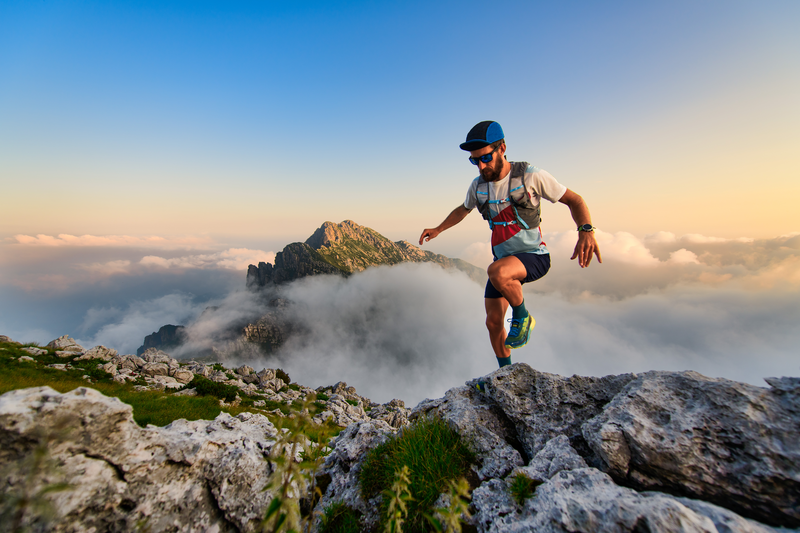
94% of researchers rate our articles as excellent or good
Learn more about the work of our research integrity team to safeguard the quality of each article we publish.
Find out more
REVIEW article
Front. Nutr. , 22 February 2024
Sec. Food Chemistry
Volume 11 - 2024 | https://doi.org/10.3389/fnut.2024.1359813
This article is part of the Research Topic Extracts From Plants and Other Natural Sources: Application, Characterization, Optimization, and Their Use View all 10 articles
Perilla frutescens (L.) Britton is an annual herb plant of the Perilla genus in the Labiatae family, which is commonly utilized as an edible and medicinal resource. Polysaccharides are among the major components and essential bioactive compounds of P. frutescens, which exhibit a multitude of biological activities, including antioxidant, antitumor, anti-fatigue, immunoregulation, hepatoprotective, anti-inflammatory, and lipid-lowering effects. As a natural carbohydrate, P. frutescens polysaccharide has the potential to be utilized in the development of drugs and functional materials. In this paper, we provide an overview of progress made on the extraction, purification, structural characterization, and bioactivity of polysaccharides from different parts of P. frutescens. The challenges and opportunities for research are discussed, along with the potential development prospects and future areas of focus in the study of P. frutescens polysaccharides.
Perilla frutescens (L.) Britton, also known as zisu in China, is an annual herb plant of the Labiatae family (1, 2). Although P. frutescens is widespread in Asian countries (Figure 1A) (3, 4), China is proposed to be the main genetic center of this species, where it has been cultivated for more than 2,000 years (5, 6). According to the variation of plant leaf color, P. frutescens can be divided into two main varieties circulating in China: P. frutescens var. arguta (the lower part of the leaf is red or purple) and P. frutescens var. frutescens (the upper and lower leaf surfaces are green) (7, 8). P. frutescens is considered a medicine and food homologous plant, as the dried stems, leaves (Figure 1B), and seeds (Figure 1C) can be used as a natural herbal medicine for pain relief, hemostasis, relief of cough, purgative, detoxification, relief of stomach upset, dissipating colds, and anti-inflammation (8–10). As part of the daily diet, P. frutescens leaves can be used in barbecue, sashimi, sushi, and as a culinary condiment given their aromatic flavor (9, 11). Additionally, the oil of P. frutescens seeds can be used in baking pastries as an alternative to hydrogenated oils or cream (9). P. frutescens is becoming a more popular item in home cooking as it is increasingly recognized as a health-promoting food source.
Figure 1. Distribution diagram of P. frutescens in the world (A); stems and leaves of P. frutescens (B); seeds of P. frutescens (C).
In recent years, the chemical composition of P. frutescens has been extensively investigated (12). P. frutescens contains multiple active ingredients, including polysaccharides, anthocyanins, flavonoids, terpenoids, phenols, volatile oils, fatty acids, and proteins (13, 14). Among these compounds, polysaccharide is an essential component, which has been increasingly researched for various applications owing to its advantages of natural origin, safety, and low toxicity (15). The polysaccharides obtained from P. frutescens have proven to exhibit antioxidant, hepatoprotective, antitumor, and immunomodulatory activities (16–19), highlighting their broad application prospects in the food and biomedicine industries. To date, studies in this field have mainly focused on the small-molecule compounds of P. frutescens and their pharmacological effects; however, a comprehensive review of the macromolecular polysaccharides of P. frutescens is lacking. Hence, in the present paper, the extraction, purification, structural characteristics, and biological activities of P. frutescens polysaccharides are systematically summarized with a view to further expand their application areas.
The extraction of polysaccharides from raw materials is the first and key step in their effective utilization. Therefore, identifying optimal extraction methods has been a primary research focus. P. frutescens polysaccharides are mainly extracted from the leaf and seed meal (a by-product of the seed after oil extraction) of the plant. Typically, a suitable solvent (such as petroleum ether, butane, or n-hexane) is selected to degrease the P. frutescens raw material in a Soxhlet extractor prior to polysaccharide extraction (15, 18, 20). Polysaccharides are a class of highly polar, highly water-soluble, and ethanol-insoluble substances that can be obtained by hot water extraction (HWE) in combination with ethanol precipitation (21). For instance, Zhou and Sheng (22) prepared polysaccharides from P. frutescens seeds using HWE at 80 °C followed by 70% ethanol alcohol precipitation. To increase the polysaccharide yield, response surface methodology (RSM) can be used to optimize the extraction conditions. For example, Ding (23) determined the following optimized HWE conditions for the extraction of P. frutescens leaf polysaccharide using RSM: material-to-liquid ratio of 1:30, extraction time of 4 h, extraction three times, and ethanol concentration for precipitation of 60% (v/v). Under the above conditions, the extraction yield of crude P. frutescens leaf polysaccharide reached up to 5.22 ± 0.17%. HWE has advantages of being a relatively simple, low-cost, and non-polluting process (24); however, this method suffers from the drawbacks of a long operating time, low yield, and the need for repetitive operations (25). Thus, HWE should be combined with other innovative technologies such as ultrasonic-assisted extraction (UAE), microwave-assisted extraction (MAE), and ultrasonic-assisted enzyme extraction (UAEE).
UAE can promote the release and dissolution of intracellular and cell wall polysaccharides through the high shear pressure generated via cavitation, which has the benefits of a high extraction rate, simple operation, and low solvent dosage (26). Zhang et al. (18) optimized the experimental scheme for the extraction of polysaccharides from P. frutescens seed meal (PSMP) by UAE through RSM, obtaining an average extraction yield of 6.137 ± 0.062% using a liquid-to-solid ratio of 26.00 mL/g, extraction temperature of 43.00°C, ultrasonic time of 52.00 min, and ultrasonic power of 229.00 W. Compared with the time required using HWE, the extraction time for obtaining P. frutescens polysaccharides could be shortened by two-thirds and the extraction temperature was reduced by 33.70–57.40% with UAE (20).
MAE has the advantages of high permeability, selectivity, and extraction efficiency (27), demonstrating its suitability for the extraction of P. frutescens polysaccharide. Microwave is an electromagnetic wave with a frequency in the range of 300 MHz to 300 GHz (24). Microwave heating is produced by the ionic conduction of dissolved ions and molecular friction due to the dipolar rotation of polar solvents (28). The optimized conditions for the MAE of P. frutescens seed polysaccharides were determined to be a liquid-to-feed ratio of 25 mL/g, microwave power of 480 W, and microwave processing time of 3 min, which resulted in a yield of 9.06% with subsequent HWE (90°C, 3 h) (29). In another study, using the MAE method to extract polysaccharides from P. frutescens leaf powder with a short microwave treatment (800 W) of only 30 s after hot water immersion (80°C) for 2 h resulted in improvement of the yield to 3.99% compared to a yield of only 2.21% obtained with the HWE method, which required a total extraction time of 6 h at 80°C (30). Therefore, the extraction of P. frutescens polysaccharides by MAE can save substantial time while improving the efficiency compared with the HWE method.
