- 1Department of Biochemistry, Biophysics and Molecular Biology, Iowa State University, Ames, IA, United States
- 2Department of Molecular Biology, Genetic Engineering and Biotechnology Research Institute, University of Sadat City, Sadat City, Egypt
We have evaluated the floral nectars of nine species from different sections of the genus Nicotiana. These nine species effectively cover the genus. We found that the nectary glands from these different species showed similar developmental regulation with swelling of nectaries during the first half of development and a distinct color change in the nectary gland as development approaches anthesis. When we examined the composition of the nectar from these nine different species we found that they were similar in content. Carbohydrate compositions of these various nectars varied between these species with N. bonariensis showing the highest and N. sylvestris lowest level of sugars. Based upon the amount of carbohydrates, the nectars fell into two groups. We found that hydrogen peroxide accumulated in the nectars of each of these species. While all species showed the presence of hydrogen peroxide in nectar, the quantitative amounts of hydrogen peroxide which was very high in N. rustica and N. bonariensis, suggesting be a common characteristic in short flower Nicotiana species. We further found that the antioxidant ascorbate accumulated in nectar and β-carotene accumulated in nectaries. β-carotene was most high in nectaries of N. bonariensis. We also examined the presence of proteins in the nectars of these species. The protein profile and quantities varied significantly between species, although all species have showed the presence of proteins in their nectars. We performed a limited proteomic analysis of several proteins from these nectars and determined that each of the five abundant proteins examined were identified as Nectarin 1, Nectarin 3, or Nectarin 5. Thus, based upon the results found in numerous species across the genus Nicotiana, we conclude that the mechanisms identified are similar to those mechanisms found in previous studies on ornamental tobacco nectars. Further, these similarities are remarkably conserved, throughout the genus Nicotiana.
Introduction
The floral nectary is a unique organ that undergoes a complex developmental pathway. Over the past two decades, we have investigated the biochemistry of floral nectar and the floral nectary gland. These studies have focused on an interspecific cross of Nicotiana langsdorffii × N. sanderae (LxS8). This cross has a number of advantages that permit the biochemical analysis of these tissues. First, plants of this cross have very large nectary glands and produce copious quantities of floral nectar. This has permitted large-scale biochemical analyses of both floral nectar and the floral nectary gland. These studies have shown the floral nectar contains a limited array of proteins termed Nectarins (Carter et al., 1999; Carter and Thornburg, 2004a; Naqvi et al., 2005; Park and Thornburg, 2009). These nectar proteins function together in a novel biochemistry pathway termed the Nectar Redox Cycle. The nectar redox cycle is an oxidative cycle that produces very high levels of hydrogen peroxide as a defense compound (Carter and Thornburg, 2004a).
In nectaries of Nicotiana, this oxidative process is initiated by a NADPH oxidase (Carter et al., 2007) that produces high levels of superoxide (Thornburg et al., 2003) and subsequently, the Nectarin 1 superoxide dismutase in Nectar Redox Cycle pathway converts the superoxide into high levels of hydrogen peroxide, up to 4 mM, in nectar (Carter and Thornburg, 2000), that is toxic to multiple microorganisms (Carter et al., 2007). These levels of hydrogen peroxide serve to protect flowers from microbial infections (Thornburg et al., 2003).
In addition, we have also characterized the biochemistry of the nectary gland during floral development. These studies have shown that the nectary glands accumulate very high levels of photosynthate that is stored as starch during the first 4–5 days of floral development which is termed the growth phase (Ren et al., 2007a). Subsequently, about floral stage 9, (about 24 h before anthesis) there is shift in metabolism from starch anabolism to starch catabolism (Ren et al., 2007a) that results in the release of high levels of free sugar that flows into the biosynthesis of antioxidants (ascorbate and β-carotene) (Ren et al., 2007b) and into nectar via the sugar transporter SWEET9 (Lin et al., 2014). This shift in metabolism is transcriptionally controlled by a novel floral transcription factor, MYB305 (Liu et al., 2009). MYB305 is expressed about floral stage 6 (Liu et al., 2009), prior to the metabolic switch that leads to starch breakdown and sugar production. Of note, knockdown of the MYB305 protein in floral nectaries results in plants with reduced production of antioxidants as well as reduced levels of sugar in floral nectar.
Accompanying this maturation process, the nectary morphs into the plant’s premier secretory organ. The primary component of nectar secretions is a carbohydrate-rich material as a reward for pollinator activity. In Nicotiana plants the secretion of nectar begins about floral stage S10, and reaches a maximum at floral stage S12. There are three main carbohydrates that make up the nectar of most species (Bolten et al., 1979). The carbohydrates produced not only enter the secretion pathway to form nectar, but carbohydrates such as glucose, can also act as precursors for the biosynthesis of important nectar/nectary compounds such as ascorbate, oxalate and β-carotene that are crucial in redox metabolism (Horner et al., 2007).
In addition to compensating pollinators for visiting, tobacco nectar also shows defensive properties (Thornburg et al., 2003) while some of these defenses are related to redox activity (Carter and Thornburg, 2004b,c). There are also proteinaceous defenses in the genus Nicotiana (Carter and Thornburg, 2000, Carter and Thornburg, 2004a). The major nectar protein (NEC1) begins to be expressed about floral Stage 10 (Carter et al., 1999) and nectar secretion itself begins prior to Stage 11 (Ren et al., 2007b). Nectar from the interspecific cross produces a limited array of proteins that function together to a developmental NADPH oxidase is expressed initiating the Nectar Redox Cycle (Carter and Thornburg, 2004a) just before anthesis at Stage 12 (Carter et al., 2007).
To extend these observations, we have also examined the nectar biochemistry from Petunia sp., a close relative of tobacco. Those studies demonstrated that the nectar biochemistry of petunia is significantly different that or ornamental tobacco. First, petunia does not produce the high levels of hydrogen peroxide that are found in tobacco nectars and second, the nectar proteins found in petunia nectar are very different from those produced in tobacco nectars (Hillwig et al., 2010b). Because petunia and tobacco nectars are so very different, we initiated the current work is evaluate nectar biochemistry throughout the genus Nicotiana. We therefore have chosen a number of Nicotiana species that broadly represent the breadth of the Nicotiana to characterize their nectar production.
Materials and Methods
Materials
The materials used in these studies were obtained from either Fisher Chemical Co1. or Sigma Chemical Co2. and were of the highest quality available.
Plant Materials
The tobacco species used for this study are shown in Table 3. Seeds were obtained from the United States Department of Agriculture National Genetic Resources Program3, plants were grown from seed in the greenhouse and when approximately 15 cm tall, these plants were transplanted to individual 30 cm pots containing a local potting mix. Plants were grown under 16 h day/8 h night conditions until flowering. Flowers were staged as described in (Koltunow et al., 1990).
Floral Anatomy
To evaluate the floral morphology of these different Nicotiana sp., we characterized the size of the floral opening, where insects enter, the floral size, depth of the floral tube. Analysis of each of these features was characterized from at least 10 flowers each from three different tobacco plants.
Floral size was measured using a digital micrometer, placing one tine at the base of the flower and the other at the corolla-floral tube junction. Likewise, the floral opening was also measured using a digital micrometer, placing both tines at opposite faces of the corolla’s opening. The depth of the floral corolla was measured by inserting a short length of monofilament fishing line (30# test) until it stopped at the base of the gynoecium. The corolla-floral tube junction was then marked on the line, and after removing the line the depth of the corolla was measured.
Carbohydrate Analyses
Nectar was collected from flowers, in the first hours of the day, as previously described (Carter et al., 1999; Naqvi et al., 2005). For quantitative analyses, 100 μL of raw nectar was collected and diluted (1:1000) using double distillated water. The samples were immediately returned to the laboratory for carbohydrate quantification. The levels of sucrose, glucose, and fructose were evaluated using the sucrose/D-glucose/D-fructose determination kit (Boehringer Mannheim/r-Biopharm4, catalog no. 10716260035), according to the manufacturer’s directions.
Protein Quantification
Protein concentrations were determined by the dye-binding method described by (Bradford, 1976), with bovine serum albumin (BSA) as the standard.
SDS-PAGE
Protein profiles from raw nectar were analyzed by SDS-PAGE according method described by (Laemmli, 1970).
