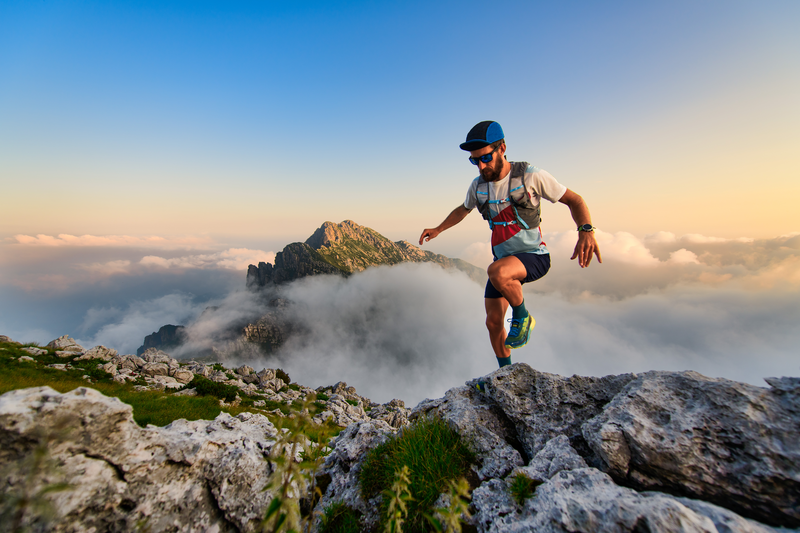
95% of researchers rate our articles as excellent or good
Learn more about the work of our research integrity team to safeguard the quality of each article we publish.
Find out more
SYSTEMATIC REVIEW article
Front. Pharmacol. , 11 September 2024
Sec. Ethnopharmacology
Volume 15 - 2024 | https://doi.org/10.3389/fphar.2024.1452545
This article is part of the Research Topic Efficacy and Mechanism of Herbal Medicines and Their Functional Compounds in Preventing and Treating Cardiovascular Diseases and Cardiovascular Disease Risk Factors - Volume II View all 12 articles
Background: Salvianolic acid B is the most abundant water-soluble component in the traditional Chinese medicine Danshen and can reduce myocardial ischemia-reperfusion (MI/R) injury through multiple targets and pathways. However, the role of SalB in protecting the myocardium from ischemia/reperfusion injury remains unclear.
Purpose: To perform a preclinical systematic review and meta-analysis to assess the efficacy of Sal B in an animal model of myocardial infarction/reperfusion (MI/R) and to summarize the potential mechanisms of Sal B against MI/R.
Methods: Studies published from inception to March 2024 were systematically searched in PubMed, Web of Science, Embase, China National Knowledge Infrastructure Wanfang, and VIP databases. The methodological quality was determined using the SYRCLE RoB tool. The R software was used to analyze the data. The potential mechanisms are categorized and summarized.
Results: 32 studies containing 732 animals were included. The results of the meta-analysis showed that Sal B reduced myocardial infarct size (p < 0.01), and the cardiological indices of CK-MB (p < 0.01), CK (p < 0.01), LDH (p < 0.01), and cTnI (p < 0.01) compared to the control group. In addition, Sal B increased cardiac function indices, such as LVFS (p < 0.01), -dp/dt max (p < 0.01), +dp/dt max (p < 0.01), and cardiac output (p < 0.01). The protective effects of Sal B on the myocardium after I/R may be mediated by attenuating oxidative stress and inflammation, promoting neovascularization, regulating vascular function, and attenuating cardiac myocyte apoptosis. Publication bias was observed in all the included studies. Further studies are required to elucidate the extent of the cardioprotective effects of SalB and the safety of its use.
Conclusion: To the best of our knowledge, this is the first meta-analysis of Sal B in the treatment of MI/R injury, and Sal B demonstrated a positive effect on MI/R injury through the modulation of key pathological indicators and multiple signaling pathways. Further studies are needed to elucidate the extent to which SalB exerts its cardioprotective effects and the safety of its use.
Systematic Review Registration: https://www.crd.york.ac.uk/PROSPERO/.
Myocardial infarction (MI) is caused by rupture or erosion of the epicardial coronary artery, coronary artery thrombosis, or atherosclerotic plaque formation, resulting in stenosis or occlusion of the coronary artery, leading to ischemia and hypoxia in the area of the myocardium supplied by the occluded artery. Severe and persistent ischemia and hypoxia cause irreversible damage to the myocardium, leading to death (Reed et al., 2017). According to statistics, more than seven million people worldwide are diagnosed with acute myocardial infarction (AMI) each year, and approximately 2.4 million people die of acute myocardial infarction (AMI) each year in the United States alone, resulting in an enormous health and economic burden (Yeh et al., 2010). Prompt reperfusion is the only way to save ischemic myocardium in myocardial infarction. Reperfusion has been shown to limit infarct size, improve long-term myocardial function, change the healing pattern of infarcted areas, and, more importantly, reduce mortality (Hausenloy et al., 2017). Data from France showed that between 1995 and 2015, the use of reperfusion therapy increased from 82% to 49%, whereas the 1-year mortality rate of reperfusion-treated patients decreased from 11.9% to 5.9% (Puymirat et al., 2019). Much experimental and clinical evidence supports the hypothesis that reperfusion causes additional myocardial damage. Neutrophil aggregation, calcium overload or redistribution, impaired mitochondrial energy synthesis, and burst production of oxygen free radicals occur during the reperfusion of coronary blood to ischemic tissues, further inducing myocardial injury and cardiomyocyte death, known as reperfusion injury (Heusch and Gersh, 2017). This has resulted in the current clinical dilemma that the mortality and morbidity associated with heart failure due to AMI remain high despite the increased use of reperfusion and improved methods (Sánchez-Hernández et al., 2020). Therefore, there is an urgent need for an effective therapy that can target myocardial reperfusion injury and reduce the size of the myocardial infarction. Myocardial ischemia/reperfusion (MI/R) injury is a complex pathology involving several mechanisms and molecules that cause damage to the heart. However, there are currently no satisfactory therapeutic strategies for mitigating myocardial ischemia-reperfusion injury, which may be since targeting one mechanism at a time may not be sufficient to produce a robust effect in a clinical situation where many uncontrolled variables commonly coexist (Heusch, 2020). Therefore, a multitargeted agent may be a potential avenue for addressing MI/R.
Danshen, a traditional Chinese medicine, is widely used in the treatment of angina pectoris, gastric pain, arthralgia, and menstrual disorders and is the main ingredient of Compound Danshen Dripping Pills, Danshen injections, and other medicines in the clinic (Li et al., 2018). The pharmacological effects of Salvia miltiorrhiza are mainly based on water-soluble components such as salicylic acid and fat-soluble components such as tanshinone, among which salvianolic acid B(Sal B) is the water-soluble component with the highest content (Wei et al., 2023). In recent years, researchers have focused on this promising small-molecule compound, and more preclinical studies on Sal B have been conducted. These studies suggest that Sal B exerts protective effects on ischemia-reperfusion myocardium through multiple targets, mainly through its anti-inflammatory and antioxidant effects, modulation of vasodilatation and contraction, promotion of neovascularization, and regulation of cell death functions (Ho and Hong, 2011). SalB also exerts anticoagulant, hypolipidemic, and indirect cardioprotective effects. SalB is a robust and active ingredient with an multi-target promising impact; however, no SalB injections are available on the market. A comprehensive assessment and systematic review of preclinical animal studies is essential before SalB enters clinical trials. Systematic reviews and meta-analyses can not only provide important information about the possibility of translating the results of experimental animal studies into clinical practice but also validate the efficacy, safety, and optimal dosage (Ritskes-Hoitinga et al., 2014).
To the best of our knowledge, no previous review has systematically summarized the effects of SalB in MI/R models.
