- Department of Plant and Microbial Biology, University of Minnesota Twin Cities, St. Paul, MN, United States
Over 75% of crop species produce nectar and are dependent on pollinators to achieve maximum seed set, yet little is known about the mechanisms regulating nectar secretion. The phytohormone jasmonic acid (JA) is recognized to be involved in several plant processes including development and defense. JA was also recently shown to positively influence nectar secretion in both floral and extrafloral nectaries. For example, endogenous JA levels peak in flowers just prior to nectar secretion, but the details of how JA regulates nectar secretion have yet to be elucidated. We have found that the octadecanoid pathway does indeed play a role in the production and regulation of floral nectar in Arabidopsis. Null alleles for several JA biosynthesis and response genes had significantly reduced amounts of nectar, as well as altered expression of genes known to be involved in nectar production. We additionally identified crosstalk between the JA and auxin response pathways in nectaries. For example, the nectar-less JA synthesis mutant aos-2 showed no auxin response in nectaries, but both nectar production and the auxin response were restored upon exogenous JA and auxin treatment. Conversely, coi1-1, a JA-Ile-insensitive receptor mutant, displayed no auxin response in nectaries under any circumstance, even in older flowers that produced nectar. Surprisingly, opr3-1, a mutant for 12-oxophytodienoate reductase 3 [an enzyme further down the JA biosynthetic pathway that reduces 12-oxo phytodienoic acid (OPDA)], produced no nectar in newly opened flowers, but did secrete nectar in older flowers. Furthermore, a similar phenotype was observed in coi1-1. Cumulatively, these observations strongly suggest an indispensable role for an octadecanoic acid- and auxin-dependent, but JA- and COI1-dispensible, pathway in regulating nectar production in Arabidopsis.
Introduction
Nectar is a major factor for enhancing plant-mutualist interactions. Nearly 90% of all plant species, including 75% of domesticated crops, benefit from animal-mediated pollination, which is usually facilitated by floral nectar (Klein et al., 2007; Calderone, 2012). Floral nectar is offered to increase pollinator visitation, while extra-floral nectar is used to attract mutualist insects that provide protection from herbivory (Heil, 2011). Despite the importance of nectar in attracting pollinators to promote outcrossing and help plants achieve maximum seed set, relatively little is known about the molecular regulation of nectar secretion.
Arabidopsis thaliana flowers, although highly self-fertile, surprisingly still produce nectar, which is thought to contribute to outcrossing events in natural populations (Hoffman et al., 2003; Kram and Carter, 2009). Due to the wealth of genomic resources and its close relatedness to agriculturally important relatives in the Brassicaceae family – that often require effective pollinator visitation for achieving maximum seed set – Arabidopsis has been an important model for studying the genetic and molecular mechanisms required for nectary function (Kram and Carter, 2009). Arabidopsis flowers have two types of nectaries: median and lateral. The lateral nectaries are located at the base of the short stamen and secrete >99% of total floral nectar (Davis et al., 1998; Kram and Carter, 2009). Median nectaries are located at the base of petals and long stamens and produce little to no nectar. Immature lateral nectaries accumulate starch, which is then broken down at anthesis and the resulting sugars are eventually secreted into the floral nectar (Stage 13–15; newly opened flowers, pollen shed and nectar secretion) (Ren et al., 2007; Kram and Carter, 2009). The nectar produced by these lateral nectaries of Arabidopsis (and most Brassicaceae species) is hexose-rich (nearly all glucose and fructose) (Davis et al., 1998).
A few recent reports have enhanced our understanding of nectar production. The current literature increasingly supports an eccrine-based secretion model for floral nectar in the Brassicaceae (Roy et al., 2017). Eccrine-based secretion relies on plasma membrane-localized pores and transporters to export nectar metabolites from parenchymal cells in the nectary. In one model, nectary starch is degraded and re-synthesized into sucrose by sucrose phosphate synthases (SPS) and other enzymes (Lin et al., 2014). Next, the sucrose is exported into the apoplastic space via the sucrose uniporter AtSWEET9 (Lin et al., 2014). In the apoplastic space CELL WALL INVERTASE 4 (AtCWINV4) catalyzes the hydrolysis of sucrose into hexose monomers, glucose and fructose (Ruhlmann et al., 2010). This invertase activity creates both a constant driving force for sucrose export and a negative water potential causing water to move toward sugars and create water droplets. Perhaps not surprisingly, knockout mutants of SPS, SWEET9, and CWINV4 all lack nectar production. Although we are beginning to understand key genes involved in the process of nectar secretion, the ways in which these processes are regulated is also still poorly understood.
In order to support effective mutualist visitation and proper pollination, floral nectar production must be carefully coordinated with petal opening, pollen shed, stigma receptivity and pollinator activity. Therefore, it is not surprising that floral nectar production would require hormonal regulation to ensure its production is tightly coordinated with these other important processes. Even though proper regulation of nectar secretion is essential to its overall function – effectively manipulating pollinator visitation (Pyke, 2016) – the impacts of each of the phytohormones has remained rather elusive in relation to nectar production. Some studies have examined the relative impacts of auxin (IAA, indole acetic acid) (Bender et al., 2013), gibberellins (Wiesen et al., 2016), and jasmonic acid (JA) (Radhika et al., 2010) with regard to their roles in regulating floral nectar secretion as outlined below.
It is well established that auxin is an important phytohormone that heavily regulates both developmental processes as well as responses to biotic and abiotic stresses (Chapman and Estelle, 2009; Lokerse and Weijers, 2009; Leyser, 2010; Zhao, 2010; Weijers et al., 2018). IAA activates transcriptional responses through binding to the TIR1 F-box receptor, which leads to the ubiquitin-mediated degradation of AUX/IAA transcriptional repressors and the de-repression of auxin response factors (ARFs) thus activating auxin response genes (Dharmasiri et al., 2005). IAA is important for regulating proper floral development too (Aloni et al., 2006). Furthermore, recent reports have shown that auxin-related genes display nectary-enriched expression profiles in the Brassicaceae (Kram et al., 2009; Hampton et al., 2010). Interestingly, PIN6, an auxin efflux transporter, was shown to have a positive effect on total nectar production as PIN6 overexpressers showed an increase in total nectar production and PIN6 knockdown mutants show a decrease in nectar production in Stage 14–15 flowers (Bender et al., 2013). When Arabidopsis inflorescences were treated with exogenous auxin there was a 2 – 10-fold increase in total nectar production in Arabidopsis and Brassica napus, whereas the auxin transport inhibitor NPA decreased nectar output (Bender et al., 2013).
In addition to IAA, JA is another phytohormone known to play important roles in plant flower development as well as plant defense (Wasternack and Hause, 2013). JA is a lipid-derived hormone whose biosynthesis occurs via the octadecanoic pathway and begins with the cleaving of alpha-linolenic acid (C18:3) off of lipid bilayers by phospholipases (e.g., Ishiguro et al., 2001) (see Supplementary Figure S1 for diagram of full synthetic pathway). The primary mode of JA signaling occurs through a similar mechanism as described for IAA. JA signals through the COI1 F-box protein that forms a Skp-Cullin-F-box (SCF) ubiquitin ligase complex that leads to the ubiquitination and degradation of JAZ repressors in the presence of jasmonoyl-L-isoleucine (JA-Ile), the bioactive form of JA (Kelley and Estelle, 2012; Nagels Durand et al., 2016). Some previous reports have shown that JA plays a role in the regulation of nectar production. For example, JA levels in B. napus flowers peak just prior to anthesis which, as previously mentioned, is coincidental with the onset of nectar production (Radhika et al., 2010). Furthermore, exogenous floral application of phenidone, a chemical inhibitor of JA synthesis, lowered nectar production while exogenous JA increased total nectar (Radhika et al., 2010). In tobacco, JA’s importance in regulating nectar secretion is also evident. Tobacco flowers silenced for JA synthesis and response have no nectar and show altered starch utilization (Liu and Thornburg, 2012; Wang et al., 2014). For example, the JA-responsive transcription factor NtMYB305 was shown to be required for nectary maturation and nectar secretion (Liu et al., 2009; Liu and Thornburg, 2012; Wang et al., 2014). These mutants also showed defects in starch accumulation. Furthermore, Stitz et al. (2014) showed that JA-Ile signaling through COI1 is required for nectar production in tobacco flowers. Although there is a small body of work implicating that JA and auxin are involved in nectary maturation and nectar secretion, the details as to exactly how these hormones regulate these processes is still an area that requires further investigation.
Phytohormones do not work in isolation and the coordinated interactions between JA, IAA, and GA make it difficult to study the sole influence that each of these hormones may have on nectary function. For instance, in Arabidopsis IAA acts through ARF6 and ARF8 to induce JA synthesis leading to the expression of MYB21 and MYB24 which together play important roles in flower maturation (Nagpal et al., 2005; Reeves et al., 2012). To add to the complexity of this hormonal coordination, gibberellin-deficient flowers show reduced levels of JA and lower expression of MYB21/24 (Cheng et al., 2009). Using combinations of JA biosynthesis and response mutants, in addition to transgenic lines with altered auxin levels, here we demonstrate that the crosstalk between JA and IAA is essential for the regulation of nectary function.
Materials and Methods
Plant Material and Growth Condition
All plants were grown on peat-based medium with vermiculite and perlite (Pro-Mix BX; Premier Horticulture) in individual pots. Arabidopsis seeds were sterilized in a 33% bleach solution + 0.01% Triton mix for 10 min and subsequently washed five times with water before being planted. The plants were either housed in a Percival AR66LX growth chamber with 16 h day/8 h night, photosynthetic flux of 150 μmol m-2 sec-1 and at 23°C or in a growth room with same 16 h day/8 h night cycle at 22°C. It should be noted that plants used for any direct comparisons were grown at the same time, in the same trays, under the same growth conditions to limit environmental effects on nectar production. Arabidopsis seed for wild-type (Col-0), dad1-1 (SALK_138439), and jar1-11 (CS67935) were obtained from the Arabidopsis Biological Resource Center (ABRC). DR5::GUS plants were previously described (Ulmasov et al., 1997). Homozygous mutants of myb21-4, opr3-1, coi1-1, and aos-2 (SALK_017756) were provided by the Reed Group at the University of North Carolina at Chapel Hill (Reeves et al., 2012). Both coi1-1 and myb21-4 are sterile and not rescuable via exogenous JA application, as such they were carried as heterozygous mutants by backcrossing to wild-type Col-0 and subsequent selection for homozygous mutants in downstream experiments. Jas9-VENUS (Stock N2105629) and mJas-9 Venus (Stock N2105630) seeds were ordered from Nottingham Arabidopsis Stock Center (NASC) and previously described by Larrieu et al., 2015. All T-DNA mutants were genotyped with gene specific primers (Supplementary Table S1) flanking the T-DNA insertion site and the T- DNA specific primer “LBb1.3” to screen for the T-DNA insert as described at: http://signal.salk.edu/tdnaprimers.2.html.
