- 1School of Basic Medicine, Jinggangshan University, Ji’an, China
- 2Online Collaborative Research Center for Evidence-Based Medicine Ministry of Education, Jinggangshan University, Ji’an, China
- 3School of Clinical Medicine, Jinggangshan University, Ji’an, China
- 4School of Mathematics and Physics, Jinggangshan University, Ji’an, China
- 5State Key Laboratory of Cardiovascular Diseases, Shanghai East Hospital, School of Medicine, Tongji University, Shanghai, China
- 6School of Chinese Medicine, Jinggangshan University, Ji’an, China
- 7School of Foreign Languages, Jinggangshan University, Ji’an, China
- 8Department of Emergency, The People’s Hospital of Baoan Shenzhen, Shenzhen, China
Background: Previous researches have demonstrated an association between carotenoids and elongated telomeres. Nonetheless, there is scant scientific evidence examining this relationship in individuals who are overweight or obese, a demographic more predisposed to accelerated aging. This study aims to elucidate the correlation between serum carotenoid concentrations and telomere length within this population group.
Methods: Data were sourced from the 2001–2002 National Health and Nutrition Examination Survey, encompassing 2,353 overweight or obese participants. The levels of α-carotene, β-carotene (both trans and cis isomers), β-cryptoxanthin, lutein/zeaxanthin, and trans-lycopene were quantified via high-performance liquid chromatography. Telomere length was assessed using quantitative polymerase chain reaction.
Results: Following adjustment for potential confounders, telomere length exhibited an increase of 1.83 base pairs (bp) per unit elevation in β-carotene levels (β = 1.83; 95% CI: 0.48, 3.18). Within the fully adjusted model, telomere length incremented by 1.7 bp per unit increase in serum β-carotene among overweight individuals (β = 1.7; 95% CI: 0.1, 3.3), and by 2.6 bp per unit increase among obese individuals (β = 2.6; 95% CI: 0.1, 5.0). Furthermore, restricted cubic spline analysis revealed a linear relationship between β-carotene levels and telomere length, whereas a non-linear association was observed between β-cryptoxanthin levels and telomere length.
Conclusion: This investigation indicates that higher serum β-carotene concentrations are linked with extended telomere length in overweight and obese populations in the United States. These findings warrant further validation through prospective studies.
1 Introduction
Serum carotenoids are well-recognized natural antioxidants, with over 95% of carotenoids in human blood circulation primarily consisting of β-carotene, α-carotene, β-cryptoxanthin, lutein/zeaxanthin, and lycopene. These carotenoids exhibit potent antioxidant properties, mitigating damage induced by reactive oxygen species and inhibiting lipid peroxidation (1). Additionally, carotenoids are involved in cellular signaling pathways associated with inflammation and oxidative stress (OS), thereby exerting a modulatory effect on both OS and inflammation (2).
Telomeres, located at the termini of linear chromosomes, are composed of thousands of TTAGGG nucleotide sequence repeats, serving to protect chromosome ends from deterioration and preventing chromosomal fusion (3). Genetic factors play a crucial role in determining telomere length. Concurrently, the preservation of telomere length is integral to genomic stability and aging. Throughout an individual’s lifespan, telomeres progressively shorten with each cell division (4). In essence, various environmental factors impacting genomic stability, aging, oxidative and inflammatory responses—such as diet, smoking, obesity, and physical activity—contribute to alterations in telomere length (5, 6).
Despite the established correlation between serum carotenoids and telomere length, there remains a paucity of information regarding this relationship in overweight or obese individuals. To our knowledge, this is the inaugural study exploring the association between serum carotenoids and telomere length in overweight or obese populations within the United States. Over recent decades, the prevalence of obesity has surged globally, attributed to shifts in dietary habits and lifestyle choices (7). Obesity, characterized by the excessive accumulation of adipose tissue (AT), involves the release of adipokines from AT, which regulate various biological processes such as inflammation, insulin resistance, and glucose and lipid metabolism, thereby contributing to the pathogenesis of obesity-related diseases (8).
Therefore, the objective of this study is to investigate the relationship between serum carotenoid concentrations and telomere length among individuals classified as overweight or obese, using data from the 2001/2002 cycle of the National Health and Nutrition Examination Survey (NHANES). Given the antioxidant properties of carotenoids, we hypothesize that higher carotenoid levels may attenuate telomere shortening in the study population.
2 Methods
2.1 Study population
The National Health and Nutrition Examination Survey (NHANES) is an extensive research initiative designed to evaluate the health and nutritional status of adults and children in the United States. NHANES has received formal approval from the US Centers for Disease Control and Prevention’s Research Ethics Review Board, with written informed consent obtained from all study participants. The datasets generated and analyzed in this study are publicly accessible on the NHANES official website.1
Our study population was derived from the 2001–2002 NHANES database. Initially, we screened 11,039 participants to identify overweight and obese individuals, excluding those with a BMI ≤ 25 kg/m2 (N = 7,023). Further exclusions were made for individuals with missing data on telomere length, carotenoid levels, education, poverty income ratio (PIR), physical activity, energy intake, congestive heart failure, cancer or malignancy, hypertension, smoking, and alcohol consumption. 2,353 participants were included in our analysis (Figure 1).