Enzyme-assisted extraction of polysaccharides involves the use of enzymes capable of breaking down the cell wall, which offers benefits of environmental friendliness, low-energy consumption, and high extraction efficiency (31, 32). Under the optimal conditions of 1771.85 U/g cellulase, enzyme activation temperature of 53.7°C, and enzyme digestion time of 36.2 min, the extraction rate of P. frutescens leaf polysaccharides reached 17.91 mg/g (33). Ultrasonic extraction can further enhance the affinity of the enzyme for the substrate and thereby increase the speed of the enzymatic reaction (24). Therefore, enzyme-assisted extraction can be combined with UAE (i.e., UAEE) to effectively increase the extraction rate. Recently, Li et al. (34) optimized the UAEE process to extract polysaccharides from P. frutescens leaves according to a single-factor test and Box-Behnken design (BBD), obtaining a yield of 3.84% when the liquid-to-solid ratio was 41:1, enzymatic time was 40 min, enzymatic temperature was 49 °C, and ultrasonic power was 204 W. Subsequently, Zhang et al. (35) applied RSM analysis with a BBD to identify the optimal conditions for the UAEE of P. frutescens seed meal polysaccharides (PSMP). With the optimized conditions of a compound enzyme dose of 6.6%, liquid-to-solid ratio of 25 mL/g, extraction time of 61 min, and extraction temperature of 62°C, the PSMP yield was 7.711 ± 0.201%.
In addition to the methods described above, various novel approaches for extracting polysaccharides have also been proposed, including pressurized-liquid extraction, supercritical-fluid extraction, ionic-liquid extraction, and pulsed electric field-assisted extraction (27, 32). However, these novel approaches have not yet been employed for extracting polysaccharides from P. frutescens, requiring further investigation in the future.
The crude polysaccharide obtained after ethanol precipitation normally contains impurities such as proteins, pigments, and small molecules, necessitating subsequent separation and purification processes to obtain the pure polysaccharide (36). The Sevag method is commonly used to remove proteins from crude polysaccharide solutions, which is based on the principle of protein denaturation in chloroform and other organic solvents (17). In brief, the crude polysaccharide solution is blended with Sevag’s reagent (1-butanol/chloroform, 1/4 v/v) at a certain proportion, the mixture is shaken well, and the supernatant is collected after centrifugation to remove some of the proteins (37, 38). The Sevag method offers advantages of mild conditions, without causing the denaturation of polysaccharides (39), and can achieve a good effect of removing proteins from the crude P. frutescens polysaccharide solution after multiple repeated operations (20, 23, 34). Zhang (30) discovered that under the conditions of a 4:1 ratio of chloroform to n-butanol, 1:1 ratio of polysaccharide solution to Sevag reagent, 35 min of deproteinization time, and two deproteinization steps, the deproteinization rate of P. frutescens leaf polysaccharide reached 73.6%. However, the deproteinized P. frutescens polysaccharide solution needs to be further dialyzed to remove residual Sevag reagent and other small-molecule impurities (15, 35).
To obtain homogeneous polysaccharides, further isolation and purification of the crude polysaccharides is required. Column chromatography is the most extensively applied approach for the purification of polysaccharides owing to its good purification effect and simple operation procedures (40–42). Column chromatography mainly includes ion-exchange column chromatography and gel-column chromatography (43). Ion-exchange chromatography involves the use of either cation- or anion-exchange resins (44), with anionic columns most frequently used in the purification of P. frutescens polysaccharides. Anion-exchange chromatography enables strong binding to acidic polysaccharides without interacting with neutral polysaccharides, thus, the neutral polysaccharides are eluted first (45) and those obtained from different fractions can be separated by a stepwise elution process using solutions with different ionic strengths (46). For example, Li et al. (34) eluted four types of P. frutescens leaf polysaccharides on a DAE-Cellulose 52 column (2.6 × 30 cm) at a flow rate of 0.6 mL/min using different concentrations of sodium chloride solution (0, 0.1, 0.2, 0.3, and 0.5 mol/L).
Gel-column chromatography (Sephadex-G series and Sephacryl-S series) is an effective method to separate polysaccharides with different molecular weights (47). High-molecular-weight polysaccharides will be eluted first with the mobile phase, while the low-molecular-weight polysaccharides will diffuse into the pores of the gel and elute later (48). Ding et al. (15) used a Sephadex G-200 gel column (1.6 cm × 30 cm) to purify and obtain a P. frutescens leaf polysaccharide (PFP) with purity reaching up to 89.73%. Different columns can be used synergistically in the separation and purification of polysaccharides to achieve a better effect. Kim et al. (16) first used a DEAE-Toyopearl 650 M column (4.0 × 30 cm) to isolate one polysaccharide, designated PFB-1-0. This polysaccharide was then eluted using a Sephadex G-100 gel column (2.5 × 94 cm) with 0.2 mol/L NaCl at a flow rate of 0.2 mL/min to obtain fractions PFB-1-0i and PFB-1-0ii with 43.2 ± 2.5% and 82.8 ± 4.8% purity, respectively. In the majority of studies, DEAE-52 cellulose, DEAE-Toyopearl 650 M, Sephadex G-100 gel, and Sephadex G-200 gel chromatography columns are employed for the separation of P. frutescens polysaccharides, the eluate is collected, concentrated, and freeze-dried to finally obtain purified P. frutescens polysaccharides. The flow chart of the extraction and purification procedure of P. frutescens polysaccharides is displayed in Table 1 and Figure 2.
Figure 2. Schematic diagram of the extraction and purification process of P. frutescens polysaccharide.
Structural characterization of polysaccharides is important since the structure is closely related to biological activity (54). The key structural features of polysaccharides include their monosaccharide composition, molecular weight (Mw), chemical components, and bonding information (55). Different plant parts (i.e., the roots, leaves, stems, or seeds), extraction methods, and isolation and purification steps can lead to variations in polysaccharide structure (56). The structural characteristics of polysaccharides derived from different parts of P. frutescens are summarized in Table 2.
Monosaccharide composition is an essential foundation for the structural analysis of polysaccharides. The acid hydrolysis method is used to completely break the glycosidic bonds of polysaccharides; after hydrolysis, the polysaccharide sample is neutralized, filtered, and derivatized for analysis of monosaccharide composition (57). High-performance liquid chromatography (HPLC), gas chromatography-mass spectrometry (GC-MS), gas chromatography (GC), gas-liquid chromatography, and ion chromatography (IC) are common methods employed to measure the monosaccharide composition and molar ratio of polysaccharides (15, 16, 47, 48). Using GC-MS analysis, the monosaccharide composition of a PSMP was determined to comprise rhamnose (Rha), arabinose (Ara), xylose (Xyl), mannose (Man), glucose (Glu), and galactose (Gal) at a mass ratio of 3.196%: 43.901%: 21.956%: 4.244%: 4.706%: 21.997% (18). Using IC analysis, the PFP extracted from the leaves was reported to comprise six major monosaccharides, including Rha, Ara, Gal, Glu, Xyl, and galacturonic acid (GalA), with the Glu and Gal monosaccharides being the most abundant (15). Furthermore, analysis of P. Frutescens polysaccharides extracted from the leaves and purified with DEAE-Toyopearl 650 M and Sephadex G-100 column chromatography showed that the proportions of Ara, Xyl, and Gal were gradually reduced during the progression of purification, whereas the proportion of Man and Glu significantly increased, which ultimately accounted for the major portion of the obtained P. frutescens leaf polysaccharides (16). Subsequent GC analysis showed that the PFB-1-0 fraction consisted of Ara (8.9%), Xyl (0.9%), Man (25.9%), Glu (16.8%), and Gal (24.9%) after DEAE-Toyopearl 650 M column chromatography purification, and the PFB-1-0-ii fraction consisted of Ara (9.6%), Xyl (0.2%), Man (39.8%), Glu (45.6%), and Gal (14.5%) after Sephadex G-100 column chromatography purification.
