Corrigendum: When the population of an endangered marine mollusc (Patella ferruginea) increases almost three-fold in ten years. Reality or fiction?
- Laboratorio de Biología Marina de la Universidad de Sevilla (LBMUS)/ Área de Investigación I+D+i del Acuario de Sevilla/ Estación de Biología Marina del Estrecho (Ceuta), Universidad de Sevilla, Seville, Spain
The critically endangered species Patella ferruginea (Gastropoda, Patellidae), endemic to the western Mediterranean, has breeding populations in both natural and artificial habitats, the latter of which are generally linked to port infrastructures. Over the past decade, the temporal change of this species’ population has been monitored (structure and density) using exhaustive censuses along Ceuta’s coast (Strait of Gibraltar), one of the few stronghold populations within the entire Mediterranean basin. This study focuses on the population dynamics of P. ferruginea in Ceuta and the environmental factors that affect the structure of this population, such as wave exposure, coastline heterogeneity, substratum roughness, substratum lithology, and chlorophyll-a concentration. Different potential negative interactions were also considered: angling, shell fishing, bathing in the intertidal, bathing near the intertidal, recreational boating and temporary migrant campsites nearby. The results have shown in the period 2011-2021, the estimated size of P. ferruginea population has increased by 200 %, from 55,902 to 168,463 individuals (of which 131,776 are adults). The subpopulation with the greatest increase in these years was the one settled on dolomitic rip-raps inside the Ceuta’s harbor, with an increase of 1,288%. The results of the present study indicate that Ceuta hosts the main population of this endangered species through its distributional range (Western Mediterranean), being a source population on the Southern Iberian Peninsula that its preservation must be prioritized. Statistical modelling has shown that the adult density of P. ferruginea is positively influenced by coastal heterogeneity, habitat area and substratum roughness, but negatively by vertical inclination, concentration of chlorophyll-a, and anthropogenic impact. These results also support the concept of ¨Artificial Marine Micro-Reserves¨ as a new area-based conservation measure according with the IUCN guidelines, as these will contribute to setting up a network of these source populations that promote genetic flow among populations, with eventual recolonization throughout its original distribution.
1 Introduction
Coastal areas host half of the world’s cities and more than half of the world’s population, with population density near the coast three times higher than the global average (Creel, 2003; Small and Nicholls, 2003; Airoldi and Beck, 2007). Shoreline urbanization combined with the increase of tourist, recreational and commercial activities, has resulted in the introduction and proliferation of artificial structures in marine coastal habitats worldwide (Bulleri and Chapman, 2010; Firth et al., 2016). The human impact associated with these structures has been reported to affect biological processes on all spatial and temporal scales (Airoldi et al., 2005). Among them, fragmentation of natural habitats by urbanization processes can cause a reduction in population size and connectivity of species, and thus increase their risk of extinction in the face of certain permanent disturbances (Aguilera et al., 2020; Chase et al., 2020).
Limpets are considered keystone species in rocky coastal ecosystems as they maintain the composition and structure of the biological community and, when they disappear from their habitats, cause a cascading effect on them (Raffaelli and Hawkins, 1996). Therefore, limpets may be the most important group of grazers in temperate rocky intertidal zones, because their feeding activity limits the growth of macroalgae and controls barnacle numbers (Branch, 1981).
Among limpets, the larger species are frequently the most ecologically relevant, being defined as ‘giant limpets’ (shell length greater than 100 mm). With this classification a total of 14 species in the world can be found, three of them catalogued as threatened (Espinosa and Rivera-Ingraham, 2017). Patella ferruginea Gmelin, 1791 (Gastropoda: Patellidae) belongs to this classification, as it achieves a maximum recorded shell length of 115 mm (Supplementary Figure 1-S). It is endemic to the Western Mediterranean Sea and it is considered the most endangered marine invertebrate (Ramos, 1998), together with Pinna nobilis, within the European coastline (Vázquez-Luis et al., 2017; Lattos et al., 2021).
Like other limpet species, P. ferruginea is a broadcast spawner with external fertilization so that the urban development of coastal areas has dramatically fragmented its habitat (Airoldi et al., 2005; Firth et al., 2016). Moreover, the conspicuous star‐shaped shell and large sizes that P. ferruginea can reach has made this species the target of human collection since the Quaternary (Espinosa, 2006; Espinosa et al., 2009; Rivera-Ingraham et al., 2011a; Guallart et al., 2013a).
On account of these anthropogenic impacts, its distribution range has progressively been contracted, and P. ferruginea has nearly disappeared from European continental coasts, except for the small and fragmented populations present in Andalusia Southern Spain, Liguria (Italy) and Southern France (Cottalorda et al., 2004; Espinosa et al., 2014b; Luque et al., 2018; Ferranti et al., 2019; CMAOT, 2020). The remaining most notable populations are found in scattered North African gated communities: Ceuta, Melilla, Chafarinas Islands, Habibas Islands, and the island of Zembra (Espinosa et al., 2014b; Luque et al., 2018). In this regard, it has recently been identified that possibly the population located in Ceuta (Strait of Gibraltar, North Africa) plays the role of source population in the area, maintaining nearby sink populations in the south of the Iberian Peninsula (Espinosa et al., 2018) according to the “source-sink population model” (see Pulliam, 1988; Pulliam, 1996).
This study focuses on the population dynamics of P. ferruginea in Ceuta and the environmental factors that shape the population size structure. Consequently, the objectives of the present study are i) to determine the current status of this P. ferruginea population, ii) track the any change in the population in the last 10 years, iii) to confirm the result of the ¨population viability analysis¨ (PVA) prediction method performed by Espinosa et al. (2018) of the P. ferruginea population in Ceuta and iv) explore the environmental factors affecting the populations of P. ferruginea.
2 Materials and methods
2.1 Study area
The Strait of Gibraltar is a very important geological region, bordering by the Mediterranean, Lusitanian and Mauritanian biogeographic provinces, so it is a zone in which marine flora and fauna from the Mediterranean and Atlantic overlap (Coll et al., 2010). Likewise, it is one of the world’s busiest hubs for maritime traffic, as it is a place where many commercial routes converge and where important harbors are present (Algeciras, Gibraltar and Tangier Med), which makes it an area with high risk of disturbances, impacts and environmental disasters (Piniella and Walliser, 2013: Nachite et al., 2020).
The harbor of Ceuta, a Spanish city in North Africa, has an unusual structure, because it is located between two bays (North and South) connected to both by the Royal Moat (Figure 1A). This defensive construction, built by the Portuguese during the 16th century, increases water movement and exchange within the structure (shorting the residence time of the water mass), when compared to other conventional harbors, maintaining moderate oxygen levels in the water and sediment (Guerra-García et al., 2004a; Guerra-Garcia and García-Gómez, 2005; Sánchez-Badorrey et al., 2021).
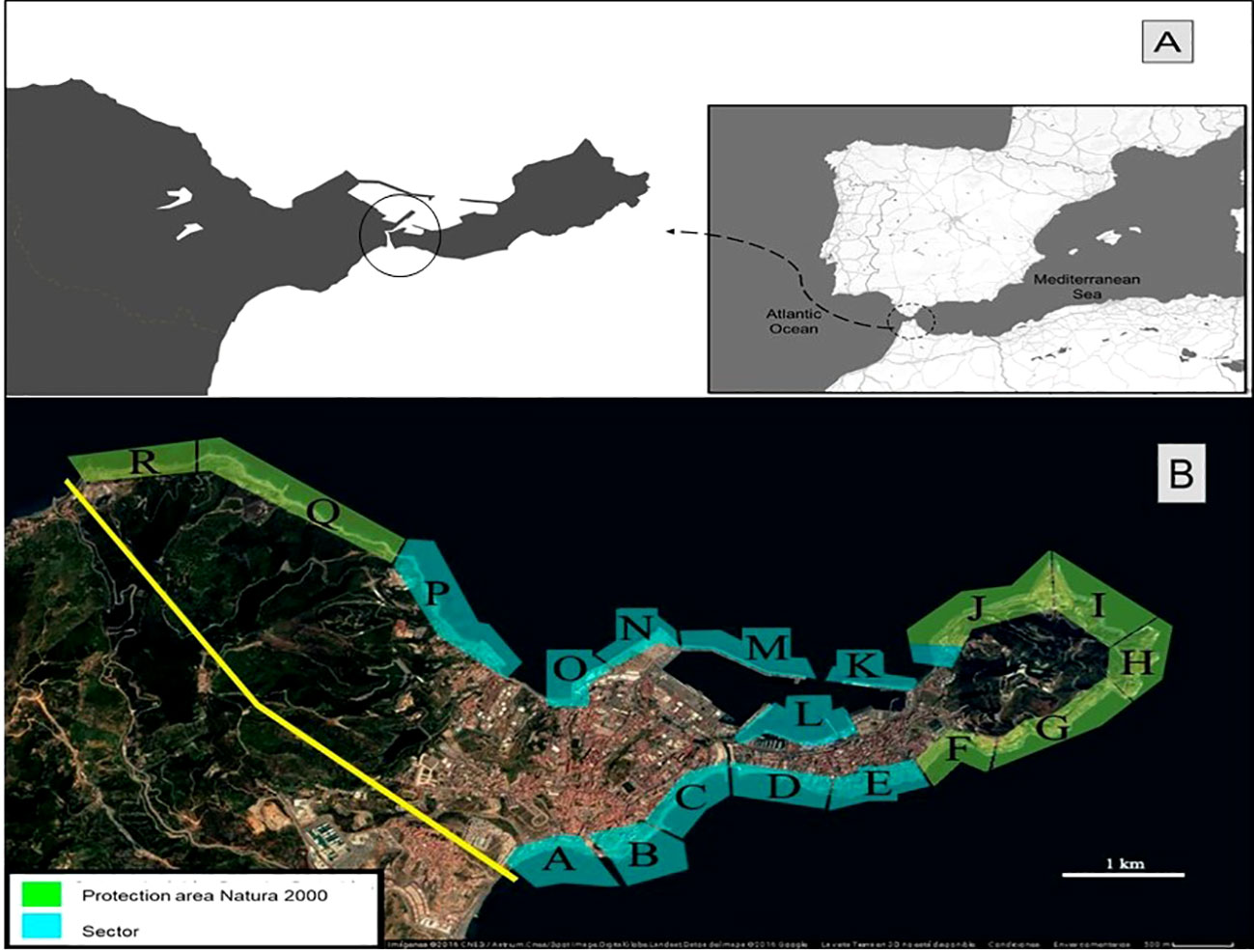
Figure 1 (A) Location of Ceuta, inside the black circle can be found the royal Moat; (B) Sectorization of the coastline to estimate the population size distribution of P. ferruginea. Source: Google Earth.
