- 1Key Laboratory of Wetland Ecology and Environment, Northeast Institute of Geography and Agroecology, Chinese Academy of Sciences, Changchun, China
- 2School of Economics and Management, Qiqihar University, Qiqihar, China
Introduction: The peat carbon pool stores 30% of the total global soil carbon accounting for 3–4% of the global land surface. The stability of the peatland carbon pool is a key factor affecting global carbon cycling that is seriously disturbed by climate change and regional human activities. However, the impact of these factors on carbon pool stability remains poorly understood.
Methods: Based on the physicochemical properties and carbon compounds of 973 peat samples from Jilin Province (China), which are widely distributed in different altitude regions of the Changbai Mountains, we investigated the stability of the carbon pool in different dominant plants and degradation types of peatlands and assessed the effects of regional environmental factors on the peatland carbon pool.
Results and Discussion: Our results showed that the carbohydrate content of peat soils in different peatland types ranged from 33.2 ± 6.9% to 40.5 ± 4.8%, and the aromatic content ranged from 19.8 ± 1.2% to 22.7 ± 2.3%. Bulk density is the most important physicochemical factor, and annual average temperature is the most important environmental factor that influences carbon stability. The effects of selected environmental factors on the peatland carbon pool covered by different plants were different, and the carbon stability in shrub peatlands is more sensitive to climate characteristics than in peatlands dominated by the other two plant types. Peatland degradation decreases the carbon stability in herb and herb/shrub peatlands and increases the carbon stability in shrub peatlands, leading the peatland carbon pool to be more easily influenced by regional human activities than natural peatlands.
1. Introduction
Peatlands store approximately 500 ± 100 Pg of carbon, which accounts for 30% of the global soil carbon pool and only 3% of the total global land area (Yu et al., 2010; Loisel et al., 2014). Water-saturated and low-pH environments are the major causes of carbon storage in peatlands, which is much higher than that in other ecosystems (Ye et al., 2012; Wu and Roulet, 2014; Loisel et al., 2017). Peatlands are mainly distributed across mid- and high-latitude regions, which are more sensitive to climate change than other regions, and the peatland carbon pool has become vulnerable to climate change in recent years (Solomon et al., 2007; Xu et al., 2018; Loisel et al., 2020). The stability of the peatland carbon pool acts as a key factor that affects its response to climate change and must be evaluated (Leifeld and Menichetti, 2018; Qiu et al., 2020; Cong et al., 2022).
Organic matter chemistry is widely used as a key factor for evaluating the decomposition process and chemical stability of peatland carbon pools (Sjögersten et al., 2016), and several typical chemical compounds of organic matter have been widely selected as indicators (Wilson et al., 2016; Cong et al., 2020). As the carbohydrate content in peat soils increases, organic matter is more easily decomposed, and more CO2 is released through microbial activities under aerobic environment, which means that an increase in carbohydrate content decreases the stability of the peatland carbon pool (Leifeld et al., 2012). Phenolic acid acts as an important factor that inhibits microbial activities and decreases the decomposition rate of organic matter (Fenner and Freeman, 2020). Thus, the release of phenolic compounds from shrubs has been widely hypothesized to be a key factor that increases carbon stability and protects the peatland carbon pool in warm and dry environments (Wang et al., 2015). The aromatic contents in peat soils in low-latitude peatlands with warm environments are significantly higher than those in high-latitude peatlands with cold environments, and the chemical stability of the carbon pool in low-latitude peatlands is significantly higher than that in high-latitude peatlands (Hodgkins et al., 2018). Furthermore, as the mean July temperature increased and the annual precipitation increased, the carbohydrate content in peat soils decreased and the aromatic content increased markedly, indicating an increase in the stability of the peatland carbon pool (Cong et al., 2020). These results indicate that peatland carbon pools with high aromatic contents and low carbohydrate contents are more stable than others, and these special carbon chemical compounds are majorly influenced by regional climate factors (Verbeke et al., 2022).
In addition to climatic factors, chemical compounds of organic matter are mainly influenced by their sources and the storage environment (Heller et al., 2015). Considering the dominant plant community and plant biomass in peatlands, plant litter from herbs and shrubs acts as the major organic matter (OM) source, and the carbon compounds of OM in the surface soils of these two types of plant communities are significantly different (Normand et al., 2017). In general, more lignin content is available in shrub stems than in herb plant litter, which causes the aromatic contents and the stability of the carbon pool in shrub peatlands to be higher than that in herb peatlands (Wang et al., 2015). In recent years, the increase in human activities has also become an important disturbance factor that has serious effects on peatlands, such as decreasing the water table and accumulating more nutrient/pollution elements (Gao et al., 2018). For example, the increase in atmospheric nitrogen deposition not only increases gross ecosystem production but also increases the decomposition rates of the soil carbon pool (Bragazza et al., 2006; Wu et al., 2015), which accelerates the carbon cycling rates in peatlands and may also cause serious changes in the stability of the peatland carbon pool. Serious environmental disturbances may also cause peatland degradation, such as a decrease in the water table through drained or climate drying and an increase in pH values through the accumulation of more nutrients (Dohong et al., 2017; Šimanauskienė et al., 2019). The degradation of peatlands has serious effects on peat soil accumulation and promotes peat decomposition (Drollinger et al., 2020). Liable carbon compounds are more easily decomposed in degraded peatland carbon pools and increase the residual carbon recalcitrance to environmental changes, which may also cause the chemical stability of residual carbon compounds to be higher than that in natural peatland carbon pools (Leifeld et al., 2020). Thus, the dominant plant types, degradation, climate characteristics, and regional human activities not only cause serious changes in the peatland environment but also have potential effects on the chemical compounds of organic matter and the stability of the peatland carbon pool. However, these effects remain uncertain.
Peatlands in Jilin Province (China) are mainly covered by herb and shrub plants and are located in the Changbai Mountains, which are widely distributed in different altitudes from 17 to 1,550 m (Xing et al., 2015). The different altitudes caused the peatlands in this region to develop under different climatic characteristics and regional environmental disturbances. In addition, the increasing population in Northeast China during the 20th century caused serious effects on peatlands in the Changbai Mountains and more serious effects on low-altitude peatlands than those located at high altitudes (Gao et al., 2016). The marked differences in climatic factors and anthropogenic disturbances may also have serious effects on the stability of peatland carbon pools in different altitude regions; however, these are still unclear.
To address these knowledge gaps, the goals of this study were to assess the stability of the peatland carbon pool in Jilin Province based on detailed physiochemical properties and carbon chemistry analysis of peat soils in different peatland types. Second, we wanted to identify the differences in peatland carbon pools under the different dominant plant types and evaluate whether peatland degradation causes serious effects on the peatland carbon pool. Finally, by comparing the natural and anthropogenic factors with the peatland carbon pool stability, we aimed to clarify the effects of climate characters and human activities on the stability of the peatland carbon pool in Jilin Province.
2. Materials and methods
2.1. Site description and sampling
The peatlands studied in Jilin Province are mainly located in the middle part of the Changbai Mountains in Northeast China. In this study, we collected 973 peat samples from typical peatlands in Jilin Province, which cover the different dominant plants in natural and degraded peatlands and are widely distributed in different altitude regions (Figure 1). Based on the dominant plants, the peatlands were divided into herb peatland (i.e., the dominant plants are Carex sp., Typha orientalis Presl), herb/shrub peatland (i.e., the dominant plants are Betula platyphylla Suk., Spiraea salicifolia L., Carex sp.), and shrub peatland (i.e., the dominant plants are Betula platyphylla Suk., Spiraea salicifolia L., Sphagnum palustre L.). Unlike natural peatlands, the degraded peatlands are threatened by regional human activities (e.g., graze, drainage, and reclamation) or extreme climate change (e.g., drought). Most of degraded peatlands are distributed in the middle and south of Jilin Province and the natural peatlands are distributed in the eastern of Jilin Province. The sampling sites were randomly selected in 2013 and were widely distributed in the middle of the Changbai Mountains to reflect the regional peatland carbon pool. The studied peatlands have a temperate continental monsoon climate with mean annual temperatures of −0.5–6°C and a mean annual precipitation of 500–900 mm (Fick and Hijmans, 2017). The regional human influence index (HII) of selected peatlands ranged from 10 to 41 (WCS and CIESIN, 2005), and the altitudes ranged from 17 to 1,550 m. Considering the number of peat samples and peat sites, one samples from each site would be better than several samples from different depth in several selected typical peatlands for evaluating the chemical stability of peatland carbon pool in Jilin Province. The peat samples for each site were a mixture of the whole peat layer to ensure that the samples reflected the average carbon content and stability of the peatland carbon pool at selected sites. The location of each site was determined using a portable global positioning system (GPS; Figure 1). The samples were stored in polyethylene plastic bags and brought to the laboratory for analysis. The samples were loosely disaggregated to facilitate air-drying at 20°C.
