- 1The First Clinical College, Gannan Medical University, Ganzhou, Jiangxi, China
- 2Department of Urology, The First Affiliated hospital of Gannan Medical University, Ganzhou, China
- 3Institute of Urology, The First Affiliated Hospital of Ganna Medical University, Ganzhou, China
- 4Department of Jiangxi Engineering Technology Research Center of Calculi Prevention, Gannan Medical University, Ganzhou, Jiangxi, China
Prostate cancer is the most prevalent malignant tumor in men across developed countries. Traditional diagnostic and therapeutic methods for this tumor have become increasingly difficult to adapt to today’s medical philosophy, thus compromising early detection, diagnosis, and treatment. Prospecting for new diagnostic markers and therapeutic targets has become a hot topic in today’s research. Notably, exosomes, small vesicles characterized by a phospholipid bilayer structure released by cells that is capable of delivering different types of cargo that target specific cells to regulate biological properties, have been extensively studied. Exosomes composition, coupled with their interactions with cells make them multifaceted regulators in cancer development. Numerous studies have described the role of prostate cancer-derived exosomal proteins in diagnosis and treatment of prostate cancer. However, so far, there is no relevant literature to systematically summarize its role in tumors, which brings obstacles to the later research of related proteins. In this review, we summarize exosomal proteins derived from prostate cancer from different sources and summarize their roles in tumor development and drug resistance.
Introduction
Prostate cancer (PCa) is a highly prevalent and the second highest cause of cancer-related mortalities in men. Although PCa incidence is lower in Asia, relative to that in Europe and the United States, there is a continuous increasing trend (1). Currently, clinical treatment of PCa faces numerous challenges, due to its progression to Castration-resistant prostate cancer (CRPC)and a high rate of bone metastasis. Therefore, prospecting for new diagnostic and therapeutic targets is imperative to effective management of the malignancy. Previous studies have shown that novel diagnostic and therapeutic pathways, represented by exosomes, have potential for solving such problems. For example, miR-21 in PCa-derived exosomes (PCaDE) was found to inhibit apoptosis thereby promoting survival of cancer cells (2), whereas miR-423-5p was differentially expressed in PCa bone metastases a phenomenon that provided a basis for diagnosis of potential bone metastases (3). On the other hand, long non-coding RNA (lncRNA)was associated with vascular regeneration, tumor survival and metastasis, as well as tumor microenvironment (TME) establishment (4). Apart from RNA, prostate cancer-derived exosomal proteins (PCaDEPr), such as Exportin1(XPO1) which is present in all PCa cell lines exosomes, have been studied. Notably, this nuclear protein which is involved in nucleoplasmic exportation of the carry signal protein, not only plays a crucial role in the tumorigenic signaling pathway but also increases with the Gleason score (5, 6). This may be a new avenue for diagnosis and treatment of advanced PCa.
Exosomes are small membranous vesicles with a diameter of 30-150 nm that are formed by cells budding inward to form early endosomes that subsequently evolve into multi-vesicular bodies, which then fuse with the plasma membrane and are eventually released into the extracellular matrix. They participate in intercellular signaling by carrying various biomolecules such as proteins and nucleic acids, and regulate the pathophysiological processes of the organism (7). Exosomal proteins include endosomal proteins, plasma proteins and nuclear proteins. In PCa, these proteins have been shown to have a higher level of glycosylation than cellular ones (1). In addition, exosomes carry both membrane transport and fusion proteins, such as RabGTPases, Annexin, flotillins1(Flot), microvesicle-forming proteins Alix and Tsg101, as well as lipid-associated protein families including CD9, CD81, CD82and CD63 integrin proteins (8–11). Notably, the four transmembrane proteins play an important role in exosome-mediated regulation of cellular homeostasis components (Figure 1).
Although several studies have described the role of exosomal proteins in PCa, precise markers for PCa development have not been elucidated. Therefore, identification of the main types of PCaDEPr, coupled with elucidating the precise roles and underlying mechanisms of action for these proteins in cancer are imperative to guiding future developmental studies. Recent studies have demonstrated that exosomes proteins derived from PCa cell lines, plasma, tissues and urine are closely associated with tumor development (Figure 2). Therefore, understanding the roles played by these proteins, coupled with elucidating their underlying mechanisms of action in tumors will enable better targeting of these proteins for clinical treatment and improve the quality of survival of PCa patients (Figure 3).
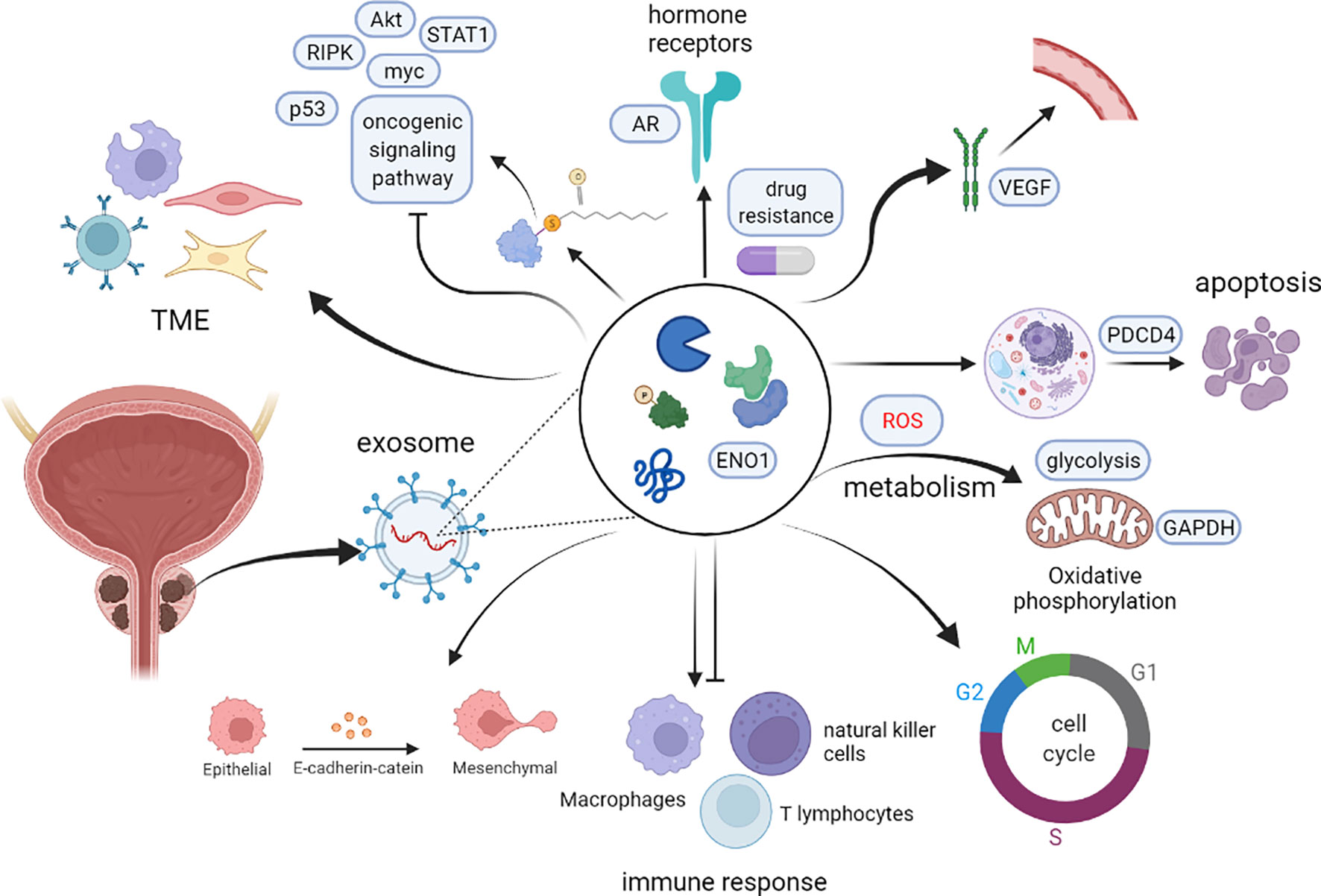
Figure 3 The potential role of PCaDEPrs in cancer. Exosomes originating from the tumor cell play a crucial role in tumor development. During tumor initiation, they mediate apoptosis, lipid metabolism, TME, and tumorigenic signaling, and also interfere with the cell cycle to induce cancer development. During tumor survival and progression, they regulate remodeling the tumor microenvironment, hormonal regulation and metabolic alterations, as well as lysosomal function and distribution, and inhibition of cancer cell apoptosis. During tumor metastasis, PCaDEPrs can contribute to EMT transformation, trigger microenvironment alteration, and establishment of a pre-metastatic ecological niche. Finally, they can also regulate tumor resistance to chemotherapeutic agents.
PCaDEPr From Different Sources
PCaDEPr in the Cell Line
Findings from several exosomal proteomics and subsequent functional validation in PCa cell lines have shown that exosomal proteins secreted by the cell lines play an important role in both tumorigenesis and development (Table 1). The exosomal proteins secreted by PCa cell lines are relatively high in tetraspanins such as CD9, CD82, CD61, heat shock protein (HSP) family HSP90, HSP70, and integrin proteins ITGA3, ITGB1, etc., and previous studies have confirmed these Proteins may play a role in the occurrence and development of tumors. Kurozumi et al. found that knocking down ITGA3 and ITGB1 significantly downregulated phosphorylation of FAK, SRC, AKT and ERK1/2 proteins, thereby markedly inhibiting migration and invasion of PCa cells (16). Similarly, Ramteke et al. extracted exosomes from LNCaP and PC3 cells exposed to hypoxic (1% O2) and normoxic (21% O2) media and found that CD63, CD81, HSP90, HSP70, Annexin II were expressed at higher levels in the hypoxic environment and that hypoxia enhanced the invasiveness and motility of LNCaP and PC3 cells as confirmed by cell invasion assays. Further research found that this may be related to the above-mentioned proteins promoting the formation of pre-metastatic niche in cancer cells and inducing stem cell proliferation and epithelial–mesenchymal transition (EMT) transformation (38). Furthermore, an exosomal protein study by Jinlu et al. found that the exosomal protein PKM2 secreted by C4-2B cells can be transported to bone marrow stromal cells (BMSC) via exosomes and upregulate CXCL12 production in BMSC in a HIF-1α-dependent manner to promote bone metastasis of PCa (45).
PCaDEPr in Plasma
Studies evaluating the clinical value of exosomal proteins in PCa have confirmed that plasma-derived exosomal proteins play a key role in tumor survival and metastasis, among others (Table 2). Notably, PCa patients exhibit significantly higher levels of proteins involved in substance metabolism (P-gp), bioactive enzymes (NEU3, C1r), and cell survival (Survivin, PIF1) in their plasma exosomes, relative to cell lines, and have reportedly been associated with a variety of tumor survival and metastasis. Bergelson et al. found that NEU3 is highly expressed in various cancers such as colon cancer and renal cancer, and significantly inhibits the apoptosis of cancer cells. Another study found that NEU3, as an enzyme that specifically hydrolyzes gangliosides, can reduce the ganglioside-mediated immune activation process (54). This result suggests that NEU3 may act as an immunosuppressant in tumors. Kishi et al. detected the expression of Survivin in the tissues of 82 PCa patients and found that its expression was positively correlated with the pathological stage, Gleason score (ranges from 1-5 and describes how much the cancer from a biopsy looks like healthy tissue (lower score) or abnormal tissue (higher score)) and cell proliferation activity of PCa, and could inhibit cell apoptosis (58). Additional research evidences have shown that plasma exosomal proteins may also have bidirectional effects on tumors. For example, P-gp in exosomes was reportedly elevated in doxorubicin-resistant PCa (18), while another study showed that it enhanced the anti-cancer ability of anti-cancer cytokines, such as CD4+ T cells, in ovarian cancer (84). Conversely P-gp was also found to activate expression of pro-tumor progressive M2 type macrophages (85). Collectively, these findings suggest that plasma exosomeal protein P-gp may be a potential therapeutic target for tumors.
PCaDEPr in Urine
Numerous studies have shown that urinary exosomal proteins from PCa patients play a non-negligible role in tumors (Table 3). The urine exosomes are more abundant in substance synthesis (Sepiapterin), signaling (Ras GTPase, Flot-2), tight junction (Claudin-3, δ-catenin) and other proteins compared to the plasma exosomes. Wu et al. compared SMMC-7721 containing epiapterin reductase (SPR) with SMMC-7721(human hepatocarcinoma cells) containing this mutant and concluded that SPR might be a tumor promoter in HCC (hepatocellular carcinoma). Results from further cellular experiments, as well as analysis of a nude mouse xenograft model, revealed that SPR depletion inhibited HCC cell proliferation and promoted apoptosis, affirming that SPR may regulate hepatocellular carcinoma progression via the FoxO3a/Bim pathway (a transcriptional target in apoptosis regulation in vivo and in vitro) (99). On the other hand, Hazarika et al. performed immunohistochemical staining of Flot-2 and found significantly higher intensities in metastatic melanoma from lymph nodes or visceral sites relative to those in nevi and primary melanoma and their results indicated that overexpression of Flot-2 promoted tumor cell proliferation and vascular regeneration (124). In addition, Flot-2 reportedly plays a role in promoting tumor metastasis, such as and has been shown to induce metastasis in nasopharyngeal carcinoma by activating the NF-κB and PI3K/Akt3 signaling pathways (125). This factor has also been shown to regulate the cell cycle and induce EMT, thereby promoting growth and metastasis of HCC (126). Lin et al. found that knocking down the expression of Claudin 3(CLDN3) resulted in significant changes in the phenotype of ovarian cancer cells, and further studies found that this would significantly downregulate the expression level of E-cadherin and upregulate the expression of N-cadherin. Therefore, CLDN3 may be involved in regulation of the EMT to promote metastasis in ovarian cancer (47). Exploration of the value of urinary exosomal proteins during early diagnosis of PCa is of great importance for subsequent clinical use, owing to the ease of obtaining urine samples. Results from differential protein analysis between healthy men and PCa patients revealed that 246 proteins were differentially expressed, 221 of which were significantly upregulated in exosomes of PCa patients (86). Taken together, these findings suggest that exosomal proteins may have potential as diagnostic and therapeutic markers in PCa.
PCaDEPr in Tissues
Apart from plasma and urine exosomal proteins from PCa patients, exosomal proteins from PCa tissues have also been extensively studied (Table 4). For example, results from mass spectrometry analysis revealed that PCa tissue exosomal protein types are mainly involved in vesicle transport and composition (Annexin A5, Annexin A3), biotransformation enzymes (such as Glutathione synthetase, and D-3-phosphoglycerate dehydrogenase), cytoskeletal molecules (Syntenin-1) and other related proteins. Moreover, previous studies have confirmed that these proteins play a role in tumor initiation and progression. Tang et al. demonstrated that Annexin A5 could activate the PI3K/Akt/mTOR signaling pathway to regulate the EMT process and matrix metalloproteinase (MMP) expression thereby significantly promoting proliferation, migration and invasion of renal cancer cells both in vitro and in vivo (145). Kennedy et al. reported that Glutathione plays a role as an intracellular antioxidant in cancer, a where it regulates reactive oxygen species (ROS)-mediated signaling pathways, including NF-kB and MAPK/ERK, to maintain tumor survival and induce tumorigenesis (128). ROS, which are closely related to Glutathione, were found to regulate Cav-1 expression in human lung cancer H460 cells, thereby modulating their migration and invasion. However, different ROS exert different effects in tumors. Superoxide anion and hydrogen peroxide were found to significantly downregulate Cav-1 expression and inhibit both cell migration and invasion, while hydroxyl radicals reportedly upregulated Cav-1 expression and also promoted cell migration and invasion (163). With regards to chemoresistance, Iwamoto et al. reported that Syntenin-1 was upregulated in rectal cancer (CRC) tumor tissues, while its downregulation mediated a significant downregulation of prostaglandin E2 receptor (PTGER2). On the other hand, silencing PTGER2 decreased chemoresistance of cancer stem cells to oxaliplatin. Taken together, these results indicated that Syntenin-1 may promote chemoresistance in cancer cells (147).
