- 1Department of Dermatology, Heidelberg University Hospital, Heidelberg, Germany
- 2Department of Dermatology, Affiliated Hospital of Guizhou Medical University, Guiyang, China
- 3Department of Anesthesiology and Intensive Care, University Hospital Essen, Essen, Germany
- 4Department of Anesthesiology, Heidelberg University Hospital, Heidelberg, Germany
- 5Department of Nephrology, Heidelberg University Hospital, Heidelberg, Germany
Sepsis is a severe life-threatening syndrome caused by dysregulated host responses to infection. Biomarkers that allow for monitoring the patient’s immune status are needed. Recently, a flow cytometry-based detection of in vivo inflammasome activation by formation of cytoplasmic aggregates of ASC (apoptosis-associated speck-like protein containing a caspase recruitment domain) has been proposed. Here we report on the frequency of ASC-speck+ leukocytes correlating with the survival of sepsis. 25 patients with sepsis were sampled consecutively for 7 days. Blood, serum samples and patient data were collected according to the guidelines of the PredARRT-Sep-Trial. Flow cytometric analysis was performed on fresh whole blood samples to investigate the formation of ASC-specks in leukocyte subsets. Serum samples were analyzed for production of IL-1ß, IL-18 and additional inflammatory markers. ASC-speck formation was found to be increased in leukocytes from sepsis patients compared to healthy donor controls. The absolute number of ASC-speck+ neutrophils peaked on day 1. For monocytes, the highest percentage and maximum absolute number of ASC-speck+ cells were detected on day 6 and day 7. Inflammatory cytokines were elevated on day 1 and declined thereafter, with exception of IL-18. Survival analysis showed that patients with lower absolute numbers of ASC-speck+ monocytes (<1,650 cells/ml) on day 6 had a lower probability to survive, with a hazard ratio (HR) of 10.178. Thus, the frequency of ASC-speck+ monocytes on day 6 after onset of sepsis may serve to identify patients at risk of death from sepsis.
Introduction
Sepsis remains a major cause of mortality in intensive care units (ICU) worldwide (1–3). The dysregulated host responses in sepsis allows for excessive inflammation and immunosuppression (4). A hyper-inflammatory response predominates at the early stage, anti-inflammatory mechanisms manifest concomitantly, leading to long-term immune suppression. Owing to advances in care, most patients with sepsis survive the initial phase. However, if sepsis persists, patients enter a protracted hypo-inflammatory phase with significant immunosuppression (5–9) and the majority of non-surviving patients die in this phase (9–11). Failure in controlling the primary infection or the acquisition of secondary infections, caused by opportunistic bacteria and fungi, are the major cause of death during this period (10, 12). The risk of late-deaths from sepsis appears to be directly related to the immunosuppressive state of leukocytes (13, 14).
Monitoring the immune status of sepsis patients and the application of biomarker-guided interventions are needed for designing future therapeutic interventions allowing protection of these patients from late death (13). Biomarkers identifying patients at risk are largely missing (15). Inflammasome activation is of central importance for immune defense against infection and is a key component of sepsis pathophysiology (16–21). Inflammasomes are large protein complexes formed on encounter of microbial or damage associated stimuli (22). They regulate at least two host responses during sepsis: maturation and secretion of the pro-inflammatory cytokines interleukin-1β (IL-1β) as well as IL-18 and induction of pyroptosis, a rapid lytic form of programmed cell death (23–25). Central to the function of most inflammasome structures is the adapter molecule apoptosis-associated speck-like protein containing a caspase-recruitment domain (ASC). When inflammasomes are activated and assembled, ASC relocalizes from its diffuse cytoplasmatic distribution at steady state into a single speck, serving as a supramolecular signaling platform. This is followed by caspase-1 activation, thereby, cleaving the pro-peptide to generate mature and bioactive IL-1β and IL-18. The aberrant activation of inflammasomes has been reported in several autoimmune and chronic inflammatory diseases, such as cryopyrin-associated periodic syndrome (CAPS), familial mediterranean fever (FMF), gout and asthma. However, little work has been devoted to the role of inflammasomes in acute inflammatory diseases. Lee et al. reported that downregulation of nucleotide-binding and oligomerization domain-like receptor (NLR) family pyrin domain (PYD)containing 3 (NLRP3) inflammasome activation leads to increased survival in a polymicrobial sepsis mouse model (17). Another study found that NLRP3 inflammasome knockout mice were more susceptible to S. schenckii infection than wild-type mice, suggesting that inflammasomes contribute to host protection as well (26). Caspase-1 was shown to predict the outcome of sepsis (27–29), in that high caspase-1 activation during the first day of sepsis correlated with poor sepsis outcome (29). However, little work has been devoted to the role of ASC, the upstream process of caspase-1, in sepsis. The in vivo ASC-speck formation within blood leukocytes is a distinguishing feature of inflammasome formation and permits a direct and quantitative study of blood samples by flow cytometry. This assay was well validated (30–32) and has been implemented previously (33, 34).
Given the potential relevance of in vivo ASC-speck formation as a biomarker of systemic immune activation, we studied ASC-speck formation in leukocytes of sepsis patients during the first week after onset. Here, we report on a flow cytometry-based detection of ASC-specks in monocytes serving as a potential biomarker of patients at risk of death from sepsis.
Methods
Sample Collection
We report data of 141 samples from 25 patients with sepsis or septic shock, representing a subgroup of patients participating in the PredARRT-Sep-Trial (DRKS-ID: DRKS00012446) (35), hospitalized in the surgical ICU of Heidelberg University Hospital from September 2017 to February 2018. Some factors resulted in missing samples, which were, death of the patient, surgery during the time period of sampling, technical errors, vascular conditions not allowing to draw blood and transfer to another hospital unit (Supplementary Figure 1). Demographics and clinical characteristics of enrolled patients are shown in Supplementary Table 1. The study has been approved by the local Ethics Committee (file number: S-200/2017) according to the latest Declaration of Helsinki. All patients or their legal designees gave written informed consent before sample collection. The criteria for inclusion were based on the Sepsis-3 definition and clinical criteria (36). 19 samples of healthy controls were obtained from volunteers (file number: S-305/2010) without immunosuppression, autoimmune or infectious diseases. Upon the onset of sepsis, heparinized blood and serum tubes were drawn once daily for seven days. Within blood samples, leukocytes were stained directly and analyzed by flow cytometry. Serum sample were stored at -80°C for further investigation.
Patient Data Collection
Parallel to collecting blood samples to investigate ASC-speck formation, clinical and laboratory data were recorded. Disease severity was determined using acute physiology and chronic health evaluation II (APACHE II), sequential organ failure assessment (SOFA) and simplified acute physiology score II (SAPS II) scores. The white blood cell count (WBC) and the concentration of c-reactive protein (CRP), lactate dehydrogenase (LDH) and procalcitonin (PCT) were also measured. Patient survival was followed up for 90 days.
