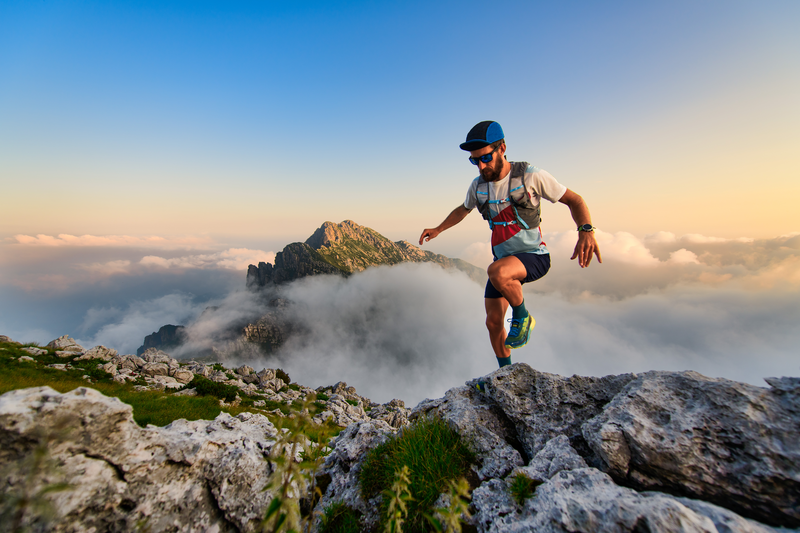
95% of researchers rate our articles as excellent or good
Learn more about the work of our research integrity team to safeguard the quality of each article we publish.
Find out more
ORIGINAL RESEARCH article
Front. Nutr. , 14 January 2025
Sec. Food Chemistry
Volume 11 - 2024 | https://doi.org/10.3389/fnut.2024.1531185
Tea flower, with characteristic flavor formed during blooming, are a significant tea resource. However, studies on the volatile compounds of tea flower and their aroma characteristics during flowering are scarce. In this study, the odor characteristics of tea flower during blooming were comprehensively investigated by GC–MS, PCA, ACI determination and sensory evaluation. The tea flower of unopened buds (TF-S1) contained the highest alcohol amounts, while fully opened tea flowers (TF-S3) had the highest heterocyclic compounds. Half-opened tea flowers (TF-S2) had the most volatile compounds, including high levels of linalool and its oxides, and low levels of (Z)-3-hexen-1-ol. Acetoin and cosmene were first identified in TF-S1 and TF-S2, respectively. The major ACI components differed, with linalool being prominent exhibiting ACI above 27 in all samples. Acetophenone, unique to TF-S2 with ACI of 57.35, contributed to sweet odor. Furthermore, PCA analysis and sensory evaluation revealed distinct aroma characteristics among the samples. Overall, TF-S2 and TF-S3 had higher volatile amounts and better aroma properties with floral, powdery or almond-like odors. These results advance the understanding of aroma properties of tea flower during blooming, and provide a reference for resource utilization and promotion of the application in food or cosmetics industries.
The tea flower, derived from Camellia sinensis (L.) O. Kuntze, is a reproductive organ of the tea plant and constitutes an edible portion of the tea bush. It has increasingly become the second tea resource after tea leaves, which can be used to make scented teas and beverages (1). Studies have shown that tea flowers have similar bioactive compounds to those found in tea leaves, which contains polyphenols, caffeine, polysaccharides, amino acids, 2-ketobutyric acid, phenolamides (2–4). These compounds contribute to various health-promoting benefits, including antioxidant, anti-cancer, anti-inflammatory, anti-cholesterol, antifungal, hypoglycemic, hypotensive, immunomodulatory, improvement of dermal hyperpigmentation and maintaining intestinal health (5–14). Regarding the excellent biological activities, tea flowers have been paid more attentions in health products, and hold potential for application in the development of functional foods and medicines over recent years (15, 16). On the other hand, tea flower is renowned for its aroma properties, characterized by pleasant floral, fragrance, fresh, fruity and sweet odors (17), and is rich in active ingredients, such as tea polyphenols and amino acids, making it an excellent candidate for beverage production (18), particularly enhancing the fruitiness of kombucha (19). Additionally, research by Khat-Udomkiri et al. (20) highlights the potential of tea flower extract in cosmetic and pharmaceutical applications, as it effectively protects fibroblast cells from oxidative damage. To date, the majority of research has concentrated on the health benefits in vitro and the analysis of non-volatile secondary metabolites of tea flowers (21, 22), while comparatively fewer studies have investigated their volatile compounds and aroma characteristics.
Aroma is a crucial quality aspect of flowers or products produced thereform, affecting their sensory characteristics and economic value (23). Flowers exhibit different aroma profiles due to the presence of diverse volatile compounds, which find applications in the food flavoring and cosmetic industries (24). Furthermore, the quality and market value of tea flower related products are significantly influenced by the presence of volatile compounds. The aroma profile of tea flower is a critical factor in determining its market appeal and consumer acceptance (25). A pleasant and natural aroma can enhance the user experience and contribute to the perceived efficacy of the product, thereby increasing its market value. Notably, certain volatile compounds may also possess antimicrobial or anti-inflammatory effects, showing additional skin benefits, which can further boost the market value of tea flowers (26).
The volatile compounds in flowers are mainly formed during blooming (50), and those formed at different flowering stages possess distinct aroma characteristics. However, the volatile compounds in tea flowers, either in volatile compositions, their variations or aroma profiles at different blooming stages, have been rarely studied and reported so far. It would greatly affect the comprehensive development and utilization of tea flowers. Notably, tea flowers have been assigned as a new food resource in China, where they are abundant, with an annual output exceeding 1.8 billion kilograms (14). Given their potential as a food source and the lack of research on their aroma characteristics, it is crucial to analyze the volatile compounds and aroma profiles of tea flowers at different flowering stages. This research will not only contribute to a better understanding of tea flower aroma development but also support the comprehensive development and utilization of this abundant resource.
