. Ecol. 23, 85.
Sharma, B. R., Jaiswal, S., and Ravindra, P. V. (2022). Modulation of gut microbiota by bioactive compounds for prevention and management of type 2 diabetes. Biomed. Pharmacother. 152, 113148. doi:10.1016/j.biopha.2022.113148
Shen, X. L., Zhao, T., Zhou, Y., Shi, X., Zou, Y., and Zhao, G. (2016). Effect of oat β-glucan intake on glycaemic control and insulin sensitivity of diabetic patients: A meta-analysis of randomized controlled trials. Nutrients 8 (1), 39. doi:10.3390/nu8010039
Shiby, V. K., and Mishra, H. N. (2013). Fermented milks and milk products as functional foods—a review. Crit. Rev. Food Sci. Nutr. 53 (5), 482–496. doi:10.1080/10408398.2010.547398
Shirkhan, F., Mirdamadi, S., Mirzaei, M., Akbari-adergani, B., and Nasoohi, N. (2022). The effect of inulin on properties of functional fermented milk produced by native strains isolated from traditional Iranian yogurt. J. Food Technol. Nutr. 5–20. doi:10.30495/jftn.2022.62166.11142
Showpanish, K., Sonhom, N., Janyaphisan, T., Woraprayote, W., and Rumjuankiat, K. (2022). Isolation and optimization to enhance anti-Streptococcussuis bacteriocin production by Lactobacillusplantarum RB01-SO. Int. J. Agric. Technol. 18 (2), 809–828.
Sivamaruthi, B. S., Kesika, P., and &Chaiyasut, C. (2018). Impact of fermented foods on human cognitive function— a review of outcome of clinical trials. Sci. Pharm. 86 (2), 22. doi:10.3390/scipharm86020022
Skrypnik, K., Bogdański, P., Łoniewski, I., Reguła, J., and Suliburska, J. (2018). Effect of probiotic supplementation on liver function and lipid status in rats. Acta. Sci. Pol. Technol. Aliment. 17 (2), 185–192. doi:10.17306/J.AFS.0554
Song, J., Peng, S., Yang, J., Zhou, F., and Suo, H. (2021). Isolation and identification of novel antibacterial peptides produced by Lactobacillus fermentum SHY10 in Chinese pickles. Food Chem. 348, 129097. doi:10.1016/j.foodchem.2021.129097
Sun, L., Zhang, Y., Guo, X., Zhang, L., Zhang, W., Man, C., et al. (2020). Characterization and transcriptomic basis of biofilm formation by Lactobacillus plantarum J26 isolated from traditional fermented dairy products. LWT 125, 109333. doi:10.1016/j.lwt.2020.109333
Tanaka, Y., Tran, P. O. T., Harmon, J., and Robertson, R. P. (2002). A role for glutathione peroxidase in protecting pancreatic β cells against oxidative stress in a model of glucose toxicity. Proc. Natl. Acad. Sci. U. S. A. 99 (19), 12363–12368. doi:10.1073/pnas.192445199
Tang, C., Zhou, W., Shan, M., Lu, Z., and Lu, Y. (2022). Yogurt-derived Lactobacillus plantarum Q16 alleviated high-fat diet-induced non-alcoholic fatty liver disease in mice. Food Sci. Hum. Wellness 11 (5), 1428–1439. doi:10.1016/j.fshw.2022.04.034
Townsend, N., Wilson, L., Bhatnagar, P., Wickramasinghe, K., Rayner, M., and Nichols, M. (2016). Cardiovascular disease in Europe: epidemiological update 2016. Eur. Heart J. 37 (42), 3232–3245. doi:10.1093/eurheartj/ehw334
Wa, Y., Yin, B., He, Y., Xi, W., Huang, Y., Wang, C., et al. (2019). Effects of single probiotic-and combined probiotic-fermented milk on lipid metabolism in hyperlipidemic rats. Front. Microbiol. 10, 1312. doi:10.3389/fmicb.2019.01312
Wang, G., Song, J., Huang, Y., Li, X., Wang, H., Zhang, Y., et al. (2022). Lactobacillus plantarum SHY130 isolated from yak yogurt attenuates hyperglycemia in C57BL/6J mice by regulating the enteroinsular axis. Food Funct. 13 (2), 675–687. doi:10.1039/D1FO02387J
WHO (2022a). Diabetes; fact sheet N˚317, geneva, Switzerland. Available at: https://www.who.int/news-room/fact-sheets/detail/diabetes (Accessed on April 21st, 2022).