PCaDEPr in Tumor Cell Initiation and Proliferation
The basic understanding of tumorigenesis is uncontrolled cell proliferation or uncontrolled apoptosis. In addition to changes in tumor cells, changes also occur in the tumor microenvironment, including variations in structure and function of stromal cells such as fibroblasts, lymphocytes, epithelial cells, and matrix molecules, like growth factors and cytokines. Previous studies have shown that PCaDEPr can induce tumor cell initiation and proliferation processes by regulating metabolic, apoptotic, and TME pathways.
Inhibition of Apoptosis Induces Cell Tumorigenesis
Apoptosis is an orderly and coordinated process of cell death that occurs under physiological and pathological conditions. In cancer, dying cells do not receive apoptotic signals, due to an imbalance between cell division and cell death, a phenomenon that causes normal cells to tumorize. Apoptosis can induce development of cancer cells through intrinsic and extrinsic pathways, which involve action of many proteins that regulate apoptosis and these proteins are also present in prostate cancer-derived exosomes. For example, Sepiapterin reductase (SPR), an important regulator of tetrahydrobiopterin (BH4) biosynthesis, has been shown to be a promoter of various tumors. Zhang et al. found that ROS-mediated apoptosis could be induced by knocking down SPR expression to inhibit progression of breast cancer cells (100). Similarly, Basu et al. found a strong association between S100 Calcium Binding Protein P (S100P) expression and prostate tumorigenesis, with S100P expression mediating basal apoptosis and impeding camptothecin-induced apoptosis. Moreover, silencing of S100P significantly inhibited growth of 22Rv1 cells, while overexpressing S100P in PC3 cells resulted in increased proliferation of tumor cells (164). In addition, other exosomal proteins interact with apoptosis-related proteins to induce tumorigenesis. Ingo et al. found that ornithine decarboxylase (ODC) and Sepiapterin reductase (SPR) proteins interacted to elevate ODC activity, thereby inhibiting apoptosis and inducing neuroblastoma cell genesis (165). Exosomal protein phosphatase and tensin homolog (PTEN) was shown to negatively regulate expression of the cyclin-dependent kinase (CDK) inhibitor p27 (KIP1), thereby inhibiting apoptosis (166). Previous studies have shown that S100 calcium-binding protein A6 (S100A6) interacts with p53 to affect oligomerization and activity of p53, thereby reducing its ability to promote apoptosis (167, 168). Moreover, tumor cells can also induce cancer by secreting exosomes to eliminate proteins that initiate apoptosis. Diederick et al. showed that cancer cells can remove PDCD6IP, a protein involved in programmed cell death, by exosome secretion to inhibit apoptosis, explained by high PDCD6IP abundance in PCa-derived exosomes and low abundance in autologous tumor cells this possibility (6). Collectively, these studies indicate that exosomal proteins can induce tumorigenesis and proliferation by inhibiting the apoptotic process both directly and indirectly.
Involvement in Substance Metabolism and Oxidative Stress
Alteration of pathways regulating cellular substance metabolism is more common in cancer, compared to normal tissue cells. In fact, alterations in normal cellular substance metabolism have been implicated in convergence of cells to a tumor state. Previous studies have shown that alterations in tumor metabolism include glycolysis, lipid hydrolysis, increased nutrient utilization, and increased production of biosynthetic intermediates required for cell growth and proliferation. Liu et al. found that Fatty acid synthase (FASN) protein was upregulated in exosomes derived from Vertebral-Cancer of the Prostate (VCaP) cells. Additional studies have demonstrated that FASN catalyzes formation of long-chain fatty acids from acetyl coenzyme A, malonyl coenzyme A and NADPH, to promote proliferation of VCaP cells, and that inhibition of FASN effectively and selectively kills cancer cells (166). Qin et al. demonstrated that ADP-ribosylation factor-like 8b (Arl8b) Arl8b depletion reduced the ability of PCa cells to establish subcutaneous xenografts in mice. Under a low nutrient environment, Arl8b maintained efficient metabolism in PCa cells thereby allowing them maintain their excessive proliferative capacity by promoting lipid hydrolysis. Metabolic defects in the proliferation of cells with low Arl8b expression inhibit tumor growth initiation in vivo. The phenomenon may be attributed to the fact that Arl8b depletion impairs intracellular neutral lipid hydrolysis, thereby shifting the metabolic profile to an abnormal lipogenic phenotype, which subsequently impairs glucose utilization and limits the propensity for cytokinesis (169). In addition, Webber et al. demonstrated that the exosomal protein TGFβ1 secreted by cancer-associated fibroblasts enhanced proliferation of PCa cells under both hypoxic and low nutrient environments by inhibiting mitochondrial oxidative phosphorylation and elevating anaerobic glycolysis (170).
Oxidative stress, a series of adaptive responses caused by an imbalance between ROS and the body’s antioxidant system, plays a key role in cancer development and progression. Previous studies have shown that by interfering with the normal redox state of cells, oxidative stress causes generation of peroxides and free radicals that subsequently damage cellular proteins, lipids and DNA, thereby causing tumor development. For example, ROS can either initiate or stimulate tumorigenesis and support the transformation and proliferation of cancer cells. Over-proliferation of tumor cells is often accompanied by high ROS production, and thrives under such oxidative load conditions. At the same time, tumor cells can optimize the ROS-driven cell proliferation process by increasing their antioxidant capacity, to avoid the ROS threshold that triggers senescence, apoptosis and iron-induced cell death (171). Previous studies have shown that some proteins in exosomes can influence ROS expression during oxidative stress in cells, thereby affecting the tumor initiation process. For example, Qin et al. reported that ectopic expression of six transmembrane epithelial antigen of prostate 4 (STEAP4) in PCa cells significantly increased tumor cell proliferation and colony formation, suggesting that STEAP4 may be playing a role in tumor growth. This phenomenon may be explained by the fact that high STEAP4 expression not only downregulates IRS-1, PI3K and AKT phosphorylation but also impairs insulin-mediated GLUT4 translocation, thereby resulting in ROS-associated mitochondrial dysfunction (169). In another study, silencing of STEAP4 significantly inhibited growth of mouse PCa xenografts in a mouse model. Furthermore, STEAP4 expression was found to mediate elevation of ROS levels probably by increasing levels of ferrous iron in cells after using it as a redox intermediate (electron donor) to generate free radicals. In addition, STEAP4 expression reportedly depleted production of NADPH (172), an inhibitor of ROS production, thereby resulting elevated ROS production. Notably, persistently high ROS levels were found to promote cancer development, owing to is oncogenic nature (113).
Activation of TME Regulatory and Tumorigenic Signaling Pathways
The tumor microenvironment refers to the surrounding microenvironment where tumor cells exist, including surrounding blood vessels, immune cells, fibroblasts, bone marrow-derived inflammatory cells, adipose stem cells and various signaling molecules and the extracellular matrix (ECM). During early stages of cancer development, tumor cells appropriately regulate the microenvironment. For example, various microenvironmental changes, such as adjustment of the ECM, immune response, stromal stem cell transformation and induction of angiogenesis, can be triggered during tumor initiation (173). Recent studies have shown that PCaDEPr may promote production of tumor cells through this pathway. Zakaria et al. found that the PCa cell microenvironment disrupts adipose-derived stem cells in PCa patients to induce tumor transformation, but unlike normal stem cells, the use of PCa cell-conditioned medium effectively triggers conversion of adipose-derived stem cells into prostate-like tumor lesions in vivo. Furthermore, exosomal proteins, namely Rab1a, Rab1b, and Rab11a, in PCa were found to recapitulate the formation of prostate tumorigenic mimics generated by adipose-derived stem cells triggered by PCa cell conditioned medium. In fact, the use of PCa cell-derived conditioned medium (CM) or exosomes was found to effectively trigger adipose stem cells to undergo genetic instability, mesenchymal-to-epithelial transformation (MET), and oncogenic transformation, thereby inducing PCa in vivo. This may be explained by the fact that exosomes deliver oncogenic factors, such as Rab proteins (Rab1a, Rab1b, and Rab11a) translocated to pASCs to inhibit large tumor suppressor kinase 2 (LATS2) and programmed downregulation of cell death protein 4 (PDCD4), thereby promoting tumor growth (40). In the tumor vascular microenvironment, Dominique et al. showed that HSP27 interacted with CD283, thereby inducing NF-κB activation, which subsequently led to vascular endothelial growth factor (VEGF)-mediated angiogenesis in the tumor microenvironment (174).
In addition, PCaDEPrs have also been shown to induce tumorigenesis by modulating alterations in tumorigenic signaling pathways. For example, flotillins that are also present in tumor-derived exosomes. Jang et al. showed that palmitoylation of Flot-1 could regulate proliferation of PCa cells by activating the IGF-1R signaling pathway. Moreover, palmitoylation (S-palmitoylation) modification of Flot-1 was found to regulate intracellular signaling proteins p53, STAT1 (signal transducer and activator of transcription 1) and IκBα (nuclear factor of kappa light polypeptide gene enhancer in B-cells inhibitor, alpha), thereby inducing oncogenic effects (175). Furthermore, Takahashi-Niki et al. found that Parkinson disease protein 7 (DJ-1) binds to Topors/p53BP3 (176), both in vitro and in vivo, thereby releasing the monoglycosylated form of p53 and helping to restore the transcriptional activity of p53. Recent research evidence showed that DJ-1 directly binds to Sirtuin1 (Sirt1), to stimulate Sirt1 deacetylase activity. Furthermore, DJ-1 downregulated the transcriptional activity of sirt1-suppressed sirt1 target p53 (177). Taken together, these results indicated that p53 is closely associated with DJ-1, suggesting presence of a finely regulated circuit between both proteins during tumorigenesis and apoptosis. Previous studies have also shown that major facilitator superfamily domain containing 12 (MFSD12), which is highly expressed in melanoma, induces proliferation of melanoma cells via the PI3K- AKT signaling pathway (102).
Interference With the Cell Cycle
Continued unregulated growth of cancer cells is a fundamental abnormality during cancer development and progression. In fact, the first step in the process, tumor initiation, is believed to be the basis for initiation of abnormal proliferation of individual cells. Subsequent cell proliferation causes growth of clonally derived tumor cell populations. Numerous studies have identified a number of proteins that regulate proliferation of the cell cycle, to subsequently trigger tumorigenesis. For example, one study showed that estrogen stimulates the proliferative cycle of endometrial cells, while exposure to excess estrogen significantly increases the risk of endometrial cancer in women (178). PCaDE also contains similar proteins that interfere with cell cycle processes, to promote tumor cell development. For instance, Bosch et al. demonstrated that Ca2+ and calmodulin (CaM) play a key role in proliferation and viability of a variety of cells, including PCa. This phenomenon may be attributed to the fact that CaM interacts with various proteins that regulate the cell cycle, including p21Cip1, D1-Cdk4 and CaM kinase II, to control their activities and nuclear localization, thereby influencing proliferation of tumor cells (179). Moreover, cell cycle protein A in LNCaP cellular extracts was found to directly or indirectly bind to CaM, indicating that its expression is sensitive to the inhibitory effect of the anti-CaM drug W-7. Notably, this indicates that CaM regulates expression of cell cycle protein A in PCa cells to induce over-proliferation of LNCaP cells (96). In addition, previous studies have shown that expression of MFSD12, a novel suppressor gene in lung cancer, and its protein, can control cell cycle distribution, matrix attachment and cell motility, thereby regulating tumor growth and development. MFSD12 was significantly upregulated in melanoma tissues, with interreference in its expression in A2058 and M14 melanoma cells found to significantly suppress tumor cell proliferation. Results from flow cytometry analysis confirmed that silencing MFSD12 expression mediated increase and decrease in the proportion of cells in the G1and S phases, respectively, suggesting that MFSD12-induced proliferation is associated with promotion of the G1 phase (102).
PCaDEPr in Cancer Survival and progression
Evasion of death is imperative to cancer cells’ persistence and their subsequent progression. Several tumor survival proteins are present in the tumor survival microenvironment, where they play a key role in regulatory processes including apoptosis (180), metabolism (181), immune escape, nutrient transport, hypoxic environment and drug resistance, that promote tumor cell survival. Proteins related to apoptosis and also present in PCa exosomes, such as Bcl-2, inhibitor of apoptosis (IAP) and heat shock protein (HSP) and proteins related to cell metabolism, such as glucose transporter 1 (GLUT1) and Ras, etc. Considered a family of survivin proteins of tumor cells (180). Abnormal expression of these proteins is associated with a series of biological regulatory processes that promote cancer cell survival, proliferation, and treatment resistance (181). In addition, cancer cells employ progression as a means for tumors to maintain survival, thus tumor survival is closely associated with progression. Tumor progression is characterized by rapid changes in the tumor phenotype, a phenomenon that has made tumors to become more aggressive. Exosomal proteins are thought to play various roles in progression of various tumor types, including remodeling of the tumor microenvironment, promoting epithelial mesenchymal transition (EMT), angiogenesis induction, promoting migration, invasion and immune escape of cancer cells, as well as regulating the corresponding signaling pathways (182).
Remodeling the Tumor Microenvironment
Immune escape is an important aspect in tumor survival, as tumor cells can only proliferate, migrate and invade tissues if they escape killing by immune cells, such as phagocytes, T cells, and NK cells. The exosomal proteins, which are secreted by cancer cells support immune escape to promote tumor cell survival. For instance, exosomal proteins support immune cell migration (such as neutrophils, macrophages and regulatory T cells) to secondary sites, suppress immune responses to tumors by inhibiting the efficacies of antigen-presenting cells, such as dendritic cells. They can also impair immune functions of T and NK cells by activating apoptosis (183, 184). Moriwaki et al. demonstrates that tumor cells lacking GDP-mannose-4,6-dehydratase (GMDS) can evade NK cell-mediated tumor immune surveillance by acquiring resistance to tumor necrosis factor-related apoptosis-inducing ligand (TRAIL)-induced apoptosis (105). Aled et al. found that TGF-β-positive exosomes downregulated natural-killer group 2, member D(NKG2D) expressions in NK and CD8+ T cells, which in turn impaired immune effector functions (185). TGF-β-rich exosome inhibits lymphocyte responses to IL-2, thereby altering the tumor microenvironment to promote immune escape functions of tumor cells (186). In addition, documented those expressions of some purinergic receptors directly or indirectly inhibit T cells and NK cells effects, thereby suppressing immune responses to primary tumors. For instance, oncogenic exosomes with elevated CD39 and CD73 levels can promote adenosine production, thereby enhancing regulatory T cell and myeloid cell proliferation to suppress immune functions (187). Interestingly, exsomeal proteins have also been shown to promote tumor progression by activating tumor-associated immune cells. Wang et al. found that LAMP 2a contributes to tumor progression by degrading PRDX1 (peroxiredoxin 1) and CRTC1 (CREB-regulated transcriptional coactivator 1), which enhances tumor-associated macrophage activation (188). These studies confirm that cancer-derived exosomal proteins can mediate the escape of tumor cells from immune surveillance to promote their survivability.