Therefore, we performed a quality assessment and meta-analysis of preclinical studies using SalB in animal models to form a chain of clinical evidence and provide rigorous and systematic scientific support for further clinical studies.
This review was designed and reported according to the Preferred Reporting Items for Systematic Reviews and Meta-Analyses (PRISMA) 2020 statement (Page et al., 2021). The protocol was registered in PROSPERO (registration number: CRD42024526832).
We searched the following six databases: PubMed, EMBASE, Web of Science, China’s National Knowledge Infrastructure (CNKI), WanFang, and the VIP database (VIP). The search strategy is based on the search components “Myocardial Ischemia,” “Myocardial I/R,” and “Salvianolic acid.” The full search strategy is provided in the Supplementary Table 1. The search was limited from its inception to March 2024. We also screened the reference lists of the included studies to identify additional eligible studies.
To minimize bias, the inclusion criteria were as follows: ①animal model of MI/R (modeling methods include ligation of LAD, ligation-reperfusion, and injection of ISO); ②The treatment group received only Sal B at any dose and treatment modality; ③The control group received only an equivalent amount of saline or vehicle or no treatment; ④The primary outcome of animal studies was infarct size, cardiac markers (CK, CK-MB, LDH, AST, and cTnI) and echocardiogram indicators (+dp/dt max, -dp/dt max, LVEF, LVFS, and cardiac output). Secondary outcomes were serum or protein levels associated with myocardial injury and biomarkers related to Sal B mechanisms.
The exclusion criteria were as follows: ①Case report, clinical trial, review, meeting abstract; ②The treatment group that received salvianolic acid complex or received Sal B in conjunction with other treatments; ③Animals with other disease comorbidities or No MI/R model; ④In vitro or ex vivo studies; ⑤No predetermined outcome index; ⑥No control group.
Two reviewers independently screened the retrieved studies’ titles and/or abstracts using the search technique to identify articles that met the inclusion criteria mentioned above. They then retrieved all the texts of these possibly eligible studies and separately determined their eligibility. If they cannot agree on whether a particular study qualifies, they will be consulted with a third reviewer to resolve the dispute. To evaluate the research quality and the evidence synthesis, two impartial reviewers gathered data from the included studies using standardized pre-pilot forms.
The extracted information will include: ①first author name and year of publication; ②Specific details on the animals in each study, including species, number, sex, and body weight; ③MI/R model and the anesthetic method used to prepare the model; ④Information about Sal B treatment, including dose, method of administration, course of treatment; as well as corresponding information in the control group; ⑥The mean and standard deviation (SD) of the results. If the article presented results from many different time points or multiple doses of SalB, only data from the last time point or the highest dose group were extracted. Because some of the data were supplied only in graphical form, we attempted to contact the authors for further clarification. If we did not receive a response, we used digital ruler software to determine the value of the graph.
The two reviewers conduct an independent quality assessment using the SYRCLE’s RoB tool (Hooijmans et al., 2014a), and any disagreements are resolved through consultation with the third reviewer.
R was used for statistical analysis. Heterogeneity was determined using the Q statistical test (p < 0.05, considered statistically significant) and the I2 statistical test. Owing to the nature and diversity of animal studies, the random effects model may better reflect reality; thus, we used a random effects model for every outcome. If at least 10 independent comparisons were available, formal subgroup analyses were performed for the modeling method, dosage, method of administration, timing of administration, and species, and sensitivity analyses were performed to assess the robustness of the meta-analysis results. All results were continuous variables; therefore, standardized mean differences (SMDs) and 95% confidence intervals (95%CI) were used to express them. Publication bias was assessed using funnel plots and Egger’s regression test.
A total of 888 articles were retrieved from the online database, 181 of which remained after duplicates were removed. 92 articles were retained after screening titles and abstracts. After full-text screening and assessment, 34 studies met the inclusion criteria. Because complete data were unavailable for two articles, 32 studies were ultimately included in this meta-analysis (Figure 1).
Sprague-Dawley rats were used in 22 studies, Wistar rats in seven studies, ICR mice in one study, New Zealand rabbits, and 4-Way Ovoss in one study. In terms of sex, both female and male animals were used in 10 studies; one study used all female animals, one study did not explicitly report the sex of the animals, and all other studies used male animals. Fourteen of the included studies used ligation of the LAD, 13 used ligation-reperfusion, and the remaining five used ISO injection modeling. The three main routes of administration were intravenous (n = 15), intraperitoneal (n = 11), and gavage (n = 6). The dosage ranged from 3 to 120 mg/kg. In addition, the timing of Sal B administration varied, with 15 studies administering the drug prophylactically before modeling, 13 studies administering the drug after modeling or surgery, and the treatment time points of four studies were at other times. The treatment lasted 28 days Table 1 presents the characteristics of the included studies.
Overall, 64.69% of the criteria were marked as “unclear” because basic information on the methodology was missing. For six criteria, all studies scored “unclear,” namely, Sequence generation (selection bias); Baseline characteristics (selection bias); Allocation concealment (selection bias); Blinding investigators (performance bias); Random outcome assessment (measurement bias); Blinding outcome assessor (measurement bias). Among these studies, 53.12% reported that animals were randomly grouped and housed in rooms with the same controlled temperature and moderation. One study had a high risk of bias in selective reporting because the results were not fully reported. Eight studies did not report complete data and were therefore considered high-risk. Given the high number of “unclear” scores, none of the studies were considered to be at low risk of bias. The percentage of inter-evaluator agreement for the risk of bias assessment was 79.6%. The results of the quality assessment of the included studies are shown in Table 2.
Nineteen studies reported the infarct size, but data were unavailable for one of these studies. Finally, 18 studies involving 309 animals were included in the meta-analysis. The results showed that Sal B was effective in reducing myocardial infarct size compared to the control group (SMD = −4.23, 95%CI(-5.88, −2.58), p < 0.01, I2 = 85%) (Figure 2). However, there was significant heterogeneity across the studies; therefore, sensitivity and subgroup analyses were conducted to explore the potential sources of heterogeneity. Sensitivity analyses were conducted by omitting individual studies that showed reliable results for infarct size (Supplementary Figure 1). Subsequently, separate subgroup analyses were performed based on modeling, administration method, time, dosage, and species (Table 3). The results showed that only the dosage subgroups were statistically significant. In addition, a substantial reduction in heterogeneity was found when blocking the LAD and Intravenous injection. Lower heterogeneity was likewise found in the dose less than 20 mg/kg dose subgroup, other administration time subgroup, and Wistar rat subgroup, but this was not statistically significant.
Figure 2. The forest plot: effect of Sal B for reducing the infarction size compared with the control group.
CK, CK-MB, LDH, AST, and cTnI are common markers of myocardial injury and are often measured in combination to monitor the extent of myocardial injury in clinical practice. This meta-analysis indicated that CK (SMD = −1.88, 95%CI(-2.42, −1.33), p < 0.01, I2 = 47%), CK-MB (SMD = −4.06, 95%CI(-5.35, −2.77), p < 0.01, I2 = 81%), LDH (SMD = −4.13, 95%CI(-5.93, −2.33), p < 0.01, I2 = 87%), and cTnI (SMD = −4.71, 95%CI(-7.14, −2.28), p < 0.01, I2 = 80%) were reduced after the Sal B intervention. The SMD for the treatment effect of Sal B on AST was not statistically significant (SMD = −3.71, 95%CI(-9.16, 1.73), p = 0.18, I2 = 92%) (Figure 3).
Figure 3. The forest plot of cardiac markers: effects of Sal B for decreasing CK (A), CK-MB (B), LDH (C), cTnI (E) compared with the control group. No significant effect of Sal B for AST (D) compared with the control group.
