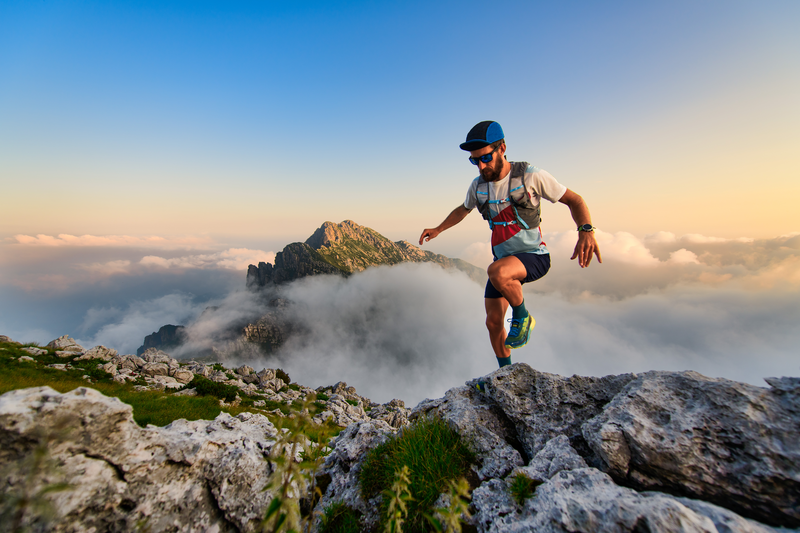
94% of researchers rate our articles as excellent or good
Learn more about the work of our research integrity team to safeguard the quality of each article we publish.
Find out more
ORIGINAL RESEARCH article
Front. Nutr. , 05 July 2022
Sec. Nutrition, Psychology and Brain Health
Volume 9 - 2022 | https://doi.org/10.3389/fnut.2022.931734
At present, it is a trend to use dietary supplements to prevent age-related cognitive impairment. This study aimed to investigate the effects of a dietary supplement enriched with micronutrients, phosphatidylserine, and docosahexaenoic acid on cognitive performance using a D-galactose (D-gal) induced aging rat model. Seven-month-old male Sprague-Dawley rats were randomly divided into five groups, including the control group, D-gal model group, and low-dose (2 g/kg body weight), medium-dose (6 g/kg body weight), and high-dose (10 g/kg body weight) dietary supplement intervention groups, which were investigated for 13 weeks. The dietary supplement intervention was found to improve cognitive performance in Morris water maze test, increase superoxidase dismutase activity, reduce malondialdehyde activity, decrease tumor necrosis factor-α and interleukin-6 concentrations, inhibit the activation of astrocytes, and elevate brain-derived neurotrophic factor protein and mRNA expression in the brains of D-gal-induced aged rats. This dietary supplement customized for the aged can be applied to the restoration of cognitive performance by enhancing antioxidant and anti-neuroinflammatory abilities, up-regulating neurotrophic factors, and inhibiting the activation of astrocytes. These results will be useful for future studies focused on implementation in humans.
Among all organs, the brain is the most vulnerable to oxidative stress owing to its high metabolic activity and lipid content, as well as its limited antioxidant defense mechanisms during aging (1). Thus, increased oxidative stress, which can incur structural and functional alterations in the hippocampus, ultimately leads to decrements in learning and memory, decision-making speed and sensory perception (2). To the best of our knowledge, age-related cognitive impairment is one of the major causes of disability and dependency in human aging (3).
Several human studies (4–8) and fundamental research (7, 9–11) have shown that some nutrients such as antioxidants, B vitamins, phosphatidylserine (PS), long-chain n-3 polyunsaturated fatty acids (n-3 PUFA) and polyphenols can ameliorate age-related cognitive decline and decrease the risk of dementia. Some underlying mechanisms that can potentially support the protective effects of these nutrients against brain aging are the restoration of the microstructural integrity of the hippocampus (12), prevention of glutamatergic synapse and astroglial aging (13), decreased oxidative stress and neuroinflammation (14), and increased expression of brain neurotrophins (15). Based on this situation, cognition-beneficial supplements or “nootropics” have become increasingly popular (16). However, many commercial cognition-beneficial supplements consist of agents (such as piracetam) without the approval from Food and Drug Administration (FDA) for sale (16). Furthermore, the intake levels of phytochemicals such as polyphenols have not been thoroughly investigated, and assessments of polyphenol intake levels are not fully reliable. In addition, excess or deficiency of the nutrient intake attributed to the arbitrary intake of commercial cognition-beneficial supplements—not according to one’s own nutritional status—will raise safety concerns (17). Thus, it is necessary to design dietary supplements, which can accommodate the optimal dietary intakes of the targeted age group, to avoid the risk of insufficient or excessive nutrient intake.
Due to physical changes with aging, older adults face more serious challenges in the attainment of appropriate nutrients. In order to investigate the dietary intakes of the elderly, we conducted a survey among institutional 600 older adults (aged 65 or above) in China, and found that calcium, iron, zinc, selenium, vitamin B1, vitamin B2, and vitamin C intakes of them were all lower than Chinese dietary reference intakes (DRIs) (18, 19). Therefore, a dietary supplement, mainly focused on the essential nutrients, was designed to (1) compensate for the insufficient dietary intake of micronutrients among older adults in China to reach the Chinese DRIs; and (2) improve cognitive function. The composition of the dietary supplement includes 11 vitamins, six minerals, docosahexaenoic acid (DHA), and PS as per the suggestions from experts of the Chinese Nutrition Society.
Chronic administration of D-galactose (D-gal) for 6–10 weeks to induce brain senescence and mimic the effects of natural aging has been a widely accepted model for studying the effects of therapeutic interventions on brain aging (20–23). Therefore, in the present study, we examined the effects of the dietary supplement on age-related cognitive impairment using a D-gal-induced aged rat model. The Morris water maze (MWM) test was conducted to evaluate cognitive performance. Histopathological changes in the brain were analyzed using hematoxylin-eosin (H&E) and Nissl staining. Immunofluorescence analysis was used to assess the activation of astrocytes. The hippocampus and serum concentrations and activities of superoxidase dismutase (SOD) and malondialdehyde (MDA), and serum concentrations of tumor necrosis factor-α (TNF-α) and interleukin-6 (IL-6) were measured. The expression of brain-derived neurotrophic factor (BDNF) in the hippocampus was assessed using western blot and RT-PCR. The results of our study will provide information for future clinical studies regarding this intervention.
D-gal was purchased from Sigma Aldrich (St Louis, MO, United States). ELISA kits (Rat TNF-α ELISA kit, Rat IL-6 ELISA kit, ELK Biotechnology, Wuhan, China) were used to detect TNF-α and IL-6 levels. Anti-BDNFs (ab108319), anti-GAPDH (ab37168), and anti-glial fibrillary acidic protein (GFAP) (ab33922) were purchased from Abcam (MA, United States). HRP goat anti-rabbit antibody (AS1107) was purchased from Aspen Biotechnology (Wuhan, China).
Sprague-Dawley male rats (6 weeks old, SPF grade, weight: 229–295 g) were obtained from Shanghai SIPPR-BK Laboratory Animal Co., Ltd. (SCXK 2013-0016). The animals were housed in ventilated cages (four rats per cage) kept on a 12 h light/12 h dark cycle (light on from 08:00 to 20:00) at 23°C ± 2°C, with humidity of 50 ± 10%. Food and distilled water were provided ad libitum.
