- 1School of Ocean and Earth Science, National Oceanography Centre, University of Southampton Waterfront Campus, Southampton, United Kingdom
- 2Biodiversity, Evolution, and Adaptation Team, British Antarctic Survey, Cambridge, United Kingdom
In 2018 RRS James Clark Ross investigated the marine benthic biodiversity of the Prince Gustav Channel area which separates the eastern coast of the Antarctic Peninsula from James Ross Island. The southern end of this channel had been covered by the Prince Gustav Ice Shelf until its collapse in 1995. Benthic samples were collected by an epibenthic sledge at six stations (200–1,200 m depth) in the channel and adjacent Duse Bay. In total 20,307 live collected mollusc specimens belonging to 50 species and 4 classes (Solenogastres, Bivalvia, Gastropoda, and Scaphopoda) were identified. The area may be characterised by it’s low species richness (ranging from 7 to 39 species per station) but high abundances (specifically of the Scaphopods with 11,331 specimens). The functional traits of the community were dominated by motile development and mobility type. Assemblage analyses of the molluscan species abundances within the Prince Gustav Channel stations sit distinct, with no pattern by depth or location. However, when bivalve assemblages were analysed with reference to the wider Weddell Gyre region (15 stations from 300 to 2,000 m depth), the Prince Gustav Channel sits distinct from the other Weddell Gyre stations with a higher dissimilarity between the deeper or more geographically distant areas. The Prince Gustav Channel is undergoing colonisation following the recent ice shelf collapse. With many Antarctic ice shelves threatened under climate warming, this area, with future monitoring, may serve as a case study of benthic faunal succession.
Introduction
The Antarctic Peninsula is the most northerly part of mainland Antarctica and in recent years has experienced dramatic warming of both the atmosphere and ocean as a result of climate change (Skvarca et al., 1998; Vaughan et al., 2003; Turner et al., 2005). Floating ice shelves, fed by the continental ice sheet, are located at the coastline and as such are sensitive to any changes in atmospheric or oceanic circulation or temperature (Rott et al., 1996). Ice shelves around the Antarctic Peninsula have retreated by approximately 75% in the past 50 years (Cook and Vaughan, 2010). The Larsen Ice Shelf, previously described as ‘one of the two great areas of shelf ice in the Weddell Sea’ by Mason (1950), has since undergone significant reduction and collapse. In 1957 the Prince Gustav Ice Shelf (PGIS), the most northerly portion (Ferrigno, 2006), was separated from the main Larsen Ice Shelf. By January 1995, a 4,200 km2 of the northern Larsen Ice shelf (Larsen A) and the PGIS broke away, eventually causing the shelf to collapse (Rott et al., 1996). In 2002 the Larsen B part collapsed (Rack and Rott, 2004) and in 2017 a 5,800 km2 iceberg (A68) broke off the Larsen C part (Marchant, 2017).
The investigated Prince Gustav Channel area (PGC) is defined in this study as Duse Bay (DB) and the Prince Gustav Channel, situated in the western Weddell Sea and the region affected by the Weddell Gyre (Figure 1; Vernet et al., 2019). The Prince Gustav Channel is a narrow passageway separating the eastern coast of the Antarctic Peninsula from the volcanic James Ross Island (Figure 1B). The Prince Gustav Channel has an over-deepened trough with a maximum depth of 1,500 m as a result of glacial quarrying, made shallower to about 1,300 m as a result of infill by several hundred meters of glacial sediments (Camerlenghi et al., 2001). Currents in the Prince Gustav Channel have a net flow from the northern entrance of the channel (∼24 km at its widest and ∼9 km at its narrowest) westward and south through the channel (Camerlenghi et al., 2001). The southern entrance of the Prince Gustav Channel is approximately 12.5 km at its widest, 8 km at its narrowest. Duse Bay is a sheltered inlet, with an entrance approximately 7 km wide, along the east side of the Antarctic Peninsula, adjacent and connected to the Prince Gustav Channel (Figure 1B).
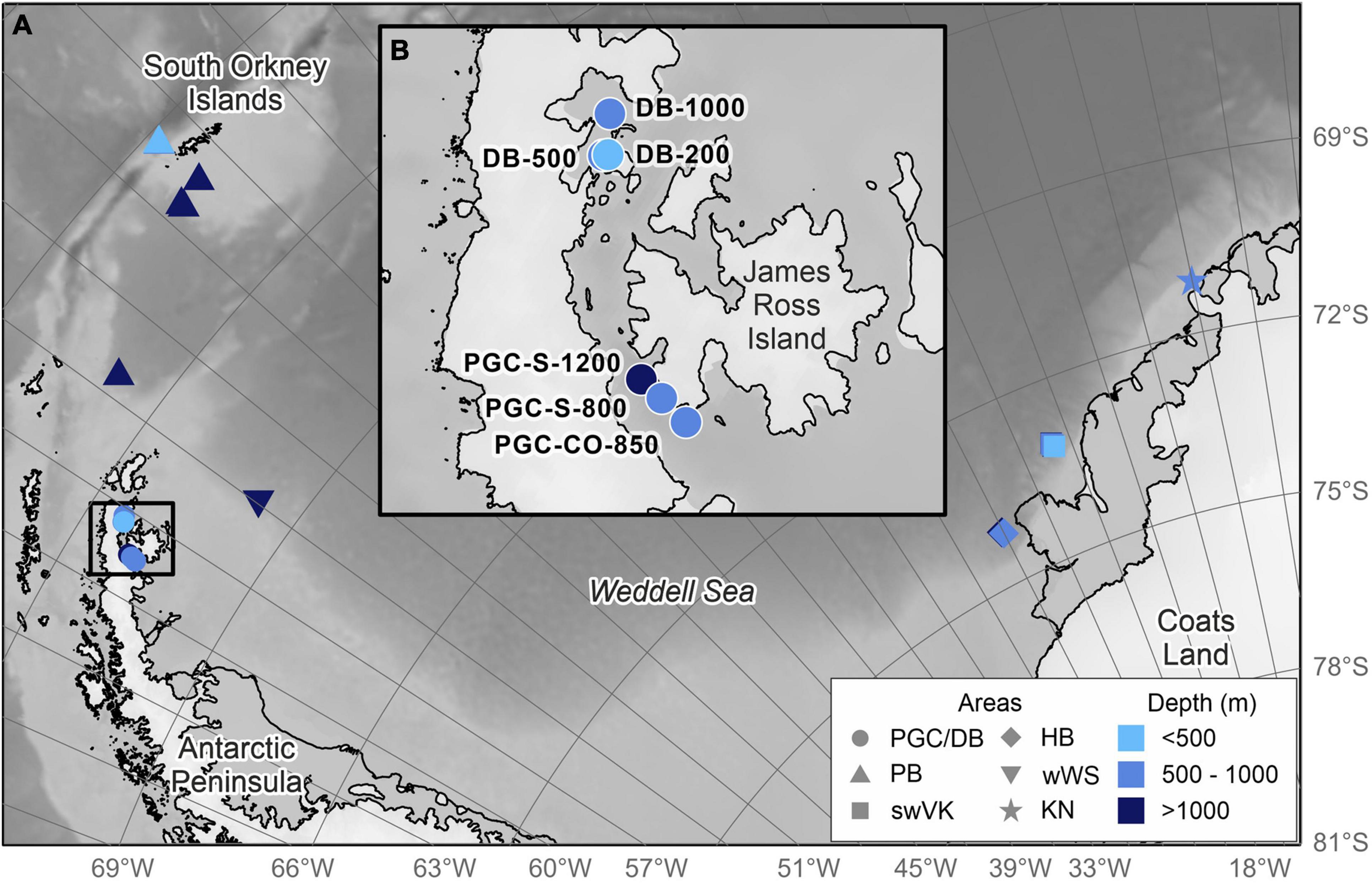
Figure 1. Location of stations within the study area. (A) The western Weddell Gyre region including the Weddell Sea and South Orkney Islands. DB, Duse Bay; PGC, Prince Gustav Channel; PB, Powell Basin; swVK, southwest of Vestkapp; HB, Halley Bay; wWS, western Weddell Sea; KN, Kap Norvegia. (B) The Prince Gustav Channel area. S, South; CO, Cape Obelisk.
In the past 200 years the PGC has undergone dramatic changes in both ice cover and environmental conditions as a result of the aforementioned collapse of the Larsen A and Prince Gustav ice shelves. A number of geological surveys were undertaken to the PGC to explore the contourites, deglaciation, and climate change (Camerlenghi et al., 2001; Roberts et al., 2011; Sterken et al., 2012) but no benthic biological sampling of the area occurred until the RRS James Clark Ross JR17003a expedition in 2018 (Linse, 2018). To date, studies from this expedition include in-situ image analysis of the benthic habitat heterogeneity and biodiversity in the PGC (Almond et al., 2021), diversity analysis of the annelid, and nematode faunas in the PGC benthos (Drennan et al., 2021; Pantó et al., 2021), peracarid crustaceans from the Weddell Gyre region (WG), including the PGC (Di Franco et al., 2020, 2021).
The Antarctic continental shelf is a highly specialised ecosystem as a result of several unique characteristics (Clarke, 1996). One is the unusually deep continental shelves; global continental shelves are at approximately 100–200 m deep, however, as a result of isostatic depression, the Antarctic continental shelves may be 450–1,000 m deep (Clarke and Johnston, 2003). In addition, shelf habitats are influenced by ice, with iceberg and brash ice scouring events and the sediments are predominantly glacial-marine (Clarke, 1996). Next to by seasonality, primary productivity within the Southern Ocean is highly influenced by sea ice presence and duration, coastal polynyas, and the iron input from melting ice shelves, impacting biological export to the benthos (Smith and Comiso, 2008; Arrigo et al., 2015).
Biological research on and description of new species of the Antarctic benthos dates back to the Heroic Age of Antarctic Exploration at the end of the 19th century and early 20th century (Griffiths, 2010). Since then the biodiversity of the Southern Ocean, including the macrobenthos have been studied considerably (Griffiths, 2010). One of the most-studied phyla in the Southern Ocean are the molluscs, second in the collection records only to the arthropods (De Broyer and Danis, 2011; Schiaparelli and Linse, 2014). Molluscs have a strong and deep-time fossil record in Antarctica (Whittle et al., 2019) and developed different adaptations to life in the Southern Ocean (Clarke and Crame, 2010; Peck, 2018), supporting studies into their evolutionary histories, phylogeographies, and predicting their futures (e.g., González-Wevar et al., 2019; Moles et al., 2019). As a species rich and diverse taxon, the Mollusca are often used as a model group for both biodiversity records and benthic community assemblage analyses from shallow waters to the deep sea (e.g., Arnaud et al., 2001; Linse et al., 2006; Troncoso and Aldea, 2008; Linse, 2014; Schiaparelli and Linse, 2014; Amsler et al., 2015; Rosenfeld et al., 2017).