The Mw not only affects the physical properties of polysaccharides, such as viscosity and solubility, but also affects their biological activities, forming the basis for characterizing the properties of polysaccharides (58). To date, the Mw of P. Frutescens polysaccharide has mainly been analyzed using HPLC and high-performance gel-permeation chromatography (HPGPC) detection techniques (16, 23, 51). Based on the available literature, the Mw of P. frutescens polysaccharide ranges from approximately 1.6 × 103 Da to 1.18 × 106 Da. For example, PFB-1-0-ii obtained from extraction of P. frutescens leaves using HWE and ethanol precipitation, followed by further separation and purification on a DEAE-Toyopearl 650 M column and a Sephadex G-100 chromatographic column, had an Mw of 10 kDa determined by HPGPC (16). By contrast, Niu et al. (52) obtained three main polysaccharide fractions from P. frutescens leaves by HWE, ethanol precipitation, and further purification on a DEAE-52 column with an Mw of 2.2 kDa and 1589.8 Da for PFP-2 and PFP-4, respectively, whereas PFP-3 was mainly composed of two segments with an Mw of 5.2 and 40.0 kDa, respectively, as determined by HPGPC. The difference in Mw among these P. frutescens polysaccharides is mainly attributed to variations in factors such as source, treatment method, and separation equipment. Similar variations in Mw have also been observed in studies on polysaccharides from Bupleuri Radix, and Nelumbo nucifera Gaertn. (lotus), and Radix Hedysari (26, 59, 60).
The biological significance of polysaccharides is intimately associated with their complex structural properties and unique backbone features (47). Therefore, determining the chemical structure of polysaccharides is an important element in the investigation of their pharmacological effects and improvement of their applications (61). Chromatographic techniques, spectroscopic analysis, and other chemical analyses are effective methods for studying the structural characteristics of P. frutescens polysaccharides, including the types and linkages of sugar residues. According to the results of Fourier-transfer infrared spectroscopy (FT-IR), P. frutescens seed meal polysaccharide had five absorption peaks at 3,356 cm−1 (O−H), 2931 cm−1 (C−H), 1658 cm−1 (−C=O and −CHO), 1415 cm−1 (C−O), and 1,317 and 1,245 cm−1 (−COOH). The strong peaks noted at 1,072 and 1,047 cm−1 suggested the presence of galactopyranose and arabinofuranose in the backbone and branches of the sugar chain. GC-MS and one-dimensional nuclear magnetic resonance (NMR) spectrum (1H, 13C) analyses showed that P. frutescens seed meal polysaccharide was composed of β-D-Xyl, α-L-Ara, β-D-Gal, and β-L-Ara sugar residues, and was free of glucuronic acid (18). Moreover, Li et al. (53) obtained a homogenous polysaccharide faction of PFSP-2-1 from P. frutescens seed. Based on FT-IR, IC, methylation, and one-and two-dimensional NMR analysis, the structure of PFSP-2-1 showed a backbone of →1)-Araf-(5→1)-Galp-(6→1)-Galp-(6→1)-Araf-(5→1)-Araf-(5→1)-Galp-(6→1)-Araf-(5→1)-Araf-(5→1)-Araf-(3→1)-Xylp-(4→; and three branches consisting of →1,6)-Galp-(3→1)-Arap, →1,6)-Galp-(3→1,3)-Galp-(6→1)-Araf, →1,6)-Galp-(3→1)-Arap, and →1,3)-Araf-(5→1)-Glcp-(4→1)-Galp-(3→1)-Glcp. The link sites were at position C-3 of →3,6)-Galp-(1→, C-3 of →3,6)-Galp-(1→, and C-5 of →3,5)-Araf-(1→, respectively (Figure 3). Using FT-IR, IC, and NMR spectroscopy, PFP was confirmed to be an acidic polysaccharide with a backbone comprising six sugar residues: α-L-Araf-(1→, →6)-β-D-Galp-(1→, →4)-α-D-Glcp-(1→, →1,4)-β-D-Xylp-(1→, →4)-α-GalpA-(1→, and →2,4)-α-L-Rhap-(1→ (15). However, the detailed chemical structures of polysaccharides from P. frutescens leaf, such as the linkage of sugar residues, have been not reported to date. Therefore, the detailed structures of P. frutescens polysaccharides from different sources and parts of the plant need to be analyzed more deeply and comprehensively to further understand the physicochemical and biological properties of P. frutescens polysaccharides.
Figure 3. Diagram of the planar structure of P. frutescens polysaccharide (PFSP-2-1) (53).
Conformation refers to the three-dimensional structure created by the penetrating bonds formed by the molecular structure of the polymer and the physical force penetrating the space (62). Polysaccharides possess complicated substituents and diversified chemical structures, and their spatial conformation largely determines the various biological functions (63). Scanning electron microscopy (SEM) observations showed that the apparent morphology of polysaccharides obtained from P. frutescens leaves represents a combination of reticulated, lamellar, and chained forms, while the surface of the polysaccharides extracted from the P. frutescens seed meal is dominated by meshes and sheets (20) (Figure 4). Ding (23) carried out a comprehensive characterization of the morphological features of P. frutescens leaf polysaccharides via Congo red staining, circular dichroism, and SEM observations. The results revealed an overall orderly spatial structure of the P. frutescens polysaccharide, without a triple-helix structure, and the surface was smooth and flaky. Zhao et al. (51) similarly found a smooth lamellar structure on the surface of a P. Frutescens polysaccharide extracted from the leaves based on SEM observations.
Figure 4. SEM micrographs of P. frutescens leaf polysaccharides (A: PLP-0.1-I; C; PLP-0.2-I; E: PLP-0.3-I) and P. frutescens seed meal polysaccharides (B: PSMP-0.1-I; D: PSMP-0.2-I; F: PSMP-0.3-I) (20).
P. frutescens exhibits numerous edible and medicinal benefits, and its polysaccharide is an essential component conferring these characteristics. Indeed, P. frutescens polysaccharide possess a variety of biological activities, including antioxidant, antitumor, anti-fatigue, immunoregulation, hepatoprotective, anti-inflammatory, and lipid-lowering effects, which are summarized in Figure 5.