2.2 Assessment of serum carotenoid levels
Serum specimens for carotenoid measurement were processed, stored, and shipped to the National Center for Disease Control and Prevention’s Department of Laboratory Sciences for analysis. The primary carotenoids measured in NHANES 2001–2002 were α-carotene, trans-β-carotene, cis-β-carotene, β-cryptoxanthin, combined lutein/zeaxanthin, and trans-lycopene. These measurements were conducted using high-performance liquid chromatography with photodiode array detection. Detailed laboratory procedures and quality control methods for serum carotenoid measurements are available elsewhere (9). The serum concentrations of total carotenoids were calculated by summing the concentrations of the five carotenoids listed above.
2.3 Assessment of telomere length
For DNA analysis, whole blood samples were collected from participants, and quantitative polymerase chain reaction (qPCR) was performed to determine telomere length (T/S ratio) related to standard reference DNA in Dr. Elizabeth Blackburn’s laboratory in San Francisco, CA. Further details on telomere length determination are available on the laboratory section’s website.2 The inter-assay coefficient of variation was 6.5%. A T/S ratio to base pair conversion was utilized, with the conversion formula being 3,274 + 2413*(T/S). Rigorous quality control reviews were conducted by the Centers for Disease Control and Prevention before linking telomere data to the NHANES 2001–2002 public data files.
2.4 Assessment of covariates
Demographic information included age, sex, education, race, poverty income ratio (PIR), BMI, and energy intake. Questionnaire data covered physical activity, smoking status, and alcohol consumption status. BMI was calculated as weight/height2 (kg/m2). Ethnicity was categorized as non-Hispanic white, non-Hispanic Black, Mexican American, other Hispanic, or other races. Physical activity levels were classified into no aerobic activity, low-level exercise, moderate-level exercise, and high-level exercise. The NHANES definitions were used to classify physical activity levels, ranging from predominantly sedentary to high-load activities. Smokers were defined as individuals who had smoked more than one hundred cigarettes in their lifetime, while drinkers were participants who consumed at least 12 alcoholic drinks of any type in a given year.
Medical history variables included hypertension, defined as mean systolic blood pressure ≥ 140 mmHg, mean diastolic blood pressure ≥ 90 mmHg, or self-reported hypertension. Data on congestive heart failure and cancer or malignancy were obtained through self-report questionnaires.
