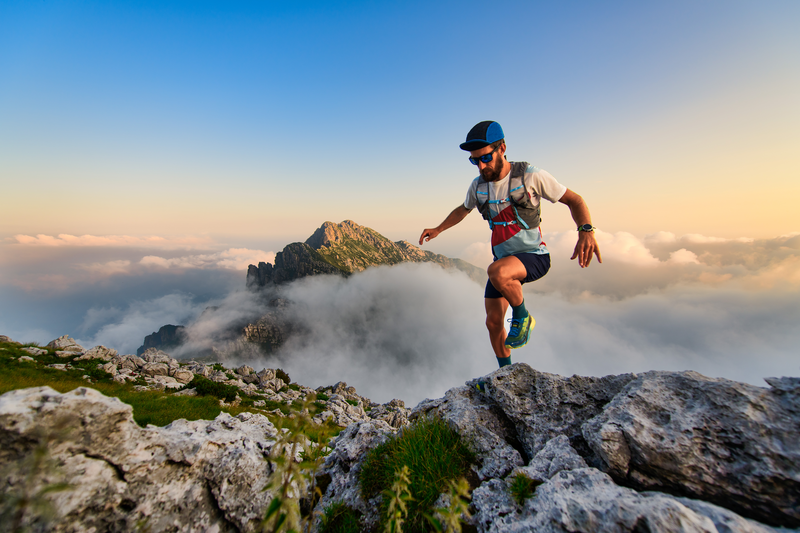
95% of researchers rate our articles as excellent or good
Learn more about the work of our research integrity team to safeguard the quality of each article we publish.
Find out more
SYSTEMATIC REVIEW article
Front. Pharmacol. , 17 December 2024
Sec. Ethnopharmacology
Volume 15 - 2024 | https://doi.org/10.3389/fphar.2024.1478558
This article is part of the Research Topic Efficacy and Mechanism of Herbal Medicines and Their Functional Compounds in Preventing and Treating Cardiovascular Diseases and Cardiovascular Disease Risk Factors - Volume II View all 12 articles
Purpose: Salvianolate for injection (SFI) is a widely used treatment for acute myocardial infarction (AMI). This study aims to assess the efficacy and safety of SFI in treating AMI by synthesizing evidence from published randomized controlled trials (RCTs).
Methods: Seven databases were searched for relevant RCTs published up to 1 July 2024. Two investigators independently conducted the literature searches, data extraction, and quality assessment. Subgroup and sensitivity analyses were performed to address potential heterogeneity. Data analyses were conducted using RevMan 5.4 software.
Result: Thirty RCTs with a total of 3,931 participants were included in the study and analyzed. The results revealed that SFI significantly reduced major adverse cardiac events (MACEs) (RR = 0.34, 95% CI: 0.24 to 0.49, p < 0.05). In addition, SFI lowered creatine kinase-MB (CK-MB) (MD = −5.65, 95% CI: −9.55 to −1.76, p < 0.05) and improved left ventricular ejection fraction (LVEF) (MD = 6.2, 95% CI: 4.82 to 7.57, p < 0.05). Further reductions were observed in C-reactive protein (CRP) (MD = −6.17, 95% CI: −8.11 to −4.23, p < 0.05), malondialdehyde (MDA) (MD = −1.95, 95% CI: −2.08 to −1.83, p < 0.05), and endothelin-1 (ET-1) (MD = −12.27, 95% CI: −17.13 to −7.40, p < 0.05). The incidence of adverse events did not significantly differ between the EG and CG [RR = 0.74, 95% CI: 0.42 to 1.33, p = 0.32].
Conclusion: This study suggests that SFI may be a promising alternative therapy for treating AMI without increasing the risk of adverse events. However, our findings may be limited by the quality of the existing studies. High-quality RCTs are needed to provide more robust evidence.
Systematic Review Registration: https://www.crd.york.ac.uk/PROSPERO/, identifier CRD42024567279.
Acute myocardial infarction (AMI) is a critical cardiovascular condition characterized by the sudden obstruction of coronary arteries, typically resulting from atherosclerotic plaque rupture or thrombus formation, leading to myocardial cell necrosis (Reed et al., 2017). Globally, AMI is responsible for 9.14 million fatalities, constituting 49.2% of all cardiovascular disease (CVD)-related deaths (Nichols et al., 2014; Yeh et al., 2010). The immediate management of AMI prioritizes the rapid restoration of blood flow to the occluded coronary artery to achieve myocardial reperfusion, with percutaneous coronary intervention (PCI) being the preferred therapeutic approach (Gunnar et al., 1990; De Luca et al., 2008). Concurrently, a combination of antiplatelet agents, anticoagulants, vasodilators, and other supportive medications is administered to maintain myocardial function and mitigate further damage (Windecker et al., 2013; Levine et al., 2016; Puymirat et al., 2017; Valgimigli and Gragnano, 2020). Although these interventions effectively reduce myocardial injury in the acute phase, reperfusion injury may occur, potentially causing additional damage to cardiomyocytes during the restoration of blood flow (Puymirat et al., 2017). Moreover, current treatment strategies predominantly focus on acute management, inadequately addressing the long-term structural and functional recovery of the heart. Consequently, AMI patients continue to face elevated morbidity and mortality rates in the long term.
Danshen, scientifically known as Salvia miltiorrhiza Bunge, is a traditional Chinese medicinal botanical drug utilized in the treatment of various conditions, including myocardial infarction, ischemic stroke, and hepatitis (Ho and Hong, 2011; Xu et al., 2018). Salvianolate for injection (SFI), a metabolite derived from Danshen, received approval from the Chinese Food and Drug Administration in 2005 for the treatment of CVD (Cao et al., 2021; Zhang et al., 2016). This formulation primarily comprises salvianolic acid B (Sal-B) and its homologous metabolites (Li W. et al., 2018; Hu et al., 2024). SFI has been reported to confer cardiovascular benefits through multiple mechanisms, including anti-inflammatory, anti-apoptotic, anti-ischemia reperfusion injury, and anti-fibrotic effects (Liang et al., 2021; Xu et al., 2023; Qiu et al., 2018; Jiang et al., 2023; Ma et al., 2019). In China, SFI has been employed in clinical settings as an adjunctive therapy for AMI. However, a comprehensive literature review evaluating its efficacy and safety has not been conducted. Therefore, this study aims to elucidate the cardioprotective effects of SFI in patients with AMI through a systematic review of existing research.
The protocol were registered in the PROSPERO, with registration number CRD42024567279. This study was carried out following the protocol and in compliance with the PRISMA 2020 guidelines.
Studies were included according to the four criteria:
(1) Study type: randomized controlled trials (RCTs) assessing the efficacy and safety of SFI for AMI, with no status, language, or data restrictions;
(2) Participants: patients diagnosed with AMI according to the diagnostic criteria outlined in the 2023 European Society of Cardiology (ESC) guidelines (Byrne et al., 2023), the Fourth Universal Definition of Myocardial Infarction (2018) (Thygesen et al., 2018), or the American College of Cardiology (ACC)/American Heart Association (AHA) guidelines (O'Gara et al., 2013). These criteria include typical ischemic symptoms, electrocardiographic changes indicative of ischemia (such as ST-segment elevation or new left bundle branch block), and elevated cardiac troponin levels with a rise and/or fall indicative of myocardial injury;
(3) Interventions: the experimental groups (EG) received both SFI and conventional therapy (CT), while control groups (CG) received only CT. The courses and dosages of SFI were not restricted;
(4) Outcomes: the primary efficacy outcome was evaluated by major adverse cardiac events (MACEs). The secondary outcomes included myocardial injury markers, such as creatine kinase-MB (CK-MB), cardiac troponin I (cTnI), and lactate dehydrogenase (LDH); cardiac function indices, including N-terminal pro-B-type natriuretic peptide (NT-proBNP), left ventricular ejection fraction (LVEF), left ventricular end-diastolic diameter (LVEDD), left ventricular end-diastolic volume (LVEDV), and left ventricular end-systolic volume (LVESV). Inflammatory markers included C-reactive protein (CRP), tumor necrosis factor-alpha (TNF-α), interleukin-6 (IL-6), and toll-like receptor-4 (TLR-4). Oxidative stress markers were malondialdehyde (MDA) and superoxide dismutase (SOD). Vascular endothelial function was assessed using endothelin-1 (ET-1) and nitric oxide (NO). Hemorheological indicators included whole blood specific viscosity (WBSV), plasma specific viscosity (PSV), and fibrinogen. Platelet function was evaluated by measuring P-selectin (CD62p) and CD63. Safety was assessed by adverse events.
Studies were excluded according to the four criteria:
(1) Unclear reporting of interventions;
(2) Insufficient data for statistical analysis;
(3) Duplicate publications or data;
(4) Conference abstracts, reviews, and technical reports.
