- 1Departamento de Ecología Humana, Centro de Investigación y de Estudios Avanzados del IPN - Unidad Mérida, Merida, Mexico
- 2Red de Ambiente y Sustentabilidad, Instituto de Ecología, A. C, Xalapa, Mexico
The impact of ancient urban occupations on sustainability has recently become a topic of interest for archaeologists as well as many other scholars. Much of this archaeological research has focused on documenting the longevity of ancient cities and elucidating the social and economic strategies employed at the urban and regional scales to promote urban sustainability. In this article, we add to this discussion by addressing the issue of sustainability by considering the impact of environmental legacies left by ancient cities after their abandonment. Using a series of cases from pre-Hispanic Mesoamerica [OMYK (Coba), Kiuic, Lagunas de Yalahau, and Tlaxcallan], we show through ethnoecological and historical ecological research that in some cases pre-Hispanic people, living in urban zones, affected “intermediate” disturbances that increased biodiversity, biomass, and sustainability by creating second natures that have endured for centuries.
Introduction
Since its introduction in wider academic debates in the early 1970s (e.g., Meadows et al., 1972), the concept of sustainability and its cousin sustainable development have become extremely popular across academic, political, and economic discourse. Despite its popularity and 50 years of publications, a single clear and formal definition of sustainability has not emerged. Instead, an ever-growing number of definitions have been proposed and applied to myriad topics (Dresner, 2008; Giovannoni and Fabietti, 2014). Archaeologists have traditionally focused on arguments concerning the longevity of settlements, especially in cities and regions in complex societies (Smith, 2010; Smith et al., 2021). Accordingly, they have tended to explore strategies deployed to maintain societies and settlements for centuries or millennia (McNeil, 2012; Isendahl and Smith, 2013; Lentz et al., 2014; Smith et al., 2021; Feinman et al., 2023). These endeavors have produced and continue to produce admirable results that help us better understand how factors such as rural intensification, markets, and collective action contribute to longevity across cultures (e.g., Carballo et al., 2022; Feinman et al., 2023).
While this has been a useful pathway, we wish to examine the issue of sustainability from another perspective in this study. Despite the longevity of some settlements and regional polities, change through collapse and reorganization is a common aspect of human experience. In somewhat banal terms, change is inevitable, and perpetual social and economic sustainability is impossible. Thus, we propose to examine how human transformation of the environment, especially in the case of ancient urbanism in Mesoamerica, has contributed to or enhanced environmental sustainability. To achieve this, we first consider the role of resilience in sustainability. We then explored the relationship between resilience and biodiversity, as well as biomass. Finally, we consider the impact of urbanism in pre-Hispanic Mesoamerica on environmental sustainability from the perspective of historical ecology and the longue durée (e.g., Balée, 2006). This perspective will be applied to northern Maya lowland and Central Mexican landscape management (Figure 1). In the case of the northern Maya lowlands, we consider the impact of urbanization on OMYK (Coba), Kiuic, and Lagunas de Yalahau. For Central Mexico, we considered the city of Tlaxcallan. The results of these studies indicate that these cities were abandoned because of a variety of factors such as social, economic, and environmental changes. However, they have all left behind legacy environments or landscape palimpsests that have endured for centuries or millennia. These landscapes feature greater biodiversity and biomass (or natural capital) than pre-urban environments and adjacent zones, which lack ancient landscape modifications. Accordingly, we argue that ancient Mesoamerican urbanism, in some cases, increased biodiversity and biomass, thereby contributing to greater resilience and enduring legacies of enhanced environmental sustainability.
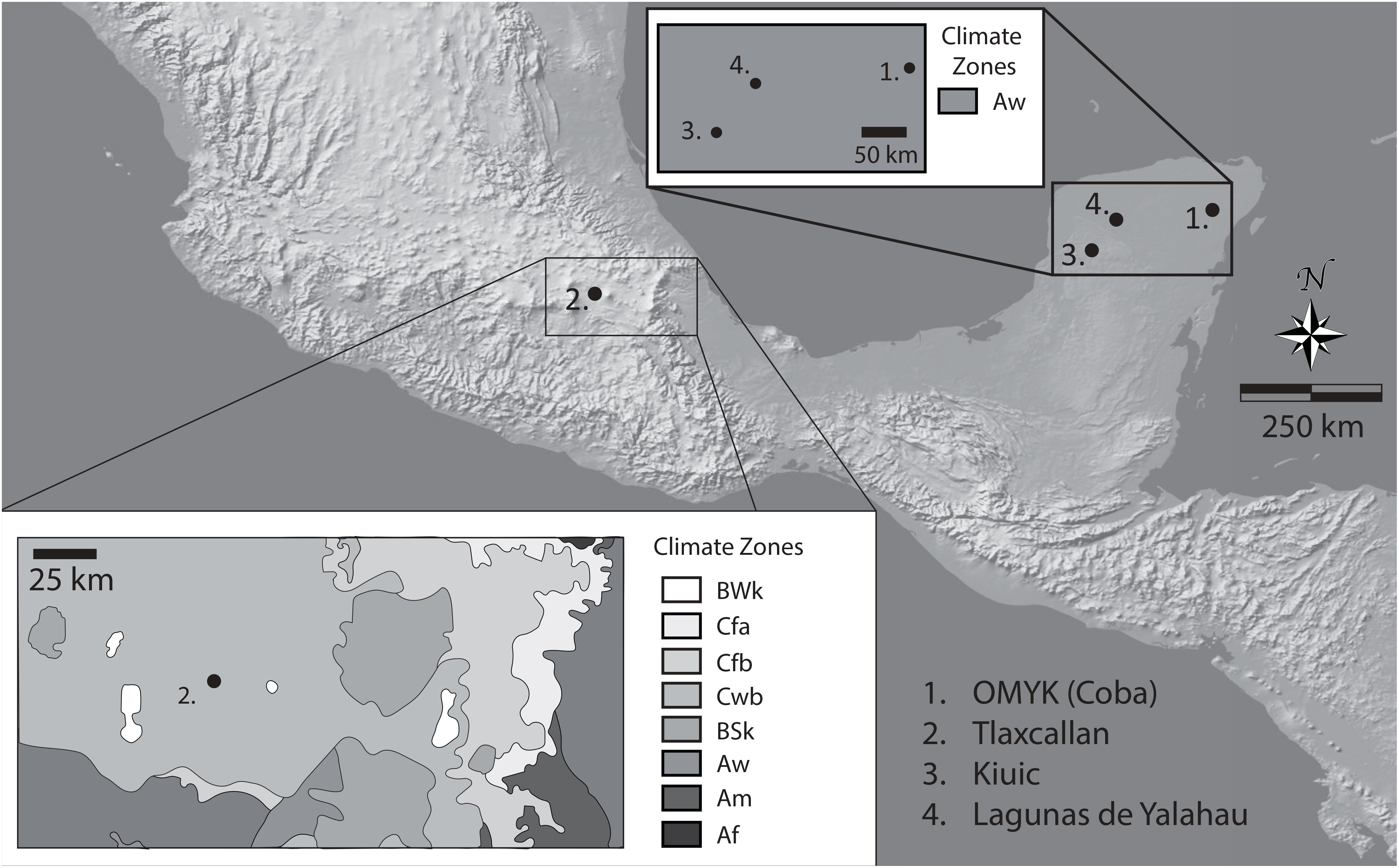
Figure 1 Shaded relief map showing topographic settings and insets showing the environmental settings of the case study sites.
Background
Sustainability, resilience, and biodiversity
Most definitions of sustainability either implicitly or explicitly rely on the concept of carrying capacity (or ecological footprint analysis) (cf., Goodland, 1995; Wackernagel and Rees, 1998; Giovannoni and Fabietti, 2014). Therefore, any particular resource or an entire “system” has a limit to which it can indefinitely support use or extraction. Exceeding that limit (e.g., overuse) will deplete said resources or systems to the point that they collapse. Accordingly, sustainability refers to extraction or use that remains below that limit and allows the resource or system to regenerate, persist, or survive (Daly, 1990; Costanza and Patten, 1995; Goodland, 1995). Many scholars have subdivided the concept of sustainability along three axes: 1) social sustainability, 2) economic or business sustainability, and 3) environmental sustainability (Goodland, 1995; Giovannoni and Fabietti, 2014). Here, we focus on environmental sustainability (Costanza and Patten, 1995; Goodland, 1995; Giovannoni and Fabietti, 2014; Carballo et al., 2022; Feinman et al., 2023; and Hicks, 1946 for discussions on social and economic sustainability).
The most straightforward definition of environmental sustainability we identified in the literature defines it as non-depletion of “natural capital” (Costanza and Patten, 1995; Goodland, 1995; Giovannoni and Fabietti, 2014). In other words, the rate of extraction or consumption of renewable natural resources for any activity or goal is maintained below the rate at which that resource renews itself (Costanza and Patten, 1995; Goodland, 1995; Giovannoni and Fabietti, 2014). For nonrenewable resources, sustainability focuses on reducing consumption or extraction to a minimum level coupled with the search for and implementation of alternative sources (e.g., replacement of oil extraction with solar energy). Alternatively, environmental sustainability seeks to maintain ecosystem services into the distant future, including reducing/mitigating/eliminating waste and contamination (Goodland, 1995; Giovannoni and Fabietti, 2014).
Theoretical and empirical studies have demonstrated that a relationship exists between sustainability and resilience arising from the nature of ecological change. According to Holling (1996:31) ecological change is “episodic, with slow accumulation of natural capital such as biomass or nutrients, punctuated by sudden releases and reorganization of that capital as the result of internal or external … processes….” Consequently, resilience may be defined as the ability of an environment or landscape to absorb disturbances (stresses) without experiencing sudden release or reorganization (Holling, 1996:31–32). Thus, greater resilience results in longer periods without reorganization, allowing environments or landscapes to persist producing greater sustainability. Theory and research have demonstrated that greater biodiversity results in a larger number of species performing the same role in the environment (Peterson et al., 1998). Because different species respond differently to disturbances or stresses, greater species diversity provides more robustness to ecological processes, which increases resilience (Tilman and Downing, 1994; Holling, 1996). This is because diversity allows for greater reinforcement of ecological interactions, which, in turn, dampens disruptions (Peterson et al., 1998). For example, Frank and McNaughton (1991) found that grasslands with greater diversity of grass species recovered more quickly from droughts than in areas with lower diversity (Tilman and Downing, 1994). Naeem et al. (1994) used experimental plots to show that greater species richness is associated with greater carbon dioxide consumption, more light capture, denser canopies, and higher productivity.