Headspace (HS) extraction is a convenient and straightforward method to extract aroma compounds and does not produce artifacts during operation. This method is commonly employed for extracting and enriching volatile compounds, including those found in flowers (27). Thereafter, the gas chromatography–mass spectrometry (GC–MS) is applied for the separation and analysis of volatile compounds in complex matrix (28, 29). In the current study, the aroma compounds in tea flowers were extracted using HS and analyzed by GC–MS to reveal their aroma compositions and odor profiles at different blooming stages, aiming to provide scientific foundation for the development and resource utilization of tea flowers, ultimately enhancing the overall quality of related products.
The fresh tea flowers (Camellia sinensis cv. Population) of three different flowering stages, which were of flower buds (stage I, unopened buds, TF-S1), half opened tea flowers (stage II, including the ones that petal started to split, TF-S2) and fully opened tea flowers (stage III, TF-S3). The detailed descriptions and states of tea flowers from three flowering stages were provided in Supplementary material and illustrated in Figure 1. These tea flower samples were collected at Guangde City (Anhui province, China) in October 2019, then immediately deep frozen in liquid nitrogen and stored in a −80°C ultra-low freezer before processing. The tea flower samples were freeze-dried using freeze dryer (ALPHA 1–4 LD plus, Martin Christ Freeze Dryer, Germany) and powdered to pass through a 350 μm sieve. All authentic standards were obtained from Sigma Aldrich (Shanghai, China) unless specified otherwise. The detail information of reference compounds and their suppliers are shown in Supplementary Table S1. A mixture of C5-C28 n-alkanes were purchased from Supelco, United States.
Figure 1. The identified volatile compounds in TF-S1 (A), TF-S2 (B) and TF-S3 (C). The bubble diagram of volatile compounds, where circles of identical colors signify compounds belonging to the same volatile category, and the size of each circle corresponds to their relative amount.
Volatile compounds in tea flowers were extracted by HS using a method similar to that reported previously (30) with some modification. Three grams of dried tea flowers were directly placed into a 20 mL headspace vial, and covered by a headspace auto-loading crimp cap with septa. Volatile compounds were analyzed on a GC (7890A, Agilent, Santa Clara, CA, United States) coupled to a headspace injector (Agilent 7697A, Agilent, United States) with splitless mode. The oven temperature, manifold temperature, and transfer-line temperature were set at 120, 140, and 150°C, respectively. The incubation and injection time were 20 min and 60 s, respectively. The temperature program was initially set at 50°C, held for 5 min, increased to 210°C at a rate of 3°C min−1 and retained 3 min, raised to 230°C at 10°C min−1 and subsequent maintained at 230°C for 2 min, finally increased to 280°C at a rate of 10°C min−1 and held for another 10 min. Injection volume was 1 μL.
The GC–MS analytical procedure for the analyzing volatile compounds in tea flowers, as described by Guo et al. (31) were followed, with minor modification. Separation was achieved by a fused-silica capillary column (DB-5, 30 m × 0.25 mm, 0.25 μm film thickness, J&W Scientific, Folsom, CA, United States). Helium gas (1 mL/min) was used as a mobile phase. The mass spectrometer was operated in electron ionization mode (positive ion, 70 eV). Ion source and transfer-line temperatures were 250 and 150°C, respectively. The detection electric pressure was 1905 V, the mass spectra were acquired in full scan mode from 30 to 500 amu. Retention indices (RI) were calculated from the retention time of n-alkanes by linear interpolation. The peak was deconvoluted and the compounds were identified by comparing the mass spectral data with those of the NIST virtual library (NIST 2017 version), references and in-house database (30–33). Components were tentatively identified by agreement of their retention times, retention index and mass spectra with published data and, if available, positively identified with those of authentic compounds. The amounts of the identified volatile compounds were expressed as the average of the peak area of three replicates.
Aroma character impact (ACI), which was the percentage of the ratio of the concentration of a volatile compound to its odor threshold value, was applied to compare aroma contributions of the identified volatile compounds in a mixture (34), which was determined by the Equation 1:
where Ci is the concentration of the volatile compound and Ti is its corresponding odor threshold value in air; Rai is the relative amount (peak area) of the volatile compound in tea flowers during flowering.
The method of sensory analysis on tea flower aroma was from Guo et al. (33) with modification. The tea flower samples from different stages of flowering were subjected to a sensory test. Three-digit numbers were used to code samples, and they were randomly offered to panelists, the intensity values and aroma descriptors of samples were recorded by evaluators. The aroma attributes, including floral, powdery, green, citrus, waxy, fragrance, and almond-like odor were recorded by panelists. As for the TF-S2, the green odor was noted following by the floral odor, which was a little different from the green flavor of TF-S1. The intensities of the aroma attributes were scored using a scale from 0 to 10, the higher the score, the stronger the intensity, where 0 = none or not perceptible intensity, 3 = weak intensity, 5 = moderate intensity, 7 = high intensity, and 10 = extremely high intensity. Each sample was evaluated three times by each panelist on different days. Data were expressed as mean values.
The tea flower samples were evaluated by a well-trained panel of 10 members (four males and six females, age from 27 ~ 45). All assessors had more than 5 years of experience in the descriptive sensory analysis of natural plant and flower related products. Panelists were trained by a series of important volatile compounds, including (E)-2-pentenal for its fruity odor, (Z)-3-hexenal and hexanal for their green and grassy scents, (E,E)-2,4-nonadienal for its fatty odor, phenethyl alcohol, linalool and its oxides for their floral fragrances, phenylacetaldehyde for its honey-like odor, α-terpineol for its woody odor, maltol for its sweet odor, as well as the flavor of actual products such as almonds.
All experiments were replicated 3 times. Statistical analysis was performed with one-way analysis of variance (ANOVA) and Duncan’s multiple-range tests by use of SPSS Statistics 22.0 (SPSS Inc., Chicago, IL, United States). All comparisons were considered statistically significant if p-value <0.05. In addition, the circular stacking diagrams were generated using Chiplot.1
Totally 63 volatile compounds were identified in tea flowers with different flowering stages of TF-S1 (38), TF-S2 (49), and TF-S3 (35) (Table 1). The identified components belonged to various chemical groups, including alcohols, aldehydes, alkenes, ketones, esters, heterocyclics, hydrocarbons and aromatics, as shown in Figure 1. The proportions and numbers of these volatile categories were different among the three samples (Figures 2A,B). Notably, alcohols, aldehydes and heterocyclics were the most abundant compounds, accounting for more than 18% of the total volatiles amount in each sample. With the advancement of flower growth, the proportions of alcohols and esters were diminished, whereas the heterocyclic compounds were enhanced. The peak proportion of heterocyclic compounds was observed in TF-S3, reaching to 41.06%, which was 1.87 and 1.38 times of that in TF-S1 and TF-S2, respectively. In addition, twenty-two volatile compounds were commonly found in all three samples, accounting for 34.92% of the total identified compounds. However, 7, 17, and 2 volatiles were uniquely identified in TF-S1, TF-S2, and TF-S3 samples, respectively (Figures 2C–E).
