- 1Marine and Environmental Sciences Centre (MARE)/Aquatic Research Network (ARNET), Laboratório de Ciências do Mar, Universidade de Évora, Sines, Portugal
- 2Departamento de Biologia, Universidade de Évora, Évora, Portugal
- 3The Marine Biological Association of the United Kingdom, The Laboratory, Plymouth, United Kingdom
- 4School of Ocean and Earth Science, University of Southampton, Southampton, United Kingdom
- 5School of Biological and Marine Sciences, University of Plymouth, Plymouth, United Kingdom
Limpets are keystone grazers on rocky shores. We studied spatial patterns of four co-occurring patellids (Patella ulyssiponensis, Patella depressa, Patella rustica, Patella vulgata) and one siphonariid (Siphonaria pectinata) across rockpools and their surrounds. Our approach considered each rockpool as a concentric system of three micro-habitats where limpets were censused (species, counts, and size of individuals): inside rockpool, edge zone, and surrounding open-rock. The whole intertidal zone was sampled on six rocky shores with contrasting landscape variability (amount of open-rock and other micro-habitats) in Southwest Portugal. Additionally, open-rock surfaces far away (>25cm) from rockpools were surveyed on the mid-shore. Three groups of predictors (“physical, habitat-composition, and connectivity variables”) were assessed for each rockpool system. Limpets of various sizes of the three most common species—P. ulyssiponensis, P. depressa, and S. pectinata—were found to coexist inside and outside rockpools on all shores, with rockpools extending their vertical distribution upwards. Patella vulgata was rare; P. rustica was absent from rockpools and their edges. Intra-specific connectivity (correlations in density of the same species between adjacent micro-habitats) showed a decreasing gradient with distance from the pool, being sharper for P. ulyssiponensis (a largely rockpool-resident) than for P. depressa and S. pectinata (suggesting their emergence from rockpools to edges and surrounding open-rock). Abundance and size structure of both P. depressa and S. pectinata were similar on mid-shore open-rock surrounding rockpools and far from rockpools. Landscape variability explained distribution of P. depressa (shores with less open-rock had more and smaller limpets). Habitat-composition (assemblages and substrata) was more important than physical predictors in explaining among-rockpool variability of each limpet species. Densities of P. ulyssiponensis and P. depressa inside rockpools were positively associated with cover of coralline algae and negatively associated with sand cover. Less explanatory power was found in predictive models of S. pectinata, suggesting a more opportunistic nature. There was limited evidence of inter- or intra-specific competition of the three species in rockpools and open-rock. Competition between P. ulyssiponensis and P. depressa was most likely in rockpool edges. Rockpool-generated mosaic landscapes are linked by intra-specific connectivity of limpet populations and modulated by interactions among different limpet species.
1 Introduction
Spatial patterns of keystone species and their coexistence are fundamental for understanding organization of benthic communities and informing marine conservation (Tanner et al., 1994; Ortiz et al., 2017). Given the ecological importance of limpets on rocky shores (Branch, 1981; Hawkins and Hartnoll, 1983; Branch et al., 1985; Fretter and Graham, 1994; Hodgson, 1999; Coleman et al., 2006), their spatial distribution has been extensively investigated on several scales worldwide (e.g., Creese, 1980; Williams and Morritt, 1995; Lima et al., 2006; Vermeij, 2017; Broitman et al., 2018). Coexisting limpets may be from the same (e.g., Aguilera et al., 2013; Freitas et al., 2023a; Freitas et al., 2023b) or different (e.g., Creese and Underwood, 1982; Aguilera et al., 2019) phylogenetically related groups, with similar or distinct traits (e.g., Davies, 1970; Wolcott, 1973; Aguilera and Navarrete, 2012). Many studies have documented distribution patterns of sympatric patellogastropods in South Africa (e.g., Branch, 1971; Branch, 1975; Branch, 1976; Branch and Marsh, 1978; Carneiro, 2021) and the North-East Atlantic (e.g., Boaventura et al., 2002a; Boaventura et al., 2002b; Firth and Crowe, 2008; Casal et al., 2018; Oróstica et al., 2020; Freitas et al., 2023a; Freitas et al., 2023b). On North-East Atlantic shores, patellid limpets have long-been recognized as keystone grazers (e.g., Hawkins and Hartnoll, 1983; Jenkins et al., 2005; Coleman et al., 2006; Henriques et al., 2017). Patellids can co-occur with Siphonaria pectinata on Atlantic and Mediterranean rocky shores in South Europe and North Africa (Rivera-Ingraham et al., 2011; Rubal et al., 2013; Vasconcelos et al., 2021; Slama et al., 2022).
Limpet species can co-occur in two contrasting intertidal micro-habitats: freely draining emersed surfaces (open-rock) and depressions retaining water during low tide (rockpools or tidepools) (Firth and Crowe, 2008; Firth et al., 2009; Noël et al., 2009; Seabra et al., 2020). On open-rock, patterns of distribution, abundance, and size structure of populations of individual species of limpets have been traditionally described vertically with tidal level (e.g., Orton, 1929; Lewis, 1954; Blackmore, 1969), horizontally with different wave exposure (e.g., Evans, 1947; Evans, 1957; Thompson, 1979; Thompson, 1980; Ocaña, 2003; Silva et al., 2003), or on larger geographic scales (e.g. Lewis, 1986; Rubal et al., 2015). Local-scale micro-habitat variation also can determine distribution patterns of limpets living outside rockpools, such as mussel clumps (Lewis and Bowman, 1975; Silva et al., 2003), fucoid and barnacle patches (Hartnoll and Hawkins, 1985; Hawkins et al., 1992; Johnson et al., 1997; Burrows and Hawkins, 1998; Jenkins et al., 1999; Moore et al., 2007; Marzinelli et al., 2012), vertical surfaces, overhangs or crevices (Wolcott, 1973; Garrity, 1984; Williams and Morritt, 1995; Gray and Hodgson, 1998; Aguilera and Navarrete, 2011), sunny versus shaded rock (Lima et al., 2016; Seabra et al., 2016), or substrata associated with crypsis by polymorphic species (e.g., Giesel, 1970; Sorensen and Lindberg, 1991). Such studies have demonstrated the role of landscape variability (the mosaic of micro-habitats available on each shore) for the underlying processes determining limpet survival or growth, with consequences for spatial heterogeneity of assemblages (Hawkins et al., 2019). Inside rockpools, high densities of early life-history stages of patellids or siphonariids consistently occur (e.g., Lewis and Bowman, 1975; Bowman, 1981; Ostalé-Valriberas et al., 2018). The role of rockpools as nurseries for coexisting limpets has been experimentally demonstrated across different regions (Delany et al., 1998; Seabra et al., 2020). Rockpools extend the upper limits of Patella ulyssiponensis (Evans, 1947; Thompson, 1979; Firth and Crowe, 2008), possibly due to its association with crustose-coralline algae (hereafter CCA) (Delany et al., 2002; Seabra et al., 2019; Gomes et al., 2022). While this pattern has been described for other low-shore organisms that are susceptible to desiccation (Goss-Custard et al., 1979; Kooistra et al., 1989; Araújo et al., 2006), unequivocal quantitative evidence is still lacking for other limpets (but see Menconi et al., 1999 for crevices influencing vertical distribution of Mediterranean patellids).
Connectivity is likely to occur between limpet populations inhabiting rockpools versus open-rock. These are clearly distinct environments, especially physically when the tide is out and in their algal communities (Metaxas and Scheibling, 1993; Araújo et al., 2006). However, bi-directional movements of individual limpets across the two micro-habitats happen (Delany et al., 2002; Noël et al., 2009). As limpet intra-specific competition occurs inside rockpools (Firth et al., 2009; Firth and Crowe, 2010), coupled with the importance of these micro-habitats as limpet nurseries (Seabra et al., 2020), rockpools may act as sources of limpet emigration to surrounding open-rock areas. Conversely, as limpets with home scars outside rockpools enter rockpools when foraging at high tide (Noël et al., 2009), rockpools could act as feeding grounds or refuges from desiccation or other stresses, attracting limpets from surrounding open-rock. Limpets also establish home scars along the edge of rockpools (Delany et al., 1998). Rockpool edges are interfaces straddling neighboring micro-habitats with different physical and biological characteristics (e.g., insolation, evaporation, algal and microbial food): permanently submerged inside-pool areas and tidally emersed outside-pool areas slightly away from pools. Patterns of limpet population structure have not been previously described for this pool to open-rock transition nor the wider mosaics of micro-habitats created by the presence of pools, likely to be important at emerging landscape scales.
Large variability at small spatial scales occurs among different rockpools due to their physical and biological characteristics, with consequences for biodiversity and ecosystem functioning (Metaxas et al., 1994; Griffin et al., 2010). The influence of rockpool characteristics on species richness, community structure and functioning has been examined (e.g., shore height, Kooistra et al., 1989; surface area, Underwood and Skilleter, 1996; depth, Martins et al., 2007; volume, Wolfe and Harlin, 1988; slope of pool substratum, Firth et al., 2014; presence of sea urchin grazers, Benedetti-Cecchi and Cinelli, 1995; abundance of molluscan grazers, Masterson et al., 2008; topographic-complexity, Schaefer et al., 2023). Moreover, several variables have been identified as important predictors of abundance and dynamics of invertebrate populations in rockpools or in surrounding rock (e.g., geographic location, Souza and Matthews-Cascon, 2019; shore height, Johnson, 2001; rockpool substrata and biota, Carvalho et al., 2021; distance to rockpool edge, Noël et al., 2009; presence of coexisting species of limpets in the same micro-habitat, Firth and Crowe, 2008). However, the potential effects of other rockpool characteristics (e.g., shape, distance to low-water mark) or proxies of connectivity among populations of mobile invertebrates across rockpool edges (e.g., densities of coexisting species of limpets in adjacent micro-habitats) have not been studied yet.
We studied the four limpets of the genus Patella (P. ulyssiponensis, P. depressa, P. rustica, and P. vulgata) and one siphonariid limpet (S. pectinata) that coexist on the continental Portuguese coast. Patterns of distribution, abundance, and/or size structure of these species and underlying driving mechanisms have been examined for open-rock within shore levels, mostly for adults (Boaventura et al., 2002a; Boaventura et al., 2002b; Boaventura et al., 2003; Rubal et al., 2013; Lima et al., 2016; Seabra et al., 2016). The distribution and abundance of juvenile limpets, especially their association with rockpools or patchy micro-habitats at different shore levels plus temporal and spatial variation in recruitment, have also been investigated (Guerra and Gaudencio, 1986; Silva et al., 2003; Seabra et al., 2019; Seabra et al., 2020). Preliminary observations suggest much small-scale variability in spatial patterning of patellid and siphonariid populations across rockpools and their surrounds. Here, we take an integrative view along the continuous gradient of shore height of the intertidal zone, focusing on micro-habitats both inside and outside rockpools and their interfaces creating mosaic landscapes at each tidal level (hereafter “rockpool-generated landscapes”).
Our overall aim was to examine the spatial patterns of co-occurrence of intertidal limpets in rockpool-generated landscapes describing variability of limpet populations within each micro-habitat and connectivity among them. Each species was quantified (number and size of individuals) in the following micro-habitats: inside-rockpools (POOL), on the narrow edge-zone around the rim of rockpools (EDGE), and on open-rock surrounding rockpools (NEAR). Surveys were made over the whole intertidal gradient on six natural rocky shores in the southwest of continental Portugal (Figure 1), to assess diversity, distribution, abundance, and size structure of limpets throughout the distinct micro-habitats and among shores. Additionally focusing only on the mid-shore, areas of open-rock at a minimum distance of 25 cm from any rockpool (FAR) were also surveyed on the same shores, to measure the density of all limpet species-size classes occurring within these areas. Three main objectives were addressed (Table 1). First, (1) we described the distribution of all limpet species present in each micro-habitat on the six shores, and we examined the spatial variation in abundance and size of the most common limpet species at different horizontal scales. Specifically, (1a) we tested how the density and size structure of each of the three most abundant species (P. ulyssiponensis, P. depressa, and S. pectinata) within each of three micro-habitats (POOL, EDGE, and NEAR) varied among shores and within each shore. For these three species, (1b) we also tested if the total density of the same species was correlated between adjacent micro-habitats to assess their intra-specific connectivity across rockpool-generated landscapes. For the two most common species on mid-shore open-rock (P. depressa and S. pectinata), (1c) we compared density and size structure between open-rock surfaces surrounding mid-shore rockpools (MidNEAR) and further away from rockpools (FAR), and assessed whether the pattern of proximity to rockpools (MidNEAR versus FAR) varied among shores of differing landscape. Landscape variability among shores was described in terms of contrasting heterogeneity in the presence of intertidal micro-habitats, by measuring the relative occurrence of open-rock, rockpools, crevices, overhangs, and channels, in mid-shore areas within each shore. Second, (2a) we described any differences with shore height in abundance of each limpet species within each micro-habitat and shore, and (2b) we compared the vertical distribution patterns of the most common species (P. ulyssiponensis, P. depressa, and S. pectinata) between areas inside versus outside rockpools, specifically testing the following hypothesis: inside rockpools (POOL), each species occurs further upshore than its occurrence on the open-rock (NEAR). Third, (3) we described the small-scale (among rockpools) variability in density and size-class structure of individual species (P. ulyssiponensis, P. depressa, and S. pectinata) within each micro-habitat (POOL, EDGE, and NEAR), and we investigated which predictive variables explain most of these spatial patterns. For every species and micro-habitat, we assessed the influence of the following groups of predictors: i) metrics describing size, shape, topography, and position of rockpools and surrounding rock (hereafter “physical variables”); ii) both inorganic (e.g., rock and sand) and biotic (algae and invertebrates) variables describing composition of the substratum coverage and benthic assemblages (hereafter, “habitat-composition variables”—cover of major substrata types and sessile taxa, plus density of other limpet species and molluscan grazers in the same micro-habitat); and iii) “connectivity variables” describing inter-specific influences on connectivity (i.e., density of other limpet species in adjacent micro-habitats).
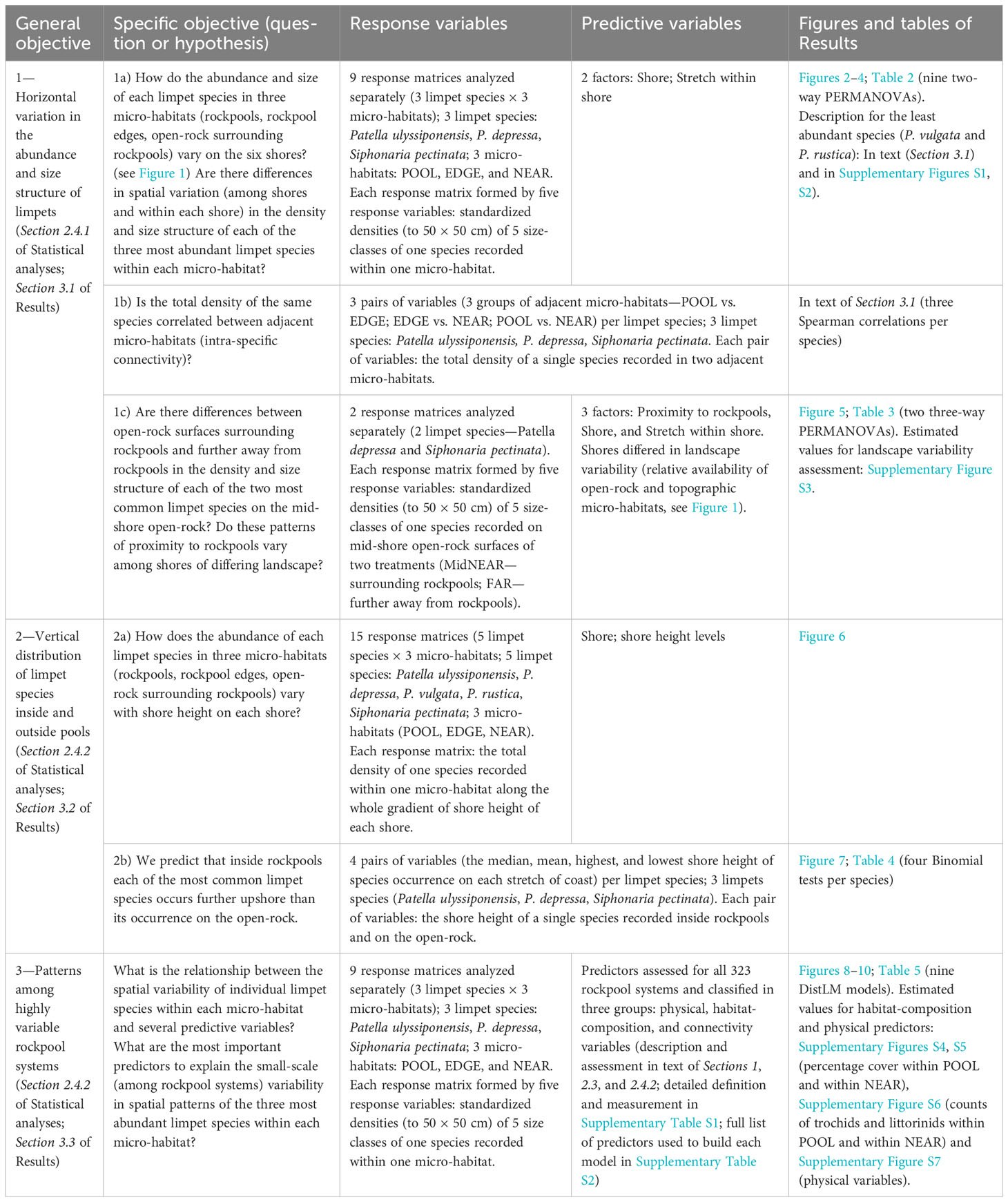
Table 1 Summary-table outlining the general objectives of the present study, specific research questions or hypotheses, corresponding response and predictive variables, and figures and/or tables of Results (including Supplementary Material).
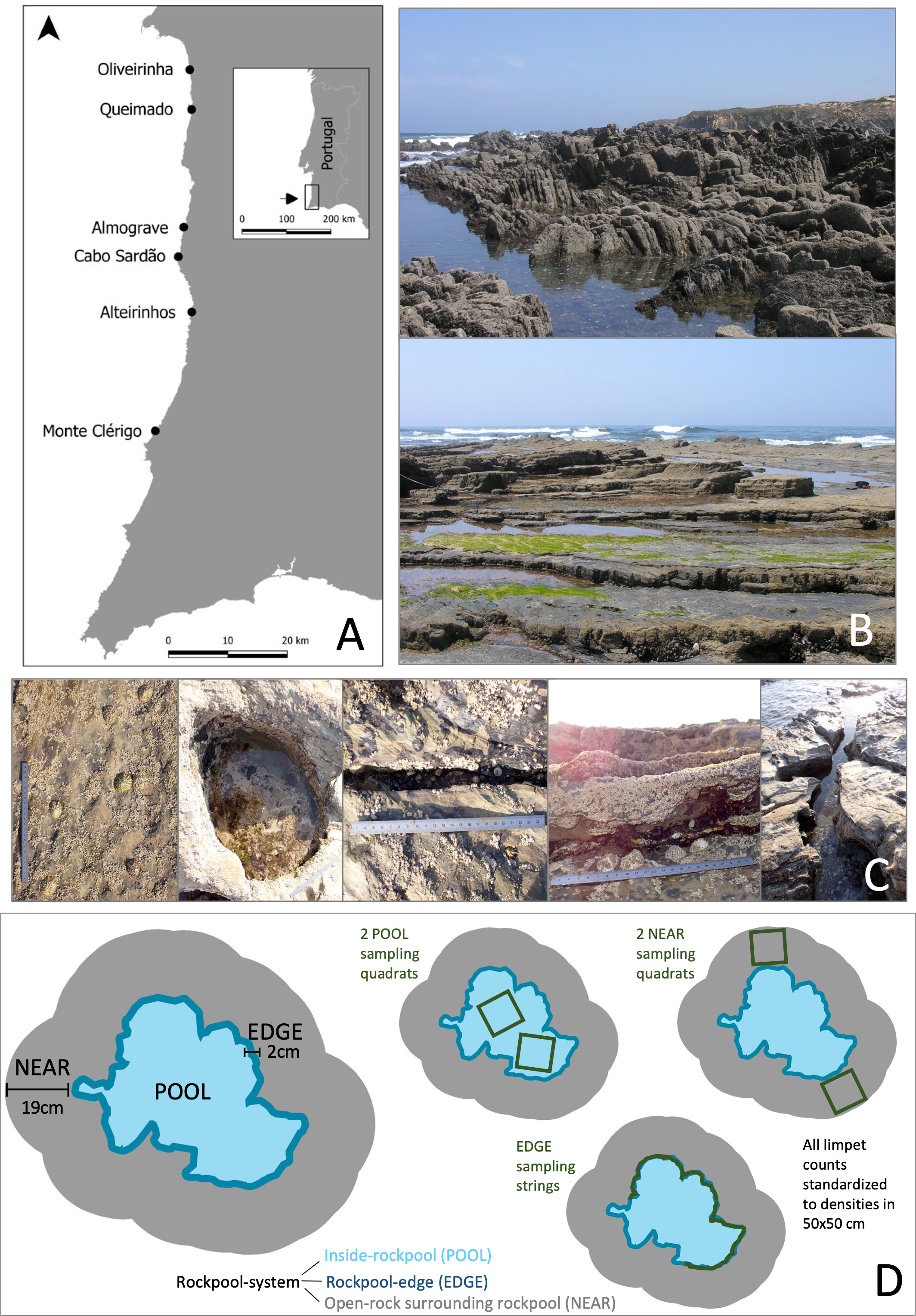
Figure 1 (A) Map of the study region and the six sampled shores; (B) shores varied in geomorphology, namely, in the cleavage orientation of shale strata, from flatter-layered platforms (Oliveiriinha, Queimado, and Monte Clérigo—example of Monte Clérigo on photograph below) to steeper bedrock (Almograve and Cabo Sardão; intermediate orientation in Alteirinhos—example of Almograve on photograph above); (C) five micro-habitat categories considered for landscape variability assessment (open-rock, rockpool, crevice, overhang, and channel); (D) diagram representing a rockpool system, with its three concentric micro-habitats (POOL, EDGE, and NEAR) and respective sampling units (POOL and NEAR quadrats, EDGE strings).