The keywords used were “salvianolate,” “salvianolic acids,” “danshen polyphenolate salts,” “acute myocardial infarction,” and “randomized controlled trial” in both English and Chinese. Literature search was carried out independently by two investigators (PFC and HZ) in seven databases, including PubMed, Web of Science, EMBASE, Cochrane Library, CNKI, VIP, and Wanfang. The search period spanned from each database’s inception to 1 July 2024. Additionally, references from similar systematic reviews were manually checked to ensure all relevant studies were included. Detailed search strategies and screening processes are provided in Supplementary Table S1.
Two investigators (PFC and HZ) independently screened studies by examining titles, abstracts, and full texts according to the eligibility criteria. Any disputes were settled by the senior reviewer (DZS).
The following information was extracted, including trial characteristics (first author, publication year, country, follow-up period); patient characteristics (sample size, gender, age); SFI treatments (start time, administration frequency, dosage, duration); and treatment outcomes.
Two investigators (PFC and HZ) independently assessed the quality and risk of bias using the RoB 2 tool proposed by Cochrane (Sterne et al., 2019). The tool used algorithms to assign responses to signaling questions and generate a risk-of-bias judgment. We assessed five domains: the randomization process, deviations from intended interventions, missing outcome data, outcome measurement, and selective reporting. Each was categorized as either “low risk,” “some concerns,” or “high risk.”
In this study, data analysis was performed using the RevMan 5.4. Continuous variables were expressed as weighted mean differences (WMD) and 95% confidence intervals (CIs), while dichotomous variables were presented as pooled risk ratios (RR) and 95% CIs. Heterogeneity among the RCTs was evaluated using the Cochrane’s Q test and I2 statistic. Significant statistical heterogeneity was indicated by p < 0.05 or I2 > 50%, in which case a random-effects model was employed to assess outcomes; otherwise, a fixed-effects model was applied. For analyses involving only two trials, a random-effects model was chosen regardless of heterogeneity significance to ensure result accuracy. A p-value of less than 0.05 denoted a statistically significant difference.
Subgroup and sensitivity analyses were performed to identify the sources of heterogeneity when I2 was ≥50%. Subgroup analysis focused on treatment duration and follow-up time. Sensitivity analysis involved omitting one study at a time to identify sources of heterogeneity related to sample, gender, age, and interventions.
For datasets with 10 or more trials, a funnel plot was used to assess publication bias. While we planned to use Egger’s or Begg’s test, these tests were deemed unreliable for datasets with fewer than 10 trials, and thus were not performed.
Figure 1 illustrates the database search process and study identification. Initially, 343 potentially relevant articles were identified (PubMed: 35, Embase: 42, Web of Science: 38, Cochrane Library: 9, CNKI: 85, Wanfang: 76, VIP: 40, other sources: 18). After excluding 207 duplicate records and 95 ineligible records through title and abstract screening, 41 articles underwent full-text review. Of these, 11 were excluded due to non-RCT study design, inaccurate data, or ineligible interventions. The excluded studies and reasons are detailed in Supplementary Table S2. Ultimately, 30 studies (Shen L. et al., 2020; Ou et al., 2020; Wang, 2018; Wang et al., 2017; Yi et al., 2017; Zu-shan et al., 2016; Wu et al., 2016; Wang et al., 2013; Ye et al., 2014; Qiu, 2019; Zhu and Wang, 2018; Tang, 2023; Liu et al., 2020; Liu et al., 2022; Zhang et al., 2022; Zhang et al., 2017; Hu Xiaochun et al., 2023; Hongmei et al., 2017; Jizheng and Lu, 2020; Guo, 2017; Duan and Jian-Hong, 2016; Dong et al., 2019; He et al., 2014; Hou and Yunfeng, 2018; Li et al., 2020; Zhu and Wang, 2022; Fu, 2024; Zhao, 2021; Lan et al., 2011; Lin et al., 2023) were included in our study.
Table 1 summarizes the characteristics of the 30 RCTs included, involving a total of 3,931 participants (1,972 in the EG and 1,959 in the CG). There were no statistically significant differences in baseline information between the EG and CG. The patients’ ages ranged from 50.38 ± 5.01 to 71.56 ± 8.37 years. The treatment duration with SFI varied from 1 to 21 days. Following the ConPhyMP guidelines (Heinrich et al., 2022), all the included RCTs focused on “type A” extracts, which are listed in the national pharmacopeia and have licensed applications. Detailed information on the source, composition, and chemical properties of SFI used in these trials is provided in Supplementary Table S3.
Among the 30 studies included, all used random sequence generation. However, 12 studies (Wang et al., 2017; Yi et al., 2017; Wang et al., 2013; Ye et al., 2014; Qiu, 2019; Liu et al., 2022; Zhang et al., 2017; Hongmei et al., 2017; Jizheng and Lu, 2020; He et al., 2014; Hou and Yunfeng, 2018; Lan et al., 2011) did not detail the randomization method, resulting in an “some concerns.” These missing details introduce potential risks of selection bias, which could affect the reliability of the reported outcomes. Eighteen studies provided clear descriptions of their randomization methods, including 15 studies (Shen L. et al., 2020; Ou et al., 2020; Wang, 2018; Zu-shan et al., 2016; Wu et al., 2016; Tang, 2023; Zhang et al., 2022; Hu Xiaochun et al., 2023; Guo, 2017; Duan and Jian-Hong, 2016; Dong et al., 2019; Li et al., 2020; Zhu and Wang, 2022; Fu, 2024; Lin et al., 2023) using random number tables, 2 study (Zhu and Wang, 2018; Zhao, 2021) using coin-toss method, 1 study (Liu et al., 2020) using drew lots method, and were evaluated as “low risk.” Regarding measurement of the outcome and mising outcome data. Three study (Shen L. et al., 2020; Ou et al., 2020; Wu et al., 2016) reported some details regarding the methods of allocation concealment, and rated as “low risk.” Three RCTs (Qiu, 2019; Duan and Jian-Hong, 2016; He et al., 2014) were rated as “high risk” due to incomplete data, which could introduce bias into the analysis. The remaining studies were classified as “some concerns” due to insufficient information on these aspects, raising concerns about the potential for unrecognized biases. Regarding the selection of reported results, we rated nine trials (Shen L. et al., 2020; Ou et al., 2020; Wu et al., 2016; Ye et al., 2014; Liu et al., 2020; Guo, 2017; Dong et al., 2019; He et al., 2014; Lan et al., 2011) as “low risk” because the adverse events or adverse reactions were mentioned in the results. However, for the remaining studies, the absence of reported safety events raises the possibility of selective reporting bias (Figure 2).
Nine RCTs (Shen L. et al., 2020; Ou et al., 2020; Wu et al., 2016; Liu et al., 2020; Zhang et al., 2022; Jizheng and Lu, 2020; Hou and Yunfeng, 2018; Zhu and Wang, 2022; Fu, 2024) reported the MACEs. As the heterogeneity was not significant (p = 0.64, I2 = 0%), a fixed-effects model was used to analysis. The result showed that the occurrence of MACEs in the EG was significantly lower than in the CG, with the difference being statistically significant [RR = 0.34, (0.24–0.49), p < 0.05] (Figure 3).
Eight RCTs (Yi et al., 2017; Zhu and Wang, 2018; Hu Xiaochun et al., 2023; Jizheng and Lu, 2020; Guo, 2017; Li et al., 2020; Fu, 2024; Lin et al., 2023) reported the CK-MB. The meta-analysis revealed a significant reduction in CK-MB concentrations in the EG compared to the CG [MD = −5.65, (−9.55 to −1.76), p < 0.05; I2 = 95%, random-effects model]. Six RCTs (Yi et al., 2017; He et al., 2014; Li et al., 2020; Zhu and Wang, 2022; Lan et al., 2011; Lin et al., 2023) reported the cTnI. Meta-analysis showed that cTnI was significantly reduced in EG compared to CG [MD = −1.27, (−1.90 to −0.64), p < 0.05; I2 = 95%]. Three RCTs (Hu Xiaochun et al., 2023; Dong et al., 2019; Fu, 2024) reported the LDH, and meta-analysis showed that the LDH was significantly reduced in EG compared to CG [MD = −17.86, (−23.52 to −12.20), p < 0.05; I2 = 40%] (Figure 4).