Table 1. The identified volatile compounds and their odor description of tea flowers during blooming.
Figure 2. The volatile profiling of tea flowers during flowering. The number (A) and proportion (B) of volatile compounds in tea flowers. The volatile compounds uniquely identified in TF-S1 (C), TF-S2 (D) and TF-S3 (E). Compound nos. Correspond to Table 1.
A total of 41 volatiles were detected in TF-S1, with 38 volatile compounds successfully identified (Table 1). The TF-S1 samples tended to contain the highest proportion of alcohols (37.13%), among which phenylethyl alcohol, linalool and 2-heptanol were the three most abundant compounds, accounting for 77.81% of the total alcohols detected. Phenylethyl alcohol imparting floral, rose-like odors (35), is the product of glycoside hydrolysis in tea (36, 37). 2-Heptanol having herbal flavor is derived from lipid degradation, with the unsaturated fatty acids such as palmitoleic acid and oleic acid as precursors (36). Linalool, a contributor for floral odor in teas, is recognized as the key aroma compound in all tea samples (38, 39). In addition, benzyl alcohol, linalool oxide I and linalool oxide II, which contribute to floral odors (36), were identified in TF-S1 but were of low abundance. Among the seven aldehydes identified in TF-S1, 2-hexenal and hexanal exhibiting green or grassy odors (38, 39), were the major volatile compounds. Particularly, the amount of 2-hexenal was significantly higher than the other aldehydes, nearly twice as much as hexanal. In addition to the two components and (E)-2-hexenal, the other four aldehydes, namely benzaldehyde, octanal, benzeneacetaldehyde and nonanal have floral or fruity odors (36), which are the important contributors to tea aroma. The two most abundant heterocyclic compounds were pyranone and furfural, comprising 81.78% of the total amounts of heterocyclic compounds. The former was detected in tea related products for the first time, while the latter was commonly identified in baked teas as a contributor of almond-like and caramel-like odors (30, 40, 41). Most of the identified esters had green or fatty flavor, in which (E)-3-hexen-1-ol acetate and methyl salicylate were the major compounds with high amounts. Methyl salicylate is considered as an important aroma compound for tea quality, and is mainly liberated by hydrolyzing glycosides in tea (38, 39, 42). Three ketones, including acetoin, were identified in TF-S1. Acetoin with pleasant milky odor (43) is rarely detected in previous tea samples. In addition, only one chemical compound with a relatively low amount, was identified in alkenes, hydrocarbons, and aromatics.
Totally 35 volatiles were identified in TF-S3 using GC–MS technology (Table 1). In contrast to TF-S1, these identified components belonged to seven chemical groups, with no ketones detected in the sample. The volatile compositions, amounts, and proportions of volatile categories were different from those in TF-S1. Similarly, alcohols, aldehydes and heterocyclic compounds were the most abundant, accounting for 86.91% of the total identified volatiles, while alkenes were the least abundant (0.15%). Limonene was the sole alkene compound identified, and its amount did not significantly differ from that in TF-S1. In comparison to TF-S1, five heterocyclic compounds including 2-furanmethanol, 2-acetylfuran, 2,5-dimethylpyrazine, 5-methyl-2-furanmethanol and 1-ethyl-1H-pyrrole-2-carboxaldehyde were newly identified. The former two compounds have been found in large-leaf yellow tea under high intensity of roasting treatment (30), and the latter one exhibiting roasted or smoky notes (41) is generated in the final product Shuixian oolong tea after full fire processing (32). The formation of 5-methyl-2-furanmethanol is in relation to Maillard reaction from D-glucose and L-theanine (32), and 2,5-dimethylpyrazine, which imparts characteristic roasted peanutty flavor, is considered as the key aroma compound in tea (32). In terms of the common volatiles in TF-S1 and TF-S3, heterocyclic compounds were still dominated by pyranone, accounting for 69.05% of the total amounts of this group in TF-S3. Additionally, the amounts of these common volatile compounds, except for 2-acetylpyrrole, raised significantly as the tea flower progressed from stage I to stage III. A total of 11 alcohols were identified in the sample, of which 2-(vinyloxy)ethanol and maltol absent in TF-S1. Maltol having caramel-like odor, which is an important flavor ingredient detected in Japanese soy sauce (44), was not found in previous tea samples. Meanwhile, trace amounts of alcohols in TF-S1, such as 6-methyl-5-hepten-2-ol, hotrienol and geraniol, which are identified in Wuyi rock tea (31), were not detected in TF-S3. Although phenylethyl alcohol, linalool and 2-heptanol remained the three most abundant alcohols in TF-S3, the amounts of the former two volatiles dropped compared to TF-S1. With the flowers development (from stage I to stage III), the amounts of aldehydes and esters with green flavor were decreased, whereas the aldehydes with floral odors or the esters with fatty notes were enhanced. Octanal, (E)-2-hexenal and 3(Z)-hexenyl butanoate were not found in TF-S3.
