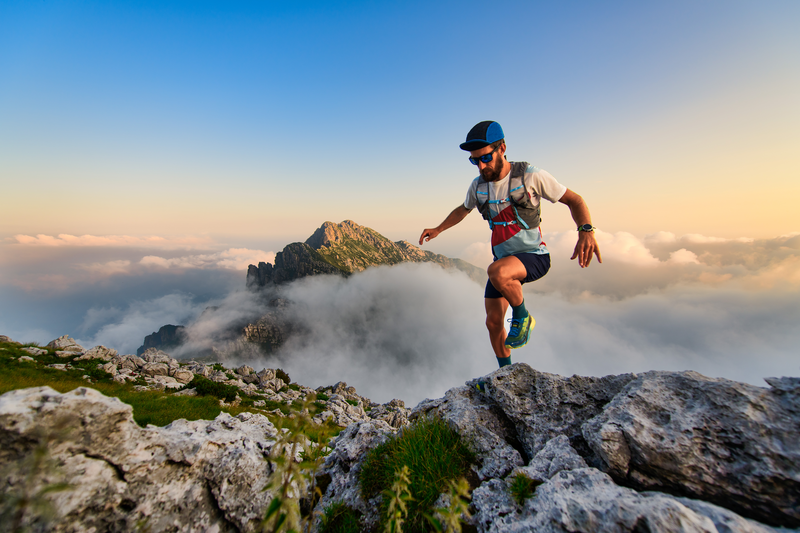
94% of researchers rate our articles as excellent or good
Learn more about the work of our research integrity team to safeguard the quality of each article we publish.
Find out more
SYSTEMATIC REVIEW article
Front. Nutr. , 11 August 2023
Sec. Nutrition and Metabolism
Volume 10 - 2023 | https://doi.org/10.3389/fnut.2023.1117387
A correction has been applied to this article in:
Corrigendum: Remarkable impacts of probiotics supplementation in enhancing of the antioxidant status: results of an umbrella meta-analysis
Introduction: Numerous meta-analyses have demonstrated the beneficial effects of probiotics on oxidative stress biomarkers, although some studies have contradictory results. Therefore, the current research was conducted to obtain a precise and definite understanding on the impact of probiotics on oxidative stress biomarkers in adults.
Methods: We conducted a comprehensive systematic search of results on Scopus, PubMed, Embase, Web of Science, and Google Scholar dating up to March 2022. Fifteen meta-analyses were included in this umbrella meta-analysis. The random-effects model was employed to obtain the overall effect size. Subgroup analyses were carried out based on supplementation dosage and duration, mean age, and study population.
Results: Our results indicated that probiotics supplementation meaningfully decreased serum malondialdehyde (MDA) (ESWMD = −0.56, 95% CI: −0.72, −0.39; p < 0.001, and ESSMD = −0.50, 95% CI: −0.66, −0.34; p < 0.001). Moreover, the findings showed that probiotics resulted in a significant increase in total antioxidant capacity (TAC) (ESWMD = 29.18, 95% CI: 16.31, 42.04; p < 0.001, and ESSMD = 0.25, 95% CI: 0.02, 0.47; p = 0.032), total glutathione (GSH) (ESWMD: 30.65; 95% CI: 16.94, 44.35, p < 0.001), and nitric oxide (NO) (ESWMD: 1.48; 95% CI: 0.31, 2.65, p = 0.013; I2 = 51.7%, p = 0.043).
Discussion: Probiotics could be considered a strong agent in the reinforcement of antioxidant status and preventing the incidence of chronic diseases.
The interaction of oxygen with certain molecules can cause the formation of highly reactive atoms named free radicals with unpaired electrons in their external shell, which can behave as oxidants (1). An imbalance between the reactive oxygen species (ROS), including superoxide (O2–), hydroxyl (OH–), and hydrogen peroxide (H2O2), and the body antioxidant defense system is defined as oxidative stress. Oxidative stress has been associated with a wide range of non-communicable and chronic diseases such as cardiovascular diseases, diabetes, Alzheimer's disease, cancer, and chronic obstructive pulmonary disease (2–4).
Intake of antioxidant vitamin supplementation, such as A, C, and E, as well as polyphenols and fruits and vegetables, is a common strategy to reinforce the antioxidant defense system (5–7). Nevertheless, evidence of a positive effect of probiotics in reducing oxidative stress and related diseases is also growing (5, 8). According to the World Health Organization (WHO) definition, probiotics are living microorganisms with certain benefits for human health when administered in a suitable amount (9). Certain strains of microorganisms can exhibit probiotic properties. For a strain to be called a “probiotic”, it must meet a number of requirements regarding safety, functionality, and technical suitability. The safety profile is determined based on strain origin, level of antibiotic resistance, and lack of association with pathogenic strains. Lactobacillus, Bifidobacterium, Lactococcus, Streptococcus, and Enterococcus species are the probiotic microorganisms mainly used in humans (10–14). More than 500 different bacteria reside in an adult human gastrointestinal tract as a source of probiotics, and many of the probiotic species used today have been isolated from the human gut. Besides the human GIT, dairy and dairy-related products, such as fermented milk and kefir, and non-dairy fermented substrates, such as meat and fruits, are good sources of probiotics (15–17).
Gut dysbiosis, which is the condition of the abnormal predominance of pathogenic over non-pathogenic microorganisms, is one of the confirmed causes of oxidative stress in the body (18, 19). Studies have demonstrated the beneficial role of probiotics in reconstruction of intestinal microbiota through various mechanisms including the maintenance of intestinal homeostasis (20).
Several studies have evaluated the effect of probiotic supplementation on a variety of disorders, and review studies have reported different results of probiotic effects. Several studies revealed no significant improvement in total antioxidant capacity (TAC) (21, 22) and malondialdehyde (MDA) after probiotic administration (23, 24), while in some other studies probiotics intake resulted in significant improvement in serum levels, TAC, total glutathione (GSH), and MDA (25, 26). Due to the reported contradictory results, we conducted the present umbrella meta-analysis to investigate the overall effect of probiotics on oxidative stress biomarkers in adults.
