- 1Department of Biological Sciences and Marine Research Institute, University of Cape Town, Cape Town, South Africa
- 2Department of Forestry, Fisheries and Environment, Cape Town, South Africa
Integrating observations and experiments, we address the progressive effects of three alien species, the mussels Mytilus galloprovincialis and Semimytilus patagonicus, and the barnacle Balanus glandula, on limpet species in South Africa. We describe four aspects: (1) Interactions among algae, the limpet Scutellastra granularis and M. galloprovincialis. (2) The influences of B. glandula on S. granularis and on the periwinkle Afrolittorina knysnaensis. (3) Transformation of the zonation of S. granularis by the successive arrival of these three aliens. (4) Assessment of how effective the published predictors of the effects of M. galloprovincialis have been when applied to S. patagonicus. We conclude: (a) Scutellastra granularis improves mussel survival and condition by regulating algae that would otherwise overgrow and smother the mussels. (b) Balanus glandula has largely occupied the upper half of rocky shores, and at high densities depletes or eliminates suitable bare-rock habitat for S. granularis. However, it has positive effects on another gastropod, A. knysnaensis. (c) Mytilus galloprovincialis dominates the midshore and has positive effects on the recruitment of S. granularis, but negatively influences adults of this limpet, so that its size composition, density, reproductive output and zonation are all altered by this mussel. (d) Semimytilus patagonicus presents different challenges to those created by M. galloprovincialis, settling at much greater densities, lower down the shore, and reaching smaller maximum sizes. Rather than generating a favourable habitat for epizootic S. granularis recruits and juveniles, it almost completely excludes that limpet. We conclude that the influences of alien species are not readily predictable, depending on the nature of the invader and recipient species, environmental conditions and complex interactions among species. Collectively, the three alien species now cover almost all zones on wave-exposed rocky shores, completing the ‘zonation squeeze’ on limpets, but are less influential on wave-sheltered shores and in years when recruitment is low.
1 Introduction
This paper deals with three of the alien species that have occupied the shores of southern Africa, namely Mytilus galloprovincialis (Mediterranean mussel), Semimytilus patagonicus (bisexual mussel) and Balanus glandula (Pacific barnacle), and their interactions with the indigenous limpets, particularly Scutellastra granularis, as well as the effects of B. glandula on the indigenous periwinkle Afrolittorina knysnaensis.
All of these alien species have become dominant on southern African rocky shores and have spread substantially from their points of origin. Mytilus galloprovincialis arrived in the late 1970s (Grant and Cherry, 1985) and now covers over 2000 km of the coast from northern Namibia to the south-east coast of South Africa. Balanus glandula was first formally recorded in South Africa in 2008 but probably arrived as early as 1992 (Jenkins et al., 2008; Laird and Griffiths, 2008; Simon-Blecher et al., 2008). It currently occurs over 500 km of the southern west coast. Semimytilus patagonicus (until recently known as S. algosus) originated from Chile, arrived in Namibia as early as 1930 and abruptly spread from there to South Africa in 2009, where it now occurs over almost the entire west coast and has penetrated to a limited extent on the south coast (De Greef et al., 2013; Alexander et al., 2015a; Ma et al., 2020a; Ma et al., 2020b; Zeeman et al., 2020).
The sequential arrival of these species at Marcus Island in Saldanha Bay on the West Coast is documented by Robinson et al. (2007) and Sadchatheeswaran et al. (2015; 2018), who showed that (a) after its arrival, M. galloprovincialis formed deep beds and increased habitat complexity, consequently augmenting community diversity; (b) S. patagonicus failed to have these effects, and (c) in zones where B. glandula later replaced M. galloprovincialis, the simpler monolayered structure of this barnacle substantially reduced complexity and diversity.
The interactions between M. galloprovincialis and the indigenous brown mussel Perna perna on the south coast of South Africa have attracted particular attention (Erlandsson et al., 2006; Zardi et al., 2006; Hanekom, 2007; Nicastro et al., 2007; Zardi et al., 2007; Nicastro et al., 2008; von der Meden et al., 2008; Nicastro et al., 2010a; Nicastro et al., 2010b; von der Meden et al., 2010; Lathlean et al., 2016; synthesized in Branch and Branch, 2018). In brief, survival of M. galloprovincialis is greater higher on the shore, and that of P. perna lower on the shore, hence their partial segregation and continued coexistence.
Interactions between M. galloprovincialis and the limpet S. granularis have also been explored (Griffiths et al., 1992; Hockey and van Erkom Schurink, 1992; Branch et al., 2010). In short, dense beds of the mussel provide a haven for recruits of the limpet, boosting its numbers; but adults of the limpet are crowded out when they become too large to occupy mussel shells (Supplementary Figure S1). The demographics and normal zonation pattern of the limpet have consequently been transformed. Before the arrival of M. galloprovincialis, the limpet was one of a group of limpets that Branch (1975; 1976) termed ‘migratory’, in the sense that they settle low on the shore and then progressively migrate upshore as their size and tolerance to physical to physical stresses increases. Arrival of M. galloprovincialis has overturned that, as we describe in this paper.
Alien species are one of the major threats to the integrity of marine systems, increasingly transforming and homogenizing the nature of ecosystems world-wide (Thompson et al., 2002; Robinson et al., 2007; Pyšek et al., 2020), being ‘a potent driver of change with no sign of saturation’ (Ros et al., 2023, p81). They are amply living up to the prognosis for the future made in 2008 that by 2025 their rate of spread would have radically increased (Branch et al., 2008a). In South Africa alone, the rate at which alien species have been recorded has escalated exponentially from just one in 1950 to 95 by 2023 (Robinson et al., 2005; Griffiths et al., 2009; 2010; Mead et al., 2011a; Mead et al., 2011b; Robinson et al., 2016; Robinson et al., 2020; Van Wilgen et al., 2023).
Jennsen (2015) expresses a pessimistic view: ‘You have loosed a chaotic, unstable element into the Mosaic. They will destroy everything.’ The effects of alien species are, however, not all unmitigatedly negative. Some, such as the ascidian Pyura praepucialis in Chile increase habitat complexity and enhance biodiversity as a result (Castilla et al., 2004). Arrival of the Mediterranean or blue mussel Mytilus galloprovincialis in South Africa has boosted the food supplies of the African Black Oystercatcher Haematopus moquini, improving its conservation status by trebling of the rate at which pairs of birds raise two chicks rather than one (Hockey and van Erkom Schurink, 1992; Coleman and Hockey, 2008).
A central focus of our paper is that alien species have complex and unpredictable effects on the structure and functioning of South African rocky shores. Part of the reason is that their effects of are moderated by a range of factors, including geographic distribution (Ma et al., 2023), zonation (Rius and McQuaid, 2006), wave action (Steffani and Branch, 2003a; Steffani and Branch, 2003b; Hampton and Griffiths, 2007; Branch et al., 2010), responses to predators (Alexander et al., 2015a), resistance to desiccation (Nicastro et al., 2010a), attachment strength (Zeeman et al., 2018), the effects of upwelling (Xavier et al., 2007), and the relative sizes of organisms (Branch and Steffani, 2004).
A second focus of the paper is the interplay of patellid limpets with alien species. Patellids are dominant grazers and can influence alien species either directly by removing them or indirectly by controlling other species such as algae that may influence them. Firth (2021, p30) describes limpets as ‘the champion grazers of the rocky intertidal zone’. From the pioneering experimental removals of Patella spp. in Great Britain (Jones, 1946; Lodge, 1948) that demonstrated limpet grazing and not wave action was responsible for keeping algal growth in check, through multiple other experiments, removal of limpets has consistently resulted in algal proliferation (Southward, 1964; Branch, 1981; Hawkins and Hartnoll, 1983; Branch, 1985; Farrell, 1988; Dye, 1995; Jenkins et al., 1999; Lindegarth et al., 2001; Arrontes et al., 2004; Phillips and Hutchison, 2008; Maneveldt et al., 2009; Tejada-Martinez et al., 2016). A common pattern is that removal of limpets is followed sequentially by development of a film of diatoms and sporelings, then emphemeral algae such as Ulva spp., and finally slower-growing corticated algae (Hawkins, 1981).
Added to this, limpets can act is ‘bulldozers’, reducing the abundance of small settlers of species such as barnacles (Menge et al., 2010; Ellrich et al., 2020). The situation is even more complex when other members of the community indirectly influence the outcome of interactions. Barnacles may aid settlement and survival of algae, but algal settlement can increase the mortality rate of the barnacles and enhance recruitment of limpets that then deplete the algae (Hartnoll and Hawkins, 1985). Cycles may arise in which the abundances of algae and limpets alternate over roughly 10-year periods (Little et al., 2017).
Considering the multiplicity of factors influencing the effects of both alien species and grazers, it is not simple to forecast their precise interactions, which can range from competitive exclusion to facilitation (Miyamoto and Noda, 2004).
This paper addresses four aspects of interaction between alien species and limpets on South African shores. The first concerns a three-way interplay between M. galloprovincialis, algae and S. granularis. Grazing by the limpet on shells of the mussel may control algal growth, but the benefits (or otherwise) of this for the mussel are not easy to predict, as grazing on the mussel shells weakens them (Day et al., 2000) and may offset any benefits. Second, the influence of B. glandula on the abundances of both S. granularis and the periwinkle Afrolittorina knysnaensis is explored. The latter was selected because of previous correlative evidence of the influence of B. glandula on it (Laird and Griffiths, 2008), which we verify experimentally. Third, the influences of M. galloprovincialis, S. patagonicus and B. glandula on the zonation of S. granularis are described. Finally, we turn to predictions previously made about why M. galloprovincialis has successfully dominated large portions of the coast of southern Africa (Branch and Steffani, 2004) and, drawing on both our results and information in the literature, we consider the applicability of these predictions when applied to the later arrival and spread of S. patagonicus.
We address four specific hypotheses:
1. Grazing by the limpet S. granularis will benefit M. galloprovincialis by preventing algal overgrowth. Conversely, the mussel will support high densities of S. granularis, but only small individuals.
2. Dense settlements of the alien barnacle B. glandula will exclude S. granularis but will enhance densities of the periwinkle A. knysnaensis.
3. The ‘normal’ progressively upshore migration of S. granularis to establish an increasing gradient of size and a decreasing gradient of density will be interrupted by the arrival of M. galloprovincialis and further altered by S. patagonicus and B. glandula.
4. Factors that successfully predicted the invasive capabilities of M. galloprovincialis will not be the same as those applicable to S. patagonicus.
2 Materials and methods
2.1 Interactions among limpets, mussels and algae
Manipulative experiments were conducted at two sites just south of Groenriviermond on the west coast of South Africa (Figure 1), approximately 450 km north of Cape Town: Island Point (30°54.93´S; 17°36.17´E) and Nina’s Site (30°54.88´S; 17°36.28´E), from January to June 2000, after which the plots were destructively sampled, and measurement of the variables taken as described below. Steffani and Branch (2003a) class both sites as exposed, experiencing average wave forces of 10.0-12.0 × 103 N m-2, and they fall in an area of active upwelling with high productivity (Andrews and Hutchings, 1980), and high algal, limpet and mussel biomass (Bustamante et al., 1995b). Tidal range was 1.9 m.
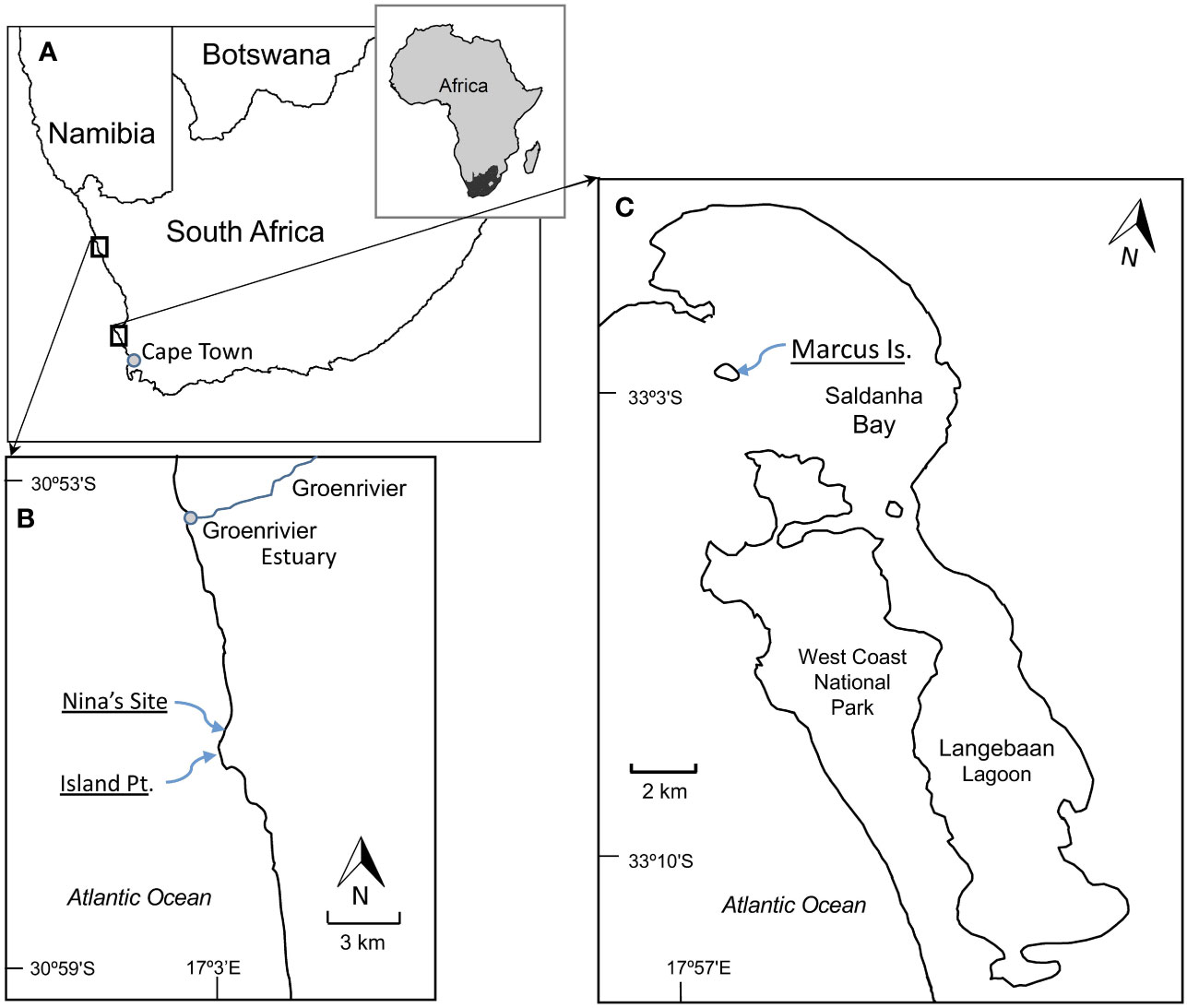
Figure 1 Maps of Africa and South Africa (A) and details (B, C) of the study sites (names underlined).