The aims of this study are to (1) describe the macrobenthic molluscan abundance, richness and diversity of the previously unstudied PGC area of the Weddell Sea that is vulnerable to climate change, setting the current baseline for future biodiversity studies, (2) describe the assemblage structure and functional traits of the macrobenthic mollusc assemblages in the PGC, and (3) understand the biogeographic position of the PGC within the Southern Ocean by comparing the bivalve assemblage to other areas within the wider Weddell Gyre region.
Materials and Methods
Sample Collection
The RRS James Clark Ross expedition JR10073a to the PGC took place between February and March 2018 (Linse, 2018). Sampling occurred at three stations in DB and three stations in the Southern end of the Prince Gustav Channel, twice at PGC-S and once PGC-CO (Figure 1 and Table 1). The distinction between PGC-S and PGC-CO was made because, prior to 1995, PGC-CO had been covered by the now collapsed PGIS. DB stations are considered a separate unit to the PGC stations due to their restricted geographic location.
Macrobenthic Mollusca were sampled with an epibenthic sledge at six stations (Linse, 2018). The epibenthic sledge was towed for 10 min at a mean velocity of 1 knot and trawling distances were then calculated from the start of trawling to the time the sledge left the seafloor, following equation #4 of Brenke (2005). The collected material was preserved in 96% pre-cooled ethanol, kept at −20°C while onboard for 4 months and then stored at ambient temperature in Cambridge.
In the laboratory, samples were initially sorted under a stereomicroscope to class level and, whenever possible, classified to morphospecies level (specimens identified by morphology without a genetic identification). Juvenile specimens were assigned to family or genus level. Live specimen numbers were counted and thanatocoenosis were not taken into account. The molluscan dataset includes all morphospecies and abundance counts from all six stations and include four classes (Solenogastres, Bivalvia, Gastropoda, and Scaphopoda). The bivalve and gastropod datasets are sub-datasets of the molluscan data. Morphospecies will hereon in be referred to as ‘species.’
For comparison between stations, abundance data were standardised to 1,000 m2 haul lengths. PGC bivalve assemblages were compared with other available bivalve assemblage data collected by epibenthic sledge from further stations of equivalent depth within the Weddell Gyre region (Figure 1). Bivalve assemblage data from 15 stations across 6 areas in the Weddell Gyre region, at depths from 300 to 2,000 m, were used in this comparative analysis (Linse and Anderson, 2021).
Biodiversity Analyses
To assess patterns in species richness, specimen abundances, biodiversity and assemblage structure of the macrobenthic molluscs present in the epibenthic sledge samples, univariate (species abundance, richness and diversity indices) and multivariate (cluster analysis, rarefaction analyses, SIMPROF, and SIMPER) analyses were performed with R v4.0.4 (R Core Team, 2020), RStudio v1.2.5 (RStudio Team, 2019) with the packages ‘ggplot2’ (Wickham, 2016), and ‘viridis’ (Garnier et al., 2021) and PRIMER6 (Clarke and Gorley, 2006).
Species Abundance, Richness, and Diversity
Univariate analyses for diversity indices were run for all molluscan taxa, bivalves only and gastropods only. For comparison, four commonly used diversity indices were run: Margalef species richness (D), Pielou evenness (J′), Shannon-Wiener diversity [H′(loge)], and Simpson diversity (I-λ’) (Clarke and Gorley, 2006). Diversity indices are used to quantitatively analyse biodiversity with a dataset, with each index assessing different assemblage features including richness, evenness, or diversity. We refer to species only found with one specimen as ‘singletons’ and species found with two specimens as ‘doubletons.’ Abundances were summed to class level. Non-standardised epibenthic sledge counts were compiled into percentage stacked bar plots for relative mollusc abundances. The percentage relative abundance plot compares the assemblage composition (by class) between stations regardless of total abundance. The stacked standardised abundance (1,000 m–2) plots compare the total abundances by class at each station.
Functional Traits
Definitions of functional traits follow Hogg et al. (2018). For this study, development type [categories: (1) lecithotrophic, (2) planktotrophic, (3) brooder, and (4) direct developer] reproduction mode [categories: (1) gonochoric, (2) hermaphroditic, and (3) asexual], mobility [categories: (1) crawler, (2) burrower, and (3) sessile (encrusting and erect)] and lifestyle [categories: (1) infauna, (2) epifauna, (3) epibiotic, (4) demersal, and (5) pelagic] were considered (Linse and Anderson, 2021). Additional traits used by Hogg et al. (2018), like substratum affinity, were largely unknown and excluded. Taxa were ascribed functional traits as per current literature (e.g., Hain, 1990; Hain and Arnaud, 1992; WoRMS Editorial Board, 2021). Some traits are not always known at an individual species level and were taken from higher taxon levels, such as family or genus.
Bremner et al. (2006) describes ecological functioning with three methods of biological trait analyses; one of which is to use fuzzy coding analyses. After taxa were ascribed functional traits from sub-descriptions, taxa were scored on the extent that they expressed these categories, enabling any variations in trait exhibition to be taken into account (Chevene et al., 1994). As per Bremner et al. (2006) a scale of 0–3 was used; 0 being no expression of that trait category, or unknown, and 3 being only expression within that trait category. The taxa abundance by station table and biological trait score by taxa tables were then multiplied. Trait category scores were multiplied by station abundances, to form a functional trait by station by category table. Category scores by taxa were summed, resulting in a station by category table for molluscan taxa (Linse and Anderson, 2021). A relative percentage stack plot of abundance by station was formed per functional trait.
Assemblage Structure
To analyse patterns in assemblage structure, for molluscan, gastropods and bivalves multivariate cluster analyses were formed in PRIMER6 (Clarke and Gorley, 2006). Standardised abundances (1,000 m–2) were then pre-treated with a range of transformations from no transformation through to presence-absence. A square root transformation was used for all PGC community assemblage analyses. Weddell Gyre samples were fourth root transformed. A Bray-Curtis analysis of resemblance was used to compare the composition similarity between stations and areas.
A similarity profile (SIMPROF) was run (Clarke and Gorley, 2006) to test for evidence of the groupings within the dataset. A similarity percentages routine (SIMPER) was run on the SIMPROF groups (Clarke and Gorley, 2006) to understand intra-group similarities, inter-group dissimilarities and to identify the driving species and abundances behind these. No cut off for contributing species was applied (100%) to the dissimilarity nor the similarity.
The Weddell Gyre region data were split into three groupings: all PGC stations, WG-shallow (≤000 m), and WG-deep (>1,000 m) for subsequent rarefaction analyses (number of Expected Species (ES) and species accumulation). The species accumulation ran for 999 permutations (Clarke and Gorley, 2006) and the output values were plotted (RStudio Team, 2019; R Core Team, 2020). The ES values for each group were calculated for the ES-32 value of each station and averaged across the group. Three WG stations had specimen numbers lower than 32 (wWS-1000, PB-1600, HB-1000) so were removed from these analyses.
Results
Prince Gustav Channel Biodiversity, Functional Traits, and Assemblage Structure
The 6 epibenthic sledge trawls across PGC yielded 20,307 specimens from 4 classes, 25 families and 50 species (Table 1, Linse and Anderson, 2021).
Gastropoda (320 specimens) and Bivalvia (8,622 specimens) were the two most species rich classes with 24 and 18 species, respectively (Table 2). Although the least species rich (2 species), the most abundant class was the Scaphopoda (11,331 specimens). The most abundant species was the scaphopod Cadulus thielei with 11,019 specimens. The most abundant bivalve species was Yoldiella valettei (6,725 specimens) followed by Thyasira debilis (1,167 specimens) and Yoldiella sabrina (248 specimens). The most abundant gastropod species was Lissotesta sp. (53 specimens) followed by Lissotesta strebeli (44 specimens) and Onoba georgiana (39 specimens). Ten species (Solenogastres sp.2, Limatula hodgesoni, Cyclochlamys gaussianus, Lyonsia arcaeformis, Dickdellia labioflecta, Kerguelenatica delicatula, Omalogyra antarctica, Melanella sp., Cuthona sp. and Skeneidae sp. 2) occurred as singletons only and six species (Solenogastres sp. 5 & 6, Torellia smithi, Mangeliidae sp., Philinidae sp., Falsitromina sp.) as doubletons in the samples while others, e.g., Cuspidaria infelix, Ptychocardia vanhöffeni, Falsitromina sp., Mangellidae sp. and Margarella cf. whiteana, were represented by mostly juvenile specimens.
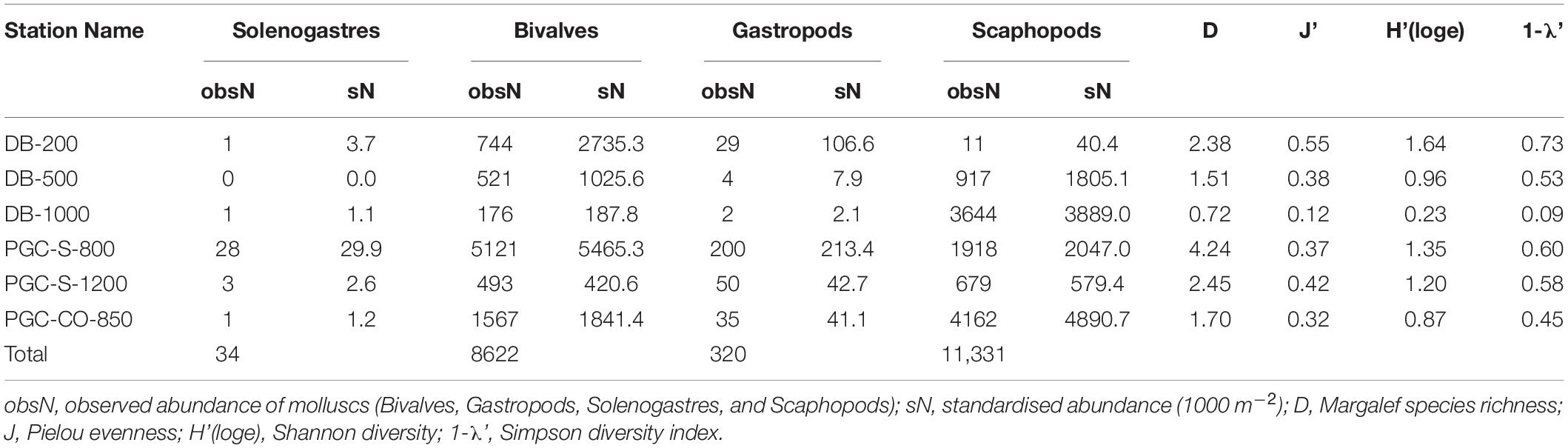
Table 2. Station names (DB, Duse Bay; PGC, Prince Gustav Channel; S, South; CO, Cape Obelisk) with raw and standardised abundances (1000 m–2) and diversity indices.