The dietary supplement was manufactured by Richen Nutritional Co., Ltd. (Shanghai, China). The compositions of the dietary supplement were determined by the suggestions, based on the dietary survey of 600 older adults (aged 65 or above) in China, by experts at the Chinese Nutrition Society (Table 1) (18, 19). It was designed to improve cognitive function while compensating for the insufficient dietary intake of micronutrients, by providing older adults with at least one sachet (per 20 g) of dietary supplements per day. The fatty acid of this dietary supplement (per 20 g) is composed of 100 mg algal-oil-derived DHA and 100 mg soybeans-derived PS. The commercial basal chow diet (AIN-93M) and the experimental diets were obtained from TROPHIC Animal Feed High-tech Co., Ltd. (Nantong, China) (24). The intervention dose was converted from the human oral dose using the human-rat species coefficient issued by the American FDA as follows (25): 20 g (human oral dose) ÷ 60 kg (standard weight of human adult) × 6.2 (the human-rat species coefficient) × 360 g (standard weight of adult rats) = 0.74 g. Based on the average intake of an adult rat per day (approximate 35 g of feed), 21 g of the dietary supplement was added to 1,000 g of feed. According to the method of function evaluation issued by the China FDA, three intervention groups should be set up in animal experiments with five times the recommended dose in human body (for rats) as one of the groups (26). Therefore, we set up low-, medium-, and high-dose groups of rats that were administered dietary supplements that were 1, 3, and 5 times the recommended human dose, respectively. To prepare the low-, medium-, and high-dose experimental diets, 1,000 g of the AIN-93M diet were mixed with approximately 21, 63, and 105 g of the dietary supplement, respectively. These doses correspond to 1 sachet (20 g), 3 sachets (60 g), or 5 sachets (100 g) of dietary supplements taken orally per day in humans. The compositions of the background AIN-93M diet, and the final amount of low-, medium- and high-dose treatment diets are listed in Table 2. All feeds were sealed and stored in the refrigerator at −20°C and were used within 1 week after opening.
Table 1. Composition of the dietary supplement customized for older adults (20 g per package per day).
60 Sprague-Dawley male rats (6 weeks old, SPF grade, weight: 229–295 g) were randomly divided into five groups using a randomized block design according to body weight (n = 12 for each group). After 1 week of adaptation, the rats in the control group were fed the AIN-93M diet and treated with 0.9% saline (subcutaneous injection, s.c.). The rats in the D-gal model group (D-gal) were fed the AIN-93M diet with D-gal (300 mg/kg/day, s.c.). The rats in the low-, medium-, and high-doses of the intervention groups were treated with D-gal (300 mg/kg/day, s.c.) and were simultaneously fed with the low, medium, and high doses (2, 6, and 10 g/kg body weight dietary supplement, respectively) of the experimental diets. Since the energy differences in the AIN-93M diet, and low-, medium-, and high-dose experimental diets were subtle, the treatment diets were considered to be isocaloric.
The brain aging model requires D-gal injections of 50–500 mg/kg body weight for 6–10 weeks (21). Therefore, we chose chronic administration of D-gal at a dose of 300 mg/kg/day, s.c. for 10 weeks. Previous studies have shown that supplementation with micronutrients for 8–16 weeks can improve the cognitive performance of aged rats (27, 28). At first, the duration of the dietary intervention was preset as 12 weeks. However, the MWM test lasted for 1 week in which rats were still fed with the corresponding intervention diets. Therefore, the dietary intervention, in reality, lasted for 13 weeks. The experimental protocol is shown in Figure 1. The institutional and national guidelines for the care and use of animals were followed and all experimental procedures involving animals were approved by the animal ethics committee of Fudan University (approval number 201810002Z). All surgeries were performed under anesthesia and efforts were made to minimize any suffering.
Figure 1. The experimental protocol of the study. After 1 week of adaptation (week –1 ∼ 0), animal modeling (week 1∼10) and the dietary supplement intervention (week 1 ∼ 13) were introduced simultaneously. After 12 weeks of supplementation, we performed the Morris water maze test (week 13), including 5 days of place navigation test and 1 day of spatial probe test. After the Morris water maze test, the rats were fasted overnight and sacrificed for subsequent tests.
Spatial learning and memory were determined using the MWM test (29, 30). Following 1 day of pre-test, the MWM test, starting in the 13th week, lasted for 6 days (including 5 days of place navigation test and 1 day of spatial probe test) (Figure 1). Besides, in order to minimize the effect of the circadian cycle, the test was performed between 8:00 and 16:00 (10). Rats were trained to use a variety of visual cues located on the pool wall to find a hidden submerged transparent platform in a circular pool (diameter 120 cm, height 45 cm). The inside of the pool was black and filled with 22°C ± 1°C water to achieve a depth of 30 cm. The pool was conceptually divided into four equal quadrants by imaging lines. During the navigation training, the invisible platform was located in the center of the fourth quadrant and remained fixed for each trial. The training was initiated by placing the rats in the water maze, from different randomly chosen start positions located in the four quadrants, in such a way that they all faced the wall of the pool. The sequence of the starting positions was constant for all rats on the first day but was changed on the training days. Each rat underwent four trials every day with a 3-min inter-trial interval. If the rat did not find the platform in the allotted time (within 90 s), it was manually guided toward it. Upon finding the hidden platform, the rat was allowed to rest on it for 10 s. After each trial, the rats were dried and kept in a dry plastic cage filled with towels to dry off. Experimental data such as time taken by the rat to reach the platform, distance covered by the rat, swimming speed, time spent in each quadrant, and the number of times a rat crossed the position of the platform were recorded. On the sixth day, the platform was removed to perform the probe trial. The rats were placed such that they were facing the wall of the tank from the second quadrant. During the test, the path length and time spent in the target quadrant, as well as the number of times a rat crossed the platform location were recorded. The rats were maintained on their respective diets throughout the duration of this test. A video camera was installed on the ceiling of the pool (placed at the center) and was attached to a video tracking system (SuperMaze software, Shanghai Xinruan Information Technology Co., Ltd., China) to monitor swim paths and latencies.
The general appearance of the rats was observed daily throughout the study and the body weight of the rats was recorded weekly. After behavioral studies, the rats were fasted overnight and anesthetized with 10% chloral hydrate (dissolved in normal saline, 4 mL/kg body weight). Serum samples were separated from inferior vena cava and were stored at −80°C for subsequent experiments. Next, the rats were perfused transcardially with normal saline. The heart, liver, spleen, and thymus gland were isolated, blotted dry, and weighed to calculate the organ index according to the following equation: organ index = organ weight (g)/body weight (g) × 100%.
The brains of the rats were carefully removed and washed with ice-cold normal saline and weighed separately. In each group, four brains were used for histopathological examination and immunofluorescence analysis and another eight brains were used for biochemical analysis. Brains were sagittally cut into two halves and paraffin-embedded. The left hemispheres were post-fixed in 4% paraformaldehyde for histopathological examination and immunofluorescence analysis. The hippocampi were immediately separated from the right hemispheres on a cold plate, collected in 0.5 mL EP tubes, and stored at −80°C for biochemical analysis, western blotting, and mRNA expression studies.
H&E and Nissl staining were performed (31) for histological examination and evaluation of neuronal loss.