Determination of Intracellular ASC-Specks
Intracellular ASC-speck formation in vivo was determined by flow cytometry on fresh whole blood samples according to previous studies (30–32). The gating strategy is shown in Supplementary Figure 2. The following antibodies were used: CD3-PerCP, CD19-PerCP, CD56-PerCP, CD66b-PerCP, ASC-PE, and HLA-DR PE-Cy7 are from BioLegend (San Diego, CA); CD14 APC-Cy7 and CD16 Krome Orange are from BD Bioscience (Heidelberg, Germany) and Beckman Coulter (Krefeld, Germany), respectively. Samples of sepsis patients and healthy controls were processed in exactly the same way. In brief, 300 µl of fresh whole blood was incubated with the antibodies (CD3, CD19, CD56, CD66b, HLA-DR, CD14, and CD16) for 15 min at room temperature (RT) in the dark. Erythrocytes were lysed using FACS lysing solution (BD Biosciences) and leukocytes were fixed simultaneously. Cells were washed and permeabilized using 0.1% saponin followed by incubation with anti-ASC monoclonal antibody for 40 min at 4°C in the dark. After staining, cells were washed and flow cytometry was performed on a Gallios Flow Cytometer (Beckman Coulter). Counting beads (Molecular Probes Invitrogen, Paisley, UK) were added into each sample before acquisition to study absolute numbers of different leukocytes subsets. Flowjo software (Version 10.0.7 for windows) was used for flow cytometric analysis.
Serum Cytokines and Chemokine Levels
Serum samples of patients on day 1, day 3, day 5, day 6, and day 7 were investigated. Levels of different cytokines including IL-1β, IL-18, IL-6, tumor necrosis factor-α (TNF-α), monocyte chemoattractant protein-1 (MCP-1), interferon-γ (IFN-γ), IL-12p70, IL-8, IL-10, thymic stromal lymphopoietin (TSLP), IL-1α, granulocyte macrophage colony stimulating factor (GM-CSF), IFN-α2, IL-23, IL-12p40, IL-15, IL-11, IL-27, IL-33, and IL-17A were determined using the legendplex multi-analyte flow assay kit human inflammation panel and human cytokine panel 2 (BioLegend). Assays were performed according to the manufacturer’s instructions.
Statistical Analysis
Statistical analysis was undertaken using legendplex data analysis software (version 8.0), prism (version 5.0, graph pad software, La Jolla, CA, USA) and SPSS (version 21.0; SPSS Inc., Chicago, IL, USA). Comparisons of demographics and clinical characteristics between different groups were assessed using independent samples t tests, Mann-Whitney U tests, chi-square tests, or Fisher’s exact tests, as appropriate. Dot plots and bar-graph data are represented as median or mean ± standard error of the mean (SEM). The difference of ASC-speck+ cells between healthy controls and patients was determined by Mann-Whitney U test. Multiple comparisons among different time points of patient samples were analyzed by Kruskal–Wallis test. Correlations were assessed by Pearson coefficient. Receiver operating characteristic (ROC) curve analysis and the area under the ROC curve (AUROC) was applied to identify the optimal cutoff value. Kaplan-Meier analysis was used to calculate overall survival and the log-rank test was performed to assess the differences between survival curves. In univariate analysis, variables associated with mortality were assessed using the log-rank test. The predictors with a p value of <0.05 from the univariate analyses were included in multivariate analysis using a Cox proportional hazards model. Independent predictors of mortality were identified in the multivariate analysis and hazard ratios were computed for significant risk factors. For complete analysis, a two-tailed p value of less than 0.05 was considered as statistically significant.
Results
ASC-Speck Formation Among Blood Leukocytes of Sepsis Patients
After inflammasome activation, ASC assembles to a protein complex, termed “speck”. The intracytoplasmic redistribution of ASC from a diffuse state into a single speck can be detected by a decreased width of the pulse of emitted fluorescence after staining with ASC-specific antibodies. Initially, we validated this flow cytometric assay (30–32) by using peripheral blood mononuclear cells (PBMCs) stimulated with lipopolysaccharide (LPS) and/or adenosine triphosphate (ATP) (Supplementary Figure 2A and Figure 1A). As expected, following cell stimulation with LPS and ATP, we observed a marked increase of inflammasome formation in monocytes. When studying patient samples and healthy controls (Supplementary Figures 2B, C and Figure 1B) we detected a far higher frequency of ASC-speck formation in monocytes and neutrophils in a representative sepsis patient. Further analysis showed that most of the ASC-speck+ monocytes belong to the population of CD14++CD16- classical monocytes (Supplementary Figure 3). The flow cytometric gating strategy and respective isotype controls are shown in Supplementary Figure 2 and the patient characteristics are provided in Supplementary Table 1.
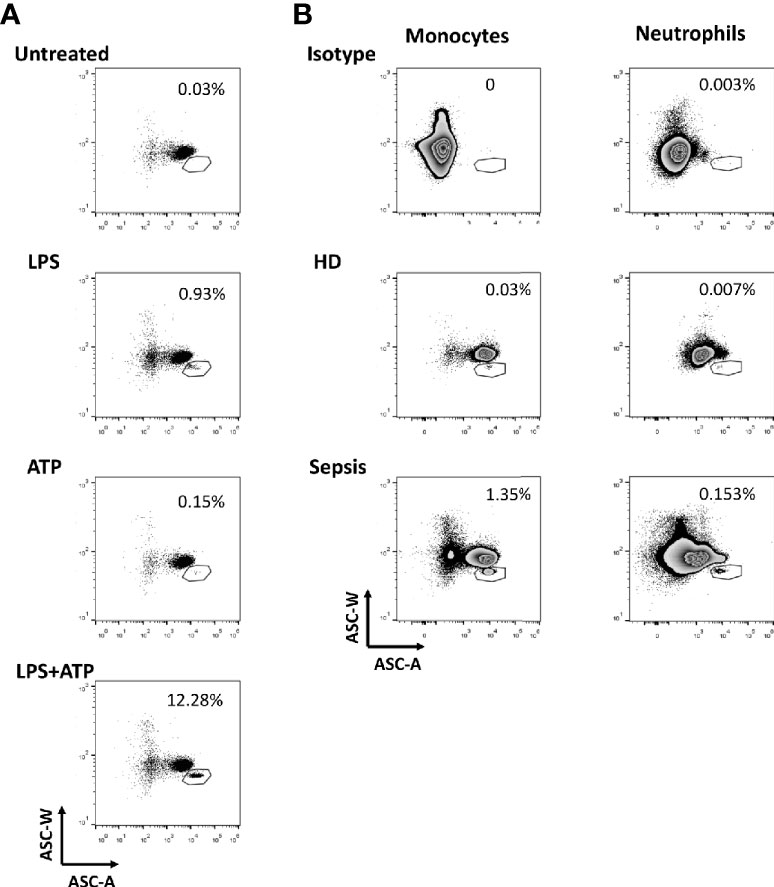
Figure 1 Formation of ASC-speck among leukocytes. (A) Detection of ASC-speck formation in peripheral blood mononuclear cells (PBMCs) of healthy donors after stimulation. Displayed are gated monocytes from gradient purified PBMCs (gating strategy is shown in Supplementary Figure 2A) cultured under different conditions including stimulation with lipopolysaccharides (LPS), with adenosine triphosphate (ATP) and with LPS + ATP. Gates were placed to include cells with a relatively low ASC-W: ASC-A profile (low W:A) that represent ASC-speck+ cells. Numbers shown next to the gates are the percentages of the ASC-speck+ cells from the HLA-DR+ monocyte population. Here, we confirmed that this flow cytometry assay allows the quantification of ASC speck-positive cells in samples. (B) Detection of ASC-speck formation in whole blood from sepsis patients and healthy donors. Displayed are gated monocytes and neutrophils from whole blood (gating strategy is shown in Supplementary Figure 2B). ASC-speck+ cells were defined by relatively low W:A in monocytes and neutrophils. Numbers shown next to the gates are the percentages of the ASC-speck+ cells from the HLA-DR+ monocyte and neutrophil population.