Lowest standardised molluscan abundances (1,000 m–2) observed in this study were found at the deepest station PGC-S-1200 with 1045 individuals per 1,000 m2 and highest at PGC-S-800 with 7,267 individuals per 1,000 m2 (Table 1 and Figure 2A). Species richness was highest at PGC-S-800 with 39 species and lowest at DB-1000 with 7 species. Average species richness was 19 species. The lowest gastropod species richness and standardised abundances were at DB-1000 with 1 species and 2 individuals per 1,000 m2. Highest gastropod species richness and abundance was at PGC-S-1200 with 19 species and 213 individuals per 1,000 m2 (Table 2). Lowest bivalve species richness and standardised abundance occurred at DB-1000 with 4 species and 188 individuals per 1,000 m2. Highest bivalve species richness was at PGC-S-1200 with 14 species and 5465 individuals per 1,000 m2.
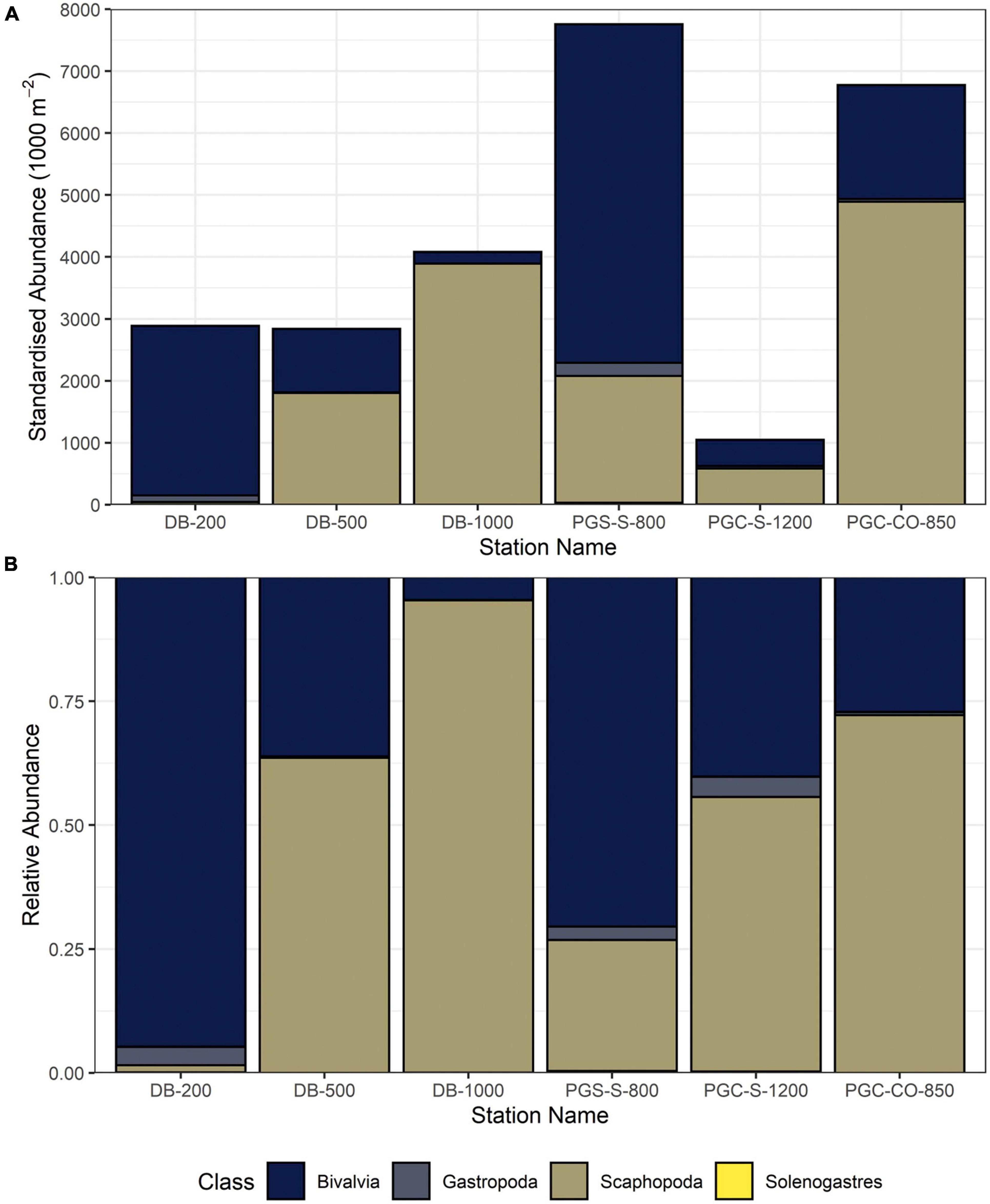
Figure 2. Relative and standardised abundances of molluscan classes by station (DB, Duse Bay; PGC, Prince Gustav Channel; S, South; CO, Cape Obelisk). (A) Relative abundances. (B) Standardised abundances (per 1,000 m2) of molluscan classes.
Observed relative abundances per class differed between stations and depth (Figure 2B); no general consistent pattern was found. Bivalve relative abundance dominated at Station DB-200 and PGC-S-800 but scaphopods dominated at the remainder of the stations between 55 and 95% (mean of 2,264 specimens). All four classes were represented at each station.
DB-200 is the most diverse (Shannon-Wiener - 1.64, Simpson - 0.73) and even (0.55) station, although PGC-S-800 is the richest (4.24). Station DB-1000 is the least rich (0.72), even (0.12), and diverse (Shannon-Wiener - 0.23, Simpson - 0.09) (Table 2).
In order to assess if certain trait categories dominate the molluscan assemblage in the PGC, categories of the development, mobility, lifestyle, and reproductions traits were evaluated. In the molluscan dataset regarding the trait development, station DB-200 and PGC-S-800 were dominated by lecithotrophic species (Bivalvia – 8 species), meanwhile the remaining stations were dominated by planktotrophic taxa (Scaphopoda – 1 species) (Figure 3). In the mobility trait category burrowing taxa (48 species) dominated over crawling taxa (2 species) at all stations but DB-200 and PGC-S-800. The mobility type for both gastropods and bivalves was entirely crawling taxa. In the reproductive mode trait, asexual reproducing taxa were absent while hermaphrodite taxa, represented by 8 bivalve species, were the minority compared to the dominant gonochoric taxa (40 species). The lifestyle traits were dominated by infaunal taxa (15 species and 28,439 individuals) over epifauna (21 species and 323 individuals) and epibiotic taxa (4 species and 66 individuals). Gastropod lifestyle was dominated by epifauna (19 species), with some epibiotic taxa (2 species) at PGC-S-800. Infaunal bivalve taxa dominated (13 species) over epibiotic taxa which were present (2 species) at all stations apart from DB-500.
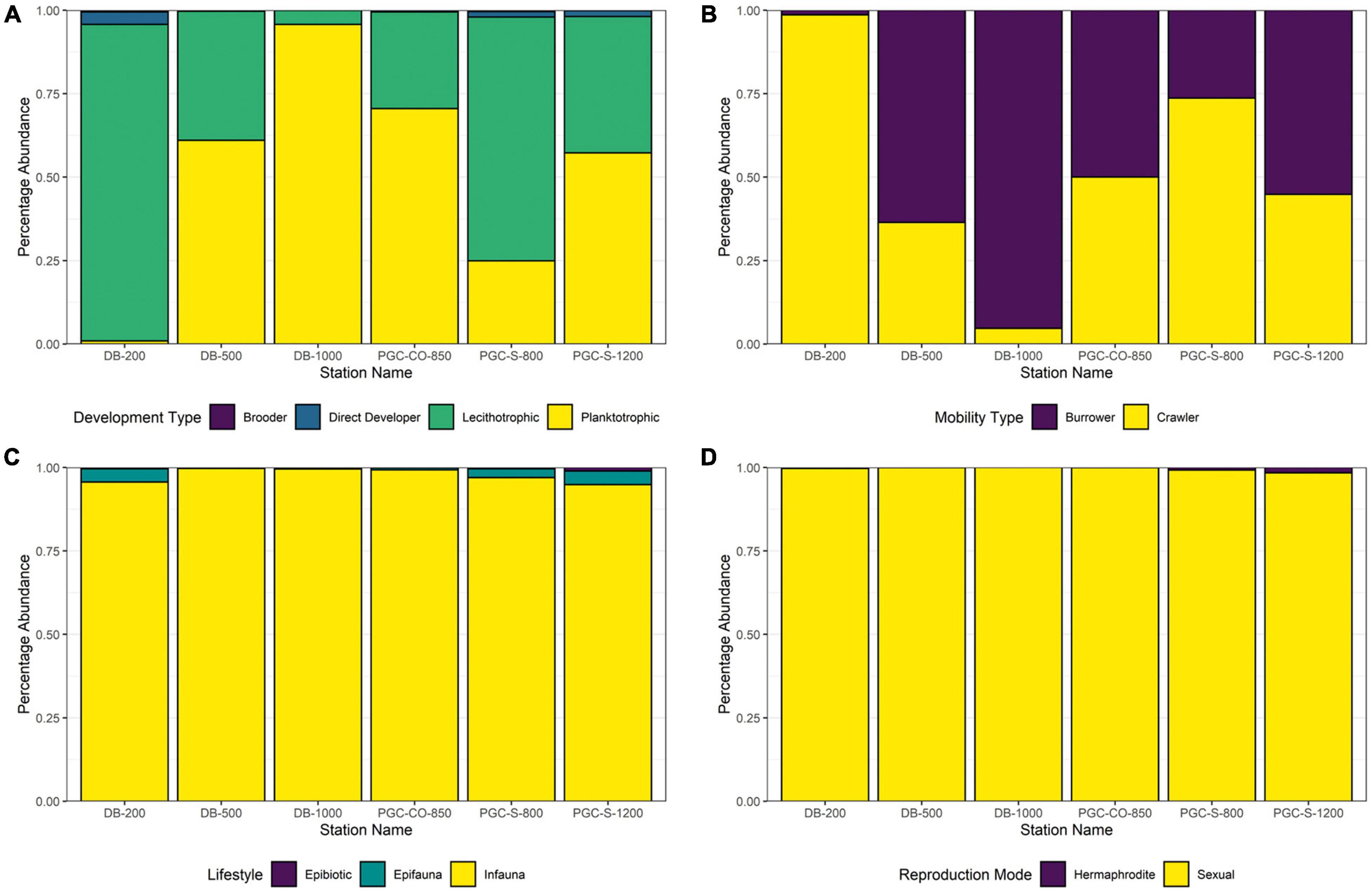
Figure 3. Contributions of functional traits to molluscan relative abundances by station (DB, Duse Bay; PGC, Prince Gustav Channel; S, South; CO, Cape Obelisk). (A) Development type. (B) Mobility type. (C) Lifestyle. (D) Reproduction Mode.
The similarity cluster based on the standardised molluscan abundances (1,000 m–2) showed all stations to sit distinct from each other (Figure 4). Similarity analyses at class level for both bivalves and gastropods showed the same results (results not shown).