Five replicate plots per treatment of 30×30 cm were established at each site (five replicates × four treatments × two sites). These were installed at the mid-tide level (tidal height 0.9 m), where M. galloprovincialis comprised 97% of all mussels present, and the limpet S. granularis constituted 95% of all limpets present. Plots that were designed to exclude limpets were surrounded by 5-cm-wide band of lead oxide antifouling paint, to control ingress or egress of limpets. Undisturbed control plots and three treatments were employed, as visualized in Figure 2A: (1) Control plots that contained both mussels and limpets (+M+L). (2) Limpet removal plots from which all limpets were removed (+M–L) and exclusion maintained by ongoing monthly removals. (3) Mussel removal plots (–M+L) in which mussels were removed, but any limpets that entered were allowed to remain there. (4) Removal of both mussels and limpets (–M–L). Monitoring took place monthly, when plots were inspected and the required treatments maintained; but only data for the terminal sampling are presented, partly to avoid temporal pseudo-replication, but also because that was when the plots were sampled destructively to allow full assessment.
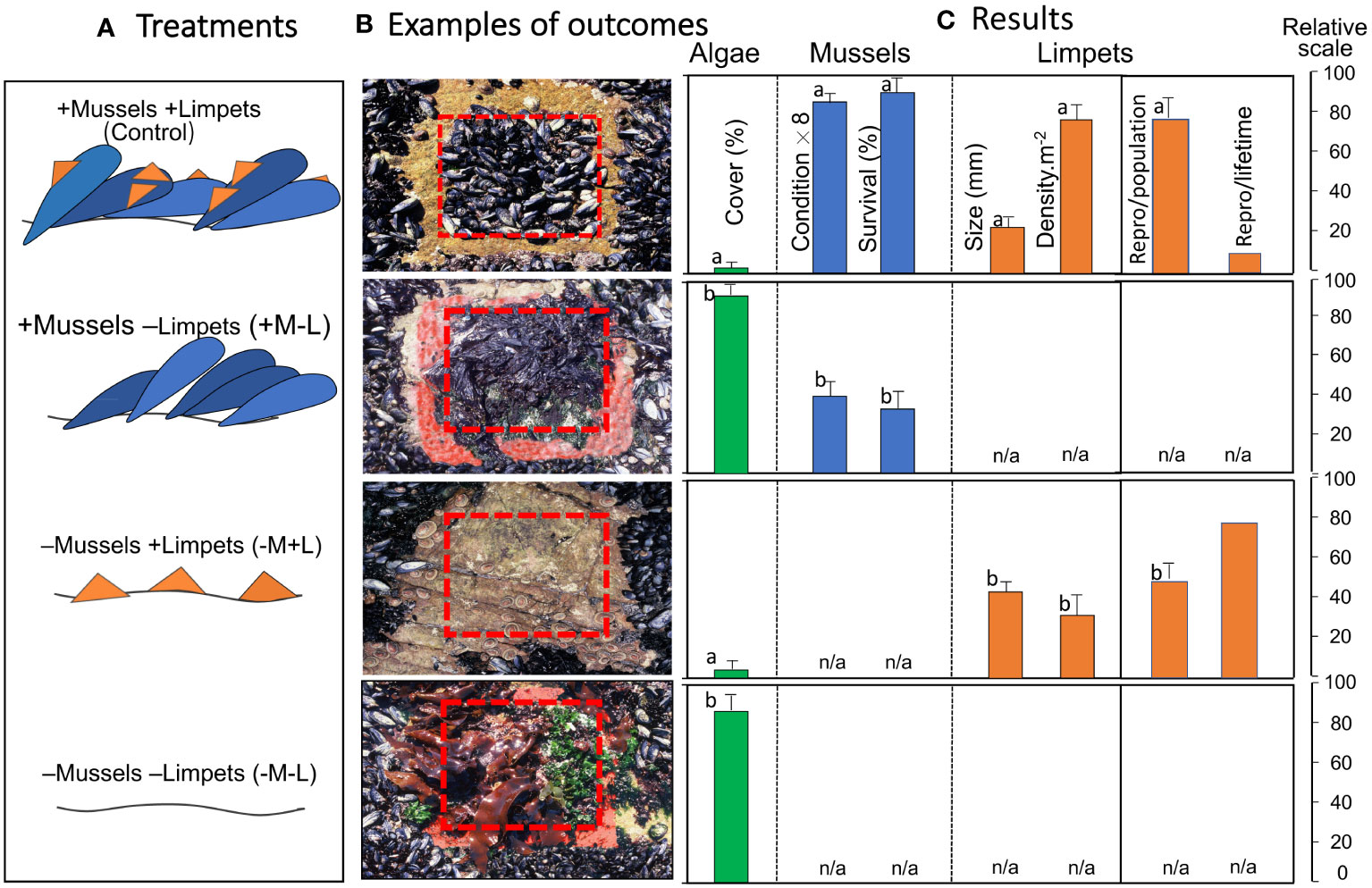
Figure 2 (A) Design of a field experiment to assess the interplay between the alien mussel M. galloprovincialis, the limpet S. granularis and algae. (B) Visual examples of outcomes. (C) Results showing the proliferation of algae in absence of limpets, better condition and survival of mussels in presence of limpets, and a drastic decline in lifetime reproductive output of (smaller) limpets that live on mussels versus rock, despite their greater densities. Data are mean values +1SD, n = 10; except for reproductive output per lifetime, which is a single calculated value. The data are standardised to the same scale of 0-100 in units specified for each variable, but values for condition have been multiplied by a factor of eight to improve visibility. Repro/population = the reproductive output (g wet mass m-2 yr-1) of the population, calculated from replicate plots. Repro/lifetime = the reproductive outputs (g wet mass) per individual calculated over its lifetime, assuming individuals remain on shells or on rocks. No error term is attached to this value as it constitutes a single calculation. n/a, not applicable due to the absence or one or more taxa from the treatment. Lower case letters above bars differ if treatment were significantly different.
The outcomes of these treatments were assessed by measuring: (a) algal % cover and composition; (b) mussel condition and percentage survival relative to original densities at the start of the experiment; (c) limpet sizes, densities, reproductive output for the populations (wet g m-2), and life-time reproductive output per individual (wet g indivual-1). For all variables except condition and reproductive output, scoring was done in situ and (where feasible) a second estimate obtained using photographs of plots. The greater of these two values was accepted as being the most accurate. Population reproductive output was calculated from the counts and size composition of limpets measured per plot, converting sizes to ages using regressions taken from data in Branch (1975), and ages to wet-mass reproductive output per annum per plot, based on conversions derived from data in Branch (1974a). Life-time outputs per individual were calculated for standard individuals occupying mussels or rock, taking into account average survivorship per year, and outputs per annum summed over a lifespan, also based on survivorship and size-related annual reproductive outputs in Branch (1974a; 1974b). These two measures are distinctly different and not necessarily correlated. The population reproductive output estimates the output per annum per unit area for each plot, so that a mean (and SD) value can be calculated for the population as a whole. The life-time reproduction is a single composite measure of what an individual (standard) limpet will achieve over its estimated lifespan. The former measure is relevant to population dynamics; the latter to the evolutionary reproductive success of individuals that remain on mussels or live on rocks. Both can be calculated for limpets on either mussels or rocks, making the assumption that individuals will remain there throughout their lives.
Mussel condition was determined from subsamples of 20 mussels (40-50 mm shell length) per plot taken at the end of the experiment and was measured as (dry) body mass divided by shell mass × 100 (Steffani and Branch, 2003a). For mussel survival, only mussels classed as adult, i.e., > 35 mm shell length, were counted, as those smaller than that were too difficult to score accurately at the start of the experiment. For the same reason, for limpet densities, only individuals > 10mm were counted.
To test for possible ‘cage’ (i.e., paint) effects on limpet presence or absence and algal growth, procedural controls with paint applied just at the corners of plots were established. We hypothesized entry of limpets would not be precluded by the paint, and that if limpets did enter the plots, they would control algal growth, but that if they did not, algal growth would proliferate to the same extent as in fully enclosed plots excluding limpets.
2.2 Barnacles, limpets and periwinkles
Interactions between B. glandula and S. granularis, and between B. glandula and the littorinid Afrolittorina knysnaensis were assessed at Marcus Island [33°02.59´S; 17°58.26´E; Figure 1)], starting April 2012, shortly after the barnacle was detected there, during a period when it covered extensive portions of the mid to high shore at tidal heights of 0.99 to 1.39 m above spring low-tide levels (SLT). Tidal range was 1.96 m and wave force rated as exposed, averaging 11.3 × 103 N m-2 (Sadchatheeswaran et al., 2015).
Interactions were assessed in two ways: (a) by first determining correlations between the numbers of barnacles and either limpets or littorinids, which provided information on natural patterns of relative abundance, and (b) by using manipulative experiments that followed immediately after the correlative data had been collected, to experimentally explore the responses of limpets or littorinids to barnacle cover, providing an independent cause-and-effect test of the relationship between barnacles and these species.
Correlations between the abundances B. glandula (% cover) and S. granularis and A. knysnaensis (counts) were determined from 50 randomly placed 14.1 × 14.1 cm quadrats, in the mid shore for S. granularis, and high shore for A. knysnaensis. Densities were converted to numbers m-2 for presentation.
For the manipulative experiment, six replicates of three treatments were established in plots of 20 × 20 cm at these two respective shore heights, in each of two areas separated by 50 m. Treatments comprised (a) control plots of in which B. glandula covered 95-100% of the substratum (Supplementary Figure S2), which were left unaltered (+Balanus), (b) removal plots of the same size in which 50% of the barnacles were removed, and (c) all barnacles were removed by scraping to bedrock (-Balanus). Numbers of any S. granularis that occupied plots were recorded after 1 month, and sizes estimated from either the total sample, or from a subsample of the first 50 individuals encountered per plot if numbers exceeded this. Subsampling was employed only after test sampling showed that 50 individuals were sufficient to secure a mean measure within 3% accuracy of that from total samples of up to 90 individuals.
2.3 Effects of alien species on zonation of S. granularis
To test the extent to which the arrival of M. galloprovincialis, S. patagonicus and B. glandula has altered the previously described progressive ‘migratory’ up-shore movement of S. granularis (Branch, 1975; see also Table 1, and hypothesis 3 in introduction), data on the densities and sizes of S. granularis were obtained from Marcus Island in April 1970 before the detection of any alien species; in April 2001 after M. galloprovincialis had become established and was at its peak abundance, and in April 2012 when M. galloprovincialis had diminished but S. patagonicus and B. glandula were established. On five replicate vertical transects up the shore and within quadrats of 1.0 × 0.5 m, five zones were surveyed, designated as Infratidal (0.0-0.1 m above SLT), Low (0.25-0.30 m), Mid (0.85-0.95 m), High (1.10-0.26 m) and Top (1.30-0.1.56 m). Densities were converted to numbers m-2.