In total, 52 volatile compounds were detected in TF-S2, with 49 volatiles being identified (Table 1). Among these, alcohols (21.26%), aldehydes (27.58%) and heterocyclic compounds (29.85%) comprised 78.69% of the total amount. The number and amounts of identified volatiles in TF-S2 were much higher than those of TF-S1 and TF-S3. Moreover, the proportions or amounts of alkenes, ketones and hydrocarbons were enhanced significantly. The amount of limonene, a representative component common to all three samples, was almost three times higher in TF-S2 compared to the other two samples. Cosmene, an unsaturated alkene commonly found in herbal essential oils (45) and a bound-form volatile compound of Rubus corchorifolius fruit (46), was detected in tea flowers firstly. Different from TF-S1, three new ketones were identified in TF-S2, with 6-methyl-5-hepten-2-one being the least amount. This compound is identified in oolong tea as a carotenoid-derived aroma compound (36, 47). Acetophenone imparts sweet, or citrus flavor, which is derived from L-phenylalanine in tea flowers (48), was also found in tea leaves (49). It was identified in high abundance only in TF-S2, differing slightly from the results of Joshi et al. (50) due to variations in location, variety, sample status, and aroma extraction methods of the tea flowers. Although the largest number of esters were identified in TF-S2, their proportions significantly decreased from stage I to stage II. Among them, (E)-3-hexen-1-ol acetate and methyl salicylate remained the two compounds with the highest amounts. Similar to esters, the proportions of alcohols first decreased and then increased with the advancement of flower growth, with the lowest observed in TF-S2. However, the amounts of alcohols imparting floral flavor, such as linalool, linalool oxide I and linalool oxide II, increased significantly. These are aroma-active compounds in tea, with linalool being a vital contributor of the floral odor in tea (36), and the most abundant alcohols in TF-S2. In practice, linalool has a floral odor with green flavor, which might be the main source of floral and green notes in the sensory evaluation of TF-S2. Four aldehydes, namely 2-methyl-2-pentenal, heptanal, methional and decanal were only identified in TF-S2. Heptanal and decanal are produced by lipid oxidation in tea (36), and contribute to the fruity or floral flavor of tea flowers (36, 51). Methional, with a low threshold value (0.06 ppb in air), is usually recognized as the precursor of methanethiol (52). Among the common aldehydes, the amounts of hexanal, 2-hexenal, benzaldehyde, and nonanal were significantly higher in TF-S2 than those of the other two samples, while benzeneacetaldehyde presented an opposite pattern.
Eleven heterocyclics were identified in TF-S2, among which furfural, 2-phetylfuran and 2-acetylpyrrole were the common heterocyclics in three tea flower samples. Furfural and 2-phetylfuran were most abundant in TF-S2, whereas TF-S2 had the least amount of 2-acetylpyrrole. In comparison to TF-S3, TF-S2 had the significantly lower amounts of 2,5-dimethylpyrazine but much higher amounts of 2-acetylfruan, 5-methyl-2-furanmethanol and 2-furanmethanol. 5-Methyl-2-furancarboxaldehyde, which has been found in large-leaf yellow tea and oolong tea after high intensity of roasting treatment (30, 32) together with 3(2H)-pyridazinone were solely identified in TF-S2. In addition, hexanoic acid with acrid odor (53) was identified in all samples. The amounts of hexanoic acid gradually increased with the development of blooming, while their proportion first declined and then raised during flowering, being lowest in TF-S2 (1.51%) and highest in TF-S3 (2.67%).
As the flowering stage progressed, TS-S2 possessed a significantly greater number and amounts of volatiles, most of which had floral and fruity odors as well as the pleasant flavor. Their amounts had a significant increasement compared with the other two tea flower samples. Meanwhile, some volatile compounds with green or fatty odors decreased or volatilized during flowering. These findings were in line with those of Joshi et al. (50), who observed that half-opened tea flowers had the most volatiles, and the highest levels of linalool, linalool oxide I & II, benzaldehyde, and acetophenone, which was only identified in TF-S2.
To further understand intuitively the aroma characteristics of tea flowers and distinguish the differences among tea flowers from various flowering stages, the PCA was conducted on the identified volatiles (Figure 3). As can be seen in Figure 3A, the accumulated contribution rate of the first two principal components (PC 1 for 71.6%, PC 2 for 25.4%) was 97.0%, which appeared to represent the sufficient information of tea flower samples. Notably, the three samples were well separated according to the flowering stages. Specifically, TF-S1 exhibited high scores on the negative PC 1 and positive PC 2, where the loadings of characteristic volatile compounds including hotrienol, geraniol, 2-nonanone, 6-methyl-5-hepten-2-ol, acetoin, 2-(1,3-butadienyl)-1,3,5-trimethylbenzene were prominent. These compounds, along with phenylethyl alcohol and benzeneacetaldehyde, were uniquely identified in the initial stages of florescence of tea flower. This suggests that the different aroma properties of TF-S1 compared to TF-S2 or TF-S3 might be attributed to its uniquely volatile compounds. On the other hand, TF-S3 showed high scores on both the negative PC 1 and negative PC 2, containing high loadings of compounds, such as 2,5-dimethylpyrazine, 1,3-dimethylbenzene, butyl hexadecanoate, 2-acetylpyroole, tricosane, pyranone. Among these, pyranone was the most abundant aroma compound in TF-S3 of the late stages of tea flowering (Figure 3B). In addition, the levels of benzeneacetaldehyde, 2-heptanol, N-acetyl-4(H)-pyridine, and 2,4-dihydroxy-2,5-dimethyl-3(2H)-furan-3-one were similar in TF-S1 and TF-S3, suggesting they were co-characteristic aroma compounds in both samples. TF-S2 scored high on the positive PC 1, which contained high loadings of benzyl alcohol, (E)-3-hexen-1-ol acetate, 3(Z)-hexenyl butanoate, linalool oxide II, linalool, linalool oxide I, benzaldehyde, furfural, 2-acetylfuran, 5-methyl-2-furanmethanol, 2-pentylfuran, 2-furanmethanol, maltol. These characteristic volatiles with floral, fruity, almond-like odors, along with heterocyclic compounds, were of most abundance in the half-opened tea flowers. The PCA results indicated that the fully and half-opened tea flowers possessed large amounts of volatiles with floral or fruity odors and heterocyclic compounds, whereas volatile compounds imparting green or fatty odors were abundant in the initial stages of tea flower blossoming.
Figure 3. PCA analysis of tea flowers in different flowering stages. The score plot (A) and biplot (B) of PCA analysis. Compound nos. Correspond to Table 1.
However, it is important to note that the aroma impact of a component depends on its concentration and odor threshold value. Therefore, a comprehensive analysis of the aroma contributions of volatile compounds in tea flowers is necessary, and the actual aroma profile of tea flowers should be assessed from a sensorial point of view.