2 Materials and methods
2.1 Study species
On the Portuguese coast, the warm-temperate P. depressa on the mid-shore and P. ulyssiponensis lower down are the most abundant limpet species on open-rock (Guerra and Gaudencio, 1986; Boaventura et al., 2002b), with P. ulyssiponensis occupying pools at higher shore levels (Guerra and Gaudencio, 1986). The less common cold-temperate P. vulgata occurs between low and high shore levels (Cabral and Simões, 2007), mostly found on the lower-mid shore (Boaventura et al., 2002b), frequently in shade or local shelter (Lima et al., 2016). Warm-temperate P. rustica is mostly restricted to steep high-shore levels on exposed shores (Boaventura et al., 2002b; Lima et al., 2006). In SW Portugal, S. pectinata occurs on open-rock and pools across all tidal levels (CIEMAR, 2018; Seabra et al., 2020), being particularly abundant in wave-sheltered sites (CIEMAR, 2018), with much small-scale variation in abundance on mid-shore open-rock (Rubal et al., 2013).
2.2 Study region and shores
Surveys were made in Southwest Portugal (Figure 1A), within the “Parque Natural do Sudoeste Alentejano e Costa Vicentina,” a marine park where the rocky littoral is characterized by high biodiversity (Horta e Costa et al., 2018), and many rockpools. Intertidal limpets (Patella spp. and S. pectinata) are abundant (e.g., Boaventura et al., 2002b); harvesting of patellids is mostly permitted and spatially widespread across the marine park, being considered a regularly exercised but low-intensity activity in terms of frequency and harvest yields with low (P. depressa and P. vulgata) to medium (P. ulyssiponensis) social–economic importance relative to other rocky-intertidal species (Castro et al., 2020 and references therein). Six natural wave-exposed shores were selected from easily accessible locations with extensive reefs along 60 km of coastline (Figure 1A): i) Oliveirinha (37°53′12.38″N, 8°47′47.97″W), ii) Queimado (37°49′35.95″N, 8°47′33.60″W), iii) Almograve (37°38′54.42″N, 8°48′21.96″W), iv) Cabo Sardão (37°36′13.09″N, 8°48′56.91″W), v) Alteirinhos (37°31′12.40″N, 8°47′22.93″W, and vi) Monte Clérigo (37°20′23.34”N, 8°51′25.88”W). All shores were of the dominant rock type on this coast (shale sedimentary/schist metamorphic rock, Boaventura et al., 2002b). Shores differed in cleavage orientation of strata (Figure 1B), from flat-layered platforms (Oliveirinha, Queimado and Monte Clérigo—hereafter “flatter shores”) to steep bedrock (Almograve and Cabo Sardão—hereafter “steeper shores”); Alteirinhos has an intermediate/mixed pattern of flat and steep surfaces. These geomorphological differences drive landscape variability of these shores in terms of heterogeneity of intertidal micro-habitats. The tidal regime is semidiurnal with 3.5 m maximum amplitude.
2.3 Field surveys
2.3.1 Survey design and sampling procedures (landscape variability, limpets, and habitat-composition variables)
Surveys were made during low tides from August to October 2009, on days without rainfall. On each shore, two stretches of 10 m were surveyed. In each stretch, a 10-m chain was deployed along eight transects running sea parallel, haphazardly spread at different distances from low-water mark along the whole shore. In each transect, the micro-habitats present at 21 points spaced at 50-cm intervals along the chain were recorded, by assigning one of the following categories to each point: open-rock, rockpool, channel, crevice, or overhang (Figure 1C). The percentage frequency of occurrence was calculated for each category on each transect. The mean percentages of micro-habitat occurrence over all transects sampled on the mid-shore of the same stretch were then assessed as a measure of landscape variability. From the whole set of rockpools intersected by transects, a minimum of 25 rockpools per stretch were randomly selected and individually marked in the field for sampling (numbered removable epoxy tag on adjacent rock). We sampled 323 rockpools (Oliveirinha, 52; Queimado, 51; Almograve, 57; Cabo Sardão, 56; Alteirinhos, 53; Monte Clérigo, 54).
Each rockpool was treated as a system of three concentric micro-habitats (Figure 1D): i) inside-rockpool (POOL); ii) edge of rockpool (EDGE); and iii) open-rock surrounding the edge (NEAR). POOL was sampled using two equal-sized quadrats deployed on the bottom of each rockpool, encompassing inside-pool surfaces located at least 2cm away from the pool waterline. POOL quadrat-size varied with rockpool size; so that a minimum of 50% of the total surface area of rockpool-bottom was covered by the two sampling quadrats. For each rockpool, the size of the POOL quadrat was selected from eight differently sized quadrats, made from ~1.3-cm grid of plastic wire mesh, with the following areas (respective quadrat-size in number of grid units): 40 cm2 (5×5), 161 cm2 (10×10), 361 cm2 (15×15), 640 cm2 (20×20), 1,005 cm2 (25×25), 1,444 cm2 (30×30), 2,581 cm2 (40×40), and 4,032 cm2 (50×50). EDGE was defined as the thin rim comprising a 2-cm wide band surrounding the pool waterline, including a 1-cm wide marginal inside-pool surface and a 1-cm wide marginal outside-pool surface. EDGE was sampled with strings of 25×2 cm length deployed along the pool waterline, using enough strings to cover a minimum of 50% of the perimeter of each rockpool. NEAR was defined as the adjacent outside-pool area of open-rock, bounded by a halo-line positioned at approximately 2 cm away from the pool waterline and extended to a constant width of 19 cm. NEAR was sampled by two quadrats of 361 cm2 (19×19 cm) randomly deployed within open-rock surfaces surrounding each rockpool. All quadrats and strings were made of flexible material to fit the topography of sampled surfaces. For each variable assessed within POOL or NEAR, a replicate consisted of the mean obtained from the two sampling quadrats of the same micro-habitat of every rockpool system. For variables assessed within EDGE, a replicate consisted of the sum obtained from all strings sampled along the edge of every rockpool. Additionally, mid-shore surfaces of open-rock located at least 25 cm away from any rockpool (FAR) were sampled by six replicate quadrats of 361 cm2 per stretch. For comparison with FAR replicates, a subset of six NEAR replicates per stretch, all located around mid-shore rockpools, was selected during surveys (hereafter “MidNEAR replicates”).
Species identification, allocation to size classes (maximum shell length—MSL, measured with calipers), and counts were made for all limpets within the four micro-habitats (POOL, EDGE, NEAR, and FAR). Five size classes were considered for both Patella spp. (MSL, ≤ 1 cm; 1–2 cm, 2–3 cm, 3–4 cm, and ≥ 4 cm) and S. pectinata (MSL, ≤ 0.5 cm, 0.5–1 cm, 1–2 cm, 2–3 cm, and 3–4 cm). Every individual limpet within the sampling areas was recorded for the respective species-size class, micro-habitat, and individual rockpool system. There were two exceptions: a) juvenile limpets (MSL ≤ 1 cm for patellids and MSL ≤ 0.5 cm for S. pectinata, see Seabra et al., 2020) within POOL were sub-sampled using two quadrats of 40 cm2; and b) limpets with MSL ≤ 0.5 cm were not sampled within EDGE due to the transitional nature of this micro-habitat. All limpet counts were then standardized to densities in 50×50 cm.
Within both POOL and NEAR of every rockpool system, we assessed the following habitat-composition variables (details in Supplementary Table S1): i) the percentage cover of 15 space-occupying categories (rock, sand, Lichinaceae, Verrucariaceae, cyanophytes, crustose non-coralline algae, CCA, articulated coralline algae, seaweed, Porifera, sea anemones, barnacles, mussels, sea urchins, and other sessile invertebrates), i.e., substratum types and functional groups of sessile organisms and ii) counts of two groups of non-limpet mobile grazer gastropods, i.e., trochids (including Steromphala umbilicalis, S. pennanti, Phorcus sauciatus, and P. lineatus) and littorinids (Melarhaphe neritoides), both standardized to densities in 50×50 cm. All taxa were visually identified in the field to the lowest possible taxonomic resolution and then lumped into functional groups of sessile or mobile organisms.
2.3.2 Physical characteristics of rockpools
A set of 19 physical variables was assessed for each of the 323 sampled rockpools, in the field or through image analysis (details in Supplementary Table S1). Each variable corresponds to a quantitative or qualitative metric associated with size (perimeter, surface area, and volume), shape (circularity, roundness, aspect ratio, and curved or straight edges), topography (maximum and mean depth, POOL and NEAR slope, POOL and NEAR topographic complexity, “confinement”), or position (straight and contoured distance to the nearest rockpool, shore height, distance to low-water mark, and “barriers”) of rockpools and/or surrounding rock. “Confinement” was the height of the rocks surrounding the pool (1, pool flushes with surrounding rock; 2, low walls, <3 cm; 3, medium-height walls, 3–6 cm; 4, high walls, 6–10 cm; 5, very-high walls, >10 cm and often with obtuse angles). “Barriers” was presence or absence of outcrops of rock to seaward at <1 m from the pool. Although a few variables applied only to a single micro-habitat (volume, maximum, and mean depth: POOL-only; slope and topographic complexity: separately assessed inside pools and on surrounding rock), most were physical descriptors of each rockpool system across POOL, EDGE, and NEAR (shape and position variables applying to the three micro-habitats; perimeter and surface area of a rockpool are proportional to the ones of its surrounds).
2.4 Statistical analyses
Prior to multivariate analyses described in Sections 2.4.1 and 2.4.3, each response matrix—formed by five response variables, i.e., standardized densities (to 50×50 cm) of the five size classes of a single limpet species recorded on all replicates of a single micro-habitat (hereafter “population size structure and density” or “size-class densities” of an individual species within a micro-habitat)—was transformed (as a pre-treatment of the analysis for a given species-by-micro-habitat combination): i) fourth-root transformation applied to response matrices inside rockpools (POOL); ii) square-root transformation applied to response matrices of the other micro-habitats (EDGE or NEAR or MidNEAR vs. FAR). All multivariate analyses were based on Euclidean distances calculated from transformed size-class densities. All the procedures for multivariate analyses were implemented in PERMANOVA+ for PRIMER package (Anderson et al., 2008), an add-on to PRIMER v7 (Clarke and Gorley, 2015).
2.4.1 Horizontal variation in the abundance and size structure of limpets
Two-way non-parametric multivariate analyses of variance (PERMANOVA, Anderson, 2001) were made separately for each of the three species of limpets (P. ulyssiponensis, P. depressa, and S. pectinata), within each of the three micro-habitats (POOL, EDGE, and NEAR), to test for differences in limpet populations among the six shores and among the two stretches within each shore (shore—fixed factor with six levels; stretch—random factor with two levels, nested in shore; sample size varied between 25 and 30 rockpools per stretch).
To assess intra-specific connectivity, correlations of the total density of the same species between pairs of micro-habitats (POOL and EDGE; EDGE and NEAR; and POOL and NEAR) were made separately for the three species (P. ulyssiponensis, P. depressa, and S. pectinata) using Spearman's coefficient (n = 323 rockpool systems).
Three-way PERMANOVAs were performed separately for each of two species (P. depressa and S. pectinata) to test for differences in limpet populations living on mid-shore open-rock between areas close to and further away from pools, and among shores and stretches (proximity to rockpools—fixed factor with two levels: MidNEAR and FAR; Shore—fixed factor with six levels; Stretch—random factor with two levels, nested in Shore; n = 6). In the case of P. depressa, for which a significant interaction was found between Proximity and Shore, non-metric multidimensional scaling (MDS) was then done separately for each level of factor Proximity (MidNEAR, FAR), to visualize the distance among stretches of all shores and correlation vectors of the response variables (size-class densities of P. depressa). In duplicate MDS plots, vectors of predictors of landscape variability were overlaid (i.e., mean percentages of occurrence of five micro-habitat categories within stretches of every shore).
PERMANOVA tests employed permutation of residuals under a reduced model using 999 permutations and type III (partial) sum of squares. Where differences were detected by PERMANOVA, pair-wise tests determined which levels of each factor differed. The similarity percentages breakdown (SIMPER) procedure (Clarke, 1993) was applied to identify which response variables were the major contributors to the differences between groups detected by pair-wise tests (size classes most responsible for multivariate distances between significantly different factor levels).
2.4.2 Vertical distribution of limpets inside and outside rockpools
Binomial tests (Sokal and Rohlf, 1995) assessed if upward extension occurred in three species of limpets (P. ulyssiponensis, P. depressa, and S. pectinata) inside rockpools (POOL), compared to outside rockpools (NEAR). Data were assembled separately for each species, through the following steps: i) every replicate where the presence of a given species was found was selected from the 323 replicates of both POOL and NEAR; ii) for each of the two micro-habitats, the shore-height records of all selected replicates were considered; iii) from these records, the median, mean, maximum, and minimum values of shore height within each micro-habitat (four pairs of variables per species) were calculated for each sampled stretch of coast (12 stretches, corresponding to two stretches within each of six shores). The general null hypothesis that the median, mean, highest, or lowest shore height of a limpet species occurrence is similar between the two micro-habitats was analyzed by individual binomial tests. In each binomial test, 12 POOL and 12 NEAR values were compared.
2.4.3 Patterns among highly variable rockpool systems
Distance-based linear models (DistLM) were used to examine the relationship between the small-scale variability of individual limpet species inside pools, at the edge of pools or in the surrounding open-rock (size-class densities of a target species within a micro-habitat) and several predictors assessed for each rockpool system (physical, habitat-composition, and connectivity variables). Predictive variables were initially assembled for each target species within each micro-habitat (full list of predictive variables used to build the model for each response matrix in Supplementary Table S2). The total density of every other co-occurring species of limpets were included as follows: i) habitat-composition variables if co-existing with the target species in the same micro-habitat or ii) connectivity variables if estimated in an adjacent micro-habitat. Connectivity variables were coded (e.g., PU_adjacent_Pool) by the abbreviation of species name (P. ulyssiponensis—PU; P. depressa—PD; S. pectinata—SP) followed by “_adjacent_” and the micro-habitat code (Pool; Edge; Near). In the two cases where significant differences were detected among shores by the previously described two-way PERMANOVA tests (Section 2.4.1), specifically for P. depressa within NEAR and for S. pectinata within EDGE, the factor shore was included as an additional predictive variable for DistLM.
After preliminary procedures of elimination and transformation of predictive variables, their selection was made with BEST procedure and based on AIC criterion (methodological details given in Supplementary Text). After obtaining the “BEST final model” for each response matrix, we further selected the “top predictors” as those that contributed most to explain total variation and for which the inclusion in this model added a minimum of 1% in R2. Finally, distance-based redundancy analysis (db-RDA) plots were produced to identify the response variables (size classes) that best described the variability of each target species within each micro-habitat, and their association with the “top predictors.” Correlation vectors of response variables and “top predictors” were overlaid in duplicate db-RDA plots for comparison.
A second DistLM analysis was ran for each species within each micro-habitat, with the “top-predictors” of the “BEST final model” (obtained by the first DistLM analyses) classified in three indicator groups of predictors: physical, habitat-composition, and connectivity variables. We assessed the contribution of each of these groups to the overall explanation of final models.
3 Results
A total of 15,619 limpets were recorded. Within each micro-habitat, the numbers sampled and percentage of each species were as follows: 7,603 limpets within pools (POOL: 54% P. ulyssiponensis, 32% P. depressa, 14% S. pectinata, 0.1% P. vulgata, and 0.6% of non-identified juvenile patellids); 3,472 limpets around pool edges (EDGE: 58% P. depressa, 35% P. ulyssiponensis, 6% S. pectinata, and 1% P. vulgata); 3,771 limpets on open-rock surfaces near to pools (NEAR: 79% P. depressa, 12% P. ulyssiponensis, 6% S. pectinata, 2% P. rustica, and 1% P. vulgata) and 773 limpets on mid-shore open-rock surfaces further away from pools (FAR: 95% P. depressa, 4% S. pectinata, and 1% P. rustica). Patella ulyssiponensis, P. depressa, and S. pectinata were present across POOL, EDGE, and NEAR on all shores (Figures 2–4), while the two other patellids were rare (Supplementary Figures S1, S2).
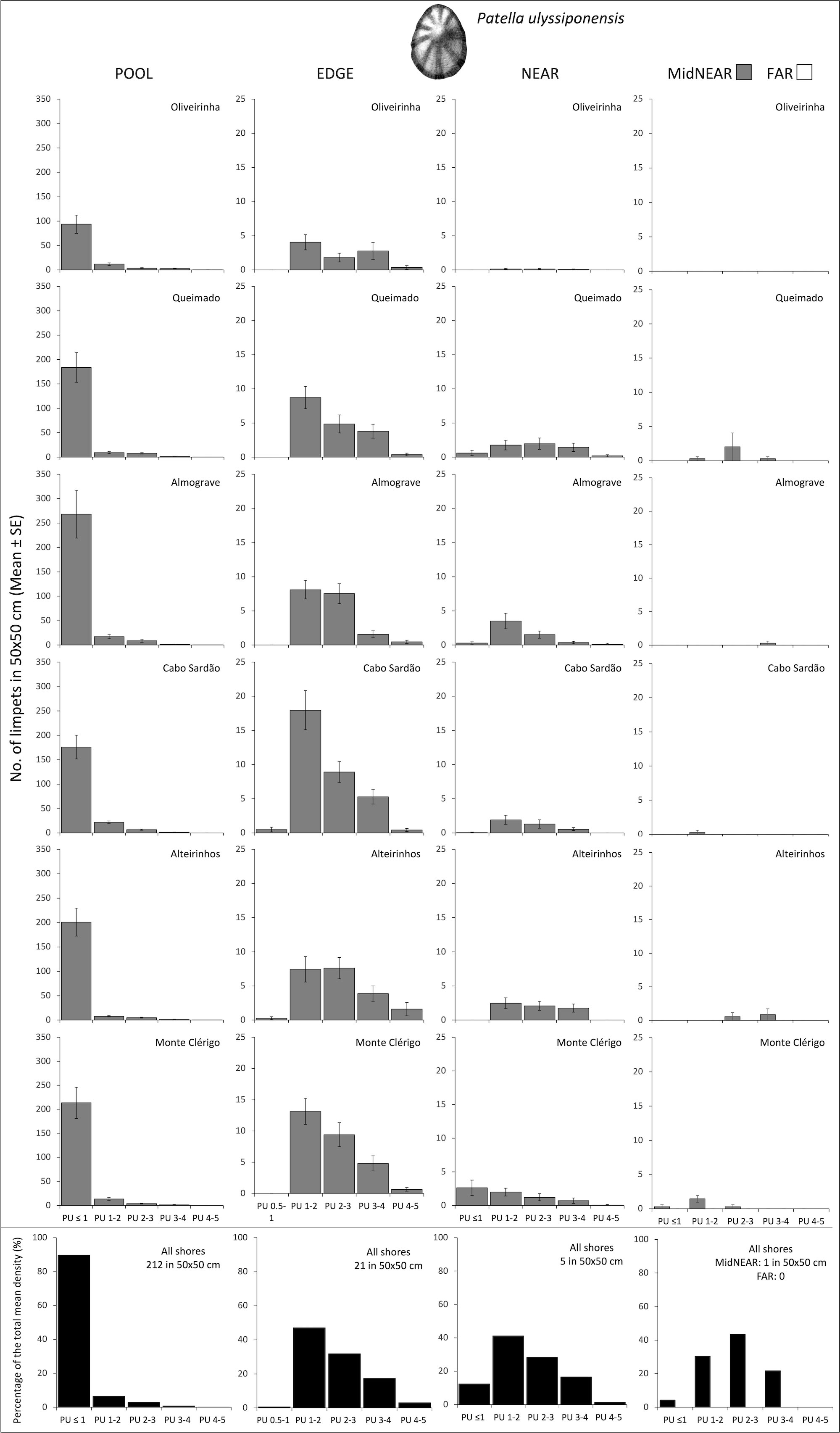
Figure 2 Densities and size-class structure of Patella ulyssiponensis on six shores within POOL (inside rockpools), EDGE (edge of rockpools), and NEAR (open-rock surrounding rockpools) micro-habitats (three left columns); and within areas of mid-shore open-rock at two proximity categories from rockpools (MidNEAR and FAR, far right column). For POOL, EDGE, and NEAR, the sample size in each shore was the following: 52 in Oliveirinha, 51 in Queimado, 57 in Almograve, 56 in Cabo Sardão, 53 in Alteirinhos, and 54 in Monte Clérigo. For MidNEAR and FAR, n = 12 on each shore. Gray bars are mean (± SE) number of limpets of five size classes per 50×50 cm. Note different y-axis scale between graphs of POOL vs. others. Black bars (bottom row) are percentages of every size class relatively to the total density of this species (value given top right of each graph) within each micro-habitat and across all shores. NB P. ulyssiponensis was absent in FAR.
3.1 Horizontal variation in the abundance and size structure of limpets
3.1.1 Patella ulyssiponensis
On every shore, there was a consistent pattern of decreasing abundance of this species from pools to further away: the total mean density of P. ulyssiponensis was 10 times higher in POOL compared to EDGE, four times higher in EDGE compared to NEAR, and null within FAR (Figure 2). Consistently on all shores, nearly 90% of the total mean density within pools corresponded to PU ≤ 1 cm (Figure 2, POOL). Within the surrounding micro-habitats, the modal size class was PU 1–2 cm on most shores (Figure 2, EDGE and NEAR). This species was present on mid-shore open-rock areas near pools on five shores in very low abundances (the highest total mean density of 3 per 50 × 50 cm was recorded in Queimado) with variable size structure among shores (Figure 2, MidNEAR).
Population size structure and density within pools did not differ among shores or stretches within each shore (Table 2, PU within POOL). Within the surrounding micro-habitats, differences were only found between stretches (Table 2, PU within EDGE and NEAR). Correlations of the total density of P. ulyssiponensis between adjacent micro-habitats were all positively significant between POOL and EDGE (rs = 0.495, p<0.001), between EDGE and NEAR (rs = 0.423, p<0.001), and between POOL and NEAR (rs = 0.136, p=0.014).