2.2. Physical and chemical analysis
The bulk density of peat soils was obtained from the weight of peat soils with known volumes dried in an oven at 105°C for 12 h. The pH of the peat soils was measured directly using a pH meter, and the weight ratio of peat soils to water was 0.1. The sources of residual fiber in peat samples were mainly from the undecomposed plant litters, and the percentage of fiber content was used to define the peat decomposition level. With the fiber proportion increasing, more undecomposed plant litters and the peat decomposition degree decreasing. The fiber content was determined from the dry weight of fibers retained on a 0.15 mm sieve as a percentage of oven-dried mass, and the ratio of the weight loss of peat to total peat was regarded as the decomposition degree of peat samples (Abd Rahman and Ming, 2015). Soil organic carbon was determined by external heating potassium dichromate oxidation method, and details of this method was described in Zhao et al. (2012).
2.3. Carbohydrate and aromatic contents
The carbohydrate and aromatic contents of peat soils were estimated based on FTIR spectra and selected peat heights, which were area-normalized and baseline corrected (Cong et al., 2020). The FTIR spectra of the peat soils were obtained using a Spectrum Two FTIR spectrometer (PerkinElmer, United States) using KBr pellets (150 mg dried KBr and 1 mg peat soil). Measurements were recorded from 4,000 to 300 cm−1 using a resolution of 1 cm−1. The carbohydrate content was estimated based on the regression equations of the heights of the FTIR carb (1,030 cm−1), and the aromatic content were estimated based on the height of the FTIR arom15 (1,510 cm−1) and arom16 (1,615 cm−1; Hodgkins et al., 2018).
2.4. Statistical method
2.4.1. Two-Way analysis of variance
The physicochemical and carbon properties of peat soils (i.e., bulk density, decomposition degree, pH, carbon content, carbohydrate content, and aromatic content) were analyzed using a two-way analysis of variance (ANOVA, SPSS 22.0, IBM, Armonk, United States). For peatlands in Jilin Province, the peatlands were classified by dominant plant types and divided into herb peatlands, herb/shrub peatlands, and shrub peatlands. Thus, peatland-dominant plant types (i.e., herbs, herb/shrub, and shrub) and degradation (i.e., degraded or natural) were regarded as two distinct factors, and their effects on the tested variables were evaluated through two-way ANOVA. Significant differences are reported at a probability level of 0.05, unless otherwise stated.
2.4.2. Random forest
The importance of the selected environmental factors on peat physicochemical properties was calculated using a random forest (RF) model. The RF classification was conducted using the “randomForest” package in R software. The selected soil physicochemical properties (bulk density, decomposition degree, and pH) and environmental factors (altitude, annual precipitation, annual average temperature, degradation, HII, and dominant plant type) were considered as explanatory variables, and the carbon properties were considered as the response variables. Seventy percent of the selected data were regarded as the training dataset, and the RF then searched for the predictor that best partitioned the response variable in the training dataset. The relative importance of the selected environmental factors for each physicochemical property of peat soils was calculated based on the increase in the mean squared error (%IncMSE).
3. Results
3.1. Physicochemical properties of peat soils in Jilin Province
The average values with standard error of the bulk density, pH, and decomposition degree of peat soils in Jilin Province are shown in Table 1, and box plots of these properties are shown in Figure 2. The bulk density of peat soils in Jilin Province ranged from 0.23 ± 0.10 g.cm−3 to 0.40 ± 0.10 g.cm−3. In herb/shrub peatlands, the bulk density were 0.23 ± 0.10 g.cm−3 in natural peatlands and 0.29 ± 0.12 g.cm−3 in degraded peatlands, which were markedly lower than those in other peatland types. The decomposition degree of peat soils ranged from 62.5 ± 17.6% to 81.6 ± 10.4%, and the decomposition degree in degraded peatlands was markedly higher than that in natural peatlands, especially for peatlands covered by shrubs, which was 62.5 ± 17.6% in natural peatlands and 81.6 ± 10.4% in degraded peatlands. The pH of peat soils ranged from 4.91 ± 0.34 to 5.09 ± 0.30. Based on the two-way ANOVA results, both the dominant plant types and degradation had significant effects on the bulk density and decomposition degree of peat soils (Table 2). However, only the dominant plant types had significant effects on the pH of peat soils, and the effects of degradation on the pH of peat soils were not significant.
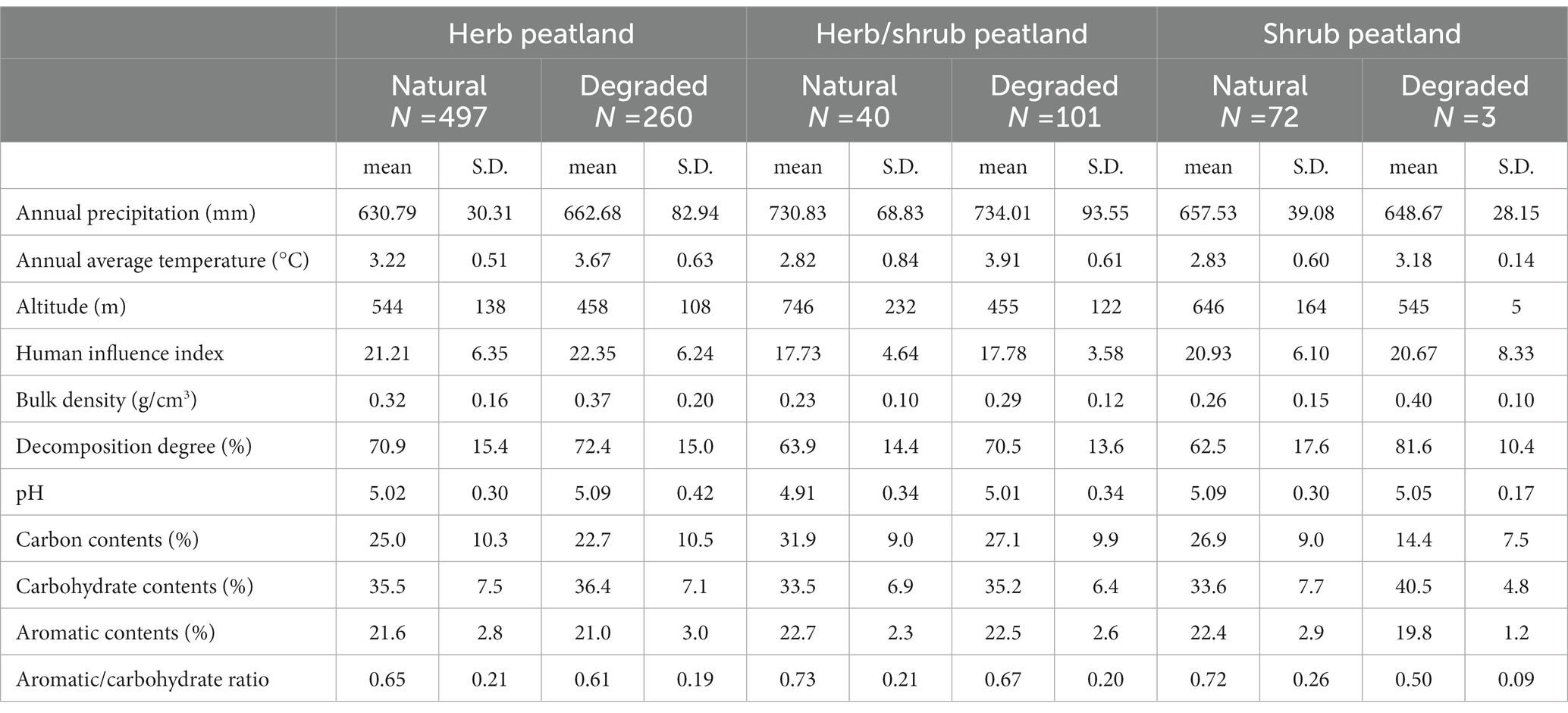
Table 1. Basic information of selected peat soils in different dominated plant types of natural and degraded peatland.