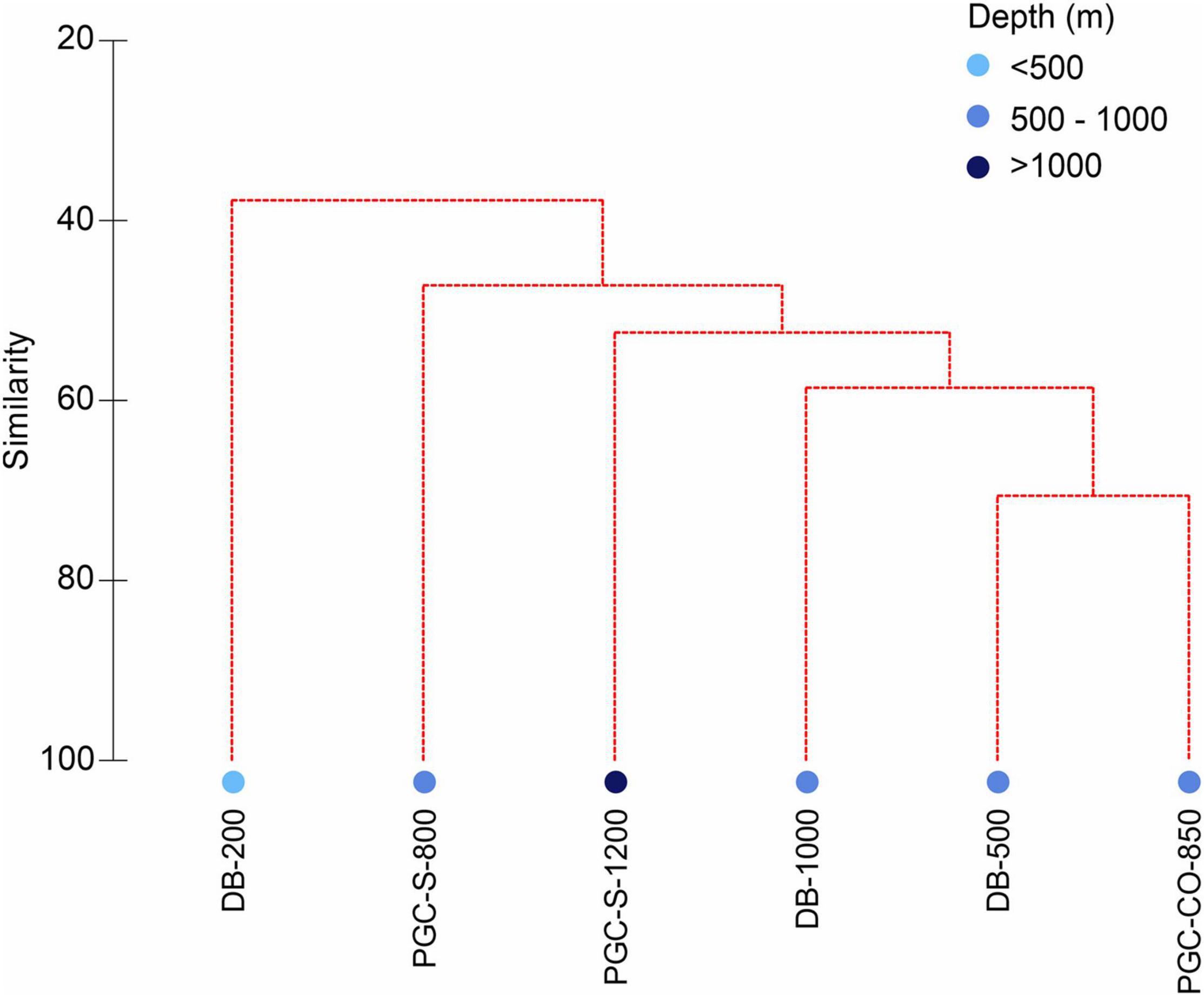
Figure 4. PGC molluscan similarity species cluster analysis by station (DB, Duse Bay; PGC, Prince Gustav Channel; S, South; CO, Cape Obelisk). Square root transformation and Bray Curtis similarity of resemblance.
Weddell Gyre Bivalve Assemblage Structure
The bivalve assemblages of the PGC were compared with available bivalve assemblage data from other areas of comparable depth within the WG. The PGC had the lowest species richness with 18 bivalve species, followed by the Weddell Gyre Deep (WG-deep) group with 26 species and Weddell Gyre Shallow (WG-shallow) with 35. The WG-deep group had the lowest standardised mean species abundances of 127 individuals per station, the WG-shallow group had an average of 1,104 individuals per station, and the PGC had the greatest species abundance of 1,960 individuals per station.
Rarefaction analyses found the PGC stations had a lower average ES-32 diversity of 3.8 (ES-32 range 2.8–5.2) than the WG-deep with an average at 5.4 (ES-32 range 4–6.9) and the WG-shallow with an average at 5.3 (ES-32 range 3.6–6.6). The species accumulation curves (Figure 5) across the three groups showed that the species richness across the PGC was lower than for the WG-deep and WG-shallow. At station 6, the highest comparable point, the PGC had 18 species, WG-deep had 24 and WG-shallow had 30 species. The PGC curve appeared to be flattening off and even with the additional stations in the WG groups, the curves continued to increase, with the shallow grouping showing the highest diversity.
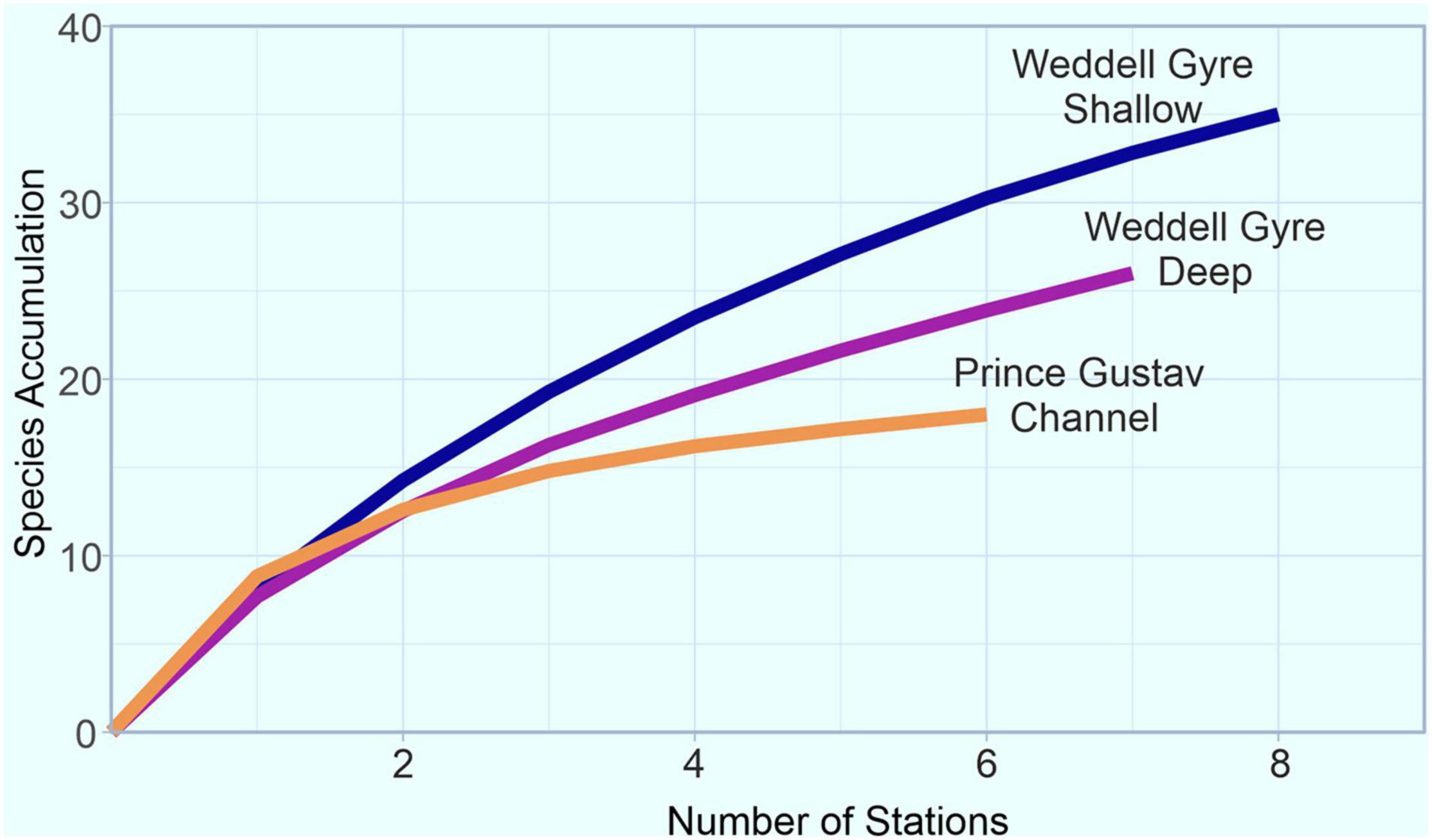
Figure 5. Species accumulation across number of stations for the Weddell Gyre (WG) region divided into three groups: WG-shallow, WG-deep, and Prince Gustav Channel (PGC).
The SIMPROF analysis to identify significant clusters identified 6 main groupings based on location and depth (Figure 6). Groups A and B were PGC based groupings, Group C consisted of shallow water samples from the Powel Basin (PB, western Weddell Sea), Group D was comprised of mostly deep-water samples from around the wider Weddell Gyre, Group E were shallow water samples from the eastern Weddell Sea, Group F were low diversity and low abundance samples and HB-1000 is an outlier. HB-1000 was likely an outlier as a result of the very low diversity and abundance at the station with only two species, Yoldiella cf ecaudata and Dacrydium sp. 1 each with a standardised abundance of 3 individuals per 1,000 m2.