In addition to regulation of immune microenvironments, exosomal proteins are involved in construction of other tumor microenvironments to maintain tumor survival. For example, tumor cell over proliferation leads to the development of hypoxic environments, therefore, the ability to regulate tumor cell tolerance to hypoxic environments is necessary for tumor cell survival, and some proteins in exosome can play this function. Hypoxia-inducible factor-1α (HIF-1α), a master transcription factor, is stable under hypoxic conditions. It regulates the expressions of several target genes and enhances the adaptability of tumor cells to hypoxia (189). Zhong et al. found that under hypoxic conditions, DJ-1 is involved in regulation of HIF-1α transcriptional activities, promoting PCa adaptation to hypoxic environments (190). Inflammatory microenvironments can also affect tumor survival and progression. For instance, DJ-1 is involved in creation of inflammatory tumor microenvironments. In their study, Chien et al. found elevated levels of IL-1β in cultured macrophages from DJ-1 DJ-1 Knockdown mice and DJ-1 knockdown mice (191). It was also confirmed that the inflammatory microenvironment generated by DJ-1 dysregulation sustained melanoma survival at the point of lung metastasis. Another study found that adipose-derived stem cells (ADSCs) induced by exosomal proteins exhibited typical characteristics of tumor-associated myofibroblasts, and could induce the phenotype and function of myofibroblasts in ADSCs by activating intracellular signaling pathways. Increased expression of smooth muscle actin (α-SMA) and tumor-promoting factors such as the stromal cell-derived factor 1 (SDF-1) and TGF-β. These outcomes are associated with increased expressions of TGF-β receptors I and II in exosomes (189). Therefore, exosomal proteins contribute to the generation of tumor-associated myofibroblasts in the tumor stroma to construct an extracellular matrix environment suitable for tumor survival.
Hormone Receptor Regulation and Metabolic Reprogramming
Although the emergence of castration-resistant PCa poses difficulties for androgen deprivation therapy (ADT), androgen depletion and hormonal regulation have been the mainstay of advanced disease treatment since the landmark discovery of Huggins and Hodges (192). The PCaDEPr that are associated with hormone receptor regulation, including adhesion spot protein (VCL), play an important role in cancer progression. Kawakami et al. reported that the VCL, through which integrins associate with the actin cytoskeleton, promotes paclitaxel resistance-associated PCa invasion. They found that VCL levels were highest in CRPC, negative or very low in BPH and non-CRPC, and confirmed that VCL overexpressions promotes PCa progression by altering androgen receptor (AR) levels (21). Iwamoto et al. found that Syntenin-1 levels are positively correlated with prostaglandin E2 receptor (PTGER2) levels and promotes rectal cancer cell progression (147). In addition to regulation of hormone receptors, these proteins mediate hormone levels, thereby activating hormone receptors. Abiraterone acetate (CYP17A1), an integrase involved in adrenal steroid conversion and de novo synthesis of androgens, is involved in CRPC production. Attard et al. used an inhibitor of CYP17A1 synthesis to treat 21 desmoresistant PCa patients. They reported a decrease in serum androstenedione, dehydroepiandrosterone (DHEA) and testosterone levels in vivo, and in CRPC patients. The antifungal drug, ketoconazole, is similar to CYP17A1 inhibitors and suppresses testosterone synthesis (193). Therefore, CYP17A1 promotes androgen expressions, which have a significant role in AR activation. Therefore, PCaDEPr mediated regulation of hormone receptors may be one of the pathways in cancer progression.
Metabolic processes are crucial for cell survival, especially tumor cells. The exosomal proteins have the ability to regulate substance metabolism-related proteins to induce metabolic reprogramming and provide energy as well as biosynthetic pathways to tumor cells. For example, glucose transporter protein 1 (GLUT1) regulates cellular glucose uptake and responds to suppressed intracytoplasmic glucose levels. Cheng et al. found that Rab25(member RAS oncogene family) regulates GLUT1 transport to cell surfaces to enhance glucose uptake and ultimately increases glycogen reserves as well as ATP levels in ovarian cancer cells (194). Even though dysregulated glucose metabolism is important for metabolic reprogramming in tumor cells, metabolic reprogramming in tumor cells also involves lipid storage and mobilization. Walther et al. found that Rab GTPases regulates GLUT (glucose transporter protein) transport and lipid droplet (LD) formation during glucose and lipid metabolism in cancer cells. Lipid droplets (LD) have a role in intracellular lipid storage and maintenance of intracellular levels of free lipids and energy homeostasis (195). Wu et al. reported that Rab8a regulates lipid droplet fusion and cancer cell growth in hepatocellular carcinoma (196), thereby maintaining hepatocellular carcinoma cell survival.
Regulation of Lysosomal Functions and Distribution
Lysosomes are important components of the endosomal system. They are involved in various biological processes, including macromolecular degradation, antigen presentation, intracellular pathogen destruction, plasma membrane repair, exosomesrelease, cell adhesion/migration, and apoptosis. Functional states and spatial distributions of lysosomes are closely associated with cancer cell proliferation, energy metabolism, invasion and metastasis, as well as immune escape. Invasiveness of radiation-surviving cancer cells is associated with altered lysosomal exocytosis induced by activation of Arl8b present in prostate cancer-derived exosomes. Ping-Hsiu Wu et al. found that after radiation, Arl8b, a small GTPase that regulates lysosomal transport, increased its binding to its effector-SifA and kinesin-interacting protein (SKIP) through the regulation of the BORC (Biogenesis of lysosome-related organelles complex) subunit. Knockdown of Arl8b or the BORC subunit suppressed lysosomal cytokinesis and invasiveness of radiation-surviving breast cancer tumor cells. In vivo, suppression of Arl8b levels inhibited radiation-induced invasive tumor growth and distant metastasis (109). Moreover, Arl8b is also a key regulator of lysosomal localization (197). The active form of Arl8b is mainly located in the lysosome, where it regulates lysosomal transport to the cell periphery (198). The cis-transport of lysosomes from the center of microtubule tissues to the cell periphery is regulated by the BORC/Arl8b/SKIP complex (199). Therefore, Arl8b regulates spatial distribution of lysosomes and protease release through lysosomal localization, leading to elevated tumor cell invasiveness. In addition, as a key protein in lysosomal functions, cathepsin D is widely found in PCa-derived exosomes and is associated with tumor progression. Yong et al. found that cathepsin D levels are positively correlated with colorectal cancer malignancy, and that patients with elevated cathepsin D levels have lower survival rates (200).
Inhibition of Cancer Cell Apoptosis
Homeostatic balance in an organism is maintained by programmed cell death or apoptosis. In addition to being associated with tumor survival, apoptosis is also closely associated late survival of tumor cells. In cancer patients, tumor cells also undergo their own apoptosis, leading to the death of cancer cells. However, some biomolecules such as proteins present in prostate cancer-derived exosomes inhibit this process to keep tumor cells alive. Hahm et al. reported that induction of lysosomal-associated membrane protein 2A (LAMP2A) expression inhibited the apoptotic abilities of prostate cells, thereby enhancing cancer cell survival. LAMP2A protein knockdown in PC-3 and 22Rv1 cells significantly increased the apoptotic rate in both cells, confirming that LAMP2A is involved in induction and activation of the apoptotic protein (Bcl-2) (201). Ding et al. documented that LAMP2A downregulation significantly increased positive apoptosis-staining of hepatocellular carcinoma cells, while decreasing Ki-67(a staining for lipid membranes) staining, confirming that LAMP2A contributes to cancer persistence by inhibiting apoptosis and promoting cell proliferation (202). Moreover, in primary breast cancer samples, DJ-1 levels were negatively correlated with PTEN immunoreactivity and positively correlated with PKB (Protein kinase B)/Akt hyperphosphorylation. Co-expressions of DJ-1 and PTEN completely rescued the apoptotic processes of PTEN-induced tumor cells (112).
Apoptosis affects tumorigenesis and has an important role in late tumor progression. Therefore, interference with apoptosis can promote cancer progression. In vitro and in vivo, elevated caveolin-1 expressions in metastatic mice and human PCa cells have been reported, suggesting that inhibition of apoptosis promotes tumor progression. Overexpressions of caveolin-1 in LNCaP) or upregulation of Cav-1 in androgen-insensitive LNCaP clones makes these cells resistant to apoptosis (203). Li et al. found a significant association between elevated glucosamine-6-Phosphate Deaminase 1 (GNPDA1) levels and advanced tumor stage, TNM (the TNM classification of malignant tumors) stage or grade, and the subsequent apoptotic staining analysis revealed that elevated GNPDA1 levels inhibited HCC cell apoptosis (104). Therefore, GNPDA1 promotes hepatocellular carcinoma progression by inhibiting HCC cell apoptosis.
PCaDEPr in Cancer Transfer
Approximately 90% of human cancer-associated deaths are attributed to metastases (204). One of the hallmarks of malignancy is a high degree of invasiveness and metastatic capacity. During development of most cancer types, sooner or later, the primary tumor mass produces free cells that invade adjacent tissues and migrate to distant sites, where they establish new tumor cell colonies. Presumably, the processes involved in invasion and metastasis are: separation from the primary tumor mass, reorganization/remodeling of the extracellular matrix, cell migration, recognition, movement through endothelial cells and vascular circulation, as well as colonization and proliferation within the ectopic stroma. The key and initial to all these processes is an increased ability of cancer cells to move themselves and escape the control of normal physiological regulation. Various biomolecules, including proteins, are involved in regulation of tumor cell invasion and metastasis. This could be because, proteins can influence the tumor microenvironment, EMT, target microenvironment, vascular regeneration, and metastatic signaling pathways to induce distant tumor cell metastasis.
Establishment of pre-Metastatic Ecological Niches
Tumor cells require a permissive environment in terms of nutrients, extracellular matrix and immune cells to successfully metastasize to distant organs. Therefore, tumor-adapted metastable environments are particularly important for tumor metastasis, and the process of constructing these microenvironments involve the establishment of pre-metastatic ecological niches. Studies on metabolic networks and seeding mechanisms of cancer cells in specific environments have revealed that some integrin proteins are involved in establishment of these ecological niches. During metastasis, tumor cells must acquire the ability to remodel the extracellular matrix (ECM) to achieve invasion and metastasis. Some exosomal proteins are involved in regulation of this process. Bijnsdorp et al. found that Integrin Subunit Alpha 3 (ITGA3) and Integrin beta-1 (ITGB1) were highly expressed in urinary esosomes of metastatic PCa patients, and that ITGA3 and ITGB1, as well as ITGA3 in exosomes, stimulated non-cancerous epithelial cell migration and invasion. This enables the progression and distant metastasis of cancer cells (205). Moreover, the immunosuppressive microenvironment is important in development of pre-metastatic niches, and some exosomal proteins in PCa are involved in establishment of immunosuppressive microenvironments. Allard et al. found that synergistic actions of two extracellular nucleotidases (CD39 and CD73), constituted the main source of extracellular adenosine in TME and were jointly involved in development of immunosuppressive TME, such as through tumor kinetics to redirect ATP to the immunosuppressive adenosine-rich tumor microenvironment (206).
Vascular regeneration of tumor cells enhances the migratory as well as metastatic capacities of tumor cells, in addition to providing them with a favorable nutritional environment (207). Some exosomal proteins promote distant tumor cell metastasis through this process. Gesierich et al. reported that quadruple transmembrane protein-8 (Tspan8)-positive exosomes promoted endothelial cell production and increased the expressions of vascular endothelial growth factors as well as growth factor receptors in fibroblasts, thereby promoting angiogenesis in pancreatic and gastric cancers (208). Chen et al. found that in patients with metastatic colon cancer, high serum Galectin-3 levels were associated with elevated serum G-CSF, IL-6 and sICAM1 levels, which interacts with the vascular endothelium to increase the expressions of vascular cell adhesion protein type I (VCAM-1) on endothelial cell surfaces, leading to increased cancer cell-endothelial adhesion and increased endothelial cell migration and small vessel formation (209). In addition, intramembrane cleavage mediated by γ-secretase, a large protease complex consisting of a catalytic subunit (presenilin-1 or presenilin-2) and auxiliary subunits (Pen-2, Aph1 and nicastrin), is an important link in the Notch signaling pathway. Zeng et al. documented that γ-secretase affects cancer metastasis after Notch activation cascade reactions, probably because γ-secretase promotes angiogenesis in solid tumors through Notch signaling (210).
In conclusion, the establishment of metastatic ecotone, including immunosuppression and angiogenesis suggests that PCaDEPr is involved in mediating the establishment of pre-metastatic ecotone in tumors, thereby inducing cancer metastasis.
Alterations of Microenvironments at the Target Site
Adaptive regulation of the microenvironment at tumor colonization sites prior to metastasis is important for tumor colonization. Recently, exosomal proteins have been shown to promote tumor cell colonization of tissues and organs by modulating the tumor metastasis target site microenvironments. In addition to alterations of tumor microenvironments at the target site, there are changes in bone colonization processes, such as the number and structures of outcomes and osteoclasts. Prior to the arrival of tumor cells, primary tumors actively regulate the nutritional, extracellular matrix and immune environments of distant organs by secreting regulatory factors, thus producing a permissive and supportive ecological niche for tumor survival at the metastatic site. In tumor bone cell metastasis, malignant communication between PCa cells and bone cells (osteoblasts and osteoclasts) is established. Casimiro et al. found that PCa cells provide osteoblasts with osteogenic cytokines [e.g. bone morphogenetic proteins (BMPs), platelet-derived growth factors (PDGF), endothelin-1 (ET1)] and osteolytic factors [e.g. MMPs and vascular endothelial growth factor (VEGF)], which enables these cells to make bone-derived cell growth factors (211). Itoh et al. identified the ETS Proto-Oncogene 1 (Ets1) protein in PCa-derived exosomes to be an osteoblast differentiation-related transcription factor and found it to be a candidate inducer of osteoblast differentiation (19). A standard exosomal protein study found that exosome-mediated translocation of pyruvate kinase M2 (PKM 2) from PCa cells into BMSCs promotes PCa bone metastasis. Moreover, the PKM2 protein upregulates hypoxia-inducible factor 1α (HIF-1α) in BMSCs to promote CXCL12 expressions in stromal cells. Biologically, exosome-mediated PKM2 transport of prostate tumor origin is a key mediator of PCa bone metastasis (45).
EMT Transformation and Regulation of Cell Motility
Prior to metastasis, tumor cells are detached from their original sites through loss of attachment and adhesion capacities and metastasize to their target sites with blood or lymphatic chemotaxis, eventually undergoing clonal growth at metastatic sites. Therefore, epithelial-mesenchymal transition processes of EMT formation are important in initiation of cancer metastasis. Various molecules, including exosomal proteins, are involved in EMT transformation in tumor cells. The loss of E-cadherin is associated with the loss of intercellular contacts, disruption of the E-cadherin-catenin complex, abnormal activation of β-catenin signaling as well as cytoskeletal changes. This is critical for cells to lose their epithelial polarity and acquire aggressive phenotypes. In primary PCa, suppressed E-cadherin levels and elevated nucleus β-catenin levels are strongly associated with metastasis and poor prognostic outcomes. Zhang et al. observed elevated E-cadherin levels and suppressed N-cadherin as well as wave protein levels in response to melanopsin depletion. Silencing of melanopsin was associated with suppressed total and activated β-catenin levels. In a subsequent study, it was noted that when melanopsin was downregulated, PCa cells exhibited decreased proliferation, migration and invasion abilities (101). In addition to melanopsin, Rab3D induces epithelial mesenchymal transformation. Tauro et al. found that Rab3D regulates EMT transformation of tumor cells by activating the Akt/GSK-3β/Snail signaling pathways (212). In addition, overexpressing cells with melanopsin-like Rab2A suppresses E-calmodulin while elevating N-calmodulin, wave protein, and fibronectin levels, which affects the EMT phenotype (118).