Plants expressing the auxin synthesis gene iaaM under the control of the nectary-specific SWEET9 promoter were generated by PCR amplifying the iaaM gene out of Pseudomonas savastanoi genomic DNA using the primer pair “iaaM ORF-F” and “iaaM ORF-R” and then ligating it into the XmaI and SmeI sites downstream of the nectary-specific SWEET9 promoter in the plant transformation vector pPMK1 (Bender et al., 2012). This vector was subsequently given the name pPMK21. Agrobacterium tumefaciens (GV3101) cells were transformed to carry the pPMK21 vector and used to transform Arabidopsis using the floral-dip method described by Clough and Bent (1998). Transformed seedlings were selected on one half Muarshige and Skoog medium plates with 50 μg/ml kanamycin.
Hormone Treatment of Flowers
1-naphthalene acetic acid (1-NAA) (Cat #N0640) and methyl jasmonate (Cat #392707) were obtained from Sigma-Aldrich. 1-NAA stocks were prepared in ethanol. Treatment of flowers with 10 μM 1-NAA or 500 μM methyl jasmonate was conducted by dipping the inflorescence tips in the appropriate hormone solution dissolved in a 0.05% aqueous Tween 20 solution the evening before testing nectar production. Flowers were phenotyped for the production of nectar and anther dehiscence, or RNA was isolated via the protocol described below.
Gene Expression Analysis
For qRT PCR analysis, RNA was isolated from tissues (minimum of 10 flowers per biological replicate) with the Absolutely RNA Miniprep kit according to the manufacturer’s instructions (Agilent, Catalog #400800). RNA quality was confirmed by spectrophotometric analysis and agarose gel electrophoresis. For cDNA preparation, total RNA (500–1,000 ng) was used as template for Promega’s Reverse Transcription System (Promega, Catalog #A3500). 10 ng of the resulting cDNA was added to the real time PCR reaction mix, which included 10 μl of 2× Brilliant III Ultra-Fast QPCR Master Mix (Agilent Technologies, Cat #600880), 0.4 μl of each forward and reverse primer (10 μM stock), 0.4 μl ROX dye (high), and 6.8 μl nuclease-free H2O. Primers were designed using the online primer design tool “QuantPrime.” The Applied Biosystems StepOnePlus thermocycler was used for real-time PCR, and results were analyzed with Applied Biosystems StepOne software (v2.3). Three biological replicates with a minimum of two technical replicates were performed for each experiment.
Histochemical Staining for Starch and GUS
Starch staining was performed as described by Ruhlmann et al. (2010). Freshly collected flowers were dipped in Lugols iodine solution (Fischer, Cat # S93408) for 5 min after a 1-min vacuum infiltration in the same. Sepals were removed carefully before beginning staining to improve stain permeation. Flowers were subsequently rinsed in deionized water twice to wash off excess stain and subsequently imaged under a dissecting microscope. GUS staining was performed as described previously (Jefferson et al., 1987). For GUS staining, flowers were dissected to remove sepals and subsequently dipped in a GUS staining solution, kept under 15 psi vacuum for 15 min and incubated at 37°C for 16 h. The stain was subsequently removed and the flowers were washed with 90% ethanol thrice, with each wash lasting 2–3 h. The final wash was with 70% ethanol overnight. The flowers were then transferred to deionized water and imaged under a dissecting microscope.
Microscopic Analysis
Flowers expressing mJas9-Venus or Jas9-Venus were viewed either using an Olympus BX-53 fluorescence microscope (YFP cube) or a Nikon A1si spectral confocal microscope attached to a Nikon TE2000 motorized inverted microscope (Nikon USA, Melville, NY, United States) using the 514 nm laser line (laser power 25.4). The emitted fluorescence signal was collected using the 32-channel spectral detector at 6.0 nm spectral resolution using the 20× objective (n.a. 0.75) with zoom setting of 4. Optical sections were collected at 0.5 μm increments then spectrally unmixed using Nikon Elements software ver. 5.1.2.
Results
Exogenous Methyl Jasmonate Induces Nectar Secretion in Arabidopsis JA Synthesis Mutants
Jasmonic acid has been suggested to play a role in nectar production (Heil, 2001; Radhika et al., 2010; Wang et al., 2014). In order to examine the role of JA in Arabidopsis nectar production, we first observed mutants of the JA biosynthesis genes DELAYED ANTHER DEHISCENCE (DAD1, AT2G44810) and ALLENE OXIDE SYNTHASE (AOS, AT5G42650). AOS is a single copy gene indispensable for JA synthesis (Park et al., 2002), whereas DAD1 encodes a filament-specific phospholipase involved in JA synthesis in flowers, but plants are not entirely JA deficient (Ishiguro et al., 2001). The first two open flowers (equivalent of Stage 14 in Col-0) of both aos-2 and dad1-1 produced no nectar while appearing to maintain proper nectary morphology (Figures 1B,C). The mutants also exhibited male sterility (data not shown), which was a previously known phenotype to each mutant (Ishiguro et al., 2001; Park et al., 2002). When these synthesis mutants were exogenously treated with the volatile methylated form of JA, methyl jasmonate (MeJA), nectar production was rescued in recent fully opened flowers (equivalent to Stage 14 flowers in wild-type; Figures 1E,F). Wild-type plants showed no obvious change in nectar volume in response to MeJA treatment (Figure 1D). Since JA synthesis mutants are sterile, their flowers do not quickly dehisce like wild-type, instead their ‘older’ flowers (third open flower and down from the meristem) remain open. Interestingly, these ‘older’ open flowers in dad1-1 produced nectar, whereas those of aos-2 did not (Supplementary Figures S1, S2). The relationship between nectar secretion in ‘young’ and ‘old’ flowers of JA synthesis and response mutants is further explored below. Cumulatively, these results further support an essential role for JA in regulating nectar production.
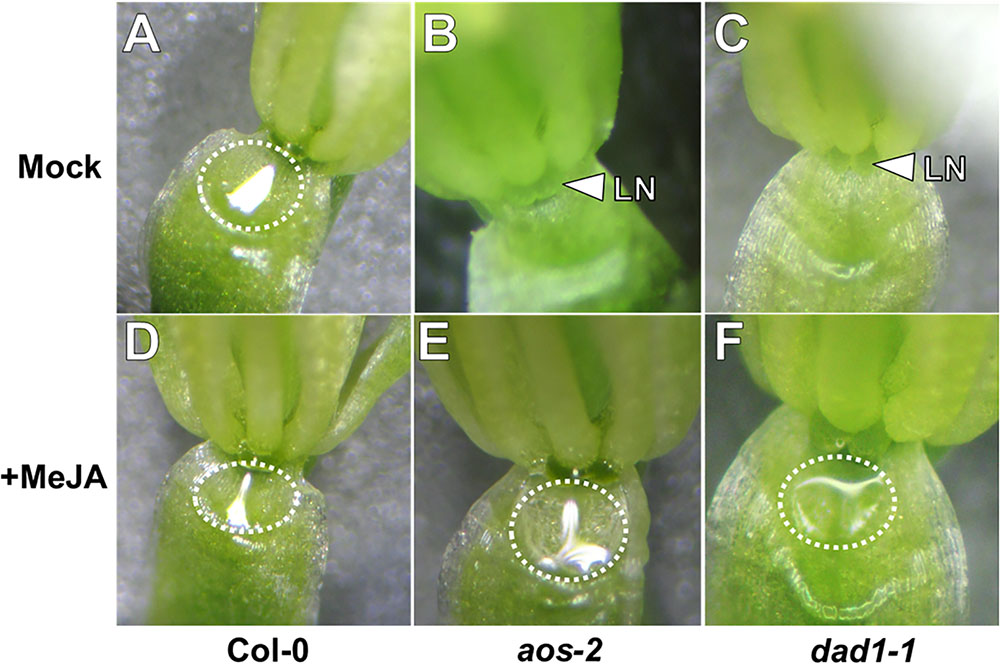
FIGURE 1. Exogenous methyl jasmonate induces nectar secretion in Arabidopsis JA synthesis mutants. Mock treated wild-type flowers at Stage 14 (fully open) secrete nectar (A), whereas the equivalent flowers of JA synthesis mutants (aos-2, B and dad1-1, C) do not. Exogenous application of MeJA (500 μM; D–F) induces nectar secretion in JA synthesis mutant flowers (E,F). Arrowheads indicate the location of the lateral nectaries (LN) and dashed circles outline the presence of nectar droplets.
Exogenous Methyl Jasmonate and Auxin Induce Nectar Secretion and Auxin Responses in aos-2 Nectaries
Bender et al. (2013) previously showed that auxin homeostasis is important for nectar secretion. Cross talk between auxin and JA is also important for floral development (Nagpal et al., 2005; Varaud et al., 2011; Reeves et al., 2012), therefore we hypothesized that JA may be involved in crosstalk with auxin to regulate nectary function and an altered auxin response would be observed in the JA mutants. To further explore this hypothesis, aos-2 was crossed into the auxin-responsive DR5::GUS reporter line and whole flowers were subjected to histochemical GUS staining (Ulmasov et al., 1997). In the wild-type background the DR5::GUS reporter showed a strong auxin response in both the median and lateral nectaries (Supplementary Figure S2A). However, there was no signal in the nectaries of the aos-2 background, which produce no nectar (Figures 2A,D). When flowers were treated with MeJA, both nectar production and the nectary auxin response were rescued (Figures 2B,E).