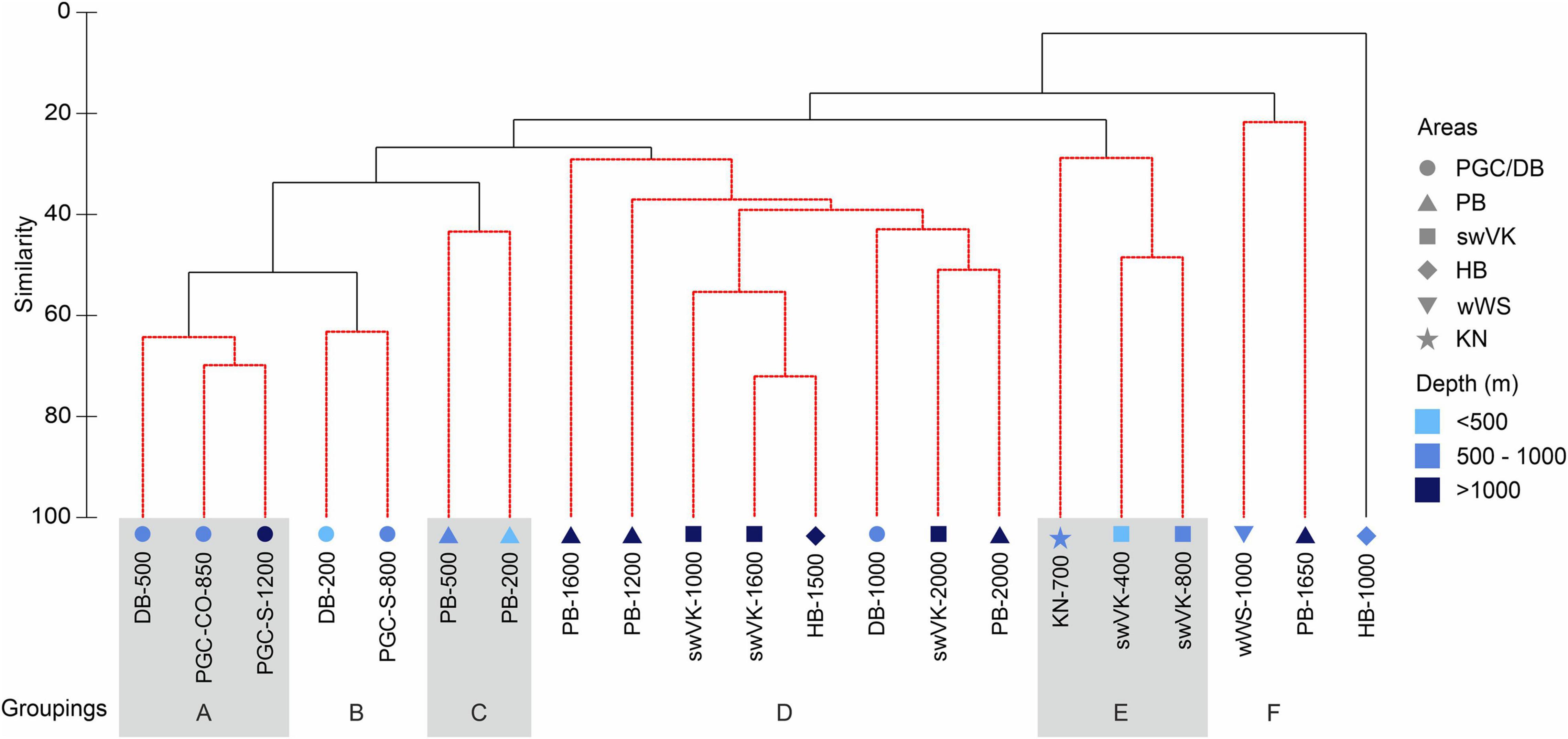
Figure 6. Weddell Gyre region similarity species cluster analysis by station. DB, Duse Bay; PGC, Prince Gustav Channel; PB, Powell Basin; swVK, southwest of Vestkapp; HB, Halley Bay; wWS, western Weddell Sea; KN, Kap Norvegia; DB, Duse Bay; PGC, Prince Gustav Channel; S, South; CO, Cape Obelisk. Fourth root transformation and Bray Curtis similarity of resemblance. SIMPROF groupings in red.
The SIMPER analysis showed that the two SIMPROF derived PGC groups had higher intra-group similarity (A had 66.15% and B 63.22%) than the PB and WG groups (C 43.39%, D 39.18%, E 35.36% and the lowest group F with 21.69%). Twelve species characterised 80% of the intra-group similarities (between 2 and 6 species per group). One species, Yoldiella valettei, contributed significantly to five of the six (A–D) groups’ intra-group similarity, between 10.23 and 40.3% of these similarities. Axinulus antarcticus contributed to 5 of the 6 groups, but only significantly to three groupings (C at 27.11%, and over 50% in E and F) (Supplementary Material). Dacrydium albidum contributed 51.33% to group F’s similarity.
Dissimilarity between the different groupings is shown in Table 3. The two PGC groups (A and B) come out as least dissimilar to each other (48.54%), supporting the existence of a distinct PGC assemblage. The group geographically closest to the PGC stations, the shallow water PB stations (group C) clustered closest to Figure 6 and had the lowest level of dissimilarity with (Supplementary Material), groups A and B. All the western Weddell Sea groups (A, B, and C) had high dissimilarities (over 70%) with group E (shallow water eastern Weddell Sea). Deep water stations from the rest of the Weddell Gyre grouped together as a single group, D with dissimilarities to other groups ranging between 65.6 and 82.18%. The biggest dissimilarities were found between the PGC groups and group F as a result of the high abundances within groups A and B and the low abundance region of group F as well as differences in species present (8 species with A and 10 species with B).
Five of the 49 bivalve species (Thyasira debilis, Yoldiella valettei, Yoldiella sabrina, Axinulus antarcticus, and Cuspidaria kerguelensis) are the main drivers to the groupings and are responsible for at least 10% of the inter-group dissimilarities, as a result of presence and absence, or differing abundances (Supplementary Material). The main species driving the dissimilarity of 48.54% between the two PGC groups (A and B) are as a result of differing abundances of T. debilis (10.73%), the presence of 7 other species in both groupings with differing abundances (46.54%) and the absence of three species (Adacnarca nitens, Ptychocardia vanhöffeni and Thracia meridionalis) from A (20.76%) and Waldo parasiticus from B (4.39%).
The differences between the two PGC groupings (A and B) with the rest of the Weddell Gyre groups were largely driven by the high abundances of Yoldiella valettei in the PGC, with low abundances elsewhere, and a complete absence from group F. Thyasira debilis was present in the PGC and deep Weddell Gyre and was seen in high abundance in group B. T. debilis was completely absent from groups C, E and F samples.
Differences in abundances of Yoldiella valettei were the main drivers for the difference between A (18.41%) and B (14.19%) with group E. The absence of seven species in group E present in group A and 8 found in group B also had a large influence on this dissimilarity (Supplementary Material). The difference between E and the PB shallow stations (group C) were also as a result of absence of 9 species from group E and of five species with differing abundances.
Discussion
Prince Gustav Channel Molluscan Biodiversity
Prior to the JR10073a expedition in 2018, the PGC had not been sampled for benthic fauna, despite being a region of recent dramatic environmental change (Linse, 2018). These samples represent our first insights into the communities that occupy an area over 20 years after it lost its ice shelf (Di Franco et al., 2020; Almond et al., 2021; Drennan et al., 2021; Pantó et al., 2021). The Mollusca are one of the best studied Antarctic benthic marine taxa with well-established taxonomy and biogeographic patterns (e.g., González-Wevar et al., 2019, 2021), making them an ideal group to investigate the biodiversity of the PGC. The expedition collected 6 epibenthic sledge samples from the PGC, representing 20,307 molluscan specimens from 50 species. Of the eight molluscan classes, which all occur in Antarctica, four were present within our samples (Solenogastres, Bivalvia, Gastropoda, and Scaphopoda).
Previous Molluscan biodiversity analyses in the Weddell Gyre and Southern Ocean characterised the typical species which are also present within the PGC samples (e.g., Dell, 1972, 1990; Hain, 1990; Troncoso et al., 2007; De Broyer et al., 2014; Schiaparelli et al.,, 2014). Comparing our material with Schiaparelli et al., (2014), across 18 Ross Sea stations, from similar depths, using a fine meshed Rauschert dredge, they collected 8,359 specimens from 161 species. Although Schiaparelli et al., (2014) sampled three times as many stations, they collected under half (∼40%) the number of specimens but two and a half times as many species as this study including representatives of monoplacophorans and polyplacophorans. These differences are likely a reflection of the regional differences in abundance (higher in the PGC) and diversity (higher in the Ross Sea) but may also be complicated by the higher sampling effort in the Ross Sea study (Gamito, 2010; Brandt et al., 2016).
At a community level, the PGC stations were unusually dominated by scaphopods, making up 56% of all individuals collected. In contrast, scaphopods made up just 0.9% of the total collected from the Ross Sea (Schiaparelli et al.,, 2014) and 8% in the Bellingshausen Sea (Troncoso et al., 2007). Cadulus thielei made up 54% (11,019 specimens) PGC molluscan abundance. In the original description of Cadulus thielei (Plate, 1909) reports only 5 specimens collected at 380 m (Plate, 1909) in the East Antarctic. Aldea and Troncoso (2008) reported just two specimens in 157 and 438 m depth from 2 stations of 47 stations sampled in the Bellingshausen Sea and Western Antarctic Peninsula. These few records suggested a rare species. Cadulus thielei has never previously been recorded below 438 m, but in the PGC they were found at all stations and in depths from 200 to 1,200 m. Given its reproductive mode (planktotrophic), and abundance within the PGC, C. thielei seems to be a good candidate as a colonising species and even a possible pioneer species.
In typical Antarctic shelf communities, the dominant group between gastropod and bivalve abundances usually favours the gastropods. 71% (5,965) of individuals collected by Schiaparelli et al., (2014) in the Ross Sea were gastropods, and 16% (1,323) were bivalves. In striking contrast, the PGC gastropods made up just 2% of the total abundance and bivalves 42%. There are approximately 600 gastropod (Schiaparelli and Linse, 2014) and 160 bivalve (Linse, 2014) species known from Antarctic waters. However, regionally the relative diversity of these groups can vary. In the PGC bivalve and gastropod species numbers were relatively even at 18 and 23 species, respectively, a difference of 5 species or 12% of the total. In contrast, in the Ross Sea, gastropods outnumbered the bivalves by 113 to 36 species, a difference of 77 species or 52% of the total (Schiaparelli et al.,, 2014). However, in the Bellingshausen Sea Troncoso et al. (2007) found 33 species of gastropods and 25 species of bivalves, a difference of 8 species or 14% of the total, making the PGC appear to be more similar in structure to the Bellingshausen Sea than the Ross Sea. Gastropod taxa accounted for 46% of total species richness in the PGC, compared with 53% in the Bellingshausen Sea (Troncoso et al., 2007) and 72% in the Ross Sea (Schiaparelli et al.,, 2014). Bivalve species made up 26% of the PGC richness, 40% of the Bellingshausen Sea and 22% of the Ross Sea bivalve diversity.
These regional differences in diversity and abundance imply that the PGC mollusc assemblage is different to both the Ross and Bellingshausen Seas and particularly depauperate when compared to the Ross Sea diversity. The unusually high abundance of scaphopods and the richness and abundance of bivalves relative to gastropods, suggests that there are some physical or environmental drivers causing these differences in the community compared to previously studied regions.
Our findings are comparable with those from Deception Island (Angulo-Preckler et al., 2018). Deception Island is the caldera of an active volcano, and although not recently impacted by ice shelf collapse, the recolonisation of Deception Island after the 1970 volcanic eruption may be analogous to events in the PGC as a consequence of the narrow entrance to the bay. Angulo-Preckler et al. (2018) suggest distance to the open sea was a factor favouring a gradient of community composition during recolonisation. Angulo-Preckler et al. (2018) found echinoderms dominated the epifauna, with oligochaetes, polychaetes and bivalves dominating the infauna. Although Deception Island is significantly smaller in area (11 km long by 7 km wide) than the PGC they have both had comparatively short amounts of time for their recolonisation.