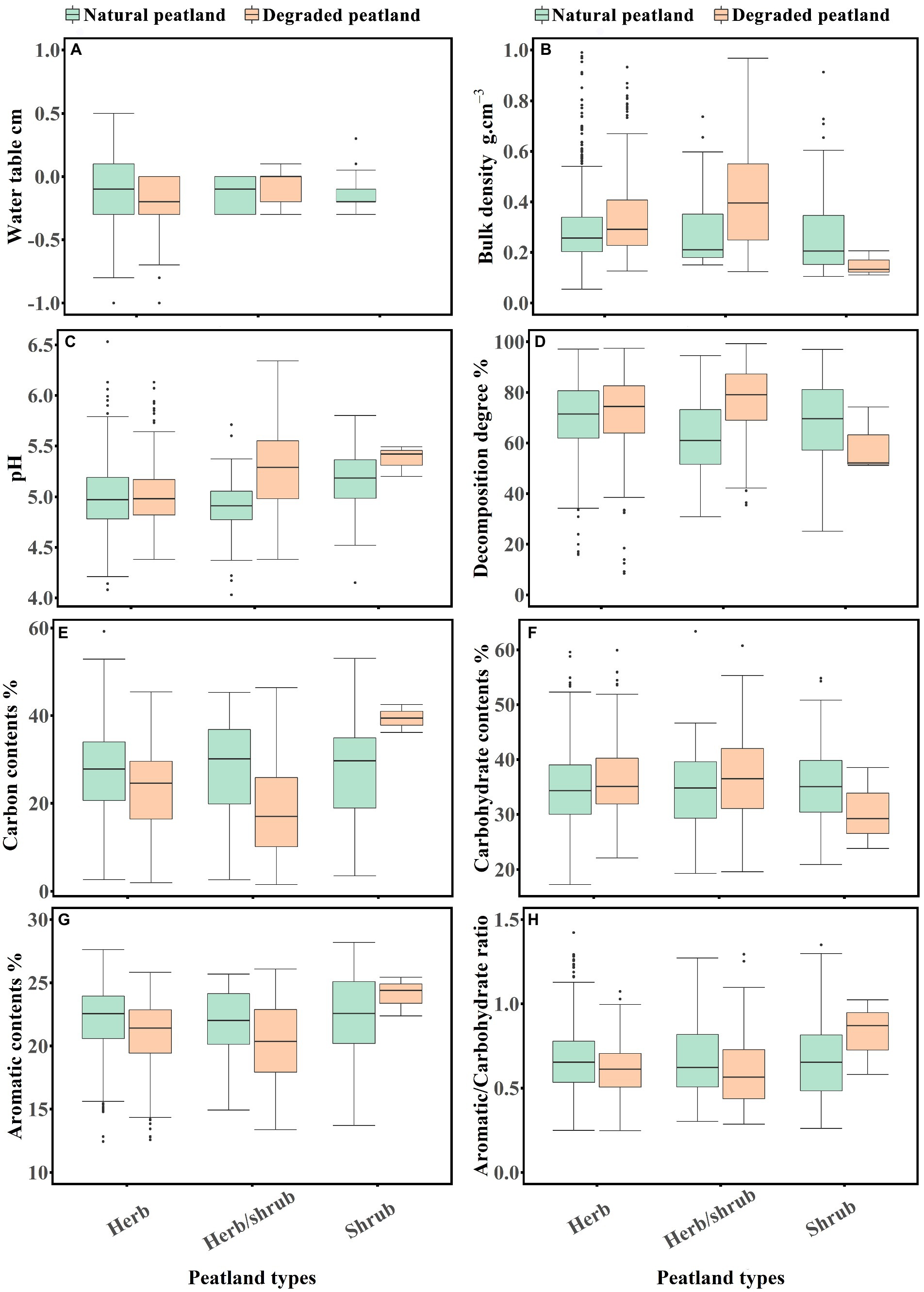
Figure 2. Boxplot of water table (A), bulk density (B), pH (C), decomposition degree (D), carbon contents (E), carbohydrate contents (F), aromatic contents (G), and the ratio of aromatic/carbohydrate (H) of peat soils in different dominated plant types of natural and degraded peatland.
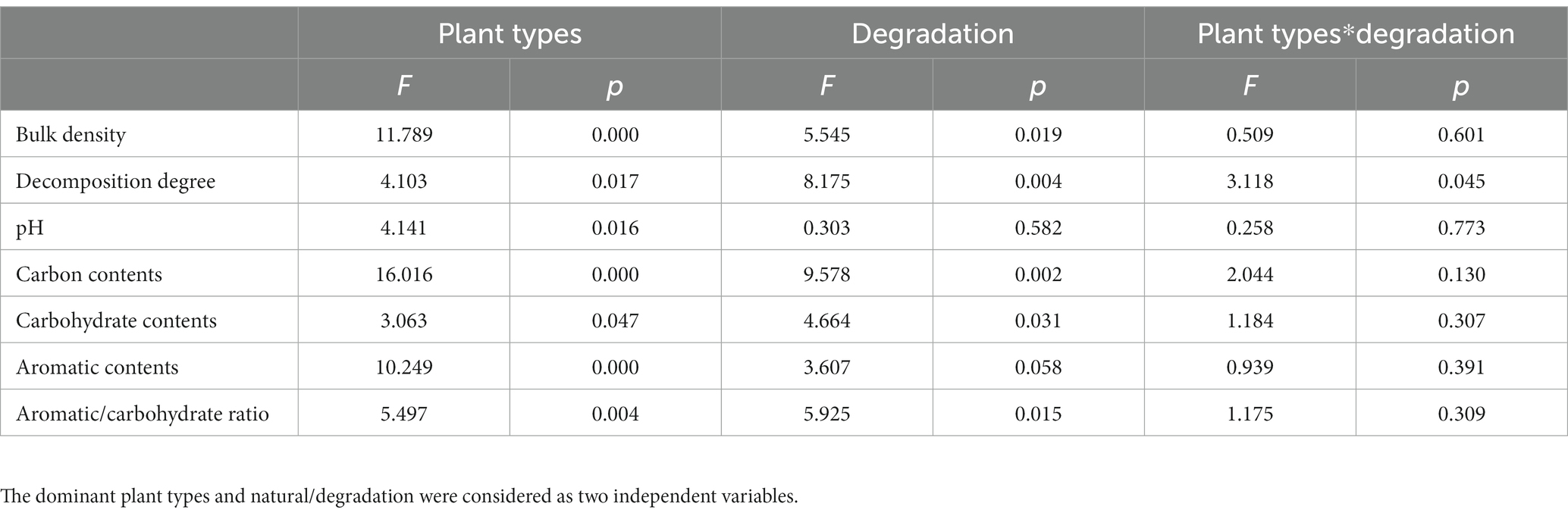
Table 2. Two-way ANOVA for typical physicochemical properties, carbon contents, and carbon compounds in peat soils.
3.2. Carbon contents and chemical stability of peat soils in Jilin Province
The carbon content of peat soils in Jilin Province in different peatland types ranged from 14.4 ± 7.5% to 31.9 ± 9.0%, and the lowest carbon contents were observed in degraded shrub peatlands (Table 1; Figure 2). The carbon content of peat soils in degraded peatlands was significantly lower than that of natural peatlands, and the effects of dominant plant types on carbon content were also significant. Carbohydrate contents of peat soils in Jilin province ranged from 33.2 ± 6.9% to 40.5 ± 4.8%, and the average carbohydrate contents in different peatland types (i.e., dominant plant types and degradation) were significant different. Compared to natural peatlands, carbohydrate content in degraded peatlands were significantly higher than those in natural peatlands. Conversely, the aromatic content in degraded peatlands were slightly lower than those in natural peatlands. However, the effects of degradation on the aromatic content were not significant. In shrub peatlands, the average aromatic content in degraded peatlands was 19.8 ± 1.2%, which was markedly lower than that in natural peatlands which was 22.4 ± 2.9%. In others, the aromatic contents were approximately 22% and ranged from 21.0 ± 3.0% to 22.7 ± 2.3%. Interestingly, the effects of the interaction between dominant plant types and degradation on both these variables were not significant.
3.3. Importance of typical factors on peatland carbon pool
The importance of the selected environmental factors, dominant plant types, and physicochemical properties of peat soils on peatland carbon pools is shown in Figure 3. The IncMSE results shown that the importance of physicochemical properties for the peatland carbon pool are stronger than the selected environmental factors and the dominant plant types. Bulk density was listed as the most important factor for all four indicators of the peatland carbon pool, ranging from 35.30 to 89.36%. The importance of bulk density on carbon content was 89.36%, which is obviously higher than that of other factors, in which the IncMSE values only ranged from 0.95 to 21.53%. The degree of decomposition was the second most important factor (21.53%) for the carbon content. Compared with the bulk density and decomposition degree, the impact of pH on the peatland carbon pool was markedly lower, ranging from 0.57 to 6.56%. Among the environmental factors, altitude was the most important factor (12.47%) for carbon content, and the annual average temperature was the most important factor for carbon chemical stability. Compared to climate factors, the importance of the HII for peatland carbon pools was markedly lower, ranging from 0.95 to 6.43%. The importance of plant types on the peatland carbon pool was slightly higher than that of degradation and ranged from 6.26 to 9.49%.