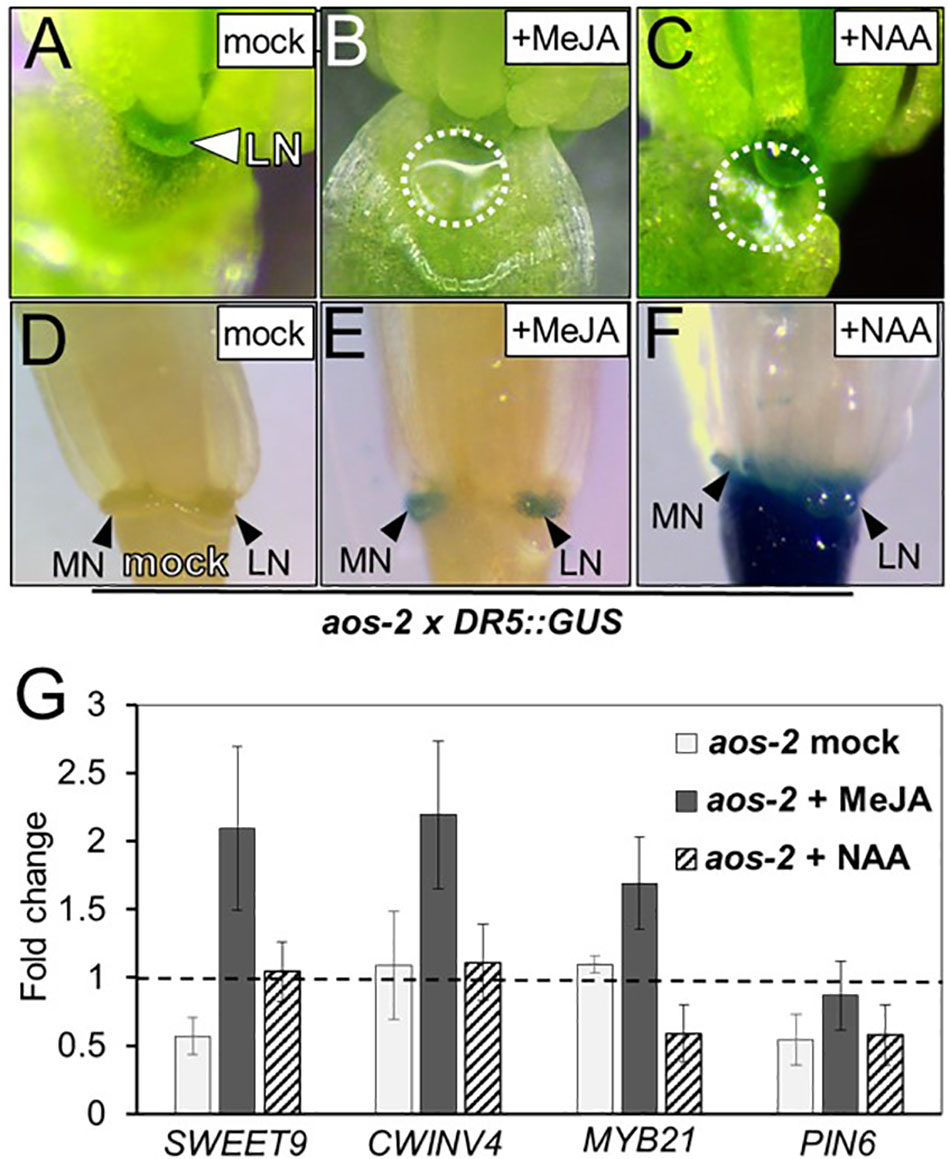
FIGURE 2. Exogenous methyl jasmonate and auxin induce nectar secretion and auxin responses in aos-2 nectaries. Mock treated aos-2 flowers do not secrete nectar (A) and lack the nectary auxin response (D) observed in wild-type plants. Treatment of aos-2 flowers with either MeJA (500 μM) or the synthetic auxin NAA (10 μM) induces nectar secretion (B,C) and the nectary auxin response (E,F). The expression of key genes involved in nectar production were evaluated in whole aos-2 Stage 14 flowers after treatment with MeJA or NAA by qRT-PCR (G). Data is presented as fold-change in expression of each gene normalized to Col-0 (dashed line).
Since previous reports have shown auxin treatment can increase nectar production (Bender et al., 2013), we decided to test if exogenous application of 1-naphthaleneacetic acid (NAA) – a synthetic membrane permeable auxin – could rescue nectar production in JA mutants. As with MeJA, floral crowns exogenously treated with 10 μM NAA displayed a restoration of nectar production (Figure 2C).
Given the dramatic change of nectar production in these JA biosynthesis mutants, we examined the expression of previously characterized genes known to be involved in nectar production in aos-2 flowers exogenously treated with either MeJA or NAA (Figure 2G). Genes targeted for qRT-PCR analysis in JA mutants included SWEET9, a sucrose transporter (Lin et al., 2014), CWINV4, an invertase responsible for cleaving sucrose into hexose sugars (Ruhlmann et al., 2010), PIN6, an auxin efflux transporter (Bender et al., 2013) and MYB21, an ortholog of the JA-inducible tobacco transcription factor MYB305, which is required for nectar secretion (Liu et al., 2009; Liu and Thornburg, 2012; Reeves et al., 2012; Wang et al., 2014).
SWEET9 and PIN6 transcripts were downregulated in nectar-less mock treated aos-2 flowers relative to Col-0 (wild-type) (Figure 2G), which in itself could explain the loss of nectar production (Bender et al., 2013; Lin et al., 2014). Exogenous treatment of MeJA restored nectar production (Figure 2E) and induced the expression of SWEET9, CWINV4, and MYB21 by ∼2-fold compared to wild-type and ∼4-fold relative to the mock treated aos-2 specifically for SWEET9 (Figure 2G). aos-2 flowers treated with exogenous NAA also produced nectar and restored SWEET9 transcript to wild-type levels, but MYB21 transcript abundance was downregulated and had little effect on PIN6 transcript level relative to mock-treated Col-0 (Figure 2G).
Nectary-Derived Auxin Can Rescue Nectar Secretion in aos-2
Exogenous auxin (NAA) restored nectar production in aos-2 flowers (Figure 2), but since NAA could only be applied to whole flowers it was not clear if the auxin-dependent signaling was limited to the nectaries. Thus, we decided to engineer transgenic aos-2 that could produce high endogenous auxin specifically in the nectaries by expressing the auxin biosynthesis gene iaaM (Klee et al., 1987) under control of the nectary-specific SWEET9 promoter (Bender et al., 2013; Lin et al., 2014). This construct, SWEET9pro::iaaM, was first transformed into wild-type plants. The subsequent SWEET9pro::iaaM transgenic line was then crossed into aos-2 and phenotyped. The first two fully open flowers (‘young,’ Figure 3A) of aos-2 x SWEET9pro::iaaM did not produce nectar (Figure 3C) similar to both the ‘young’ and ‘old’ flowers of aos-2 alone (Figures 1B, 3B). The ‘old’ flowers (open flowers 3–5 of down from the meristem) of aos-2 × SWEET9pro::iaaM did produce nectar (Figure 3D). Hence the nectary-derived auxin eventually caused nectar to be produced in the aos-2 background. It should be noted that the anthers never dehisced in aos-2, either with or without SWEET9pro::iaaM, so fertilization was not a cause of the auxin signaling.
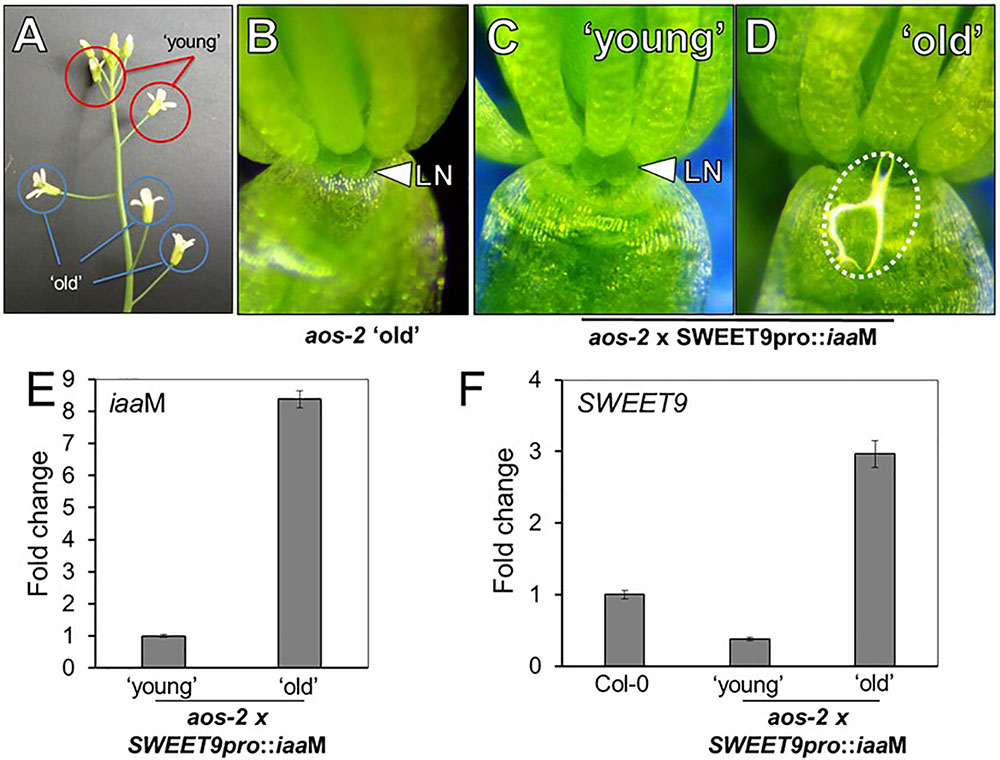
FIGURE 3. Endogenous auxin induces nectar secretion in aos-2. (A) Relative locations of ‘young’ and ‘old’ flowers in JA-synthesis mutants, like aos-2. ‘Old’ aos-2 flowers produced either no nectar (B) or a very faint glistening of fluid on the inner surface of the sepal. ‘Young’ aos-2 flowers expressing the auxin synthesis gene iaaM under control of the SWEET9 promoter do not produce nectar (C), whereas the corresponding ‘old’ flowers produce large nectar droplets (D). (E) iaaM expression is strongly induced in ‘old’ aos-2 × SWEET9pro::iaaM flowers (qRT-PCR data normalized to ‘young’ flowers). (F) Expression of SWEET9 transcripts in Col-0 and ‘young’ and ‘old’ aos-2 × SWEET9pro::iaaM flowers (qRT-PCR data normalized to Col-0 flowers).