The occurrence of mollusc singletons and doubletons in samples (species found as only one or two individuals) varies greatly across the Ross Sea, West Antarctica, and the PGC; in all cases doubletons were rarer than singletons (Schiaparelli et al., 2006; Schiaparelli et al.,, 2014; Aldea et al., 2009). Proportions of singletons ranged from ∼22% in the Ross Sea and Peter I Island up to 72.2% on the Eights Coast, with the new PGC samples being the lowest at 20% (Linse and Anderson, 2021). Percentages of species found twice ranged from 6.5% at the South Shetland Islands to 18.9% at Peter I Island, with the PGC samples fitting within that range at 12%. Southern Ocean gastropods are known to be more regionally endemic and less homogenous in their distribution than their bivalve counterparts (Griffiths et al., 2009). This is reflected in the numbers of singletons of both taxa in the PGC. Gastropods represented 67% of the total singleton and doubleton species (15). A higher proportion of gastropod species (42%) were either singletons or doubletons, contrasting with only 17% (3 species) of bivalves being singletons and no doubletons. The general trend in Antarctic benthic molluscs is that most species are rare, and few species are common (Clarke et al., 2007). This does not hold true for the PGC with 72% of bivalves and 42% of gastropods being from multiple stations and 69% of all gastropod and bivalve species having more than 2 individuals (up to a maximum of 6,725). The lower prevalence of singletons in the PGC samples is probably a reflection of its lower overall diversity but may also be linked to the limited number of sampling stations.
The developmental and motility functional traits are key for the mobility (and hence colonisation ability) of species. Lecithotrophic and planktotrophic (both with planktonic dispersal phases) species accounted for 98% of the developmental modes found in the PGC. The lower diversity of gastropods in the PGC may be explained by, despite being able to crawl as adults, their dominant developmental type is direct developing (with no planktonic dispersal phase). With reduced access to the PGC due to geographic location and dynamic ice history, most species are likely to be brought in by the currents. The remote position of the PGC, sheltered by James Ross Island and frequent sea-ice cover of the area, separates it from the main current flow of the Weddell Gyre. Little is known about the currents within the PGC but there is a net westward flow through the channel to the south, in the absence of oceanographic models the source of this is unknown (Camerlenghi et al., 2001). As a result of local topography water is accelerated through the Channel proper up to 40 cm s–1; a much greater velocity than the Weddell Gyre current (10 cm s–1).
The most abundant mollusc species found at the PGC stations, Cadulus thielei (11,019 specimens), Yoldiella valettei (6,725 specimens) and Thyasira debilis (1,167 specimens) have motile life stages, with C. thielei a planktotrophic species and Y. valettei and T. debilis lecithotrophic. The trait dominance of motile, planktonic dispersing species in the PGC, suggests recolonisation and the non-mobile, brooding species will be the last to colonise. The dominance of the planktotrophic and crawling Scaphopod fauna at all stations, apart from at DB-200 which is a lecithotrophic bivalve dominated community, suggesting some selection for having mobile life stages in the PGC.
The dominance of scaphopods (lecithotrophic and planktotrophic burrowing species) and increased depth range for some species (specifically C. thielei), suggests some form of ‘founder effect’ occurring (although for traits and life modes rather than genetics) (Joly, 2011; Waters et al., 2013). The founder effect is the loss of variation in the establishment of new populations, with the high abundance of few species, e.g., the scaphopods in the PGC, dominating the habitat and making it potentially difficult for newly arriving species to become established, especially less mobile taxa.
Weddell Gyre Molluscan Assemblages
The Weddell Sea, which includes a large shelf area, has been described as a species rich region for bivalves in the Southern Ocean (Linse et al., 2006; Linse, 2014) and a significant area for Antarctic molluscan evolution (Linse et al., 2006; Griffiths et al., 2009). The WG is a strong, clock-wise current systems in the Atlantic sector of the Southern Ocean and heavily influences the Weddell Sea and its geological, hydrographical and biological processes (Vernet et al., 2019). The area of the WG region investigated in this study spans approximately 2,000 km between the most distant stations analysed, which is a much larger geographic coverage than the PGC (∼90 km). Due to the large size, the WG has the potential for a much wider range of environmental conditions and habitats than the PGC, increasing its scope for habitat and species diversity (Starmans and Gutt, 2002; Linse, 2014). Large shelf areas such as in the Weddell and Ross seas (Schiaparelli et al., (2014) sampled stations up to 500 km apart) have been found to be richer in species than shelf-depth areas at smaller, more isolated islands, e.g., Peter I Island or Heard Island (Linse et al., 2006; Troncoso et al., 2007; Troncoso and Aldea, 2008; Linse, 2014). Given its geographic proximity and apparent oceanographic connectivity with the PGC, comparisons with the WG region can be used to understand the biogeographic status of the PGC.
Bivalve species richness typically decreases with depth in the Southern Ocean, below 1,200 m species richness is approximately half that compared to the shelf (Brandt et al., 2009). Although there was a decrease in species richness between the WG shallow (30 species at 6 stations and ES-32 5.32) and WG deep (24 species and ES-32 5.39) stations, this 50% drop in richness was not observed in these samples. This may be a result of not having sampled sufficient numbers of stations to represent the rarer or more patchy species (Figure 5). Regardless of the impact of depth on bivalve species richness in the Southern Ocean, the PGC had a much lower species richness (18 species and ES-32 3.81) than both the shallow and deep WG groups, confirming the PGC to be a species poor area. Although the shallow stations split off into groups based on geographical location, the majority of deep WG stations (>1,000 m) grouped significantly together (Figure 5). This strong grouping is a result of many species being absent from the deep stations that are present in the shallower groups, characterising the deep group as relatively species poor. The PGC groups (A and B) were characterised by the high abundances of a few species such as Yoldiella valettei. As a result, it is the combination of low species richness, and high abundance that characterise the PGC as different from the deep WG.
High abundances were characteristic of the PGC, making them different from the majority of the remaining WG groupings, apart from Group C (the two shallowest PB stations) which has almost as high abundances per station (3,519) as Group B (4,142) and much higher than Group A (1,096). Previous studies in the PB region have found depth to be a structuring factor (Galkin et al., 2021). As such the separation of the deep and shallow PB stations comes as no surprise. The high abundances (especially in Yoldiella valettei and Y. sabrina), geographical proximity, and shallow depth of the shallow water PB stations may explain the similarity with the PGC. Regional differences in Weddell Sea and Southern Ocean molluscs have previously been found (Linse et al., 2006; Ellingsen et al., 2007), as such it may be as a result of the large geographical distance between the PGC stations group A and B with the shallow west Weddell Gyre group (E) and Group F (with wWS-1000 station) that results in the high dissimilarity between groups.
Colonisation of the Prince Gustav Channel Area
The physical impact of ice on Antarctic benthic communities and their subsequent recovery varies with depth, topography and community type. Shallow-water, nearshore (∼10 m depth) benthic communities have been found to return to their previous states within 10 years (Zwerschke et al., 2021) of iceberg scouring. Other studies predicted that an iceberg scour on the Antarctic shelf can take up to 340 years to recolonise (Gutt et al., 1996; Gutt, 2001), without the added impediment of being in a geographically isolated area (Peck et al., 1999; Gutt and Starmans, 2002). These studies showed that the biggest influence on recovery was the recruitment driven by the proximity and composition of neighbouring communities. Given the limited access to the PGC via the narrow ends of the channel, it is possible that post-ice shelf collapse colonisation of the area may be slower and more selective than in less restricted shelf areas.
Ecosystems under ice shelves are assumed oligotrophic and hence impoverished in benthic life (Ingels et al., 2021), not only this but low species diversity is often a characteristic of an area of impoverishment or a consequence of rapidly changing environmental conditions, ice cover and/or food availability (Gage, 2004; Clarke, 2008). It is unlikely that the low molluscan biodiversity observed the PGC is due to limited food availability, especially given the high abundances found. As elsewhere in the Antarctic sea ice zone, the seasonal sea-ice in the PGC stabilises the water column, resulting in substantial phytoplankton blooms in spring (Nedwell et al., 1993; Clarke, 1996). The low overall diversity and high abundances of species that usually contribute a small proportion to established communities may be as a result of the process of sub-ice shelf benthos being replaced by more open water species (Gutt et al., 2011). This hypothesis seems to be supported by the high abundance of motile species with a motile life stage within the PGC.
The Southern Ocean is known for its unusually high number of brooders and direct developers within the benthic invertebrate diversity but also the ecological dominance of broadcasters in terms of abundance along nearshore areas (Poulin et al., 2002; Pearse et al., 2009). Species with planktonic life stages have been found to be highly abundant (Poulin et al., 2002) in disturbed regions of Antarctic coastal waters which would be under constant recolonisation. Given the PGC’s higher similarity with its neighbouring shallow water area along the edges of the Powell Basin, the origin of the recolonisation is likely from the nearby shallow water fauna. However, the tight clustering of the PGC stations suggests the colonisation is still far from complete, with many regionally common species still absent from the PGC and a dominance of species that normally occur in far lower numbers.
The expedition JR10073a was the first attempt at sampling the benthos of the PGC since the collapse of the PGIS. The conditions encountered included heavy and shifting sea ice, hampering the ability to target planned sampling locations and collect replicant samples. These first observations suggest that the biodiversity and community structure of the PGC is distinct from its neighbours, but with only 6 locations sampled to date and no replication, further detailed investigation is required.
Ice shelves cover around 30% of the Antarctic continental shelf, representing a major global habitat of over 1.5 million km2 (Ingels et al., 2018). Climate change is expected to decrease the stability of 14% to 18% of Antarctic ice shelves over the coming century under low to moderate warming scenarios (1.5°C and 2°C), and up to 34% of all ice shelves at 4°C (Gilbert and Kittel, 2021). As an area of ongoing colonisation following ice shelf collapse, the continued monitoring of the development of the PGC fauna would serve as an ideal case study to investigate the future of Antarctic benthic habitats currently covered by vulnerable ice shelves.
Data Availability Statement
The original contributions presented in the study are included in the article/Supplementary Material, further inquiries can be directed to the corresponding author.
Author Contributions
KL collected the samples in the Southern Ocean, counted and identified the PGC molluscan specimens, and provided the comparative data. MA analysed the data, prepared the figures, and wrote the manuscript with conceptual and analytical assistance from HG, KL, and PF. All authors have contributed to previous versions and approved the final, submitted manuscript.
Funding
KL and HG are part of the British Antarctic Survey Polar Science for Planet Earth Program by the Natural Environment Research Council (NERC) [NC-Science] and RSS James Clark Ross expedition JR17003a was funded by the NERC urgency grant NE/R012296/1.
Conflict of Interest
The authors declare that the research was conducted in the absence of any commercial or financial relationships that could be construed as a potential conflict of interest.
Publisher’s Note
All claims expressed in this article are solely those of the authors and do not necessarily represent those of their affiliated organizations, or those of the publisher, the editors and the reviewers. Any product that may be evaluated in this article, or claim that may be made by its manufacturer, is not guaranteed or endorsed by the publisher.
Acknowledgments
We are grateful to the captain and crew of RRS James Clark Ross expedition JR17003a for their support, especially for our epibenthic sledge deployments, during multiple expeditions. We thank Hilary Blagborough, Angelika Brandt, Davide di Franco, and various students for their help in sorting epibenthic sledge samples from JR17003a and the Weddell Gyre dataset.