Heterogeneity was high for the latter four indicators, but only LDH had more than ten publications; thus, we performed sensitivity and subgroup analyses only for LDH. By progressively eliminating each trial from the meta-analysis, no significant differences were observed between the pre-and post-sensitivity pooled effects of LDH (Supplementary Figure 2). The results of the subgroup analyses showed that the differences between subgroups were not statistically significant (Table 4). Heterogeneity was reduced in the ISO group and the subgroup treated with doses less than 20 mg/kg; however, the difference was not statistically significant.
Echocardiographic indicators, such as + dp/dt max, -dp/dt max, LVEF, LVFS, and cardiac output, can describe changes in cardiac function and structure in MI/R models. The results of this meta-analysis suggest that Sal B had a significant effect in increasing + dp/dt max (SMD = 5.75, 95%CI(2.53, 8.96), p < 0.01, I2 = 90%), -dp/dt max (SMD = 4.47, 95%CI(3.77, 5.16), p < 0.01, I2 = 0%), LVFS(SMD = 2.78, 95%CI(0.77, 4.79), p < 0.01, I2 = 89%), and cardiac output (SMD = 5.07, 95%CI(4.36, 5.79), p < 0.01, I2 = 0%) compared to the control group (Figure 4). However, Sal B had no apparent effect on LVEF (SMD = 4.36, 95%CI(-0.19, 8.92), p = 0.06, I2 = 94%).
Figure 4. The forest plot of cardiac function: effects of Sal B for increasing + dp/dt max (A), -dp/dt max (B), LVFS (D), Cardiac Output (E) compared with the control group. No significant effect of Sal B for LVEF (C) compared with the control group.
Oxidative stress can be measured using reactive oxygen species (ROS), oxidative products, and antioxidant enzymes. MDA levels indicate the degree of lipid peroxidation. SOD and CAT are antioxidant enzymes that scavenge free radicals and prevent oxidative damage. Summarizing the available reports on the cardioprotective effects of Sal B, it was reported that Sal B intervention reduced ROS (SMD = −7.81, 95%CI(-10.77, −4.84), p < 0.01, I2 = 71%) and MDA (SMD = −4.90 95%CI(-6.54, −3.26), p < 0.01, I2 = 90%) levels and increased SOD (SMD = 4.36, 95%CI(1.87, 6.85), p < 0.01, I2 = 91%), CAT (SMD = 5.51, 95%CI(2.10, 8.91), p < 0.01, I2 = 87%) and GSH (SMD = 5.55, 95%CI (0.22, 10.88), p = 0.04, I2 = 88%) levels as compared to the control group (Figure 5). These results suggest that Sal B is a cardioprotective agent by restoring the oxidative-antioxidant balance.
Figure 5. The forest plot of oxidative stress indicators: effects of Sal B for decreasing ROS (A), MDA (C), and increasing SOD (B), CAT (D), and GSH (E) compared with the control group.
Overexpression of inflammatory markers is an indication of MI/R. It is associated with myocardial injury in the early stages of ischemia/reperfusion and myocardial repair after injury. The results of this meta-analysis revealed that Sal B alleviated the expression of inflammatory factors including IL-1β(SMD = −3.26, 95%CI(-4.13, −2.39), p < 0.01, I2 = 70%), IL-6(SMD = −1.74, 95%CI(-2.54, −0.95), p < 0.01, I2 = 0%) and TNF-α(SMD = −2.85 95%CI(-4.03, −1.66), p < 0.01, I2 = 76%) (Figure 6).
Figure 6. The forest plot of inflammation indicators: effects of Sal B for decreasing IL-1β (A), IL-6 (B), TNF-α (C) compared with the control group.
Neovascularization is an important compensatory mechanism after MI, and the vascular endothelial growth factor (VEGF) is a major biological mediator of angiogenesis in vivo. Promoting normal vasodilatory and contractile functions is likewise one of the important methods for alleviating MI/R injury. NO and ET are the vasodilatory and vasoconstrictive factors, respectively. Therefore, the VEGF, NO, and ET levels were considered in this meta-analysis. The results showed that SalB treatment significantly increased VEGF (SMD = 15.17, 95%CI(1.58, 28.75), p = 0.03, I2 = 94%) and NO levels (SMD = 4.68, 95%CI(1.36, 8.00), p < 0.01, I2 = 85%). Meta-analyses showed no significant effect on ET (SMD = −10.28, 95%CI(-26.02, 5.47), p = 0.20, I2 = 93%) (Figure 7).
Figure 7. The forest plot of neovascularization and vasoregulation: effects of Sal B for increasing VEGF (A), NO (B) compared with the control group. No significant effect of Sal B for ET (C) compared with the control group.
Bax and Bcl-2 are markers of apoptosis, and the TUNEL assay is commonly used to detect apoptosis ratios. The results revealed that SalB significantly decreased Bax (SMD = −11.08, 95%CI(-19.84, −2.33), p < 0.01, I2 = 94%) and elevated Bcl-2 (SMD = 11.52, 95%CI(0.94, 22.11), p < 0.01, I2 = 93%). The level of apoptosis detected by the TUNEL also declined compared to the control group (SMD = −6.02, 95%CI(-8.23, −3.81), p < 0.01, I2 = 76%) (Figure 8).
Figure 8. The forest plot of apoptosis indicators: effects of Sal B for decreasing Bax (A) and TUNEL (C) and increasing Bcl-2 (B) compared with the control group.
Publication bias is a phenomenon in which the results of an experiment determine the likelihood of publication, usually leading to an overestimation of positive results. Funnel plots and Egger’s regression tests were used to analyze the publication bias of infarction size. Visual inspection of the funnel plots revealed a large amount of asymmetry, suggesting potential publication bias, as confirmed by Egger’s test (p < 0.05) (Supplementary Figures 3A, B). Trim-and-fill methods were used to correct for potential publication bias (Supplementary Figure 3C). Although trim-and-fill analyses resulted in corrected novel effect sizes (SMD = −2.27, 95%CI(-4.46, −0.09), p = 0.04), the association between Sal B and infarct size remained statistically significant.
To the best of our knowledge, this is the first preclinical systematic review and meta-analysis investigating the effects of SalB on MI/R. Thirty-two studies involving a total of 32 papers involving 732 animals were included. The meta-analysis showed that Sal B has a protective effect on the myocardium after ischemia-reperfusion injury and a positive effect on reducing infarct size, improving cardiac function, and reducing myocardial injury. The cardioprotective effects of SalB were mediated by attenuating oxidative stress and inflammation, promoting neovascularization, modulating vascular function, and attenuating cardiomyocyte apoptosis (Figure 9). A high degree of heterogeneity was observed among the included studies; further subgroup and sensitivity analyses were performed. The results of the subgroup analyses showed that the dose of the drug administered could be a potential source of heterogeneity. In contrast, the differences between subgroups for the time of administration, mode of administration, and modality of modeling were not statistically significant. They did not significantly reduce the heterogeneity across subgroups within the group. There was some publication bias among the included studies. In conclusion, Sal B has a clear protective effect against MI/R; however, the exact magnitude of the cardioprotective effect of Sal B should be interpreted with caution.
MI/R injury involves multiple pathological processes, such as cell death (including apoptosis, autophagy, and ferroptosis), oxidative stress, inflammatory response, vascular endothelial dysfunction, and neovascularization (Ibáñez et al., 2015). There is no satisfactory therapeutic approach in the current clinical practice, and multi-targeted small-molecule drugs may be a solution. Sal B is a promising small-molecule drug for treating MI/R. However, the progression of Sal B from basic research to clinical practice has been hampered by the lack of a comprehensive understanding of its underlying mechanisms. To gain a more comprehensive understanding of the role of Sal B in MI/R, its specific mechanisms and pathways are summarized in this review. In brief, Sal B exerted antioxidant, anti-inflammatory, and modulatory effects on vascular function and regulated cell death (Figure 10).