2.5 Statistical analysis
Continuous variables were presented as mean ± standard deviation, while categorical variables were expressed as counts (percentages). Serum total carotenoids were divided into quartiles. Baseline characteristics across different quartiles were assessed using chi-square tests and analysis of variance (Table 1). Generalized linear models were constructed to evaluate the relationship between serum carotenoids and telomere length in overweight or obese participants. Logistic regression models were used to assess the relationship between each quartile of serum carotenoids and their lowest quartile, with linear trends calculated by treating carotenoid quartiles as continuous variables (Table 2). Similar analyses were conducted in non-overweight and non-obese individuals (Supplementary Table S1). Further regression analyses were performed by categorizing participants into overweight (BMI < 30 kg/m2) and obese (BMI ≥ 30 kg/m2) subgroups based on obesity thresholds (Table 3).
Three models were employed to adjust for potential confounders identified in previous studies (10). Model I was adjusted for sex and age, Model II included additional demographic characteristics such as education, race, PIR, BMI, physical activity, and energy intake, and Model III further adjusted for medical history variables including congestive heart failure, cancer or malignancy, hypertension, smoking, and alcohol consumption. Stratified analyses were conducted to determine the relationship between serum carotenoids and telomere length across various subgroups based on sex, education, race, physical activity, congestive heart failure, cancer or malignancy, hypertension, smoking, and alcohol consumption (Figure 2). Lastly, a restricted cubic spline model with five nodes was utilized to examine the relationship between each serum carotenoid and telomere length (Figure 3).
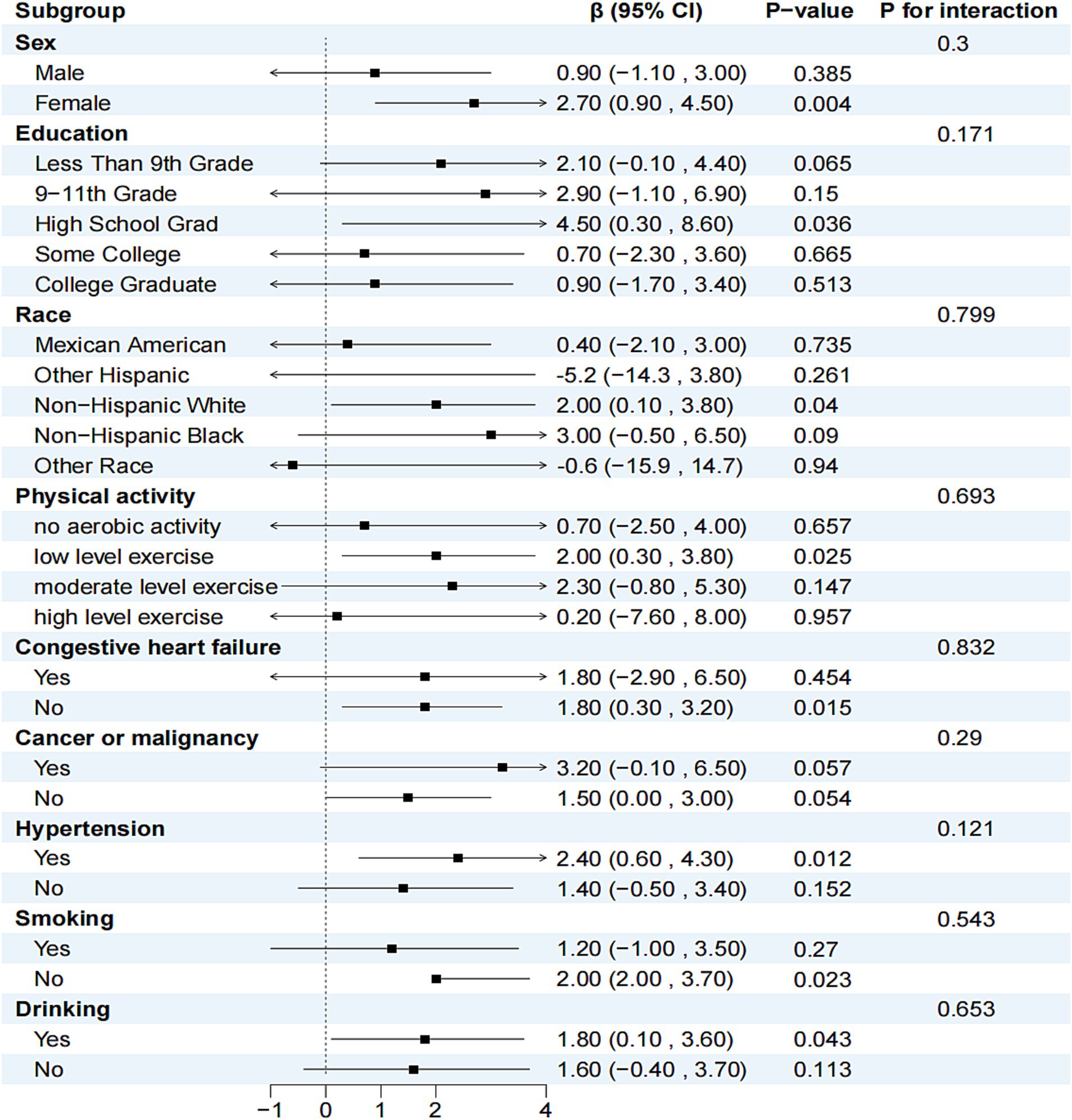
Figure 2. Relationship between serum β-carotene and telomere length in different subgroups. In addition to the stratification variables themselves, sex, education, race, physical activity, congestive heart failure, cancer or malignancy, hypertension, smoking and drinking were adjusted.
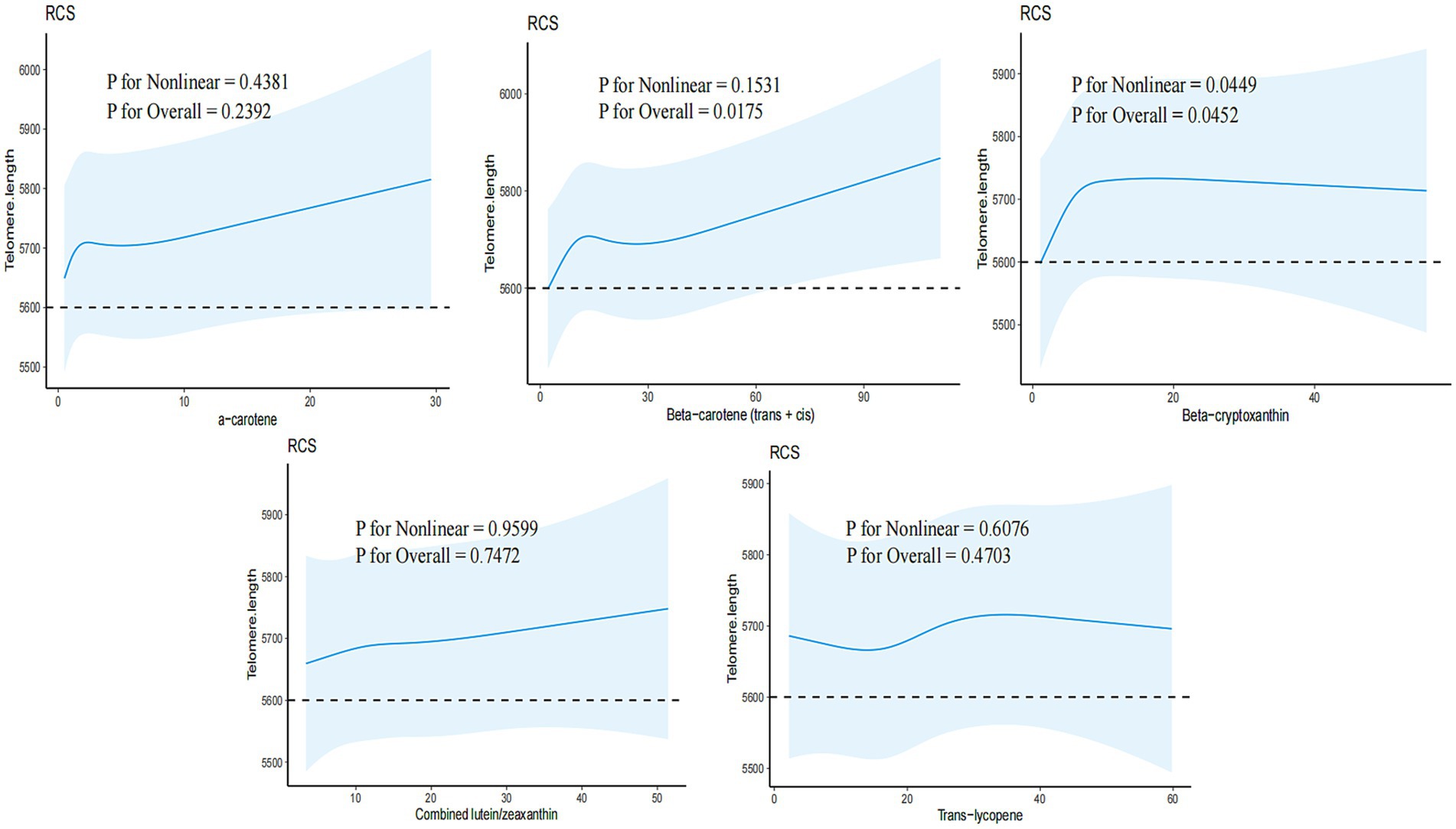
Figure 3. Association between serum β-carotene and telomere length in overweight and obese people. Adjusted for age, sex, education, race, PIR, BMI, physical activity, energy, congestive heart failure, cancer or malignancy, hypertension, smoking, and drinking.