Historical ecology, longue durée, and landscape palimpsests
Our approach to environmental sustainability is informed by the research program on Historical Ecology (e.g., Balée, 2006; Szabó, 2015; Armstrong et al., 2017). Therefore, we take a holistic or monistic view of the environment such that humans and their actions are considered part of nature and not separate from it. In this approach, the environment is conceptualized as a landscape, which is the outcome of the interaction of human activity, abiotic (or physico-chemical) factors, and biotic factors (e.g., flora, fauna, and fungi). Thus, humans are historical agents of change that produce a second nature, landscape palimpsests (manuscripts on which more successive texts have been written), which are the accumulation of humanly induced, as well as abiotically and biotically induced, change over thousands to tens of thousands of years (the longue durée) (Crumley, 2022). Historical ecologists have demonstrated empirically that intermediate scale “disturbances” can result in changes in biota that persist over the longue durée (Balée, 2006). These disturbances include a) controlled use of fire, b) modification of hydrological systems, c) mineral and biological enrichment of soils, d) domestication and diversification of plant and animal species, e) changes in species distribution and abundance, f) co-evolutionary histories with wildlife, and g) the complete shaping of useful oligarchic meta-landscapes through complex successive vegetation management (Rivera-Núñez and Fargher, 2021).
Intermediate-scale disturbance mold environments, over time, into mosaic landscapes defined by a patchwork of different types of forests and/or grasslands (e.g., oligarchical, sacred groves, unmodified forests, prairies, etc.), with enriched alpha (refers to diversity at the local scale, often expressed in number of species or richness in a functional community), beta (refers to changes or differences in species diversity between two localities, by counting/comparing, for example, the unique species between each area), and/or gamma biodiversity (refers to a measure of global diversity within a region or at the landscape scale) in some cases (Balée et al., 2020; Andermann et al., 2022). In cases where biodiversity increases, these second natural landscapes should be more resilient, and thus, exhibit greater sustainability. This can be understood through the hypothesis of mid-range transformations proposed by Connell (1978) to explain how certain controlled disturbances can generate biodiversity peaks in tropical rain forests because intermediate transformations can match in succession k-selected and r-selected species (Figure 2).
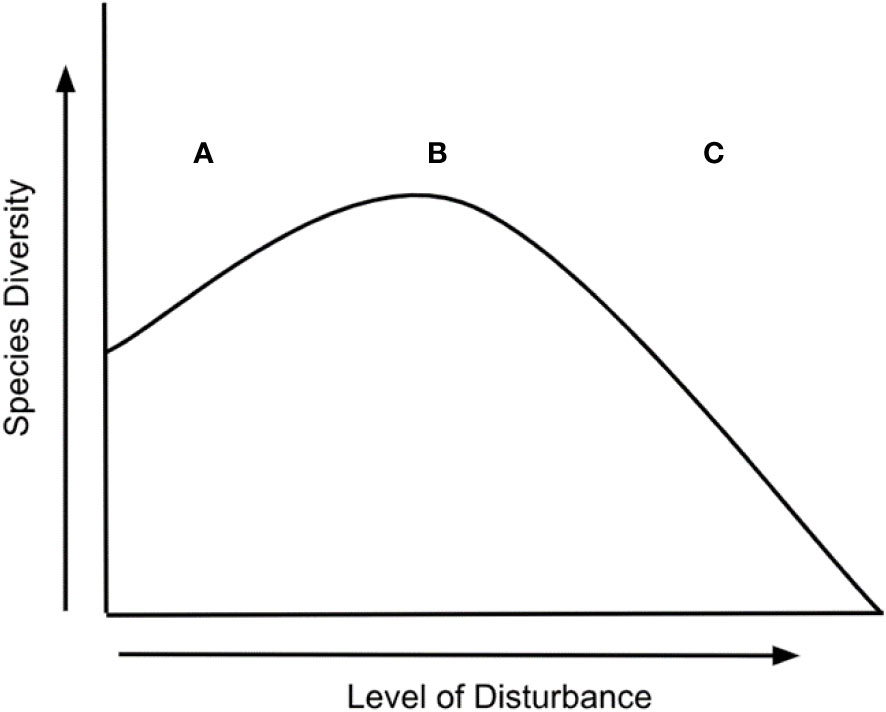
Figure 2 Species diversity scenarios proposed by the human-mediated disturbance hypothesis: (A) at low levels of ecological disturbance, species richness decreases as competitive exclusion increases; (B) at intermediate levels of disturbance, diversity is maximized because species that thrive at both early and late successional stages can coexist; and (C) at high levels of disturbance, species richness is decreased because of an increase in species movement [Modified from Connell (1978) and Balée (2006)].
Background on pre-Hispanic Mesoamerican urbanism
Ecological settings of case studies
The Preclassic–Classic Period cities of Coba (OMYK), Kiuic, and Lagunas de Yalahau were located in the northern Maya lowlands (Figure 1). All were located in Aw (tropical wet-dry) climates, according to the Köppen–Geiger classification. This region has an average temperature range of 24°C in January to 29°C in July. Rainfall follows a gradient from Northwest to Southeast, ranging from less than 800 mm to more than 1,100 mm. The region experiences a three-season climate (rainy, nortes, dry) due to exposure to cold fronts crossing the Gulf of Mexico from the US that collide with warm, moist tropical air, generating rainfall during the winter (December–February). Most precipitation occurs during the hurricane season from June to November, and the region experiences a mid-summer drought in July. The region is covered by dry semi-deciduous forests owing to the strong seasonal variation in rainfall. These forests are dominated by chaca or West Indian birch (Bursera simaruba), kitam che’ (Caesalpinia gaumeri), sak ts’iits’il che’ (Gymnopodium floribundum), ja’abin or Jamaican dogwood (Piscidia piscipula), and k’an chuunup (Thouinia paucidentata) (White and Hood, 2004). In drier zones, kibche’ (Guettarda elliptica), wild tamarind (Lysiloma bahamense), and ta’tsi’ or black breadfruit (Neea choriophylla) are common, whereas in wetter areas, boob ch’iich’ (Coccoloba cozumelensis and Coccoloba spicata), chiim kuuts (Croton reflexifolius), pichi che’ (Eugenia buxifolia), t’iitil (Gymnanthes lucida), and chi’ kéej or little sapote (Manilkara zapota) predominate (White and Hood, 2004).
Conversely, the late pre-Hispanic city of Tlaxcallan was located in the central plateau of Mexico (Figure 1). Although, the latitude of this zone places it in the tropics, its elevation plays a major role in the climate. Accordingly, in the Köppen–Geiger climate classification system, Mexico’s central plateau is classified as having a subtropical highland climate (Cwb). The region experiences cold, comparatively dry winters and cool wet summers. Average annual temperatures range between 14°C and 19°C, and rainfall ranges between 600 mm/y and 1,100 mm/y (Wilken, 1970). Most of the rainfall falls between June and August (Wilken, 1970). The rainy season is punctuated by a mid-summer drought, typically during July. The vegetation of the region is dominated by pine–oak–alder forests (Lozano-García et al., 2013: Figure 3A). Ash, willow, hornbeam, cypress, ragweed, flowers (e.g., aster, daisy, sunflower), amaranths, and grasses are present in smaller amounts (Lozano-García et al., 2013: Figure 3A). Finally, agave and nopal are conspicuous members of the landscape, but their pollen density is significantly lower than that of the other species.
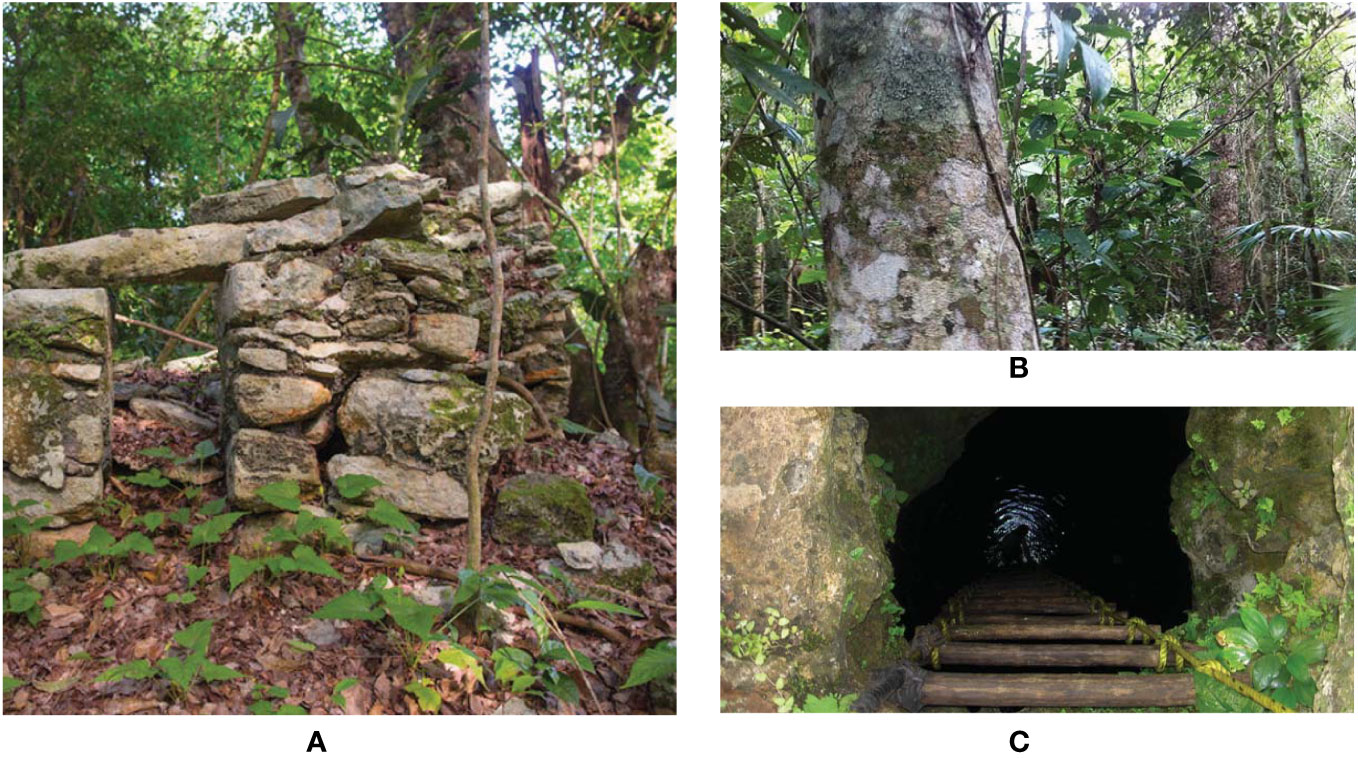
Figure 3 Composition of a ka’kab ka’ax (ancestor’s forest) landscape unit in Otoch Ma’ax Yetel Kooh, Yucatan: (A) miniature temple belonging to the “East Coast” Maya construction style that corresponds to Coba’s Late Postclassic architecture; (B) large Ramon tree (Brosimum alicastrum) located next to the miniature temple; and (C) funerary and ceremonial cenote adjacent to the temple and the forest dominated by Ramon in which more than two hundred human skulls were recorded.