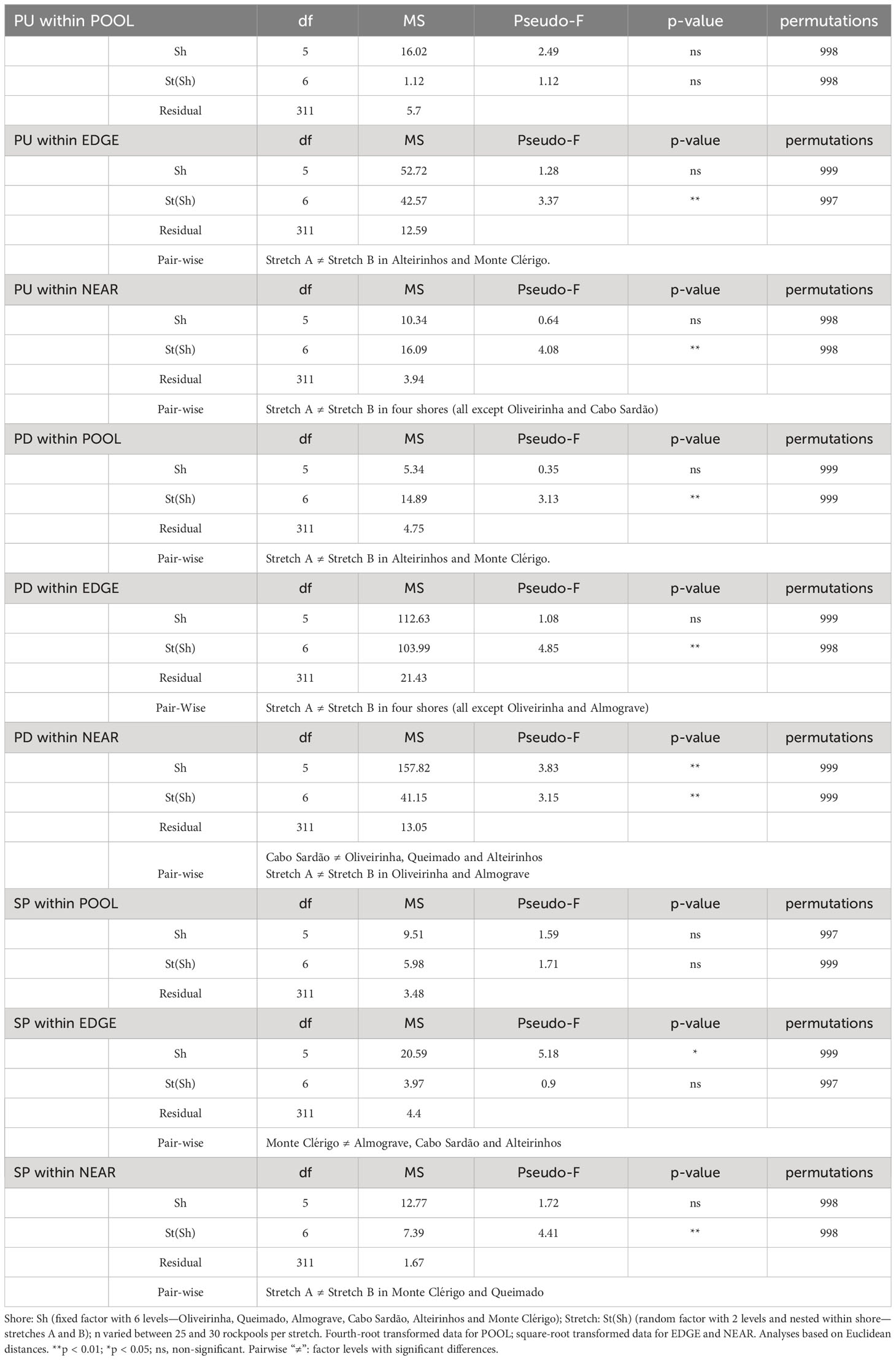
Table 2 Two-way PERMANOVA on the spatial variation (among shores and stretches) of size-class densities of a species (Patella ulyssiponensis—PU, P. depressa—PD and Siphonaria pectinata—SP) within POOL (inside rockpools), EDGE (in the edge of rockpools) and NEAR (on the open-rock surrounding rockpools) micro-habitats.
3.1.2 Patella depressa
On every shore, the abundance of this species was consistently higher inside rockpools compared to the surrounding micro-habitats: the total mean density of P. depressa in POOL was three times higher than in EDGE and five times higher than in NEAR (Figure 3). Inside pools and consistently on all shores, more than 90% of the total mean density corresponded to juveniles (PD ≤ 1cm) (Figure 3, POOL). The modal size class along pool edges was PD 2–3 cm on all shores except Cabo Sardão, where the size structure was dominated by PD 1–2 cm (Figure 3, EDGE). On the open-rock surfaces, the modal size class was either PD 1–2 cm or PD 2–3 cm, the former consistently on the two steeper shores (Almograve and Cabo Sardão) and the latter consistently on the three flatter shores (Figure 3, NEAR, MidNEAR, and FAR).
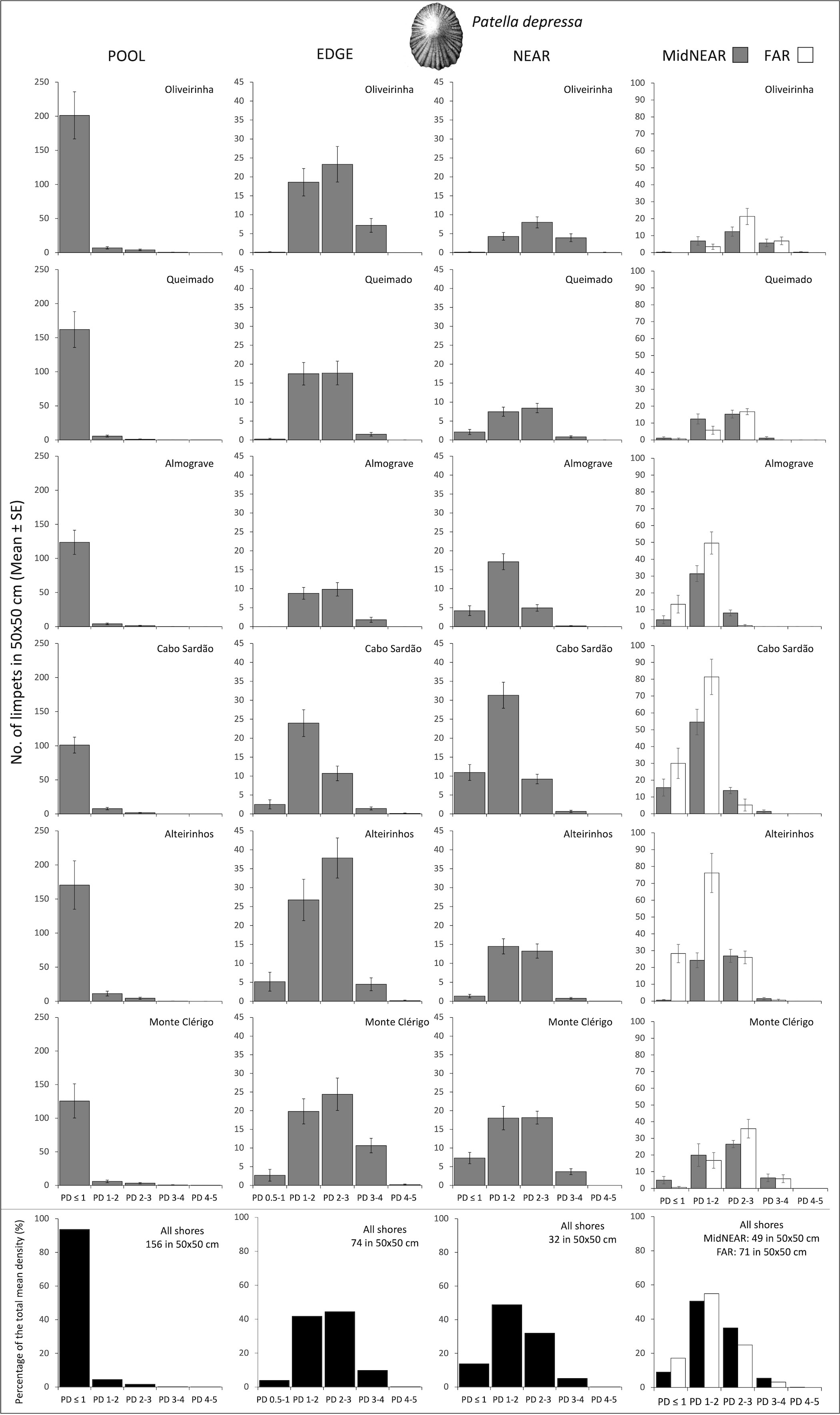
Figure 3 Densities and size-class structure of Patella depressa on six shores within POOL (inside rockpools), EDGE (edge of rockpools), and NEAR (open-rock surrounding rockpools) micro-habitats (three left columns); and within areas of mid-shore open-rock at two proximity categories from rockpools (MidNEAR and FAR, far right column). Gray and white bars are mean (± SE) number of limpets of five size classes per 50×50 cm. Note different y-axis scale: between graphs of POOL vs. others, and between graphs of MidNEAR and FAR vs. others. Sample size indicated in caption of Figure 1. Black and white bars (bottom row) are percentages of every size class relatively to the total density of this species (value given top right of each graph) within each micro-habitat and across all shores.
Population size structure and density inside and around the edge of pools did not differ among shores but were significantly different among stretches within shores (Table 2, PD within POOL and EDGE). On the open-rock near pools, there were significant differences in population size structure and density among shores and among stretches; pair-wise tests detected differences between Cabo Sardão and three other shores (Oliveirinha, Queimado, and Alteirinhos), and between the two stretches of Alteirinhos and Monte Clérigo (Table 2, PD within NEAR). Differences among shores were mostly explained by higher densities of the two smallest size classes (mainly PD 1–2 but also PD ≤ 1) in Cabo Sardão than in the other shores (SIMPER, Figure 3). Cabo Sardão was the shore where the highest abundance was recorded for this species within NEAR (total mean density of 52 limpets per 50×50 cm) (Figure 3). Correlations of the total density of P. depressa between adjacent micro-habitats were positively significant between POOL and EDGE (rs = 0.610, p<0.001) and between EDGE and NEAR (rs = 0.120, p=0.031), but non-significant between POOL and NEAR (rs = 0.076, p=0.173).
Regarding population size structure and density of P. depressa on mid-shore open-rock surfaces at different proximity to rockpools, a significant interaction was found between factors Proximity and Shore, and significant differences were found among stretches at Oliveirinha and Alteirinhos (Table 3). Pair-wise tests on Proximity detected no differences between mid-shores surfaces close to and far from pools on any shore (Table 3, MidNEAR = FAR). Pair-wise tests on Shore revealed a different among-shore pattern for the two categories of Proximity. For mid-shores surfaces near to pools, the most evident pattern of variation was between Cabo Sardão and all shores other than Almograve (Table 3, MidNEAR); this was due to a higher density of PD 1–2 cm and PD ≤ 1 cm in Cabo Sardão (SIMPER, Figures 3, 5A); Cabo Sardão was the shore with the lowest relative proportion of open-rock (Figure 5B) (20% for the average of the two stretches, Supplementary Figure S3). For mid-shores surfaces far from pools, significant differences were found between the three flatter shores and the group of two steeper and one intermediate shores (Table 3, FAR); this reflected a higher density of PD 1–2 cm and PD ≤ 1 cm in the group formed by steeper and intermediate shores (Almograve, Cabo Sardão, and Alteirinhos) (SIMPER, Figures 3, 5C); these shores had more crevices and less open-rock (Figure 5D), their relative proportions of crevices (33%) and open-rock (23%) being four times higher and two times lower on average compared to the ones recorded in flatter shores (8% and 49%, respectively) (Supplementary Figure S3).
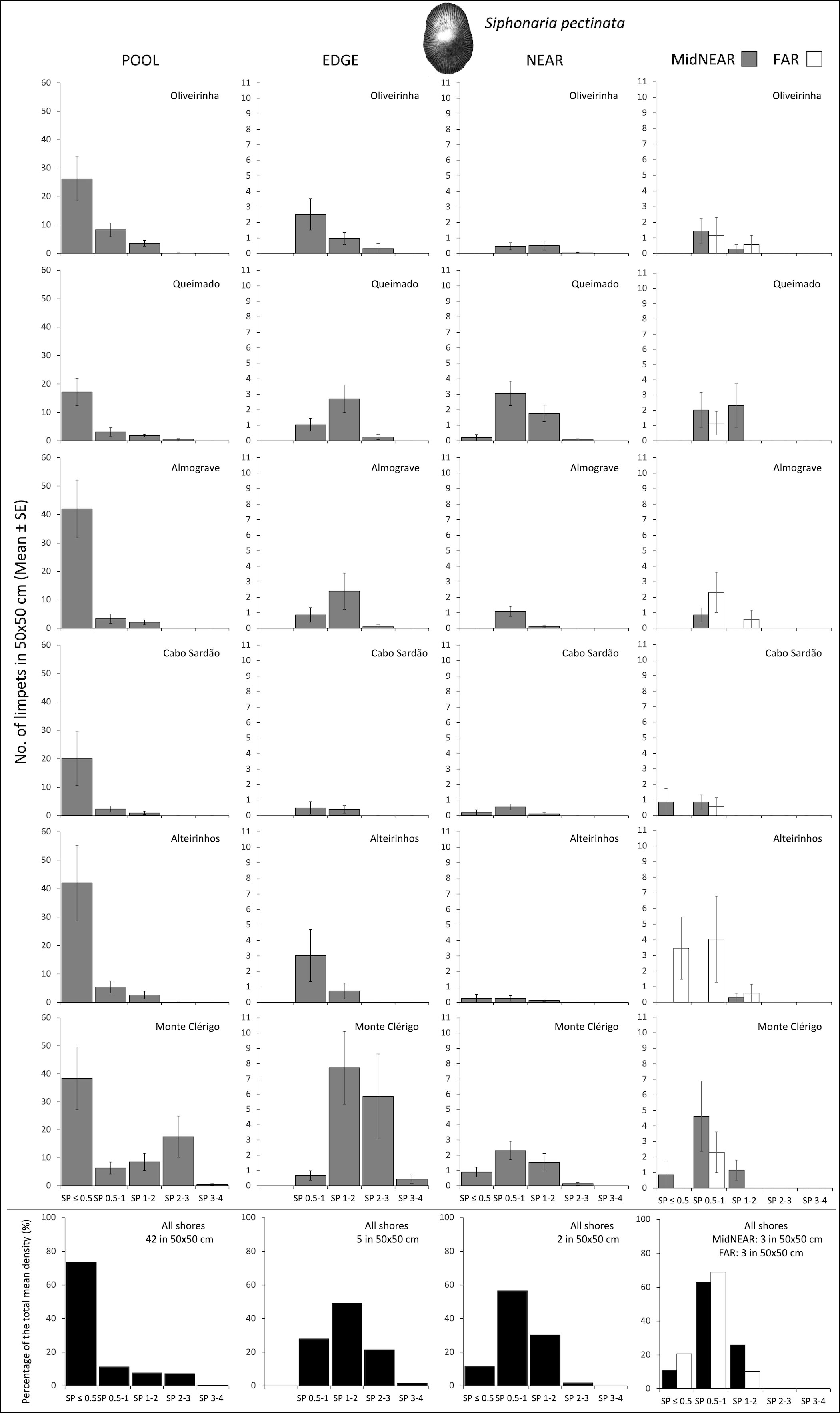
Figure 4 Densities and size-class structure of Siphonaria pectinata on six shores within POOL (inside rockpools), EDGE (edge of rockpools), and NEAR (open-rock surrounding rockpools) micro-habitats (three left columns); and within areas of mid-shore open-rock at two proximity categories from rockpools (MidNEAR and FAR, far right column). Gray and white bars are mean (± SE) number of limpets of five size classes per 50×50 cm. Note different y-axis scale between graphs of POOL vs. others; sample size indicated in caption of Figure 1. Black and white bars (bottom row) are percentages of every size class relatively to the total density of this species (value given top right of each graph) within each micro-habitat and across all shores.
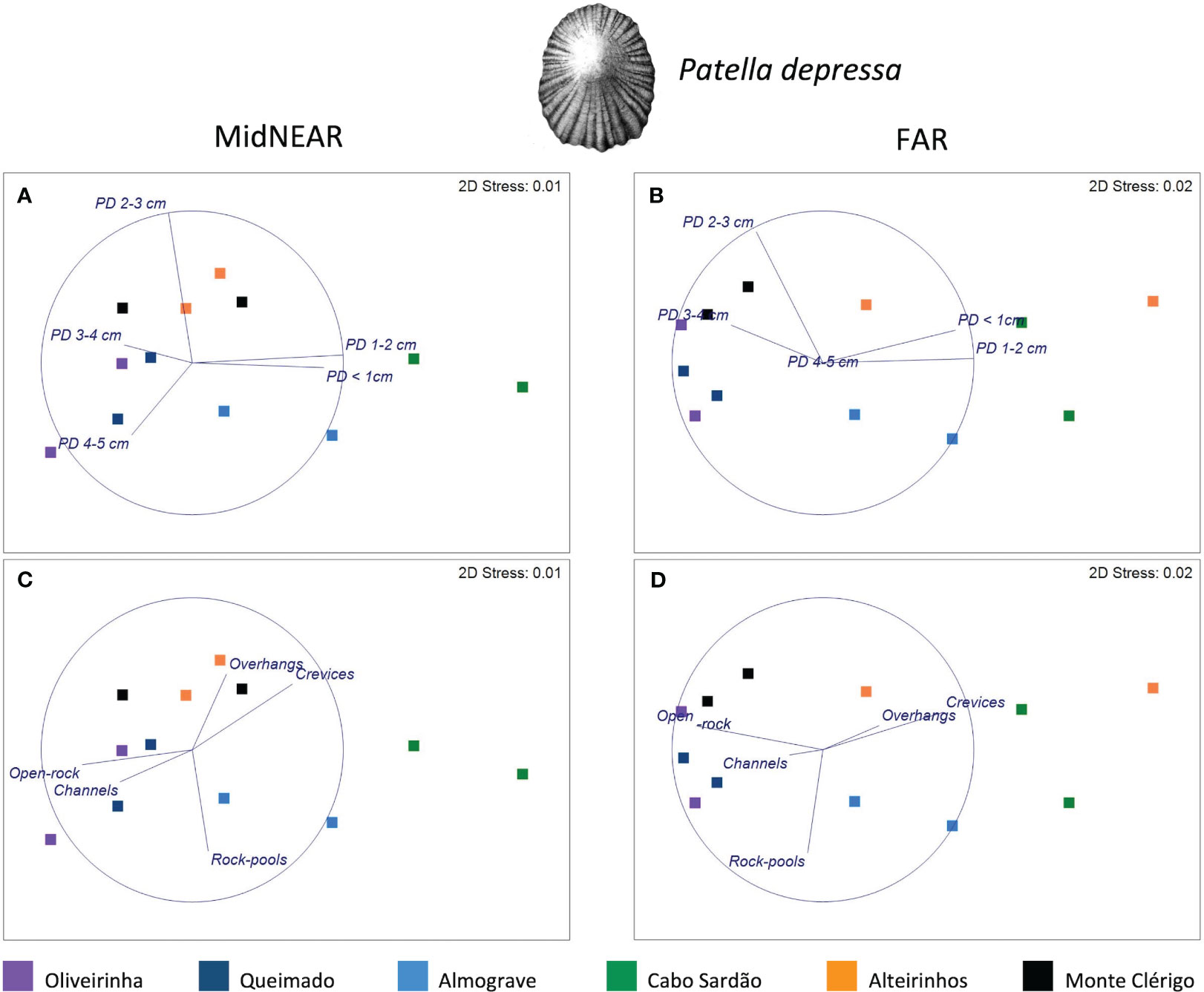
Figure 5 MDS plots for Patella depressa within areas of mid-shore open-rock at two proximity categories from rockpools: MidNEAR (A, B) and FAR (C, D). Overlaid vectors of the response variables, i.e., densities of five size classes (A, C); overlaid vectors of predictors of landscape variability, i.e., proportions of open-rock and topographic micro-habitats assessed on the mid-shore of two coastal stretches on every shore (B, D).