ASC-Speck+ Neutrophils and Monocytes Are Increased at Different Time Points in Sepsis Patients
During the first week of sepsis we observed dynamic changes in the frequency of ASC-speck+ leukocytes in sepsis patients. Both the absolute number (Figure 2A) and percentage (Figure 2B) of ASC-speck+ monocytes were found increased on day 6 and day 7 compared to healthy donors. An increased absolute number of ASC-speck+ neutrophils was only observed on day 1 (Figure 2C). Over time we did not observe significant changes in the percentage of ASC-speck+ neutrophils (Figure 2D).
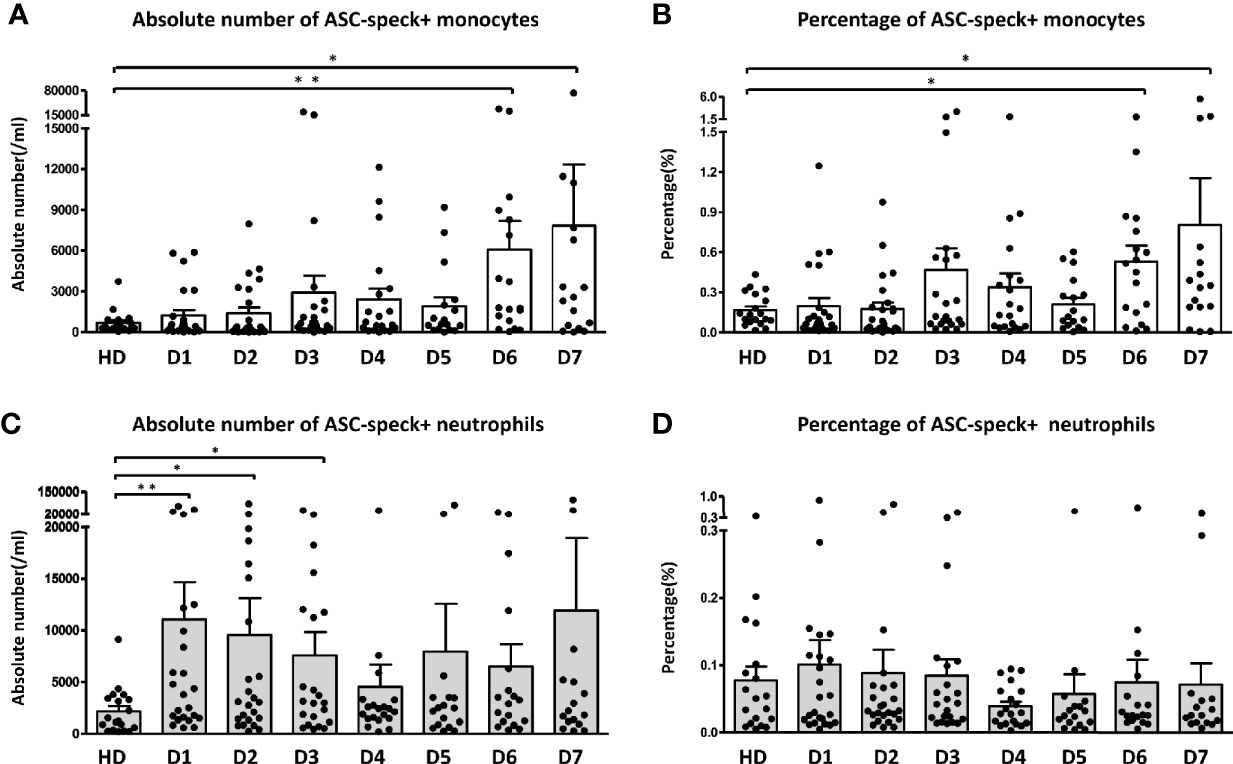
Figure 2 Dynamic change of ASC-speck+ cells in different leukocyte subsets. Both absolute number (A) and percentage (B) of ASC-speck+ monocytes increased significantly on day 6 and 7. The absolute number (C) of ASC-speck+ neutrophils increased during the first three days. However, no significant change of the percentage (D) of ASC-specks in neutrophils within seven days has been observed. The difference between healthy donors and patients was determined by Mann-Whitney test. *p < 0.05, **p < 0.01. Healthy donors n = 19; Sepsis patients day1 n = 24, day 2 n = 24, day 3 n = 22, day 4 n = 20, day5 n = 17, day 6 n = 18, day 7 n = 16. Dot plots and bar graph data are represented as mean ± SEM.
Dynamic Change of Downstream Cytokines and Clinical Parameters
IL-1β and IL-18, the downstream cytokines secreted upon inflammasome activation, were determined in serum samples to further confirm the role of ASC-speck+ leukocytes in sepsis patients (Figure 3 and Supplementary table 2). The concentration of IL-1β and IL-18 were found increased on day 1 compared to healthy controls. IL-1β values decreased back to baseline levels on day 7, whereas increased levels of IL-18 were maintained in the serum during the first week of sepsis. Other inflammatory cytokines were found elevated on day 1 and decreased thereafter (Supplementary Figure 4 and Supplementary Table 2). The differences of cytokines between survivors and non-survivors were not significant (Supplementary Table 3).
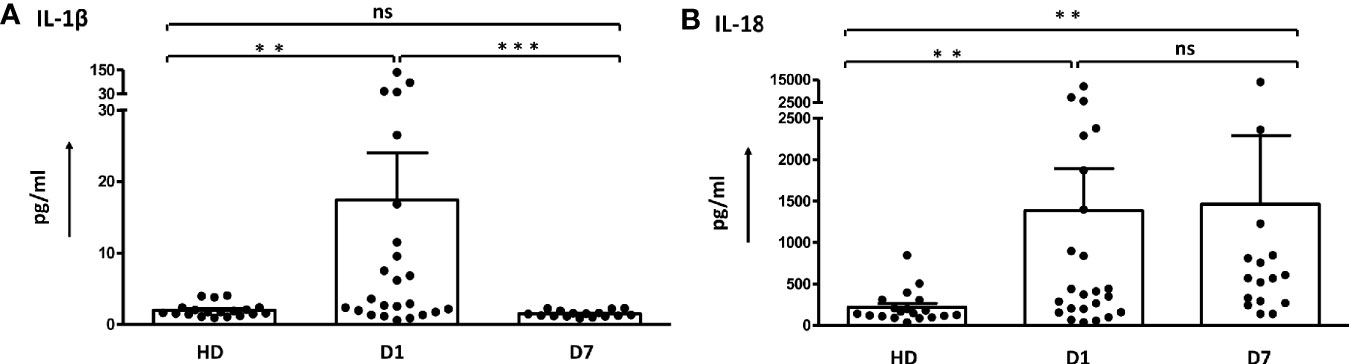
Figure 3 Dynamic change of serum levels of IL-1β and IL-18 in sepsis patients. (A) The concentration of IL-1β in sepsis patients was higher than in healthy donors on day 1 and decreased on day 7. (B) Values for IL-18 detected in sepsis patients remained increased. The difference was determined by Mann-Whitney test. **p < 0.01, ***p< 0.001. ns, not significant. Healthy donors n = 19; Sepsis patients day1 n = 24, day 7 n = 16. Dot plots and bar graph data are represented as mean ± SEM.
The dynamic changes of LDH, WBC and PCT are depicted in Supplementary Figure 5A. There was a positive correlation between the frequency of ASC-speck+ monocytes (days 5–7) and the concentration of LDH and the WBC on days 5–7 (Supplementary Figures 5B–D).