Historical chronology of urbanism in pre-Hispanic Mesoamerica
Archaeological research demonstrates that cities emerged in Mesoamerica during the Middle Preclassic period (900–300 B.C.E.). Among the earliest and most important cities are Monte Albán (founded c. 500 B.C.E.), in the Valley of Oaxaca, Nakbe, in the El Mirador Basin, Guatemala (founded c. 400 B.C.E.), and Nixtun-Ch’ich’, in the Peten, Guatemala (peaked between 800 and 500 B.C.E.) (Sharer and Traxler, 2006; Blanton et al., 2022; Pugh et al., 2022). During the subsequent Late-Terminal Preclassic (300 B.C.E.–C.E. 250) and Classic Periods (C.E. 250–950), cities flourished across Mesoamerica at places like Teotihuacan, Tikal, Calakmul, Caracol, Monte Albán, Cacaxtla-Xochitecatl, Chichen Itza, Matacapan, El Tajin, etc. (Blanton et al., 1993; Sharer and Traxler, 2006; Serra Puche and Lazcano Arce, 2012). Beginning about C.E. 700 and continuing to 950, Mesoamerican cities experienced a tumultuous period. Teotihuacan erupted in civil strife and collapsed, Cacaxtla-Xochitecatl experienced significant eruptions from Popocatepetl and mudslides off Iztaccihuatl resulting in an exodus, and in the Maya lowlands, the urban centers, which had grown during the Early and Late Classic (e.g., Calakmul, Tikal, Caracol, etc.), experienced profound sociopolitical and economic changes generated variously by internal conflicts among elites, droughts, and/or military confrontations between polities, which caused abandonment, migration, and displacement of the population and the emergence of new regional powers, such as Uxmal and Chichen Itzá in the Terminal Classic (Blanton et al., 1993; Peterson and Haug, 2005; Sharer and Traxler, 2006; Webster et al., 2007; Medina-Elizalde et al., 2010; Serra Puche and Lazcano Arce, 2012; Mumary Farto, 2016). Thus, the period after C.E. 950 until the Spanish conquest (C.E. 1521–1697) saw a reorganization and a new period of urbanism that peaked with the Aztec Triple Alliance capital of Tenochtitlan-Tlatelolco (Blanton et al., 1993).
Pre-Hispanic urbanism and their environmental legacies
Case I: landscape sustainability through the historical legacy of kanan ka’ax in the Maya lowlands
Background on Kanan Ka’ax
Recent research has demonstrated that kanan ka’ax (which roughly translates as taking care of the forest by using it) has a deep history in the Maya lowlands. Starting as early as the Preclassic (1500–250 B.C.E.) and even possibly during the Archaic Period (8000–1500 B.C.E.), experience and experimentation with cultivation, forestry, soil, and hydrologic management served as the basis for refining the complex itinerant agroforestry system of the Classic Period, known as the “Maya Forest-Garden” (Ford and Nigh, 2015). The Maya Forest-Garden is a sophisticated and intensive (in labor and yield) agricultural and silvicultural management system that begins with the generation of space for planting annual crops of maize, beans, and squash in association with up to 60 other crop species and up to 90 varieties, a Mesoamerican polyculture known as milpa maya or Ich Kool (Hernández Xolocotzi et al., 1995; Rodríguez-Robayo et al., 2020). During the first phase of the Maya Forest-Garden, two practices take place that are of utmost importance to guarantee the eventual environmental sustainability of landscape management: 1) walking the bush (kimbal ka’ax) for the highly specialized selection of sites suitable for initiating a cycle of agroforestry succession; and 2) sophisticated burning by the Yum Ik’Ob (wind tenders) in charge of clearing the forest through a level, low-temperature burn that brings nutrients to the soil and favors the conservation of seeds in the substrate, as well as vegetative reproduction (Nigh and Diemont, 2013; Rivera-Núñez et al., 2020). After milpa cultivation, there are seven additional phases of successive vegetation strata that transition from mixed shrub and tree combinations to old-growth forests because of the competition generated by previous human selection. This process culminates in a forest garden dominated by harvestable species or a functional oligarchic meta-landscape (Ford and Nigh, 2015). The seven phases of vegetative succession, with active utilization of species for food, medicine, utensils, and bioconstruction materials for dwellings, are as follows (Gómez-Pompa, 1987; cf. González-Cruz et al., 2014:63–65):
Sak’aab
Includes the first three years after milpa. It is characterized by low fertility soils with a minimum amount of leaf litter and a predominance of bushes or weeds. This successional stage is characterized by a high presence of Viguiera dentata.
Sak’aab hubche’
Tropical forest from 3 to 5 years old. Vines are established, leaf litter accumulates, and soil recovery begins. V. dentata remains the dominant species.
Hubche’
Tropical forest from 5 to 8 years old. This stage marks the first dramatic change in the plant community due to high mortality of grasses and seedlings from the Sak’aab and Sak’aab hubche’. Sabal mexicana and Brosimum alicastrum are the dominant species.
Ka’anal hubche’
Tropical forest from 8 to 15 years old. It is low forest with a dominance of small trees and a notable decrease in the number of vines. Lysiloma latisiliquum and B. simaruba are dominant.
Kelenche’
Tropical forest from 16 to 30 years old. This is equivalent to a high forest site, where the canopy is approximately 10 m high. The vegetation community is mostly composed of trees. M. zapota and Enterolobium ciclocarpum are the dominant species.
Suhuy ka’ax
Refers to a 30- to 50-year-old tropical forest. It is characterized by the presence of large trees with a canopy greater than 15 m in height. The trees present at this stage are mostly timber or useful for firewood. Simaruba glauca is the dominant tree species.
Ka’anal ka’ax
This is an old tropical forest at least 50 years old, from which few resources are extracted because it is considered sacred and a mosaic for preservation of the continuity of forest processes. Cordia sp., Thrinax radiata, and B. alicastrum are dominant.
What is interesting in terms of environmental sustainability is that, over the centuries, the Maya have managed to combine an agricultural strategy that balances supplying their livelihood needs with the recovery of forest cover and maintenance of the environmental services provided by tropical forests (Fedick, 2010). Ethnobotanical studies in the Maya Forest-Garden area have reported that approximately 90% of the plant species are harvested in some way by farm families (Allen et al., 2003; Ford and Nigh, 2015). In addition, in areas of great subsistence, wildlife populations are maintained in a good state of conservation. For example, there are annual reports of the presence of jaguar (Panthera onca), puma (Puma concolor), ocelot (Leopardus siedii), Mesoamerican tapir (Tapirus bairdii), spider monkey (Ateles georffroyi), mantled saraguato (Alouatta pigra), pecari (Pecari tajacu), white-tailed deer (Odocoileus virginianus), and marsh crocodile (Crocodylus moreletti).
Landscape sustainability in Otoch Ma’ax Yetel Kooh-Coba
Otoch Ma’ax Yetel Kooh (OMYK—House of the Monkey and the Jaguar, in Yucatec Mayan) is a Natural Protected Area of just over 5,000 hectares located in the northeast of the Yucatan Peninsula, 18 kilometers from Coba’s pre-Hispanic ceremonial core. In 2002, OMYK was decreed by Mexico’s federal government as an area of flora and fauna protection due to its high biodiversity in a reduced geographic space, as well as for its good state of environmental sustainability. Because of its proximity to one of the largest pre-Hispanic cities built in the northern Maya lowlands, since 2012 Rivera-Núñez and Fargher-Navarro have conducted research in ecological anthropology and ethnohistory to test the hypothesis that this enormous biological wealth and excellent state of environmental sustainability are, to a large extent, a product of the pre-Hispanic legacy of Maya management and not only the result of a biophysical condition per se, as the dominant currents of environmental conservation argue. As we will explain in the following lines, our hypothesis turned out to be accurate and can be explained through a Maya environmental management ethos called kanan ka’ax. An ethos that is still in force in the area, as well as in the regions of the Yucatan Peninsula, which are still strongly Maya in cultural terms.
OMYK was occupied with some fluctuations from the Preclassic to the Late Postclassic period (2500 B.C.E.–C.E. 1500) (Kurnick et al., 2023). The 3,000 years of occupation have been dated through platforms, stelae, ceramic fragments, and funerary remains (Benavides and Zapata, 1991). According to dating, OMYK is older than Coba, but it became part of this city in the Late Terminal Classic (C.E. 730–1000) when Coba reached an estimated 50,000 inhabitants and covered 80 km2 (Folan et al., 2009). Following a downturn in the Early Postclassic (C.E. 1000–1300), Coba recovered during the Late Postclassic (C.E. 1300–1500), and OMYK was again brought into its orbit (Folan et al., 2009). Accordingly, OMYK is a residential and commercial area that was incorporated into Coba’s administrative domain (Folan et al., 2009).
Research suggests that the initial occupation at OMYK may have corresponded to the Maya settlement trend associated with areas known as bajos (Dunning et al., 2002). Bajos are periodically flooded grasslands located between 1 and 20 m above sea level and are usually located near lagoons; therefore, their soils are seasonally saturated by water inflows (Dunning et al., 2002). These areas were initially occupied during the Preclassic period to generate flood-recession agricultural fields by altering the water levels. During subsequent use in Preclassic and Classic, sedimentation of these environments through point deforestation and (highly controlled) erosion, as well as by redepositing more fertile sediments from other types of substrates, converted these areas into highly productive agroforestry landscapes (Beach et al., 2006). Instead of finding soils that correspond to those expected for humid tropical environments in OMYK (e.g., Acrisols-Lixisols), we find highly fertile anthroposols for agricultural purposes. They are characterized by black, brown, and red humus-carbonate strata that are identified by the Maya ethnoclassification of soils as ek lu’um, chac lu’um, and kankab, respectively (Barrera-Bassols and Toledo, 2005; Rivera-Núñez et al., 2020).
By ethnographically elucidating old-growth landscape mosaics (ka’anal ka’ax) with OMYK elders, we uncovered a profound Maya knowledge system of tropical forest management that goes well beyond vegetative succession, including management knowledge related to relief types, soils, and foliage, which is of particular importance to our interest in historical ecology and the management history of landscape mosaics (Table 1). They told us about the ka’kab ka’ax (ancestor´s forest), characterized by the presence of miniature temples, which served as small shrines for the veneration of agricultural deities related to fertility and water, nearby cenotes (collapsed water-filled dolines) with funerary remains, and forests densely dominated by long-lived Ramon trees (B. alicastrum) (Figure 3). For the elders of Maya communities, the ka’kab ka’ax are a reminder that they, as heirs of the ancient Maya, must maintain the legacy of kanan ka’ax (taking care of the forest) because, as a key collaborator explained, “we are not separated from the forest, we are part of the forest, from the forest we get everything to live and that is why we must take care of it so that it remains in order with its Yum Ka’ax (owners and lords).” Hence, they maintain an agricultural ceremonial calendar where they ask for permission to plant the milpa, for subsistence hunting, praying for rain, giving thanks for the first harvests, and organize complex community rituals to re-establish order in the forest.