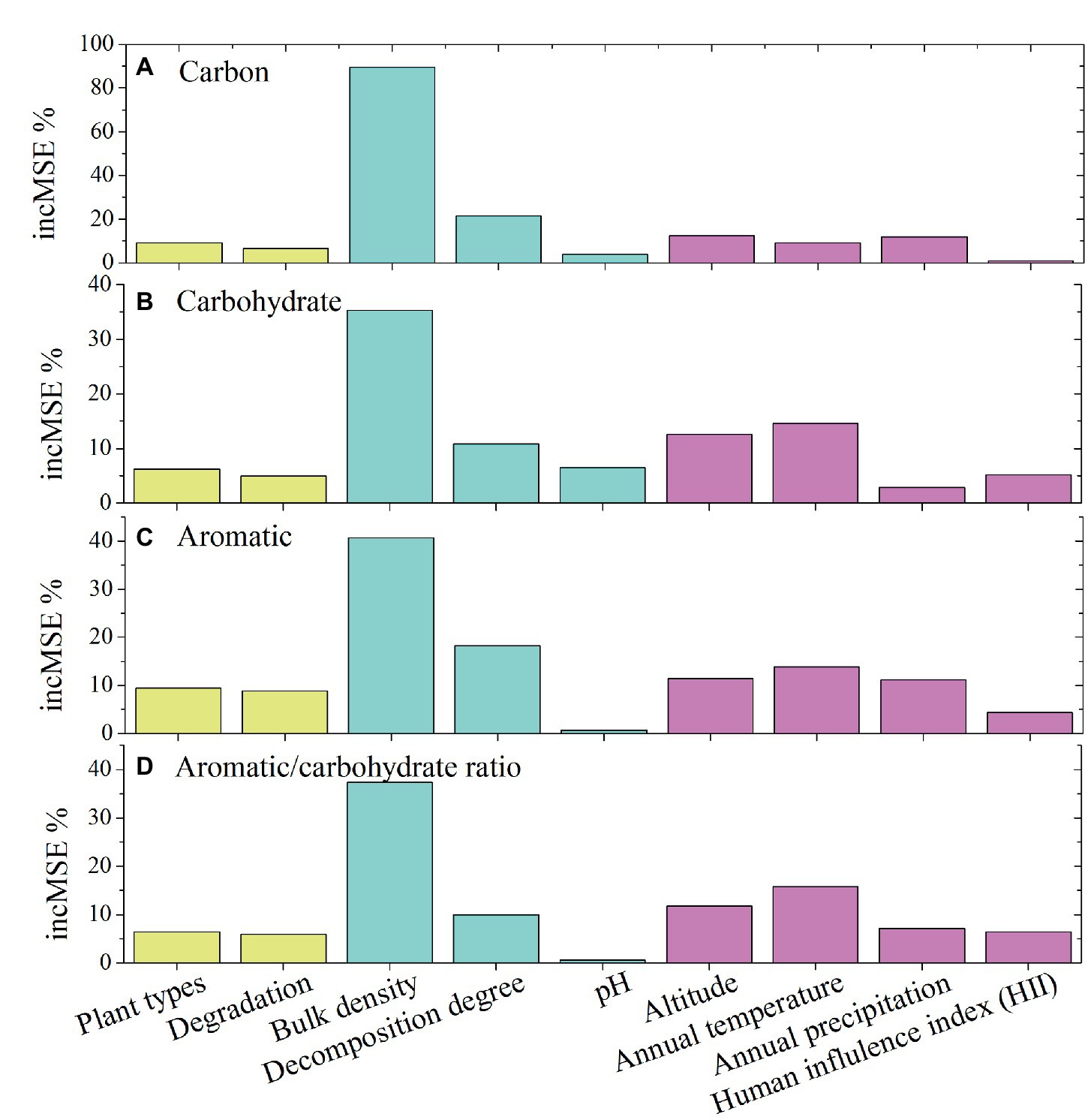
Figure 3. Based on random forest analysis, the increase in mean squared error (%IncMSE) of selected environmental factors to carbon contents (A), carbohydrate contents (B), aromatic contents (C), and the ratio of aromatic/carbohydrate (D).
4. Discussion
4.1. Effects of dominant plant types and degradation on peat soil properties
The chemical properties of OM in peatland carbon pools are affected by the natural conditions of formation, land use, and drainage (Heller et al., 2015; Negassa et al., 2019; Leifeld et al., 2020). Because local plants are a major source of OM in peatlands, the dominant plant community acts as one of the major factors influencing the chemical properties of OM in the peatland carbon pool (Paul, 2016). The effects of the dominant plant types on natural and degraded peat soil properties and carbon pools are shown in Figure 2.
In shrub peatlands, the pH of peat soils was higher and the bulk density was lower than those in peatlands covered by the other two plant types. The decomposition degree in herb/shrub peatlands was lower than that in the others. The pH of peat soils in peatlands, which are influenced by surface water (i.e., fen), are higher than those only influenced by rain water (i.e., bog; Rydin and Jeglum, 2013). Due to the high pH in shrub peatlands, we speculated that more shrub peatlands in Jilin province are influenced by surface water than herb peatlands. Additionally, the water table of peatlands are also important that affect the plants, and the water table in shrub peatlands is lower than that in herb peatlands (Keddy, 2010; Rydin and Jeglum, 2013). Thus, changes in the water table also act as another factor that influences the physicochemical properties of peat soils. In natural peatlands, many studies have reported that the decomposition of peat soils always increases with decreasing water table (Clarkson et al., 2013; Munir et al., 2014). The decomposition of peat soils in herb and shrub peatlands was similar in our study and obviously higher than that in herb/shrub peatlands, where the water table should be at the middle level of these three peatland types. However, because herb plant litter contains more carbohydrates and less aromatics than shrub plant litter (Wang et al., 2021a), herb plant litter is more easily decomposed than shrub plants. Thus, the decomposition degree of peat soils in herb and shrub peatlands was similar. We also speculated that the reason that the low decomposition degree of peat soils in herb/shrub peatlands is that the plant litter sources in herb/shrub peatlands are contain more stable compounds than herb peatlands and the decomposition environment are more restrictive under higher water tables than in shrub peatlands.
Compared to peat physicochemical properties, the effects of peatland-dominant plant types on carbon content, carbohydrate content, and aromatic content were lower, and the differences in the carbon pool in peatlands covered by different plants were weak. Although the aromatic content in shrub plants was higher than that in herb plants, there was no obvious difference in aromatic content between shrub and herb peatlands. Compared to carbohydrate compounds, aromatic compounds are recalcitrant and limiting the decomposition of peatland carbon pool (Fenner and Freeman, 2020). The major reason for the similar contents of residual aromatics and carbohydrates in herb peatlands and shrub peatlands is speculated to be that the decomposition processes of peat soils cause less aromatic compounds and more carbohydrate compounds from the herb plant to decompose, which cause the aromatic compounds contents increasing in residual herb peatland carbon pool.
Interestingly, the effects of degradation on peat physicochemical properties and carbon pools in peatlands covered by different plant types varied (Figure 3). The bulk density and decomposition degree in the degraded herb and herb/shrub peatlands were markedly higher than those in natural peatlands. These two properties in degraded shrub peatlands were markedly lower than those in natural peatlands. A similar trend was also found in carbohydrate content and the effects of degradation on the carbon and aromatic contents of peatlands covered by different plant types. The most important characteristic of peatland degradation is the decreasing in the water table (Guo et al., 2013; Han et al., 2021). Due to there was no clear water table for measuring the degradation of herb/shrub peatlands and shrub peatlands, especially for dry degraded peatlands. The available water table data for these two peatland types could not be used to reflect the real water level conditions. For herb peatlands, the water table could be measured in most of the degraded herb peatlands, and the results showed that the water table in degraded peatlands was clearly lower, and the decomposition degree of peat soils was markedly higher than that in natural peatlands (Figure 2). Decreasing the water table also decreases the biomass of herb plants and increases the biomass of shrub plants (Lou et al., 2018), which may be the major factor for plant litter residuals in degraded herb and herb/shrub peatlands.
The increase in the degree of decomposition and changes in plant litter sources also cause changes in the stability of the peatland carbon pool (Leifeld et al., 2012). More carbohydrate and less aromatic contents in degraded peatlands means the chemical stability in degradation herb and herb/shrub peatlands was slightly lower than that in natural peatlands (Hodgkins et al., 2018). In the present study, increasing the degree of decomposition increased the bulk density and decreased the carbon content, which was clearly found in herb peatlands and herb/shrub peatlands. However, the effects of degradation on shrub peatlands were opposite to those of the other peatland types. Unlike herb plants, decreasing the water table in degraded peatlands is beneficial for shrub plant growth, and the aboveground biomass of shrub plants increases under low water table environments (Lou et al., 2015). With more plant litter from the shrub plant residue, more carbon with high aromatic contents in shrub plant litter accumulated in the peatland carbon pool, which increased the stability of the peatland carbon pool and decreased the decomposition degree of peat soils in degraded shrub peatlands (Straková et al., 2012; Cong et al., 2022). Thus, the different effects of degradation on dominant plant communities are the major factors that cause the degradation decreases the carbon content and carbon chemical stability in herb and herb/shrub peatlands and causes opposite effects on shrub peatlands.
