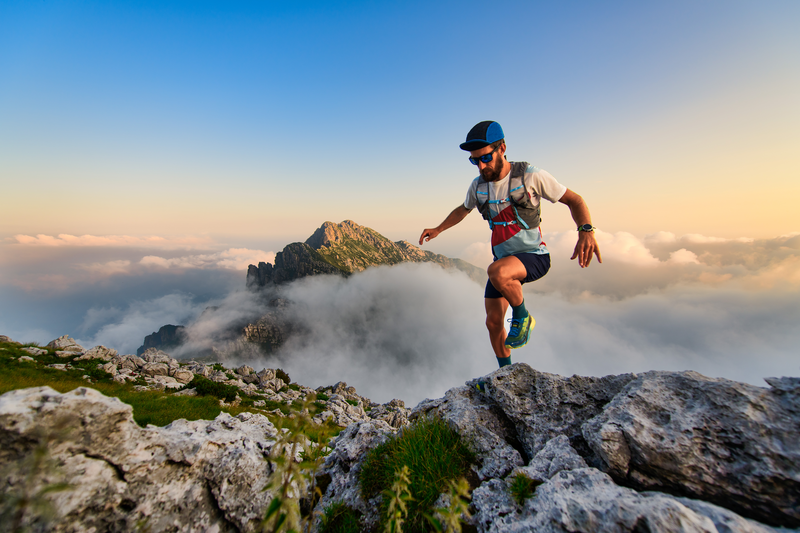
94% of researchers rate our articles as excellent or good
Learn more about the work of our research integrity team to safeguard the quality of each article we publish.
Find out more
ORIGINAL RESEARCH article
Front. Nutr. , 09 February 2022
Sec. Food Chemistry
Volume 9 - 2022 | https://doi.org/10.3389/fnut.2022.833906
This article is part of the Research Topic Enhancement of Nutritional Profile/Biological Activity of Plant-Based Foods by Fermentation View all 18 articles
A correction has been applied to this article in:
Expression of Concern: Fermentation and storage characteristics of “fuji” apple juice using Lactobacillus acidophilus, Lactobacillus casei and Lactobacillus plantarum: microbial growth, metabolism of bioactives and in vitro bioactivities
Fruit juices have been widely used as the substrates for probiotic delivery in non-dairy products. In this study, three lactic acid bacteria (LAB) strains, including Lactobacillus acidophilus, Lactobacillus casei and Lactobacillus plantarum, were selected to ferment apple juice. During 72-h of fermentation, these LAB strains grew well in the apple juice with significant increases in viable cell counts (from 7.5 log CFU/mL to 8.3 log CFU/mL) and lactic acid content (from 0 to 4.2 g/L), and a reduction of pH value (from 5.5 to around 3.8). In addition, the antioxidant and antibacterial capacities of fermented apple juice in vitro were significantly improved through the phenolic and organic acid metabolisms. After storage at 4°C for 30 days, the total amino acid content of fermented apple juice was significantly increased, although the viable cell counts and total phenolic content were decreased (p < 0.05). Furthermore, the stored fermented apple juices still possessed antibacterial and in vitro antioxidant activities. Overall, all the selected LAB strains could be suitable for apple juice fermentation and can effectively improve their biological activities.
Probiotics are living microorganisms and potential functional foods, which have a positive impact on the human body by improving the microbial balance in the human intestine (1). Lactic acid bacteria (LAB) are important probiotics, and they are often used in yogurt and other dairy products. In recent years, the researches on fermented fruit and vegetable juices by lactic acid bacteria have gradually emerged. Meanwhile, the related products in the Chinese market are still limited, which could not meet the consumer's demand. After the fermentation of fruit and vegetable juices by LAB strains, their nutritional and functional values are improved, which not only gives fruit and vegetable juice a special taste and taste, but also extends their shelf life (2, 3).
Apple is one of the most consumed fruits in the world, especially in “fuji” and related varieties which are widely accepted by consumers because of its excellent sensory properties (4, 5). They are characterized by high sugar content and low acidity (6). With the improvement of living standards, consumers are more concerned about the freshness, originality, physicochemical properties, and nutritional values of food products including fruit juice (7). Apple juice has some positive impacts on human health due to its rich contents of polyphenols such as isoflavones, flavonoids and phenolic acids (8). Although fruit juices are rich in minerals, sugars and vitamins, they contain low amounts of free peptides and amino acids, which is adverse to the growth and metabolism of human intestinal microorganisms (9). To address this problem, LAB strains are widely used for the fermentation of fruit and vegetable substrates due to their excellent tolerability in acidic environments. In addition, the nutrients of apples are retained due to the fermentation by probiotics, resulting in unique flavor and efficacy (8). In the literature, Chen et al. (10) studied the effect of four LAB strains on the flavors of fermented apple juice. The results showed that the total acid concentration and microbial activity under the fermentation of lactic acid bacteria increased markedly, and new volatile compounds with important flavors were produced. Kwaw et al. (11) reported that the effects of lactic acid bacteria on the color, phenolics (such as anthocyanins) and antioxidant activity of mulberry juice. The results found that lactic acid fermentation can enrich the bioactive components in mulberry juice. Similarly, Vivek et al. (12) found an increase trend of antioxidant activity, total phenolic and anthocyanin contents in “Sohiong” juice fermented by L. plantarum, confirming the suitability and feasibility of developing a non-dairy based probiotic drinks from Sohiong juice. Kaprasob et al. (13) also reported an increase in phenolic compounds, vitamin C and other antioxidants in cashew apple juices fermented by L. casei, L. plantarum and L. acidophilus. In theory, by using the selected three lactic acid bacteria, apple juice can be processed into value-added products. However, there is still limited researches about the effect of LAB on the fermentation and preservation of apple juice.
In this study, three potential probiotics, L. plantarum, L. acidophilus and L. casei, were selected to ferment apple juice. The changes of organic acids, sugars, free amino acids, and phenolics during fermentation and storage (4°C for 30 days) were evaluated. Finally, this study can provide the basic knowledge of the biotransformation of relevant components in apples by LAB fermentation, and guide the development of fermented apple juice products with high nutritional value and safety.
Fresh apple was provided by Xuanwu Fruit Store, Nanjing, Jiangsu Province. Ethanol, acetonitrile, Folin-Ciocalteu reagent, organic acid standards (oxalate acid, pyruvic acid, malic acid, citric acid, succinic acid and lactic acid), phenolic acid standards (gallic acid, protocatechuic acid, catechin, procyanidin B2, chlorogenic acid, hydroxybenzoic acid, caffeic acid, ferulic acid, rutin and quercetin), amino acid standards (Asp, Thr, Ser, Glu, Gly, Ala, Cys, Val, Met, Ile, Leu, Tyr, Phe, Lys, His, Arg, Pro) and sugar standards (fructose, sorbitol, glucose and sucrose) were all bought from Yuanye Biotechnology Co., Ltd (Shanghai, China).
Three commercial LAB strains L. acidophilus BNCC 185342, L. casei ATCC 393, L. plantarum BNCC 337796 were provided by Beijing Beina Chuanglian Biotechnology Research Institute (Beijing, China). All stains were activated in MRS broth (Bo Microbiology Technology Co., Ltd. Shanghai) at 37°C for 24 h to achieve a final concentration at 9.0 log CFU/mL.