Hydrogen Peroxide in Nectar
Hydrogen peroxide in nectar was evaluated using the FOX reagent according by (Bleau et al., 1998; Hillwig et al., 2010b). Twenty-five microliters of nectar was added to 975 μL of distilled water. For analysis of H2O2 200 μL of diluted nectar was used in the assay reaction with Fox reagent. The FOX reagent contained sulfuric acid 1.2 mM, xylenol orange 0.1 mM, ferrous ammonium sulfate 0.25 mM and sorbitol 0.1 mM. The H2O2 concentration in nectar was determined from a standard curve by measurement of the absorbance 560 nm.
β-Carotene Analysis
To evaluate the levels of β-carotene in nectaries, we isolated 40 mg of nectary tissue from stage 12 flowers of each species. Care was taken to insure that non-nectary tissue was excluded from these samples as described (Horner et al., 2007). Carotenoids were extracted from the homogenate using two 1 mL aliquots of acetone followed by a 1 mL aliquot of hexane. The organic layers were combined, dehydrated with anhydrous sodium sulfate, evaporated to dryness, and taken up in 50-μL hexane for analysis. Carotenoid levels were estimated by absorption at 450 nm. The β-carotene was confirmed within each species by thin layer chromatography (TLC) on silica gel plates using an acetone: hexane (9:1) mobile solvent as previously described (Horner et al., 2007).
Ascorbic Acid Analysis
The ascorbic acid analysis was performed according method described by Horner et al. (2007). For these analyses, either nectar was harvested from stage 12 flowers of each species. For analysis of raw nectar, 50 μL of nectar was added to 150 μL of distilled water. An aliquot of 50 μL was used for assay in a total volume of 2 ml of 1% oxalic acid. This was titrated to a pink endpoint with 0.05% 2,6-dichlorophenolindophenol (DCIP) in 0.1 M phosphate buffer, pH 7.0. A standard curve using ascorbate 0–20 μg of ascorbate was prepared to quantitate levels of ascorbate.
Mass Spectrometry (LC-MS/MS)
The proteomic analysis was performed to identify proteins in nectars at the Iowa State University protein facility5. The nectar of different species was initially analyzed by SDS-PAGE according method described previously (Laemmli, 1970). Afterwards, the selected bands were excised and the pieces transferred to a 0.6 mL methanol, washed and then added 20 μl of 1% acetic acid. Next, the proteins were digested in solution with trypsin/Lys-C (Promega). The peptides were then separated by liquid chromatography and analyzed by MS/MS by fragmenting each peptide on Q ExactiveTM Hybrid Quadrupole-Orbitrap Mass Spectrometer from Thermo Scientific. Raw data were analyzed using Thermo Scientific’s Proteome Discoverer Software and the data searched using publically available databases. Bovine serum albumin was used as an internal calibration standard.
Statistical Analysis
To perform the biochemical analysis we used three different tobacco plants and the floral nectar or nectaries collected from multiple flowers of each single plant to compose three independent biological replicates. The one way ANOVA test was performed to determine if there is a significant difference between mean of the each specie from the total and Tukey’s test, at p < 0.05 significance level, was used to analyze the differences between species. The statistical analysis was performed using the NCSS statistical software6.
Results
From earlier studies, we observed that the nectar of ornamental tobacco differed significantly from the nectar of the closely related petunia (Hillwig et al., 2010a). To investigate this observation, we decided to examine the nectars from a variety of tobacco species across the genus Nicotiana to determine whether differences were observed within the tobacco genus. Because our earlier work was done with a species cross that fell within the section Alatae. Then based upon the phylogenetic studies of Bogani et al. (1997), Chase et al. (2003), and Clarkson et al. (2004) we selected five additional Nicotiana sections to enhance diversity within the genus Nicotiana. We obtained seed from numerous species of these sections from the United States Department of Agriculture National Genetic Resources Program7. These were grown to maturity and based upon growth characteristics as well as previously published analyses of pollinator preferences, we chose the Nicotiana species shown in Table 1 for these analyses.
Once the selected plant species were growing and flowering, we compared the floral characteristics of these species. For these values, we measured floral opening (throat width), the corolla length (floral base to corolla), and the depth of the floral corolla. Our interests were to determine the size of a pollinating insect that could enter the floral opening, as well as the depth of the corolla to determine the minimum length of the pollinator’s proboscis. This analysis shown in Table 2 illustrate that there are different categories of flower size among our selected group. Long flowers (>7 cm) include the N. alata and N. sylvestris (qs = 1.53, p < 0.899). The shortest flowers (<2.5 cm) include N. rustica (qs = 33.22, p < 0.01) and N. bonariensis (qs = 34.22, p < 0.01). The intermediate sized flowers ranged from (2.5 cm to 5 cm) encompass the remainder N. benthamiana (qs = 19.42, p < 0.01), N. plumbaginifolia (qs = 20.44, p < 0.01), N. glauca (qs = 22.49, p < 0.01), N. clevelandii (qs = 25.55, p < 0.01), and N. langsdorffii (qs = 29.22, p < 0.01). We also determined the depth of the corolla and we found that the ratio of the corolla depth to the flower size was different, from 68% in N. glauca to 90% in N. alata. Thus, we found that the length of the floral tube is indicative of the length of the pollinator’s proboscis required to reach any nectar at the base of the flower. Also shown in Table 1 is the pollinator syndrome that is used by these species. In species with long flowers (N. alata and N. sylvestris) are preferred by hawkmoth, while species with intermediate or short size flowers (N. glauca or N. langsdorffii) are preferred by bird, hummingbird or bee.
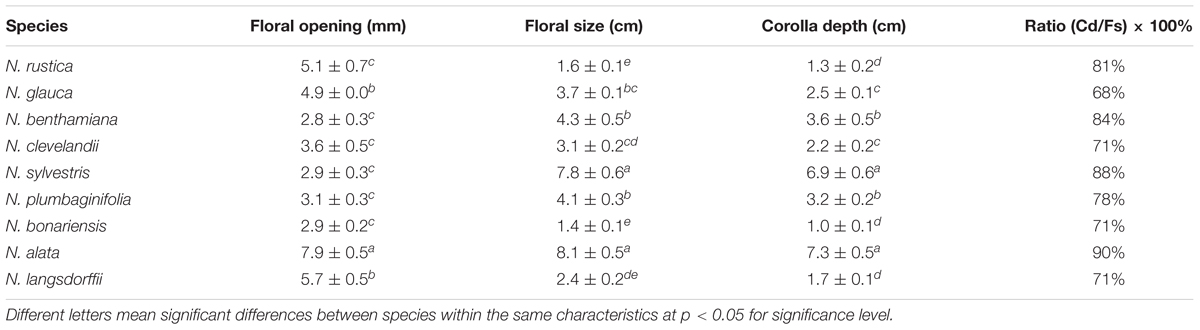
TABLE 2. Floral characteristics among the selected Nicotiana sp. dimensions were determined as outlined in the Section “Materials and Methods.”
Carbohydrate in Nectar
To begin analysis of nectar from these species, we examined the nectar carbohydrate from each of the selected plant species. Using a Sucrose/Glucose/Fructose analysis kit from Boehringer Mannheim/r-Biopharm, we measured each of these components and determined the molar ratios of each of these sugars in the different nectars (Table 3). These carbohydrate composition data (Figure 1) show two different groups: Group 1 – N. glauca, N. benthamiana, N. clevelandii, N. alata, N. sylvestris, N. rustica, and N. plumbaginifolia composed mainly of night flowering Nicotiana species showed the lower levels of sugars (<560 mM), while that day flowering Nicotiana species N. bonariensis and N. langsdorffii, showed the higher levels of sugars content (>1000 mM ). For most of these species such as, N. benthamiana, N. clevelandii, N. sylvestris, and N. plumbaginifolia, the molar ratio of Glucose to Fructose was very similar. However, for a few species, notably the day flowering Nicotiana species, N. glauca and N. rustica, there was significantly more Fructose than Glucose. Similar observations have been made for these species (Tiedge and Lohaus, 2017), and recent findings Tiedge and Lohaus (2017) suggest that the differences in nectar sugars composition may be implicated with different mechanisms of secretion between day/night flowering Nicotiana species.