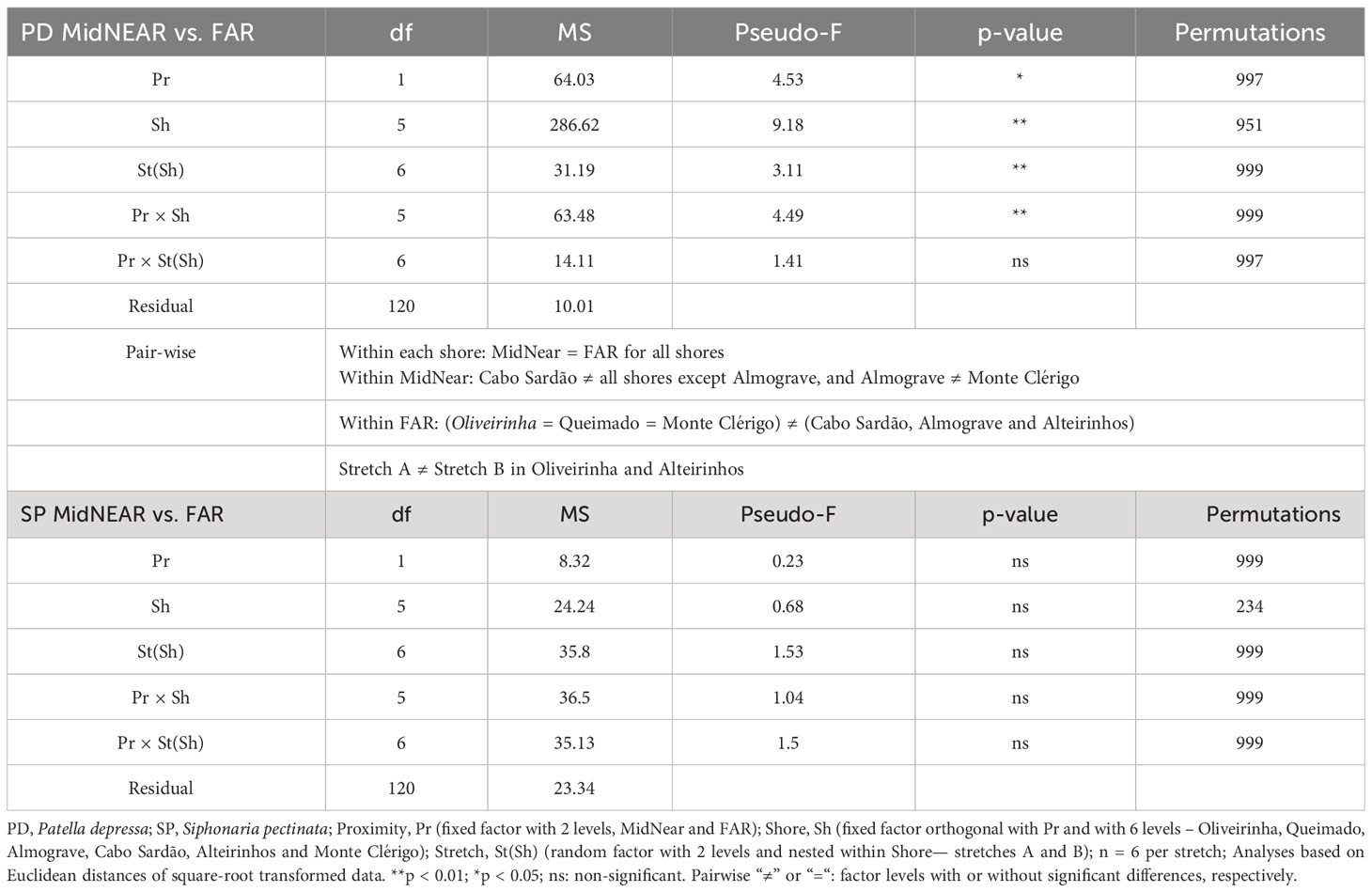
Table 3 Three-way PERMANOVA comparing size-class densities of P. depressa (PD) and Siphonaria pectinata (SP) between mid-shore open-rock areas located at two proximity categories to rockpools, i.e., adjacent to rockpools (MidNEAR) and located at least 25 cm away from any rockpool (FAR), and also among shores and stretches within shores.
3.1.3 Patella vulgata
This species (Supplementary Figure S1) did not occur at Queimado, and only one individual was recorded at Oliveirinha. It was consistently found in very low densities across the other four shores—the highest total mean density of three limpets per 50×50 cm was recorded inside pools in Alteirinhos. The presence of P. vulgata was most consistently found: (i) along pool edges, compared to the other micro-habitats and (ii) in Cabo Sardão, compared to the other shores. Inside pools (POOL), P. vulgata individuals were either juveniles (PV ≤ 1cm) or small-sized (PV 1–2 cm). Within the surrounding micro-habitats (EDGE and NEAR), the commonest size classes were PV 1–2 cm and PV 2–3 cm. Within open-rock far from pools (FAR), only PV ≤ 1cm or PV 2–3 cm were recorded.
3.1.4 Patella rustica
This species (Supplementary Figure S2) was absent from POOL and EDGE and did not occur at Oliveirinha or Queimado, being consistently present on four shores within NEAR in very low densities—the highest total mean density of four limpets per 50×50 cm was recorded in Almograve—and variable size-structure—the modal size class was either PR 1–2 cm or PR 2–3 cm, depending on the shore. Only a few sampled individuals occurred on the mid-shore open-rock of the two steeper shores: Almograve (both within MidNEAR and FAR) and Cabo Sardão (only within FAR).
3.1.5 Siphonaria pectinata
On every shore, this species was consistently more abundant inside rockpools compared to the surrounding micro-habitats: on average, the total mean density of S. pectinata in POOL was eight times higher than in EDGE and 19 times higher than in NEAR (Figure 4). Inside pools, the size structure of S. pectinata was dominated by juveniles (SP ≤ 0.5cm) on all shores (74% on average), although a comparatively lower proportion of juveniles (54%) and exceptionally higher densities of the three larger size classes were found in Monte Clérigo (Figure 4). Within pool surrounding areas, the modal size class depended on the shore, being either SP 0.5–1 cm, SP 1–2 cm, or equal proportions of these two size classes (Figure 4, EDGE, NEAR, and MidNEAR). Away from pools, the modal size class was SP 0.5–1 cm on all shores (Figure 4, FAR). Monte Clérigo had the highest abundance of S. pectinata within all micro-habitats (POOL, EDGE, and NEAR) and mid-shore categories (MidNEAR and FAR) (Figure 4). However, no significant differences were found among shores or stretches within each shore in population size structure and density within pools (Table 2, SP within POOL). Within pool edges, differences in population size structure and density were significant among shores and not among stretches, with Monte Clérigo differing significantly from Almograve, Cabo Sardão, and Alteirinhos (Table 2, SP within EDGE). Differences between Monte Clérigo and the other shores were mostly explained by higher densities of SP 1–2 cm and SP 2–3 cm within rockpool edges of Monte Clérigo (SIMPER, Figure 4). Moreover, the highest total mean density within EDGE (15 individuals per 50×50 cm) was at Monte Clérigo (Figure 4). On open-rock near pools, only differences among stretches were significant (Table 2, SP within NEAR). Correlations of the total density of S. pectinata between adjacent micro-habitats were positively significant between POOL and EDGE (rs = 0.455, p<0.001) and between EDGE and NEAR (rs = 0.214, p<0.001) but non-significant between POOL and NEAR (rs = 0.066, p=0.236). Population size structure and density of S. pectinata on mid-shore open-rock did not differ with respect to proximity to rockpools, or among shores or stretches (Table 3).
3.2 Vertical distribution of limpet species inside and outside pools
The complete range of shore levels was not present on all shores: it was not possible to sample areas at <1.5 m above C.D. at Almograve; the two highest shore levels (>2.5 m above C.D) were absent at Monte Clérigo (Figure 6). Moreover, the total number of rockpool systems sampled at the five shore levels differed among shores (N.B. sample sizes in Figure 6 caption).
3.2.1 Patella ulyssiponensis
The highest abundance inside rockpools was consistently recorded at the second lowest interval of shore height (1.5–2 m above C.D.), decreasing progressively at higher shore levels. This was observed on all shores except Monte Clérigo, where a slightly higher abundance was found on the upper mid shore (2–2.5 m) (Figure 6, POOL). The highest abundance of this species in the open-rock surrounding rockpools was always recorded at the lowest shore level available on each of the six shores, declining upshore to complete absence (in Oliveirinha and Queimado), or sharply on the other four shores (Figure 6, NEAR). A similar pattern was found for rockpool edges, but with a less-abrupt decrease in abundance with increasing shore height (Figure 6, EDGE).
3.2.2 Patella depressa
Within every micro-habitat, this species was generally most abundant at mid-shore levels; the exception was Monte Clérigo, where abundances of P. depressa within all the three micro-habitats were similar across all three shore levels (Figure 6). On the other five shores within pools and their edges, very low densities or complete absence were found at the lowest shore level, with most individuals occurring from 1.5 to 3 m above C.D. at Oliveirinha and Queimado and higher than 2 m above C.D. at Almograve, Cabo Sardão, and Alteirinhos (Figure 6, POOL and EDGE). Outside rockpools, P. depressa was almost absent for the two higher shore levels at Oliveirinha and Queimado, while it was present across all shore levels at Almograve, Cabo Sardão, and Alteirinhos; the highest abundances were found at the 1.5–2 m level on three shores (Oliveirinha, Queimado, and Alteirinhos) and from 2 to 3 m above C.D. at Almograve and Cabo Sardão (Figure 6, NEAR).
3.2.3 Patella vulgata
There were no clear vertical patterns in this low-density range-edge species; where present, it occurred mostly on pool edges at various heights on different shores (Figure 6).
3.2.4 Patella rustica
This species was most abundant at the highest shore levels, being usually found on the steeper shores (Almograve and Cabo Sardão) (Figure 6).
3.2.5 Siphonaria pectinata
The vertical distribution patterns of this species were the most variable among shores; the highest abundances within pools were found on the mid-shore and/or upper shore (Figure 6, POOL). On all shores except Monte Clérigo, a consistent absence or low abundance both inside and in the edge of pools was found at the two lower shore levels (Figure 6, POOL and EDGE). Inside pools, it was found at high shore levels, being most abundant at 2.5–3 m at Oliveirinha, Almograve, Cabo Sardão, and Alteirinhos; the distribution inside pools at Queimado was vertically discontinuous as mostly restricted to two separate levels (2–2.5 m and >3 m), with maximum abundance at the highest level (Figure 6, POOL). On pool edges, it was most abundant at mid-tidal heights in Oliveirinha and Queimado (1.5–3 m above C.D.), while only being found at higher levels (>2.5 m above C.D.) in Almograve, Cabo Sardão, and Alteirinhos (Figure 6, EDGE). Outside rockpools, it was mostly found on the lower shore levels in Oliveirinha and Queimado, and at mid-tidal levels (from 2 to 3 m above C.D.) at Almograve, Cabo Sardão, and Alteirinhos (Figure 6, NEAR). In Monte Clérigo, the highest abundances within both pools and pool edges were found at the highest level, whereas the highest abundance on open-rock was at the lowest level (Figure 6).
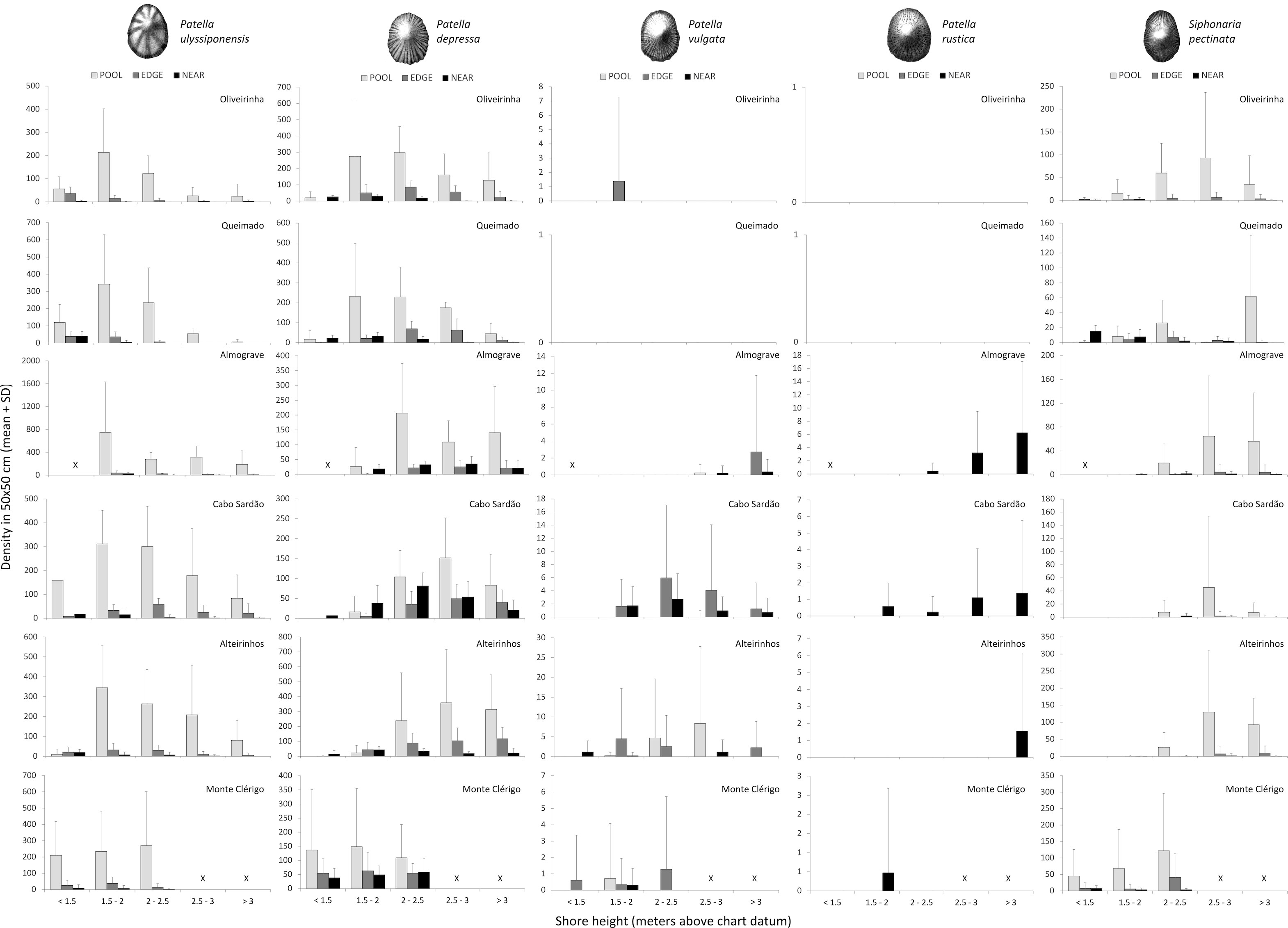
Figure 6 Total density of five limpet species along the vertical intertidal gradient of six shores within POOL (inside rockpools, light grey), EDGE (edge of rockpools, dark grey), and NEAR (open-rock surrounding rockpools, black) micro-habitats. Values are mean (± SD) number of limpets per 50×50 cm at five intervals of shore height; note different y-axis scale among shores and among species. Variable sample size among intervals of shore height on different shores—for the intervals <1.5, 1.5–2, 2–25, 2.5–3 > 3 m above chart datum, respectively: 3, 18, 11, 6, and 14 in Oliveirinha; 6, 15, 18, 3, and 9 in Queimado; 0, 6, 8, 16, and 27 in Almograve; 1, 6, 14, 25, and 10 in Cabo Sardão; 6, 16, 10, 12, and 9 in Alteirinhos; 20, 22, 12, 0, and 0 in Monte Clérigo. The symbol “X” means not available shore level.
Our hypothesis, that inside rockpools (POOL) each of the most common species (P. ulyssiponensis, P. depressa, and S. pectinata) would occur higher up the shore than on open-rock (NEAR), was generally supported (Figure 7; Table 4). This pattern was consistent across most of the 12 sampled coastal stretches, both when considering extreme (minimum and maximum) or central-location (median and mean) values of shore height (Table 4; Figures 7A). There was an exception: the lowest shore height where the presence of P. ulyssiponensis was recorded in each stretch did not differ between pools and open-rock (Table 4). Compared to open-rock, the median shore height inside rockpools was extended upwards in 0.6 m for P. ulyssiponensis, 0.2 m for P. depressa, and 0.7 m for S. pectinata (Figures 7B).
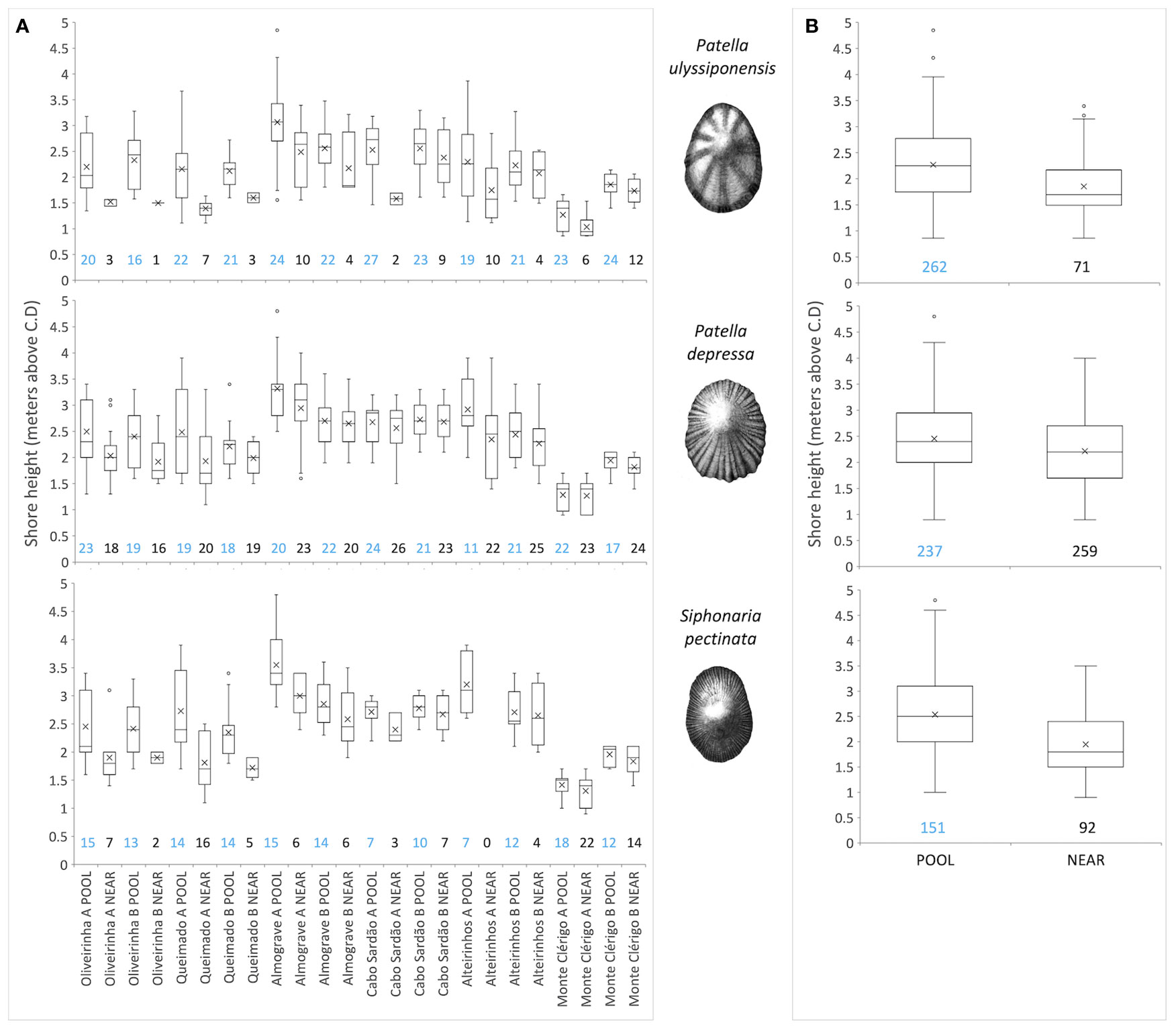
Figure 7 Vertical distribution of three species of limpets inside (POOL) and outside (NEAR) rockpools. Values are shore-height records (meters above chart datum) of all rockpools (POOL) or open-rock surfaces (NEAR) where each species was present (from a total of 323 sampled rockpools and surrounding open-rock). (A) Data for each micro-habitat on each of 12 coastal stretches (stretch A and B sampled within each of six shores). (B) Data for each micro-habitat across all shores and stretches. Boxplots show interquartile range (IQR—the first quartile Q1 to the third quartile Q3; box), median (horizontal line), mean (cross symbol), “minimum” (Q1 − 1.5 × IQR) and “maximum”(Q3 + 1.5 × IQR) (whiskers) and outliers (dots) of standardized shore height. Numbers below boxplots correspond to the respective number of rockpools (blue, POOL) and open-rock surfaces (black, NEAR).
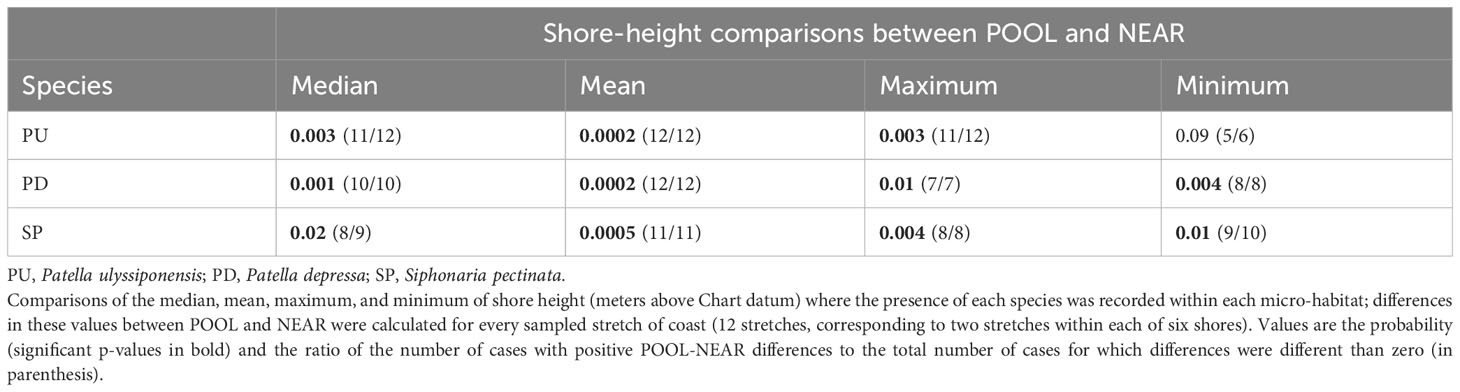
Table 4 Binomial tests comparing the vertical distribution of three species of intertidal limpets between micro-habitats inside (POOL) and outside (NEAR) rockpools.
3.3 Patterns among highly variable rockpool systems
The different sets of predictors assessed for all 323 rockpool systems and used in DistLM analyses reflected the great variability among rockpools present on all shores, encompassing cover of different substrata or sessile organisms (Supplementary Figures S4, S5), density of trochids and littorinids (Supplementary Figure S6), physical characteristics (Supplementary Figure S7), and total density of each co-occurring limpet species (Figures 2–4).
3.3.1 Patella ulyssiponensis
The models retrieved by DistLM analyses for P. ulyssiponensis inside rockpools (PU POOL) and on the open-rock surrounding rockpools (PU NEAR) explained identical proportions of the variability among replicates (44%), higher than the model regarding this species in the edge of rockpools (PU EDGE, 34%) (Table 5).