Reactive oxygen species (ROS) are oxygen radicals in biological bodies, consisting of oxygen and oxygenated highly active molecules (e.g., superoxide anion, tissue peroxidation products, and free radicals). Typically, appropriate levels of ROS can facilitate immunity, repair, survival, and growth (64, 65). However, the excessive production of ROS will disrupt the dynamic redox balance, causing the human body to suffer damage, leading to multiple diseases (66). Hence, supplementation of antioxidants exogenously is essential for the body to fight or mitigate oxidative stress damage. P. frutescens polysaccharides demonstrate potential as effective antioxidants. The antioxidant activities of P. frutescens polysaccharides have been evaluated in vitro by examining the potential for scavenging 2,2-diphenyl-1-picrylhydrazyl (DPPH), 2,2′-azino-bis (3-ethylbenzothiazoline-6-sulfonic acid) diammonium salt (ABTS), hydroxyl (•OH), superoxide anion (O2-•), and nitrite ion (NO2-) radicals, along with iron-reduction capacity assays (14, 20, 37). For example, 1,000 μg/mL of a P. frutescens seed meal polysaccharide (PMP) showed scavenging rates on •OH, O2-•, NO2-, and DPPH radicals of 49.70 ± 2.10%, 21.76 ± 1.09%, 60.75 ± 2.19%, and 72.95 ± 4.19%, respectively (67). The scavenging ability of PMP for O2-• showed a certain dose-dependent effect at a polysaccharide mass concentration range of 62.5–1,000 μg/mL. However, Zhu et al. (68) showed that the scavenging rate of P. frutescens seed meal polysaccharide on O2-• radicals exhibited a trend of initial increase followed by a decrease; when the concentration of the polysaccharide reached 1,000 μg/mL, the formation of free radicals was promoted rather than suppressed. The reason for these discrepant effects could be attributed to the different structures and compositions of polysaccharides. Moreover, a PSMP exhibited a 73.83% scavenging rate of •OH radicals at 5,000 μg/mL and a 91.10% scavenging rate of ABTS radicals at 600 μg/mL (18). In general, the high antioxidant activity of polysaccharides is related to the enrichment of uronic acid groups (39). However, no uronic acid was detected in the PSMP, and the antioxidant properties were instead attributed to the presence of Man and Glu (18). Overproduction of ROS induced by oxidative stress also plays a key role in neuronal damage. In H2O2-induced neurotoxic cellular assays, P. frutescens polysaccharide reduced ROS production, lowered malondialdehyde (MDA) levels, increased intracellular superoxide dismutase (SOD) activity, and counteracted H2O2-induced neuronal cell death by activating the PI3K/AKT pathway and negatively regulating the mitogen-activated protein kinase (MAPK) and nuclear factor-kappa B (NF-κB) pathways in HT22 hippocampus cells (14).
Cancer remains a major global public health challenge. In the last few years, polysaccharides have attracted considerable attention in cancer research owing to their reported antitumor activity and low side effects (69). The antitumor effects of P. Frutescens polysaccharides could be attributed to their strong immunomodulatory abilities (15, 17). Treatment of H22 tumor-bearing mice with a P. frutescens leaf polysaccharide (PFP) at doses of 100 mg/kg and 300 mg/kg for three weeks significantly decreased tumor volume and weight and reduced thymic atrophy and splenomegaly in mice in a dose-dependent manner (15). In vitro investigations further showed that the PFP also inhibited tumor proliferation by blocking the S-phase of the cell cycle and decreasing mitochondrial membrane potential. The antitumor effect of PFP may be related to the Gal component. Treatment of tumor-bearing mice with P. frutescens seed polysaccharide (0.1, 0.3, and 0.5 mg for 10 days) significantly reduced the levels of lactate dehydrogenase, aldolase, and interleukin (IL)-10; increased the levels of IL-2 and tumor necrosis factor-alpha (TNF-α) in the serum of mice; and down-regulated the expression of the anti-apoptotic protein Bcl-2 and up-regulated the expression of the pro-apoptotic protein Bax (17). Collectively, these results indicated that P. frutescens seed polysaccharide can inhibit the growth of tumor cells in vivo by enhancing the autoimmunity of mice. Li et al. (53) found that a P. frutescens seed polysaccharide (PFSP-2-1) could significantly inhibit the growth of three types of hepatocellular carcinoma cells (HepG2, Hep3b, and SK-Hep-1), and the inhibitory effect gradually increased with the increase of PFSP-2-1 concentration. It has been hypothesized that Ara and Xyl in PFSP-2-1 are some of the factors inhibiting the growth of liver cancer cells. Moreover, the triple-helix high-level structure of PFSP-2-1 is also an important factor in its anti-tumor activity. In summary, P. frutescens polysaccharides exhibit excellent antitumor properties; however, the therapeutic effects will need to be further investigated on more cancer models along with determination of the conformational relationships.
The liver is a crucial organ with essential functions in regulating metabolism, biotransformation, and detoxification (70). Factors such as autoimmune aggression of liver cells, medication misuse, alcohol consumption, infections with viruses, and cardiovascular disease predispose the liver to injury (71, 72). Several studies have demonstrated that P. frutescens polysaccharides have a hepatoprotective effect, which may be associated with their antioxidant and anti-inflammatory activities (19, 73). Li et al. (29) reported that P. frutescens seed meal polysaccharide administered at doses of 100, 300, and 500 mg/kg for 7 days alleviated CCl4-induced acute liver injury in an animal model. Specifically, the P. frutescens seed meal polysaccharide decreased serum aspartate aminotransferase (AST) and alanine aminotransferase (ALT) activities, as well as liver and spleen coefficients. In a type 2 diabetic rat liver injury model induced by high-fat and high-sugar feeding combined with intraperitoneal injection of streptozotocin, gavage of P. frutescens leaf polysaccharide at 0.15, 0.30, 0.60 g/kg for 28 days significantly reduced the levels of MDA, C-reactive protein, IL-6, TNF-α, and acetylated forkhead transcription factor protein in liver tissues, while the activities of glutathione peroxidase (GSH-Px), catalase (CAT), and SOD as well as the expression of sirtuin 1 (SIRT1) and FoxO1 proteins in liver tissues were significantly elevated (73). Thus, the P. frutescens leaf polysaccharide could improve type 2 diabetes-induced rat liver injury by inhibiting oxidative stress and inflammation, while regulating activation of the SIRT1/FoxO1 signaling pathway. Additionally, Tao et al. (19) found that P. frutescens leaf polysaccharide significantly improved hepatocyte inflammatory cell infiltration, bleeding, and liver steatosis in a mouse model of alcoholic liver injury disease (ALD) induced by chronic ethanol gavage. After gavage of the P. frutescens polysaccharides, the contents of IL-1β, IL-6, TNF-α, and MDA in the liver tissues of ALD mice were significantly decreased; the SOD and GSH-Px activities were significantly increased; and the relative expression levels of p-AMPKα/AMPKα and SIRT1 were significantly increased. These findings suggested that P. frutescens polysaccharide from the leaves might ameliorate liver injury in ALD model mice by modulating the activity of the SIRT1-AMPK signaling pathway in the liver tissues.
Immunomodulation involves recognition of the body’s own and external substances, along with maintenance of the body’s physiological balance via the immune response to external antigens, which plays an essential role in the resistance to infections, tumors, and other diseases (31, 74). Natural polysaccharides play a vital role in immune regulation by stimulating immune cells such as T lymphocytes, B cells, macrophages, and cytotoxic T cells (75). To date, the immunomodulatory activity of P. frutescens polysaccharides has been demonstrated in a variety of in vitro and in vivo models. Kwon et al. (49) obtained a fraction from the crude polysaccharides extracted from P. frutescens leaves (PFB-1) by DEAE-Toyopearl 650 M chromatographic column elution named PFB-1-0, which significantly increased lysosomal enzyme activity as well as nitric oxide and TNF-α levels in mouse peritoneal macrophages. Moreover, PFB-1 could stimulate the production of IL-6 and granulocyte-macrophage colony-stimulating factors in mice. In a corroborative study, Kim et al. (16) showed that the active polysaccharide fractions (PFB-1, PFB-1-0, PFB-1-0-ii) from P. frutescens leaves at a concentration range of 1–100 μg/mL promoted macrophage lysosomal enzyme activity in a concentration-dependent manner. The immunomodulatory activity of these four P. frutescens leaf polysaccharides was attributed to the Man, Gal, and Glu components. Moreover, the high-molecular-weight P. Frutescens polysaccharide exhibited more effective macrophage-stimulating activity than the low-molecular-weight P. Frutescens polysaccharide. PFB-1-0-ii showed the best effect among all polysaccharide fractions, with 100 μg/mL PFB-1-0-ii increasing macrophage lysosomal enzyme activity by 245% in comparison to that of the untreated control. Together, these studies indicate that P. Frutescens polysaccharides have the potential to be developed into drugs and functional foods with novel immunostimulatory activities; however, the interaction mechanism between polysaccharides and the immune system remains unclear, warranting further research.