4.2. Effects of peat physicochemical properties on peatland carbon pool
The bulk density, decomposition degree, and pH of peat soils were selected as physicochemical property indicators to evaluate the effects of peat physicochemical properties on carbon content and stability in the peatland carbon pool, and the results are shown in Figure 4. The results of the RF analysis showed that the importance of the bulk density on the peatland carbon pool was markedly higher than that of the other two factors (Figure 3). The effects of bulk density on carbon content in peat soils were negative for all peatland types, and the slope for natural herb/shrub peatlands was the highest. Carbon decomposition and mineral element accumulation in peatlands are the major reasons for the increase in the bulk density of peat soils (Bao et al., 2012; Kareksela et al., 2015; Wang et al., 2021b). With the bulk density of peat soils increasing, more carbon decomposed and less carbon residual in peat soils which is the major factor that explain the negative correlation between bulk density and carbon contents. While, as the bulk density increased, the carbohydrate content increased and the aromatic content decreased, which caused a marked decrease in the aromatic/carbohydrate ratios. More mineral elements available in peat soils with high bulk density was speculate to support more nutrients for plant growth, and more leaves with high carbohydrate contents in surface plant under sufficient nutrition environment (Poorter et al., 2012; Wang et al., 2015). More leaves with high carbohydrate contents as organic matter sources under high nutrients environment is speculated as a potential reason that cause the stability of the peatland carbon pool decreased with increasing bulk density.
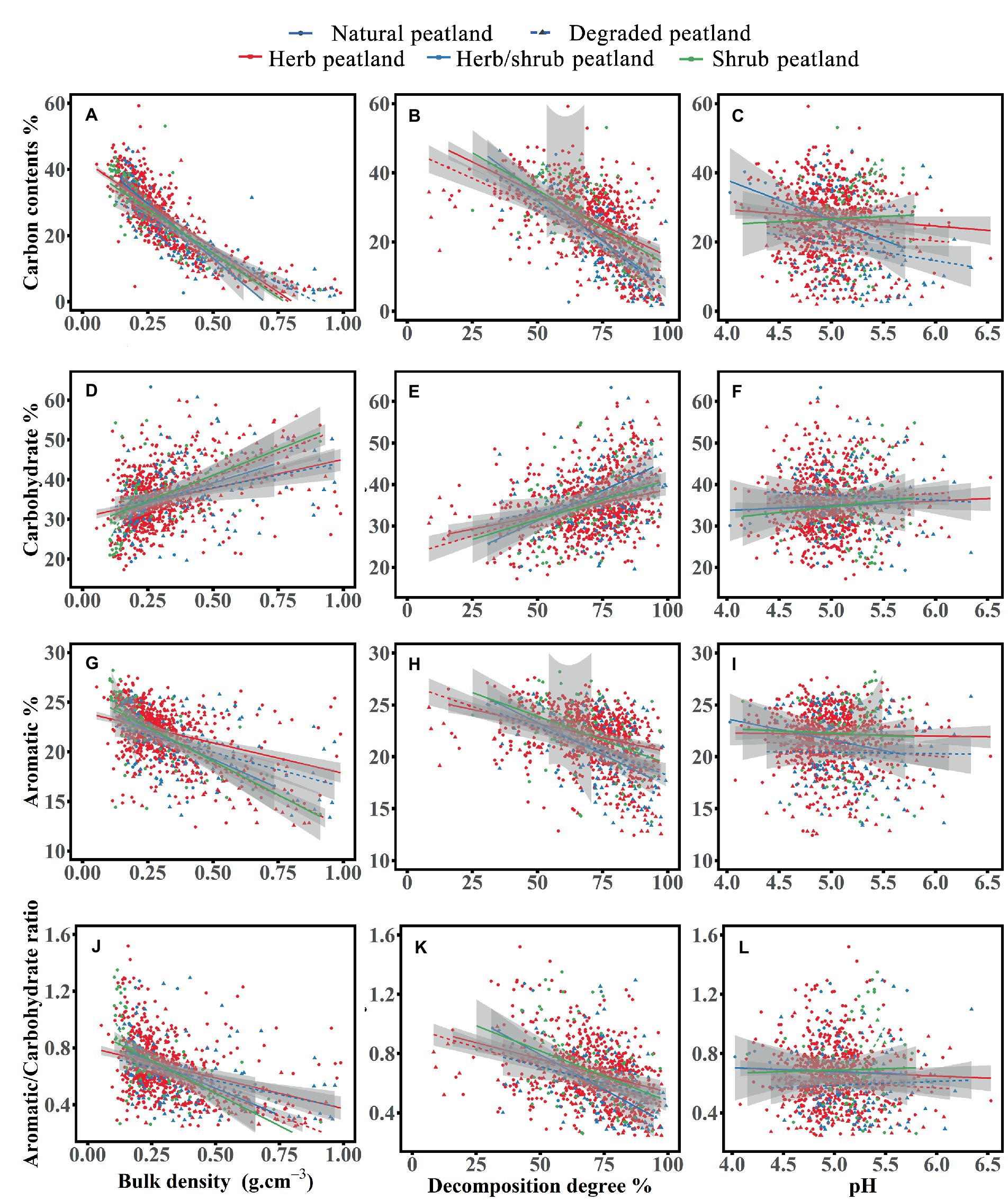
Figure 4. Relation between carbon contents (A–C), carbohydrate contents (D–F), aromatic contents (G–I), and aromatic/carbohydrate ratio (J–L) with bulk density, degree of decomposition, and pH in different dominated plant types of natural and degraded peatland. The trend lines were fitted with a linear model, and the grey shadows indicate 95% confidence interval of fitted lines.
As the bulk density increases, a marked decreasing trend in natural peatlands indicates that the carbon content in natural peatlands is more sensitive to decomposition and mineral accumulation than that in degraded peatlands. However, the effects of bulk density on carbohydrate and aromatic contents in natural/degraded peatlands covered by different plants were different. For herb and shrub peatlands, the carbohydrate and aromatic contents in natural peatlands were less sensitive than those in degraded peatlands. This result indicates that the stability of the natural peatland carbon pool is less sensitive to bulk density than that of degraded peatlands. However, the opposite trend for herb/shrub peatlands showed that the increase in bulk density decreased the stability of the carbon pool in natural herb/shrub peatlands. The decreasing trend of the fitted line also showed that the effects of bulk density on carbon content in natural herb/shrub peatlands were more marked than in others, and the effects of bulk density on carbon stability (i.e., aromatic/carbohydrate ratio) in degraded shrub peatlands were more marked than in other peatland types.
The high degree of decomposition in our selected method indicates that high amount of undecomposed plant litter (i.e., fibers) is decomposed and converted to organic matter in peat soils (Abd Rahman and Ming, 2015). Similar to bulk density, the effects of decomposition degree on carbon, aromatic content, and the aromatic/carbohydrate ratio in all peatland types were the same and negative, with opposite effects on carbohydrate content. Due to the carbohydrate compounds are more easily decomposed than aromatic compounds (Leifeld et al., 2012), residual plant litters with high aromatic and low carbohydrate contents in peat soils were not easy decomposed and converted to organic matter. And more undecomposed plant litters with high aromatic compounds contents was speculated as an important reason that cause high aromatic and low carbohydrate available in low decomposition degree of peat soils. What is more, high amount of available aromatics in weakly decomposed plant litter may also act as a major reason that inhibit the organic matter decomposition and increase carbon stability (Fenner and Freeman, 2020; Wilson et al., 2022). As the decomposition degree of peat soils increased, most of plant litter converted to organic matter, and the decrease in carbon content and aromatic/carbohydrate ratios showed that the decomposition processes not only caused carbon loss also decreased the stability of the peatland carbon pool.
Unlike bulk density and decomposition, the effects of pH on the selected carbon properties of different peatland types were different. For example, the effects of pH on carbon content in degraded and natural shrub peatlands were positive, whereas the effects on other peatland types were negative. The effects of pH on carbohydrate and aromatic contents in degraded shrub peatlands were more marked than in others. In degraded shrub peatlands, as the pH increased, the aromatic content increased and the carbohydrate content decreased markedly, and the aromatic/carbohydrate ratio also increased more markedly than in the others. The pH of peatlands is significantly influenced by water sources and nutrients availability, and peat soils with low pH are mainly found in peatlands, where the only water source is rainwater and low available nutrients (Rydin and Jeglum, 2013). As the pH increases, more nutrients are available in peat soils and cause organic matter to decompose more easily under high microbial metabolic activities (Ye et al., 2012; Zhao et al., 2022). For natural peatlands, the effects of pH on herb/shrub peatlands were more marked than in others, which indicates that the effects of water and nutrient sources in herb/shrub peatlands were also more marked than in others. However, except for degraded shrub peatlands, the weak effects of pH on the aromatic/carbohydrate ratios in all peatland types indicate that the effects of water sources and available nutrients on carbon stability were weak. Thus, the influence of increasing pH on the peatland carbon pool mainly decreased the carbon content in peat soils and had weak effects on the stability of peatland carbon pools.