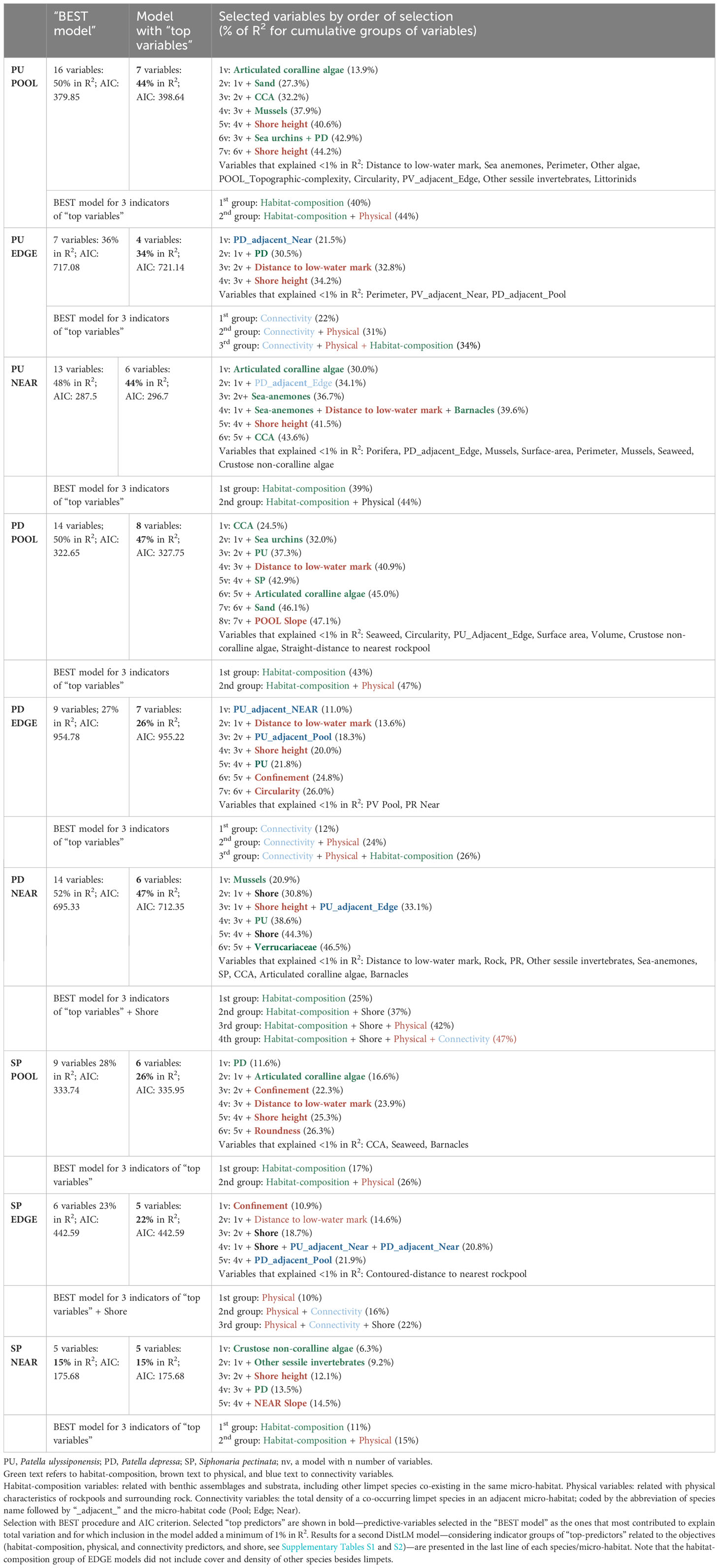
Table 5 Distance based linear models (DistLM) for three species of limpets (P. ulyssiponensis, PU; P. depressa, PD; S. pectinata, SP) within three neighboring micro-habitats (POOL, EDGE, and NEAR).
In both PU POOL and PU NEAR models, habitat-composition variables were the most important group of “top-predictors” for structuring P. ulyssiponensis size-class densities (40% and 39%, respectively, Table 5). Two habitat-composition variables estimated as percentage cover were selected in common for these models (Table 5): articulated coralline algae (the first selected variable in both models, contributed alone to explain 13% and 30% of the variation inside and outside rockpools, respectively) and CCA (explained 5% and 2% of the variation inside and outside rockpools, respectively). Both were positively associated with overall abundance of this species within both micro-habitats: articulated coralline algae was mostly related with density of P. ulyssiponensis juveniles (PU ≤ 1cm) inside rockpools and with densities of P. ulyssiponensis adults (all size classes except PU ≤ 1 cm) outside rockpools; whereas CCA was mostly related with P. ulyssiponensis adults inside and outside rockpools (Figures 8A, C). Other habitat-composition predictors were selected exclusively for the PU POOL model: the cover of sand (also including detritus and cobbles in minor proportions, Supplementary Table S2) was negatively related with densities of P. ulyssiponensis adults, whereas both the cover of mussels and sea urchins were positively related with densities of P. ulyssiponensis, particularly juveniles (Figure 8A). Both the cover of sea anemones and barnacles were selected exclusively for the PU NEAR model (Table 5), both positively related with density of P. ulyssiponensis juveniles outside rockpools and responsible for the segregation of a few samples (squares in the bottom part of the db-RDA plot, Figure 8C).
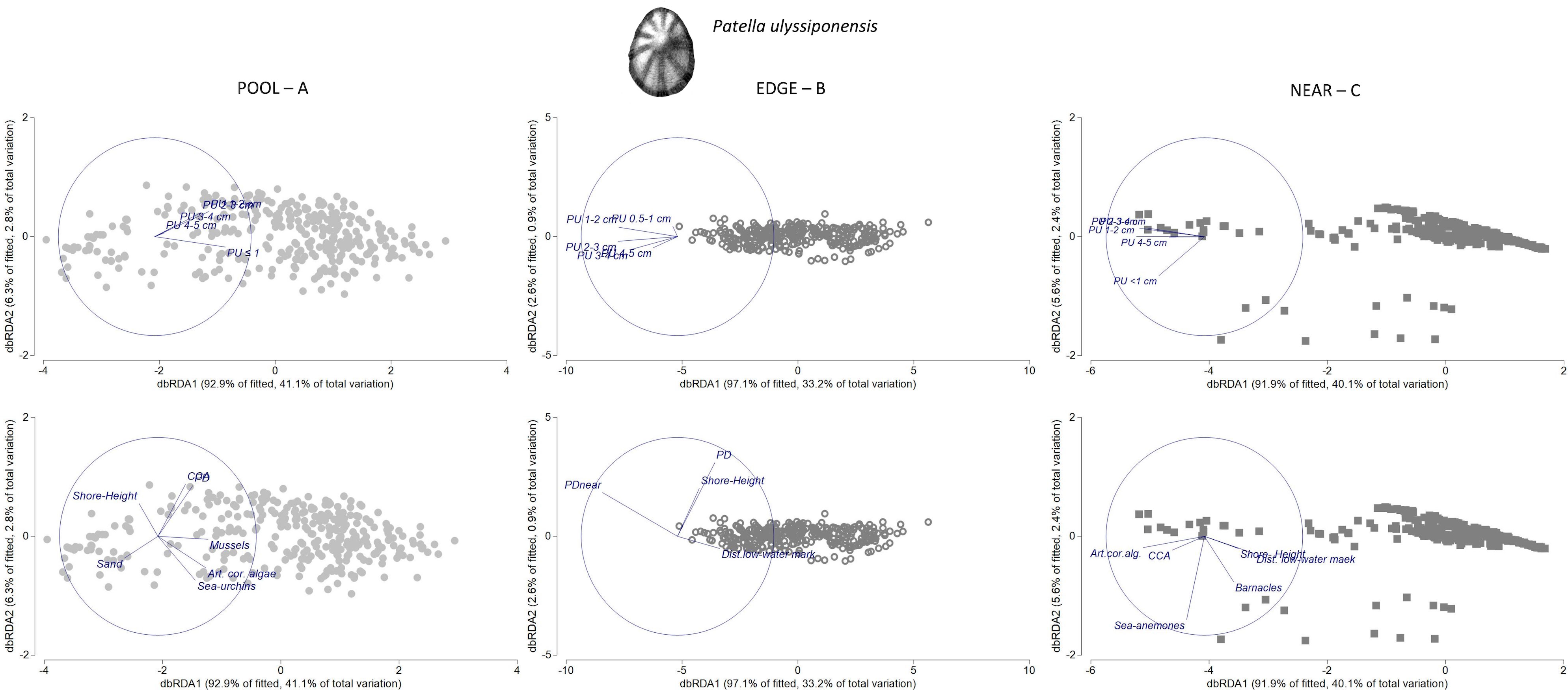
Figure 8 Distance-based redundancy analysis (db-RDA) plots for the spatial variability in abundance and size structure of Patella ulyssiponensis within each of three neighboring micro-habitats: (A) POOL (rockpools, gray circles); (B) EDGE (edge of rockpools, open circles); (C) NEAR (open-rock adjacent to rockpools, gray squares), (n = 323 samples). Vectors on top plots are response variables (size-class densities); vectors on bottom plots are “top predictors” selected by distance-based linear models (DistLM, see Table 5). On the plot in the top left, the superimposed vector labels correspond to: PU 1–2 and PU 2–3. On the plot in the top middle, the superimposed vector labels correspond to: PU 2–3 and PU 3–4.
The group of physical variables was also important for PU POOL and PU NEAR (adding another 5% of explanation to the models), while connectivity variables were not selected for these models (Table 5). Shore height was selected as a “top predictor” for both models (Table 5), with a general negative association found between this predictor and P. ulyssiponensis abundance inside and outside rockpools, particularly negatively related with density of juveniles inside rockpools and with densities of adults outside rockpools (Figures 8A, C). Outside rockpools, distance to low-water mark was also negatively related with densities of P. ulyssiponensis adults (Figure 8C).
In the PU EDGE model, the most prominent predictor was a connectivity variable, which alone explained 22% of the model variation: the total density of P. depressa on the surrounding open-rock (PD_adjacent_Near) (Table 5). This variable was positively associated with densities of P. ulyssiponensis of the smallest size classes (PU 0.5–1 cm, PU 1–2 cm) around rockpool edges (Figure 8, middle). The other variables responsible for the spatial pattern of P. ulyssiponensis at rockpool edges were distance to low-water mark (higher shore heights had lower overall abundance of P. ulyssiponensis), shore height, and the abundance of the congener P. depressa (PD) (both negatively associated with the abundance of larger P. ulyssiponensis) (Table 5; Figure 8B).
3.3.2 Patella depressa
Both DistLM models produced for P. depressa inside rockpools (PD POOL) and on the open-rock surrounding rockpools (PD NEAR) explained 47% of variation among rockpool systems, while the model regarding the EDGE explained 26% of the variability (Table 5).
The group of habitat-composition predictors was the most important for explaining P. depressa size-class densities on both PD POOL and PD NEAR models (43% and 25%, respectively); the cover of CCA and mussels contributed to more than 20% of the variation inside and outside rockpools respectively (Table 5). These predictors greatly influenced spatial structuring within each micro-habitat (samples along the horizontal axis db-RDA1), with CCA positively associated with overall abundance of P. depressa inside rockpools and mussels positively associated with overall abundance of P. depressa outside rockpools (Figures 9A, C). The other habitat-composition variables that also explained variation inside rockpools were cover of sea urchins—negative relationship with juveniles (PD ≤ 1cm); total density of P. ulyssiponensis within the same micro-habitat (PU) and cover of articulated coralline algae—negative relationships with the largest size class (PD 4–5 cm); total density of S. pectinata within the same micro-habitat (SP)—positive relation with P. depressa adults; and cover of sand—negative relation with P. depressa adults (Table 5; Figure 9A). Outside rockpools, besides the above-mentioned positive association with mussels, Verrucariaceae cover was negatively associated with density of small-sized P. depressa (PD 1–2 cm), and the total density of P. ulyssiponensis within the same microhabitat (PU) was negatively associated with density of large-sized P. depressa (PD 2-3 cm) (Figure 9C).
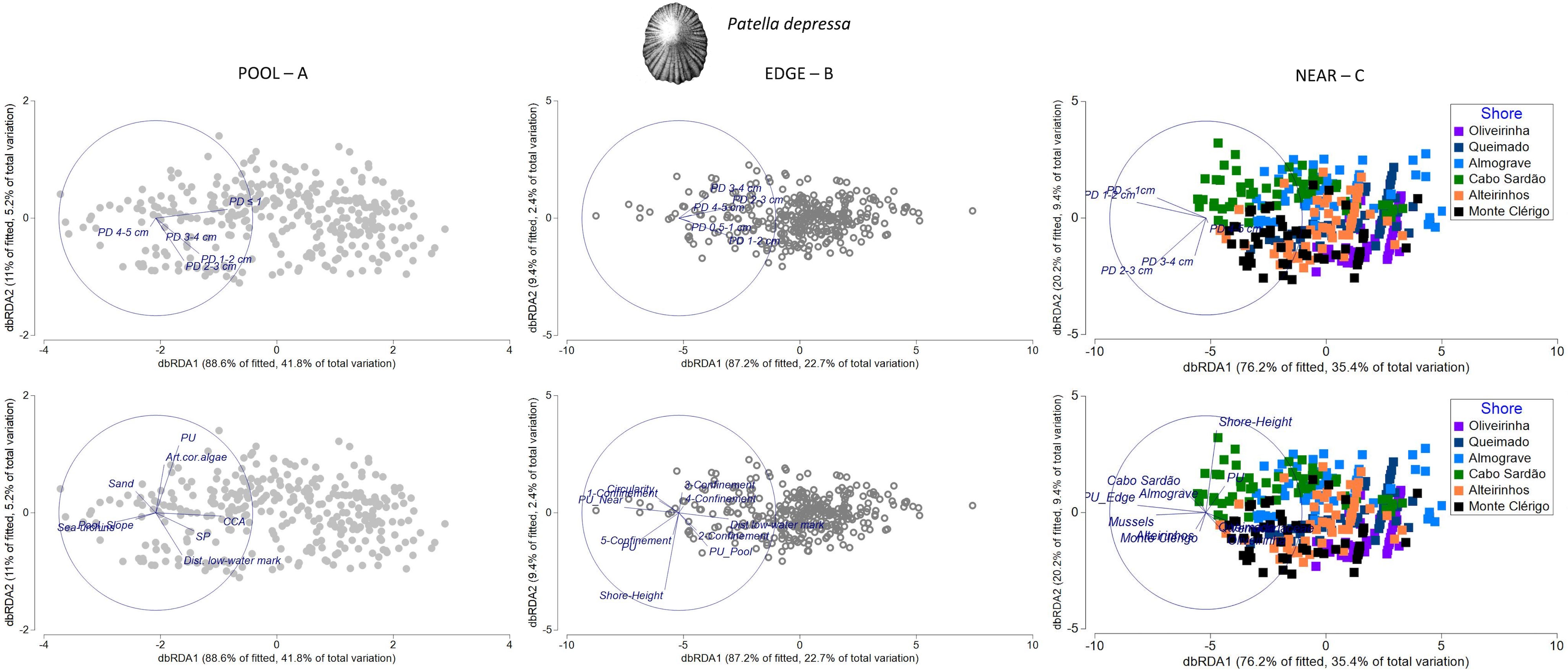
Figure 9 Distance-based redundancy analysis (db-RDA) plots for the spatial variability in abundance and size structure of Patella depressa within each of three neighboring micro-habitats: (A) POOL (rockpools, gray circles); (B) EDGE (edge of rockpools, open circles); and (C) NEAR (open-rock adjacent to rockpools, gray squares), (n = 323 samples). Vectors on top plots are response variables (size-class densities); vectors on bottom plots are “top predictors” selected by distance-based linear models (DistLM, see Table 5). On the plot of the bottom right corner, the superimposed vector labels correspond to: Alteirinhos and Monte Clérigo (at the left); Queimado and Verrucariaceae and Oliveirinha (at the right, coincident with several sample symbols).
The group of physical predictors was also important for the spatial patterns of both PD POOL and PD NEAR (adding 4% and 5% of explanation to these models, respectively). Distance to low-water mark (included on both models) and/or shore height (included on PD NEAR model) were the physical variables most important for explaining the variation in P. depressa among samples within each micro-habitat (Table 5). Inside rockpools, distance to the low-water mark was positively associated with densities of mid- and large-sized P. depressa (PD 2–3 cm, and PD 3–4cm) (Figure 9, top). Outside rockpools, shore height was negatively associated with density of PD 3–4 cm (Figure 9C). An additional physical variable, the slope of the rockpool bottom, was negatively associated with PD ≤ 1cm inside rockpools (Figure 9A).
While no connectivity variable was selected for the PD POOL model (Table 5), the total density of P. ulyssiponensis in rockpool edges (PU_adjacent_Edge) was selected for the PD NEAR model, being positively associated with density of PD 1–2 cm outside rockpools (Figure 9, bottom). Furthermore, shore was included as a factor in the PD NEAR model (contributing to 12% of total explanation, Table 5), with the following positive associations: i) Cabo Sardão and Almograve with densities of the smallest size classes (PD ≤ 1 cm and PD 1–2 cm); ii) Oliveirinha and Queimado with density of the largest size class (PD 4–5 cm); and iii) Alteirinhos and Monte Clérigo with densities of mid and large-sized P. depressa (PD 2–3 cm and PD 3–4 cm) (Figure 9C).
For P. depressa present around rockpool edges (PD EDGE), the structuring among samples was mainly driven by association with two connectivity variables acting in opposite directions (accounting for 12% of explanation, Table 5): a negative relationship with the total density of P. ulyssiponensis on the surrounding open-rock (PU_adjacent_Near) and a positive relationship with the total density of P. ulyssiponensis inside rockpools (PU_adjacent_Pool) (Figure 9B). The second group of predictors selected for the PD EDGE model was the one of physical variables, namely, distance to low-water mark (positive relation with overall abundance of P. depressa), shore height, and circularity (negative association with abundance of P. depressa in general) and confinement (Figure 9B). It is worth noting the negative relationship between very-high confinement (category 5, the most-recessed pools) and density of large-sized P. depressa (PD 3–4 cm) (Figure 9, middle). Finally, a habitat-composition variable was also selected for this PD EDGE model: abundance of P. ulyssiponensis within the same micro-habitat (PU)— negatively related with large-sized P. depressa (Table 5; Figure 9B).
3.3.3 Siphonaria pectinata
The DistLM models for S. pectinata inside rockpools (SP POOL) and in the edge of rockpools (SP EDGE) explained similar proportions of the variability (26% and 22%, respectively), higher than the model for this species on the open-rock surrounding rockpools (SP NEAR—15%).
In SP POOL and SP NEAR models, habitat-composition predictors were most important for the spatial patterns of S. pectinata (17% and 11%, respectively) (Table 5). The first selected predictor in the SP POOL model was the total density of P. depressa in the same micro-habitat (PD) (Table 5), for which a positive relation with density of S. pectinata juveniles (SP ≤ 0.5cm) was suggested (Figure 10A). The other habitat-composition predictor included in the SP POOL model was the cover of articulated coralline algae (Table 5), for which a negative relationship was suggested with the density of the middle size class (SP 1–2 cm) (Figure 10A). For the SP NEAR model, three different habitat-composition predictors were included: cover of crustose non-coralline algae was positively related with densities of small-sized S. pectinata (SP ≤ 0.5 cm and SP 0.5–1 cm); the variable “Other sessile invertebrates” was negatively associated with densities of larger S. pectinata (SP 1–2 cm and SP 2–3 cm); and the total density of P. depressa in the same micro-habitat (PD) was positively associated with densities of small-sized S. pectinata (SP ≤ 0.5 cm and SP 0.5–1 cm) (Table 5; Figure 10C).
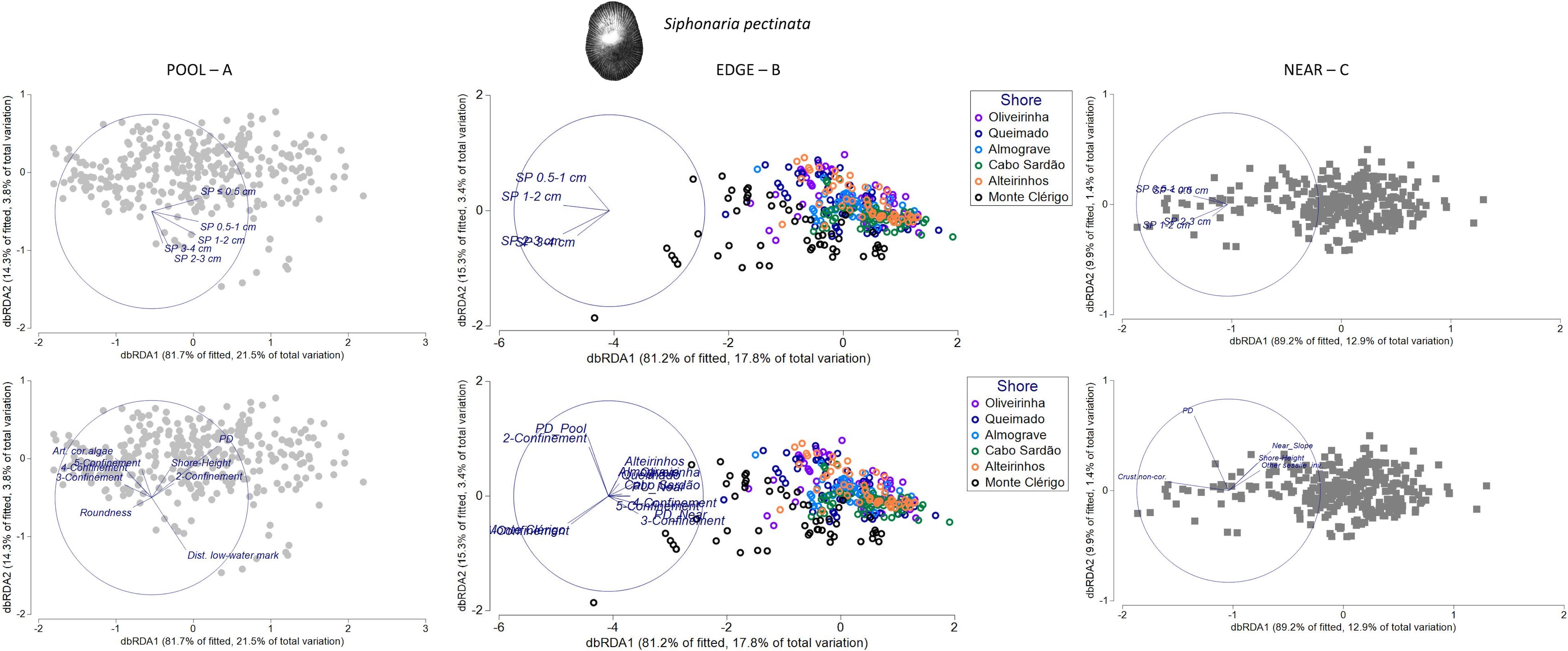
Figure 10 Distance-based redundancy analysis (db-RDA) ordination plots for the spatial variability in abundance and size structure of Siphonaria pectinata within each of three neighboring micro-habitats: (A) POOL (rockpools, gray circles), (B) EDGE (edge of rockpools, colored circles), and (C) NEAR (open-rock adjacent to rockpools, gray squares), (n = 323 samples). Vectors on top plots are response variables (size-class densities); vectors on bottom plots are “top predictors” selected by distance-based linear models (DistLM, see Table 5). On the plot in the top middle, the superimposed vector labels correspond to: SP 2–3 and SP 3–4. On the plot in the bottom middle, the superimposed vector labels correspond to: Monte Clérigo and 1-Confinement (at the left); Oliveirinha, Queimado, and Almograve (at the right); Cabo Sardão and PU Near (at the right); 4-Confinement and 5-Confinement (at the right). On the plot in the top right, the superimposed vector labels correspond to: SP < 1 and SP 0.5–1.