Delving deeper into ecolinguistic terms regarding the meaning of the word ka’ax (colloquially translated as forest), the Cordemex Maya Dictionary does not define it simply as a forest, but as a space where agricultural crops, groves, dwellings, and jungles intermingle. This, together with the cosmology that the ka’ax has owners and lords, indicates land-sharing type management models, on the one hand, and the domain of relational ontologies, on the other. Based on relational ontologies, the concept of “nature” is a culturally minority notion that represents a Western belief with a strong Christian (Edenic) imprint in which society is separated from the environment (Denevan, 1992; Gambon and Bottazzi, 2021). In contrast, many indigenous peoples perceive relational webs between humans and non-humans that must be kept in balance to achieve profound sustainability of life (De la Cadena, 2015). Therefore, many indigenous peoples welcome inclusive conservation proposals where the use of the environment is integrated with its preservation (land sharing). In contrast, they reject agendas that seek forest transition by denying their livelihoods through land-sparing conservation schemes (Perfecto et al., 2019).
In synthesis, the historical ecology approach allowed us to elucidate that the OMYK, which is a natural area far from urban occupations, is a landscape palimpsest with more than four thousand years of anthropogenic intervention that came to form a residential and commercial part of the Maya city of Coba. In this sense, the environmental sustainability that today is appreciated and valued in this area is defined, to a great extent, by the historical legacy (longue durée) of kanan ka’ax: a Maya ethos that is passed on from generation to generation, to take care of the forest by using and venerating it, not by imposing green enclosures. Kanan ka’ax, in addition to an ethic of care, implies a complex system of local knowledge and management practices; through it the people of OMYK have built an oligarchic landscape with high levels of biodiversity, where most of the plant species are useful for Maya families, while wildlife is maintained because excellent levels of forest cover persist.
Landscape sustainability at Kiuic
The archaeological site of Kiuic, located 95 km south of Merida, Yucatan, Mexico, is situated on the eastern frontier of the Bolonchen, a region of cone karst hills and small valleys with comparatively deep soils located along the Campeche-Yucatan border. The site was originally occupied during the Middle Preclassic (circa 800 B.C.E.) and reached its peak during the Terminal Classic (C.E. 700–950) (Simms et al., 2012). The city of Kiuic in the Terminal Classic was the capital of an important Maya polity and was a major center (Simms et al., 2012). The urban core covers approximately 25 ha and is surrounded by a suburban zone that may have been approximately 290 ha in area (estimate based on the length of the PARB LiDAR transect of the suburban zone) (see May Ciau, 2018). Assuming population densities of 50 people/ha in the urban core and 15–20/ha in the suburban zone, a moderate estimate of population would place the figure at 5,500–7,000. Importantly, a large portion of the site was never reoccupied following abandonment between approximately C.E. 950 and 1000. During the Postclassic, individuals returned to the site to perform rituals in the ruins, but archaeological evidence suggests that these were temporary visits (May Ciau, 2018). During the Colonial Period, a ranch (Rancho Kiuic) was established south of the urban core and lightly occupied until Kiuic became a private reserve in 1998 (https://www.kaxilkiuic.org.mx/9eserve-biocultural-kaxil-kiuic/). Thus, the site has only seen minor reuse since its abandonment.
To understand the legacy forest created by the pre-Hispanic occupation of Kiuic, May Ciau (2018) used the PARB LiDAR transect to study an area 2.5 km x 0.5 km extending west from the urban core. She divided this zone into 3,115 20 m x 20 m sampling quadrants organized into three strata (urban, suburban, and rural) and randomly selected 46 quadrants for analysis (20 rural, 17 suburban, and 9 urban) (May Ciau, 2018). She also intentionally sampled 28 additional quadrants (seven rural, 14 suburban, and seven urban) to ensure coverage of archaeological features by vegetation (May Ciau, 2018). Within each quadrant, she identified and mapped all soil types as well as all trees larger than 6.36 cm in diameter and 1.3 m in height (May Ciau, 2018). This sampling strategy resulted in the identification of 2,902 trees at the genus or species level in 74 quadrants (May Ciau, 2018).
May Ciau’s (2018) results demonstrate that the archaeological site is covered with multiple oligarchical forests, instead of a homogenous “natural” forest (cf., Table 1). These forests are differentially distributed across the site zones (urban, suburban, and rural) and include diverse communities within these zones (the results are summarized from May Ciau, 2018). In the rural area, Principal Components Analysis (PCA) showed that 14 species explain 46.26% of the variation in species composition and Factor Analysis (FA) identified two communities, consisting of chunlok, kaa poch, k’ann chunuup, and tsalam in one and iik che’, k’annan chunuup, tsalam, and xuul in the second. In the suburban area, PCA demonstrated that seven species explained 59.3% of the variation, and FA identified a community dominated by chac pich’ che’, cruz k’iix, kaas kaat, and ya’ax el’. Finally, in the urban area, PCA showed that nine species explain 61.8% of the variation in composition, and FA identified two communities, one dominated by cruz k’iix, ja’abin, k’aan chunuup, and k’i’ik’ che’, and the other by boojum, chakaj, ja’abin, and ya’ax ek’. These results demonstrate that the kanan ka’ax ethos directed landscape management practices during the pre-Hispanic occupation, resulting in intermediate disturbances that produced legacy forests, similar to OMYK, which probably resulted in lower alpha diversity in any specific zone, but increased beta biodiversity within and among the site’s zones.
Landscape sustainability at Lagunas de Yalahau
The archaeological site of Lagunas de Yalahau is located approximately 50 km southeast of Merida, Yucatan, Mexico. A systematic archaeological survey demonstrated that this site was occupied from the Early Classic (C.E. 300–600) through the Late-Terminal Classic (C.E. 600–950) (Antorcha-Pedemonte, 2023). It reached its peak during the Terminal Classic, and the urban core covered approximately 1 km2 (Antorcha-Pedemonte, 2023). This city contained multiple monumental cores and housed an estimated 2,500–5,000 people. Importantly, the site was nestled among a series of aguadas (depression with impermeable soils that form shallow (seasonal) lagoons), including the Yalahau Lagoon, which is the largest body of surface water in the State of Yucatan, Mexico (Antorcha-Pedemonte, 2023). Furthermore, the site contains 18 cenotes.
To study the impact of human occupation on the site’s landscape, Antorcha-Pedemonte (2023) undertook a holistic study that combined systematic archaeological survey, historic archival research, ethnographic interviews, and the collection of unconsolidated sediment cores from the floors of three cenotes (two within the site, Kikil and Oxola, and one located several kilometers beyond the site’s limits in an area that lacks evidence of pre-Hispanic and historic occupations, Ciil). These cores were subjected to isotopic analysis for δ13C and δ18O, and chemical analysis using pXRF (Antorcha-Pedemonte, 2023). These results allow us to track changes in C3 and C4 vegetation, variation in precipitation, and human occupation from aproximately 6,000 years ago up to approximately C.E. 1800. Because the site experienced significant deforestation during the Colonial–Independence period from industrial-scale henequen production, we relied on sediment cores to track environmental changes associated with and following the pre-Hispanic occupation.
The analytical results allow us to track changes in vegetation that result from human disturbance or environmental variation (e.g., changes in precipitation). Here, we used the Kikal core to track changes within the pre-Hispanic city and Ciil to monitor variations in an environment with only minor human modifications. The results of the δ13C, δ18O, and chemical analyses demonstrated that for almost the entire sequence recorded in the Kikal core, which spans the pre-Hispanic urban occupation, δ13C remained between −25‰ and −30‰ (Antorcha-Pedemonte, 2023). These data indicate that the city was heavily forested throughout its occupation and the impact of climatic variation was mitigated by anthropogenic landscape changes. Following a drought around the Middle Classic (circa C.E. 500–600), δ13C values surpassed −24‰ (Antorcha-Pedemonte, 2023), which indicates a transition to a forest-savanna mosaic. Interestingly, during subsequent and more intense droughts during the Terminal Classic and Early Postclassic, δ13C values remained steadily below −25‰ (Antorcha-Pedemonte, 2023). Comparatively, the Ciil core shows strong variation with cycling between forest values (−25‰ and −30‰) and forest-savanna mosaic values (above −24‰) coupled with changes in precipitation (Antorcha-Pedemonte, 2023).
First and foremost, these results indicate that human disturbance associated with the urban occupation at Lagunas de Yalahau produced an anthropogenic landscape that was more resilient to drought cycles, as evidenced by the Kikil core, than the much less impacted forest documented in the Ciil core. Furthermore, the Kikil core demonstrates that despite intense and prolonged drought conditions during the Terminal Classic and Early Postclassic, the site’s forest cover persisted, even after human abandonment. Thus, we suggest that following the Middle Classic drought, the Maya appear to have “built back better” and created a landscape that was more resilient in the face of droughts and persisted as a legacy environment, for several centuries, until deforestation for henequen production destroyed it. Importantly, we note that archaeological surveys demonstrate a large number of landesque modifications to the city’s environment, which would have conserved water and soil humidity (Antorcha-Pedemonte, 2023). These include building terraces along the edges of aguadas that extend out into the water, as well as reservoirs, among others. These features were probably mostly or entirely constructed during the Late-Terminal Classic, based on the archaeological survey results. Thus, we have chemical and isotopic evidence that the intermediate disturbance associated with Late-Terminal Classic urbanism produced a landscape that was more resilient than “natural” forests and demonstrably more sustainable. We also have evidence that the decline of the ethos of kanan ka’ax, with the advent of henequen production and Western ecology, resulted in the decline of the forest (just as our collaborator in OMYK predicted).
Case II: landscape sustainability through the historical legacy of Central Mexican land management
Background on Central Mexican (Nahua and Otomí) landscape management
Indigenous agro-forestry production strategies in Central Mexico are comparable to the ethos of kanan ka’ax. However, they have received less attention from researchers and have been highly influenced by European practices, including the significant incorporation of domesticated livestock. As Mountjoy and Gliessman (1988) pointed out, the mountainous landscape is covered with layers of ashfall that have been transformed into alternating layers of duripan (tepetate) and clay horizons covered with a thin A horizon. Given the topography and climate (with powerful summer rains, especially during hurricanes), A-horizon soils are especially prone to erosion, and once stripped away, the underlying duripan hardens into an impermeable layer that supports only sparse vegetation (Wilken, 1970; Mountjoy and Gliessman, 1988).