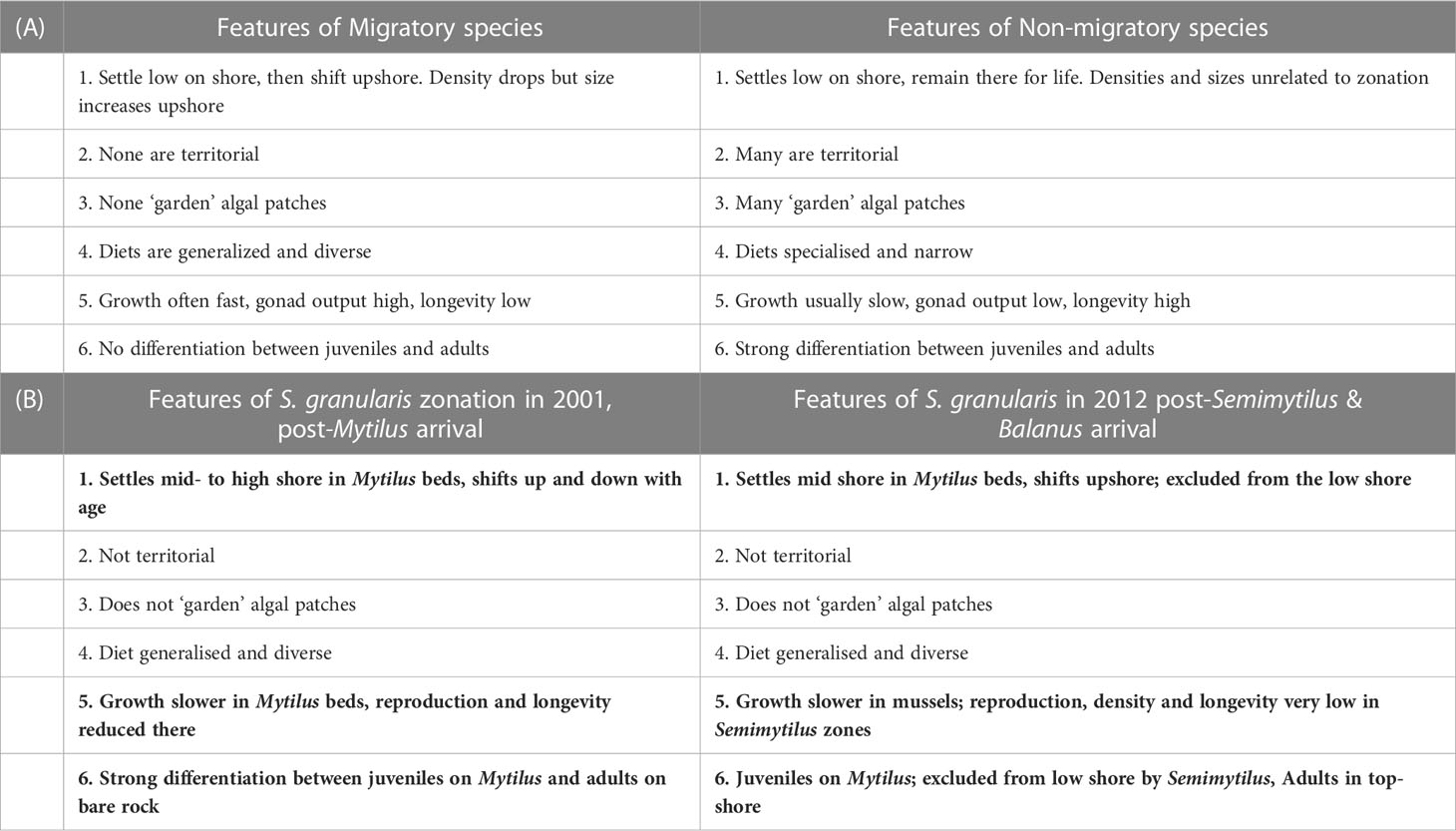
Table 1 (A) Summary of features characterising migratory and non-migratory limpets, derived from Branch (1975) and Lindberg (2007), and (B) how these have changed (bold entries) or not changed for Scutellastra granularis after the arrival of first Mytilus galloprovincialis, and later Semimytilus patagonicus and Balanus glandula.
2.4 Predictors of the invasive success of S. patagonicus
To assess the features that could be used to predict successful invasions of respectively M. galloprovincialis and S. patagonicus, we integrated our results with published material (mainly Branch and Steffani, 2004; Zeeman et al., 2018, but also papers cited in Table 2), based on a literature search of journal articles in google and scholar.google.com for key words Semimytilus algosus, Semimytilus patagonicus, Mytilidae, mytilids, mussels.
2.5 Statistical analyses
For interactions among limpets, mussels and algae at Groenrivier, a two-way analysis of variance (ANOVA) with treatments nested in sites showed that differences between sites were non-significant for all variables: ANOVA1,4, p > 0.25, so the data for the two sites were pooled (Quinn and Keough, 2002). In the case of algal cover, for which four treatment levels could be compared, the assumptions of normality and equality of variance for ANOVA could not be met even after transformations, so a non-parametric Kruskal-Wallis test was applied, followed by Dunn post-hoc comparisons among treatments. For the remaining variables where only two treatment levels existed (mussel condition/survival, limpet densities/sizes, population reproductive output), the assumptions of normality (assessed with normal probability plots) and of equal variances (accepted if ratios of variance did not differ by more than a factor of five; Quinn and Keough, 2002) were met without transformation, and Students t-tests applied. Life-time individual reproductive outputs were calculated for idealized individuals, thus yielding single values for limpets on mussels versus rocks, so no statistical tests of their difference were possible.
For the field-sampled relationship between B. glandula on the densities of respectively S. granularis and A. knysnaensis, Pearson’s correlation coefficients were determined. For the manipulative experiment, differences between the two areas were again non-significant (ANOVA1,5 P >0.25 in all cases) so the data were pooled for analysis. Densities of S. granularis could not be normalized and the variances remained unequal even after transformation because there were multiple zero values for the +Balanus treatment. We therefore applied Kruskal-Wallis tests followed by Dunn post-hoc comparisons among treatments. For Afrolittorina knysnaensis abundance, assumptions of equality of variance and normality (assessed as above) were met and the data analyzed without transformation. For sizes, normality and equality of variance were achieved by log-log transformations and one-way ANOVAs were applied to those data, followed by Tukey post-hoc tests of any significantly different data.
To test for differences in densities and for sizes of S. granularis amongst the five zones, the years (1970, 2001 and 2012) and their interaction, a two-way ANOVA was run with years and zones as fixed factors, using the aov function in R (R Core Team, 2019). For density, the data were log (x+1) transformed to meet assumptions of normality and equality of variance. The data for sizes did not require transformation, and were averaged for each quadrat (i.e., within each zone and transect) to achieve a balanced ANOVA design. No limpets were present in three of the five replicate samples in the infratidal zone and in these cases missing size data were substituted with dummy values without altering the mean and variance of the model cell. Tukey HSD was used for posthoc comparisons.
3 Results
3.1 Interactions among limpets, mussels and algae
There was no significant ‘cage’ (i.e., paint) effect. Limpets entered cage control plots in 7/10 cases, and inhibited algal growth, the abundance of which remined low and did not differ significantly from the respective +M+L and –M+L treatments (t-tests, df =8, p > 0.05 in all treatments). For the remaining control plots (3/10), limpets failed to enter, algae flourished and reached levels not significantly different from +M–L and –M–L treatments (t-tests, df =8, p > 0.05 in all treatments). In short, limpets were not inhibited from entering by partial ‘cages’, and algal growth depended on the presence or absence of limpets, not on the extent of the paint enclosure.
In the experimental plots that were fully enclosed with paint, the limpet S. granularis never entered plots from which they had been removed. In plots containing limpets, their densities remained within 85-100% of initial densities. Thus, the –L and +L conditions were effectively maintained.
In plots with the limpet S. granularis present (+M+L and -M+L), minimal amounts of algae became established, whereas plots from which the limpet was excluded (+M-L and -M-L) developed a prolific cover of close to 100% (Figure 2). The differences were significant (Kruskal-Wallis H = 29.95, df = 36; p < 0.0001), and Dunn post-hoc tests showed that significant differences (p < 0.05) were restricted to the two +L treatments versus the two -L treatments.
In plots that contained mussels, body condition and survivorship of mussels were both significantly lower in –L treatments, in which the mussels were covered by a dense algal growth, than in +L plots (Figure 2), with the differences being significant (t-tests = 20.18 and 28.58 respectively, df = 18, p < 0.0001 in both cases).
Limpet sizes were significantly smaller, and limpet densities significantly greater, in plots that contained mussels than in those lacking mussels (Figure 2; t-tests = 8.28 and 9.79 respectively, df =18, p < 0.0001 in both cases). Limpet reproductive output calculated per unit area for the population on mussels was about 1.33 times that of limpets on rocks in areas without mussels, the difference being significant (t-test = 2.58, df = 18, p = 0.018). Conversely, life-time reproductive output of those on rocks was about 7.9 times greater than that of limpets on mussels (Figure 2).
3.2 Barnacles, limpets and periwinkles
In the mid shore to high shore at Marcus Island, densities of S. granularis were significantly negatively correlated with those of the alien barnacle B. glandula, whereas those of the littorinid Afrolittorina knysnaensis were significantly positively correlated with B. glandula (Figure 3).
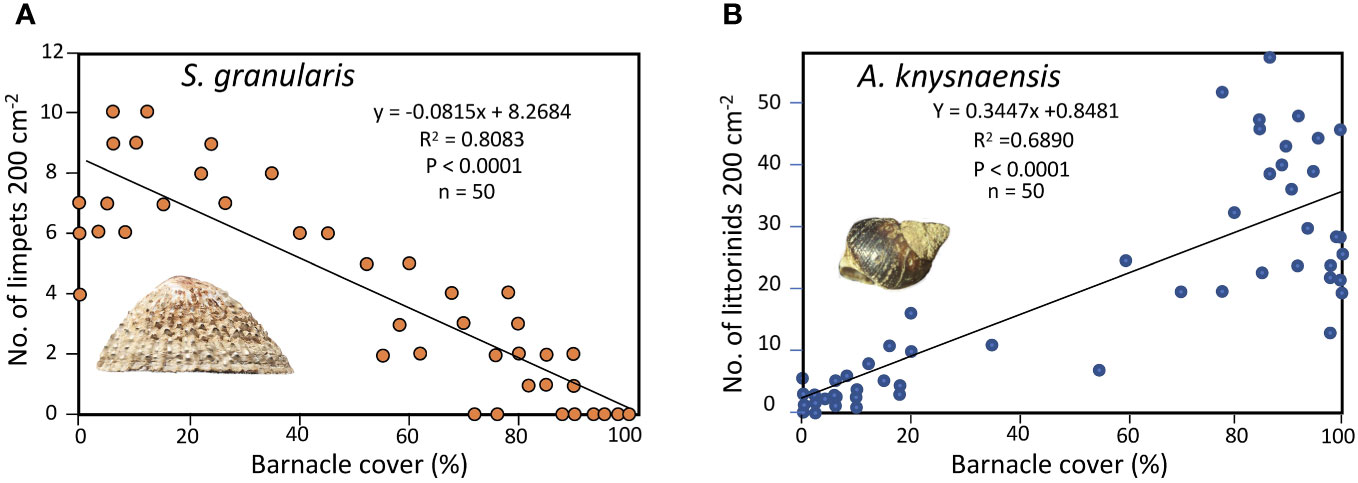
Figure 3 Densities of (A) the limpet Scutellastra granularis and (B) the littorinid Afrolittorina knysnaensis relative to the percentage cover of the alien barnacle Balanus glandula at Marcus Island, South Africa.
In the experimental plots in which the barnacles were left untouched, thinned by 50% or removed altogether (Figure 4A), the densities and sizes of S. granularis that became established were significantly different among all three treatments (Respectively Kruskal-Wallis H = 21.210, df = 2, n = 26, P < 0.0001, Dunn post-hoc tests P < 0.05 among all three treatment for sizes; and ANOVA F2,27 = 66.807, P < 0.0001 and Tukey posthoc tests P < 0.05 among all treatments for densities).
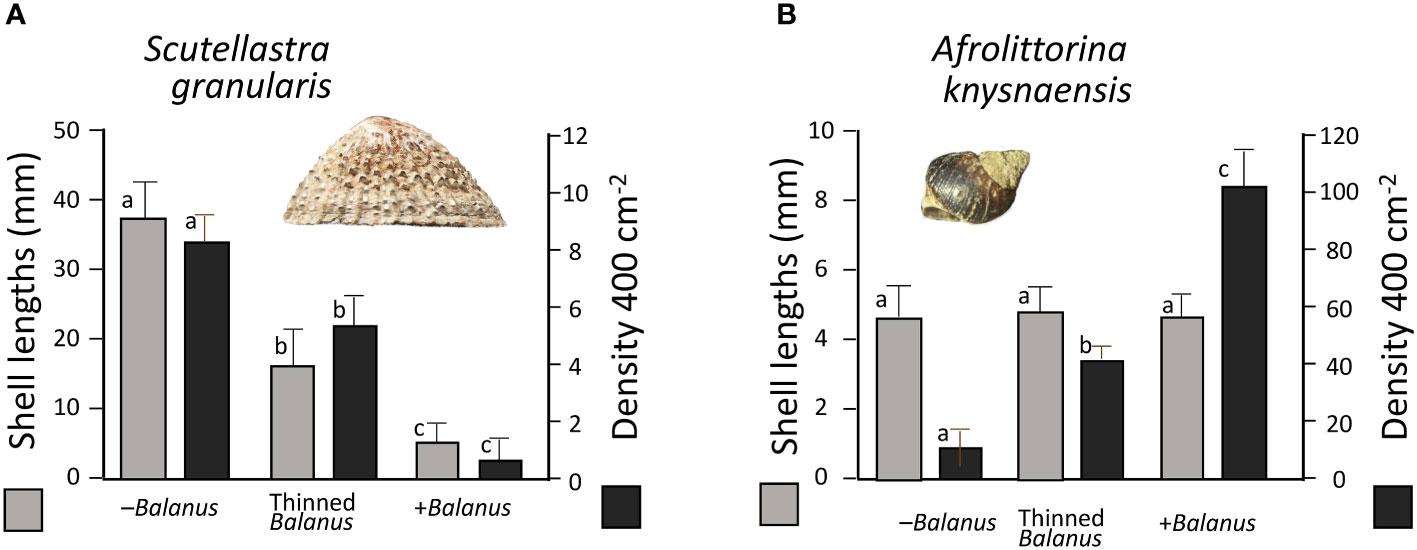
Figure 4 The sizes and densities of (A) Scutellastra granularis and (B) A. knysnaensis at Marcus Island, in relation to the three treatments of –Balanus (complete removal of barnacles), Thinned Balanus (removal of 50%) and +Balanus (natural density of barnacles close to 100%). Values are means +1SD; lowercase letters differ for treatments that were significantly different on post-hoc tests.
