- 1Department of Biology, College of Natural and Computational Sciences, Debre Markos University, Debre Markos, Ethiopia
- 2Department of Medicine and Health Sciences, University of Molise, Campobasso, Italy
- 3Tropical and Infectious Diseases Research Center, Jimma University, Jimma, Ethiopia
- 4Faculty of Health Sciences, School of Medical Laboratory Sciences, Jimma University, Jimma, Ethiopia
- 5Department of Agriculture, Environment and Food Sciences, Università degli Studi del Molise, Campobasso, Italy
- 6Department of Pharmaceutical and Health Sciences, Università degli Studi di Catania, Catania, Italy
Mosquito-borne diseases such as malaria, dengue fever, West Nile virus, chikungunya, Zika fever, and filariasis have the greatest health and economic impact. These mosquito-borne diseases are a major cause of morbidity and mortality in tropical and sub-tropical areas. Due to the lack of effective vector containment strategies, the prevalence and severity of these diseases are increasing in endemic regions. Nowadays, mosquito infection by the endosymbiotic Wolbachia represents a promising new bio-control strategy. Wild-infected mosquitoes had been developing cytoplasmic incompatibility (CI), phenotypic alterations, and nutrition competition with pathogens. These reduce adult vector lifespan, interfere with reproduction, inhibit other pathogen growth in the vector, and increase insecticide susceptibility of the vector. Wild, uninfected mosquitoes can also establish stable infections through trans-infection and have the advantage of adaptability through pathogen defense, thereby selectively infecting uninfected mosquitoes and spreading to the entire population. This review aimed to evaluate the role of the Wolbachia symbiont with the mosquitoes (Aedes, Anopheles, and Culex) in reducing mosquito-borne diseases. Global databases such as PubMed, Web of Sciences, Scopus, and pro-Quest were accessed to search for potentially relevant articles. We used keywords: Wolbachia, Anopheles, Aedes, Culex, and mosquito were used alone or in combination during the literature search. Data were extracted from 56 articles’ texts, figures, and tables of the included article.
1. Introduction
Due to their high adaptation capacity to various environments, mosquitoes have endured for millions of years (Couper et al., 2021). Different pathogenic, endosymbiont and symbiotic organisms have the ability to infect them. The main carriers of human pathogens are various species of mosquitoes from the genera Aedes, Anopheles, and Culex. Those mosquito genera are vectors of emerging and reemerging human diseases caused by pathogens, such as protozoan parasites, viruses, and nematodes (Iturbe-Ormaetxe et al., 2011).
Among the protozoan parasitic diseases, malaria is caused by different Plasmodium species. It is a life-threatening disease spread to humans by the bite of infected female Anopheles mosquitoes. According to the World Health Organization (WHO) report in 2022, globally, more than 3.2 billion people (almost half the world’s population) are at risk of malaria; furthermore, 245 million malaria cases have been recorded, with a mortality of 0.6 million. Children are the most affected group of patients. Malaria is also a great burden from an economic point of view; $ 12 billion is lost per year in economic productivity in Africa alone (WHO, 2022).
Similarly, among mosquito-borne viral diseases, viruses belonging to the Flaviviridae family, such as Dengue virus, Zika virus, yellow fever virus, chikungunya virus, and West Nile virus, can be transmitted to humans by Aedes aegypti and Ae. albopictus.
About half of the world’s population is at risk of dengue, which is estimated to infect 100–400 million people yearly. It is found in tropical and subtropical climates worldwide, mainly in urban and semi-urban areas (Leta et al., 2018). Likewise, West Nile fever is caused by an RNA virus, namely West Nile virus (WNV). The virus causes severe disease in birds, horses, and other mammals, but most human infections occur through the bite of infected mosquitoes. About 1 in 150 infected people develop neurological disease and die. It is common in Africa, Europe, the Middle East, North America, and Western Asia (Leta et al., 2018; CDC, 2023). In addition, Yellow fever is caused by an arbovirus and is transmitted to humans through the bites of infected Aedes and Haemagogus mosquitoes. It is a high-impact high-threat disease with the risk of cross-boundary transmission (Leta et al., 2018; WHO, 2023a).
Moreover, the Zika virus is transmitted to humans through the bites of infected mosquitoes, mainly Ae. aegypti, particularly in tropical regions. Zika virus infection clinical manifestation is similar to other arboviruses, with fever, skin rash, conjunctivitis, muscle and joint pain, fatigue, and headache (Leta et al., 2018; WHO, 2023b). On the other hand, Chikungunya fever is caused by an RNA virus belonging to the alphavirus genus, the Togaviridae family. Infection in humans occurs through the bite of infected female mosquitoes (commonly Ae. aegypti and Ae. albopictus). More than 2 million cases arise each year. The disease is now identified in more than 110 countries (Bettis et al., 2022).
Among nematode infections transmitted by mosquito vectors, filariasis is mainly caused by the filarial worm Wuchereria bancrofti, and less commonly Brugia malayi and Brugia timori. Anopheles is the main filariasis vector in Africa, however, in the Americas the main vector is Culex. It is also transmitted by the bite of infected Aedes and Mansonia species. Filariasis has been considered a neglected tropical disease. However, it is the second leading cause of permanent malformation and disability, next to leprosy worldwide. Lymphatic filariasis affects the lymphatic system and causes abnormal enlargement of body parts, which can cause pain, severe disability, and social stigma. It affects more than 120 million of people in 72 tropical and subtropical countries. Over 882 million people in 44 countries worldwide remain threatened by lymphatic filariasis and require preventive chemotherapy to stop the spread of this parasitic infection (Bizhani et al., 2021; WHO, 2021).
To reduce the threat and burden of these vector-borne diseases, insecticides have been widely used in the last many years. However, due to the frequent and prolonged use of insecticides to control insect disease vectors and pests of crops, mosquitoes developed resistance to several classes of insecticides. As a result, bacteria belonging to the Wolbachia genus have been proposed as potential candidates for mosquito-borne disease control strategies (van den Berg et al., 2021). A brief timeline of Wolbachia isolation, the impact of infection, and utilization as a prevention method is presented in Figure 1 (Werren and O’Neill, 1997; Carrington et al., 2011; Kamtchum-Tatuene et al., 2017; Dorigatti et al., 2018).
Wolbachia is a genus of Gram-negative, non-spore-forming, obligate intracellular parasitic bacteria that frequently infect mosquitoes. It is a member of the Alphaproteobacteria belonging to the Rickettsiales order. The bacterium was first isolated in 1924 by Hertig and Wolbach from the Cx. pipiens germlines (Hertig and Wolbach, 1924). Later in 1936, Hertig, designated it as Wolbachia pipientis (Hertig, 1936; Philip, 1956).
In the last two decades, different strains of Wolbachia were isolated and identified by genome sequencing: Wolbachia wAna, Wolbachia wSim, Wolbachia wMel, and Wolbachia wMoj from Drosophila species (Salzberg et al., 2005). Then different Wolbachia strains are grouped into two major phylogenetic lineages. More than 18 clades, ranging from A to R, have been identified, and almost all were isolated from arthropods (Landmann, 2019). The general distribution of Wolbachia strains and their associated supergroups in mosquitoes are summarized in Figure 2 (Inácio da Silva et al., 2021).
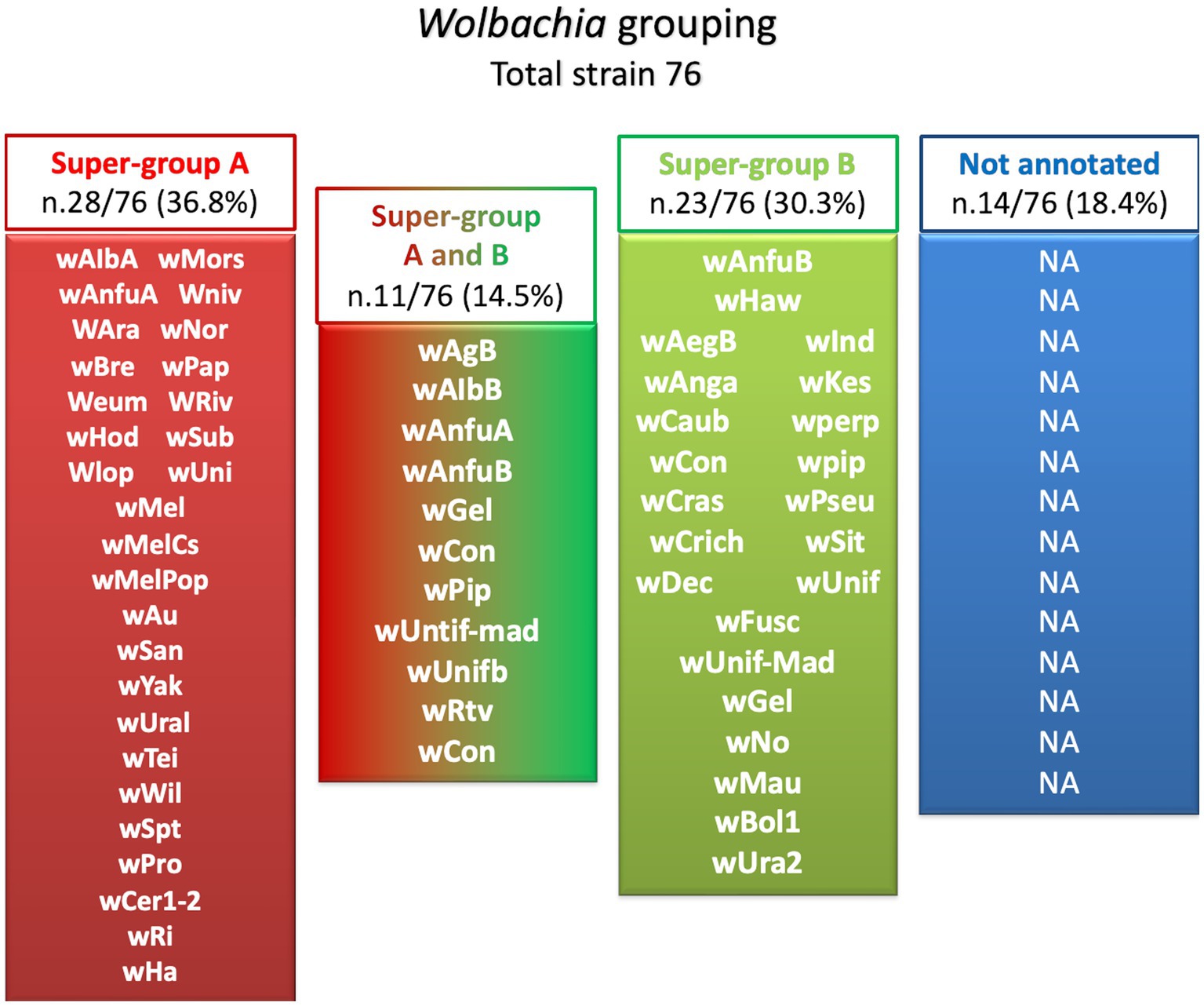
Figure 2. Wolbachia strains isolated from mosquito grouping. Of the 76 isolated Wolbachia strains, 28 (36.8%) belong to super-group A (Red), 23 strains (30.3%) are also grouped in super-group B (Green), 11 strains (14.5%) are also grouped under both A and B (Red and Green), while 14 (18.4%) were non annotated (Blue).