Paraffin sections were prepared, dewaxed, and rinsed thrice with distilled water. Citrate buffer (pH 6.0) was used for heat-induced antigen retrieval. The sections were treated with 3% bovine serum albumin (BSA) for 30 min to eliminate non-specific binding. The sections were then incubated with a primary antibody (anti-GFAP, 1:300) at 4°C in a light-proof wet box overnight, followed by incubation with a secondary antibody (HRP goat anti-rabbit antibody, 1:400) at room temperature (25–30°C) for 50 min. The sections were washed three times with phosphate-buffered saline (PBS) (pH 7.4) on a decolorizing shaking bed and each washing step was performed for 5 min. After the sections were slightly dried, DAPI dye solution was dripped into the circle and incubated at room temperature for 10 min. Brain sections were washed and visualized with anti-fluorescence quenching (Record Biotechnology Co., Ltd, Shanghai, China). The sections were observed under an inverted fluorescence microscope (Nikon, Tokyo, Japan) (ultraviolet excitation wavelength 330–380 nm, emission wavelength 420 nm, FITC green excitation wavelength 465–495 nm, emission wavelength 515–555 nm, CY3 red excitation wavelength 510–560 nm, emission wavelength 590 nm, 200× magnification) and the images were collected. The screenshots from the dentate gyrus (DG) region (200× magnification) were captured in four slides for each group. The staining of the GFAP-positive cells was analyzed using computerized planimetry Image-pro plus 6.0 (Media Cybernetics, Inc., Rockville, MD, United States). The results of four separate measurements for the DG section are expressed as mean ± standard error.
The hippocampi of the rats were homogenized in tissue total protein lysis buffer (1 g tissue in 9 mL lysis buffer). The homogenate was then centrifuged at 4,000 × g for 10 min and the supernatant was used for the subsequent tests. The concentration of protein was measured using a commercial bicinchoninic acid (BCA) protein assay kit and BSA was used as the standard. MDA levels and SOD activity in both the hippocampus homogenate and serum were assayed using commercially available kits (Nanjing Jiancheng Bioengineering Research Institute, Nanjing, China) according to the manufacturer’s instructions (32).
Serum concentrations of TNF-α and IL-6 were measured using TNF-α and IL-6 ELISA kits (ELK Biotechnology, Wuhan, China) according to the manufacturer’s instructions.
Total RNA was isolated from the hippocampus using Trizol reagent (#15596-026, Ambion, Austin, TX, United States) according to the manufacturer’s instructions. The RNA integrity was confirmed by A260/280 ratio and gel electrophoresis (Beijing Junyi Electrophoresis Co., Ltd.) analysis, cDNA was synthesized from 3.52 μg RNA sample, 2 μL Oligo (dT) 18 (10 μM), 4 μL dNTP (2.5 mM), 4 μL 5XHiscript Buffer, 0.5 μL ribonuclease inhibitor, up to 20 μL RNase free ddH2O at 25°C for 5 min, 50°C for 15 min, 85°C for 5 min followed by 4°C for 10 min by HiScript Reverse Transcriptase (RNase H) (Vazyme Biotech Co., Ltd., Nanjing, China). The cDNA was diluted 5 times and 4 μL cDNA, 0.4 μL forward primer (10 μM), 0.4 μL reverse primer (10 μM), 10 μL SYBR Green qPCR Master Mix (Vazyme Biotech Co., Ltd., Nanjing, China), 0.4 μL 50X ROX Reference Dye 2, and 4.8 μL H2O were used for quantitative RT-PCR that was performed with gene-specific primers on a QuantStudio™ 6 Flex real-time thermocycler (Applied Biosystems, Forster City, CA, United States). Rat-specific primers were designed and synthesized by TSING KE Biological Technology (Beijing, China). Primer sequences used for the GAPDH (internal control) and BDNF amplification are shown in Table 3. The following cycle conditions were used: 50°C for 2 min, 95°C for 10 min, followed by 40 cycles of 95°C for 30 s, and 60°C for 30 s. Relative expression levels of gene expression were calculated and expressed as 2–Δ Δ Ct. Data are expressed as the relative level of the target gene expression in the young control group normalized to that of GAPDH. Each experiment was performed in triplicate with eight independent samples per group.
The hippocampi were rinsed 2–3 times with pre-cooled PBS to remove any blood and were completely homogenized with a homogenizer in ice-cold radio-immunoprecipitation assay lysis buffer (Aspen Biotechnology, Wuhan, China). Protease inhibitor cocktail (Aspen Biotechnology) was added to the hippocampi minutes before homogenization. The homogenate was transferred to a centrifuge tube for oscillation and was then left in an ice bath for 30 min. The homogenate was centrifuged at 13,000 × g for 5 min (4°C) and the supernatant was collected for subsequent analysis. The protein content of the supernatant was estimated using a BCA protein assay kit (Aspen Biotechnology).
Samples were mixed with 5X protein buffer (Aspen Biotechnology) and incubated for 5 min at 98°C before loading. Equal amounts of protein (40 μg) were separated using 12% sodium dodecyl sulfate-polyacrylamide gel electrophoresis and were then transferred to polyvinylidene difluoride membranes (Merck Millipore, Billerica, MA, United States). After blocking with 5% (w/v) skim milk for 1 h, the membranes were incubated with the primary antibodies [BDNF (1:1,000) and GAPDH (1:10,000)] overnight at 4°C, followed by incubation with HPR goat anti-rabbit antibody (Aspen Biotechnology) at room temperature for 30 min. The membranes were rinsed with buffer and the proteins were detected using an ECL detection reagent (Aspen Biotechnology) according to the manufacturer’s instructions. The X-ray films were scanned and quantitative analysis of each band area and density in blots was performed using AlphaEaseFC software (Alpha Innotech, CA, United States).
Statistical analyses were performed with SPSS R24.0.0.0 (IBM Corporation, NY, United States) and Prism 7 for Mac OS X Version 7.0a (GraphPad Software Inc., San Diego, CA, United States). A p-value < 0.05 was considered statistically significant and the results are expressed as the mean ± standard error of the mean (SEM). The primary outcome was the time spent in the target quadrant, as well as the number of times a rat crossed the platform location in the MWM test. The secondary outcomes included the immunofluorescence analysis, the hippocampus and serum concentrations and activities of SOD and MDA, and serum concentrations of TNF-α and IL-6, as well as the expression of BDNF in the hippocampus. The group size was based on previous experimental protocols to allow for the detection of differences for the MWM test with a sufficient power of 80% at the level of significance of 0.05 (10). Twelve rats were included in each treatment group to obtain valid statistical data and account for unexpected mortality during the 13 weeks of treatment. The one-way ANOVA was conducted on comparisons of different groups, followed by the post hoc Tukey test. A repeated-measures two-way ANOVA was used for comparison of the main effects of time (day) and treatment and the time × treatment interaction in the navigation training. A trend analysis was also performed for the primary outcomes, i.e., time spent in the targeted quadrant and the number of times crossing the targeted quadrant.
After 10 weeks of D-gal treatment, rats in the D-gal group showed reduced enthusiasm and physical inactivity, together with loss of hair, compared to the rats in the control group. The mean energy of food consumption of the rats in the five groups was similar each week (Figure 2A); however, the mean body weight gain of the rats in the D-gal group was significantly lower than that of the rats in the control group (p < 0.05) (Figure 2B). Moreover, the rats in the three dietary supplement groups showed a significant increase in their body weight compared with the rats in the D-gal group (p < 0.05). Meanwhile, the organ indices for all organs except the brain were significantly smaller in the D-gal group than those in the control group (Figures 2C–G) (p < 0.05). The brain indices of the D-gal-induced aged rats showed a declining trend (p = 0.095), while they were not substantially different from those of the control group. However, the heart index (Figure 2D) and thymus index (Figure 2G) of the rats in the medium- and high-dose groups were significantly increased compared with those in the D-gal group. The spleen index (Figure 2F) of the rats in three treatment groups were all significantly increased compared with those in the D-gal group. Only the liver indices in the high-dose group were significantly increased, compared with those in the D-gal group (Figure 2E) (all p < 0.05).