All analyses were performed using a two-sided significance level (p < 0.05) with the statistical software packages R3 and Empower Stats.4
3 Results
3.1 Baseline characteristics of participants
The baseline characteristics of the 2,353 participants included in this study are presented in Table 1. The mean age of the subjects was 49.4 ± 17.6 years, with 1,171 males (49.8%). Participants in the highest serum total carotenoid group (Q4) were more likely to be female, older, and had a higher proportion of college graduates or higher education levels. This group also tended toward lower or moderate physical activity compared to other groups, had a lower mean BMI, and consumed less energy. Additionally, they were less likely to smoke or have conditions such as congestive heart failure.
3.2 Relationship between serum carotenoids and telomere length
The relationship between serum carotenoids and telomere length is detailed in Table 2. After multivariate adjustment, a significant relationship was observed between β-carotene (trans + cis) and telomere length, whereas no association was found with α-carotene, β-cryptoxanthin, lutein/zeaxanthin, and trans-lycopene. For continuous carotenoid levels, telomere length increased by 1.83 base pairs (bp) per unit increase in β-carotene levels (β = 1.83; 95% CI: 0.48, 3.18). When carotenoid levels were divided into quartiles, a significant positive correlation was found between the highest quartile and telomere length compared with the lowest quartile for β-carotene (OR = 73.1; 95% CI: 5.18, 140.84) and β-cryptoxanthin (OR = 92.36; 95% CI: 22.37, 162.35). Across all models, trend tests indicated statistically significant associations for β-carotene (p for trend <0.05). No such association was observed in non-overweight and non-obese individuals, as shown in Supplementary Table S1. In the fully adjusted model, telomere length increased by 1.7 bp per unit increase in serum β-carotene in overweight individuals (β = 1.7; 95% CI: 0.1, 3.3), and by 2.6 bp per unit increase in obese individuals (β = 2.6; 95% CI: 0.1, 5.0), as shown in Table 3.
3.3 Subgroup analysis
The relationship between serum β-carotene and telomere length within subgroups is shown in Figure 2. Subgroups were stratified by sex, education, ethnicity, physical activity, congestive heart failure, cancer or malignancy, hypertension, smoking, and alcohol consumption. After adjusting for variables other than the stratification variable itself, no significant interaction was found between β-carotene levels and potential confounders of telomere length (p > 0.05 for each interaction). Supplementary Tables S2–S5 present stratified analyses and interactions between the other four carotenoids and telomere length, with results similar to those for β-carotene.
3.4 Restricted cubic spline model
The dose–response relationship between carotenoid levels and telomere length is illustrated in Figure 3. No linear deviation from telomere length was observed for β-carotene (p for Nonlinear = 0.1531; p for Overall = 0.0175). However, a non-linear relationship was detected between β-cryptoxanthin and telomere length (p for Nonlinear = 0.0449; p for Overall = 0.0452), with a significant relationship below the threshold of 17.6 μg/dL. No nonlinear relationship was observed for the other three carotenoids.
4 Discussion
Our study found that increasing serum carotenoid levels were significantly associated with longer telomere lengths in overweight or obese U.S. populations. Specifically, β-carotene showed a linear correlation with telomere length, while β-cryptoxanthin showed a non-linear correlation. The other three carotenoids were not statistically significant. The increase in carotenoid levels had a more significant effect on telomere length in obese individuals compared to overweight individuals. Notably, no such relationship was found between carotenoids and telomere length in non-overweight or non-obese individuals.
Previous studies have shown a significant positive relationship between telomere length and self-reported high dietary intake of vegetables and β-carotene (11), particularly in women not using multivitamins (12). Serum carotenoid levels have also been highlighted as objective markers of dietary intake. A study from Austria indicated that higher plasma concentrations of lutein, zeaxanthin, and vitamin C were associated with longer leukocyte telomere length in normal older adults, suggesting a protective role for these vitamins in telomere maintenance (3). Similarly, an increase in blood carotenoid levels was significantly associated with longer leukocyte telomeres in 3,660 adults from NHANES (10). And this result was replicated in a larger cohort, serum carotenoids generally showed a positive correlation with leukocyte telomere length (13).