The group of physical predictors also contributed to the SP POOL and SP NEAR models (adding another 9% and 4% of explanation, respectively). Common to both models was the selection of shore height, which was positively associated with S. pectinata abundance inside rockpools, namely, with juveniles within POOL (SP ≤ 0.5cm), and negatively associated with S. pectinata abundance outside rockpools, namely, with mid- and large-sized S. pectinata within NEAR (SP 1–2 cm and SP 2–3 cm) (Table 5; Figures 10A, C). The other physical predictors selected in SP POOL model were the following: confinement—particularly the negative relationship between high confinement and the largest size classes (SP 2–3 cm and SP 3–4 cm); distance to low-water mark—positive association with these largest size classes; and roundness—negative relation with juveniles (SP ≤ 0.5cm) (Table 5; Figure 10A). The other physical variable selected in SP NEAR model was Slope NEAR, showing a negative relationship with mid- and large-sized SP (SP 1–2 cm and SP 2–3 cm) (Table 5; Figure 10C).
In both the SP POOL and SP NEAR models, no connectivity variables were selected (Table 5). In contrast, three connectivity variables were selected for the SP EDGE model (responsible for 6% of total explanation): P. ulyssiponensis within open-rock surfaces surrounding rockpools (PU_adjacent_Near)—negative association with mid-sized SP (SP 1–2 cm); P. depressa within open-rock surfaces surrounding rockpools (PD_adjacent_Near)—negative association with small-sized S. pectinata (SP 0.5–1 cm); and P. depressa inside rockpools (PD_adjacent_Pool)—positive association with small-sized S. pectinata (Table 5; Figure 10B). However, in the SP EDGE model, the variable that stood out was confinement, a physical predictor that explained 11% of the variation (Table 5), the most evident pattern being the positive associations between low confinement (category 2) and small-sized S. pectinata (SP 0.5–1 cm) and between very-low confinement (category 1) and the largest size classes (SP 2–3 cm and SP 3–4 cm) (Figure 10B). Besides the connectivity variables and confinement, the factor shore was selected in this model (Table 5), for which the most evident pattern was the positive association between the largest size classes (SP 2–3 cm and SP 3–4 cm) and Monte Clérigo (Figure 10B). This shore had the highest percentage of very-low confinement (category 1): 24% of rockpools (Supplementary Figure S7).
In summary, the cover of articulated coralline algae was a mutual habitat-composition “top predictor” in models of the three species inside rockpools and of P. ulyssiponensis on the open-rock, whereas the cover of mussels and crustose non-coralline algae were the most relevant “top predictors,” respectively, for P. depressa and S. pectinata on the open-rock (Table 5).
4 Discussion
4.1 Summary of main findings
Coexistence of mobile species that share common habitats may arise from differences in the spatial patterns of different species at small scales. This can be caused by overlap in populations of individual species with different centers of distribution or with life-history-driven time windows of occupancy within the same habitat, and by connectivity of any species across adjacent habitats. Our most important results (relative to the specific objectives) were i) Limpets of various sizes of the three most common species—P. ulyssiponensis, P. depressa and S. pectinata—coexist on all shores, both inside and outside rockpools, and along a large part of the vertical tidal gradient within rockpools, thus sharing much of the available rocky-intertidal space in SW Portugal (objectives 1a and 2); ii) low abundance of the cold-water species P. vulgata scattered across micro-habitats on shores in SW Portugal, being more common at pool-edges and the wider landscape at Cabo Sardão, which might act as possible refuges for this range-edge species (objective 1a); iii) absence of P. rustica from rockpools and their edges, reflecting occupancy of open-rock and steeper shores (objective 1a); iv) a decreasing gradient of intra-specific connectivity from rockpools to their edges and surrounding open-rock was found for the three most common species (significant positive correlations in the abundance of the same species between pairs of adjacent micro-habitats), being steeper in P. ulyssiponensis than in P. depressa and S. pectinata, possibly due to P. ulyssiponensis being largely resident in rockpools and to gradual emergence (migration outward from rockpool-nursery areas) of the other two species onto open-rock (objective 1b); v) mid-shore populations of P. depressa and S. pectinata did not differ between open-rock near to and far away from rockpools, which can be considered a single habitat for these species (objective 1c); vi) landscape variability within shores, and hence the micro-habitat mosaics available, likely influenced among-shore patterns of abundance and size of P. depressa on mid-shore open-rock, with shores with less open-rock and more crevices associated with more numerous and smaller limpets (objective 1c); vii) habitat-composition of the substratum and benthic assemblages was generally a more important proximate factor than physical predictors in explaining variability of each limpet species both within rockpools and open-rock (3); viii) inter-specific influence on connectivity explained a great part of the variability of the three main species within rockpool edges, emphasizing the importance of this interface micro-habitat for limpet migration and for interactions between different limpet species (both positive and negative relationships were found between the spatial pattern of each individual limpet species at rockpool edges and the abundance of other limpet species in adjacent micro-habitats) (objective 3).
4.2 Diversity, dominance, and rare species
Five species of intertidal limpets comprising four patellids and one siphonariid co-occur in SW Portugal: P. ulyssiponensis was the dominant species within POOL; P. depressa was dominant within EDGE, NEAR, and FAR; S. pectinata was the third ranked species in abundance across all micro-habitats; P. vulgata infrequently occurred mostly within EDGE and NEAR; and P. rustica was exclusively present on NEAR and FAR. Rockpools did not seem to act as nursery grounds for the two least abundant species (P. vulgata and P. rustica), although a few P. vulgata juveniles were present within rockpools at Alteirinhos and Monte Clérigo. This agrees with a previous study (including Oliveirinha; Seabra et al., 2020), where limpet recruitment was measured within pools regularly cleared of limpets for 3 years: only 22 P. vulgata (all juveniles) and no P. rustica were recorded out of a total of 13,791 recruits (unpublished data). In Ireland, P. vulgata was described to occur in pools “in very small numbers” (Firth and Crowe, 2008: 15 juveniles in 30×30 quadrats in several shores and levels), but still in much higher (~20 times) densities than the ones reported here. Vertical distribution of these rare species (P. vulgata across mid-high levels and P. rustica mostly at high shore, as described in Section 2.1) and low densities of P. vulgata on open-rock were generally consistent with previous studies in our region (e.g., Rubal et al., 2013). The abundances of P. vulgata and P. rustica were consistently higher on shores with steeper cleavage of schist strata (Almograve and Cabo Sardão), where higher mean values of NEAR Slope and NEAR Topographic index (Supplementary Figure S7) and a higher frequency of crevices were found (Supplementary Figure S3). This agreed with previously described positive associations of these species with the following habitat features: P. vulgata with topographically irregular or damp surfaces (Cabral and Simões, 2007) and shady open-rock at mid-high shore (Seabra et al., 2011; Lima et al., 2016; Seabra et al., 2016), and P. rustica with steep open-rock surfaces of exposed shores (Lima et al., 2006) and crevices (Menconi et al., 1999). Further studies dedicated to these two species should be made on the SW Portuguese coast, given its biogeographic importance for both species (close to the southern limit of P. vulgata; not far from the northern limit of P. rustica and included in the region where it is most abundant in Portugal—e.g., Boaventura et al., 2002b). The role of micro-habitats surrounding rockpools, steeper surfaces, or a potentially colder regime in Cabo Sardão (a Cape, likely with upwelling-enriched waters) as potential thermal refuges for southern-edge populations of P. vulgata in this coast deserve further investigation.
4.3 Vertical distribution and among-shore patterns
When considering the micro-habitat inside-rockpools, the three most common limpets are present along most of the intertidal gradient, being abundant at many shore heights on all shores. The vertical distribution patterns on the open-rock were as expected from past work (e.g., Boaventura et al., 2002b), showing zonation of the three species at different tidal levels (low-shore P. ulyssiponensis, mainly mid-shore P. depressa, S. pectinata scattered and non-consistent zonation pattern across shores). The hypothesis of extension of the upper distribution limit in areas inside pools compared to contiguous areas outside pools was supported for the three common species. The presence of P. ulyssiponensis in rockpools at conspicuously higher shore levels than the low shore (where it is commonly found on the open-rock) has long been described (e.g., Evans, 1947; Firth and Crowe, 2008). The upper limits of subtidal and lower-intertidal organisms are generally pushed upwards by rockpools, as shown by many macroalgae (e.g., Femino and Mathieson, 1980; Araújo et al., 2006), seagrasses (Dethier, 1984) and also by the limpet Acmaea mitra and other invertebrates in Canada (Green, 1971). There was no such extension in the lower distribution limit (minimum value of shore height) of P. ulyssiponensis, suggesting equal habitat suitability between rockpools and open-rock at low shore (Firth and Crowe, 2008). For P. depressa and S. pectinata, the lower distribution limit also varied between micro-habitats. Within the open-rock, spatial and temporal variations in both vertical distribution limits of P. depressa in Portugal was previously related to sea-water temperature (Nobre et al., 2019). Here, the highest vertical extension was found for S. pectinata (difference in the median shore height between inside and outside rockpools, Figure 7). This species can be particularly abundant upon open-rock and in rockpools located at both extremes of the vertical gradient (e.g., low-shore open-rock vs. splash pools) (Seabra et al., 2020; this study). Amelioration against abiotic stress due to permanent submersion has been the main explanation for rockpools supporting more diverse assemblages compared to open-rock (Metaxas et al., 1994; Firth et al., 2014), and for the role of rockpools as nurseries for limpets due to sensitivity of their early-life stages to desiccation (e.g., Lewis and Bowman, 1975; Seabra et al., 2020). A recent study on the metabolic stress responses of Cellana toreuma in China demonstrated that limpets (size not specified) on rockpools suffer less cold stress than those living on open-rock during low tide in winter (Sun et al., 2023). Any environmental differences at low tide from pools to open-rock become greater at higher tidal levels (e.g., Huggett and Griffiths, 1986) increasing the ecological importance of pools.
Differences in density and size structure of limpets among shores were not found for P. ulyssiponensis within any of the three micro-habitats of rockpool systems, but were found for P. depressa within NEAR and S. pectinata within EDGE. The general pattern of higher abundance and lower individual size of P. depressa was found on the NEAR micro-habitat of steeper shores compared to flatter shores. This pattern could be a consequence of lower growth rates (probably due to the less available open-rock space and consequent limited foraging activity) and/or higher recruitment (possibly due to the higher abundance of crevices) onto open-rock of steeper shores. Among-shore variation detected for S. pectinata was due to a higher abundance, namely, of larger size classes, within pool edges in Monte Clérigo. This was the most sheltered and extensive gently sloping shore, with the shallowest, less topographically complex, and least-confined rockpools, likely to warm quickly (the lowest values of maximum and mean depth, POOL topographic index and slope, and confinement, Supplementary Figure S7). Such conditions are associated with high abundance (Rubal et al., 2013; Slama et al., 2021) and high growth rate (Ocaña, 2003) of S. pectinata. However, as Monte Clérigo is the most southern shore, this pattern might also reflect geographic affinity of S. pectinata. For all species and micro-habitats, sporadic or frequent variation was found between stretches within each shore, which could be related to smaller-scale variation in physical and biological processes.
4.4 Horizontal distribution and intra-specific connectivity across micro-habitats
On all shores, there were many more limpets of the three common species inside rockpools than in the surrounding open-rock: P. ulyssiponensis was 44 times, S. pectinata was 19 times, and P. depressa was 5 times more abundant within POOL compared to NEAR. This pattern was mostly due to newly settled and juvenile limpets, confirming that rockpools were undoubtfully important nurseries for these three species in this region (Seabra et al., 2020). Regarding only the adults (patellids of MSL > 1cm and siphonariids of MSL > 0.5cm), on all shores, there were five times more P. ulyssiponensis adults and six times more S. pectinata adults inside than outside rockpools (POOL versus NEAR, on average), but there were three times more P. depressa adults outside than inside rockpools (NEAR versus POOL, on average). This suggests that rockpools provide important adult habitats for P. ulyssiponensis and S. pectinata, whereas they serve primarily as nurseries for P. depressa. Moreover, P. depressa was also the species for which the gradient in abundance between adjacent micro-habitats was less sharp, namely, between POOL and EDGE (EDGE being a continuum from POOL for P. depressa, since its abundance was only three times higher inside rockpools than their edges; the same ratio was 10 and 8, respectively for P. ulyssiponensis and S. pectinata). This horizontal pattern suggested a gradual transition through migration out of pools (similarly to P. vulgata in Ireland, Delany et al., 1998), perhaps moderated by intra-age class interactions (Boaventura et al., 2003). Furthermore, whereas the abundance of P. ulyssiponensis within EDGE was consistently higher compared to NEAR on all shores (four times on average), this was only found on four and five of the six shores, respectively, for P. depressa and S. pectinata. Therefore, pool edges were mostly an intermediate zone from inside to outside rockpools, possibly acting as a frontier for the largely pool-resident P. ulyssiponensis above the low shore and a potential a transition route for migratory species (P. depressa and S. pectinata). The ontogenic distinction between residence within pools until adulthood (P. ulyssiponensis) vs. emigration of old juveniles of other patellids was previously described (e.g., Delany et al., 1998).
Considering intra-specific connectivity at the scale of the rockpool system, the strength of correlations in the abundance of the same species between pairs of adjacent micro-habitats lessened with distance from the pool. This pattern was found in common for all species, suggesting emergence of vagrant juveniles of the three species from their settlement sites within rockpools to drier open-rock only suitable for older/larger limpets (e.g., Bowman, 1981; Bowman, 1985). However, the positive relationship between rockpools and open-rock was only direct for P. ulyssiponensis (the only species with a significant correlation between POOL and NEAR). A direct connection from POOL to NEAR was not found for P. depressa and S. pectinata, although a continuum of positive relationships was found between rockpools and their edges and between edges and their surrounding open-rock. For these two species compared to P. ulyssiponensis, a more gradual connection was suggested between rockpools vs. open-rock at this horizontal small scale. Patella ulyssiponensis abundance consists mostly of rockpool populations, with spill-over to open-rock only possible on the low shore. This might be due to similarity in habitat suitability between rockpools and open-rock at low shore or to intra-specific interactions (Firth and Crowe, 2010).
The two most abundant species at the mid-shore open-rock (P. depressa and S. pectinata) did not differ with proximity to rockpools (MidNEAR and FAR areas). Therefore, at this tidal level, the open-rock surrounding rockpools can be considered as part of the open-rock habitat in general. Once emerged from mid-shore rockpools and their edges, limpets of these species likely form unique populations living across the mid-shore open-rock. Landscape variability was associated with among-shore differences in population structure of P. depressa on the mid-shore. This pattern could be interpreted as intra-specific connectivity with nurseries at this horizontal scale: shores with less open-rock and more crevices might have more abundant and smaller-sized limpets due to a higher connection to nursery areas (less-fragmented mosaics of patchy micro-habitats for recently settled limpets) across the mid-shore landscape. Mid-shore crevices were identified as important juvenile micro-habitats for P. depressa and S. pectinata in SW Portugal (Seabra et al., 2020). However, landscape variability did not seem important for S. pectinata on the mid-shore. Our results on overall patterns of intra-specific connectivity suggested migration outward from nursery areas. Further studies are needed on this process and on survival of early-life stages and ontogenetic shifts in traits (e.g., tolerance of emersion, Bowman, 1981) of these species across micro-habitats.
4.5 Patterns among highly variable rockpool-systems
The interpretation of DistLM models and relationships highlighted by db-RDA must be made with caution, as based on associations between response and predictive variables without investigating the causes of variation with manipulative experiments. Our study prompts future experimental work on the variables identified to be most relevant for the spatial patterns of each species within each micro-habitat. For all three species, the group of habitat-composition variables explained most of the variation in response matrices of the three species within both rockpools and open-rock. Previous studies showed the proximate importance of biotic predictors in determining molluscan patterns (e.g., Gonçalves et al., 2023). Nevertheless, physical factors can ultimately shape assemblage composition in pools (Huggett and Griffiths, 1986; Legrand et al., 2018), which is characterized by high small-scale variability (Araújo et al., 2006; Rubal et al., 2011; Bertocci et al., 2012). In general, the percentage of explained variation in EDGE models was lower than that in POOL and NEAR models, probably due to the smaller number of habitat-composition variables assessed within EDGE, (as the percentage cover of sessile taxa and the density of mobile grazers other than limpets were not measured on pool edges). A much greater percentage of the variation was explained for the two patellids (44% in P. ulyssiponensis, 47% in P. depressa, in both POOL and NEAR models) compared to S. pectinata (26% in POOL and 15% in NEAR). This lower explanatory power of S. pectinata models and the lack of rigid vertical distribution (see above) was probably related to an opportunistic nature. For instance, their presence in extremely shallow and very-low-confined splash-zone rockpools (where extreme physical–chemical conditions are likely) was consistent with distribution patterns of other siphonariid species (Hodgson, 1999). Hodgson (1999) also described several traits of siphonariids responsible for their success in occupancy of environmentally harsh micro-habitats, including efficient respiration in air and water, optimized foraging activity, unpalatability to predators, facultative metabolic rate depression, and possible anaerobiosis under unfavorable conditions. Their ability to re-hydrate more rapidly compared to patellogastropods, coupled with greater tolerances to salinity fluctuations (Hodgson, 1999), may also enable a wider range of conditions to be occupied by S. pectinata.
Inter-specific influences on connectivity were remarkably important for all three species in characterizing their variability within the transition environment (pool edges), but not within POOL and NEAR micro-habitats (connectivity variables selected only in the P. depressa NEAR model). On the contrary, abundances of other limpet species in the same micro-habitat were selected in most models, suggesting both positive facilitation and negative interactions between different limpet species (see below).
Inside pools, the relationships that seem to be established between different species are mostly positive, namely between patellids (the exception was a negative relationship between the abundance of P. ulyssiponensis and the largest size-class of P. depressa), and between P. depressa and S. pectinata (POOL dbRDA plots with predictor vectors). This agrees with Firth and Crowe (2010), who did not find evidence of inter-specific competition of P. ulyssiponensis and P. vulgata inside rockpools. There was also no evidence of intra-specific competition inside pools, as the different size classes of the same species also suggest positive relationships (POOL dbRDA plots with limpet-size vectors). This is not in agreement with Firth and Crowe (2010), who found evidence of intra-specific competition of P. ulyssiponensis inside rockpools. Abundances of both patellids inside rockpools were positively related with cover of CCA and articulated coralline algae and negatively related with sand cover. Observation of these patellids within “Lithothamnia-lined pools” and coralline turfs (Evans, 1947; Kooistra et al., 1989; Benedetti-Cecchi and Cinelli, 1996) and their grazing on these algal groups (Kooistra et al., 1989; Delany et al., 2002) has long been described. While siphonariids are known to be tolerant to sand inundation (Hodgson, 1999), negative effects of sediment have been found on grazing activity and survival of patellids (e.g., Airoldi and Hawkins, 2007). Contrasting patterns between the two patellids were observed inside pools: higher P. ulyssiponensis density occurred at lower shore, along with more sea urchins cover; P. depressa density was higher with distance from low-water mark and less sea urchins. Similar contrasting associations with Paracentrotus lividus within low-shore rockpools were found for juveniles of the two species in this region (Seabra et al., 2019; Seabra et al., 2020). The highest abundances of S. pectinata within pools and at pool edges were associated with the lowest category of confinement and the shore with more pools of this category (Monte Clérigo). For S. pectinata inside pools, a positive relationship with distance to low-water mark was found (similarly with P. depressa), and a higher abundance of S. pectinata was related to lower coverage of articulated coralline algae (contrarily with P. depressa). Indeed, S. pectinata in SW Portugal is frequently found at high-shore rockpools dominated by bare rock (juveniles, Seabra et al., 2020; adults, personal observations).