The Preferred Reporting Items for Systematic Reviews and Meta-Analyses (PRISMA) statement guidelines were used to develop the present umbrella meta-analysis. The protocol of this study has been registered in the international prospective register of systematic reviews (PROSPERO) under number CRD42023399865.
The international scientific databases of PubMed, Scopus, Web of Science, Cochrane Central Library, and EMBASE were searched for results dating up to March 2022. The following search pattern was utilized to explore related articles: “Probiotics” [Mesh] OR “probiotics” [All Fields] OR “probiotic” [All Fields] OR “Saccharomyces” [Title/Abstract] OR “Lactobacillus” [Title/Abstract] OR “Bifidobacterium” [Title/Abstract] OR “Lactobacillus casei” [Title/Abstract] OR “Bifidobacterium bifidum” [Title/Abstract] OR “Lactobacillus fermentum” [Mesh] OR “bifidobacterium” [All Fields] AND “Oxidative Stress” [MeSH terms] OR “Oxidative Stress” [Title/Abstract] OR “Total Antioxidant Capacity” [Title/Abstract] OR “antioxidant” [Title/Abstract] OR “Oxidant” [Title/Abstract] OR “reactive oxygen species” [Title/Abstract] OR “Malondialdehyde” [Title/Abstract] OR “glutathione” [Title/Abstract] OR “TAC” [Title/Abstract] OR “GSH” [Title/Abstract] OR “MDA” [Title/Abstract] OR “Nitric Oxide” [MeSH terms] AND “systematic review” [Publication Type] OR “meta-analysis” [Title/Abstract]. The wild-card term “*” was utilized to boost the sensitivity of the search method. The articles were limited to those written in the English language.
The PICO criteria for the present umbrella meta-analysis were as follows: Population/Patients (P): adults, 18 years old or above; Intervention (I): probiotics; Comparison (C): control or placebo group; Outcome (O): stress oxidative biomarkers including nitric oxide (NO), GSH, TAC, and MDA. Meta-analysis studies investigating the impact of probiotics supplementation on stress oxidative biomarkers and providing effect sizes and corresponding confidence intervals (CI) for each outcome were included in the present study. We excluded in vitro and in vivo studies, observational studies, case reports, quasi-experimental studies, and controlled clinical trials.
Two independent reviewers (VM and FHK) examined the methodological quality of the included studies by the AMSTAR questionnaire (27). The AMSTAR questionnaire consists of 11 questions, and 11 is the maximum possible score. Articles with a score of 7 or higher were considered of good quality.
The screening and inclusion process of the studies based on the eligibility criteria were conducted by VM and FMK as two independent reviewers. We reviewed the abstracts and titles of the studies in the first step. Then, the full text of included studies was evaluated to determine the eligibility of the studies. Any disagreements were resolved by the senior author's decision (MZ). The name of the first author, year of the publication, sample size, intervention duration, study location, probiotics dosage, effect size [weighted mean difference (WMD) and standardized mean difference (SMD)], and confidence intervals (CIs) for NO, MDA, TAC, and GSH were extracted from the selected articles.
We calculated the overall effect size by pooling the effect size and CI of each included study. The analysis was performed separately for SMD and WMD due to their natural differences. The analysis was performed using the random-effects model in case of a high-heterogeneity amount, and the fixed-effects model was employed in case that the amount of heterogeneity was low. The I2 statistic and Cochran's Q test were used to determine between-study heterogeneity. Significant heterogeneity was considered as follows: I2 value >50% or P < 0.1 for the Q test. To recognize the probable sources of heterogeneity, subgroup analysis was performed according to the dose of probiotics, study population, sample size, and duration of intervention. We conducted a sensitivity analysis to determine whether the overall effect size was dependent on a particular study. The publication bias was evaluated using the Begg's and Egger's tests and funnel plot evaluation. In case of the presence of publication bias, trim-and-fill analysis was used to modify the publication bias. Egger's test and funnel plot evaluation were not performed when the total number of observations for each outcome was <10. All of the statistical analyses were performed using Stata software version 16.0 (Stata Corporation, College Station, TX, US). A p-value lower than 0.05 was considered significant.
A total number of 302 articles were obtained from the systematic search, among which 240 were thoroughly reviewed by titles and abstracts after 62 duplicate articles were excluded. Eventually, 29 articles were chosen for full-text examination, among which 15 meta-analyses were included in the umbrella meta-analysis. The PRISMA flowchart (Figure 1) represents the study selection process. The study participants' age ranged from 29 to 79, and the included studies were conducted between 2018 and 2021. The duration of interventions ranged from 6 to 14 weeks. The average dosage of probiotics in the current study varied from 1 × 1010 to 8 × 1010 CFU.
The studies were performed in Iran (23, 25, 26, 28–30), China (21, 22, 31–34), Malaysia (24), Egypt (35), and Brazil (36). The quality of trials included in the meta-analyses was assessed by the Cochrane Risk of Bias Tool (37) and Jadad scores (38), and almost all RCTs included in the meta-analyses were of high quality (Table 1). The characteristics of the studies qualified for this umbrella meta-analysis and the quality assessment results for the RCTs qualified in the meta-analyses are outlined in Table 1.
A total of 6 of the 21 meta-analyses were rated as high quality, 13 as moderate quality, and 2 as low quality. Detailed results are presented in Table 2.
Eleven meta-analyses that included 2,605 participants revealed significant reduction in MDA levels (ES WMD = −0.56, 95% CI: −0.72, −0.39; p < 0.001) (Figure 2A). Inter-study heterogeneity was found to be significant (I2 = 791%; p < 0.001). The subgroup analysis revealed that probiotic supplementation among subjects under 50 years of age with T2DM and a dosage of <0.4 × 1010 CFU substantially reduced MDA levels (Table 3). According to the sensitivity analysis, the removal of any of the studies did not affect the overall effect size estimate. Small-study effect was not detected using Egger's and Begg's tests (p = 0.896 and 0.999, respectively). Publication bias was also not identified through visual assessment of the funnel plot (Supplementary Figure S1).