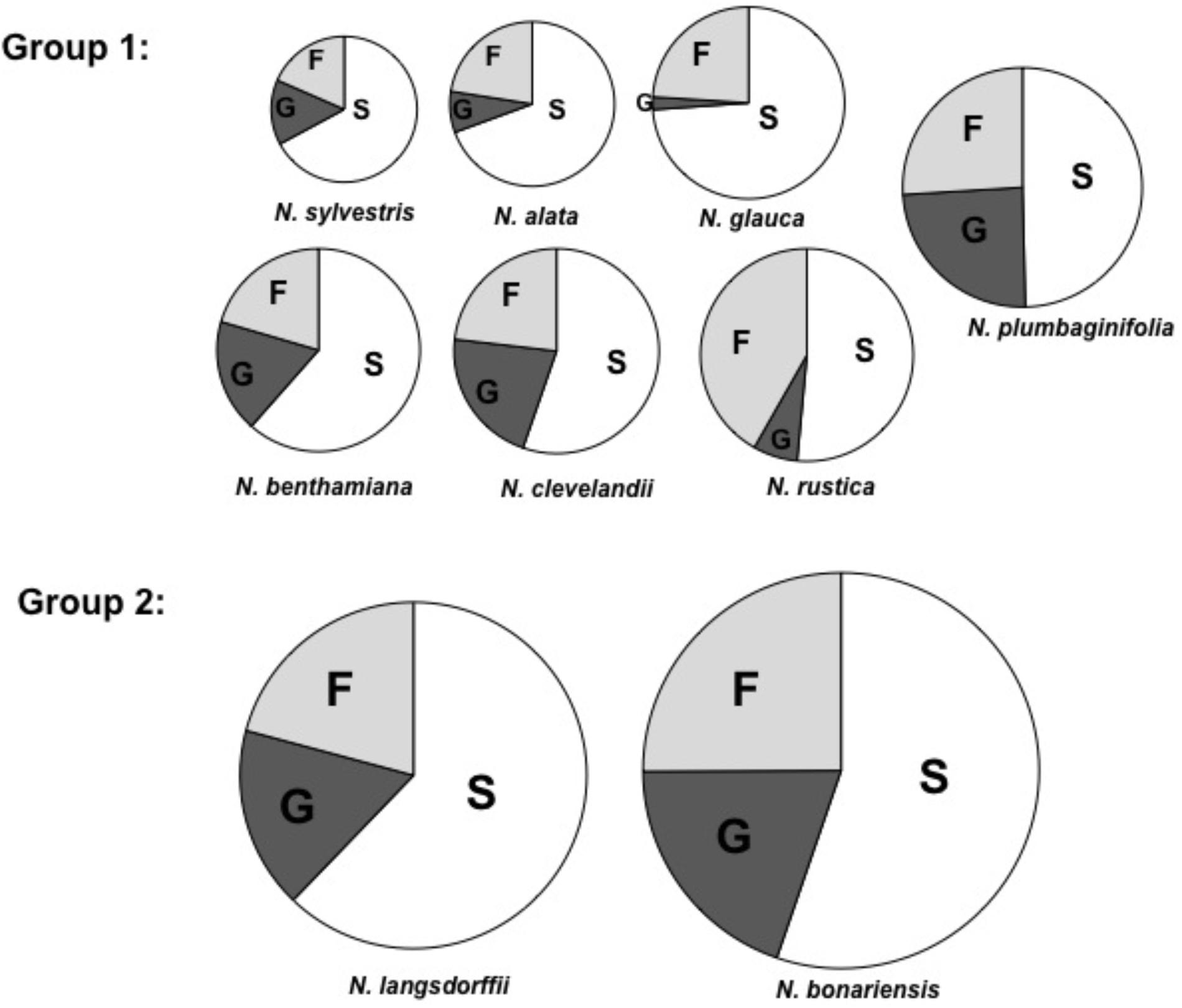
FIGURE 1. Carbohydrate composition of selected tobacco species. Proportions of fructose (F), glucose (G), and sucrose (S) in nectar. The area of each circle represents the total mass of sugar found in each nectar sample. Each segment represents the mean of three samples. Averages ± standard deviation (N = 3). All characteristics of each nectar are present in Table 3. Group 1, total nectar carbohydrate < 560 mM, Group 2, total nectar carbohydrate > 1000 mM.
Nectary Carotenoids
After analyzing the floral characteristics and the carbohydrate composition of the nectars, we then examined the gynoecium and nectary gland of each of these species. Because of our interest in the development of the floral nectary during the process of floral growth, we examined the gynoecia of these species at four different floral stages: Stage 6 (pre secretion), Stage 9 (at the time of the metabolic switch, (Ren et al., 2007b), Stage 12 (anthesis, with full nectar secretion) and the Post-fertilization Stage (48 h after pollination). These stages are shown in Supplementary Figures S1–S4. In all cases, the gynoecium and nectary gland from each species increase in size and the color changes from light yellow or lime green at the earliest stages to a bright orange in nectaries of mature stages. The observed changes were very similar to the development of the nectaries of the interspecific cross LxS8 (Horner et al., 2007). Based upon the obvious swelling of the nectaries and the noticeable color changes, we hypothesized that similar developmental pathways (involving carotenoid accumulation (Horner et al., 2007) and starch buildup and breakdown (Ren et al., 2007b) likely exist in these different Nicotiana species. One striking feature that we observed was extreme levels of carotenoids that were present in the nectaries of N. bonariensis. This is shown best by comparing the color of N. bonariensis (Supplementary Figure S3, #15 and #24) with similarly staged nectaries of the other species in Supplementary Figure S3.
To confirm our hypothesis that similar processes were occurring in the nectaries of these different species, we investigated the biochemistry of these different nectary glands. In LxS8, the orange color arises from β-carotene that is produced from isopentenyl pyrophosphate (IPP) arising from the dimethylallyl pyrophosphate (DMAPP) pathway (Horner et al., 2007). Therefore, we examined the level of β-carotene that was present in the Stage 12 floral nectaries of each species. As shown in Figure 2A, a bright orange pigment that co-chromatographed with β-carotene (Rf = 0.95) was present in the nectaries of each of the Nicotiana species. The amount of β-carotene varied significantly between N. bonariensis and other species showing the highest levels. Other species such as N. sylvestris, N. benthamiana, and N. glauca showed much lower levels of β-carotene. Note that several intermediate pigments that were also yellow were also observed. These were identified as lutein and xanthophyll by virtue of their Rfs = 0.80 and 0.17 (Schoefs, 2005). For each of the selected species, we further spectroscopically quantified the level of β-carotene. The results shown in Figure 2B, mirror the levels that were chromatographically identified in Figure 2A. In this analysis, N. bonariensis showed the highest levels of β-carotene (p < 0.001, df = 17, n = 18), confirming the observations of nectaries shown in the Supplementary Figure S3.