Supplementary Material
The Supplementary Material for this article can be found online at: https://www.frontiersin.org/articles/10.3389/fmars.2021.771369/full#supplementary-material
References
Aldea, C., Olabarria, C., and Troncoso, J. S. (2009). Spatial patterns of benthic diversity in molluscs from West Antarctica. Antarct. Sci. 21, 341–353. doi: 10.1111/j.1365-294X.2012.05572.x
Aldea, C., and Troncoso, J. S. (2008). Systematics and distribution of shelled molluscs (Gastropoda, Bivalvia and Scaphopoda) from the South Shetland Islands to the Bellingshausen Sea, West Antarctica. Iberus 26, 43–117.
Almond, P. M., Linse, K., Dreutter, S., Grant, S. M., Griffiths, H. J., Whittle, R. J., et al. (2021). In-situ image analysis of habitat heterogeneity and benthic biodiversity in the prince gustav channel, Eastern Antarctic Peninsula. Front. Mar. Sci. 8:614496. doi: 10.3389/fmars.2021.614496
Amsler, M. O., Huang, Y. M., Engl, W., McClintock, J. B., and Amsler, C. D. (2015). Abundance and diversity of gastropods associated with dominant subtidal macroalgae from the western Antarctic Peninsula. Polar Biol. 38, 1171–1181. doi: 10.1007/s00300-015-1681-4
Angulo-Preckler, C., Figuerola, B., Núñez-Pons, L., Moles, J., Martín-Martín, R., Rull-Lluch, J., et al. (2018). Macrobenthic patterns at the shallow marine waters in the caldera of the active volcano of Deception Island, Antarctica. Cont. Shelf Res. 157, 20–31. doi: 10.1016/j.csr.2018.02.005
Arnaud, P. M., Troncoso, J. S., and Ramos, A. (2001). Species diversity and assemblages of macrobenthic Mollusca from the South Shetland Islands and Bransfield Strait (Antarctica). Polar Biol. 24, 105–112. doi: 10.1007/s003000000183
Arrigo, K. R., Van Dijken, G. L., and Strong, A. L. (2015). Environmental controls of marine productivity hot spots around Antarctica. J. Geophys. Res. 120, 5545–5565. doi: 10.1002/2015jc010888
Brandt, A., Linse, K., Ellingsen, K. E., and Somerfield, P. J. (2016). Depth-related gradients in community structure and relatedness of bivalves and isopods in the Southern Ocean. Prog. Oceanogr. 144, 25–38.
Brandt, A., Linse, K., and Schuller, M. (2009). Bathymetric distribution patterns of Southern ocean macrofaunal taxa: Bivalvia, Gastropoda, Isopoda and Polychaeta. Deep Sea Res. 1 Oceanogr. Res. Pap. 56, 2013–2025. doi: 10.1016/j.dsr.2009.06.007
Bremner, J., Rogers, S., and Frid, C. (2006). Methods for describing ecological functioning of marine benthic assemblages using biological traits analysis (BTA). Ecol. Indic. 6, 609–622. doi: 10.1016/j.ecolind.2005.08.026
Brenke, N. (2005). An epibenthic sledge for operations on marine soft bottom and bedrock. Mar. Technol. Soc. J. 39, 10–21. doi: 10.4031/002533205787444015
Camerlenghi, A., Domack, E., Rebesco, M., Gilbert, R., Ishman, S., Leventer, A., et al. (2001). Glacial morphology and post-glacial contourites in northern PrinceGustav Channel (NW Weddell Sea, Antarctica). Mar. Geophys. Res. 22, 417–443.
Chevene, F., Doledeac, S., and Chessel, D. (1994). A fuzzy coding approach for the analysis of long-term ecological data. Freshw. Biol. 31, 295–309. doi: 10.1111/j.1365-2427.1994.tb01742.x
Clarke, A. (1996). “Benthic marine habitats in Antarctica,” in Foundations for Ecological Research West of the Antarctic Peninsula, eds R. M. Oss, E. E. Hofmann, and L. B. Quetin (Washington, D.C: American Geophysical Union), 123–133.
Clarke, A. (2008). Antarctic marine benthic diversity: patterns and processes. J. Exp. Mar. Biol. Ecol. 366, 48–55. doi: 10.1016/j.jembe.2008.07.008
Clarke, A., and Crame, J. A. (2010). Evolutionary dynamics at high latitudes: speciation and extinction in polar merine faunas. Philos. Trans. R. Soc. B 365, 3655–3666. doi: 10.1098/rstb.2010.0270
Clarke, A., Griffiths, H. J., Linse, K., Barnes, D. K., and Crame, J. A. (2007). How well do we know the Antarctic marine fauna? A preliminary study of macroecological and biogeographical patterns in Southern Ocean gastropod and bivalve molluscs. Div. Distrib. 13, 620–632. doi: 10.1111/j.1472-4642.2007.00380.x
Clarke, A., and Johnston, N. M. (2003). Antarctic marine benthic diversity. Oceanogr. Mar. Biol. 41, 47–114.
Cook, A. J., and Vaughan, D. G. (2010). Overview of areal changes of the ice shelves on the Antarctic Peninsula over the past 50 years. Cryosphere 4, 77–98. doi: 10.5194/tc-4-77-2010
De Broyer, C., and Danis, B. (2011). How many species in the Southern Ocean? Towards a dynamic inventory of the Antarctic marine species. Deep Sea Res. 2 Top. Stud. Oceanogr. 58, 5–17. doi: 10.1016/j.dsr2.2010.10.007
De Broyer, C., Koubbi, P., Griffiths, H. J., Raymond, B., Udekem D’Acoz, C. D., Van De Putte, A. P., et al. (eds) (2014). Biogeographic Atlas of the Southern Ocean. Cambridge: Scientifc Committee on Antarctic Research.
Dell, R. K. (1990). Antarctic Mollusca. With special reference to the fauna of the Ross Sea. Wellington: Royal Society of New Zealand.
Di Franco, D., Linse, K., Griffiths, H. J., and Brandt, A. (2021). Drivers of abundance and spatial distribution in Southern Ocean peracarid crustacea. Ecol. Indic. 128:107832. doi: 10.1016/j.ecolind.2021.107832
Di Franco, D., Linse, K., Griffiths, H. J., Haas, C., Saeedi, H., and Brandt, A. (2020). Abundance and distributional patterns of benthic peracarid crustaceans from the Atlantic Sector of the Southern Ocean and Weddell Sea. Front. Mar. Sci 7:554663. doi: 10.3389/fmars.2020.554663
Drennan, R., Dahlgren, T. G., Linse, K., and Glover, A. G. (2021). Annelid fauna of the prince gustav channel, a previously ice-covered seaway on the Northeastern Antarctic Peninsula. Front. Mar. Sci. 7:595303. doi: 10.3389/fmars.2020.595303
Ellingsen, K. E., Brandt, A., Ebbe, B., and Linse, K. (2007). Diversity and species distribution of polychaetes, isopods and bivalves in the Atlantic sector of the deep Southern Ocean. Polar Biol. 30, 1265–1273. doi: 10.1007/s00300-007-0287-x
Ferrigno, J. G. (2006). Coastal-Change and Glaciological Map of the Trinity Peninsula Area and South Shetland Islands, Antarctica, 1843-2001. Washington, DC: US Department of the Interior.
Gage, J. D. (2004). Diversity in deep-sea benthic macrofauna: the importance of local ecology, the larger scale, history and the Antarctic. Deep Sea Res. 2 Top. Stud. Oceanogr. 51, 1689–1708. doi: 10.1016/j.dsr2.2004.07.013
Galkin, S. V., Minin, K. V., Udalov, A. A., Chikina, M. V., Frey, D. I., Molodtsova, T. N., et al. (2021). Benthic assemblages of the powell basin. Oceanology 61, 204–219. doi: 10.1134/s0001437021020053
Gamito, S. (2010). Caution is needed when applying margalef diversity index. Ecol. Indic. 10, 550–551. doi: 10.1016/j.ecolind.2009.07.006
Garnier, S., Ross, N., Rudis, R., Camargo, A. P., Sciani, M., and Scherer, C. (2021). Rvision – Colorblind-Friendly Color Maps for R. R Package Version 0.6.2.
Gilbert, E., and Kittel, C. (2021). Surface melt and runoff on antarctic ice shelves at 1.5°c, 2°c, and 4°c of future warming. Geophys. Res. Lett. 48:e2020GL091733.
González-Wevar, C. A., Gérard, K., Rosenfeld, S., Saucède, T., Naretto, J., Díaz, A., et al. (2019). Cryptic speciation in Southern Ocean Aequiyoldia eightsii (Jay, 1839): miopliocene trans-drake passage separation and diversification. Prog. Oceanogr. 174, 44–54. doi: 10.1016/j.pocean.2018.09.004
González-Wevar, C. A., Segovia, N. I., Rosenfeld, S., Noll, D., Maturana, C. S., Hüne, M., et al. (2021). Contrasting biogeographical patterns in Margarella (Gastropoda: Calliostomatidae: Margarellinae) across the Antarctic Polar Front. Mol. Phylogenet. Evol. 156:107039. doi: 10.1016/j.ympev.2020.107039
Griffiths, H. J. (2010). Antarctic marine biodiversity – what do we know about the distribution of life in the Southern Ocean? PLoS One 5:e11683. doi: 10.1371/journal.pone.0011683
Griffiths, H. J., Barnes, D. K. A., and Linse, K. (2009). Towards a generalized biogeography of the Southern Ocean benthos. J. Biogeogr. 36, 162–177. doi: 10.1111/j.1365-2699.2008.01979.x
Gutt, J. (2001). On the direct impact of ice on marine benthic communities, a review. Polar Biol. 24, 553–564. doi: 10.1007/s003000100262
Gutt, J., Barratt, I., Domack, E., D’udekem D’acoz, C., Dimmler, W., Grémare, A., et al. (2011). Biodiversity change after climate-induced ice-shelf collapse in the Antarctic. Deep Sea Res. 2 Top. Stud. Oceanogr. 58, 74–83.
Gutt, J., and Starmans, A. (2002). “Quantification of iceberg impact and benthic recolonisation patterns in the Weddell Sea (Antarctica),” in Proceedings of the Ecological Studies in the Antarctic Sea Ice Zone, (Berlin: Springer), 210–214.
Gutt, J., Starmans, A., and Dieckmann, G. (1996). Impact of iceberg scouring on polar benthic habitats. Mar. Ecol. Prog. Series 137, 311–316. doi: 10.3354/meps137311
Hain, S. (1990). Die Beschalten Benthischen Mollusken (Gastropoda und Bivalvia) des Weddellmeeres, Antarktis= The Benthic Seashells (Gastropoda and Bivalvia) of the Weddell Sea, Antarctica. Berichte zur Polarforschung. Ph. D. thesis. Vasco da Gama: Polar Research, 70.