However, most previous studies have focused on the general adult population, neglecting groups more prone to accelerated aging, such as overweight or obese individuals (14). This longitudinal study highlights the significant finding that telomere shortening begins at a remarkably early age in children with obesity (15). This aligns with the understanding that obesity reduces telomere length by persistently affecting systemic inflammation and redox homeostasis (16). The urgent need for preventive measures and early interventions is emphasized to mitigate the long-term health consequences of obesity on telomere dynamics and associated metabolic disorders. For example, obese mice have shown reduced telomere length in oocytes and embryos (17), and overweight and obese children have significantly shorter telomeres compared to children with normal BMI (18). A collaborative cross-sectional meta-analysis of 87 observational studies also demonstrated that higher BMI is associated with shorter telomeres, particularly in young adults (19). Thus, maintaining a healthy body weight is crucial to delay telomere shortening and the development of related diseases. Additionally, a meta-analysis has demonstrated that psychological stress is linked to a reduction in telomere length (16), with high levels correlating with chronic diseases such as obesity and abdominal fat accumulation (20). Furthermore, beyond varying stress levels, socioeconomic status also influences telomere length. A cohort study from FFCWS identified poverty as a predictor of changes in telomere length among women (21). Moreover, certain stressors unique to women may further exacerbate this effect (22).
Mechanistically, increases in oxidative stress and chronic inflammation are key contributors to telomere shortening (23). Reactive oxygen species from oxidative stress can cause breaks in DNA and interfere with the replication of telomeric repeats, leading to an increased rate of telomere shortening. Chronic inflammation increases inflammatory mediators, which also promote telomere shortening. Conversely, telomere shortening in leukocytes leads to decreased immune function and increased secretion of pro-inflammatory factors (24, 25), creating a vicious cycle (26). Obesity exacerbates this cycle by increasing oxidative stress and chronic inflammation (16), potentially due to adipocyte proliferation and hypertrophy leading to adipose tissue hypoxia (27). Therefore, obese individuals may have shorter somatic telomere lengths and are more susceptible to premature aging and reduced cell lifespan (28–30). Our findings support this trend, with lower BMI associated with longer telomeres across all populations included.
In this study, serum β-carotene levels were significantly associated with longer telomeres, while β-cryptoxanthin showed no significant relationship beyond a certain concentration. α-carotene, lutein/zeaxanthin, and trans-lycopene were not statistically significant. This difference may be due to the study population size, statistical methods, and choice of confounding variables. Further carefully designed studies are needed to assess the effects of these carotenoids on telomeres (10).
Despite these differences, carotenoids still play a significant role in protecting against telomere loss. Tocopherol (vitamin E) and β-carotene work synergistically to quench reactive oxygen species (ROS). Specifically β-carotene neutralize peroxyl radicals, leading to the formation of a carotenoid radical cation (CAR•+). This CAR• + can be reduced back to β-carotene by cellular antioxidants like tocopherol, thereby recycling β-carotene and reducing the propagation of lipid peroxidation (31). Carotenoids have anti-inflammatory properties, and increased serum concentrations can reduce the production of inflammatory mediators, potentially protecting telomeres from inflammatory damage (3). As potent antioxidants, they can neutralize free radicals and reduce oxidative stress, thus delaying telomere shortening in obese individuals (10, 32–34). Obesity-related unhealthy lifestyles, such as poor dietary habits and lack of exercise, may lead to reduced carotenoid intake, indirectly affecting telomere length. Hormonal fluctuations in obesity may also impact telomere length, and carotenoids may influence telomere length through hormone modulation or other signaling pathways (35).
For provitamin A carotenoids, including α-carotene, β-carotene, and β-cryptoxanthin, β-carotene, are abundantly found in yellow-orange fruits and green leafy vegetables. Notable sources of β-carotene include carrots, pumpkins, and celery (36). β-carotene may exhibit pro-oxidative properties at high concentrations or high oxygen partial pressures (10). The narrative review by Baliou et al. (2024) highlights the diverse benefits of the Mediterranean diet on telomere biology. It suggests that a diet rich in carotenoids from natural food sources may be more effective than supplementation in preserving telomere length, thereby helping to mitigate the progression of age-related diseases (37). β-carotene in adipose tissue may be metabolized into thrombotic or atherogenic derivatives, increasing the risk of cardiovascular disorders (38, 39). Conversely, non-provitamin A carotenoids, including lutein/zeaxanthin and trans-lycopene, have been reported to prevent DNA damage (40). Follow-up studies are needed to determine if specific carotenoids differentially protect telomere length. Notably, several studies have indicated that β-carotene supplementation alone, particularly at high doses, is linked to adverse outcomes, including an increased risk of all-cause mortality and a higher likelihood of lung cancer among individual at elevated risk for this disease (41, 42). Therefore, for daily intake, a mixed consumption of various carotenoids is recommended to avoid excessive intake of any single carotenoid class (43). Carotenoids may help reduce the risk of telomere shortening in obese individuals, underscoring the importance of a balanced dietary approach. Such as the Mediterranean diet, which offers broader nutritional support compared to isolated supplementation.
However, our study has limitations. First and foremost, our study utilized only the 2001–2002 cycle of the NHANES database (13). Further research and periodic studies are necessary to validate our findings. Also, as a cross-sectional study, it only explores the relationship between serum carotenoids and telomere length in obese individuals without establishing causality. More longitudinal studies and intervention trials are needed to clarify these associations and explore differences across gender, age, and ethnic groups (16). Additionally, there is no precise definition of high carotenoid concentrations, which may introduce bias.
Despite these limitations, our study highlights the potential relationship between serum carotenoids and telomere length in obese individuals, suggesting that increasing carotenoid intake may help delay telomere shortening, cellular aging, and related diseases in this population.