Cell motility is key in cancer invasion and metastasis. The loss of cell-cell adhesion and enhanced cell-matrix interactions are essential for enhanced tumor cell motility (213). Four-transmembrane proteins are associated with various processes, including signal transduction pathways, cell activation, proliferation, motility, adhesion, tissue differentiation, angiogenesis, tumor progression, and metastasis (214, 215)and are present in urinary exosomes of PCa patients. Even though most tetra-transmembrane proteins are downregulated in metastatic tumors, the CD151 glycoprotein was the first member of the tetra-transmembrane protein to be identified as a metastasis promoter. This shows that the tetra-transmembrane superfamily protein CD151 promotes cancer migration and metastasis (216). Detchokul et al. revealed that CD151 can regulate the redistribution of adhesion components required for cell migration as well as invasion and the process of targeted delivery of matrix degrading enzymes, confirming that CD151 promotes cell motility and tumor invasion (217). Gesierich et al. found that colocalization of integrin β4 with CD151 activates PKC to promote integrin internalization, thereby increasing tumor cell motility (218). Ang et al. found that CD151-transfected LNCaP cells had greater motility, compared to controls and that PC3 cells with CD151 knockdown showed reduced motility. However, the responsible mechanisms have not been elucidated (219). In conclusion, CD151 induces distant cancer cell metastasis by regulating tumor cell motility.
Activation of Metastatic Signaling Pathways
Tumor-associated exosomal proteins have the ability to mediate the activation of common signaling pathways to induce tumor cell metastasis. Hao et al. found that CD44 or CD147 knockdown downregulated p-Akt and p-Erk levels in PC3 cells and inhibited the activations of PI3K/Akt and MAPK/Erk signaling pathways. The administration of drugs that selectively target CD44/CD147 alone or in combination with docetaxel restricted CaP metastasis (50). Therefore, CD44 and CD147 enhances the metastatic abilities of CaP cells, possibly by activating PI3K and MAPK pathways. He et al. found that DJ-1 knockdown markedly suppressed invasive and migration abilities of pancreatic cancer cells, inhibited the expressions and activities of uPA and induced cytoskeletal disruption. These outcomes may have been because DJ-1 downregulation inhibited SRC and ERK1/2 phosphorylation, which suppressed SRC and ERK signaling pathways-mediated expressions of uPA (220). Yang et al. reported that the exosomal protein (Rab3D) was highly expressed in malignant breast cancer but not in normal tissues and benign breast tumors. The knockdown of Rab3D significantly inhibited the migration abilities of breast cancer cells, which was confirmed to be mediated by Rab3D activations of AKT/GSK-3β/Snail signaling pathways (116). In addition, exosoemal proteins are involved in intermediate pathways of metastatic signaling pathways to induce cancer metastasis. Boscher et al. found that EGF activations of downstream integrin signaling pathways in breast cancer adenocarcinoma epithelial cells induces tumor metastasis dependent on synergistic actions of Galectin 3 and p-Caveolin-1 (221). Thus, PCaDEPr activates multiple tumor metastasis signaling pathways to induce cancer metastasis.
PCaDEPr in Cancer Drug Resistance
Tumor cell sensitivity to chemotherapeutic agents is essential for cancer drug therapy. Many biological factors modulate the sensitivity as well as resistance of tumors to chemotherapeutic agents (222, 223). In patients with prostate tumors, exosomal proteins have been shown to be essential for the development of drug resistance. With increasing administrations of chemotherapeutic drugs, the rates of tumor drug resistance have been increasing year by year. Therefore, elucidation of the mechanisms involved in chemotherapeutic resistance to identify new therapeutic targets is the direction of today’s oncology research. Previous exosomes studies found that PCaDEPr regulates tumor sensitivity to drugs through various pathways. For example, Survivin is expressed in PCa-derived exosomes and its downregulation sensitizes PCa cells to chemotherapeutic agents (59). Doxorubicin is a chemotherapeutic agent that usually becomes ineffective against tumor cells over time due to chemoresistance. Breast cancer cells lacking LAMP2A exhibit increased sensitivity to this drug (224). In addition, LAMP2-mediated autophagy in PCa-derived exosomes modulates lung cancer cell resistance to temozolomide (225). Pedram et al. found that resistance of DU145 and PC-3 to docetaxel and paclitaxel was partly due to P-gp expressions and confirmed that P-gp protein levels in exosomes reflect P-gp levels in PCa cells (226).
In cisplatin-resistant ovarian cancer cells, claudin-4 was overexpressed 7.2-fold and was one of the most overexpressed proteins, suggesting that it may be associated with cisplatin resistance in ovarian cancer. Expressions of claudin, including claudin-3, -4 and -7, were markedly higher in chemoresistant ovarian cancer cells than in chemo-sensitive ovarian cancer cells. Their high expressions were positively correlated with ovarian cancer resistance to chemotherapy (227). Liu et al. found that elevated levels of synaptic binding protein-like 4 (SYTL4), a Rab effector in vesicular transport, are associated with poor prognostic outcomes in TNBC (triple negative breast cancer, referring to breast cancer lacking estrogen receptor (ESr or Er), progesterone receptor (Pr) expression with lack of epidermal growth factor receptor-2 gene (HER) expression), especially in paclitaxel treated TNBC. It has been postulated that SYTL4 confers resistance to paclitaxel in triple-negative breast cancer (110).
These findings demonstrate that PCaDEPr plays an important role in promoting drug resistance in tumor cells.
Summary and Outlook
With further research on PCaDE, tumor-derived exosomal proteins have attracted special attention. In this review, we discuss recent advances in research related to PCaDEPrs from the perspective of promoting tumorigenesis and progression. The role of these exosomal proteins present in cells or other tumors is also highlighted, although this does not mean that they remain such in specific tumor exosomes. However, because of this, this may provide researchers who identify differential proteins by routine protein analysis for subsequent functional validation with new directions for these exosomal proteins in prostate cancer research.
Although PSA is of great value as a commonly used tumor marker in the diagnosis and prognosis of prostate cancer, it has undeniable limitations, especially for the early diagnosis of bone metastatic prostate cancer. Exosomes may have more potential than PSA for therapeutic purposes, with a number of publications reporting that interference with exosome production and expression of exosome-containing substances will significantly reduce tumor metastasis and aggressiveness. In addition, important progress has been made in the study of drug-loaded exosomes, modified exosomes, and MSC exosomes in disease therapy. However, several questions remain to be addressed in future studies:1. With the study of exosome proteomics, more and more different kinds of proteins have been discovered one after another. However, it is not possible to conclude that the extracted proteins are necessarily present in exosomes according to the current database, so a more rigorous and extensive study is still needed to clarify the types of substances contained in tumor-derived exosomes in order to exclude heterogeneous proteins. 2. Due to the limitations of current extraction techniques, it is difficult to extract exosomes with 100% purity, and exosomes themselves contain a variety of secretory proteins, so it is difficult to determine the exact source of secretory proteins in exosomes of somatic fluid origin: exosomal origin? Body fluids themselves contain? 3. We found that tumors can release some exosomes rich in protective proteins that can inhibit cancer progression, so extracting these exosomes for interfering with tumor progression may be a new avenue for tumor therapy. 4. A large number of studies have found that some proteins present in exosomes and with protective effects significantly decrease with cancer progression. it remains unclear whether the effect of exosomes derived from primary and bone metastatic PCa on the establishment of the target microenvironment is persistent or transient, and further studies of these exosomes are therefore still necessary.
Bone metastatic prostate cancer and the emergence of CRPC types pose great difficulties in the treatment of PCa. Recent literature has demonstrated that tumor-derived exosomal proteins can be transported to distant metastatic targets, creating “fertile ground” to promote cancer metastasis. This may offer hope for finding ways to diagnose and treat bone metastases from prostate cancer. Furthermore, exploring the role of tumor-derived exosomes in cancer development may be a way to address these challenges. The successful treatment of these complex cancers depends on our full understanding of the single actions or interactions and mechanisms of action of the various components of exocytosis. We elucidated on the various functions and possible mechanisms of exosomeal proteins in PCa body fluids or tissues during tumor development. The exosomeal proteins can influence tumor initiation, progression, and drug resistance processes through various complex mechanisms. Elucidation of the mechanisms through which biomolecules, such as proteins, act on these processes will make it possible for us to target these proteins for cancer treatment. However, the most suitable exosomes molecular target for the diagnosis and treatment of PCa has yet to be identified, and the clinical applications of exosomes are associated with some challenges. For instance, exosomes isolation and extraction methods are still limited to the laboratory, relatively harsh storage conditions for exosomes, and medical costs. With rapid advances in exosome-related technologies and in-depth research on PCaDEPr, applications of exosomal proteins in the diagnosis and treatment of PCa will soon be realized.
Author Contributions
SF and KL searched for literature and wrote the first draft of this article. SF edited tables and figures. JZ and GZ reviewed the manuscript and polished the grammar. All authors approved the final version submitted and agree on its submission to this journal.
Funding
This work was supported by the National Natural Science Foundation of China (grant nos. 81760462 and 81860456).
Conflict of Interest
The authors declare that the research was conducted in the absence of any commercial or financial relationships that could be construed as a potential conflict of interest.
Publisher’s Note
All claims expressed in this article are solely those of the authors and do not necessarily represent those of their affiliated organizations, or those of the publisher, the editors and the reviewers. Any product that may be evaluated in this article, or claim that may be made by its manufacturer, is not guaranteed or endorsed by the publisher.
References
1. Lorenc T, Klimczyk K, Michalczewska I, Słomka M, Kubiak-Tomaszewska G, Olejarz W. Exosomes in Prostate Cancer Diagnosis, Prognosis and Therapy. Int J Mol Sci (2020) 21(6):2118. doi: 10.3390/ijms21062118
2. Liu CM, Hsieh CL, Shen CN, Lin CC, Shigemura K, Sung SY. Exosomes From the Tumor Microenvironment as Reciprocal Regulators That Enhance Prostate Cancer Progression. Int J Urol (2016) 23(9):734–44. doi: 10.1111/iju.13145
3. Guo T, Wang Y, Jia J, Mao X, Stankiewicz E, Scandura G, et al. The Identification of Plasma Exosomal miR-423-3p as a Potential Predictive Biomarker for Prostate Cancer Castration-Resistance Development by Plasma Exosomal miRNA Sequencing. Front Cell Dev Biol (2020) 8:602493. doi: 10.3389/fcell.2020.602493
4. Dragomir M, Chen B, Calin GA. Exosomal lncRNAs as New Players in Cell-to-Cell Communication. Transl Cancer Res (2018) 7(Suppl 2):S243–s252. doi: 10.21037/tcr.2017.10.46
5. Stade K, Ford CS, Guthrie C, Weis K. Exportin 1 (Crm1p) is an Essential Nuclear Export Factor. Cell (1997) 90(6):1041–50. doi: 10.1016/S0092-8674(00)80370-0
6. Duijvesz D, Burnum-Johnson KE, Gritsenko MA, Hoogland AM, Vredenbregt-van den Berg MS, Willemsen R, et al. Proteomic Profiling of Exosomes Leads to the Identification of Novel Biomarkers for Prostate Cancer. PloS One (2013) 8(12):e82589. doi: 10.1371/journal.pone.0082589
7. Kowal J, Tkach M, Théry C. Biogenesis and Secretion of Exosomes. Curr Opin Cell Biol (2014) 29:116–25. doi: 10.1016/j.ceb.2014.05.004
8. Kowal J, Arras G, Colombo M, Jouve M, Morath JP, Primdal-Bengtson B, et al. Proteomic Comparison Defines Novel Markers to Characterize Heterogeneous Populations of Extracellular Vesicle Subtypes. Proc Natl Acad Sci USA (2016) 113(8):E968–77. doi: 10.1073/pnas.1521230113
9. Andreu Z, Yáñez-Mó M. Tetraspanins in Extracellular Vesicle Formation and Function. Front Immunol (2014) 5:442. doi: 10.3389/fimmu.2014.00442
10. Shahir M, Mahmoud Hashemi S, Asadirad A, Varahram M, Kazempour-Dizaji M, Folkerts G, et al. Effect of Mesenchymal Stem Cell-Derived Exosomes on the Induction of Mouse Tolerogenic Dendritic Cells. J Cell Physiol (2020) 235(10):7043–55. doi: 10.1002/jcp.29601
11. Huang D, Chen J, Hu D, Xie F, Yang T, Li Z, et al. Advances in Biological Function and Clinical Application of Small Extracellular Vesicle Membrane Proteins. Front Oncol (2021) 11:675940. doi: 10.3389/fonc.2021.675940
12. Fhu CW, Ali A. Fatty Acid Synthase: An Emerging Target in Cancer. Mol (Basel Switzerland) (2020) 25(17):3935. doi: 10.3390/molecules25173935
13. Azizian NG, Li Y. XPO1-Dependent Nuclear Export as a Target for Cancer Therapy. J Hematol Oncol (2020) 13(1):61. doi: 10.1186/s13045-020-00903-4
14. Li HJ, Ke FY, Lin CC, Lu MY, Kuo YH, Wang YP, et al. ENO1 Promotes Lung Cancer Metastasis via HGFR and WNT Signaling-Driven Epithelial-To-Mesenchymal Transition. Cancer Res (2021) 81(15):4094–109. doi: 10.1158/0008-5472.CAN-20-3543
15. Husi H, Skipworth RJ, Cronshaw A, Stephens NA, Wackerhage H, Greig C, et al. Programmed Cell Death 6 Interacting Protein (PDCD6IP) and Rabenosyn-5 (ZFYVE20) are Potential Urinary Biomarkers for Upper Gastrointestinal Cancer. Proteomics Clin Appl (2015) 9(5-6):586–96. doi: 10.1002/prca.201400111
16. Kurozumi A, Goto Y, Matsushita R, Fukumoto I, Kato M, Nishikawa R, et al. Tumor-Suppressive microRNA-223 Inhibits Cancer Cell Migration and Invasion by Targeting ITGA3/ITGB1 Signaling in Prostate Cancer. Cancer Sci (2016) 107(1):84–94. doi: 10.1111/cas.12842
17. Pellinen T, Blom S, Sánchez S, Välimäki K, Mpindi JP, Azegrouz H, et al. ITGB1-Dependent Upregulation of Caveolin-1 Switches Tgfβ Signalling From Tumour-Suppressive to Oncogenic in Prostate Cancer. Sci Rep (2018) 8(1):2338. doi: 10.1038/s41598-018-20161-2
18. Kato T, Mizutani K, Kameyama K, Kawakami K, Fujita Y, Nakane K, et al. Serum Exosomal P-Glycoprotein is a Potential Marker to Diagnose Docetaxel Resistance and Select a Taxoid for Patients With Prostate Cancer. Urol Oncol (2015) 33(9):385.e15–20. doi: 10.1016/j.urolonc.2015.04.019
19. Itoh T, Ito Y, Ohtsuki Y, Ando M, Tsukamasa Y, Yamada N, et al. Microvesicles Released From Hormone-Refractory Prostate Cancer Cells Facilitate Mouse Pre-Osteoblast Differentiation. J Mol Histol (2012) 43(5):509–15. doi: 10.1007/s10735-012-9415-1
20. Wan X, Kim SY, Guenther LM, Mendoza A, Briggs J, Yeung C, et al. Beta4 Integrin Promotes Osteosarcoma Metastasis and Interacts With Ezrin. Oncogene (2009) 28(38):3401–11. doi: 10.1038/onc.2009.206
21. Kawakami K, Fujita Y, Kato T, Mizutani K, Kameyama K, Tsumoto H, et al. Integrin β4 and Vinculin Contained in Exosomes are Potential Markers for Progression of Prostate Cancer Associated With Taxane-Resistance. Int J Oncol (2015) 47(1):384–90. doi: 10.3892/ijo.2015.3011
22. Hosseini-Beheshti E, Pham S, Adomat H, Li N, Tomlinson Guns ES. Exosomes as Biomarker Enriched Microvesicles: Characterization of Exosomal Proteins Derived From a Panel of Prostate Cell Lines With Distinct AR Phenotypes. Mol Cell Proteomics (2012) 11(10):863–85. doi: 10.1074/mcp.M111.014845
23. Chen CY, Lin YS, Chen CH, Chen YJ. Annexin A2-Mediated Cancer Progression and Therapeutic Resistance in Nasopharyngeal Carcinoma. J Biomed Sci (2018) 25(1):30. doi: 10.1186/s12929-018-0430-8
24. Chu Y, Lai YH, Lee MC, Yeh YJ, Wu YK, Tsao W, et al. Calsyntenin-1, Clusterin and Neutrophil Gelatinase-Associated Lipocalin are Candidate Serological Biomarkers for Lung Adenocarcinoma. Oncotarget (2017) 8(64):107964–76. doi: 10.18632/oncotarget.22438
25. Watt F, Martorana A, Brookes DE, Ho T, Kingsley E, O'Keefe DS, et al. A Tissue-Specific Enhancer of the Prostate-Specific Membrane Antigen Gene, FOLH1. Genomics (2001) 73(3):243–54. doi: 10.1006/geno.2000.6446
26. Li S, Ma YM, Zheng PS, Zhang P. GDF15 Promotes the Proliferation of Cervical Cancer Cells by Phosphorylating AKT1 and Erk1/2 Through the Receptor Erbb2. J Exp Clin Cancer Res CR (2018) 37(1):80. doi: 10.1186/s13046-018-0744-0
27. Kwon HJ, Min SY, Park MJ, Lee C, Park JH, Chae JY, et al. Expression of CD9 and CD82 in Clear Cell Renal Cell Carcinoma and its Clinical Significance. Pathol Res Pract (2014) 210(5):285–90. doi: 10.1016/j.prp.2014.01.004
28. Mizutani K, Terazawa R, Kameyama K, Kato T, Horie K, Tsuchiya T, et al. Isolation of Prostate Cancer-Related Exosomes. Anticancer Res (2014) 34(7):3419–23.