Low Absolute Number of ASC-Speck+ Blood Monocytes in Sepsis Non-Survivors
Next, we asked whether 90-day sepsis survivors and non-survivors differ in their frequency of ASC-speck+ blood leukocytes. As shown in Figure 4A this analysis revealed that sepsis non-survivors have significantly lower absolute number of ASC-speck+ monocytes on day 6 after the onset of sepsis. A further analysis was undertaken using ROC curves demonstrating that the absolute number of ASC-speck+ monocytes on day 6 discriminated with highest accuracy between survivors and non-survivors (Figure 4B). The AUROC for predicting survival was 0.875 [95% confidence interval (CI): 0.699–1.051]. The cut-off level of ASC-speck positive monocytes was 1,650 cells/ml with a specificity 83.33% (95% CI: 51.59–97.91%) and a sensitivity 83.33% (95% CI: 35.88–99.58%). Correspondingly, Kaplan-Meier curves were calculated to investigate whether the absolute number of ASC-speck+ monocytes serves as a putative biomarker in sepsis. These studies revealed that patients with lower absolute number of ASC-speck+ monocytes (<1,650 cells/ml) on day 6 showed an inferior survival [p = 0.0079, hazard ratio (HR) = 10.23, 95% CI: 1.840–56.94] (Figure 4C). Demographics and clinical characteristics of patients with high (>1,650 cells/ml) and low (<1,650 cells/ml) absolute number of ASC-speck+ monocytes are shown in Supplementary Table 4. The cut-off values for other parameters were also calculated by ROC curve analysis (Supplementary Table 5). Finally, the univariate and multivariate analysis (Table 1) showed that absolute number of ASC-speck+ monocytes is an independent predictor of 90-day mortality in sepsis patients (p = 0.035, HR = 10.178, 95% CI: 1.180–87.786).
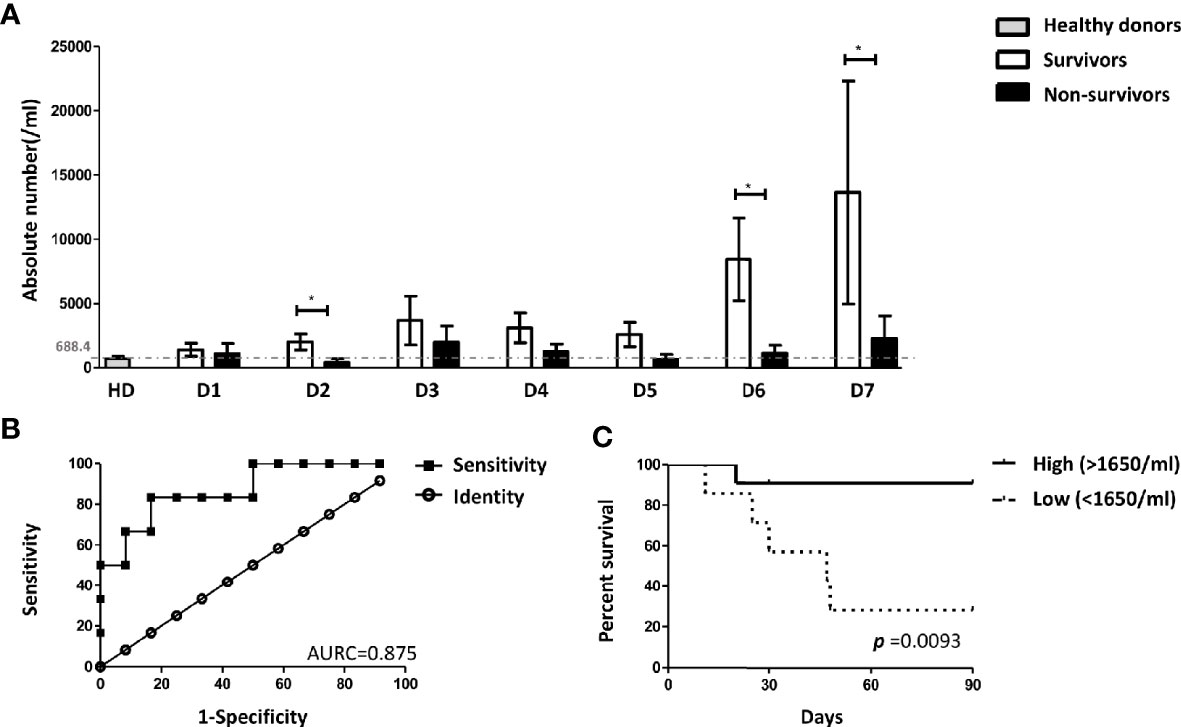
Figure 4 The absolute number of ASC-speck+ monocytes predicted the 90-days survival of sepsis patients. (A) Patients with a higher absolute number of ASC-specks on day 6 have a favorable outcome within 90 days *p < 0.05. Healthy donors n = 19; sepsis patients (survivors) day 1 n = 17, day 2 n = 17, day 3 n = 16, day 4 n = 14, day 5 n = 11, day 6 n = 12, day 7 n = 10; sepsis patients (non-survivors) day 1 n = 7, day 2 n = n = 7, day 3 n = 6, day 4 n = 6, day 5 n = 6, day 6 n = 6, day 7 n = 6. (B) Receiver operating characteristic (ROC) curve analysis on day 6 showed the AUROC for the absolute number of ASC-speck+ monocytes predicting survival was 0.875 (p = 0.011, 95% confidence interval (CI): 0.699–1.051) and the best the cutoff value was 1650 cells/ml with the highest sensitivity (83.33%) and specificity (83.33%). (C) Kaplan-Meier analysis revealed that patients with higher levels of ASC-speck positive monocytes (>1650 cells/ml) on day 6 have better survival [p = 0.0079, hazard ratio (HR) = 10.23, 95% CI: 1.840–56.94]. The patient cohort included 11 survivors and 7 non-survivors.
In addition, we analyzed the expression of HLA-DR on CD14+ monocytes (Supplementary Figure 6). Gating was done as shown in Supplementary Figure 6A. When compared with healthy donors, the HLA-DR expression of monocytes obtained from sepsis patients was significantly reduced (Supplementary Figure 6B). Although not statistically significant monocyte HLA-DR expression was higher in survivors than in non-survivors (Supplementary Figures 6C–E).
Discussion
Patients with prolonged immune suppression after sepsis frequently die from recurrent or hospital acquired infections (6–10, 12, 13). Reliable biomarkers identifying patients at risk of late death from sepsis are missing (15).
In the study presented here we identified a reduced in vivo inflammasome activation of blood monocytes as a prognostic factor of late death from sepsis. Recognition and killing of invading organisms by monocytes are important mechanisms of a protective innate inflammatory immune response. Equally important is the role of monocytes in presenting antigens by means of HLA molecules. Multiple studies have demonstrated clearly that following sepsis, monocytes have a diminished capacity for both of these responses. Specifically, they secrete fewer cytokines when stimulated (37) and down-regulate expression of HLA receptors (38, 39). This down-regulation of monocyte function generally predicts increased risk of secondary infection and poor prognosis (40).
Inflammasome activation is of central importance for the immune defense against infections and its role in sepsis pathophysiology has been highlighted by several studies recently (16–21). Among the different inflammasomes, NLRP3 and absent in melanoma 2 (AIM2) contain the adaptor molecule ASC. Inflammasomes such as the NLR family PYD containing 1 (NLRP1) and the NLR Family Caspase Recruitment Domain (CARD) Containing 4 (NLRC4) assemble in the absence of ASC, however, the presence of ASC enhances their activation and caspase-1 function (41, 42). Among the different inflammasomes NLRP3 is activated in response to the widest array of stimuli, including components from bacterial, viral, and fungal pathogens as well as endogenous molecules, for example uric acid crystals and stress signals, such as ATP and ion fluxes (43–50) converge into a common activating signal, which is the potassium efflux (51–54).