The contribution of an individual component to the overall aroma of tea flowers is determined by its aroma character impact (ACI). The ACI values of aroma compounds in tea flowers were calculated based on the threshold data from reported references, and the ACI of identified volatiles is presented in Supplementary Table S2. Totally, 8 volatile compounds had ACI values higher than one in three samples, of which 7, 5, and 6 volatiles with ACI greater than one were identified in TF-S1, TF-S2, and TF-S3, respectively (Table 2). Generally, these aroma character impact molecules mainly imparted floral, sweet or green notes, contributing 97.49, 97.78, and 97.68% to the aroma of TF-S1, TF-S2, and TF-S3, respectively (Table 2).
Among the identified volatiles, linalool was the only volatile compound with an ACI above 27 in all samples. It also made the most significant contribution to the aroma character in TF-S1 and TF-S3, indicating that it had the positive influence on floral or green notes in tea flower samples, consistent with the findings from Gao et al. (54) that linalool has been reported to be the potential key aroma compound in tea flowers from albino cultivars with the highest relative abundance. In contrast, for TF-S2, acetophenone imparting sweet odor was the most prominent aroma contributor (ACI = 57.35). Nonanal, which contributes floral or green odors, had the third highest ACI value of 8.30, whereas the remaining compounds played a much less important role in the aroma contribution. In comparison to TF-S2, acetophenone was not found in TF-S1, where phenylethyl alcohol (ACI = 17.35), hexanoic acid (ACI = 7.58), (E)-2-hexenal (ACI = 1.16), and methyl salicylate (ACI = 3.83) had significantly higher ACI values. Similarly to TF-S1, the volatiles phenylethyl alcohol (ACI = 15.50), hexanoic acid (ACI = 12.60), nonanal (ACI = 7.90), and methyl salicylate (ACI = 1.60) were notable contributors for TF-S3. However, (E)-2-hexenal with green flavor was not observed in TF-S3. Notably, Cui et al. (55) discovered that acetophenone is the most abundant volatile compound in tea flower at the fully-opened stage. The significant different might be due to the distinct tea cultivar utilized and the different aroma extraction techniques employed.
The radar profile illustrating the sensory aroma attributes of tea flowers during flowering stage is shown in Figure 4. The detailed scores on intensity of aroma attributes were shown in Supplementary Table S3. Only moderate green and weak fragrance odors were recorded in TF-S1, while TF-S3 had extremely high intensity of powdery odor, coupled with strong floral and waxy odors, as well as moderate citrus and fragrance notes. Compared to TF-S3, TF-S2 possessed higher intensity of floral odor and lower intensity of powdery odor. Notably, TF-S2 also featured a special characteristic of almond-like odor with high intensity, potentially attributed to the abundant presence of furfural, which imparts such an aroma. As for the green flavor in TF-S2, it differed from that of noted in TF-S1, accompanied with the floral odor, possibly originating from linalool. However, further research is required to ascertain the exact contribution of linalool to the floral and green notes of half-opened tea flowers. The green flavor was not noted in TF-F3 might be associated with the absence of (E)-2-hexenal, which has been considered a positive contributor to green odor in tea flower (54). Practical sensory evaluation results revealed that half-opened and fully-opened tea flowers had different aroma characteristics, the latter exhibited powdery odor, whereas, the former was dominated by floral note, both with extremely high intensities. Linalool and phenylethyl alcohol might be the potential contributors of powdery odor in TF-S3, while acetophenone and linalool contributed to the floral note in TF-S2. The unique aroma characteristics of tea flowers at different stages of flowering could be harnessed to create distinctive and appealing products. The TF-S2 had strong floral and almond-like odors, with sensory scores of 7.84 and 6.77, respectively, making it an ideal choice for use in perfumes and cosmetics to impart a fresh and inviting scent. Similarly, the powdery odor of TF-S3, with a sensory score of 7.39, could add a unique and intriguing note to beverages and other products. Further research is encouraged to explore the full potential of tea flowers in these and other applications.
In the present study, the volatile compositions and aroma profiles of tea flowers at different developmental stages were analyzed using HS-GC–MS technology, complemented by ACI determination and sensory evaluation. The volatiles in tea flowers were dominated by alcohols, aldehydes and heterocyclic compounds, with variations across stages. TF-S2 tended to contain higher proportions of aldehydes, ketones, hydrocarbons, and alkenes, but lower alcohols and esters levels compared to flower buds and fully opened tea flowers. Eight volatiles, including linalool (ACI >27 in all samples) and acetophenone (unique to TF-S2), were the key aroma compounds. Half-opened tea flowers exhibited strong floral odor and the characteristic almond-like flavor, while fully opened tea flowers had strong powdery and moderate floral odors. Overall, half-opened or fully-opened tea flowers had higher amounts of total volatiles and superior aroma characteristics. These findings provide the theoretical basis for the understanding the aroma profiles and application performance of tea flowers, suggesting further research into their physicochemical composition, bioactivity, and the practical application in functional foods, beverages, and food flavor industries.
We would like to dedicate this article with profound affection to the memory of first author’s mother, who passed away at the end of September 2024. She provided many valuable opinions and suggestions for the shaping of this article, and it is due to her constant encouragement and support that the author has been able to persist in the scientific research work.
The original contributions presented in the study are included in the article/Supplementary material, further inquiries can be directed to the corresponding authors.
XG: Conceptualization, Data curation, Formal analysis, Funding acquisition, Investigation, Methodology, Project administration, Resources, Software, Supervision, Writing – original draft, Writing – review & editing. LW: Formal analysis, Investigation, Writing – review & editing. XH: Formal analysis, Writing – review & editing. QZ: Funding acquisition, Writing – review & editing.
The author(s) declare that financial support was received for the research, authorship, and/or publication of this article. This work was supported by the Key Scientific Research Project of Higher Education Institutions in Henan Province (24B210015).
The authors declare that the research was conducted in the absence of any commercial or financial relationships that could be construed as a potential conflict of interest.
All claims expressed in this article are solely those of the authors and do not necessarily represent those of their affiliated organizations, or those of the publisher, the editors and the reviewers. Any product that may be evaluated in this article, or claim that may be made by its manufacturer, is not guaranteed or endorsed by the publisher.