For A. knysnaensis (Figure 4B), densities in the three treatments differed significantly (Kruskal-Wallis H = 25.865, df = 2, n = 30, P < 0.0001; Dunn post-hoc test P < 0.05 among all three treatments). Sizes did not, however, differ among treatments (ANOVA F = 0.059, df = 2,27, P = 0.94).
3.3 Effects of alien species on zonation of S. granularis
The densities of S. granularis on Marcus Island were significantly affected by an interaction between zones and years (ANOVA, F = 34.52, DF = 8,60, P <0.0001). Post-hoc Tukey tests showed that this was because of a shift from a progressive decrease in limpet density up the shore in 1970, to (a) a concentration in the mid and high shore, where the limpet increased from around 20 m-2 in 1970 to about 65 m-2 in 2001 when M. galloprovincialis had become dominant, and (b) declines in abundance of S. granularis in 2012 to about 2 m-2 in the infratidal and low-shore zones (Figure 5A) with the arrival and establishment of S. patagonicus, and to around 5-10 m-2 in the high zone associated with declines in M. galloprovincialis abundance and the range of its zonation, and the establishment of B. glandula in the high shore.
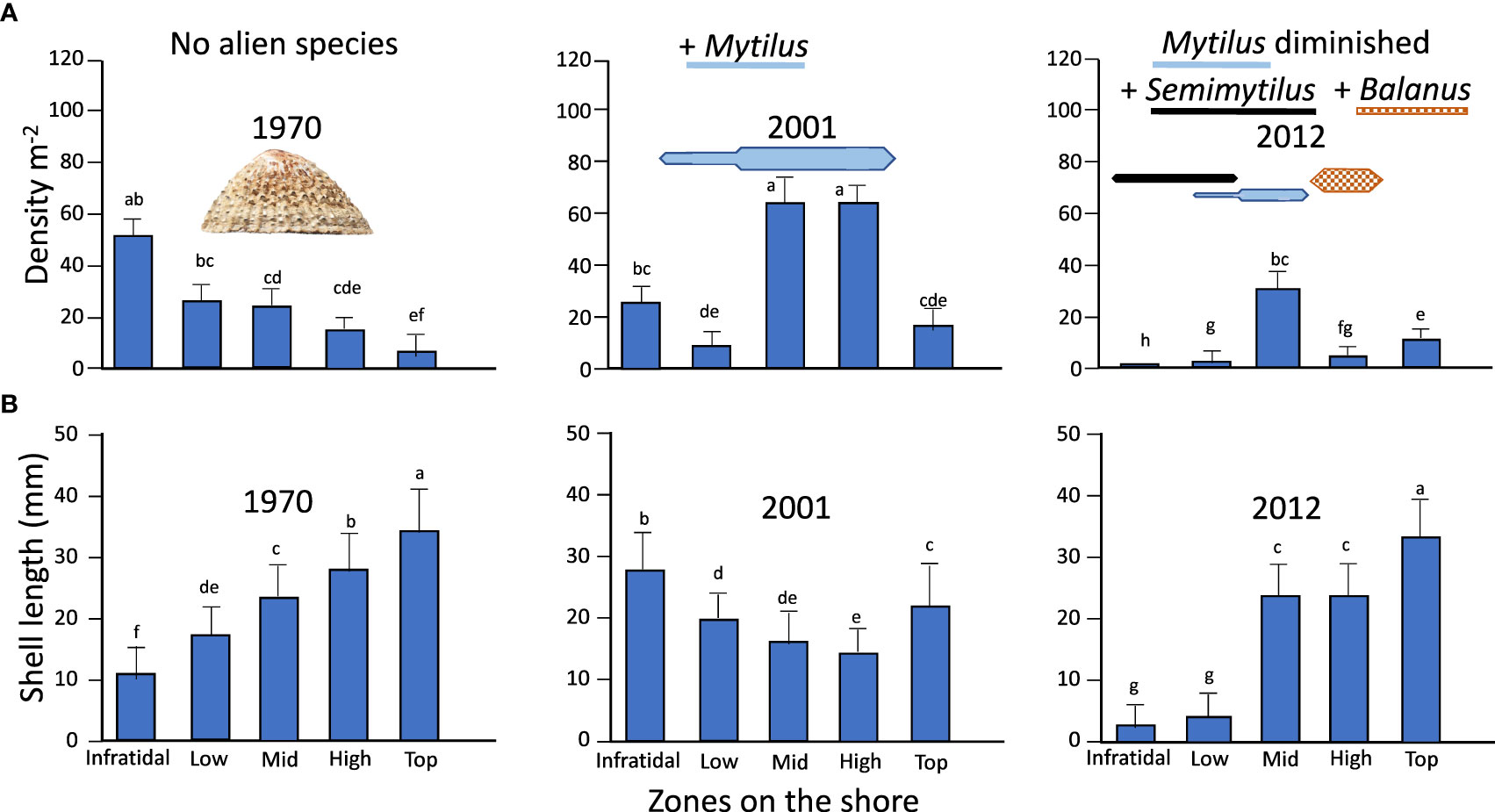
Figure 5 Densities (A) and sizes (B) of the limpet S. granularis in relation to zonation, in 1970 prior to arrival of alien species, in 2001 after arrival of M. galloprovincialis and in 2012 when M. galloprovincialis had diminished and S. patagonicus and B. glandula had arrived. Kite diagrams non-quantitatively show the relative zonation and abundance of alien species, coded by species. Values are means +1SD. Lower-case letters are shared if values are not significantly different.
The sizes (shell lengths) of S. granularis (Figure 5B) also changed among years and zones with a significant interaction (ANOVA, F = 226.1, DF = 8,60, P <0.0001), which affected interpretation of the effects of year and zone. The interaction arose mainly from a reversal in of the 1970 pattern of an upshore increase in size, to one with smallest sizes in the mid and high shore, and then a restoration in 2012 to close to the original 1970 pattern of zonation. Tukey post-hoc tests showed this was attributable to (a) declines in the sizes of limpets in the mid to top-shore in 2001, associated with the occupation of M. galloprovincialis shells by limpet recruits and juveniles in the mid and high shore, and their migration from there into both the infratidal and the top-shore zones, (b) radical reductions of sizes of the limpet in 2012 in the infratidal and lowshore, coinciding with the arrival of S. patagonicus, and (c) increases in size in the mid- to high shore with a reduction in the cover of M. galloprovincialis and arrival of B. glandula in the mid to high shore. Sizes in the top-shore, which was above the zonation range of any of the alien species, remained the highest or the second-highest values across all three periods.
In the context of classifying limpets as either migratory or non-migratory, Table 1A summarises the characteristics of these two groups, and Table 1B lists how the zonation patterns of S. granularis have changed from before to after the arrival of alien species.
3.4 Predictors of the invasive success of S. patagonicus
Table 2 synthesises nine potential predictors of invasion success. All proved applicable to M. galloprovincialis; but only seven were relevant for S. patagonicus, as we amplify in the discussion.
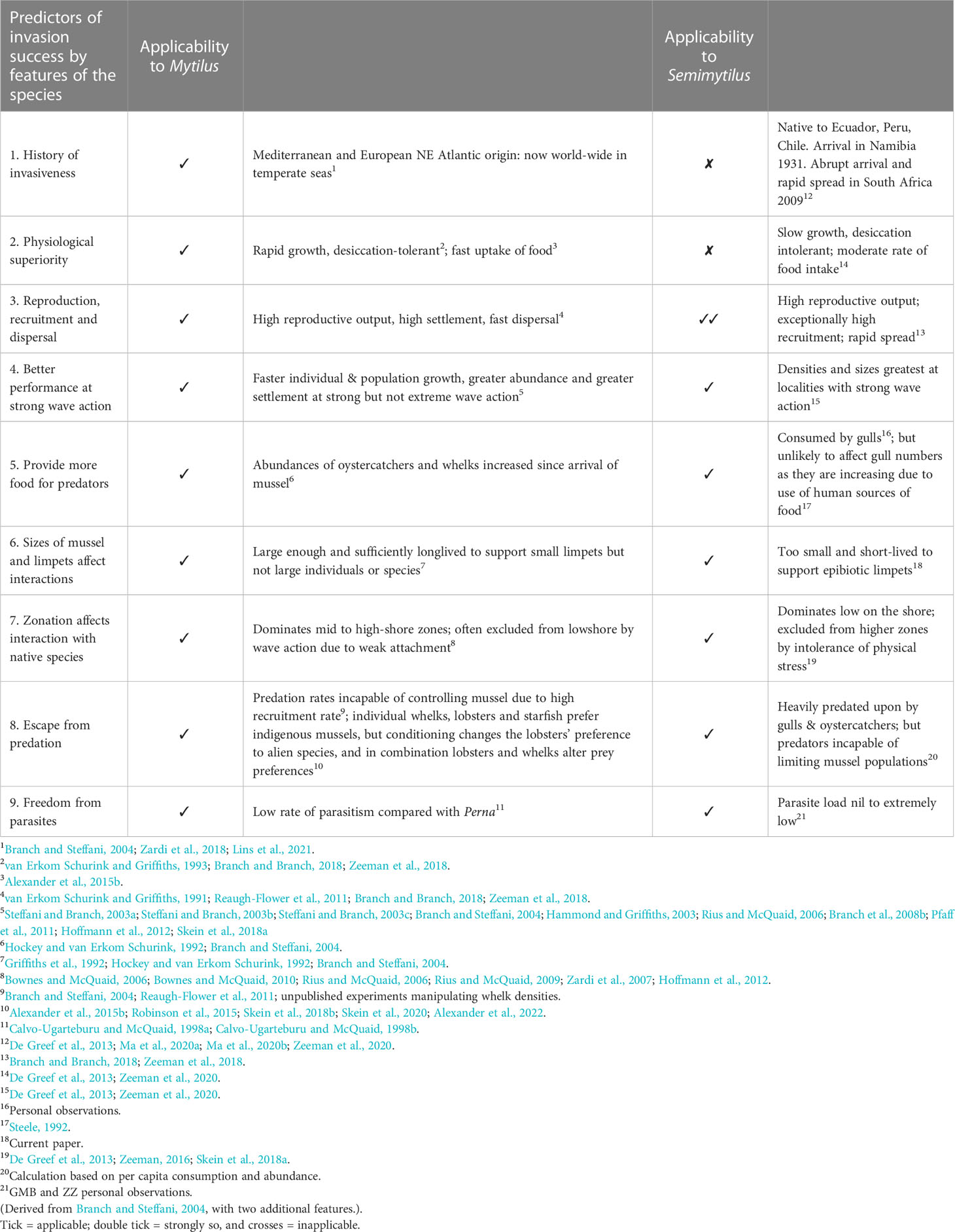
Table 2 Efficacy of applying the characteristics of Mytilus galloprovincialis to predict its invasive capabilities and effects as an alien species, compared to their efficacy when applied to Semimytilus patagonicus.
4 Discussion
As McQuaid and Arenas (2009, pp 316-317) note: ‘the effects of invasive species are highly dependent on their ecological context … difficult to predict … idiosyncratic and context-dependent’. Indeed, that was true in our results, with differences emerging even between the two invasive mussels, despite their being members of the same family, Mytilidae. Nevertheless, key principles emerged about the factors influencing the effects of the alien species:
1. The interactions were between organisms with very different trophic needs and lifestyles: sessile phototrophic algae, mobile herbivorous grazers, and sessile filter feeders. Space was the common resource over which competition took place. Space is an absolute requirement (Branch, 1984) and if it is insufficient, exclusion of subordinate competitors is likely. However, offsetting this, intertidal organisms can provide secondary space for epibionts even if they dominate primary space.
2. Size matters. Occupation of secondary space on shells depends on the relative size of the host and the epibiont.
3. Abundance powerfully affects competitive capability, and is influenced by settlement and recruitment rates, which can vary temporally and spatially, often with considerable vagaries (Underwood and Fairweather, 1989).
4. Ecological traits have proved valid predictors of the competitive advantages and invasive capabilities of M. galloprovincialis (Branch and Steffani, 2004). These include tolerance to thermal stress and desiccation, which can set differential vertical zonation patterns and potentially lead to spatial segregation that ameliorates competition. Differential adaptations to wave action and upwelling may determine horizontal differences in abundance, again moderating competition. In the concluding section of this discussion, we revisit the conclusions of Branch and Steffani (2004) to inquire how many of the successful predictions made about the invasive capability of M. galloprovincialis are applicable to S. patagonicus.
We explore these perspectives below, in the context of the four experimental and comparative cases we examined.