Six RCTs (Wang, 2018; Qiu, 2019; Hu Xiaochun et al., 2023; Hongmei et al., 2017; Duan and Jian-Hong, 2016; He et al., 2014; Lan et al., 2011) reported the NT-proBNP. The meta-analysis revealed that the NT-proBNP in the EG was significantly lower compared to the CG [MD = −24.36, (−28.87 to −19.86), p < 0.05; I2 = 8%]. Fifteen RCTs (Shen L. et al., 2020; Wang, 2018; Wu et al., 2016; Qiu, 2019; Tang, 2023; Liu et al., 2020; Liu et al., 2022; Zhang et al., 2017; Hu Xiaochun et al., 2023; Jizheng and Lu, 2020; Duan and Jian-Hong, 2016; He et al., 2014; Hou and Yunfeng, 2018; Fu, 2024; Zhao, 2021; Lin et al., 2023) reported the LVEF, and meta-analysis revealed that the LVEF in EG was significantly higher compared to the CG [MD = 6.2, (4.82–7.57), p < 0.05; I2 = 87%]. Six RCTs (Tang, 2023; Zhang et al., 2017; Jizheng and Lu, 2020; Hou and Yunfeng, 2018; Fu, 2024; Zhao, 2021) reported the LVEDD, and revealed that the LVEDD in EG is significantly lower compared to the CG [MD = −3.06, (−4.01 to −2.12), p < 0.05; I2 = 11%]. Four RCTs (Liu et al., 2022; Hu Xiaochun et al., 2023; Hou and Yunfeng, 2018; Lin et al., 2023) reported the LVEDV, and showed that the LVEDV in EG is significantly lower compared to the CG [MD = −13.75, (−23.33 to −4.16), p < 0.05; I2 = 93%]. Five RCTs (Liu et al., 2022; Hu Xiaochun et al., 2023; Hou and Yunfeng, 2018; Zhao, 2021; Lin et al., 2023) reported the LVESV, and revealed that the LVESV in EG is significantly lower compared to the CG [MD = −8.88, (−13.10 to −4.66), p < 0.05; I2 = 92%] (Figure 5).
Ten RCTs (Wang, 2018; Zu-shan et al., 2016; Qiu, 2019; Jizheng and Lu, 2020; Guo, 2017; Duan and Jian-Hong, 2016; He et al., 2014; Zhao, 2021; Lan et al., 2011; Lin et al., 2023) reported the CRP. The meta results suggested that SFI significantly improved CRP [MD = −6.17, (−8.11 to −4.23), p < 0.05; I2 = 97%]. Six RCTs (Qiu, 2019; Tang, 2023; Zhang et al., 2022; Duan and Jian-Hong, 2016; Zhu and Wang, 2022; Zhao, 2021) reported the TNF-α amd meta results suggested that SFI significantly improved TNF-α [MD = −4.87, (−7.12 to −2.63), p < 0.05; I2 = 96%]. Four RCTs (Qiu, 2019; Zhang et al., 2022; Duan and Jian-Hong, 2016; Li et al., 2020) reported the IL-6, and suggested that SFI significantly improved IL-6 [MD = −11.38, (−17.35 to −5.40), p < 0.05; I2 = 96%]. Three RCTs (Wang, 2018; Wang et al., 2017; Wang et al., 2013) reported the TLR-4, and suggested that SFI significantly improved TLR-4 [MD = −4.28, (−6.42 to −2.14), p < 0.05; I2 = 91%] (Figure 6).
Nine RCTs (Wu et al., 2016; Ye et al., 2014; Qiu, 2019; Liu et al., 2020; Hongmei et al., 2017; Guo, 2017; Duan and Jian-Hong, 2016; Dong et al., 2019; Zhu and Wang, 2022) reported the MDA. Compared with CT, results suggested that SFI can significantly reduce the MDA [MD = −1.95, (−2.08 to −1.83), p < 0.05; I2 = 0%]. Eight RCTs (Zu-shan et al., 2016; Wu et al., 2016; Qiu, 2019; Zhu and Wang, 2018; Hongmei et al., 2017; Duan and Jian-Hong, 2016; Dong et al., 2019; Zhu and Wang, 2022) reported the SOD. Compared with CT, results suggested that SFI can significantly increase the SOD [MD = 22.29, (12.86–31.73), p < 0.05; I2 = 99%] (Figure 7).
Seven RCTs (Wu et al., 2016; Ye et al., 2014; Qiu, 2019; Zhang et al., 2022; Hu Xiaochun et al., 2023; Guo, 2017; Hou and Yunfeng, 2018) reported the ET-1. The meta-analysis indicated that EG was more effective than CG on reducing the level of ET-1 [MD = −12.27, (−17.13 to −7.40), p < 0.05; I2 = 91%]. Eight RCTs (Zu-shan et al., 2016; Wu et al., 2016; Ye et al., 2014; Qiu, 2019; Liu et al., 2020; Zhang et al., 2022; Hu Xiaochun et al., 2023; Guo, 2017) reported the NO, and meta-analysis indicated that EG was more effective than CG on increasing the NO [MD = 15.78, (13.05–18.52), p < 0.05; I2 = 90%] (Figure 8).
Two RCTs (Dong et al., 2019; He et al., 2014) reported the WBSV. The results showed no statistically significant difference in WBSV between EG and CG [MD = −0.41, (−0.84 to 0.03), p > 0.05; I2 = 0%]. Three RCTs (Dong et al., 2019; He et al., 2014; Lin et al., 2023) reported the PSV. The results showed that PSV levels improved better in EG than in CG [MD = −0.54, (−0.62 to −0.46), p < 0.05; I2 = 40%]. Two RCTs (He et al., 2014; Lin et al., 2023) reported the fibrinogen. The results showed that fibrinogen levels improved better in EG than in CG [MD = −0.68, (−1.06 to −0.29), p < 0.05; I2 = 74%] (Figure 9).
Three RCTs (Ye et al., 2014; Qiu, 2019; Guo, 2017) reported the CD62p. Meta-analysis showed that EG was more effective than CG on reducing the level of CD62p [MD = −1.95, (−3.59 to −0.32), p < 0.05; I2 = 97%]. Three RCTs (Ye et al., 2014; Qiu, 2019; Guo, 2017) reported the CD63. Meta-analysis showed that EG was more effective than CG on reducing the level of CD63 [MD = −2.89, (−5.33 to −0.44), p < 0.05; I2 = 98%] (Figure 10).
Nine RCTs (Shen L. et al., 2020; Ou et al., 2020; Wu et al., 2016; Ye et al., 2014; Liu et al., 2020; Guo, 2017; Dong et al., 2019; He et al., 2014; Lan et al., 2011) reported the adverse events. The Meta-analysis showed that the incidence of adverse events did not significantly differ between the EG and CG [RR = 0.74, 95% CI: 0.42 to 1.33, p = 0.32]. Given the non-significant heterogeneity (p = 1.00, I2 = 0%), a fixed-effects model was employed for the analysis (Figure 11). Across the included studies, four studies (Ou et al., 2020; Liu et al., 2020; He et al., 2014; Lan et al., 2011) found no adverse events in both the EG and CG. Other studies documented adverse events related to the AMI, including dizziness, rash, thrombocytopenia, hypotension, hemorrhage, and arrhythmia, with similar frequencies observed between the EG and CG. Overall, the reported adverse events were infrequent and comparable, indicating no major safety concerns related to SFI.
One subgroup analysis stratified by the follow-up duration (1-month, 3-month, and 6-month) indicated that SFI could decrease MACEs at 3-month checkpoint [RR = 0.38, (0.15–0.91), p < 0.05] and 6-month [RR = 0.25, (0.15–0.41), p < 0.05]. Nonetheless, there was no notable difference was observed at the 1-month [RR = 0.52, (0.25–1.09), p > 0.05] (Supplementary Figure 1).
Another subgroup analysis by the treatment duration of SFI (7-days or 14-days), indicated that SFI could decrease MACEs at 7-days checkpoint [RR = 0.49, (0.26–0.92), p < 0.05] and 14-days [RR = 0.29, (0.19–0.45), p < 0.05]. In addition, SFI could decrease CK-MB at 14-days checkpoint [MD = −7.35, (−11.39 to −3.32), p < 0.05]. However, there was no notable difference observed at the 7-days checkpoint of CK-MB [MD = 2.05, (−3.17–7.28), p > 0.05] (Supplementary Figure 2).
When significant heterogeneity was observed (I2 ≥ 50%) in outcomes such as CK-MB, cTnI, LVEF, LVEDV, LVESV, CRP, TNF-α, IL-6, ET-1, and NO, we conducted a sensitivity analysis. This analysis suggested that the variability could be attributed to differences in participant sample size, age, gender, and SFI intervention durations across trials. Excluding these studies substantially reduced the heterogeneity, with minimal impact on the overall results (Supplementary Table S4).
Publication bias for LVEF and CRP was assessed using a funnel plot, as there were ten or more trials available. The results showed a symmetrical inverted funnel shape, indicating a low likelihood of publication bias for both LVEF and CRP (Supplementary Figure 3).
To the best of our knowledge, this is the first study to systematically evaluate the efficacy and safety of SFI for AMI treatment. The findings reveal a substantial decrease in MACEs among AMI patients receiving SFI treatment. Additionally, SFI was found to mitigate myocardial injury, enhance cardiac function, reduce inflammatory responses and oxidative stress, and improve vascular endothelial, hemorheological, and platelet function. These effects likely contribute to the cardioprotective mechanism of action of SFI in AMI. Safety evaluations indicate that SFI does not increase the risk of adverse events, particularly bleeding. These findings highlight the efficacy and safety of SFI, demonstrating enhanced structural and functional outcomes in AMI patients and suggesting SFI as a promising treatment option for AMI.