2.2 Sampling methods
2.2.1 Patella ferruginea census
A census on P. ferruginea population in Ceuta was carried out during 2019 and 2020 (14 months). To estimate the status and evolution of the P. ferruginea population, the sampling methodology repeated the one carried out by Rivera-Ingraham et al. (2011b) in 2010 in the same area. The complete coastline was divided into 18 sectors; accounting for the type of substratum (natural, dolomitic rip-rap and concrete cubes) and its geographic orientation (see sectors at Figure 1B).
To know the ¨population size structure¨ of P. ferruginea’s population, the 20% of the entire rocky midlittoral, where the species occurs, was sampled at each sector. Ten meter linear transects were proportionally distributed within each sector separated by 40 m. The shell length of the specimens found within the intertidal at each transect were measured to the nearest millimetre using a calliper (Guerra-García et al., 2004b; Espinosa, 2009b; Rivera-Ingraham et al., 2011b). Finally, 4,560 m of rocky midlittoral coastline were sampled out of a total of 23,617 m of rocky coastline present in the city of Ceuta (Table 1).
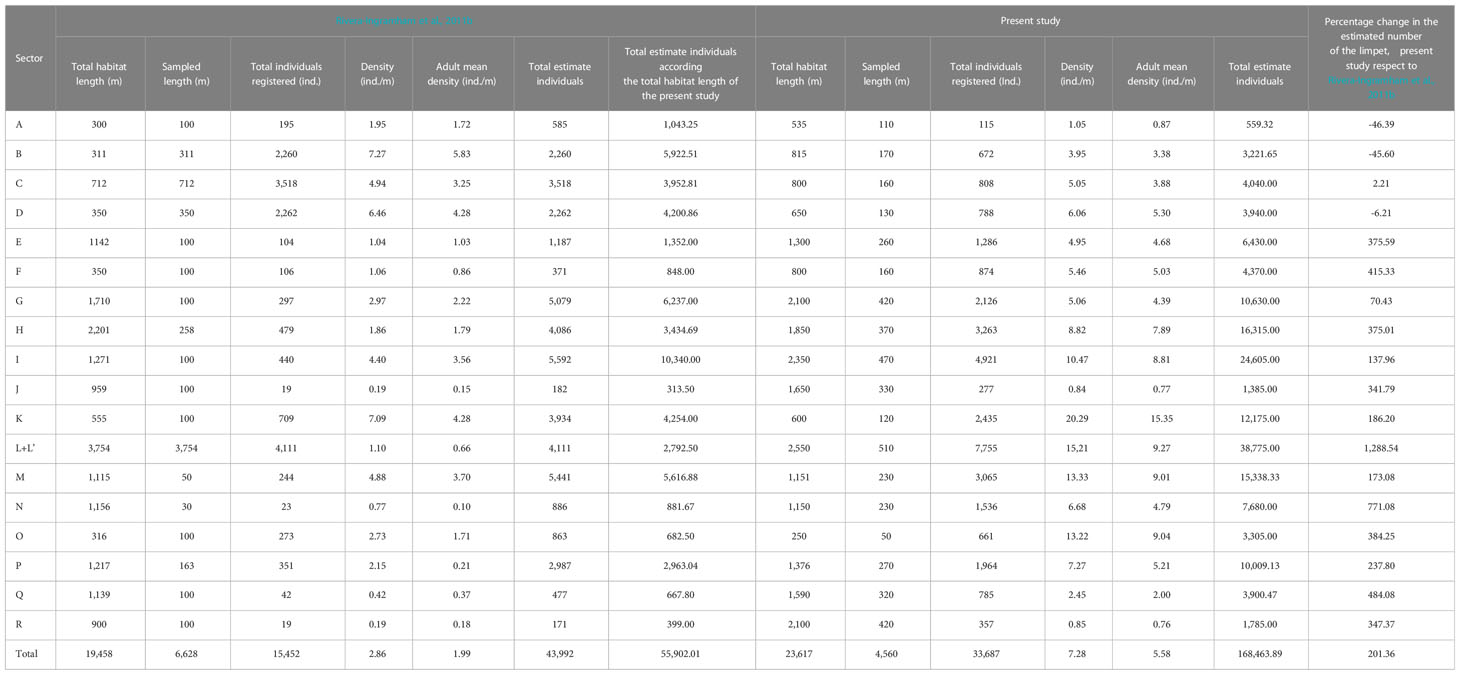
Table 1 Comparison of the mean density, adult mean density and the total estimate individuals between both studies about P. ferruginea population of Ceuta (change from 2010 to 2020).
To estimate the total number of individuals in each sector and thus of the population, the results were extrapolated from the total length (m-1) of P. ferruginea habitat in the sector. In the present study, recruits were defined as individuals smaller than 30 mm and adults greater than or equal to 30 mm (Espinosa et al., 2006) and the “class size” was categorize for every 10 mm of shell length (e.g., class size 3 pertains to individuals from 21mm to 30mm).
To track any change of the population structure in the last 10 years, it has been considered that in the preceding work performed by Rivera-Ingraham et al. (2011b). In the said study, the total length of rocky shoreline where the species could potentially be present was calculated by using 1:9,000 scale maps obtained using Google Earth©. Therefore, the estimations from the previous census have been recalculated, according to the used estimate of coastline length in the present work (10 m resolution), in order to make both estimations comparable and to be able to evaluate the percent increase or decrease of the population in each sector.
The percentage change in the number of individuals (%) between both studies was calculated using the equation:
2.2.2 Environmental factors
Once the sampling of P. ferruginea was completed, the environmental factors were studied. All environmental factors were studied in the same transect that were randomly selected in each sector (three transects per sector, 54 transects in total) in July of 2022.
Substratum lithology of each sector was characterized by collecting three rock chips from each sector, which were powdered in the laboratory using a ball mill with stainless steel balls as grinding media. Each rock chip was obtained by hammer and chisel. The elemental composition and calcination percentage of each sample was quantified by X- ray fluorescence (XRF) using an AXIOS spectrometer. Mineralogic absorption spectra were detected by X-ray diffraction (XRD) using a powder diffractometer (Bruker D8 Advance) equipped with a high temperature chamber (Anton Paar XRK 900) and a fast response/high sensitivity detector (Bruker Vantec 1) with radial Soller slits (full methodology can be accessed in Valverde et al., 2015). Afterwards, XRF and XRD data were interpreted to quantify the crystallinity and lithology composition of each sample using DRIFAC.EVA.4.1 software.
To assess the influence of microalgal abundance on P. ferruginea demographic, the chlorophyll-a concentration on the substratum surface was estimated in the same rock samples used to study lithology. The resulting slurry was treated with 20 ml of 100% ethanol to extract the pigments. Then, samples were frozen and maintained in darkness until analysis (minimum of 48 h) according with the protocol used by Kido and Murray (2003). Samples were filtered through a Whatman GF/C filter and measurements of absorbance were carried out with a spectrophotometer (Pharmacia Biotech Novaspec II).
Given the large extension of the study area, some environmental factors like chlorophyll a were collected once, being a snapshot of the environment. Moreover, for the Chl-a we took the 54 samples in two consecutive days of July 2020 using a zodiac and sampling in the same hour of the day. The chlorophyll-a concentration was calculated according to the formula of Thompson et al. (1999):
Where, 12.2 = constant for ethanol, A6655 = net absorbance of solution at 665 nm, v = final volume of solution (20 ml), d = path length of cell (1.6 cm), V = surface area of sample (100cm2). Chlorophyll-a concentration is expressed in µg cm-2.
The wave exposure was quantified for each sector based on a fetch model index developed by Howes et al. (1994) (see Terrón-Sigler et al., 2018). Fetch models have been successfully used to predict marine community patterns (e.g. Ros et al., 2016) by providing quantitative estimates of wave exposure using a combination of two indices: maximum fetch (Fi) and modified effective fetch (Fe). Maximum fetch is defined as the maximum fetch distance in km measured from the point of interest. When a vector does not find and obstacle (i.e. open ocean), a value of 1,000 km is conventionally used. Effective fetch (Fe) is calculated from the equation:
Where Өi is the angle between the shore-normal and the directions 0°, 45° to the left ad 45° to the right, and Fi is the fetch distance in km along the relevant vector. Combining the values obtained for each index, the wave exposure class of each sector was determined based on the classification proposed by Howes et al. (1994). The different Fi values (-45°, 0° and 45°) were calculated for each transect studied using Google Earth©.
The heterogeneity of the coast (macroscale roughness) was calculated by lying a 10 m flexible measuring tape along the upper mid-littoral, which was allocated following as closely as possible the contours of the bare substratum along the transect. Using the same methodology, three replicates were recorded on each transect randomly selected along the height of the rocky midlittoral (habitat of P. ferruginea). This information was used to obtain was used to obtain the area of P. ferruginea habitat in each sector: profile of longitudinal x profile of height (m).
Regarding microscale roughness, three 25 cm profile gauges per transect with 0.5 mm pins were pushed onto the bare rock within the same transects already selected for macroscale roughness to record substrata surface heterogeneity (Frost et al., 2005). The resulting profiles were photographed, and the images were digitally processed with Adobe© Photoshop to obtain two-coloured images. The length of the contour of the profile was obtained with ImageJ software. In both cases (macro- and microscale), substratum roughness was calculated as in Rivera-Ingraham et al., 2011b; Rivera-Ingraham et al., 2011c and Sedano et al., 2020 using the equation by Blanchard and Bourget (1999): . Where Tr is the contour measured between two points and Ts the linear distance between those points.
The inclination of the substratum was taken recording three replicates within the already selected transects using a digital clinometer (see Rivera-Ingraham et al., 2011c).
The impact of human activity in the limpet’s habitat (intertidal zone) was sampled in July 2021, since it is the month of the year when more people are present within the littoral (personal observations from Ostalé-Valriberas). In the present study, the variable “impact of human activity” was restricted to the direct interaction of the citizen with the rocky intertidal. Each sector was visited twice by boat to quantify the different interactions. Sampling was conducted on two weekends in July due to the large number of citizens around the shoreline on these days. The first weekend sampled was from 11 a.m. to 2 p.m. in the morning and the second from 6 p.m. to 9 p.m., each sector was observed for half an hour each day. In each sampling session, all the sectors were visited. Each interaction was scored from 1 to 3 to categorise the different impacts on P. ferruginea habitat. The difference interactions registered were angling (x3), shell fishing (x3), bathing in the intertidal (x2), bathing near the intertidal (x1), recreational boat nearby (x1) and irregular camp (x1) (the concept ¨irregular immigrant camp¨ derives from the exceptional migratory problem of 17th May of 2021 in which the local administration assistance was overwhelmed, see investigation of Casey and Bautista (2021). To estimate the used index for the GLM, the total of each interaction was multiplied by the impact value on the habitat (e.g. 9 people bathing in the intertidal (x2) gives a value of 18). In the methodology for quantifying human activity, value 1 is “low impact” and 3 is “high impact”.