Fresh apples (“Red Fuji” apple from Xuanwu Fruit Store, Nanjing, Jiangsu Province) were washed, peeled, nucleated, and cut into small pieces. Next, 0.15% (w/w) ascorbic acid (also known as vitamin C) was added during crushing. Then, apple slurries were filtered through four layers of gauze and centrifuged at 6,000 × g for 20 min. The supernatant was collected, and the soluble solid content and pH were adjusted to 13% using glucose and 6.5 using 1N NaOH, separately.
Apple juice was sterilized at 110°C for 10 min before fermentation. After cooling to room temperature, 8 mL of inoculum containing various LAB strains were added into 400 mL of apple juice, respectively. The initial viable bacterial count in apple juice was about 7.5 log CFU/mL. Next, apple juice with different LAB strains were placed into an incubator to ferment for 72 h at 37°C. After that, fermented juices were immediately stored at 4oC for 30 days. Unfermented apple juice was selected as a control group. Samples were collected for analysis during fermentation at 0, 12, 24, 48, 72 h and storage at 10, 20, 30 d. Before the physicochemical analysis, the collected samples were centrifuged at 4°C and 12,000 × g for 15 min.
The number of viable bacteria was determined by the plate counting method. Specifically, 1 mL fermented juices were diluted serially with sterile saline to 104-1010 dilutions. Inoculation of 0.1 mL of the diluted samples were plated on the MRS agar. The plates were incubated at 37°C for 36–48 h. Plates containing 30–300 colonies were counted and recorded as log CFU/mL (14). The pH of fermented apple juice was monitored by a digital pH meter (PHS-3C, Shanghai INESA Scientific Instrument Co., Ltd, Shanghai, China).
The browning index of unfermented and fermented apple juice samples were measured referring to Tiwari et al. (15). 3 mL of centrifuged apple juice was thoroughly mixed with 3 mL of anhydrous ethanol, and then the mixture was centrifuged at 4°C and 12,000 × g for 15 min. The supernatant was collected, and its absorbance was measured at 420 nm in a spectrophotometer (LabTech UV9100, USA).
Organic acids were determined by a LC-2010A system (Shimadzu Company, Japan) according to the previously reported method (16). The mobile phase is KH2PO4 (0.08 mol/L, pH 2.9). An Agilent TC-C18 chromatographic column (250 × 4.6 mm i.d., 5 mm) was applied for the separation of organic acids and it was maintained at 30°C. The injection volumes of the samples or standards were 20 μL, and the flow rate was 0.7 mL/min. Organic acids were detected using an ultraviolet detector (DAD) at 210 nm. The content of each organic acid was expressed as mg/L.
The sugars were determined by a HPLC-ELSD system (Agilent Technologies, USA., Alltech-ELSD 3300 evaporative light scattering detector: Otai Technology, USA) coupled with a Grace Prevail Carbohydrate ES column (250 × 4.6 mm i.d., 5 mm) according to the previously reported method (17). The mobile phase consisted of 25% (v/v) H2O and 75% (v/v) acetonitrile. The solvent flow rate was 1.0 mL/min and the injection volume was 10 μL. The column temperature was maintained at 30°C. The drift tube temperature of the ELSD detector was 80°C, the flow rate of nitrogen gas was 1.5 L/min. The content of each sugar was expressed as mg/L.
The apple juice collected at different time intervals was filtered through 0.45 μm membranes. The amino acids were analyzed by a L-8900 automatic amino acid analyzer (Hitachi Technology Company, Japan). The content of each free amino acid was expressed as mg/L.
The method for the determination of total phenols was the Folin-Ciocalteu method (18). Specifically, 0.2 mL of diluted apple juice sample was mixed with 1.5 mL of 7.5% (w/v) Na2CO3 and 1.5 mL of Folin-Ciocalteu (10-fold dilution). After standing at 25°C in darkness for 40 min, and the absorbance was measured at 765 nm. The total phenolic content was standardized against gallic acid and expressed as gallic acid equivalents/L.
The phenolic substances were analyzed using a Shimadzu-HPLC system coupled with an Inertsil ODS-3 column (250 × 4.6 mm i.d., 5 mm) (LC-2010A, Shimadzu Corporation, Japan) according to the previously reported method (19, 20). The column was maintained at 25°C, and the injection volume was 20 μL. The binary mobile phase consisted of (A) glacial acetic acid aqueous (1%, v/v) and (B) glacial acetic acid methanol (1%, v/v). The samples were eluted at a flow rate of 0.6 mL/min with the following gradients: 0–10 min, 10–26% B; 10–25 min, 10–26% B; 25–45 min, 40–65% B; 45–55 min, 65–95% B; 55–58 min, 95–100% B; 58–65 min, 100% B. Flavonoids and phenolic acids in samples were detected at 350 and 280 nm respectively. The results were expressed in mg/L.
The free radical scavenging activity of apple juice was assessed by the ABTS·+ method, as described in previous paper (21). The calibration curve was made using Trolox as the standard, and the results were expressed as mmol Trolox/L. Ferric reducing antioxidant power (FRAP) was determined according to our previously reported method (21). FeSO4 was employed as the standard to obtain the calibration curve, and the results were expressed as mmol Fe 2+/L.
The experiments were repeated three times. The test data were analyzed by Excel, Origin 8.0, Minitab, and SPSS 20.0 software. Significance was defined at p < 0.05, and Duncan's test was used.
Changes in viable cell counts and pH value of apple juice fermented with L. acidophilus, L. casei and L. plantarum during 72-h fermentation and the subsequent 30-day storage are shown in Figure 1, which can reflect the growth status of these strains. As can be seen from Figure 1A, L. acidophilus, L. casei and L. plantarum all grew well in the apple juice, and there was no significant difference in their viable cell count during the fermentation process (p ≥ 0.05). At the latter period of fermentation (from 48 to 72 h), the viable cell counts of L. acidophilus, L. casei and L. plantarum were still above 8.3 log CFU/mL. This result demonstrates that the growth of L. acidophilus, L. casei and L. plantarum metabolized vigorously. Meanwhile, this result also confirmed that apple juice can be a suitable substrate for the fermentation of the selected strains. Additionally, the viable cell counts of three LAB strains were maintained above 8.0 log CFU/mL in apple juice during the first 20 days of storage at 4°C. However, during the storage of 20–30 days, the viable cell counts of L. casei decreased rapidly to 5.4 ± 0.04 log CFU/mL, following L. acidophilus (5.6 ± 0.13 log CFU/mL) and L. plantarum (5.7 ± 0.04 log CFU/mL). These results are in line with the findings reported by Yoon et al. (22), which states that L. casei and L. plantarum were able to grow in cabbage juice without supplementing nutrients. With the increase of storage time, the substances that can be used by LAB in the apple juice were dramatically reduced with a marked accumulation of the metabolites (13). Eventually, the growth of the LAB reached the death phase, when the viable cell counts of LAB rapidly decreased.
Figure 1. Evolutions of viable cell counts (A), pH value (B) and browning index (C) in apple juices fermented by the three LAB strains during fermentation and refrigerated storage (4°C, 30 days).
LAB strains can metabolize sugar and produce organic acids, thus changing the pH value of apple juice during fermentation and storage (23). The change in pH values of apple juice fermented with L. acidophilus, L. casei and L. plantarum is shown in Figure 1B. The pH values of apple juice fermented by the three LAB strains had a minor change in the first 12 h of fermentation, and then experienced a rapid drop between 12 and 72 h (from 5.5~4.0). To be specific, after 48-h fermentation, the maximum reduction of pH value was obtained in apple juice fermented with L. casei (3.8 ± 0.02), following that fermented with L. acidophilus (3.9 ± 0.01) and L. plantarum (4.0 ± 0.02). In the storage period, the pH value remained stable, and there was no significant difference among the juices fermented by the three different strains (p ≥ 0.05). The variations of the pH values were consistent with the report of Chen et al. (10), who studied the effects of four LAB strains on the flavor characteristics of fermented apple juice.
