- 1Research and Development, Molekule Inc, Tampa, FL, United States
- 2Clean Energy Research Center, Department of Chemical, Biological and Materials Engineering, University of South Florida, Tampa, FL, United States
- 3Department of Chemistry, Sri Guru Teg Bahadur Khalsa College, Anandpur Sahib, Punjab, India
- 4Department of Physics, Gujranwala Guru Nanak Khalsa College, Ludhiana, India
- 5Department of Environment Studies, Panjab University, Chandigarh, India
- 6NanoBioTech Laboratory, Department of Environmental Engineering, Florida Polytechnic University, Lakeland, FL, United States
Despite ongoing public health measures and increasing vaccination rates, deaths and disease severity caused by severe acute respiratory syndrome coronavirus 2 (SARS-CoV-2) and its new emergent variants continue to threaten the health of people around the world. Therefore, there is an urgent need to develop novel strategies for research, diagnosis, treatment, and government policies to combat the variant strains of SARS-CoV-2. Since the state-of-the-art COVID-19 pandemic, the role of selenium in dealing with COVID-19 disease has been widely discussed due to its importance as an essential micronutrient. This review aims at providing all antiviral activities of nanoselenium (Nano-Se) ever explored using different methods in the literature. We systematically summarize the studied antiviral activities of Nano-Se required to project it as an efficient antiviral system as a function of shape, size, and synthesis method. The outcomes of this article not only introduce Nano-Se to the scientific community but also motivate scholars to adopt Nano-Se to tackle any serious virus such as mutated SARS-CoV-2 to achieve an effective antiviral activity in a desired manner.
1 Introduction
The transmission of coronaviruses (CoV) in animals has a long history (Ruiz-Aravena et al., 2022). However, the earliest pathogenic transmission from animal to human was detected as a severe acute respiratory syndrome (SARS) in Guangdong province, China, in 2002, and after a decade, one more pathogenic coronavirus, Middle East respiratory syndrome coronavirus (MERS-CoV), was identified in Middle Eastern countries (Cui et al., 2019; Dhama et al., 2020; Shereen et al., 2020). The recent COVID-19 outbreak originated in the city of Wuhan, China, and rapidly spread all over the world and has become a new global public health crisis (Cui et al., 2019; Zhu et al., 2020). After the declaration of novel coronavirus disease as a pandemic by the World Health Organization (WHO), researchers in academics and pharmaceuticals have been increasingly engaged in the search for effective antiviral drugs and therapeutic treatment options. The emergence of SARS-CoV-2 variants through mutations to their genetic code is a growing concern for public health. The Centers for Disease Prevention and Control (CDC), United States, recently revised the classification of variants and included another class of variants named as variant being monitored (VBM) in addition to three preceding classes of SARS-CoV-2 variants, that is, variant of concern (VOC), variant of interest (VOI), and variant of high consequence (VOHC), to prioritize global monitoring, selective and rapid diagnosis, research to explore pathogenesis, and treatments of higher efficacy (Centers for Disease Prevention and Control, 2022).
The structural proteins of SARS-CoV-2 include four different structural proteins, such as spike (S) glycoprotein, membrane (M) protein, small envelope glycoprotein (E), and nucleocapsid (N) protein, and are responsible for viral replication and propagation (Schoeman and Fielding, 2019; Yadav et al., 2021). Like other viruses, SARS-CoV-2 is susceptible to genetic evolution while transmitting from one host person to others through mutations over the time (Harvey et al., 2021). These changes have little or no impact; however, some mutations could alter its pathogenic or transmission potential of the virus and can amplify disease severity (Young et al., 2020). Throughout the pandemic, several variants of SARS-CoV-2 have been identified around the world. So far, five variants, namely, B.1.1.7 (Alpha), B.1.351 (Beta), P.1 (Gamma), B.1.617.2 (Delta), and B.1.1.529 (Omicron), have been listed as variants of concern (World Health Organization, 2021). Despite the remarkable progresses of vaccine and drug development against COVID-19 disease, the emergences of new variant strains of SARS-CoV-2 can not only result in an increased rate of spread, disease severity, and mortality but also threaten to overturn the significant progress made so far in halting the spread of SARS-CoV-2. SARS-CoV-2 variants have raised concerns about resistance to neutralizing by escaping from host antibody responses and have become a stumbling block to ending this pandemic. Therefore, prevention, vaccination adoption, and advancing research are key factors for the management of the COVID-19 pandemic.
Various kinds of nanotechnology-based materials have been studied in biomedical science, including metal NPs, quantum dots (QDs), carbon-based nanosystems, and polymeric nanomaterials (Chenthamara et al., 2019; Aflori, 2021; Singh et al., 2021a; Ma et al., 2021; Dhiman et al., 2022a; Dhiman et al., 2022b; Chaudhary et al., 2022). In the biomedicine field, nanoparticles hold great promise for a variety of applications, including drug developments, diagnostic techniques, drug delivery, gene delivery, nanodrugs, and prostheses and implants (Pelaz et al., 2017; Kaushik, 2019; Malachowski and Hassel, 2020; Nienhaus et al., 2020; Li et al., 2022; Mostafavi et al., 2022; Xie et al., 2022). These applications are revolutionary in the biomedical field and result in new materials, techniques, and devices with more sensitivity, selectivity, and sophistication, which are required for this field (Kaushik et al., 2020; Ahmadivand et al., 2021; Sharma et al., 2021). In the ongoing pandemic, nanotechnology plays an important role in dealing with SARS-CoV-2 and its variants (Paliwal et al., 2020; Gage et al., 2021; Sadique et al., 2021; Varahachalam et al., 2021; Dubey et al., 2022; Kujawska et al., 2022). Unlike many other metals, Se is an essential element in the human body. Therefore, Nano-Se having very low toxicity has better medicinal properties over other metal nanoparticles (Ferro et al., 2021). In the last two decades, biological activities of selenium nanoparticles (Nano-Se) toward various diseases have been extensively studied (Figure 1), which has transformed the Se nanoparticle chemistry as a major branch of study in nanotechnology (Khurana et al.; 2019; Shi et al., 2021; Bisht et al., 2022). The capabilities of Se-containing species to act as an antiviral in nature make it notable and potentially useful in the current COVID-19 pandemic (Kieliszek, 2022; Singh et al., 2022). In the case of SARS-CoV-2, Se deficiency is related to increased susceptibility to infections (Khatiwada and Subedi 2021; Majeed et al., 2022). This observation has been observed in many findings from different countries, where the selenium status was associated with cure rates of COVID-19 disease (Zhang D. W et al., 2020; Erol et al., 2021; Majeed et al., 2021). Moreover, studies on other virus-related diseases such as HIV, influenza, and Ebola demonstrated the benefit of Se supplementation (Harthill, 2011; Ivory et al., 2017; Muzembo et al., 2019). A considerable number of research articles and review articles on synthesis, characterization, and the wide range of applications of Se nanoparticles are proof of the growth in this field. This review projects the potential of Se nanoparticles and its decorated systems for antiviral applications systematically. This review article aims to summarize the studied antiviral properties of selenium nanoparticles and other related materials.
2 Origin of selenium
In nature, Se is a common element and exists in organic and inorganic forms. The typical organic forms of Se are selenocysteine and selenomethionine, which are present in the human body, while the inorganic forms include selenite and selenate, which are available in plants (Gupta and Gupta, 2017). Since the discovery of Se in 1817, the role of selenium in the human body has evolved and its deficiency has been shown to correlate with the progression of HIV to AIDS, cardiovascular disease, infertility, Keshan disease, cognitive decline, and impaired mineralization of bones and teeth (Dworkin, 1994; Benstoem et al., 2015; Pieczyńska and Grajeta, 2015; Munguti et al., 2017; Yang et al., 2022). On Earth, Se is unevenly distributed and concentrated in different forms, which leads to variation in Se intake throughout the population of the world (Kieliszek et al., 2022). It is estimated that the diets of at least one billion people in the world lack sufficient Se for their well-being (Haug et al., 2007). An adequate selenium status is essential for regulating many physiological processes in the human body (Table 1).
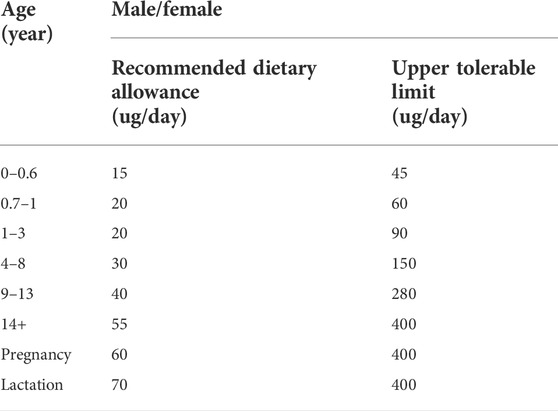
TABLE 1. Selenium intake for children and adults (Institute of Medicine Food and Nutrition Board, 2000).
Se cannot be produced by human cells and must be ingested through food (Kieliszek and Blazejak, 2016). Selenium enters the food chain through plants uptake from the soil. Crops cultivated in soil with a low Se concentration are correlated with Se deficiency in the human population. Selenium biofortification in plant strategies to produce an adequate Se amount in foods can be helpful in overcoming Se deficiency and improving human health. Apart from traditional biofortification methods, researchers continue their efforts to find new advanced Se biofortification methods, including genetic engineering, microbial-based biofortification, and through organic matter recycling (Sarwar et al., 2020). In human diet, the main contributors to Se intake are plant-based products (46.5%) (Hu et al., 2021). Animal-based foods are the second highest source of Se intake, in which fish, meat, meat-based products, milk, dairy products, and eggs are the main dietary sources (Hu et al., 2021). Selenium has vital roles in animals; they must ingest selenium through food, and in biological processes they transform the inorganic Se form to the organic form (Se-Cys). The selenium amount in animal-based foods depends upon the selenium in the plants or food that animals eat and the species of animals (Hu et al., 2021). The incorporation of Se in animal feed can increase the Se content in animal-based products and result in indirect introduction of selenium into the human body (Pavlovic et al., 2018).
3 Clarity on Se toxicity
The first scientific finding on Se as a toxic form was found through multiple experiments conducted at the Wyoming and South Dakota Agricultural Experiment Stations in 1929 (Zwolak, 2020). In these experiments, it was found that crops grown on specific soil caused an alkali disease to livestock (Christophersen et al., 2013). However, with the confirmation of an essential role as a trace element in the diet of mammals (Kieliszek and Blazejak, 2016), the focus of most research efforts shifted to exploring Se deficiency and has prompted studies on the biological activities of different forms of selenium species (Dhau et al., 2014a; Dhau et al., 2015). The United States Department of Agriculture in 1974 approved inorganic selenium as food additive to animal feeds in the identified regions where selenium was at a subadequate level in soil (Surai et al., 2018). In 1978, scientists recognized selenocysteine moiety as a catalytic site in glutathione peroxidase, later named the “twenty-first” amino acid by Bock et al.(1991) (Schmidt and Simonovic, 2012).
All forms of Se are potentially toxic to living species, with the toxicity dependent upon the chemical nature of Se, dose, the route of administration, age of species, physiological state, and nutrition and dietary interactions (Nogueira et al., 2004; Christophersen et al., 2013; Singh et al., 2021b). Selenium poisoning in humans has been reported in different situations, such as accidental ingestion, industrial mishappenings, and environmental toxicity (Nuttall, 2006). The most serious events of environmental toxicity to humans occurred in China with symptoms including loss of hair and cracked nails (Huang et al., 2013). The lowest observed adverse effect level (LOAEL) for selenium is 1540–1600 μg/day of Se while 5,000 μg/day of Se is the toxic level, which can cause selenosis (Stoffaneller and Morse, 2015; Prabhu and Lei, 2016; Ibrahim et al., 2019). Selenosis is the term used for conditions resulting from chronic selenium toxicity and has symptoms of loss of hair, damaged nails, cardiac complications, and respiratory problems (Stoffaneller and Morse, 2015; Ibrahim et al., 2019).