The Supplementary material for this article can be found online at: https://www.frontiersin.org/articles/10.3389/fnut.2024.1531185/full#supplementary-material
1. Shi, L, Gu, Y, Wu, D, Wu, X, Grierson, D, Tu, Y, et al. Hot air drying of tea flowers: effect of experimental temperatures on drying kinetics, bioactive compounds and quality attributes. Int J Food Sci Technol. (2018) 54:526–35. doi: 10.1111/ijfs.13967
2. Cai, Y, Liu, S, Ge, X, Cheng, L, and Zhang, X. Inhibitory effect of tea flower polysaccharides on oxidative stress and microglial oxidative damage in aging mice by regulating gut microbiota. Food Funct. (2024) 15:11444–57. doi: 10.1039/d4fo03484h
3. Chen, D, Wang, A, Lv, J, Tang, C, Jin, C, Liu, J, et al. Structural and digestive characters of a heteropolysaccharide fraction from tea (Camellia sinensis L.) flower. Food Chem. (2024) 21:101058. doi: 10.1016/j.fochx.2023.101058
4. Liu, H, Liu, Y, Han, H, Lu, C, Chen, H, and Chai, Y. Identification and characterization of phenolamides in tea (Camellia sinensis) flowers using ultra-high-performance liquid chromatography/Q-Exactive orbitrap mass spectrometry. Food Chem. (2023) 424:136402. doi: 10.1016/j.foodchem.2023.136402
5. Chen, D, Chen, G, Chen, C, Zeng, X, and Ye, H. Prebiotics effects in vitro of polysaccharides from tea flowers on gut microbiota of healthy persons and patients with inflammatory bowel disease. Int J Biol Macromol. (2020) 158:968–76. doi: 10.1016/j.ijbiomac.2020.04.248
6. Chen, D, Chen, G, Ding, Y, Wan, P, Peng, Y, Chen, C, et al. Polysaccharides from the flowers of tea (Camellia sinensis L.) modulate gut health and ameliorate cyclophosphamide-induced immunosuppression. J Funct Foods. (2019) 61:103470. doi: 10.1016/j.jff.2019.103470
7. Chen, D, Chen, G, Sun, Y, Zeng, X, and Ye, H. Physiological genetics, chemical composition, health benefits and toxicology of tea (Camellia sinensis L.) flower: A review. Food Res Int. (2020) 137:109584. doi: 10.1016/j.foodres.2020.109584
8. Chen, D, Ding, Y, Ye, H, Sun, Y, and Zeng, X. Effect of long-term consumption of tea (Camellia sinensis L.) flower polysaccharides on maintaining intestinal health in BALA/c mice. J Food Sci. (2020) 85:1948–55. doi: 10.1111/1750-3841.15155
9. Chen, D, Wang, A, Lv, J, Peng, Y, Zheng, Y, Zuo, J, et al. Tea (Camellia sinensis L.) flower polysaccharide attenuates metabolic syndrome in high-fat diet induced mice in association with modulation of gut microbiota. Int J Biol Macromol. (2024) 279:135340. doi: 10.1016/j.ijbiomac.2024.135340
10. Chen, F, Chen, YP, Wu, H, Li, Y, Zhang, S, Ke, J, et al. Characterization of tea (Camellia sinensis L.) flower extract and insights into its antifungal susceptibilities of aspergillus flavus. BMC Compl Med Ther. (2023) 23:286. doi: 10.1186/S12906-023-04122-5
11. Dissanayake, CY, Moon, HH, Yang, KM, Lee, Y, and Han, CH. The effects of green tea (Camellia sinensis) flower extract on melanin synthesis in B16-F10 melanoma cells. Korean J Vet Res. (2018) 58:65–72. doi: 10.14405/kjvr.2018.58.2.65
12. Gao, Y, Han, Z, Xu, Y, and Yin, J. Chemical composition and anti-cholesterol activity of tea (Camellia sinensis) flowers from albino cultivars. Front Nutr. (2023) 10:1142971. doi: 10.3389/FNUT.2023.1142971
13. Ren, N, Chen, L, Li, B, Rankin, GO, Chen, YC, and Tu, Y. Purified tea (Camellia sinensis (L.) Kuntze) flower saponins induce the p53-dependent intrinsic apoptosis of cisplatin-resistant ovarian cancer cells. Int J Mol Sci. (2020) 21:4324. doi: 10.3390/ijms21124324
14. Xia, C, Tu, Y, Yang, Z, Jin, Y, and Xia, H. Antioxidative activity of essential oil of flowers of tea (Camellia sinensis L.) plants extractd by supercritical carbon dioxide. J Biomed Sci. (2018) 7:10–8. doi: 10.4172/2254-609X.100089
15. Chen, D, Chen, G, Wan, P, Hu, B, Chen, L, Ou, S, et al. Digestion under saliva, simulated gastric and small intestinal conditions and fermentation in vitro induced by human gut microbiota of polysaccharides form the flowers of Camellia sinensis. Food Funct. (2017) 8:4619–29. doi: 10.1039/c7fo01024A
16. Chen, D, Ding, Y, Chen, G, Sun, Y, Zeng, X, and Ye, H. Components identifidation and nutritional value exploration of tea (Camellia sinensis L.) flower extract: evidence for functional food. Food Res Int. (2020) 132:109100. doi: 10.1016/j.foodres.2020.109100
17. Shi, L, Kim, E, Yang, L, Huang, Y, Ren, N, Li, B, et al. Effect of a combined microwave-assisted drying and air drying on improving active nutraceutical compounds, flavor quality, and antioxidant properties of Camellia sinensis L. (cv. Longjing 43) flowers. Food Qual Saf. (2021) 5:1–7. doi: 10.1093/fqsafe/fyaa040
18. Wu, Z, Li, X, Xu, X, Xing, A, Xu, Y, Yang, X, et al. Quality components identification and formation analysis of tea (Camellia sinensis L.) flower beverages from three cultivars. LWT. (2023) 181:114739. doi: 10.1016/j.lwt.2023.114739
19. Huang, Y-B, Zou, C, Gao, Y, Yin, J-F, Contursi, P, Zhang, S, et al. Kombucha beverages made from Camellia nitidissima chi and Camellia sinensis flowers – physicochemical properties, sensory properties and bioactivity. Int J Gastron Food Sci. (2024) 37:100964. doi: 10.1016/J.IJGFS.2024.100964