Color is an important quality indicator for apple juice, and the fermentation of LAB in fruit and vegetable substrates can change their color (11). The change in color of apple juice fermented with L. acidophilus, L. casei and L. plantarum during the 72-h fermentation and the subsequent 30-day storage are shown in Figure 1C. The Browning Index value of apple juice increased slightly during the first 12 h of fermentation, and then decreased rapidly during the fermentation between 12 and 24 h, which was consistent with the variation of pH. The Browning Index value showed a downward trend during the period of 24-h fermentation and 30-day storage at 4°C. This may be due to the metabolism of phenolics in apple juice caused by LAB fermentation and the decrease of pH in apple juice, which inhibited the color change caused by Maillard reaction (24).
The changes in organic aicds and sugars in apple juice fermented with L. acidophilus, L. casei and L. plantarum during the 72-h fermentation and the subsequent 30-day storage are summarized in Supplementary Table 1. Organic acids are important products derived from LAB metabolism, which can improve the flavor and palatability of fruit juices (25). Meanwhile, organic acids can increase the acidity of the juice and inhibit the growth of spoilage and pathogen microorganisms. Therefore, organic acids have a profound influence on the storage characteristics and flavor of fermented fruit and vegetable juices (25). As shown in Supplementary Table 1, six organic acids including oxalic acid, pyruvic acid, malic acid, lactic acid, citric acid, and succinic acid can be identified in the fermented apple juice with L. acidophilus, L. casei and L. plantarum.
Lactic acid content was increased significantly after fermentation owing to the biosynthesis pathway of LAB metabolism, and the highest concentration was 4,191.6 ± 31.6 mg/L by L. casei. Malic acid is the main source of sour flavor in unfermented apple juice. Before fermentation, it had the highest content of 1,166.5 ± 14.7 mg/L in apple juice (26, 27). Malic acid can be bio-transferred to lactic acid under the catalysis of the malolactic enzyme produced by LAB, thus weakening the acidity and providing a mildly sour taste of apple juice (28). The existence of this metabolic pathway can be confirmed by the similarity between the increasing amount of lactic acid content and the decreasing amount of malic acid content.
Citric acid content (730.7 ± 13.1 mg/L in unfermented juice) was decreased after lactic acid fermentation as a result of its decomposition into various products, such as diacetyl, lactic acid, and acetic acid (29). Pyruvate is an intermediate product of the basic metabolic pathways of organisms such as tricarboxylic acid cycle, glycolysis, and other metabolic pathways. The pyruvic acid content experienced an increasing trend and reached a maximum concentration of 130.2 ± 1.4 mg/L after fermentation by L. plantarum for 24 h, and then followed a decreasing trend. It has been confirmed that pyruvic acid can be produced from glucose through glycolysis, which may be related to the rising phase of pyruvic acid. In addition, pyruvic acid can also be used to form the amino acid alanine and converted into lactic acid or ethanol via fermentation, which may be associated with its decline at the end of fermentation (30). The trends in the content of oxalic acid and succinic acid in fermented apple juice were similar to that of pyruvic acid. In addition, the content of total organic acids increased during fermentation and then remained stable during the storage period, which may be due to the inhibition of the metabolism of lactic acid bacteria at low temperatures.
Before fermentation, fructose is the major sugar (52.0 ± 2.3 mg/L) in apple juice. During the fermentation process, the content of glucose in all fermented samples experienced a continuous decrease from 17.7 ± 0.8 mg/L to about 11.7 ± 0.1 mg/L, although there was no significant difference in the content of glucose among the apple juice fermented with different microbial strains (p ≥ 0.05). This trend can be explained by the reason that the fermentation of the selected LAB strains is facultative heterologous fermentation, and glucose and fructose are converted into lactic acid through the Embden-Meyerhof pathway. The content of sucrose in apple juice fermented with L. acidophilus, L. casei and L. plantarum was reduced continuously, which can be attributed to the hydrolysis of sucrose by galactosidase in LAB strains. This trend is consistent with the results of Wang et al. (31) who used LAB strains to ferment soy milk. The contents of glucose, fructose and sorbitol were also increased significantly (P < 0.05) during storage. This may be due to the inhibition of the respiration of LAB strains by monosaccharides in an environment of low temperature and high acidity. Meanwhile, the degradation of sucrose by galactosidase may also take place in LAB strains (32–34).
Amino acids are important flavoring compounds and their compositions have a profound influence on the evaluation of sensory properties of apple juice (35). For LAB fermentation, amino acids may first undergo decarboxylation, deamination, transamination, and desulfurization reactions, followed by amine conversion. Eventually, the aldehydes generated from amino acid metabolism are oxidized or reduced to the corresponding carboxylic acids and alcohols (36). Seventeen free amino acids could be detected in the apple juice samples fermented by the three LAB strains. Among them, Thr possessed the highest amount in unfermented samples (695.3 ± 13.2 mg/L). To clarify the effect of LAB strains on the metabolism of amino acids, two-dimensional analysis of the principal component analysis (PCA) was used to analyze the profile of free amino acids during 72-h fermentation. Meanwhile, the original data about the content of each amino acid are prepared in Table 1. Two principal components, PC1 and PC2, were extracted that accounted for 67.7% and 19.7% of the total variance in seventeen variable systems, respectively. The unfermented sample was distributed on the negative side of PC1. During 72-h fermentation, a significant shift of the distribution could be observed among fermented apple juice (Figure 2A). This phenomenon indicates that the profile of amino acids in apple juice changed obviously during 72-h fermentation. For example, the PC1 value of unfermented apple juice was−7.31. After fermentation for 72 h, the PC1 values for apple juice fermented with L. acidophilus, L. plantarum, and L. casei increased to 2.42, 2.49, and 2.47. According to the loading plot (Figure 2B), Leu, Ala, Val, His, Ile, Met, Thr and Phe distributed on the negative side of PC1, and Tyr, Lys, Pro, Arg, Glu, Asp, Cys and Gly distributed on the positive side. These amino acids placed to the right such as Pro, Cys and Gly in the loading plot were close and positively correlated. However, amino acids on the negative side were negatively correlated. In addition, some amino acids including Leu, Val, Ala, His, Ile, Met, Thr, Phe, Pro, Cys, Gly and Asp distributed far from the origin of the first PC. Therefore, LAB fermentation of apple juice may have a higher influence on the content of these amino acids. Based on the locations of samples and the attributes, it can be found that the contents of Leu, Ala, Val, His, Ile, Met, Thr and Phe in apple juice were generally decreased with fermentation. In contrast, the lactic acid fermentation led to the increment of the amounts of Tyr, Lys, Pro, Arg, Glu, Asp, Cys and Gly. Besides, it can be observed that the fermented apple juice by the three microbial strains at the same stage had similar PC1 values, indicating that PC2 is mainly responsible for the discrimination of the samples fermented by different strains. For example, after 72-h fermentation, the contents of Asp, Cys and Gly in apple juice fermented with L. acidophilus, L. plantarum, and L. casei were 979.4 ± 1.0 mg/L, 972.9 ± 1.0 mg/L, 969.3 ± 16.8 mg/L; 6.2 ± 0 mg/L, 6.2 ± 0.2 mg/L, 5.8 ± 0.1 mg/L and 80.3 ± 1.2 mg/L, 86.9 ± 1.7 mg/L, 85.3 ± 1.2 mg/L, respectively. And the PC2 values of Asp, Cys and Gly were−0.17, 0.17, and 0.10 (Figure 2B). This result implies that different strains have different capacities to metabolize some amino acids such as Asp, Cys and Gly and others.