Figure 2. Forest plot with mean difference and 95% confidence intervals (CIs), and the effects of probiotics supplementation on MDA levels according to WMD (A) and SMD (B) analysis.
Our analysis findings based on four meta-analyses revealed that probiotic supplementation considerably decreased MDA levels (ESSMD= −0.50, 95% CI: −0.66, −0.34; p < 0.001), with no considerable between-studies heterogeneity (I2 = 0.0%; p = 0.552) (Figure 2B). The overall effect size was not affected by removing any study following the sensitivity analysis. Begg's test revealed no evidence of publication bias (p = 0.471).
The pooled estimate of meta-analyses revealed significant improvement in the TAC levels following probiotics supplementation (ESWMD = 29.18, 95% CI: 16.31, 42.04; p < 0.001); (I2 = 13.1%; p = 0.328) (Figure 3A). Subgroup analysis also indicated a significant impact of probiotics on TAC levels in studies with T2DM patients and a dosage of <0.4 × 1010 CFU (Table 3). In the sensitivity analysis, omitting each study did not substantially alter the pooled effect size for TAC. No publication bias was detected following Begg's test (p = 0.266).
Figure 3. Forest plot with mean difference and 95% confidence intervals (CIs), and the effects of probiotics supplementation on TAC levels according to WMD (A) and SMD (B) analysis.
The overall analysis of the data from five studies demonstrated that probiotics supplementation significantly increased TAC levels (ESSMD = 0.25, 95% CI: 0.02, 0.47; p = 0.032) (Figure 3B). However, no significant degree of heterogeneity existed (I2 = 55.4%, p = 0.062). The pooled effect size did not alter when each study was excluded from the sensitivity analysis. No publication bias was found by Begg's test (p = 0.998).
The results of the pooled analysis demonstrated that probiotics had a significant increase in GSH levels (ESWMD: 30.65; 95% CI: 16.94, 44.35, p < 0.001) (Figure 4A). There was no significant between-study heterogeneity (I2 = 24.5%, p = 0.218). Probiotics supplementation in studies with T2DM patients, a dosage of <0.4 × 1010 CFU, and an intervention duration of ≥10 weeks led to a remarkable increase in the GSH level compared to other subgroups (Table 3). The pooled effect size was not affected by the exclusion of any individual study using sensitivity analysis. No small-study effect was detected by Egger's and Begg's tests (p = 0.614 and 0.858, respectively). The funnel plot (Supplementary Figure S2) likewise did not reveal an uneven distribution of studies.
Figure 4. Forest plot with mean difference and 95% confidence intervals (CIs), and the effects of probiotics supplementation on GSH levels according to WMD (A) and SMD (B) analysis.
Of the included studies, three studies reported the effect of probiotic supplementation on GSH levels with 1,122 participants. The results demonstrated that probiotic supplementation led to no meaningful increase in the GSH level (ESSMD: 0.23; 95% CI: −0.01, 0.48, p = 0.061) (Figure 4B). However, heterogeneity among the studies was high (I2 = 64.0%, p = 0.062). As a result of Begg's test, no publication bias was identified (p = 0.296).
The results indicated the meaningful effect of probiotics supplementation on NO levels (ESWMD: 1.48; 95% CI: 0.31, 2.65, p = 0.013; I2 = 51.7%, p = 0.043) (Figure 5A). Probiotics supplementation in the context of a dosage of <0.4 × 1010 CFU, mean age of <50 years, and duration of intervention of <10 weeks was greater than the overall results (Table 3). In the sensitivity analysis, any single study excluded did not impact the overall effect size. The finding of Begg's test was not significant in respect to detected publication bias (p = 0.618).
Figure 5. Forest plot with mean difference and 95% confidence intervals (CIs), and the effects of probiotics supplementation on NO levels according to WMD (A) and SMD (B) analysis.
Probiotics supplementation had no significant impact on NO levels (ESSMD: 0.02; 95% CI: −0.32, 0.36, p = 0.921; I2 = 48.7%, p = 0.119) (Figure 5B). The sensitivity analysis did not influence the pooled effect size by excluding any particular study. There was no evidence of significant publication bias (p = 0.999 for Begg's test).
According to our umbrella analysis, probiotics had improving effects on oxidative stress status and antioxidant biomarkers. Examining SMD and WMD showed that probiotics significantly improved antioxidant and oxidative stress biomarkers in both examinations, resulting in the ineffectiveness of standard deviation in the final result. WMD depends on the weight of each study (39). Therefore, the greater improving effect size in the WMD estimation was not surprising.
Lower dosages (<0.4 × 1010 CFU) of probiotics led to a maximum decrease in MDA and increase in GSH, TAC, and NO levels. Shorter (<10-week) and longer (≥10-week) durations of probiotic supplementation had the most improving effects on oxidative stress and antioxidant status, respectively. The beneficial effect of probiotics on MDA in the short term may be due to its improving effect on SOD activity in the early stages of oxidative stress. MDA is a secondary lipid peroxidation product generated by the oxidation of arachidonic acid and larger PUFAs (40). Superoxide dismutase (SOD) is the first line of defense against oxidation (41). However, due to the limited number of studies on SOD, it was not included in our analysis. Roshan et al., in a meta-analysis study, revealed that probiotics have an improving effect on SOD activity (42). GSH is involved in the next steps against oxidative stress, with the neutralization of H2O2 produced by SOD activity (41). Therefore, longer durations of probiotics are needed to affect GSH as well. TAC is not a specific measure and only presents the total status of antioxidant capacity. TAC does not evaluate SOD, glutathione peroxidase (GPx), and catalase activity (43). Theoretically, as a total antioxidant index, a longer duration of supplementation may be needed to affect TAC. However, due to the limited number of studies of short durations, performing subgroup analysis was not possible. NO acts as a double-edged sword in the mechanism of oxidative stress. A decrease in its synthesis is associated with endothelial dysfunction and subsequent thrombosis, vasospasm, vascular inflammation, and proliferation of vascular smooth muscle cells. On the other hand, increasing the production of free radicals in oxidative stress due to reaction with NO causes the production of peroxynitrite, which contributes to vascular oxidative stress (44, 45). Therefore, proper bioavailability of NO along with increasing antioxidant capacity and reducing the production of free radicals following probiotic supplementation can lead to the improvement of endothelial dysfunction and inflammation. In terms of administered dosage, a safety assessment of probiotics revealed that a low dose of probiotics may have more preventing usages than high dosages (46).