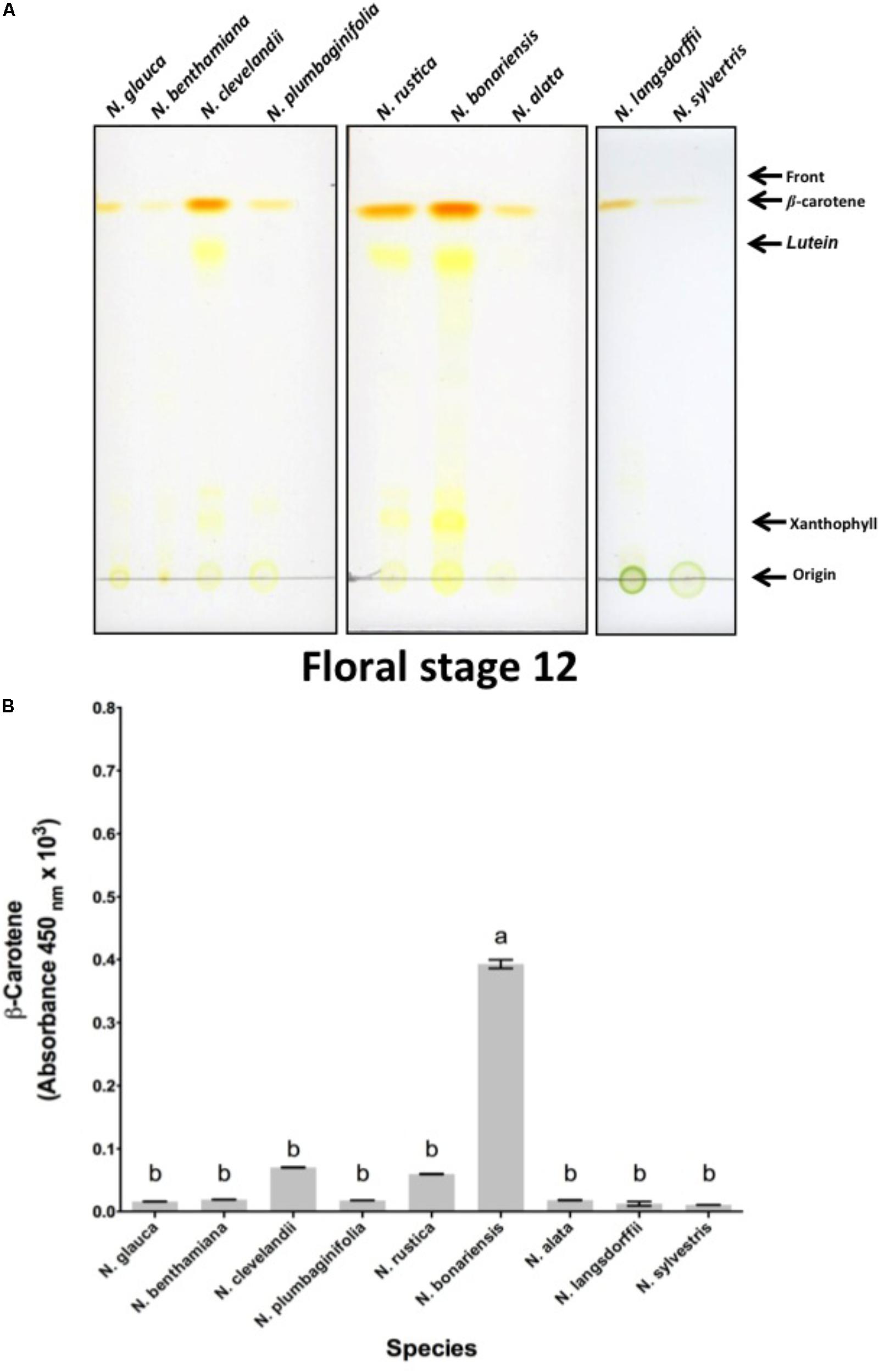
FIGURE 2. Analysis of nectary-expressed carotenoids from different species of Nicotiana sp. (A) Silica gel thin-layer chromatogram (TLC) of carotenoids isolated from stage 12 nectaries of N. glauca; N. benthamiana; N. clevelandii; N. plumbaginifolia; N. rustica; N. bonariensis and N. alata; N. langsdorffii; and N. sylvestris. The origin, solvent front, and migration of various pigments are indicated. Solvent used was 9:1 acetone: hexane solvent. (B) Absorbance (450 nm) of β-carotene in nectary extracts at stage 12. Averages ± standard deviation (N = 3). Species evaluated: 1, N. glauca; 2, N. benthamiana; 3, N. clevelandii; 4, N. plumbaginifolia; 5, N. rustica; 6, N. bonariensis; 7, N. alata; 8, N. langsdorffii; and 9, N. sylvestris. Different letters mean statistical differences between groups.
In addition to the presence of β-carotene in the nectary gland, the LxS8 interspecific cross also showed an additional antioxidant present in soluble nectar, ascorbate (Carter and Thornburg, 2004b). To determine whether these Nicotiana species also express this important nectar antioxidant, we evaluated whether ascorbate was present in the nectar of these selected species. The highest levels ascorbate was evidenced in N. alata and varied significantly (p < 0.001, df = 25, n = 27) of the other species, while, N. langsdorffii and N. sylvestris showed the lower levels, Figure 6.
Hydrogen Peroxide in Nectar
Previous analyses have demonstrated that LxS8 tobacco nectar had high levels of hydrogen peroxide (Carter et al., 1999). To determine whether other Nicotiana species also showed high levels of hydrogen peroxide, nectars were collected and their hydrogen peroxide content were measured with the FOX reagent method as described in Section “Materials and Methods.” As shown in Figure 3, the nectar of all species do indeed contain hydrogen peroxide. However, two species, N. rustica and N. bonariensis had very high levels of hydrogen peroxide, 2.14 and 1.84 μmol.ml-1, respectively. This could correlate with the high levels of sugars that were found in these species, especially in N. bonariensis. The high levels of sugar demonstrated in these species (Table 3) could increase the attractiveness of pollinators, having easier access to the nectar due to the floral characteristics (Table 2) increasing the colonization by microorganisms. The high levels of hydrogen peroxide would be a mechanism of control of microorganisms in nectar. In the other species, we found that the level of hydrogen peroxide was lower. Reasons for this are unclear, but may be related by altered regulation between the species in these complex pathways.
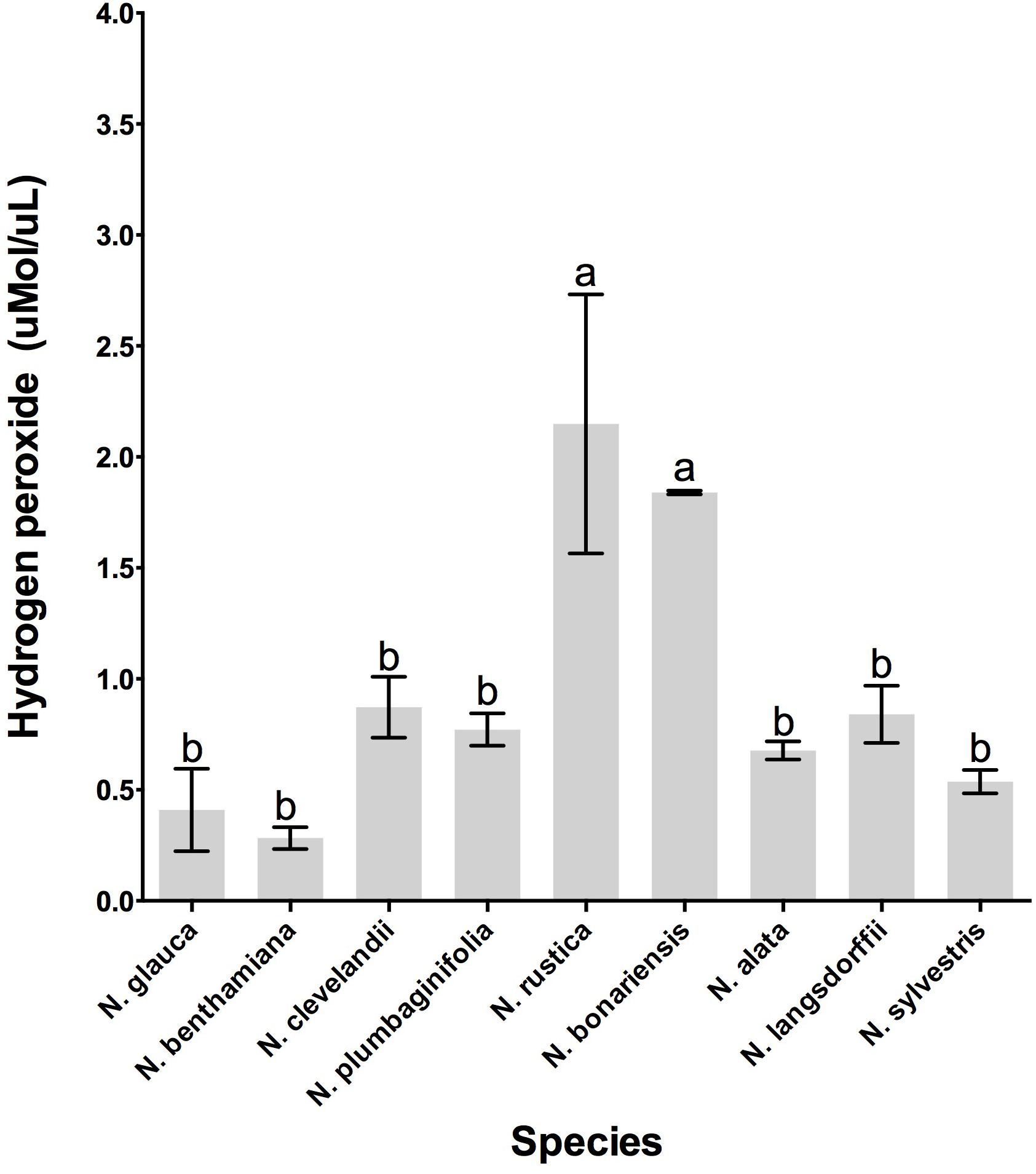
FIGURE 3. Hydrogen peroxide production in the floral nectar of the selected Nicotiana species. Averages ± standard deviation (N = 3). Species evaluated: 1, N. glauca; 2, N. benthamiana; 3, N. clevelandii; 4, N. plumbaginifolia; 5, N. rustica; 6, N. bonariensis; 7, N. alata; 8, N. langsdorffii; and 9, N. sylvestris. Different letters mean statistical differences between groups.