Se in nanodimensional forms, that is, nanoselenium (Nano-Se), is less toxic than other forms of selenium (organic and inorganic) (Hosnedlova et al., 2018; Bhattacharjee et al., 2019; Bisht et al., 2022). Selenium-based nanomaterials have been investigated for many medical applications, such as diagnosis and imaging, antioxidant, antibacterial and antiviral, and chemotherapy (Sakr et al., 2018; Khurana et al., 2019; Bisht et al., 2022). Nanoselenium has the advantage of selenium in its zero-oxidation state (Se0), which introduces high bioavailability and lower toxicity (Wang et al., 2007; Shi et al., 2010). Cytotoxicity analysis of Nano-Se and SeO2 demonstrated that Se nanoparticles were six times less toxic against MCF-7 cells (Forootanfar et al., 2014). In another study, selenium nanoparticles were studied for safety, toxicities, biocompatibility, and antitumor activity in animal models and in addition to the high value of therapeutic effectiveness, no significant value of toxicity was found (Mittal and Banerjee, 2021). To deal with the toxic nature of the selenium species at higher concentrations, Dhau et al. (2014b)explored the polymer matrix as a sustained and controlled release drug device for selenium compounds. Discoveries such as these open new possibilities for the development of new classes of selenium-based drugs.
4 Prospects for Nano-Se to tackle SARS-CoV-2 variants
In general, the development of new drugs is a difficult and lengthy process, which takes at least 10 years on an average to complete the journey from initial discovery to the marketplace (Mohs and Greig, 2017). The ongoing pandemic caused by SARS-CoV-2 and its muted variants has recognized the urgency to discover new antiviral drugs and, with this, the idea of repurposing existing drugs to treat infectious viruses emerged as an attractive strategy (Martinez, 2022). All over the world, various health agencies and research groups have performed various studies such as computational research, clinical trials, and other experiments to study repurposed standard drugs and potential drug candidates to treat COVID-19 symptoms (Wang and Guan, 2021). Jin et al. (2020)investigated a pool of 10,000 molecules including standard drugs, drug molecules in clinical phases, and pharmacologically relevant molecules to identify efficient inhibitors of main protease (Mpro), a key enzyme of SARS-CoV-2, using the combination of structure-based drug design, virtual drug screening, and high-throughput screening. Of the results, out of six active compounds against SARS-CoV-2 Mpro, ebselen exhibited promising antiviral activity in cell-based assays (Jin et al., 2020). The main protease, Mpro activity, is critical for breaking the viral polyprotein into further protein units for virus replication and pathogenesis (Moghadasi et al., 2020). The position of SARS-CoV-2 Mpro inhibitor in the prevention of SARS-Cov-2 propagation is shown in Figure 2 (Mengist et al., 2020). Ebselen [2-phenyl-1,2-benzisoselazol-3(2H)-one] is an organoselenium compound, which is most widely studied as a GPx mimetic and has also been found to possess antiviral properties against a number of viruses such as human immunodeficiency virus type 1 (HIV-1), hepatitis C virus (HCV), influenza A virus, Zika virus, herpes simplex virus type 1 (HSV-1), and coxsackie virus (Wójtowicz et al., 2004; Mukherjee et al., 2014; Singh et al., 2015; Oostwoud et al., 2016; Sharma et al., 2017a; Sands and Back., 2018; Simanjuntak et al., 2018; Zhang J et al., 2020). In addition to ebselen, researchers have been exploring the other selenium compounds for various biological activities (Dhau et al., 2014b; Sharma et al., 2017b; Dhau et al., 2021; Kumar et al., 2022). Amporndanai et al. (2021)investigated the binding modes of ebselen derivatives in Mpro through high-resolution co-crystallography and further explored their chemical reactivity using spectroscopic techniques. The authors found that two ebselen derivatives have better inhibitory effectiveness than ebselen against Mpro enzyme and SARS-CoV-2 replication. These studies have fueled the discussion and research on the potentials of synthetic forms of selenium to manage SARS-COVID-19 (Kieliszeka and Lipinskib, 2020; Seale et al., 2020; Siesa and Parnham, 2020; Zhang J et al., 2020; He et al., 2021; Khatiwada and Subedi, 2021; Kieliszek, 2022; Pedrosa et al., 2022).
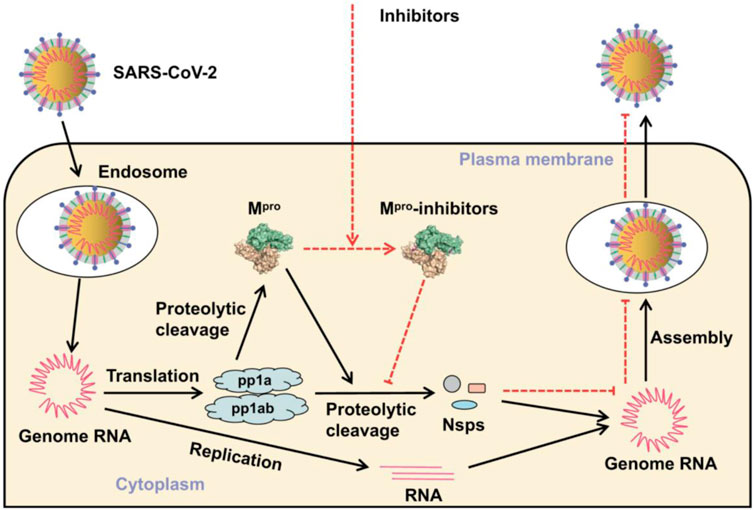
FIGURE 2. Position of the inhibitor in the prevention of SARS-CoV-2 replication (Mengist et al., 2020).
In biological science, the research on Se-containing species was started with the focus on the treatment of oxidative stress, which can be responsible for several diseases (Tinggi, 2008). In the early studies on SARS-CoV-2, scientific findings concluded that its infection in the respiratory system can activate nuclear factor kappa B (NF-κB), which leads to multiple inflammatory and autoimmune diseases (Hirano and Murkami, 2020; Davies et al., 2021). Selenium is well-established to affect NF-kB activity and contributes to reducing viral-related infection (Hiffler and Rakotoambinina, 2020). Nuclear factor kappa B (NF-κB) transcription factors control several critical physiological processes, including oxidative stress, inflammation, cell growth, apoptosis, immune responses, and the expression of certain viral genes (Lin et al., 2009; Oeckinghaus and Ghosh, 2009). Kumari et al. (2018)tested a combination of curcumin-loaded Se nanoparticles and CD44-targeted doxorubicin-loaded nanoparticles for anticancer activity and found a lower expression of NF-κB. Ge et al. (2021)compared the alleviation of Cd-induced heart inflammation through inhibiting the NF-κB pathway using three different forms of Se, namely, Se-enriched yeast (organic form), sodium selenite (inorganic form), and Nano-Se (prepared through bioreduction), and demonstrated superior results with the nanoform of Se. Researchers have investigated the role of essential metals as micronutrients in surviving the COVID-19 challenge. Micronutrients including zinc (Zn), copper (Cu), iron (Fe), and selenium (Se) are involved in the better management of the immune system functioning against viral infections, including severe acute respiratory syndrome coronavirus 2 (SARS-CoV-2) (Renata et al., 2022). Zhang D. W. et al. (2020)performed a study including 17 Chinese cities and showed that Se insufficiency was associated with an elevated COVID-19 mortality risk. Selenium supplements have proven health benefits in other viral disease prevention (Zhou et al., 2018).
The trace element Se is known for a regular functioning of the immune system by increasing the production of immunoglobulin G and M antibodies and leading to increased activity of T cells and macrophages in human health. This biological outcome of Se obtained from its presence in more than 25 selenoproteins was identified in humans (Calder et al., 2020; Maltseva et al., 2022). Five glutathione peroxidases (GPx1-4 and GPx6), three thioredoxin reductases (TrxR1-3), and three deiodinases are the major types in the list of 25 selenoproteins (Bates et al., 2000; Moghadaszadeh and Beggs, 2006; Flohé et al., 2022). GPx1 is a cytosolic enzyme present in all tissues, and a decrease in this level causes an increase in the production of reactive oxygen species (ROS), which leads to oxidative stress, activation of NF-κB transcription, and cell apoptosis (Youn et al., 2008; Lubos et al., 2011; Ren et al., 2020).
5 Antiviral properties of Nano-Se
Nano-Se are fascinating species that have been explored by researchers to produce advanced materials for various applications (Chaudhary and Mehta, 2014; Dong et al., 2014; Peng et al., 2015; Kolay et al., 2019; Ferro et al., 2021). Many biological studies were performed using Nano-Se, including antiviral, antimicrobial, anti-oxidant, anticancer, antidiabetic, and antiparasitic (Khurana et al., 2019; Shi et al., 2021; Bisht et al., 2022). Several antiviral investigations were performed using Nano-Se and their conjugates (summarized in Table 2). The progress in the development of nanoselenium species and related systems as antiviral active agents is discussed in the remaining section.
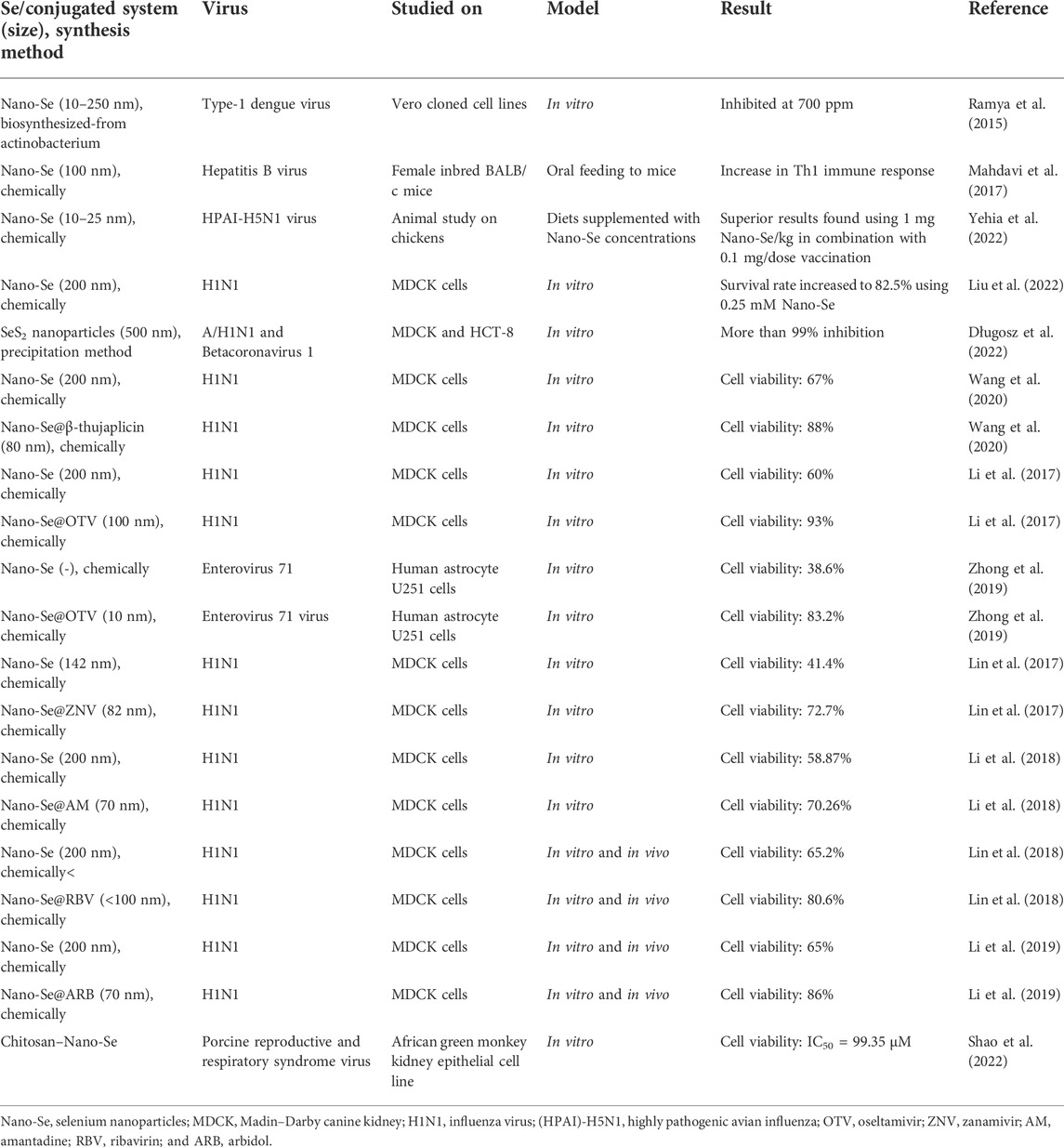
TABLE 2. Nanoselenium particles and their conjugated systems with studied antiviral activity against different viruses.