Patients with glucose intolerance have been shown to benefit more from the antioxidant effects of probiotic supplementation. One of the underlying factors in the development of diabetes mellitus is oxidative stress. Hyperglycemia through the polyol pathway, auto-oxidation, and increased production of advanced glycation end products (AGEs) contributes to the elevation of oxidative stress (47). Probiotic supplementation has also been demonstrated to exert antioxidant effects in patients with chronic kidney disease (CKD) and autoimmune diseases. The decrease of NO bioavailability and inflammation are the causes of oxidative stress in CKD patients. Moreover, the loss of antioxidant vitamins through the dialysis process and the production of ROS on the surface of dialysis membranes by the activation of neutrophils lead to oxidative stress in these patients (48). Oxidative stress through post-translational modifications of proteins is involved in the breaking of immunological tolerance and subsequent autoimmune reactions (49).
Various underlying mechanisms have been proposed for the association between probiotics and the antioxidant defense system. One is their ability to chelate metal ions. Cell-free supernatants of lactic acid bacteria (LAB) strains have been shown to exhibit the ability to chelate with metal ions such as ferrous and cupric ions (50–52). Moreover, probiotics have their own antioxidant system including SOD and catalase (53). LeBlanc et al. reported that mice with Crohn's disease receiving SOD and catalase-producing LAB had an increased antioxidant capacity in the gut (54). Probiotics also produce various metabolites related to the antioxidant system including GSH, butyrate, and B vitamins. Folate is important in the efficiency of DNA replication, repair, and methylation (55). Numerous studies have reported that various probiotic strains including LAB and Bifidobacteria are able to produce folate and enhance its status in human and animal models (56–58). B. longum and B. bifidum have been shown to be able to produce thiamin (59); moreover, L. fermentum can produce riboflavin (60). Furthermore, the ability of some strains in the production of vitamin B12 has been shown in studies (61–63). Elevation of the homocysteine level as the main consequence of B-vitamins deficiency is the cause of oxidative stress in this condition (64). Results on fat-soluble vitamins are limited, and an exact conclusion cannot be obtained (65). Kullisaar et al. reported that Lactobacillus fermentum E-3 and E-18 had significant levels of GSH (66). In addition, a whole GSH system was found in Lactobacillus fermentum ME-3 (67). The beneficial effect of sodium butyrate on the oxidative status through the partial activation of nuclear factor-erythroid factor 2-related factor 2 (Nrf2)-dependent genes has been reported in an in vivo investigation (68). Butyrate is a short-chain fatty acid (SCFA) whose production by Clostridium butyricum was studied in an in vivo model (69). In addition, probiotics can regulate signaling pathways related to antioxidant responses including the induction of Nrf2-Keap1-ARE (70, 71), mitogen-activated protein kinases (MAPKs) (72), and protein kinase C (PKC) (72), as well as the inhibition of nuclear factor kappa-B (NFκB) (73). Kelch-like ECH-associated protein 1 (Keap-1) is a molecular switcher that activates Nrf2 when cells are counteracted with free radicals. Subsequently, Nrf2 increases the expression of antioxidant enzymes and detoxifying proteins by binding to the antioxidant response element (ARE) (74). NFκB is the main transcription factor involved in the regulation of inflammatory pathways (75). Both MAPKs and PKC are the enzymes involved in the signaling pathways leading to the regulation of cell growth. Probiotic-secretory proteins through the PKC- and MAPK-dependent mechanism protect intestinal integrity (72). Another possible antioxidant aspect of probiotics is their inhibitory effect on enzymes producing free radicals, including the NADPH oxidase (NOX) complex (76), cyclo-oxygenase (COX) (77), and cytochrome P450 (CYP) enzymes (78).
In terms of bias, 11 of included studies reported that the majority of their analyzed clinical trials had a low risk of bias. Moreover, 5 and 8 of the 15 included systematic review studies had a high and moderate risk of bias, respectively. Therefore, our obtained results can be almost reliable. However, there are some limitations to our study. First, due to the limited number of studies, subgroup analysis based on study duration on TAC was not performed. Second, due to the presence of a wide range of health conditions, subgroup analysis based on diseases other than diabetes mellitus was not possible. This problem also applies to different strains of probiotics.
Probiotics in low dosages can be considered as antioxidant agents. Shorter (<10-week) and longer (≥10-week) durations of probiotic supplementation have the most improving effects on oxidative stress and antioxidant status, respectively. Patients with different health conditions such as T2DM, CKD, and autoimmune diseases can benefit from probiotic supplementation.
The original contributions presented in the study are included in the article/Supplementary material, further inquiries can be directed to the corresponding authors.
VM and MZ designed research. AF and VM conducted the systematic search. MZ, AP, and FH-K screened the articles. FH-K, AJ, and PM extracted the data. MZ analyzed and interpreted the data. AJ and FH-K drew the tables. MZ, VM, and AF wrote the paper. ZG had primary responsibility for the final content. All authors read and approved the final manuscript.
The research protocol was approved and supported by the Student Research Committee, Tabriz University of Medical Sciences (Registration code: 70114).
The authors declare that the research was conducted in the absence of any commercial or financial relationships that could be construed as a potential conflict of interest.
All claims expressed in this article are solely those of the authors and do not necessarily represent those of their affiliated organizations, or those of the publisher, the editors and the reviewers. Any product that may be evaluated in this article, or claim that may be made by its manufacturer, is not guaranteed or endorsed by the publisher.