SalB protects the heart by regulating cardiomyocyte death. Various pathways regulate cardiomyocyte death during MI/R, including apoptosis, ferroptosis, and autophagy, among others (Xiang et al., 2024). Cardiomyocyte apoptosis is a genetically regulated, actively ordered, and self-terminating process involving various enzymes. Caspase-3, Bcl-2, and BAX are important regulators of apoptosis. Caspase-3 is at the crossroads of apoptosis-related signaling pathways. Activating caspase-3 promotes downstream protease cascades that irreversibly amplify apoptotic signaling pathways. Bcl-2 exerts antiapoptotic effects and prevents apoptosis at multiple levels. Bax belongs to a class of pro-apoptotic proteins that promote the subsequent apoptotic cascade by disrupting the outer mitochondrial membrane (Del et al., 2019). Several studies have shown that Sal B can regulate the expression of Caspase-3, Bcl-2, and Bax and thus exert anti-apoptotic effects on cardiomyocytes. Furthermore, several studies have explored the pathways upstream of these three apoptotic regulators. Sal B inhibited mitochondrial ROS accumulation, which consequently inhibited the downstream JNK/MAPK pro-apoptotic signaling pathway (Xu et al., 2023a). In addition, the PI3K-Akt pathway is also one of the upstream pathways in which Sal B alleviates apoptosis (Liu et al., 2020a).
Ferroptosis is a recently discovered type of regulated cell death (RCD) in recent years (Zhao et al., 2023). The core biochemical features of ferroptosis are iron overload and lipid peroxidation. Iron overload refers to the excessive accumulation of intracellular Fe2+. TfR1 and Fpn1 present on the cell membrane are channels for transporting Fe3+ and Fe2+. FTH1(ferritin heavy chain-1) is a component of ferritin that catalyzes the storage of Fe2+, thereby reducing free iron levels (Zhang et al., 2024). FTH1 is often used as a biomarker of ferroptosis. The acidic and highly reducing intracellular environment during the early stages of reperfusion promotes the release of iron and iron from ferritin. Iron uptake, utilization, and recirculation balance are disrupted (Xiang et al., 2024). Free iron ions accumulate and catalyze the Fenton reaction, producing increased ROS. Sal B attenuates intracellular free iron accumulation and reduces mitochondrial ROS by decreasing the expression of TfR1 and promoting high expression of FTH1, thereby protecting the myocardium from ferroptosis (Xu et al., 2023a). Lipid peroxidation is another characteristic of ferroptosis. Glutathione peroxidase 4 (GPX4) is the most important intracellular anti-lipid peroxidase and a central regulator of ferroptosis. In the early and middle stages of myocardial infarction, GPX4 expression is markedly reduced, causing the accumulation of lipid peroxides and ultimately leading to ferroptosis (Han et al., 2023). Sal B reduces ubiquitination-proteasome degradation of GPX4 and attenuates MI/R-induced ferroptosis. It was also found that another pathway by which salt B inhibits lipid peroxidation is the inhibition of the Nrf2/HO-1 pathway (Shen et al., 2022).
Autophagy is a lysosome-dependent degradation pathway (Popov et al., 2023). Autophagic lysosomes play a crucial role in autophagy. It degrades damaged cytoplasmic structures and produces amino acids, free fatty acids, and other protein and energy synthesis substances. Autophagy is beneficial during ischemia in MI/R. Autophagy helps cardiomyocytes adapt to environments such as hypoxia and starvation and delays the occurrence of irreversible cell damage (Dong et al., 2019). However, excessive autophagy during reperfusion can induce the progressive consumption of cellular constituents, leading to autophagic cell death. Thus, moderate autophagy activation is cardioprotective and ensures the availability of energy substrates (Bravo-San Pedro et al., 2017). Autophagy is regulated by specific autophagy-related (Atg) genes, among which Atg5 is involved in the formation of phagocytic vesicle expansion on the one hand and promotes the recruitment of LC3II on the other, contributing to the transition from LC3Ⅰ to LC3Ⅱ. LC3II and LC3II/Ⅰ are key indicators of autophagic activity (Sciarretta et al., 2018). In addition, Beclin1 and its complexes are involved in the formation of the isolation membrane as well as the recruitment of Atg proteins to the autophagosomal membrane, and their elevation marks the initiation of autophagy (Shi et al., 2019). Sal B upregulates autophagy lysosomes and the expression of LC3II/Ⅰ and Beclin1 in a myocardial ischemia model in mice induced by ISO. This suggests that Sal B enhances the autophagic activity during myocardial ischemia (Chen et al., 2023). However, whether the effect of Sal B on autophagy during reperfusion is consistent with that during the ischemic process has not yet been investigated.
Oxidative stress is considered one of the major factors that cause myocardial injury in MI/R. During MI/R, particularly during reperfusion, the balance between ROS generation and scavenging is disrupted. A large accumulation of oxygen free radicals damages subcellular membranes and structural systems through several mechanisms (Granger and Kvietys, 2015; Bugger and Pfeil, 2020). Several studies have revealed that Sal B attenuates oxidative stress by reducing ROS production and increasing the expression of the antioxidants SOD, CAT, and GSH(31–34). GPX1 is an important antioxidant enzyme responsible for scavenging ROS accumulated in cells. Ubiquitination and degradation of GPX1 are mediated by TRIM8. Studies have shown that SalB inhibits TRIM8 expression and reduces GPX1 degradation, resulting in accelerated ROS elimination (Lu et al., 2022).
Macrophages regulate the MI/R repair processes. In the early stages of MI/R, M1 macrophages predominate and produce oxidative metabolites and proinflammatory factors. During the following 3–5 days, M2 macrophages gradually replace M1, and M2 macrophages produce anti-inflammatory factors and promote scar formation and angiogenesis (Xu et al., 2023b). Therefore, timely regulation of their transition to the M2 phenotype is essential to ensure cardiac tissue healing and avoid adverse remodeling and systolic dysfunction. Changing the mode of macrophage energy metabolism is one way of changing the polarization direction (Kim et al., 2021). Sal B inhibits the activation of mTORC1 and glycolysis mediated by Rag D, resulting in the inhibition of M1 polarization and an increase in M2 macrophages, ultimately leading to a reduction in myocardial inflammatory factor infiltration and cardioprotective effects (Zhao et al., 2020).
Regulation of vascular endothelial function and promotion of neovascularization are also important for improving MI/R. MI/R injury leads to an imbalance between vasodilatory and vasoconstrictive factors, manifesting as a marked increase in the content of vasoconstrictive factors and a decrease in the release of vasodilatory factors. This imbalance causes vasoconstriction and spasms, leading to worsening of partial ischemia and hypoxia. Sal B can inhibit the release of ET and TXB2 from the vascular endothelium and increase the content of NO and PGI2, thereby regulating endothelial dysfunction and increasing the myocardial blood supply (Wang et al., 2004; Zhang et al., 2008; Yang et al., 2008; Xue et al., 2014). This suggests that this effect may be achieved through the AMPK/PI3k/Akt pathway (Pan et al., 2011). Neovascularization is another approach to repair MI/R damage. VEGF is one of the most widely studied positive regulators of vascular neovascularisation. It also promotes the proliferation and differentiation of vascular endothelial cells. PKD1 is an upstream protein of VEGF that mediates the proliferation, migration, and lumen formation of endothelial progenitors and stem cells. It was found that Sal B induced VEGF synthesis by regulating the PKD1/HDAC axis and the PKD1/HIF-α axis, which promoted neovascularization of ischemic myocardial tissues and increased microcirculatory blood supply in ischemic myocardium (Liu et al., 2020b; Liu et al., 2020c). Additionally, Sal B activates the Nrf2/HO-1 pathway and induces VEGF synthesis, which is involved in the regulation of neovascularization after ischemic injury (Zhou et al., 2018).