4.1 Interactions among limpets, mussels and algae
The capacity of limpets to control algal growth is amply demonstrated by a plethora of limpet removal experiments (see references in the introduction). In addition, evidence arises from marine protects areas (MPAs) in which protection from harvesting has increased limpet abundances and consequently decreased algal abundance (Micheli et al., 2005; Martins et al., 2010; Baliwe et al., 2022). In inaccessible areas such as islands, limpet numbers are often greater than in accessible mainland localities, increasing food supplies to predators such as oystercatchers which, in turn, reduce the numbers of limpets and confine them to vertical surfaces out of reach (Hockey and Branch, 1983; Branch, 1985; Branch et al., 1987; Lindberg et al., 1998). The effects of limpets on macroalgae cascade downwards to encrusting algae, which benefit because they would otherwise be overgrown (Steneck, 1982), and their speed of lateral growth – and, hence, ability to occupy space – is increased by grazing (Vanmari and Maneveldt, 2019). The ripple effects of grazers such as limpets thus extend up and down interaction chains.
Whereas the influence of S. granularis on macroalgal growth was predictable, the effects of algae on mussels were not. Others have reported that overgrowth by algae has detrimental effects on mussels, reducing growth and reproductive output (Dittman and Robles, 1991), density (Albrecht and Reise, 1994), productivity (Metri et al., 2002) and survivorship (O’Connor et al., 2006).
Our research on the three-way interaction between limpets, mussels and algae showed conclusively that M. galloprovincialis benefitted from the presence of limpets on their shells, improving their body condition and survival. The nearest parallel to our study is one by Miyamoto and Noda (2004), who tested the effects of grazers (two Lottia spp. and two littorinids) on algae growing on mussels. Unlike our study, they concluded that grazers had only minimal effects on the growth of algae. Grazing effects on host mussels are thus not universal.
Because M. galloprovincialis is relatively large, its shells provide secondary habitat for limpets, but limpets may use this only if they are sufficiently small. Miyamoto and Noda (2004) recorded densities of small (6-14 mm) Lottia spp. were 20 times greater on the mussel Septifer virgatus than on bare rock. Small (mostly juvenile, 3-25 mm) S. granularis reached much higher densities on mussels that on bare rock; but larger individuals (adults 25-65 mm) were excluded from the mussel beds and limited to bare rock, as previously reported (Griffiths et al., 1992; Hockey and van Erkom Schurink, 1992; Branch et al., 2008b).
While densities of S. granularis rise significantly on mussel beds, mean sizes are reduced. Individuals of < 0.8 g wet somatic mass (ca. 10-24 mm shell length) are pre-reproductive. At larger sizes, reproductive output per annum increases linearly with somatic mass and exponentially with shell length (Branch, 1974a; Branch, 1974b). Our results (Figure 2) show that taking into account densities and sizes and translating these to reproductive output yields two interesting facts. Firstly, reproductive output for the population (g yr-1 m-2) is about 30% greater in mussel beds than that for limpets living on bare rock, with the greater densities of limpets in mussels more than offsetting their smaller sizes there. Secondly, and in contrast, from an evolutionary, life-time perspective, each individual limpet will achieve a much greater output – around seven times – if it spends its life on rock rather than in mussels, because it will achieve a much greater maximum size and greater longevity. This approximation is of course simplistic in that it assumes a given limpet will spend its whole life on mussels or on rock: but it is not unrealistic, given that the extensive cover of M. galloprovincialis in many parts of the west coast of South Africa (Supplementary Figure S1) leaves them no option but to occupy mussel shells, and the fact that prior to the arrival of this mussel (and in areas where it is sparse or absent), S. granularis spends its entire life on bare rock (Griffiths et al., 1992; Hockey and van Erkom Schurink, 1992).
Mytilus galloprovincialis has much worse effects on another co-occurring indigenous South African limpet, Scutellastra argenvillei, which is much larger (up to 95 mm) and matures sexually at about 47 mm. Dense mussel beds reduce its density, survivorship and homing fidelity (Ruiz Sebastián et al., 2002), confining it to small patches (Supplementary Figure S3), and no S. argenvillei ever reaches sexual maturity on mussel shells (Steffani and Branch, 2005).
Wave action does, however, moderate the effects of M. galloprovincialis on S. argenvillei. Abundance of M. galloprovincialis peaks at strong (but not extreme) levels of wave action, where food supply for the mussel is optimal (Bustamante and Branch, 1996); and the densities, sizes and reproductive potential of S. argenvillei are substantially reduced under those conditions. In contrast, at moderate levels of wave action the mussel settles and grows at lower rates, and the two species co-exist with minimal effects on each other (Steffani and Branch, 2003b; Steffani and Branch, 2003c; Branch and Steffani, 2004). Both the size of S. argenvillei and the intensity of wave action thus influence the outcome.
Various other factors influence the magnitude, speed and even direction of such interactions among mussels, barnacles, algae and limpet including geographic differences related to physical stress (Arrontes et al., 2004; Jenkins et al., 2005; Coleman et al., 2006; Crowe et al., 2011) and differences in mode of feeding. Not all limpets are conventional roam-and-scrape feeders. Some defend algal patches or garden specific algae (Stimson, 1973; Branch, 1975; Branch et al., 1992; McQuaid and Froneman, 1993), or trap drift algae (Bustamante et al., 1995a; Bustamante and Branch, 1996), and their effects on algal growth will be substantially less. The effects of limpet grazing on algae also depend on algal productivity. In New Zealand, limpet grazing has substantial effects at low-nutrient sites but not at upwelling sites (Guerry and Menge, 2017). Our study at Groenriver was, however, conducted in an area of active upwelling (Andrews and Hutchings, 1980) and high algal productivity (Bustamante et al., 1995b), yet the effects of limpet exclusion were decisive.
4.2 Barnacles, limpets and periwinkles
Our results show that the barnacle B. glandula has negative effects on S. granularis but positive effects on littorinids. There are many other examples of negative barnacle-limpet interactions (Dayton, 1971; Branch, 1976; Hawkins, 1983; Dungan, 1986; Santini et al., 2019; Ellrich et al., 2020), but in New South Wales, Creese (1982) showed that a small species of limpet, Patelloida latistrigata, benefits from the presence of barnacles that provide a refuge from competition with a larger species of limpet, Cellana tramoserica, which is excluded because it cannot move over and feed effectively on barnacles. Again, size matters.
The effects of B. glandula on S. granularis are particularly severe for two reasons. First, indigenous barnacles are generally scarce on the west coast of South Africa (Boland, 1997). In particular, there is a large gap in distribution of the native high-shore Chthamalus dentatus between Namibia and the south coast of South Africa (with genetic differences between the two populations of C. dentatus suggesting they are separate species; Motro et al., 2023). This gap is now filled by B. glandula. Second, B. glandula predominates in the high shore where there was previously a void in occupation of the shore by barnacles. Our demonstrations that the barnacle B. glandula has negative effects on S. granularis but positive effects on littorinids, support previous findings by respectively Griffiths et al. (1992) and Laird and Griffiths (2008).
4.3 Effects of alien species on zonation of S. granularis
The successive advents of M. galloprovincialis, S. patagonicus and B. glandula have radically altered the zonation patterns of S. granularis. Prior to their arrival, S. granularis was recognized as a typical member of a group of ‘migratory’ limpets that Branch (1975; 1976) distinguished on the grounds that they settle low on the shore and then migrate progressively upwards as they age, in contrast to ‘non-migratory’ species that remain in the same zone as they settle throughout their lives. Table 1A summarises the features of migratory and non-migratory southern African species of patellid limpets. Hobday (1995) has demonstrated for the limpet Lottia digitalis that this zonation pattern is maintained by migration. Arrival of M. galloprovincialis changed those patterns (Table 1B). Most notably, recruits and juveniles of S. granularis became concentrated in beds of M. galloprovincialis and then shifted both up and down the shore with an increase in size (and age). The advent of S. patagonicus and B. glandula brought further changes. Low on the shore where S. patagonicus predominates, S. granularis is virtually excluded and no individuals over 8 mm recorded were recorded there. Upwards migration of S. granularis from the (diminished) recruitment beds of M. galloprovincialis into the top zone did restore a semblance of the size-zonation pattern originally present, but high densities of B. glandula in the high zones largely exclude the limpet. Both S. patagonicus and B. glandula constitute a poor substratum for the limpet: the former because of its small size and short life, which make it an unsuitable host, and the latter because its rough texture makes attachment and feeding problematic – both difficulties that Creese (1982) suggests large limpets will experience among barnacles.
4.4 Predictors of the invasive success of S. patagonicus
Ros et al. (2023) bemoan that little research has compared whether the ecosystem impacts of alien species are related to their life-history traits. Based on comparisons of such traits among South African mussels, Branch and Steffani (2004) advanced seven predictors of whether the characteristics of M. galloprovincialis influence its capacity to invade. With subsequent research, including the results reported in this paper, it is possible to extend the list of predictors for M. galloprovincialis to nine (Table 2), and to then use those traits to explore the extent to which they have proven valid predictors of the invasions recorded for S. patagonicus.
The almost world-wide invasion of temperate waters by M. galloprovincialis made it an obvious predictor that it would invade South Africa (Table 2, item 1). It was not, however a useful predictor of the arrival of S. patagonicus, which was first described from material in Argentina (d´Orbigny, 1842, p 647), but has not been recorded there since (Signorelli and Pastorino, 2021), apart from transient records of it on fishing-boat hulls in 2013 (Bigatti et al., 2014). Thus, there was no evidence of it invading other parts of the world before its arrival in Namibia in 1931. Its subsequent spread in that country, abrupt and dense settlement in South Africa in 2009 and recent rapid range expansion there (Zeeman et al., 2018; Ma et al., 2020a; Ma et al., 2020b) were therefore not events predictable by any history of invasions elsewhere. Likewise, the physiological superiority exhibited by M. galloprovincialis in terms of its rapid growth, desiccation tolerance, shell strength and food uptake rate (Alexander et al., 2015b; Zeeman et al., 2018) associated with its invasion capabilities was not evident in S. patagonicus (Table 2, item 2).
The most successful predictor of the capacity of S. patagonicus to invade and spread in Namibia and South Africa was its astounding rate of recruitment (Table 2, item 3), reaching around 2-5 million recruits m-2 in the low shore, orders of magnitude greater than that of any of the indigenous mussels (Reaugh-Flower et al., 2011). The remaining predictors of invasion capability (Table 2, items 4-9) serve as well for M. galloprovincialis as for S. patagonicus, both of which reach greatest densities (and maximal effects) on shores with strong wave action, and provide additional food for predators, although none of the known predators provide any predator-driven resistance to invasion by S. patagonicus (Robinson et al., 2015; Skein et al., 2020). The brief lifespan, high densities and particularly the small size of S. patagonicus are firm predictors of its almost complete exclusion of limpets. This accords with other limpet-mussel interactions in South Africa. For example, the relatively tiny (20-30-mm) Scutellastra aphanes lives almost its entire live on shells of Perna perna (Robson, 1986) whereas the large (80-100 mm) S. argenvillei is largely excluded by dense beds of M. galloprovincialis (Steffani and Branch, 2003a; Steffani and Branch, 2003b; Steffani and Branch, 2003c; Branch and Steffani, 2004).
Skein et al. (2021) have broadened the consideration of characteristics that influence the invasion success of alien species to include features of both prey and predator. For prey, they list gregarious behaviour, high fecundity, high recruitment, dispersal potential and refuge from predation; for predators, preference for alien over native species, high feeding rate and high abundance feature. All the features listed for prey are applicable to M. galloprovincialis, S. patagonicus and B. glandula. High reproductive capacity and high to exceptionally high settlement and recruitment are important features for M. galloprovincialis and S. patagonicus, and Skein et al. (2021) make the point that this may overwhelm any predatory biotic resistance to alien species. The same is true for B. glandula (Robinson et al., 2015). In short, although there are several know predators of the two alien mussels, none seem positioned to provide effective biotic resistance to them. Both M. galloprovincialis (Calvo-Ugarteburu and McQuaid, 1998a; Calvo-Ugarteburu and McQuaid, 1998b) and S. patagonicus (personal observations) are relatively free of parasites, which is likely to increase their invasive capabilities.
Four factors are, however, likely to ameliorate the effects of the alien mussels and barnacle: wave action, upwelling, zonation and recruitment. All three alien species predominate on wave-exposed shores and are less abundant at upwelling headlands than downstream bays (Pfaff et al., 2011). Their recruitment is diminished on sheltered shores and at upwelling headlands and, correlated with this, adult abundances are reduced (Hoffmann et al., 2012), reducing their competitive capability.
The three alien species are also differentially zoned: B. glandula is concentrated in the high shore (Hoffmann et al., 2012; Supplementary Figure S2), M. galloprovincialis predominates in the mid to high shore, although it can extend to cover the low shore (Hoffmann et al., 2012; De Greef et al., 2013) and S. algosus is strictly a low-shore species (De Greef et al., 2013) that extends well into the subtidal zone (Skein et al., 2018a). However, in combination, S. patagonicus, M. galloprovincialis and B. glandula now exert a ‘zonation squeeze’ that covers all but the highest (top) zone on the shore (Sadchatheeswaran et al., 2015; Supplementary Figure S4).
Finally, recruitment of many marine organisms is patchy, both spatially and temporally (Underwood and Fairweather, 1989). For mussels and barnacles, concentration of settlement and recruitment at bays downstream of upwelling headland and on wave-exposed shore focuses their effects there (Pfaff et al., 2011). More importantly, periodic ‘sweepstake’ settlements take place, with periods of several years between which settlement and recruitment are very low to absent (Reaugh-Flower et al., 2011). Maximal effects are felt when settlement is dense, but between such events mussel and barnacle populations diminish in vertical extent and abundance, as we recorded for M. galloprovincialis between 2001 and 2012.