To determine if gene expression could account for nectar secretion observed in aos-2 × SWEET9pro::iaaM, we first verified that iaaM expression was induced in ‘old’ vs. ‘young’ flowers (Figure 3E). We hypothesized that the low expression of SWEET9 in the aos-2 background (Figure 2G) would correspondingly keep iaaM levels low in younger aos-2 × SWEET9pro::iaaM flowers. The gradual increase in endogenous auxin, driven by control of the SWEET9 promoter, subsequently would result in a positive feedback loop and nectar production in ‘old’ aos-2 × SWEET9pro::iaaM flowers. Indeed, SWEET9 transcripts in aos-2 × SWEET9pro::iaaM flowers were significantly downregulated in ‘young’ flowers, but upregulated >3-fold in ‘old’ flowers relative to Stage 14 Col-0 flowers (open and secreting nectar) (Figure 3F). As a control, flowers from both ‘young’ and ‘old’ aos-2 not harboring the SWEET9pro::iaaM transgene were examined for SWEET9 expression, which demonstrated that SWEET9 is not induced in ‘old’ flowers not carrying iaaM (Supplementary Figure S3), suggesting that SWEET9 expression in aos-2 × SWEET9pro::iaaM flowers is dependent on endogenous auxin synthesis. These results cumulatively support the hypothesis that endogenous auxin can rescue aos-2 nectar secretion phenotypes downstream of JA.
The JA Receptor COI1 Is Not Required for Nectar Secretion, but Is Required for the Nectary Auxin Response
In light of the apparent dependence of nectar secretion on JA, an interesting phenotype was observed in the ‘old’ flowers of the JA-biosynthesis mutants dad1-1 and opr3-1. Specifically, ‘young’ dad1-1 and opr3-1 flowers behaved similarly to those of the JA-deficient aos-2 by not producing nectar, but their ‘old’ flowers secreted large nectar droplets (Supplementary Figure S2B and Figure 4A), unlike older aos-2 flowers (Figure 3B). However, these results should be considered in the context of the fact that neither dad1-1 nor opr3-1 are completely JA-deficient [there is partial functional redundancy in the case of DAD1 (Ishiguro et al., 2001)] and opr3-1 is a leaky mutant (Chehab et al., 2011). Regardless of this fact, ‘young’ nectarless flowers crossed with DR5::GUS displayed no nectary auxin response, whereas the older flowers with nectar did have a robust auxin response specifically in the nectaries, although only in the median nectaries in the case of opr3-1 (Figure 4A and Supplementary Figure S2B), again reinforcing a connection between auxin signaling and nectar secretion downstream of JA.
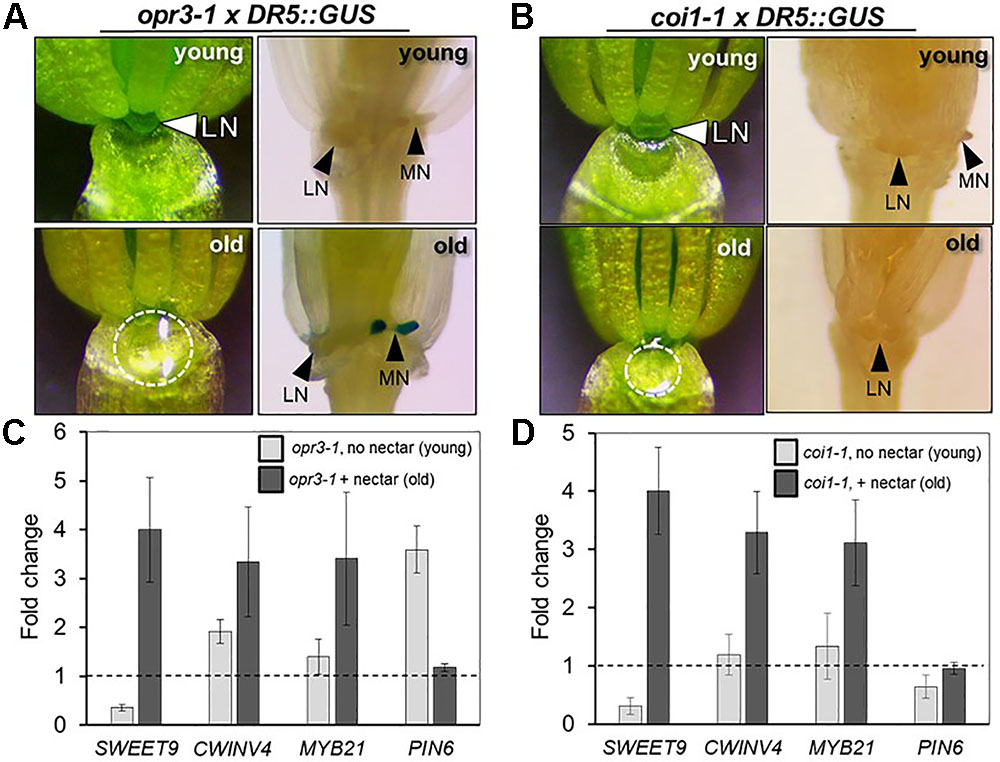
FIGURE 4. The JA receptor COI1 is not required for nectar secretion, but is required for the nectary auxin response. The ‘young’ flowers of opr3-1 (A) and coi1-1 (B) do not produce nectar, whereas the corresponding ‘old’ flowers secrete nectar. ‘Old’ opr3-1 flowers (with nectar) display a strong auxin response in nectaries (A, lower right), but the corresponding ‘old’ coi1-1 flowers with nectar do not (B, lower right). The expression of key genes involved in nectar secretion was examined in whole flowers by qRT-PCR in both ‘young’ (no nectar) and ‘old’ (with nectar) of opr3-1 (C) and coi1-1 (D). qRT data was normalized to Col-0 (dashed line).
Previous reports have shown that the F-box protein CORONATINE INSENSITIVE 1 (COI1), which is a component of a JA receptor critical for JA-mediated signaling, is indispensable for floral starch metabolism and nectar secretion in tobacco flowers (Wang et al., 2014). Therefore, in addition to our JA biosynthesis mutants, we examined the reportedly JA-insensitive coi1-1 in Arabidopsis. As also observed in aos-2 × SWEET9pro::iaaM, dad1-1 and opr3-1, there was a distinct difference in floral nectar secretion between ‘young’ and ‘old’ flowers. ‘Young’ coi1-1 flowers (equivalent to Stage 14 in Col-0), showed a nectar-less phenotype, whereas the ‘old’ flowers did produce nectar (Figure 4B), suggesting the presence of a COI1-independent pathway responsible for regulating certain aspects of nectar secretion in Arabidopsis. Further support for the notion of a COI1-dispensible route for nectar secretion comes from the fact that both exogenous NAA and MeJA induced nectar secretion in ‘young’ coi1-1 flowers (Supplementary Figure S4).
We also examined a mutant of JASMONATE RESISTANT 1 (JAR1) (AT2G46370), jar1-11, which is deficient in the JAR1 enzyme that conjugates JA to isoleucine in order to generate the bioactive JA-Ile. JA-Ile interacts directly with COI1 to mediate JA signal transduction (Staswick and Tiryaki, 2004). Interestingly jar1-11, which has previously been shown to accumulate JA-Ile levels to only 10% of that in WT upon wounding (Suza and Staswick, 2008), still produced nectar (Supplementary Figure S5) suggesting that either the low levels of JA-Ile are adequate for signaling nectar production or that there may be an alternative signaling module involved for nectar secretion.
There were notable changes in the expression of essential genes required for nectar production in the aos-2 mutant that may account for the absence of nectar (Figures 2, 3). We thus hypothesized that a similar pattern of gene expression may be observed in opr3-1 and coi1-1 that may cause the ‘young’ and ‘old’ nectar phenotype. Young nectarless opr3-1 and coi1-1 flowers showed a strong reduction in the expression of SWEET9 (required for nectar production), whereas older flowers with nectar displayed a ∼3-to-4-fold induction of SWEET9, CWINV4, and MYB21 relative to wild-type) (Figures 4C,D). Interestingly, PIN6 expression begins 3.5-fold higher in ‘young’ opr3-1 flowers but drops off to wild-type levels in ‘old’ flowers (Figure 4C). No significant difference in PIN6 (auxin transporter) expression was observed in either the ‘young’ or ‘old’ flowers of coi1-1 relative to Col-0 (Figure 4D). However, coi1-1 × DR5::GUS failed to display an auxin response in either ‘young’ (no nectar) or ‘old’ (with nectar) flowers (Figure 4B). These results imply the presence of a COI1-independent pathway for nectar secretion, but that COI1 is required for the auxin responses observed in the nectaries of other JA-biosynthesis mutants.
JA Responses Are High in Nectaries During Nectar Production as Revealed by a Biosensor
A recently developed JA reporter system can be used to observe jasmonate signaling in planta (Larrieu et al., 2015). In brief, this system was created based on the knowledge that the Jas motif in JAZ proteins is required for their degradation upon JA-induced signaling, thus releasing the repression of JA-inducible genes (Yan et al., 2009; Wasternack and Hause, 2013). The Jas motif of AtJAZ9 was fused with VENUS, a fast maturing YFP and placed under the control of the CaMV 35S promoter. These lines were designated as Jas9-VENUS and robust studies confirmed their use as a JA biosensor (Larrieu et al., 2015). A mutant version, mJas9-VENUS, was also engineered such that it cannot be targeted for degradation by COI1, even in the presence of bioactive JA-Ile (Larrieu et al., 2015). Thus, Jas9-VENUS is rapidly degraded in tissues undergoing active JA responses and an be monitored by a reduction in fluorescence, whereas stabilized mJas9-VENUS is not degraded.
We hypothesized that the JA signaling and response in the nectaries of a Stage 14 flower would be high and thus lead to active degradation of Jas9-VENUS, manifesting as no or very low signal in a Jas9-VENUS line whereas the mJas9-VENUS line would show a nuclear signal in the nectaries. To confirm that the plants being tested indeed had a VENUS-dependent fluorescence signal, 5-day-old seedlings grown on half strength MS media were imaged with a fluorescence microscope. Seedlings with a positive signal for both Jas9- and mJas9-VENUS signal in the roots (Figures 5E–H) were transplanted to soil. Fluorescence imaging of Stage 14 flowers reveal a strong signal in the nuclei of the nectaries of the mJas9-VENUS lines whereas the signal was absent in the Jas9-VENUS nectaries (Figures 5A–D) suggesting that JA signaling was active in nectaries during nectar production. To confirm that the signal was not due to autofluorescence we imaged Stage 14 mJas9-VENUS flower nectaries with a confocal microscope and spectrally unmixed the image to differentiate VENUS from autofluorescence (Supplementary Figure S6). These results indicate that JA is acting as primary signal directly in the nectaries and not in other proximal floral tissues that may be transmitting secondary signals to the nectaries.