The inflammatory response also plays an important role in the myocardial injury caused by MI/R. During ischaemia, inflammation is activated. During reperfusion, restoration of blood flow and oxygen delivery further activates inflammatory signalling pathways. Inflammatory vesicles are sensors that link injury to inflammation. NLRP3 inflammatory vesicles orchestrate the inflammatory response in the absence of pathogens by inducing downstream caspase-1 cleavage and releasing the inflammatory factors IL-1β and IL-18 (Algoet et al., 2023). Sal B promotes the inactivation of NLRP3 inflammatory vesicles via several pathways. First, Sal B can further induce NLRP3 inactivation by inhibiting intracellular ROS production during myocardial ischemia, increasing the mitochondrial membrane potential, regulating the expression levels of SIRT1, Parkin, and PINK1 proteins, and promoting mitochondrial autophagy (Hu et al., 2020). Secondly, Sal B could activate AMPK and PGC-1α-mediated mitochondrial biogenesis via SIRT1, which in turn blocked NLPR3-mediated inflammatory response (Li et al., 2022). Finally, Sal B blocks TLR4 dimerization through competitive chimerism with MD-2 and reduces HMGB1 expression, thereby inhibiting the TLR4/NF-κB pathway, reducing the inflammatory cascade, and attenuating MI/R injury (Hu et al., 2019).
This study systematically reviewed the potential efficacy and mechanism of action of SalB in an animal model of MI/R. This systematic review and meta-analysis had several limitations when interpreting the results.
1) The literature search was conducted only on commonly used Chinese and English databases; articles published in other languages may have been ignored. The included studies were conducted in China, hindering our results’ generalizability.
2) Pre-specifying the methodological details of the meta-analysis reduces the risk of inappropriate post hoc analyses and selective result reporting (reporting only the results of subgroup analyses showing significant effects) (Bannach-Brown et al., 2024). Therefore, subgroups were established in advance for this meta-analysis. The subgroup analyses for infarct size and LDH levels showed statistically significant differences only between the dose subgroups for myocardial infarct size. Differences between the subgroups regarding administration method, administration time, modeling, and species were not statistically significant. However, no statistical differences between these subgroups should never be interpreted as evidence that the covariates are not related to the effect size (Hooijmans et al., 2022). In addition, the fact that substantial heterogeneity within groups remained after subgroup analyses does not mean that subgroups were not a source of heterogeneity, as these results were obtained under different experimental conditions and need to be investigated in further depth (Hooijmans et al., 2014b).
3) Among the included studies, the duration of administration varied considerably, ranging from one dose to 28 consecutive days. However, considering the diversity of dosing durations in different studies and the limitations of the number of studies, this heterogeneity was not explored, although it may be an important source of heterogeneity.
4) Publication bias was found in this study, which is common in preclinical systematic reviews and meta-analyses (Korevaar et al., 2011; Marks-Anglin and Chen, 2020). Subsequently, this study used the trim-and-fill methods to adjust for publication bias. The results indicated that the direction of the identified effect was reliable. In conclusion, the precise overall effect estimates calculated in this review should be interpreted cautiously, and more high-quality studies are needed to clarify the specific effect sizes.
In clinical practice, systematic reviews of animal studies can facilitate better scientific research and improve translation, offering the possibility of providing evidence for the potential translational value of animal models for humans (Hooijmans et al., 2018). For future preclinical studies, a systematic review of published studies can reduce unnecessary repetition of costly animal experiments. At the same time, a systematic review and synthesis of evidence can expose biases and deficiencies in the methodology of individual studies, thus reducing misinterpretation of evidence.
The present study systematically reviewed 32 included studies and found that only one study was conducted using female animals, ten did not differentiate between animal sexes, and all other studies included males. However, there were differences in the degree of tolerance to MI/R between the sexes. In animal models of MI/R, females have a smaller infarct size, reduced ischemic systolic dysfunction, and limited fibrotic remodeling compared to males (Medzikovic et al., 2023). Reliable sex subgroup analyses were not possible due to the lack of detailed reporting on the sex of the individual metrics originating from the included studies. Few studies on female animals are currently available, highlighting the need for further studies.
Safe and effective dose ranges are essential for future animal experiments and clinical studies. Interestingly, the different studies’ dosages ranged from 3 to 480 mg/kg; in almost every study, the maximum dose was more effective, with no reports of adverse effects. However, in clinical practice, there is a safe dose range for any drug. A small-sample clinical trial in a Chinese population explored the safe dose of SalB. Adverse events (SAEs) in the single-ascending-dose (SAD) and multiple-ascending-dose (MAD) studies were observed with an incidence of approximately 50% (Cheng et al., 2023). Therefore, further investigation of the optimal SalB dose range of Sal B is required.
The risk of bias assessment showed that the risk of bias for most of the included studies was rated as “unclear” because essential sources of bias, such as allocation sequence concealment, baseline characteristics, and implementation of blinding, were missing from the report. It is difficult to judge the impact of these sources of bias on the results. The lack of methodological details may distort the interpretation of the study results, and there is a risk of overestimating the effect of the intervention. Future animal studies should refine their methodological reporting as much as possible, adhering to the ARRIVE guidelines for reporting animal studies (Percie du Sert et al., 2020).
Overall, the results of this systematic review and meta-analysis suggest that Sal B has a protective effect against MI/R injury, which may be accomplished through a complex multi-target and multi-pathway mechanism. This study provides an evidence-based assessment and reference evidence for future basic and clinical studies on SalB. Further studies are needed to elucidate the extent to which Sal B exerts its cardioprotective effects and the safety of its use.
The original contributions presented in the study are included in the article/Supplementary Material, further inquiries can be directed to the corresponding author.
YY: Writing–original draft, Methodology, Investigation, Formal Analysis, Data curation, Conceptualization. ZS: Writing–review and editing, Software, Methodology, Investigation, Data curation. XS: Writing–review and editing, Software, Methodology, Investigation, Data curation. JZ: Writing–review and editing, Validation, Supervision, Methodology, Formal Analysis. TT: Writing–review and editing, Validation, Supervision, Methodology, Formal Analysis. XZ: Writing–review and editing, Validation, Supervision, Methodology, Formal Analysis. KY: Writing–review and editing, Supervision, Funding acquisition, Conceptualization.
The author(s) declare that financial support was received for the research, authorship, and/or publication of this article. The authors declare that this research was supported by Central High Level Traditional Chinese Medicine Hospital Project of eye Hospital China Academy of Chinese medical science (grant numbers GSP2-02).
We would like to thank Editage (www.editage.cn) for English language editing.
The authors declare that the research was conducted in the absence of any commercial or financial relationships that could be construed as a potential conflict of interest.
All claims expressed in this article are solely those of the authors and do not necessarily represent those of their affiliated organizations, or those of the publisher, the editors and the reviewers. Any product that may be evaluated in this article, or claim that may be made by its manufacturer, is not guaranteed or endorsed by the publisher.
The Supplementary Material for this article can be found online at: https://www.frontiersin.org/articles/10.3389/fphar.2024.1452545/full#supplementary-material
Algoet, M., Janssens, S., Himmelreich, U., Gsell, W., Pusovnik, M., Van den Eynde, J., et al. (2023). Myocardial ischemia-reperfusion injury and the influence of inflammation. Trends Cardiovasc Med. 33 (6), 357–366. doi:10.1016/j.tcm.2022.02.005
Bannach-Brown, A., Rackoll, T., Kaynak, N., Drude, N., Aquarius, R., Vojvodić, S., et al. (2024). Navigating PROSPERO4animals: 10 top tips for efficient pre-registration of your animal systematic review protocol. BMC Med. Res. Methodol. 24 (1), 20. doi:10.1186/s12874-024-02146-0
Bravo-San Pedro, J. M., Kroemer, G., and Galluzzi, L. (2017). Autophagy and mitophagy in cardiovascular disease. Circ. Res. 120 (11), 1812–1824. doi:10.1161/CIRCRESAHA.117.311082
Bugger, H., and Pfeil, K. (2020). Mitochondrial ROS in myocardial ischemia reperfusion and remodeling. Biochim. Biophys. Acta Mol. Basis Dis. 1866 (7), 165768. doi:10.1016/j.bbadis.2020.165768
Chen, H. X., Xu, L., and Xu, B. H. (2012). Effect of salvianolic acid B on angiogenesis of ischemic myocardium in myocardial ischemia rats. Chin. J. Exp. Traditional. 18, 180–183. doi:10.13422/j.cnki.syfjx.2012.20.056
Chen, Q., Xu, Q. Y., Zhu, H., Wang, J., Sun, N., Bian, H., et al. (2023). Salvianolic acid B promotes angiogenesis and inhibits cardiomyocyte apoptosis by regulating autophagy in myocardial ischemia. Chin. Med. 18 (1), 155. doi:10.1186/s13020-023-00859-wAvailable at: https://www.embase.com/search/results?subaction=viewrecord&id=L2026873317&from=export.