5 Conclusions
Returning to the hypotheses outlined in the introduction:
1. Grazing by the limpet S granularis did benefit M. galloprovincialis by preventing algal overgrowth, improving survivorship and condition of the mussel. In turn, the mussel supported high densities of small individuals of S. granularis; but excluded large ones.
2. Dense settlements of the alien barnacle B. glandula in the high shore excluded S. granularis but enhanced densities of the periwinkle A. knysnaensis.
3. The previously described progressively upshore migration of S. granularis was disrupted by the arrival of M. galloprovincialis, which concentrated recruits of the limpet in the mid to high shore; and further altered by S. patagonicus and B. glandula, which largely excluded the limpet from respectively the low- and high-shore zones.
4. Factors that predicted the invasive success of M. galloprovincialis were largely applicable to S. patagonicus, with the most important departures being (a) the absence of an invasive history that would have forecast its arrival in Namibia, and thence South Africa; and (b) the slow growth, intolerance of physical stresses and relatively low rate of food uptake in S. patagonicus that are at odds with its successful invasions and spread. Pivotal to its success is its astonishingly high rate of recruitment.
Factors mitigating the negative effects of the alien species were (a) differences in their zonation, response to wave action and upwelling that partially separate species; (b) differences among species in their relative sizes, which determined whether the alien species could provide a secondary substratum for native species; and (c) fluctuations in recruitment that grant respite between periods of heavy settlement of alien species. Generalities did, thus emerge, but each alien was sufficiently different from the others to be unique in its interactions. As Silverberg (2015) says: ‘The main thing about aliens is that they are alien. They feel no responsibility for fulfilling any of your expectations’.
Data availability statement
The raw data supporting the conclusions of this article will be made available by the authors, without undue reservation.
Author contributions
GB conceived and supervised the research, undertook data collection, analysis and writing. NS was responsible for the work on limpet-mussel-algae interaction including the experiments, data collection and revision of that section of the paper. MP and NB were mainly involved with work on changing zonation patterns, including data gathering, analysis and revision of the text. ZZ was responsible for work on Semimytilus and helped revise that section of the paper. All authors contributed to the article and approved the submitted version.
Funding
Funding was received from the Andrew Mellon Foundation; the University of Cape Town and the South African National Research Foundation.
Acknowledgments
SANParks granted access and permission to work on Marcus Island. Janine Dunlop provided assistance with references and Claudio Velasquez, Margo Branch, Saachi Sadchatheeswaran, Tammy Robinson contributed to fieldwork. Guido Patorino updated our information on the status of Semimytilus in South America. All are thanked with appreciation. This paper is dedicated to Professors Alan Southward (1928-2007) and Brian Allanson (1928-2022), both of whom were ‘impatient of administrators’ and ‘devoted much time to encouraging young scientists’ and who were also PhD examiners for the first author eons ago.
Conflict of interest
The authors declare that the research was conducted in the absence of any commercial or financial relationships that could be construed as a potential conflict of interest.
Publisher’s note
All claims expressed in this article are solely those of the authors and do not necessarily represent those of their affiliated organizations, or those of the publisher, the editors and the reviewers. Any product that may be evaluated in this article, or claim that may be made by its manufacturer, is not guaranteed or endorsed by the publisher.
Supplementary material
The Supplementary Material for this article can be found online at: https://www.frontiersin.org/articles/10.3389/fmars.2023.1190456/full#supplementary-material
References
Albrecht A., Reise K. (1994). Effects of Fucus vesiculosus covering intertidal mussel beds in the Wadden Sea. Helgol. Wiss. Meeresunters 48, 243256. doi: 10.1007/bf02367039
Alexander M. E., Adams R., Dick J. T. A., Robinson T. B. (2015b). Forecasting invasions: resource use by mussels informs invasion patterns along the South African coast. Mar. Biol. 162, 2493–2500. doi: 10.1007/s00227-015-2742-5
Alexander M. E., Raven H. J., Robinson T. B. (2015a). Foraging decisions if a native whelk, Trochia cingulata Linnaeus, and the effects of invasive mussels on prey choice. J. Exp. Mar. Biol. Ecol. 470, 26–33. doi: 10.1016/j.jembe.2015.04.023
Alexander M. A., Skein L., Robinson T. B. (2022). Rapid learning in a native predator shifts diet preferences towards invasive prey. Biol. Lett. 18, 20210655. doi: 10.1098/rbl.2021.0655
Andrews W. R. H., Hutchings L. (1980). Upwelling in the Southern Benguela Current. Prog. Oceanogr. 9, 1–81. doi: 10.1016/0079-6611(80)90015-4
Arrontes J., Arena F., Fernandez C., Rico J. M., Oliveros J., Martinez B., et al. (2004). Effects of grazing by limpets on mid-shore species assemblages in northern Spain. Mar. Ecol. Prog. Ser. 277, 117–133. doi: 10.3354/meps277117
Baliwe N. G., Pfaff M. C., Branch G. M. (2022). Assessing the effects of no-take zones in a marine protected area spanning two ecoregions and rock substrate types. Front. Mar. Sci. 9. doi: 10.3389/fmars.2022.893260
Bigatti G., Signorelli J. H., Schwindt E. (2014). Potential invasion of the Atlantic coast of South America by Semimytilus algosus (Gould 1850). BioInvasions Rec. 3, 241–246. doi: 10.3391/bir.2014.3.4.05
Boland J. M. (1997). Is the geographic pattern in the abundance of South African barnacles due to pre-recruitment or post-recruitment factors? S. Afr. J. Mar. Sci. 18, 63–73. doi: 10.2989/025776197784161036
Bownes S. J., McQuaid C. D. (2006). Will the invasive mussel Mytilus galloprovincialis Lamarck replace the indigenous Perna perna L. on the South coast of South Africa? J. Exp. Mar. Biol. Ecol. 338, 14–151. doi: 10.1016/j.jembe.2006.07.006
Bownes S. J., McQuaid C. D. (2010). Mechanisms of habitat segregation between and invasive and an indigenous mussel: settlement, post-settlement mortality and recruitment. Mar. Biol. 156, 991–1006. doi: 10.1007/s00227-009-1143-z
Branch G. M. (1974a). The ecology of Patella Linnaeus from the Cape Peninsula, South Africa. 2. Reproductive cycles. Trans. R. Soc S. Afr. 41, 111–160. doi: 10.1080/00359197409520068
Branch G. M. (1974b). The ecology of Patella Linnaeus from the Cape Peninsula, South Africa. 3. Growth rates. Trans. R. Soc S. Afr. 41, 161–193. doi: 10.1080/00359197409520069
Branch G. M. (1975). Mechanisms reducing intraspecific competition in Patella spp.: migration, differentiation and territorial behaviour. J. Anim. Ecol. 44, 575–600. doi: 10.2307/3612
Branch G. M. (1976). Interspecific competition experienced by South African Patella spp. J. Anim. Ecol. 4, 507–529. doi: 10.2307/3888
Branch G. M. (1981). The biology of limpets: physical factors, energy flow, and ecological interactions. Oceanogr. Mar. Biol. Ann. Rev. 19, 235–380.
Branch G. M. (1984). Competition between marine organisms: ecological and evolutionary implications. Oceanogr. Mar. Biol. Ann. Rev. 22, 429–593. doi: 10.1201/9781482267242-14
Branch G. M. (1985). “Limpets: their role in littoral and sublittoral community dynamics,” in The ecology of rocky coasts. Eds. Moore P. G., Seed R. (London: Hodder and Stoughton), 97–116.
Branch G. M., Barkai A., Hockey P. A. R., Hutchings L. (1987). Biological interactions: causes or effects of variability in the Benguela ecosystem? S. Afr. J. Mar. Sci. 5, 425–445. doi: 10.2989/025776187784522144
Branch G. M., Branch M. L. (2018). Living shores – interacting with southern Africa’s marine ecosystems (Cape Town: Struik Nature).
Branch G. M., Harris J. M., Parkins C., Bustamante R. H., Eekhout S. (1992). “Algal ‘gardening’ by grazers: a comparison of the ecological effects of territorial fish and limpets,” in Plant-animal interactions in the marine benthos. Special Volume, No. 46. Eds. John D. M., Hawkins S. J., Price J. H. (Oxford: Systematics Association Clarendon Press), 405–423.
Branch G. M., Odendaal F., Robinson T. B. (2008b). Long-term monitoring of the arrival, expansion and effects of the alien mussel Mytilus galloprovincialis relative to wave action. Mar. Ecol. Prog. Ser. 370, 171–183. doi: 10.3354/meps07626
Branch G. M., Odendaal F., Robinson T. B. (2010). Competition and facilitation between the alien mussel Mytilus galloprovincialis and indigenous species: moderation by wave action. J. Exp. Mar. Biol. Ecol. 383, 65–78. doi: 10.3354/meps07626
Branch G. M., Steffani C. N. (2004). Can we predict the effects of alien species? a case-history of the invasion of South Africa by Mytilus galloprovincialis (Lamarck). J. Exp. Mar. Biol. Ecol. 300, 189–215. doi: 10.1016/j.jembe.2003.12.007
Branch G. M., Thomson R. C., Crowe T. P., Castilla J. C., Langmead O., Hawkins S. J. (2008a). “Rocky intertidal shores: prognosis for the future,” in Aquatic ecosystems; trends and global prospects. Ed. Polunin N. (Cambridge: Cambridge University Press), 209–225.
Bustamante R. H., Branch G. M. (1996). The dependence of intertidal consumers on kelp-derived organic matter on the west coast of South Africa. J. Exp. Mar. Biol. Ecol. 196, 1–28. doi: 10.1016/0022-0981(95)00093-3
Bustamante R. H., Branch G. M., Eekhout S. (1995a). Maintenance of an exceptional intertidal grazer biomass in South Africa: subsidy by subtidal kelps. Ecology 76, 2314–2329. doi: 10.1016/0022-0981(95)00093-3
Bustamante R. H., Branch G. M., Eekhout S., Robertson B., Zoutendyk P., Schleyer M., et al. (1995b). Gradients of intertidal productivity around the coast of South Africa and their relationships with consumer biomass. Oecologia 102, 189–201. doi: 10.1007/BF00333251
Calvo-Ugarteburu G., McQuaid C. D. (1998a). Parasitism and invasive species: effects of digenetic trematodes on mussels. Mar. Ecol. Prog. Ser. 169, 149–163. doi: 10.3354/meps169149
Calvo-Ugarteburu G., McQuaid C. D. (1998b). Parasitism and introduced species: epidemiology of trematodes in the intertidal mussels Perna perna and Mytilus galloprovincialis. J. Exp. Mar. Biol. Ecol. 220, 47–65. doi: 10.1016/s0022-0981(97)00083-x
Castilla J. C., Lagos N. A., Cerda M. (2004). Marine ecosystem engineering by the alien ascidian Pyura praeputialis on a mid-intertidal rocky shore. Mar. Ecol. Prog. Ser. 268, 119–130. doi: 10.3354/meps268119
Coleman R., Hockey P. A. R. (2008). Effects of an alien invertebrate species and wave action on prey selection by African black oystercatchers (Haematopus moquini). Aust. Ecol. 33, 232–240. doi: 10.1111/j.1442-9993.2008.01864.x
Coleman R. A., Underwood A. J., Benedetti-Cecchi L., Åberg P., Arenas F., Arrontes J., et al. (2006). A continental scale evaluation of the role of limpet grazing on rocky shores. Oecologia 147, 556–564. doi: 10.1007/s00442-005-0296-9
Creese R. G. (1982). Distribution and abundance of the acmaeid limpet Patelloida latistrigata, and its interaction with barnacles. Oecologia 52, 85–96. doi: 10.1007/bf00389010
Crowe T. P., Frost N. J., Hawkins S. J. (2011). Interactive effects of losing key grazers and ecosystem engineers vary with environmental context. Mar. Ecol. Prog. Ser. 430, 223–234. doi: 10.3354/meps09023
d´Orbigny A. D. (1842). Voyage dans l’Amerérique méridionale (le Brazil, la république orientale de l’Uruguay, la république Argentine, la patagonie, la république du chili, la république Bolivia, la république pérou), exécute pendant le annés 1826, 1827, 1928. 1832 et 1833. Eds. Bertrand P., Levrault V. (Paris: Tome cinquème, 3e partie: Mollusques).