The chemical metabolites of Danshen extract are primarily categorized into two groups: water-soluble metabolites and lipophilic phenanthraquinones. The principal lipophilic phenanthraquinones include Tanshinone I, Tanshinone IIA, and Tanshinone IIB. The major water-soluble metabolites comprise Danshensu, Rosmarinic Acid, Lithospermic Acid, Salvianolic Acid A, and Sal-B (Ho and Hong, 2011; Wang et al., 2019; Li ZM. et al., 2018). Sal-B, also referred to as lithospermic acid B, is a key bioactive metabolite found in the hydrophilic extracts of Salvia miltiorrhiza, with a molecular formula of C36H30O16 and a molecular weight of 718 (Figure 12). The SFI formula is predominantly composed of Sal-B, which has been recognized for its cardiovascular protective effects, particularly in mitigating oxidative stress, inflammation, and myocardial injury. Sal-B’s magnesium salt derivative, magnesium tanshinoate B (MTB), may offer enhanced therapeutic potential in treating AMI due to the physiological benefits of magnesium ions on cardiac muscle function (Xiao et al., 2020). Given that Sal-B constitutes 80% of the active metabolites in SFI and has been more extensively studied than other metabolites, this investigation primarily focuses on the pharmacological effects of Sal-B in AMI treatment.
Oxidative stress-induced injury plays a crucial role in causing severe and irreversible damage to cardiomyocytes in AMI. Sal-B exhibits potent antioxidant activity, effectively neutralizing free radicals and attenuating myocardial cell damage caused by oxidative stress (Xiao et al., 2020; Wu et al., 2009). The cardioprotective effects of Sal-B are mediated through the enhancement of SOD activity and the reduction of MDA production. Furthermore, Sal-B significantly protects bone marrow-derived endothelial progenitor cells from oxidative stress-induced injury by inhibiting the MKK3/6-p38, MAPK-ATF2, and ERK1/2 signaling pathways, thereby reducing intracellular reactive oxygen species (ROS) levels (Tang et al., 2014). Research conducted by Gao et al. demonstrated that Sal-B protects human umbilical vein endothelial cells (HUVECs) from oxidative stress, partially through the enhancement of autophagy via activation of the AMPK pathway and suppression of the mTOR pathway (Gao S. et al., 2019). Additionally, Lu et al. (2022) reported that Sal-B shields cardiomyocytes from ischemia/reperfusion (I/R)-induced oxidative stress both in vitro and in vivo, a process partially mediated by the TRIM8/GPX1 axis.
The inflammatory response plays a pivotal role in myocardial injury and remodeling following AMI. Sal-B has been demonstrated to inhibit the release of various inflammatory mediators, including CRP, TNF-α, IL-16, and TLR-4, primarily through the modulation of the TNF-α/NF-κB and TLR pathways. Researches conducted by Hu Y. et al. (2019) and Hu et al. (2020) revealed that Sal-B significantly attenuated AMI injury induced by subcutaneous isoproterenol (ISO) injection in a rat model. Sal-B treatment was observed to markedly reduce intracellular ROS production, inhibit NLRP3 inflammasome activation, and decrease apoptosis in H9C2 cardiomyocytes. Furthermore, Sal-B has been shown to promote the formation of anti-inflammatory M2 macrophages by regulating macrophage polarization, thereby mitigating myocardial damage caused by the inflammatory response (Li et al., 2021; Zou et al., 2022).
Cardiomyocyte apoptosis represents a key mechanism of myocardial injury following AMI. Sal-B has been found to reduce markers of myocardial damage, including CK-MB, cTnI, and LDH. The anti-apoptotic effects of Sal-B are mediated through the regulation of the Bcl-2/Bax expression ratio and the suppression of caspase activation (Wang et al., 2022; Chen HM. et al., 2017). Additionally, Sal-B exerts cardioprotective effects by activating the PI3K/Akt signaling pathway, thereby enhancing cell survival signals (Liu et al., 2007). Research conducted by Liu et al. (Liu et al., 2020) demonstrated that Sal-B significantly ameliorated myocardial I/R injury in a dose-dependent manner. This protective effect was characterized by a reduction in myocardial infarction size, decreased expression of myocardial injury markers, attenuated cell apoptosis, enhanced PI3K/Akt expression, and inhibition of high mobility group box 1 (HMGB1) expression.
Sal-B has been shown to exhibit antiplatelet and anticoagulant properties through multiple mechanisms. The metabolite reduces the expression of CD62p on the platelet surface and inhibits platelet activation induced by adenosine diphosphate (ADP), collagen, and thrombin (Chen et al., 2023). Moreover, Sal-B demonstrates anticoagulant effects by decreasing thrombin production and fibrin formation, thereby preventing blood clot formation. A study conducted by Liu et al. (Liu et al., 2014) elucidated two primary mechanisms underlying the antiplatelet effects of Sal-B: firstly, as a P2Y12 receptor antagonist, Sal-B significantly inhibits the interaction between ADP and the P2Y12 receptor; secondly, it inhibits phosphodiesterase (PDE) activity in platelets, preventing cyclic adenosine monophosphate (cAMP) degradation and thus suppressing platelet activation. Furthermore, Sal-B has been observed to reduce Ca2+ mobilization within platelets, further contributing to its antiplatelet aggregation effects (Song et al., 2017; Chen RC. et al., 2017).
Endothelial dysfunction has been identified as a crucial factor in the pathophysiology of AMI. Sal-B has been demonstrated to improve endothelial function through the promotion of endothelial cell survival and repair mechanisms. Extensive research has elucidated that Sal-B enhances the synthesis and release of NO, thereby improving vasodilation and reducing endothelial cell apoptosis (Ling et al., 2017). Furthermore, Sal-B exerts protective effects on endothelial cells by attenuating oxidative stress and inflammatory responses (Ren et al., 2016; Ko et al., 2020). A study conducted by Chen et al. (Chen et al., 2001) revealed that Sal-B primarily safeguards vascular endothelial cells through the concentration-dependent reduction of vascular cell adhesion molecule-1 (VCAM-1) and intercellular adhesion molecule-1 (ICAM-1) activity.
Pharmacological investigations have confirmed that hemodynamic alterations represent a primary etiological factor in thrombosis formation. Fibrinogen plays a pivotal role in platelet aggregation, a reduction in fibrinogen levels can lead to decreased thrombus formation. Sal-B has been shown to significantly lower fibrinogen and lipid peroxide levels, while concomitantly increasing high-density lipoprotein (HDL) concentrations. These effects contribute to improved blood viscosity and a reduction in the nitric oxide/endothelin (NO/ET) ratio (Dong et al., 2019; He et al., 2014; Lin et al., 2023). Moreover, Sal-B enhances vascular dilation and improves hemodynamics through the modulation of vascular smooth muscle cell function (He H. et al., 2008). Clinical studies have demonstrated that Sal-B administration significantly reduces blood viscosity, increases red blood cell deformability, and improves microcirculation in patients with AMI (He HB. et al., 2008).
Sal-B has been demonstrated to mitigate fibrosis and cardiac remodeling while promoting angiogenesis. In AMI rat models, Sal-B selectively inhibits MMP-9 activity, effectively augments left ventricular wall thickness, enhances cardiac contractility, and attenuates myocardial fibrosis (Ma et al., 2015; Sun et al., 2021; Luo et al., 2023). The metabolite inhibits type I collagen production in the LX-2 cell line under non-TGF-β2 stimulation and exerts anti-fibrotic effects through the inhibition of p38 and ERK signaling pathways (Luo et al., 2023; Gao H. et al., 2019; Li et al., 2020). Clinical studies have revealed that Sal-B significantly elevates serum VEGF levels in AMI patients, thereby promoting vascular endothelial cell proliferation and migration and facilitating new blood vessel formation (He HB. et al., 2008; Chen et al., 2022; Dhapare and Sakagami, 2018). Furthermore, Sal-B enhances hypoxia-induced angiogenesis and promotes myocardial repair through the activation of the HIF-1α signaling pathway (Yang et al., 2016; Wei et al., 2018).
The findings of this study suggest that SFI may play a therapeutic role in the AMI population. Subgroup analysis further revealed that SFI significantly reduced MACEs at 3 and 6 months follow-up, while no significant reduction was observed at 1 month. These results indicate that SFI can potentially reduce mortality and the incidence of recurrent myocardial infarction, thereby improving the long-term prognosis of AMI patients. Additional subgroup analysis demonstrated SFI’s efficacy in reducing MACE at 7 and 14 days of treatment. However, SFI reduced CK-MB at 14 days, with no significant difference observed at 7 days. Based on these findings, a treatment duration of 2 weeks is recommended.