To monitor the systemic inflammatory responses in sepsis we studied in vivo inflammasome activation identified by ASC-speck formation in blood leukocytes during the first week of sepsis. Both neutrophils and monocytes are important cell subsets capable of inflammasome activation after stimulation. Being the most abundant leukocytes in the blood, neutrophils are the first line defense in protecting the host from infection or tissue damage (55). In this initial phase of sepsis bacterial derived pathogen-associated molecular patterns (PAMPs) may directly stimulate cells through their pathogen recognition receptors (PRRs). Activation of inflammasomes, release of cytokines, phagocytosis, degranulation and neutrophil extracellular trap formation are considered instrumental for this wave of host defense (4, 52–54). During the first 3 days of sepsis, neutrophils showed a significantly increased formation of ASC-specks. A short-lived rise of multiple inflammatory serum parameters (IL-1ß, TNF-α, IL-6, IL-8, MCP-1, PCT and CRP) accompanied this early response. Correspondingly, PCT values indicating bacteremia peaked on day 1 and declined thereafter, showing that infection in our patients was rapidly controlled (56).
Unlike other cytokines, IL-18 and IL-1ß are synthesized as precursor proteins and require enzymatic cleavage by caspase-1 to generate soluble, biologically active cytokines. In general, IL-1ß and IL-18 are produced and regulated differentially, and serum levels may not run in parallel (Figure 3 and Supplementary Figure 4A). Secretion of mature IL-1ß requires cell activation, is usually short lived and largely inflammasome dependent (57, 58). In contrast, IL-18 secretion is partially inflammasome-independent, is increased and sustained after stimulation but also constitutively expressed in neutrophils, monocytes, intestinal epithelial cells and endocrine tissues, such as the adrenal gland (42, 58–61).
The key finding of this study was an increase in the absolute number and the percentage of ASC-speck+ monocytes on day 6 and 7 of sepsis. A detailed analysis then clearly revealed that patients with lower absolute numbers of ASC-speck+ monocytes on day 6 showed an inferior 90-day survival.
The stimulus of inflammasome activation in monocytes on day 6 is not clear. Multiple molecular and cellular signaling events, including ionic flux, mitochondrial dysfunction, the production of reactive oxygen species (ROS), and lysosomal damage, have been shown to activate the NLRP3 inflammasomes. We found LDH values to be still upregulated. LDH levels are directly related to tissue injury, serving as a marker of necrosis and hypoxia; collectively called danger-associated molecular patterns (DAMPs) (62, 63). Similar to PAMPs also DAMPs are recognized via PRR and may have caused inflammasome activation of immune cells on day 6 of sepsis. Interestingly, we found a correlation of serum LDH levels and ASC-speck+ monocytes on days 5–7 of sepsis (Supplemental Figure 5).
The role of inflammasome activation in sepsis has been addressed recently. In a study by Giamarellos-Bourboulis et al., reduced in vitro NLRP3 inflammasome activation and IL-1ß production of monocytes stimulated with monosodium urate crystal was observed in sepsis patients compared to healthy controls (64). A study by Weighardt et al. found higher IL-1ß production of ex vivo stimulated monocytes in sepsis survivors compared to non-survivors (65). Similar results were obtained by Martinez-Garcia et al. (20); interestingly, these authors also addressed a molecular mechanism responsible for the hyporesponsiveness of monocytes. The authors showed that activation of cells via the purinergic ATP receptor P2X7 results in mitochondrial dysfunction causing inflammasome inhibition and immunosuppression of monocytes (20). Therefore, ATP release with P2X7 receptor activation as a consequence of different treatments or complications already prior to sepsis can be seen as one possibility to disarm monocyte inflammasomes causing immunoparalysis and late death from sepsis (20).
Interestingly, low level in vivo formation of ASC-specks in monocytes during early sepsis (day 1 to 5) was found in all patients, which happened despite the obvious PAMP-induced neutrophil activation evidenced by increased numbers of ASC-speck+ neutrophils and increased cytokine serum levels. A reduced inflammasome activation of monocytes during early sepsis was previously found in studies on in vitro activated monocytes (64, 65) and appears to be a general phenomenon. Whether this dysfunction of monocytes resulted from the initial PAMP-induced and granulocyte-dominated inflammatory response, is not known. Subsequent immunorestoration with successful induction of ASC-speck+ monocytes (>1,650 cells/ml) on day 6 of sepsis—possibly stimulated by DAMPs—appeared highly beneficial in terms of sepsis survival. Thus, some patients appear capable of escaping the hypo-inflammatory phase while others with lower amount of ASC-speck+ monocytes on day 6 may still be locked in a state of “immunoparalysis” associated with inferior survival. Enhanced inflammasome activation of monocytes is associated with increased rates of cell death by pyroptosis and indicative of a higher monocyte turnover (66). Ultimately, it is currently unknown whether ASC-speck+ monocytes directly save patients from post sepsis mortality or whether ASC-speck+ monocytes are indicative for a competent immune system, with a higher monocyte turnover, ready to fight off invading pathogens.
The activation of inflammasome involves two distinct steps. Firstly, a transcription factor nuclear factor-κB (NF-κB)-dependent manner, such as the binding of LPS to its receptor Toll-like Receptor (TLR)4, induces elevated expression of pro-IL-1ß. Secondly, P2X7 receptor-mediated K+ efflux induces inflammasome assembly, as well as the subsequent maturation and release of IL-1ß (52, 67, 68). ASC-speck+ monocyte numbers increased significantly during the later phase of sepsis (day 6 and day 7), when, however, serum IL-1ß levels were back to normal. This may appear as a contradiction at first; however, detection of ASC-speck in blood leukocytes represents only one source of the total IL-1ß producing cells contributing to IL-1ß serum levels, and the IL-1ß assay detected not only the inflammasome-dependent mature form of IL-1ß but also the unprocessed pro-IL-1ß, which is released upon cell lysis caused by multiple pathways including pyroptosis. In addition, production of IL-1ß depends on a two-step process regulated at the mRNA- in addition to the inflammasome level.
Monocytes are not only essential elements in innate immune responses, they also express MHC-class II and are regarded to play an important role in orchestrating adaptive immunity. Previous studies reported that persisting monocyte deactivation, characterized by a decrease in HLA-DR expression from the initial period, was associated with higher mortality and the recovery of function of monocytes was observed in survivors within 10 days (11, 38, 69–72). As previously described, we noted that HLA-DR expression of monocytes in sepsis patients was significantly reduced (70, 71). There was a clear trend that the difference of HLA-DR expression on day 6 compared to the first 4 days was higher in patients surviving sepsis. These differences did not reach statistical significance in our cohort (Supplementary Figure 6). In addition, for our patients we did not observe a statistically significant association of survival and different clinical scores (APACHE II, SOFA, or SAPS II).
Studies with murine sepsis models showed the different roles of inflammasomes. Wegiel et al. reported that the NLRP3 inflammasome is relevant for bacterial clearance in mice (73). While Lee et al. found that genetic deficiency of NLRP3 inhibited inflammatory responses and permitted enhanced survival of septic mice (17). In line with the notion that overwhelming inflammation is detrimental Dolinay et al. reported that caspase-1–dependent inflammatory responses involving the production and activation of IL-18 may play a role in the propagation of acute respiratory distress syndrome (74). The focus of many studies is the overwhelming inflammatory response at the beginning of sepsis leading to immunoparalysis and death. We instead monitored the patients for one week and identified a marker correlating with immune recovery and sepsis survival.