Proteins in Nectar
To determine whether the different Nicotiana species also showed the presence of proteins in their nectars, we quantitated the amount of protein present in the nectars from each of these species. As shown in Figure 4 the variability of nectar proteins was quite large, with some species such as N. glauca and N. sylvestris having very little protein in their nectars (0.044 μg protein/μL of nectar) while other species such as N. clevelandii, N. rustica, and N. bonariensis containing higher concentrations of protein in their nectars (up to 0.778 μg protein/μL of nectar).
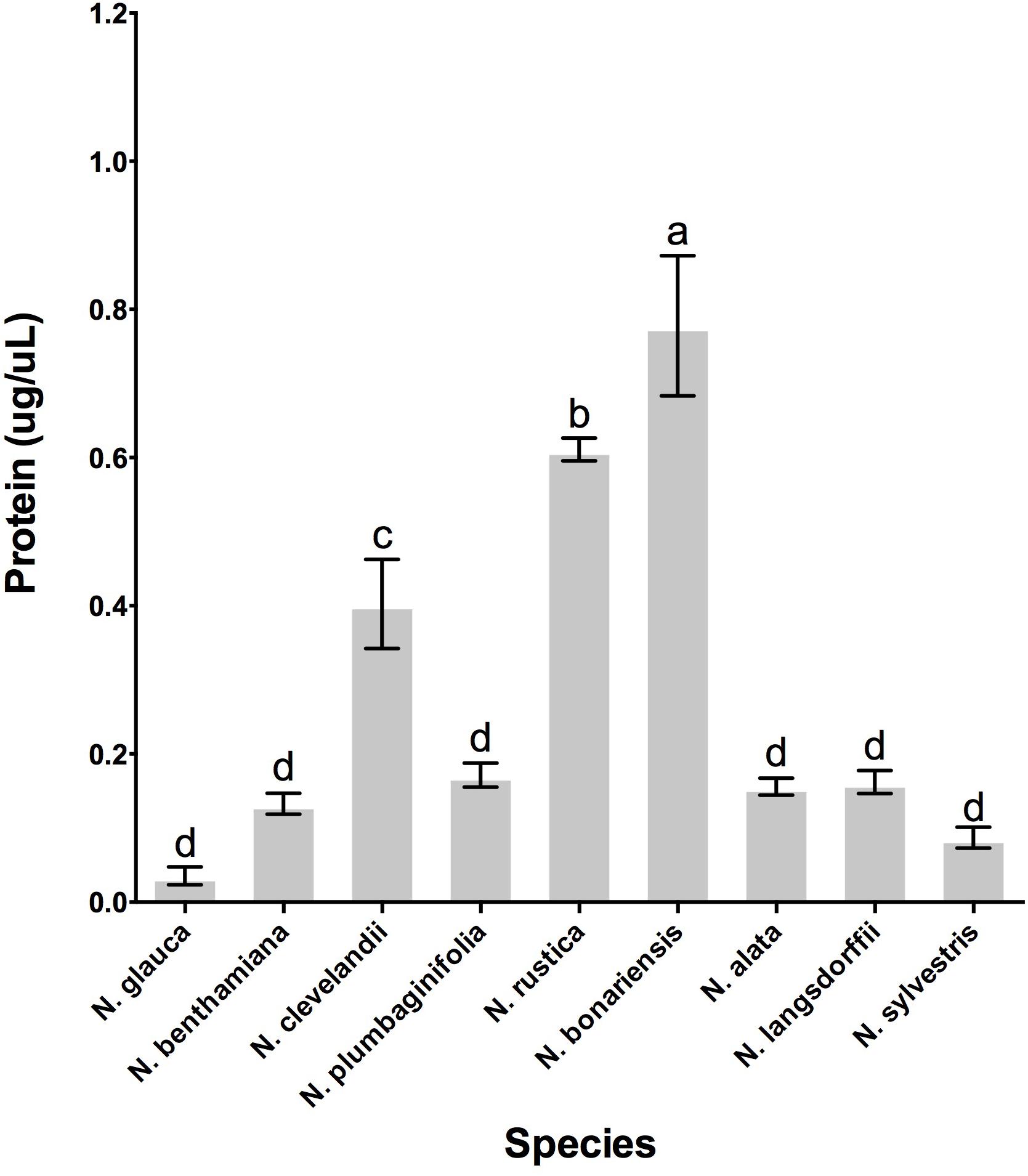
FIGURE 4. Protein accumulation in the nectar of various Nicotiana sp. Species evaluated: 1, N. glauca; 2, N. benthamiana; 3, N. clevelandii; 4, N. plumbaginifolia; 5, N. rustica; 6, N. bonariensis; 7, N. alata; 8, N. langsdorffii; and 9, N. sylvestris. Different letters mean statistical differences between groups.
Once we had confirmed that these species do indeed contain nectar proteins, we next wanted to identify the nectar proteins in these different species. First we investigated the profile of proteins in nectars among the species. SDS-PAGE analysis showed different profiles of the proteins in nectars Figure 5 distributed between 70 and 20 kDa. The protein profile of N. rustica, N. bonariensis, N alata, and N. langsdorffii were very similar to the protein profile observed in the LxS8 interspecific cross (Carter and Thornburg, 2004a) and suggested that the nectarins found in LxS8 may also accumulate in nectar of these other Nicotiana species. The protein quantification also varied significantly (p < 0.001, df = 17, n = 27) among the species.
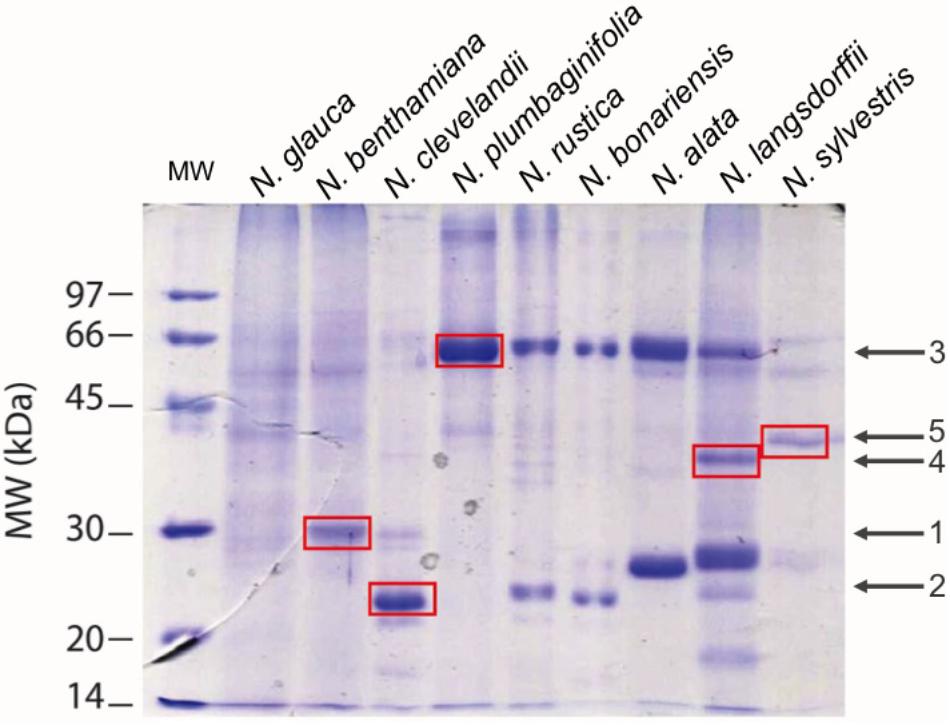
FIGURE 5. Patterns of nectar proteins by SDS-PAGE 12.5%. In each lane was applied 1.25 μgP of raw nectar. Averages ± standard deviation. The standard profile was obtained from the analysis of three different independent experiments (N = 3). The proteins were stained with Coomassie Blue. Averages ± standard deviation (N = 3).
Based upon the SDS PAGE protein gels, it initially appears that there are many different proteins present in these different Nicotiana species. Therefore, we excised five of these proteins from the gel (identified by red spots) and following trypsin digestion; we subjected them to proteomic analysis. The results of this analysis is shown in Table 4 and in detail in Supplementary Figures S5A–C. The spots 1 (from N. benthamiana) and 2 (from N. clevelandii) shown in Table 4, were identified as Nectarin 1-like superoxide dismutases although these proteins had showed differences between the predicted molecular mass by SDS-PAGE 28 and 23 kDa, and the mass found by mass spectrometry 24.6 kDa. The different molecular weight likely is due the degree of glycosylation of Nectarin 1 proteins as was showed previously by Carter et al. (1999).