Our findings contribute to the growing body of evidence on the clinical applications of SFI. Yang et al. (Yang et al., 2020) conducted a retrospective analysis using national health insurance data in China to assess the economic impact of SFI in coronary heart disease (CHD). The study demonstrated that SFI was associated with lower hospitalization costs and shorter hospital stays compared to other treatments, such as Danhong and alprostadil injections, highlighting its potential to reduce the financial burden on patients and healthcare systems. However, their focus on economic outcomes left a gap in clinical efficacy assessment. By integrating our RCT-based clinical findings with Yang et al.’s economic insights, we emphasize the dual value of SFI—not only as a clinically effective treatment for AMI but also as a cost-efficient therapy for broader cardiovascular care.
Similarly, Shen Y. et al. (2020) explored SFI’s therapeutic potential in a different clinical setting—diabetic nephropathy. Their meta-analysis revealed that SFI improved renal function, reduced oxidative stress, and lowered inflammatory markers, demonstrating its potential beyond cardiovascular conditions. These therapeutic effects align with the cardioprotective benefits observed in our study. However, both studies, including ours, face limitations such as variability in methodological quality, differences in treatment regimens, and a lack of long-term follow-up. These limitations underscore the need for larger, high-quality RCTs to validate salvianolate’s long-term efficacy and safety across different clinical populations and to explore optimal dosing strategies for both cardiovascular and non-cardiovascular applications.
This study highlights SFI as a promising alternative treatment option for AMI. Despite these encouraging results, several key areas warrant further investigation. While SFI has demonstrated notable effectiveness in reducing MACEs, determining the optimal dosage to ensure maximum efficacy and minimal side effects remains crucial. Future research should prioritize dosage optimization and conduct long-term follow-ups to evaluate the sustained efficacy and safety of SFI across diverse patient populations. The study also reveals SFI’s significant improvement of myocardial injury markers, cardiac function, and inflammatory responses. However, its precise mechanisms of action remain elucidated. Future studies should delve into the molecular and cellular mechanisms of SFI to enhance understanding of its role in AMI management and to identify potential biological pathways and targets.
This study presents several notable strengths. Firstly, a comprehensive search was conducted across multiple databases without language or time restrictions. Secondly, the involvement of two independent investigators in study selection, data extraction, and bias assessment minimized the potential for errors. Thirdly, the application of rigorous standards in assessing and reviewing eligible trials ensured methodological robustness and aimed to draw unbiased conclusions. Lastly, an in-depth discussion of the mechanism of Sal-B in AMI treatment was provided.
Nevertheless, certain limitations must be acknowledged. Our findings should be interpreted with caution due to the risk of bias identified in some included studies. In particular, the absence of clear randomization methods and incomplete outcome reporting in some studies raise serious concerns about selection and reporting biases. These limitations suggest that some of the included studies may not be sufficiently reliable for assessing the efficacy and safety of SFI. To address these concerns, we conducted sensitivity analyses by excluding high-risk studies and found that the main results remained consistent, indicating a certain degree of robustness. In addition, subgroup analyses based on study characteristics showed no significant differences, further supporting the stability of our findings. However, the quality of safety reporting across the studies remains a concern. Some studies reported no adverse events, which could reflect either genuine safety or incomplete monitoring, increasing the possibility of selective reporting bias. Moreover, we acknowledge that the overall quality of the evidence remains a concern, and the limitations of some included studies restrict the strength of our conclusions. Therefore, while our findings provide preliminary evidence for the therapeutic potential of SFI, future studies should employ rigorous RCT designs with clear randomization methods, transparent reporting, and comprehensive outcome documentation to ensure more reliable and conclusive assessments.
This study suggests that SFI may be a promising alternative therapy for treating AMI without increasing the risk of adverse events. However, our findings may be limited by the quality of the existing studies. High-quality RCTs are needed to provide more robust evidence.
The original contributions presented in the study are included in the article/Supplementary Material, further inquiries can be directed to the corresponding authors.
PC: Data curation, Writing–original draft, Writing–review and editing. HZ: Software, Writing–original draft. ZG: Conceptualization, Data curation, Writing–review and editing. DS: Conceptualization, Writing–review and editing. JZ: Data curation, Formal Analysis, Writing–review and editing.
The author(s) declare that financial support was received for the research, authorship, and/or publication of this article. This work was supported by the project of Hospital capability enhancement project of Xiyuan Hospital, CACMS. (NO. XYZX0404-15, NO. XYZX0204-02, and NO. XYZX0201-03).
The authors declare that the research was conducted in the absence of any commercial or financial relationships that could be construed as a potential conflict of interest.
All claims expressed in this article are solely those of the authors and do not necessarily represent those of their affiliated organizations, or those of the publisher, the editors and the reviewers. Any product that may be evaluated in this article, or claim that may be made by its manufacturer, is not guaranteed or endorsed by the publisher.
The Supplementary Material for this article can be found online at: https://www.frontiersin.org/articles/10.3389/fphar.2024.1478558/full#supplementary-material
SUPPLEMENTARY FIGURE S1 | Subgroup analysis stratified by follow-up time of MACEs.
SUPPLEMENTARY FIGURE S2 | Subgroup analysis stratified by treatment time of SAI [(A) MACEs; (B) CK-MB].
SUPPLEMENTARY FIGURE S3 | The publication bias [(A) LVEF; (B) CRP].
Byrne, R. A., Rossello, X., Coughlan, J. J., Barbato, E., Berry, C., Chieffo, A., et al. (2023). 2023 ESC Guidelines for the management of acute coronary syndromes. Eur. Heart J. 44 (38), 3720–3826. doi:10.1093/eurheartj/ehad191
Cao, W., Yang, Q., Zhang, W., Xu, Y., Wang, S., Wu, Y., et al. (2021). Drug-drug interactions between salvianolate injection and aspirin based on their metabolic enzymes. Biomed. Pharmacother. 135, 111203. doi:10.1016/j.biopha.2020.111203
Chen, H. M., Luo, H., Zeng, W. B., Liu, B., Huang, J. C., Liu, M., et al. (2017a). Salvianolic acid B attenuates oxidized low-density lipoprotein-induced endothelial cell apoptosis through inhibition of oxidative stress, p53, and caspase-3 pathways. Chin. J. Integr. Med. doi:10.1007/s11655-016-2645-4
Chen, J., Wang, Y., Wang, S., Zhao, X., and Zhao, L. (2022). Salvianolic acid B and ferulic acid synergistically promote angiogenesis in HUVECs and zebrafish via regulating VEGF signaling. J. Ethnopharmacol. 283, 114667. doi:10.1016/j.jep.2021.114667
Chen, Q., Xu, Q., Zhu, H., Wang, J., Sun, N., Bian, H., et al. (2023). Salvianolic acid B promotes angiogenesis and inhibits cardiomyocyte apoptosis by regulating autophagy in myocardial ischemia. Chin. Med. 18 (1), 155. doi:10.1186/s13020-023-00859-w
Chen, R. C., Sun, G. B., Ye, J. X., Wang, J., Zhang, M. D., and Sun, X. B. (2017b). Salvianolic acid B attenuates doxorubicin-induced ER stress by inhibiting TRPC3 and TRPC6 mediated Ca(2+) overload in rat cardiomyocytes. Toxicol. Lett. 276, 21–30. doi:10.1016/j.toxlet.2017.04.010
Chen, Y. H., Lin, S. J., Ku, H. H., Shiao, M. S., Lin, F. Y., Chen, J. W., et al. (2001). Salvianolic acid B attenuates VCAM-1 and ICAM-1 expression in TNF-alpha-treated human aortic endothelial cells. J. Cell Biochem. 82 (3), 512–521. doi:10.1002/jcb.1176
De Luca, G., Suryapranata, H., and Marino, P. (2008). Reperfusion strategies in acute ST-elevation myocardial infarction: an overview of current status. Prog. Cardiovasc Dis. 50 (5), 352–382. doi:10.1016/j.pcad.2007.11.004
Dhapare, S., and Sakagami, M. (2018). Salvianolic acid B as an anti-emphysema agent I: in vitro stimulation of lung cell proliferation and migration, and protection against lung cell death, and in vivo lung STAT3 activation and VEGF elevation. Pulm. Pharmacol. Ther. 53, 107–115. doi:10.1016/j.pupt.2018.10.001
Dong, Y., Li, Y., Luo, S., and Duan, B. (2019). Application of salvianolate in the treatment of ischemic myocardial injury in emergency patients. Shaanxi Chin. Med. 40 (07), 871–874. doi:10.3969/j.issn.1000-7369.2019.07.016
Duan, X., and Jian-Hong, L. (2016). Effect of salvianolate in the treatment of acute myocardial infarction with hypertension after PCI. J. Qiqihar Med. Coll. 37 (31), 3911–3912.
Fu, X. (2024). Effect of coronary intervention combined with salvianolate in the treatment of hypertension and acute myocardial infarction. Knowl. Prev. Treat. Cardiovasc. Dis. 14 (02), 34–37.