5 Conclusion
In conclusion, this study suggests that serum β-carotene is linearly and positively associated with longer telomere length in overweight and obese U.S. populations. Compared with overweight participants, obese participants ingested more β-carotene better for delaying telomere shortening. While the potential role of other carotenoids in delaying aging cannot be denied, further confirmation through future prospective studies is needed.
Data availability statement
The raw data supporting the conclusions of this article will be made available by the authors, without undue reservation.
Ethics statement
The studies involving humans were approved by the National Center for Health Statistics (NCHS). The studies were conducted in accordance with the local legislation and institutional requirements. The participants provided their written informed consent to participate in this study. Written informed consent was obtained from the individual(s) for the publication of any potentially identifiable images or data included in this article.
Author contributions
JW: Conceptualization, Project administration, Supervision, Validation, Writing – review & editing. FX: Conceptualization, Data curation, Formal analysis, Investigation, Methodology, Project administration, Software, Visualization, Writing – original draft, Writing – review & editing. WZ: Data curation, Formal analysis, Investigation, Project administration, Writing – original draft. DY: Investigation, Project administration, Resources, Writing – original draft, Writing – review & editing. YX: Methodology, Project administration, Software, Writing – review & editing. MS: Data curation, Formal analysis, Investigation, Validation, Writing – original draft, Writing – review & editing. RZ: Investigation, Project administration, Supervision, Validation, Writing – review & editing. JB: Investigation, Project administration, Supervision, Validation, Writing – review & editing. XX: Investigation, Project administration, Supervision, Validation, Writing – review & editing. LC: Data curation, Investigation, Supervision, Validation, Writing – review & editing. AZ: Methodology, Software, Validation, Writing – review & editing. KZ: Writing – review & editing. TF: Writing – review & editing. BL: Funding acquisition, Project administration, Supervision, Writing – review & editing. LX: Project administration, Supervision, Writing – review & editing. XZ: Investigation, Project administration, Supervision, Validation, Writing – review & editing.
Funding
The author(s) declare that financial support was received for the research, authorship, and/or publication of this article. This work was supported by the National Natural Science Foundation of China [grant no. 52163015].
Acknowledgments
The authors thank all the NHANES study participants for their assistance.
Conflict of interest
The authors declare that the research was conducted in the absence of any commercial or financial relationships that could be construed as a potential conflict of interest.
Publisher’s note
All claims expressed in this article are solely those of the authors and do not necessarily represent those of their affiliated organizations, or those of the publisher, the editors and the reviewers. Any product that may be evaluated in this article, or claim that may be made by its manufacturer, is not guaranteed or endorsed by the publisher.
Supplementary material
The Supplementary material for this article can be found online at: https://www.frontiersin.org/articles/10.3389/fnut.2024.1479994/full#supplementary-material
Footnotes
1. ^https://wwwn.cdc.gov/nchs/NHANES/Default.aspx
2. ^http://cdc.gov/nchs/nhanes
4. ^www.empowerstats.com, X&Y Solutions, Inc.
References
1. Terao, J . Revisiting carotenoids as dietary antioxidants for human health and disease prevention. Food Funct. (2023) 14:7799–824. doi: 10.1039/D3FO02330C
2. Wang, M, Tang, R, Zhou, R, Qian, Y, and Di, D. The protective effect of serum carotenoids on cardiovascular disease: a cross-sectional study from the general US adult population. Front Nutr. (2023) 10:1154239. doi: 10.3389/fnut.2023.1154239
3. Crous-Bou, M, and Molinuevo, J-L. Sala-Vila a: plant-rich dietary patterns, plant foods and nutrients, and telomere length. Adv Nutr. (2019) 10:S296–303. doi: 10.1093/advances/nmz026
4. Blackburn, EH, Epel, ES, and Lin, J. Human telomere biology: a contributory and interactive factor in aging, disease risks, and protection. Science. (2015) 350:1193–8. doi: 10.1126/science.aab3389
5. Patel, CJ, Manrai, AK, Corona, E, and Kohane, IS. Systematic correlation of environmental exposure and physiological and self-reported behaviour factors with leukocyte telomere length. Int J Epidemiol. (2017) 46:44–56. doi: 10.1093/ije/dyw043
6. Güneşliol, BE, Karaca, E, Ağagündüz, D, and Acar, ZA. Association of physical activity and nutrition with telomere length, a marker of cellular aging: a comprehensive review. Crit Rev Food Sci Nutr. (2023) 63:674–92. doi: 10.1080/10408398.2021.1952402
7. Blüher, M . Obesity: global epidemiology and pathogenesis. Nat Rev Endocrinol. (2019) 15:288–98. doi: 10.1038/s41574-019-0176-8
8. Pham, D-V, Nguyen, T-K, and Park, P-H. Adipokines at the crossroads of obesity and mesenchymal stem cell therapy. Exp Mol Med. (2023) 55:313–24. doi: 10.1038/s12276-023-00940-2