4.3. Effects of environmental factors on peatland carbon pool
To assess the effects of environmental factors on the carbon content and stability of the peatland carbon pool, the altitude, annual average temperature, annual precipitation, and HII were selected as regional climate and human factors, and the correlation between these factors and carbon properties is shown in Figure 5. The importance of altitude for carbon content was highest in three of the four selected potential factors, and for carbon stability, it was slightly lower than the annual average temperature (Figure 3). With increasing altitude, the carbon and aromatic contents in herb-peatlands and herb/shrub peatlands increased, and the carbohydrate content decreased. Opposite trends were observed in shrub peatlands, and the contents of carbon and aromatics decreased markedly in high-altitude peatlands. Based on global peat samples, a previous study found that carbohydrate content was greater and aromatic content was lower at higher altitudes in similar latitudes (Verbeke et al., 2022), which was similar to the effects on shrub peatlands in our study. Thus, the response of the carbon pool in peatlands covered by different plant types to altitude increase is different and should be considered individually. Furthermore, the effects of altitude on carbohydrate and aromatic content in natural peatlands were more marked than those in degraded peatlands. Additionally, no obvious changes were observed for carbohydrate and aromatic contents in degraded peatlands in different altitude regions, and only carbon contents were increased slightly with an increase in altitude. The increase in altitude also markedly increased the aromatic/carbohydrate ratio in the natural peatlands and was more obvious in herb/shrub peatlands. The increase in the aromatic/carbohydrate ratio indicates that the chemical stability of the peatland carbon pool increased, which indicates that the peatland carbon pool in the high-altitude region was more stable than that in the low-altitude region.
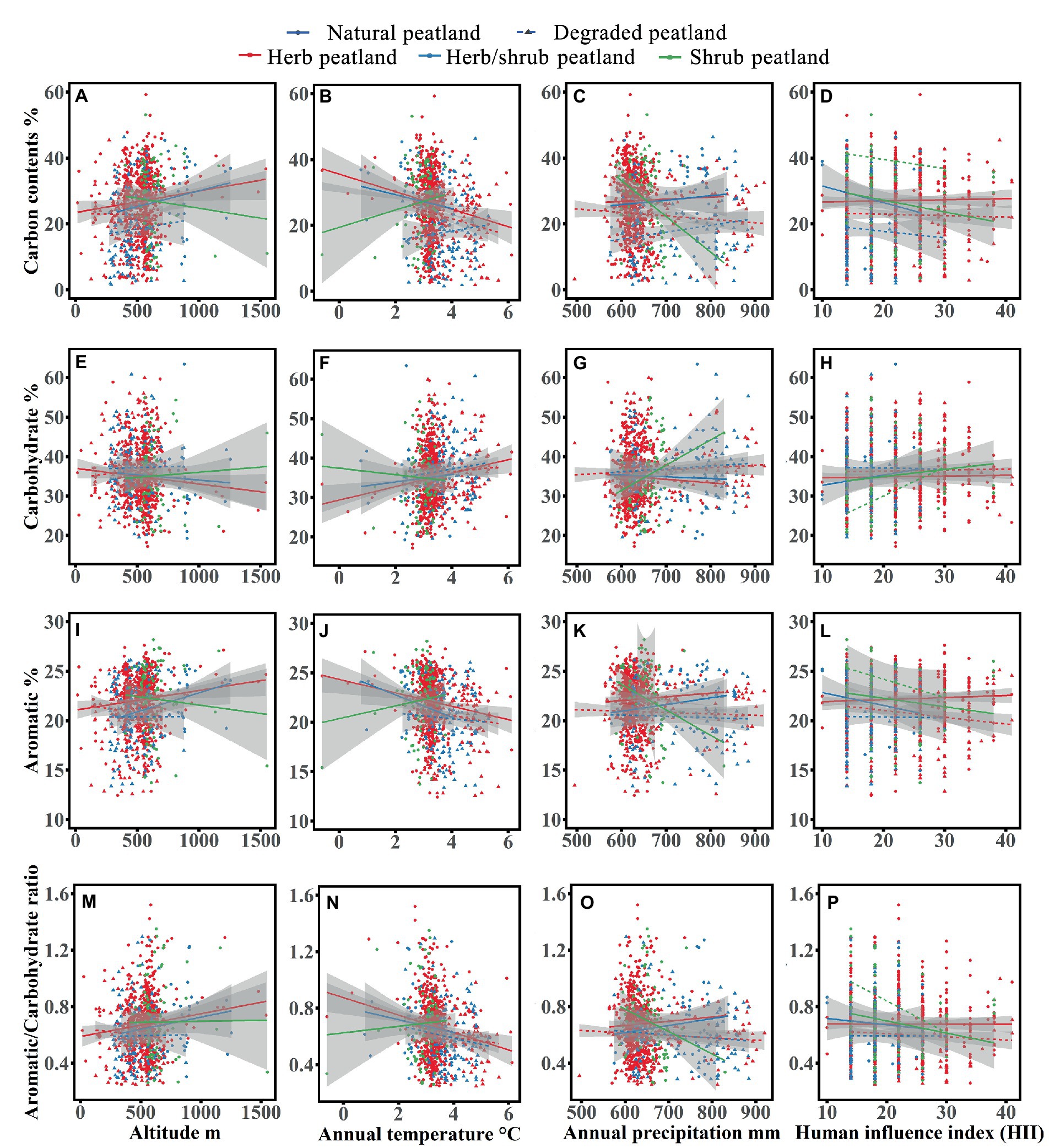
Figure 5. Relation between carbon contents (A–D), carbohydrate contents (E–H), aromatic contents (I–L), and aromatic/carbohydrate ratio (M–P) with altitude, mean annual temperature, annual precipitation, and human influence index (HII) in different dominated plant types of natural and degraded peatland. The trend lines were fitted with linear model, and the grey shadows indicate 95% confidence interval of fitted lines.
Temperature and precipitation also act as two important climatic factors that have serious effects on the peatland carbon pool (Figure 5). Similar to altitude, the effects of temperature and precipitation on the carbon pool in different peatland types were also different. With an increase in the annual average temperature, the carbon and aromatic contents in the shrub peatlands increased markedly, and the carbohydrate content decreased. The shrub peatland carbon pool in the high-temperature region was more stable than that in the low-temperature region, which is similar to that observed in previous studies at the global scale (Hodgkins et al., 2018; Verbeke et al., 2022). However, the effects of annual average temperature on herb and herb/shrub peatlands were the opposite, and high aromatic/carbohydrate ratios occurred in those located in the low annual average temperature region. The increase in annual precipitation decreased carbon stability in shrub peatlands and increased carbon stability in others. The high slope of the trend line between carbon properties and annual precipitation in shrub peatlands indicates that shrub peatland carbon pools are more easily influenced by precipitation than in other peatland types. Because the water tables in shrub peatlands are lower than in others (Rydin and Jeglum, 2013), the importance of precipitation for shrub peatland water sources is stronger, and the changes in precipitation more easily cause water table fluctuations in shrub peatlands, which ultimately causes the dominate plant and the decomposition degree in the peatland carbon pool to change. The increase in precipitation increased the water table and biomass of herbs or mosses in shrub peatlands, which was speculated to be the major reason for the decrease in shrub peatlands.
Except for carbohydrate content, the importance of HII in the peatland carbon pool was the lowest among the four selected factors. With HII increasing, the carbon content in shrub and herb/shrub peatlands decreased and the carbohydrate content slightly increased. The effects of HII on aromatic content in the peatland carbon pool were more marked than those of the aromatic content of degraded shrub peatlands. Similar to the aromatic contents, the effects of HII on the carbohydrate content and aromatic/carbohydrate ratio in degraded shrub peatlands were also the strongest, and the stability of the peatland carbon pool decreased with increasing HII. Although the effects of HII on natural shrub peatlands were slightly lower than those of degraded shrub peatlands, the increase in HII also decreased the stability of natural shrub peatlands more than that of natural herb and herb/shrub peatlands. The increase in the HII mainly caused more nutrients and pollution elements to accumulate in natural peatlands and decreased the surface water table due to regional agricultural water consumption (Gao et al., 2018). Thus, our results show that the carbon pool in shrub peatlands was more sensitive to regional human activities and climate change than in other peatland types. Compared with natural peatlands, degradation caused the shrub peatland carbon pool to be more easily influenced by regional human activities.