Ramya et al. (2015) prepared Se nanoparticles through aerobic biogenic synthesis from actinobacteria, having an average particle size of 10–250 nm. They investigated applications of these nanoparticles for antibiofilm, antioxidant, wound healing, cytotoxic, and antiviral activities. The antiviral activity of biosynthesized Se nanoparticles was tested against the type-1 dengue virus using different concentrations (100–1000 ppm) of Se nanoparticles. The biosynthesized Se nanoparticles showed maximum inhibition of type-1 dengue viral growth at 700 ppm concentration.
Selenium is a vital micronutrient in the human body that plays a dominant role in many processes such as the maintenance of cellular redox homeostasis in nearly all tissues, thyroid hormone metabolism, modulation of the immune system by antiviral properties, and enhancement of humoral immunity response (Huang et al., 2012). Mahdavi and co-workers explored the effect of Se nanoparticles on inducing a Th1cytokine pattern after hepatitis B surface antigen (HBsAg) vaccination in animal models. In this study, authors measured lymphocyte proliferation, interferon gamma, interleukin 4, and total antibody by enzyme-linked immunosorbent assay (ELISA) (Mahdavi et al., 2017). The results clearly demonstrated that oral administration of synthetic Se nanoparticles to mice helped in increasing Th1 immune response and caused the production of cytokines, which play a crucial role in the immune response against viral infection and other diseases.
Nano-Se of 200 nm was prepared from combining Na2SeO3 (0.1 M) and vitamin C and verified for inhibition of influenza (H1N1) virus (Liu et al., 2022). Influenza A (H1N1) virus infection in humans not only leads to respiratory diseases but also occasionally causes severe lethal pneumonia and nervous system damage. As influenza viruses become less susceptible to antiviral drugs, therefore, in the search of new potential drugs with different modes of action, Liu et al. investigated selenium nanoparticles for an antiviral study against H1N-infected Madin–Darby canine kidney (MDCK) cells. In this investigation, a change in morphology of MDCK cells infected by H1N1 was observed using the microscopic technique and shrinkage of cytoplasm, loss of cell integrity, and lower cell numbers were found (Figure 3A). The cell survival rate after treatment with H1N1 virus was studied using different concentrations of selenium nanoparticles by MTT assay. Results are shown in Figure 3, recommending that selenium nanoparticles (Nano-Se) with 0.25 mM concentration increased the survival rate up to 82.5% compared to the control samples. In a mechanistic investigation, GPx1 activity was determined using different concentrations of Nano-Se and we found that improving the level of GPx1 plays a role in the inhibition of H1N1 influenza virus-induced apoptosis.
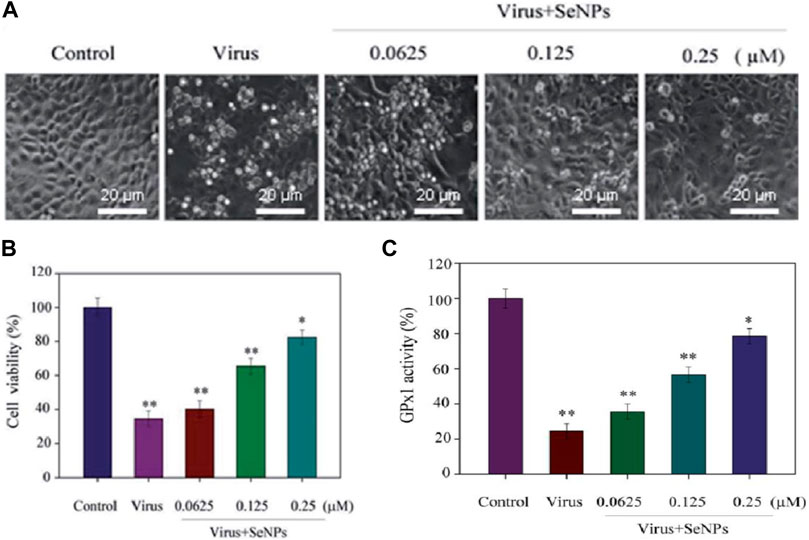
FIGURE 3. (A) Phase contrast microscopic study on the morphology of H1N1-infected MDCK cells using different concentrations of selenium nanoparticles. (B) Antiviral activity through cell viability percentage using the MTT assay. (C) GPx activity percentage using different concentrations of Nano-Se (Liu et al., 2022).
Yehia et al. (2022) utilized a combination of Nano-Se and inactivated highly pathogenic avian influenza for studying the improvement in chicken immunity. Two methods of Nano-Se supplementation were used: first in diets with Nano-Se concentrations (0.25–1 mg/kg) and then vaccinated and in the second method, 0.5 ml of the vaccine used with 0.02–0.1 mg/dose of Nano-Se. The group of chickens having selenium supplementation of 1 mg Nano-Se/kg showed a considerably higher antibody titer at four and following weeks in the post-vaccination study. In both groups, Nano-Se supplementation via diet or vaccine dose resulted in a lower viral shedding and milder symptoms of inflammation in the lung, trachea, spleen, and liver. Among all chickens, the best vaccine efficacy was observed in a group of chickens fed with 1 mg Nano-Se/kg in combination with 0.1 mg/dose vaccination.
Długosz et al. (2022) (Figure 4) have reported the preparation of Se sulfide nanoparticles using the precipitation method, in which aqueous solutions of sodium sulfide and selenium chloride in the presence of cinnamon, curcumin, and cayenne pepper extracts were extracted using natural deep eutectic solvents (NDESs). This innovative method produced selenium sulfide nanoparticles stabilized with extract of species in natural deep eutectic solvents and has increased bioactivity toward microorganisms. Natural deep eutectic solvents were based on the different combinations of citric acid, propanediol, betaine, proline, lactic acid–glucose, and betaine, and the authors found that using these solvents, the highest content of active ingredients from the spices could be extracted. The average size of selenium sulfide nanoparticles observed by X-ray diffraction analysis was about 500 nm. The antiviral activity of as prepared selenium sulfide nanoparticles was tested against human influenza virus A/H1N1 and Betacoronavirus 1. Using the nanoform of selenium sulfide in curcuma extract, the antiviral results showed a 5.5 log reduction in TCID50 virus titer against A/H1N1, which means more than 99.99% virus inactivation and in the case of HCoV-OC43 virus, and the decrease in the virus infectivity was 99.93% with the virus titer reduction logTCID50 of 3.2.
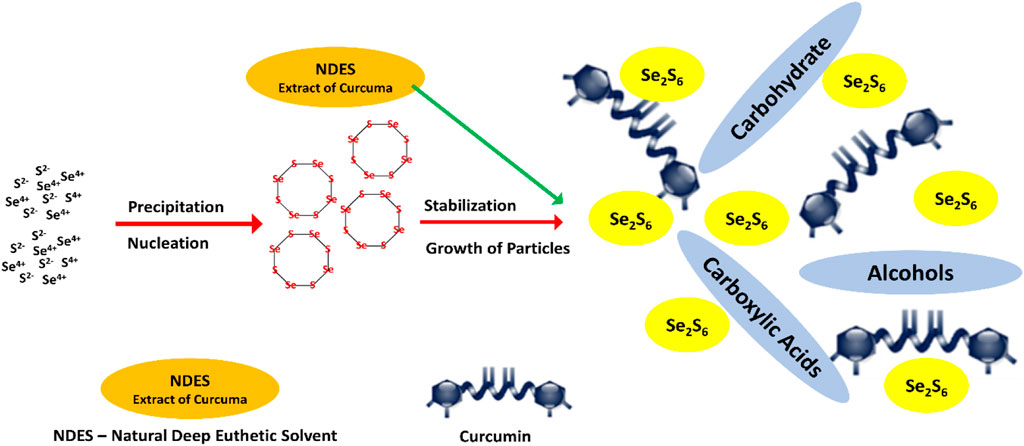
FIGURE 4. Synthesis of Se sulfide nanoparticles in NDES extract of curcuma (Długosz et al., 2022).
The other developments in the search for new antiviral drugs have been strategically based upon the conjugation of Nano-Se with standard antiviral drugs. Most of these studies present in the literature are based on the antiviral activity against H1N1 virus. H1N1 is a subtype of influenza A virus and is a common human pathogenic agent, which causes serious respiratory illness (Lin et al., 2017). In 2009, the pandemic caused by the influenza virus started in Mexico, and the most recent SARS-CoV-2 pandemic has severely affected humankind, causing numerous deaths, and both remain a serious problem in public health (Wang et al., 2020). Both these viruses may circulate simultaneously, which can cause more serious respiratory diseases. Bao et al. (2021)developed systematic animal models (ferrets and mice) of co-infection of H1N1 with SARS-CoV-2 for studying the consequences and pathogenesis of sequential infections. This study showed that both viruses together can enhance the condition of pneumonia in ferrets and mice and concluded that an effective prevention strategy from these viruses is concurrent vaccinations against these viruses. These types of findings may be helpful in developing systems against multivirus infections that lead to improvement in public health.
Selenium nanoparticles (Nano-Se) were conjugated with zanamivir (ZNV) and explored for antiviral properties (Lin et al., 2017). Zanamivir is an antiviral drug used as an inhibitor for effective treatment against influenza A and influenza B viruses. The antiviral activities of ZNV, Nano-Se, and ZNV-decorated selenium nanoparticles (Nano-Se@ZNV) were studied using the MTT assay. The results of this study indicated that ZNV-decorated Nano-Se have a greater antiviral activity compared to the Nano-Se and ZNV alone. Madin–Darby canine kidney cell viability after treatment with Nano-Se@ZNV reached 72.7% from 62.2% cells treated with ZNV (Figure 5A). Furthermore, the RNA level of H1N1 after treatment with different groups (ZNV, Nano-Se, and Nano-Se@ZNV) was determined, and it indicated that the cells treated with Nano-Se@ZNV had a lower RNA level of H1N1 virus than those with the standard antiviral drug alone (Figure 5B). H1N1 influenza virus induced apoptosis of MDCK cells, in which caspase-3 plays a pivotal role. Therefore, the authors studied caspase-3 activity of MDCK cells infected by H1N1 virus in different groups and found 273% and 161% in cases without treatment and Nano-Se@ZNV treatment, respectively; results are shown in Figure 5C. This substantial drop demonstrates the antiviral activity of Nano-Se@ZNV.
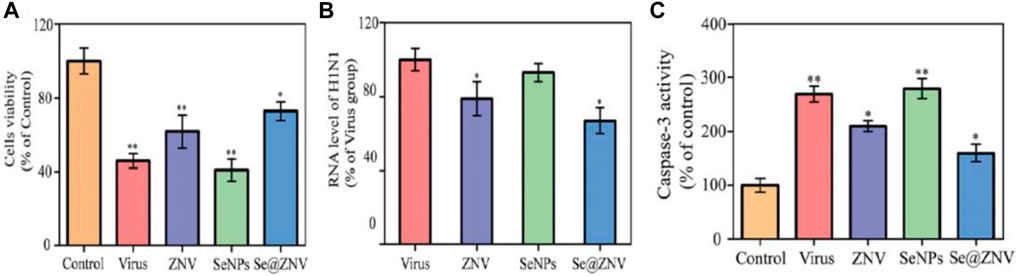
FIGURE 5. (A) Antiviral activity through cell viability percentage. (B) Percentage of the RNA level of H1N1. (C) Caspase-3 activity of infected MDCK cells (Lin et. al., 2017).