9. Laboratory Procedure Manual . (2007). Available at: https://wwwn.cdc.gov/Nchs/Nhanes/2001-2002/L06VIT_B.htm.
10. Min, K-B, and Min, J-Y. Association between leukocyte telomere length and serum carotenoid in US adults. Eur J Nutr. (2017) 56:1045–52. doi: 10.1007/s00394-016-1152-x
11. Marcon, F, Siniscalchi, E, Crebelli, R, Saieva, C, Sera, F, Fortini, P, et al. Diet-related telomere shortening and chromosome stability. Mutagenesis. (2012) 27:49–57. doi: 10.1093/mutage/ger056
12. Xu, Q, Parks, CG, DeRoo, LA, Cawthon, RM, Sandler, DP, and Chen, H. Multivitamin use and telomere length in women. Am J Clin Nutr. (2009) 89:1857–63. doi: 10.3945/ajcn.2008.26986
13. Mazidi, M, Kengne, AP, Cheskin, LJ, and Banach, M. Serum lipophilic antioxidants levels are associated with leucocyte telomere length among US adults. Lipids Health Dis. (2018) 17:164. doi: 10.1186/s12944-018-0781-x
14. Salmon, AB . Beyond diabetes: does obesity-induced oxidative stress drive the aging process? Antioxidants (Basel). (2016) 5:24. doi: 10.3390/antiox5030024
15. Toupance, S, Karampatsou, SI, Labat, C, Genitsaridi, S-M, Tragomalou, A, Kassari, P, et al. Longitudinal Association of Telomere Dynamics with obesity and metabolic disorders in young children. Nutrients. (2022) 14:191. doi: 10.3390/nu14235191
16. Mathur, MB, Epel, E, Kind, S, Desai, M, Parks, CG, Sandler, DP, et al. Perceived stress and telomere length: a systematic review, meta-analysis, and methodologic considerations for advancing the field. Brain Behav Immun. (2016) 54:158–69. doi: 10.1016/j.bbi.2016.02.002
17. Ge, J, Li, C, Sun, H, Xin, Y, Zhu, S, Liu, Y, et al. Telomere dysfunction in oocytes and embryos from obese mice. Front Cell Dev Biol. (2021) 9:617225. doi: 10.3389/fcell.2021.617225
18. Reljic, D, Koller, A, Herrmann, HJ, Ekici, AB, and Neurath, MF. Zopf Y: differential effects of very-low-volume exercise modalities on telomere length, inflammation, and Cardiometabolic health in obese metabolic syndrome patients: a subanalysis from two randomized controlled trials. Antioxidants (Basel). (2023) 12:1847. doi: 10.3390/antiox12101847
19. Gielen, M, Hageman, GJ, Antoniou, EE, Nordfjall, K, Mangino, M, Balasubramanyam, M, et al. Body mass index is negatively associated with telomere length: a collaborative cross-sectional meta-analysis of 87 observational studies. Am J Clin Nutr. (2018) 108:453–75. doi: 10.1093/ajcn/nqy107
20. Dallman, MF, Pecoraro, N, Akana, SF, La Fleur, SE, Gomez, F, Houshyar, H, et al. Chronic stress and obesity: a new view of "comfort food". Proc Natl Acad Sci USA. (2003) 100:11696–701. doi: 10.1073/pnas.1934666100
21. Assari, S, Dezfuli, M, Peyrovinasab, A, and Zare, H. Does adulthood socioeconomic status predict subsequent telomere length in racially and ethnically diverse women? J Biomed Life Sci. (2024) 4:47–59. doi: 10.31586/jbls.2024.1023
22. Gianakos, I . Gender roles and coping with work stress. Sex Roles. (2000) 42:1059–79. doi: 10.1023/A:1007092718727
23. Lin, J, and Epel, E. Stress and telomere shortening: insights from cellular mechanisms. Ageing Res Rev. (2022) 73:101507. doi: 10.1016/j.arr.2021.101507
24. Effros, RB . Kleemeier award lecture 2008--the canary in the coal mine: telomeres and human healthspan. J Gerontol A Biol Sci Med Sci. (2009) 64:511–5. doi: 10.1093/gerona/glp001
25. Sebastián, C, Herrero, C, Serra, M, Lloberas, J, Blasco, MA, and Celada, A. Telomere shortening and oxidative stress in aged macrophages results in impaired STAT5a phosphorylation. J Immunol. (2009) 183:2356–64. doi: 10.4049/jimmunol.0901131
26. Wolkowitz, OM, Mellon, SH, Epel, ES, Lin, J, Dhabhar, FS, Su, Y, et al. Leukocyte telomere length in major depression: correlations with chronicity, inflammation and oxidative stress - preliminary findings. PLoS One. (2011) 6:e17837. doi: 10.1371/journal.pone.0017837
27. Liu, F, He, J, Wang, H, Zhu, D, and Bi, Y. Adipose morphology: a critical factor in regulation of human metabolic diseases and adipose tissue dysfunction. Obes Surg. (2020) 30:5086–100. doi: 10.1007/s11695-020-04983-6
28. Tiainen, AMK, Männistö, S, Blomstedt, PA, Moltchanova, E, Perälä, MM, Kaartinen, NE, et al. Leukocyte telomere length and its relation to food and nutrient intake in an elderly population. Eur J Clin Nutr. (2012) 66:1290–4. doi: 10.1038/ejcn.2012.143
29. García-Calzón, S, Gea, A, Razquin, C, Corella, D, Lamuela-Raventós, RM, Martínez, JA, et al. Longitudinal association of telomere length and obesity indices in an intervention study with a Mediterranean diet: the PREDIMED-NAVARRA trial. Int J Obes. (2014) 38:177–82. doi: 10.1038/ijo.2013.68