The Supplementary Material for this article can be found online at: https://www.frontiersin.org/articles/10.3389/fnut.2023.1117387/full#supplementary-material
Supplementary Figures. The results of funnel plot for the effect of probiotics on oxidative stress.
1. Chandrasekaran A, Idelchik MD, Melendez JA. Redox control of senescence and age-related disease. Redox Biol. (2017) 11:91–102. doi: 10.1016/j.redox.2016.11.005
2. Brigelius-Flohé R. Tissue-specific functions of individual glutathione peroxidases. Free Radical Biol Med. (1999) 27:951–65. doi: 10.1016/S0891-5849(99)00173-2
3. Jones DP. Redefining oxidative stress. Antioxid Redox Signal. (2006) 8:1865–79. doi: 10.1089/ars.2006.8.1865
4. Valko M, Leibfritz D, Moncol J, Cronin MT, Mazur M, Telser J. Free radicals and antioxidants in normal physiological functions and human disease. Int J Biochem Cell Biol. (2007) 39:44–84. doi: 10.1016/j.biocel.2006.07.001
5. Poljsak B. Strategies for reducing or preventing the generation of oxidative stress. Oxid Med Cell Longev. (2011) 2011:194586. doi: 10.1155/2011/194586
6. Zabłocka A, Janusz M. The two faces of reactive oxygen species. Advn Hygiene Exper Med. (2008) 62:118–24. doi: 10.1007/978-1-84628-782-4_12
7. Zhang X, Tao N, Wang X, Chen F, Wang M. The colorants, antioxidants, and toxicants from nonenzymatic browning reactions and the impacts of dietary polyphenols on their thermal formation. Food Funct. (2015) 6:345–55. doi: 10.1039/C4FO00996G
8. Vasquez EC, Pereira T, Peotta VA, Baldo MP, Campos-Toimil M. Probiotics as beneficial dietary supplements to prevent and treat cardiovascular diseases: uncovering their impact on oxidative stress. Oxid Med Cell Longev. (2019) 2019:3086270. doi: 10.1155/2019/3086270
9. Hill C, Guarner F, Reid G, Gibson GR, Merenstein DJ, Pot B, et al. Expert consensus document: The International Scientific Association for Probiotics and Prebiotics consensus statement on the scope and appropriate use of the term probiotic. Nat Rev Gastroenterol Hepatol. (2014) 11:506. doi: 10.1038/nrgastro.2014.66
10. Dudek-Wicher R, Junka A, Paleczny J, Bartoszewicz M. Clinical trials of probiotic strains in selected disease entities. Int J Microbiol. (2020) 2020:8854119. doi: 10.1155/2020/8854119
11. Haghshenas B, Kiani A, Nami Y. Probiotic potential and safety evaluation of lactic acid bacteria isolated from colostrum. J Biosafety. (2021) 14:37–60.
12. Nami Y, Haghshenas B, Khosroushahi AY. Molecular identification and probiotic potential characterization of lactic acid bacteria isolated from human vaginal microbiota. Adv Pharmaceut Bull. (2018) 8:683. doi: 10.15171/apb.2018.077
13. Nami Y, Haghshenas B, Yari Khosroushahi A. Effect of psyllium and gum Arabic biopolymers on the survival rate and storage stability in yogurt of Enterococcus durans IW 3 encapsulated in alginate. Food Sci Nutr. (2017) 5:554–63. doi: 10.1002/fsn3.430
14. Nami Y, Kiani A, Elieh-Ali-Komi D, Jafari M, Haghshenas B. Impacts of alginate–basil seed mucilage–prebiotic microencapsulation on the survival rate of the potential probiotic Leuconostoc mesenteroides ABRIINW. N18 in yogurt. Int J Dairy Technol. (2023) 76:138–48. doi: 10.1111/1471-0307.12909
15. Fontana L, Bermudez-Brito M, Plaza-Diaz J, Munoz-Quezada S, Gil A. Sources, isolation, characterisation and evaluation of probiotics. Br J Nutr. (2013) 109:S35–50. doi: 10.1017/S0007114512004011
16. Kiani A, Nami Y, Hedayati S, Elieh Ali Komi D, Goudarzi F, Haghshenas B. Application of Tarkhineh fermented product to produce potato chips with strong probiotic properties, high shelf-life, and desirable sensory characteristics. Front Microbiol. (2021) 12:657579. doi: 10.3389/fmicb.2021.657579
17. Kiani A, Nami Y, Hedayati S, Jaymand M, Samadian H, Haghshenas B. Tarkhineh as a new microencapsulation matrix improves the quality and sensory characteristics of probiotic Lactococcus lactis KUMS-T18 enriched potato chips. Sci Rep. (2021) 11:12599. doi: 10.1038/s41598-021-92095-1
18. Bellavia M, Tomasello G, Romeo M, Damiani P, Lo Monte AI, Lozio L, et al. Gut microbiota imbalance and chaperoning system malfunction are central to ulcerative colitis pathogenesis and can be counteracted with specifically designed probiotics: a working hypothesis. Med Microbiol Immunol. (2013) 202:393–406. doi: 10.1007/s00430-013-0305-2
19. Prakash S, Rodes L, Coussa-Charley M, Tomaro-Duchesneau C. Gut microbiota: next frontier in understanding human health and development of biotherapeutics. Biologics. (2011) 5:71–86. doi: 10.2147/BTT.S19099
20. Wilkins T, Sequoia J. Probiotics for gastrointestinal conditions: a summary of the evidence. Am Fam Physician. (2017) 96:170–8.