In another study, Nano-Se were functionalized with antiinfluenza drug oseltamivir (OTV) and their synergic effect on antiviral properties against H1N1 virus was explored (Li et al., 2017). OTV-modified Nano-Se (Nano-Se@OTV) as globular nanocomposites were prepared in a spherical shape of size 100 nm. Transmission electron microscopy (TEM) and size distribution results showed a reduced size of Nano-Se@OTV compared to Nano-Se (Figures 6A–D). In vitro antiviral activity results indicated that Nano-Se@OTV are superior for inactivation of H1N1 virus compared to the Nano-Se and OTV alone. Using TEM characterization, the authors investigated the effect of Nano-Se@OTV on the H1N1 morphology (Figures 6E,F). Using Nano-Se@OTV, another study was conducted on enterovirus 71 (EV71) in the human astrocytoma cell model (Zhong et al., 2019). Enterovirus 71 (EV71) is a single-stranded RNA virus and has been associated with hand, foot, and mouth disease (HFMD) in young children. It was found that Nano-Se@OTV were able to penetrate into human astrocyte U251 cells and suppressed EV71 proliferation. Further, in a detailed mechanism study, the authors found that protection of EV71-infected U251 cells from apoptosis was initiated by the mitochondrial pathway, thereby reducing the generation of reactive oxygen species.
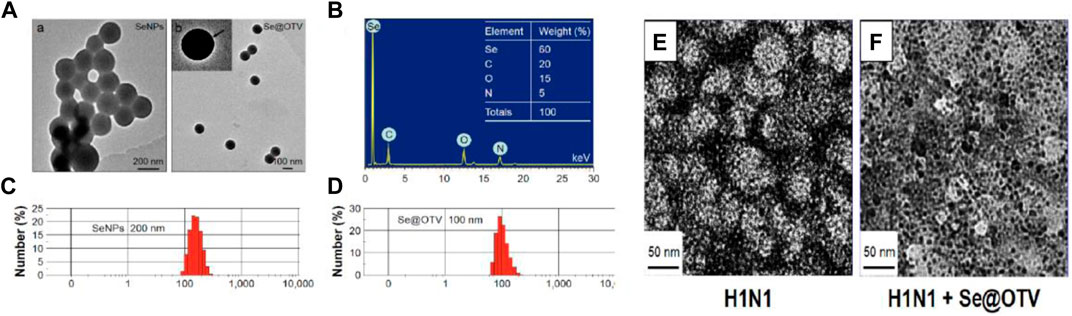
FIGURE 6. (A) Transmission electron microscopy images of Nano-Se and Nano-Se@OTV. (B) Energy-dispersive X-ray analysis of Nano-Se@OTV. (C,D) Size distribution of Nano-Se and Nano-Se@OTV. (E) Control samples of H1N1. (F) Treated sample of H1N1 with Nano-Se@OTV (Li et al., 2017).
Li et al. (2018)used amantadine (AM) to decorate the surface of Nano-Se (Nano-Se@AM) and reported that Nano-Se@AM has the ability to inhibit H1N1 influenza virus to infect MDCK cells through suppression of the neuraminidase activity. In MTT assay, MDCK cells have cell viability of 32.34, 53.23, 58.87, and 79.26% for virus alone, virus with AM, virus with Nano-Se, and virus with Nano-Se@AM, respectively (as shown in Figure 7A). Again, similar to previous studies on the combination of Nano-Se and standard drugs, Nano-Se@AM showed superior performance toward antiviral properties. The neuraminidase enzymatic activity study was used to investigate the antiinfluenza mechanism and identified that Nano-Se@AM bind tightly to the neuraminidase protein, resulting in the difficulty of the attachment of H1N1 virus to MDCK cells as the responsible factor for the antiinfluenza action of Nano-Se@AM. Moreover, in the mechanistic study, the ROS generation was recorded using dichlorodihydrofluorescein (DCF) assay. In the control, the infection of MDCK cells with H1N1 influenza virus without any treatment increased the reactive oxygen species generation up to 420%. Using Nano-Se@AM, the ROS generation level was reduced to 130% (shown in Figure 7B). This study showed that ROS was a potential novel target for antiviral drug development.
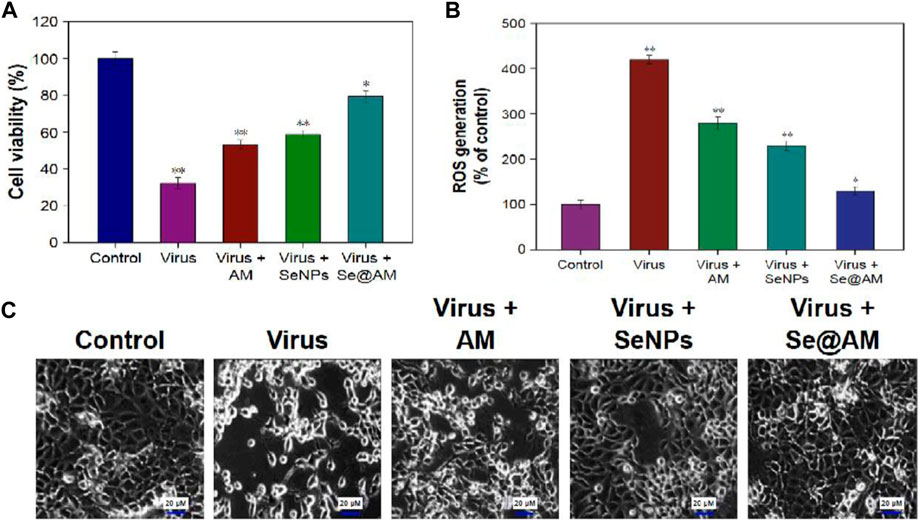
FIGURE 7. (A) Antiviral activity through cell viability percentage using the MTT assay. (B) ROS generation using different treatments detected by DCF fluorescence intensity. (C) Phase contrast microscopy images showing morphological changes in H1N1-infected MDCK cells (Li et al., 2018).
Ribavirin (RBV)-loaded selenium nanoparticles (Nano-Se@RBV) with a diameter of 65–100 nm were studied for inhibition of H1N1 influenza virus infection (Lin et al., 2018). Nano-Se@RBV increased MDCK cell viability after H1N1 infection and reduced viral titers in the culture supernatant by resisting the caspase-3 activation pathway. The authors further extended the antiviral activity study in in vivo models using H1N1 influenza-infected mice. It was observed that levels of lung injury and DNA damage were diminished in in vivo samples using Nano-Se@RBV compared to untreated groups. The result on restriction of activation of the caspase-3 signaling pathway coincidence between in vitro and in vivo studies. H&E and tunnel staining were used for demonstrating the efficiency of Nano-Se@RBV for prevention of DNA and lung damage during H1N1 infection (Figure 8).
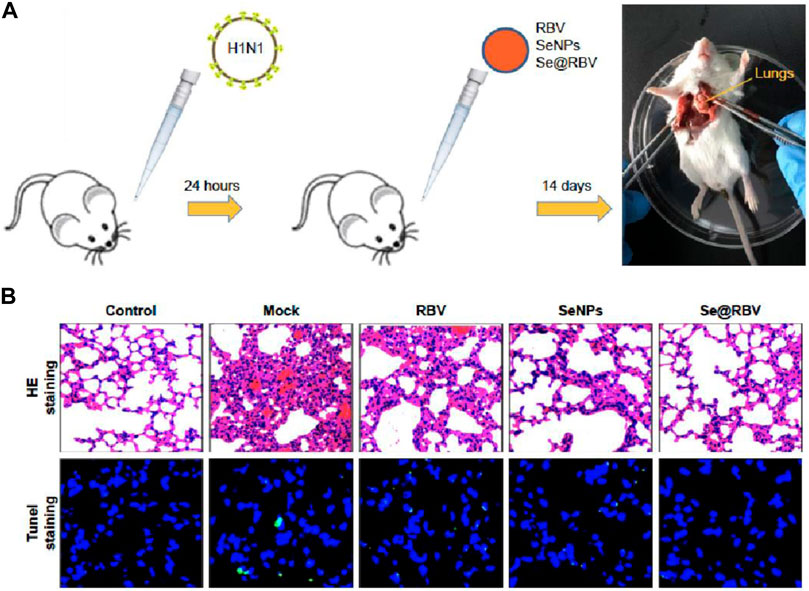
FIGURE 8. (A) Mice infected by H1N1 virus was treated with physiological saline (Mock), RBV, Nano-Se, or Nano-Se@RBV. (B) HE staining and tunnel staining (Lin et al., 2018).
In another study, arbidol (ARB)-loaded selenium nanoparticles (Nano-Se@ARB) showed effectiveness in reduction of the virus by following the pathway of the inhibition of hemagglutinin and neuraminidase (Li et al., 2019). Also, suppression of virus-induced oxidative stress of H1N1 infected MDCK cells through a signaling cascade involving p53, and AKT played an important role in antiviral activity. Novel functionalized selenium nanoparticles by β-thujaplicin (Nano-Se@TP) were evaluated for antiinfluenza activity (Wang et al., 2020). Effects and mechanisms of Nano-Se@TP in inhibiting H1N1 from infecting MDCK cells were studied. In a detailed mechanistic study, authors demonstrated that Nano-Se@TP inhibited caspase-3-mediated apoptosis by inhibition of ROS-mediated AKT and p53 signaling pathways.
Shao et al. (2022)used chitosan, a nontoxic, easily biodegradable, and biocompatible linear polysaccharide to coat Nano-Se. The prepared chitosan-coated Nano-Se investigated for antiviral performance against porcine reproductive and respiratory syndrome virus (PRRSV) infected African green monkey kidney cell line MARC-145. The results revealed that the increase in apoptosis rates induced by PRRSV infection was considerably reduced by chitosan–Nano-Se through suppression of oxidative stress by the combination of increased GSH-Px activity, promoted GSH production, and inhibited H2O2 synthesis. In a cell viability experiment, the IC50 value of chitosan–Nano-Se for African green monkey kidney cell line MARC-145 was recorded at 99.35 μM (Figure 9).
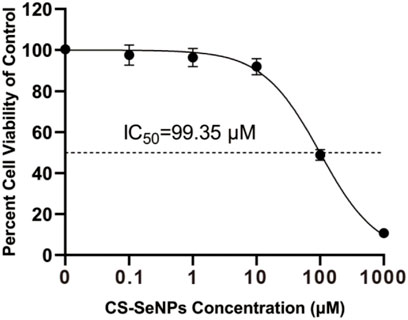
FIGURE 9. Cell viability of African green monkey kidney cell line MARC-145 on treatment using different concentrations (0–1000 μM) of chitosan–Nano-Se for 48 h by Cell Counting Kit-8 (Shao et al., 2022).
6 Challenges and prospects
SARS-CoV-2 infectious diseases present one of the major public health challenges of the 21st century. As compared to previous SARS-CoV-1 infection, SARS-CoV-2 infection has shown 40 times more transmission efficiency and a 3.3% higher fatality rate (Tiwari et al., 2022). SARS-CoV-2 variants have been causing continuous threat to public health and challenges to governments, health agencies, and researchers to end this pandemic. Therefore, researchers are urgently exploring different materials for the use in efficient COVID-19 pandemic management (Kaushik et al., 2020; Mujawar et al., 2020; Yalcin and Kaushik, 2021). In the past two decades, many efforts have been devoted by researchers in order to develop nanoselenium for various applications, including biological science and material science. However, there are still many knowledge gaps for the use of nanoselenium in the field of biomedical science. In the biomedical field, there are always challenges associated with exploring novel molecules (Figure 10).