30. el Bouazzaoui, F, Henneman, P, Thijssen, P, Visser, A, Koning, F, Lips, MA, et al. Willems van Dijk K, van Harmelen V: adipocyte telomere length associates negatively with adipocyte size, whereas adipose tissue telomere length associates negatively with the extent of fibrosis in severely obese women. Int J Obes. (2014) 38:746–9. doi: 10.1038/ijo.2013.175
31. Saini, RK, Prasad, P, Lokesh, V, Shang, X, Shin, J, and Keum, Y-S. Lee J-H: carotenoids: dietary sources, extraction, encapsulation, bioavailability, and health benefits-a review of recent advancements. Antioxidants (Basel). (2022) 11:795. doi: 10.3390/antiox11040795
32. Houben, JMJ, Moonen, HJJ, van Schooten, FJ, and Hageman, GJ. Telomere length assessment: biomarker of chronic oxidative stress? Free Radic Biol Med. (2008) 44:235–46. doi: 10.1016/j.freeradbiomed.2007.10.001
33. Ma, D, Zhu, W, Hu, S, Yu, X, and Yang, Y. Association between oxidative stress and telomere length in type 1 and type 2 diabetic patients. J Endocrinol Investig. (2013) 36:1032–7. doi: 10.3275/9036
34. Babizhayev, MA, Savel'yeva, EL, Moskvina, SN, and Yegorov, YE. Telomere length is a biomarker of cumulative oxidative stress, biologic age, and an independent predictor of survival and therapeutic treatment requirement associated with smoking behavior. Am J Ther. (2011) 18:e209–26. doi: 10.1097/MJT.0b013e3181cf8ebb
35. Schmidt, TT, Tyer, C, Rughani, P, Haggblom, C, Jones, JR, Dai, X, et al. High resolution long-read telomere sequencing reveals dynamic mechanisms in aging and cancer. Nat Commun. (2024) 15:5149. doi: 10.1038/s41467-024-48917-7
36. Gebregziabher, BS, Gebremeskel, H, Debesa, B, and Ayalneh, D. Carotenoids: dietary sources, health functions, biofortification, marketing trend and affecting factors – a review. J Agric Food Res. (2023) 14:100834. doi: 10.1016/j.jafr.2023.100834
37. Baliou, S, Ioannou, P, Apetroaei, M-M, Vakonaki, E, Fragkiadaki, P, Kirithras, E, et al. The impact of the Mediterranean diet on telomere biology: implications for disease management-a narrative review. Nutrients. (2024) 16:525. doi: 10.3390/nu16152525
38. Liu, S, Wu, Q, Wang, S, and He, Y. Causal associations between circulation β-carotene and cardiovascular disease: a Mendelian randomization study. Medicine (Baltimore). (2023) 102:e36432. doi: 10.1097/MD.0000000000036432
39. Qiu, Z, Chen, X, Geng, T, Wan, Z, Lu, Q, Li, L, et al. Associations of serum carotenoids with risk of cardiovascular mortality among individuals with type 2 diabetes: results from NHANES. Diabetes Care. (2022) 45:1453–61. doi: 10.2337/dc21-2371
40. Azqueta, A . Collins AR: carotenoids and DNA damage. Mutat Res. (2012) 733:4. doi: 10.1016/j.mrfmmm.2012.03.005
41. Schwingshackl, L, Boeing, H, Stelmach-Mardas, M, Gottschald, M, Dietrich, S, Hoffmann, G, et al. Dietary supplements and risk of cause-specific death, cardiovascular disease, and Cancer: a systematic review and Meta-analysis of primary prevention trials. Adv Nutr. (2017) 8:27–39. doi: 10.3945/an.116.013516
42. O'Connor, EA, Evans, CV, Ivlev, I, Rushkin, MC, Thomas, RG, Martin, A, et al. Vitamin and mineral supplements for the primary prevention of cardiovascular disease and Cancer: updated evidence report and systematic review for the US preventive services task force. JAMA. (2022) 327:2334–47. doi: 10.1001/jama.2021.15650
Keywords: β-carotene, carotenoids, NHANES, obesity, telomeres
Citation: Wang J, Xie F, Zhu W, Ye D, Xiao Y, Shi M, Zeng R, Bian J, Xu X, Chen L, Zhu A, Zhu K, Fan T, Liu B, Xiao L and Zhang X (2024) Relationship between serum carotenoids and telomere length in overweight or obese individuals. Front. Nutr. 11:1479994. doi: 10.3389/fnut.2024.1479994
Edited by:
Xihan Guo, Yunnan Normal University, ChinaReviewed by:
Kulvinder Kochar Kaur, Kulvinder Kaur Centre for Human Reproduction, IndiaKaijian Hou, Shantou University, China
Copyright © 2024 Wang, Xie, Zhu, Ye, Xiao, Shi, Zeng, Bian, Xu, Chen, Zhu, Zhu, Fan, Liu, Xiao and Zhang. This is an open-access article distributed under the terms of the Creative Commons Attribution License (CC BY). The use, distribution or reproduction in other forums is permitted, provided the original author(s) and the copyright owner(s) are credited and that the original publication in this journal is cited, in accordance with accepted academic practice. No use, distribution or reproduction is permitted which does not comply with these terms.
*Correspondence: Bin Liu, TGl1YmluMjAxMkBqZ3N1LmVkdS5jbg==; Liyan Xiao, MzA3MjU3Mzc3M0BxcS5jb20=; Xiaoming Zhang, emhhbmdtdXhpMDMxMEAxNjMuY29t
†These authors have contributed equally to this work and share first authorship