Day E., Branch G. M., Viljoen C. (2000). How costly is molluscan shell erosion? A comparison of two limpets with contrasting shell structures. J. Exp. Mar. Biol. Ecol. 243, 185–208. doi: 10.1016/s0022-0981(99)00120-3
Dayton P. K. (1971). Competition, disturbance, and community organisation: the provision and subsequent utilisation of space in a rocky intertidal community. Ecol. Monogr. 41, 351–389. doi: 10.2307/1948498
De Greef K., Griffiths C. L., Zeeman Z. (2013). Déjà vu? a second Mytilus mussel Semimytilus algosus, invades South Africa’s west coast. Afr. J. Mar. Sci. 35, 307–313. doi: 10.2989/1814232X.2013.829789
Dittman D., Robles C. (1991). The effects of algal epiphytes on the mussel Mytilus californianus. Ecology 72, 286–296. doi: 10.2307/1938922
Dungan M. L. (1986). Three-way interactions: barnacles, limpets, and algae in a Sonoran Desert rocky intertidal zone. Am. Nat. 127, 292–316. doi: 10.1086/284486
Dye A. H. (1995). The effects of excluding limpets from the lower balanoid zone of rocky shores in Transkei, South Africa. S. Afr. J. Mar. Sci. 15, 9–15. doi: 10.2989/025776195784156313
Ellrich J. A., Yorisue T., Momota K. (2020). Limpet disturbance effects on barnacle recruitment are related to recruitment density but not recruit size. Peer J 8, e9190. doi: 10.7717/peerj.9190
Erlandsson J., Pal P., McQuaid C. D. (2006). Re-colonisation rate differs between co-existing indigenous and invasive intertidal musselsfollowing major disturbance. Mar. Ecol. Prog. Ser. 320, 169–176. doi: 10.3354/meps320169
Farrell T. M. (1988). Community stability: effects of limpet removal and reintroduction in a rocky intertidal community. Oecologia 75, 190–197. doi: 10.1007/bf00378596
Firth L. (2021). What have limpets ever done for us? On the past and present provisioning and cultural services of limpets. Int. Rev. Environ. History 7, 5–45. doi: 10.22459/ireh.07.02.2021.01
Grant W. S., Cherry M. I. (1985). Mytilus galloprovincialis Lmk. in southern Africa. J. Exp. Mar. Biol. Ecol. 90, 179–191. doi: 10.1016/0022-0981(85)90119-4
Griffiths C. L., Hockey P. A., Van Erkom Schurink C., Le Roux P. J. (1992). Marine invasive aliens on South African shores: implications for community structure and trophic functioning. S. Afr. J. Mar. Sci. 12, 713–722. doi: 10.2989/02577619209504736
Griffiths C. L., Mead A., Robinson T. B. (2010). A brief history of marine bio-invasions in South Africa. Afr. Zool. 44, 241–247. doi: 10.3377/004.044.0212
Griffiths C. L., Robinson T. B., Mead A. (2009). “The status and distribution of marine alien species in South Africa,” in Biological invasions in marine ecosystems, vol. 204. Eds. Rilov G., Crooks J. A. (Heidelberg: Springer-Verlag), 393–408.
Guerry A. D., Menge B. A. (2017). Grazer impacts on algal community structure vary with the coastal upwelling regime. J. Exp. Mar. Biol. Ecol. 48, 10–23. doi: 10.1016/j.jembe.2016.12.011
Hammond W., Griffiths C. L. (2003). Influence of wave exposure on South African mussel beds and their associated infaunal communities. Mar. Biol. 144, 547–552. doi: 10.1007/s00227-003-1210-9
Hampton S. L., Griffiths C. L. (2007). Why Carcinus maenas cannot get a grip on South Africa’s wave-exposed shores. Afr. J. Mar. Sci. 29, 123–126. doi: 10.2989/AJMS.2007.29.1.11.76
Hanekom N. (2007). Invasion of an indigenous Perna perna mussel bed on the south coast of South Africa by an alien mussel Mytilus galloprovincialis and its effect on the associated fauna. Biol. Inv. 10, 233–244. doi: 10.1007/s10530-007-9125-x
Hartnoll R. G., Hawkins S. J. (1985). Patchiness and fluctuations on moderately exposed shores. Ophelia 24, 53–63. doi: 10.1080/00785236.1985.10426619
Hawkins S. G. (1981). The influence of Patella grazing on the fucoid/barnacle mosaic on moderately exposed rocky shores. Kieler Meerersforsch. Sonderh. 5, 537–543.
Hawkins S. J. (1983). Interactions of Patella and macroalgae with settling Semibalanus balanoides (L.). J. Exp. Mar. Biol. Ecol. 71, 55–72. doi: 10.1016/0022-0981(83)90104-1
Hawkins S. J., Hartnoll R. G. (1983). Grazing of intertidal algae by marine invertebrates. Oceanogr. Mar. Biol. Ann. Rev. 21, 195–282.
Hobday A. (1995). Body-size variation exhibited by an intertidal limpet: influence of wave exposure, tidal height and migratory behavior. J. Exp. Mar. Biol. Ecol. 18, 29–45. doi: 10.1016/0022-0981(95)00009-g
Hockey P. A. R., Branch G. M. (1983). Oystercatchers and limpets: impact and implications a preliminary assessment. Ardea 72, 199–206.
Hockey P. A. R., van Erkom Schurink C. (1992). The invasive biology of the mussel Mytilus galloprovincialis on the southern African coast. Trans. R. Soc S. Afr. 48, 123–139. doi: 10.1080/00359199209520258
Hoffmann V., Pfaff M. C., Branch G. M. (2012). Spatio-temporal patterns of larval supply and settlement of intertidal invertebrates reflect a combination of passive transport and larval behaviour. J. Exp. Mar. Biol. Ecol. 418–419, 83–90. doi: 10.1016/j.jembe.2012.03.008
Jenkins S. R., Coleman R. A., Santina P. D., Hawkins S. J., Burrows M. T., Hartnoll R. G. (2005). Regional scale differences in the determinism of grazing effects in the rocky intertidal. Mar. Ecol. Prog. Ser. 287, 77–86. doi: 10.3354/meps287077
Jenkins S. R., Hawkins S. J., Norton T. A. (1999). Direct and indirect effects of a macroagal canopy and limpet grazing in structuring a sheltered inter-tidal community. Mar. Ecol. Prog. Ser. 188, 81–92. doi: 10.3354/meps188081
Jenkins S. R., Laird M. C., Griffiths C. L. (2008). Present distribution and abundance of the introduced barnacle Balanus glandula Darwin in South Africa. Afr. J. Mar. Sci. 30, 93–100. doi: 10.2989/ajms.2008.30.1.9.459
Jennsen G. S. (2015). Sidespace: aurora renegrades. Book one (Hauppauge, New York: Hypernova Publishing).
Laird M. C., Griffiths C. L. (2008). Present distribution and abundance of the introduced barnacle Balanus glandula Darwin in South Africa. Afr. J. Mar. Sci. 30, 93–100. doi: 10.2989/ajms.2008.30.1.9.459
Lathlean J. A., Seuront L., McQuaid C. D., Ng T. P. T., Zardi G. I., Nicastro K. R. (2016). Cheating the locals: invasive mussels steal and benefit from the cooling effects of indigenous mussels. PloS One 11 (3), e0152556. doi: 10.1371/journal.pone.0152556
Lindberg D. R. (2007). Reproduction, ecology, and evolution of the Indo-Pacific limpet Scutellatra flexuosa. Bull. Mar. Sci. 81, 2019–2234.
Lindberg D. R., Estes J. A., Warheit. K. I. (1998). Human influences on trophic cascades along rocky shores. Ecol. Applic. 8, 880–890. doi: 10.1890/1051-0761(1998)008[0880:hiotca]2.0.co;2
Lindegarth M., Åberg P., Cervin G., Nilsson P. G. (2001). Effects of grazing on the structure of mid-shore, intertidal assemblages on moderately exposed rocky shores of the Swedish west coast. Mar. Ecol. Prog. Ser. 212, 29–38. doi: 10.3354/meps212029
Lins D. M., Zbawicka M., Wenne R., Pocwirz-Kotus A., Molina J. R. A., Alves L. P., et al. (2021). Ecology and genetics of Mytilus galloprovincialis: threat to bivalve aquaculture in southern Brazil. Aquaculture 540, 73653. doi: 10.1016/j.aquaculture.2021.736753
Little C., Trowbridge C. D., Pilling G. M., Stirling P., Morritt D., Williams G. A. (2017). Long-term fluctuations in intertidal communities in an Irish sea-lough: limpet-fucoid cycles. Estuar. Coast. Shelf Sci. 196, 70–82. doi: 10.1016/j.ecss.2017.06.036
Lodge S. M. (1948). Algal growth in the absence of Patella on an experimental strip of foreshore, Port St. Mary, Isle of Man. Proc. Trans. Liverpool Biol. Soc 56, 78–85.
Ma K. C. K., McQuaid C. D., Pulfrich A., Robinson T. B. (2020b). Status of a decennial marine invasion by the biexual mussel Semimytilus algosus (Gould 1850) in South Africa. Afr. J. Mar. Sci. 42, 507–515. doi: 10.2989/1814232x.2020.1820376
Ma K. C. K., Monsinjon J. R., Froneman P. W., McQuaid C. V. D. (2023). Thermal stress gradient causes inctreasingly negative effects towards the range limit of an invasive mussel. Sci. Tot. Env. 865, 161184. doi: 10.1016/j.scitotenv.2022.161184
Ma K. C. K., Zardi G. I., McQuaid C. D., Nicastro K. R. (2020a). Historical and contemporary range expansion of an invasive mussel, Semimytilus algosus, in Angola and Namibia despite data scarcity in an infrequently surveyed region. PloS One 15 (9), e0239167. doi: 10.1371/journal/pone.0239167
Maneveldt G. W., Eager R. C., Bassier A. (2009). Effects of long-term exclusion of the limpet Cymbula oculus (Born) on the distribution of intertidal organisms on a rocky shore. Afr. J. Mar. Sci. 31, 171–179. doi: 10.2989/ajms.2009.31.2.5.877
Martins G. M., Thompson R. C., Neto A. I., Hawkins S. J., Jenkins S. R. (2010). Exploitation of intertidal grazers as a driver of community divergence. J. Appl. Ecol. 47, 1282–1289. doi: 10.1111/j.1365-2664.2010.01876.x
McQuaid C. D., Arenas F. (2009). “Biological invasions: insights from marine benthic communities,” in Marine hard bottom communities, vol. 206. Ed. Whal M. (Berlin Heidelberg: Springer-Verlag), 309–320. doi: 10.1007/b76710_22
McQuaid C. D., Froneman P. W. (1993). Mutualism between the territorial intertidal limpet Patella longicosta and the crustose alga Ralfsia verrucosa. Oecologia 96, 128–133. doi: 10.1007/bf00318040
Mead A., Carlton J. T., Griffiths C. L., Rius M. (2011a). Introduced and cryptic marine and estuarine species in South Africa. J. Nat. Hist. 45, 2463–2524. doi: 10.1080/00222933.2011.595836
Mead A., Carlton J. T., Griffiths C. L., Rius M. (2011b). Revealing the scale of marine bio-invasions in developing regions: a South African re-assessment. Biol. Inv. 13, 1991–2008. doi: 10.1007/s10530-011-0016-9
Menge B. A., Foley M. M., Pamplin J., Murphy G., Pennington C. (2010). Supply-side ecology, barnacle recruitment, and rocky intertidal community dynamics: do settlement surface and limpet disturbance matter? J. Exp. Mar. Biol. Ecol. 392, 160–175. doi: 10.1016/j.jembe.2010.04.032
Metri R., da Rocha R. M., Marenzi A. (2002). Epibiosis reduction on productivity in a mussel culture of Perna (Linné, 1758). Braz. Arch. Bio. Technol. 45, 325–331. doi: 10.1590/s1516-89132002000300011
Micheli F., Benedetti-Cecchi L., Gambaccini S., Bertocci I., Borsini C., Osio G. C., et al. (2005). Cascading human impacts, marine protected areas, and the structure of Mediterranean reef assemblages. Ecol. Monogr. 75, 81–102. doi: 10.1890/03-4058
Miyamoto Y., Noda T. (2004). Effects of mussels on competitively inferior species: competitive exclusion to facilitation. Mar. Ecol. Prog. Ser. 276, 293–298. doi: 10.3354/meps276293
Motro U., Simon-Blecher N., Frumin S., Achituv Y. (2023). Brewed in the African pot: the phylogeography of the toothed barnacle Chthamalus dentatus (Chthamaloidea: Chthamalidae). Mar. Biol. Res. doi: 10.1080/17451000.2023.2193899
Nicastro K. R., Zardi G. I., McQuaid C. D. (2007). Behavioural response of invasive Mytilus galloprovincialis and indigenous Perna perna mussels exposed to risk of predation. Mar. Ecol. Prog. Ser. 336, 169–175. doi: 10.3354/meps336169
Nicastro K. R., Zardi G. I., McQuaid C. D. (2008). Movement behaviour and mortality in invasive and indigenous mussels: resilience and resistance strategies at different spatial scales. Mar. Ecol. Prog. Ser. 372, 119–126. doi: 10.3354/meps07671
Nicastro K. R., Zardi G. I., McQuaid C. D. (2010a). The role of gaping behaviour in habitat partitioning between coexisting intertidal mussels. BMC Ecol. 10, 17. doi: 10.1186/1472-6785-10-17
Nicastro K. R., Zardi G. I., McQuaid C. D. (2010b). Differential reproductive investment, attachment strength and mortality of invasive and indigenous mussels across heterogeneous environments. Biol. Inv. 12, 2165–2177. doi: 10.1007/s10530-009-9619-9
O’Connor N. E., Crowe T. P., McGrath D. (2006). Effects of epibiotic algae on survival, biomass and recruitment of mussels, Mytilus L. (Bivalvia: Mollusca). J. Exp. Mar. Biol. Ecol. 328, 265–276. doi: 10.1016/j.jembe.2005.07.013
Pfaff M. C., Branch G. M., Wieters E. A., Branch R. A., Broitman B. R. (2011). Upwelling intensity and wave exposure determine recruitment of intertidal mussels and barnacles in the Southern Benguela upwelling region. Mar. Ecol. Prog. Ser. 425, 141–152. doi: 10.3354/meps09003
Phillips N. E., Hutchison E. (2008). Grazer effects on algal assemblages and mussel recruitment in two different mid-intertidal communities in the Cook Strait, New Zealand. N. Z. J. Mar. Freshw. Res. 42, 297–306. doi: 10.1080/00288330809509957
Pyšek P., Hulme P. E., Simberloff D., Bacher S., Blackburn T. M., Carlton J. T., et al. (2020). Scientists’ warning on invasive alien species. Biol. Rev. 95, 1511–1534. doi: 10.1111/brv.12627
Quinn G. P., Keough M. J. (2002). Experimental design and data analysis for biologists (Cambridge: Cambridge University Press).