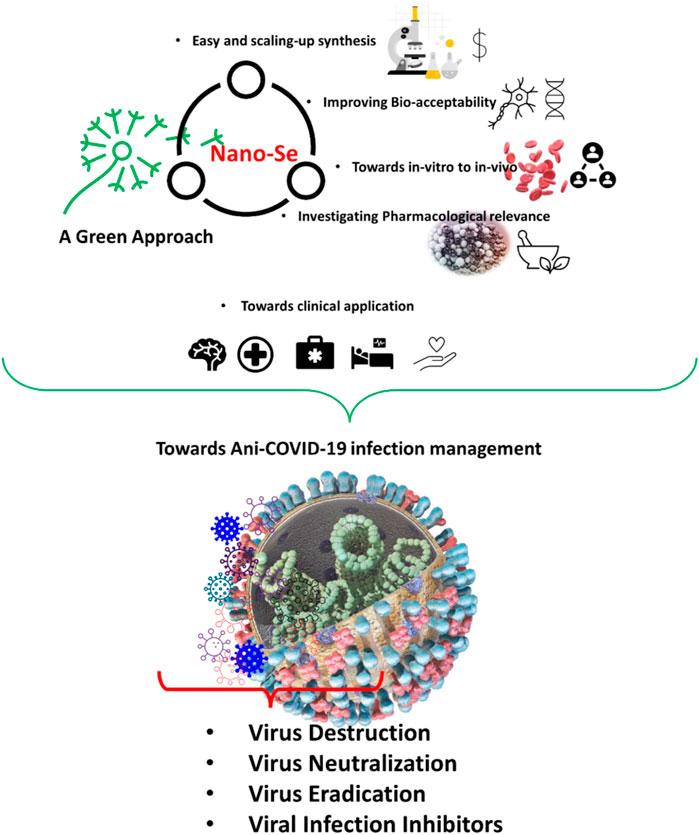
FIGURE 10. Prospects of Nano-Se toward using a green approach for efficient biomedical application, especially with a focus on tackling SARS-CoV-2 to manage COVID-19 infection management.
Se is widely distributed in the human body in the form of 25 selenoproteins, which are important in many physiological processes. These selenoproteins are mainly involved in the regulation of oxidative stress through antioxidative properties. The hypothesis that synthetic selenium species may be superior anti-oxidants than the natural antioxidants has led to research on selenium. Regarding synthetic selenium species, the first issue which comes to mind is the toxicity of selenium species. Different forms of selenium have vastly different toxic potentials. Nano-Se and related systems show excellent biological properties together with their lower toxicity. In order to really use nanoselenium to tackle muted SARS-CoV-2 for efficient COVID-19 management, it is necessary to have a clear and systematic investigation on easy scale-up synthesis using green approach, antiviral activity, mechanism study, and toxicology study. Green synthesis offers various advantages over chemical synthesis, such as ease in adopting large-scale production, easy handling, less-expensive materials, and eco-friendliness (Kalishwaralal et al., 2016; Dhawan et al., 2021). Furthermore, detailed in vivo studies with rigorous clinical trials and pharmacological studies are required to explore Nano-Se for biomedical applications (Figure 10).
7 Conclusion and viewpoint
This comprehensive review article explored the need for effective and efficient nanoassisted technologies to tackle mutated SARS-CoV-2 to manage COVID-19 infection. Since the emergence of the COVID-19 outbreak, policymakers, scientific communities, and health professionals have demanded a collaborative approach to develop effective approaches for ending this ongoing pandemic. Nanomaterials emerge as an important anti-SARS-CoV-2 agent, which can tackle SARS-CoV-2 via trapping, neutralizing, and eradication, and are required for efficient COVID-19 management even in a personalized manner. To achieve these tasks, we are propose and explore Nano-Se as an efficient nanostructure capable of managing COVID-19 infection due to its excellent antiviral activity capable of neutralizing and eradicating SARS-CoV-2 variants. This article also raised the demand for additional efforts to promote acceptable and affordable Nano-Se as a part of COVID-19 infection management planning. Nano-Se can be a part of protective and treatment strategies in a personalized manner. However, further optimization is required (in laboratories, clinics, and community) considering FDA approval; therefore, a significant future research must be planned in this direction.
Author contributions
AS visualized and articulated the article. PS, RK, and AK contributed to editing, review, and finalizing the article.
Funding
This article is supported by article processing changes provided by Frontiers in Nanotechnology (Code: DSC-05033211106PRD).
Acknowledgments
The authors acknowledge respective affiliated departments and institutions for providing support and facilities.
Conflict of interest
AS was employed by the company Molekule Inc.
The remaining authors declare that the research was conducted in the absence of any commercial or financial relationships that could be construed as a potential conflict of interest.
Publisher’s note
All claims expressed in this article are solely those of the authors and do not necessarily represent those of their affiliated organizations, or those of the publisher, the editors, and the reviewers. Any product that may be evaluated in this article, or claim that may be made by its manufacturer, is not guaranteed or endorsed by the publisher.
References
Aflori, M. (2021). Smart nanomaterials for biomedical applications-A review. Nanomater. (Basel) 11, 396. doi:10.3390/nano11020396
Ahmadivand, A., Gerislioglu, B., Ramezani, Z., Kaushik, A., Manickam, P., and Ghoreishi, S. A. (2021). Functionalized terahertz plasmonic metasensors: Femtomolar-level detection of SARS-CoV-2 spike proteins. Biosens. Bioelectron. X. 177, 112971. doi:10.1016/j.bios.2021.112971
Amporndanai, K., Meng, X., Shang, W., Jin, Z., Rogers, M., Zhao, Y., et al. (2021). Inhibition mechanism of SARS-CoV-2 main protease by ebselen and its derivatives. Nat. Commun. 12, 3061. doi:10.1038/s41467-021-23313-7
Bao, L., Deng, W., Qi, F., Lv, Q., Song, Z., Liu, J., et al. (2021). Sequential infection with H1N1 and SARS-CoV-2 aggravated COVID-19 pathogenesis in a mammalian model, and co-vaccination as an effective method of prevention of COVID-19 and influenza. Sig. Transduct. Target. Ther. 6, 200. doi:10.1038/s41392-021-00618-z
Bates, J. M., Spate, V. L., Morris, J. S., Germain, D. L. S., and Galton, V. A. (2000). Effects of selenium deficiency on tissue selenium content, deiodinase activity, and thyroid hormone economy in the rat during development. Endocrinology 141, 2490–2500. doi:10.1210/endo.141.7.7571
Benstoem, C., Goetzenich, A., Kraemer, S., Borosch, S., Manzanares, W., Hardy, G., et al. (2015). Selenium and its supplementation in cardiovascular disease--what do we know? Nutrients 7, 3094–3118. doi:10.3390/nu7053094
Bhattacharjee, A., Basu, A., and Bhattacharya, S. (2019). Selenium nanoparticles are less toxic than inorganic and organic selenium to mice in vivo. Nucleus 62, 259–268. doi:10.1007/s13237-019-00303-1
Bisht, N., Phalswal, P., and Khanna, P. K. (2022). Selenium nanoparticles: A review on synthesis and biomedical applications. Mat. Adv. 3, 1415–1431. doi:10.1039/d1ma00639h
Bock, A., Forchhammer, K., Heider, J., Leinfelder, W., Sawers, G., Veprek, B., et al. (1991). Selenocysteine: The 21st amino acid. Mol. Microbiol. 5, 515–520. doi:10.1111/j.1365-2958.1991.tb00722.x
Calder, P. C., Carr, A. C., Gombart, A. F., and Eggersdorfer, M. (2020). Optimal nutritional status for a well-functioning immune system is an important factor to protect against viral infections. Nutrients 12, 1181. doi:10.3390/nu12041181
Centers for Disease Prevention and Control (2022). SARS-CoV-2 variant classifications and definitions. Available at: https://www.cdc.gov/coronavirus/2019-ncov/variants/variant classifications.html.
Chaudhary, S., and Mehta, S. K. (2014). Selenium nanomaterials: Applications in electronics, catalysis and sensors. J. Nanosci. Nanotechnol. 14, 1658–1674. doi:10.1166/jnn.2014.9128
Chaudhary, V., Mostafavi, E., and Kaushik, A. (2022). De-coding Ag as an efficient antimicrobial nano-system for controlling cellular/biological functions. Matter 5, 1995–1998. doi:10.1016/j.matt.2022.06.024
Chenthamara, D., Subramaniam, S., Ramakrishnan, S. G., Krishnaswamy, S., Essa, M. M., Lin, F. H., et al. (2019). Therapeutic efficacy of nanoparticles and routes of administration. Biomater. Res. 23, 20. doi:10.1186/s40824-019-0166-x
Christophersen, O. A., Lyons, G., Haug, A., and Steinnes, E. (2013). “Selenium,” in Heavy metals in soils. Environ. Pollut. 22. Editor B. Alloway (Dordrecht: Springer). doi:10.1007/978-94-007-4470-7_16
Cui, J., Li, F., and Shi, Z. L. (2019). Origin and evolution of pathogenic coronaviruses. Nat. Rev. Microbiol. 17, 181–192. doi:10.1038/s41579-018-0118-9
Davies, D. A., Adlimoghaddam, A., and Albensi, B. C. (2021). The effect of COVID-19 on NF-κB and neurological manifestations of disease. Mol. Neurobiol. 58, 4178–4187. doi:10.1007/s12035-021-02438-2
Dhama, K., Patel, S. K., Sharun, K., Pathak, M., Tiwari, R., Yatoo, M. I., et al. (2020). SARS-CoV-2 jumping the species barrier: Zoonotic lessons from SARS, MERS and recent advances to combat this pandemic virus. Travel Med. Infect. Dis. 37, 101830. doi:10.1016/j.tmaid.2020.101830
Dhau, J. S., Singh, A., Brandão, P., and Félix, V. (2021). Synthesis, characterization, X-ray crystal structure and antibacterial activity of bis[3-(4-chloro-N, Ndiethylpyridine- 2-carboxamide)] diselenide. Inorg. Chem. Commun. 133, 108942. doi:10.1016/j.inoche.2021.108942
Dhau, J. S., Singh, A., Singh, A., Sharma, N., Brandão, P., Félix, V., et al. (2015). A mechanistic study of the synthesis, single crystal X-ray data and anticarcinogenic potential of bis(2-pyridyl)selenides and -diselenides. RSC Adv. 5, 78669–78676. doi:10.1039/C5RA15577K
Dhau, J. S., Singh, A., Singh, A., and Sooch, B. S. (2014b). A Study on the antioxidant activity of pyridylselenium compounds and their slow release from poly(acrylamide) hydrogels. Phosphorus Sulfur Silicon Relat. Elem. 189, 687–699. doi:10.1080/10426507.2013.844143
Dhau, J. S., Singh, A., Singh, A., Sooch, B. S., Brandão, P., and Félix, V. (2014a). Synthesis and antibacterial activity of pyridylselenium compounds: Self-assembly of bis(3-bromo-2-pyridyl)diselenide via intermolecular secondary and π⋯π stacking interactions. J. Organomet. Chem. 766, 57–66. doi:10.1016/j.jorganchem.2014.05.009
Dhawan, G., Singh, I., Dhawan, U., and Kumar, P. (2021). Synthesis and characterization of nanoselenium: A step-by-step guide for undergraduate students. J. Chem. Educ. 98, 2982–2989. doi:10.1021/acs.jchemed.0c01467
Dhiman, N., Ghosh, S., Mishra, Y. K., and Tripathi, K. M. (2022a). Prospects of nano-carbons as emerging catalysts for enzyme-mimetic applications. Mat. Adv. 3, 3101–3122. doi:10.1039/D2MA00034B
Dhiman, N., Pradhan, D., and Mohanty, P. (2022b). Heteroatom (N and P) enriched nanoporous carbon as an efficient electrocatalyst for hydrazine oxidation reaction. Fuel 314, 122722. doi:10.1016/j.fuel.2021.122722