A constrained ordination approach to assess how well the biological data relate to different abiotic variables that characterize the different sectors was carried out. The parameters considered were heterogeneity of the coast (macroscale roughness), microscale roughness, area of P. ferruginea habitat, concentration of chlorophyll-a, inclination of the substratum, wave exposure (fetch) of the littoral, impact of human activity and rock elemental composition.
2.3 Statistical analyses
2.3.1 Patella ferruginea census
Among the sectors, a PERMANOVA analysis was carried out between the different size classes to differentiate the population size structure of P. ferruginea distribution. Before the analyses, a square root transformation along with the Bray Curtis similarity index was applied to different size classes. Differences among sectors were established using nNMDS (non-metric multidimensional scaling) and cluster analysis.
The change of the P. ferruginea population in the last 10 years was determined by comparing the adult density between the present study and preceding work performed by Rivera-Ingraham et al. (2011b). To obtain this result, Euclidean distance matrices were calculated and tested using univariate PERMANOVA.
PERMANOVA analyses were performed using the PRIMER v.6+PERMANOVA package (Clarke and Gorley, 2005; Anderson et al., 2008).
2.3.2 Environmental parameters
The effect in the P. ferruginea population’s structure (adult density and adult mean size) of different environmental parameters were modelled using generalized linear models (GLMs; McCullagh and Nelder, 1989). Models were carried out using normal distributions and identity link functions to determine which of the parameters gave the best fit to the data (see Cayuela, 2010). The environmental parameters added to the model were the percentage of calcium oxide in the substratum (CaO %), chlorophyll-a concentration, wave exposure (fetch), roughness of substratum (macroscale and microscale), area of P. ferruginea habitat, inclination of the littoral and anthropogenic impact. Prior to the analysis, environmental variables were tested for multicollinearity, assuming collinearity when variance inflation factor (VIF) values were much greater than 1. Model selection was carried out based on Akaike’s information criterion for small sample sizes (AICc) (Burnham and Anderson, 2002). Variability explained by the best models was computed using deviance values (D2), comparing residual deviance with the deviance of a null model (null deviance). Among all significant models, the minimal Akaike value indicated the best model that explained the greatest proportion of variance under the restriction that all the predictors in the model must be significant (see Cayuela, 2010; Ros et al., 2015).
The density of recruits (individuals< 30 mm) was not considered in the GLM because their presence is highly fluctuating throughout the year (recruits of this species settle on the intertidal rock between December and March) (see Espinosa, 2006), and it was impossible to sample all sectors at the same time in those months.
Generalized Lineal Models were carried out using SPSS 25.0© (IBM, New York, NY, USA) (Pallant, 2020).
3 Results
3.1 Present status of Patella ferruginea in Ceuta
In the 20% of the P. ferruginea habitat (4,560 m), it was registered 33,687 individuals so that it was estimate that the population of Ceuta have 168,464 individuals distributed in a total of 23,617 m of rocky coastline (Table 1). From the whole population, it was estimated that 131,775.87 were adult individuals (≥ 30 mm). PERMANOVA analysis showed that there were significant differences between the population structure (different size classes) of the different sectors (Table 2).
Individuals of P. ferruginea were present in all sampled sectors (Figure 2), with the greater mean density of individuals and adults occurring in the dolomitic rip-rap inside the harbour (sector L), with a mean density of 32.2 ind./m and adult mean density of 19.57 ind/m (Figure 3). The greatest values per transect were achieved in this sector (54.84 ind./m and 30.84 ind./m of adults), although the concrete seawalls in the same area (subsector L’) had one of the lowest values. The natural habitat with greater P. ferruginea densities was Acantilados de la Sirena (sector H) with 8.82 ind./m and 7.89 ind./m of adults.
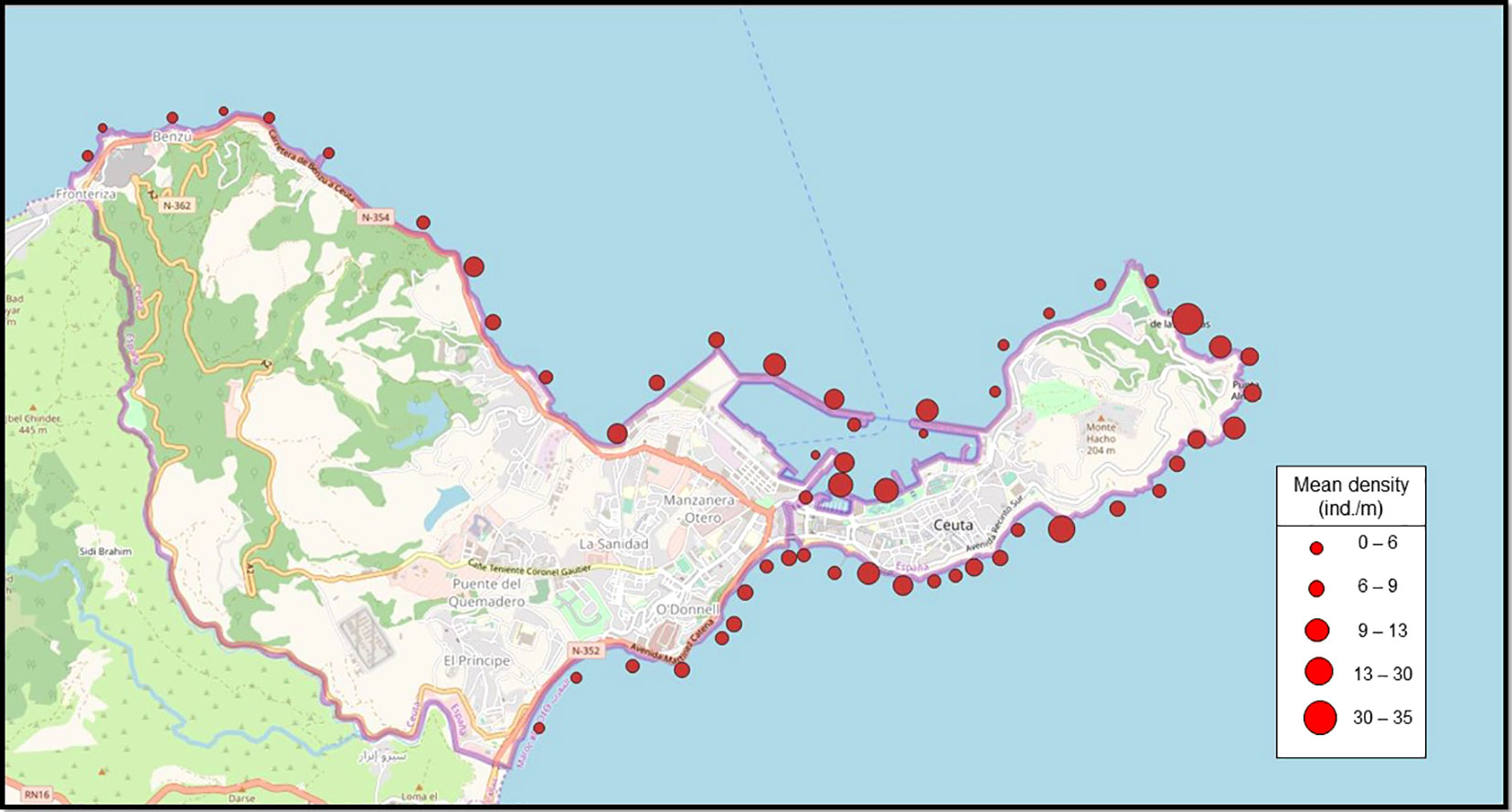
Figure 2 General distribution of P. ferruginea in Ceuta. Circle diameter corresponds to the density of individuals. Google Earth.
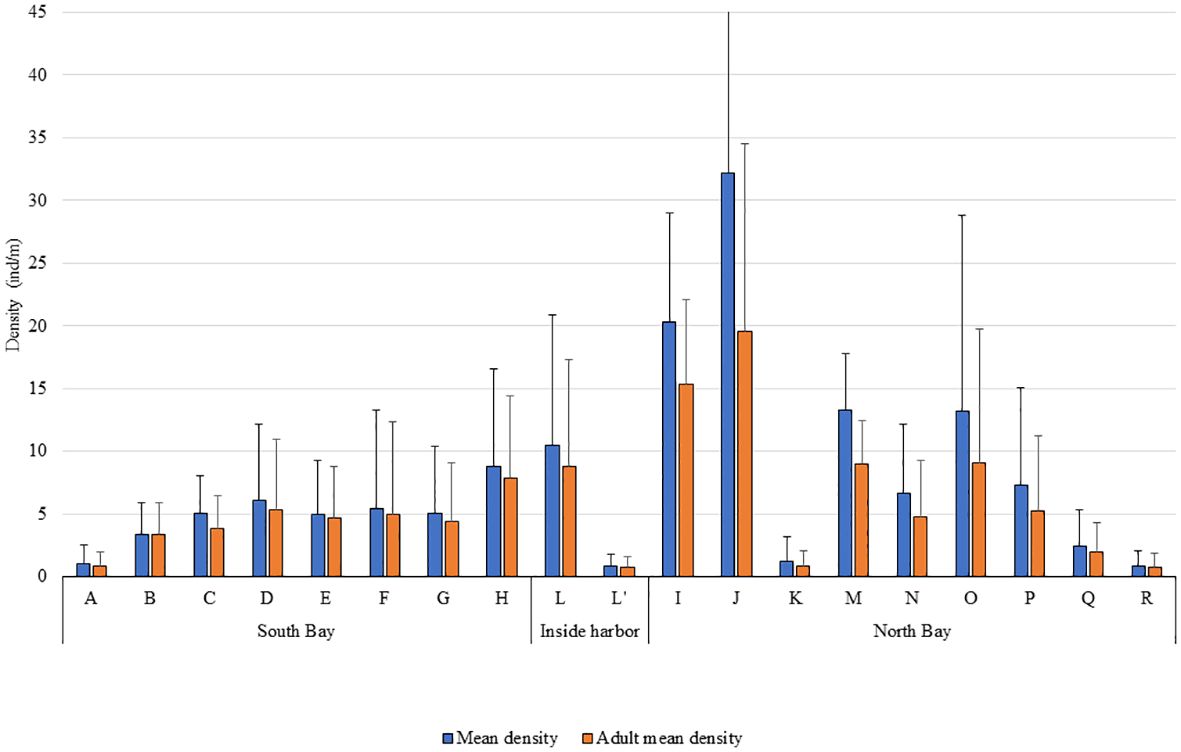
Figure 3 Mean density and adult mean density of P. ferruginea for each of the sectors and standard deviation.