Figure 2. Effects of the dietary supplement on mean energy of food consumption (A), mean body weight gain (B), and organ indices (C–G) in D-gal induced aged rats at the end of supplementation (week 13). The data are presented as mean ± SEM (n = 12). *p < 0.05 when compared with the control group; #p < 0.05 when compared with the D-gal group. D-gal, D-galactose; SEM, standard error of mean.
The MWM test was used to investigate the effects of the dietary supplement on cognitive impairment in D-gal-induced aged rats. Figures 3A,B revealed D-gal group as the only group that did not show a progressive decline in either escape latency or swimming distance with training. Repeated measures two-way ANOVA for escape latencies and path to a hidden platform during MWM acquisition revealed significant main effects for day and for treatment (Ftreatment = 69.12, p < 0.001; Fday = 478.07, p < 0.001; Finteraction = 2.15, p > 0.05). The results demonstrated that the spatial learning ability of the rats in the D-gal group was significantly impaired during the 5-day navigation training because their escape latency and swimming distance were longer than those of the control group (Figure 3C). However, the escape latency and swimming distance of rats in the dietary supplement intervention groups (low-, medium-, and high-dose) were significantly decreased, compared with those in the D-gal group (all p < 0.05) (Figures 3A,B).
Figure 3. Effects of the dietary supplement on learning and memory impairment in Morris water maze test (n = 4 in each group). The escape latencies (A), swimming distance (B), the typical swimming path on the fifth day (C) in the navigation training, the time in the targeted quadrant (D), platform crossing times (E), and the typical swimming path (F) in the probe trial. Values are presented as means ± SEM. *p < 0.05 when compared with the control group; #p < 0.05 when compared with the D-gal group. D-gal, D-galactose; SEM, standard error of mean.
In the spatial probe trial, rats in the D-gal group spent less time in the targeted quadrant (Figure 3D) and crossed the location of the removed platform fewer times (Figure 3E) than the rats in the control group (both p < 0.05), whereas the rats in the three dietary supplement intervention groups spent more time in the targeted quadrant and crossed the target location more times than the rats in the D-gal group (all p < 0.05) (Figures 3D–F). The dose-effect trends of the time spent in the targeted quadrant (Ptrend < 0.001) and the number of times crossing the targeted quadrant (Ptrend < 0.001) among the three dietary supplement groups were statistically different.
The histopathological changes in the DG region of the brain were further explored using H&E (Figure 4A) and Nissl staining (Figure 4B). Neurons in the DG region of the rats in the control group showed normal morphology with well-preserved cytoplasm, prominent nucleus, and nucleolus. However, neuronal degeneration and nucleus shrinkage were observed in the neurons in the hippocampal DG regions of the rats in the D-gal group. In contrast, the neuron damage was alleviated in the dietary supplement intervention groups.
Figure 4. The representative photomicrographs of H&E staining (A), Nissl staining (B) and immunofluorescence staining of GFAP (green) (C,D) in the DG region of the hippocampus (n = 4 in each group). In photomicrographs of immunofluorescence, astrocytes were positive for GFAP with ramified branches. The images of the scale bar represent 100 μm, magnification = 200X. Values are presented as means ± SEM. *p < 0.05 when compared with the control group; #p < 0.05 when compared with the D-gal group. DG, dentate gyrus; GFAP, glial fibrillary acidic protein; SEM, standard error of mean.
GFAP expression indicates the overaction and inflammatory response of astrocytes in hippocampal aging (33). The D-gal-treated rats showed higher GFAP expression in the DG regions, compared with the rats in the control group (Figures 4C,D). However, the expressions of GFAP in the DG region in the three intervention groups were decreased, compared with those of the rats in the D-gal group (all p < 0.05).
MDA and SOD concentrations in the hippocampi and serum were measured to assess the effect of dietary supplement intervention on oxidative capacities. The D-gal treatment significantly decreased the SOD activities and increased the MDA concentrations (Figures 5A–D). In contrast, the low-, medium-, and high-dose dietary supplement treatments down-regulated MDA accumulation in both serum and brain, and up-regulated production of SOD in serum (Figures 5A–C). However, only medium- and high-dose dietary supplement treatments up-regulated the SOD activities in brain (Figure 5D).
Figure 5. Effects of the dietary supplement on MDA (A,C) and SOD (B,D) contents in both serum and brain tissues, and serum IL-6 (E) and TNF-α (F) concentrations (n = 8 in each group). Values are presented as means ± SEM. *p < 0.05 when compared with the control group; #p < 0.05 when compared with the D-gal group. IL-6, interleukin-6; MDA, malondialdehyde; SEM, standard error of mean; SOD, superoxidase dismutase; TNF-α, tumor necrosis factor-α.
The serum concentrations of TNF-α and IL-6 in the D-gal group were significantly higher than those of the control group (Figures 5E,F). The dietary supplement intervention (low-, medium-, and high-dose) restrained the D-gal-induced alterations in the serum levels of pro-inflammatory cytokines (all p < 0.05).
Western blot analysis revealed that the hippocampal BDNF expression levels in the D-gal group were significantly decreased, compared with those of the control group (p < 0.05), whereas the three dietary supplement intervention group showed substantially increased BDNF expression levels compared to that of the D-gal group (all p < 0.05) (Figures 6A,B). BDNF mRNA expression in the hippocampus demonstrated a similar trend (Figure 6C).
Figure 6. Effects of the dietary supplement on expression of BDNF in hippocampi (n = 8 in each group). Supplement of Dietary supplement can increase the expression of BDNF protein levels (A,B) and the expression of BDNF mRNA levels (C) in hippocampi. Values are presented as means ± SEM. *p < 0.05 when compared with the control group; #p < 0.05 when compared with the D-gal group. BDNF, brain-derived neurotrophic factor; D-gal, D-galactose; SEM, standard error of mean.
The present study aimed to investigate whether the dietary supplement could improve age-related cognitive impairments using the D-gal-induced aged rat model. In the aged rats induced by D-gal, it was discovered that the dietary supplement intervention reduced learning and memory deficits, reversed changes in lower organ indices, decreased MDA levels, increased SOD activities, decreased TNF-α and IL-6 concentrations, attenuated astrocyte reactivities, and upregulated expression of BDNF.
Aging is characterized by changes in appearance, body weight, and organ indices (34). The administration of D-gal in rats can accelerate the development of these symptoms (21), manifested as significant decreases in heart, liver, thymus, and spleen indices (35, 36). In line with the results, our study also demonstrated that the administration of D-gal for 10 weeks caused atrophy of the heart, liver, spleen, and thymus. Nevertheless, administration of the dietary supplement for 13 weeks restored the organ indices of the rats in three supplement groups closer, than in the D-gal group, to those of the control group, in which the medium dose of the dietary supplement increased heart, spleen, and thymus indices, and the high dose of the dietary supplement increased heart, spleen, liver, and thymus indices.