Conclusion
Inflammasome research and monocyte function are of particular interest in sepsis (18, 20, 21). In the study presented here, in vivo inflammasome activation was identified by ASC-speck formation in blood leukocytes during the first week of sepsis. We found that both neutrophils and monocytes are important cell subsets capable of inflammasome activation in sepsis. Statistical analysis demonstrated that the frequency of ASC-speck+ monocytes on day 6 after onset of sepsis may serve as a marker for immune monitoring and identifying patients at risk of death.
Although it remains unclear how ASC-speck+ monocytes rescue patients from death of sepsis, our present results provide a novel link between in vivo inflammasome activation in monocytes, immune competence and sepsis survival. The inflammasome has well-known pleiotropic and potentially “double-edged sword” effects in sepsis as a regulator of inflammation and as an integral part of the immune response. Future studies are required to describe the exact role of the rapidly performed flow cytometric assay to identify ASC-speck+ monocytes in making clinical decisions in sepsis.
Data Availability Statement
The original contributions presented in the study are included in the article/Supplementary Material. Further inquiries can be directed to the corresponding author.
Ethics Statement
The studies involving human participants were reviewed and approved by Ethics committee of the University of Heidelberg, described in the Methods in more detail. The patients/participants provided their written informed consent to participate in this study.
Author Contributions
JC, SO, FA, FF, CR, and SM performed the experiments. JC, SO, TB, FU, CN, MW, CM, and KS designed the project and interpreted the data. JC, SO, and KS. wrote the manuscript. TB, FU, CN, MW, and CM critically read the manuscript and facilitated sample collection. KS has final responsibility for decision to submit publication. All authors contributed to the article and approved the submitted version.
Conflict of Interest
The authors declare that the research was conducted in the absence of any commercial or financial relationships that could be construed as a potential conflict of interest.
Acknowledgments
We would like to thank Ramona Geske for critically reading the manuscript.
Supplementary Material
The Supplementary Material for this article can be found online at: https://www.frontiersin.org/articles/10.3389/fimmu.2020.613745/full#supplementary-material
References
1. Vincent JL, Marshall JC, Namendys-Silva SA, Francois B, Martin-Loeches I, Lipman J, et al. Assessment of the worldwide burden of critical illness: the intensive care over nations (ICON) audit. Lancet Respir Med (2014) 2(5):380–6. doi: 10.1016/s2213-2600(14)70061-x
2. Fleischmann C, Scherag A, Adhikari NK, Hartog CS, Tsaganos T, Schlattmann P, et al. Assessment of Global Incidence and Mortality of Hospital-treated Sepsis. Current Estimates and Limitations. Am J Respir Crit Care Med (2016) 193(3):259–72. doi: 10.1164/rccm.201504-0781OC
3. Angus DC. The lingering consequences of sepsis: a hidden public health disaster? JAMA (2010) 304(16):1833–4. doi: 10.1001/jama.2010.1546
4. van der Poll T, van de Veerdonk FL, Scicluna BP, Netea MG. The immunopathology of sepsis and potential therapeutic targets. Nat Rev Immunol (2017) 17(7):407–20. doi: 10.1038/nri.2017.36
5. Schefold JC, Hasper D, Reinke P, Monneret G, Volk HD. Consider delayed immunosuppression into the concept of sepsis. Crit Care Med (2008) 36(11):3118. doi: 10.1097/CCM.0b013e31818bdd8f
6. Hotchkiss RS, Karl IE. The pathophysiology and treatment of sepsis. N Engl J Med (2003) 348(2):138–50. doi: 10.1056/NEJMra021333
7. Hall MW, Knatz NL, Vetterly C, Tomarello S, Wewers MD, Volk HD, et al. Immunoparalysis and nosocomial infection in children with multiple organ dysfunction syndrome. Intensive Care Med (2011) 37(3):525–32. doi: 10.1007/s00134-010-2088-x
9. Boomer JS, To K, Chang KC, Takasu O, Osborne DF, Walton AH, et al. Immunosuppression in patients who die of sepsis and multiple organ failure. JAMA (2011) 306(23):2594–605. doi: 10.1001/jama.2011.1829
10. Torgersen C, Moser P, Luckner G, Mayr V, Jochberger S, Hasibeder WR, et al. Macroscopic postmortem findings in 235 surgical intensive care patients with sepsis. Anesth Analg. (2009) 108(6):1841–7. doi: 10.1213/ane.0b013e318195e11d
11. Monneret G, Lepape A, Voirin N, Bohe J, Venet F, Debard AL, et al. Persisting low monocyte human leukocyte antigen-DR expression predicts mortality in septic shock. Intensive Care Med (2006) 32(8):1175–83. doi: 10.1007/s00134-006-0204-8
12. Otto GP, Sossdorf M, Claus RA, Rodel J, Menge K, Reinhart K, et al. The late phase of sepsis is characterized by an increased microbiological burden and death rate. Crit Care (2011) 15(4):R183. doi: 10.1186/cc10332
13. Hotchkiss RS, Monneret G, Payen D. Sepsis-induced immunosuppression: from cellular dysfunctions to immunotherapy. Nat Rev Immunol (2013) 13(12):862–74. doi: 10.1038/nri3552
14. Fattahi F, Ward PA. Understanding Immunosuppression after Sepsis. Immunity (2017) 47(1):3–5. doi: 10.1016/j.immuni.2017.07.007
15. Raith EP, Udy AA, Bailey M, McGloughlin S, MacIsaac C, Bellomo R, et al. Prognostic Accuracy of the SOFA Score, SIRS Criteria, and qSOFA Score for In-Hospital Mortality Among Adults With Suspected Infection Admitted to the Intensive Care Unit. JAMA (2017) 317(3):290–300. doi: 10.1001/jama.2016.20328
16. Fahy RJ, Exline MC, Gavrilin MA, Bhatt NY, Besecker BY, Sarkar A, et al. Inflammasome mRNA expression in human monocytes during early septic shock. Am J Respir Crit Care Med (2008) 177(9):983–8. doi: 10.1164/rccm.200703-418OC
17. Lee S, Nakahira K, Dalli J, Siempos II, Norris PC, Colas RA, et al. NLRP3 Inflammasome Deficiency Protects against Microbial Sepsis via Increased Lipoxin B4 Synthesis. Am J Respir Crit Care Med (2017) 196(6):713–26. doi: 10.1164/rccm.201604-0892OC
18. Jorgensen I, Miao EA. Pyroptotic cell death defends against intracellular pathogens. Immunol Rev (2015) 265(1):130–42. doi: 10.1111/imr.12287
19. Mankan AK, Dau T, Jenne D, Hornung V. The NLRP3/ASC/Caspase-1 axis regulates IL-1beta processing in neutrophils. Eur J Immunol (2012) 42(3):710–5. doi: 10.1002/eji.201141921
20. Martinez-Garcia JJ, Martinez-Banaclocha H, Angosto-Bazarra D, de Torre-Minguela C, Baroja-Mazo A, Alarcon-Vila C, et al. P2X7 receptor induces mitochondrial failure in monocytes and compromises NLRP3 inflammasome activation during sepsis. Nat Commun (2019) 10(1):2711. doi: 10.1038/s41467-019-10626-x