Furthermore, the data suggest that Nectarin 1 is one of the main proteins found in the nectar of N. benthamiana and N. clevelandii. The major protein identified in nectar of N. plumbaginifolia was identified as Nectarin 5, spot 3, Table 4. The theoretical molecular weight was 59.8, very similar to obtained by SDS PAGE 60 kDa. The spots 4 from N. langsdorffii and 5 from N. sylvestris were identified as a Nectarin 3-like protein.
Discussion
Because of previously observed significant differences between the nectars of the genus Nicotiana (Carter and Thornburg, 2004a) and the genus petunia (Hillwig et al., 2010a), we have investigated the nectars of a broad group of Nicotiana species to determine whether significant differences in nectars exist within this genus. To attract their pollinators, the plants offer floral nectar secreted into the floral tube at the base of the ovary that constitute a rich source of sugars, amino acids, vitamins and other ingredients which provides a rich reward to pollinators (Carter et al., 2006). However, the selected species used in this study include several different pollinator syndromes, Table 1.
It is known that several factors such as sugar composition, amino acids, organic acids and inorganic ions can affect the visitation of pollinators (Kessler and Baldwin, 2007; Afik et al., 2014; Tiedge and Lohaus, 2017). In addition, another important aspect as the floral biology can affect the access of the pollinators (Ackermann and Weigend, 2006). Thus, we conducted a study to understand the relationship between floral biology and the biochemistry of nectar from different genus of Nicotiana. As shown in Table 2, species like N. benthamiana, N. clevelandii N. plumbaginifolia showed intermediate flowers or in other species such as N. alata and N. sylvestris long flowers. Due to the floral characteristics these species have access to nectar more limited requiring specialized pollinators with long proboscis like hawkmoth. On the other hand, species like N. rustica, N. bonariensis, and N. langsdorffii showed short flowers indicating that nectar can be more easily accessed and has different composition. The nectar sugar concentration also differed among Nicotiana species, being divided into two groups. The sugars were higher in species with short flowers such as N. bonariensis and N. langsdorffii, while that other species showed lower concentrations (Figure 1). In species with intermediate or long flowers, there was no observed correlation between the floral length and the concentration of sugar. Recently, (Tiedge and Lohaus, 2017) showed that this correlation is associated with the floral opening period. Day flowering Nicotiana species such as, N. rustica and N. langsdorffii show higher level of sugar than night flowering Nicotiana species. In addition of the floral biology, the nectar sugar composition is another factor that can significantly affect the visitation of pollinators (Torres and Galetto, 2002; Wolff, 2006; Witt et al., 2013). Sucrose represents one of the main sugars found in nectar (Chalcoff et al., 2006). The analysis of nectar carbohydrate composition from Nicotiana species Table 3 showed that sucrose was the major sugar in floral nectar in all species analyzed. Three species, N. alata, N. glauca, and N. sylvestris, showed molar ratio (S/G + F) ≥ 1.00. From these species only N. glauca having intermediate-length flowers has been described as diurnal flowering species, while N. alata and N. sylvestris are night-flowering species. The higher sucrose to hexose molar ratio was previously shown in nectar of long night-flowering N. alata and N. sylvestris and common feature of night Nicotiana flowering species (Tiedge and Lohaus, 2017). The high content of sucrose in nectar of night-flowers or with long floral tubes is associated with higher starch storage in nectaries and different mechanisms of nectar secretion (Tiedge and Lohaus, 2017). Furthermore, the high content of sucrose is related to decrease in viscosity, which facilitates suction by pollinators with long proboscis (Nicolson et al., 2013; Tiedge and Lohaus, 2017). In addition, during the night with lower temperatures, the evaporation effect is reduced and is not necessary high osmolarity for nectar secretion. Thus, long flowered plants takes advantage of these conditions to secrete sucrose, a carbohydrate with low osmolarity (Witt et al., 2013). In addition to sucrose, the nectar of Nicotiana species also presented glucose and fructose in their composition and among the hexoses analyzed, fructose was the predominant sugar (Table 3). In some Nicotiana species studied, the fructose/glucose (F/G) molar ratio was higher or equal 1.0. However, an extremely high molar ratio (F/G) of 11.0 was observed in nectar of N. glauca, followed by N. rustica, with molar ratio of 10.0 (Table 3). Recently a high molar ratio (F/G) of 12.6 for nectar of N. glauca was described, suggesting that this feature is characteristic of this species. The high content of fructose in nectars has been associated with increase sweetness, thus increasing pollinator reward (Tiedge and Lohaus, 2017). Besides the floral characteristics and carbohydrate composition of the nectars, we also examined the biochemistry of nectary gland. In all species, the nectary gland increased in size and changed color as result of carotenoids accumulation Supplementary Figures S1–S4. Carotenoids (b-carotene) were observed in nectaries, and extreme levels were observed in nectaries of N. bonariensis Figures 2A,B. In nectaries, the production of carotenoids and ascorbate provides an antioxidant defense against the high level of hydrogen peroxide found in nectar (Horner et al., 2007). During development of nectaries, the high level accumulation of carotenoids in the nectaries starts about stage 9 when nectaries undergo a metabolic shift and starch are degraded to produce glucose. This glucose is then available to the methylerythritol phosphate (MEP) pathway, which leads to the production of IPP, the carotenoid precursor. The high levels of carotenoids are thought protect nectary cells from the severe oxidative processes that occur as a result of the Nectar redox cycle (Carter and Thornburg, 2004c).
In fact, the nectar of N. bonariensis showed one of the highest content of sugars and hydrogen peroxide (Figure 3). Thus, the high levels of carotenoids and other pigments such as lutein and xanthophyll may function as an additional defense to high level of hydrogen peroxide in nectar of this species. Similar observation was found in nectar of N. rustica, species with short flowers. In N. rustica, the hydrogen peroxide content showed the highest levels among all species tested (Figure 3), however, there appeared to be no correlation with the high levels of carotenoids or ascorbate. Although, N. rustica has intermediate sugar content, the short size flowers could facilitate the access pollinators and growth of microorganisms. Thus, the highest hydrogen peroxide content would be an additional nectar defense. The ascorbate is another important antioxidant involved in the Nectar Redox Cycle. Ascorbate was detected in nectar of all species, however, N. alata showed the highest levels (Figure 6). Ascorbate accumulates at high levels in nectaries at stage 12 (2 μg/nectary) (Horner et al., 2007), composing the nectar during the secretion process and integrating the Nectar Redox Cycle. As previously described, the Nectar Redox Cycle is the remarkable biochemistry pathway responsible for production of high levels of hydrogen peroxide in nectar. The SDS-PAGE analysis showed different profiles of the proteins in nectars Figure 6. N. plumbaginifolia, N. langsdorffii, N. bonariensis, and N. alata from Alatae section had similar profile. The proteomics analysis of the main protein in nectar of N. plumbaginifolia identified as Nectarin 5, spot 3, Table 4, being this protein was very evident Alatae section. This is indicative that Nectarin 5 has a central role in the production of peroxide in Alatae section. Other species such as N. glauca and N. sylvestris had very little protein in their nectars, low abundance without majority proteins. In ornamental tobacco nectar, the nectarins are secreted as array of five proteins and accumulate to almost 250 mg/ml in nectar (Carter et al., 1999). The low abundance of proteins in the nectar N. glauca, N. sylvestris, and N. benthamiana may be associated the low content of hydrogen peroxide quantified these nectars. The very limited production of hydrogen peroxide suggesting that a different mechanism may exist for antimicrobial defense, as RNase activities described to petunia nectar (Hillwig et al., 2010a). Based upon these observations, we conclude that, although the oxidative processes that were first identified and characterized in the LxS8 interspecific cross, including the presence of hydrogen peroxide in nectar as well as antioxidants in both soluble nectar (ascorbate) and in nectary tissues (β-carotene) have been identified in all species, there are species-specific differences are found throughout the genus Nicotiana. Further, the major nectar proteins that we identified from these species belonged to the nectarin family of proteins (especially, Nec1, Nec3, and Nec5).