Indigenous practices have taken this situation into consideration. At least 3,000 years ago, hillslope management focused on combining soil conservation with water control strategies. This was accomplished through the construction of sloping terraces, channels, and settling tanks (cajetes) (Mountjoy and Gliessman, 1988). These terraces served to maintain soils in place, whereas the channels directed excess rainfall not absorbed by terraces, along with suspended sediment collected during downpours, into cajetes. Thus, cajetes allowed for water to slowly seep downward, reducing its erosive potential and the recovery of soils that could be dug out and scattered over terraces (Mountjoy and Gliessman, 1988). Importantly, successful cajete/terrace systems require the coordinated effort of many farmers working individually to transform entire hillsides because piecemeal applications increase the destructive capacity of rainfall-driven erosion (Mountjoy and Gliessman, 1988). Soil and water control, fertility, and productivity of terraces are maintained through intercropping, crop rotation, fallowing, management of permanent terrace edge vegetation, and forest patches (Mountjoy and Gliessman, 1988). Field-edge vegetation includes agaves, nopal cactus, fruit trees, and a variety of shrubs and other annuals (Wilken, 1970; Mountjoy and Gliessman, 1988). Wetland raised field-edge vegetation is also cultivated on valley bottoms (Anaya et al., 1987). The most important plants are alders (Alnus firmifolia), lesser waterparsnip (Berula erecta), rushes (Juncus sp.), and Douglas falsewillow (Baccharis glutinosa) (Anaya et al., 1987). Alder leaves, lesser waterparsnips, and rush leaves are used as green manure to control weeds (Anaya et al., 1987). Thus, intercropping, management of annuals along field edges, and forests increase diversity and promote allelopathy, thereby producing sustainable agro-forestry landscapes (Anaya et al., 1987).
Landscape sustainability at Tlaxcallan
Fargher-Navarro directed a long-term mapping and excavation project at the Late Postclassic city of Tlaxcallan, located 100 km east of Mexico City, from 2007 to 2018 (results below are partially summarized from Fargher et al., 2010; Fargher et al., 2011a; Fargher et al., 2011b; Fargher et al., 2016; Fargher et al., 2020; Fargher et al., 2022, but include unpublished data presented here). Based on a systematic full-coverage archaeological survey and large-scale excavations on nine terraces, this project demonstrated that Tlaxcallan was initially occupied between approximately C.E. 1250 and 1300 based on radiocarbon dates. The city rapidly expanded over the next 150 years to become the largest and most important city in Mexico’s Tlaxcala-Puebla Valley. At its peak, the city covered 450 ha and housed somewhere between 30,000 and 40,000 people. In 1519, Cortés and the Conquistadores first visited the city and then lived there through 1520 and 1521, while they prepared for the siege of Aztec Tenochtitlan-Tlatelolco. Importantly, the city spread across a series of hillslopes and hilltops. This forced Tlaxcaltecas to build stone-faced residential terraces, as well as numerous plazas and temples with retaining walls and terraces and a network of paved roads with stairways, to transform this sloped landscape into a functional urban environment.
During the systematic survey, dense concentrations of Late Postclassic diagnostic ceramics in plow zone horizons, as well as modern road cuts, pits, and stripping for construction that exposed buried stratigraphy, confirmed that the city housed more than 1,000 terraces, roads, plazas, and platforms at its peak. The stratigraphy of every systematically excavated terrace demonstrates that cultural deposits sit directly on bare duripan (bedrock) (Figure 4). Furthermore, soil was so scarce at the outset of occupation that elevations of the same duripan surfaces had to be laboriously pecked away, and the debris ground to create sediment that could be used to fill in depressions in the surface to produce flat living surfaces behind low terrace walls. Once occupation began, organics (e.g., food waste) and debris, such as pottery, stone tools, and other artifacts, produced by households were mixed with ground duripans and possibly sediments brought to the site to create terrace fill, as households remolded and expanded their residential lots. Thus, by the end of the city’s occupation in the early 16th century (shortly after the Spanish Conquest), Tlaxcaltecas had created an entirely new landscape with patches of comparatively deep soils (1–2 m or more) covering anywhere from approximately 1,000 m2 to 12,500 m2 that could be colonized by diverse forms of vegetation (Figures 4A–C). Today, these archaeological features are covered with dense vegetation, including diverse tree species, grasses, wildflowers, agaves, and shrubs (Figures 4D, E). Where these terraces have been brought under cultivation in the present, we see evidence of field-edge vegetation management, fallowing, and maintenance of forest patches to conserve fertility and productivity (cf., Mountjoy and Gliessman, 1988). The areas within the site and along its boundaries that were not modified for human occupation continued to be nearly barren bedrock hillsides, covered only by dispersed shrubby vegetation and a few trees (Figure 4F). The comparison of cultural deposits with adjacent natural hillslopes demonstrates that intermediate scale disturbances generated by Tlaxcallan’s urban occupation, in this case, has resulted in greater biodiversity and biomass. Specifically, the humanly mitigated disturbances significantly increased alpha biodiversity, beta biodiversity (considering both terraced and natural hillslopes), and gamma diversity.
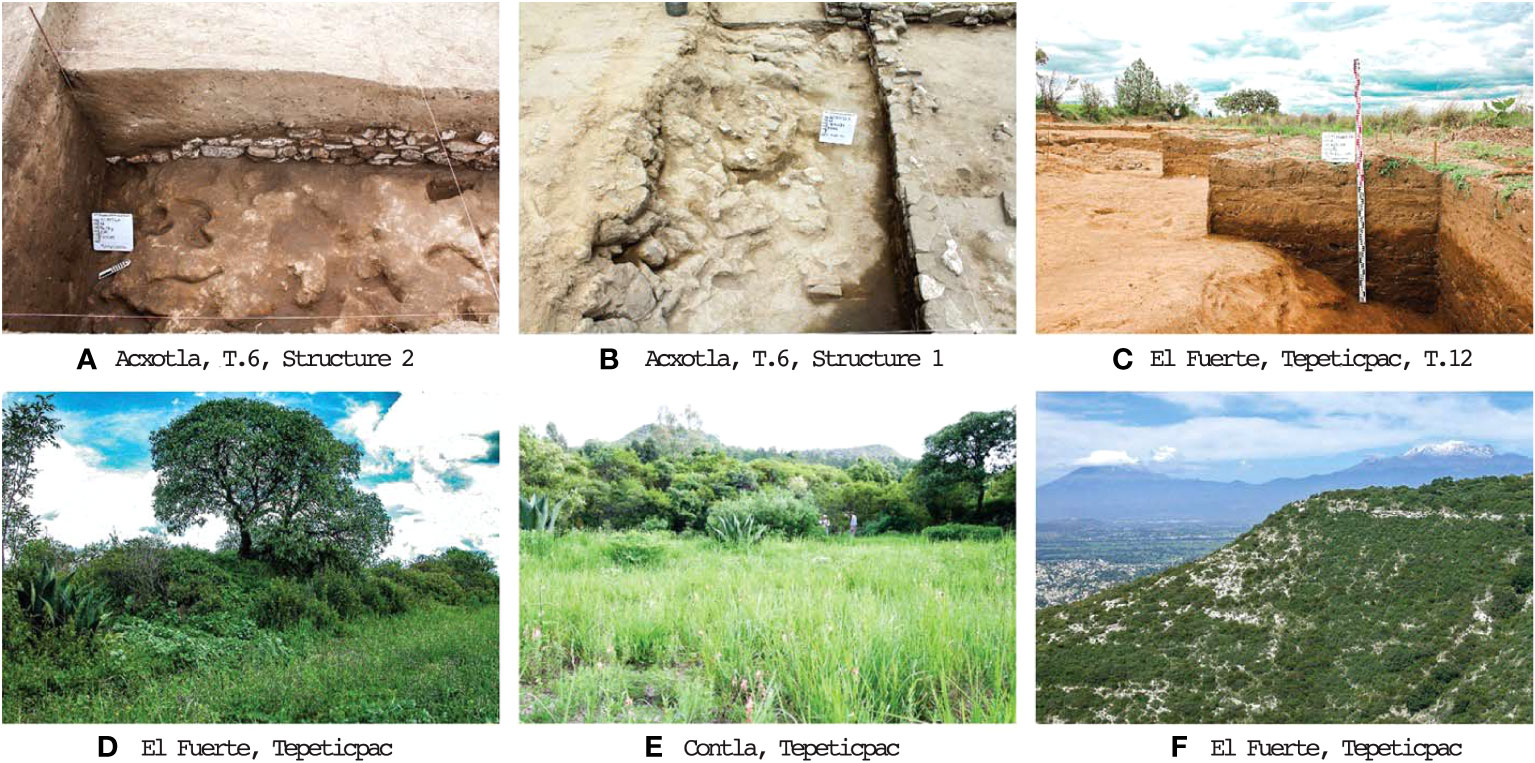
Figure 4 Archaeological excavations showing cultural deposits directly on duripan bedrock (A, B), depth of cultural deposits on duripan bedrock (C), diversity and biomass of vegetation on terraces (D, E), diversity and biomass of vegetation on “natural” hillslopes (F).
Discussion
Lowland Maya cities and their forest-gardens
The analyzed cases of OMYK (Coba), Kiuic, and Lagunas de Yalahau are evident examples of that both small and large Maya cities that flourished during the Preclassic-Classic in the northern Maya lowlands formed what in archaeological and historical ecology research is known as forest-garden cities (Ford and Nigh, 2015; Crumley, 2017). Forest-gardens are forests domesticated by local populations to satisfy their livelihoods while safeguarding forest resources. They feature interweaving of different gradients of crops and enrichment of forest units with remnants of mature forest in an agroforestry sequence under successive management that continuously transforms the landscape configuration from one mosaic to another (Wiersum, 2004). Within the classification of agroforestry systems, forest-gardens are often understood as intermediate systems, as they are precisely at the interface between the natural forest and specialized tree crop plantations (Angelsen et al., 2000). Furthermore, from an anthropological point of view, forest-gardens are management schemes that perfectly embody land-use systems in which a culture-nature continuum exists (Wartman et al., 2018). Examples of forest-gardens have been studied mainly in Papua New Guinea (Bourke, 1985), Indonesia (Michon and De Foresta, 1999; Mulyoutami et al., 2009), Thailand (Withrow-Robinson et al., 1998), Brazil (Denevan et al., 1984; Posey, 1985; Balée et al., 2020), Ecuador (Balée et al., 2023), Bolivia (Erickson, 2000), and Mexico (Gómez-Pompa and Kaus, 1990; Ford and Nigh, 2015).
The main intermediate scale “disturbances,” employed by the ancient Maya to build forest-gardens within the administrative boundaries of their cities, where residential patio groups were located (Arnauld et al., 2012), resulted in: a) the translocation and enrichment of anthropogenic soils (Nigh and Diemont, 2013); b) sophisticated uses of fire for agricultural purposes (Nigh and Diemont, 2013); c) complex management of forest succession and fallow (Fedick, 1996); d) enrichment of forest and agricultural units such as the Pet kot with fruit trees or the management of milpa borders with tolche for protection from environmental disturbances (Gómez-Pompa, 1987; Levy-Tacher et al., 2019); and e) the management of landscape topography for the regulation of water permeability levels, drainage, and water storage through rejolladas, aguadas, and akalches (Gunn et al., 2002; Reese-Taylor et al., 2016). Such intermediate disturbances have been shaped over centuries or thousands of years (longue durée) in such a way that they still bear their mark on landscape palimpsests (Rivera-Núñez and Fargher, 2021; Antorcha-Pedemonte, 2023), as evidenced by the contemporary identification of an ancestral forest landscape unit (ka’kab ka’ax). Similarly, environmental management ethics such as kanan ka’ax (taking care of the forest by using it) are often considered a pre-Hispanic historical legacy by contemporary Maya communities (Rivera-Núñez et al., 2020).