Gao, H., Bo, Z., Wang, Q., Luo, L., Zhu, H., and Ren, Y. (2019b). Salvanic acid B inhibits myocardial fibrosis through regulating TGF-β1/Smad signaling pathway. Biomed. Pharmacother. 110, 685–691. doi:10.1016/j.biopha.2018.11.098
Gao, S., Li, S., Li, Q., Zhang, F., Sun, M., Wan, Z., et al. (2019a). Protective effects of salvianolic acid B against hydrogen peroxide-induced apoptosis of human umbilical vein endothelial cells and underlying mechanisms. Int. J. Mol. Med. 44 (2), 457–468. doi:10.3892/ijmm.2019.4227
Gunnar, R. M., Bourdillon, P. D., Dixon, D. W., Fuster, V., Karp, R. B., Kennedy, J. W., et al. (1990). ACC/AHA guidelines for the early management of patients with acute myocardial infarction. A report of the American College of Cardiology/American Heart Association Task Force on Assessment of Diagnostic and Therapeutic Cardiovascular Procedures (subcommittee to develop guidelines for the early management of patients with acute myocardial infarction). Circulation 82 (2), 664–707. doi:10.1161/01.cir.82.2.664
Guo, X. (2017). Effect of salvianolate in the treatment of acute myocardial infarction with hypertension after PCI. J. Med. Forum 38 (04), 13–16.
He, H., Shi, M., Yang, X., Zeng, X., Wu, L., and Li, L. (2008a). Comparison of cardioprotective effects using salvianolic acid B and benazepril for the treatment of chronic myocardial infarction in rats. Naunyn Schmiedeb. Arch. Pharmacol. 378 (3), 311–322. doi:10.1007/s00210-008-0287-6
He, H. B., Yang, X. Z., Shi, M. Q., Zeng, X. W., Wu, L. M., and Li, L. D. (2008b). Comparison of cardioprotective effects of salvianolic acid B and benazepril on large myocardial infarction in rats. Pharmacol. Rep. 60 (3), 369–381.
He, T., He, Q., Zhu, Y., Wu, J., and Xu, S. (2014). Clinical study of salvianolate in treatment of non-ST segment elevation myocardial infarction. Chin. J. General Med. 12 (05), 735–736. doi:10.16766/j.cnki.issn.1674-4152.2014.05.030
Heinrich, M., Jalil, B., Abdel-Tawab, M., Echeverria, J., Kulić, Ž., McGaw, L. J., et al. (2022). Best Practice in the chemical characterisation of extracts used in pharmacological and toxicological research-The ConPhyMP-Guidelines. Front. Pharmacol. 13, 953205. doi:10.3389/fphar.2022.953205
Ho, J. H., and Hong, C. Y. (2011). Salvianolic acids: small compounds with multiple mechanisms for cardiovascular protection. J. Biomed. Sci. 18 (1), 30. doi:10.1186/1423-0127-18-30
Hongmei, L., Pin, F., and Zhang, W. (2017). Effects of salvianolate combined with atorvastatin on oxidative stress injury and inflammatory factors in elderly patients with acute myocardial infarction. Hebei Med. 39 (12), 1842–1844. doi:10.3969/j.issn.1002-7386.2017.12.024
Hou, L., and Yunfeng, F. (2018). Effects of salvianolate injection on myocardial injury, neuroendocrine hormone, vascular endothelial function and cardiac function associated with PCI. J. Mod. Integr. Chin. West. Med. 27 (23), 2573–2576. doi:10.3969/j.issn.1008-8849.2018.23.019
Hu, S., Wen, J., Fan, X. D., and Li, P. (2024). Study on therapeutic mechanism of total salvianolic acids against myocardial ischemia-reperfusion injury based on network pharmacology, molecular docking, and experimental study. J. Ethnopharmacol. 326, 117902. doi:10.1016/j.jep.2024.117902
Hu, X., Cong, Y., Ma, R., Zhou, Y., and Li, H. (2023). Perioperative clinical observation of salvianolate assisted percutaneous coronary intervention for acute myocardial infarction. Chin. Pharm. Ind. 32 (18), 93–97. doi:10.3969/j.issn.1006-4931.2023.18.020
Hu, Y., Li, Q., Pan, Y., and Xu, L. (2019). Sal-B alleviates myocardial ischemic injury by inhibiting TLR4 and the priming phase of NLRP3 inflammasome. Molecules 24 (23), 4416. doi:10.3390/molecules24234416
Hu, Y., Wang, X., Li, Q., Pan, Y., and Xu, L. (2020). Salvianolic acid B alleviates myocardial ischemic injury by promoting mitophagy and inhibiting activation of the NLRP3 inflammasome. Mol. Med. Rep. 22 (6), 5199–5208. doi:10.3892/mmr.2020.11589
Jiang, Y., Cai, Y., Han, R., Xu, Y., Xia, Z., and Xia, W. (2023). Salvianolic acids and its potential for cardio-protection against myocardial ischemic reperfusion injury in diabetes. Front. Endocrinol. (Lausanne) 14, 1322474. doi:10.3389/fendo.2023.1322474
Jizheng, L., and Lu, Y. (2020). Effect of salvianolate combined with ticagrelor in the treatment of ST-segment elevation myocardial infarction and its influence on cardiac function indexes and serological indexes. J. Qiqihar Med. Coll. 41 (10), 1255–1257. doi:10.3969/j.issn.1002-1256.2020.10.028
Ko, Y. S., Jin, H., Park, S. W., and Kim, H. J. (2020). Salvianolic acid B protects against oxLDL-induced endothelial dysfunction under high-glucose conditions by downregulating ROCK1-mediated mitophagy and apoptosis. Biochem. Pharmacol. 174, 113815. doi:10.1016/j.bcp.2020.113815
Lan, N., Peng, X., and Wu, X. (2011). Clinical observation of salvianolate injection combined with percutaneous coronary intervention in the treatment of non-ST segment elevation myocardial infarction. Shanghai J. Chin. Med. 45 (01), 41–43. doi:10.16305/j.1007-1334.2011.01.027
Levine, G. N., Bates, E. R., Bittl, J. A., Brindis, R. G., Fihn, S. D., Fleisher, L. A., et al. (2016). 2016 ACC/AHA guideline focused update on duration of dual antiplatelet therapy in patients with coronary artery disease: a report of the American College of Cardiology/American heart association task force on clinical practice guidelines: an update of the 2011 ACCF/AHA/SCAI guideline for percutaneous coronary intervention, 2011 ACCF/AHA guideline for coronary artery bypass graft surgery, 2012 ACC/AHA/ACP/AATS/PCNA/SCAI/STS guideline for the diagnosis and management of patients with stable ischemic heart disease, 2013 ACCF/AHA guideline for the management of ST-elevation myocardial infarction, 2014 AHA/ACC guideline for the management of patients with non-ST-elevation acute coronary syndromes, and 2014 ACC/AHA guideline on perioperative cardiovascular evaluation and management of patients undergoing noncardiac surgery. Circulation 134 (10), e123–e155. doi:10.1161/CIR.0000000000000404
Li, C. L., Liu, B., Wang, Z. Y., Xie, F., Qiao, W., Cheng, J., et al. (2020). Salvianolic acid B improves myocardial function in diabetic cardiomyopathy by suppressing IGFBP3. J. Mol. Cell Cardiol. 139, 98–112. doi:10.1016/j.yjmcc.2020.01.009
Li, J., Chen, Y. H., Li, L. Z., Wang, F., Song, W., Alolga, R. N., et al. (2021). Omics and transgenic analyses reveal that salvianolic acid B exhibits its anti-inflammatory effects through inhibiting the mincle-syk-related pathway in macrophages. J. Proteome Res. 20 (7), 3734–3748. doi:10.1021/acs.jproteome.1c00325
Li, W., Polachi, N., Wang, X., Chu, Y., Wang, Y., Tian, M., et al. (2018a). A quality marker study on salvianolic acids for injection. Phytomedicine 44, 138–147. doi:10.1016/j.phymed.2018.02.003
Li, Z. M., Xu, S. W., and Liu, P. Q. (2018b). Salvia miltiorrhizaBurge (Danshen): a golden herbal medicine in cardiovascular therapeutics. Acta Pharmacol. Sin. 39 (5), 802–824. doi:10.1038/aps.2017.193
Li, S., Wu, Z., Chang, Y., and Ding, H. (2020). Effect of salvianolate injection combined with Rosuvastatin in the treatment of acute myocardial infarction and its effect on inflammatory response. Chin. J. Med. 22 (01), 24–27.
Liang, Y., Liu, H., Fang, Y., Lin, P., Lu, Z., Zhang, P., et al. (2021). Salvianolate ameliorates oxidative stress and podocyte injury through modulation of NOX4 activity in db/db mice. J. Cell Mol. Med. 25 (2), 1012–1023. doi:10.1111/jcmm.16165
Lin, W., Liu, Z., and Bo, L. (2023). Effect of recombinant human TNK tissue type plasminogen activator combined with salvianolic acid on quality of life and prognosis of patients with acute myocardial infarction after PCI. Jilin Med. Sci. 44 (08), 2276–2279.