21. Den H, Dong X, Chen M, Zou Z. Efficacy of probiotics on cognition, and biomarkers of inflammation and oxidative stress in adults with Alzheimer's disease or mild cognitive impairment—A meta-analysis of randomized controlled trials. Aging (Albany NY). (2020) 12:4010. doi: 10.18632/aging.102810
22. Chan KY, Wong MMH, Pang SSH, Lo KKH. Dietary supplementation for gestational diabetes prevention and management: A meta-analysis of randomized controlled trials. Arch Gynecol Obstet. (2021) 303:1381–91. doi: 10.1007/s00404-021-06023-9
23. Rudbane SMA, Rahmdel S, Abdollahzadeh SM, Zare M, Bazrafshan A, Mazloomi SM. The efficacy of probiotic supplementation in rheumatoid arthritis: a meta-analysis of randomized, controlled trials. Inflammopharmacology. (2018) 26:67–76. doi: 10.1007/s10787-017-0436-y
24. Hasain Z, Roos NAC, Rahmat F, Mustapa M, Ali RAR, Mokhtar NM. Diet and pre-intervention washout modifies the effects of probiotics on gestational diabetes mellitus: a comprehensive systematic review and meta-analysis of randomized controlled trials. Nutrients. (2021) 13:3045. doi: 10.3390/nu13093045
25. Ardeshirlarijani E, Tabatabaei-Malazy O, Mohseni S, Qorbani M, Larijani B, Baradar Jalili R. Effect of probiotics supplementation on glucose and oxidative stress in type 2 diabetes mellitus: A meta-analysis of randomized trials. DARU J Pharmac Sci. (2019) 27:827–37. doi: 10.1007/s40199-019-00302-2
26. Bohlouli J, Namjoo I, Borzoo-Isfahani M, Kermani MA, Zehi ZB, Moravejolahkami AR. Effect of probiotics on oxidative stress and inflammatory status in diabetic nephropathy: A systematic review and meta-analysis of clinical trials. Heliyon. (2021) 7:e05925. doi: 10.1016/j.heliyon.2021.e05925
27. Shea BJ, Grimshaw JM, Wells GA, Boers M, Andersson N, Hamel C, et al. Development of AMSTAR: a measurement tool to assess the methodological quality of systematic reviews. BMC Med Res Methodol. (2007) 7:1–7. doi: 10.1186/1471-2288-7-10
28. Amirani E, Milajerdi A, Mirzaei H, Jamilian H, Mansournia MA, Hallajzadeh J, et al. The effects of probiotic supplementation on mental health, biomarkers of inflammation and oxidative stress in patients with psychiatric disorders: A systematic review and meta-analysis of randomized controlled trials. Complement Ther Med. (2020) 49:102361. doi: 10.1016/j.ctim.2020.102361
29. Tamtaji OR, Milajerdi A, Reiner Ž, Asemi Z, Dadgostar E, Heidari-Soureshjani R, et al. A systematic review and meta-analysis: the effects of probiotic supplementation on metabolic profile in patients with neurological disorders. Complement Ther Med. (2020) 53:102507. doi: 10.1016/j.ctim.2020.102507
30. Zamani B, Sheikhi A, Namazi N, Larijani B, Azadbakht L. The effects of supplementation with probiotic on biomarkers of oxidative stress in adult subjects: A systematic review and meta-analysis of randomized trials. Probiotics Antimicrob Proteins. (2020) 12:102–11. doi: 10.1007/s12602-018-9500-1
31. Zhang J, Ma S, Wu S, Guo C, Long S, Tan H. Effects of probiotic supplement in pregnant women with gestational diabetes mellitus: a systematic review and meta-analysis of randomized controlled trials. J Diab Res. (2019) 2019. doi: 10.1155/2019/5364730
32. Chen Y, Yue R, Zhang B, Li Z, Shui J, Huang X. Effects of probiotics on blood glucose, biomarkers of inflammation and oxidative stress in pregnant women with gestational diabetes mellitus: A meta-analysis of randomized controlled trials. Med Clínica. (2020) 154:199–206. doi: 10.1016/j.medcli.2019.05.041
33. Jiang J, Chu C, Wu C, Wang C, Zhang C, Li T, et al. Efficacy of probiotics in multiple sclerosis: a systematic review of preclinical trials and meta-analysis of randomized controlled trials. Food Funct. (2021) 12:2354–77. doi: 10.1039/D0FO03203D
34. Wang H, Wang D, Song H, Zou D, Feng X, Ma X, et al. The effects of probiotic supplementation on renal function, inflammation, and oxidative stress in diabetic nephropathy: a systematic review and Meta-analysis of randomized controlled trials. Mater Express. (2021) 11:1122–31. doi: 10.1166/mex.2021.1888
35. AbdelQadir YH, Hamdallah A, Sibaey EA, Hussein AS, Abdelaziz M, AbdelAzim A, et al. Efficacy of probiotic supplementation in patients with diabetic nephropathy: a systematic review and meta-analysis. Clin Nutr ESPEN. (2020) 40:57–67. doi: 10.1016/j.clnesp.2020.06.019
36. Krüger JF, Hillesheim E, Pereira ACSN, Camargo CQ, Rabito EI. Probiotics for dementia: a systematic review and meta-analysis of randomized controlled trials. Nutr Rev. (2021) 79:160–70. doi: 10.1093/nutrit/nuaa037
37. Higgins JP, Altman DG, Gøtzsche PC, Jüni P, Moher D, Oxman AD, et al. The Cochrane Collaboration's tool for assessing risk of bias in randomised trials. BMJ. (2011) 18:343. doi: 10.1136/bmj.d5928
38. Clark HD, Wells GA, Huët C, McAlister FA, Salmi LR, Fergusson D, et al. Assessing the quality of randomized trials: reliability of the Jadad scale. Control Clin Trials. (1999) 20:448–52. doi: 10.1016/S0197-2456(99)00026-4
39. Andrade C. Mean difference, standardized mean difference (SMD), and their use in meta-analysis: as simple as it gets. J Clin Psychiatry. (2020) 81:11349. doi: 10.4088/JCP.20f13681
40. Ayala A, Muñoz MF, Argüelles S. Lipid peroxidation: production, metabolism, and signaling mechanisms of malondialdehyde and 4-hydroxy-2-nonenal. Oxid Med Cell Longev. (2014) 2014:360438–360438. doi: 10.1155/2014/360438
41. Abd El HA. Lipid peroxidation end-products as a key of oxidative stress: effect of antioxidant on their production and transfer of free radicals. in Lipid peroxidation. IntechOpen (2012).