Besides mosquitoes, these intracellular bacteria selectively infect arthropods, nematodes, and other organisms but are harmless to humans (Osei-Poku et al., 2012). It forms endosymbiotic relationships that range from parasitism to mutualism (Zug and Hammerstein, 2012; Sullivan, 2017). Parasitism and mutualism are host, environment, temperature, and density-dependent induced by the same genetic machinery and shifted by selection (Bordenstein et al., 2009; Zug and Hammerstein, 2015; Rohrscheib et al., 2016). Parasitism persistently affects several hosts’ biological indicators, such as physiology, immunity, and host development (Werren et al., 2008; Gutzwiller et al., 2015). The host’s capacity for reproduction was also altered. Additionally, it makes arthropods sterile, and infertile, with reduced longevity, which strongly impacts male mosquitoes (Werren and Windsor, 2000; Ahmed et al., 2015; Sicard et al., 2019). On the other hand, mutualism provides resistance to viral pathogens or the provision of metabolites during host nutritional stress (Allman et al., 2020; Kaur et al., 2021).
The bacterium has the ability to be transmitted vertically through insect eggs and spread horizontally across populations (Hedges et al., 2008; Zug and Hammerstein, 2012; Duron et al., 2015). A vertically transmitted Wolbachia is frequently found in the insect’s endosymbionts, with a 28%–30% prevalence of naturally infected mosquitoes (Kittayapong et al., 2000; Dorigatti et al., 2018; Inácio da Silva et al., 2021). Furthermore, there are different types of symbiont transmission, from vertical (genetic) to horizontal (infectious), with horizontal transmission opting for parasitism. In contrast, vertically transmitted endosymbionts evolve toward reciprocity (Zug and Hammerstein, 2015) among naturally infected genera: Aedes, Culex, Drosophila, and other insect species (Osei-Poku et al., 2012; Sicard et al., 2019; Inácio da Silva et al., 2021) but not commonly reported in Ae. aegypti and Anopheles species.
Even though Wolbachia infection is transmitted between unrelated species, it spreads more quickly among related species. As a result, strains that naturally exist in mosquitoes are suitable for trans-infection into different vector species, enabling bacterial diffusion among mosquito populations (Turelli and Hoffmann, 1991).
Wolbachia-infected mosquitoes reduce mosquito-borne diseases by reducing competent mosquito populations or the vector’s number of mosquitoes and/or pathogen replication (Yen and Failloux, 2020). This is due to CI stimulated by the dynamics of Wolbachia strains introduced into a mosquito population and immune modulation (Kambris et al., 2010), which are triggered to change the host’s behavior and the pathogenic transmission effect (Sinkins et al., 2005; Hedges et al., 2008; Kambris et al., 2009, 2010; Dorigatti et al., 2018). This phenomenon reduces pathogen replication and disease transmissions by vectors to humans and/or animals.
In this review, we focused on assessing the role of Wolbachia infection in the genera Aedes, Anopheles, and Culex in reducing vector-borne diseases. Global electronic databases (PubMed, Web of Sciences, Scopus, and Pro-Quest) were used to search potentially relevant and most recent articles published from 2000 to 2022. Wolbachia, Anopheles, Aedes, Culex, and mosquito were used alone or in combination as a keyword during the literature search. The search was conducted from November 15th to December 12th, 2022. Papers were chosen according to topic pertinence; only research articles published in English and articles with all the required information were included in the review. Data were extracted from the included articles’ texts, figures, and tables, of the included articles. Preliminary 946 articles were accessed, of which only 56 were used for review, as shown in Supplementary Figure S1 and Supplementary Table S1.
2. Wolbachia strain and mosquito infection
This study retrieved 56 original studies. Of these, 32 and 13 studies reported infection of Aedes and Anopheles species by Wolbachia. Other 11 original studies revealed infection in Culex species (Supplementary Figure S1 and Supplementary Table S1).
2.1. Wolbachia infection in Aedes species
The genus Aedes includes more than 950 species and is one of the most widespread mosquito genera in the world (Rogers, 2023). Among them, Ae. aegypti and Ae. albopictus are the most known biological vectors of vector-borne diseases (Brelsfoard and Dobson, 2011; Silva et al., 2017; Damiani et al., 2022) and are included in this study. These two main species are primarily responsible for spreading filariasis, dengue, yellow fever, chikungunya, West Nile Virus, and Zika fever, which can result in serious human diseases (Hoey, 2000; Brasil et al., 2016). These illnesses are a major public health problem resulting in millions of infections and thousands of fatalities yearly (Caragata et al., 2021).
Due to the disease’s severity and the limitation of current prevention patterns, entomopathogenic bacteria have been explored to enhance current control measures and proposed as an effective strategy to reduce the increasing problem of vector-borne diseases (Turley et al., 2009; Mohanty et al., 2016; Yen and Failloux, 2020).
A recent molecular study by Li et al. (2023) in the Chinese province of Hainan revealed that the prevalence of Wolbachia was 86.7% from field-collected Ae. albopictus (Li et al., 2023). Another study conducted in eastern Thailand by Kittayapong et al. (2002) demonstrated the maternal transmission of Wolbachia from field-collected Ae. albopictus was nearly 100%. Wild infections also have efficient vertical transmission across host generations, essential for symbiosis. Even though there was no natural infection report of Ae. aegypti populations by Wolbachia, many studies indicated trans-infection techniques to infect non-wild infected mosquito populations (Turley et al., 2009; Walker et al., 2011; Jeffries and Walker, 2015; O’Neill, 2018; Ding et al., 2020; Liew et al., 2021). This technique established stable vertical transmission in Ae. albopictus, Ae. aegypti and Anopheles species (McMeniman et al., 2009; Iturbe-Ormaetxe et al., 2011; Calvitti et al., 2014).
2.1.1. Wolbachia infection and its effect on Aedes species
Wolbachia infection on Aedes is becoming an increasingly popular alternative candidate strategy for controlling vector-borne disease transmission (Brelsfoard and Dobson, 2011). According to research findings, infected females can successfully mate with infected and uninfected males and give live Wolbachia-positive offspring (Sinkins, 2004; O’Neill, 2018). On the other hand, when uninfected females mate with infected males, they produce non-viable eggs (Figure 3) (Charlat et al., 2001; Poinsot et al., 2003; Werren et al., 2008; Brelsfoard and Dobson, 2011; Beebe et al., 2021). As a result of male sperm infection, haploid cells do not effectively fuse with uninfected eggs, causing the failure of embryonic development or early embryonic death (Caragata et al., 2021). Other research findings pointed out that Wolbachia-infected male nutrition can be linked to reduced fertility and fecundity in mates (Islam and Dobson, 2006; Beebe et al., 2021). This disrupts the normal development of the zygote produced by infected males and uninfected females mating (Serbus et al., 2008).