In addition to the above biological activities, P. Frutescens polysaccharides also contribute to other health dimensions, including anti-fatigue, anti-inflammatory, and lipid-lowering effects. In a mouse model of fatigue induced by exhaustive swimming, gavage of P. Frutescens polysaccharides extracted from the leaves (5, 10, and 20 mg/kg for 4 weeks) significantly increased the forceful swimming time of mice, as well as the blood glucose level, liver glycogen content, and muscle glycogen content after exercise (76). These findings demonstrated a protective effect of the P. Frutescens polysaccharide in accelerating the removal of certain fatigue-causing metabolic substances, maintaining the normal physiological function of cells, and delaying fatigue. Wang et al. (77) tested the effectiveness of P. Frutescens leaf polysaccharide as an anti-inflammatory agent in a rat model of smoking combined with lipopolysaccharide-induced chronic obstructive pulmonary disease (COPD); treatment with 20 mg/kg P. Frutescens polysaccharide for 4 weeks in vivo resulted in improvement of lung function in COPD model rats. P. Frutescens polysaccharide treatment significantly decreased the expression levels of IL-8, TNF-α, Ras homologous protein A, Wnt5a, and p-JNK; reduced the thickness of bronchial wall and smooth muscle; and improved lung histopathologic changes. The inhibitory effect of P. Frutescens leaf polysaccharide on inflammation in COPD rats was mainly achieved by inhibiting the Wnt/PCP signaling pathway. In addition, Liu et al. (78) demonstrated the ameliorative effects of a P. frutescens seed polysaccharides (PFSP) on abnormal lipid metabolism and oxidative stress induced by a high-fat diet in mice. Supplementation of the PFSP (50, 100, and 200 mg/kg) in the feed for 8 weeks significantly decreased the levels of AST, ALT, triglyceride, total cholesterol, low-density lipoprotein, blood glucose, MDA, and the mRNA and protein expression levels of fatty acid synthesis-related genes. By contrast, PFSP supplementation increased the levels of high-density lipoprotein, fatty acid catabolism-related genes (CPT-1, ATGL) mRNA and protein expression, and the activities of SOD, GSH-Px, and CAT. Collectively, PFSP ameliorated high-fat diet-induced oxidative stress and fatty liver in mice. Based on the above research, the detailed biological effects or mechanisms of P. Frutescens polysaccharides are summarized in Table 2.
Perilla frutescens is a highly promising edible and medicinal homologous resource; as the main components of P. frutescens, the polysaccharides of this herb have received increasing research attention in recent years. In this review, the extraction, purification, structural characterization, and associated biological activities of P. Frutescens polysaccharides have been summarized. Despite the remarkable advances achieved in P. Frutescens polysaccharide research, there are still several opportunities and challenges that remain to be resolved (Table 3).
Table 3. Summary of biological activities of polysaccharides from P. frutescens (“↓,” decrease; “↑,” increase).
First, studies have been carried out to extract P. Frutescens polysaccharides by HWE, MAE, UAE, enzyme-assisted extraction, and UAEE. There is still a great deal of scope for the development of other extraction methods. In particular, efforts should be focused on developing new environmentally friendly and high-yield extraction technologies. Second, the isolation and purification methods of P. Frutescens polysaccharides are currently limited to the laboratory level; thus, these methods should be further optimized to obtain reproducible and stable products that are suitable for industrial-scale production. Third, investigations on the structure of P. Frutescens polysaccharides have primarily focused on the monosaccharide composition and molecular weight. However, research is lacking on the more detailed structures of the sequence of monosaccharides, types, and positions of glycosidic bonds, and structural fragments. Various classical approaches, including FT-IR, NMR, X-ray diffraction, atomic force microscopy, and methylation analysis, can be applied to characterize the chemical structure of P. Frutescens polysaccharides. Fourth, to date, researchers have mostly focused on the antioxidant activity of P. Frutescens polysaccharides, with fewer studies on other bioactivities and their relationship with P. Frutescens polysaccharides structure. Therefore, it is imperative to establish more appropriate pharmacological models to investigate the additional biological activities of P. Frutescens polysaccharides and further study the structure-activity relationships of the polysaccharide, as well as the exact molecular mechanisms underlying the observed activities. Finally, research on P. Frutescens polysaccharides is still limited to theoretical studies in animal and in vitro experiments, with a notable lack of relevant clinical, toxicity, and pharmacokinetic studies. Developing and applying P. Frutescens polysaccharides as nutraceuticals or natural medicines is a huge challenge. Hence, a large number of clinical trials should be carried out to ensure the efficacy and safety of P. Frutescens polysaccharides toward creating novel products with P. Frutescens polysaccharides as the active ingredient.
In summary, P. Frutescens polysaccharides possess diverse biological functions, which offer extensive prospects in the fields of food and medicine; however, there is still a long way to go to achieve the transformation of P. Frutescens polysaccharides into practical functional foods, nutraceuticals, or natural medicines. We hope that more researchers will pay attention to PFP in the future and further investigate its precise structure, active mechanism, and clinical utilization, to offer a scientific foundation for its further development and utilization as functional foods and therapeutic drugs.
LZ: Validation, Writing – original draft, Writing – review & editing. LG: Writing – review & editing. KW: Conceptualization, Writing – review & editing. CR: Data curation, Writing – review & editing. YG: Methodology, Writing – review & editing. JL: Investigation, Software, Writing – review & editing. SY: Project administration, Writing – review & editing. XZ: Formal analysis, Writing – review & editing. XY: Resources, Writing – review & editing. YZ: Methodology, Writing – review & editing. BL: Writing – review & editing. SL: Writing – review & editing.
The author(s) declare financial support was received for the research, authorship, and/or publication of this article. This work was supported by the National Key Research and Development Program of China (2021YFD2100902) and the Agricultural Science and Technology Innovation Project of Heilongjiang Academy of Agricultural Sciences (CX23GG16-04).
The authors declare that the research was conducted in the absence of any commercial or financial relationships that could be construed as a potential conflict of interest.
All claims expressed in this article are solely those of the authors and do not necessarily represent those of their affiliated organizations, or those of the publisher, the editors and the reviewers. Any product that may be evaluated in this article, or claim that may be made by its manufacturer, is not guaranteed or endorsed by the publisher.