The hippocampus plays an important role in spatial learning and memory (37). However, neurogenesis in the DG area of the hippocampus shows a continuous decline as the brain ages (38). This decline can be attributed to the age-related exhaustion of neural stem cells and progenitor cells (NSCs/NPCs) through their conversion into mature hippocampal astrocytes (39). Furthermore, the reduction in neurogenesis contributes to cognitive deficits in hippocampal learning and spatial memory (40). In the present study, the dietary supplement promoted retention of normal neurons, which manifested in the form of an increased number of normal neurons in the H&E and Nissl staining. Folate in the dietary supplement may promote neurogenesis by stimulating the proliferation of NSCs (41). Vitamin B12 and DHA may also contribute to the microstructural integrity of the hippocampus as they act as precursors for the formation of neuronal membranes and synapses (12, 42). Additionally, vitamins A and D may improve hippocampal neurogenesis by regulating the retinoic acid signaling pathway on the action of glucocorticoids and differentiation of NPCs (43, 44).
Chronic injection of D-gal can disrupt the oxidative/anti-oxidative balance, exert reactive oxygen species (ROS), and lead to impaired learning and memory function (45). Excessive ROS not only potently inhibits neurogenesis but also increases neuroinflammation and neurodegeneration (46). Our results showed that the dietary supplement protected the hippocampus against oxidative stress by enhancing the SOD activity (one of the important anti-oxidative enzymes) to remove ROS accumulated during aging. The decrease in the MDA level, an indication of lipid peroxidation, also contributed to the reduction of ROS. Many nutrients including vitamins C, E, and D, and iron are associated with oxidative stress; moreover, oxidative stress and inflammation pathways are interrelated (47–49). For example, the administration of vitamin E (α-tocopherol at a dose of 100 mg/kg once daily via oral gavage for 6 weeks) has been shown to prevent high-fat high-carbohydrate diet-induced memory impairment, with normalized SOD activity, thiobarbituric acid reactive substances levels, and oxidized glutathione in the hippocampus of adult male Wistar rats (50). Similarly, in male Wistar rats, vitamin D supplementation preserves high-fat induced cognitive impairment, increases glutathione peroxidase and SOD activities, and reduces MDA concentration (51). These results suggest that the dietary supplement may attenuate D-gal-induced cognitive impairment, possibly by activating antioxidant enzymes and eliminating free radicals in the hippocampus.
The presence of long-lasting increments in pro-inflammatory cytokines in the hippocampus, such as Il-6, IL-1β, and TNF-α, has led to the implication of neuroinflammation in brain aging and cognitive impairments (52). Additionally, GFAP-positive neurons, which serve as markers of activated astrocytes, have also been identified as a major brain-derived source of inflammatory cytokines (53). In our study, the serum levels of IL-6 and TNF-α were significantly lower in the dietary supplement groups than those in the D-gal group, possibly due to the anti-inflammatory activities of the nutrients in dietary supplements. In 22-month-old Wistar rats fed an n-3 deficient diet from birth, poor n-3 PUFA status exacerbates reduced glutamatergic transmission and its related astroglial regulation in the hippocampus (13). However, the amelioration of cognitive deficits came off in conjunction with the picking-up of the level of DHA in the brain in old senescence-accelerated prone 8 mice (54). Further, the administration of vitamin C (200 and 400 mg/kg body weight) can restore colchicine induced memory impairments and also decrease hippocampal TNF-α and IL-1β concentrations in colchicine induced Alzheimer’s disease rats (48). Vitamin D supplementation (1 μg/kg vitamin D daily by intragastric gavage for 10 days) also attenuates neuroinflammation through the up-regulation of the anti-inflammatory cytokines (IL-10, IL-4, and TGF-β) mRNA in a Parkinson’s disease animal model (55). Another study found that supplementation of DHA, eicosapentaenoic acid, uridine, choline, phospholipids, folate, vitamins B12, vitamin B6, vitamin C, vitamin E, and selenium decreases assessed neuroinflammatory response and improves the neural function and structure, which is assessed by magnetic resonance imaging and positron emission tomography, in middle cerebral artery occlusion C57BL/6J mice after ischemic stroke (56). These results suggest that the dietary supplement can counteract neuroinflammation and astrogenesis to protect the hippocampal function.
BDNF is one of the most important neurotrophic factors in the brain and primarily exists in the hippocampus to regulate learning and memory capacity (57). It plays a central role in neurogenesis, survival, growth, and synaptic plasticity (58), whose expression decreases during aging (59). The neuroprotective effects of BDNF in the regulation of neurocognitive functions, such as learning, memory, synaptic transmission, and plasticity, have been demonstrated in some studies (60). BDNF can also decrease inflammatory factors (including TNF-α, IL-1β, and IL-6), increase anti-inflammatory factors (IL-10), and inhibit hippocampal apoptosis to reduce neuroinflammation (61). The supplement of vitamin B1 (10 mg/kg body weight by oral gavage) every other day for 30 days has been found to increase hippocampal BDNF levels and improve spatial memory performance in a chronic stress model of Wistar rats (62). Another study showed that supplementation of a diet mixed with vitamin B12 and fish oil derived DHA and eicosapentaenoic acid, increases BDNF levels in both the hippocampus and cortex and improves cognitive performance in Wistar albino rats (15). Moreover, vitamin D can reverse cognitive impairments by the elevation of hippocampal BDNF concentrations in high-fat diet-induced obese Wistar rats (orally with 500 IU/kg vitamin D for 5 weeks) (63) and protect against hippocampal apoptosis in seizure Sprague Dawley rats model (orally with 500 IU/kg for 2 weeks) (64). In accordance with the above results, our results indicate that the dietary supplement increased the BDNF levels in the D-gal-induced aged rats, which is consistent with the observed improvement in cognitive function. Hence, we believe that the improvement of cognition could be partially attributed to the increase in the BDNF levels.
In this study, we designed a cognition-beneficial dietary supplement targeting Chinese older adults based on the dietary survey and investigated the effect of the dietary supplement on cognition using D-gal-induced aged rats. The results provide a theoretical basis for future clinical studies regarding this intervention. We observed that the time spent in the targeted quadrant and the number of times crossing the targeted quadrant have a dose effect among the three dietary supplement groups. In addition, as treatment dose increased, parameters including serum MDA, SOD, IL-6, TNF-α, and BDNF in hippocampi tend to, respectively, draw near their optimal values. However, even the low-dose dietary supplement can improve the cognitive deficits of the rats, which means one sachet (20 g) per day of this dietary supplement may potentially improve the cognitive decline in the elderly. In terms of population-based intervention studies, the Chinese DRIs for older adults need to be considered, the optimization of a supplement approach in humans, maybe at a dose of 1–2 sachets/day, requires further clinical trials.
However, some limitations remain in the study. First, the aim of the study was to evaluate the overall effect of the dietary supplement on cognitive impairment in aged rats, so we only investigated the effect of integral dietary supplement. The identification of the specific nutrient which counteracts cognitive impairment is beyond the scope of this study, while it remains unknown whether there is a synergistic or antagonistic effect. For instance, a combination of neuroprotective compounds may have synergistic actions that promote neuroimmune protection, reduce the effects of aging, and improve the absorption, bioavailability and actions of neuroprotective compounds in the brain (28). Many nutrients (such as folate, vitamin B12, vitamin A, iron and zinc) are found to be capable of regulating n-3 PUFA synthesis (65). Second, as we didn’t aim to observe the effect of the dietary supplement on micronutrient levels in rats, we did not examine the concentrations of the nutrients in blood and tissues. According to previous literature, dietary supplements containing micronutrients and n-3 PUFA can be well absorbed by rats when mixed into feed (15, 66). The concentrations of the nutrients in blood will subsequently be assessed in population-based intervention studies. Moreover, the MWM test is an approach sufficient for assessing cognitive function on its own (67), so we only used the MWM test to assess cognitive function. Future studies using more cognitive assessments are needed to further validate the effectiveness of cognitive enhancement of this dietary supplement.