WHO (2022b). Global Health Estimates: Life expectancy and leading causes of death and disability. Geneva, Switzerland: Fact sheet N˚317. Available at: https://www.who.int/data/gho/data/themes/mortality-and-global-health-estimates (Accessed on April 21st, 2022).
Wuyts, S., Van Beeck, W., Allonsius, C. N., van den Broek, M. F., and &Lebeer, S. (2020). Applications of plant-based fermented foods and their microbes. Curr. Opin. Biotechnol. 61, 45–52. doi:10.1016/j.copbio.2019.09.023
Yadav, H., Jain, S., and Sinha, P. R. (2007). Antidiabetic effect of probiotic dahi containing Lactobacillusacidophilus and Lactobacilluscasei in high fructose fed rats. Nutrition 23 (1), 62–68. doi:10.1016/j.nut.2006.09.002
Yadav, H., Jain, S., and Sinha, P. R. (2008). The effect of probiotic dahi containing Lactobacillus acidophilus and Lactobacilluscasei on gastropathic consequences in diabetic rats. J. Med. Food 11 (1), 62–68. doi:10.1089/jmf.2006.136
Yadav, R., Dey, D. K., Vij, R., Meena, S., Kapila, R., and Kapila, S. (2018). Evaluation of anti-diabetic attributes of lactobacillus rhamnosus MTCC: 5957, lactobacillus rhamnosus MTCC: 5897 and lactobacillus fermentum MTCC: 5898 in streptozotocin induced diabetic rats. Microb. Pathog. 125, 454–462. doi:10.1016/j.micpath.2018.10.015
Yi, R., Tan, F., Zhou, X., Mu, J., Li, L., Du, X., et al. (2020). Effects of Lactobacillus fermentum CQPC04 on lipid reduction in C57BL/6J mice. Front. Microbiol. 11, 573586. doi:10.3389/fmicb.2020.573586
Yoon, H., Lee, Y., Park, H., Kang, H. J., Ji, Y., and Holzapfel, W. H. (2021). Lactobacillus johnsonii BFE6154 ameliorates diet-induced hypercholesterolemia. Probiotics Antimicrob. Proteins, 1–9. doi:10.1007/s12602-021-09859-4
Zeng, Z., Guo, X., Zhang, J., Yuan, Q., and Chen, S. (2021). Lactobacillus paracasei modulates the gut microbiota and improves inflammation in type 2 diabetic rats. Food Funct. 12 (15), 6809–6820. doi:10.1039/D1FO00515D
Zhao, J., Wang, L., Cheng, S., Zhang, Y., Yang, M., Fang, R., et al. (2022). A potential synbiotic strategy for the prevention of type 2 diabetes: Lactobacillus paracasei JY062 and exopolysaccharide isolated from Lactobacillus plantarum JY039. Nutrients 14 (2), 377. doi:10.3390/nu14020377
Zheng, J., Wittouck, S., Salvetti, E., Franz, C. M., Harris, H., Mattarelli, P., et al. (2020). A taxonomic note on the genus Lactobacillus: Description of 23 novel genera, emended description of the genus Lactobacillus Beijerinck 1901, and union of Lactobacillaceae and Leuconostocaceae. doi:10.7939/r3-egnz-m294
Keywords: lactobacillus, hypolipidemic, hypoglycemic, GRAS, metabolic
Citation: Ghatani K, Thapa S and Chakraborty P (2022) Hypolipidemic and hypoglycemic nature of lactobacillus strains in fermented vegetable and dairy products. Front. Food. Sci. Technol. 2:955990. doi: 10.3389/frfst.2022.955990
Received: 29 May 2022; Accepted: 04 October 2022;
Published: 20 October 2022.
Edited by:
Shashi Kant Bhatia, Konkuk University, South KoreaReviewed by:
Asghar Kamboh, Sindh Agriculture University, PakistanNaseer Ahmad Bhat, University of Kashmir, India
Copyright © 2022 Ghatani, Thapa and Chakraborty. This is an open-access article distributed under the terms of the Creative Commons Attribution License (CC BY). The use, distribution or reproduction in other forums is permitted, provided the original author(s) and the copyright owner(s) are credited and that the original publication in this journal is cited, in accordance with accepted academic practice. No use, distribution or reproduction is permitted which does not comply with these terms.
*Correspondence: Kriti Ghatani, Z2hhdGFuaWtAbmJ1LmFjLmlu