1. Adam, G, Robu, S, Flutur, MM, Cioanca, O, Vasilache, IA, Adam, AM, et al. Applications of Perilla frutescens extracts in clinical practice. Antioxidants. (2023) 12:727. doi: 10.3390/antiox12030727
2. Sa, KJ, Jang, SJ, Lee, S, Park, H, Cho, J, Sung, J, et al. Characterization of volatile compounds of Perilla crop (Perilla Frutescens L.) in South Korea. Appl Biol Chem. (2023) 66:1–13. doi: 10.1186/s13765-023-00801-6
3. Tavan, M, Hanachi, P, Mirjalili, MH, and Dashtbani-Roozbehani, A. Comparative assessment of the biological activity of the green synthesized silver nanoparticles and aqueous leaf extract of Perilla Frutescens (L.). Sci Rep. (2023) 13:6391. doi: 10.1038/s41598-023-33625-x
4. Wang, P, Jin, B, Lian, C, Guo, K, and Ma, C. Comparative analysis of polycyclic aromatic hydrocarbons and halogenated polycyclic aromatic hydrocarbons in different parts of Perilla Frutescens (L.) Britt. Molecules. (2022) 14:738–54. doi: 10.3390/molecules14020738
5. Zhou, XJ, Yan, LL, Yin, PP, Shi, LL, Zhang, JH, Liu, YJ, et al. Structural characterisation and antioxidant activity evaluation of phenolic compounds from cold-pressed Perilla frutescens Var Arguta seed flour. Food Chem. (2014) 164:150–7. doi: 10.1016/j.foodchem.2014.05.062
6. Wang, R, Zhang, Q, Feng, C, Zhang, J, Qin, Y, Meng, L, et al. Advances in the pharmacological activities and effects of Perilla ketone and Lsoegomaketone. Evid Based Complement Alternat Med. (2022) 2022:1–10. doi: 10.1155/2022/8809792
7. Chen, J, Zhang, D, Wang, Q, Yang, A, Zheng, Y, and Wang, L. Comprehensive comparison of two color varieties of Perillae folium by Gc-Ms-based Metabolomic approach. Molecules. (2022) 27:6792. doi: 10.3390/molecules27206792
8. Ethnomedicinal, AH. Phytochemical and pharmacological investigations of Perilla frutescens (L.) Britt. Molecules. (2018) 24:102. doi: 10.3390/molecules24010102
9. Wu, X, Dong, S, Chen, H, Guo, M, Sun, Z, and Luo, H. Perilla frutescens: a traditional medicine and food homologous plant. Chin Herb Med. (2023) 15:369–75. doi: 10.1016/j.chmed.2023.03.002
10. Fujiwara, Y, Kono, M, Ito, A, and Ito, M. Anthocyanins in Perilla plants and dried leaves. Phytochemistry. (2018) 147:158–66. doi: 10.1016/j.phytochem.2018.01.003
11. Ahmed, HM, Al-Zubaidy, MA, and Othman-Qadir, G. Biological investigations on macro-morphological characteristics, polyphenolic acids, antioxidant activity of Perilla Frutescens (L) Britt. Grown under open field. Saudi J Biol Sci. (2022) 29:3213–22. doi: 10.1016/j.sjbs.2022.01.059
12. Hou, T, Netala, VR, Zhang, H, Xing, Y, Li, H, and Zhang, Z. Perilla frutescens: a rich source of pharmacological active compounds. Molecules. (2022) 27:3578. doi: 10.3390/molecules27113578
13. Huang, S, Nan, Y, Chen, G, Ning, N, Du, Y, Lu, D, et al. The role and mechanism of Perilla frutescens in Cancer treatment. Molecules. (2023) 28:5883. doi: 10.3390/molecules28155883
14. Byun, EB, Cho, EJ, Kim, YE, Kim, WS, and Byun, EH. Neuroprotective effect of polysaccharide separated from Perilla frutescens Britton Var. Acuta kudo against H2O2-induced oxidative stress in HT22 Hippocampus cells. Biosci Biotechnol Biochem. (2018) 82:1344–58. doi: 10.1080/09168451.2018.1460572
15. Ding, SY, Yan, ZQ, Liu, HP, Chen, P, Shi, SY, and Chang, ML. Structural characterization and antitumor activity of a polysaccharide extracted from Perilla frutescens Var. frutescens. Ind Crop Prod. (2022) 187:115334. doi: 10.1016/j.indcrop.2022.115334
16. Kim, YJ, Hwang, JS, and Kwon, KH. Extraction, purification and macrophage stimulatory activity of polysaccharide isolated from leaves of Perilla frutescens Britton Var. Crispa. Prog Nutr. (2019) 21:399–405. doi: 10.23751/pn.v21i1-S.5525
17. Liu, Z, Yin, H, Yang, A, An, Q, Li, C, and Jin, T. Separation, purification and anti-tumor activity of polysaccharides from Perilla frutescens seeds. Food Sci. (2022) 43:158–65. doi: 10.7506/spkx1002-6630-20210708-087
18. Zhang, H, Li, H, Zhang, Z, and Hou, T. Optimization of ultrasound-assisted extraction of polysaccharides from Perilla seed meal by response surface methodology: characterization and in vitro antioxidant activities. J Food Sci. (2021) 86:306–18. doi: 10.1111/1750-3841.15597
19. Tao, YD, Tian, HJ, Li, Y, and Luo, XY. Protective effect of Perilla frutescens polysaccharides on alcoholic liver injury in mice. Northwest J Pharm. (2022) 37:51–6. doi: 10.3969/j.issn.1004-2407.2022.02.010
20. Zhang, HJ. Isolation, purification, structural characterization and application of Perilla polysaccharides. North Univ China. (2022). doi: 10.27470/d.cnki.ghbgc.2022.000026
21. Li, X, Chen, C, Leng, A, and Qu, J. Advances in the extraction, purification, structural characteristics and biological activities of Eleutherococcus senticosus polysaccharides: a promising medicinal and edible resource with development value. Front Pharmacol. (2021) 12:753007. doi: 10.3389/fphar.2021.753007
22. Zhou, QC, and Sheng, GH. Pyrolytic and kinetic characteristics of the thermal decomposition of Perilla frutescens polysaccharide. PLoS One. (2012) 7:e52597. doi: 10.1371/journal.pone.0052597
23. Ding, SY. Study on extraction, structure characterization and anti-tumor activity of polysaccharide from Perilla frutescens var. frutescens. Tianjin Univ Sci Technol. (2022). doi: 10.27359/d.cnki.gtqgu.2022.000729
24. Ke, L, Duan, X, Cui, J, Song, X, Ma, W, Zhang, W, et al. Research Progress on the extraction technology and activity study of Epimedium polysaccharides. Carbohydr Polym. (2023) 306:120602. doi: 10.1016/j.carbpol.2023.120602
25. Jing, Y, Yan, M, Liu, D, Tao, C, Hu, B, Sun, S, et al. Research Progress on the structural characterization, biological activity and product application of polysaccharides from Crataegus pinnatifida. Int J Biol Macromol. (2023) 244:125408. doi: 10.1016/j.ijbiomac.2023.125408
26. Yu, X, Miao, Z, Zhang, L, Zhu, L, and Sheng, H. Extraction, purification, structure characteristics, biological activities and pharmaceutical application of Bupleuri Radix polysaccharide: a review. Int J Biol Macromol. (2023) 237:124146. doi: 10.1016/j.ijbiomac.2023.124146
27. Ahmad, MM, Chatha, SAS, Iqbal, Y, Hussain, AL, Khan, I, and Xie, F. Recent trends in extraction, purification, and antioxidant activity evaluation of plant leaf-extract polysaccharides. Biofuels Bioprod Biorefin. (2022) 16:1820–48. doi: 10.1002/bbb.2405
28. Yang, Y, Lei, Z, Zhao, M, Wu, C, Wang, L, and Xu, Y. Microwave-assisted extraction of an acidic polysaccharide from Ribes nigrum L.: structural characteristics and biological activities. Ind Crop Prod. (2020) 147:112249. doi: 10.1016/j.indcrop.2020.112249
29. Li, C, Song, Y, and Sun, Q. Extraction of polysaccharide from Perilla frutescens seed with microwave and its Hepatoprotective activity. Chin Agri Sci Bulltin. (2014) 30:285–90. Available at: https://webofscience.clarivate.cn/wos/alldb/full-record/CSCD:5104335.
30. Zhang, LH. Study on the extraction and antioxidant activity of Perilla leaf polysaccharide. Fujian Agriculture and Forestry University (2013). Available at: https://kns.cnki.net/kcms2/article/abstract?v=WVDzDAe5jxaZxI7s-UrUg1EFJDlNVIOZad9BlVOQ_KdRsAsAqovyqBv7NoiYVTVMRNmI0sAlr-tKmZM2bPA4RbM21-yk-TmxSioaGb9hRYyOwde0BGzqXbLzWZmQQOQp0Cy-Z8IBKsliJqiLw1CLJA==&uniplatform=NZKPT&language=CHS.
31. Luo, L, Xue, J, Shao, Z, Zhou, Z, Tang, W, Liu, J, et al. Recent developments in Salvia miltiorrhiza polysaccharides: isolation, purification, structural characteristics and biological activities. Front Pharmacol. (2023) 14:1139201. doi: 10.3389/fphar.2023.1139201
32. Kumar, M, Hasan, M, Sharma, A, Suhag, R, Maheshwari, C, Radha,, et al. Tinospora cordifolia (Willd.) Hook.F. & Thomson polysaccharides: a review on extraction, characterization, and bioactivities. Int J Biol Macromol. (2023) 229:463–75. doi: 10.1016/j.ijbiomac.2022.12.181
33. Lv, CX, Li, MM, Xu, XM, Li, JR, Zhu, SM, and Liu, RR. Optimization of Cellulase extraction process of Perilla frutescens polysaccharides by response surface analysis. Food Chem. 34:6–10.