Table 1. Changes in the contents of free amino acids (mg/L) of apple juice during lactic acid fermentation and the subsequent refrigerated storage period (4°C, 30 days).
Figure 2. Principal component analysis of free amino acid profile in apple juice during fermentation by the three LAB strains [(A), score plot; (B), loading plot] and changes in total free amino acids (C). “Control,” Unfermented apple juice; “La,” Apple juice fermented by L. acidophilus; “Lp”, Apple juice fermented by L. plantarum; “Lc”, Apple juice fermented by L. casei. In (A), the number followed with the name of strain indicated the time of fermentation.
The contents of Asp and Gly were increased significantly during fermentation (P < 0.05), which may improve the flavor of fermented apple juice (37). LAB can metabolize threonine to pyruvate and ammonia through threonine dehydratase, resulting in a significant decrease in threonine content (P < 0.05) during the first 24-h fermentation. It has been reported that lactic acid bacteria can alleviate environmental acid stress by increasing the expression of enzymes consuming cytoplasm (38). Ammonia produced by amino acid metabolism can accept protons and promote acid-base balance in microbial cells (29), which is confirmed by the increase in ammonia contents from 3.5 ± 0.1 mg/L to 121.0 ± 0.2 mg/L, 121.0 ± 2.4 mg/L, 120.2 ± 0.01 mg/L during fermented by L. acidophilus, L. plantarum, and L. casei, respectively. Moreover, the contents of Phe, Val, Ile and Met also showed a downward trend throughout fermentation, which may be related to the decarboxylase (4) and transaminase (39, 40) produced by LAB. Decarboxylase decomposes free amino acids to produce primary amine and release carbon dioxide, while transaminases can catalyze the inter-conversion of amino acids into alpha-ketonic acid (38) and the transamination reaction between branched-chain amino acids (i.e., leucine) and alpha-keto-beta-methyl valerate/alpha-keto isopropionate (41).
During storage, almost all the mentioned amino acids were increased significantly during the first 10-day storage (P < 0.05). To be specific, the contents of Asp, Phe and Gly in apple juice fermented by L. plantarum increased from about 972.9 ± 1.0 mg/L, 7.2 ± 0.1 mg/L and 86.9 ± 1.7 mg/L to approximately 1496.4 ± 20.2 mg/L, 11.7 ± 0.1 mg/L and 106.8 ± 1.6 mg/L, respectively. Meanwhile, the contents of Met, Ile, Leu had no obvious changes in the early period of storage. In the contrast, the content of Thr was decreased from 370.6 mg/L to below 1 mg/L. In the subsequent storage period, there was no significant change in the content of all the amino acids (P ≥ 0.05).
Besides, the content of total amino acids in apple juice fermented by three LAB strains was studied. The results are shown in Figure 2C. The total amino acid content in fermented apple juice was decreased slightly in the first 24 h of fermentation, and then increased gradually. During the first 10 days of storage at 4°C, the content of total amino acids in apple juice fermented by L. acidophilus, L. plantarum, and L. casei were increased from 1.88 ± 0.002 g/L, 1.88 ± 0.01, and 1.88 ± 0.04 g/L to 2.26 ± 0.02 g/L, 2.22 ± 0.02 g/L, and 2.27 ± 0.03 g/L, respectively. Then maintained at this level until the end of storage.
Gallic acid, protocatechuic acid, catechin, proanthocyanidin B2, chlorogenic acid, p-hydroxybenzoic acid, coffee acid, syringic acid, phloxic acid, p-coumaric acid, ferulic acid, cinnamaldehyde, p-vinyl guaiacol, rutin and quercitrin can be tentatively identified in the fermented apple juice. Gallic acid, protocatechuic acid, catechin, proanthocyanidin B2, caffeic acid, rutin ferulate and quercetin were the main phenolic substances in fermented apple juice, and their contents were summarized in Table 2. After 48-h fermentation by L. acidophilus, L. plantarum, and L. casei, the content of ferulic acid was declined by 11.1, 18.5, and 11.1%, and the caffeic acid content was reduced by 41.7, 50, and 50%, respectively. These results indicated that the selected LAB strains were able to biotransform ferulic acid and caffeic acid. This phenomenon can be explained by two possible pathways. One is that phenolic acid decarboxylases synthesized by LAB can catalyze the non-oxidative decarboxylation of phenolic acids to generate their corresponding p-vinyl derivatives (42). Another reason is that ferulic acid and caffeic acid could be transformed to dihydrocaffeic acid and dihydroferulic acids through the side chain hydrogenation (43–46). The ferulic acid content in apple juice fermented by L. plantarum was decreased faster than those fermented by L. acidophilus and L. casei (P < 0.05), guessing that L. plantarum was more capable to synthesize the enzymes involved in the metabolism of ferulic acid.
Table 2. Changes in the contents of individual phenolic compounds (mg/L) of apple juices during lactic acid fermentation and the subsequent refrigerated storage period (4°C, 30 days).
The content of ferulic acid was significantly decreased from around 4.7 mg/L to about 0.8 mg/L (P < 0.05) after storage for 10 days, which may also be related to the metabolism of ferulic acid into 4-vinyl guaiacol and other substances (43). Also, caffeic acid content increased rapidly, which could be attributed to the conversion of dihydrocaffeic acid to caffeic acid (47). Meanwhile, the content of catechin in apple juice fermented by L. acidophilus, L. plantarum, and L. casei were increased from 10.1 ± 0.1 mg/L, 9.9 ± 0.3 mg/L, 10.5 ± 0.4 mg/L to 12.6 ± 0.9 mg/L, 14.0 ± 0.5 mg/L, and 14.7 ± 0.3 mg/L while the content of protocatechuic acid were decreased from 24.4 ± 0.09 mg/L, 22.8 ± 2.6 mg/L, 25.1 ± 0.3 mg/L to 16.2 ± 0.8 mg/L, 18.9 ± 2.5 mg/L, and 18.2 ± 0.6 mg/L in the first 10-day storage. It has been reported that LAB can metabolize protocatechuic acid to catechin through the decarboxylation reaction (48). The study of Annalisa et al. also demonstrated the metabolic correlation between protocatechuic acid and catechin (49). It was also found that the changes of rutin and quercetin were almost the same during fermentation and storage at 4°C. Moreover, the contents of rutin and quercetin were almost stable throughout fermentation and there was no significant difference among the three LAB fermented samples (P ≥ 0.05). However, the contents of rutin and quercetin in all the samples decreased significantly after storage for 10 days (P < 0.05), which may be attributed to the uncoupling of beta-glucosidase in lactic acid bacteria (50). Meanwile, rutin and quercetin could be biotransformed to low molecular weight metabolites, such as 2-(3,4-dihydroxyphenyl) acetic acid, 2-(3-hydroxyphenyl) acetic acid, 3,4-dihydroxybenzoic acid and others (51). The gallic acid content was increased during 72-h fermentation, but decreased during storage at 4°C for 30 days. In contrast, the content of proanthocyanidin B2 was decreased rapidly within the first 12 h of fermentation and then stayed stable in the following 36 h of fermentation. Similar to gallic acid, the proanthocyanidin B2 content was decreased significantly during storage.