20. Khat-Udomkiri, N, Gatnawa, G, Boonlerd, N, and Myo, H. Valorization of Camellia sinensis flowers in cosmetic and pharmaceutical applications: optimization of microwave-assisted glycerin extraction. Waste Biom Valor. (2024) 15:323–35. doi: 10.1007/S12649-023-02148-X
21. Ye, H, Hu, J, Peng, S, Zong, W, Zhang, S, Tong, L, et al. Determination of the chemical compounds of Shuchazao tea flowers at different developmental stages and in young shoots using 1H NMR-based metabolomics. Monat Chem. (2022) 153:409–17. doi: 10.1007/S00706-022-02928-6
22. Zhang, T, Ma, X, Zhou, Y, Yang, H, Wang, Y, Chen, T, et al. Metabolite profiling of external and internal petals in three different colors of tea flowers (Camellia sinensis) using widely targeted metabolomics. Meta. (2023) 13:784. doi: 10.3390/metabo13070784
23. Wang, T, Xie, A, Zhang, D, Liu, Z, Li, X, Li, Y, et al. Analysis of the volatile components in flowers of Paeonia lactiflora pall. And Paeonia lactiflora pall. Var Trichocarpa. Am J Plant Sci. (2021) 12:146–62. doi: 10.4236/ajps.2021.121009
24. Schwab, W, Davidovich-Rikanati, R, and Lewinsohn, E. Biosynthesis of platn-derived flavor compounds. Plant J. (2008) 54:712–32. doi: 10.1111/j.1365-313X.2008.03446.x
25. Joshi, R, Poonam,, Saini, R, Guleria, S, Babu, GDK, Kumari, M, et al. Characterization of volatile components of tea flowers (Camellia sinensis) growing in Kangra by GC/MS. Nat Prod Commun. (2011) 6:1155–8. doi: 10.1017/S0022029911000471
26. Tran, GB, Pham, TV, Le, AT, Nguyen, NH, Vo, NHH, and Do, BH. Chemical composition and the anti-inflammatory effect of volatile compounds from Anaxagorea luzonensis A. Gray Z Naturfors C. (2024) 79:329–36. doi: 10.1515/znc-2023-0177
27. Rowshan, V, and Najafian, S. Headspace analyses of leaf and flower of Citrus limetta (lemon), Citrus maxima (pomelo), Citrus sinensis (Orange), and Citrus medica (Cedrum) for volatile compounds by combiPAL system technique. J Herbs Spices Med Plants. (2013) 19:418–25. doi: 10.1080/10496475.2013.818605
28. Norani, M, Ebadi, MT, and Ayyari, M. Volatile constituents and antioxidant capacity of seven Tussilago farfara L. populations in Iran. Sci Hortic. (2019) 257:108635. doi: 10.1016/j.scienta.2019.108635
29. Zhou, Y, Abbas, F, Wang, Z, Yu, Y, Yue, Y, Li, X, et al. HS-SPME-GC-MS and electronic nose reveal differences in the volatile profiles of Hedychium flowers. Molecules. (2021) 26:5425. doi: 10.3390/molecules26175425
30. Guo, X, Ho, CT, Schwab, W, Song, C, and Wan, X. Aroma compositions of large-leaf yellow tea and potential effect of theanine on volatile formation in tea. Food Chem. (2019) 280:73–82. doi: 10.1016/j.foodchem.2018.12.066
31. Guo, X, Ho, CT, Wan, X, Zhu, H, Liu, Q, and Wen, Z. Changes of volatile compounds and odor profiles in Wuyi rock tea during processing. Food Chem. (2021) 341:128230. doi: 10.1016/j.foodchem.2020.128230
32. Guo, X, Song, C, Ho, CT, and Wan, X. Contribution of L-theanine to the formation of 2,5-dimethylpyrazine, a key roasted peanutty flavor in oolong tea during manufacturing processes. Food Chem. (2018) 263:18–28. doi: 10.1016/j.foodchem.2018.04.117
33. Guo, X, and Wang, P. Aroma characteristics of lavender extract and essential oil from Lavandula angustifolia mill. Molecules. (2020) 25:5541. doi: 10.3390/molecules25235541
34. Yang, X. Aroma constituents and alkylamides of red and green huajiao (Zanthoxylum bungeanum and Zanthoxylum schinifolium). J Agric Food Chem. (2008) 56:1689–96. doi: 10.1021/jf0728101
35. Chen, XM, Kobayashi, H, Sakai, M, Hirata, H, Asai, T, Ohnishi, T, et al. Functional characterization of rose phenylacetaldehyde reductase (PAR), an enzyme involved in the biosynthesis of the scent compund 2-phenylethanol. J Plant Physiol. (2011) 168:88–95. doi: 10.1016/j.jplph.2010.06.011
36. Ho, CT, Zheng, X, and Li, S. Tea aroma formation. Food Sci Human Wellness. (2015) 4:9–27. doi: 10.1016/j.fshw.2015.04.001
37. Yang, Z, Baldermann, S, and Watanabe, N. Recent studies of the volatile compounds in tea. Food Res Int. (2013) 53:585–99. doi: 10.1016/j.foodres.2013.02.011
38. Wang, K, Liu, F, Liu, Z, Huang, J, Xu, Z, Li, Y, et al. Comparison of catechins and volatile compounds among different types of tea using high performance liquid chromatograph and gas chromatograph mass spectrometer. Int J Food Sci Technol. (2011) 46:1406–12. doi: 10.1111/j.1365-2621.2011.02629.x
39. Wang, K, and Ruan, J. Analysis of chemical components in green tea in relation with perceived quality, a case study with Longjing teas. Int J Food Sci Technol. (2009) 44:2476–84. doi: 10.1111/j.1365-2621.2009.02040.x
40. Qu, F, Zhu, X, Ai, Z, Ai, Y, Qiu, F, and Ni, D. Effect of different drying methods on the sensory quality and chemical components of black tea. LWT Food Sci Technol. (2019) 99:112–8. doi: 10.1016/j.lwt.2018.09.036
41. Yang, P, Song, H, Lin, Y, Guo, T, Wang, L, Granvogl, M, et al. Differences of characterisitic aroma compounds in Rougui tea leaves with different roasing temperatures analyzed by switchable GC-O-MS and GC×GC-O-MS and sensory evaluation. Food Funct. (2021) 12:4797–807. doi: 10.1039/d1fo00165e