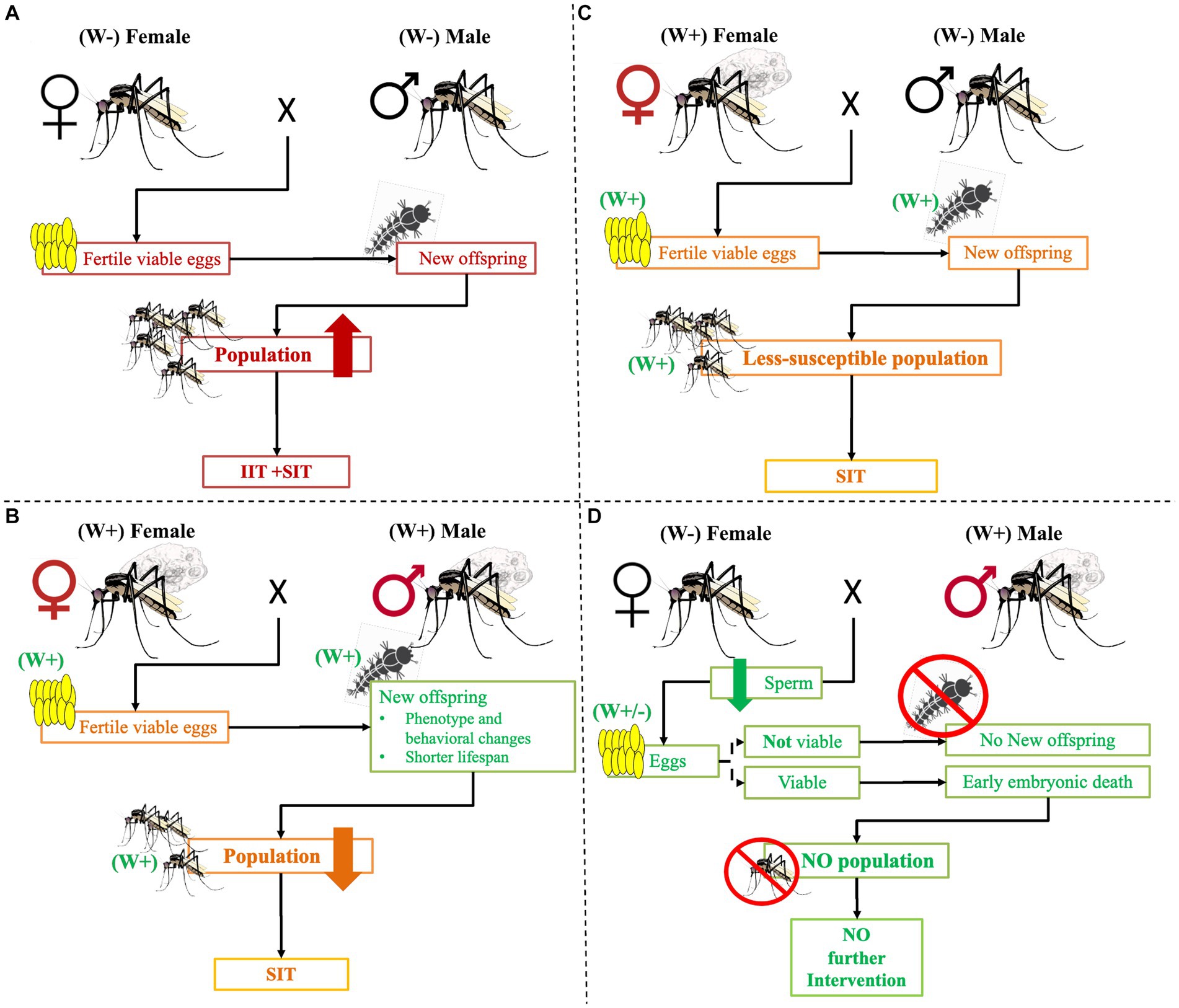
Figure 3. The possible crosses between Wolbachia-infected and/or uninfected mosquitos. Maternal transmission of Wolbachia effects. (A) When Wolbachia uninfected female and male mosquitoes mate, they will give a viable egg that will continue the next generation and disease transmission. To tackle it, intervention, IIT, and/or SIT are needed. Similarly, (B) when infected females and males mate, they produce infected viable eggs. However, the development of the offspring continues the phenotype, adult behaviors may change, the life span is short, and the population declines. As a result, no more diseases frequently occur, but intervention like SIT is still needed. (C) When infected females mate with uninfected males, they produce infected viable eggs that can grow but are less susceptible to developing pathogens and transmitting diseases. The adult life span may be short and may not effectively bite humans and other hosts. Nevertheless, control intervention such as SIT is needed. (D) When an uninfected female mating with an infected male, no more viable egg is produced or early embryonic death occurs in the new generation. As a result, no more intervention is needed. Key: W+: Wolbachia infected, W−: Wolbachia uninfected, ×: Mates.
The study conducted in Crevalcore, Italy by Puggioli et al. (2016) focused on using a specific Ae. albopictus line that was genetically modified to produce sterile males generated by introducing wPip in the ARwP line. The finding showed bidirectional reproductive barriers between infected and uninfected mosquitoes, meaning that when infected males mate with uninfected females or vice versa, the eggs produced fail to develop or hatch, thus leading to a reduction in the overall mosquito population (Puggioli et al., 2016). Similarly, the study conducted by Moretti et al. (2018) in Italy focused on a genetically manipulated line of Ae. albopictus mosquitoes using ARwP-M reduced Ae. albopictus populations. This suggested the introduction of a combined ARwP-M line, which carries wPip and wMel-induced sterility and virus protection to fight Ae. Albopictus-borne viruses. This could be a potential strategy for controlling Ae. albopictus populations and reducing the transmission of chikungunya and dengue viruses (Moretti et al., 2018).
Based on this, to reduce the Ae. albopictus population in the field, Zheng et al. (2019) used Incompatible Insect Technology (IIT), which utilizes sterilization with the maternally inherited endosymbiont Wolbachia, but the accidental release of females infected with the same strain of Wolbachia as the released males could comprise its effectiveness (Zheng et al., 2019). In this scenario, a study conducted in Nanyang, Singapore by Ong et al. (2022) reported that an IIT combined with a sterile insect technique (IIT-SIT) releasing X-ray irradiated Wolbachia-infected male mosquitoes resulted in a 98% reduction in Ae. aegypti populations also showed an 88% reduction in the incidence of dengue fever (Ong et al., 2022).
Likewise, an on-field trial performed in South Miami, United States, by Mains et al. (2019) showed the release of many infected Ae. aegypti males significantly reduced the egg-hatching rate in areas populated by infected males, consistent with the CI expectations. Similarly, the number of Ae. aegypti were significantly reduced in areas where infected males were getting infected compared to untreated areas, reducing the Zika virus burden (Mains et al., 2019). Moreover, the release of sterile or incompatible males resulted in the suppression of both wild-type and wMel-infected Ae.aegypti populations, confirming the utility of bidirectional incompatibility in the field (Beebe et al., 2021) as demonstrated in northern Queensland, Australia by Beebe et al. (2021).
The main limitation of the IIT is releasing infected male mosquitos maternally inherited. To overcome this constraint, a combination strategy between IIT techniques and the Sterile Insect Technique (SIT) was also tested, whereby overwhelming numbers of sterile insects are released into the wild (Zheng et al., 2019; Villegas-Chim et al., 2022). SIT is a control method applied against agricultural pests as well as human disease vector populations, by providing the release of sterile or incompatible males (Werren et al., 2008). According to Iturbe-Ormaetxe et al. (2011), Wolbachia transinfection experiments are more successful when the donor and recipient organisms are closely related.
Likewise, Moreira et al. (2009a,b) and Bian et al. (2010) demonstrated that bacterial infection can occur in different body parts of Ae.aegypti like the midgut, fat body, brain, and salivary gland, with a high prevalence in the reproductive tissues, both ovaries and testicles (Bi and Wang, 2020). Another study conducted in Mexico by Mejia et al. (2022) found relatively greater Wolbachia densities in reproductive tissues than those in the somatic tissues (Mejia et al., 2022). This implies that reproductive parts infection can inhibit the vector fecundity and fertility.
Beyond the reproductive system, the brain also is a target for Wolbachia infection, affecting oviposition and host-seeking behavior. However, this condition does not alter the attraction of mosquitoes to the human odor (Wiwatanaratanabutr et al., 2010; Turley et al., 2014) but rather, the blood-feeding ability by affecting the proboscis’s anatomy (Turley et al., 2009; Moreira et al., 2009a; Bian et al., 2010).
A study by Caragata et al. (2016a) demonstrated that Wolbachia infection can induce diet-nutritional stress in Ae. aegypti reducing vector susceptibility versus Dengue virus and the avian malarial parasite Plasmodium gallinaceum. Similarly, Geoghegan et al. (2017) found that infection alters lipid/cholesterol metabolism including differential cholesterol and lipid profiles (Geoghegan et al., 2017). These findings suggest a possible competition for nutrients between Wolbachia and other pathogens inhibiting replication and shortening vector life span.
According to De Oliveira et al. (2017), Ae. albopictus demonstrated greater competitive ability in a variety of laboratory settings. Its larvae outperformed Ae. aegypti (both infected and uninfected groups) in terms of development and performance index survival rate. Wolbachia boosted the larval survival rate of Ae. aegypti. This finding indicated that larval density greatly impacts the competition for nutrients in infected vectors (De Oliveira et al., 2017).
According to a study conducted by Islam and Dobson (2006) on the impact of Wolbachia on Ae. albopictus, uninfected larvae, had the best survival rate, partly because males infected with wAlbB or wAlbA had lower survival rates. Dutra et al. (2016), recorded similar results at Penn State Brazil and found that wMel infection of Ae. aegypti caused faster larval growth in males and females at greater densities but did not affect females living in less crowded settings (Dutra et al., 2016). While wMelPop infection of Ae. aegypti exhibited highly inhibitory effects of larval food level, the effect of strain alone was not significant, according to a different study conducted in Queensland, Australia by Kho et al. (2016). These differences may be due to bacterial density and host susceptibility. Thus a higher density causes more pronounced effects. For instance, W. pipientis strain wMelPop is known for shortening life spans when inserted into the main dengue vector Ae. aegypti (Thomas et al., 2011; Yeap et al., 2014) but not in Ae. albopictus (Mousson et al., 2010), as demonstrated by Wiwatanaratanabutr et al. (2010).
According to Ross et al. (2017) wMel and wMelPop-CLA infections of Ae. aegypti could not be transmitted to the next generation when mosquitoes were exposed to 26–37°C across all life stages. In contrast, under the same temperature range, an increase in infection density allowed the infection to be inherited from mother to offspring (Ross et al., 2017).
Besides vertical transmission, innate immune priming is also strain and density-dependent. Indeed, epithelial cells that are also involved in regulating innate immune responses to bacteria and parasites produce a significant number of reactive oxygen species and antimicrobial peptides (Ryu et al., 2010; Pircalabioru et al., 2016). In Ae. aegypti, an increased level of reactive oxygen compounds suppresses the replication of West Nile virus (Hussain et al., 2013), Dengue virus (Bian et al., 2010; Frentiu et al., 2014), Chikungunya virus (Aliota et al., 2016a), and Zika virus (Aliota et al., 2016b). Furthermore, it confers resistance to various ribonucleic acid (RNA) viruses and virus-induced death in flies, but it reduces adult vectorial capacity (Mohanty et al., 2016). Replication of West Nile virus is significantly reduced in the presence of Wolbachia by the alteration of GATA4 expression which inhibits virus assembly (Hussain et al., 2013). Those imply that Wolbachia infection has evolutionary, biological, and developmental impacts on mosquito vectors.
On the other hand, Quek et al. (2022) found that in the absence of Wolbachia, microfilariae quickly lose their capacity to develop in the mosquito vector because of their inability to break out of their shells and get through the gut wall. They also showed that the enzyme chitinase, essential for microfilariae to leave their shells, was low in Wolbachia-depleted microfilariae, preventing them from leaving their shells. When chitinase was added to Wolbachia-depleted microfilariae in a lab, they could break out of their shells just as well as the ones that were not treated. So, it looks like Wolbachia has a big role in the transmission of filariasis and suggests that anti-Wolbachia treatment mediates a more accelerated impact on the elimination of lymphatic filariasis (Quek et al., 2022).