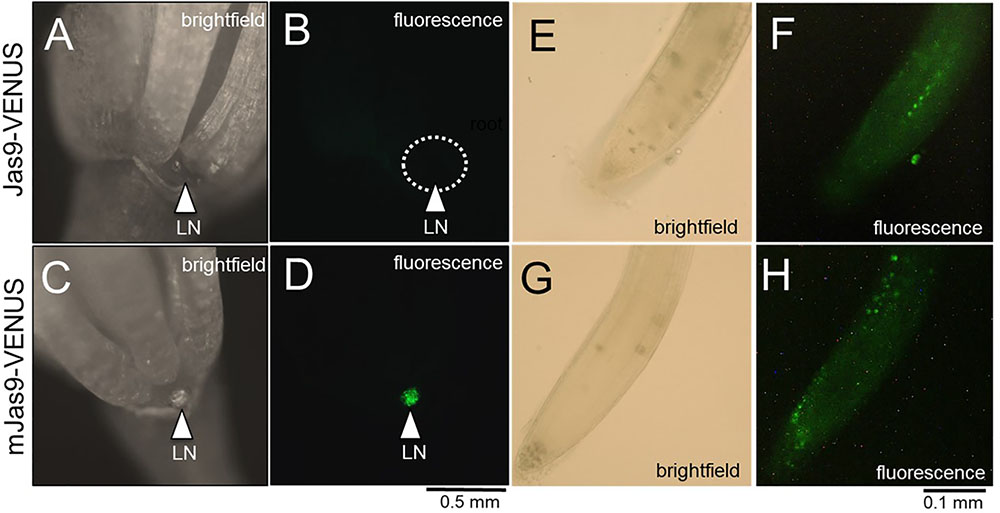
FIGURE 5. The JA response in mature lateral nectaries. Mature lateral nectaries (Stage 14 flowers with nectar) were evaluated for active JA responses via the Jas9-VENUS and mJas9-VENUS system. Jas9-VENUS is degraded in the presence of active JA signaling (decreased fluorescence), whereas mJas9-VENUS is not degraded (stable fluorescence). (A) Jas9::VENUS brightfield and (B) corresponding fluorescence in a Stage 14 nectary. (C) mJas9::VENUS brightfield and (D) corresponding fluorescence image in a Stage 14 nectary. The roots of both Jas9::VENUS (E,F) and mJas9::VENUS (G,H) display strong VENUS-dependent signal (punctate spots represent nuclei in G,H).
MYB21 Is Required for Nectar Production
The transcription factor MYB21 is JA inducible and has been shown to play a critical role in stamen maturation and overall flower development (Stintzi and Browse, 2000; Stracke et al., 2001; Mandaokar et al., 2006; Cheng et al., 2009; Song et al., 2011). More specifically to nectar regulation, MYB305, the tobacco ortholog of MYB21, was reported to directly play a role in nectar production through the regulation of starch metabolism in tobacco flowers (Liu and Thornburg, 2012; Wang et al., 2014). MYB305 mutants accumulate lower levels of starch in their nectaries and have reduced levels of nectar production (Liu and Thornburg, 2012). Reeves et al. (2012) also showed that 20 nectary specific genes were down-regulated in the myb21-5 myb24-5 double mutant. Even with these reports in the literature, there has yet to be a report on the role of MYB21 in Arabidopsis nectary function.
myb21-4 is a null mutant previously described as having a premature stop codon (Trp116∗) (Reeves et al., 2012). As might be expected, the flowers of these mutants produce no nectar (Figure 6A). Perhaps unsurprisingly, nectar production was not rescued by exogenous application of MeJA (Figure 6B) since MYB21 is required downstream of JA signaling (Reeves et al., 2012). To further elucidate what genes MYB21 may be regulating we examined the expression of SWEET9, CWINV4, and PIN6 via qRT-PCR analysis. All three genes showed a severe reduction in expression (>80%) (Figure 6C). Our data supports that the activity of MYB21 in the floral nectary indeed works downstream of JA and has a critical role in regulating the expression of several genes known to be essential for nectar production.
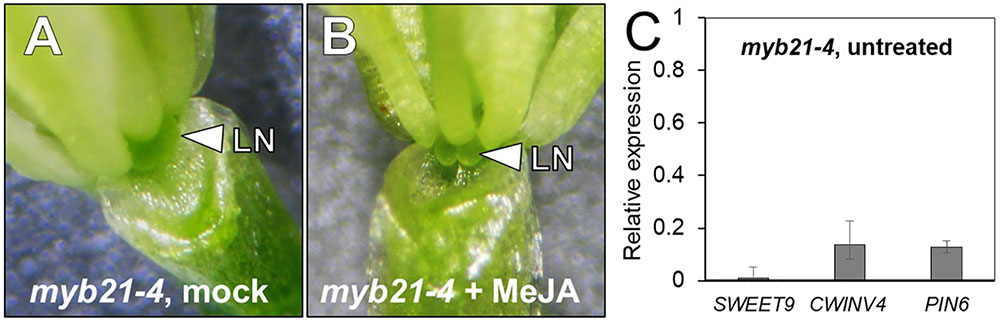
FIGURE 6. MYB21 is required for nectar production. The flowers of mock- and MeJA treated myb21-4 do not produce nectar (A,B). The expression of key genes involved in nectar secretion was examined in whole untreated myb21-4 flowers by qRT PCR (C). Fold-change was calculated relative to Col-0 (wild-type expression = 1).
Starch Metabolism Is Altered in Nectaries of JA Mutants
Starch metabolism has been shown to be important in the process of proper nectary function (Paschold et al., 2008; Ruhlmann et al., 2010; Lin et al., 2014; Wang et al., 2014). Starch buildup is required in nectaries prior to nectar production and is rapidly broken down to produce sucrose and hexoses during the secretory phase. In tobacco, starch metabolism was severely compromised in a coi1 mutant leading to an absence of nectar production (Wang et al., 2014). We hypothesized that aos-2, a JA biosynthetic mutant, would also display defects in starch metabolism. To test this hypothesis, Stage 14 WT and aos-2 flowers were stained with Lugol’s iodine and imaged under a dissecting microscope. The distinct staining of the flower peduncle with iodine suggests a buildup of starch whereas lesser staining is consistent with the breakdown of the starch for nectar production. Also, the stomata on the nectaries stain distinctively when nectar secretion is occurring. Our study correlates a breakdown of starch with nectar secretion in the WT flowers (Figures 7A,B) whereas the aos-2 flower peduncles have increased starch accumulation and a lack of stomatal staining (Figures 7C,D), as expected by the nectarless phenotype. The starch staining patterns observed in aos-2 are very similar to those observed in cwinv4 and sweet9 mutants (Ruhlmann et al., 2010; Lin et al., 2014). Furthermore, since coi1 mutants in tobacco showed defects in nectary starch metabolism and degradation (Wang et al., 2014), we hypothesized there may be starch defects in the Arabidopsis coi1-1 mutant. In the nectarless ‘young’ flowers of coi1-1 we indeed saw strong staining in both the peduncle and the nectary of the flower (Figures 7E,F), whereas in the ‘old’ flowers that do produce nectar, we see some breakdown of the starch in the peduncle and a mobilization of starch products to the guard cells of the nectary stomata (Figures 7G,H). This result confirms JA’s role in starch breakdown and subsequent nectar production.
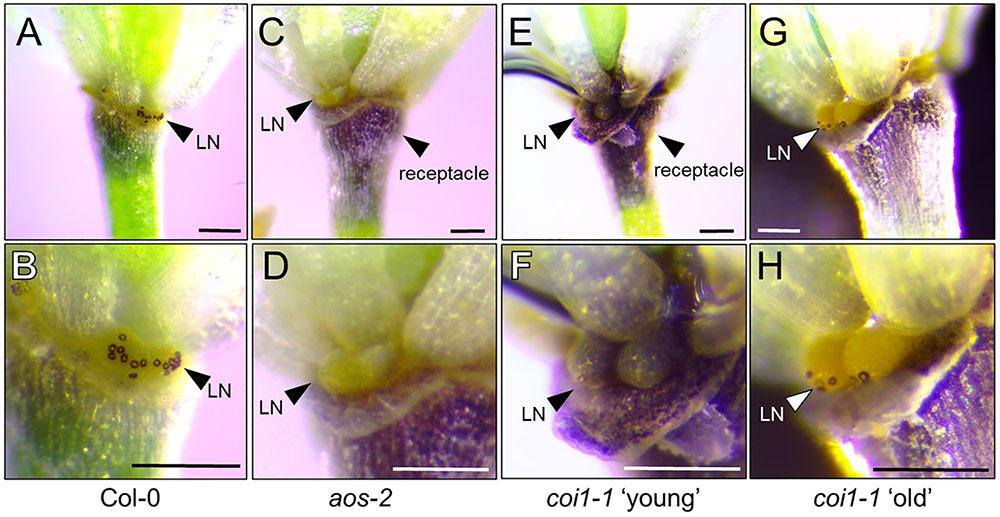
FIGURE 7. Starch accumulation patterns in aos-2 and coi1-1 nectaries. Each pair of images are of the same flower at different magnifications stained for starch accumulation. (A,B) Wild-type Col-0; (C,D) aos-2; (E,F) coi1-1 ‘young’, (G,H) coi1-1 old. Arrowheads either point out the lateral nectaries (LN) or the receptacle. Note the stained stomates in (B,H). Scale bars = 100 microns.