34. Li, H, Zhang, H, Zhang, Z, and Cui, L. Optimization of ultrasound-assisted enzymatic extraction and in vitro antioxidant activities of polysaccharides extracted from the leaves of Perilla frutescens. Food Sci Technol. (2020) 40:36–45. doi: 10.1590/fst.29518
35. Zhang, H, Li, H, Netala, VR, Hou, T, and Zhang, Z. Optimization of complex enzyme-ultrasonic synergistic extraction of water-soluble polysaccharides from Perilla frutescens seed meal: purification, characterization and in vitro antioxidant activity. J Food Process Preserv. (2022) 46:e16201. doi: 10.1111/jfpp.16201
36. Gong, H, Gan, X, Li, Y, Chen, J, Xu, Y, Shi, S, et al. Review on the genus Polygonatum polysaccharides: extraction, purification, structural characteristics and bioactivities. Int J Biol Macromol. (2023) 229:909–30. doi: 10.1016/j.ijbiomac.2022.12.320
37. Keltoum, AK, Lilia, B, Selma, N, Khaoula, QD, Nora, G, and Dalila, B. Anti-inflammatory and antioxidant activity of the hot water-soluble polysaccharides from Anacyclus pyrethrum (L.) lag. Roots. J Ethnopharmacol. (2021) 281:114491. doi: 10.1016/j.jep.2021.114491
38. Chen, F, Huang, S, and Huang, G. Preparation, activity, and antioxidant mechanism of Rice bran polysaccharide. Food Funct. (2021) 12:834–9. doi: 10.1039/d0fo02498h
39. Qin, D, Han, S, Liu, M, Guo, T, Hu, Z, Zhou, Y, et al. Polysaccharides from Phellinus Linteus: a systematic review of their extractions, purifications, structures and functions. Int J Biol Macromol. (2023) 230:123163. doi: 10.1016/j.ijbiomac.2023.123163
40. Li, B, and Huang, G. Preparation, structure-function relationship and application of Grifola umbellate polysaccharides. Ind Crop Prod. (2022) 186:115282. doi: 10.1016/j.indcrop.2022.115282
41. Li, J, and Huang, G. Extraction, purification, separation, structure, derivatization and activities of polysaccharide from Chinese date. Process Biochem. (2021) 110:231–42. doi: 10.1016/j.procbio.2021.08.018
42. Tang, W, Liu, D, Yin, J, and Nie, S. Consecutive and progressive purification of food-derived natural polysaccharide: based on material, extraction process and crude polysaccharide. Trends Food Sci Technol. (2020) 99:76–87. doi: 10.1016/j.tifs.2020.02.015
43. Ai, X, Yu, P, Li, X, Lai, X, Yang, M, Liu, F, et al. Polysaccharides from Spirulina platensis: extraction methods, structural features and bioactivities diversity. Int J Biol Macromol. (2023) 231:123211. doi: 10.1016/j.ijbiomac.2023.123211
44. Ji, X, Peng, Q, Yuan, Y, Shen, J, Xie, X, and Wang, M. Isolation, structures and bioactivities of the polysaccharides from jujube fruit (Ziziphus Jujuba mill.): a review. Food Chem. (2017) 227:349–57. doi: 10.1016/j.foodchem.2017.01.074
45. Ren, Y, Bai, Y, Zhang, Z, Cai, W, and Flores, DRA. The preparation and structure analysis methods of natural polysaccharides of plants and Fungi: a review of recent development. Molecules. (2019) 24:3122. doi: 10.3390/molecules24173122
46. Ji, X, Hou, C, Shi, M, Yan, Y, and Liu, Y. An insight into the research concerning Panax ginseng C. A. Meyer polysaccharides: a review. Food Rev Intl. (2020) 38:1149–65. doi: 10.1080/87559129.2020.1771363
47. Liu, H, Wei, S, Shi, L, and Tan, H. Preparation, structural characterization, and bioactivities of polysaccharides from Psidium guajava: a review. Food Chem. (2023) 411:135423. doi: 10.1016/j.foodchem.2023.135423
48. Borjigin, G, Wei, F, Jiang, S, Li, Q, and Yang, C. Extraction, purification, structural characterization and biological activity of polysaccharides from Fritillaria: a review. Int J Biol Macromol. (2023) 242:124817. doi: 10.1016/j.ijbiomac.2023.124817
49. Kwon, KH, Kim, KI, Jun, WJ, Shin, DH, Cho, EJ, and Hong, BS. In vitro and in vivo effects of macrophage-stimulatory polysaccharide from leaves of Perilla frutescens Var. crispa. Biol Pharm Bull. (2002) 25:367–71. doi: 10.1081/SCC-120023425
50. Liang, LX, Huang, XC, and Huang, XT. An Experimenton using ultrasonic cleaning machine and Cellulase to extract polysaccharides from Perilla leaves and their antioxidant activity. Farm Prod Process. (2022) 14:27–32. doi: 10.16693/j.cnki.1671-9646(X).2022.07.036
51. Zhao, YN, Guo, JT, Zhou, TT, Li, MH, and Li, HZ. Modification of Perilla frutescens polysaccharides with selenic acid and analysis of their physicochemical structural characteristics. Food Chem. (2023) 44:1–9. doi: 10.7506/spkx1002-6630-20230206-048
52. Niu, XR, Zhu, TT, Gao, ZJ, Han, CY, Liu, EW, Han, LF, et al. Separation and purification of Perilla frutescens polysaccharides and their antioxidant activities in vitro. Food Res Dev. (2023) 44:30–5. doi: 10.12161/j.issn.1005-6521.2023.09.005
53. Li, H, Liu, M, Liu, Z, Cheng, L, Li, M, and Li, C. Purification, structural characterization, and antitumor activity of a polysaccharide from Perilla seeds. Int J Mol Sci. (2023) 24:15904. doi: 10.3390/ijms242115904
54. Hu, YC, Hu, JL, Li, J, Wang, J, Zhang, X, Wu, X, et al. Physicochemical characteristics and biological activities of soluble dietary fibers isolated from the leaves of different quinoa cultivars. Food Res Int. (2023) 163:112166. doi: 10.1016/j.foodres.2022.112166
55. Luan, F, Ji, Y, Peng, L, Liu, Q, Cao, H, Yang, Y, et al. Extraction, purification, structural characteristics and biological properties of the polysaccharides from Codonopsis pilosula: a review. Carbohydr Polym. (2021) 261:117863. doi: 10.1016/j.carbpol.2021.117863
56. Xue, T, Ruan, K, Tang, Z, Duan, J, and Xu, H. Isolation, structural properties, and bioactivities of polysaccharides from Althaea officinalis Linn.: a review. Int J Biol Macromol. (2023) 242:125098. doi: 10.1016/j.ijbiomac.2023.125098
57. Zhou, W, Zhou, X, Wang, Y, Ling, Y, Li, P, and Zhang, F. Research Progress on pretreatment and analysis methods for polysaccharides in traditional Chinese medicine. J Instrum Anal. (2020) 39:1168–75. doi: 10.3969/j.issn.1004-4957.2020.09.017
58. Li, Y, Liang, J, Gao, JN, Shen, Y, Kuang, HX, and Xia, YG. A novel Lc-Ms/Ms method for complete composition analysis of polysaccharides by Aldononitrile acetate and multiple reaction monitoring. Carbohydr Polym. (2021) 272:118478. doi: 10.1016/j.carbpol.2021.118478
59. Wang, M, Hu, W, Wang, Q, Yang, B, and Kuang, HX. Extraction, purification, structural characteristics, biological activities, and application of the polysaccharides from Nelumbo nucifera Gaertn. (Lotus): a review. Int J Biol Macromol. (2023) 226:562–79. doi: 10.1016/j.ijbiomac.2022.12.072