21. Goncalves AC, Ferreira LS, Manente FA, de Faria CMQG, Polesi MC, de Andrade CR, et al. The NLRP3 inflammasome contributes to host protection during Sporothrix schenckii infection. Immunology (2017) 151(2):154–66. doi: 10.1111/imm.12719
22. Prochnicki T, Latz E. Inflammasomes on the Crossroads of Innate Immune Recognition and Metabolic Control. Cell Metab (2017) 26(1):71–93. doi: 10.1016/j.cmet.2017.06.018
24. Sahoo M, Ceballos-Olvera I, del Barrio L, Re F. Role of the inflammasome, IL-1beta, and IL-18 in bacterial infections. ScientificWorldJournal (2011) 11:2037–50. doi: 10.1100/2011/212680
25. Schroder K, Tschopp J. The inflammasomes. Cell (2010) 140(6):821–32. doi: 10.1016/j.cell.2010.01.040
26. Goncalves AC, Ferreira LS, Manente FA, de Faria C, Polesi MC, de Andrade CR, et al. The NLRP3 inflammasome contributes to host protection during Sporothrix schenckii infection. Immunology (2017) 151(2):154–66. doi: 10.1111/imm.12719
27. Matute-Bello G. Targeting caspase-1 in sepsis: a novel approach to an old problem. Intensive Care Med (2007) 33(5):755–7. doi: 10.1007/s00134-007-0589-z
28. Xu XE, Liu L, Wang YC, Wang CT, Zheng Q, Liu QX, et al. Caspase-1 inhibitor exerts brain-protective effects against sepsis-associated encephalopathy and cognitive impairments in a mouse model of sepsis. Brain Behav Immun (2019) 80:859–70. doi: 10.1016/j.bbi.2019.05.038
29. Wang YC, Liu QX, Liu T, Xu XE, Gao W, Bai XJ, et al. Caspase-1-dependent pyroptosis of peripheral blood mononuclear cells predicts the development of sepsis in severe trauma patients: A prospective observational study. Med (Baltimore) (2018) 97(8):e9859. doi: 10.1097/md.0000000000009859
30. Sester DP, Zamoshnikova A, Thygesen SJ, Vajjhala PR, Cridland SO, Schroder K, et al. Assessment of Inflammasome Formation by Flow Cytometry. Curr Protoc Immunol (2016) 114:14 40 1–14 40 29. doi: 10.1002/cpim.13
31. Sester DP, Thygesen SJ, Sagulenko V, Vajjhala PR, Cridland JA, Vitak N, et al. A novel flow cytometric method to assess inflammasome formation. J Immunol (2015) 194(1):455–62. doi: 10.4049/jimmunol.1401110
32. Hoss F, Rolfes V, Davanso MR, Braga TT, Franklin BS. Detection of ASC Speck Formation by Flow Cytometry and Chemical Cross-linking. Methods Mol Biol (2018) 1714:149–65. doi: 10.1007/978-1-4939-7519-8_10
33. Ahmad F, Mishra N, Ahrenstorf G, Franklin BS, Latz E, Schmidt RE, et al. Evidence of inflammasome activation and formation of monocyte-derived ASC specks in HIV-1 positive patients. AIDS (2018) 32(3):299–307. doi: 10.1097/QAD.0000000000001693
34. Chung IC, Chen LC, Tsang NM, Chuang WY, Liao TC, Yuan SN, et al. Mitochondrial Oxidative Phosphorylation Complex Regulates NLRP3 Inflammasome Activation and Predicts Patient Survival in Nasopharyngeal Carcinoma. Mol Cell Proteomics. (2020) 19(1):142–54. doi: 10.1074/mcp.RA119.001808
35. Nusshag C, Rupp C, Schmitt F, Krautkramer E, Speer C, Kalble F, et al. Cell Cycle Biomarkers and Soluble Urokinase-Type Plasminogen Activator Receptor for the Prediction of Sepsis-Induced Acute Kidney Injury Requiring Renal Replacement Therapy: A Prospective, Exploratory Study. Crit Care Med (2019) 47(12):e999–e1007. doi: 10.1097/ccm.0000000000004042
36. Singer M, Deutschman CS, Seymour CW, Shankar-Hari M, Annane D, Bauer M, et al. The Third International Consensus Definitions for Sepsis and Septic Shock (Sepsis-3). JAMA (2016) 315(8):801–10. doi: 10.1001/jama.2016.0287
37. West MA, Heagy W. Endotoxin tolerance: a review. Crit Care Med (2002) 30(1 Suppl):S64–73. doi: 10.1097/00003246-200201001-00009
38. Tschaikowsky K, Hedwig-Geissing M, Schiele A, Bremer F, Schywalsky M, Schuttler J. Coincidence of pro- and anti-inflammatory responses in the early phase of severe sepsis: Longitudinal study of mononuclear histocompatibility leukocyte antigen-DR expression, procalcitonin, C-reactive protein, and changes in T-cell subsets in septic and postoperative patients. Crit Care Med (2002) 30(5):1015–23. doi: 10.1097/00003246-200205000-00010
39. Flohe S, Scholz M. HLA-DR monitoring in the intensive care unit–more than a tool for the scientist in the laboratory? Crit Care Med (2009) 37(10):2849–50. doi: 10.1097/CCM.0b013e3181ad7ac9
40. Lukaszewicz AC, Grienay M, Resche-Rigon M, Pirracchio R, Faivre V, Boval B, et al. Monocytic HLA-DR expression in intensive care patients: interest for prognosis and secondary infection prediction. Crit Care Med (2009) 37(10):2746–52. doi: 10.1097/CCM.0b013e3181ab858a
41. Yang Y, Wang H, Kouadir M, Song H, Shi F. Recent advances in the mechanisms of NLRP3 inflammasome activation and its inhibitors. Cell Death Dis (2019) 10(2):128. doi: 10.1038/s41419-019-1413-8
42. Latz E, Xiao TS, Stutz A. Activation and regulation of the inflammasomes. Nat Rev Immunol (2013) 13(6):397–411. doi: 10.1038/nri3452
43. Indramohan M, Stehlik C, Dorfleutner A. COPs and POPs Patrol Inflammasome Activation. J Mol Biol (2018) 430(2):153–73. doi: 10.1016/j.jmb.2017.10.004
44. Mariathasan S, Weiss DS, Newton K, McBride J, O’Rourke K, Roose-Girma M, et al. Cryopyrin activates the inflammasome in response to toxins and ATP. Nature (2006) 440(7081):228–32. doi: 10.1038/nature04515
45. Martinon F, Petrilli V, Mayor A, Tardivel A, Tschopp J. Gout-associated uric acid crystals activate the NALP3 inflammasome. Nature (2006) 440(7081):237–41. doi: 10.1038/nature04516
46. Dostert C, Pétrilli V, Van Bruggen R, Steele C, Mossman BT, Tschopp J. Innate immune activation through Nalp3 inflammasome sensing of asbestos and silica. Science (2008) 320(5876):674–7. doi: 10.1126/science.1156995
47. Eisenbarth SC, Colegio OR, O’Connor W, Sutterwala FS, Flavell RA. Crucial role for the Nalp3 inflammasome in the immunostimulatory properties of aluminium adjuvants. Nature (2008) 453(7198):1122–6. doi: 10.1038/nature06939
48. Ichinohe T, Lee HK, Ogura Y, Flavell R, Iwasaki A. Inflammasome recognition of influenza virus is essential for adaptive immune responses. J Exp Med (2009) 206(1):79–87. doi: 10.1084/jem.20081667
49. Joly S, Sutterwala FS. Fungal pathogen recognition by the NLRP3 inflammasome. Virulence. (2010) 1(4):276–80. doi: 10.4161/viru.1.4.11482
50. Kanneganti TD, Body-Malapel M, Amer A, Park JH, Whitfield J, Franchi L, et al. Critical role for Cryopyrin/Nalp3 in activation of caspase-1 in response to viral infection and double-stranded RNA. J Biol Chem (2006) 281(48):36560–8. doi: 10.1074/jbc.M607594200