42. Roshan H, Ghaedi E, Rahmani J, Barati M, Najafi M, Karimzedeh M, et al. Effects of probiotics and synbiotic supplementation on antioxidant status: A meta-analysis of randomized clinical trials. Clinical Nutrition ESPEN. (2019) 30:81–8. doi: 10.1016/j.clnesp.2019.02.003
43. Rubio CP, Hernández-Ruiz J, Martinez-Subiela S, Tvarijonaviciute A, Ceron JJ. Spectrophotometric assays for total antioxidant capacity (TAC) in dog serum: an update. BMC Vet Res. (2016) 12:166. doi: 10.1186/s12917-016-0792-7
44. Lubos E, Handy DE, Loscalzo J. Role of oxidative stress and nitric oxide in atherothrombosis. Front Biosci. (2008) 13:5323–44. doi: 10.2741/3084
45. Förstermann U. Nitric oxide and oxidative stress in vascular disease. Pflugers Arch. (2010) 459:923–39. doi: 10.1007/s00424-010-0808-2
46. Li X-Q, Zhu Y-H, Zhang H-F, Yue Y, Cai Z-X, Lu Q-P, et al. Risks associated with high-dose Lactobacillus rhamnosus in an Escherichia coli model of piglet diarrhoea: intestinal microbiota and immune imbalances. PLoS ONE. (2012) 7:e40666–e40666. doi: 10.1371/journal.pone.0040666
47. Tangvarasittichai S. Oxidative stress, insulin resistance, dyslipidemia and type 2 diabetes mellitus. World J Diabetes. (2015) 6:456–80. doi: 10.4239/wjd.v6.i3.456
48. Kao MPC, Ang DSC, Pall A, Struthers AD. Oxidative stress in renal dysfunction: mechanisms, clinical sequelae and therapeutic options. J Hum Hypertens. (2010) 24:1–8. doi: 10.1038/jhh.2009.70
49. Smallwood MJ, Nissim A, Knight AR, Whiteman M, Haigh R, Winyard PG. Oxidative stress in autoimmune rheumatic diseases. Free Radical Biol Med. (2018) 125:3–14. doi: 10.1016/j.freeradbiomed.2018.05.086
50. Lee J, Hwang KT, Chung MY, Cho DH, Park CS. Resistance of Lactobacillus casei KCTC 3260 to reactive oxygen species (ROS): role for a metal ion chelating effect. J Food Sci. (2005) 70:m388–91. doi: 10.1111/j.1365-2621.2005.tb11524.x
51. Lin MY, Yen CL. Antioxidative ability of lactic acid bacteria. J Agric Food Chem. (1999) 47:1460–6. doi: 10.1021/jf981149l
52. Ahire JJ, Mokashe NU, Patil HJ, Chaudhari BL. Antioxidative potential of folate producing probiotic Lactobacillus helveticus CD6. J Food Sci Technol. (2013) 50:26–34. doi: 10.1007/s13197-011-0244-0
53. Kleniewska P, Hoffmann A, Pniewska E, Pawliczak R. The Influence of Probiotic Lactobacillus casei in Combination with Prebiotic Inulin on the Antioxidant Capacity of Human Plasma. Oxid Med Cell Longev. (2016) 2016:1340903–1340903. doi: 10.1155/2016/1340903
54. LeBlanc JG, del Carmen S, Miyoshi A, Azevedo V, Sesma F, Langella P, et al. Use of superoxide dismutase and catalase producing lactic acid bacteria in TNBS induced Crohn's disease in mice. J Biotechnol. (2011) 151:287–93. doi: 10.1016/j.jbiotec.2010.11.008
55. Duthie SJ. Folate and cancer: how DNA damage, repair and methylation impact on colon carcinogenesis. J Inherit Metab Dis. (2011) 34:101–9. doi: 10.1007/s10545-010-9128-0
56. Rossi M, Amaretti A, Raimondi S. Folate production by probiotic bacteria. Nutrients. (2011) 3:118–34. doi: 10.3390/nu3010118
57. Pompei A, Cordisco L, Amaretti A, Zanoni S, Raimondi S, Matteuzzi D, et al. Administration of folate-producing bifidobacteria enhances folate status in Wistar rats. J Nutr. (2007) 137:2742–6. doi: 10.1093/jn/137.12.2742
58. Strozzi GP, Mogna J. Quantification of folic acid in human feces after administration of Bifidobacterium probiotic strains. J Clin Gastroenterol. (2008) 42:S179–84. doi: 10.1097/MCG.0b013e31818087d8
59. Magnúsdóttir S, Ravcheev D, de Crécy-Lagard V, Thiele I. Systematic genome assessment of B-vitamin biosynthesis suggests co-operation among gut microbes. Front Genet. (2015) 6:148. doi: 10.3389/fgene.2015.00148
60. Cárdenas N, Laiño JE, Delgado S, Jiménez E, Valle MJ, de Giori GS, et al. Relationships between the genome and some phenotypical properties of Lactobacillus fermentum CECT 5716, a probiotic strain isolated from human milk. Appl Microbiol Biotechnol. (2015) 99:4343–53. doi: 10.1007/s00253-015-6429-0
61. De Angelis M, Bottacini F, Fosso B, Kelleher P, Calasso M, Di Cagno R, et al. Lactobacillus rossiae, a vitamin B12 producer, represents a metabolically versatile species within the Genus Lactobacillus. PLoS ONE. (2014) 9:e107232–e107232. doi: 10.1371/journal.pone.0107232
62. Li P, Gu Q, Yang L, Yu Y, Wang Y. Characterization of extracellular vitamin B(12) producing Lactobacillus plantarum strains and assessment of the probiotic potentials. Food Chem. (2017) 234:494–501. doi: 10.1016/j.foodchem.2017.05.037
63. Fang H, Kang J, Zhang D. Microbial production of vitamin B(12): a review and future perspectives. Microb Cell Fact. (2017) 16:15–15. doi: 10.1186/s12934-017-0631-y
64. Bito T, Misaki T, Yabuta Y, Ishikawa T, Kawano T, Watanabe F. Vitamin B12 deficiency results in severe oxidative stress, leading to memory retention impairment in Caenorhabditis elegans. Redox Biol. (2017) 11:21–9. doi: 10.1016/j.redox.2016.10.013