2.2. Wolbachia infection in Anopheles mosquitoes
Among all retrieved articles in this review, 13 were on Wolbachia infection of Anopheles species (Supplementary Figure S1 and Supplementary Table S1). There are more than 460 recognized species in the genus Anopheles. An. gambiae and An. funestus are the most significant global malaria vectors (Sinka et al., 2012; Wiebe et al., 2017). Currently, An.stepheni is going to be the main concern for malaria transmission in Africa. The genus Anopheles is most well-known for conveying malaria but also transmits other diseases like filarial worms (Coetzee, 2020; Kientega et al., 2022).
The first recorded on-field infection of Wolbachia in Anopheles species was reported in Burkina Faso by Baldini et al. (2014) using 16S rRNA gene analysis from An. gambiae reproductive tissue. This study also isolated a new strain of Wolbachia, namely wAnga. Similarly, other research conducted in Senegal reported the first Wolbachia on-field infection in another species, namely An.funestus, using the 16S rRNA gene and isolating new strains called wAnfu-A and wAnfu-B (Niang et al., 2018). In 2022 Waymire et al. detected Wolbachia haplotypes in wild Anopheles stephensi in eastern Ethiopia (Waymire et al., 2022). Despite this evidence, according to a phylogenies screening conducted in 2019 by Chrostek and Gerth on Wolbachia 16S rRNA presence in An. gambiae there is no congruence between host and symbiont phylogenies (Chrostek and Gerth, 2019).
2.2.1. Wolbachia infection and parasite development Anopheles species
Once the Anopheles infection is established, the inherent mechanism is similar to the Aedes and Culex species, as shown in Figure 2 (Hughes et al., 2011). However, the role of Wolbachia in inhibiting the malaria parasites in Anopheles mosquitoes is still not well-known (Straub et al., 2020), In vitro trans-infection of An. gambiae with wMelPop and wAlbB strains performed by Hughes et al. (2011) demonstrated the bacteria distributed throughout the fat body, head, sensory organs, and other tissues.
On the other hand, a study conducted in Pennsylvania, United States, by Hughes et al. (2012) found that the wAlbB strain significantly increases P. berghei oocyst levels in the infected An. gambiae midgut while wMelPop modestly suppresses oocyst levels. Another study from East Lansing, United States, by Joshi et al. (2014), reported that wAlbB infections of An. stephensi had reduced female fecundity and caused a minor decrease in male mating competitiveness. Later, Joshi et al. (2017) revealed wAlbB infection in An. stephensi led to a reduction in parasite numbers of up to 92% at the sporozoite stage and more than half at the oocyst stage. This finding implies that wAlbB strain infections can reduce the parasite density depending on the Plasmodium species and vector population. This evidence is in agreement with what was reported by Baldini et al. (2018) on natural Wolbachia infection in the malaria mosquito Anopheles arabiensis in Tanzania.
Another study conducted in Dangassa, Mali, by Gomes et al. (2017) found the Wolbachia infection in the field-collected An. coluzzii was positive for wAnga and revealed a significantly lower prevalence and intensity of P. falciparum sporozoite. Similarly, in Bobo-Dioulasso, Burkina Faso, Shaw et al. (2016) revealed that Wolbachia infections in natural Anopheles populations affect egg laying and negatively correlate with Plasmodium development. Finally, in 2020 Wong et al. reported that Wolbachia infection in An. gambiae is able to reduce the mosquito life span and provide resistance to pathogen infection (Wong et al., 2020).
2.3. Wolbachia infection in Culex species
Among all retrieved articles in this review, 11 have as subjects the epidemiology and infection of Culex species (Supplementary Table S1). The genus Culex has several species; however, Cx. pipiens and Cx. quinquefasciatus, reviewed in the selected studies, are vectors for various human diseases, such as arbovirus diseases like the West Nile virus, Japanese encephalitis, and filariasis (Harbach, 1985; Omar, 1996; Paramasivan et al., 2003).
The first Wolbachia infection in mosquitoes was reported from Cx. pipiens reproductive tissues by Hertig and Wolbach (1924). Later on, different studies showed that the prevalence of Wolbachia in this species ranges from 65% to 100% in field-collected females and nearly 100% in males (Karami et al., 2016; Bergman and Hesson, 2021).
2.3.1. Wolbachia infection and its effect on the Culex species
The mode of infection and its effect on Culex physiology is similar to Aedes. However, Hague et al. (2020) demonstrated that the infected host raises temperature preference. In contrast to the uninfected, most hosts infected with Wolbachia supergroup A prefer cooler temperatures than uninfected ones, On the other hand, supergroup B infected hosts prefer warmer temperatures (Hague et al., 2020). These findings suggest that Wolbachia infection-inducing host behavior’s alterations facilitate bacterial replication and disease spread (Moreira et al., 2009b; Glaser and Meola, 2010; Caragata et al., 2016b). Interestingly similar evidence has not been reported for Aedes and Anopheles.
According to Atyame et al. (2011) a considerable amount of Wolbachia diversity can be generated within a single host species in a short time, and playing a key role in their evolution. Furthermore, a recent study by Zhang et al. (2020) clarified the immune system’s role in Cx. pipiens infection, according to the author the competition for scarce nutrients may not be the primary cause of Wolbachia-mediated pathogen suppression, as evidenced by the fact that the presence of Wolbachia per se does not always alter pathogen infections. Instead, it is brought on by host immunological reactions (Zhang et al., 2020).
In vitro insecticide susceptibility studies by Berticat et al. (2002) and Duron et al. (2006) showed that the symbiotic maternally inherited Wolbachia affected Cx. quinquefasciatus and Cx. pipiens insecticide resistance depending on infection density and the type of insecticide used (Berticat et al., 2002; Duron et al., 2006). Therefore, a medium-density infection synergises with deltamethrin and other organophosphates, but not with Dichloro-diphenyl-trichloroethane (DDT) (Berticat et al., 2002; Duron et al., 2006; Shemshadian et al., 2021). Likewise, Echaubard et al. (2010) also reported that a medium-density infection in Cx. pipiens made the vector insecticide-susceptible whereas with a higher density infection caused insecticide-resistance. These results may partially explain the presence of high-density infections in pesticide-resistant mosquitoes in the field (Echaubard et al., 2010).
Similarly, in vitro infection with wPipSJ made Cx. quinquefasciatus less susceptible to entomopathogenic bacteria as demonstrated by Díaz-Nieto et al. (2021). These findings agree with on-field records, where Cx. quinquefasciatus infected by wPipSJ are more resistant to Bacillus wiedmannii var. thuringiensis, and B. thuringiensis subsp. israelensis, and Lysinibacillus sphaericus bacterial infections (Díaz-Nieto et al., 2021).
3. Discussion
Integrated Mosquito Management (IMM) strategies are currently the best option for reducing mosquito populations (CDC, 2020). This implementation is based on understanding mosquito biology, ecology, and mosquito pathogen interaction. Indeed, IMM programs employ several strategies, together with insecticides, such as larval breeding source reduction through community participation and biological control techniques like predatory fish and symbiotic bacteria (Dodson et al., 2017; CDC, 2020).
Currently, scientific evidence has underscored the appropriate use of the symbiotic bacteria W. pipientis as a new weapon in the fight against mosquitoes as vector-borne diseases. Compared to insecticide-based methods, it has the advantage of potentially being more cost-effective and environmentally friendly (Iturbe-Ormaetxe et al., 2011). In addition, Wolbachia infection density was positively correlated with insecticides, making this management strategy even more attractive (Berticat et al., 2002; Duron et al., 2006; Shemshadian et al., 2021). These suggest that reducing vector population and other pathogen replication in the host also increases the vectors’ susceptibility to different insecticides.
When considering Wolbachia infection as a pathogen for inhibition and population reduction, factors such as strain, density, distribution, and infection frequency must be considered (Bian et al., 2010). The mechanism of Wolbachia infection to protect the host from pathogens is immune priming, in which symbiotic infection upregulates basal immune responses and primes insect defenses against subsequent pathogen infections (Ye et al., 2013). However, Hughes et al. (2012) reported that the wAlbB strain significantly increases P. berghei oocyst levels in the infected An. gambiae. These various effects imply that Wolbachia strains differ in their interactions with the host and/or pathogen, and these variations may be used to elucidate the molecular processes that prevent pathogen development in mosquitoes.
In addition to strain and density, the distribution of bacterial infections within the mosquito’s body also significantly impacts mosquito population decline. Infection of the reproductive tract causes host reproductive failure due to CI (Li et al., 2023). Reproductively infected mosquitoes cannot produce viable offspring or transmit the bacteria to their offspring (Sinkins, 2004; O’Neill, 2018). On this basis, releasing Wolbachia-infected male mosquitoes into the field decreased the fecundity and the fertility of wild mosquito populations. Wolbachia Incompatible Insect Technology (IIT) performing this strategy has proven to be a promising method for eliminating invasive mosquito populations such as Ae. aegypti and Ae. albopictus and reducing the incidence of vector-borne diseases such as dengue, chikungunya, and Zika (Pagendam et al., 2020).
To enhance the effect of population reduction of mosquitoes in the human community, IIT can be combined with radiation-based SIT, which is rearing, sterilization, and release of large numbers of male mosquitoes to mate with fertile wild females, thereby reducing offspring production from the target population (Zheng et al., 2019; Chen et al., 2023). This further reinforces the dependence on strain type and density in infection vertical transmission.
Even though the release of Wolbachia-carrying mosquitoes into communities is not immediately stopping the epidemic, it leads to mosquito population declines over several months (Iturbe-Ormaetxe et al., 2011; Liew et al., 2021). These imply that Wolbachia influences the transmission effect when mosquitoes are exposed for an extended time to obtain the capacity of Wolbachia strains to infiltrate the uninfected mosquito population in the community.
Besides, before and during the implementation of releases of Wolbachia-infected mosquitoes for mosquito population suppression or replacement. It is important to keep engaging with the community and educating them to increase their understanding of this method, including clear and specific health risk assessment information (Sánchez-González et al., 2021). In addition to maintaining community support, programs should evaluate and monitor to determine how well they reduce the mosquito population (Sánchez-González et al., 2021; Villegas-Chim et al., 2022). Household perception surveys in different areas of Singapore provided a good understanding of public acceptance and sentiments toward using Wolbachia-Aedes technology (Liew et al., 2021). In addition, Texas and California in the United States, Thailand, Mexico, and Australia have released Wolbachia-infected mosquitoes and reported a significant drop in Ae. aegypti mosquitoes to control dengue, chikungunya, and Zika also gaining acceptance in the community (Wiwatanaratanabutr et al., 2010; Torres et al., 2020; Villegas-Chim et al., 2022).