29. Yang XF, Wu CJ, Chen L, Alyea EP, Canning C, Kantoff P, et al. CML28 is a Broadly Immunogenic Antigen, Which is Overexpressed In Tumor Cells. Cancer Res (2002) 62(19):5517–22.
30. Fedele C, Singh A, Zerlanko BJ, Iozzo RV, Languino LR. The αvβ6 Integrin is Transferred Intercellularly via Exosomes. J Biol Chem (2015) 290(8):4545–51. doi: 10.1074/jbc.C114.617662
31. Trerotola M, Ganguly KK, Fazli L, Fedele C, Lu H, Dutta A, et al. Trop-2 is Up-Regulated in Invasive Prostate Cancer and Displaces FAK From Focal Contacts. Oncotarget (2015) 6(16):14318–28. doi: 10.18632/oncotarget.3960
32. Kumar D, Gupta D, Shankar S, Srivastava RK. Biomolecular Characterization of Exosomes Released From Cancer Stem Cells: Possible Implications for Biomarker and Treatment of Cancer. Oncotarget (2015) 6(5):3280–91. doi: 10.18632/oncotarget.2462
33. Zhu C, Kong Z, Wang B, Cheng W, Wu A, Meng X. ITGB3/CD61: A Hub Modulator and Target in the Tumor Microenvironment. Am J Trans Res (2019) 11(12):7195–208.
34. Vences-Catalán F, Duault C, Kuo CC, Rajapaksa R, Levy R, Levy S. CD81 as a Tumor Target. Biochem Soc Trans (2017) 45(2):531–5. doi: 10.1042/BST20160478
35. Miyata Y, Nakamoto H, Neckers L. The Therapeutic Target Hsp90 and Cancer Hallmarks. Curr Pharm Design (2013) 19(3):347–65. doi: 10.2174/138161213804143725
36. Albakova Z, Armeev GA, Kanevskiy LM, Kovalenko EI, Sapozhnikov AM. HSP70 Multi-Functionality in Cancer. Cells (2020) 9(3):587. doi: 10.3390/cells9030587
37. Wang T, Wang Z, Niu R, Wang L. Crucial Role of Anxa2 in Cancer Progression: Highlights on its Novel Regulatory Mechanism. Cancer Biol Med (2019) 16(4):671–87. doi: 10.20892/j.issn.2095-3941.2019.0228
38. Ramteke A, Ting H, Agarwal C, Mateen S, Somasagara R, Hussain A, et al. Exosomes Secreted Under Hypoxia Enhance Invasiveness and Stemness of Prostate Cancer Cells by Targeting Adherens Junction Molecules. Mol Carcinog (2015) 54(7):554–65. doi: 10.1002/mc.22124
39. Colak S, Ten Dijke P. Targeting TGF-β Signaling in Cancer. Trends Cancer (2017) 3(1):56–71. doi: 10.1016/j.trecan.2016.11.008
40. Abd Elmageed ZY, Yang Y, Thomas R, Ranjan M, Mondal D, Moroz K, et al. Neoplastic Reprogramming of Patient-Derived Adipose Stem Cells by Prostate Cancer Cell-Associated Exosomes. Stem Cells (2014) 32(4):983–97. doi: 10.1002/stem.1619
41. Liu S, Liang J, Liu Z, Zhang C, Wang Y, Watson AH, et al. The Role of CD276 in Cancers. Front Oncol (2021) 11:654684. doi: 10.3389/fonc.2021.654684
42. Lu Q. δ-Catenin Dysregulation in Cancer: Interactions With E-Cadherin and Beyond. J Pathol (2010) 222(2):119–23. doi: 10.1002/path.2755
43. Lu Q, Zhang J, Allison R, Gay H, Yang WX, Bhowmick NA, et al. Identification of Extracellular Delta-Catenin Accumulation for Prostate Cancer Detection. Prostate (2009) 69(4):411–8. doi: 10.1002/pros.20902
44. Feng Y, Xiong Y, Qiao T, Li X, Jia L, Han Y, et al. A Key Player in Carcinogenesis and Potential Target in Cancer Therapy. Cancer Med (2018) 7(12):6124–36. doi: 10.1002/cam4.1820
45. Dai J, Escara-Wilke J, Keller JM, Jung Y, Taichman RS, Pienta KJ, et al. Primary Prostate Cancer Educates Bone Stroma Through Exosomal Pyruvate Kinase M2 to Promote Bone Metastasis. J Exp Med (2019) 216(12):2883–99. doi: 10.1084/jem.20190158
46. Agarwal R, D'Souza T, Morin PJ. Claudin-3 and Claudin-4 Expression in Ovarian Epithelial Cells Enhances Invasion and is Associated With Increased Matrix Metalloproteinase-2 Activity. Cancer Res (2005) 65(16):7378–85. doi: 10.1158/0008-5472.CAN-05-1036
47. Lin X, Shang X, Manorek G, Howell SB. Regulation of the Epithelial-Mesenchymal Transition by Claudin-3 and Claudin-4. PLoS One (2013) 8(6):e67496. doi: 10.1371/journal.pone.0067496
48. Kharaziha P, Chioureas D, Rutishauser D, Baltatzis G, Lennartsson L, Fonseca P, et al. Molecular Profiling of Prostate Cancer Derived Exosomes may Reveal a Predictive Signature for Response to Docetaxel. Oncotarget (2015) 6(25):21740–54. doi: 10.18632/oncotarget.3226
49. Díaz MI, Díaz P, Bennett JC, Urra H, Ortiz R, Orellana PC, et al. Caveolin-1 Suppresses Tumor Formation Through the Inhibition of the Unfolded Protein Response. Cell Death Dis (2020) 11(8):648. doi: 10.1038/s41419-020-02792-4
50. Hao J, Madigan MC, Khatri A, Power CA, Hung TT, Beretov J, et al. In Vitro and In Vivo Prostate Cancer Metastasis and Chemoresistance can be Modulated by Expression of Either CD44 or CD147. PLoS One (2012) 7(8):e40716. doi: 10.1371/journal.pone.0040716
51. Xiao Y, Zhong J, Zhong B, Huang J, Jiang L, Jiang Y, et al. Exosomes as Potential Sources of Biomarkers in Colorectal Cancer. Cancer Lett (2020) 476:13–22. doi: 10.1016/j.canlet.2020.01.033
52. Ishizuya Y, Uemura M, Narumi R, Tomiyama E, Koh Y, Matsushita M, et al. The Role of Actinin-4 (ACTN4) in Exosomes as a Potential Novel Therapeutic Target in Castration-Resistant Prostate Cancer. Biochem Biophys Res Commun (2020) 523(3):588–94. doi: 10.1016/j.bbrc.2019.12.084
53. Khurana S, Chakraborty S, Lam M, Liu Y, Su YT, Zhao X, et al. Familial Focal Segmental Glomerulosclerosis (FSGS)-Linked α-Actinin 4 (ACTN4) Protein Mutants Lose Ability to Activate Transcription by Nuclear Hormone Receptors. J Biol Chem (2012) 287(15):12027–35. doi: 10.1074/jbc.M112.345421
54. Bergelson LD. Serum Gangliosides as Endogenous Immunomodulators. Immunol Today (1995) 16(10):483–6. doi: 10.1016/0167-5699(95)80032-8
55. Hata K, Tochigi T, Sato I, Kawamura S, Shiozaki K, Wada T, et al. Increased Sialidase Activity in Serum of Cancer Patients: Identification of Sialidase and Inhibitor Activities in Human Serum. Cancer Sci (2015) 106(4):383–9. doi: 10.1111/cas.12627
56. Locke JA, Fazli L, Adomat H, Smyl J, Weins K, Lubik AA, et al. A Novel Communication Role for CYP17A1 in the Progression of Castration-Resistant Prostate Cancer. Prostate (2009) 69(9):928–37. doi: 10.1002/pros.20940
57. Hurwitz MD, Kaur P, Nagaraja GM, Bausero MA, Manola J, Asea A. Radiation Therapy Induces Circulating Serum Hsp72 in Patients With Prostate Cancer. Radiother Oncol (2010) 95(3):350–8. doi: 10.1016/j.radonc.2010.03.024
58. Kishi H, Igawa M, Kikuno N, Yoshino T, Urakami S, Shiina H. Expression of the Survivin Gene in Prostate Cancer: Correlation With Clinicopathological Characteristics, Proliferative Activity and Apoptosis. J Urol (2004) 171(5):1855–60. doi: 10.1097/01.ju.0000120317.88372.03
59. Khan S, Jutzy JM, Valenzuela MM, Turay D, Aspe JR, Ashok A, et al. Plasma-Derived Exosomal Survivin, A Plausible Biomarker for Early Detection of Prostate Cancer. PLoS One (2012) 7(10):e46737. doi: 10.1371/journal.pone.0046737
60. Krishn SR, Singh A, Bowler N, Duffy AN, Friedman A, Fedele C, et al. Prostate Cancer Sheds the αvβ3 Integrin In Vivo Through Exosomes. Matrix Biol (2019) 77:41–57. doi: 10.1016/j.matbio.2018.08.004
61. Kwon MJ. Emerging Roles of Claudins in Human Cancer. Int J Mol Sci (2013) 14(9):18148–80. doi: 10.3390/ijms140918148
62. Gagou ME, Ganesh A, Thompson R, Phear G, Sanders C, Meuth M. Suppression of Apoptosis by PIF1 Helicase in Human Tumor Cells. Cancer Res (2011) 71(14):4998–5008. doi: 10.1158/0008-5472.CAN-10-4404
63. Ding L, Wang Z, Yan J, Yang X, Liu A, Qiu W, et al. Human Four-and-a-Half LIM Family Members Suppress Tumor Cell Growth Through a TGF-Beta-Like Signaling Pathway. J Clin Invest (2009) 119(2):349–61. doi: 10.1172/JCI35930
64. Terayama M, Yamada K, Hagiwara T, Inazuka F, Sezaki T, Igari T, et al. Glutathione S-Transferase Omega 2 Regulates Cell Growth and the Expression of E-Cadherin via Post-Transcriptional Down-Regulation of β-Catenin in Human Esophageal Squamous Cells. Carcinogenesis (2020) 41(7):875–86. doi: 10.1093/carcin/bgz189
65. Bolomsky A, Heusschen R, Schlangen K, Stangelberger K, Muller J, Schreiner W, et al. Maternal Embryonic Leucine Zipper Kinase is a Novel Target for Proliferation-Associated High-Risk Myeloma. Haematologica (2018) 103(2):325–35. doi: 10.3324/haematol.2017.172973
66. Barrios N, González-Pérez E, Hernández R, Campuzano S. The Homeodomain Iroquois Proteins Control Cell Cycle Progression and Regulate the Size of Developmental Fields. PLoS Genet (2015) 11(8):e1005463. doi: 10.1371/journal.pgen.1005463
67. Berezovsky AD, Poisson LM, Cherba D, Webb CP, Transou AD, Lemke NW, et al. Sox2 Promotes Malignancy in Glioblastoma by Regulating Plasticity and Astrocytic Differentiation. Neoplasia (New York N.Y.) (2014) 16(3):193–206, 206.e19-25. doi: 10.1016/j.neo.2014.03.006
68. Lei M. The MCM Complex: Its Role in DNA Replication and Implications for Cancer Therapy. Curr Cancer Drug Targets (2005) 5(5):365–80. doi: 10.2174/1568009054629654
69. Sim EU, Lee CW, Narayanan K. The Roles of Ribosomal Proteins in Nasopharyngeal Cancer: Culprits, Sentinels or Both. biomark Res (2021) 9(1):51. doi: 10.1186/s40364-021-00311-x
70. Patnaik D, Estève PO, Pradhan S. Targeting the SET and RING-Associated (SRA) Domain of Ubiquitin-Like, PHD and Ring Finger-Containing 1 (UHRF1) for Anti-Cancer Drug Development. Oncotarget (2018) 9(40):26243–58. doi: 10.18632/oncotarget.25425
71. Walsh PC. Heterogeneity of Genetic Alterations in Prostate Cancer: Evidence of the Complex Nature of the Disease. J Urol (2002) 168(4 Pt 1):1635–6.