65. Barkhidarian B, Roldos L, Iskandar MM, Saedisomeolia A, Kubow S. Probiotic supplementation and micronutrient status in healthy subjects: a systematic review of clinical trials. Nutrients. (2021) 13:3001. doi: 10.3390/nu13093001
66. Kullisaar T, Zilmer M, Mikelsaar M, Vihalemm T, Annuk H, Kairane C, et al. Two antioxidative lactobacilli strains as promising probiotics. Int J Food Microbiol. (2002) 72:215–24. doi: 10.1016/S0168-1605(01)00674-2
67. Kullisaar T, Songisepp E, Aunapuu M, Kilk K, Arend A, Mikelsaar M, et al. Complete glutathione system in probiotic Lactobacillus fermentum ME-3. Prikl Biokhim Mikrobiol. (2010) 46:527–31. doi: 10.1134/S0003683810050030
68. Ma N, Abaker JA, Bilal MS Dai H, Shen X. Sodium butyrate improves antioxidant stability in sub-acute ruminal acidosis in dairy goats. BMC Vet Res. (2018) 14:275. doi: 10.1186/s12917-018-1591-0
69. Endo H, Niioka M, Kobayashi N, Tanaka M, Watanabe T. Butyrate-producing probiotics reduce nonalcoholic fatty liver disease progression in rats: new insight into the probiotics for the gut-liver axis. PLoS ONE. (2013) 8:e63388. doi: 10.1371/journal.pone.0063388
70. Wang LX, Liu K, Gao DW, Hao JK. Protective effects of two Lactobacillus plantarum strains in hyperlipidemic mice. World J Gastroenterol. (2013) 19:3150. doi: 10.3748/wjg.v19.i20.3150
71. Gao D, Gao Z, Zhu G. Antioxidant effects of Lactobacillus plantarum via activation of transcription factor Nrf2. Food Funct. (2013) 4:982–9. doi: 10.1039/c3fo30316k
72. Seth A, Yan F, Polk DB, Rao RK. Probiotics ameliorate the hydrogen peroxide-induced epithelial barrier disruption by a PKC- and MAP kinase-dependent mechanism. Am J Physiol Gastrointest Liver Physiol. (2008) 294:G1060–9. doi: 10.1152/ajpgi.00202.2007
73. Bhardwaj R, Singh BP, Sandhu N, Singh N, Kaur R, Rokana N, et al. Probiotic mediated NF-κB regulation for prospective management of type 2 diabetes. Mol Biol Rep. (2020) 47:2301–13. doi: 10.1007/s11033-020-05254-4
74. Ulasov AV, Rosenkranz AA, Georgiev GP, Sobolev AS. Nrf2/Keap1/ARE signaling: Towards specific regulation. Life Sci. (2022) 291:120111. doi: 10.1016/j.lfs.2021.120111
75. Liu T, Zhang L, Joo D, Sun SC. NF-κB signaling in inflammation. Signal Transduct Targeted Ther. (2017) 2:1–9. doi: 10.1038/sigtrans.2017.23
76. Gómez-Guzmán M, Toral M, Romero M, Jiménez R, Galindo P, Sánchez M, et al. Antihypertensive effects of probiotics Lactobacillus strains in spontaneously hypertensive rats. Mol Nutr Food Res. (2015) 59:2326–36. doi: 10.1002/mnfr.201500290
77. Patel B, Kumar P, Banerjee R, Basu M, Pal A, Samanta M, et al. Lactobacillus acidophilus attenuates Aeromonas hydrophila induced cytotoxicity in catla thymus macrophages by modulating oxidative stress and inflammation. Mol Immunol. (2016) 75:69–83. doi: 10.1016/j.molimm.2016.05.012
Keywords: systematic review, probiotics, umbrella meta-analysis, malondialdehyde (MDA), oxidative stress biomarkers
Citation: Musazadeh V, Faghfouri AH, Zarezadeh M, Pakmehr A, Moghaddam PT, Hamedi-Kalajahi F, Jahandideh A and Ghoreishi Z (2023) Remarkable impacts of probiotics supplementation in enhancing of the antioxidant status: results of an umbrella meta-analysis. Front. Nutr. 10:1117387. doi: 10.3389/fnut.2023.1117387
Received: 07 December 2022; Accepted: 20 March 2023;
Published: 11 August 2023.
Edited by:
Marija Takic, University of Belgrade, SerbiaReviewed by:
Babak Haghshenas, Kermanshah University of Medical Sciences, IranCopyright © 2023 Musazadeh, Faghfouri, Zarezadeh, Pakmehr, Moghaddam, Hamedi-Kalajahi, Jahandideh and Ghoreishi. This is an open-access article distributed under the terms of the Creative Commons Attribution License (CC BY). The use, distribution or reproduction in other forums is permitted, provided the original author(s) and the copyright owner(s) are credited and that the original publication in this journal is cited, in accordance with accepted academic practice. No use, distribution or reproduction is permitted which does not comply with these terms.
*Correspondence: Zohreh Ghoreishi, em9ocmVoLmdob3JlaXNoeUBnbWFpbC5jb20=; Arian Jahandideh, QXJpYW5qYWhhbmRpZGVoQHlhaG9vLmNvbQ==
†These authors have contributed equally to this work and share first authorship
Disclaimer: All claims expressed in this article are solely those of the authors and do not necessarily represent those of their affiliated organizations, or those of the publisher, the editors and the reviewers. Any product that may be evaluated in this article or claim that may be made by its manufacturer is not guaranteed or endorsed by the publisher.
Research integrity at Frontiers
Learn more about the work of our research integrity team to safeguard the quality of each article we publish.