Greater average shell length was registered in sector E, in which mean size was 584 mm and the adult mean size 603 mm (Figure 4). The biggest individual measured had a maximum shell length of 113 mm (Supplementary Figure 1-S). The average shell size of Ceuta’s population was 447 mm and the mean adult size 508 mm.
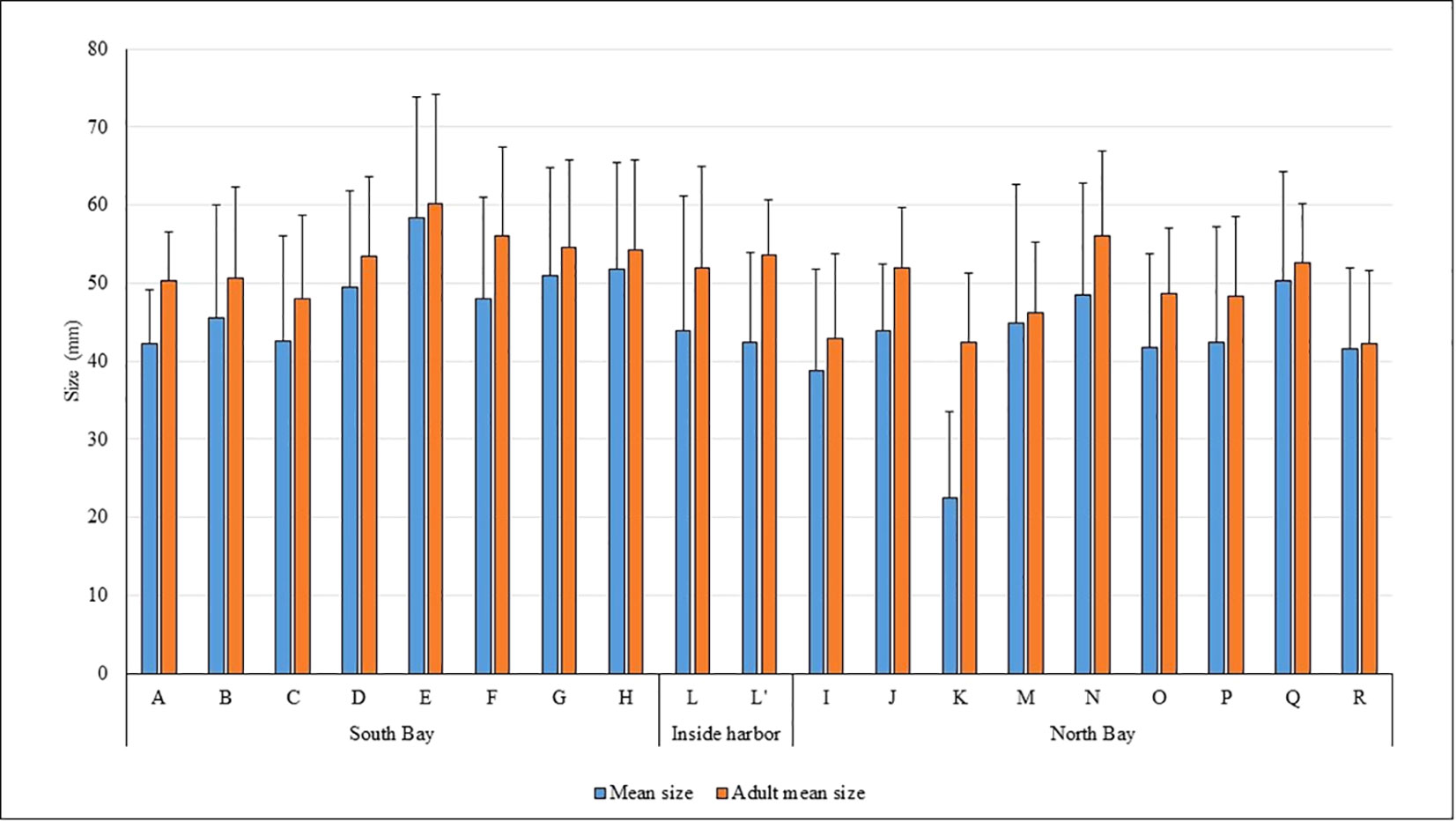
Figure 4 Mean size and adult mean size of P. ferruginea for each of the sectors and standard deviation.
Multivariate analyses revealed differences in the structure of P. ferruginea population (abundances within size classes) among sectors (Figures 5–7). The combination of NMDS and Cluster analyses showed the segregation of sector L from other sectors. The subpopulations most similar to sector L were K, M and O, all these sectors were composed of rip-raps made with quarry rocks (Supplementary Table 4-S). On the contrary, coinciding with the less dense sectors, were the sectors R, J, A and L’ (Table 1; Figures 2, 3).
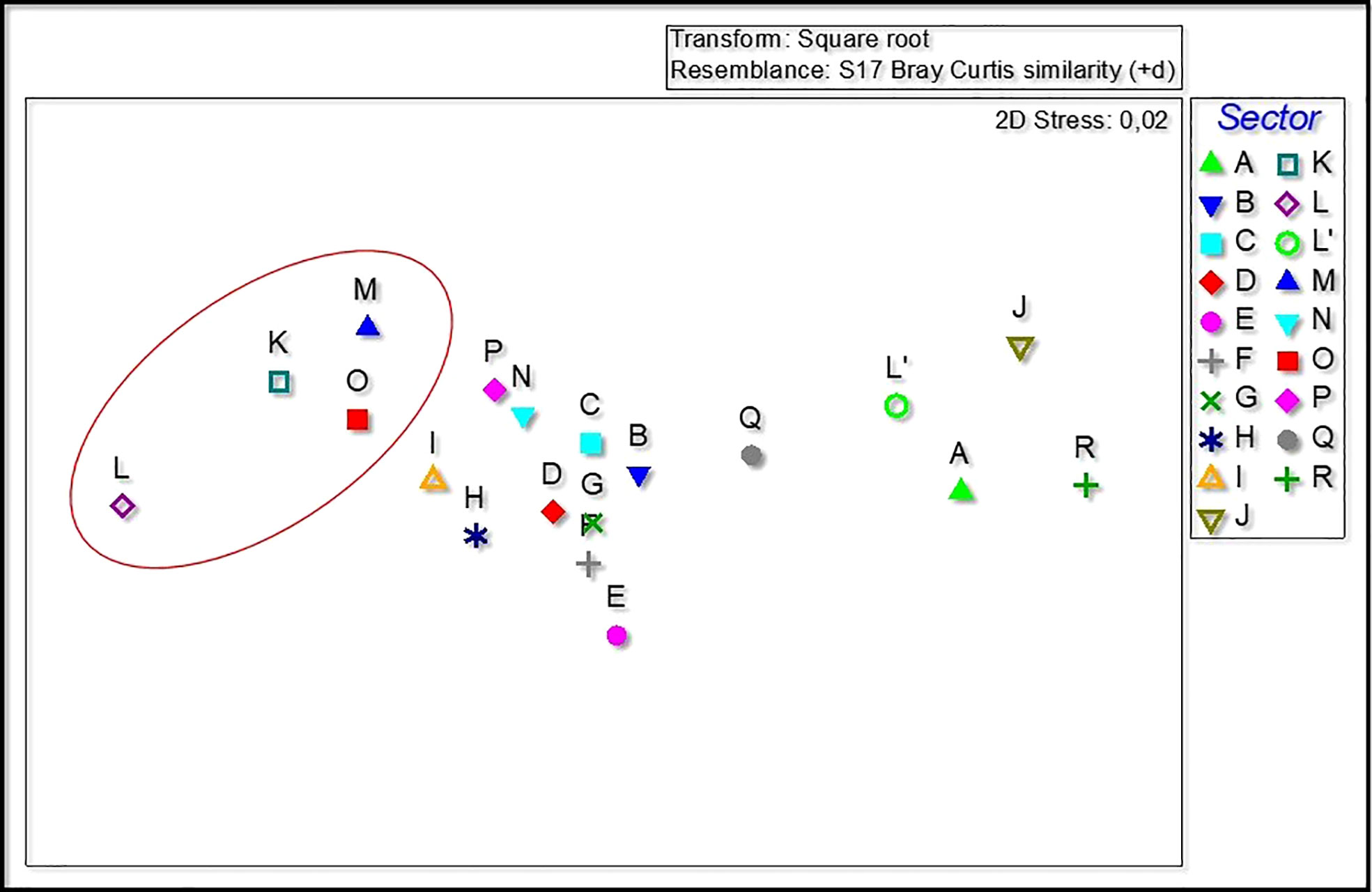
Figure 5 nMDS computed using the abundances of each size class and showing PCO centroids for each of the sectors. Red circle: sectors with dolomitic rip-rap of the commercial harbor where the access is restricted with a great heterogeneity substratum (macro and micro scale).