The changes of individual phenolic compounds during 72-h fermentation were analyzed by PCA (Figures 3A,B). PC1 and PC2 explained 94% of the total variance of all the “fuji” apple juices. According to Figure 3A, the unfermented sample was located on the positive side of PC1. And apple juices fermented with L. acidophilus, L. plantarum, and L. casei at different stage were distributed on the different PC1 region, implying that PC1 can be considered as a contributor to distinguish the unfermented and fermented samples. In the loading plot of phenolic acids (Figure 3B), three phenolics, including cinnamaldehyde, phloretin, and p-vinyl guaiacol, were located on the negative side of PC1. In contrast, the other 13 phenolic acids were located on the positive side of PC1. Among them, p-vinyl guaiacol, phloretin, cinnamaldehyde, quercetin and ferulic acid were placed nearby the origin of first PC. Therefore, LAB fermentation may have minor effects on these phenolic acids. However, protocatechuic acid, gallic acid, p-hydroxybenzoic acid, p-coumaric acid, chlorogenic acid and syringic acid were all placed far from the origin of first PC and positively correlated. This result implied that LAB fermentation has some positive effects on the phenolic acids in apple juice.
Figure 3. Principal component analysis of phenolic profile in apple juice during fermentation by the three LAB strains [(A), the score plots; (B), the loading plots] and changes in total phenolic content (C). “Control,” Unfermented apple juice; “La,” Apple juice fermented by L. acidophilus; “Lp”, Apple juice fermented by L. plantarum; “Lc”, Apple juice fermented by L. casei. The number followed with the name of strain indicated the time of fermentation.
The changes in total phenolic content in apple juices fermented by the three LAB strains are shown in Figure 3C. Overall, the total phenolic content was declined after 72-h fermentation (P < 0.05). During the first 12 h of fermentation, the total phenolic contents of apple juice fermented with different strains all declined from 1.37 g/L to around 1.27 g/L. However, the total phenolic content in apple juice fermented by L. casei was increased to 1.35 ± 0.01 g/L after 72 h of fermentation (P < 0.05), while that in apple juice fermented by L. plantarum was decreased to 1.27 ± 0.02 g/L. The loss of phenolics may be due to the interaction between phenolics and proteins in apple juice during fermentation, which could produce insoluble complexes (4). Another potential reason is that phenolics are the inhibitors of the growth of L. plantarum, and LAB can synthetize a series of enzymes (benzyl alcohol dehydrogenase, decarboxylase, tannase) to degrade phenolics (42, 52). The total phenolic contents in apple juice fermented by the three LAB strains were close after fermentation. In the first 10 day of storage, the total phenolic content decreased continuously to about 0.95 g/L. After that, no significant change in the total phenolic content was observed in the subsequent 20-day storage. Similar results were found by Wang et al. (53), the total phenolic content of fermented mango juice experienced a rapid decrease during the first 10-d storage and then stable. This phenomenon may be caused by the presence of dissolved oxygen which resulted in the oxidation of phenolic compounds during the first 10-d storage (54). However, phenolic compounds were more stable with the consumption of oxygen during the later storage stage.
The antioxidant properties of apple juice are mainly related to vitamin C and phenolics (55, 56). ABTS·+ method (free radical scavenging capacity) and FRAP method (iron reduction capacity) were used to evaluate the effect of LAB fermentation on the antioxidant activity of apple juice in vitro. The results of antioxidant activity of fermented apple juice are shown in Figure 4. Generally, LAB fermentation enhanced the ABTS·+ radical scavenging capacity of the apple juice fermented by L. acidophilus, L. plantarum, and L. casei were increased from 36.41 ± 1.1 mmol Trolox/L to 49.22 ± 3.0 mmol Trolox/L, 54.03 ± 1.7 mmol Trolox/L, 51.94 ± 2.4 mmol Trolox/L, which was consistence with the trend in pomegranate juice (57) and jussara pulp (58) fermented by LAB. On the other hand, both ABTS·+ radical scavenging capacity and ferric reducing power of fermented apple juice declined significantly during the first 10-day storage, which could be related to the aforementioned decrease of total phenolic content in fermented apple juice. In the next 20-day storage, their trends have different performance. The ferric reducing power was stable, whereas the ABTS·+ radical scavenging capacity was even increased. Overall, the ferric reducing ability of fermented apple juice has similar trend with the change of total phenolic acid content whereas the ABTS·+ radical scavenging capacity of fermented apple juice were increased during fermentation. Such inconsistent changes in ferric reducing ability and ABTS·+ radical scavenging capacity of fermented juice were also found by Wang et al. (9) who used LAB to ferment apple juice. There are two possible reasons for this phenomenon. First one is that the dissolved oxygen and microbial strains can affect the ABTS·+ radical scavenging capacity of fermented apple juice (9). Another one is that the bioconversion of phenolic acids during fermentation (59). Ozgen et al. (60) compared the ABTS·+ radical scavenging activities of four phenolic acids (gallic acid, chlorogenic acid, caffeic acid and ascorbic acid), and reported that gallic acid had the highest ABTS·+ radical scavenging activities, followed by caffeic acid. Zhang et al. (61) illustrated that gallic acid and caffeic acid had more contribution to the ABTS·+ radical scavenging activity as compared to ferulic acid. And in our study, we also found an increase in these phenolic acid contents (gallic acid, caffeic acid and so on).
Figure 4. The antioxidant activity of unfermented and LAB-fermented apple juice in vitro. (A) ABTS·+ radical scavenging activity, (B) ferric reducing antioxidant power.
In addition, microbial contamination is an important reason of food spoilage. E. coli (G−) and S. aureus (G+) are the most common microorganisms in contaminated food (62). In this study, the antimicrobial activities of unfermented and fermented apple juice against the growth of E. coli and S. aureus were evaluated, and the results are shown in Figure 5. Before fermentation, the samples had weak antibacterial activities. After 72-h fermentation, the diameters of the inhibition circles against E. coli and S. aureus were 23.78 ± 0.1 mm and 17.53 ± 1.0 mm for the apple juice fermented by L. plantarum, 23.74 ± 1.1 mm and 16.81 ± 0.6 mm for the juice fermented by L. casei, and 23.76 ± 0.9 mm and 17.13 ± 0.8 mm for the juice fermented by L. acidophilus. It is supposed that the antibacterial properties of fermented apple juice may be related to bacteriocin, diacetyl, organic acid and hydrogen peroxide produced by lactic acid bacteria metabolism (63). Although LAB fermentation improved the antimicrobial activities, there was no significant difference in the areas of inhibition zone of apple juice fermented by the three LAB strains after 72-h fermentation. Interestingly, after storage for 30 days, the diameter of the inhibition circle against E. coli for all the juices even increased. The apple juice fermented with L. acidophilus had the largest inhibition circles (26.41 ± 0.3 mm) followed by those fermented with L. plantarum (25.03 ± 0.6 mm) and L. casei (24.31 ± 0.9 mm). In addition, the diameter of the inhibition circle against S. aureus for the samples fermented with L. acidophilus did not change significantly after 30-day storage, whereas the antimicrobial ability of samples fermented with L. casei and L. plantarum against S. aureus got weakened with storage. Generally, the antimicrobial activities of unfermented and fermented apple juice can be contributed by the acid production, these acids can lower pH and create an unfavorable environment for pathogens and deteriorating microorganisms (64). Additionally, LAB can produce some antimicrobial compounds such as H2O2 and bacteriocin, which can inhibit microbial growth (21). Therefore, such changes in antimicrobial activities after fermentation and storage may be influenced by the change of organic acids and viable cell counts of LAB.