Ling, W. C., Liu, J., Lau, C. W., Murugan, D. D., Mustafa, M. R., and Huang, Y. (2017). Treatment with salvianolic acid B restores endothelial function in angiotensin II-induced hypertensive mice. Biochem. Pharmacol. 136, 76–85. doi:10.1016/j.bcp.2017.04.007
Liu, C. L., Xie, L. X., Li, M., Durairajan, S. S. K., Goto, S., and Huang, J. D. (2007). Salvianolic acid B inhibits hydrogen peroxide-induced endothelial cell apoptosis through regulating PI3K/Akt signaling. PLoS One 2 (12), e1321. doi:10.1371/journal.pone.0001321
Liu, H., Liu, W., Qiu, H., Zou, D., Cai, H., Chen, Q., et al. (2020). Salvianolic acid B protects against myocardial ischaemia-reperfusion injury in rats via inhibiting high mobility group box 1 protein expression through the PI3K/Akt signalling pathway. Naunyn Schmiedeb. Arch. Pharmacol. 393 (8), 1527–1539. doi:10.1007/s00210-019-01755-7
Liu, L., Li, J., Zhang, Y., Zhang, S., Ye, J., Wen, Z., et al. (2014). Salvianolic acid B inhibits platelets as a P2Y12 antagonist and PDE inhibitor: evidence from clinic to laboratory. Thromb. Res. 134 (4), 866–876. doi:10.1016/j.thromres.2014.07.019
Liu, T., Cao, G., Wang, W., Wang, L., Liu, Y., and Li, N. (2020). Effects of salvianolate on oxidative stress, cardiac function and vascular endothelial function in patients with emergency PCI. J. Mod. Chin. West. Integr. Med. 29 (10), 1089–1092. doi:10.3969/j.issn.1008-8849.2020.10.016
Liu, Z., Yuan, Y., and Chen, Y. (2022). Effects of salvianolate on cardiac function and coagulation function in elderly patients with acute myocardial infarction after PCI. Guizhou Med. 46 (10), 1623–1625.
Lu, B., Li, J., Gui, M., Yao, L., Fan, M., Zhou, X., et al. (2022). Salvianolic acid B inhibits myocardial I/R-induced ROS generation and cell apoptosis by regulating the TRIM8/GPX1 pathway. Pharm. Biol. 60 (1), 1458–1468. doi:10.1080/13880209.2022.2096644
Luo, H., Fu, L., Wang, X., Yini, X., Ling, T., and Shen, X. (2023). Salvianolic acid B ameliorates myocardial fibrosis in diabetic cardiomyopathy by deubiquitinating Smad7. Chin. Med. 18 (1), 161. doi:10.1186/s13020-023-00868-9
Ma, L., Guan, Y. Q., and Du, Z. D. (2015). Salvianolic acid B down-regulates matrix metalloproteinase-9 activity and expression in tumor necrosis factor-α-induced human coronary artery endothelial cells. Chin. Med. J. Engl. 128 (19), 2658–2663. doi:10.4103/0366-6999.166037
Ma, L., Tang, L., and Yi, Q. (2019). Salvianolic acids: potential source of natural drugs for the treatment of fibrosis disease and cancer. Front. Pharmacol. 10, 97. doi:10.3389/fphar.2019.00097
Nichols, M., Townsend, N., Scarborough, P., and Rayner, M. (2014). Cardiovascular disease in Europe 2014: epidemiological update. Eur. Heart J. 35 (42), 2929. doi:10.1093/eurheartj/ehu378
O'Gara, P. T., Kushner, F. G., Ascheim, D. D., Casey, D. E., Chung, M. K., de Lemos, J. A., et al. (2013). 2013 ACCF/AHA guideline for the management of ST-elevation myocardial infarction: a report of the American College of Cardiology foundation/American heart association task force on practice guidelines. Circulation 127 (4), e362–e425. doi:10.1161/CIR.0b013e3182742cf6
Ou, Y., Sun, S. J., Shi, H. M., Luo, J. F., Luo, X. P., Shen, Y. Z., et al. (2020). Protective effects of salvianolate on myocardial injury or myocardial infarction after elective percutaneous coronary intervention in NSTE-ACS patients: a randomized placebo-controlled trial. Chin. J. Integr. Med. 26 (9), 656–662. doi:10.1007/s11655-020-2728-0
Puymirat, E., Simon, T., Cayla, G., Cottin, Y., Elbaz, M., Coste, P., et al. (2017). Acute myocardial infarction: changes in patient characteristics, management, and 6-month outcomes over a period of 20 Years in the FAST-MI program (French registry of acute ST-elevation or non-ST-elevation myocardial infarction) 1995 to 2015. Circulation 136 (20), 1908–1919. doi:10.1161/CIRCULATIONAHA.117.030798
Qiu, H., Liu, W., Lan, T., Pan, W., Chen, X., Wu, H., et al. (2018). Salvianolate reduces atrial fibrillation through suppressing atrial interstitial fibrosis by inhibiting TGF-β1/Smad2/3 and TXNIP/NLRP3 inflammasome signaling pathways in post-MI rats. Phytomedicine 51, 255–265. doi:10.1016/j.phymed.2018.09.238
Qiu, J. (2019). Protective effect of salvianolate on myocardium in patients with acute myocardial infarction after PCI. J. Cardio-Cerebrovascular Dis. Integr. Chin. West. Med. 17 (02), 256–259. doi:10.12102/j.issn.1672-1349.2019.02.026
Reed, G. W., Rossi, J. E., and Cannon, C. P. (2017). Acute myocardial infarction. Lancet 389 (10065), 197–210. doi:10.1016/S0140-6736(16)30677-8
Ren, Y., Tao, S., Zheng, S., Zhao, M., Zhu, Y., Yang, J., et al. (2016). Salvianolic acid B improves vascular endothelial function in diabetic rats with blood glucose fluctuations via suppression of endothelial cell apoptosis. Eur. J. Pharmacol. 791, 308–315. doi:10.1016/j.ejphar.2016.09.014
Shen, L., Xu, R., Yin, J., Lu, H., Zhang, S., Huang, Z., et al. (2020a). Effects of salvianolate on myocardial perfusion after primary percutaneous catheter intervention in patients with ST-segment elevation myocardial infarction: a multicenter, randomized, double-blind, placebo-controlled study. Ann. Transl. Med. 8 (18), 1185. doi:10.21037/atm-20-6081
Shen, Y., Wang, S., Liu, Y., Ge, L., Xia, L., Zhang, X., et al. (2020b). The effects of salvianolate combined with western medicine on diabetic nephropathy: a systematic review and meta-analysis. Front. Pharmacol. 11, 851. doi:10.3389/fphar.2020.00851
Song, Q., Han, X., Xue, Y., Song, T., Chu, X., Zhang, X., et al. (2017). Effects of salvianolic acid B on L-type calcium channels and myocardial contractility in isolated rat ventricular myocytes and hERG K(+) channels expressed in HEK293 cells. Naunyn Schmiedeb. Arch. Pharmacol. 390 (8), 791–799. doi:10.1007/s00210-017-1381-4
Sterne, J., Savović, J., Page, M. J., Elbers, R. G., Blencowe, N. S., Boutron, I., et al. (2019). RoB 2: a revised tool for assessing risk of bias in randomised trials. BMJ 366, l4898. doi:10.1136/bmj.l4898
Sun, Z., Luo, D., Wang, S., Huang, R., and Dong, P. (2021). Salvianolic acid B inhibits myofibroblast differentiation and extracellular matrix accumulation in nasal polyp fibroblasts via the TGF-β1 signaling pathway. Mol. Med. Rep. 23 (6), 478. doi:10.3892/mmr.2021.12117
Tang, C. (2023). Effects of salvianolate on cardiac function and microinflammatory indexes in patients with acute myocardial infarction. Halbin Med. 43 (06), 6–8. doi:10.3969/j.issn.1001-8131.2023.06.003
Tang, Y., Jacobi, A., Vater, C., Zou, X., and Stiehler, M. (2014). Salvianolic acid B protects human endothelial progenitor cells against oxidative stress-mediated dysfunction by modulating Akt/mTOR/4EBP1, p38 MAPK/ATF2, and ERK1/2 signaling pathways. Biochem. Pharmacol. 90 (1), 34–49. doi:10.1016/j.bcp.2014.04.008
Thygesen, K., Alpert, J. S., Jaffe, A. S., Chaitman, B. R., Bax, J. J., Morrow, D. A., et al. (2018). Fourth universal definition of myocardial infarction (2018). J. Am. Coll. Cardiol. 72 (18), 2231–2264. doi:10.1016/j.jacc.2018.08.1038
Valgimigli, M., and Gragnano, F. (2020). The benefit and harm of treating STEMI: between ischemia, bleeding, and reperfusion injury. Eur. Heart J. Acute Cardiovasc Care 9 (4), 259–261. doi:10.1177/2048872620934298
Wang, J., Xu, J., Gong, X., Yang, M., Zhang, C., and Li, M. (2019). Biosynthesis, chemistry, and pharmacology of polyphenols from Chinese Salvia species: a review. Molecules 24 (1), 155. doi:10.3390/molecules24010155
Wang, Q. Q., Wang, M., Li, Y., Liu, Y. H., and Sun, L. Q. (2022). Attenuation of oxidative stress-induced cell apoptosis and pyroptosis in RSC96 cells by salvianolic acid B. Chin. J. Integr. Med. 28 (3), 243–248. doi:10.1007/s11655-021-3507-2
Wang, X., Ye, M., Wang, J., Wang, X., Wang, Y., and Zhu, X. (2013). Effects of salvianolate on reactive oxygen species and toll-like receptors in CD14∼+ monocytes after PCI in acute myocardial infarction. J. Cardiopulm. Vasc. Dis. 32 (03), 243–246. doi:10.3969/j.issn.1007-5062.2013.03.002
Wang, X., Zhang, X., Ye, M., and Mi, Y. (2017). Effects of salvianolate on the adhesion of CD14∼+ monocytes and toll-like receptors in patients with acute ST-segment elevation myocardial infarction after interventional therapy. J. Cardiopulm. Vasc. Dis. 36 (02), 82–85. doi:10.3969/j.issn.1007-5062.2017.02.002
Wang, Z. R. (2018). Effect of salvianolate on myocardial remodeling indexes in patients with acute ST-segment elevation myocardial infarction after coronary intervention. J. Community Med. 16 (09), 51–52.