60. Mo, X, Guo, D, Jiang, Y, Chen, P, and Huang, L. Isolation, structures and bioactivities of the polysaccharides from Radix Hedysari: a review. Int J Biol Macromol. (2022) 199:212–22. doi: 10.1016/j.ijbiomac.2021.12.095
61. Zeng, J, Luan, F, Hu, J, Liu, Y, Zhang, X, Qin, T, et al. Recent research advances in polysaccharides from Undaria pinnatifida: isolation, structures, bioactivities, and applications. Int J Biol Macromol. (2022) 206:325–54. doi: 10.1016/j.ijbiomac.2022.02.138
62. Ain, NU, Wu, S, Li, X, Li, D, and Zhang, Z. Isolation, characterization, pharmacology and biopolymer applications of licorice polysaccharides: review. Materials. (2022) 15:3654. doi: 10.3390/ma15103654
63. Kirschner, KN, and Woods, RJ. Solvent interactions determine carbohydrate conformation. Proc Natl Acad Sci USA. (2001) 98:10541–5. doi: 10.1073/pnas.191362798
64. McCord, JM. The evolution of free radicals and oxidative stress. Am J Med. (2000) 108:652–9. doi: 10.1016/S0002-9343(00)00412-5
65. Reczek, CR, and Chandel, NS. Ros-dependent signal transduction. Curr Opin Cell Biol. (2015) 33:8–13. doi: 10.1016/j.ceb.2014.09.010
66. Yu, Y, Shen, M, Song, Q, and Xie, J. Biological activities and pharmaceutical applications of polysaccharide from natural resources: a review. Carbohydr Polym. (2018) 183:91–101. doi: 10.1016/j.carbpol.2017.12.009
67. Wang, SB, Kong, LX, and Liu, JJ. In vitro antioxidant activity of Perilla frutescens meal polysaccharides and their protective effect on oxidative damage of liver cells. China Oils Fats. (2023):1–10. doi: 10.19902/j.cnki.zgyz.1003-7969.230284
68. Zhu, JF, Deng, YX, Ran, YL, Tang, CH, and Yu, HB. Study on the antioxidant activity of Perilla frutescens meal polysaccharide Pwps. J Chongqing Technol Business Univ. (2012) 29:83–6. Available at: https://kns.cnki.net/kcms2/article/abstract?v=-0THPtffOh0_SnYDmMVQ8kiE_ofJsTLPVJ0q1lGa95LY0Q5H8s3kCArkCfAZLm8UZ0ujvFasoqEQuQrgFoIsk_Hz4ePbls3el25FIveZi5CeWIqLk0zA0SrCFvtQ_i2sMsD9IciG664=&uniplatform=NZKPT&language=CHS.
69. Li, N, Wang, C, Georgiev, MI, Bajpai, VK, Tundis, R, Simal-Gandara, J, et al. Advances in dietary polysaccharides as anticancer agents: structure-activity relationship. Trends Food Sci Technol. (2021) 111:360–77. doi: 10.1016/j.tifs.2021.03.008
70. Chen, S, Guan, X, Yong, T, Gao, X, Xiao, C, Xie, Y, et al. Structural characterization and Hepatoprotective activity of an acidic polysaccharide from Ganoderma lucidum. Food Chem X. (2022) 13:100204. doi: 10.1016/j.fochx.2022.100204
71. Zhang, Y, Yao, L, Liu, Y, Chen, B, Wang, C, Gong, K, et al. Acidic polysaccharide from corn silk: structural & conformational properties and hepatoprotective activity. Int J Biol Macromol. (2023) 236:123851. doi: 10.1016/j.ijbiomac.2023.123851
72. Qu, J, Huang, P, Zhang, L, Qiu, Y, Qi, H, Leng, A, et al. Hepatoprotective effect of plant polysaccharides from natural resources: a review of the mechanisms and structure-activity relationship. Int J Biol Macromol. (2020) 161:24–34. doi: 10.1016/j.ijbiomac.2020.05.196
73. Sun, GP, Yuan, L, Fang, XL, and Yang, HB. Study on the role and mechanism of Perilla frutescens polysaccharide in ameliorating liver injury in type 2 diabetic rats. J Chin Med Mater. (2020) 43:037. doi: 10.13863/j.issn1001-4454.2020.11.037
74. Cai, W, Wong, K, and Huang, Q. Isolation, structural features, rheological properties and bioactivities of polysaccharides from Lignosus rhinocerotis: a review. Int J Biol Macromol. (2023) 242:124818. doi: 10.1016/j.ijbiomac.2023.124818
75. Wen, Y, Peng, D, Li, C, Hu, X, Bi, S, Song, L, et al. A new polysaccharide isolated from morchella importuna fruiting bodies and its immunoregulatory mechanism. Int J Biol Macromol. (2019) 137:8–19. doi: 10.1016/j.ijbiomac.2019.06.171
76. Liu, SH. Protective effect of Perilla frutescens leaf polysaccharides on exercise induced fatigue in mice induced by exhaustive swimming. Heilongjiang Sci Technol Inform. (2015) 35:115–6. Available at: https://kns.cnki.net/kcms2/article/abstract?v=-0THPtffOh0M530Ke8zt7uKdmrdDcgcln3WKuV8TmojW6hGLDTFKO-gYXc2x3WO9-qgX7IATfRXhVi4ky-doS0ZQMnzs8JhBAIVt9e4rdCIMW8VOglDS29LKBpdKOtS3NmBnym0CS_a_iTurFvJlNw==&uniplatform=NZKPT&language=CHS.
77. Wang, WJ, Liu, XM, and Zhang, GQ. Effect of Perilla leaf polysaccharide on airway inflammation and airway remodeling in COPD rats through Wnt/Pcp pathway. Chin Med Guide. (2021) 27:37–41. doi: 10.13862/j.cnki.cn43-1446/r.2021.10.007
78. Liu, M, Zhang, L, Wang, D, and Wang, CW. Effects of Perilla frutescens seeds polysaccharide on lipid metabolism and antioxidants in mice fed high-fat diet. Food Res Dev. (2022) 43:70–7. doi: 10.12161/j.issn.1005-6521.2022.18.010
Keywords: Perilla frutescens , polysaccharides, extraction, structural characterization, bioactivities
Citation: Zhu L, Guan L, Wang K, Ren C, Gao Y, Li J, Yan S, Zhang X, Yao X, Zhou Y, Li B and Lu S (2024) Recent trends in extraction, purification, structural characterization, and biological activities evaluation of Perilla frutescens (L.) Britton polysaccharide. Front. Nutr. 11:1359813. doi: 10.3389/fnut.2024.1359813
Received: 22 December 2023; Accepted: 13 February 2024;
Published: 22 February 2024.
Edited by:
Saša Đurović, Institute of General and Physical Chemistry, SerbiaReviewed by:
Gu Haofeng, Ankang University, ChinaCopyright © 2024 Zhu, Guan, Wang, Ren, Gao, Li, Yan, Zhang, Yao, Zhou, Li and Lu. This is an open-access article distributed under the terms of the Creative Commons Attribution License (CC BY). The use, distribution or reproduction in other forums is permitted, provided the original author(s) and the copyright owner(s) are credited and that the original publication in this journal is cited, in accordance with accepted academic practice. No use, distribution or reproduction is permitted which does not comply with these terms.
*Correspondence: Lijun Guan, cXFhaXBwaGhAc2luYS5jb20=; Shuwen Lu, c2h1d2VubHVAc2luYS5jb20=
Disclaimer: All claims expressed in this article are solely those of the authors and do not necessarily represent those of their affiliated organizations, or those of the publisher, the editors and the reviewers. Any product that may be evaluated in this article or claim that may be made by its manufacturer is not guaranteed or endorsed by the publisher.
Research integrity at Frontiers
Learn more about the work of our research integrity team to safeguard the quality of each article we publish.