As a result, the Maya forest-garden is an oligarchic forest dominated by food, medicinal, and bioconstruction material species for both ancient and contemporary Maya families (Ford, 2022). However, despite transforming the structural composition of the forest (to an intermediate level), the science behind the historical processes of forest-garden construction lies in achieving multi-stratum cropping systems that mimic the function of natural forests while maintaining their ecological integrity. The maintenance of ecological integrity takes place through a series of synergistic processes that are safeguarded or enhanced by local populations, such as canopy management, nutrient recirculation, pest and disease management, and protection against extreme hydrometeorological events through vegetation barriers, as well as the safeguarding of strategic habitats for wildlife (Van Noordwijk et al., 1997). Thus, some Maya cities created second natural landscapes that managed to balance the conservation of biodiversity and continuity of ecosystem services with the production and provisioning of basic human needs.
Nahua and Otomí cities of Central Mexico and their environmental management
Like the Maya ethos of kanan ka’ax, indigenous peoples of Central Mexico developed an understanding that humans are integral parts of the environment, and that by working with it, they could produce what they needed to survive, as well as maintain ecological services. However, the specifics of these strategies vary owing to topography and climate. First, like Maya, they managed the abiotic environment through a complex understanding of the relationships among topography, soils, and hydrology. On hillslopes, they used terraces, ditches, and cajetes (settling tanks) to conserve soils, enhance them, and increase the absorption of water to increase soil humidity, while mitigating the erosive capacity of intense summer deluges. In lowland swampy areas, they used ditches, canals, drains, and land reclamation to increase and direct environmental productivity. Second, over these landesque modifications, they created a patchwork of juxtaposed environmental niches that placed cultivated annuals (like maize, beans, squash, and chili pepper, etc.) adjacent to allelopathic plants, including trees, shrubs, grasses, and flowers, that enhanced agricultural production, controlled harmful weed growth and insects, and increased sustainability.
In the case of the Late Postclassic Tlaxcallan, Nahua and Otomí ethnoecology, developed during the Formative and Classic Periods, was deployed to build a city on baren hillslopes. Terraces were constructed through soil reclamation, creation of anthropogenic sediments from the bedrock itself and mixing with organic debris and artifacts. Over approximately 150 years of urban occupation at Tlaxcallan, terrace construction strategies transformed the landscape from a sparsely vegetated zone to a second nature with a patchwork of deep, rich soils that were first residential spaces and then became beds for colonizing vegetation following abandonment. Grasses, shrubs, and trees were able to flourish in the legacy landscape until these terraces were recolonized by farmers who employ allelopathic strategies that juxtaposed cultivated annuals with field-edge vegetation to enhance sustainability and help anchor soils. Thus, urban occupation at Tlaxcallan during the pre-Hispanic era combined with contemporary indigenous land-use strategies significantly increased alpha, beta, and gamma biodiversity, as well as biomass, resulting in enhanced resilience and greater sustainability than the “natural” environment that preceded human occupation.
Comparative settlement pattern analysis and environmental management
From a comparative perspective, Central Mexico and the northern Maya lowlands are characterized by distinct environmental characteristics and diverse pre-Hispanic settlement patterns (e.g., compact vs. disperse). Central Mexico, which is a mountainous zone with large plateaus, offers a diverse range of natural resources, such as coniferous forests, pediment zones populated by shrubby vegetation, cacti, and succulents, and wide alluvial plains planted with annual crops, grasses, shrubs, and trees. Due to comparatively lower temperatures in the highlands, biotic growth is slower; however, plants take advantage of deep-rich soils that accumulate nutrients and store them. Landscape building strategies from the Middle-Late Formative through the Epi-Classic emphasized a strong distinction between compact urban city-states and comparatively lowly populated rural agricultural areas (Sanders et al., 1979; Drennan, 1988). During the Postclassic, archaeological research has revealed that densely populated city -states were surrounded by a densely settled rural landscape characterized by dispersed settlements consisting of small villages and hamlets (Sanders et al., 1979; Drennan, 1988).
In all periods, alluvial zones and low piedmonts were cultivated for extended periods punctuated by brief (2–3 year) fallow periods, whereas steeper piedmont zones were used intermittently or terraced for more permanent use, and finally forests were maintained in high piedmont and mountain zones. With the transition to the Postclassic, farmers settled closer to their fields to reduce travel time to increase laboresque investment and, thereby, augment output (Drennan, 1988; Fargher, 2009). In the case of the urban occupation at Tlaxcallan, city residents settled in middle-piedmont zones with steep hillsides that necessitated the construction of terraces. While the occupation was urban, the accumulation of sediments and organic material (natural capital) behind terrace walls during the life of the city created comparatively deep (1–2 m) nutrient-rich deposits that could be rapidly colonized by various species, including grasses, succulents, cacti, shrubs, and trees, following human abandonment. The source of these colonizers was most likely plants living along the edges of terraces as well as on neighboring hillslopes. Growth would have been enhanced if abandoned terraces were incorporated into new land-use strategies focused on forest management and cultivation.
In contrast, the northern Maya lowlands were characterized by semi-deciduous forests and cenotes that were utilized through landscape strategies (e.g., kanan ka’ax), featuring more dispersed settlement patterns from the Formative through the Postclassic (Andrews and Castellanos, 2004). This dispersed pattern capitalized on the availability of water in scattered cenotes, the local terrain, and rapid growth/succession that stores nutrients (natural capital) above ground in plants. Exploiting this natural capital, the Maya focused primarily on forest polyculture strategies that directed rapid succession into a series of cultivated patches, each with its diverse suite of plants, from cultivated annuals (such as maize, beans, chili pepper), rapidly growing trees and comestible shrubs (e.g., papaya), and long-lived tree species (e.g., chaca, zapote, etc.). This included a diversity of managed parcels in different phases of succession in outfield areas, and more permanent cultivation and occupation in houselots. Based on ethnographic observations, home gardens feature up to 400 plant species for medicinal, culinary, and ornamental use, which serve for daily use and consumption, highlighting the importance of these types of gardens in urban zones (Moreno-Calles et al., 2013).
Conclusions
Examples of environmental management in the ancient cities of the northern Maya lowlands, as well as the Nahua and Otomi of Central Mexico, presented here, are clear examples of nature-based urban planning. Without essentializing or extrapolating to all Native American urbanism and environmental management strategies and not exempting them from the inherent human dynamics of flourishing and stagnation, our focal cities located in contrasting regions (tropical and temperate) managed to live for hundreds or even thousands of years. Moreover, despite the multiple socio-political reorganizations experienced by their ancient populations, the historical legacies (longue durée) of the environmental management of these pre-Hispanic cities still prevail over the landscape (palimpsests) and continue to be practiced and appreciated by contemporary inhabitants in agroforestry management systems, ecological preservation ethics, and hydraulic and hillside agricultural management practices. In this sense, such cities can be considered truly longitudinal examples of resilience and environmental sustainability. Despite structural shocks and profound reorganization, they still endure in essence and continue to show relative capacity to meet the needs of current and future generations without undermining their ecological base.
The historical cases presented in this article permeate three major lessons for thinking about urban models beyond the Western hegemonies to which our contemporary cities respond. The first relates to the understanding that urban deployment should not be inexorably opposed to natural spaces, but that cities can be intertwined with functional forest corridors, as in the case of forest-garden cities. Second, and closely related to the first point, is the fact that cities can go from being passive to active environments to satisfy their subsistence needs, mainly food, by activating an entire platform of urban, and especially peri-urban, agriculture at the points of intersection among cities, rural landscapes, and forests. Third, and encompassing the previous two lessons, is the understanding of cities as human ecosystems or better yet as historical landscapes in which people and biodiversity can coexist and thrive together, which ecologist Michael Rosenzweig (2003) calls “ecologies of reconciliation.” Thus, some cities in the past are full of messages for the urban socio-ecological future.
Data availability statement
The original contributions presented in the study are includedin the article/supplementary materials, further inquiries can bedirected to the corresponding author/s.
Author contributions
RA-P co-developed the concept for the article, collected data, interpreted data, and co-wrote, and revised the draft. TR-N co-developed the concept for the article, collected data, interpreted data, and co-wrote the draft. LF-N co-developed the concept, collected data, interpreted data, co-wrote, and edited the draft. LF-N obtained funding for the Tlaxcallan project and RA-P and LF-N obtained funding for the Lagunas de Yalahau project. All authors contributed to the article and approved the submitted version.
Funding
Funding for the survey and excavations at Tlaxcallan was provided by the National Science Foundation (Grant # BCS 0809643 and BCS 1450630, Conacyt; Grant # CB-2014-236004), the National Geographic Society, and FAMSI, Inc. (Grant #06082). Funding for the survey and collection of sediment cores at Lagunas de Yalahau was provided by Secretaria de Educación Pública-Cinvestav (Grant #165). RA-P, TR-N, and Rossana May Ciau received funding for graduate studies from Mexico’s Conacyt.
Acknowledgments
We thank Abigail Derby Lewis, Attila Gyucha, Natalia C. Piland, Rodrigo Solinis-Casparius, and Marina Alberti for inviting us to participate in the research topic: Shaping the Future: Urban Resilience and Socio-Ecological Systems Through Time. We thank the two reviewers for their comments, which helped to improve the manuscript.
Conflict of interest
The authors declare that the research was conducted in the absence of any commercial or financial relationships that could be construed as a potential conflict of interest.
Publisher’s note
All claims expressed in this article are solely those of the authors and do not necessarily represent those of their affiliated organizations, or those of the publisher, the editors and the reviewers. Any product that may be evaluated in this article, or claim that may be made by its manufacturer, is not guaranteed or endorsed by the publisher.
References
Allen M. F., Gómez-Pompa A., Fedick S. L., Jiménez-Osornio J. J. (2003). “The Maya Lowlands: a case study for the future? Conclusions,” in The lowland Maya area: Three millennia at the human-wildland interface. Eds. Gómez-Pompa A., Allen M. F., Fedick S. L., Jimenez-Osornio J. J. (New York: Food Products Press), 623–634.
Anaya A. L., Ramos L., Cruz R., Hernández J. G., Nava V. (1987). Perspectives on allelopathy in Mexican traditional agroecosystems: a case study in Tlaxcala. J. @ Chem. Eco. 13, 2083–2101. doi: 10.1007/BF01012873
Andermann T., Antonelli A., Barrett R. L., Silvestro D. (2022). Estimating alpha, beta, and gamma diversity through deep learning. Front. Plant Sci. 13. doi: 10.3389/fpls.2022.839407
Andrews A. P., Castellanos F. R. (2004). An archaeological survey of northwest Yucatán, Mexico. Mexicon 26 (1), 7–14.