Cheng, J., Long, J., Zhang, J., Han, L., Hu, Y., Liu, J., et al. (2023). Safety, tolerance, and pharmacokinetics of salvianolic acid B in healthy Chinese volunteers: a randomized, double-blind, placebo-controlled phase 1 clinical trial. Front. Pharmacol. 14, 1146309. doi:10.3389/fphar.2023.1146309
Del, Re D. P., Amgalan, D., Linkermann, A., Liu, Q., and Kitsis, R. N. (2019). Fundamental mechanisms of regulated cell death and implications for heart disease. Physiol. Rev. 99 (4), 1765–1817. doi:10.1152/physrev.00022.2018
Dong, Y., Chen, H., Gao, J., Liu, Y., Li, J., and Wang, J. (2019). Molecular machinery and interplay of apoptosis and autophagy in coronary heart disease. J. Mol. Cell Cardiol. 136, 27–41. doi:10.1016/j.yjmcc.2019.09.001
Fan, Y. C., Zhao, G. F., and Zhang, W. Z. (2004). Influence of Salvianolic acid B on pathomorphology in myocardial infarction rats. J. Tianjin Univ. Traditional Chin. Med. 23 (1), 12–14.
Granger, D. N., and Kvietys, P. R. (2015). Reperfusion injury and reactive oxygen species: the evolution of a concept. Redox Biol. 6, 524–551. doi:10.1016/j.redox.2015.08.020
Han, X., Zhang, J., Liu, J., Wang, H., Du, F., Zeng, X., et al. (2023). Targeting ferroptosis: a novel insight against myocardial infarction and ischemia-reperfusion injuries. Apoptosis 28 (1–2), 108–123. doi:10.1007/s10495-022-01785-2
Hausenloy, D. J., Botker, H. E., Engstrom, T., Erlinge, D., Heusch, G., Ibanez, B., et al. (2017). Targeting reperfusion injury in patients with ST-segment elevation myocardial infarction: trials and tribulations. Eur. Heart J. 38 (13), 935–941. doi:10.1093/eurheartj/ehw145
Heusch, G. (2020). Myocardial ischaemia–reperfusion injury and cardioprotection in perspective. Nat. Rev. Cardiol. 17 (12), 773–789. doi:10.1038/s41569-020-0403-y
Heusch, G., and Gersh, B. J. (2017). The pathophysiology of acute myocardial infarction and strategies of protection beyond reperfusion: a continual challenge. Eur. Heart J. 38 (11), 774–784. doi:10.1093/eurheartj/ehw224
Ho, J. H. C., and Hong, C. Y. (2011). Salvianolic acids: small compounds with multiple mechanisms for cardiovascular protection. J. Biomed. Sci. 18 (1), 30. doi:10.1186/1423-0127-18-30
Hooijmans, C. R., de Vries, R. B. M., Ritskes-Hoitinga, M., Rovers, M. M., Leeflang, M. M., IntHout, J., et al. (2018). Facilitating healthcare decisions by assessing the certainty in the evidence from preclinical animal studies. PLoS One 13 (1), e0187271. doi:10.1371/journal.pone.0187271
Hooijmans, C. R., Donders, R., Magnuson, K., Wever, K. E., Ergün, M., Rooney, A. A., et al. (2022). Assessment of key characteristics, methodology, and effect size measures used in meta-analysis of human-health-related animal studies. Res. Synth. Methods 13 (6), 790–806. doi:10.1002/jrsm.1578
Hooijmans, C. R., IntHout, J., Ritskes-Hoitinga, M., and Rovers, M. M. (2014b). Meta-analyses of animal studies: an introduction of a valuable instrument to further improve healthcare. ILAR J. 55 (3), 418–426. doi:10.1093/ilar/ilu042
Hooijmans, C. R., Rovers, M. M., de Vries, R. B., Leenaars, M., Ritskes-Hoitinga, M., and Langendam, M. W. (2014a). SYRCLE’s risk of bias tool for animal studies. BMC Med. Res. Methodol. 14 (1), 43. doi:10.1186/1471-2288-14-43
Hu, X. G., Xiang, X., Yang, C. Y., and Zhou, Y. S. (2010). Protective effect of salvinorin B against acute myocardial ischaemia in experimental rats. Guangdong Med. J. 31, 334–336. doi:10.13820/j.cnki.gdx.2010.03.005
Hu, Y., Li, Q., Pan, Y., and Xu, L. (2019). Sal B alleviates myocardial ischemic injury by inhibiting TLR4 and the priming phase of NLRP3 inflammasome. Molecules 24 (23), 4416. doi:10.3390/molecules24234416Available at: https://www.embase.com/search/results?subaction=viewrecord&id=L630116289&from=export.
Hu, Y., Wang, X., Li, Q., Pan, Y., and Xu, L. (2020). Salvianolic acid B alleviates myocardial ischemic injury by promoting mitophagy and inhibiting activation of the NLRP3 inflammasome. Mol. Med. Rep. 22 (6), 5199–5208. doi:10.3892/mmr.2020.11589
Ibáñez, B., Heusch, G., Ovize, M., and Van de Werf, F. (2015). Evolving therapies for myocardial ischemia/reperfusion injury. J. Am. Coll. Cardiol. 65 (14), 1454–1471. doi:10.1016/j.jacc.2015.02.032
Jiang, B., Chen, J., Xu, L., Gao, Z., Deng, Y., Wang, Y., et al. (2010). Salvianolic acid B functioned as a competitive inhibitor of matrix metalloproteinase-9 and efficiently prevented cardiac remodeling. BMC Pharmacol. 10. doi:10.1186/1471-2210-10-10
Kim, Y., Nurakhayev, S., Nurkesh, A., Zharkinbekov, Z., and Saparov, A. (2021). Macrophage polarization in cardiac tissue repair following myocardial infarction. Int. J. Mol. Sci. 22 (5), 2715. doi:10.3390/ijms22052715
Korevaar, D. A., Hooft, L., and Ter Riet, G. (2011). Systematic reviews and meta-analyses of preclinical studies: publication bias in laboratory animal experiments. Lab. Anim. 45 (4), 225–230. doi:10.1258/la.2011.010121
Li, L., Sun, L. S., and Xu, J. P. (2004). Therapeutical effects of salvianolic acid B on myocardial infarction in dogs. Chin. Tradit. Pat. Med. 26 (3), 215–218.