Figure 5. The antimicrobial activity of unfermented and LAB-fermented apple juice against the growths of (A) E. coli and (B) S. aureus in vitro. Values with different letters within each samples indicated a significant difference in content (p < 0.05).
In this study, three commercial LAB strains including L. acidophilus, L. casei, and L. plantarum were utilized to ferment apple juice. All the microbial strains presented satisfactory growth capacities in the studied apple juice environment. In general, LAB fermentation improved the antioxidant and antimicrobial capacities by metabolizing phenolics and producing lactic acid. In addition, the changes in the physicochemical properties of fermented apple juice during storage at 4°C for 30 days was also evaluated. The results showed that the total amino acid content was significantly increased, but the total phenolic content was significantly decreased. Overall, these results prove that lactic acid fermentation is a useful method to enhance the nutritional value of apple juice.
The original contributions presented in the study are included in the article/Supplementary Material, further inquiries can be directed to the corresponding author/s.
JY performed the experiments, analyzed the data, and wrote the manuscript. YS, TG, and YW analyzed the data and wrote the manuscript. HS, QZ, and CL analyzed and discussed the data. CZ, YH, and YT provided samples and discussed the data. YT designed the research content, analyzed the data, and modified the manuscript. All authors read and approved the final manuscript.
This work was financially supported by grants from the National Natural Science Foundation of China (No. 32100037), Natural Science Research General Project of Jiangsu Higher Education Institutions (No. 20KJB550008), Science and Technology Program of Kizilsu Kirghiz Autonomous Prefecture in 2021 (No. 3-25), Jiangsu Planned Projects for Postdoctoral Research Funds (No. 2019K015), Priority Academic Program Development of Jiangsu Higher Education Institutions (PAPD), and the Research and Practice Innovation Program for College Graduates of Jiangsu Province (No. KYCX20_2888).
The authors declare that the research was conducted in the absence of any commercial or financial relationships that could be construed as a potential conflict of interest.
All claims expressed in this article are solely those of the authors and do not necessarily represent those of their affiliated organizations, or those of the publisher, the editors and the reviewers. Any product that may be evaluated in this article, or claim that may be made by its manufacturer, is not guaranteed or endorsed by the publisher.
The Supplementary Material for this article can be found online at: https://www.frontiersin.org/articles/10.3389/fnut.2022.833906/full#supplementary-material
1. Yuasa M, Shimada A, Matsuzaki A, Eguchi A, Tominaga M. Chemical composition and sensory properties of fermented citrus juice using probiotic lactic acid bacteria. Food Biosci. (2020) 39:100810. doi: 10.1016/j.fbio.2020.100810
2. Dogan K, Akman PK, Tornuk F. Role of non-thermal treatments and fermentation with probiotic Lactobacillus plantarum on in vitro bioaccessibility of bioactives from vegetable juice. J Sci Food Agr. (2021) 101:4779–88. doi: 10.1002/jsfa.11124
3. Wang Z, Feng Y, Yang N, Jiang T, Xu H, Lei H. Fermentation of kiwifruit juice from two cultivars by probiotic bacteria: bioactive phenolics, antioxidant activities and flavor volatiles. Food Chem. (2022) 373:131455. doi: 10.1016/j.foodchem.2021.131455
4. Ankolekar C, Johnson K, Pinto M, Johnson D, Labbe RG, Greene D, et al. Fermentation of whole apple juice using lactobacillus acidophilus for potential dietary management of hyperglycemia, hypertension, and modulation of beneficial bacterial responses. J Food Biochem. (2012) 36:718–38. doi: 10.1111/j.1745-4514.2011.00596.x
5. Varela P, Salvador A, Fiszman S. Shelf-life estimation of 'Fuji' apples: Sensory characteristics and consumer acceptability. Postharvest Biol Tec. (2005) 38:18–24. doi: 10.1016/j.postharvbio.2005.05.009
6. Zhu G, Tian C. Determining sugar content and firmness of "Fuji' apples by using portable near-infrared spectrometer and diffuse transmittance spectroscopy. J Food Process Eng. (2018) 41:e12810.1–10. doi: 10.1111/jfpe.12810
7. Ross JA, Kasum CM. Dietary flavonoids: bioavailability, metabolic effects, and safety. Annu Rev Nutr. (2002) 22:19–34. doi: 10.1146/annurev.nutr.22.111401.144957
8. Wang X, Han M, Zhang M, Wang Y, Ren Y, Yue T, et al. In vitro evaluation of the hypoglycemic properties of lactic acid bacteria and its fermentation adaptability in apple juice. LWT- Food Sci Technol. (2021) 136:110363. doi: 10.1016/j.lwt.2020.110363
9. Wang H, Tao Y, Li Y, Wu S, Li D, Liu X, et al. Application of ultrasonication at different microbial growth stages during apple juice fermentation by Lactobacillus plantarum: Investigation on the metabolic response. Ultrason Sonochem. (2021) 73:105486. doi: 10.1016/j.ultsonch.2021.105486
10. Chen C, Lu Y, Yu H, Chen Z, Tian H. Influence of 4 lactic acid bacteria on the flavor profile of fermented apple juice. Food Biosci. (2019) 27:30–6. doi: 10.1016/j.fbio.2018.11.006
11. Kwaw E, Ma YK, Tchabo W, Apaliya MT, Wu M, Sackey AS. Effect of lactobacillus strains on phenolic profile, color attributes and antioxidant activities of lactic-acid-fermented mulberry juice. Food Chem. (2018) 250:148–54. doi: 10.1016/j.foodchem.2018.01.009
12. Vivek K, Mishra S, Pradhan RC, Jayabalan R. Effect of probiotification with Lactobacillus plantarum MCC 2974 on quality of Sohiong juice. LWT-Food Sci Technol. (2019) 108:55–60. doi: 10.1016/j.lwt.2019.03.046
13. Kaprasob R, Kerdchoechuen O, Laohakunjit N, Sarkar D, Shetty K. Fermentation-based biotransformation of bioactive phenolics and volatile compounds from cashew apple juice by select lactic acid bacteria. Process Biochem. (2017) 59:141–9. doi: 10.1016/j.procbio.2017.05.019
14. Balestra GM, Misaghi IJ. Increasing the efficiency of the plate counting method for estimating bacterial diversity. J Microbiol Methods. (1997) 30:111–7. doi: 10.1016/S0167-7012(97)00056-0
15. Tiwari BK, Muthukumarappan K, Odonnell CP. Colour degradation and quality parameters of sonicated orange juice using response surface methodology. LWT-Food Sci Technol. (2008) 41:1876–83. doi: 10.1016/j.lwt.2007.11.016