Angelsen A., Asbjørnsen H., Belcher B., Michon G., RuizPereze M. (2000). “Cultivating (in) Tropical Forests? The evolution and sustainability of intermediate systems between extractivism and plantations,” in International workshop cultivating (in) tropical forests (Lofoten, Norway: Kraemmervika), 9–18.
Antorcha-Pedemonte ,.R. R. (2023). La Antropización del Paisaje en El Parque Estatal Lagunas de Yalahau. Un estudio histoírico-holiístico. [Unpublished PhD disseration] (Mérida (Yuc: Departamento de Ecología Humana. Centro de Investigación y de Estudios Avanzados del IPN-Unidad Mérida).
Armstrong C. G., Shoemaker A. C., McKechnie I., Ekblom A., Szabó P., Lane P. J., et al. (2017). Anthropological contributions to historical ecology: 50 questions, infinite prospects. PloS One 12(2), e0171883 doi: 10.1371/journal.pone.0171883
Arnauld M. C., Manzanilla L. R., Smith M. E. (Eds.) (2012). The neighborhood as a social and spatial unit in mesoamerican cities (Tucson: University of Arizona Press).
Balée W. (2006). The research program of historical ecology. Ann. Rev. Anthro. 35, 75–98. doi: 10.1146/annurev.anthro.35.081705.123231
Balée W., Honorato de Oliveira V., dos Santos R., Amaral M., Rocha B., Guerrero N., et al. (2020). Ancient transformation, current conservation: Traditional forest management on the Iriri River, Brazilian Amazonia. Hum. Eco. 48, 1–15. doi: 10.1007/s10745-020-00139-3
Balée W., Swanson T., Zurita-Benavides M. G., Macedo J. C. R. (2023). Evidence for landscape transformation of ridgetop forests in amazonian Ecuador. Lat. Am. Ant. doi: 10.1017/laq.2022.94
Barrera-Bassols N., Toledo V. M. (2005). Ethnoecology of the Yucatec Maya: symbolism, knowledge and management of natural resources. J. Lat. Am. Geo. 4 (1), 9–41. doi: 10.1353/lag.2005.0021
Beach T., Dunning N., Luzzadder-Beach S., Cook D. E., Lohse J. (2006). Impacts of the ancient Maya on soils and soil erosion in the central Maya Lowlands. Catena 65 (2), 166–178. doi: 10.1016/j.catena.2005.11.007
Benavides A., Zapata R. L. (1991). Punta Laguna: un sitio prehispánico de Quintana Roo. Est. Cult. Maya. 18, 23–64. doi: 10.19130/iifl.ecm.1991.18.172
Blanton R. E., Feinman G. M., Kowalewski S. A., Nicholas L. M. (2022). Ancient oaxaca (Cambridge: Cambridge University Press).
Blanton R. E., Kowalewski S. A., Feinman G. M., Finsten L. M. (1993). Ancient mesoamerica: A comparison of change in three regions (Cambridge: Cambridge University Press).
Bourke R. M. (1985). Food, coffee and casuarina: an agroforestry system from the Papua New Guinea highlands. Agrofor. Sys. 2, 273–279. doi: 10.1007/BF00147038
Carballo D. M., Feinman G. M., López Corral A. (2022). Mesoamerican urbanism: Indigenous institutions, infrastructure, and resilience. Urban. Stud. doi: 10.1177/00420980221105418
Connell J. H. (1978). Diversity in tropical rain forests and coral reefs. Sci. 199, 1302–1310. doi: 10.1126/science.199.4335.1302
Costanza R., Patten B. C. (1995). Defining and predicting sustainability. Eco. Econ. 15 (3), 193–196. doi: 10.1016/0921-8009(95)00048-8
Crumley C. L. (2017). Historical ecology and the study of landscape. Land. Res. 42 (sup1), S65–S73. doi: 10.1080/01426397.2017.1399994
Crumley C. L. (2022). “Historical ecology for the past and the future: organizing at local and regional scales,” in Historical ecology: learning from the past to understand the present and forecast the future of ecosystems, coord. Guillaume decocq (Hoboken: Wiley), 285–294.
Daly H. E. (1990). Toward some operational principles of sustainable development. Eco. Econ. 2 (1), 1–6. doi: 10.1016/0921-8009(90)90010-R
De la Cadena M. (2015). Earth beings: ecologies of practice across andean worlds (Durham: Duke University Press).
Denevan W. M. (1992). The pristine myth: the landscape of the Americas in 1492. Ann. Assoc. Am. Geo. 82 (3), 369–385. doi: 10.1111/j.1467-8306.1992.tb01965.x
Denevan W. M., Treacy J. M., Alcorn J. B., Padoch C., Denslow J., Paitan S. F. (1984). Indigenous agroforestry in the Peruvian Amazon: Bora Indian management of swidden fallows. Intercien. 9, 346–357.
Drennan R. D. (1988). “Household location and compact versus dispersed settlement in prehispanic Mesoamerica,” in Household and community in the mesoamerican past. Eds. Wilk R. R., Ashmore W. (Albuquerque: University of New Mexico Press), 273–293.
Dunning N. P., Luzzadder-Beach S., Beach T., Jones J. G., Scarborough V., Culbert T. P. (2002). Arising from the bajos: The evolution of a neotropical landscape and the rise of Maya civilization. Ann. Assoc. Am. 92 (2), 267–283. doi: 10.1111/1467-8306.00290
Erickson C. L. (2000). An artificial landscape-scale fishery in the Bolivian Amazon. Nat. 408 (6809), 190–193. doi: 10.1038/35041555
Fargher L. F. (2009). A comparison of the spatial distribution of agriculture and craft specialization in five state-level societies. J. Anthro. Res. 65 (3), 353–387. doi: 10.3998/jar.0521004.0065.301
Fargher L. F., Antorcha-Pedemonte R. R., Heredia Espinoza V. Y., Blanton R. E., López Corral A., Cook R. A., et al. (2020). Wealth inequality, social stratification, and the built environment in Late Prehispanic Highland Mexico: A comparative analysis with special emphasis on Tlaxcallan. J. Anthro. Arch. 58, 101176. doi: 10.1016/j.jaa.2020.101176
Fargher L. F., Blanton R. E., Heredia Espinoza V. Y. (2010). Egalitarian ideology and political power in prehispanic central Mexico: the case of Tlaxcallan. Lat. Am. Ant. 21 (3), 227–251. doi: 10.7183/1045-6635.21.3.227
Fargher L. F., Blanton R. E., Heredia Espinoza V. Y. (2016). “The independent republic of tlaxcallan,” in The oxford handbook of the aztecs. Eds. Nichols D. L., Rodríquez-Alegría E. (Oxford: Oxford University Press), 535–542.
Fargher L. F., Blanton R. E., Heredia Espinoza V. Y. (2022). Collective action, good government, and democracy in tlaxcallan, Mexico: an analysis based on demokratia. Front. Pol. Sci. 4. doi: 10.3389/fpos.2022.832440
Fargher L. F., Blanton R. E., Heredia Espinoza V. Y., Millhauser J., Xiuhtecutli N., Overholtzer L. (2011a). Tlaxcallan: the archaeology of an ancient republic in the New World. Ant. 85 (327), 172–186. doi: 10.1017/S0003598X0006751X
Fargher L. F., Heredia Espinoza V. Y., Blanton R. E. (2011b). Alternative pathways to power in late Postclassic Highland Mesoamerica. J. Anth. Arch. 30 (3), 306–326. doi: 10.1016/j.jaa.2011.06.001
Fedick S. L. (1996). The managed mosaic: ancient maya agriculture and resource use (Salt Lake City: University of Utah Press).
Fedick S. L. (2010). The Maya Forest: Destroyed or cultivated by the ancient Maya? Proc. Nat. Acad. Sci. 107 (3), 953–954. doi: 10.1073/pnas.0913578107
Feinman G. M., Carballo D. M., Nicholas L. M., Kowalewski S. A. (2023). Sustainability and duration of early central places in prehispanic Mesoamerica. Front. Ecol. Evol. 11. doi: 10.3389/fevo.2023.1076740
Folan W. J., Hernandez A. A., Kintz E. R., Fletcher L. A., Heredia R. G., Hau J. M., et al. (2009). Coba, Quintana Roo, Mexico: a recent analysis of the social, economic and political organization of a major Maya urban center. Ancient. Meso. 20 (1), 59–70. doi: 10.1017/S0956536109000054
Ford A. (2022). Scrutinizing the paleoecological record of the maya forest. Front. Ecol. Evol. 10. doi: 10.10.3389/fevo.2022.868660
Ford A., Nigh R. (2015). The maya forest garden: eight millennia of sustainable cultivation of the tropical woodlands (Routledge: Oxfordshire).
Frank D. A., McNaughton S. J. (1991). Stability increases with diversity in plant communities: empirical evidence from the 1988 Yellowstone drought. Oikos 62 (3), 360–362. doi: 10.2307/3545501
Gambon H., Bottazzi P. (2021). The political ontology of protected area co-management: worlding and nature perceptions among stakeholders. J. Pol. Eco. 28 (1), 646–662. doi: 10.2458/jpe.3026
Giovannoni E., Fabietti G. (2014). “What is sustainability? A review of the concept and its applications,” in Integrated reporting: concepts and cases that redefine corporate accountability. Eds. Busco C., Frigo M. L., Riccaboni A., Quattrone P. (New York: Springer), 21–40.
Gómez-Pompa A. (1987). On maya silviculture. Mex. Stud./Estud. Mex. 3 (1), 1–17. doi: 10.2307/4617029
Gómez-Pompa A., Kaus A. (1990). “Traditional management of tropical forests in Mexico,” in Alternatives to deforestation: steps towards sustainable use of the amazon rain forest. Ed. Anderson A. B. (New York: Columbia University Press), 45–64.
González-Cruz G., García-Frapolli E., Fernández A. C., Rada J. M. D. (2014). Conocimiento tradicional maya sobre la dinámica sucesional de la selva. Un caso de estudio en la Península de Yucatán. Etnobio 12 (1), 60–67.
Goodland R. (1995). The concept of environmental sustainability. Ann. Rev. Eco. Sys. 26 (1), 1–24. doi: 10.1146/annurev.es.26.110195.000245
Gunn J. D., Foss J. E., Folan W. J., Carrasco M. D. R. D., Faust B. B. (2002). Bajo sediments and the hydraulic system of Calakmul, Campeche, Mexico. Ancient. Meso. 13 (2), 297–315. doi: 10.1017/S0956536102132184
Hernández Xolocotzi E., Baltazar B., Tacher L., Israel S. (1995). La milpa en Yucatán: Un Sistema de Producción Agrícola Tradicional (Mexico City: Colegio de Postgraduados).
Hicks J. (1946). Value and Capital: An Inquiry into some fundamental principles of Economic Theory (Oxford: Clarendon Press).