Li, Q., Zuo, Z., Pan, Y., Zhang, Q., Xu, L., and Jiang, B. (2022). Salvianolic acid B alleviates myocardial ischemia injury by suppressing NLRP3 inflammasome activation via SIRT1-AMPK-PGC-1α signaling pathway. Cardiovasc Toxicol. 22 (9), 842–857. doi:10.1007/s12012-022-09760-8
Li, Z. M., Xu, S. W., and Liu, P. Q. (2018). Salvia miltiorrhizaBurge (Danshen): a golden herbal medicine in cardiovascular therapeutics. Acta Pharmacol. Sin. 39 (5), 802–824. doi:10.1038/aps.2017.193
Lin, C., Liu, Z., Lu, Y., Yao, Y., Zhang, Y., Ma, Z., et al. (2016). Cardioprotective effect of Salvianolic acid B on acute myocardial infarction by promoting autophagy and neovascularization and inhibiting apoptosis. J. Pharm. Pharmacol. 68, 941–952. doi:10.1111/jphp.12567
Lin, Z. R., Chen, C., Tian, D. K., Gu, Q., Qu, S. F., Ruan, A. H., et al. (2011). Comparative study of salvinorin A and salvinorin B against myocardial ischaemia-reperfusion injury in rats. Lishizhen Med. Materia Medica Res. 22, 412–414. doi:10.3969/j.issn.1008-0805.2011.02.068
Liu, C., Chen, X. D., Sun, J. A., Zhang, S. H., and Zhang, Q. (2022). Study of the protective mechanism of salvinorin B in a rat model of myocardial infarction by inhibiting iron death through modulation of Cx43. Chin. J. Pathophysiol. 38, 1032–1039. doi:10.3969/j.issn.1000-4718.2022.06.010
Liu, H., Liu, W., Qiu, H., Zou, D., Cai, H., Chen, Q., et al. (2020a). Salvianolic acid B protects against myocardial ischaemia-reperfusion injury in rats via inhibiting high mobility group box 1 protein expression through the PI3K/Akt signalling pathway. Schmiedeb. Arch. Pharmacol. 393 (8), 1527–1539. doi:10.1007/s00210-019-01755-7
Liu, J., Chen, L., and Lu, H. (2018). Cardioprotective effect of salvianolic acid B against isoproterenol-induced inflammation and histological changes in a cardiotoxicity rat model. Trop. J. Pharm. Res. 17 (11), 2189–2197. doi:10.4314/tjpr.v17i11.12
Liu, N., Yang, L., and Liu, P. (2020c). Protective effects of Salvia divinorum B on ischaemic myocardial tissue in rats studied based on the PKD1/HDAC7 axis. Chin. Pharmacol. Bull. 36 (12), 1704–1710. doi:10.3969/j.issn.1001-1978.2020.12.014
Liu, N., Yang, L., and Mao, B. Y. (2020b). Angiogenesis promotion function of salvianolic acid B in rats with myocardial infarction via PKD1 - HIF - 1α - VEGF pathway. Chin. Pharmacol. Bull. 36 (7), 984–990. doi:10.3969/j.issn.1001-1978.2020.07.018
Lu, B., Li, J., Gui, M., Yao, L., Fan, M., Zhou, X., et al. (2022). Salvianolic acid B inhibits myocardial I/R-induced ROS generation and cell apoptosis by regulating the TRIM8/GPX1 pathway. Pharm. Biol. 60 (1), 1458–1468. doi:10.1080/13880209.2022.2096644
Marks-Anglin, A., and Chen, Y. (2020). A historical review of publication bias. Res. Synth. Methods 11 (6), 725–742. doi:10.1002/jrsm.1452
Medzikovic, L., Azem, T., Sun, W., Rejali, P., Esdin, L., Rahman, S., et al. (2023). Sex differences in therapies against myocardial ischemia-reperfusion injury: from basic science to clinical perspectives. Cells 12 (16), 2077. doi:10.3390/cells12162077
Page, M. J., McKenzie, J. E., Bossuyt, P. M., Boutron, I., Hoffmann, T. C., Mulrow, C. D., et al. (2021). The PRISMA 2020 statement: an updated guideline for reporting systematic reviews. BMJ 74, 790–799. doi:10.1016/j.rec.2021.07.010Available at: https://www.bmj.com/content/372/bmj.n71.
Pan, C., Lou, L., Huo, Y., Singh, G., Chen, M., Zhang, D., et al. (2011). Salvianolic acid B and Tanshinone IIA attenuate myocardial ischemia injury in mice by no production through multiple pathways. Ther. Adv. Cardiovasc Dis. 5 (2), 99–111. doi:10.1177/1753944710396538
Percie du Sert, N., Hurst, V., Ahluwalia, A., Alam, S., Avey, M. T., Baker, M., et al. (2020). The ARRIVE guidelines 2.0: updated guidelines for reporting animal research. PLoS Biol. 18 (7), e3000410. doi:10.1371/journal.pbio.3000410
Popov, S. V., Mukhomedzyanov, A. V., Voronkov, N. S., Derkachev, I. A., Boshchenko, A. A., Fu, F., et al. (2023). Regulation of autophagy of the heart in ischemia and reperfusion. Apoptosis 28 (1–2), 55–80. doi:10.1007/s10495-022-01786-1
Puymirat, E., Cayla, G., Cottin, Y., Elbaz, M., Henry, P., Gerbaud, E., et al. (2019). Twenty-year trends in profile, management and outcomes of patients with ST-segment elevation myocardial infarction according to use of reperfusion therapy: data from the FAST-MI program 1995-2015. Am. Heart J. 214, 97–106. doi:10.1016/j.ahj.2019.05.007
Qiao, Z., Ma, J., and Liu, H. (2011). Evaluation of the antioxidant potential of salvia miltiorrhiza ethanol extract in a rat model of ischemia-reperfusion injury. Molecules 16 (12), 10002–10012. doi:10.3390/molecules161210002
Qiao, Z., and Xu, Y. (2016). Salvianolic Acid B alleviating myocardium injury in ischemia reperfusion rats. Afr. J. Trad. Complement. Altern. Med. 13 (4), 157–161. doi:10.21010/ajtcam.v13i4.20
Reed, G. W., Rossi, J. E., and Cannon, C. P. (2017). Acute myocardial infarction. Lancet 389 (10065), 197–210. doi:10.1016/S0140-6736(16)30677-8
Ritskes-Hoitinga, M., Leenaars, M., Avey, M., Rovers, M., and Scholten, R. (2014). Systematic reviews of preclinical animal studies can make significant contributions to health care and more transparent translational medicine. Cochrane Database Syst. Rev. 2014 (3), ED000078. doi:10.1002/14651858.ED000078
Sánchez-Hernández, C. D., Torres-Alarcón, L. A., González-Cortés, A., and Peón, A. N. (2020). Ischemia/reperfusion injury: pathophysiology, current clinical management, and potential preventive approaches. Mediat. Inflamm. 2020, 8405370. doi:10.1155/2020/8405370
Sciarretta, S., Maejima, Y., Zablocki, D., and Sadoshima, J. (2018). The role of autophagy in the heart. Annu. Rev. Physiol. 80, 1–26. doi:10.1146/annurev-physiol-021317-121427
Shen, Y., Shen, X. Y., Wang, S. L., Zhang, Y. Y., Wang, Y., Ding, Y., et al. (2022). Protective effects of Salvianolic acid B on rat ferroptosis in myocardial infarction through upregulating the Nrf2 signaling pathway. Int. Immunopharmacol. 112, 109257. doi:10.1016/j.intimp.2022.109257
Shi, B., Ma, M., Zheng, Y., Pan, Y., and Lin, X. (2019). mTOR and Beclin1: two key autophagy-related molecules and their roles in myocardial ischemia/reperfusion injury. J. Cell Physiol. 234 (8), 12562–12568. doi:10.1002/jcp.28125
Song, Y. P., Kong, L. S., Wu, J., Miao, A. R., and Zhao, G. (2007). Comparison of the effect of salvianolic acid A and B on acute myocardial ischemia of rats. Chin. J. Inf. Traditional Chin. Med., 36–38.
Wang, B. H., Fu, W., and Li, Z. T. (2004). Mechanistic study on the myocardial protective effect of salvinorin B on rats with acute myocardial ischaemia/reperfusion injury. J. Tianjin Univ. Traditional Chin. Med. 23 (3), 132–133.