72. Liu Z, Johnson ST, Zhang Z, Corey DR. Expression of TNRC6 (GW182) Proteins Is Not Necessary for Gene Silencing by Fully Complementary RNA Duplexes. Nucleic Acid Ther (2019) 29(6):323–34. doi: 10.1089/nat.2019.0815
73. Anand R, Prakash SS, Veeramanikandan R, Kirubakaran R. Association Between Apolipoprotein E Genotype and Cancer Susceptibility: A Meta-Analysis. J Cancer Res Clin Oncol (2014) 140(7):1075–85. doi: 10.1007/s00432-014-1634-2
74. Welton JL, Brennan P, Gurney M, Webber JP, Spary LK, Carton DG, et al. Proteomics Analysis of Vesicles Isolated From Plasma and Urine of Prostate Cancer Patients Using a Multiplex, Aptamer-Based Protein Array. J Extracell Vesicles (2016) 5:31209. doi: 10.3402/jev.v5.31209
75. Afshar-Kharghan V. The Role of the Complement System in Cancer. J Clin Invest (2017) 127(3):780–9. doi: 10.1172/JCI90962
76. Mangogna A, Belmonte B, Agostinis C, Zacchi P, Iacopino DG, Martorana A, et al. Prognostic Implications of the Complement Protein C1q in Gliomas. Front Immunol (2019) 10:2366. doi: 10.3389/fimmu.2019.02366
77. Riihilä P, Viiklepp K, Nissinen L, Farshchian M, Kallajoki M, Kivisaari A, et al. Tumour-Cell-Derived Complement Components C1r and C1s Promote Growth of Cutaneous Squamous Cell Carcinoma. Br J Dermatol (2020) 182(3):658–70. doi: 10.1111/bjd.18095
78. Dirix LY, Salgado R, Weytjens R, Colpaert C, Benoy I, Huget P, et al. Plasma Fibrin D-Dimer Levels Correlate With Tumour Volume, Progression Rate and Survival in Patients With Metastatic Breast Cancer. Br J Cancer (2002) 86(3):389–95. doi: 10.1038/sj.bjc.6600069
79. Simpson-Haidaris PJ, Rybarczyk B. Tumors and Fibrinogen. The Role of Fibrinogen as an Extracellular Matrix Protein. Ann New York Acad Sci (2001) 936:406–25. doi: 10.1111/j.1749-6632.2001.tb03525.x
80. Lin TC, Yang CH, Cheng LH, Chang WT, Lin YR, Cheng HC. Fibronectin in Cancer: Friend or Foe. Cells (2019) 9(1):27. doi: 10.3390/cells9010027
81. Mangogna A, Varghese PM, Agostinis C, Alrokayan SH, Khan HA, Stover CM, et al. Prognostic Value of Complement Properdin in Cancer. Front Immunol (2020) 11:614980. doi: 10.3389/fimmu.2020.614980
82. Yang AJ, Wang M, Wang Y, Cai W, Li Q, Zhao TT, et al. Cancer Cell-Derived Von Willebrand Factor Enhanced Metastasis of Gastric Adenocarcinoma. Oncogenesis (2018) 7(1):12. doi: 10.1038/s41389-017-0023-5
83. Gabriel K, Ingram A, Austin R, Kapoor A, Tang D, Majeed F, et al. Regulation of the Tumor Suppressor PTEN Through Exosomes: A Diagnostic Potential for Prostate Cancer. PloS One (2013) 8(7):e70047. doi: 10.1371/journal.pone.0070047
84. Frank MH, Denton MD, Alexander SI, Khoury SJ, Sayegh MH, Briscoe DM. Specific MDR1 P-Glycoprotein Blockade Inhibits Human Alloimmune T Cell Activation In Vitro. J Immunol (Baltimore Md. 1950) (2001) 166(4):2451–9. doi: 10.4049/jimmunol.166.4.2451
85. Cory TJ, He H, Winchester LC, Kumar S, Fletcher CV. Alterations in P-Glycoprotein Expression and Function Between Macrophage Subsets. Pharm Res (2016) 33(11):2713–21. doi: 10.1007/s11095-016-1998-x
86. Øverbye A, Skotland T, Koehler CJ, Thiede B, Seierstad T, Berge V, et al. Identification of Prostate Cancer Biomarkers in Urinary Exosomes. Oncotarget (2015) 6(30):30357–76. doi: 10.18632/oncotarget.4851
87. Bhardwaj A, Singh S, Srivastava SK, Arora S, Hyde SJ, Andrews J, et al. Restoration of PPP2CA Expression Reverses Epithelial-to-Mesenchymal Transition and Suppresses Prostate Tumour Growth and Metastasis in an Orthotopic Mouse Model. Br J Cancer (2014) 110(8):2000–10. doi: 10.1038/bjc.2014.141
88. Shaughnessy R, Echard A. Rab35 GTPase and Cancer: Linking Membrane Trafficking to Tumorigenesis. Traffic (Copenhagen Denmark) (2018) 19(4):247–52. doi: 10.1111/tra.12546
89. Nedjadi T, Kitteringham N, Campbell F, Jenkins RE, Park BK, Navarro P, et al. S100A6 Binds to Annexin 2 in Pancreatic Cancer Cells and Promotes Pancreatic Cancer Cell Motility. Br J Cancer (2009) 101(7):1145–54. doi: 10.1038/sj.bjc.6605289
90. Di Virgilio F. Purines, Purinergic Receptors, and Cancer. Cancer Res (2012) 72(21):5441–7. doi: 10.1158/0008-5472.CAN-12-1600
91. Hsu DK, Chen HY, Liu FT. Galectin-3 Regulates T-Cell Functions. Immunol Rev (2009) 230(1):114–27. doi: 10.1111/j.1600-065X.2009.00798.x
92. Nakahara S, Oka N, Raz A. On the Role of Galectin-3 in Cancer Apoptosis. Apoptosis an Int J Programmed Cell Death (2005) 10(2):267–75. doi: 10.1007/s10495-005-0801-y
93. Funasaka T, Raz A, Nangia-Makker P. Galectin-3 in Angiogenesis and Metastasis. Glycobiology (2014) 24(10):886–91. doi: 10.1093/glycob/cwu086
94. Merseburger AS, Kramer MW, Hennenlotter J, Simon P, Knapp J, Hartmann JT, et al. Involvement of Decreased Galectin-3 Expression in the Pathogenesis and Progression of Prostate Cancer. Prostate (2008) 68(1):72–7. doi: 10.1002/pros.20688
95. Gauthier-Rouvière C, Bodin S, Comunale F, Planchon D. Flotillin Membrane Domains in Cancer. Cancer Metastasis Rev (2020) 39(2):361–74. doi: 10.1007/s10555-020-09873-y
96. Cifuentes E, Mataraza JM, Yoshida BA, Menon M, Sacks DB, Barrack ER, et al. Physical and Functional Interaction of Androgen Receptor With Calmodulin in Prostate Cancer Cells. Proc Natl Acad Sci USA (2004) 101(2):464–9. doi: 10.1073/pnas.0307161101
97. Liang H, Xiong Z, Li R, Hu K, Cao M, Yang J, et al. BDH2 is Downregulated in Hepatocellular Carcinoma and Acts as a Tumor Suppressor Regulating Cell Apoptosis and Autophagy. J Cancer (2019) 10(16):3735–45. doi: 10.7150/jca.32022
98. Zhang SF, Wang XY, Fu ZQ, Peng QH, Zhang JY, Ye F, et al. TXNDC17 Promotes Paclitaxel Resistance via Inducing Autophagy in Ovarian Cancer. Autophagy (2015) 11(2):225–38. doi: 10.1080/15548627.2014.998931
99. Wu Y, Du H, Zhan M, Wang H, Chen P, Du D, et al. Sepiapterin Reductase Promotes Hepatocellular Carcinoma Progression via FoxO3a/Bim Signaling in a Nonenzymatic Manner. Cell Death Dis (2020) 11(4):248. doi: 10.1038/s41419-020-2471-7
100. Zhang X, Chen Y, Wang K, Tang J, Chen Y, Jin G, et al. The Knockdown of the Sepiapterin Reductase Gene Suppresses the Proliferation of Breast Cancer by Inducing ROS-Mediated Apoptosis. Int J Clin Exp Pathol (2020) 13(9):2228–39.
101. Zhang T, Sun Y, Zheng T, Wang R, Jia D, Zhang W. MLPH Accelerates the Epithelial-Mesenchymal Transition in Prostate Cancer. Onco Targets Ther (2020) 13:701–8. doi: 10.2147/OTT.S225023
102. Wei CY, Zhu MX, Lu NH, Peng R, Yang X, Zhang PF, et al. Bioinformatics-Based Analysis Reveals Elevated MFSD12 as a Key Promoter of Cell Proliferation and a Potential Therapeutic Target in Melanoma. Oncogene (2019) 38(11):1876–91. doi: 10.1038/s41388-018-0531-6
103. Gonzalez A, Valeiras M, Sidransky E, Tayebi N. Lysosomal Integral Membrane Protein-2: A New Player in Lysosome-Related Pathology. Mol Genet Metab (2014) 111(2):84–91. doi: 10.1016/j.ymgme.2013.12.005
104. Li D, Cheng X, Zheng W, Chen J. Glucosamine-6-Phosphate Isomerase 1 Promotes Tumor Progression and Indicates Poor Prognosis in Hepatocellular Carcinoma. Cancer Manag Res (2020) 12:4923–35. doi: 10.2147/CMAR.S250094
105. Moriwaki K, Shinzaki S, Miyoshi E. GDP-Mannose-4,6-Dehydratase (GMDS) Deficiency Renders Colon Cancer Cells Resistant to Tumor Necrosis Factor-Related Apoptosis-Inducing Ligand (TRAIL) Receptor- and CD95-Mediated Apoptosis by Inhibiting Complex II Formation. J Biol Chem (2011) 286(50):43123–33. doi: 10.1074/jbc.M111.262741
106. Li Z, Xuan W, Huang L, Chen N, Hou Z, Lu B, et al. Claudin 10 Acts as a Novel Biomarker for the Prognosis of Patients With Ovarian Cancer. Oncol Lett (2020) 20(1):373–81. doi: 10.3892/ol.2020.11557
107. Andrijes R, Hejmadi RK, Pugh M, Rajesh S, Novitskaya V, Ibrahim M, et al. Tetraspanin 6 is a Regulator of Carcinogenesis in Colorectal Cancer. Proc Natl Acad Sci USA (2021) 118(39):e2011411118. doi: 10.1073/pnas.2011411118
108. Shih Ie M, Wang TL. Notch Signaling, Gamma-Secretase Inhibitors, and Cancer Therapy. Cancer Res (2007) 67(5):1879–82. doi: 10.1158/0008-5472.CAN-06-3958
109. Wu PH, Onodera Y, Giaccia AJ, Le QT, Shimizu S, Shirato H, et al. Lysosomal Trafficking Mediated by Arl8b and BORC Promotes Invasion of Cancer Cells That Survive Radiation. Commun Biol (2020) 3(1):620. doi: 10.1038/s42003-020-01339-9
110. Liu XY, Jiang W, Ma D, Ge LP, Yang YS, Gou ZC, et al. SYTL4 Downregulates Microtubule Stability and Confers Paclitaxel Resistance in Triple-Negative Breast Cancer. Theranostics (2020) 10(24):10940–56. doi: 10.7150/thno.45207
111. Arumugam T, Logsdon CD. S100P: A Novel Therapeutic Target for Cancer. Amino Acids (2011) 41(4):893–9. doi: 10.1007/s00726-010-0496-4
112. Kim RH, Peters M, Jang Y, Shi W, Pintilie M, Fletcher GC, et al. DJ-1, a Novel Regulator of the Tumor Suppressor PTEN. Cancer Cell (2005) 7(3):263–73. doi: 10.1016/j.ccr.2005.02.010
113. Scarl RT, Lawrence CM, Gordon HM, Nunemaker CS. STEAP4: Its Emerging Role in Metabolism and Homeostasis of Cellular Iron and Copper. J Endocrinol (2017) 234(3):R123–r134. doi: 10.1530/JOE-16-0594
114. Zou P, Yang Y, Xu X, Liu B, Mei F, You J, et al. Silencing of Vacuolar ATPase C Subunit ATP6V0C Inhibits the Invasion of Prostate Cancer Cells Through a LASS2/TMSG1-Independent Manner. Oncol Rep (2018) 39(1):298–306. doi: 10.3892/or.2017.6092
115. Steffan JJ, Dykes SS, Coleman DT, Adams LK, Rogers D, Carroll JL, et al. Supporting a Role for the GTPase Rab7 in Prostate Cancer Progression. PLoS One (2014) 9(2):e87882. doi: 10.1371/journal.pone.0087882
116. Yang J, Liu W, Lu X, Fu Y, Li L, Luo Y. High Expression of Small GTPase Rab3D Promotes Cancer Progression and Metastasis. Oncotarget (2015) 6(13):11125–38. doi: 10.18632/oncotarget.3575
117. Tan PY, Chang CW, Chng KR, Wansa KD, Sung WK, Cheung E. Integration of Regulatory Networks by NKX3-1 Promotes Androgen-Dependent Prostate Cancer Survival. Mol Cell Biol (2012) 32(2):399–414. doi: 10.1128/MCB.05958-11
118. Luo ML, Gong C, Chen CH, Hu H, Huang P, Zheng M, et al. The Rab2A GTPase Promotes Breast Cancer Stem Cells and Tumorigenesis via Erk Signaling Activation. Cell Rep (2015) 11(1):111–24. doi: 10.1016/j.celrep.2015.03.002
119. Riplinger SM, Wabnitz GH, Kirchgessner H, Jahraus B, Lasitschka F, Schulte B, et al. Metastasis of Prostate Cancer and Melanoma Cells in a Preclinical In Vivo Mouse Model is Enhanced by L-Plastin Expression and Phosphorylation. Mol Cancer (2014) 13:10. doi: 10.1186/1476-4598-13-10
120. Colaço A, Jäättelä M. Ragulator-A Multifaceted Regulator of Lysosomal Signaling and Trafficking. J Cell Biol (2017) 216(12):3895–8. doi: 10.1083/jcb.201710039
121. Eriksson P, Aine M, Veerla S, Liedberg F, Sjödahl G, Höglund M. Molecular Subtypes of Urothelial Carcinoma are Defined by Specific Gene Regulatory Systems. BMC Med Genomics (2015) 8:25. doi: 10.1186/s12920-015-0101-5
123. Gu TL, Cherry J, Tucker M, Wu J, Reeves C, Polakiewicz RD. Identification of Activated Tnk1 Kinase in Hodgkin's Lymphoma. Leukemia (2010) 24(4):861–5. doi: 10.1038/leu.2009.293
124. Hazarika P, McCarty MF, Prieto VG, George S, Babu D, Koul D, et al. Up-Regulation of Flotillin-2 is Associated With Melanoma Progression and Modulates Expression of the Thrombin Receptor Protease Activated Receptor 1. Cancer Res (2004) 64(20):7361–9. doi: 10.1158/0008-5472.CAN-04-0823
125. Liu J, Huang W, Ren C, Wen Q, Liu W, Yang X, et al. Flotillin-2 Promotes Metastasis of Nasopharyngeal Carcinoma by Activating NF-κb and PI3K/Akt3 Signaling Pathways. Sci Rep (2015) 5:11614. doi: 10.1038/srep11614
126. Wang CH, Zhu XD, Ma DN, Sun HC, Gao DM, Zhang N, et al. Flot2 Promotes Tumor Growth and Metastasis Through Modulating Cell Cycle and Inducing Epithelial-Mesenchymal Transition of Hepatocellular Carcinoma. Am J Cancer Res (2017) 7(5):1068–83.
127. Ronquist KG, Ronquist G, Larsson A, Carlsson L. Proteomic Analysis of Prostate Cancer Metastasis-Derived Prostasomes. Anticancer Res (2010) 30(2):285–90.