R Core Team. (2019). R: a language and environment for statistical computing (Vienna, Austria: R Foundation for Statistical Computing). Available at: https://www.R-project.org/.
Reaugh-Flower K., Branch G. M., Harris J. M., McQuaid C. D., Currie B., Dye A., et al. (2011). Scale-dependent patterns and processes of intertidal mussel recruitment around southern Africa. Mar. Ecol. Prog. Ser. 434, 101–119. doi: 10.3354/meps09169
Rius M., McQuaid C. D. (2006). Wave action and competitive interactions between the invasive mussel Mytilus galloprovincialis and the indigenous Perna perna in South Africa. Mar. Biol. 150, 69–78. doi: 10.1007/s00227-006-0322-4
Rius M., McQuaid C. D. (2009). Facilitation and competition between invasive and indigenous mussels over a gradient of physical stress. Basic Appl. Ecol. 10, 607–613. doi: 10.1016/j.baae.2009.03.008
Robinson T. B., Alexander M. E., Simon C. A., Griffiths C. L., Peters K., Sibanda S., et al. (2016). Lost in translation? standardising the terminology used in marine invasion biology and updating South African alien species lists. Afr. J. Mar. Sci. 38, 129–140. doi: 10.2989/1814232X.2016.1163292
Robinson T. B., Branch G. M., Griffiths C. L., Govender A., Hockey P. A. R. (2007). Changes in South African rocky intertidal invertebrate community structure associated with the invasion of the mussel Mytilus galloprovincialis. Mar. Ecol. Prog. Ser. 340, 163–171. doi: 10.2989/18142320509504088
Robinson T. B., Griffiths C. L., McQuaid C., Rius M. (2005). Marine alien species of South Africa-status and impacts. Afr. J. Mar. Sci. 27, 297–306. doi: 10.2989/18142320509504088
Robinson T. B., Peters K., Brooker B. (2020). “Coastal invasions: the South African context,” in Biological invasions in South Africa, vol. 14. Eds. Van Wilgen B. W., Measey J., Richardson D. M., Wilson J. R. (Berlin/Heidelberg, Germany: Springer), 229–249. doi: 10.1007/978-3-030-32394-3_9
Robinson T. B., Pope H. R., Hawken L., Binneman C. (2015). Predation-driven biotic resistance fails to restrict the spread of a sessile rocky shore invader. Mar. Ecol. Prog. Ser. 522, 169–179. doi: 10.3354/meps11167
Robson G. (1986). A new species of South African limpet, Patella aphanes, (Mollusca: Gastropoda: Patellidae), with a discussion of P. obtecta. Durban Museum Novitates 13 (22), 305–320.
Ros M., Ashton G. V., Cabezas M. P., Cacabelos E., Canning-Clode J., Carlton J. T., et al. (2023). “Marine bioinvasions in the anthropocene: challenges and opportunities,” in Coastal habitat conservation. Ed. Espinosa F. (London: Academic Press), 81–110.
Ruiz Sebastián C., Steffani C. N., Branch G. M. (2002). Homing and movement patterns of a South African limpet Scutellastra argenvillei in an area invaded by an alien mussel Mytilus galloprovincialis. Mar. Ecol. Prog. Ser. 243, 111–122. doi: 10.3354/meps243111
Sadchatheeswaran S., Branch G. M., Robinson T. B. (2015). Changes in habitat complexity resulting from sequential invasions of a rocky shore: implications for community structure. Biol. Invasions 17, 1799–1816. doi: 10.1007/s10530-014-0837-4
Sadchatheeswaran S., Branch G. M., Robinson T. B. (2018). Impacts of alien ‘ecosystem engineers’ overwhelm interannual and seasonal shifts in rocky-shore community composition on Marcus Island, South Africa. Afr. J. Mar. Sci. 40, 137–147. doi: 10.2989/1814232X.2018.1462729
Santini G., Thompson R. C., Jenkins S. R., Chelazzi G., Hartnoll R. G., Kay S. M., et al. (2019). Barnacle cover modifies foraging behabiour of the intertidal limpet Patella vulgata. J. Mar. Biol. Ass. U. K. 99, 1779–1786. doi: 10.1017/s0025315419000778
Signorelli J., Pastorino G. (2021). Semimytilus patagonicus (d´Orbigny 1842) (Bivalvia: Mytilidae) revealed as the valid name with priority over Semimytilus algosus (Gould 1850). Arch. Molluskenkd. 150 (1), 55–63. doi: 10.1127/arch.moll/150/055-063
Silverberg R. (2015). “Hannibal’s elephants,” in Dark city lights: New York short stories. Ed. Block L. (Three Rooms Press: New York).
Simon-Blecher N., Granevitze Z., Achituv Y. (2008). Balanus glandula: from North-West America to the west coast of South Africa. Afr. J. Mar. Sci. 30, 85–92. doi: 10.2989/ajms.2008.30.1.8.458
Skein L., Alexander M., Robinson T. (2018b). Contrasting invasion patterns in intertidal and subtidal mussel communities. Afr. Zool. 53, 1–6. doi: 10.1080/15627020.2018.1448420
Skein L., Alexander M. E., Robinson T. B. (2020). Co-occurring predators increase biotic resistance against invasive prey. Mar. Env. Res. 157, 104929. doi: 10.1016/j.marenvres.2020.104929
Skein L., Alexander M. E., Robinson T. B. (2021). Characteristics of native predators are more important than those of alien prey in determining the success of biotic resistance in marine systems. Aquat. Ecol. 55, 97–106. doi: 10.1007/s10452-020-09814-5
Skein L., Robinson T. B., Alexander M. E. (2018a). Impacts of mussel invasions on the prey preferences of two native predators. Behav. Ecol. 29, 353–359. doi: 10.1093/beheco/arx172
Southward A. J. (1964). Limpet grazing and the control of vegetation on rocky shores (Blackwell Scientific: Oxford).
Steele W. K. (1992). Diet of Hartlaub’s gull Larus hartlaubii and the kelp gull L. dominicanus in the southwestern Cape Province, South Africa. Ostrich 63, 68–82. doi: 10.1080/00306525.1992.9633952
Steffani C. N., Branch G. M. (2003a). Growth rate, condition, and shell shape of Mytilus galloprovincialis: responses to wave exposure. Mar. Ecol. Prog. Ser. 246, 197–209. doi: 10.3354/meps246197
Steffani C. N., Branch G. M. (2003b). Spatial comparisons of populations of an indigenous limpet Scutellastra argenvillei and an alien mussel Mytilus galloprovincialis along a gradient of wave energy. Afr. J. Mar. Sci. 25, 195–212. doi: 10.2989/18142320309504010
Steffani C. N., Branch G. M. (2003c). Temporal changes in an interaction between an indigenous limpet Scutellastra argenvillei and an alien mussel Mytilus galloprovincialis: effects of wave exposure. Afr. J. Mar. Sci. 25, 213–229. doi: 10.2989/18142320309504011
Steffani C. N., Branch G. M. (2005). Mechanisms and consequences of competition between an alien mussel, Mytilus galloprovincialis, and an indigenous limpet, Scutellastra argenvillei. J. Exp. Mar. Biol. Ecol. 317, 127–142. doi: 10.3354/meps246197
Steneck R. S. (1982). A limpet-coralline alga association: adaptations and defenses between a selective herbivore and its prey. Ecology 63, 507–522. doi: 10.2307/1938967
Stimson J. (1973). The role of territory in the ecology of the intertidal limpet Lottia gigantea (Gray). Ecology 54, 1020–1030. doi: 10.2307/1935568
Tejada-Martinez D., López D. N., Bonta C. C., Sepúlveda R. D., Valdivia N. (2016). Positive and negative effects of mesograzers on early-colonizing species in an intertidal rocky-shore community. Ecol. Evol. 6, 5761–5770. doi: 10.1002/ece3.2323
Thompson R. C., Crowe T. P., Hawkins S. J. (2002). Rocky intertidal communities: past environmental changes, present status and predictions for the next 25 years. Environ. Conserv. 29, 168–191. doi: 10.1017/s0376892902000115
Underwood A. J., Fairweather P. G. (1989). Supply-side ecology and benthic marine assemblages. TREE 4, 16–20. doi: 10.1016/0169-5347(89)90008-6
van Erkom Schurink C., Griffiths C. L. (1991). A comparison of reproductive cycles and reproductive output in four mussel species. Mar. Ecol. Prog. Ser. 76, 123–134. doi: 10.3354/meps076123
van Erkom Schurink C., Griffiths C. L. (1993). Factors affecting the rates of growth in four South African mussel species. Aquaculture 109, 257–273. doi: 10.1016/0044-8486(93)90168-x
Vanmari D., Maneveldt G. W. (2019). Mechanisms of interference and exploitation competition in a guild of encrusting algae along a South African rocky shore. Afr. J. Mar. Sci. 41 (4), 353–359. doi: 10.2989/1814232X.2019.1666738
Van Wilgen N. J., Faulkner K. T., Robinson. T. B., South J., Beckett H., Janion-Scheepers C. (2023). “Climate change and biological invasions in South Africa,” in Invasive species and climate change, vol. 14. Ed. Ziska L. H. (Oxfordshire, UK: CAB International), 158–202.
von der Meden C. E. O., Porri F., Erlandsson J., McQuaid C. D. (2008). Coastline topography and the distribution of indigenous and invasive mussels. Mar. Ecol. Prog. Ser. 372, 135–145. doi: 10.3354/meps07731
von der Meden C. E. O., Porri F., McQuaid C. D., Faulkner K., Robey J. (2010). Fine-scale ontogenetic shifts in settlement behavour of mussels: changing responses to biofilm and conspecific settler presence in Mytilus galloprovincialis and Perna perna. Mar. Ecol. Prog. Ser. 411, 161–171. doi: 10.3354/meps08642
Xavier B. M., Branch G. M., Wieters E. (2007). Abundance, growth and recruitment of Mytilus galloprovincialis on the west coast of South Africa in relation to upwelling. Mar. Ecol. Prog. Ser. 346, 189–201. doi: 10.3354/meps07007
Zardi G. I., McQuaid C. D., Jacinto R., Lourenço C. R., Serrão E. A., Nicastro K. R. (2018). Re-assessing the origins of the invasive mussel Mytilus galloprovincialis in southern Africa. Mar. Freshw. Res. 69, 607–613. doi: 10.1071/mf17132
Zardi G. I., McQuaid C. D., Nicastro K. R. (2007). Balancing survival and reproduction: seasonality of wave action, attachment strength and reproductive output in indigenous Perna perna and invasive Mytilus galloprovincialis mussels. Mar. Ecol. Prog. Ser. 334, 155–183. doi: 10.3354/meps334155
Zardi G. I., Nicastro A. R., McQuaid C. D., Rius M., Porri F. (2006). Hydrodynamic stress and habitat partitioning between indigenous (Perna perna) and invasive (Mytilus galloprovincialis) mussels: constraints of an evolutionary strategy. Mar. Biol. 150, 79–88. doi: 10.1007/s00227-006-0328-y
Zeeman S. C. F. (2016). Genetics and ecosystem effects of the invasive Semimytilus algosus, on the west coast of South Africa (South Africa: University of Cape Town).
Zeeman Z., Branch G. M., Pillay D. (2018). Comparisons of life-history patterns of the alien invasive Semimytilus algosus and three other mussel species on the west coast of South Africa. Mar. Ecol. Prog. Ser. 607, 113–127. doi: 10.3354/meps12794
Keywords: barnacles, competition, facilitation, invasive, mussels, patellids, algae, rocky shores
Citation: Branch GM, Steffani N, Pfaff MC, Baliwe NG and Zeeman Z (2023) Complex interplays between limpets and alien species in South Africa: multispecies interactions, zonation and size effects. Front. Mar. Sci. 10:1190456. doi: 10.3389/fmars.2023.1190456
Received: 20 March 2023; Accepted: 11 May 2023;
Published: 30 May 2023.
Edited by:
Stephen John Hawkins, University of Southampton, United KingdomReviewed by:
David Schiel, University of Canterbury, New ZealandNatacha Nogueira, Direção Regional do Ambiente e Alterações Climáticas, Governo Regional da Madeira, Portugal
Copyright © 2023 Branch, Steffani, Pfaff, Baliwe and Zeeman. This is an open-access article distributed under the terms of the Creative Commons Attribution License (CC BY). The use, distribution or reproduction in other forums is permitted, provided the original author(s) and the copyright owner(s) are credited and that the original publication in this journal is cited, in accordance with accepted academic practice. No use, distribution or reproduction is permitted which does not comply with these terms.
*Correspondence: George M. Branch, bWJyYW5jaEBtd2ViLmNvLnph