The main difficulty with using Wolbachia for controlling vectors in the community is that the main vectors, like Ae.aegypti and Anopheles species, are not usually naturally infected. Trans-infection in the laboratory is necessary to ensure the bacterium is stably transmitted in these vector populations. In addition, culturing obligate intracellular bacteria is a challenge. Insect cells support Wolbachia growth, but culturing is long and difficult to manipulate cells. Modified Eagle’s Minimum Essential Medium, Schneider’s Insect Medium, Mitsuhashi-Maramorosch Insect Medium, and their one-to-one combinations are tested and effective for Wolbachia culture (Angeloni, 2021). Moreover, experts must transfer Wolbachia’s strain into a new host once it grows in the cell culture.
4. Conclusion
Different Wolbachia species and strains have been isolated at different times. These different Wolbachia species and strains commonly infect and affect mosquito species differently. Once Wolbachia-infected mosquitoes release, they may reduce or prevent disease transmission through two mechanisms: (1) by reducing mosquito population density and/or survival rate; (2) by reducing the ability of mosquitoes to transmit diseases and/or pathogen replication or development. It causes the hosts’ CI, phenotypic changes, and nutritional competition with other pathogens. These triggers reduce adult survivorship, inhibit mosquito reproduction, and prevent pathogen replication or development. Wolbachia infection from mosquitoes also sensitizes status to insecticides. Accordingly, Wolbachia can be used for biological control of mosquito-borne diseases, a public health problem in the tropical and sub-tropical world and some developed countries. Wolbachia reduces infection and transmission of diseases such as malaria, filariasis, dengue, chikungunya, yellow fever, zika, and West Nile fever.
Author contributions
AM: Conceptualization, Formal analysis, Writing – original draft, Writing – review & editing, Investigation, Validation, Visualization. GPP: Validation, Visualization, Writing – review & editing, Investigation, Supervision. DY: Validation, Writing – review & editing, Supervision. AS: Supervision, Validation, Writing – review & editing. IM: Writing – review & editing, Validation. DN: Validation, Writing – review & editing. RM: Supervision, Validation, Writing – review & editing. GA: Supervision, Validation, Writing – review & editing, Conceptualization, Data curation.
Funding
The author(s) declare financial support was received for the research, authorship, and/or publication of this article.
Conflict of interest
The authors declare that the research was conducted in the absence of any commercial or financial relationships that could be construed as a potential conflict of interest.
Publisher’s note
All claims expressed in this article are solely those of the authors and do not necessarily represent those of their affiliated organizations, or those of the publisher, the editors and the reviewers. Any product that may be evaluated in this article, or claim that may be made by its manufacturer, is not guaranteed or endorsed by the publisher.
Supplementary material
The Supplementary material for this article can be found online at: https://www.frontiersin.org/articles/10.3389/fmicb.2023.1267832/full#supplementary-material
Abbreviations
AMP, Antimicrobial peptides; CHIKV, Chikungunya virus; CI, Cytoplasmic incompatibility; DDT, Dichlorodiphenyltrichloroethane; DENV, Dengue Virus; IIT, Incompatible insect technique; IMM, Integrated mosquito management; NO, Nitric oxide; PCR, Polymerized chain reaction; RNA, Ribonucleic acid; ROS, Reactive oxygen species; SIT, Sterile insect technique.
References
Ahmed, M., Li, S., Xue, X., Yin, X., Ren, S., Jiggins, F., et al. (2015). The intracellular bacterium Wolbachia uses parasitoid wasps as phoretic vectors for efficient horizontal transmission. PLoS Pathog. 11:e1004672. doi: 10.1371/journal.ppat.1004672
Aliota, M., Peinado, S., Velez, I., and Osorio, J. (2016b). The wMel strain of Wolbachia reduces transmission of Zika virus by Aedes aegypti. Sci. Rep. 6:28792. doi: 10.1038/srep28792
Aliota, M., Walker, E., Yepes, U., Dario Velez, I., Christensen, B., and Osorio, J. (2016a). The wMel strain of Wolbachia reduces transmission of chikungunya virus in Aedes aegypt. PLoS Negl. Trop. Dis. 10:e0004677. doi: 10.1371/journal.pntd.0004677
Allman, M., Fraser, J., Ritchie, S., Joubert, D., Simmons, C., and Flores, H. (2020). Wolbachia’s deleterious impact on Aedes aegypti egg development: the potential role of nutritional parasitism. Insects 11:735. doi: 10.3390/insects11110735
Angeloni, S. K. (2021). Optimization of Wolbachia culture conditions and purification of Wolbachia. California State University. Available at: http://hdl.handle.net/20.500.12680/fq978086r
Atyame, C. M., Delsuc, F., Pasteur, N., Weill, M., and Duron, O. (2011). Diversification of Wolbachia endosymbiont in the Culex pipiens mosquito. Mol. Biol. Evol. 28, 2761–2772. doi: 10.1093/molbev/msr083
Baldini, F., Rougé, J., Kreppel, K., Mkandawile, G., Mapua, S. A., Sikulu-Lord, M., et al. (2018). First report of natural Wolbachia infection in the malaria mosquito Anopheles arabiensis in Tanzania. Parasit. Vectors 11, 1–7. doi: 10.1186/s13071-018-3249-y
Baldini, F., Segata, N., Pompon, J., Marcenac, P., Robert Shaw, W., Dabiré, R., et al. (2014). Evidence of natural Wolbachia infections in field populations of Anopheles gambiae. Nat. Commun. 5:3985. doi: 10.1038/ncomms4985
Beebe, N., Pagendam, D., Trewin, B., Boomer, A., Bradford, M., Ford, A., et al. (2021). Releasing incompatible males drives strong suppression across populations of wild and Wolbachia-carrying Aedes aegypti in Australia. Proc. Natl. Acad. Sci. 118:e2106828118. doi: 10.1073/pnas.2106828118
Bergman, A., and Hesson, J. (2021). Wolbachia prevalence in the vector species Culex pipiens and Culex torrentium in a Sindbis virus-endemic region of Sweden. Parasit. Vectors 14:428. doi: 10.1186/s13071-021-04937-6
Berticat, C., Rousset, F., Raymond, M., Berthomieu, A., and Weill, M. (2002). High Wolbachia density in insecticide–resistant mosquitoes. Proc. R. Soc. Lond. Ser. B Biol. Sci. 269, 1413–1416. doi: 10.1098/rspb.2002.2022
Bettis, A. A., L’Azou Jackson, M., Yoon, I.-K., Breugelmans, J. G., Goios, A., Gubler, D. J., et al. (2022). The global epidemiology of chikungunya from 1999 to 2020: a systematic literature review to inform the development and introduction of vaccines. PLoS Negl. Trop. Dis. 16:e0010069. doi: 10.1371/journal.pntd.0010069
Bi, J., and Wang, Y. (2020). The effect of the endosymbiont Wolbachia on the behavior of insect hosts. Insect Sci. 27, 846–858. doi: 10.1111/1744-7917.12731
Bian, G., Xu, Y., Lu, P., Xie, Y., and Xi, Z. (2010). The endosymbiotic bacterium Wolbachia induces resistance to dengue virus in Aedes aegypti. PLoS Pathog. 6:e1000833. doi: 10.1371/journal.ppat.1000833
Bizhani, N., Hashemi Hafshejani, S., Mohammadi, N., Rezaei, M., and Rokni, M. B. (2021). Lymphatic filariasis in Asia: a systematic review and meta-analysis. Parasitol. Res. 120, 411–422. doi: 10.1007/s00436-020-06991-y
Bordenstein, S., Paraskevopoulos, C., Dunning Hotopp, J., Sapountzis, P., Lo, N. B. C., Bandi, C., et al. (2009). Parasitism and mutualism in Wolbachia: what the phylogenomic trees can and cannot say. Mol. Biol. Evol. 26, 231–241. doi: 10.1093/molbev/msn243
Brasil, P., Calvet, G., Siqueira, A., Wakimoto, M., de Sequeira, P. C., Nobre, A., et al. (2016). Zika virus outbreak in Rio de Janeiro, Brazil: clinical characterization, epidemiological and virological aspects. PLoS Negl. Trop. Dis. 10:e0004636. doi: 10.1371/journal.pntd.0004636
Brelsfoard, C., and Dobson, S. (2011). Wolbachia effects on host fitness and the influence of male aging on cytoplasmic incompatibility in Aedes polynesiensis (Diptera: Culicidae). J. Med. Entomol. 48, 1008–1015. doi: 10.1603/ME10202
Calvitti, M., Moretti, R., Lampazzi, E., Bellini, R., and Dobson, S. L. (2014). Characterization of a new Aedes albopictus (Diptera: Culicidae)-Wolbachia pipientis (Rickettsiales: Rickettsiaceae) symbiotic association generated by the artificial transfer of the w pip strain from Culex pipiens (Diptera: Culicidae). J. Med. Entomol. 47, 179–187. doi: 10.1603/me09140
Caragata, E., Dutra, H., and Moreira, L. (2016b). Exploiting intimate relationships: controlling mosquito-transmitted disease with Wolbachia. Trends Parasitol. 32, 207–218. doi: 10.1016/j.pt.2015.10.011
Caragata, E., Dutra, H., Sucupira, P., Ferreira, A., and Moreira, L. (2021). Wolbachia as translational science: controlling mosquito-borne pathogens. Trends Parasitol. 37, 1050–1067. doi: 10.1016/j.pt.2021.06.007
Caragata, E., Rezende, F., Simões, T., and Moreira, L. (2016a). Diet-induced nutritional stress and pathogen interference in Wolbachia-infected Aedes aegypti. PLoS Negl. Trop. Dis. 10:e0005158. doi: 10.1371/journal.pntd.0005158