128. Kennedy L, Sandhu JK, Harper ME, Cuperlovic-Culf M. Role of Glutathione in Cancer: From Mechanisms to Therapies. Biomolecules (2020) 10(10):1429. doi: 10.3390/biom10101429
129. Zhao X, Fu J, Du J, Xu W. The Role of D-3-Phosphoglycerate Dehydrogenase in Cancer. Int J Biol Sci (2020) 16(9):1495–506. doi: 10.7150/ijbs.41051
130. Kim E, Kwak H, Ahn K. Cytosolic Aminopeptidases Influence MHC Class I-Mediated Antigen Presentation in an Allele-Dependent Manner. J Immunol (Baltimore Md. 1950) (2009) 183(11):7379–87. doi: 10.4049/jimmunol.0901489
131. Almaguel FA, Sanchez TW, Ortiz-Hernandez GL, Casiano CA. Alpha-Enolase: Emerging Tumor-Associated Antigen, Cancer Biomarker, and Oncotherapeutic Target. Front Genet (2020) 11:614726. doi: 10.3389/fgene.2020.614726
132. Chen J, Cheng X, Merched-Sauvage M, Caulin C, Roop DR, Koch PJ. An Unexpected Role for Keratin 10 End Domains in Susceptibility to Skin Cancer. J Cell Sci (2006) 119(Pt 24):5067–76. doi: 10.1242/jcs.03298
133. Guo C, Liu S, Wang J, Sun MZ, Greenaway FT. ACTB in Cancer. Clin Chim acta; Int J Clin Chem (2013) 417:39–44. doi: 10.1016/j.cca.2012.12.012
134. Calvert AE, Chalastanis A, Wu Y, Hurley LA, Kouri FM, Bi Y, et al. Cancer-Associated IDH1 Promotes Growth and Resistance to Targeted Therapies in the Absence of Mutation. Cell Rep (2017) 19(9):1858–73. doi: 10.1016/j.celrep.2017.05.014
135. Jelski W, Szmitkowski M. Alcohol Dehydrogenase (ADH) and Aldehyde Dehydrogenase (ALDH) in the Cancer Diseases. Clin Chim acta; Int J Clin Chem (2008) 395(1-2):1–5. doi: 10.1016/j.cca.2008.05.001
136. Bardella C, Pollard PJ, Tomlinson I. SDH Mutations in Cancer. Biochim Biophys Acta (2011) 1807(11):1432–43. doi: 10.1016/j.bbabio.2011.07.003
137. Huang D, Cao L, Zheng S. CAPZA1 Modulates EMT by Regulating Actin Cytoskeleton Remodelling in Hepatocellular Carcinoma. J Exp Clin Cancer Res CR (2017) 36(1):13. doi: 10.1186/s13046-016-0474-0
138. Hulin JA, Tommasi S, Elliot D, Mangoni AA. Small Molecule Inhibition of DDAH1 Significantly Attenuates Triple Negative Breast Cancer Cell Vasculogenic Mimicry In Vitro. Biomed Pharmacother (2019) 111:602–12. doi: 10.1016/j.biopha.2018.12.117
139. Scannell M, Flanagan MB, deStefani A, Wynne KJ, Cagney G, Godson C, et al. Annexin-1 and Peptide Derivatives are Released by Apoptotic Cells and Stimulate Phagocytosis of Apoptotic Neutrophils by Macrophages. J Immunol (Baltimore Md. 1950) (2007) 178(7):4595–605. doi: 10.4049/jimmunol.178.7.4595
140. Blume KE, Soeroes S, Waibel M, Keppeler H, Wesselborg S, Herrmann M, et al. Cell Surface Externalization of Annexin A1 as a Failsafe Mechanism Preventing Inflammatory Responses During Secondary Necrosis. J Immunol (Baltimore Md. 1950) (2009) 183(12):8138–47. doi: 10.4049/jimmunol.0902250
141. Huggins A, Paschalidis N, Flower RJ, Perretti M, D'Acquisto F. Annexin-1-Deficient Dendritic Cells Acquire a Mature Phenotype During Differentiation. FASEB J Off Publ Fed Am Soc Exp Biol (2009) 23(4):985–96. doi: 10.1096/fj.08-119040
142. Bist P, Shu S, Lee H, Arora S, Nair S, Lim JY, et al. Annexin-A1 Regulates TLR-Mediated IFN-β Production Through an Interaction With TANK-Binding Kinase 1. J Immunol (Baltimore Md. 1950) (2013) 191(8):4375–82. doi: 10.4049/jimmunol.1301504
143. Jorge YC, Mataruco MM, Araújo LP, Rossi AF, de Oliveira JG, Valsechi MC, et al. Expression of Annexin-A1 and Galectin-1 Anti-Inflammatory Proteins and mRNA in Chronic Gastritis and Gastric Cancer. Mediators Inflammation (2013) 2013:152860. doi: 10.1155/2013/152860
144. Ullah MF. Sulforaphane (SFN): An Isothiocyanate in a Cancer Chemoprevention Paradigm. Medicines (Basel Switzerland) (2015) 2(3):141–56. doi: 10.3390/medicines2030141
145. Tang J, Qin Z, Han P, Wang W, Yang C, Xu Z, et al. High Annexin A5 Expression Promotes Tumor Progression and Poor Prognosis in Renal Cell Carcinoma. Int J Oncol (2017) 50(5):1839–47. doi: 10.3892/ijo.2017.3942
146. Yang L, Lu P, Yang X, Li K, Qu S. Annexin A3, A Calcium-Dependent Phospholipid-Binding Protein: Implication in Cancer. Front Mol Biosci (2021) 8:716415. doi: 10.3389/fmolb.2021.716415
147. Iwamoto K, Takahashi H, Okuzaki D, Osawa H, Ogino T, Miyoshi N, et al. Syntenin-1 Promotes Colorectal Cancer Stem Cell Expansion and Chemoresistance by Regulating Prostaglandin E2 Receptor. Br J Cancer (2020) 123(6):955–64. doi: 10.1038/s41416-020-0965-9
148. van Ommeren R, Staudt MD, Xu H, Hebb MO. Advances in HSP27 and HSP90-Targeting Strategies for Glioblastoma. J Neuro-oncol (2016) 127(2):209–19. doi: 10.1007/s11060-016-2070-8
149. Hansen RK, Parra I, Hilsenbeck SG, Himelstein B, Fuqua SA. Hsp27-Induced MMP-9 Expression is Influenced by the Src Tyrosine Protein Kinase Yes. Biochem Biophys Res Commun (2001) 282(1):186–93. doi: 10.1006/bbrc.2001.4548
150. Lemieux P, Oesterreich S, Lawrence JA, Steeg PS, Hilsenbeck SG, Harvey JM, et al. The Small Heat Shock Protein Hsp27 Increases Invasiveness But Decreases Motility of Breast Cancer Cells. Invasion Metastasis (1997) 17(3):113–23.
151. Liang HH, Huang CY, Chou CW, Makondi PT, Huang MT, Wei PL, et al. Heat Shock Protein 27 Influences the Anti-Cancer Effect of Curcumin in Colon Cancer Cells Through ROS Production and Autophagy Activation. Life Sci (2018) 209:43–51. doi: 10.1016/j.lfs.2018.07.047
152. Chang X-Z, Li D-Q, Hou Y-F, Wu J, Lu J-S, Di G-H, et al. Identification of the Functional Role of Peroxiredoxin 6 in the Progression of Breast Cancer. Breast Cancer Res (2007) 9(6):1–15. doi: 10.1186/bcr1789
153. Chen T, Huang Z, Tian Y, Lin B, He R, Wang H, et al. Clinical Significance and Prognostic Value of Triosephosphate Isomerase Expression in Gastric Cancer. Medicine (2017) 96(19):e6865. doi: 10.1097/MD.0000000000006865
154. Schoentgen F, Jonic SJ, APA. PEBP1/RKIP: From Multiple Functions to a Common Role in Cellular Processes. (2018) arXiv:1802.02378. doi: doi: 10.48550/arXiv.1802.02378
155. Ishiguro H, Izumi K, Kashiwagi E, Zheng Y, Li Y, Kawahara T, et al. Semenogelin I Promotes Prostate Cancer Cell Growth via Functioning as an Androgen Receptor Coactivator and Protecting Against Zinc Cytotoxicity. Am J Cancer Res (2015) 5(2):738–47.
156. Hileman EA, Achanta G, Huang P. Superoxide Dismutase: An Emerging Target for Cancer Therapeutics. Expert Opin Ther Targets (2001) 5(6):697–710. doi: 10.1517/14728222.5.6.697
157. Hosseini SM, Okoye I, Chaleshtari MG, Hazhirkarzar B, Mohamadnejad J, Azizi G, et al. E2 Ubiquitin-Conjugating Enzymes in Cancer: Implications for Immunotherapeutic Interventions. Clin Chim acta; Int J Clin Chem (2019) 498:126–34. doi: 10.1016/j.cca.2019.08.020
158. Edechi CA, Ikeogu NM, Akaluka GN, Terceiro LEL, Machado M, Salako ES, et al. The Prolactin Inducible Protein Modulates Antitumor Immune Responses and Metastasis in a Mouse Model of Triple Negative Breast Cancer. Front Oncol (2021) 11:639859. doi: 10.3389/fonc.2021.639859
159. Markowitz J, Carson WE. 3rd, Review of S100A9 Biology and its Role in Cancer. Biochim Biophys Acta (2013) 1835(1):100–9. doi: 10.1016/j.bbcan.2012.10.003
160. Wei X, Zhou J, Hong L, Xu Z, Zhao H, Wu X, et al. Hint1 Expression Inhibits Proliferation and Promotes Radiosensitivity of Human SGC7901 Gastric Cancer Cells. Oncol Lett (2018) 16(2):2135–42. doi: 10.3892/ol.2018.8900
161. Duman C, Yaqubi K, Hoffmann A, Acikgöz AA, Korshunov A, Bendszus M, et al. Acyl-CoA-Binding Protein Drives Glioblastoma Tumorigenesis by Sustaining Fatty Acid Oxidation. Cell Metab (2019) 30(2):274–89.e5. doi: 10.1016/j.cmet.2019.04.004
162. Meng M, Sang L, Wang X. S100 Calcium Binding Protein A11 (S100A11) Promotes The Proliferation, Migration And Invasion Of Cervical Cancer Cells, And Activates Wnt/β-Catenin Signaling. Onco Targets Ther (2019) 12:8675–85. doi: 10.2147/OTT.S225248
163. Luanpitpong S, Talbott SJ, Rojanasakul Y, Nimmannit U, Pongrakhananon V, Wang L, et al. Regulation of Lung Cancer Cell Migration and Invasion by Reactive Oxygen Species and Caveolin-1. J Biol Chem (2010) 285(50):38832–40. doi: 10.1074/jbc.M110.124958
164. Basu GD, Azorsa DO, Kiefer JA, Rojas AM, Tuzmen S, Barrett MT, et al. Functional Evidence Implicating S100P in Prostate Cancer Progression. Int J Cancer (2008) 123(2):330–9. doi: 10.1002/ijc.23447
165. Lange I, Geerts D, Feith DJ, Mocz G, Koster J, Bachmann AS. Novel Interaction of Ornithine Decarboxylase With Sepiapterin Reductase Regulates Neuroblastoma Cell Proliferation. J Mol Biol (2014) 426(2):332–46. doi: 10.1016/j.jmb.2013.09.037
166. Liu H, Liu JY, Wu X, Zhang JT. Biochemistry, Molecular Biology, and Pharmacology of Fatty Acid Synthase, an Emerging Therapeutic Target and Diagnosis/Prognosis Marker. Int J Biochem Mol Biol (2010) 1(1):69–89.
167. Słomnicki ŁP, Nawrot B, Leśniak W. S100A6 Binds P53 and Affects its Activity. Int J Biochem Cell Biol (2009) 41(4):784–90. doi: 10.1016/j.biocel.2008.08.007
168. van Dieck J, Brandt T, Teufel DP, Veprintsev DB, Joerger AC, Fersht AR. Molecular Basis of S100 Proteins Interacting With the P53 Homologs P63 and P73. Oncogene (2010) 29(14):2024–35. doi: 10.1038/onc.2009.490
169. Qin DN, Zhu JG, Ji CB, Chunmei S, Kou CZ, Zhu GZ, et al. Monoclonal Antibody to Six Transmembrane Epithelial Antigen of Prostate-4 Influences Insulin Sensitivity by Attenuating Phosphorylation of P13K (P85) and Akt: Possible Mitochondrial Mechanism. J Bioenerg Biomembr (2011) 43(3):247–55. doi: 10.1007/s10863-011-9360-9
170. Webber JP, Spary LK, Sanders AJ, Chowdhury R, Jiang WG, Steadman R, et al. Differentiation of Tumour-Promoting Stromal Myofibroblasts by Cancer Exosomes. Oncogene (2015) 34(3):290–302. doi: 10.1038/onc.2013.560
171. Hayes JD, Dinkova-Kostova AT, Tew KD. Oxidative Stress in Cancer. Cancer Cell (2020) 38(2):167–97. doi: 10.1016/j.ccell.2020.06.001
172. Ying W. NAD+/NADH and NADP+/NADPH in Cellular Functions and Cell Death: Regulation and Biological Consequences. Antioxid Redox Signaling (2008) 10(2):179–206. doi: 10.1089/ars.2007.1672
173. Neophytou CM, Panagi M, Stylianopoulos T, Papageorgis P. The Role of Tumor Microenvironment in Cancer Metastasis: Molecular Mechanisms and Therapeutic Opportunities. Cancers (2021) 13(9):2053. doi: 10.3390/cancers13092053
174. Thuringer D, Jego G, Wettstein G, Terrier O, Cronier L, Yousfi N, et al. Extracellular HSP27 Mediates Angiogenesis Through Toll-Like Receptor 3. FASEB J Off Publ Fed Am Soc Exp Biol (2013) 27(10):4169–83. doi: 10.1096/fj.12-226977
175. Jang D, Kwon H, Jeong K, Lee J, Pak Y. Essential Role of Flotillin-1 Palmitoylation in the Intracellular Localization and Signaling Function of IGF-1 Receptor. J Cell Sci (2015) 128(11):2179–90. doi: 10.1242/jcs.169409
176. Shinbo Y, Taira T, Niki T, Iguchi-Ariga SM, Ariga H. DJ-1 Restores P53 Transcription Activity Inhibited by Topors/P53bp3. Int J Oncol (2005) 26(3):641–8. doi: 10.3892/ijo.26.3.641
177. Takahashi-Niki K, Ganaha Y, Niki T, Nakagawa S, Kato-Ose I, Iguchi-Ariga SMM, et al. DJ-1 Activates SIRT1 Through its Direct Binding to SIRT1. Biochem Biophys Res Commun (2016) 474(1):131–6. doi: 10.1016/j.bbrc.2016.04.084
178. Watanabe J, Kamata Y, Seo N, Okayasu I, Kuramoto H. Stimulatory Effect of Estrogen on the Growth of Endometrial Cancer Cells is Regulated by Cell-Cycle Regulators. J Steroid Biochem Mol Biol (2007) 107(3-5):163–71. doi: 10.1016/j.jsbmb.2007.03.045
179. Bosch M, Gil J, Bachs O, Agell N. Calmodulin Inhibitor W13 Induces Sustained Activation of ERK2 and Expression of P21(Cip1). J Biol Chem (1998) 273(34):22145–50. doi: 10.1074/jbc.273.34.22145
180. Pandey MK, Prasad S, Tyagi AK, Deb L, Huang J, Karelia DN, et al. Targeting Cell Survival Proteins for Cancer Cell Death. Pharmaceut (Basel Switzerland) (2016) 9(1):11. doi: 10.3390/ph9010011
181. Boroughs LK, DeBerardinis RJ. Metabolic Pathways Promoting Cancer Cell Survival and Growth. Nat Cell Biol (2015) 17(4):351–9. doi: 10.1038/ncb3124
182. Liu Y, Shi K, Chen Y, Wu X, Chen Z, Cao K, et al. Exosomes and Their Role in Cancer Progression. Front Oncol (2021) 11:639159. doi: 10.3389/fonc.2021.639159
183. Gordon SR, Maute RL, Dulken BW, Hutter G, George BM, McCracken MN, et al. PD-1 Expression by Tumour-Associated Macrophages Inhibits Phagocytosis and Tumour Immunity. Nature (2017) 545(7655):495–9. doi: 10.1038/nature22396
184. Dong H, Strome SE, Salomao DR, Tamura H, Hirano F, Flies DB, et al. Tumor-Associated B7-H1 Promotes T-Cell Apoptosis: A Potential Mechanism of Immune Evasion. Nat Med (2002) 8(8):793–800. doi: 10.1038/nm730
185. Clayton A, Mitchell JP, Court J, Linnane S, Mason MD, Tabi Z. Human Tumor-Derived Exosomes Down-Modulate NKG2D Expression. J Immunol (Baltimore Md. 1950) (2008) 180(11):7249–58. doi: 10.4049/jimmunol.180.11.7249
186. Clayton A, Mitchell JP, Court J, Mason MD, Tabi Z. Human Tumor-Derived Exosomes Selectively Impair Lymphocyte Responses to Interleukin-2. Cancer Res (2007) 67(15):7458–66. doi: 10.1158/0008-5472.CAN-06-3456
187. Novitskiy SV, Ryzhov S, Zaynagetdinov R, Goldstein AE, Huang Y, Tikhomirov OY, et al. Adenosine Receptors in Regulation of Dendritic Cell Differentiation and Function. Blood (2008) 112(5):1822–31. doi: 10.1182/blood-2008-02-136325
188. Wang R, Liu Y, Liu L, Chen M, Wang X, Yang J, et al. Tumor Cells Induce LAMP2a Expression in Tumor-Associated Macrophage for Cancer Progression. EBioMedicine (2019) 40:118–34. doi: 10.1016/j.ebiom.2019.01.045
189. Cho JA, Park H, Lim EH, Lee KW. Exosomes From Breast Cancer Cells can Convert Adipose Tissue-Derived Mesenchymal Stem Cells Into Myofibroblast-Like Cells. Int J Oncol (2012) 40(1):130–8. doi: 10.3892/ijo.2011.1193
190. Zhong H, Chiles K, Feldser D, Laughner E, Hanrahan C, Georgescu MM, et al. Modulation of Hypoxia-Inducible Factor 1alpha Expression by the Epidermal Growth Factor/Phosphatidylinositol 3-Kinase/PTEN/AKT/FRAP Pathway in Human Prostate Cancer Cells: Implications for Tumor Angiogenesis and Therapeutics. Cancer Res (2000) 60(6):1541–5.