51. Tschopp J, Schroder K. NLRP3 inflammasome activation: The convergence of multiple signalling pathways on ROS production? Nat Rev Immunol (2010) 10(3):210–5. doi: 10.1038/nri2725
52. Lamkanfi M, Dixit VM. Mechanisms and functions of inflammasomes. Cell (2014) 157(5):1013–22. doi: 10.1016/j.cell.2014.04.007
53. Muñoz-Planillo R, Kuffa P, Martínez-Colón G, Smith BL, Rajendiran TM, Núñez G. K+ efflux is the common trigger of NLRP3 inflammasome activation by bacterial toxins and particulate matter. Immunity (2013) 38(6):1142–53. doi: 10.1016/j.immuni.2013.05.016
54. Petrilli V, Papin S, Dostert C, Mayor A, Martinon F, Tschopp J. Activation of the NALP3 inflammasome is triggered by low intracellular potassium concentration. Cell Death Differ (2007) 14(9):1583–9. doi: 10.1038/sj.cdd.4402195
55. de Oliveira S, Rosowski EE, Huttenlocher A. Neutrophil migration in infection and wound repair: going forward in reverse. Nat Rev Immunol (2016) 16(6):378–91. doi: 10.1038/nri.2016.49
56. Leliefeld PH, Wessels CM, Leenen LP, Koenderman L, Pillay J. The role of neutrophils in immune dysfunction during severe inflammation. Crit Care (2016) 20:73. doi: 10.1186/s13054-016-1250-4
57. Takeuchi O, Akira S. Pattern recognition receptors and inflammation. Cell (2010) 140(6):805–20. doi: 10.1016/j.cell.2010.01.022
58. Zhu Q, Kanneganti TD. Cutting Edge: Distinct Regulatory Mechanisms Control Proinflammatory Cytokines IL-18 and IL-1beta. J Immunol (2017) 198(11):4210–5. doi: 10.4049/jimmunol.1700352
59. Bakele M, Joos M, Burdi S, Allgaier N, Poschel S, Fehrenbacher B, et al. Localization and functionality of the inflammasome in neutrophils. J Biol Chem (2014) 289(8):5320–9. doi: 10.1074/jbc.M113.505636
60. Pizarro TT, Michie MH, Bentz M, Woraratanadharm J, Smith MF Jr., Foley E, et al. IL-18, a novel immunoregulatory cytokine, is up-regulated in Crohn’s disease: expression and localization in intestinal mucosal cells. J Immunol (1999) 162(11):6829–35. 10.1016/S0016-5085(98)84319-9
61. Sugama S, Wang N, Shimokawa N, Koibuchi N, Fujita M, Hashimoto M, et al. The adrenal gland is a source of stress-induced circulating IL-18. J Neuroimmunol. (2006) 172(1–2):59–65. doi: 10.1016/j.jneuroim.2005.11.001
62. Duman A, Akoz A, Kapci M, Ture M, Orun S, Karaman K, et al. Prognostic value of neglected biomarker in sepsis patients with the old and new criteria: predictive role of lactate dehydrogenase. Am J Emerg Med (2016) 34(11):2167–71. doi: 10.1016/j.ajem.2016.06.012
63. Alves-Filho JC, Spiller F, Cunha FQ. Neutrophil paralysis in sepsis. Shock (2010) 34 Suppl 1:15–21. doi: 10.1097/SHK.0b013e3181e7e61b
64. Giamarellos-Bourboulis EJ, van de Veerdonk FL, Mouktaroudi M, Raftogiannis M, Antonopoulou A, Joosten LA, et al. Inhibition of caspase-1 activation in Gram-negative sepsis and experimental endotoxemia. Crit Care (2011) 15(1):R27. doi: 10.1186/cc9974
65. Weighardt H, Heidecke CD, Emmanuilidis K, Maier S, Bartels H, Siewert JR, et al. Sepsis after major visceral surgery is associated with sustained and interferon-gamma-resistant defects of monocyte cytokine production. Surgery. (2000) 127(3):309–15. doi: 10.1067/msy.2000.104118
66. Kelley N, Jeltema D, Duan Y, He Y. The NLRP3 Inflammasome: An Overview of Mechanisms of Activation and Regulation. Int J Mol Sci (2019) 20(13):3328. doi: 10.3390/ijms20133328
67. Savio LEB, de Andrade Mello P, da Silva CG, Coutinho-Silva R. The P2X7 Receptor in Inflammatory Diseases: Angel or Demon? Front Pharmacol (2018) 9(52):52. doi: 10.3389/fphar.2018.00052
68. Di Virgilio F, Dal Ben D, Sarti AC, Giuliani AL, Falzoni S. The P2X7 Receptor in Infection and Inflammation. Immunity (2017) 47(1):15–31. doi: 10.1016/j.immuni.2017.06.020
69. Cheron A, Floccard B, Allaouchiche B, Guignant C, Poitevin F, Malcus C, et al. Lack of recovery in monocyte human leukocyte antigen-DR expression is independently associated with the development of sepsis after major trauma. Crit Care (2010) 14(6):R208. doi: 10.1186/cc9331
70. Wu JF, Ma J, Chen J, Ou-Yang B, Chen MY, Li LF, et al. Changes of monocyte human leukocyte antigen-DR expression as a reliable predictor of mortality in severe sepsis. Crit Care (2011) 15(5):R220. doi: 10.1186/cc10457
71. Drewry AM, Ablordeppey EA, Murray ET, Beiter ER, Walton AH, Hall MW, et al. Comparison of monocyte human leukocyte antigen-DR expression and stimulated tumor necrosis factor alpha production as outcome predictors in severe sepsis: a prospective observational study. Crit Care (2016) 20(1):334. doi: 10.1186/s13054-016-1505-0
72. Landelle C, Lepape A, Voirin N, Tognet E, Venet F, Bohe J, et al. Low monocyte human leukocyte antigen-DR is independently associated with nosocomial infections after septic shock. Intensive Care Med (2010) 36(11):1859–66. doi: 10.1007/s00134-010-1962-x
73. Wegiel B, Larsen R, Gallo D, Chin BY, Harris C, Mannam P, et al. Macrophages sense and kill bacteria through carbon monoxide-dependent inflammasome activation. J Clin Invest (2014) 124(11):4926–40. doi: 10.1172/JCI72853
Keywords: inflammasome, sepsis, monocytes, biomarker, ASC-speck
Citation: Cui J, Oehrl S, Ahmad F, Brenner T, Uhle F, Nusshag C, Rupp C, Funck F, Meisel S, Weigand MA, Morath C and Schäkel K (2021) Detection of In Vivo Inflammasome Activation for Predicting Sepsis Mortality. Front. Immunol. 11:613745. doi: 10.3389/fimmu.2020.613745
Received: 03 October 2020; Accepted: 11 December 2020;
Published: 04 February 2021.
Edited by:
Pablo Pelegrin, Biomedical Research Institute of Murcia (IMIB), SpainReviewed by:
Stefan W. Ryter, Harvard Medical School, United StatesEsteban Ballestar, Josep Carreras Leukaemia Research Institute (IJC), Spain
Rksubbarao Malireddi, St. Jude Children's Research Hospital, United States
Copyright © 2021 Cui, Oehrl, Ahmad, Brenner, Uhle, Nusshag, Rupp, Funck, Meisel, Weigand, Morath and Schäkel. This is an open-access article distributed under the terms of the Creative Commons Attribution License (CC BY). The use, distribution or reproduction in other forums is permitted, provided the original author(s) and the copyright owner(s) are credited and that the original publication in this journal is cited, in accordance with accepted academic practice. No use, distribution or reproduction is permitted which does not comply with these terms.
*Correspondence: Knut Schäkel, knut.schaekel@med.uni-heidelberg.de