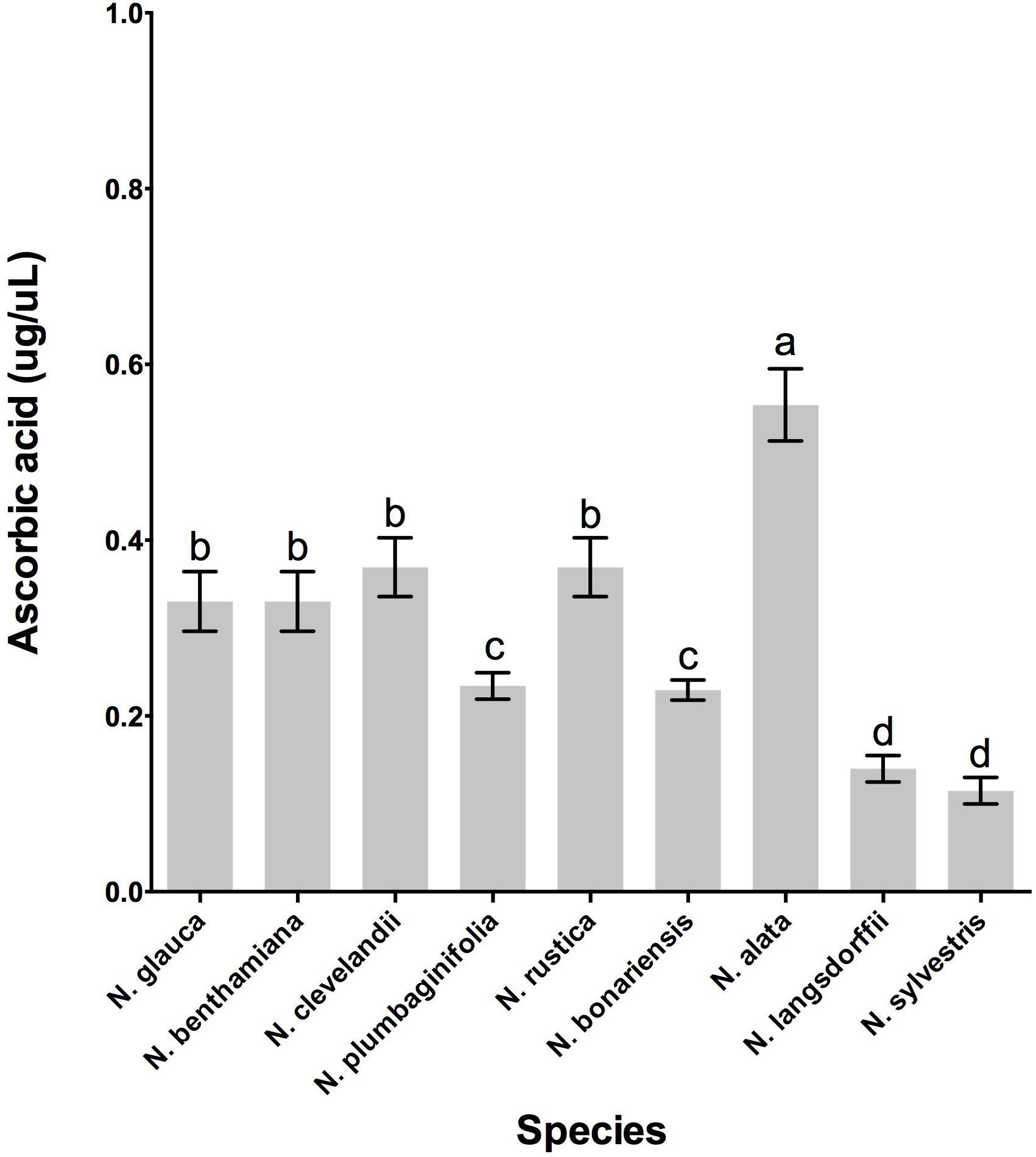
FIGURE 6. Quantity of ascorbate from nectar of nine tobacco species flowers at stage 12. Averages ± standard deviation (N = 3). Species evaluated: 1, N. glauca; 2, N. benthamiana; 3, N. clevelandii; 4, N. plumbaginifolia; 5, N. rustica; 6, N. bonariensis; 7, N. alata; 8, N. langsdorffii; and 9, N. sylvestris. Different letters mean statistical differences between groups.
Author Contributions
FS and RT designed the study, performed the biochemical assays, interpreted the experimental data, and wrote the manuscript. AG cultivated the plants and collected the samples used in all experiments. All authors read and approved the final version of the manuscript.
Funding
This work was supported by the National Science Foundation (NSF #1339246), project: Comparative Functional Genomics of Nectar and Nectaries in the Dicots.
Conflict of Interest Statement
The authors declare that the research was conducted in the absence of any commercial or financial relationships that could be construed as a potential conflict of interest.
Supplementary Material
The Supplementary Material for this article can be found online at: https://www.frontiersin.org/articles/10.3389/fpls.2018.01100/full#supplementary-material
Abbreviations
EST, expressed sequence tagged mRNA; Floral Nectary stages: S6, Stage 6 (immature; beginning of metabolic switch); S9, Stage 9 (immature; pre-secretory, S12, Stage 12 (mature; anthesis); PF, post-fertilization; LxS8, an interspecific cross of Nicotiana langsdorffii × N. sanderae long studied for nectar/nectary research.
Footnotes
- ^www.fishersci.com
- ^www.sigmaaldrich.com
- ^http://www.ars-grin.gov
- ^www.r-biopharm.com
- ^www.protein.iastate.edu
- ^www.ncss.com
- ^www.ars-grin.gov
References
Ackermann, M., and Weigend, M. (2006). Nectar, floral morphology and pollination syndrome in Loasaceae subfam. Loasoideae (Cornales). Ann. Bot. 98, 503–514. doi: 10.1093/aob/mcl136
Afik, O., Delaplane, K. S., Shafir, S., Moo-Valle, H., and Quezada-Euan, J. J. (2014). Nectar minerals as regulators of flower visitation in stingless bees and nectar hoarding wasps. J. Chem. Ecol. 40, 476–483. doi: 10.1007/s10886-014-0455-8
Anon (1972–2017a). Cleveland’s Tobacco (Nicotiana clevelandii in the Nicotianas Database. Available at: https://garden.org/plants/view/200829/Clevelands-Tobacco-Nicotiana-clevelandii/ [accessed August 2, 2017].
Anon (1972–2017b). Tobacco (Nicotiana benthamiana) in the Nicotianas Database. Available at: https://garden.org/plants/view/661958/Tobacco-Nicotiana-benthamiana/
Anon (2017). Nicotiana rustica - L. Available at: http://pfaf.org/user/Plant.aspx?LatinName=Nicotiana+rustica [accessed August 2, 2017].
Bleau, G., Giasson, C., and Brunette, I. (1998). Measurement of hydrogen peroxide in biological samples containing high levels of ascorbic acid. Anal. Biochem. 263, 13–17. doi: 10.1006/abio.1998.2801
Bogani, P., Lio, P., Intrieri, M. C., and Buiatti, M. (1997). A physiological and molecular analysis of the genus Nicotiana. Mol. Phylogenet. Evol. 7, 62–70. doi: 10.1006/mpev.1996.0356
Bolten, A. B., Feinsinger, P., Baker, H. G., and Baker, I. (1979). On the calculation of sugar concentration in flower nectar. Oecologia 41, 301–304. doi: 10.1007/BF00377434
Bradford, M. M. (1976). A rapid and sensitive method for the quantitation of microgram quantities of protein utilizing the principle of protein-dye binding. Anal. Biochem. 72, 248–254. doi: 10.1016/0003-2697(76)90527-3
Carter, C., Graham, R. A., and Thornburg, R. W. (1999). Nectarin I is a novel, soluble germin-like protein expressed in the nectar of Nicotiana sp. Plant Mol. Biol. 41, 207–216. doi: 10.1023/A:1006363508648
Carter, C., Healy, R., O’Tool, N. M., Naqvi, S. M., Ren, G., Park, S., et al. (2007). Tobacco nectaries express a novel NADPH oxidase implicated in the defense of floral reproductive tissues against microorganisms. Plant Physiol. 143, 389–399. doi: 10.1104/pp.106.089326
Carter, C., Shafir, S., Yehonatan, L., Palmer, R. G., and Thornburg, R. (2006). A novel role for proline in plant floral nectars. Naturwissenschaften 93, 72–79. doi: 10.1007/s00114-005-0062-1
Carter, C., and Thornburg, R. W. (2000). Tobacco nectarin I. Purification and characterization as a germin-like, manganese superoxide dismutase implicated in the defense of floral reproductive tissues. J. Biol. Chem. 275, 36726–36733. doi: 10.1074/jbc.M006461200
Carter, C., and Thornburg, R. W. (2004a). Is the nectar redox cycle a floral defense against microbial attack? Trends Plant Sci. 9, 320–324.