16. Lima M, Silani I, Toaldo IM, Correa LC, Biasoto ACT, Pereira GE. Phenolic compounds, organic acids and antioxidant activity of grape juices produced from new Brazilian varieties planted in the northeast region of Brazil. Food Chem. (2014) 161:94–103. doi: 10.1016/j.foodchem.2014.03.109
17. Wasik A, Mccourt J, Buchgraber M. Simultaneous determination of nine intense sweeteners in foodstuffs by high performance liquid chromatography and evaporative light scattering detection—Development and single-laboratory validation. J Chromatogr A. (2007) 1157:187–96. doi: 10.1016/j.chroma.2007.04.068
18. A MRR, B RCB. Quantification of total phenols in bio-oil using the Folin–Ciocalteu method–sciencedirect. J Anal Appl Pyrol. (2013) 104:366–71. doi: 10.1016/j.jaap.2013.06.011
19. Zhang W, Han F, He J, Duan C. HPLC-DAD-ESI-MS/MS analysis and antioxidant activities of nonanthocyanin phenolics in mulberry (Morus alba L). J Food Sci. (2008) 73:C512–518. doi: 10.1111/j.1750-3841.2008.00854.x
20. Abid M, Jabbar DS, Wu T, Hashim MM, Hu B, Lei S. Sonication enhances polyphenolic compounds, sugars, carotenoids and mineral elements of apple juice. Ultrason Sonochem. (2013) 21:93–7. doi: 10.1016/j.ultsonch.2013.06.002
21. Wang Y, Tao Y, Zhang X, Shao S, Han Y, Chu DT. Metabolic profile of ginkgo kernel juice fermented with lactic aicd bacteria: a potential way to degrade ginkgolic acids and enrich terpene lactones and phenolics. Process Biochem. (2019) 76:25–33. doi: 10.1016/j.procbio.2018.11.006
22. Yoon KY, Woodams EE, Hang YD. Production of probiotic cabbage juice by lactic acid bacteria. Bioresour Technol. (2006) 97:1427–30. doi: 10.1016/j.biortech.2005.06.018
23. Ricci A, Cirlini M, Levante A. Volatile profile of elderberry juice: Effect of lactic acid fermentation using L. plantarum, L rhamnosus and L casei strains. Food Res Int. (2018) 105:412–22. doi: 10.1016/j.foodres.2017.11.042
24. Verardo V, Moreno-Trujillo TR, Caboni MF, Garcia-Villanova B, Guerra-Hernandez EJ. Influence of infant cereal formulation on phenolic compounds and formation of Maillard reaction products. J Food Compos Anal. (2021) 104:104187. doi: 10.1016/j.jfca.2021.104187
25. Pimentel TC, Madrona GS, Garcia S, Prudencio SH. Probiotic viability, physicochemical characteristics and acceptability during refrigerated storage of clarified apple juice supplemented with Lactobacillus paracasei ssp. paracasei and oligofructose in different package type. LWT-Food Sci Technol. (2015) 63:415–22. doi: 10.1016/j.lwt.2015.03.009
26. Toit M, Engelbrecht L, Lerm E, Krieger-Weber S. Lactobacillus: the next generation of malolactic fermentation starter cultures—an overview. Food Bioprocess Tech. (2011) 4:876–906. doi: 10.1007/s11947-010-0448-8
27. Ye M, Yue T, Yuan Y. Polyphenols and organic acids evolution during apple cider fermentation. J Sci Food Agr. (2014) 94:2951–7. doi: 10.1002/jsfa.6639
28. Zhang H, Zhou F, Ji B, Nout RMJ, Yang FZ. Determination of organic acids evolution during apple cider fermentation using an improved HPLC analysis method. Eur Food Res Technol. (2008) 22:1183–90. doi: 10.1007/s00217-008-0835-9
29. Dudley EG, Steele JL. Succinate production and citrate catabolism by cheddar cheese nonstarter lactobacilli. J Appl Microbiol. (2010) 98:14–23. doi: 10.1111/j.1365-2672.2004.02440.x
30. Abdelrahman MA, Tashiro Y, Sonomoto K. Recent advances in lactic acid production by microbial fermentation processes. Biotechnol Adv. (2013) 31:877–902. doi: 10.1016/j.biotechadv.2013.04.002
31. Wang YC, Yu RC, Yang HY, Chou CC. Sugar and acid contents in soymilk fermented with lactic acid bacteria alone or simultaneously with bifidobacteria. Food Microbiol. (2003) 20:333–8. doi: 10.1016/S0740-0020(02)00125-9
32. Ortiz-Cortés LY, Ventura-Canseco L, Abud-Archila M, Ruíz-Valdiviezo VM, Velázquez-Ríos IO, Alvarez-Gutiérrez PE. Evaluation of temperature, pH and nutrient conditions in bacterial growth and extracellular hydrolytic activities of two alicyclobacillus spp. strains Arch Microbiol. (2021) 203:1–14. doi: 10.1007/s00203-021-02332-4
33. Hughes DB, Hoover DG. Viability and enzymatic activity of Bifidobacteria in Milk. J Dairy Sci. (1995) 78:268–76. doi: 10.3168/jds.S0022-0302(95)76634-6
34. KMital B, Shallenberger RH, Steinkraus K. α-Galactosidase activity of Lactobacilli. J Appl Microbiol. (1973) 26:783–8. doi: 10.1128/am.26.5.783-788.1973
35. Bermdez R, Franco D, Carballo J. Influence of muscle type on the evolution of free amino acids and sarcoplasmic and myofibrillar proteins through the manufacturing process of Celta dry-cured ham. Food Res Int. (2014) 56:226–35. doi: 10.1016/j.foodres.2013.12.023
36. Mcsweeney P, Sousa M. Biochemical pathways for the production of flavour compounds in cheeses during ripening: a review. Lait. (2000) 80:293–324. doi: 10.1051/lait:2000127
37. Mau JL, Tseng YH. Nonvolatile taste components of three strains of Agrocybe cylindracea. J Agr Food Chem. (1998) 46:2071–4. doi: 10.1021/jf971016k
38. Zuljan FA, Mortera P, Alarcón SH, Blancato VS, Espariz M, Magni C. Lactic acid bacteria decarboxylation reactions in cheese. Inter Dairy J. (2016) 62:53–62. doi: 10.1016/j.idairyj.2016.07.007
39. Liesbeth R, Mireille Y, Richard van K, Pascal C, Annette V, Emilie C, et al. Lactococcal aminotransferases Arat and Bcat are key enzymes for the formation of aroma compounds from amino acids in cheese. Int Dairy J. (2003) 13:805–12. doi: 10.1016/S0958-6946(03)00102-X
40. Tanous C, Gori A, Rijnen L, Chambellon E, Yvon M. Pathways for -ketoglutarate formation by Lactococcus lactis and their role in amino acid catabolism. Int Dairy J. (2004) 15:759–70. doi: 10.1016/j.idairyj.2004.09.011
41. Thierry A, Mailard MB, Yvon M. Conversion of L-Leucine to isovaleric acid by Propionibacterium freudenreichii TL 34 and itgp23. Appl Environ Microb. (2002) 68:608–15. doi: 10.1128/AEM.68.2.608-615.2002
42. Rodriguez H, Curiel JA, Landete JM. Food phenolics and lactic acid bacteria. Int J Food Microbiol. (2009) 132:79–90. doi: 10.1016/j.ijfoodmicro.2009.03.025
43. Knockaert D, Raes K, Wille C, Struijs K, Camp JV. Metabolism of ferulic acid during growth of Lactobacillus plantarum and Lactobacillus collinoides. J Sci Food Agr. (2012) 92:2291–6. doi: 10.1002/jsfa.5623
44. Filannino P, Bai Y, Cagno R, Gobbetti M, Gaenzle MG. Metabolism of phenolic compounds by Lactobacillus spp. during fermentation of cherry juice and broccoli puree. Food Microbiol. (2014) 46:272–9. doi: 10.1016/j.fm.2014.08.018
45. Sanchez-Maldonado AF, Schieber A, Ganzie MG. Structure-function relationships of the antibacterial activity of phenolic acids and their metabolism by lactic acid bacteria. J Appl Microbiol. (2011) 111:1176–84. doi: 10.1111/j.1365-2672.2011.05141.x