Carrington, L. B., Lipkowitz, J. R., Hoffmann, A. A., and Turelli, M. (2011). A re-examination of Wolbachia-induced cytoplasmic incompatibility in California Drosophila simulans. PLoS One 6:e22565. doi: 10.1371/journal.pone.0022565
CDC. (2020). CENTR for diseases control and prevention 2020. Integrated Mosquito Management. Available at: https://www.cdc.gov/mosquitoes/mosquitocontrol/professionals/integrated-mosquito
CDC. (2023). Centers of diseases control and preventions 2023. West Nile Virus. Available at: https://www.cdc.gov/westnile/index.html
Charlat, S., Calmet, C., and Merçot, H. (2001). On the mod resc model and the evolution of Wolbachia compatibility types. Genetics 159, 1415–1422. doi: 10.1093/genetics/159.4.1415
Chen, C., Aldridge, R., Gibson, S., Kline, J., Aryaprema, V., Qualls, W., et al. (2023). Developing the radiation-based sterile insect technique (SIT) for controlling Aedes aegypti: identification of a sterilizing dose. Pest Manag. Sci. 79, 1175–1183. doi: 10.1002/ps.7303
Chrostek, E., and Gerth, M. (2019). Is Anopheles gambiae a natural host of Wolbachia? MBio 10, 10–1128. doi: 10.1128/mBio.00784-19
Coetzee, M. (2020). Key to the females of Afrotropical Anopheles mosquitoes (Diptera: Culicidae). Malar. J. 19, 1–20. doi: 10.1186/s12936-020-3144-9
Couper, L., Farner, J., Caldwell, J., Childs, M., Harris, M., Kirk, D., et al. (2021). How will mosquitoes adapt to climate warming? elife 10:e69630. doi: 10.7554/eLife.69630
Damiani, C., Cappelli, A., Comandatore, F., Montarsi, F., Serrao, A., Michelutti, A., et al. (2022). Wolbachia in Aedes koreicus: rare detections and possible implications. Insects 13:216. doi: 10.3390/insects13020216
De Oliveira, S., Villela, D., Dias, F., Moreira, L., and Maciel De Freitas, R. (2017). How does competition among wild type mosquitoes influence the performance of Aedes aegypti and dissemination of Wolbachia pipientis? PLoS Negl. Trop. Dis. 11:e0005947. doi: 10.1371/journal.pntd.0005947
Díaz-Nieto, L., Gil, M., Lazarte, J., Perotti, M., and Berón, C. (2021). Culex quinquefasciatus carrying Wolbachia is less susceptible to entomopathogenic bacteria. Sci. Rep. 11, 1–9. doi: 10.1038/s41598-020-80034-5
Ding, H., Yeo, H., and Puniamoorthy, N. (2020). Wolbachia infection in wild mosquitoes (Diptera: Culicidae): implications for transmission modes and host-endosymbiont associations in Singapore. Parasit. Vectors 13, 1–6. doi: 10.1186/s13071-020-04466-8
Dodson, B., Andrews, E., Turell, M., and Rasgon, J. (2017). Wolbachia effects on Rift Valley fever virus infection in Culex tarsalis mosquitoes. PLoS Negl. Trop. Dis. 11:e0006050. doi: 10.1371/journal.pntd.0006050
Dorigatti, I., Mccormack, C., Nedjati-Gilani, G., and Ferguson, N. (2018). Using Wolbachia for dengue control: insights from modelling. Trends Parasitol. 34, 102–113. doi: 10.1016/j.pt.2017.11.002
Duron, O., Labbé, P., Berticat, C., Rousset, F., Guillot, S., Raymond, M., et al. (2006). High Wolbachia density correlates with cost of infection for insecticide resistant Culex pipiens mosquitoes. Evolution 60, 303–314. doi: 10.1111/j.0014-3820.2006.tb01108.x
Duron, O., Noël, V., McCoy, K. D., Bonazzi, M., Sidi-Boumedine, K., Morel, O., et al. (2015). The recent evolution of a maternally-inherited endosymbiont of ticks led to the emergence of the Q fever pathogen, Coxiella burnetii. PLoS Pathog. 11:e1004892. doi: 10.1371/journal.ppat.1004892
Dutra, H., Lopes Da Silva, V., Da Rocha Fernandes, M., Logullo, C., Maciel-De-Freitas, R., and Moreira, L. (2016). The influence of larval competition on Brazilian Wolbachia-infected Aedes aegypti mosquitoes. Parasit. Vectors 9, 1–5. doi: 10.1186/s13071-016-1559-5
Echaubard, P., Duron, O., Agnew, P., Sidobre, C., Noël, V., Weill, M., et al. (2010). Rapid evolution of Wolbachia density in insecticide resistant Culex pipiens. Heredity 104, 15–19. doi: 10.1038/hdy.2009.100
Frentiu, F., Zakir, T., Walker, T., Popovici, J., Pyke, A., van den Hurk, A., et al. (2014). Limited dengue virus replication in field-collected Aedes aegypti mosquitoes infected with Wolbachia. PLoS Negl. Trop. Dis. 8:e2688. doi: 10.1371/journal.pntd.0002688
Geoghegan, V., Stainton, K., Rainey, S. M., Ant, T. H., Dowle, A. A., Larson, T., et al. (2017). Perturbed cholesterol and vesicular trafficking associated with dengue blocking in Wolbachia-infected Aedes aegypti cells. Nat. Commun. 8:526. doi: 10.1038/s41467-017-00610-8
Glaser, R., and Meola, M. (2010). The native Wolbachia endosymbionts of Drosophila melanogaster and Culex quinquefasciatus increase host resistance to West Nile virus infection. PLoS One 5:e11977. doi: 10.1371/journal.pone.0011977
Gomes, F., Hixson, B., Tyner, M., Ramirez, J., Canepa, G., Alves e Silva, T. L., et al. (2017). Effect of naturally occurring Wolbachia in Anopheles gambiae sl mosquitoes from Mali on Plasmodium falciparum malaria transmission. Proc. Natl. Acad. Sci. 114, 12566–12571. doi: 10.1073/pnas.1716181114
Gutzwiller, F., Carmo, C., Miller, D., Rice, D., Newton, I., Hawley, R., et al. (2015). Dynamics of Wolbachia pipientis gene expression across the Drosophila melanogaster life cycle. G3 5, 2843–2856. doi: 10.1534/g3.115.021931
Hague, M., Caldwell, C., and Cooper, B. (2020). Pervasive effects of Wolbachia on host temperature preference. MBio 11, e01768–e01720. doi: 10.1128/mBio.01768-20
Harbach, R. (1985). Pictorial keys to the genera of mosquitoes, subgenera of Culex and the species of Culex occurring in southwestern Asia and Egypt, with a note on the subgeneric placement of Culex deserticola (Diptera: Culicidae). Mosq. Syst. 17, 83–107.
Hedges, L., Brownlie, J., O'Neill, S., and Johnson, K. (2008). Wolbachia and virus protection in insects. Science 322:702. doi: 10.1126/science.1162418
Hertig, M. (1936). The rickettsia, Wolbachia pipientis and associated inclusions of the mosquito, Culex pipiens. Parasitology 28, 453–486. doi: 10.1017/S0031182000022666
Hertig, M., and Wolbach, S. (1924). Studies on Rickettsia-like micro-organisms in insects. J. Med. Res. 44, 329–374.7.
Hughes, G., Koga, R., Xue, P., Fukatsu, T., and Rasgon, J. (2011). Wolbachia infections are virulent and inhibit the human malaria parasite Plasmodium falciparum in Anopheles gambiae. PLoS Pathog. 7:e1002043. doi: 10.1371/journal.ppat.1002043
Hughes, G., Vega-Rodriguez, J., Xue, P., and Rasgon, J. (2012). Wolbachia strain wAlbB enhances infection by the rodent malaria parasite Plasmodium berghei in Anopheles gambiae mosquitoes. Appl. Environ. Microbiol. 78, 1491–1495. doi: 10.1128/AEM.06751-11
Hussain, M., Lu, G., Torres, S., Edmonds, J. H., Kay, B. H., Khromykh, A. A., et al. (2013). Effect of Wolbachia on replication of West Nile virus in a mosquito cell line and adult mosquitoes. J. Virol. 87, 851–858. doi: 10.1128/JVI.01837-12
Inácio Da Silva, L., Dezordi, F., Paiva, M., and Wallau, G. (2021). Systematic review of Wolbachia symbiont detection in mosquitoes: an entangled topic about methodological power and true symbiosis. Pathogens 10:39. doi: 10.3390/pathogens10010039
Islam, M., and Dobson, S. (2006). Wolbachia effects on Aedes albopictus (Diptera: Culicidae) immature survivorship and development. J. Med. Entomol. 43, 689–695. doi: 10.1603/0022-2585(2006)43[689:WEOAAD]2.0.CO;2
Iturbe-Ormaetxe, I., Walker, T., and O'neill, S. (2011). Wolbachia and the biological control of mosquito-borne disease. EMBO Rep. 12, 508–518. doi: 10.1038/embor.2011.84
Jeffries, C. L., and Walker, T. (2015). The potential use of Wolbachia-based mosquito biocontrol strategies for Japanese encephalitis. PLoS Negl. Trop. Dis. 9:e0003576. doi: 10.1371/journal.pntd.0003576
Joshi, D., Mcfadden, M., Bevins, D., Zhang, F., and Xi, Z. (2014). Wolbachia strain wAlbB confers both fitness costs and benefit on Anopheles stephensi. Parasit. Vectors 7, 1–9. doi: 10.1186/1756-3305-7-336
Joshi, D., Pan, X., McFadden, M. J., Bevins, D., Liang, X., Lu, P., et al. (2017). The maternally inheritable Wolbachia wAlbB induces refractoriness to Plasmodium berghei in Anopheles stephensi. Front. Microbiol. 8:366. doi: 10.3389/fmicb.2017.00366
Kambris, Z., Blagborough, A., Pinto, S., Blagrove, M., Godfray, H. C., Sinden, R. E., et al. (2010). Wolbachia stimulates immune gene expression and inhibits Plasmodium development in Anopheles gambiae. PLoS Pathog. 6:e1001143. doi: 10.1371/journal.ppat.1001143
Kambris, Z., Cook, P., Phuc, H., and Sinkins, S. (2009). Immune activation by life-shortening Wolbachia and reduced filarial competence in mosquitoes. Science 326, 134–136. doi: 10.1126/science.1177531
Kamtchum-Tatuene, J., Makepeace, B. L., Benjamin, L., Baylis, M., and Solomon, T. (2017). The potential role of Wolbachia in controlling the transmission of emerging human arboviral infections. Curr. Opin. Infect. Dis. 30, 108–116. doi: 10.1097/QCO.0000000000000342
Karami, M., Moosa-Kazemi, S., Oshaghi, M., Vatandoost, H., Sedaghat, M., Rajabnia, R., et al. (2016). Wolbachia endobacteria in natural populations of Culex pipiens of Iran and its phylogenetic congruence. J. Arthropod. Borne Dis. 10, 347–363.