Długosz, O., Ochnik, M., Sochocka, M., Franz, D., Orzechowsk, B., Anna, C-K., et al. (2022). Antimicrobial and antiviral activity of selenium sulphide nanoparticles synthesised in extracts from spices in natural deep eutectic solvents (NDES). Sustain. Mater. Technol. 32, e00433. doi:10.1016/j.susmat.2022.e00433
Dong, H., Quintilla, A., Cemernjak, M., Popescu, R., Gerthsen, D., Ahlswede, E., et al. (2014). Colloidally stable selenium@copper selenide core@shell nanoparticles as selenium source for manufacturing of copper–indium–selenide solar cells. J. Colloid Interface Sci. 415, 103–110. doi:10.1016/j.jcis.2013.10.001
Dubey, A. K., Chaudhry, S. K., Singh, H. B., Gupta, V. K., and Kaushik, A. (2022). Perspectives on nano-nutraceuticals to manage pre and post COVID-19 infections. Biotechnol. Rep. (Amst). 33, e00712. doi:10.1016/j.btre.2022.e00712
Dworkin, B. M. (1994). Selenium deficiency in HIV infection and the acquired immunodeficiency syndrome (AIDS). Chem. Biol. Interact. 91, 181–186. doi:10.1016/0009-2797(94)90038-8
Erol, S. A., Polat, N., Akdas, S., Ayral, P. A., Anuk, A. T., Tokalioglu, E. O., et al. (2021). Maternal selenium status plays a crucial role on clinical outcomes of pregnant women with COVID‐19 infection. J. Med. Virol. 93, 5438–5445. doi:10.1002/jmv.27064
Ferro, C., Florindo, H. F., and Santos, H. A. (2021). Selenium nanoparticles for biomedical applications: From development and characterization to therapeutics. Adv. Healthc. Mat. 10, 2100598. doi:10.1002/adhm.202100598
Flohé, L., Toppo, S., and Orian, L. (2022). The glutathione peroxidase family: Discoveries and mechanism. Free Radic. Biol. Med. 187, 113–122. doi:10.1016/j.freeradbiomed.2022.05.003
Forootanfar, H., Adeli-Sardou, M., Nikkhoo, M., Mehrabani, M., Amir-Heidari, B., Shahverdi, A. R., et al. (2014). Antioxidant and cytotoxic effect of biologically synthesized selenium nanoparticles in comparison to selenium dioxide. J. Trace Elem. Med. Biol. 28, 75–79. doi:10.1016/j.jtemb.2013.07.005
Gage, A., Brunson, K., Morris, K., Wallen, S. L., Dhau, J., Gohel, H., et al. (2021). Perspectives of manipulative and high-performance nanosystems to manage consequences of emerging new severe acute respiratory syndrome coronavirus 2 variants. Front. Nanotechnol. 3, 700888. doi:10.3389/fnano.2021.700888
Ge, J., Guo, K., Zhang, C., Talukder, M., Lv, M. W., Li, J. Y., et al. (2021). Comparison of nanoparticle-selenium, selenium-enriched yeast and sodium selenite on the alleviation of cadmium-induced inflammation via NF-kB/IκB pathway in heart. Sci. Total Environ. 773, 145442. doi:10.1016/j.scitotenv.2021.145442
Gupta, M., and Gupta, S. (2017). An overview of selenium uptake, metabolism, and toxicity in plants. Front. Plant Sci. 7, 2074. doi:10.3389/fpls.2016.02074
Harthill, M. (2011). Review: Micronutrient selenium deficiency influences evolution of some viral infectious diseases. Biol. Trace Elem. Res. 143, 1325–1336. doi:10.1007/s12011-011-8977-1
Harvey, W. T., Carabelli, A. M., Jackson, B., Gupta, R. K., Thomson, E. C., Harrison, E. M., et al. (2021). SARS-CoV-2 variants, spike mutations and immune escape. Nat. Rev. Microbiol. 19, 409–424. doi:10.1038/s41579-021-00573-0
Haug, A., Graham, R. D., Christophersen, O. A., and Lyons, G. H. (2007). How to use the world's scarce selenium resources efficiently to increase the selenium concentration in food. Microb. Ecol. Health Dis. 19, 209–228. doi:10.1080/08910600701698986
He, L., Zhao, J., Wang, L., Liu, Q., Fan, Y., Li, B., et al. (2021). Using nano-selenium to combat coronavirus disease 2019 (COVID-19)? Nano Today 36, 101037. doi:10.1016/j.nantod.2020.101037
Hiffler, L., and Rakotoambinina, B. (2020). Selenium and RNA virus interactions: Potential implications for SARS-CoV-2 infection (COVID-19). Front. Nutr. 7, 164. doi:10.3389/fnut.2020.00164
Hirano, T., and Murakami, M. (2020). COVID-19: A new virus, but a familiar receptor and cytokine release syndrome. Immunity 52, 731–733. doi:10.1016/j.immuni.2020.04.003
Hosnedlova, B., Kepinska, M., Skalickova, S., Fernandez, C., Ruttkay-Nedecky, B., Peng, Q., et al. (2018). Nano-selenium and its nanomedicine applications: A critical review. Int. J. Nanomedicine 13, 2107–2128. doi:10.2147/IJN.S157541
Hu, W., Zhao, C., Hu, H., and Yin, S. (2021). Food sources of selenium and its relationship with chronic diseases. Nutrients 13, 1739. doi:10.3390/nu13051739
Huang, Y., Wang, Q., Gao, J., Lin, Z., Bañuelos, G. S., Yuan, L., et al. (2013). Daily dietary selenium intake in a high selenium area of Enshi, China. Nutrients 5, 700–710. doi:10.3390/nu5030700
Huang, Z., Rose, A. H., and Hoffmann, P. R. (2012). The role of selenium in inflammation and immunity: From molecular mechanisms to therapeutic opportunities. Antioxid. Redox Signal. 16, 705–743. doi:10.1089/ars.2011.4145
Ibrahim, S., Kerkadi, A., and Agouni, A. (2019). Selenium and health: An update on the situation in the Middle East and north africa. Nutrients 11, 1457. doi:10.3390/nu11071457
Institute of Medicine, Food and Nutrition Board (2000). Dietary reference intakes: Vitamin C, vitamin E, selenium, and carotenoids. Washington, DC: National Academy Press
Ivory, K., Prieto, E., Spinks, C., Armah, C. N., Goldson, A. J., Dainty, J. R., et al. (2017). Selenium supplementation has beneficial and detrimental effects on immunity to influenza vaccine in older adults. Clin. Nutr. 36, 407–415. doi:10.1016/j.clnu.2015.12.003
Jin, Z., Du, X., Xu, Y., Deng, Y., Liu, M., Zhao, Y., et al. (2020). Structure of Mpro from SARS-CoV-2 and discovery of its inhibitors. Nature 582, 289–293. doi:10.1038/s41586-020-2223-y
Kalishwaralal, K., JeyabharathiSundar, S. K., and Muthukumaran, A. (2016). A novel one-pot green synthesis of selenium nanoparticles and evaluation of its toxicity in zebrafish embryos. Artif. Cells Nanomed. Biotechnol. 44, 471–477. doi:10.3109/21691401.2014.962744
Kaushik, A. (2019). Biomedical nanotechnology related grand challenges and perspectives. Front. Nanotechnol. 1, 1. doi:10.3389/fnano.2019.00001
Kaushik, A. K., Dhau, J. S., Gohel, H., Mishra, Y. K., Kateb, B., Kim, N.-Y., et al. (2020). Electrochemical SARS-CoV-2 sensing at point-of-care and artificial intelligence for intelligent COVID-19 management. ACS Appl. Bio Mat. 3, 7306–7325. doi:10.1021/acsabm.0c01004
Kaushik, A. (2021). Manipulative magnetic nanomedicine: The future of COVID-19 pandemic/endemic therapy. Expert Opin. Drug Deliv. 18, 531–534. doi:10.1080/17425247.2021.1860938
Khatiwada, S., and Subedi, A. (2021). A mechanistic link between selenium and coronavirus disease 2019 (COVID-19). Curr. Nutr. Rep. 10, 125–136. doi:10.1007/s13668-021-00354-4
Khurana, A., Tekula, S., Saifi, M. A., Venkatesh, P., and Godugu, C. (2019). Therapeutic applications of selenium nanoparticles. Biomed. Pharmacother. 111, 802–812. doi:10.1016/j.biopha.2018.12.146
Kieliszek, M., Bano, I., and Zare, H. A. (2022). A comprehensive review on selenium and its effects on human health and distribution in Middle eastern countries. Biol. Trace Elem. Res. 200, 971–987. doi:10.1007/s12011-021-02716-z
Kieliszek, M., and Błażejak, S. (2016). Current knowledge on the importance of selenium in food for living organisms: A review. Molecules 21, 609. doi:10.3390/molecules21050609
Kieliszek, M. (2022). Selenium in the prevention of SARS-CoV-2 and other viruses. Biol. Trace Elem. Res. 19, 1–8. doi:10.1007/s12011-022-03208-4
Kieliszeka, M., and Lipinskib, B. (2020). Selenium supplementation in the prevention of coronavirus infections (COVID-19). Med. Hypotheses 143, 109878. doi:10.1016/j.mehy.2020.109878
Kolay, A., Maity, D., Ghosal, P., and Deepa, M. (2019). Selenium nanoparticle-decorated silicon nanowires with enhanced liquid-junction photoelectrochemical solar cell performance. J. Phys. Chem. C 123, 8614–8622. doi:10.1021/acs.jpcc.9b00062
Kujawska, M., Mostafavi, E., and Kaushik, A. (2022). SARS-CoV-2 getting into the brain; neurological phenotype of COVID-19, and management by nano-biotechnology. Neural Regen. Res. 18, 519. doi:10.4103/1673-5374.346486
Kumar, R., Bhasin, K. K., Dhau, J. S., and Singh, A. (2022). Synthesis and characterization of 3-pyridylchalcogen compounds. Inorg. Chem. Commun. 139, 109344. doi:10.1016/j.inoche.2022.109344
Kumari, M., Purohit, M. P., Patnaik, S., Shukla, Y., Kumar, P., and Gupta, K. C. (2018). Curcumin loaded selenium nanoparticles synergize the anticancer potential of doxorubicin contained in self-assembled, cell receptor targeted nanoparticles. Eur. J. Pharm. Biopharm. 130, 185–199. doi:10.1016/j.ejpb.2018.06.030
Li, X., Bhullar, A. S., Binzel, D. W., and Guo, P. (2022). The dynamic, motile and deformative properties of RNA nanoparticles facilitate the third milestone of drug development. Adv. Drug Deliv. Rev. 186, 114316. doi:10.1016/j.addr.2022.114316
Li, Y., Lin, Z., Gong, G., Guo, M., Xu, T., Wang, C., et al. (2019). Inhibition of H1N1 influenza virus-induced apoptosis by selenium nanoparticles functionalized with arbidol through ROS-mediated signaling pathways. J. Mat. Chem. B 7, 4252–4262. doi:10.1039/c9tb00531e
Li, Y., Lin, Z., Guo, M., Zhao, M., Xia, Y., Wang, C., et al. (2018). Inhibition of H1N1 influenza virus-induced apoptosis by functionalized selenium nanoparticles with amantadine through ROSmediated AKT signaling pathways. Int. J. Nanomedicine 13, 2005–2016. doi:10.2147/IJN.S155994
Li, Y., LinGuo, Z. M., Xia, Y., Zhao, M., Wang, C., Xu, T., et al. (2017). Inhibitory activity of selenium nanoparticles functionalized with oseltamivir on H1N1 influenza virus. Int. J. Nanomedicine 12, 5733–5743. doi:10.2147/IJN.S140939
Lin, Y.-C., Hsu, E-C., and Ting, L.-P. (2009). Repression of Hepatitis B viral gene expression by transcription factor nuclear factor-kappaB. Cell. Microbiol. 11, 645–660. doi:10.1111/j.1462-5822.2008.01280.x