Discussion
The Octadecanoic Acid Biosynthetic Pathway Is Required for Nectary Function
Arabidopsis mutants deficient in the octadecanoic pathway did not secrete nectar (Figures 1–4), particularly in ‘young’ open flowers that are the equivalent of Stage 14 wild-type flowers, which actively secrete nectar. Perhaps most telling, aos-2 did not produce nectar droplets in either ‘young’ or ‘old’ flowers, although exogenous MeJA did restore nectar production (Figures 1, 2). AOS is a single copy gene indispensable for the jasmonate biosynthesis pathway. Interestingly, the ‘old’ flowers of dad1-1 and opr3-1 did eventually produce nectar, which coincided with the expression of SWEET9, a sucrose transporter indispensable for nectar secretion (Lin et al., 2014). These results could possibly be explained by the fact that neither dad1-1 nor opr3-1 are completely JA deficient. DAD1 encodes a phospholipase with extensive functional redundancy, as demonstrated by the fact that dad1-1 mutants are defective in anther dehiscence and pollen development but are still able to accumulate JA upon wounding via the DAD1 leaf homolog DONGLE (Ishiguro et al., 2001; Hyun et al., 2008). OPR3 is reportedly essential for JA synthesis, but opr3-1 has a leaky phenotype when exposed to pathogen attack (Chehab et al., 2011). It was also recently reported that in the absence of OPR3, OPDA could enter the β-oxidation route to produce 4,5-didehydro-JA which can subsequently act as a precursor to JA and JA-Ile (Chini et al., 2018). These results perhaps suggest that JA may still be able to accumulate to sufficient concentrations in dad1-1 and opr3-1 to restore the necessary signaling to rescue nectar secretion. However, it is important to note that anther dehiscence was not observed in any of the JA synthesis or response mutants, in either young or old flowers (data not shown). These results suggest that JA is not accumulating to high levels in these mutants and indicate that anther dehiscence is not required for nectar production.
COI1 Is Dispensable for Nectar Secretion in Arabidopsis
The presence of nectar in ‘old’ opr3-1 and coi1-1 flowers (Figure 4) could also indicate the presence of an octadecanoic acid-dependent but JA- and COI1-dispensable signaling pathway in relation to nectary function. The substrate for OPR3 is cis-OPDA, which accumulates in opr3-1 instead of being converted to JA (Supplementary Figure S1). cis-OPDA has been reported to be a potent signaling molecule that can regulate gene expression in either a COI1-dependent or COI-independent fashion (Dave and Graham, 2012). For JA responses to occur, JA-Ile is usually rapidly synthesized in response to an environmental or developmental cue which then binds the F-box protein COI1, which targets JAZ repressors for degradation via the 26S proteasome (Sheard et al., 2010; Pérez and Goossens, 2013; Wasternack and Hause, 2013). This subsequently liberates the transcription factors that drive JA-related gene expression. In the jar1-11 mutant, where JA-Ile levels are severely compromised (Suza and Staswick, 2008), nectar production proceeds normally in ‘young’ flowers (Supplementary Figure S5). This suggests that the low levels of JA-Ile might suffice for nectar production or that nectar production might not require the well-established COI1-dependent JA regulation pathway in Arabidopsis. This latter supposition further gains support from the observation that nectar production proceeds normally in older flowers of the coi1-1 loss-of-function mutant. It is remarkable though that younger coi1-1 flowers produced no nectar even at Stages 13–14 when nectar secretion normally begins, indicating a COI1-dispensible pathway might require a time lag in responding to OPDA- or JA-dependent signaling. This delayed nectar phenotype is perhaps even more interesting in light of the reports that JA-Ile/COI1 signaling in wild tobacco flowers (Nicotiana attenuata) is required for nectar production (Stitz et al., 2014). Overall, this discrepancy should be further explored across species to better understand the conserved processes that are essential for nectar regulation.
MYB21 Is the Apparent Ortholog of Tobacco MYB305
MYB21 is a JA-responsive transcription factor previously reported to be important for stamen elongation and floral maturation (Reeves et al., 2012). It is also closely related to tobacco MYB305 (NtMYB305) (Ren et al., 2007), which is required for nectar production and the expression of nectary-specific genes (Liu et al., 2009; Liu and Thornburg, 2012). In our study, MYB21 expression was enhanced in aos-2 flowers exogenously treated with MeJA, supporting its JA-inducibility (Figure 2). myb21-4 flowers also did not produce nectar and also lacked the expression of genes required for nectar production – AtSWEET9, AtCWINV4, and AtPIN6 all displayed large decreases in expression in myb21-4 (Figure 6). SWEET9 acts as a sucrose transporter, putatively transporting sucrose out of nectary parenchyma cells (Lin et al., 2014). After sucrose export, CWINV4 converts this disaccharide into its hexose monomers, glucose and fructose (Ruhlmann et al., 2010). Null mutants for these genes phenocopy one another as neither produce nectar, while maintaining normal nectary structure, and have heavy starch accumulation in the floral receptacle (Ruhlmann et al., 2010; Lin et al., 2014). The regulation of these nectar sugar transporters and invertases is vital for proper nectar production and secretion. Thus, MYB21 appears to regulate both CWINV4 and SWEET9 expression, in addition to PIN6, but it is currently unknown if MYB21 directly or indirectly controls their expression. It was interesting to note the expression of SWEET9 was strongly reduced in the younger flowers of JA mutants such as aos-2, opr3-1, and coi1-1 but gradually increased in the older flowers or in response to endogenous and exogenous auxin. These results suggest that SWEET9 expression proceeds via a COI1-dispensable, but MYB21-dependent route (Figure 8).
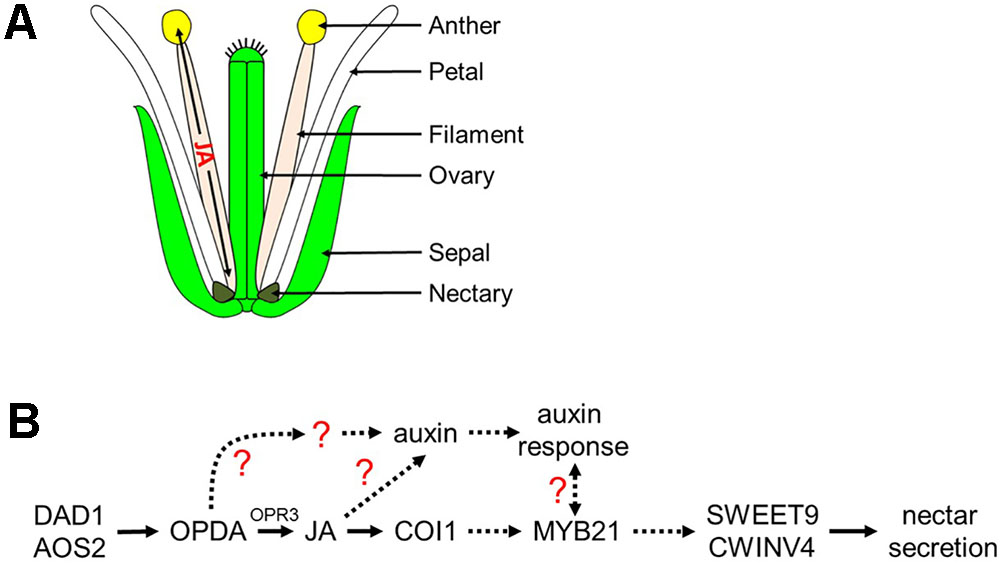
FIGURE 8. Proposed model of jasmonic acid migration after synthesis in the filament and regulation of nectar secretion. (A) JA synthesized in the anther filament migrates down the filament to the nectary to promote nectar secretion, and travels to the anther where it promotes the dehiscence of anthers and pollen maturation. (B) JA regulation of nectar secretion. Under standard conditions, JA or its precursors are synthesized in the filament leading to the induction of MYB21 expression which in turn is required for nectar secretion via the expression of SWEET9 and CWINV4. JA also regulates auxin responses within the nectaries, another hormonal factor involved in nectar secretion. A possible role for a JA- and COI1-dispensible pathway via OPDA for nectar secretion is indicated; dashed arrows represent hypothetical interactions based on limited data.
Crosstalk Between JA and Auxin Pathways
There are a number of well-known interactions between auxin (IAA) and JA in plants, both in terms of homeostasis and downstream response (Pérez and Goossens, 2013; Wasternack and Hause, 2013). One of the key discoveries of our study was a further elucidation for the role of JA during nectar production and how it coordinates with auxin responses. Our study reveals that treatment of flowers with exogenous synthetic auxin (1-NAA) can rescue nectar production defects in JA biosynthetic mutants such as aos-2 and dad1-1, which fail to produce nectar in ‘young’ open flowers (Figure 2). We also found that auxin responses in the nectaries of the ‘young’ open flowers of dad1-1, aos-2 and opr3-1 were absent as revealed by the DR5::GUS auxin reporter system. This suggests that the octadecanoic acid biosynthetic pathway is required for nectary auxin responses and subsequent nectar production. Auxin responses reappeared in aos-2 flowers treated with MeJA suggesting that jasmonates can increase auxin biosynthesis in the nectaries. It remains to be tested whether free auxin levels are indeed diminished in the mutant flowers and whether they do increase after the MeJA treatment. However, we did demonstrate that nectary-derived auxin biosynthesis (via SWEET9pro::iaaM) can rescue nectar production in the aos-2 background, likely by inducing SWEET9 expression (Figure 3). Cumulatively, these results indicate that auxin acts downstream of JA to induce nectar secretion.
The complete absence of an auxin response in coi1-1 nectaries, even after nectar production occurred in older flowers, is particularly interesting (Figure 4). The possibility that nectar production occurs independently of COI1 in Arabidopsis has been discussed previously. This suggests that nectar production and a canonical auxin response via the TIR1 pathway in the nectary are separable events. Both auxin and JA use a similar mechanism of signaling by forming specialized co-receptor complexes known as SKP1-Cullin-F-box protein (SCF) E3 ubiquitin ligase complexes. TIR1 and COI1 act as the F-box proteins providing specificity for auxin and JA in these complexes, respectively. This complex subsequently binds their target proteins, Aux/IAA (auxin) and JAZ (JA), respectively, which are then degraded via the ubiquitin-proteasome system (Kelley and Estelle, 2012) thus allowing hormone specific transcriptional activation to proceed. Both these hormones are perceived by shared components of a SCF-E3 ligase system, and it has been demonstrated that disruption in the complexes can cause an impairment of hormonal responses (del Pozo et al., 2002; Quint et al., 2005; Moon et al., 2007; Pérez and Goossens, 2013). Thus, there is a possibility that the loss of COI1 disrupts the equilibrium of the shared aspects of the ubiquitin-proteasome system thus affecting auxin signaling and responses too.
An Alternative Mechanism of JA Involvement in Nectar Production?