Hain, S., and Arnaud, P. (1992). Notes on the reproduction of high-Antarctic molluscs from the Weddell Sea. Polar Biol. 12, 303–312. doi: 10.1007/978-3-642-77595-6_35
Hogg, O. T., Huvenne, V. A. I, Griffiths, H. J., and Linse, K. (2018). On the ecological relevance of landscape mapping and its application in the spatial planning of very large marine protected areas. Sci. Total Environ. 626, 384–398. doi: 10.1016/j.scitotenv.2018.01.009
Ingels, J., Aronson, R. B., and Smith, C. R. (2018). The scientific response to Antarctic ice-shelf loss. Nat. Clim Chang. 8, 848–851. doi: 10.1038/s41558-018-0290-y
Ingels, J., Aronson, R. B., Smith, C. R., Baco, A., Bik, H. M., Blake, J. A., et al. (2021). Antarctic ecosystem responses following ice-shelf collapse and iceberg calving: science review and future research. Wiley Interdiscip. Rev. Clim. Change 12:e682.
Joly, E. (2011). The existence of species rests on a metastable equilibrium between inbreeding and outbreeding. An essay on the close relationship between speciation, inbreeding and recessive mutations. Biol. Direct 6:62. doi: 10.1186/1745-6150-6-62
Linse, K. (2014). “Chapter 5.11. bivalvia,” in Biogeographic Atlas of the Southern Ocean, eds C. De Broyer, P. Koubbi, H. J. Griffiths, B. Raymond, and C. D. Udekem D’acoz (Cambridge: Scientific Committee on Antarctic Research), 126–128.
Linse, K. (2018). RRS James Clark Ross JR17003A Cruise Summary Report. Liverpool: British Oceanographic Data Centre.
Linse, K., and Anderson, M. (2021). Macrobenthic Mollusca from Prince Gustav Channel and Duse Bay, Eastern Antarctic Peninsula collected by epibenthic sledge in March 2018. Vasco da Gama: Polar Data Centre.
Linse, K., Griffiths, H. J., Barnes, D. K. A., and Clarke, A. (2006). Biodiversity and biogeography of Antarctic and sub-Antarctic mollusca. Deep Sea Res. 2 Top. Stud. Oceanogr. 53, 985–1008. doi: 10.1016/j.dsr2.2006.05.003
Marchant, J. (2017). Giant iceberg’s split exposes hidden ecosystem. Nat. News 549:443. doi: 10.1038/549443a
Moles, J., Avila, C., and Malaquias, M. A. E. (2019). Unmasking Antarcic mollusc linaeges: novel evidence from philinoid snails (Gastropod: Cephalaspidea). Cladistics 35, 487–513. doi: 10.1111/cla.12364
Nedwell, D., Walker, T., Ellis-Evans, J., and Clarke, A. (1993). Measurements of seasonal rates and annual budgets of organic carbon fluxes in an Antarctic coastal environment at Signy Island, South Orkney Islands, suggest a broad balance between production and decomposition. Appl. Environ. Microbiol. 59, 3989–3995. doi: 10.1128/aem.59.12.3989-3995.1993
Pantó, G., Pasotti, F., Macheriotou, L., and Vanreusel, A. (2021). Combining traditional taxonomy and metabarcoding: assemblage structure of nematodes in the shelf sediments of the Eastern Antarctic Peninsula. Front. Mar. Sci 8:629706. doi: 10.3389/fmars.2021.629706
Pearse, J. S., Mooi, R., Lockhart, S. J., and Brandt, A. (2009). “Brooding and species diversity in the Southern Ocean: selection for brooders or speciation within brooding clades?,” in Smithsonian at the Poles: Contributions to International Polar Year Science, eds I. Krupnik, M. A. Lang, and S. E. Miller (Washington, D.C: Smithsonian Institution Scholarly Press), 181–196.
Peck, L., Brockington, S., Vanhove, S., and Beghyn, M. (1999). Community recovery following catastrophic iceberg impacts in a soft-sediment shallow-water site at Signy Island, Antarctica. Mar. Ecol. Prog. Ser. 186, 1–8.
Peck, L. S. (2018). Antarctic marine biodiversity: adaptations, environments and responses to change. OMBAR 56, 105–236. doi: 10.1201/9780429454455-3
Plate, L. H. (1909). “Deutsche südpolar expedition 1901-1903,” in Deutsche Südpolar-Expedition, 1901-1903, im Auftrage des Reichsamtes des Innern, ed. E. V. Drygalski (Berlin: G. Reimer), 1–6.
Poulin, E., Plama, A. T., and Féral, J.-P. (2002). Evolutionary versus ecological success in Antarctic benthic invertebrates. Trends Ecol. Evol. 17, 218–222. doi: 10.1016/s0169-5347(02)02493-x
R Core Team (2020). R: A Language and Environment for Statistical Computing. R Foundation for Statistical Computing. Vienna: R Core Team.
Rack, W., and Rott, H. (2004). Pattern of retreat and disintegration of the Larsen B ice shelf, Antarctic Peninsula. Ann. Glaciol. 39, 505–510. doi: 10.3189/172756404781814005
Roberts, S. J., Hodgson, D. A., Sterken, M., Whitehouse, P. L., Verleyen, E., Vyverman, W., et al. (2011). Geological constraints on glacio-isostatic adjustment models of relative sea-level change during deglaciation of Prince Gustav Channel, Antarctic Peninsula. Q. Sci. Rev. 30, 3603–3617. doi: 10.1016/j.quascirev.2011.09.009
Rosenfeld, S., Aldea, C., Ojeda, J., Marambio, J., Hüne, M., Troncoso, J., et al. (2017). Molluscan assemblages associated with Gigartina beds in the Strait of Magellan and the South Shetland Islands (Antarctica): a comparison of composition and abundance. Polar Res. 36:1297915. doi: 10.1080/17518369.2017.1297915
Rott, H., Skvarca, P., and Nagler, T. (1996). Rapid Collapse of Northern Larsen Ice Shelf, Antarctica. Science 271, 788–792.
Schiaparelli, S., Ghiglione, C., Alvaro, M. C., Griffiths, H. J., and Linse, K. (2014). Diversity, abundance and composition in macrofaunal molluscs from the Ross Sea (Antarctica): results of fine-mesh sampling along a latitudinal gradient. Polar Biol. 37, 859–877. doi: 10.1007/s00300-014-1487-9
Schiaparelli, S., and Linse, K. (2014). “Chapter 5.10. gastropoda,” in Biogeographic Atlas of the Southern Ocean, eds C. De Broyer, P. Koubbi, H. J. Griffiths, B. Raymond, and C. D. Udekem D’acoz (Cambridge: Scientific Committee on Antarctic Research), 122–125.
Schiaparelli, S., Lörz, A.-N., and Cattaneo-Vietti, R. (2006). Diversity and distribution of mollusc assemblages on the Victoria Land coast and the Balleny Islands, Ross Sea, Antarctica. Antarct. Sci. 18, 615–631. doi: 10.1017/s0954102006000654
Skvarca, P., Rack, W., Rott, H., and Donángelo, T. I. Y. (1998). Evidence of recent climatic warming on the eastern Antarctic Peninsula. Anna. Glaciol. 27, 628–632. doi: 10.1126/science.1069270
Smith, W. O., and Comiso, J. C. (2008). Influence of sea ice on primary production in the Southern Ocean: a satellite perspective. J. Geophys. Res. 113: C05S93.
Starmans, A., and Gutt, J. (2002). Mega-epibenthic diversity: a polar comparison. Mar. Ecol. Prog. Ser. 225, 45–52.
Sterken, M., Roberts, S. J., Hodgson, D. A., Vyverman, W., Balbo, A. L., Sabbe, K., et al. (2012). Holocene glacial and climate history of Prince Gustav Channel, northeastern Antarctic Peninsula. Q. Sci. Rev. 31, 93–111. doi: 10.1016/j.quascirev.2011.10.017
Troncoso, J. S., and Aldea, C. (2008). Macrobenthic mollusc assemblages and diversity in the West Antarctica from the South Shetland Islands to the Bellingshausen Sea. Polar Biol. 31, 1253–1265.
Troncoso, J. S., Aldea, C., Arnaud, P., Ramos, A., and García, F. (2007). Quantitative analysis of soft-bottom molluscs in the Bellingshausen Sea and around Peter I Island. Polar Res. 26, 126–134. doi: 10.1111/j.1751-8369.2007.00033.x
Turner, J., Colwell, S. R., Marshall, G. J., Lachlan-Cope, T. A., Carleton, A. M., Jones, P. D., et al. (2005). Antarctic climate change during the last 50 years. Int. J. Climatol. 25, 279–294. doi: 10.1002/joc.1130
Vaughan, D. G., Marshall, G. J., Connolley, W. M., Parkinson, C., Mulvaney, R., Hodgson, D. A., et al. (2003). Recent rapid regional climate warming on the Antarctic Peninsula. Clim. Change 60, 243–274.
Vernet, M., Geibert, W., Hoppema, M., Brown, P. J., Haas, C., Hellmer, H. H., et al. (2019). The Weddell gyre, southern ocean: present knowledge and future challenges. Rev. Geophys. 57, 623–708. doi: 10.1029/2018rg000604
Waters, J. M., Fraser, C. I., and Hewitt, G. M. (2013). Founder takes all: density-dependent processes structure biodiversity. Trends Ecol. Evol. 28, 78–85. doi: 10.1016/j.tree.2012.08.024
Whittle, R. J., Witts, J. D., Bowman, V. C., Crame, J. A., Francis, J. E., and Ineson, J. (2019). Nature and timing of biotic recovery in Antarctic benthic marine ecosystems following the cretaceous-paleogene (K-Pg) mass extinction. Palaeontology 62, 919–934. doi: 10.1111/pala.12434
WoRMS Editorial Board (2021). World Register of Marine Species (WoRMS) [Online]. Available online at: http://www.marinespecies.org at VLIZ (accessed August 5, 2021).
Keywords: assemblage structure, Bivalvia, Scaphopoda, biodiversity, Southern Ocean, Weddell Sea
Citation: Anderson MPBC, Fenberg PB, Griffiths HJ and Linse K (2021) Macrobenthic Mollusca of the Prince Gustav Channel, Eastern Antarctic Peninsula: An Area Undergoing Colonisation. Front. Mar. Sci. 8:771369. doi: 10.3389/fmars.2021.771369
Received: 06 September 2021; Accepted: 19 November 2021;
Published: 08 December 2021.
Edited by:
Alberto Basset, University of Salento, ItalyReviewed by:
Alejandro H. Buschmann, University of Los Lagos, ChileSebastian Rosenfeld, University of Magallanes, Chile
Copyright © 2021 Anderson, Fenberg, Griffiths and Linse. This is an open-access article distributed under the terms of the Creative Commons Attribution License (CC BY). The use, distribution or reproduction in other forums is permitted, provided the original author(s) and the copyright owner(s) are credited and that the original publication in this journal is cited, in accordance with accepted academic practice. No use, distribution or reproduction is permitted which does not comply with these terms.
*Correspondence: Madeline P. B. C. Anderson, bWE4ZzE3QHNvdG9uLmFjLnVr