Carter, C. J., and Thornburg, R. W. (2004b). Tobacco Nectarin III is a bifunctional enzyme with monodehydroascorbate reductase and carbonic anhydrase activities. Plant Mol. Biol. 54, 415–425.
Carter, C. J., and Thornburg, R. W. (2004c). Tobacco nectarin V is a flavin-containing berberine bridge enzyme-like protein with glucose oxidase activity. Plant Physiol. 134, 460–469.
Chalcoff, V. R., Aizen, M. A., and Galetto, L. (2006). Nectar concentration and composition of 26 species from the temperate forest of South America. Ann. Bot. 97, 413–421. doi: 10.1093/aob/mcj043
Chase, M. W., Knapp, S., Cox, A. V., Clarkson, J. J., Butsko, Y., Joseph, J., et al. (2003). Molecular systematics, GISH and the origin of hybrid taxa in Nicotiana (Solanaceae). Ann. Bot. 92, 107–127. doi: 10.1093/aob/mcg087
Clarkson, J. J., Knapp, S., Garcia, V. F., Olmstead, R. G., Leitch, A. R., and Chase, M. W. (2004). Phylogenetic relationships in Nicotiana (Solanaceae) inferred from multiple plastid DNA regions. Mol. Phylogenet. Evol. 33, 75–90. doi: 10.1016/j.ympev.2004.05.002
Hillwig, M. S., Kanobe, C., Thornburg, R. W., and Macintosh, G. C. (2010a). Identification of S-RNase and peroxidase in petunia nectar. J. Plant Physiol. 168, 734–738. doi: 10.1016/j.jplph.2010.1010.1002
Hillwig, M. S., Liu, X., Liu, G., Thornburg, R. W., and Macintosh, G. C. (2010b). Petunia nectar proteins have ribonuclease activity. J. Exp. Bot. 61, 2951–2965. doi: 10.1093/jxb/erq119
Horner, H., Healy, R., Ren, G., Fritz, D., Seames, C., and Thornburg, R. (2007). Amyloplast to chromoplast conversion in developing ornamental tobacco floral nectaries provides sugar for nectar and antioxidants for protection. Am. J. Bot. 94, 12–24. doi: 10.3732/ajb.94.1.12
Kaczorowski, R. L., Gardener, M. C., and Holtsford, T. P. (2005). Nectar traits in Nicotiana section Alatae (Solanaceae) in relation to floral traits, pollinators and mating system. Am. J. Bot. 92, 1270–1283. doi: 10.3732/ajb.92.8.1270
Kessler, D., and Baldwin, I. T. (2007). Making sense of nectar scents: the effects of nectar secondary metabolites on floral visitors of Nicotiana attenuata. Plant J. 49, 840–854. doi: 10.1111/j.1365-313X.2006.02995.x
Koltunow, A. M., Truettner, J., Cox, K. H., Wallroth, M., and Goldberg, R. B. (1990). Different temporal and spatial gene expression patterns occur during anther development. Plant Cell 2, 1201–1224. doi: 10.1105/tpc.2.12.1201
Laemmli, U. K. (1970). Cleavage of structural proteins during the assembly of the head of bacteriophage T4. Nature 227, 680–685. doi: 10.1038/227680a0
Lin, I. W., Sosso, D., Chen, L. Q., Gase, K., Kim, S. G., Kessler, D., et al. (2014). Nectar secretion requires sucrose phosphate synthases and the sugar transporter SWEET9. Nature 508, 546–549. doi: 10.1038/nature13082
Liu, G., Ren, G., Guirgis, A., and Thornburg, R. W. (2009). The MYB305 transcription factor regulates expression of nectarin genes in the ornamental tobacco floral nectary. Plant Cell 21, 2672–2687. doi: 10.1105/tpc.108.060079
Mahr, S. (2013). Flowering Tobacco, Nicotiana sylvestris. Available at: http://wimastergardener.org/article/flowering-tobacco-nicotiana-sylvestris/ [accessed August 2, 2017].
Naqvi, S. M., Harper, A., Carter, C., Ren, G., Guirgis, A., York, W. S., et al. (2005). Nectarin IV, a potent endoglucanase inhibitor secreted into the nectar of ornamental tobacco plants. Isolation, cloning, and characterization. Plant Physiol. 139, 1389–1400. doi: 10.1104/pp.105.065227
Nicolson, S. W., de Veer, L., Kohler, A., and Pirk, C. W. (2013). Honeybees prefer warmer nectar and less viscous nectar, regardless of sugar concentration. Proc. Biol. Sci. 280:20131597. doi: 10.1098/rspb.2013.1597
Ollerton, J., Watts, S., Connerty, S., Lock, J., Parker, L., Wilson, I., et al. (2012). Pollination ecology of the invasive tree tobacco Nicotiana glauca: comparisons across native and non-native ranges. J. Pollinat. Ecol. 9, 85–95.
Park, S., and Thornburg, R. (2009). Biochemistry of nectar proteins. J. Plant Biol. 52, 27–34. doi: 10.1007/s12374-008-9007-5
Ren, G., Healy, R. A., Horner, H. T., James, M. G., and Thronburg, R. W. (2007a). Expression of starch metabolic genes in the developing nectaries of ornamental tobacco plants. Plant Sci. 173, 621–637. doi: 10.1016/j.plantsci.2007.08.012
Ren, G., Healy, R. A., Klyne, A. M., Horner, H. T., James, M. G., and Thronburg, R. W. (2007b). Transient starch metabolism in ornamental tobaco nectaries regulates nectar composition and release. Plant Sci. 173, 277–290. doi: 10.1016/j.plantsci.2007.05.008
Schoefs, B. (2005). Plant pigments: properties, analysis, degradation. Adv. Food Nutr. Res. 49, 41–91. doi: 10.1016/S1043-4526(05)49002-6
Thornburg, R., Carter, C., Powell, A., Rizhsky, L., Mittler, R., and Horner, H. (2003). A major function of the tobacco floral nectary is defense against microbial attack. Plant Syst. Evol. 238, 211–218. doi: 10.1007/s00606-003-0282-9
Tiedge, K., and Lohaus, G. (2017). Nectar sugars and amino acids in day- and night-flowering Nicotiana species are more strongly shaped by pollinators’ preferences than organic acids and inorganic ions. PLoS One 12:e0176865. doi: 10.1371/journal.pone.0176865
Torres, C., and Galetto, L. (2002). Are nectar sugar composition and corolla tube length related to the diversity of insects that visit Asteraceae flowers? Plant Bio. 4, 360–366. doi: 10.1055/s-200232326
Witt, T., Jurgens, A., and Gottsberger, G. (2013). Nectar sugar composition of European Caryophylloideae (Caryophyllaceae) in relation to flower length, pollination biology and phylogeny. J. Evol. Biol. 26, 2244–2259. doi: 10.1111/jeb.12224
Keywords: Nicotiana, floral nectar, nectaries, carbohydrate in nectar, nectary carotenoids, hydrogen peroxide, proteins in nectar
Citation: Silva FA, Guirgis A and Thornburg R (2018) Nectar Analysis Throughout the Genus Nicotiana Suggests Conserved Mechanisms of Nectar Production and Biochemical Action. Front. Plant Sci. 9:1100. doi: 10.3389/fpls.2018.01100
Received: 26 March 2018; Accepted: 09 July 2018;
Published: 30 July 2018.
Edited by:
Amy Litt, University of California, Riverside, United StatesReviewed by:
Li-Qing Chen, University of Illinois at Urbana–Champaign, United StatesMichael Nicolas, Centro Nacional de Biotecnología (CNB), Spain
Copyright © 2018 Silva, Guirgis and Thornburg. This is an open-access article distributed under the terms of the Creative Commons Attribution License (CC BY). The use, distribution or reproduction in other forums is permitted, provided the original author(s) and the copyright owner(s) are credited and that the original publication in this journal is cited, in accordance with accepted academic practice. No use, distribution or reproduction is permitted which does not comply with these terms.
*Correspondence: Robert Thornburg, dGhvcm5AaWFzdGF0ZS5lZHU=