We should also consider alternative routes of how jasmonates and related oxylipins might affect nectar production. A potential for JA regulation of nectar secretion via control of water movement also exists. Proper timing of pollen release and nectar secretion in flowering plants can be vital for reproduction, therefore, there may be a close singular upstream mechanism between anther dehiscence and nectar secretion. Baum et al. (2001) characterized many male sterile mutants to be deficient in nectar production. This same correlation between anther dehiscence/male-sterility and nectar production was observed in different male sterile mutants of our study. Ishiguro et al. (2001) determined that water transport was halted in the vascular tissue of dad1-1 anthers and suggested that JA regulates water transport in the male organs. Later, Ruhlmann et al. (2010) suggested that the high amount of nectar sugars leads to the flow of water out of the nectary, creating the nectar droplet presented at the base of the sepal. Perhaps water transport in the nectaries is disrupted by the lack of JA which leads to the absence of nectar in the JA synthesis and response mutants. Therefore, only when the proper JA response can be restored in the JA synthesis mutants can the water transport/nectar secretion be restored as well. However, the molecular mechanism by which water flow is disrupted to dad1-1 stamens, and perhaps nectaries, is currently unknown.
Cumulatively, the results from this study implicate the octadecanoic acid biosynthetic pathway and auxin as indispensable regulators of nectar secretion independent of COI1 in Arabidopsis. We propose a model (Figure 8) in which cis-OPDA may act as a signaling molecule upstream of MYB21 and auxin responses leading to the expression of SWEET9 and CWINV4. Still, certain aspects of the proposed model are unclear, particularly the connection between the auxin response and MYB21 (dashed line with question mark), as exogenous treatment of aos-2 flowers with NAA induced SWEET9 expression and nectar secretion while concurrently downregulating MYB21 expression (Figure 2). A possible explanation for the apparent discrepancy between MYB21 and SWEET9 expression in auxin-treated aos-2 flowers is that qRT-PCR analyses were done with whole flowers (due to the extremely small size of Arabidopsis nectaries) and MYB21 is not a nectary-specific gene (Reeves et al., 2012), unlike SWEET9 and CWINV4 (Ruhlmann et al., 2010; Lin et al., 2014). Therefore, it is possible that MYB21 expression might be downregulated in non-nectary tissues of NAA-treated aos-2 flowers, while actually being upregulated in nectaries. Further studies will focus on examining this crosstalk and IAA, JA, and MYB21 in nectar secretion, as well as their respective control of CWINV4 and SWEET9 expression.
Author Contributions
AS, RR, PK, MJ, and CC designed and executed the experiments. AS, RR and CC wrote the final manuscript.
Funding
This work was supported by grants from the United States National Science Foundations to CC (1339246 and 0820730).
Conflict of Interest Statement
The authors declare that the research was conducted in the absence of any commercial or financial relationships that could be construed as a potential conflict of interest.
Acknowledgments
We would like to thank Dr. Jason Reed for supplying mutant seeds for the study. We also thank the University Imaging Centers at the University of Minnesota for experimental and technical support (http://uic.umn.edu/).
Supplementary Material
The Supplementary Material for this article can be found online at: https://www.frontiersin.org/articles/10.3389/fpls.2018.01060/full#supplementary-material
References
Aloni, R., Aloni, E., Langhans, M., and Ullrich, C. I. (2006). Role of auxin in regulating Arabidopsis flower development. Planta 223, 315–328. doi: 10.1007/s00425-005-0088-9
Bender, R., Klinkenberg, P., Jiang, Z., Bauer, B., Karypis, G., Nguyen, N., et al. (2012). Functional genomics of nectar production in the Brassicaceae. Flora Morphol. Distrib. Funct. Ecol. Plants 207, 491–496. doi: 10.1016/j.flora.2012.06.005
Baum, S. F., Eshed, Y., and Bowman, J. L. (2001). The Arabidopsis nectary is an ABC-independent floral structure. Development 128, 4657–4667.
Bender, R. L., Fekete, M. L., Klinkenberg, P. M., Hampton, M., Bauer, B., Malecha, M., et al. (2013). PIN6 is required for nectary auxin response and short stamen development. Plant J. 74, 893–904. doi: 10.1111/tpj.12184
Calderone, N. W. (2012). Insect pollinated crops, insect pollinators and US agriculture: trend analysis of aggregate data for the period 1992-2009. PLoS One 7:e37235. doi: 10.1371/journal.pone.0037235
Chapman, E. J., and Estelle, M. (2009). Mechanism of auxin-regulated gene expression in plants. Annu. Rev. Genet. 43, 265–285. doi: 10.1146/annurev-genet-102108-134148
Chehab, E. W., Kim, S., Savchenko, T., Kliebenstein, D., Dehesh, K., and Braam, J. (2011). Intronic T-DNA insertion renders Arabidopsis opr3 a conditional jasmonic acid-producing mutant. Plant Physiol. 156, 770–778. doi: 10.1104/pp.111.174169
Cheng, H., Song, S., Xiao, L., Soo, H. M., Cheng, Z., Xie, D., et al. (2009). Gibberellin acts through jasmonate to control the expression of MYB21, MYB24, and MYB57 to promote stamen filament growth in Arabidopsis. PLoS Genet. 5:e1000440. doi: 10.1371/journal.pgen.1000440
Chini, A., Monte, I., Zamarreño, A. M., Hamberg, M., Lassueur, S., Reymond, P., et al. (2018). An OPR3-independent pathway uses 4,5-didehydrojasmonate for jasmonate synthesis. Nat. Chem. Biol. 14, 171–178. doi: 10.1038/nchembio.2540
Clough, S. J., and Bent, A. F. (1998). Floral dip: a simplified method for Agrobacterium-mediated transformation of Arabidopsis thaliana. Plant J. 16, 735–743. doi: 10.1046/j.1365-313X.1998.00343.x
Dave, A., and Graham, I. A. (2012). Oxylipin signaling: a distinct role for the jasmonic acid precursor cis-(+)-12-oxo-phytodienoic acid (cis-OPDA). Front. Plant Sci. 3:42. doi: 10.3389/fpls.2012.00042
Davis, A. R., Pylatuik, J. D., Paradis, J. C., and Low, N. H. (1998). Nectar-carbohydrate production and composition vary in relation to nectary anatomy and location within individual flowers of several species of Brassicaceae. Planta 205, 305–318. doi: 10.1007/s004250050325
del Pozo, J. C., Dharmasiri, S., Hellmann, H., Walker, L., Gray, W. M., and Estelle, M. (2002). AXR1-ECR1-dependent conjugation of RUB1 to the Arabidopsis Cullin AtCUL1 is required for auxin response. Plant Cell 14, 421–433. doi: 10.1105/tpc.010282
Dharmasiri, N., Dharmasiri, S., and Estelle, M. (2005). The Arabidopsis F-box protein TIR1 is an auxin receptor. Nature 435, 446–451. doi: 10.1038/nature03542
Hampton, M., Xu, W. W., Kram, B. W., Chambers, E. M., Ehrnriter, J. S., Gralewski, J. H., et al. (2010). Identification of differential gene expression in Brassica rapa nectaries through expressed sequence tag analysis. PLoS One 5:e8782. doi: 10.1371/journal.pone.0008782
Heil, M. (2001). Extrafloral nectar production of the ant-associated plant, Macaranga tanarius, is an induced, indirect, defensive response elicited by jasmonic acid. Proc. Natl. Acad. Sci. U.S.A. 98, 1083–1088. doi: 10.1073/pnas.031563398
Heil, M. (2011). Nectar: generation, regulation and ecological functions. Trends Plant Sci. 16, 191–200. doi: 10.1016/j.tplants.2011.01.003
Hoffman, M. H., Bremer, M., Schneider, K., Burger, F., Stolle, E., and Moritz, G. (2003). Flower visitors in a natural population of Arabidopsis thaliana. Plant Biol. 5, 491–494.