Outside pools (NEAR), apart from the negative relationship between the abundance of P. ulyssiponensis and large-sized (2-3 cm) P. depressa, there were no other negative relationships established between the two patellids and with S. pectinata, suggesting limited evidence of inter-specific competition. This agrees with experimental evidence of weak inter-specific competition between P. vulgata and P. depressa on open-rock in Portugal (Boaventura et al., 2002a). A negative relationship with shore height was observed in this micro-habitat for the three species, possibly reflecting greater recruitment, survival, and growth lower down (Guerra and Gaudencio, 1986). The other highlighted relationships outside rockpools were the following positive associations: i) P. ulyssiponensis with cover of both articulated coralline algae and CCA; ii) P. depressa with mussel cover and the factor shore; and iii) S. pectinata with crustose non-coralline algae. Adults of S. pectinata living outside rockpools in SW Portugal are very conspicuous within Ralfsia verrucosa and Nemoderma tingitanum patches at low and lower-mid shore open-rock (personal observations). This association might suggest promotion of settlement, growth, and/or survival of these algae by S. pectinata as described for S. sirius and Ralfsia crusts (Iwasaki, 1993 in Hodgson, 1999) or mutualism as found between P. longicosta and Ralfsia verrucosa (McQuaid and Froneman, 1993).
The influence of CCA, namely, “Lithothamia” sensu Hawkins and Jones (1992), on the recruitment of North-East Atlantic patellids both inside and outside pools has been described (Bowman, 1981; Delany et al., 2002; Seabra et al., 2019; Castejón et al., 2021). Patella ulyssiponensis among Corallina spp. on the open-rock, and patellid juveniles among mussels inside and outside pools were previously observed in Portugal (Guerra and Gaudencio, 1986). Articulated coralline algae are an important constituent of the flora of rockpools and lower-shore open-rock (Evans, 1957; Boaventura et al., 2002c; Firth and Crowe, 2008), often forming a turf full of small mussels at the thalli base (personal observations). Thus, the association with these algae inside and outside pools might be due to an association with mussels. Mytilus galloprovincialis patches retaining moisture on the open-rock of Oliveirinha was the unique CCA-absent habitat with abundant P. ulyssiponensis juveniles (Seabra et al., 2019), also important for P. depressa juveniles (Seabra et al., 2020).
In EDGE models, reciprocal negative relationships were established between the two patellids, and the abundance of one species outside pools was negatively related to the abundance of its congener at pool edges. A positive relationship was also established between the abundance of S. pectinata on pool-edges and the abundance of P. depressa within pools. Moreover, the greater abundances of P. depressa and P. ulyssiponensis outside pools were negatively related to S. pectinata on pool edges. As EDGE was an interface, most of the other relevant predictors were common to the ones selected for inside-pool and open-rock models, such as shore height that indicated a negative relationship with the abundance of both patellids at pool edges or the very-low confinement associated with a greater abundance of S. pectinata at pool edges.
4.6 Concluding remarks
Limpets of the three most common species in SW Portugal co-occur in connected populations across a landscape of rockpools, pool edges, and open-rock micro-habitats. Rockpools create a mosaic of micro-habitats that differ most when the tide is out (Huggett and Griffiths, 1986), likely sharpening ecological edge effects with the surrounding rock; pools can also create patchiness when the tide is in due to larval settlement (Castejón et al., 2021) or to foraging activity (Noël et al., 2009). Various species of limpets use these micro-habitats differentially, due to individual preferences on habitat-composition and physiological optima that can change ontogenetically (Davies, 1970; Delany et al., 1998; Delany et al., 2002). The three most common species in SW Portugal use rockpools as nursery grounds (Seabra et al., 2020). Subsequently, largely permanent residence into pools (P. ulyssiponensis—except on the low shore) or gradual emergence onto open-rock (P. depressa and S. pectinata) likely happen. Thus, different species have differential occupancy across highly localized horizontal gradients and edge transitions of rockpool systems, which are superimposed on their vertical and among-shore patterns at broader scales. Shore topography ultimately creates landscape variability, which provides nursery areas (Seabra et al., 2020) or refuges (Sun et al., 2023) for limpets, allows coexistence of different limpet species (Firth and Crowe, 2010), and boosts biodiversity (Firth et al., 2014), thereby contributing to ecosystem functioning (Griffin et al., 2010). Our study suggests that rockpool-generated mosaics are strongly linked by intra-specific connectivity of limpet populations with patterns modulated by interactions between different limpet species at rockpool edges. Much further experimental work is required to explore the underlying causes of the described patterns and associations with habitat-composition predictors revealed in our study, including the effects of limpets on rockpool communities (as their role as keystone species has not been studied for rockpool micro-habitats in Portugal). The importance of rockpools for patellids harvested in SW Portugal may have implications for regional monitoring programs in intertidal systems, assessing effectiveness of protection in special areas of the marine park where fishing is totally or partially forbidden and other human activities are regulated (Castro et al., 2021). More attention should be given to the role of rockpools in sustaining limpet populations in the context of anthropogenic disturbance including harvesting, as part of biodiversity surveillance and conservation of temperate reefs.
Data availability statement
The raw data supporting the conclusions of this article will be made available by the authors, without undue reservation.
Ethics statement
Ethical review and approval was not required for the study on animals in accordance with the local and EU legislation, and institutional requirements. Limpets are not included in the listing of animals under article 2nd of the Directive n.° 2010/63/UE, of the European Parliament and Conseil from 22nd September 2010. The work was descriptive and not involving sacrifice or stress to the animals, as sampling was a completely descriptive and non-destructive survey.
Author contributions
MS, TC, and SH conceived the idea and designed the study. MS was responsible for data collection and visualization and wrote the first draft of the manuscript. NP was involved in field surveys, data curation, and manuscript preparation. TC and MS analyzed the data. TC and SH participated in data interpretation and manuscript writing also providing supervision. All authors read and commented on the manuscript. All authors contributed to the article and approved the submitted version.
Funding
This study had the support of FCT through the strategic project UIDB/04292/2020 awarded to MARE and through project LA/P/0069/2020 granted to the Associate Laboratory ARNET and the doctoral grant awarded to MIS (SFRH/BD/16693/2004). SJH was funded by the Marine Biological Association of the UK (Dept 40).
Acknowledgments
We would like to express our gratitude to the scientific illustrator Ivan Gromicho, for the drawings of limpets presented in Figures 2–10. We thank volunteer students (Verónica Sanita, Ricardo Branca, Tiago Neves, and Francisco Calado) that help on sampling of physical variables. We also thank to the two reviewers for their constructive comments, which considerably improved the first version of the manuscript. Professor M. J. Anderson provided advice on statistics during the manuscript revision. During revison, Dr. João J. Castro provided advice on issues related to regional conservation and management.
Conflict of interest
The authors declare that the research was conducted in the absence of any commercial or financial relationships that could be construed as a potential conflict of interest.
Publisher’s note
All claims expressed in this article are solely those of the authors and do not necessarily represent those of their affiliated organizations, or those of the publisher, the editors and the reviewers. Any product that may be evaluated in this article, or claim that may be made by its manufacturer, is not guaranteed or endorsed by the publisher.
Supplementary material
The Supplementary Material for this article can be found online at: https://www.frontiersin.org/articles/10.3389/fmars.2023.1206159/full#supplementary-material
Supplementary Figure S1 | Densities and size-class structure of Patella vulgata on six shores within POOL (inside-rockpools), EDGE (edge of rockpools) and NEAR (open-rock adjacent to rockpools) micro-habitats (three left columns); and within areas of mid-shore open-rock at two proximity categories from rockpools (MidNEAR and FAR, far right column). Grey and white bars are mean (± SE) number of limpets of five size-classes per 50x50 cm; Note different y-axis scale between graphs of POOL vs. others; number of replicates indicated in caption of Figure 2. Black and white bars (bottom row) are percentages of every size-class relatively to the total density of this species (value given top right of each graph) within each micro-habitat and across all shores.
Supplementary Figure S2 | Densities and size-class structure of Patella rustica on six shores within POOL (inside-rockpools), EDGE (edge of rockpools) and NEAR (open-rock adjacent to rockpools) micro-habitats (three left columns); and within areas of mid-shore open-rock at two proximity categories from rockpools (MidNEAR and FAR, far right column). Grey and white bars are mean (± SE) number of limpets of five size-classes per 50x50 cm; number of replicates indicated in caption of Figure 2. Black and white bars (bottom row) are percentages of every size-class relatively to the total density of this species (value given top right of each graph) within each micro-habitat and across all shores.
Supplementary Figure S3 | Mean proportion of occurrence of emersed open-rock, rockpools, crevices, overhangs, and channels on the mid-shore of twelve coastal stretches (two random stretches, (A, B), within each of six shores). Number of replicates within each stretch varied between 2 and 4 transects parallel to the sea.
Supplementary Figure S4 | Percentage cover of space-occupying categories within the POOL micro-habitat. Values are mean % cover (± SE) of each substratum type or functional group of sessile organisms recorded inside-rockpools on six shores. The number of replicates in each shore was the following: 52 in Oliveirinha, 51 in Queimado, 57 in Almograve, 56 in Cabo Sardão, 53 in Alteirinhos and 54 in Monte Clérigo.
Supplementary Figure S5 | Percentage cover of space-occupying categories (substratum types and functional groups of sessile organisms) within NEAR micro-habitat. Values are mean (± SE) cover estimates assessed on open-rock surfaces of six shores using the following semi-quantitative index (and respective percent cover intervals): 0 (not found); 1 (< 1%); 2 (1-5%); 3 (5-10%); 4 (10-25%); 5 (25-50%); 6 (50-75%); 7 (75-90%); 8 (90-95%); 9 (> 95%). The number of replicates in each shore was the following: 52 in Oliveirinha, 51 in Queimado, 57 in Almograve, 56 in Cabo Sardão, 53 in Alteirinhos and 54 in Monte Clérigo.
Supplementary Figure S6 | Densities (mean ± SE) of the total number of trochids (including the species Steromphala umbilicalis, Steromphala pennanti, Phorcus sauciatus, Phorcus lineatus) and the total number of littorinids Melarhaphe neritoides within POOL and NEAR micro-habitats. The number of replicates in each shore was the following: 52 in Oliveirinha, 51 in Queimado, 57 in Almograve, 56 in Cabo Sardão, 53 in Alteirinhos and 54 in Monte Clérigo.
Supplementary Figure S7 | Physical variables of rockpool-systems on six shores of SW Portugal (see Table 1 for description of each variable). The number of replicates in each shore was the following: 52 in Oliveirinha, 51 in Queimado, 57 in Almograve, 56 in Cabo Sardão, 53 in Alteirinhos and 54 in Monte Clérigo. For clarity, outliers were not shown for four variables: NEAR topographic complexity, surface-area, volume and roundness. Physical variables were used as predictors in DistLM models for which the response-variables were size-class densities of a limpet species present within each of three neighboring micro-habitats of rockpool-systems: inside-rockpools (POOL), rockpool-edges (EDGES), and surrounding emersed open-rock (NEAR).
SUPPLEMENTARY TABLE S1 | Definition and measurement details of all predictive-variables of three groups (physical, habitat-composition and connectivity) assessed for every sampled rockpool (n = 323). Under the column “Micro-habitat”, “only” is indicated for variables that refer exclusively to one micro-habitat, whereas “All” identifies those variables that were considered as physical descriptors of each rockpool-system across POOL, EDGE, and NEAR micro-habitats.
SUPPLEMENTARY TABLE S2 | List of all predictive-variables used to build the distance-based linear model (DistLM) for each of the three species (Patella ulyssiponensis – PU; P. depressa – PD; Siphonaria pectinata – SP) within each of the three micro-habitats (POOL; EDGE; NEAR). “Transf.” refers to transformation applied as pre-treatment before DistLM analyses; “sqrt” refers to square root transformation; * refers to a variable (maximum-depth) that was eliminated due to collinearity with another variable (mean-depth).
SUPPLEMENTARY TEXT | Methodological details of distance-based linear models (DistLM) to examine the relationship between the spatial structure of individual limpet species within each micro-habitat and several predictors (physical, habitat-composition, and connectivity variables).
References
Aguilera M. A., Navarrete S. A. (2011). Distribution and activity patterns in an intertidal grazer assemblage: influence of temporal and spatial organization on interspecific associations. Mar. Ecol. Prog. Ser. 431, 119–136. doi: 10.3354/meps09100
Aguilera M. A., Navarrete S. A. (2012). Inter-specific competition for shelters in territorial and gregarious intertidal grazers: consequences for individual behaviour. PloS One 7 (9), e46205. doi: 10.1371/journal.pone.0046205
Aguilera M. A., Valdivia N., Broitman B. R. (2013). Spatial niche differentiation and coexistence at the edge: co-occurrence distribution patterns in Scurria limpets. Mar. Ecol. Prog. Ser. 483, 185–198. doi: 10.3354/meps10293
Aguilera M. A., Weiß M., Thiel M. (2019). Similarity in predator-specific anti-predator behavior in ecologically distinct limpet species, Scurria viridula (Lottiidae) and Fissurella latimarginata (Fissurellidae). Mar. Biol. 166, 1–13. doi: 10.1007/s00227-019-3485-5
Airoldi L., Hawkins S. J. (2007). Negative effects of sediment deposition on grazing activity and survival of the limpet Patella vulgata. Mar. Ecol. Prog. Ser. 332, 235–240. doi: 10.3354/meps332235
Anderson M. J. (2001). Permutation tests for univariate or multivariate analysis of variance and regression. Can. J. Fish. Aquat. 58 (3), 626–639. doi: 10.1139/f01-00
Anderson M., Gorley R. N., Clarke K. (2008). PERMANOVA+ for primer: Guide to software and statistical methods (Plymouth, UK: PRIMER-E).
Araújo R., Sousa-Pinto I., Bárbara I., Quintino V. (2006). Macroalgal communities of intertidal rock pools in the northwest coast of Portugal. Acta Oecol. 30 (2), 192–202. doi: 10.1016/j.actao.2006.04.002
Benedetti-Cecchi L., Cinelli F. (1995). Habitat heterogeneity, sea urchin grazing and the distribution of algae in littoral rock pools on the west coast of Italy (western Mediterranean). Mar. Ecol. Prog. Ser. 126, 203–212. doi: 10.3354/meps126203
Benedetti-Cecchi L., Cinelli F. (1996). Patterns of disturbance and recovery in littoral rock pools: nonhierarchical competition and spatial variability in secondary succession. Mar. Ecol. Prog. Ser. 135, 145–161. doi: 10.3354/meps135145
Bertocci I., Araújo R., Incera M., Arenas F., Pereira R., Abreu, et al. (2012). Benthic assemblages of rock pools in northern Portugal: seasonal and between-pool variability. Sci. Mar. 76 (4), 781–789. doi: 10.3989/scimar.03669.21A
Blackmore D. T. (1969). Studies of Patella vulgata L. Growth, reproduction and zonal distribution. J. Exp. Mar. Biol. Ecol. 3 (2), 200–213. doi: 10.1016/0022-0981(69)90018-5
Boaventura D., Alexander M., Della Santina P., Smith N. D., Ré P., da Fonseca L. C., et al. (2002c). The effects of grazing on the distribution and composition of low-shore algal communities on the central coast of Portugal and on the southern coast of Britain. J. Exp. Mar. Biol. Ecol. 267 (2), 185–206. doi: 10.1016/S0022-0981(01)00372-0
Boaventura D., da Fonseca L. C., Hawkins S. J. (2002a). Analysis of competitive interactions between the limpets Patella depressa Pennant and Patella vulgata L. on the northern coast of Portugal. J. Exp. Mar. Biol. Ecol. 271 (2), 171–188. doi: 10.1016/S0022-0981(02)00044-8
Boaventura D., Da Fonseca L. C., Hawkins S. J. (2003). Size matters: competition within populations of the limpet Patella depressa. J. Anim. Ecol. 72 (3), 435–446. doi: 10.1046/j.1365-2656.2003.00713.x
Boaventura D., Ré P., Da Fonseca L. C., Hawkins S. J. (2002b). Intertidal rocky shore communities of the continental Portuguese coast: Analysis of distribution patterns. Mar. Ecol. 23, 69–90. doi: 10.1046/j.1439-0485.2002.02758.x
Bowman R. S. (1981). The morphology of Patella spp. juveniles in Britain, and some phylogenetic inferences. J. Mar. Biolog. Assoc. U. K. 61 (3), 647–666. doi: 10.1017/S0025315400048104
Bowman R. S. (1985). “The biology of the limpet Patella vulgata L. in the British Isles: spawning time as a factor determining recruitment success,” in The Ecology of Rocky Coasts: essays presented to JR Lewis, D. Sc. Eds. Moore P. G., Seed R. (London: Hodder and Stoughton), 178–193.
Branch G. M. (1971). The ecology of Patella Linnaeus from the cape Peninsula, South Africa I. Zonation, movements and feeding. Afr. Zool. 6 (1), 1–38. doi: 10.1080/00445096.1971.11447402
Branch G. M. (1975). Mechanisms reducing intra-specific competition in Patella spp.: migration, differentiation and territorial behaviour. J. Anim. Ecol. 44 (2), 575–600. doi: 10.2307/3612
Branch G. M. (1976). Inter-specific competition experienced by South African Patella species. J. Anim. Ecol. 45 (2), 507–529. doi: 10.2307/3888
Branch G. M. (1981). The biology of limpets: physical factors, energy flow, and ecological interactions. Ann. Rev. Mar. Sci. 19, 235–380. http://pascal-francis.inist.fr/vibad/index.php?action=getRecordDetail&idt=PASCALZOOLINEINRA82X0007825.
Branch G. M., Marsh A. C. (1978). Tenacity and shell shape in six Patella species: adaptive features. J. Exp. Mar. Biol. Ecol. 34 (2), 111–130. doi: 10.1016/0022-0981(78)90035-7
Branch G. M., Trueman E. R., Clarke M. R. (1985). “Limpets: evolution and adaptation,” in The Mollusca Vol. 10. Eds. Trueman E. R., Clarke M. R. (FL: Academic Press, Inc), 187–220.
Broitman B. R., Aguilera M. A., Lagos N. A., Lardies M. A. (2018). Phenotypic plasticity at the edge: Contrasting population-level responses at the overlap of the leading and rear edges of the geographical distribution of two Scurria limpets. J. Biogeogr. 45 (10), 2314–2325. doi: 10.1111/jbi.13406
Burrows M. T., Hawkins S. J. (1998). Modelling patch dynamics on rocky shores using deterministic cellular automata. Mar. Ecol. Prog. Ser. 167, 1–13. doi: 10.3354/meps167001
Cabral J. P., Simões J. (2007). The southern limit of distribution of Patella vulgata. Iberus 25, 57–75. doi: 10.5281/zenodo.4532807
Carneiro A. P. (2021). Diversity and Distribuition of Patellid Limpets Along the Southwestern African Coast (Benguela Current) (Faro (PT: Universidade do Algarve).
Carvalho J., Mendonça V., Vinagre C., Silva A. (2021). Environmental factors impacting the abundance and distribution of amphipods in intertidal rock pools. J. SeaRes. 172, 102035. doi: 10.1016/j.seares.2021.102035
Casal G., Aceña-Matarranz S., Fernández-Márquez D., Fernández N. (2018). Distribution and abundance patterns of three coexisting species of Patella (Mollusca Gastropoda) in the intertidal areas of the NW Iberian Peninsula: Implications for management. Fish. Res. 198, 86–98. doi: 10.1016/j.fishres.2017.10.011
Castejón D., Nogueira N., Andrade C. A. (2021). Limpet larvae (Patella aspera Röding 1798), obtained by gonad dissection and fecundation in vitro, settled and metamorphosed on crustose coralline algae. J. Mar. Biolog. Assoc. U. K. 101 (7), 991–1002. doi: 10.1017/S0025315421000916
Castro J. J., Quintella B. R., Afonso C. M. L., Almeida P. R., Andrade M., Antunes, et al. (2021). Relatório final de execução, modelo de monitorização e medidas de gestão. Relatório, projeto MARSW Sistemas de Informação e Monitorização da Biodiversidade Marinha das Áreas Classificadas do Sudoeste Alentejano e Costa Vicentina (Sines: Universidade de Évora, Faculdade de Ciências da Universidade de Lisboa, MARE – Centro de Ciências do Mar e do Ambiente, CCMAR – Centro de Ciências do Mar e Universidade do Algarve).
Castro J. J., Quintella B. R., Almeida P. R., Antunes A. C., Antunes M., Bentes L., et al. (2020). Caracterização da pesca na área marinha do Parque Natural do Sudoeste Alentejano e Costa Vicentina. Relatório, projeto MARSW – Sistemas de Informação e Monitorização da Biodiversidade Marinha das Áreas Classificadas do Sudoeste Alentejano e Costa Vicentina (Sines: Universidade de Évora, Faculdade de Ciências da Universidade de Lisboa, MARE – Centro de Ciências do Mar e do Ambiente, CCMAR – Centro de Ciências do Mar e Universidade do Algarve).
CIEMAR (2018). Substrato duro intertidal. Monitorização de Ambientes Marinhos do Porto de Sines - MAPSi 2015/2017. Relatório final (Sines: Laboratório de Ciências do Mar da Universidade de Évora).
Clarke K. R. (1993). Non‐parametric multivariate analyses of changes in community structure. Austral Ecol. 18 (1), 117–143. doi: 10.1111/j.1442-9993.1993.tb00438.x
Clarke K. R., Gorley R. N. (2015). Getting started with PRIMER v7 Vol. 20 (Plymouth: PRIMER-E: Plymouth, Plymouth Marine Laboratory), 1.
Coleman R. A., Underwood A. J., Benedetti-Cecchi L., Aberg P., Arenas F., Arrontes J., et al. (2006). A continental scale evaluation of the role of limpet grazing on rocky shores. Oecologia 147, 556–564. doi: 10.1007/s00442-005-0296-9
Creese R. G. (1980). An analysis of distribution and abundance of populations of the high-shore limpet, Notoacmea petterdi (Tenison-Woods). Oecologia 45, 252–260. doi: 10.1007/BF00346466
Creese R. G., Underwood A. J. (1982). Analysis of inter-and intra-specific competition amongst intertidal limpets with different methods of feeding. Oecologia 53, 337–346. doi: 10.1007/BF00389010
Davies P. S. (1970). Physiological ecology of Patella IV. Environmental and limpet body temperatures. J. Mar. Biolog. Assoc. U. K. 50 (4), 1069–1077. doi: 10.1017/S0025315400005956
Delany J., Myers A. A., McGrath D. (1998). Recruitment, immigration and population structure of two coexisting limpet species in mid-shore tidepools, on the west coast of Ireland. J. Exp. Mar. Biol. Ecol. 221 (2), 221–230. doi: 10.2307/1942457
Delany J., Myers A. A., McGrath D. (2002). “A comparison of the interactions of the limpets Patella vulgata and Patella ulyssiponensis with crustose coralline algae,” in New survey of Clare Island: v. 3: marine intertidal ecology. Ed. Myers A. (Dublin: The Royal Irish Academy) 3, 79–90.