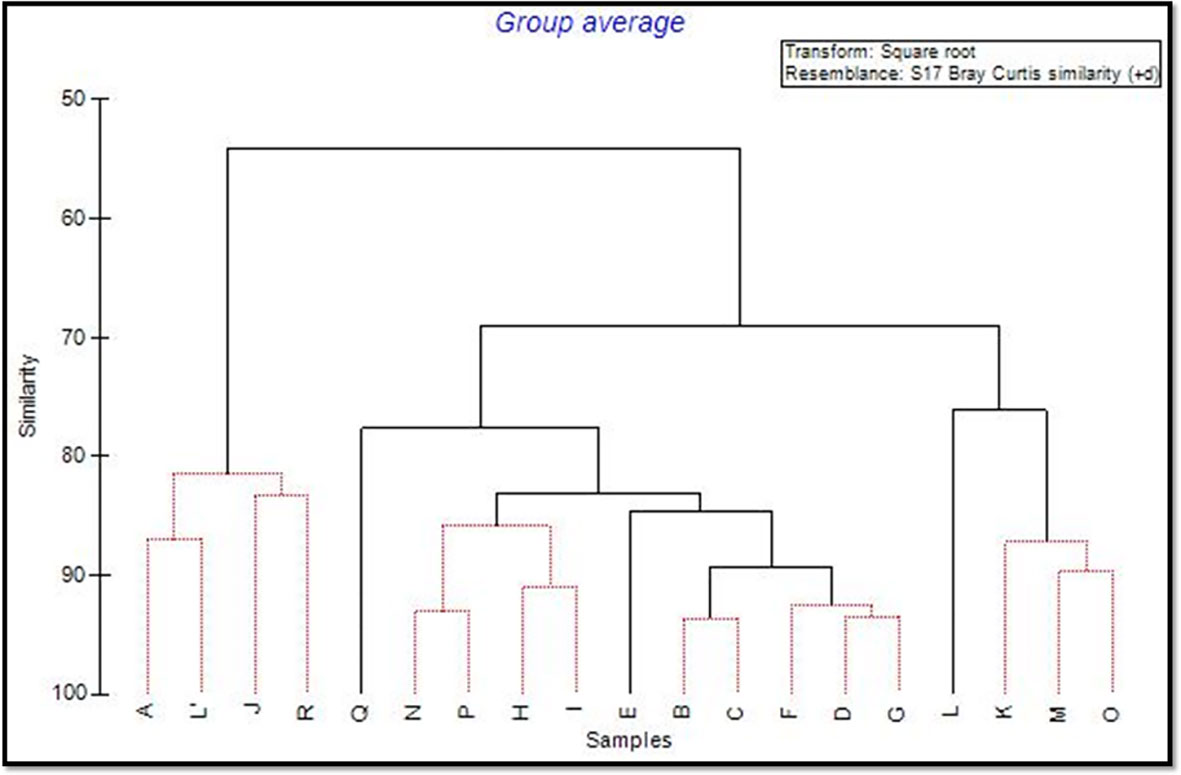
Figure 6 Cluster analysis computed using the mean abundances of the different size classes of P. ferruginea for each of the sectors considered. Discontinuous lines indicate significantly different groups of sectors segregated by SIMPROF analysis.
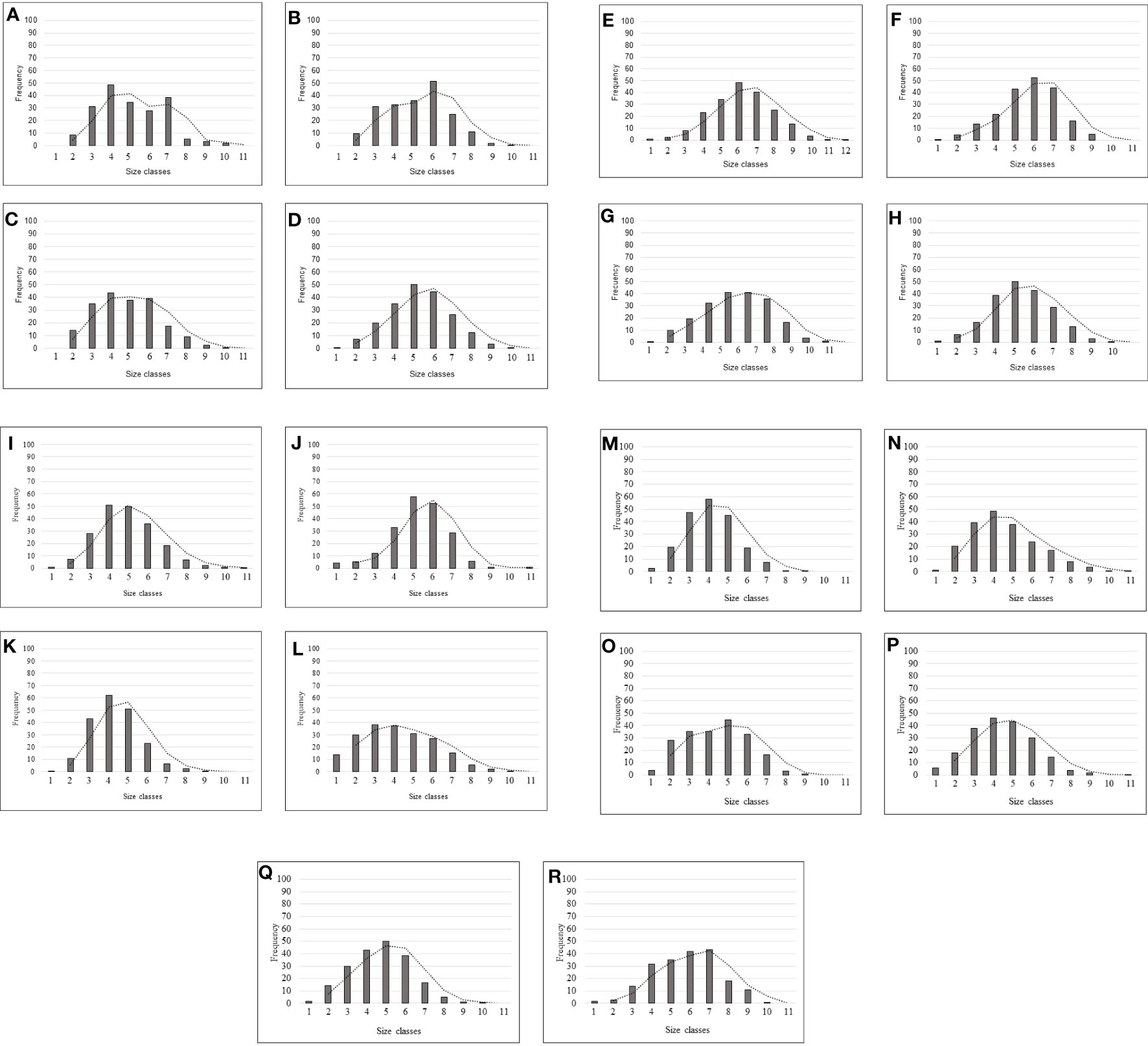
Figure 7 Size class frequencies of P. ferruginea for each of the sectors considered to know the population structure (trend line). Letters indicate the sampled sectors (see Figure 1B).
3.2 Change in the population structure of the Patella ferruginea population in the last 10 years
Recalculating the total estimated individuals from the study of Rivera-Ingraham et al. (2011b) according to the total habitat length of the present study, the population has increased from 55,902 to 168,463 individuals. This means that the population has increased by an average of 201.36% compared to the existing population 10 years ago (Table 1).
The subpopulation with the higher increase in these years was the one inhabiting dolomitic rip-rap inside the harbor (sector L), with an increase of 1,288%. On the opposite side, the subpopulations with higher decrease were those on sectors A and B with a -46.39% and -45.60% respectively (Table 1).
Univariate PERMANOVA showed that the adult mean density (ind./m) of the present study was significantly higher than the Rivera-Ingraham et al. (2011b) study (Pseudo-F = 14.68; p< 0.001).
3.3 Effects of environmental parameters on population structure
According to an omnibus test, only the variable adult density was significant. The VIF values show that the variables used to evaluate their influence on adult density were not correlated with each other.
The model demonstrated that the adult density is significantly influenced by heterogeneity (macroscale), microscale roughness, inclination of the littoral, chlorophyll-a concentration and anthropogenic impact. According to AICc values, the best model was the combination of these five parameters, reporting the 85.9% of the variability explained (Table 3). Adult density showed a positive correlation with the macroscale and microscale variables, while the variables inclination, chlorophyll-a concentration and anthropogenic impact showed a negative correlation.
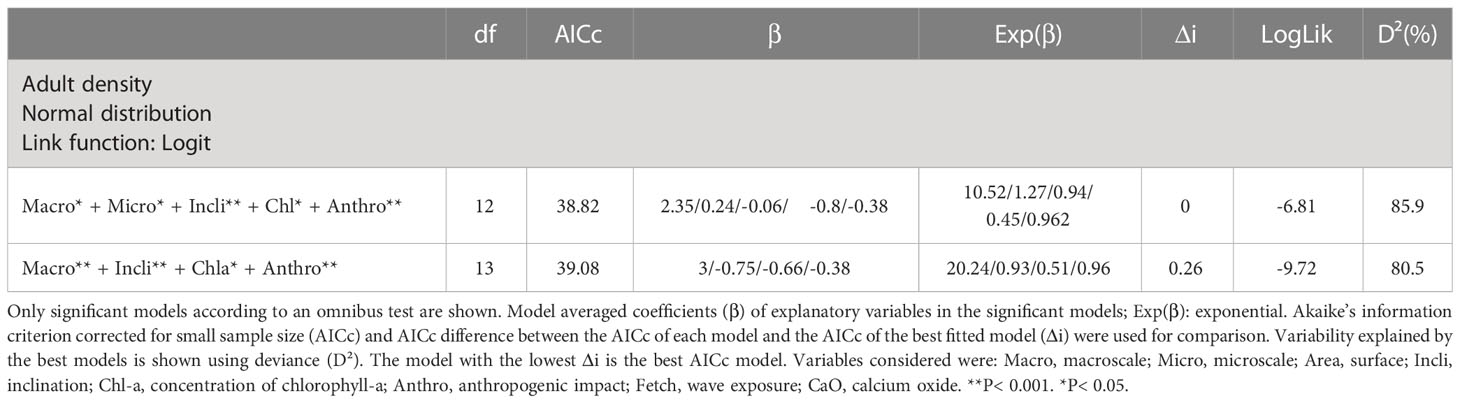
Table 3 Model selection results explaining the environmental parameters that have influence in the adult density and adult mean size.
Analyzing the results obtained for the microalgae (Chl-a concentration) in each sector, it is observed that the highest values (marked in green in Table 1-S) correspond to the natural substratum (sectors: R, B, D and G) and the lowest (marked in red in Table 1-S) correspond to the artificial substratum (sectors: N, O, K and L) (see Table 1 and Figure 2).
The adult density did not show any significant correlation with the variables area of habitat, wave exposure (fetch) and percentage of calcium oxide in the substratum (CaO %). The results of the environmental factors used in the GLM are shown as Supplementary Material (Table 1-S). The results of impact human activity (Supplementary Table 2-S) and rock elemental composition (elemental composition, Supplementary Table 3-S); mineralogy composition, Supplementary Table 4-S) were show like Supplementary Material.