Hyun, Y., Choi, S., Hwang, H. J., Yu, J., Nam, S. J., Ko, J., et al. (2008). Cooperation and functional diversification of two closely related galactolipase genes for jasmonate biosynthesis. Dev. Cell 14, 183–192. doi: 10.1016/j.devcel.2007.11.010
Ishiguro, S., Kawai-Oda, A., Ueda, J., Nishida, I., and Okada, K. (2001). The DEFECTIVE IN ANTHER DEHISCIENCE gene encodes a novel phospholipase A1 catalyzing the initial step of jasmonic acid biosynthesis, which synchronizes pollen maturation, anther dehiscence, and flower opening in Arabidopsis. Plant Cell 13, 2191–2209. doi: 10.1105/tpc.010192
Jefferson, R. A., Kavanagh, T. A., and Bevan, M. W. (1987). GUS fusions: beta-glucuronidase as a sensitive and versatile gene fusion marker in higher plants. EMBO J. 6, 3901–3907. doi: 10.1073/pnas.1411926112
Kelley, D. R., and Estelle, M. (2012). Ubiquitin-mediated control of plant hormone signaling. Plant Physiol. 160, 47–55. doi: 10.1104/pp.112.200527
Klee, H. J., Horsch, R. B., Hinchee, M. A., Hein, M. B., and Hoffmann, N. L. (1987). The effects of overproduction of two Agrobacterium tumefaciens T-DNA auxin biosynthetic gene products in transgenic petunia plants. Genes Dev. 1, 86–96. doi: 10.1101/gad.1.1.86
Klein, A.-M., Vaissiere, B. E., Cane, J. H., Steffan-Dewenter, I., Cunningham, S. A., Kremen, C., et al. (2007). Importance of pollinators in changing landscapes for world crops. Proc. R. Soc. B Biol. Sci. 274, 303–313. doi: 10.1098/rspb.2006.3721
Kram, B. W., and Carter, C. J. (2009). Arabidopsis thaliana as a model for functional nectary analysis. Sex. Plant Reprod. 22, 235–246. doi: 10.1007/s00497-009-0112-115
Kram, B. W., Xu, W. W., and Carter, C. J. (2009). Uncovering the Arabidopsis thaliana nectary transcriptome: investigation of differential gene expression in floral nectariferous tissues. BMC Plant Biol. 9:92. doi: 10.1186/1471-2229-9-92
Larrieu, A., Champion, A., Legrand, J., Lavenus, J., Mast, D., Brunoud, G., et al. (2015). A fluorescent hormone biosensor reveals the dynamics of jasmonate signalling in plants. Nat. Commun. 6:6043. doi: 10.1038/ncomms7043
Leyser, O. (2010). The power of auxin in plants. Plant Physiol. 154, 501–505. doi: 10.1104/pp.110.161323
Lin, I. W., Sosso, D., Chen, L., Gase, K., Kim, S., Kessler, D., et al. (2014). Nectar secretion requires sucrose phosphate synthases and the sugar transporter SWEET9. F1000 Prime 508, 546–549. doi: 10.1038/nature13082
Liu, G., Ren, G., Guirgis, A., and Thornburg, R. W. (2009). The MYB305 transcription factor regulates expression of nectarin genes in the ornamental tobacco floral nectary. Plant Cell 21, 2672–2687. doi: 10.1105/tpc.108.060079
Liu, G., and Thornburg, R. W. (2012). Knockdown of MYB305 disrupts nectary starch metabolism and floral nectar production. Plant J. 9, 377–388. doi: 10.1111/j.1365-313X.2011.04875.x
Lokerse, A. S., and Weijers, D. (2009). Auxin enters the matrix - assembly of response machineries for specific outputs. Curr. Opin. Plant Biol. 12, 520–526. doi: 10.1016/j.pbi.2009.07.007
Mandaokar, A., Thines, B., Shin, B., Lange, M. B., Choi, G., Koo, Y. J., et al. (2006). Transcriptional regulators of stamen development in Arabidopsis identified by transcriptional profiling. Plant J. 46, 984–1008. doi: 10.1111/j.1365-313X.2006.02756.x
Moon, J., Zhao, Y., Dai, X., Zhang, W., Gray, W. M., Huq, E., et al. (2007). A new CULLIN 1 mutant has altered responses to hormones and light in Arabidopsis. Plant Physiol. 143, 684–696. doi: 10.1104/pp.106.091439
Nagels Durand, A., Pauwels, L., and Goossens, A. (2016). The ubiquitin system and jasmonate signaling. Plants 5:E6. doi: 10.3390/plants5010006
Nagpal, P., Ellis, C. M., Weber, H., Ploense, S. E., Barkawi, L. S., Guilfoyle, T. J., et al. (2005). Auxin response factors ARF6 and ARF8 promote jasmonic acid production and flower maturation. Development 132, 4107–4118. doi: 10.1242/dev.01955
Park, J. H., Halitschke, R., Kim, H. B., Baldwin, I. T., Feldmann, K. A., and Feyereisen, R. (2002). A knock-out mutation in allene oxide synthase results in male sterility and defective wound signal transduction in Arabidopsis due to a block in jasmonic acid biosynthesis. Plant J. 31, 1–12. doi: 10.1046/j.1365-313X.2002.01328.x
Paschold, A., Bonaventure, G., Kant, M. R., and Baldwin, I. T. (2008). Jasmonate perception regulates jasmonate biosynthesis and JA-Ile metabolism: the case of COI1 in Nicotiana attenuata. Plant Cell Physiol. 49, 1165–1175. doi: 10.1093/pcp/pcn091
Pérez, A. C., and Goossens, A. (2013). Jasmonate signalling: a copycat of auxin signalling? Plant Cell Environ. 36, 2071–2084. doi: 10.1111/pce.12121
Pyke, G. H. (2016). Floral Nectar: pollinator attraction or manipulation? Trends Ecol. Evol. 31, 339–341. doi: 10.1016/j.tree.2016.02.013
Quint, M., Ito, H., Zhang, W., and Gray, W. M. (2005). Characterization of a novel temperature-sensitive allele of the CUL1/AXR6 subunit of SCF ubiquitin-ligases. Plant J. 31, 1713–1723. doi: 10.1109/TMI.2012.2196707
Radhika, V., Kost, C., Boland, W., and Heil, M. (2010). The role of jasmonates in floral nectar secretion. PLoS One 5:e9265. doi: 10.1371/journal.pone.0009265
Reeves, P. H., Ellis, C. M., Ploense, S. E., Wu, M. F., Yadav, V., Tholl, D., et al. (2012). A regulatory network for coordinated flower maturation. PLoS Genet. 8:e1002506. doi: 10.1371/journal.pgen.1002506
Ren, G., Healy, R. A., Klyne, A. M., Horner, H. T., James, M. G., and Thornburg, R. W. (2007). Transient starch metabolism in ornamental tobacco floral nectaries regulates nectar composition and release. Plant Sci. 173, 277–290. doi: 10.1016/j.plantsci.2007.05.008
Roy, R., Schmitt, A. J., Thomas, J. B., and Carter, C. J. (2017). Review: nectar biology: from molecules to ecosystems. Plant Sci. 262, 148–164. doi: 10.1016/j.plantsci.2017.04.012
Ruhlmann, J. M., Kram, B. W., and Carter, C. J. (2010). CELL WALL INVERTASE 4 is required for nectar production in Arabidopsis. J. Exp. Bot. 61, 395–404. doi: 10.1093/jxb/erp309
Sheard, L. B., Tan, X., Mao, H., Withers, J., Ben-Nissan, G., Hinds, T. R., et al. (2010). Jasmonate perception by inositol-phosphate-potentiated COI1-JAZ co-receptor. Nature 468, 400–405. doi: 10.1038/nature09430
Song, S., Qi, T., Huang, H., Ren, Q., Wu, D., Chang, C., et al. (2011). The jasmonate-ZIM domain proteins interact with the R2R3-MYB transcription factors MYB21 and MYB24 to affect jasmonate-regulated stamen development in Arabidopsis. Plant Cell 23, 1000–1013. doi: 10.1105/tpc.111.083089
Staswick, P. E., and Tiryaki, I. (2004). The oxylipin signal jasmonic acid is activated by an enzyme that conjugates it to isoleucine in Arabidopsis. Plant Cell 16, 2117–2127. doi: 10.1105/tpc.104.023549.1
Stintzi, A., and Browse, J. (2000). The Arabidopsis male-sterile mutant, opr3, lacks the 12-oxophytodienoic acid reductase required for jasmonate synthesis. Proc. Natl. Acad. Sci. U.S.A. 97, 10625–10630. doi: 10.1073/pnas.190264497
Stitz, M., Hartl, M., Baldwin, I. T., and Gaquerel, E. (2014). Jasmonoyl-l-Isoleucine coordinates metabolic networks required for anthesis and floral attractant emission in wild tobacco (Nicotiana attenuata). Plant Cell 26, 3964–3983. doi: 10.1105/tpc.114.128165
Stracke, R., Werber, M., and Weisshaar, B. (2001). The R2R3-MYB gene family in Arabidopsis thaliana. Curr. Opin. Plant Biol. 4, 447–456. doi: 10.1016/S1369-5266(00)00199-0
Suza, W. P., and Staswick, P. E. (2008). The role of JAR1 in Jasmonoyl-l-isoleucine production during Arabidopsis wound response. Planta 227, 1221–1232. doi: 10.1007/s00425-008-0694-4
Ulmasov, T., Murfett, J., Hagen, G., and Guilfoyle, T. J. (1997). Aux/lAA proteins repress expression of reporter genes containing natural and highly active synthetic auxin response elements. Plant Cell 9, 1963–1971. doi: 10.1105/tpc.9.11.1963
Varaud, E., Brioudes, F., Szecsi, J., Leroux, J., Brown, S., Perrot-Rechenmann, C., et al. (2011). AUXIN RESPONSE FACTOR8 regulates Arabidopsis petal growth by interacting with the bHLH transcription factor BIGPETALp. Plant Cell 23, 973–983. doi: 10.1105/tpc.110.081653
Wang, W., Liu, G., Niu, H., Timko, M. P., and Zhang, H. (2014). The F-box protein COI1 functions upstream of MYB305 to regulate primary carbohydrate metabolism in tobacco (Nicotiana tabacum L. cv. TN90). J. Exp. Bot. 65, 2147–2160. doi: 10.1093/jxb/eru084
Wasternack, C., and Hause, B. (2013). Jasmonates: biosynthesis, perception, signal transduction and action in plant stress response, growth and development. An update to the 2007 review in annals of botany. Ann. Bot. 111, 1021–1058. doi: 10.1093/aob/mct067
Weijers, D., Nemhauser, J., and Yang, Z. (2018). Auxin: small molecule, big impact. J. Exp. Bot. 69, 133–136.
Wiesen, L. B., Bender, R. L., Paradis, T., Larson, A., Perera, M. A. D. N., Nikolau, B. J., et al. (2016). A Role for GIBBERELLIN 2-OXIDASE6 and gibberellins in regulating nectar production. Mol. Plant 9, 753–756. doi: 10.1016/j.molp.2015.12.019
Yan, J., Zhang, C., Gu, M., Bai, Z., Zhang, W., Qi, T., et al. (2009). The Arabidopsis CORONATINE INSENSITIVE1 protein is a jasmonate receptor. Plant Cell 21, 2220–2236. doi: 10.1105/tpc.109.065730
Keywords: nectar, nectaries, jasmonic acid, auxin, F-box proteins, SWEET9
Citation: Schmitt AJ, Roy R, Klinkenberg PM, Jia M and Carter CJ (2018) The Octadecanoid Pathway, but Not COI1, Is Required for Nectar Secretion in Arabidopsis thaliana. Front. Plant Sci. 9:1060. doi: 10.3389/fpls.2018.01060
Received: 31 March 2018; Accepted: 29 June 2018;
Published: 08 August 2018.
Edited by:
Stefan de Folter, Centro de Investigación y de Estudios Avanzados del Instituto Politécnico Nacional (CINVESTAV-IPN), MexicoReviewed by:
Paul Staswick, University of Nebraska–Lincoln, United StatesChristiane Gatz, Georg-August-Universität Göttingen, Germany
Copyright © 2018 Schmitt, Roy, Klinkenberg, Jia and Carter. This is an open-access article distributed under the terms of the Creative Commons Attribution License (CC BY). The use, distribution or reproduction in other forums is permitted, provided the original author(s) and the copyright owner(s) are credited and that the original publication in this journal is cited, in accordance with accepted academic practice. No use, distribution or reproduction is permitted which does not comply with these terms.
*Correspondence: Clay J. Carter, Y2pjYXJ0ZXJAdW1uLmVkdQ==
†These authors have contributed equally to this work.
‡Present address: Mengyuan Jia, Huck Institutes of Life Sciences, Pennsylvania State University, University Park, State College, PA, United States