42. Lv, S, Wu, Y, Li, C, Xu, Y, Liu, L, and Meng, Q. Comparative analysis of Pu-erh and Fuzhuan teas by fully automatic headspace solid-phase microextraction coupled with gas chromatography-mass spectrometry and chemometric methods. J Agric Food Chem. (2014) 62:1810–8. doi: 10.1021/jf405237u
43. Tian, H, Yu, B, Yu, H, and Chen, C. Evaluation of the synergistic olfactory effects of diacetyl, acetaldehyde, and acetoin in a yoghurt matrix using odor threshold, aroma intensity, and electronic nose analyses. J Dairy Sci. (2020) 103:7957–67. doi: 10.3168/jds.2019-17495
44. Feng, Y, Cai, Y, Sun-Waterhouse, D, Cui, C, Su, G, Lin, L, et al. Approaches of aroma extraction dilution analysis (AEDA) for headspace solid phase microextraction and gas chromatography-olfactometry (HS-SPME-GC-O): altering sample amount, diluting the sample or adjusting split ratio? Food Chem. (2015) 187:44–52. doi: 10.1016/j.foodchem.2015.03.138
45. Katalin, V, Boglárka, CL, Andrea, L, and Judit, H. Antifungal activity and composition of essential oils of Conyza canadensis herbs and roots. Sci World J. (2012) 2012:1–5. doi: 10.1100/2012/489646
46. Yang, YN, Zheng, FP, Yu, AN, and Sun, BG. Changes of the free and bound volatile compounds in Rubus corchorifolius L. f. Fruit during ripening. Food Chem. (2019) 287:232–40. doi: 10.1016/j.foodchem.2019.02.080
47. Kawakami, M, and Kobayashi, A. Carotenoid-derived aroma compounds in tea. Abstr Paper Am Chem Soc. (2000) 802:U32. doi: 10.1021/bk-2002-0802.ch011
48. Dong, F, Yang, Z, Baldermann, S, Kajitani, Y, Ota, S, Kasuga, H, et al. Characterization of L-phenylalanine metabolism to acetophenone and 1-phenylethanol in the flowers of Camellia sinensis using stable isotope labeling. J Plant Physiol. (2012) 169:217–25. doi: 10.1016/j.jplph.2011.12.003
49. Dong, F, Zhou, Y, Zeng, L, Peng, Q, Chen, Y, Zhang, L, et al. Elucidation of differential accumulation of 1-phenylethanol in flowers and leaves of tea (Camellia sinensis) plants. Molecules. (2016) 21:1106–15. doi: 10.3390/molecules21091106
50. Joshi, R, Poonam,, and Gulati, A. Biochemical attributes of tea flowers (Camellia sinensis) at different developmental stages in the Kangra region of India. Sci Hortic. (2011) 130:266–74. doi: 10.1016/j.scienta.2011.06.007
51. Wang, C, Zhang, C, Kong, Y, Peng, X, Li, C, Liu, S, et al. A comparative study of volatile components in Dianhong teas from fresh leaves of four tea cultivars by using chromatography-mass spectrometry, multivariate data analysis, and descriptive sensory analysis. Food Res Int. (2017) 100:267–75. doi: 10.1016/j.foodres.2017.07.013
52. Gijs, L, Perpète, P, Timmermans, A, and Collin, S. 3-Methylthiopropionaldehyde as precursor of dimethyl trisulfide in aged beers. J Agric Food Chem. (2000) 48:6196–9. doi: 10.1021/jf0007380
53. Cheon, Y, Kim, JS, Park, JB, Heo, P, Lim, JH, Jung, GY, et al. A biosynthetic pathway for thexanoic acid production in Kluyveromyces marxianus. J Biotechnol. (2014) 182-183:30–6. doi: 10.1016/j.jbiotec.2014.04.010
54. Gao, Y, Chen, Y, Wang, F, Chen, J, Chen, G, Xu, Y, et al. Volatile composition and aroma description of tea (Camellia sinensis) flowers from albino cultivars. Horticulturae. (2023) 9:610. doi: 10.3390/horticulturae9050610
Keywords: tea (Camellia sinensis) flower, volatile compounds, aroma profiles, aroma character impact (ACI), blooming stage
Citation: Guo X, Wang L, Huang X and Zhou Q (2025) Characterization of the volatile compounds in tea (Camellia sinensis L.) flowers during blooming. Front. Nutr. 11:1531185. doi: 10.3389/fnut.2024.1531185
Received: 20 November 2024; Accepted: 26 December 2024;
Published: 14 January 2025.
Edited by:
Ying Gao, Tea Research Institute, Chinese Academy of Agricultural Sciences, ChinaReviewed by:
Tiantian Tian, National University of Singapore (Suzhou) Research Institute, ChinaCopyright © 2025 Guo, Wang, Huang and Zhou. This is an open-access article distributed under the terms of the Creative Commons Attribution License (CC BY). The use, distribution or reproduction in other forums is permitted, provided the original author(s) and the copyright owner(s) are credited and that the original publication in this journal is cited, in accordance with accepted academic practice. No use, distribution or reproduction is permitted which does not comply with these terms.
*Correspondence: Xiangyang Guo, eGlhbmd5YW5nLmd1b0BhaGF1LmVkdS5jbg==; Qiying Zhou, emhvdXF5QHh5bnUuZWR1LmNu
Disclaimer: All claims expressed in this article are solely those of the authors and do not necessarily represent those of their affiliated organizations, or those of the publisher, the editors and the reviewers. Any product that may be evaluated in this article or claim that may be made by its manufacturer is not guaranteed or endorsed by the publisher.
Research integrity at Frontiers
Learn more about the work of our research integrity team to safeguard the quality of each article we publish.