4 Discussion
The results have shown that from 2011 to 2021 the population or P. ferruginea increased by about 200%. Currently, the population numbers 168,464 individuals, of which 131,776 are adults. In about a decade, the population has grown from 55,902 to 168,464 individuals. Recent studies have shown that there has been recolonization and repopulation of P. ferruginea in some localities of the Andalusia, Melilla, Alboran and Chafarinas island (Casado et al., 2017; Maestre et al., 2018; CMAOT, 2020). Therefore, Ceuta’s population could be an important contributor to the recolonization of these areas, especially when considering that nearly 80% of its individuals are adults. Nevertheless, the situation of P. ferruginea is still critical because the individuals settled in Andalucía are the last stronghold of continental Europe, depending heavily on recruits from North African populations (CMAOT, 2020). These results show that the area of Ceuta is a ¨stronghold¨ for this endangered species and support the hypothesis that this population has the role of a ¨source population¨ of new recruits for other nearby ¨sink populations¨ in the metapopulation of the Alboran Sea supporting the hypothesis proposed by Espinosa et al., 2018.
These conclusions can be supported by different oceanographic and biological studies carried out in this biogeographic area. In this sense, it is known that all P. ferruginea populations from the Alboran Sea and North Africa up to Tunisia belong to the same genetic cluster (Casu et al., 2011). Dispersal studies showed that the directionality of the surface currents of the Alboran Sea, especially those near the Strait of Gibraltar in its first loop go from south to north (therefore, the potential larval input should go from south to north and not the other way around, at least from the coast of Ceuta) (see Periáñez, 2007). Likewise, dispersal studies of P. ferruginea larvae in the Alboran Sea show simulated regional trajectories for virtual larvae released from Ceuta with strong prevailing westerly wind at high and low spring tide conditions with a Pelagic Larval Duration of 14 days during January 2016 can cross and complete the first loop of the Alboran Sea (Stephen Warr unpublished data). The settlement ability of larvae of this species is reached 3-4 days after fertilization, depending on water temperature, and crawling and swimming pediveliger larvae have a phase of 7-32 days (probably up to 40 days) until metamorphosis to juvenile occurs (Ferranti et al., 2022), which would argue that the population studied in Ceuta should be classified as a “source population” according to the “source-sink population model” (see Pulliam, 1988; Pulliam, 1996).
Although the sampling effort was larger than previous studies, in light of the results a review of the existing literature, reveals that the population in Ceuta is the largest within the species’ natural distribution (see Table 4).
The mean density of the population has increased from 2.33 ind/m in 2010 (Rivera-Ingraham et al., 2011b) to 7.39 ind/m in 2020. This is in agreement with the output of the population viability analysis (PVA) model carried out by Espinosa et al. (2018), and based on monitoring during a ten-year period. This model indicates the unlikeliness of the population in Ceuta facing extinction within the next 50 years. This confirms the effectiveness of the control and temporary monitoring programs of the population proposed in Spain’s National Conservation Strategy for P. ferruginea, established in 2008 by the Spanish Ministry of Environment (MMAMRM, 2008).
The increase of Ceuta’s population could be due to two different factors. Firstly, there are some littoral zones with difficult or restricted access, such as sectors H and I, which are a natural cliffs, and sectors K, L and M, which are access-restricted areas in the commercial harbor of artificial structure type riprap (great heterogeneity, macroscale and microscale). These sectors would be therefore protected from anthropogenic impact, which has been identified as the main factor promoting the decline of the species (Ostalé-Valriberas et al., 2022 and references therein). Secondly, there has been an improvement in social awareness of the conservation status of this endangered species, promoted by dissemination activities performed by NGOs and the environmental administration, and increased enforcement by local authorities. In this sense, many projects and news have been published in social media publications (Mar, 2020), giving notoriety to P. ferruginea and turning it into a flagship species. In this sense, flagship species attract funding, increase awareness, and promote the conservation of habitats, acting as umbrella species (Smith and Sutton, 2008; Home et al., 2009).
An analysis of the evolution of the population of P. ferruginea over the last two decades in a biggest area distribution, the Alboran Sea, shows an increase in the number of individuals (Maestre et al., 2018; CMAOT, 2022). The control by the local authorities (environmental administration and security forces) in the application of the strict legislation that protects the species (community, national and regional regulations), in addition to the increase in the awareness of the local population through environmental education, together with the advances in research on the species give an impression of good results.
Statistical modeling has shown that P. ferruginea adult density increases with coastal heterogeneity (macroscale in meters) and substratum roughness (microscale in cm), and decreases with slope, chlorophyll-a concentration and anthropogenic impact. Among the different environmental factors, the coastal heterogeneity (m) has the most impact on the adult density. A one-point increase in the macroscale value favors adult density by 10.52 while a one-point increase in the microscale value (cm) favors it only by 1.27. Therefore, coastal and substratum heterogeneity at multiple scales must be taken into consideration for designing sites for further protection (Marine protected areas or Area-based conservation measures such as Artificial marine micro-reserves), to establish critical areas for its conservation or for future translocation procedures for reinforcement and/or reintroduction, or due to destruction of the original habitat (see MMAMRM, 2008; García-Gómez et al., 2011; García-Gómez et al., 2015; IUCN-WCPA, 2019; Agung et al., 2022; Ostalé-Valriberas et al., 2022).
The vertical slope of the coastline negatively influences the density of P. ferruginea adults. Within vertical substrata such as cliffs or seawalls, a smaller surface (area) of settlement for planktonic larvae is available, which explains the result.
A practical example of these results has been shown in the different abundances between the subpopulation settled inside the harbor (sectors L and L’). Yet still sharing the same environment, the subsector of dolomitic rip-rap (L) and the vertical concrete wall (L’) show the most different results according to the population structure (number of individuals in each size class) (see Figures 5, 6).
Analyzing the results obtained for the Chl-a concentration in each sector, it is observed that there are other environmental factors that more strongly affect the species.
According to the results of previous studies, the accessibility and visitation of the coastal areas negatively affect limpet populations of P. ferruginea, biasing the populations structure via harvesting of the greatest sizes (mostly females) and therefore affecting the adult density (see Figure 7; Table 2-S) (Espinosa et al., 2009; Ostalé-Valriberas et al., 2022). In fact, the results of the present study show that a one-point decrease in the value of accessibility increases the adult density by 0.96.
5 Conservational implications
The results indicate that Ceuta is the main population of this endangered species in its entire range, being a source population on the Southern Iberian Peninsula that should be conserved as a priority. According to the Spanish National Conservation Strategy, sectors H, I, K, L and M have the highest number of adults and should therefore be categorized and managed as Critical Areas (MMAMRM, 2008; see Supplementary Figure 2-S). It could benefit many species of conservation concern also settled in the same habitat (“umbrella effect”), as demonstrated by Ostalé-Valriberas et al. (2022).
Previous studies have shown that 90% of the populations of a metapopulation are usually “sink populations” and only 10% are “source populations” (Pulliam, 1988), so this population is of great ecological relevance for this species.
The results also support the concept of “Artificial Marine Micro-Reserves” as a new area-based management tool for marine conservation, establishing a network of these source populations that promote gene flow between populations with eventual recolonization of their original distribution (García-Gómez et al., 2011; Ostalé-Valriberas et al., 2022) (Supplementary Figure 2-S).
Finally, for future translocation procedures, the reported results could serve as a guide to select the most suitable receptor sites. Such areas should have high values of macro- or microscale heterogeneity and, similarly, should avoid vertical substrata and areas with anthropogenic impact.
Data availability statement
The original contributions presented in the study are included in the article/Supplementary Material. Further inquiries can be directed to the corresponding author.
Author contributions
EO-V, JS-V, FE, G-GJ conceptualization of the work and development the methodology. EO-V, FE application of statistical, mathematical, computational, or other formal techniques to analyze or synthesize study data. EO-V, AL, AA-A, JS-V, AP-P: Conducting a research and investigation process, specifically performing the experiments, or data/evidence collection. EO-V writhing the original draft, visualization work and supervision. AP-P, JS-V, FE, G-GJ writing -review & editing. EO-V, AL Management and coordination responsibility for the research activity planning and execution. EO-V, G-GJ acquisition of the financial support for the project leading to this publication. All authors contributed to the article and approved the submitted version.
Funding
The present study was financed with the Cooperation Agreement between two public administrations, the Sociedad, Obras, Infraestructuras y Medio Ambiente de Ceuta S.A.U. (OBIMASA) and the Fundación de Investigación de la Universidad de Sevilla (FIUS). We are grateful to Ana Garciá and Francisco Javier Martínez-Medina for their work in the Cooperation Agreement. JS-V was supported by a FPI Grant (PRE2018-086266) from Ministerio de Ciencia, Innovación y Universidades (Project CGL 2017-82739-P) co-financed by ERDF European Union and Agencia Estatal de Investigación, Gobierno de España.
Acknowledgments
The authors would like to express their gratitude to our colleague Celeste Dominguez-Monge from the Laboratorio de Biología Marina of the University of Sevilla who helped us in the sampling process and in the mineral analysis. We are also particularly grateful for the assistance given by the diving center WaterGame Gran Azul, especially by Javier Ibañez and José Manuel Ávila. We also thank the editor of Frontiers in Marine Science, Professor Alan Hodgson, and the two reviewers for their constructive comments, which considerably improved the first version of the manuscript.
Conflict of interest
The authors declare that the research was conducted in the absence of any commercial or financial relationships that could be construed as a potential conflict of interest.
Publisher’s note
All claims expressed in this article are solely those of the authors and do not necessarily represent those of their affiliated organizations, or those of the publisher, the editors and the reviewers. Any product that may be evaluated in this article, or claim that may be made by its manufacturer, is not guaranteed or endorsed by the publisher.
Supplementary material
The Supplementary Material for this article can be found online at: https://www.frontiersin.org/articles/10.3389/fmars.2023.1127630/full#supplementary-material
References
Aguilera M. A., Tapia J., Gallardo C., Núñez P., Varas-Belemmi K. (2020). Loss of coastal ecosystem spatial connectivity and services by urbanization: natural-to-urban integration for bay management. J. Environ. Manage. 276, 111297. doi: 10.1016/j.jenvman.2020.111297
Agung M. F., Adhuri D. S., Ferse S. C., Sualia I., Andradi-Brown D. A., Campbell S. J., et al. (2022). Marine conservation beyond MPAs: towards the recognition of other effective area-based conservation measures (OECMs) in Indonesia. Mar. Policy 137, 104939. doi: 10.1016/j.marpol.2021.104939
Airoldi L., Abbiati M., Beck M. W., Hawkins S. J., Jonsson P. R., Martin D., et al. (2005). An ecological perspective on the deployment and design of low-crested and other hard coastal defence structures. Coast. Eng. 52, 1073–1087. doi: 10.1016/j.coastaleng.2005.09.007
Airoldi L., Beck M. W. (2007). Loss, status and trends for coastal marine habitats in Europe. Oceanography Mar. Biology: Annu. Rev. 45, 345–405.