Wei, J., Wu, J., Xu, W., Nie, H., Zhou, R., Wang, R., et al. (2018). Salvianolic acid B inhibits glycolysis in oral squamous cell carcinoma via targeting PI3K/AKT/HIF-1α signaling pathway. Cell Death Dis. 9 (6), 599. doi:10.1038/s41419-018-0623-9
Windecker, S., Bax, J. J., Myat, A., Stone, G. W., and Marber, M. S. (2013). Future treatment strategies in ST-segment elevation myocardial infarction. Lancet 382 (9892), 644–657. doi:10.1016/S0140-6736(13)61452-X
Wu, D., Zhu, J., Cai, W., Wang, G., and Teng, M. (2016). Effects of salvianolate on oxidative stress and vascular endothelial function in patients with acute myocardial infarction after PCI. J. Pract. Clin. Med. 20 (19), 16–19. doi:10.7619/jcmp.201619005
Wu, H. L., Li, Y. H., Lin, Y. H., Wang, R., Li, Y. B., and Tie, L. (2009). Salvianolic acid B protects human endothelial cells from oxidative stress damage: a possible protective role of glucose-regulated protein 78 induction. Cardiovasc Res. 81 (1), 148–158. doi:10.1093/cvr/cvn262
Xiao, Z., Liu, W., Mu, Y. P., Zhang, H., Wang, X. N., Zhao, C. Q., et al. (2020). Pharmacological effects of salvianolic acid B against oxidative damage. Front. Pharmacol. 11, 572373. doi:10.3389/fphar.2020.572373
Xu, J., Wei, K., Zhang, G., Lei, L., Yang, D., Wang, W., et al. (2018). Ethnopharmacology, phytochemistry, and pharmacology of Chinese Salvia species: a review. J. Ethnopharmacol. 225, 18–30. doi:10.1016/j.jep.2018.06.029
Xu, Q., Shen, Y., Zhao, J., and Shen, J. (2023). Salvianolate injection for hypertensive nephropathy patients who were using valsartan: a systematic review and meta-analysis. Front. Pharmacol. 14, 1119150. doi:10.3389/fphar.2023.1119150
Yang, L., Chen, X., Oi Lam Ung, C., Zhu, H., Hu, H., and Han, S. (2020). Clinical and economic evaluation of salvianolate injection for coronary heart disease: a retrospective study based on national health insurance data in China. Front. Pharmacol. 11, 887. doi:10.3389/fphar.2020.00887
Yang, M. C., You, F. L., Wang, Z., Liu, X. N., and Wang, Y. F. (2016). Salvianolic acid B improves the disruption of high glucose-mediated brain microvascular endothelial cells via the ROS/HIF-1α/VEGF and miR-200b/VEGF signaling pathways. Neurosci. Lett. 630, 233–240. doi:10.1016/j.neulet.2016.08.005
Ye, M., Hui, A., and Zeng, Y. (2014). Effect of salvianolic acid on vascular endothelium and platelet activation in patients with acute myocardial infarction after PCI. J. Anhui Med. 18 (11), 2178–2181. doi:10.3969/j.issn.1009-6469.2014.11.056
Yeh, R. W., Sidney, S., Chandra, M., Sorel, M., Selby, J. V., and Go, A. S. (2010). Population trends in the incidence and outcomes of acute myocardial infarction. N. Engl. J. Med. 362 (23), 2155–2165. doi:10.1056/NEJMoa0908610
Yi, Z., Shi, X., and Pan, R. (2017). Effect of salvianolate on blood flow in patients with acute ST-segment elevation myocardial infarction after percutaneous coronary intervention. Prev. Treat. cardio-cerebrovascular Dis. 17 (01), 32–34. doi:10.3969/j.issn.1009-816x.2017.11
Zhang, Y., Chen, N., Zhang, M., and Wei, X. (2022). Effects of salvianolate on inflammation and vascular endothelial function in elderly patients with acute myocardial infarction after percutaneous coronary intervention. Henan Med. Res. 31 (22), 4098–4101. doi:10.3969/j.issn.1004-437X.2022.22.015
Zhang, D., Wu, J., Liu, S., Zhang, X., and Zhang, B. (2016). Salvianolate injection in the treatment of unstable angina pectoris: a systematic review and meta-analysis. Med. Baltim. 95 (51), e5692. doi:10.1097/MD.0000000000005692
Zhang, X., Chen, Z., Huang, S., Zhang, Q., Jin, L., and Deng, M. (2017). Effect of salvianolate on myocardial microcirculation after PCI in diabetic patients with acute myocardial infarction. Zhejiang J. Integr. Chin. West. Med. 27 (01), 27–29.
Zhao, J. (2021). To investigate the effects of salvianolate combined with rosuvastatin on cardiac function and inflammatory indexes in elderly patients with acute myocardial infarction. Curr. Med. 27 (33), 181–182. doi:10.3969/j.issn.1009-4393.2021.33.078
Zhu, G., and Wang, D. (2018). Effects of salvianolate on cardiac function and hypersensitive C-reactive protein in patients with acute myocardial infarction. Mod. Hosp. 18 (05), 713–714. doi:10.3969/j.issn.1671-332X.2018.05.027
Zhu, G., and Wang, D. (2022). Effect of salvianolic acid injection combined with Rosuvastatin on the inflammatory pathway of NF-κB in elderly patients with acute myocardial infarction. Chin. J. Gerontology 41 (16), 3400–3404. doi:10.3969/j.issn.1005-9202.2021.16.003
Zou, T., Gao, S., Yu, Z., Zhang, F., Yao, L., Xu, M., et al. (2022). Salvianolic acid B inhibits RAW264.7 cell polarization towards the M1 phenotype by inhibiting NF-κB and Akt/mTOR pathway activation. Sci. Rep. 12 (1), 13857. doi:10.1038/s41598-022-18246-0
Keywords: salvianolic acids, salvianolic acid B, acute myocardial infarction, randomized controlled trials, systematic review
Citation: Chen P, Zhang H, Gao Z, Shi D and Zhang J (2024) Efficacy and safety of salvianolate injection in treating acute myocardial infarction: a meta-analysis and systematic literature review. Front. Pharmacol. 15:1478558. doi: 10.3389/fphar.2024.1478558
Received: 10 August 2024; Accepted: 27 November 2024;
Published: 17 December 2024.
Edited by:
Kuo Gao, Beijing University of Chinese Medicine, ChinaReviewed by:
Jiahong Wang, Shenyang Pharmaceutical University, ChinaCopyright © 2024 Chen, Zhang, Gao, Shi and Zhang. This is an open-access article distributed under the terms of the Creative Commons Attribution License (CC BY). The use, distribution or reproduction in other forums is permitted, provided the original author(s) and the copyright owner(s) are credited and that the original publication in this journal is cited, in accordance with accepted academic practice. No use, distribution or reproduction is permitted which does not comply with these terms.
*Correspondence: Dazhuo Shi, c2hpZGF6aHVvQDEyNi5jb20=; Jie Zhang, emhhbmdqaWVfYnlAMTYzLmNvbQ==
†These authors have contributed equally to this work
Disclaimer: All claims expressed in this article are solely those of the authors and do not necessarily represent those of their affiliated organizations, or those of the publisher, the editors and the reviewers. Any product that may be evaluated in this article or claim that may be made by its manufacturer is not guaranteed or endorsed by the publisher.
Research integrity at Frontiers
Learn more about the work of our research integrity team to safeguard the quality of each article we publish.