Lin, Z., Li, Y., Gong, G., Xia, Y., Wang, C., Chen, Y., et al. (2018). Restriction of H1N1 influenza virus infection by selenium nanoparticles loaded with ribavirin via resisting caspase-3 apoptotic pathway. Int. J. Nanomedicine 13, 5787–5797. doi:10.2147/IJN.S177658
Lin, Z., Li, Y., Guo, M., Xiao, M., Wang, C., Zhao, M., et al. (2017). Inhibition of H1N1 influenza virus by selenium nanoparticles loaded with zanamivir through p38 and JNK signaling pathways. RSC Adv. 7, 35290–35296. doi:10.1039/c7ra06477b
Liu, X., Chen, D., Su, J., Zheng, R., Ning, Z., Zhao, M., et al. (2022). Selenium nanoparticles inhibited H1N1 influenza virus-induced apoptosis by ROS-mediated signaling pathways. RSC Adv. 12, 3862–3870. doi:10.1039/d1ra08658h
Lubos, E., Loscalzo, J., and Handy, D. E. (2011). Glutathione peroxidase-1 in health and disease: From molecular mechanisms to therapeutic opportunities. Antioxid. Redox Signal. 15, 1957–1997. doi:10.1089/ars.2010.3586
Ma, W., Zhan, Y., Zhang, Y., Mao, C., Xie, X., and Lin, Y. (2021). The biological applications of DNA nanomaterials: Current challenges and future directions. Sig. Transduct. Target. Ther. 6, 351. doi:10.1038/s41392-021-00727-9
Mahdavi, M., Mavandadneja, F., Yazdia, M. H., Faghfuri, E., Hashemi, H., Homayouni-Oreh, S., et al. (2017). Oral administration of synthetic seleniumnanoparticles induced robust Th1cytokine pattern after HBs antigenvaccination in mouse model. J. Infect. Public Health 10, 102–109. doi:10.1016/j.jiph.2016.02.006
Majeed, M., Nagabhushanam, K., Gowda, S., and Mundkur, L. (2021). An exploratory study of selenium status in healthy individuals and in patients with COVID-19 in a south Indian population: The case for adequate selenium status. Nutrition 82, 111053. doi:10.1016/j.nut.2020.111053
Majeed, M., Nagabhushanam, K., Prakasan, P., and Mundkur, L. (2022). Can selenium reduce the susceptibility and severity of SARS-CoV-2? A comprehensive review. Int. J. Mol. Sci. 23, 4809. doi:10.3390/ijms23094809
Malachowski, T., and Hassel, A. (2020). Engineering nanoparticles to overcome immunological barriers for enhanced drug delivery. Eng. Regen. 1, 35–50. doi:10.1016/j.engreg.2020.06.001
Maltseva, V. N., Goltyaev, M. V., Turovsky, E. A., and Varlamova, E. G. (2022). Immunomodulatory and anti-inflammatory properties of selenium-containing agents: Their role in the regulation of defense mechanisms against COVID-19. Int. J. Mol. Sci. 23, 2360. doi:10.3390/ijms23042360
Martinez, M. A. (2022). Efficacy of repurposed antiviral drugs: Lessons from COVID-19. Drug Discov. Today 27, 1954–1960. doi:10.1016/j.drudis.2022.02.012
Mengist, H. M., Fan, X., and Jin, T. (2020). Designing of improved drugs for COVID-19: Crystal structure of SARS-CoV-2 main protease M(pro). Signal Transduct. Target. Ther. 5, 67. doi:10.1038/s41392-020-0178-y
Mittal, A. K., and Banerjee, U. C. (2021). In vivo safety, toxicity, biocompatibility and anti-tumour efficacy of bioinspired silver and selenium nanoparticles. Mat. Today Commun. 26, 102001. doi:10.1016/j.mtcomm.2020.102001
Moghadasi, S. A., Becker, J. T., Belica, C., Wick, C., Brown, W. L., and ans Harris, R. S. (2020). Gain-of-Signal Assays for Probing Inhibition of SARS-CoV-2 M(pro)/3CL(pro) in Living Cells. mBio 13, e0078422. doi:10.1128/mbio.00784-22
Moghadaszadeh, B., and Beggs, A. H. (2006). Selenoproteins and their impact on human health through diverse physiological pathways. Physiol. (Bethesda) 21, 307–315. doi:10.1152/physiol.00021.2006
Mohs, R. C., and Greig, N. H. (2017). Drug discovery and development: Role of basic biological research. Alzheimer's. Dementia Transl. Res. Clin. Interventions 3, 651–657. doi:10.1016/j.trci.2017.10.005
Mostafavi, E., Dubey, A. K., Teodori, L., Ramakrishna, S., and Kaushik, A. (2022). SARS-CoV-2 omicron variant: A next phase of the COVID-19 pandemic and a call to arms for system sciences and precision medicine. MedComm 3, 1199–e128. doi:10.1002/mco2.119
Mujawar, M. A., Gohel, H., Bhardwaj, S. K., Srinivasan, S., Hickman, N., and Kaushik, A. (2020). Nano-enabled biosensing systems for intelligent healthcare: Towards COVID-19 management. Mat. Today Chem. 17, 100306. doi:10.1016/j.mtchem.2020.100306
Mukherjee, S., Weiner, W. S., Schroeder, C. E., Simpson, D. S., Hanson, A. M., Sweeney, N. L., et al. (2014). Ebselen inhibits hepatitis C virus NS3 helicase binding to nucleic acid and prevents viral replication. ACS Chem. Biol. 9, 2393–2403. doi:10.1021/cb500512z
Munguti, C. M., Rifai, M. A., and Shaheen, W. (2017). A rare cause of cardiomyopathy: A case of selenium deficiency causing severe cardiomyopathy that improved on supplementation. Cureus 9, e1627. doi:10.7759/cureus.1627
Muzembo, B. A., Ngatu, N. R., Januka, K., Huang, H. L., Nattadech, C., Suzuki, T., et al. (2019). Selenium supplementation in HIV-infected individuals: A systematic review of randomized controlled trials. Clin. Nutr. ESPEN 34, 1–7. doi:10.1016/j.clnesp.2019.09.005
Nienhaus, K., Wang, H., and Nienhaus, G. U. (2020). Nanoparticles for biomedical applications: Exploring and exploiting molecular interactions at the nano-bio interface. Mat. Today Adv. 5, 100036. doi:10.1016/j.mtadv.2019.100036
Nogueira, C. W., Zeni, G., and Rocha, J. B. T. (2004). Organoselenium and organotellurium compounds: Toxicology and pharmacology. Chem. Rev. 104, 6255–6286. doi:10.1021/cr0406559
Oeckinghaus, A., and Ghosh, S. (2009). The NF- B family of transcription factors and its regulation. Cold Spring Harb. Perspect. Biol. 1, a000034. doi:10.1101/cshperspect.a000034
Oostwoud, L. C., Gunasinghe, P., Seow, H. J., Ye, J. M., Selemidis, S., Bozinovski, S., et al. (2016). Apocynin and ebselen reduce influenza A virus-induced lung inflammation in cigarette smoke-exposed mice. Sci. Rep. 6, 20983. doi:10.1038/srep20983
Paliwal, P., Sargolzaei, S., Bhardwaj, S. K., Bhardwaj, V., Dixit, C., and Kaushik, A. (2020). Grand challenges in bio-nanotechnology to manage the COVID-19 pandemic. Front. Nanotechnol. 2, 571284. doi:10.3389/fnano.2020.571284
Pavlovic, Z., Miletic, I., Zekovic, M., Nikolic, M., and Glibetic, M. (2018). Impact of selenium addition to animal feeds on human selenium status in Serbia. Nutrients 10, 225. doi:10.3390/nu10020225
Pedrosa, L. F. C., Barros, A. N. A. B., and Leite-Lais, L. (2022). Nutritional risk of vitamin D, vitamin C, zinc, and selenium deficiency on risk and clinical outcomes of COVID-19: A narrative review. Clin. Nutr. ESPEN 47, 9–27. doi:10.1016/j.clnesp.2021.11.003
Pelaz, B., Alexiou, C., Alvarez-Puebla, R. A., Alves, F., Andrews, A. M., Ashraf, S., et al. (2017). Diverse applications of nanomedicine. ACS Nano 11, 2313–2381. doi:10.1021/acsnano.6b06040
Peng, X., Wang, L., Zhang, X., Gao, B., Fu, J., Xiao, S., et al. (2015). Reduced graphene oxide encapsulated selenium nanoparticles for high-power lithium–selenium battery cathode. J. Power Sources 288, 214–220. doi:10.1016/j.jpowsour.2015.04.124
Pieczyńska, J., and Grajeta, H. (2015). The role of selenium in human conception and pregnancy. J. Trace Elem. Med. Biol. 29, 31–38. doi:10.1016/j.jtemb.2014.07.003
Ramya, S., Shanmugasundaram, T., and Balagurunathan, R. (2015). Biomedical potential of actinobacterially synthesized selenium nanoparticles with special reference to anti-biofilm, anti-oxidant, wound healing, cytotoxic and anti-viral activities. J. Trace Elem. Med. Biol. 32, 30–39. doi:10.1016/j.jtemb.2015.05.005
Ren, X., Wang, S., Zhang, C., Hu, X., Zhou, L., Li, Y., et al. (2020). Selenium ameliorates cadmium-induced mouse leydig TM3 cell apoptosis via inhibiting the ROS/JNK/c-jun signaling pathway. Ecotoxicol. Environ. Saf. 192, 110266. doi:10.1016/j.ecoenv.2020.110266
Renata, R. B. N., Arely, G. R. A., Gabriela, L. M. A., and Esther, M. L. M. (2022). Immunomodulatory role of microelements in COVID-19 outcome: A relationship with nutritional status. Biol. Trace Elem. Res. doi:10.1007/s12011-022-03290-8
Ruiz-Aravena, M., McKee, C., Gamble, A., Lunn, T., Morris, A., Snedden, C. E., et al. (2022). Ecology, evolution and spillover of coronaviruses from bats. Nat. Rev. Microbiol. 20, 299–314. doi:10.1038/s41579-021-00652-2
Sadique, M. A., Yadav, S., Ranjan, P., Verma, S., Salammal, S. T., Khan, M. A., et al. (2021). High-performance antiviral nano-systems as a shield to inhibit viral infections: SARS-CoV-2 as a model case study. J. Mat. Chem. B 9, 4620–4642. doi:10.1039/D1TB00472G
Sakr, T. M., Korany, M., and Katti, K. V. (2018). Selenium nanomaterials in biomedicine—an overview of new opportunities in nanomedicine of selenium. J. Drug Deliv. Sci. Technol. 46, 223–233. doi:10.1016/j.jddst.2018.05.023
Sands, K. N., and Back, T. G. (2018). Key steps and intermediates in the catalytic mechanism for the reduction of peroxides by the antioxidant ebselen. Tetrahedron 74, 4959–4967. doi:10.1016/j.tet.2018.05.027
Sarwar, N., Akhtar, M., Kamran, M. A., Imran, M., Riaz, M. A., Kamran, K., et al. (2020). Selenium biofortification in food crops: Key mechanisms and future perspectives. J. Food Compost. Anal. 93, 103615. doi:10.1016/j.jfca.2020.103615
Schmidt, R. L., and Simonović, M. (2012). Synthesis and decoding of selenocysteine and human health. Croat. Med. J. 53, 535–550. doi:10.3325/cmj.2012.53.535
Schoeman, D., and Fielding, B. C. (2019). Coronavirus envelope protein: Current knowledge. Virol. J. 16, 69. doi:10.1186/s12985-019-1182-0
Seale, L. A., Torres, D. J., Berry, M. J., and Pitts, M. W. (2020). A role for selenium-dependent GPX1 in SARS-CoV-2 virulence. Am. J. Clin. Nutr. 112, 447–448. doi:10.1093/ajcn/nqaa177