Holling C. S. (1996). “Engineering resilience versus ecological resilience,” in Engineering within ecological constraints. Ed. Schulze P. C. (Washington, D.C: National Academy Press), 32–43.
Isendahl C., Smith M. E. (2013). Sustainable agrarian urbanism: The low-density cities of the Mayas and Aztecs. Cities 31, 132–143. doi: 10.1016/j.cities.2012.07.012
Kurnick S., Rogoff D., Aragón I. A. (2023). A preliminary revised life history of Punta Laguna, Yucatan, Mexico: A persistent place. Ancient. Meso. doi: 10.1017/S0956536123000093
Lentz D. L., Dunning N. P., Scarborough V. L., Magee K. S., Thompson K. M., Weaver E., et al. (2014). Forests, fields, and the edge of sustainability at the ancient Maya city of Tikal. Proceed. Nat. Acad. Sci. 111 (52), 18513–18518. doi: 10.1073/pnas.1408631111
Levy-Tacher S. I., Ramírez-Marcial N., Navarrete-Gutiérrez D. A., Rodríguez-Sánchez P. V. (2019). Are Mayan community forest reserves effective in fulfilling people’s needs and preserving tree species? J. Environ. Man. 245, 16–27. doi: 10.1016/j.jenvman.2019.04.097
Lozano-García S., Torres-Rodríguez E., Ortega B., Vázquez G., Caballero M. (2013). Ecosystem responses to climate and disturbances in western central Mexico during the late Pleistocene and Holocene. Palaeogeo. Palaeoclim. Palaeoeco. 370, 184–195. doi: 10.1016/j.palaeo.2012.12.006
May Ciau R. B. (2018). El paisaje prehispánico en el distrito de Bolonchén, Yucatán: la mirada de Kiuic y sus alrededores. [Unpublished Master’s Thesis] (Mérida (Yuc: Departamento de Ecología Humana, Centro de Investigación y de Estudios Avanzados del IPN-Unidad Mérida).
McNeil C. L. (2012). Deforestation, agroforestry, and sustainable land management practices among the Classic period Maya. Quat. Internat. 249, 19–30. doi: 10.1016/j.quaint.2011.06.055
Meadows D. H., Meadows D. L., Randers J., Behrens W. W. III (1972). The limits to growth: A report for the club of rome´s project on the predicament of mankind (New Work: Universe Books).
Medina-Elizalde M., Burns S. J., Lea D. W., Asmerom Y., von Gunten L., Polyak V., et al. (2010). High resolution stalagmite climate record from the Yucatán Peninsula spanning the Maya terminal classic period. Earth Plan. Sci. Let. 298(1–2), 255–262. doi: 10.1016/j.epsl.2010.08.016
Michon G., De Foresta H. (1999). “Agro-forests: incorporating a forest vision in agroforestry,” in Agroforestry in sustainable agricultural systems. Eds. Buck L. E., Lassoie J. P., Fernandes E. C. M. (Boca Raton, FL: CRC Press), 381–406.
Moreno-Calles A. I., Toledo V. M., Casas A. (2013). Los sistemas agroforestales tradicionales de México: una aproximación biocultural. Bot. Sci. 91 (4), 375–398. doi: 10.17129/botsci.419
Mountjoy D. C., Gliessman S. R. (1988). Traditional management of a hillside agroecosystem in Tlaxcala, Mexico: An ecologically based maintenance system. Am. J. Alter. Ag. 3 (1), 3–10. doi: 10.1017/S0889189300002058
Mulyoutami E., Rismawan R., Joshi L. (2009). Local knowledge and management of simpukng (forest gardens) among the Dayak people in East Kalimantan, Indonesia. For. Eco. Man. 257 (10), 2054–2061. doi: 10.1016/j.foreco.2009.01.042
Mumary Farto P. A. (2016). El “colapso” de las entidades políticas mayas del Clásico. Diacron. 9 (15), 23–32.
Naeem S., Thompson L. J., Lawler S. P., Lawton J. H., Woodfin R. M. (1994). Declining biodiversity can alter the performance of ecosystems. Nat 368, 734–737. doi: 10.1038/368734a0
Nigh R., Diemont S. A. (2013). The Maya milpa: fire and the legacy of living soil. Front. Ecol. Environ. 11 (s1), e45–e54. doi: 10.1890/120344
Perfecto I., Vandermeer J., Wright A. (2019). Nature’s matrix: linking agriculture, biodiversity conservation and food sovereignty (Routledge: Oxfordshire).
Peterson G., Allen C. R., Holling C. S. (1998). Ecological resilience, biodiversity, and scale. Ecosys 1, 6–18. doi: 10.1007/s100219900002
Peterson L. C., Haug G. H. (2005). Climate and the collapse of Maya civilization: A series of multi-year droughts helped to doom an ancient culture. Am. Sci. 93 (4), 322–329.
Posey D. A. (1985). Indigenous management of tropical forest ecosystems: the case of the Kayapó Indians of the Brazilian Amazon. Agrofor. Sys. 3, 139–158. doi: 10.1007/BF00122640
Pugh T. W., Rice P. M., Chan Nieto E. M., Georges J. (2022). Complexity, cooperation, and public goods: quality of place at nixtun-ch’ich’, petén, Guatemala. Front. Pol. Sci. 4, 805888. doi: 10.3389/fpos.2022.805888
Reese-Taylor K., Hernández A. A., Esquivel F. A. F., Monteleone K., Uriarte A., Carr C., et al. (2016). Boots on the ground at Yaxnohcah: Ground-truthing LiDAR in a complex tropical landscape. Ad. Arch. Prac. 4 (3), 314–338. doi: 10.7183/2326-3768.4.3.314
Rivera-Núñez T., Fargher L. (2021). The concept of ‘palimpsest’ in a reconceptualization of biodiversity conservation. Environ. Con. 48 (1), 1–4. doi: 10.1017/S0376892920000399
Rivera-Núñez T., Fargher L., Nigh R. (2020). Toward an Historical Agroecology: an academic approach in which time and space matter. Agroeco. Sus. Food Sys. 44 (8), 975–1011. doi: 10.1080/21683565.2020.1719450
Rodríguez-Robayo K. J., Méndez-López M. E., Molina-Villegas A., Juárez L. (2020). What do we talk about when we talk about milpa? A conceptual approach to the significance, topics of research and impact of the mayan milpa system. J. Rural Stud. 77, 47–54. doi: 10.1016/j.jrurstud.2020.04.029
Rosenzweig M. L. (2003). Reconciliation ecology and the future of species diversity. Oryx 37 (2), 194–205. doi: 10.1017/S0030605303000371
Sanders W. T., Parsons J. R., Santley R. S. (1979). The basin of Mexico: ecological processes in the evolution of a civilization (New York: Academic Press).
Serra Puche M. C., Lazcano Arce J. C. (2012). Abandono y retorno en el sitio de Xochitécatl-Cacaxtla, Tlaxcala. Revi. Arque. Am. 30, 43–59.
Simms S. R., Parker E., Bey G. J., Gallareta Negrón T. (2012). Evidence from escalera al cielo: abandonment of a terminal classic puuc maya hill complex in Yucatán, Mexico. J. Field Arch. 37 (4), 270–288. doi: 10.1179/0093469012Z.00000000025
Smith M. E. (2010). Sprawl, squatters and sustainable cities: Can archaeological data shed light on modern urban issues? Cam. Arch. J. 20 (2), 229–253. doi: 10.1017/S0959774310000259
Smith M. E., Lobo J., Peeples M. A., York A. M., Stanley B. W., Crawford K. A., et al. (2021). The persistence of ancient settlements and urban sustainability. Proceed. Nat. Acad. Sci. 118, 20. doi: 10.1073/pnas.2018155118
Szabó P. (2015). Historical ecology: past, present and future. Bio. Rev. 90 (4), 997–1014. doi: 10.1111/brv.12141
Tilman D., Downing J. A. (1994). Biodiversity and stability in grasslands. Nat. 367 (6461), 363–365. doi: 10.1038/367363a0
Van Noordwijk M., Tomich T. P., De Foresta H., Michon G. (1997). To segregate – or to integrate: The question of balance between production and biodiversity conservation in complex agroforestry systems. Agrofor. Today 8 (1), 6–9.
Wackernagel M., Rees W. (1998). Our ecological footprint: reducing human impact on the earth (Gabriola Island: New Society Publishers).
Wartman P., Van Acker R., Martin R. C. (2018). Temperate agroforestry: How forest garden systems combined with people-based ethics can transform culture. Sustain 10, 7. doi: 10.3390/su10072246
Webster J. W., Brook G. A., Railsback L. B., Cheng H., Edwards R. L., Alexander C., et al. (2007). Stalagmite evidence from Belize indicating significant droughts at the time of Preclassic Abandonment, the Maya Hiatus, and the Classic Maya collapse. Palaeogeo. Palaeoclim. Palaeoeco. 250 (1-4), 1–17. doi: 10.1016/j.palaeo.2007.02.022
White D. A., Hood C. S. (2004). Vegetation patterns and environmental gradients in tropical dry forests of the northern Yucatan Peninsula. J. @ Veg. Sci. 15 (2), 151–160. doi: 10.1111/j.1654-1103.2004.tb02250.x
Wiersum K. F. (2004). “Forest gardens as an ‘intermediate’ land-use system in the nature-culture continuum: characteristics and future potential,” in New vistas in agroforestry: A compendium for 1st world congress of agroforestry. Eds. Nair P. K. R., Rao M. R., Buck L. E. (Netherlands: Springer), 123–134.
Wilken G. C. (1970). The ecology of gathering in a Mexican farming region. Econ. Bot. 24 (3), 286–295. doi: 10.1007/BF02860664
Keywords: sustainability, resilience, biodiversity, urbanism, Mesoamerica, Maya lowlands, Tlaxcala, historical ecology
Citation: Antorcha-Pedemonte R, Rivera-Núñez T and Fargher-Navarro LF (2023) The impact of ancient Mesoamerican cities on long-term environmental sustainability: the view from historical ecology. Front. Ecol. Evol. 11:1237953. doi: 10.3389/fevo.2023.1237953
Received: 10 June 2023; Accepted: 20 September 2023;
Published: 30 October 2023.
Edited by:
Attila Gyucha, University of Georgia, United StatesReviewed by:
David Manuel Carballo, Boston University, United StatesStephen A. Kowalewski, University of Georgia, United States
Copyright © 2023 Antorcha-Pedemonte, Rivera-Núñez and Fargher-Navarro. This is an open-access article distributed under the terms of the Creative Commons Attribution License (CC BY). The use, distribution or reproduction in other forums is permitted, provided the original author(s) and the copyright owner(s) are credited and that the original publication in this journal is cited, in accordance with accepted academic practice. No use, distribution or reproduction is permitted which does not comply with these terms.
*Correspondence: Lane F. Fargher-Navarro, bGFuZWZhcmdoZXJAeWFob28uY29t