Anderson M. J., Gorley R. N., Clarke R. K. (2008). PERMANOVA+ para PRIMER: guía para el programa y métodos estadísticos (Plymouth, UK: PRIMER-E, Massey University), 264.
Bazairi H., Benhissoune S., Ben Haj S., Benhoussa A., Ouerghi A., Idrissi H. R. (2012). “New and updated quantitative data on the Mediterranean endangered limpet patella ferruginea gmelin 1791 along the Moroccan coast,” in XVII Iberian symposium on marine biology studies, vol. 19. (Revista de Investigación Marina), 609.
Blanchard D., Bourget E. (1999). Scales of coastal heterogeneity: influence on intertidal community structure. Mar. Ecol. Prog. Ser. 179, 163–173. doi: 10.3354/meps179163
Bulleri F., Chapman M. G. (2010). The introduction of coastal infrastructure as a driver of change in marine environments. J. Appl. Ecol. 47 (1), 26–35. doi: 10.1111/j.1365-2664.2009.01751.x
Burnham K. P., Anderson D. R. (2002). Model selection and multimodel inference. A practical information‐theoretic approach (2nd ed.). (New York, NY: Springer).
Casado M. F., del Carmen Arroyo M., Navarro M. S. V. (2017). Caracterización de las poblaciones de la lapa ferruginosa (Patella ferruginea gmelin 1971) de la costa norte del estrecho de Gibraltar. Almoraima: Rev. estudios campogibraltareños 47), 181–202.
Casey N., Bautista J. (2021) A wave of the hand sets off Spain-Morocco migrant fight. the new York times. Available at: https://www.google.com/amp/s/www.nytimes.com/2021/06/02/world/europe/spain-ceuta-migrants-morocco.amp.html.
Casu M., Rivera-Ingraham G. A., Cossu P., Lai T., Sanna D., Dedola G. L., et al. (2011). Patterns of spatial genetic structuring in the endangered limpet Patella ferruginea: implications for the conservation of a Mediterranean endemic. Genetica 139, 1293–1308. doi: 10.1007/s10709-012-9631-3
Cayuela L. (2010) Modelos lineales generalizados (GLM). Available at: https://es.scribd.com/document/353511291/3-Modelos-lineales-generalizados-CAYUELA-pdf/.
Chase J. M., Blowes S. A., Knight T. M., Gerstner K., May F. (2020). Ecosystem decay exacerbates biodiversity loss with habitat loss. Nature 584 (7820), 238–243. doi: 10.1038/s41586-020-2531-2
Clarke K. R., Gorley R. N. (2005). PRIMER: plymouth routines in multivariate ecological research (Plymouth: PRIMER-E Ltd.).
CMAOT (2019). Programa de gestión sostenible del medio Marino andaluz. informe final de resultados 2018 (Sevilla, SPAIN: Consejería de Medio Ambiente y Ordenación del Territorio (CMAOT).
CMAOT (2020). Programa de gestión sostenible del medio Marino andaluz. informe final de resultados 2019 (Sevilla, SPAIN: Consejería de Medio Ambiente y Ordenación del Territorio (CMAOT).
CMAOT (2022). Programa de gestión sostenible del medio Marino andaluz. informe final de resultados 2021 (Sevilla, SPAIN: Consejería de Medio Ambiente y Ordenación del Territorio (CMAOT).
Coll M., Piroddi C., Steenbeek J., Kaschner K., Ben Rais Lasram F., Aguzzi J., et al. (2010). The biodiversity of the Mediterranean Sea: estimates, patterns, and threats. PloS One 5 (8), e11842. doi: 10.1371/journal.pone.0011842
Coppa S., De Lucia G. A., Massaro G., Magni P. (2012). “Density and distribution of patella ferruginea in a marine protected area,” in Constraint analysis for population conservation, vol. 13. (Western Sardinia, Italy: Mediterranean Marine Science), 108–117.
Cossu A., Deluca M., Guelfi C. (2006). Distribuzione spaziale dei popolamenti a lithophyllum byssoides, a patella ferruginea e della frangia a cystoseira sp. nell’arcipelago di la maddalena Vol. 13 (Sardegna-Italia: Biologia Marina Mediterra nea), 84–85.
Cottalorda J. M., Meinesz A., Thibaut T., Chiaverini D. (2004). Représentation cartographique de l’abondance de quelques algues et invertébrés sur le littoral des îlots du rascass et de la gabinière (Parc national de port-cros, var; France). Sci. Rep. Port-Cros Natl. Park 20, 195–209.
Creel L. (2003). Ripple effects: population and coastal regions (Washington, DC: Population reference bureau), 1–7.
Cristo B., Caronni S., Floris A. (2007). Osservazioni su Patella ferruginea gmelin 1791 (Mollusca, Gastropoda) nel golfo di olbia. Biol. Marina Mediterr. 14 (2), 344–345.
Espinosa F. (2006). Caracterización biológica del molusco protegido Patella ferruginea Gmelin, 1791 (Gastropoda: Patellidae): Bases para su gestión conservación (PhD thesis). University of Sevilla, Spain, pp55.
Espinosa F. (2009b). Populational status of the endangered mollusc Patella ferruginea gmelin 1791 (Gastropoda, patellidae) on Algerian islands (SW Mediterranean). Anim. Biodiversity Conserv. 32 (1), 19–28. doi: 10.32800/abc.2009.32.0019
Espinosa F., Guerra-Garcia J. M., FA, D., Garcia-Gomez J. C. (2006). Aspects of reproduction and their implications for the conservation of the endangered limpet, Patella ferruginea. Invertebrate Reproduction & Development 49(1-2), 85–92.
Espinosa F., Navarro-Barranco C., González A. R., Maestre M., García-Gómez J. C., Benhoussa A., et al. (2014a). A combined approach to assessing the conservation status of cap des trois fourches as a potential MPA: is there a shortage of MPAs in the southern Mediterranean? doi: 10.12681/mms.775
Espinosa F., Rivera-Ingraham G. A. (2017). Biological conservation of giant limpets: the implications of large size. Adv. Mar. Biol. 76, 105–155. doi: 10.1016/bs.amb.2016.10.002
Espinosa F., Rivera-Ingraham G. A., Fa D., García-Gómez J. C. (2009). Effect of human pressure on population size structures of the endangered ferruginean limpet: toward future management measures. J. Coast. Res. 25 (4), 857–863. doi: 10.2112/08-1005.1
Espinosa F., Rivera-Ingraham G. A., Maestre M., González A. R., Bazairi H., García-Gómez J. C. (2014b). Updated global distribution of the threatened marine limpet Patella ferruginea (Gastropoda: patellidae): an example of biodiversity loss in the Mediterranean. Oryx 48 (2), 266–275. doi: 10.1017/S0030605312000580
Espinosa F., Rivera-Ingraham G. A., Ostalé-Valriberas E., García-Gómez J. C. (2018). Predicting the fate of the most endangered marine invertebrate of the Mediterranean: the power of long-term monitoring in conservation biology. Aquat. Conservation: Mar. Freshw. Ecosyst. 28 (6), 1283–1293. doi: 10.1002/aqc.2944
Ferranti M. P., Guallart J., Fanciulli G., Panzalis P. A., Chiantore M. (2022). Advancements towards restoration of the endangered limpet Patella ferruginea gmelin 1791Through controlled reproduction. Aquaculture Res. 53 (3), 782–798. doi: 10.1111/are.15614
Ferranti M. P., Monteggia D., Asnaghi V., Dagnino A., Gaino F., Moretto P., et al. (2019). Distribution of the Mediterranean ribbed limpet Patella ferruginea gmelin 1791 along the ligurian coast and implications for conservation actions. Mediterr. Mar. Sci. 20 (3), 496–501. doi: 10.12681/mms.20209
Firth L. B., Knights A. M., Bridger D., Evans A. J., Mieszkowska N., Moore P. J., et al. (2016). Ocean sprawl: challenges and opportunities for biodiversity management in a changing world. Oceanography Mar. Biology: an Annu. Rev. 54, 189–262.
Frost N. J., Burrows M. T., Johnson M. P., Hanley M. E., Hawkins S. J. (2005). Measuring surface complexity in ecological studies. Limnology Oceanography: Methods 3 (4), 203–210. doi: 10.4319/lom.2005.3.203
García-Gómez J. C., Guerra-García J. M., Espinosa F., Maestre M. J., Rivera-Ingraham G., Fa D., et al. (2015). Artificial marine micro-reserves networks (AMMRNs): an innovative approach to conserve marine littoral biodiversity and protect endangered species. Mar. Ecol. 36 (3), 259–277. doi: 10.1111/maec.12167
García-Gómez J. C., López-Fé C. M., Espinosa F., Guerra-García J. M., Rivera-Ingraham G. A. (2011). Marine artificial micro-reserves: a possibility for the conservation of endangered species living on artificial substrata. Mar. Ecol. 32 (1), 6–14. doi: 10.1111/j.1439-0485.2010.00409.x
Guallart J., Forner J. B. P., Larruscain J. P. (2013). Primeras imágenes de una forma juvenil de la lapa ferruginosa. Quercus 325), 52–53.
Guallart J., Luque A. A., Acevedo I., Calvo M. (2013b). Distribution and updated census of the ferrugineous limpet (Patella ferruginea gmelin 1791) in the littoral of melilla (SW Mediterranean). Iberus Sociedad Española Malacología 31 (1), 21–51.
Guallart J., Templado J. (2016). Distribución, abundancia y selección del hábitat de Patella ferruginea (Mollusca, Gastropoda) en las islas chafarinas (Mediterráneo suroccidental). Iberus 34 (2), 127–162.
Guerra-García J. M., Corzo J., Espinosa F., Fa D., García-Gómez J. C. (2004a). Extinction risk and harbours as marine reserves? J. Molluscan Stud. 70 (1), 96–98. doi: 10.1093/mollus/70.1.96
Guerra-García J. M., Corzo J., Espinosa F., García-Gómez J. C. (2004b). Assessing habitat use of the endangered marine mollusc Patella ferruginea (Gastropoda, patellidae) in northern Africa: preliminary results and implications for conservation. Biol. Conserv. 116 (3), 319–326. doi: 10.1016/S0006-3207(03)00201-5
Guerra-Garcia J. M., García-Gómez J. C. (2005). Oxygen levels versus chemical pollutants: do they have similar influence on macrofaunal assemblages? a case study in a harbour with two opposing entrances. Environ. pollut. 135 (2), 281–291. doi: 10.1016/j.envpol.2004.10.004
Home R., Keller C., Nagel P., Bauer N., Hunziker M. (2009). Selection criteria for flagship species by conservation organizations. Environ. Conserv. 36 (2), 139–148. doi: 10.1017/S0376892909990051
Howes D., Harper J., Owens E. (1994). Physical shore-zone mapping system for British Columbia. Report prepared by Environmental Emergency Services, Ministry of Environment. (Victoria, BC), Coastal and Ocean Resources Inc.(Sidney, BC), and Owens Coastal Consultants (Bainbridge, WA).