46. Huang J, Depaulis T, May J. Antioxidant effects of dihydrocaffeic acid in human EA. hy926 endothelial cells. J Nutr Biochem. (2005) 15:722–9. doi: 10.1016/j.jnutbio.2004.07.002
47. Ricci A, Cirlini M, Calani L. In vitro metabolism of elderberry juice polyphenols by lactic acid bacteria. Food Chem. (2019) 276:692–9. doi: 10.1016/j.foodchem.2018.10.046
48. Tabasco R. Snchez-pat NF, Monagas M, Bartolome B, Moreno-Arribas MV, Pelaez C. Effect of grape polyphenols on lactic acid bacteria and bifidobacteria growth: Resistance and metabolism. Food Microbiol. (2011) 28:1345–52. doi: 10.1016/j.fm.2011.06.005
49. He L, Xu H, Liu X, He WH, Yuan F, Hou ZQ, et al. Identification of phenolic compounds from pomegranate (Punica granatum L) seed residues and investigation into their antioxidant capacities by HPLC–ABTS+ assay. Food Res Int. (2011) 44:1161–7. doi: 10.1016/j.foodres.2010.05.023
50. Svensson L, Sekwati-monang B, Lopeslutz D, Schieber AG, Nzle MG. Phenolic acids and flavonoids in nonfermented and fermented red Sorghum (Sorghum bicolor L Moench). J Agr Food Chem. (2010) 58:9214–20. doi: 10.1021/jf101504v
51. Attri S, Sharma K, Raigond P, Goel G. Colonic fermentation of polyphenolics from sea buckthorn (Hippophae rhamnoides) berries: assessment of effects on microbial diversity by principal component analysis. Food Res Int. (2018) 105:324–32. doi: 10.1016/j.foodres.2017.11.032
52. Hashemi SMB, Khaneghah AM, Barbaf FJ, Nemati Z, Shokofti SS, Alizadeh F. Fermented sweet lemon juice (Citrus limetta) using Lactobacillus plantarum LS5: chemical composition, antioxidant and antibacterial activities. J Functional Foods. (2017) 38:409–14. doi: 10.1016/j.jff.2017.09.040
53. Wang J, Xie B, Sun Z. Quality parameters and bioactive compound bioaccessibility changes in probiotics fermented mango juice using ultraviolet-assisted ultrasonic pre-treatment during cold storage. LWT-Food Sci Technol. (2021) 137:110438. doi: 10.1016/j.lwt.2020.110438
54. Filho A, Freitas HV, Rodrigues S, Virgínia Kelly Gonalves Abreu, Pereira A. Production and stability of probiotic cocoa juice with sucralose as sugar substitute during refrigerated storage. LWT-Food Sci Technol. (2019) 99:371–8. doi: 10.1016/j.lwt.2018.10.007
55. Queirozc C, Lopes M, Fialho E, Valente-Mesquita VL. Changes in bioactive compounds and antioxidant capacity of fresh-cut cashew apple. Food Res Int. (2011) 44:1459–62. doi: 10.1016/j.foodres.2011.03.021
56. Xiao Y, Wu X, Yao X, Chen Y, Ho CT, He C, et al. Metabolite profiling, antioxidant and α-glucosidase inhibitory activities of buckwheat processed by solid-state fermentation with Eurotium cristatum YL-1. Food Res Int. (2021) 143:110262. doi: 10.1016/j.foodres.2021.110262
57. Mousavi Z, Mousavi M, Razavi S, Hadinejad M, Emam-Djomeh Z, Mirzapour M. Effect of Fermentation of pomegranate Juice by Lactobacillus plantarum and Lactobacillus acidophilus on the antioxidant activity and metabolism of sugars, organic acids and phenolic compounds. Food Biotechnol. (2013) 27:1–13. doi: 10.1080/08905436.2012.724037
58. Braga A, Mesquita L, Martins P, Habu S, Rosso VD. Lactobacillus fermentation of jussara pulp leads to the enzymatic conversion of anthocyanins increasing antioxidant activity. J Food Compos Anal. (2018) 69:162–70. doi: 10.1016/j.jfca.2017.12.030
59. Wu Y, Li S, Tao Y, Li D, Han Y, Show PL, et al. Fermentation of blueberry and blackberry juices using Lactobacillus plantarum, Streptococcus thermophilus and Bifidobacterium bifidum: Growth of probiotics, metabolism of phenolics, antioxidant capacity in vitro and sensory evaluation. Food Chem. (2021) 348:129083. doi: 10.1016/j.foodchem.2021.129083
60. Ozgen M, Reese RN, Tulio AZ, Scheerens JC, Miller AR. Modified 2,2-azino-bis-3-ethylbenzothiazoline-6-sulfonic acid (ABTS) method to measure antioxidant capacity of selected small fruits and comparison to ferric reducing antioxidant power (FRAP) and 2,2'-diphenyl-1-picrylhydrazyl (DPPH) methods. J Agri Food Chem. (2006) 54:1151–7. doi: 10.1021/jf051960d
61. Zhang B, Xia T, Duan W, Zhang Z, Wang M. Effects of organic acids, amino acids and phenolic compounds on antioxidant characteristic of Zhenjiang aromatic vinegar. Molecules. (2019) 24:3799. doi: 10.3390/molecules24203799
62. Thuibaut S, Caillon J, Huart C, Grandjean G, Lombrail P, Potel G. Susceptibility to the main antibiotics of Escherichia coli and Staphylococcus aureus strains identified in community acquired infections in France (MedQual, 2004–2007). Med Mal Al Infec. (2010) 40:74–80. doi: 10.1016/j.medmal.2009.01.011
63. Reis JA, Paula AT, Casarotti S, Penna A. Lactic acid bacteria antimicrobial compounds: characteristics and applications. Food Eng Rev. (2012) 4:124–40. doi: 10.1007/s12393-012-9051-2
Keywords: lactic acid bacteria, apple juice, fermentation, physicochemical properties, bioactivities
Citation: Yang J, Sun Y, Gao T, Wu Y, Sun H, Zhu Q, Liu C, Zhou C, Han Y and Tao Y (2022) Fermentation and Storage Characteristics of “Fuji” Apple Juice Using Lactobacillus acidophilus, Lactobacillus casei and Lactobacillus plantarum: Microbial Growth, Metabolism of Bioactives and in vitro Bioactivities. Front. Nutr. 9:833906. doi: 10.3389/fnut.2022.833906
Received: 12 December 2021; Accepted: 17 January 2022;
Published: 09 February 2022.
Edited by:
Yu Xiao, Hunan Agricultural University, ChinaReviewed by:
Hossein Kiani, University of Tehran, IranCopyright © 2022 Yang, Sun, Gao, Wu, Sun, Zhu, Liu, Zhou, Han and Tao. This is an open-access article distributed under the terms of the Creative Commons Attribution License (CC BY). The use, distribution or reproduction in other forums is permitted, provided the original author(s) and the copyright owner(s) are credited and that the original publication in this journal is cited, in accordance with accepted academic practice. No use, distribution or reproduction is permitted which does not comply with these terms.
*Correspondence: Yang Tao, eWFuZy50YW9AbmphdS5lZHUuY24=
Disclaimer: All claims expressed in this article are solely those of the authors and do not necessarily represent those of their affiliated organizations, or those of the publisher, the editors and the reviewers. Any product that may be evaluated in this article or claim that may be made by its manufacturer is not guaranteed or endorsed by the publisher.
Research integrity at Frontiers
Learn more about the work of our research integrity team to safeguard the quality of each article we publish.