5. Conclusion
In this study, we identified the stability of the peatland carbon pool dominated by different peatland types and evaluated the effects of climate factors and human activities on the carbon pool in natural and degraded peatlands. Physicochemical factors of peat soils are the most important factors (especially bulk density) for the stability of the peatland carbon pool, and the annual average temperature is the most important environmental factor. The effects of environmental factors on carbon stability in peatlands covered by different plant types varied, and even the trend lines were opposite for some specific factors (e.g., annual average temperature and HII). Owing to the low water table and high frequency of aerobic/anaerobic changes in shrub peatlands, the stability of the carbon pool in shrub peatlands is more sensitive to climate change than in other peatland types. Compared to natural peatlands, the carbon stability in degraded herb peatlands and degraded herb/shrub peatlands was lower. Although the degradation process significantly increased the carbon stability in shrub peatlands, the degradation process also caused the shrub peatland carbon pool to be more easily influenced by regional human activities than in natural peatlands.
Data availability statement
The raw data supporting the conclusions of this article will be made available by the authors, without undue reservation.
Author contributions
JC, CG, and GW designed the research and provided the funding. JC and HZ ran the experiment, JC, DH, and FM analyze the data. JC and CG wrote the manuscript with input from HZ, DH, FM, and GW. All authors contributed to the article and approved the submitted version.
Funding
Financial support was provided by the National Natural Science Foundation of China (No. 42101108, 42171103), the Education Department of Heilongjiang Province (No. 135509145), the Youth Innovation Promotion Association CAS (No. 2020235), the Young Scientist Group Project of Northeast Institute of Geography and Agroecology, Chinese Academy of Sciences (2022QNXZ01), the Jilin Association for Science and Technology (QT202126), and the China Postdoctoral Science Foundation (2020M681059).
Acknowledgments
The authors gratefully acknowledge the assistance of the Analysis and Test Center of Northeast Institute of Geography and Agroecology, Chinese Academy of Sciences. The authors thank Prof. Hongwen Yu and Dr. Na Guo for FTIR analysis.
Conflict of interest
The authors declare that the research was conducted in the absence of any commercial or financial relationships that could be construed as a potential conflict of interest.
Publisher’s note
All claims expressed in this article are solely those of the authors and do not necessarily represent those of their affiliated organizations, or those of the publisher, the editors and the reviewers. Any product that may be evaluated in this article, or claim that may be made by its manufacturer, is not guaranteed or endorsed by the publisher.
References
Abd Rahman, J., and Ming, C. C. (2015). Physico-chemical characterization of peat at different decomposition levels. Electron. J. Geotech. Eng. 20, 4011–4019.
Bao, K., Xing, W., Yu, X., Zhao, H., McLaughlin, N., Lu, X., et al. (2012). Recent atmospheric dust deposition in an ombrotrophic peat bog in great Hinggan Mountain, Northeast China. Sci. Total Environ. 431, 33–45. doi: 10.1016/j.scitotenv.2012.05.014
Bragazza, L., Freeman, C., Jones, T., Rydin, H., Limpens, J., Fenner, N., et al. (2006). Atmospheric nitrogen deposition promotes carbon loss from peat bogs. Proc. Natl. Acad. Sci. U. S. A. 103, 19386–19389. doi: 10.1073/pnas.0606629104
Clarkson, B. R., Moore, T. R., Fitzgerald, N. B., Thornburrow, D., Watts, C. H., and Miller, S. (2013). Water table regime regulates litter decomposition in Restiad Peatlands, New Zealand. Ecosystems 17, 317–326. doi: 10.1007/s10021-013-9726-4
Cong, J., Gao, C., Han, D., Li, Y., and Wang, G. (2020). Stability of the permafrost peatlands carbon pool under climate change and wildfires during the last 150 years in the northern great Khingan Mountains. China. Sci. Total Environ. 712:136476. doi: 10.1016/j.scitotenv.2019.136476
Cong, J., Gao, C., Xing, W., Han, D., Li, Y., and Wang, G. (2022). Historical chemical stability of carbon pool in permafrost peatlands in northern great Khingan Mountains (China) during the last millennium, and its paleoenvironmental implications. Catena 209:105853. doi: 10.1016/j.catena.2021.105853
Dohong, A., Aziz, A. A., and Dargusch, P. (2017). A review of the drivers of tropical peatland degradation in South-East Asia. Land Use Policy 69, 349–360. doi: 10.1016/j.landusepol.2017.09.035
Drollinger, S., Knorr, K.-H., Knierzinger, W., and Glatzel, S. (2020). Peat decomposition proxies of alpine bogs along a degradation gradient. Geoderma 369:114331. doi: 10.1016/j.geoderma.2020.114331
Fenner, N., and Freeman, C. (2020). Woody litter protects peat carbon stocks during drought. Nat. Clim. Chang. 10, 363–369. doi: 10.1038/s41558-020-0727-y
Fick, S. E., and Hijmans, R. J. (2017). WorldClim 2: new 1-km spatial resolution climate surfaces for global land areas. Int. J. Climatol. 37, 4302–4315. doi: 10.1002/joc.5086
Gao, C., Knorr, K.-H., Yu, Z., He, J., Zhang, S., Lu, X., et al. (2016). Black carbon deposition and storage in peat soils of the Changbai Mountain, China. Geoderma 273, 98–105. doi: 10.1016/j.geoderma.2016.03.021
Gao, C., Zhang, S., Liu, H., Cong, J., Li, Y., and Wang, G. (2018). The impacts of land reclamation on the accumulation of key elements in wetland ecosystems in the Sanjiang plain, Northeast China. Environ. Pollut. 237, 487–498. doi: 10.1016/j.envpol.2018.02.075
Guo, X., Du, W., Wang, X., and Yang, Z. (2013). Degradation and structure change of humic acids corresponding to water decline in Zoige peatland, Qinghai-Tibet Plateau. Sci. Total Environ. 445–446, 231–236. doi: 10.1016/j.scitotenv.2012.12.048
Han, D., Gao, C., Liu, H., Li, Y., Cong, J., Yu, X., et al. (2021). Anthropogenic and climatic driven peatland degradation during the past 150 years in greater Khingan Mountains, NE China. Land Degrad. Dev. 32, 4845–4857. doi: 10.1002/ldr.4036
Heller, C., Ellerbrock, R. H., Roßkopf, N., Klingenfuß, C., and Zeitz, J. (2015). Soil organic matter characterization of temperate peatland soil with FTIR-spectroscopy: effects of mire type and drainage intensity. Eur. J. Soil Sci. 66, 847–858. doi: 10.1111/ejss.12279
Hodgkins, S. B., Richardson, C. J., Dommain, R., Wang, H., Glaser, P. H., Verbeke, B., et al. (2018). Tropical peatland carbon storage linked to global latitudinal trends in peat recalcitrance. Nat. Commun. 9:3640. doi: 10.1038/s41467-018-06050-2
Kareksela, S., Haapalehto, T., Juutinen, R., Matilainen, R., Tahvanainen, T., and Kotiaho, J. S. (2015). Fighting carbon loss of degraded peatlands by jump-starting ecosystem functioning with ecological restoration. Sci. Total Environ. 537, 268–276. doi: 10.1016/j.scitotenv.2015.07.094
Keddy, P. A. (2010). Wetland Ecology: Principles and Conservation. Cambridge, United Kingdom: Cambridge University Press.