191. Chien CH, Lee MJ, Liou HC, Liou HH, Fu WM. Local Immunosuppressive Microenvironment Enhances Migration of Melanoma Cells to Lungs in DJ-1 Knockout Mice. PLoS One (2015) 10(2):e0115827. doi: 10.1371/journal.pone.0115827
192. Huggins C, Hodges CV. Studies on Prostatic Cancer. I. The Effect of Castration, of Estrogen and Androgen Injection on Serum Phosphatases in Metastatic Carcinoma of the Prostate. CA: Cancer J Clin (1972) 22(4):232–40. doi: 10.3322/canjclin.22.4.232
193. Attard G, Reid AH, Auchus RJ, Hughes BA, Cassidy AM, Thompson E, et al. Clinical and Biochemical Consequences of CYP17A1 Inhibition With Abiraterone Given With and Without Exogenous Glucocorticoids in Castrate Men With Advanced Prostate Cancer. J Clin Endocrinol Metab (2012) 97(2):507–16. doi: 10.1210/jc.2011-2189
194. Cheng KW, Agarwal R, Mitra S, Lee JS, Carey M, Gray JW, et al. Rab25 Increases Cellular ATP and Glycogen Stores Protecting Cancer Cells From Bioenergetic Stress. EMBO Mol Med (2012) 4(2):125–41. doi: 10.1002/emmm.201100193
195. Walther TC, Chung J, Farese RV Jrs. Lipid Droplet Biogenesis. Annu Rev Cell Dev Biol (2017) 33:491–510. doi: 10.1146/annurev-cellbio-100616-060608
196. Wu L, Xu D, Zhou L, Xie B, Yu L, Yang H, et al. Rab8a-AS160-MSS4 Regulatory Circuit Controls Lipid Droplet Fusion and Growth. Dev Cell (2014) 30(4):378–93. doi: 10.1016/j.devcel.2014.07.005
197. Raposo G, Stoorvogel W. Extracellular Vesicles: Exosomes, Microvesicles, and Friends. J Cell Biol (2013) 200(4):373–83. doi: 10.1083/jcb.201211138
198. Schiefermeier N, Scheffler JM, de Araujo ME, Stasyk T, Yordanov T, Ebner HL, et al. The Late Endosomal P14-MP1 (LAMTOR2/3) Complex Regulates Focal Adhesion Dynamics During Cell Migration. J Cell Biol (2014) 205(4):525–40. doi: 10.1083/jcb.201310043
199. Tuli A, Thiery J, James AM, Michelet X, Sharma M, Garg S, et al. Arf-Like GTPase Arl8b Regulates Lytic Granule Polarization and Natural Killer Cell-Mediated Cytotoxicity. Mol Biol Cell (2013) 24(23):3721–35. doi: 10.1091/mbc.e13-05-0259
200. Shin IY, Sung NY, Lee YS, Kwon TS, Si Y, Lee YS, et al. The Expression of Multiple Proteins as Prognostic Factors in Colorectal Cancer: Cathepsin D, P53, COX-2, Epidermal Growth Factor Receptor, C-erbB-2, and Ki-67. Gut Liver (2014) 8(1):13–23. doi: 10.5009/gnl.2014.8.1.13
201. Hahm ER, Singh KB, Kim SH, Powolny AA, Singh SV. The Role of Lysosome-Associated Membrane Protein 2 in Prostate Cancer Chemopreventive Mechanisms of Sulforaphane. Cancer Prev Res (Phila) (2020) 13(8):661–72. doi: 10.1158/1940-6207.CAPR-20-0054
202. Ding ZB, Fu XT, Shi YH, Zhou J, Peng YF, Liu WR, et al. Lamp2a is Required for Tumor Growth and Promotes Tumor Recurrence of Hepatocellular Carcinoma. Int J Oncol (2016) 49(6):2367–76. doi: 10.3892/ijo.2016.3754
203. Timme TL, Goltsov A, Tahir S, Li L, Wang J, Ren C, et al. Caveolin-1 is Regulated by C-Myc and Suppresses C-Myc-Induced Apoptosis. Oncogene (2000) 19(29):3256–65. doi: 10.1038/sj.onc.1203654
204. Sporn MB. The War on Cancer. Lancet (London England) (1996) 347(9012):1377–81. doi: 10.1016/S0140-6736(96)91015-6
205. Bijnsdorp IV, Geldof AA, Lavaei M, Piersma SR, van Moorselaar RJ, Jimenez CR. Exosomal ITGA3 Interferes With non-Cancerous Prostate Cell Functions and is Increased in Urine Exosomes of Metastatic Prostate Cancer Patients. J Extracell Vesicles (2013) 2:22079. doi: 10.3402/jev.v2i0.22097
206. Allard D, Chrobak P, Allard B, Messaoudi N, Stagg J. Targeting the CD73-Adenosine Axis in Immuno-Oncology. Immunol Lett (2019) 205:31–9. doi: 10.1016/j.imlet.2018.05.001
207. Ebos JM, Lee CR, Cruz-Munoz W, Bjarnason GA, Christensen JG, Kerbel RS. Accelerated Metastasis After Short-Term Treatment With a Potent Inhibitor of Tumor Angiogenesis. Cancer Cell (2009) 15(3):232–9. doi: 10.1016/j.ccr.2009.01.021
208. Gesierich S, Berezovskiy I, Ryschich E, Zöller M. Systemic Induction of the Angiogenesis Switch by the Tetraspanin D6.1A/CO-029. Cancer Res (2006) 66(14):7083–94. doi: 10.1158/0008-5472.CAN-06-0391
209. Chen C, Duckworth CA, Zhao Q, Pritchard DM, Rhodes JM, Yu LG. Increased Circulation of Galectin-3 in Cancer Induces Secretion of Metastasis-Promoting Cytokines From Blood Vascular Endothelium. Clin Cancer Res an Off J Am Assoc Cancer Res (2013) 19(7):1693–704. doi: 10.1158/1078-0432.CCR-12-2940
210. Zeng Q, Li S, Chepeha DB, Giordano TJ, Li J, Zhang H, et al. Crosstalk Between Tumor and Endothelial Cells Promotes Tumor Angiogenesis by MAPK Activation of Notch Signaling. Cancer Cell (2005) 8(1):13–23. doi: 10.1016/j.ccr.2005.06.004
211. Casimiro S, Guise TA, Chirgwin J. The Critical Role of the Bone Microenvironment in Cancer Metastases. Mol Cell Endocrinol (2009) 310(1-2):71–81. doi: 10.1016/j.mce.2009.07.004
212. Tauro BJ, Mathias RA, Greening DW, Gopal SK, Ji H, Kapp EA, et al. Oncogenic H-Ras Reprograms Madin-Darby Canine Kidney (MDCK) Cell-Derived Exosomal Proteins Following Epithelial-Mesenchymal Transition. Mol Cell Proteomics (2013) 12(8):2148–59. doi: 10.1074/mcp.M112.027086
213. Stuelten CH, Parent CA, Montell DJ. Cell Motility in Cancer Invasion and Metastasis: Insights From Simple Model Organisms. Nat Rev Cancer (2018) 18(5):296–312. doi: 10.1038/nrc.2018.15
214. Berditchevski F. Complexes of Tetraspanins With Integrins: More Than Meets the Eye. J Cell Sci (2001) 114(Pt 23):4143–51. doi: 10.1242/jcs.114.23.4143
215. Yánez-Mó M, Mittelbrunn M, Sánchez-Madrid F. Tetraspanins and Intercellular Interactions. Microcirc (New York N.Y 1994) (2001) 8(3):153–68. doi: 10.1111/j.1549-8719.2001.tb00166.x
216. Ang J, Lijovic M, Ashman LK, Kan K, Frauman AG. CD151 Protein Expression Predicts the Clinical Outcome of Low-Grade Primary Prostate Cancer Better Than Histologic Grading: A New Prognostic Indicator? Cancer Epidemiol Biomarkers Prev Publ Am Assoc Cancer Res Cosponsored by Am Soc Prev Oncol (2004) 13(11 Pt 1):1717–21.
217. Detchokul S, Williams ED, Parker MW, Frauman AG. Tetraspanins as Regulators of the Tumour Microenvironment: Implications for Metastasis and Therapeutic Strategies. Br J Pharmacol (2014) 171(24):5462–90. doi: 10.1111/bph.12260
218. Gesierich S, Paret C, Hildebrand D, Weitz J, Zgraggen K, Schmitz-Winnenthal FH, et al. Colocalization of the Tetraspanins, CO-029 and CD151, With Integrins in Human Pancreatic Adenocarcinoma: Impact on Cell Motility. Clin Cancer Res an Off J Am Assoc Cancer Res (2005) 11(8):2840–52. doi: 10.1158/1078-0432.CCR-04-1935
219. Ang J, Fang BL, Ashman LK, Frauman AG. The Migration and Invasion of Human Prostate Cancer Cell Lines Involves CD151 Expression. Oncol Rep (2010) 24(6):1593–7. doi: 10.3892/or_00001022
220. He X, Zheng Z, Li J, Ben Q, Liu J, Zhang J, et al. DJ-1 Promotes Invasion and Metastasis of Pancreatic Cancer Cells by Activating SRC/ERK/uPA. Carcinogenesis (2012) 33(3):555–62. doi: 10.1093/carcin/bgs002
221. Boscher C, Nabi IR. Galectin-3- and Phospho-Caveolin-1-Dependent Outside-in Integrin Signaling Mediates the EGF Motogenic Response in Mammary Cancer Cells. Mol Biol Cell (2013) 24(13):2134–45. doi: 10.1091/mbc.e13-02-0095
222. Espinosa-Sánchez A, Suárez-Martínez E, Sánchez-Díaz L, Carnero A. Therapeutic Targeting of Signaling Pathways Related to Cancer Stemness. Front Oncol (2020) 10:1533. doi: 10.3389/fonc.2020.01533
223. McCubrey JA, Abrams SL, Fitzgerald TL, Cocco L, Martelli AM, Montalto G, et al. Roles of Signaling Pathways in Drug Resistance, Cancer Initiating Cells and Cancer Progression and Metastasis. Adv Biol Regul (2015) 57:75–101. doi: 10.1016/j.jbior.2014.09.016
224. Saha T. LAMP2A Overexpression in Breast Tumors Promotes Cancer Cell Survival via Chaperone-Mediated Autophagy. Autophagy (2012) 8(11):1643–56. doi: 10.4161/auto.21654
225. Bao L, Lv L, Feng J, Chen Y, Wang X, Han S, et al. miR-487b-5p Regulates Temozolomide Resistance of Lung Cancer Cells Through LAMP2-Medicated Autophagy. DNA Cell Biol (2016) 35(8):385–92. doi: 10.1089/dna.2016.3259
226. Xu H, Hong FZ, Li S, Zhang P, Zhu L. Short Hairpin RNA-Mediated MDR1 Gene Silencing Increases Apoptosis of Human Ovarian Cancer Cell Line A2780/Taxol. Chin J Cancer Res (2012) 24(2):138–42. doi: 10.1007/s11670-012-0138-3
227. Stewart JJ, White JT, Yan X, Collins S, Drescher CW, Urban ND, et al. Proteins Associated With Cisplatin Resistance in Ovarian Cancer Cells Identified by Quantitative Proteomic Technology and Integrated With mRNA Expression Levels. Mol Cell Proteomics (2006) 5(3):433–43. doi: 10.1074/mcp.M500140-MCP200
Keywords: chemoresistance, exosomal proteins, prostate cancer, tumor markers, cancer treatment
Citation: Feng S, Lou K, Zou X, Zou J and Zhang G (2022) The Potential Role of Exosomal Proteins in Prostate Cancer. Front. Oncol. 12:873296. doi: 10.3389/fonc.2022.873296
Received: 10 February 2022; Accepted: 16 May 2022;
Published: 07 June 2022.
Edited by:
Alagarsamy Srinivasan, NanoBio Diagnostics, United StatesReviewed by:
Luciana N. S. Andrade, University of São Paulo, BrazilNagaraja Sethuraman Balakathiresan, National Institute on Alcohol Abuse and Alcoholism (NIH), United States
Copyright © 2022 Feng, Lou, Zou, Zou and Zhang. This is an open-access article distributed under the terms of the Creative Commons Attribution License (CC BY). The use, distribution or reproduction in other forums is permitted, provided the original author(s) and the copyright owner(s) are credited and that the original publication in this journal is cited, in accordance with accepted academic practice. No use, distribution or reproduction is permitted which does not comply with these terms.
*Correspondence: Junrong Zou, eWR6anJAZ211LmVkdS5jbg==; Guoxi Zhang, Z3lmeXVyb2xvZ3lAeWVhaC5uZXQ=
†These authors have contributed equally to this work and share first authorship