Kaur, R., Shropshire, J., Cross, K., Leigh, B., Mansueto, A., Stewart, V., et al. (2021). Living in the endosymbiotic world of Wolbachia: a centennial review. Cell Host Microbe 29, 879–893. doi: 10.1016/j.chom.2021.03.006
Kho, E., Hugo, L., Lu, G., Smith, D., and Kay, B. (2016). Effects of larval nutrition on Wolbachia-based dengue virus interference in Aedes aegypti (Diptera: Culicidae). J. Med. Entomol. 53, 894–901. doi: 10.1093/jme/tjw029
Kientega, M., Kranjc, N., Traoré, N., Kaboré, H., Soma, D., Morianou, I., et al. (2022). Analysis of the genetic variation of the fruitless gene within the Anopheles gambiae (Diptera: Culicidae) complex populations in Africa. Insects 13:1048. doi: 10.3390/insects13111048
Kittayapong, P., Baimai, V., and O'neill, S. (2002). Field prevalence of Wolbachia in the mosquito vector Aedes albopictus. Am. J. Trop. Med. Hyg. 66, 108–111. doi: 10.4269/ajtmh.2002.66.108
Kittayapong, P., Baisley, K., Baimai, V., and O’Neill, S. (2000). Distribution and diversity of Wolbachia infections in Southeast Asian mosquitoes (Diptera: Culicidae). J. Med. Entomol. 37, 340–345. doi: 10.1093/jmedent/37.3.340
Landmann, F. (2019). The Wolbachia endosymbionts. Microbiol. Spectr. 7:7-2. doi: 10.1128/microbiolspec.BAI-0018-2019
Leta, S., Beyene, T., De Clercq, E., Amenu, K., Kraemer, M., and Revie, C. (2018). Global risk mapping for major diseases transmitted by Aedes aegypti and Aedes albopictus. Int. J. Infect. Dis. 67, 25–35. doi: 10.1016/j.ijid.2017.11.026
Li, Y., Sun, Y., Zou, J., Zhong, D., Liu, R., Zhu, C., et al. (2023). Characterizing the Wolbachia infection in field-collected Culicidae mosquitoes from Hainan Province, China. Parasit. Vectors 16, 1–2. doi: 10.1186/s13071-023-05719-y
Liew, C., Soh, L., Chen, I., and Ng, L. (2021). Public sentiments towards the use of Wolbachia-Aedes technology in Singapore. BMC Public Health 21, 1–2. doi: 10.1186/s12889-021-11380-w
Mains, J., Kelly, P., Dobson, K., Petrie, W., and Dobson, S. (2019). Localized control of Aedes aegypti (Diptera: Culicidae) in Miami, FL, via inundative releases of Wolbachia-infected male mosquitoes. J. Med. Entomol. 56, 1296–1303. doi: 10.1093/jme/tjz051
McMeniman, C. J., Lane, R., Cass, B., Fong, A., Sidhu, M., Wang, Y., et al. (2009). Stable introduction of a life-shortening Wolbachia infection into the mosquito Aedes aegypti. Science 323, 141–144. doi: 10.1126/science.1165326
Mejia, A., Dutra, H., Jones, M., Perera, R., and Mcgraw, E. (2022). Cross-tissue and generation predictability of relative Wolbachia densities in the mosquito Aedes aegypti. Parasit. Vectors 15, 1–10. doi: 10.1186/s13071-022-05231-9
Mohanty, I., Rath, A., Mahapatra, N., and Hazra, R. (2016). Wolbachia: a biological control strategy against arboviral diseases. J. Vector Borne Dis. 53, 199–207.
Moreira, L. A., Iturbe-Ormaetxe, I., Jeffery, J. A., Lu, G., Pyke, A. T., Hedges, L. M., et al. (2009a). A Wolbachia symbiont in Aedes aegypti limits infection with dengue, chikungunya, and plasmodium. Cells 139, 1268–1278. doi: 10.1016/j.cell.2009.11.042
Moreira, L., Saig, E., Turley, A., Ribeiro, J., O'neill, S., and Mcgraw, E. (2009b). Human probing behavior of Aedes aegypti when infected with a life-shortening strain of Wolbachia. PLoS Negl. Trop. Dis. 3:e568. doi: 10.1371/journal.pntd.0000568
Moretti, R., Yen, P., Houé, V., Lampazzi, E., Desiderio, A., Failloux, A., et al. (2018). Combining Wolbachia-induced sterility and virus protection to fight Aedes albopictus-borne viruses. PLoS Negl. Trop. Dis. 12:e0006626. doi: 10.1371/journal.pntd.0006626
Mousson, L., Martin, E., Zouache, K., Madec, Y., Mavingui, P., and Failloux, A. (2010). Wolbachia modulates chikungunya replication in Aedes albopictus. Mol. Ecol. 19, 1953–1964. doi: 10.1111/j.1365-294X.2010.04606.x
Niang, E., Bassene, H., Makoundou, P., Fenollar, F., Weill, M., and Mediannikov, O. (2018). First report of natural Wolbachia infection in wild Anopheles funestus population in Senegal. Malar. J. 17, 1–6. doi: 10.1186/s12936-018-2559-z
O’Neill, S. (2018). The use of Wolbachia by the world mosquito program to interrupt transmission of Aedes aegypti transmitted viruses. Adv. Exp. Med. Biol. 1062, 355–360. doi: 10.1007/978-981-10-8727-1_24
Omar, M. (1996). A survey of bancroftian filariasis among south-east Asian expatriate workers in Saudi Arabia. Tropical Med. Int. Health 1, 155–160. doi: 10.1111/j.1365-3156.1996.tb00021.x
Ong, J., Ho, S., Soh, S., Wong, Y., Ng, Y., Vasquez, K., et al. (2022). Assessing the efficacy of male Wolbachia-infected mosquito deployments to reduce dengue incidence in Singapore: study protocol for a cluster-randomized controlled trial. Trials 23:1023. doi: 10.1186/s13063-022-06976-5
Osei-Poku, J., Han, C., Mbogo, C., and Jiggins, F. (2012). Identification of Wolbachia strains in mosquito disease vectors. PLoS One 7:e49922. doi: 10.1371/journal.pone.0049922
Pagendam, D., Trewin, B., Snoad, N., Ritchie, S., Hoffmann, A., Staunton, K., et al. (2020). Modelling the Wolbachia incompatible insect technique: strategies for effective mosquito population elimination. BMC Biol. 18:161. doi: 10.1186/s12915-020-00887-0
Paramasivan, R., Mishra, A., and Mourya, D. (2003). West Nile virus: the Indian scenario. Indian J. Med. Res. 118:108.
Philip, C. (1956). Comments on the classification of the order Rickettsiales. Can. J. Microbiol. 2, 261–270. doi: 10.1139/m56-030
Pircalabioru, G., Aviello, G., Kubica, M., Zhdanov, A., Paclet, M., Brennan, L., et al. (2016). Defensive mutualism rescues NADPH oxidase inactivation in gut infection. Cell Host Microbe 19, 651–663. doi: 10.1016/j.chom.2016.04.007
Poinsot, D., Charlat, S., and Mercot, H. (2003). On the mechanism of Wolbachia-induced cytoplasmic incompatibility: confronting the models with the facts. BioEssays 25, 259–265. doi: 10.1002/bies.10234
Puggioli, A., Calvitti, M., Moretti, R., and Bellini, R. (2016). wPip Wolbachia contribution to Aedes albopictus SIT performance: advantages under intensive rearing. Acta Trop. 164, 473–481. doi: 10.1016/j.actatropica.2016.10.014
Quek, S., Cook, D., Wu, Y., Marriott, A., Steven, A., Johnston, K., et al. (2022). Wolbachia depletion blocks the transmission of lymphatic filariasis by preventing chitinase dependent parasite escheatment. Proc. Natl. Acad. Sci. 119:e2120003119. doi: 10.1073/pnas.2120003119
Rogers, K. (2023). Aedes. Encyclopedia Britannica. Available at: https://www.britannica.com/animal/Aedes.