In summary, we observed that this dietary supplement restored D-gal-induced spatial and memory impairment, accompanied by the up-regulation of BDNF, and the enhancement of antioxidant and anti-neuroinflammatory abilities. Our results provide evidence for further clinical interventions; however, the optimization of a supplement approach in humans requires further clinical trials. Nonetheless, these findings suggest that this dietary supplement could be a promising candidate for the neuroenhancement of age-related cognitive impairments.
The original contributions presented in the study are included in the article/Supplementary Material, further inquiries can be directed to the corresponding author/s.
The animal study was reviewed and approved by the Animal Ethics Committee of Fudan University (approval number 201810002Z).
JS and QR contributed to conceptualization, methodology, supervision, and writing—original draft preparation, review and editing. QR, HX, MY, DX and YZ performed the experiments. QR and DX analyzed the data. All authors have read and agreed to the published version of the manuscript.
This research was funded by the Shanghai Municipal Health System Important and Weak Discipline (Clinical Nutrition) Project (No. 2019ZB0102).
The authors declare that the research was conducted in the absence of any commercial or financial relationships that could be construed as a potential conflict of interest.
All claims expressed in this article are solely those of the authors and do not necessarily represent those of their affiliated organizations, or those of the publisher, the editors and the reviewers. Any product that may be evaluated in this article, or claim that may be made by its manufacturer, is not guaranteed or endorsed by the publisher.
We are very grateful to the Shanghai Key Laboratory of Clinical Geriatric Medicine, Huadong Hospital affiliated to Fudan University.
The Supplementary Material for this article can be found online at: https://www.frontiersin.org/articles/10.3389/fnut.2022.931734/full#supplementary-material
BCA, bicinchoninic acid; BDNF, brain-derived neurotrophic factors; BSA, bovine serum albumin; DG, dentate gyrus; D-gal, D-galactose; DHA, docosahexaenoic acid; DRIs, dietary reference intakes; FDA, Food and Drug Administration; GFAP, glial fibrillary acidic protein; H&E, hematoxylin-eosin; IL-6, interleukin-6; MDA, malondialdehyde; MWM, Morris water maze; n-3 PUFA, n-3 polyunsaturated fatty acids; NSCs/NPCs, neural stem cells and progenitor cells; PBS, phosphate-buffered saline; PS, phosphatidylserine; ROS, reactive oxygen species; SEM, standard error of the mean; SOD, superoxidase dismutase; TNF-α, tumor necrosis factor-α.
1. Mattson MP, Arumugam TV. Hallmarks of brain aging: adaptive and pathological modification by metabolic states. Cell Metab. (2018) 27:1176–99. doi: 10.1016/j.cmet.2018.05.011
2. Fan X, Wheatley EG, Villeda SA. Mechanisms of hippocampal aging and the potential for rejuvenation. Annu Rev Neurosci. (2017) 40:251–72. doi: 10.1146/annurev-neuro-072116-031357
3. Cabeza R, Albert M, Belleville S, Craik FIM, Duarte A, Grady CL, et al. Maintenance, reserve and compensation: the cognitive neuroscience of healthy ageing. Nat Rev Neurosci. (2018) 19:701–10. doi: 10.1038/s41583-018-0068-2
4. Macpherson H, Ellis KA, Sali A, Pipingas A. Memory improvements in elderly women following 16 weeks treatment with a combined multivitamin, mineral and herbal supplement: a randomized controlled trial. Psychopharmacology (Berl). (2012) 220:351–65. doi: 10.1007/s00213-011-2481-3
5. Chan A, Remington R, Kotyla E, Lepore A, Zemianek J, Shea TB. A vitamin/nutriceutical formulation improves memory and cognitive performance in community-dwelling adults without dementia. J Nutr Health Aging. (2010) 14:224–30. doi: 10.1007/s12603-010-0054-5
6. Zwilling CE, Talukdar T, Zamroziewicz MK, Barbey AK. Nutrient biomarker patterns, cognitive function, and fMRI measures of network efficiency in the aging brain. Neuroimage. (2019) 188:239–51. doi: 10.1016/j.neuroimage.2018.12.007
7. Poulose SM, Miller MG, Scott T, Shukitt-Hale B. Nutritional factors affecting adult neurogenesis and cognitive function. Adv Nutr. (2017) 8:804–11. doi: 10.3945/an.117.016261
8. Yang W, Cui K, Li X, Zhao J, Zeng Z, Song R, et al. Effect of polyphenols on cognitive function: evidence from population-based studies and clinical trials. J Nutr Health Aging. (2021) 25:1190–204. doi: 10.1007/s12603-021-1685-4
9. Zerbi V, Jansen D, Wiesmann M, Fang X, Broersen LM, Veltien A, et al. Multinutrient diets improve cerebral perfusion and neuroprotection in a murine model of Alzheimer’s disease. Neurobiol Aging. (2014) 35:600–13. doi: 10.1016/j.neurobiolaging.2013.09.038
10. Jansen D, Zerbi V, Janssen CI, van Rooij D, Zinnhardt B, Dederen PJ, et al. Impact of a multi-nutrient diet on cognition, brain metabolism, hemodynamics, and plasticity in apoE4 carrier and apoE knockout mice. Brain Struct Funct. (2014) 219:1841–68. doi: 10.1007/s00429-013-0606-7
11. Pan Y, Araujo JA, Burrows J, de Rivera C, Gore A, Bhatnagar S, et al. Cognitive enhancement in middle-aged and old cats with dietary supplementation with a nutrient blend containing fish oil, B vitamins, antioxidants and arginine. Br J Nutr. (2013) 110:40–9. doi: 10.1017/s0007114512004771
12. Köbe T, Witte AV, Schnelle A, Grittner U, Tesky VA, Pantel J, et al. Vitamin B-12 concentration, memory performance, and hippocampal structure in patients with mild cognitive impairment. Am J Clin Nutr. (2016) 103:1045–54. doi: 10.3945/ajcn.115.116970
13. Latour A, Grintal B, Champeil-Potokar G, Hennebelle M, Lavialle M, Dutar P, et al. Omega-3 fatty acids deficiency aggravates glutamatergic synapse and astroglial aging in the rat hippocampal CA1. Aging Cell. (2013) 12:76–84. doi: 10.1111/acel.12026
14. Chen P, Chen F, Zhou B. Antioxidative, anti-inflammatory and anti-apoptotic effects of ellagic acid in liver and brain of rats treated by D-galactose. Sci Rep. (2018) 8:1465. doi: 10.1038/s41598-018-19732-0
15. Rathod RS, Khaire AA, Kale AA, Joshi SR. Effect of vitamin B12 and omega-3 fatty acid supplementation on brain neurotrophins and cognition in rats: a multigeneration study. Biochimie. (2016) 128:201–8. doi: 10.1016/j.biochi.2016.08.009
16. Cohen PA, Zakharevich I, Gerona R. Presence of piracetam in cognitive enhancement dietary supplements. JAMA Intern Med. (2020) 180:458–9. doi: 10.1001/jamainternmed.2019.5507
17. Zhang FF, Barr SI, McNulty H, Li D, Blumberg JB. Health effects of vitamin and mineral supplements. BMJ. (2020) 369:m2511. doi: 10.1136/bmj.m2511
18. Wu CF, Lu YW, Li H. Dietary intake and nutritional status of 600 elderly in 3 long-term care institutions in Shanghai. Geriatr Health Care (in Chinese). (2018) 24:30–3.