Dethier M. N. (1984). Disturbance and recovery in intertidal pools: maintenance of mosaic patterns. Ecol. Monogr. 54 (1), 99–118. doi: 10.2307/1942457
Evans R. G. (1947). “Studies on the Biology of British Limpets,” in Proceedings of the Zoological Society of London (Oxford, UK: Blackwell Publishing Ltd), 411–423. doi: 10.1111/j.1096-3642.1953.tb00179.x
Evans R. G. (1957). The intertidal ecology of some localities on the Atlantic coast of France. J. Ecol. 45 (1), 245–271. doi: 10.2307/2257088
Femino R. J., Mathieson A. C. (1980). Investigations of New England marine algae IV: The ecology and seasonal succession of tide pool algae at Bald Head Cliff, York, Maine, USA. Bot. Mar. 23, 319–332. Available at: http://pascal-francis.inist.fr/vibad/index.php?action=getRecordDetail&idt=PASCAL8010470072.
Firth L. B., Crowe T. P. (2008). Large-scale coexistence and small-scale segregation of key species on rocky shores. Hydrobiologia 614, 233–241. doi: 10.1007/s10750-008-9509-7
Firth L. B., Crowe T. P. (2010). Competition and habitat suitability: small-scale segregation underpins large-scale coexistence of key species on temperate rocky shores. Oecologia 162, 163–174. doi: 10.1007/s00442-009-1441-7
Firth L. B., Crowe T. P., Moore P., Thompson R. C., Hawkins S. J. (2009). Predicting impacts of climate-induced range expansion: an experimental framework and a test involving key grazers on temperate rocky shores. Glob. Change Biol. 15 (6), 1413–1422. doi: 10.1111/j.1365-2486.2009.01863.x
Firth L. B., Schofield M., White F. J., Skov M. W., Hawkins S. J. (2014). Biodiversity in intertidal rock pools: Informing engineering criteria for artificial habitat enhancement in the built environment. Mar. Environ. Res. 102, 122–130. doi: 10.1016/j.marenvres.2014.03.016
Freitas D., Arenas F., Vale C. G., Pinto I. S., Borges D. (2023a). Warning of warming limpets: sea temperature effects upon intertidal rocky assemblages. J. Mar. Biolog. Assoc. U. K. 103, e7. doi: 10.1017/S0025315422001096
Freitas D., Borges D., Arenas F., Pinto I. S., Vale C. G. (2023b). Forecasting distributional shifts of Patella spp. in the Northeast Atlantic Ocean, under climate change. Mar. Environ. Res. 186, 105945. doi: 10.1016/j.marenvres.2023.105945
Fretter V., Graham A. (1994). British prosobranch molluscs, their functional anatomy and ecology (London, UK: The Ray Society). doi: 10.1017/S0025315400090299
Garrity S. D. (1984). Some adaptations of gastropods to physical stress on a tropical rocky shore. Ecology 65 (2), 559–574. doi: 10.2307/1941418
Giesel J. T. (1970). On the maintenance of a shell pattern and behavior polymorphism in Acmaea digitalis, a limpet. Evolution 24 (1), 98–119. doi: 10.2307/2406717
Gomes N., Antunes C., Costa D. D. A. (2022). Insights into the Migration Routes and Historical Dispersion of Species Surviving the Messinian Crisis: The Case of Patella ulyssiponensis and Epizoic Rhodolith Lithophyllum hibernicum. Hydrobiology 1 (1), 10–38. doi: 10.3390/hydrobiology1010003
Gonçalves I., Vinagre C., Silva A. (2023). Patterns of rockpool molluscs in differing shore exposures. Mar. Biol. 170 (6), 77. doi: 10.1007/s00227-023-04223-2
Goss-Custard S., Jones J., Kitching J. A., Norton T. A. (1979). “Tide pools of Carrigathorna and Barloge Creek,” in Philosophical Transactions of the Royal Society of London, vol. 287. (London, UK: B, Biological Sciences), 1–44. doi: 10.1098/rstb.1979.0051
Gray D. R., Hodgson A. N. (1998). Foraging and homing behaviour in the high-shore, crevice-dwelling limpet Helcion pectunculus (Prosobranchia: Patellidae). Mar. Biol. 132, 283–294. doi: 10.1007/s002270050394
Green J. M. (1971). Local distribution of Oligocottus maculosus Girard and other tidepool cottids of the west coast of Vancouver Island, British Columbia. Can. J. Zool. 49 (8), 1111–1128. doi: 10.1139/z71-172
Griffin J. N., Laure M. L. N., Crowe T. P., Burrows M. T., Hawkins S. J., Thompson R. C., et al. (2010). Consumer effects on ecosystem functioning in rock pools: roles of species richness and composition. Mar. Ecol. Prog. Ser. 420, 45–56. doi: 10.3354/meps08844
Guerra M. T., Gaudencio M. J. (1986). Aspects of the ecology of Patella spp. on the Portuguese coast. Hydrobiologia 142, 57–69. doi: 10.1007/BF00026747
Hartnoll R. G., Hawkins S. J. (1985). Patchiness and fluctuations on moderately exposed rocky shores. Ophelia 24 (1), 53–63. doi: 10.1080/00785236.1985.10426619
Hawkins S. J., Hartnoll R. G. (1983). Grazing of intertidal algae by marine invertebrates. Oceanogr. Mar. Biol. 21, 195–282.
Hawkins S. J., John D. M., Price J. H. (1992). Plant-animal interactions on hard substrata in the northeast Atlantic. In: Eds. John D. M., Hawkins S. J., Price J. H. Plant-animal interactions in the marine benthos (Systematics Association). (Oxford: Oxford University Press), 1–32.
Hawkins S. J., Jones H. D. (1992). Marine Conservation Society Marine Field Course Guide 1 (London: Immel).
Hawkins S. J., Pack K. E., Firth L. B., Mieskowska N., Evans A. J., Martins G. M., et al. (2019). “The intertidal zone of the North-East Atlantic region: pattern and process,” in Interactions in the marine benthos: global patterns and processes. Eds. Hawkins S. J., Bohn K., Firth L. B., Williams G. A. (Cambridge: Cambridge University Press), 7–46. doi: 10.1017/9781108235792.003
Henriques P., Delgado J., Sousa R., Ray S. (2017). “Patellid limpets: an overview of the biology and conservation of keystone species of the rocky shores,” in Organismal and molecular malacology (London: IntechOpen Limited), 71–95. doi: 10.5772/67862
Hodgson G. (1999). The biology of siphonariid limpets (Gastropoda: Pulmonata). Oceanogr. Mar. Biol. 37, 245–314.
Horta e Costa B., Sousa I., Belackova A., Henriques N. S., Rangel M., Oliveira, et al. (2018). Relatório de Síntese da Biodiversidade Marinha da área marinha do Parque Natural do Sudoeste Alentejano e Costa Vicentina (Faro: Relatório técnico do Projecto MARSW).
Huggett J., Griffiths C. L. (1986). Some relationships between elevation, physico-chemical variables and biota of intertidal rock pools. Mar. Ecol. Prog. Ser. 29, 189–197. doi: 10.3354/MEPS029189
Iwasaki K. (1993). “Individual variation, social structure, community organization and hierarchical views in the marine context” in Mutualism and community organization: behavioural, theoretical and food-web approaches Kawanabe H., et al (eds). (Oxford: Oxford University Press), 13–36. (not seen).
Jenkins S. R., Coleman R. A., Della Santina P., Hawkins S. J., Burrows M. T., Hartnoll R. G. (2005). Regional scale differences in the determinism of grazing effects in the rocky intertidal. Mar. Ecol. Prog. Ser. 287, 77–86. doi: 10.3354/meps287077
Jenkins S. R., Norton T. A., Hawkins S. J. (1999). Interactions between canopy forming algae in the eulittoral zone of sheltered rocky shores on the Isle of Man. J. Mar. Biolog. Assoc. U. K. 79 (2), 341–349. doi: 10.3354/meps08729
Johnson M. P. (2001). Metapopulation dynamics of Tigriopus brevicornis (Harpacticoida) in intertidal rock pools. Mar. Ecol. Prog. Ser. 211, 215–224. doi: 10.3354/meps211215
Johnson M. P., Burrows M. T., Hartnoll R. G., Hawkins S. J. (1997). Spatial structure on moderately exposed rocky shores: patch scales and the interactions between limpets and algae. Mar. Ecol. Prog. Ser. 160, 209–215. doi: 10.3354/meps160209
Kooistra W. H. C. F., Joosten A. M. T., Van den Hoek C. (1989). Zonation patterns in intertidal pools and their possible causes: A multivariate approach. Bot. Mar. 32 (2), 9–26. doi: 10.1515/botm.1989.32.1.9
Legrand E., Riera P., Pouliquen L., Bohner O., Cariou T., Martin S. (2018). Ecological characterization of intertidal rockpools: Seasonal and diurnal monitoring of physico-chemical parameters. Reg. Stud. Mar. Sci. 17, 1–10. doi: 10.1016/j.rsma.2017.11.003
Lewis J. R. (1954). Observations on a high-level population of limpets. J. Anim. Ecol. 23 (1), 85–100. doi: 10.2307/1661
Lewis J. R. (1986). Latitudinal trends in reproduction, recruitment and population characteristics of some rocky littoral molluscs and cirripedes. Hydrobiologia 142, 1–13. doi: 10.1007/BF00026742
Lewis J. R., Bowman R. S. (1975). Local habitat-induced variations in the population dynamics of Patella vulgata L. J. Exp. Mar. Biol. Ecol. 17 (2), 165–203. doi: 10.1016/0022-0981(75)90029-5
Lima F. P., Gomes F., Seabra R., Wethey D. S., Seabra M. I., Cruz T., et al. (2016). Loss of thermal refugia near equatorial range limits. Glob. Change Biol. 22 (1), 254–263. doi: 10.1111/gcb.13115
Lima F. P., Queiroz N., Ribeiro P. A., Hawkins S. J., Santos A. M. (2006). Recent changes in the distribution of a marine gastropod, Patella rustica Linnaeus 1758 and their relationship to unusual climatic events. J. Biogeogr. 33 (5), 812–822. doi: 10.1111/j.1365-2699.2006.01457.x
Martins G. M., Hawkins S. J., Thompson R. C., Jenkins S. R. (2007). Community structure and functioning in intertidal rock pools: effects of pool size and shore-height at different successional stages. Mar. Ecol. Prog. Ser. 329, 43–55. doi: 10.3354/meps329043
Marzinelli E. M., Underwood A. J., Coleman R. A. (2012). Modified habitats change ecological processes affecting a non-indigenous epibiont. Mar. Ecol. Prog. Ser. 446, 119–129. doi: 10.3354/meps09472
Masterson P., Arenas F. A., Thompson R. C., Jenkins S. R. (2008). Interaction of top down and bottom up factors in intertidal rockpools: effects on early successional macroalgal community composition, abundance and productivity. J. Exp. Mar. Biol. Ecol. 363 (1-2), 12–20. doi: 10.1016/j.jembe.2008.06.001c
McQuaid C. D., Froneman P. W. (1993). Mutualism between the territorial intertidal limpet Patella longicosta and the crustose alga Ralfsia verrucosa. Oecologia 96, 128–133. doi: 10.1007/BF00318040
Menconi M., Benedetti-Cecchi L., Cinelli F. (1999). Spatial and temporal variability in the distribution of algae and invertebrates on rocky shores in the northwest Mediterranean. J. Exp. Mar. Biol. Ecol. 233 (1), 1–23. doi: 10.3354/meps215079
Metaxas A., Hunt H. L., Scheibling R. E. (1994). Spatial and temporal variability of macrobenthic communities in tidepools on a rocky shore in Nova-Scotia, Canada. Mar. Ecol. Prog. Ser. 105, 89–103. doi: 10.3354/meps105089
Metaxas A., Scheibling R. E. (1993). Community structure and organization of tidepools. Mar. Ecol. Prog. Ser. 98, 187–198. doi: 10.3354/meps098187
Moore P., Hawkins S. J., Thompson R. C. (2007). Role of biological habitat amelioration in altering the relative responses of congeneric species to climate change. Mar. Ecol. Prog. Ser. 334, 11–19. doi: 10.3354/meps334011
Nobre D., Seabra M. I., Cruz T. (2019). Patterns of the vertical distribution limits of the limpet Patella depressa on the Portuguese coast. Front. Mar. Sci. Conf. Abstract.: IMMR’18. | Int. Meeting. Mar. Res. 2018. doi: 10.3389/conf.FMARS.2018.06.00051
Noël L., Hawkins S. J., Jenkins S. R., Thompson R. C. (2009). Grazing dynamics in intertidal rockpool: connectivity in microhabitats. J. Exp. Mar. Biol. Ecol. 370 (1-2), 9–17. doi: 10.1016/j.jembe.2008.11.005
Ocaña T. M. (2003). Growth, mortality and longevity in two populations of Siphonaria pectinata (Pulmonata) at Gibraltar. J. Molluscan. Stud. 69 (2), 162–164. doi: 10.1093/mollus/69.2.162
Oróstica M. H., Hawkins S. J., Broitman B. R., Jenkins S. R. (2020). Performance of a warm-water limpet species towards its poleward range edge compared to a colder-water congener. Mar. Ecol. Prog. Ser. 656, 207–225. doi: 10.3354/meps13461
Ortiz M., Hermosillo-Nuñez B., González J., Rodríguez-Zaragoza F., Gómez I., Jordán F. (2017). Quantifying keystone species complexes: Ecosystem-based conservation management in the King George Island (Antarctic Peninsula). Ecol. Indic. 81, 453–460. doi: 10.1016/j.ecolind.2017.06.016
Orton J. H. (1929). Observations on Patella vulgata. Part III. Habitat and habits. J. Mar. Biolog. Assoc. U. K. 16 (1), 277–288. doi: 10.1017/S0025315400029805
Ostalé-Valriberas E., Sempere-Valverde J., Coppa S., García-Gómez J. C., Espinosa F. (2018). Creation of micro-habitats (tidepools) in ripraps with climax communities as a way to mitigate negative effects of artificial substrate on marine biodiversity. Ecol. Eng. 120, 522–531. doi: 10.1016/j.ecoleng.2018.06.023
Rivera-Ingraham G. A., Espinosa F., García-Gómez J. C. (2011). Effect of γ-amino butyric acid on limpet populations: towards the future management and conservation of endangered patellid species. J. Chem. Ecol. 37, 1–9. doi: 10.1007/s10886-010-9884-1
Rubal M., Veiga P., Cacabelos E., Moreira J., Sousa-Pinto I. (2013). Increasing sea surface temperature and range shifts of intertidal gastropods along the Iberian Peninsula. J. Sea. Res. 77, 1–10. doi: 10.1016/j.seares.2012.12.003
Rubal M., Veiga P., Maldonado C., Torres C., Moreira J. (2015). Population attributes and traits of Siphonaria pectinata (Mollusca: Siphonariidae) in range-edge and non range-edge populations at its Eastern Atlantic northern distribution boundary. J. Exp. Mar. Biol. Ecol. 471, 41–47. doi: 10.1016/j.jembe.2015.05.015
Rubal M., Veiga P., Vieira R., Sousa-Pinto I. (2011). Seasonal patterns of tidepool macroalgal assemblages in the North of Portugal. Consistence between species and functional group approaches. J. Sea. Res. 66 (3), 187–194. doi: 10.1016/j.seares.2011.07.003
Schaefer N., Mayer-Pinto M., Johnston E. L., Dafforn K. A. (2023). Understanding the role of microhabitats in intertidal rock pools to guide future eco-engineering designs. Mar. Biol. 170 (4), 44. doi: 10.1007/s00227-023-04196-2
Seabra M. I., Cruz T., Fernandes J. N., Silva T., Hawkins S. J. (2019). Recruitment of the limpet Patella ulyssiponensis and its relationship with crustose coralline algae: patterns of juvenile distribution and larval settlement. J. Mar. Biolog. Assoc. U. K. 99 (8), 1787–1796. doi: 10.1017/S0025315419000869
Seabra M. I., Hawkins S. J., Espírito-Santo C., Castro J. J., Cruz T. (2020). Rockpools as nurseries for co-existing limpets: Spatial and temporal patterns of limpet recruitment. Reg. Stud. Mar. Sci. 37, 101339. doi: 10.1016/j.rsma.2020.101339
Seabra R., Wethey D. S., Santos A. M., Gomes F., Lima F. P. (2016). Equatorial range limits of an intertidal ectotherm are more linked to water than air temperature. Glob. Change Biol. 22 (10), 3320–3331. doi: 10.1111/gcb.13321
Seabra R., Wethey D. S., Santos A. M., Lima F. P. (2011). Side matters: micro-habitat influence on intertidal heat stress over a large geographical scale. J. Exp. Mar. Biol. Ecol. 400 (1-2), 200–208. doi: 10.1016/j.jembe.2011.02.010
Silva A., Boaventura D., Ré P. (2003). Population structure, recruitment and distribution patterns of Patella depressa Pennant 1777 on the central Portuguese coast. Bol. Inst. Esp. Oceanogr. 19 (1/4), 461.
Slama T., Abidli S., El Menif N. T., Lahbib Y. (2022). Grazer gastropods as alternative species for monitoring butyltins contamination in harbors. J. Sea. Res. 190, 102287. doi: 10.1016/j.seares.2022.102287
Slama T., Nouet J., Chassefière E., El Menif N. T., Lahbib Y. (2021). Monitoring of coastal pollution using shell alterations in the false limpet Siphonaria pectinata. Mar. pollut. Bull. 173, 113063. doi: 10.1016/j.marpolbul.2021.113063
Sokal R. R., Rohlf F. J. (1995). Biometry: The Principles and Practice of Statistics in Biological Research. 3rd Edition (New York: W.H. Freeman and Co.).
Sorensen F. E., Lindberg D. R. (1991). Preferential predation by American black oystercatchers on transitional ecophenotypes of the limpet Lottia pelta (Rathke). J. Exp. Mar. Biol. Ecol. 154 (1), 123–136. doi: 10.1016/0022-0981(91)90078-B
Souza S. M., Matthews-Cascon H. (2019). Molluscan assemblages in rock pools on sandstone reefs: local and between pools variability. J. Shellfish. Res. 38 (1), 201–208. doi: 10.2983/035.038.0119
Sun Y. X., Hu L. S., Dong Y. W. (2023). Micro-habitat-specific diurnal metabolomic responses of the intertidal limpet Cellana toreuma to winter low temperature. iScience 26, 3. doi: 10.1016/j.isci.2023.106128
Tanner J. E., Hughes T. P., Connell J. H. (1994). Species coexistence, keystone species, and succession: a sensitivity analysis. Ecology 75 (8), 2204–2219. doi: 10.2307/1940877
Thompson G. B. (1979). Distribution and population dynamics of the limpet Patella aspera (Lamarck) in Bantry Bay. J. Exp. Mar. Biol. Ecol. 40 (2), 115–135. doi: 10.1016/0022-0981(79)90039-X
Thompson G. B. (1980). Distribution and population dynamics of the limpet Patella vulgata L. @ in Bantry Bay. J. Exp. Mar. Biol. Ecol. 45 (2), 173–217. doi: 10.1016/0022-0981(80)90058-1
Underwood A. J., Skilleter G. A. (1996). Effects of patch-size on the structure of assemblages in rock pools. J. Exp. Mar. Biol. Ecol. 197 (1), 63–90. doi: 10.1016/0022-0981(95)00145-X
Vasconcelos P., Janeiro F., Pereira F., Moura P., Carvalho A. N., Gaspar M. B. (2021). Shell shape, morphometrics and relative growth of four sympatric limpet species from the Algarve coast (southern Portugal). J. Mar. Biolog. Assoc. U. K. 101 (5), 791–800. doi: 10.1017/S0025315421000734
Vermeij G. J. (2017). The limpet form in gastropods: evolution, distribution, and implications for the comparative study of history. Biol. J. Linn. Soc Lond. 120 (1), 22–37. doi: 10.1111/bij.12883
Williams G. A., Morritt D. (1995). Habitat partitioning and thermal tolerance in a tropical limpet, Cellana grata. Mar. Ecol. Prog. Ser. 124, 89–103. doi: 10.3354/meps124089
Wolcott T. G. (1973). Physiological ecology and intertidal zonation in limpets (Acmaea): a critical look at” limiting factors”. Biol. Bull. 145 (2), 389–422. doi: 10.2307/1540048
Keywords: limpet populations, rockpools/tidepools, emergent substrata, habitat connectivity, patellids, siphonariids, mosaic landscapes, co-existence
Citation: Seabra MI, Penteado N, Cruz T and Hawkins SJ (2023) Variability and connectivity in populations of different limpet species across rockpool-generated mosaic landscapes. Front. Mar. Sci. 10:1206159. doi: 10.3389/fmars.2023.1206159
Received: 24 April 2023; Accepted: 13 October 2023;
Published: 04 December 2023.
Edited by:
Stephen J. Newman, Western Australian Fisheries and Marine Research Laboratories, AustraliaReviewed by:
Paul Brewin, South Atlantic Environmental Research Institute, Falkland IslandsYunwei Dong, Ocean University of China, China
Copyright © 2023 Seabra, Penteado, Cruz and Hawkins. This is an open-access article distributed under the terms of the Creative Commons Attribution License (CC BY). The use, distribution or reproduction in other forums is permitted, provided the original author(s) and the copyright owner(s) are credited and that the original publication in this journal is cited, in accordance with accepted academic practice. No use, distribution or reproduction is permitted which does not comply with these terms.
*Correspondence: Maria Inês Seabra, aXNlYWJyYUB1ZXZvcmEucHQ=
†These authors share last authorship