Rohrscheib, C., Frentiu, F., Horn, E., Ritchie, F., van Swinderen, B., Weible, M., et al. (2016). Intensity of mutualism breakdown is determined by temperature, not amplification of Wolbachia genes. PLoS Pathog. 12:e1005888. doi: 10.1371/journal.ppat.1005888
Ross, P., Wiwatanaratanabutr, I., Axford, J., White, V., Endersby-Harshman, N., and Hoffmann, A. (2017). Wolbachia infections in Aedes aegypti differ markedly in their response to cyclical heat stress. PLoS Pathog. 13:e1006006. doi: 10.1371/journal.ppat.1006006
Ryu, J., Ha, E., and Lee, W. (2010). Innate immunity and gut–microbe mutualism in Drosophila. Dev. Comp. Immunol. 34, 369–376. doi: 10.1016/j.dci.2009.11.010
Salzberg, S., Hotopp, J., Delcher, A., Pop, M., Smith, D., Eisen, M., et al. (2005). Serendipitous discovery of Wolbachia genomes in multiple Drosophila species. Genome Biol. 6, 1–8. doi: 10.1186/gb-2005-6-3-r2
Sánchez-González, L., Adams, L., Saavedra, R., Little, E., Medina, N., Major, C., et al. (2021). Assessment of community support for Wolbachia-mediated population suppression as a control method for Aedes aegypti mosquitoes in a community cohort in Puerto Rico. PLoS Negl. Trop. Dis. 15:e0009966. doi: 10.1371/journal.pntd.0009966
Serbus, L., Casper-Lindley, C., Landmann, F., and Sullivan, W. (2008). The genetics and cell biology of Wolbachia-host interactions. Annu. Rev. Genet. 42, 683–707. doi: 10.1146/annurev.genet.41.110306.130354
Shaw, W., Marcenac, P., Childs, L., Buckee, C., Baldini, F., Sawadogo, S., et al. (2016). Wolbachia infections in natural Anopheles populations affect egg laying and negatively correlate with Plasmodium development. Nat. Commun. 7:11772. doi: 10.1038/ncomms11772
Shemshadian, A., Vatandoost, H., Oshaghi, M., Abai, M., and Djadid, N. (2021). Relationship between Wolbachia infection in Culex quinquefasciatus and its resistance to insecticide. Heliyon 7:e06749. doi: 10.1016/j.heliyon.2021.e06749
Sicard, M., Bonneau, M., and Weill, M. (2019). Wolbachia prevalence, diversity, and ability to induce cytoplasmic incompatibility in mosquitoes. Curr. Opin. Insect Sci. 34, 12–20. doi: 10.1016/j.cois.2019.02.005
Silva, J., Magalhães Alves, D., Bottino-Rojas, V., Pereira, T., Sorgine, M., Caragata, E., et al. (2017). Wolbachia and dengue virus infection in the mosquito Aedes fluviatilis (Diptera: Culicidae). PLoS One 12:e0181678. doi: 10.1371/journal.pone.0181678
Sinka, M., Bangs, M., Manguin, S., Rubio-Palis, Y., Chareonviriyaphap, T., Coetzee, M., et al. (2012). A global map of dominant malaria vectors. Parasit. Vectors 5:69. doi: 10.1186/1756-3305-5-69
Sinkins, S. (2004). Wolbachia and cytoplasmic incompatibility in mosquitoes. Insect Biochem. Mol. Biol. 34, 723–729. doi: 10.1016/j.ibmb.2004.03.025
Sinkins, S., Walker, T., Lynd, A., Steven, A., Makepeace, B., Godfray, H. C., et al. (2005). Wolbachia variability and host effects on crossing type in Culex mosquitoes. Nature 436, 257–260. doi: 10.1038/nature03629
Straub, T., Shaw, W., Marcenac, P., Sawadogo, S., Dabiré, R., Diabaté, A., et al. (2020). The Anopheles coluzzii microbiome and its interaction with the intracellular parasite Wolbachia. Sci. Rep. 10, 13847–13841. doi: 10.1038/s41598-020-70745-0
Sullivan, W. (2017). Wolbachia, bottled water, and the dark side of symbiosis. Mol. Biol. Cell 28, 2343–2346. doi: 10.1091/mbc.e17-02-0132
Thomas, P., Kenny, N., Eyles, D., Moreira, L., O’Neill, S., and Asgari, S. (2011). Infection with the wMel and wMelPop strains of Wolbachia leads to higher levels of melanization in the hemolymph of Drosophila melanogaster, Drosophila simulans and Aedes aegypti. Dev. Comp. Immunol. 35, 360–365. doi: 10.1016/j.dci.2010.11.007
Torres, R., Hernandez, E., Flores, V., Ramirez, J., and Joyce, A. (2020). Wolbachia in mosquitoes from the Central Valley of California, USA. Parasit. Vectors 13:558. doi: 10.1186/s13071-020-04429-z
Turelli, M., and Hoffmann, A. (1991). Rapid spread of an inherited incompatibility factor in California Drosophila. Nature 353, 440–442. doi: 10.1038/353440a0
Turley, A., Moreira, L., O'neill, S., and Mcgraw, E. (2009). Wolbachia infection reduces blood-feeding success in the dengue fever mosquito, Aedes aegypti. PLoS Negl. Trop. Dis. 3:e516. doi: 10.1371/journal.pntd.0000516
Turley, A., Smallegange, R., Takken, W., Zalucki, M., O'neill, S., and Mcgraw, E. (2014). Wolbachia infection does not alter attraction of the mosquito Aedes (Stegomyia) aegypti to human odours. Med. Vet. Entomol. 28, 457–460. doi: 10.1111/mve.12063
van den Berg, H., da Silva Bezerra, H. S., al-Eryani, S., Chanda, E., Nagpal, B. N., Knox, T. B., et al. (2021). Recent trends in global insecticide use for disease vector control and potential implications for resistance management. Sci. Rep. 11:23867. doi: 10.1038/s41598-021-03367-9
Villegas-Chim, J., Martin-Park, A., Puerta-Guardo, H., Eugenia Toledo-Romaní, M., Pavía-Ruz, N., Contreras-Perera, Y., et al. (2022). “Community engagement and social assessment for Wolbachia-based suppression of natural populations of Aedes aegypti: the Mexican experience” in Mosquito research-recent advances in pathogen interactions, immunity, and vector control strategies. eds. H. Puerta-Guardo and P. Manrique-Saide (London: Intechopen)
Walker, T. J. P. H., Johnson, P. H., Moreira, L. A., Iturbe-Ormaetxe, I., Frentiu, F. D., McMeniman, C. J., et al. (2011). The wMel Wolbachia strain blocks dengue and invades caged Aedes aegypti populations. Nature 476, 450–453. doi: 10.1038/nature10355
Waymire, E., Duddu, S., Yared, S., Getachew, D., Dengela, D., Bordenstein, S. R., et al. (2022). Wolbachia 16S rRNA haplotypes detected in wild Anopheles stephensi in eastern Ethiopia. Parasit. Vectors 15, 1–11. doi: 10.1186/s13071-022-05293-9
Werren, J., Baldo, L., and Clark, M. (2008). Wolbachia: master manipulators of invertebrate biology. Nat. Rev. Microbiol. 6, 741–751. doi: 10.1038/nrmicro1969
Werren, J. H., and O’Neill, S. L. (1997). “The evolution of heritable symbionts” in Influential passengers: inherited microorganisms and arthropod reproduction. eds. S. L. O’Neill, A. A. Hoffmann, and J. H. Werren (Oxford: Oxford University Press)
Werren, J., and Windsor, D. (2000). Wolbachia infection frequencies in insects: evidence of a global equilibrium? Proc. Biol. Sci. 267, 1277–1285. doi: 10.1098/rspb.2000.1139
WHO. (2021). Global programme to eliminate lymphatic filariasis: progress report, 2020, World Health Organization Geneva
WHO. (2023a). Yellow fever. Available at: https://www.who.int/news-room/fact-sheets/detail/yellow-fever
WHO. (2023b). Zika virus disease. Available at: https://www.who.int/health-topics/zika-virus-disease#tab=tab_1
Wiebe, A., Longbottom, J., Gleave, K., Shearer, F., Sinka, M., Massey, N., et al. (2017). Geographical distributions of African malaria vector sibling species and evidence for insecticide resistance. Malar. J. 16, 85–80. doi: 10.1186/s12936-017-1734-y
Wiwatanaratanabutr, I., Allan, S., Linthicum, K., and Kittayapong, P. (2010). Strain-specific differences in mating, oviposition, and host-seeking behavior between Wolbachia-infected and uninfected Aedes albopictus. J. Am. Mosq. Control Assoc. 26, 265–273. doi: 10.2987/09-5937.1
Wong, M. L., Liew, J. W. K., Wong, W. K., Pramasivan, S., Mohamed Hassan, N., Wan Sulaiman, W. Y., et al. (2020). Natural Wolbachia infection in field-collected Anopheles and other mosquito species from Malaysia. Parasit. Vectors 13, 1–15. doi: 10.1186/s13071-020-04277-x
Ye, Y, Woolfit, M, Rancès, E, and O’Neill, S, McGraw E. (2013). Wolbachia-associated bacterial protection in the mosquito Aedes aegypti. PLoS Negl. Trop. Dis., 7,:e2362, doi: 10.1371/journal.pntd.0002362
Yeap, H. L., Axford, J. K., Popovici, J., Endersby, N. M., Iturbe-Ormaetxe, I., Ritchie, S. A., et al. (2014). Assessing quality of life-shortening Wolbachia-infected Aedes aegypti mosquitoes in the field based on capture rates and morphometric assessments. Parasit. Vectors 7, 58–13. doi: 10.1186/1756-3305-7-58
Yen, P., and Failloux, A. (2020). A review: Wolbachia-based population replacement for mosquito control shares common points with genetically modified control approaches. Pathogens 9:404. doi: 10.3390/pathogens9050404
Zhang, D., Wang, Y., He, K., Yang, Q., Gong, M., Ji, M., et al. (2020). Wolbachia limits pathogen infections through induction of host innate immune responses. PLoS One 15:e0226736. doi: 10.1371/journal.pone.0226736
Zheng, X., Zhang, D., Li, Y., Yang, C., Wu, Y., Liang, X., et al. (2019). Incompatible and sterile insect techniques combined eliminate mosquitoes. Nature 572, 56–61. doi: 10.1038/s41586-019-1407-9
Zug, R., and Hammerstein, P. (2012). Still a host of hosts for Wolbachia: analysis of recent data suggests that 40% of terrestrial arthropod species are infected. PLoS One 7:e38544. doi: 10.1371/journal.pone.0038544
Keywords: mosquito symbiont, Wolbachia, Aedes, Anopheles, Culex, mosquito, mosquito-borne diseases
Citation: Minwuyelet A, Petronio Petronio G, Yewhalaw D, Sciarretta A, Magnifico I, Nicolosi D, Di Marco R and Atenafu G (2023) Symbiotic Wolbachia in mosquitoes and its role in reducing the transmission of mosquito-borne diseases: updates and prospects. Front. Microbiol. 14:1267832. doi: 10.3389/fmicb.2023.1267832
Edited by:
Takema Fukatsu, National Institute of Advanced Industrial Science and Technology (AIST), JapanReviewed by:
Yunsheng Wang, Hunan Agricultural University, ChinaSonam Popli, University of Toledo, United States
Copyright © 2023 Minwuyelet, Petronio, Yewhalaw, Sciarretta, Magnifico, Nicolosi, Di Marco and Atenafu. This is an open-access article distributed under the terms of the Creative Commons Attribution License (CC BY). The use, distribution or reproduction in other forums is permitted, provided the original author(s) and the copyright owner(s) are credited and that the original publication in this journal is cited, in accordance with accepted academic practice. No use, distribution or reproduction is permitted which does not comply with these terms.
*Correspondence: Giulio Petronio Petronio, Z2l1bGlvLnBldHJvbmlvcGV0cm9uaW9AdW5pbW9sLml0