19. Ren Q, Xie H, Chen YQ, Wu CF, Li H, Lu YW, et al. Effect of micronutrient pack on micronutrient status and antioxidant capacities among institutional older adults in Shanghai, China. Asia Pac J Clin Nutr. (2019) 28:457–66. doi: 10.6133/apjcn.201909_28(3).0005
20. Azman KF, Zakaria RD-. Galactose-induced accelerated aging model: an overview. Biogerontology. (2019) 20:763–82. doi: 10.1007/s10522-019-09837-y
21. Shwe T, Pratchayasakul W, Chattipakorn N, Chattipakorn SC. Role of D-galactose-induced brain aging and its potential used for therapeutic interventions. Exp Gerontol. (2018) 101:13–36. doi: 10.1016/j.exger.2017.10.029
22. Singh S, Garg G, Singh AK, Tripathi SS, Rizvi SI. Fisetin, a potential caloric restriction mimetic, modulates ionic homeostasis in senescence induced and naturally aged rats. Arch Physiol Biochem. (2022) 128:51–8. doi: 10.1080/13813455.2019.1662452
23. Singh S, Kumar R, Garg G, Singh AK, Verma AK, Bissoyi A, et al. Spermidine, a caloric restriction mimetic, provides neuroprotection against normal and D-galactose-induced oxidative stress and apoptosis through activation of autophagy in male rats during aging. Biogerontology. (2021) 22:35–47. doi: 10.1007/s10522-020-09900-z
24. Reeves PG, Nielsen FH, Fahey GC Jr. AIN-93 purified diets for laboratory rodents: final report of the American institute of nutrition ad hoc writing committee on the reformulation of the AIN-76A rodent diet. J Nutr. (1993) 123:1939–51. doi: 10.1093/jn/123.11.1939
25. FDA guidance for industry. Estimating the Maximum Safe Starting Dose in Initial Clinical Trials for Therapeutics in Adult Healthy Volunteers. Rockville, MD: FDA guidance for industry (2002).
26. Evaluation method for auxiliary improvement of memory function. China Food and Drug Administration (2020).
27. Singh R, Kanwar SS, Sood PK, Nehru B. Beneficial effects of folic acid on enhancement of memory and antioxidant status in aged rat brain. Cell Mol Neurobiol. (2011) 31:83–91. doi: 10.1007/s10571-010-9557-1
28. Perez SD, Du K, Rendeiro C, Wang L, Wu Q, Rubakhin SS, et al. A unique combination of micronutrients rejuvenates cognitive performance in aged mice. Behav Brain Res. (2017) 320:97–112. doi: 10.1016/j.bbr.2016.11.019
29. Vorhees CV, Williams MT. Morris water maze: procedures for assessing spatial and related forms of learning and memory. Nat Protocols. (2006) 1:848–58. doi: 10.1038/nprot.2006.116
30. Chaung HC, Chang CD, Chen PH, Chang CJ, Liu SH, Chen CC. Docosahexaenoic acid and phosphatidylserine improves the antioxidant activities in vitro and in vivo and cognitive functions of the developing brain. Food Chem. (2013) 138:342–7. doi: 10.1016/j.foodchem.2012.10.082
31. Ji M, Su X, Liu J, Zhao Y, Li Z, Xu X, et al. Comparison of naturally aging and D-galactose induced aging model in beagle dogs. Exp Ther Med. (2017) 14:5881–8. doi: 10.3892/etm.2017.5327
32. Qu Z, Zhang J, Yang H, Huo L, Gao J, Chen H, et al. Protective effect of tetrahydropalmatine against d-galactose induced memory impairment in rat. Physiol Behav. (2016) 154:114–25. doi: 10.1016/j.physbeh.2015.11.016
33. Mandybur TI, Ormsby I, Zemlan FP. Cerebral aging: a quantitative study of gliosis in old nude mice. Acta Neuropathol. (1989) 77:507–13. doi: 10.1007/bf00687252
34. Spencer RP. Organ/body weight loss with aging: evidence for co-ordinated involution. Med Hypotheses. (1996) 46:59–62. doi: 10.1016/s0306-9877(96)90000-6
35. Chen NY, Liu CW, Lin W, Ding Y, Bian ZY, Huang L, et al. Extract of fructus cannabis ameliorates learning and memory impairment induced by D-galactose in an aging rats model. Evid Based Complement Alternat Med. (2017) 2017:4757520. doi: 10.1155/2017/4757520
36. Sun K, Yang P, Zhao R, Bai Y, Guo Z. Matrine attenuates D-Galactose-induced aging-related behavior in mice via inhibition of cellular senescence and oxidative stress. Oxid Med Cell Longev. (2018) 2018:7108604. doi: 10.1155/2018/7108604
37. Stachenfeld KL, Botvinick MM, Gershman SJ. The hippocampus as a predictive map. Nat Neurosci. (2017) 20:1643–53. doi: 10.1038/nn.4650
38. Bartsch T, Wulff P. The hippocampus in aging and disease: from plasticity to vulnerability. Neuroscience. (2015) 309:1–16. doi: 10.1016/j.neuroscience.2015.07.084
39. Encinas JM, Michurina TV, Peunova N, Park JH, Tordo J, Peterson DA, et al. Division-coupled astrocytic differentiation and age-related depletion of neural stem cells in the adult hippocampus. Cell Stem Cell. (2011) 8:566–79. doi: 10.1016/j.stem.2011.03.010
40. Drapeau E, Mayo W, Aurousseau C, Le Moal M, Piazza PV, Abrous DN. Spatial memory performances of aged rats in the water maze predict levels of hippocampal neurogenesis. Proc Natl Acad Sc USA. (2003) 100:14385–90. doi: 10.1073/pnas.2334169100
41. Liu H, Cao J, Zhang H, Qin S, Yu M, Zhang X, et al. Folic acid stimulates proliferation of transplanted neural stem cells after focal cerebral ischemia in rats. J Nutr Biochem. (2013) 24:1817–22. doi: 10.1016/j.jnutbio.2013.04.002
42. Issa AM, Mojica WA, Morton SC, Traina S, Newberry SJ, Hilton LG, et al. The efficacy of omega-3 fatty acids on cognitive function in aging and dementia: a systematic review. Dement Geriatr Cogn Disord. (2006) 21:88–96. doi: 10.1159/000090224
43. Morello M, Landel V, Lacassagne E, Baranger K, Annweiler C, Feron F, et al. Vitamin D improves neurogenesis and cognition in a mouse model of Alzheimer’s disease. Mol Neurobiol. (2018) 55:6463–79. doi: 10.1007/s12035-017-0839-1
44. Bonhomme D, Minni AM, Alfos S, Roux P, Richard E, Higueret P, et al. Vitamin A status regulates glucocorticoid availability in Wistar rats: consequences on cognitive functions and hippocampal neurogenesis? Front Behav Neurosci. (2014) 8:20. doi: 10.3389/fnbeh.2014.00020
45. Zhu J, Mu X, Zeng J, Xu C, Liu J, Zhang M, et al. Ginsenoside Rg1 prevents cognitive impairment and hippocampus senescence in a rat model of D-galactose-induced aging. PLoS One. (2014) 9:e101291. doi: 10.1371/journal.pone.0101291
46. Yuan TF, Gu S, Shan C, Marchado S, Arias-Carrión O. Oxidative stress and adult neurogenesis. Stem Cell Rev Rep. (2015) 11:706–9. doi: 10.1007/s12015-015-9603-y
47. Liu D, Ke Z, Luo J. Thiamine deficiency and neurodegeneration: the interplay among oxidative stress, endoplasmic reticulum stress, and autophagy. Mol Neurobiol. (2017) 54:5440–8. doi: 10.1007/s12035-016-0079-9