Shao, C., Yu, Z., Luo, T., Zhou, B., Song, Q., Li, Z., et al. (2022). Chitosan-coated selenium nanoparticles attenuate PRRSV replication and ROS/JNK-Mediated apoptosis in vitro. Int. J. Nanomedicine 17, 3043–3054. doi:10.2147/IJN.S370585
Sharma, N., Dhau, J. S., Singh, A., Singh, A., and Malik, A. K. (2017a). FT-IR, NMR, molecular structure and HOMO-LUMO studies of 3, 5-dimethyl-2-pyridylselenium compounds by density functional theory. Phosphorus Sulfur Silicon Relat. Elem. 192, 368–375. doi:10.1080/10426507.2016.1244205
Sharma, N., Singh, A., and Dhau, J. S. (2017b). Synthesis, characterization, and computational studies of selenium derivatives of 3, 5-dichloropyridine. J. Heterocycl. Chem. 54, 2054–2066. doi:10.1002/jhet.2804
Sharma, P. K., Kim, E.-S., Mishra, S., Ganbold, E., Seong, R.-S., Kaushik, A. K., et al. (2021). Ultrasensitive and reusable graphene oxide-modified double-interdigitated capacitive (DIDC) sensing chip for detecting SARS-CoV-2. ACS Sens. 6, 3468–3476. doi:10.1021/acssensors.1c01437
Shereen, M. A., Khan, S., Kazmi, A., Bashir, N., and Siddique, R. (2020). COVID-19 infection: Emergence, transmission, and characteristics of human coronaviruses. J. Adv. Res. 24, 91–98. doi:10.1016/j.jare.2020.03.005
Shi, L-G., Yang, R-J., Yue, W-B., Xun, W-J., Zhang, C-X., Ren, Y-S., et al. (2010). Effect of elemental nano-selenium on semen quality, glutathione peroxidase activity, and testis ultrastructure in male Boer goats. Anim. Reprod. Sci. 118, 248–254. doi:10.1016/j.anireprosci.2009.10.003
Shi, X.-D., Tian, Y.-Q., Wu, J.-L., and Wang, S.-Y. (2021). Synthesis, characterization, and biological activity of selenium nanoparticles conjugated with polysaccharides. Crit. Rev. Food Sci. Nutr. 61, 2225–2236. doi:10.1080/10408398.2020.1774497
Siesa, H., and Parnham, M. J. (2020). Potential therapeutic use of ebselen for COVID-19 and other respiratory viral infections. Free Radic. Biol. Med. 156, 107–112. doi:10.1016/j.freeradbiomed.2020.06.032
Simanjuntak, Y., Liang, J. J., Chen, S. Y., Li, J. K., Lee, Y. L., Wu, H. C., et al. (2018). Ebselen alleviates testicular pathology in mice with Zika virus infection and prevents its sexual transmission. PLoS Pathog. 14, e1006854. doi:10.1371/journal.ppat.1006854
Singh, A., Dhau, J., and Kumar, R. (2021a). “Application of carbon-based nanomaterials for removal of hydrocarbons, chapter 9, pages 205-227,” in New Frontiers of nanomaterials in environmental science. Editors R. Kumar, R. Kumar, and G. Kaur (Singapore: Springer). doi:10.1007/978-981-15-9239-3
Singh, A., Dhau, J. S., Sharma, N., and Singh, A. (2015). Synthesis of C-6 and C-3 substituted chalcogen derivatives of 2-methoxypyridine through lithiation of 2-methoxypyridine: An experimental and quantum chemical study. Inorganica Chim. Acta 432, 109–114. doi:10.1016/j.ica.2015.04.002
Singh, A., Kaushik, A., Dhau, J. S., and Kumar, R. (2022). Exploring coordination preferences and biological applications of pyridyl-based organochalcogen (Se, Te) ligands. Coord. Chem. Rev. 450, 214254. doi:10.1016/j.ccr.2021.214254
Singh, A., Maximoff, S. N., Brandão, P., and Dhau, J. S. (2021b). Crystal structures of bis(2-methoxy-3-pyridyl) diselenide and bis(2-methoxy-3-pyridyl) ditelluride: An investigation by X-ray crystallography and DFT calculations. J. Mol. Struct. 1240, 130568. doi:10.1016/j.molstruc.2021.130568
Stoffaneller, R., and Morse, L. N. (2015). A review of dietary selenium intake and selenium status in europe and the Middle East. Nutrients 7, 1494–1537. doi:10.3390/nu7031494
Surai, P. F., Kochish, I. I., Fisinin, V. I., and Velichko, O. A. (2018). Selenium in poultry nutrition: From sodium selenite to organic selenium sources. J. Poult. Sci. 55, 79–93. doi:10.2141/jpsa.0170132
Tinggi, U. (2008). Selenium: Its role as antioxidant in human health. Environ. Health Prev. Med. 13, 102–108. doi:10.1007/s12199-007-0019-4
Tiwari, S., Juneja, S., Ghosal, A., Bandara, N., Khan, R., Wallen, S. L., et al. (2022). Antibacterial and antiviral high-performance nanosystems to mitigate new SARS-CoV-2 variants of concern. Curr. Opin. Biomed. Eng. 21, 100363. doi:10.1016/j.cobme.2021.100363
Varahachalam, S. P., Lahooti, B., Chamaneh, M., Bagchi, S., Chhibber, T., Morris, K., et al. (2021). Nanomedicine for the SARS-CoV-2: State-of-the-Art and future prospects. Int. J. Nanomedicine 16, 539–560. doi:10.2147/IJN.S283686
Wang, C., Chen, H., Chen, D., Zhao, M., Lin, Z., Guo, M., et al. (2020). The inhibition of H1N1 influenza virus-induced apoptosis by surface decoration of selenium nanoparticles with β-thujaplicin through reactive oxygen species-mediated AKT and p53 signaling pathways. ACS Omega 5, 30633–30642. doi:10.1021/acsomega.0c04624
Wang, H., Zhang, J., and Yu, H. (2007). Elemental selenium at nano size possesses lower toxicity without compromising the fundamental effect on selenoenzymes: Comparison with selenomethionine in mice. Free Radic. Biol. Med. 42, 1524–1533. doi:10.1016/j.freeradbiomed.2007.02.013
Wang, X., and Guan, Y. (2021). COVID-19 drug repurposing: A review of computational screening methods, clinical trials, and protein interaction assays. Med. Res. Rev. 41, 5–28. doi:10.1002/med.21728
Wójtowicz, H., Kloc, K., Maliszewska, I., Młochowski, J., Piętka, M., and Piasecki, E. (2004). Azaanalogues of ebselen as antimicrobial and antiviral agents: Synthesis and properties. Il Farm. 59, 863–868. doi:10.1016/j.farmac.2004.07.003
World Health Organization (2021). Tracking SARS-CoV-2 variants. Available at: https://www.who.int/activities/tracking-SARS-CoV-2-variants.
Xie, X., Jiang, K., Li, B., Hou, S., Tang, H., Shao, B., et al. (2022). A small-molecule self-assembled nanodrug for combination therapy of photothermal-differentiation-chemotherapy of breast cancer stem cells. Biomaterials 286, 121598. doi:10.1016/j.biomaterials.2022.121598
Yadav, R., Chaudhary, J. K., Jain, N., Chaudhary, P. K., Khanra, S., Dhamija, P., et al. (2021). Role of structural and non-structural proteins and therapeutic targets of SARS-CoV-2 for COVID-19. Cells 10, 821. doi:10.3390/cells10040821
Yalcin, H. C., and Kaushik, A. (2021). Support of intelligent emergent materials to combat COVID-19 pandemic. emergent Mat. 4, 1–2. doi:10.1007/s42247-021-00189-3
Yang, T., Lee, S. Y., Park, K. C., Park, S. H., Chung, J., and Lee, S. (2022). The effects of selenium on bone health: From element to therapeutics. Molecules 27, 392. doi:10.3390/molecules27020392
Yehia, N., Sabour, M. A. A., Erfan, A. M., Ali, Z. M., Soliman, R. A., Samy, A., et al. (2022). Selenium nanoparticles enhance the efficacy of homologous vaccine against the highly pathogenic avian influenza H5N1 virus in chickens. Saudi J. Biol. Sci. 29, 2095–2111. doi:10.1016/j.sjbs.2021.11.051
Youn, H-S., Lim, H. J., Choi, Y. J., Lee, J. Y., Lee, M-Y., and Ryu, J-H. (2008). Selenium suppresses the activation of transcription factor NF-κB and IRF3 induced by TLR3 or TLR4 agonists. Int. Immunopharmacol. 8, 495–501. doi:10.1016/j.intimp.2007.12.008
Young, B. E., Fong, S-W., Chan, Y-H., Mak, T-M., Ang, L. W., Anderson, D. F., et al. (2020). Effects of a major deletion in the SARS-CoV-2 genome on the severity of infection and the inflammatory response : An observational cohort study. Lancet 396, P603–P611. doi:10.1016/S0140-6736(20)31757-8
Zhang, D. W, D. W., Taylor, E. W., Bennett, K., Xu, L., Yin, Z. H., Chang, S., et al. (2020). The selenium-containing drug ebselen potently disrupts LEDGF/p75-HIV-1 integrase interaction by targeting LEDGF/p75. J. Enzyme Inhib. Med. Chem. 35, 906–912. doi:10.1080/14756366.2020.1743282
Zhang, J, J., Taylor, E. W., Bennett, K., Saad, R., and Rayman, M. P. (2020). Association between regional selenium status and reported outcome of COVID-19 cases in China. Am. J. Clin. Nutr. 111, 1297–1299. doi:10.1093/ajcn/nqaa095
Zhong, J., Xia, Y., Hua, L., Liu, X., Xiao, M., Xu, T., et al. (2019). Functionalized selenium nanoparticles enhance the anti-EV71 activity of oseltamivir in human astrocytoma cell model. Artif. Cells Nanomed. Biotechnol. 47, 3485–3491. doi:10.1080/21691401.2019.1640716
Zhou, H., Wang, T., Li, Q., and Li, D. (2018). Prevention of keshan disease by seleniumsupplementation: A systematic review and meta-analysis. Biol. Trace Elem. Res. 186, 98–105. doi:10.1007/s12011-018-1302-5
Zhu, H., Wei, L., and Niu, P. (2020). The novel coronavirus outbreak in Wuhan, China. Glob. Health Res. Policy 5, 6. doi:10.1186/s41256-020-00135-6
Keywords: COVID-19 infection, SARS-CoV-2, nanoselenium, antiviral, antimicrobial
Citation: Singh A, Singh P, Kumar R and Kaushik A (2022) Exploring nanoselenium to tackle mutated SARS-CoV-2 for efficient COVID-19 management. Front. Nanotechnol. 4:1004729. doi: 10.3389/fnano.2022.1004729
Received: 27 July 2022; Accepted: 12 September 2022;
Published: 07 October 2022.
Edited by:
Kumud Malika Tripathi, Indian Institute of Petroleum and Energy (IIPE), IndiaReviewed by:
Nisha Dhiman, Indian Institute of Petroleum and Energy (IIPE), IndiaBuddolla Viswanath, Dr. Buddolla’s Institute of Life Sciences, India
Copyright © 2022 Singh, Singh, Kumar and Kaushik. This is an open-access article distributed under the terms of the Creative Commons Attribution License (CC BY). The use, distribution or reproduction in other forums is permitted, provided the original author(s) and the copyright owner(s) are credited and that the original publication in this journal is cited, in accordance with accepted academic practice. No use, distribution or reproduction is permitted which does not comply with these terms.
*Correspondence: Avtar Singh, YXZ0YXJsZGgwMDdAZ21haWwuY29t; Ajeet Kaushik, YWplZXQubnBsQGdtYWlsLmNvbQ==