IUCN-WCPA (2019). Guidelines for recognising and reporting other effective area-based conservation measures (Gland, Switzerland: IUCN).
Kallouche M. E. M., Benaissa N., Rouane-Hacene O., Soufi M. E. A., Bouderbala M., Bouras D., et al. (2020). Date endangered status of Patella ferruginea in a limpet assemblage hotspot (Plane island: south West alboran, Mediterranean sea): local distribution factors and species abundance. J. Mar. Island Cultures 9, 1–21. doi: 10.21463/jmic.2020.09.2.10
Kido J. S., Murray S. N. (2003). Variation in owl limpet Lottia gigantea population structures, growth rates, and gonadal production on southern California rocky shores. Mar. Ecol. Prog. Ser. 257, 111–124. doi: 10.3354/meps257111
Lattos A., Bitchava K., Giantsis I. A., Theodorou J. A., Batargias C., Michaelidis B. (2021). The implication of vibrio bacteria in the winter mortalities of the critically endangered Pinna nobilis. Microorganisms 9 (5), 922. doi: 10.3390/microorganisms9050922
Luque Á.A., Guallart J., González J. T., Pola M. (2018). Recopilación y análisis de la información científica disponible sobre Patella ferruginea. Sociedad Española Malacología, 74.
Maestre M. J., Espinosa F., Fa D. A., Pavón A., García-Gómez J. C. (2018). Estado de la población de Patella ferruginea en el área del estrecho de Gibraltar. Almoraima: Rev. estudios campogibraltareños 49), 115–130.
Mar (2020) La lapa que da la lata. ecologistas en acción. Available at: https://www.ecologistasenaccion.org/134671/la-lapa-que-da-la-lata/.
Marra S., de Lucia G. A., Camedda A., Espinosa F., Coppa S. (2016). New records of the distribution and conservation status of the endangered limpet Patella ferruginea in Sardinia (Italy, W Mediterranean). Aquat. Conservation: Mar. Freshw. Ecosyst. 26 (3), 607–612. doi: 10.1002/aqc.2615
MMAMRM (2008). “Estrategia de conservación de la lapa ferrugínea (Patella ferruginea) en españa,” in Conferencia sectorial de medio ambiente, ministerio de medio ambiente, medio rural y Marino(Madrid, Spain), 56.
Nachite D., Domínguez N. D. E., El M'rini A., Anfuso G. (2020). Environmental sensitivity index maps in a high maritime transit area: the Moroccan coast of the Gibraltar strait study case. J. Afr. Earth Sci. 163, 103750. doi: 10.1016/j.jafrearsci.2020.103750
Ostalé-Valriberas E., Sempere-Valverde J., Pavón-Paneque A., Coppa S., Espinosa F., García-Gómez J. C. (2022). Artificial marine micro-reserves as a new ecosystem-based management tool for marine conservation: the case of Patella ferruginea (Gastropoda, patellidae), one of the most endangered marine invertebrates of the Mediterranean. Mar. Policy 136, 104917. doi: 10.1016/j.marpol.2021.104917
Pallant J. (2020). SPSS Survival manual: a step by step guide to data analysis using IBM SPSS (Routledge).
Periáñez R. (2007). Chemical and oil spill rapid response modelling in the strait of Gibraltar–alborán Sea. Ecol. Model. 207 (2-4), 210–222. doi: 10.1016/j.ecolmodel.2007.04.033
Piniella F., Walliser J. (2013). Maritime safety in the strait of Gibraltar: taxonomy and evolution of emergencies rate in 2000-2004 period. J. Maritime Res. 10 (1), 25–30.
Pulliam H. R. (1988). Sources, sinks, and population regulation. Am. Nat. 132 (5), 652–661. doi: 10.1086/284880
Pulliam H. R. (1996). Sources and sinks: empirical evidence and population consequences (Population dynamics in ecological space and time).
Ramos M. A. (1998). Implementing the habitats directive for mollusc species in Spain. J. Conchology Special Publ. 2, 125–132.
Rivera-Ingraham G. A., Espinosa F., García-Gómez J. C. (2011a). Environmentally mediated sex change in the endangered limpet Patella ferruginea (Gastropoda: patellidae). J. Molluscan Stud. 77 (3), 226–231. doi: 10.1093/mollus/eyr007
Rivera-Ingraham G. A., Espinosa F., García-Gómez J. C. (2011b). Conservation status and updated census of Patella ferruginea (Gastropoda, patellidae) in ceuta: distribution patterns and new evidence of the effects of environmental parameters on population structure. Anim. Biodiversity Conserv. 34 (1), 83–99. doi: 10.32800/abc.2011.34.0083
Rivera-Ingraham G. A., Espinosa F., Garcia-Gomez J. C. (2011c). Ecological considerations and niche differentiation between juvenile and adult black limpets (Cymbula nigra). J. Mar. Biol. Assoc. United Kingdom 91 (1), 191–198. doi: 10.1017/S0025315410000159
Ros M., Lacerda M. B., Vázquez-Luis M., Masunari S., Guerra-García J. M. (2016). Studying exotics in their native range: can introduced fouling amphipods expand beyond artificial habitats? Biol. invasions 18 (10), 2983–3000. doi: 10.1007/s10530-016-1191-5
Ros M., Vazquez-Luis M., Guerra-Garcia J. M. (2015). Environmental factors modulating the extent of impact in coastal invasions: the case of a widespread invasive caprellid (Crustacea: amphipoda) in the Iberian peninsula. Mar. pollut. Bull. 98 (1-2), 247–258. doi: 10.1016/j.marpolbul.2015.06.041
Sánchez-Badorrey E., García-García J. A., Ortego-Jurado R., García Anguita F. J. (2021). Variabilidad climática, renovación y calidad de las aguas del puerto de ceuta y su entorno (Instituto de Estudios Ceutíes), 115–118.
Sedano F., Navarro-Barranco C., Guerra-García J. M., Espinosa F. (2020). Understanding the effects of coastal defence structures on marine biota: the role of substrate composition and roughness in structuring sessile, macro-and meiofaunal communities. Mar. pollut. Bull. 157, 111334. doi: 10.1016/j.marpolbul.2020.111334
Small C., Nicholls R. J. (2003). A global analysis of human settlement in coastal zones. J. Coast. Res. 19 (3), 584–599.
Smith A. M., Sutton S. G. (2008). The role of a flagship species in the formation of conservation intentions. Hum. Dimensions Wildlife 13 (2), 127–140. doi: 10.1080/10871200701883408
Taibi A., Oubaziz B., Ghermaoui M., Kaddour Hocine A., Bendimerad M. E. (2014). Etude de la biométrie de la patelle géante Patella ferruginea à l’île de rachgoun. Proc. BEL 3, 26–28.
Terrón-Sigler A., León-Muez D., Peñalver-Duque P., Torre F. E. (2018). A method for increasing the survival of shallow-water populations of the endemic coral Astroides calycularis. J. Mar. Biol. Assoc. United Kingdom 98 (4), 811–818. doi: 10.1017/S0025315416001806
Thompson R. C., Tobin M. L., Hawkins S. J., Norton T. A. (1999). Problems in extraction and spectrophotometric determination of chlorophyll-a from epilithic microbial biofilms: towards a standard method. J. Mar. Biol. Assoc. United Kingdom 79 (3), 551–558. doi: 10.1017/S0025315498000678
Tlig-Zouari S., Rabaoui L., Fguiri H., Ben Hassine O. K. (2010). Status, habitat and distribution of the endangered limpet Patella ferruginea along the northern and eastern Tunisian coastline: results and implications for conservation. Cahiers Biologie Mar. 51 (1), 75.
Valverde J. M., Perejon A., Medina S., Perez-Maqueda L. A. (2015). Thermal decomposition of dolomite under CO 2: insights from TGA and in situ XRD analysis. Phys. Chem. Chem. Phys. 17 (44), 30162–30176. doi: 10.1039/C5CP05596B
Vázquez-Luis M., Álvarez E., Barrajon A., Garcia-March J. R., Grau A., Hendriks I. E., et al. (2017). SOS Pinna nobilis: a mass mortality event in western Mediterranean Sea. Front. Mar. Sci. 4, 220. doi: 10.3389/fmars.2017.00220
Vela A., Leoni V. (2007). Etude des espèces de l’étage médio-littorale sur la jetée du port de commerce de bastia-recensement des effectifs de Patella ferruginea. Contrat sentinelle collectivité territorial Corse 18 p. doi: 10.12681/mms.20209
Keywords: Patella ferruginea, patellogastropoda, conservation, extinction risk, population structure, artificial marine micro-reserves, artificial substratum, source population
Citation: Ostalé-Valriberas E, Sabino-Lorenzo Á, Ali-Ahmed A, Pavón-Paneque A, Sempere-Valverde J, Espinosa F and García-Gómez JC (2023) When the population of an endangered marine mollusc (Patella ferruginea) increases almost three-fold in ten years. Reality or fiction? Front. Mar. Sci. 10:1127630. doi: 10.3389/fmars.2023.1127630
Received: 19 December 2022; Accepted: 08 June 2023;
Published: 12 July 2023.
Edited by:
Alan Hodgson, Rhodes University, South AfricaReviewed by:
Francesco Tiralongo, University of Catania, ItalyMariachiara Chiantore, University of Genoa, Italy
Copyright © 2023 Ostalé-Valriberas, Sabino-Lorenzo, Ali-Ahmed, Pavón-Paneque, Sempere-Valverde, Espinosa and García-Gómez. This is an open-access article distributed under the terms of the Creative Commons Attribution License (CC BY). The use, distribution or reproduction in other forums is permitted, provided the original author(s) and the copyright owner(s) are credited and that the original publication in this journal is cited, in accordance with accepted academic practice. No use, distribution or reproduction is permitted which does not comply with these terms.
*Correspondence: E. Ostalé-Valriberas, enriqueostalevalriberas@gmail.com