48. Sil S, Ghosh T, Gupta P, Ghosh R, Kabir SN, Roy A. Dual role of vitamin C on the neuroinflammation mediated neurodegeneration and memory impairments in colchicine induced rat model of Alzheimer disease. J Mol Neurosci. (2016) 60:421–35. doi: 10.1007/s12031-016-0817-5
49. Park UJ, Lee YA, Won SM, Lee JH, Kang SH, Springer JE, et al. Blood-derived iron mediates free radical production and neuronal death in the hippocampal CA1 area following transient forebrain ischemia in rat. Acta neuropathol. (2011) 121:459–73. doi: 10.1007/s00401-010-0785-8
50. Alzoubi KH, Khabour OF, Salah HA, Hasan Z. Vitamin E prevents high-fat high-carbohydrates diet-induced memory impairment: the role of oxidative stress. Physiol Behav. (2013) 119:72–8. doi: 10.1016/j.physbeh.2013.06.011
51. Hajiluian G, Abbasalizad Farhangi M, Nameni G, Shahabi P, Megari-Abbasi M. Oxidative stress-induced cognitive impairment in obesity can be reversed by vitamin D administration in rats. Nutr Neurosci. (2018) 21:744–52. doi: 10.1080/1028415x.2017.1348436
52. Barrientos RM, Kitt MM, Watkins LR, Maier SF. Neuroinflammation in the normal aging hippocampus. Neuroscience. (2015) 309:84–99. doi: 10.1016/j.neuroscience.2015.03.007
53. Doyen PJ, Vergouts M, Pochet A, Desmet N, van Neerven S, Brook G, et al. Inflammation-associated regulation of RGS in astrocytes and putative implication in neuropathic pain. J Neuroinflamm. (2017) 14:209. doi: 10.1186/s12974-017-0971-x
54. Currais A, Farrokhi C, Dargusch R, Armando A, Quehenberger O, Schubert D, et al. Fisetin reduces the impact of aging on behavior and physiology in the rapidly aging SAMP8 mouse. J Gerontol A Biol Sci Med Sci. (2018) 73:299–307. doi: 10.1093/gerona/glx104
55. Calvello R, Cianciulli A, Nicolardi G, De Nuccio F, Giannotti L, Salvatore R, et al. Vitamin D treatment attenuates neuroinflammation and dopaminergic neurodegeneration in an animal model of Parkinson’s disease, shifting M1 to M2 microglia responses. J Neuroimmune Pharmacol. (2017) 12:327–39. doi: 10.1007/s11481-016-9720-7
56. Wiesmann M, Zinnhardt B, Reinhardt D, Eligehausen S, Wachsmuth L, Hermann S, et al. A specific dietary intervention to restore brain structure and function after ischemic stroke. Theranostics. (2017) 7:493–512. doi: 10.7150/thno.17559
57. Phillips HS, Hains JM, Armanini M, Laramee GR, Johnson SA, Winslow JW. BDNF mRNA is decreased in the hippocampus of individuals with Alzheimer’s disease. Neuron. (1991) 7:695–702. doi: 10.1016/0896-6273(91)90273-3
58. Karpova NN. Role of BDNF epigenetics in activity-dependent neuronal plasticity. Neuropharmacology. (2014) 76:709–18. doi: 10.1016/j.neuropharm.2013.04.002
59. Buchman AS, Yu L, Boyle PA, Schneider JA, De Jager PL, Bennett DA. Higher brain BDNF gene expression is associated with slower cognitive decline in older adults. Neurology. (2016) 86:735–41. doi: 10.1212/wnl.0000000000002387
60. Kumar A, Pareek V, Faiq MA, Kumar P, Raza K, Prasoon P, et al. Regulatory role of NGFs in neurocognitive functions. Rev Neurosci. (2017) 28:649–73. doi: 10.1515/revneuro-2016-0031
61. Xu D, Lian D, Wu J, Liu Y, Zhu M, Sun J, et al. Brain-derived neurotrophic factor reduces inflammation and hippocampal apoptosis in experimental Streptococcus pneumoniae meningitis. J Neuroinflamm. (2017) 14:156. doi: 10.1186/s12974-017-0930-6
62. Dief AE, Samy DM, Dowedar F I. Impact of exercise and vitamin B1 intake on hippocampal brain-derived neurotrophic factor and spatial memory performance in a rat model of stress. J Nutr Sci Vitaminol. (2015) 61:1–7. doi: 10.3177/jnsv.61.1
63. Hajiluian G, Nameni G, Shahabi P, Mesgari-Abbasi M, Sadigh-Eteghad S, Farhangi MA. Vitamin D administration, cognitive function, BBB permeability and neuroinflammatory factors in high-fat diet-induced obese rats. Int J Obes. (2017) 41:639–44. doi: 10.1038/ijo.2017.10
64. Şahin S, Gürgen SG, Yazar U, Ince I, Kamaşak T, Acar Arslan E, et al. Vitamin D protects against hippocampal apoptosis related with seizures induced by kainic acid and pentylenetetrazol in rats. Epilepsy Res. (2019) 149:107–16. doi: 10.1016/j.eplepsyres.2018.12.005
65. Gonzalez-Soto M, Mutch DM. Diet regulation of long-chain PUFA synthesis: role of macronutrients, micronutrients, and polyphenols on delta-5/Delta-6 desaturases and elongases 2/5. Adv Nutr. (2021) 12:980–94. doi: 10.1093/advances/nmaa142
66. Latimer CS, Brewer LD, Searcy JL, Chen KC, Popovic J, Kraner SD, et al. Vitamin D prevents cognitive decline and enhances hippocampal synaptic function in aging rats. Proc Natl Acad Sci USA. (2014) 111:E4359–66. doi: 10.1073/pnas.1404477111
Keywords: dietary supplement, micronutrient, phosphatidylserine, docosahexaenoic acid, cognitive impairment, neuroinflammation, brain-derived neurotrophic factor
Citation: Ren Q, Sun J, Xu D, Xie H, Ye M and Zhao Y (2022) A Dietary Supplement Containing Micronutrients, Phosphatidylserine, and Docosahexaenoic Acid Counteracts Cognitive Impairment in D-Galactose-Induced Aged Rats. Front. Nutr. 9:931734. doi: 10.3389/fnut.2022.931734
Received: 29 April 2022; Accepted: 13 June 2022;
Published: 05 July 2022.
Edited by:
David Vauzour, University of East Anglia, United KingdomReviewed by:
Sandeep Singh, Hebrew University Hadassah Medical School, IsraelCopyright © 2022 Ren, Sun, Xu, Xie, Ye and Zhao. This is an open-access article distributed under the terms of the Creative Commons Attribution License (CC BY). The use, distribution or reproduction in other forums is permitted, provided the original author(s) and the copyright owner(s) are credited and that the original publication in this journal is cited, in accordance with accepted academic practice. No use, distribution or reproduction is permitted which does not comply with these terms.
*Correspondence: Jianqin Sun, amlhbnFpbnNAMTYzLmNvbQ==
Disclaimer: All claims expressed in this article are solely those of the authors and do not necessarily represent those of their affiliated organizations, or those of the publisher, the editors and the reviewers. Any product that may be evaluated in this article or claim that may be made by its manufacturer is not guaranteed or endorsed by the publisher.
Research integrity at Frontiers
Learn more about the work of our research integrity team to safeguard the quality of each article we publish.