Leifeld, J., Klein, K., and Wust-Galley, C. (2020). Soil organic matter stoichiometry as indicator for peatland degradation. Sci. Rep-UK 10:7634. doi: 10.1038/s41598-020-64275-y
Leifeld, J., and Menichetti, L. (2018). The underappreciated potential of peatlands in global climate change mitigation strategies. Nat. Commun. 9:1071. doi: 10.1038/s41467-018-03406-6
Leifeld, J., Steffens, M., and Galego-Sala, A. (2012). Sensitivity of peatland carbon loss to organic matter quality. Geophys. Res. Lett. 39, 1–6. doi: 10.1029/2012gl051856
Loisel, J., Gallego-Sala, A. V., Amesbury, M. J., Magnan, G., Anshari, G., Beilman, D. W., et al. (2020). Expert assessment of future vulnerability of the global peatland carbon sink. Nat. Clim. Chang. 11, 70–77. doi: 10.1038/s41558-020-00944-0
Loisel, J., van Bellen, S., Pelletier, L., Talbot, J., Hugelius, G., Karran, D., et al. (2017). Insights and issues with estimating northern peatland carbon stocks and fluxes since the last glacial maximum. Earth-Sci. Rev. 165, 59–80. doi: 10.1016/j.earscirev.2016.12.001
Loisel, J., Yu, Z., Beilman, D. W., Camill, P., Alm, J., Amesbury, M. J., et al. (2014). A database and synthesis of northern peatland soil properties and Holocene carbon and nitrogen accumulation. Holocene 24, 1028–1042. doi: 10.1177/0959683614538073
Lou, Y., Gao, C., Pan, Y., Xue, Z., Liu, Y., Tang, Z., et al. (2018). Niche modelling of marsh plants based on occurrence and abundance data. Sci. Total Environ. 616-617, 198–207. doi: 10.1016/j.scitotenv.2017.10.300
Lou, Y., Zhao, K., Wang, G., Jiang, M., Lu, X., and Rydin, H. (2015). Long-term changes in marsh vegetation in Sanjiang plain, Northeast China. J. Veg. Sci. 26, 643–650. doi: 10.1111/jvs.12270
Munir, T. M., Xu, B., Perkins, M., and Strack, M. (2014). Responses of carbon dioxide flux and plant biomass to water table drawdown in a treed peatland in northern Alberta: a climate change perspective. Biogeosciences 11, 807–820. doi: 10.5194/bg-11-807-2014
Negassa, W., Acksel, A., Eckhardt, K.-U., Regier, T., and Leinweber, P. (2019). Soil organic matter characteristics in drained and rewetted peatlands of northern Germany: chemical and spectroscopic analyses. Geoderma 353, 468–481. doi: 10.1016/j.geoderma.2019.07.002
Normand, A. E., Smith, A. N., Clark, M. W., Long, J. R., and Reddy, K. R. (2017). Chemical composition of soil organic matter in a subarctic Peatland: influence of shifting vegetation communities. Soil Sci. Soc. Am. J. 81, 41–49. doi: 10.2136/sssaj2016.05.0148
Paul, E. A. (2016). The nature and dynamics of soil organic matter: plant inputs, microbial transformations, and organic matter stabilization. Soil Biol. Biochem. 98, 109–126. doi: 10.1016/j.soilbio.2016.04.001
Poorter, H., Niklas, K. J., Reich, P. B., Oleksyn, J., Poot, P., and Mommer, L. (2012). Biomass allocation to leaves, stems and roots: meta-analyses of interspecific variation and environmental control. New Phytol. 193, 30–50. doi: 10.1111/j.1469-8137.2011.03952.x
Qiu, C., Zhu, D., Ciais, P., Guenet, B., Peng, S., and Xu, X. (2020). The role of northern peatlands in the global carbon cycle for the 21st century. Glob. Ecol. Biogeogr. 29, 956–973. doi: 10.1111/geb.13081
Rydin, H., and Jeglum, J. K. (2013). The Biology of Peatlands, 2e. Oxford, United Kingdom: Oxford University Press.
Šimanauskienė, R., Linkevičienė, R., Bartold, M., Dąbrowska-Zielińska, K., Slavinskienė, G., Veteikis, D., et al. (2019). Peatland degradation: the relationship between raised bog hydrology and normalized difference vegetation index. Ecohydrology 12:e2159. doi: 10.1002/eco.2159
Sjögersten, S., Caul, S., Daniell, T. J., Jurd, A. P. S., O'Sullivan, O. S., Stapleton, C. S., et al. (2016). Organic matter chemistry controls greenhouse gas emissions from permafrost peatlands. Soil Biol. Biochem. 98, 42–53. doi: 10.1016/j.soilbio.2016.03.016
Solomon, S., Qin, D., Manning, M., Averyt, K., and Marquis, M. (2007). Climate Change 2007-the Physical Science Basis: Working Group I Contribution to the Fourth Assessment Report of the IPCC. Cambridge, United Kingdom: Cambridge University Press.
Straková, P., Penttilä, T., Laine, J., and Laiho, R. (2012). Disentangling direct and indirect effects of water table drawdown on above- and belowground plant litter decomposition: consequences for accumulation of organic matter in boreal peatlands. Glob. Chang. Biol. 18, 322–335. doi: 10.1111/j.1365-2486.2011.02503.x
Verbeke, B. A., Lamit, L. J., Lilleskov, E. A., Hodgkins, S. B., Basiliko, N., Kane, E. S., et al. (2022). Latitude, elevation, and mean annual temperature predict peat organic matter chemistry at a global scale. Global Biogeochem. Cy. 36:e2021GB007057. doi: 10.1029/2021gb007057
Wang, Y., Paul, S. M., Jocher, M., Espic, C., Alewell, C., Szidat, S., et al. (2021b). Soil carbon loss from drained agricultural peatland after coverage with mineral soil. Sci. Total Environ. 800:149498. doi: 10.1016/j.scitotenv.2021.149498
Wang, H., Richardson, C. J., and Ho, M. (2015). Dual controls on carbon loss during drought in peatlands. Nat. Clim. Chang. 5, 584–587. doi: 10.1038/nclimate2643
Wang, H., Tian, J., Chen, H., Ho, M., Vilgalys, R., Bu, Z.-J., et al. (2021a). Vegetation and microbes interact to preserve carbon in many wooded peatlands. Commun. Earth Environ. 2, 1–8. doi: 10.1038/s43247-021-00136-4
WCS and CIESIN (2005). Last of the Wild Project, Version 2 (LWP-2): Last of the wild dataset (geographic). NASA Socioeconomic Data and Applications Center (SEDAC), Palisades, NY, 2005.
Wilson, R. M., Hopple, A. M., Tfaily, M. M., Sebestyen, S. D., Schadt, C. W., Pfeifer-Meister, L., et al. (2016). Stability of peatland carbon to rising temperatures. Nat. Commun. 7:13723. doi: 10.1038/ncomms13723
Wilson, R. M., Hough, M. A., Verbeke, B. A., Hodgkins, S. B., IsoGenie, C., Chanton, J. P., et al. (2022). Plant organic matter inputs exert a strong control on soil organic matter decomposition in a thawing permafrost peatland. Sci. Total Environ. 820:152757. doi: 10.1016/j.scitotenv.2021.152757
Wu, Y., Blodau, C., Moore, T. R., Bubier, J., Juutinen, S., and Larmola, T. (2015). Effects of experimental nitrogen deposition on peatland carbon pools and fluxes: a modelling analysis. Biogeosciences 12, 79–101. doi: 10.5194/bg-12-79-2015
Wu, J., and Roulet, N. T. (2014). Climate change reduces the capacity of northern peatlands to absorb the atmospheric carbon dioxide: the different responses of bogs and fens. Global Biogeochem. Cy. 28, 1005–1024. doi: 10.1002/2014GB004845
Xing, W., Bao, K., Gallego-Sala, A. V., Charman, D. J., Zhang, Z., Gao, C., et al. (2015). Climate controls on carbon accumulation in peatlands of Northeast China. Quaternary Sci. Rev. 115, 78–88. doi: 10.1016/j.quascirev.2015.03.005
Xu, J., Morris, P. J., Liu, J., and Holden, J. (2018). PEATMAP: refining estimates of global peatland distribution based on a meta-analysis. Catena 160, 134–140. doi: 10.1016/j.catena.2017.09.010
Ye, R., Jin, Q., Bohannan, B., Keller, J. K., McAllister, S. A., and Bridgham, S. D. (2012). pH controls over anaerobic carbon mineralization, the efficiency of methane production, and methanogenic pathways in peatlands across an ombrotrophic–minerotrophic gradient. Soil Biol. Biochem. 54, 36–47. doi: 10.1016/j.soilbio.2012.05.015
Yu, Z., Loisel, J., Brosseau, D. P., Beilman, D. W., and Hunt, S. J. (2010). Global peatland dynamics since the last glacial maximum. Geophys. Res. Lett. 37, 1–5. doi: 10.1029/2010gl043584
Zhao, X., Chen, J., Guo, M., Li, C., Hou, N., and Bai, S. (2022). Constructed wetlands treating synthetic wastewater in response to day-night alterations: performance and mechanisms. Chem. Eng. J. 446:137460. doi: 10.1016/j.cej.2022.137460
Keywords: peatland, carbohydrate, aromatic, carbon stability, FTIR, Changbai Mountain
Citation: Cong J, Gao C, Zhao H, Han D, Meng F and Wang G and (2023) Chemical stability of carbon pool in peatlands dominated by different plant types in Jilin province (China) and its potential influencing factors. Front. Ecol. Evol. 11:1171688. doi: 10.3389/fevo.2023.1171688
Edited by:
Zhongqing Yan, Chinese Academy of Forestry, ChinaReviewed by:
Meng Wang, Northeast Normal University, ChinaMinna Maria Väliranta, University of Helsinki, Finland
Copyright © 2023 Cong, Gao, Zhao, Han, Meng and Wang. This is an open-access article distributed under the terms of the Creative Commons Attribution License (CC BY). The use, distribution or reproduction in other forums is permitted, provided the original author(s) and the copyright owner(s) are credited and that the original publication in this journal is cited, in accordance with accepted academic practice. No use, distribution or reproduction is permitted which does not comply with these terms.
*Correspondence: Chuanyu Gao, gaochuanyu@iga.ac.cn; Guoping Wang, wangguoping@iga.ac.cn