Wang, X., Wang, Y., Jiang, M., Zhu, Y., Hu, L., Fan, G., et al. (2011). Differential cardioprotective effects of salvianolic acid and tanshinone on acute myocardial infarction are mediated by unique signaling pathways. J. Ethnopharmacol. 135 (3), 662–671. doi:10.1016/j.jep.2011.03.070
Wang, X. Y., Wang, M., Sun, S. J., Chen, H. X., Jiang, B. P., and Xu, L. (2016). Correlation between the protection of ischaemic myocardium by salvinorin B and the expression of inflammatory vesicles NLRP3 in rats. Chin. Pharmacol. Bull. 32, 1383–1388. doi:10.3969/j.issn.1001-1978.2016.10.011
Wei, B., Sun, C., Wan, H., Shou, Q., Han, B., Sheng, M., et al. (2023). Bioactive components and molecular mechanisms of Salvia miltiorrhiza Bunge in promoting blood circulation to remove blood stasis. J. Ethnopharmacol. 317, 116697. doi:10.1016/j.jep.2023.116697
Xia, Y., Zhang, H. J., and Nie, Y. L. (2018). Salvianolic acid B pretreatment protects myocardial ischemia/reperfusion injury through improvement of energy metabolism. Drug Eval. Res. 41, 2210–2213. doi:10.7501/j.issn.1674-6376.2018.12.014
Xiang, Q., Yi, X., Zhu, X. H., Wei, X., and Jiang, D. S. (2024). Regulated cell death in myocardial ischemia-reperfusion injury. Trends Endocrinol. Metab. 35 (3), 219–234. doi:10.1016/j.tem.2023.10.010
Xu, J., Yang, X., Sun, L., and Wu, H. (2003). Protective effect of savianolic acid B on myocardial ischemia/reperfusion in rats. Chin. Pharm. J., 35–37.
Xu, L., Deng, Y., Feng, L., Li, D., Chen, X., Ma, C., et al. (2011). Cardio-protection of salvianolic acid b through inhibition of apoptosis network. PLoS ONE 6 (9), e24036. doi:10.1371/journal.pone.0024036Available at: https://www.embase.com/search/results?subaction=viewrecord&id=L362504721&from=export.
Xu, S., Xu, C., Xu, J., Zhang, K., and Zhang, H. (2023b). Macrophage heterogeneity and its impact on myocardial ischemia-reperfusion injury: an integrative review. J. Inflamm. Res. 16, 5971–5987. doi:10.2147/JIR.S436560
Xu, X., Mao, C., Zhang, C., Zhang, M., Gong, J., and Wang, X. (2023a). Salvianolic acid B inhibits ferroptosis and apoptosis during myocardial ischemia/reperfusion injury via decreasing the ubiquitin-proteasome degradation of GPX4 and the ROS-JNK/MAPK pathways. Molecules 28 (10), 4117. doi:10.3390/molecules28104117Available at: https://www.embase.com/search/results?subaction=viewrecord&id=L641431150&from=export.
Xue, L., Wu, Z., Ji, X. P., Gao, X. Q., and Guo, Y. H. (2014). Effect and mechanism of salvianolic acid B on the myocardial ischemia-reperfusion injury in rats. Asian Pac J. Trop. Med. 7 (4), 280–284. doi:10.1016/S1995-7645(14)60038-9
Yang, F. G., Zhang, A. Y., Chen, Z. Y., Lian, Z. X., Liu, G. X., and Dong, G. X. (2008). Effects of salvianolic acid B on cardiovascular endothelial cells and platelet activation in a rabbit model of ischemia-reperfusion. J. Integr. Med. 6, 1250–1254. doi:10.3736/jcim20081208
Yeh, R. W., Sidney, S., Chandra, M., Sorel, M., Selby, J. V., and Go, A. S. (2010). Population trends in the incidence and outcomes of acute myocardial infarction. N. Engl. J. Med. 362 (23), 2155–2165. doi:10.1056/NEJMoa0908610
Zhang, C. H., Yan, Y. J., and Luo, Q. (2024). The molecular mechanisms and potential drug targets of ferroptosis in myocardial ischemia-reperfusion injury. Life Sci. 340, 122439. doi:10.1016/j.lfs.2024.122439
Zhang, L., Yuan, D. P., Xu, L., Jiang, B. P., and Fang, H. T. (2008). Protective mechanism of salvianolic acid B on myocardial ischemia-reperfusion injury of rats. Traditional Chin. Drug Res. Clin. Pharmacol., 467–469. doi:10.19378/j.issn.1003-9783.2008.06.017
Zhang, Q., Yang, L. H., and Li, H. (2013). Protective effect of salvianolic acid B on myocardium of myocardial infarction model rats and its molecular mechanism. J. Jilin Univ. Ed. 39, 268–272. doi:10.7694/jldxyxb20130217
Zhao, G. F., Zhang, H. X., and Fan, Y. C. (2004). Protective effect of salvinorin B on myocardial ischaemia-reperfusion injury in rats. J. Liaoning Univ. Traditional Chin. Med. 6 (1), 55–56. doi:10.13194/j.jlunivtcm.2004.01.56.zhaogf.037
Zhao, K., Chen, X., Bian, Y., Zhou, Z., Wei, X., and Zhang, J. (2023). Broadening horizons: the role of ferroptosis in myocardial ischemia-reperfusion injury. Naunyn Schmiedeb. Arch. Pharmacol. 396 (10), 2269–2286. doi:10.1007/s00210-023-02506-5
Zhao, M., Li, F., Jian, Y., Wang, X., Yang, H., Wang, J., et al. (2020). Salvianolic acid B regulates macrophage polarization in ischemic/reperfused hearts by inhibiting mTORC1-induced glycolysis. Eur. J. Pharmacol. 871, 172916. doi:10.1016/j.ejphar.2020.172916
Zhou, D., Quan, W., Guan, Y., Zhu, Y. R., Guo, C., Wang, Y. H., et al. (2013). Protective effect of salvinorin B on myocardial ischaemia/reperfusion injury in rats via Akt-eNOS pathway. Shaanxi J. Traditional Chin. Med. 34, 104–107.
Keywords: animal model, salvianolic acid B(Sal B), myocardial ischaemia-reperfusion, metaanalysis, preclinical studies
Citation: Yang Y, Sun Z, Sun X, Zhang J, Tong T, Zhang X and Yao K (2024) Protective effect of salvianolic acid B against myocardial ischemia/reperfusion injury: preclinical systematic evaluation and meta-analysis. Front. Pharmacol. 15:1452545. doi: 10.3389/fphar.2024.1452545
Received: 21 June 2024; Accepted: 29 August 2024;
Published: 11 September 2024.
Edited by:
Zhongfeng Li, Capital Normal University, ChinaReviewed by:
Lakshmi Manickavasagam Sundar, M.S. Ramaiah University of Applied Sciences, IndiaCopyright © 2024 Yang, Sun, Sun, Zhang, Tong, Zhang and Yao. This is an open-access article distributed under the terms of the Creative Commons Attribution License (CC BY). The use, distribution or reproduction in other forums is permitted, provided the original author(s) and the copyright owner(s) are credited and that the original publication in this journal is cited, in accordance with accepted academic practice. No use, distribution or reproduction is permitted which does not comply with these terms.
*Correspondence: Kuiwu Yao, eWFva3Vpd3VAMTI2LmNvbQ==
Disclaimer: All claims expressed in this article are solely those of the authors and do not necessarily represent those of their affiliated organizations, or those of the publisher, the editors and the reviewers. Any product that may be evaluated in this article or claim that may be made by its manufacturer is not guaranteed or endorsed by the publisher.
Research integrity at Frontiers
Learn more about the work of our research integrity team to safeguard the quality of each article we publish.