- 1College of Geographical Science / Provincial Key Laboratory of Mongolian Plateau’s Climate System, Inner Mongolia Normal University, Hohhot, China
- 2Institute of Environment and Sustainable Development in Agriculture, Chinese Academy of Agricultural Sciences, Beijing, China
- 3College of Grassland, Resources and Environment, Inner Mongolia Agricultural University, Hohhot, China
Introduction: Climate change, characterized by rising temperatures and changing precipitation patterns, has emerged as a significant global concern. Particularly, the warming potential of CH4 is 28 times greater than that of CO2, leading to an increased focus on its impact. Among various ecosystems, grasslands exhibit a high vulnerability to climate change. Grassland in Inner Mongolia is an important component of the typical grassland in Eurasian, and there was evidence that warmer and more precipitation in this area in future.
Methods: In this study, we utilized an open-top chamber (OTC) to conduct warming and precipitation experiments on a representative steppe located in Inner Mongolia in 2011. From 2017 to 2019, we monitored various factors, including soil temperature, moisture, CH4 flux, community characteristics, soil carbon nitrogen content. Subsequently, we analyzed the response of CH4 flux and its influencing factors to warming and precipitation in this typical steppe.
Results: The soil in the typical steppe acted as a CH4 sink. In 2018, CH4 flux during the growing season and t during the non-growing season were −59.31 and −21.21 under C, −56.55 and −31.17 under T, −41.34 and −24.93 under P, −50.09 and −26.51 ug C·m−2·h−1 under TP respectively. Warming stimulated absorption of CH4 during the non-growing season (25.8%), while the addition of precipitation hindered CH4 absorption during the growing season (76.37%). Warming and precipitation addition decreased the percentage of CH4 absorbed in growing season and increased that in non-growing season which account 67.66% and 32.34% under C, 59.81% and 40.19% under T, 62.71% and 37.29% under P, 62.03% and 37.97% under TP respectively. Soil temperature exhibited a positive correlation with CH4 flux (P<0.001), while the dominance of Leymus chinensis (IV-L.c.) exhibited a negative correlation with CH4 flux (P<0.01). Moreover, NH4+-N displayed a positive correlation with CH4 flux (P<0.05).
Conclusion: The findings suggest that CH4 absorption in a typical steppe may increase in a warmer future, and warming is conducive to the absorption of CH4 in the non-growing season. Not only abiotic factors had an impact on CH4 absorption, but also changes in community composition. Consequently, further exploration of the underlying mechanisms is warranted.
1 Introduction
Global warming has garnered considerable attention worldwide, with the increasing concentration of carbon dioxide (CO2), methane (CH4), and nitrous oxide (N2O) being significant contributors to climate change (IPCC, 2021). CH4 the second-largest greenhouse gas after CO2, possesses a potent warming potential and can raise surface temperatures by absorbing long-wave radiation emitted by the Earth’s surface. Grasslands represent crucial terrestrial ecosystems, and the Inner Mongolian grassland, situated at the eastern edge of Eurasia, serves as a vital ecological barrier in northern China, making it highly susceptible to climate change. Investigating the variations and influencing factors of greenhouse gas fluxes in typical grasslands and understanding the feedback and response of grassland ecosystems in the context of climate change are of utmost importance.
Scholars have explored CH4 fluxes during both the growing and non-growing seasons. For instance, CH4 absorption rates in alpine meadows on the Qinghai-Tibet Plateau are higher in summer and lower in winter (Chen W. et al., 2019). CH4 oxidative absorption occurs in temperate semi-arid grasslands during winter with a low absorption rate (Wang et al., 2011). Meadows exhibit net CH4 absorption throughout the year, primarily during the growing season, while the non-growing season weakens the sink’s ability to emit CH4 (Li et al., 2022). Warming affects CH4-producing and oxidising bacteria by altering environmental factors such as soil temperature, moisture, permeability, and nutrient levels within the ecosystem (Dijkstra et al., 2011; Dijkstra et al., 2013; Lin et al., 2015), consequently affecting soil CH4 flux. The results showed that the methane oxidation rate was 0.14 ± 0.04mg·cm−2·d−1 in grasslands with an annual average temperature of −7.8°C (Li et al., 2020). In alpine grasslands with an annual average temperature of 0.08°C, the methane oxidation rate significantly increased with a 1°C temperature rises (Li et al., 2020). In the range of −10 to 30°C, the amount of methane oxidation increases with the increase of temperature (Chen et al., 2010). Furthermore, when the soil temperature rises from 18°C to 28°C, the activity of methanotrophs increases (Ma et al., 2016). Changes in precipitation affect the production or oxidation processes of soil CH4 and regulate its emission or absorption (Xu et al., 2015a). For example, reduced precipitation promotes CH4 oxidation in tropical and temperate forest soils, thereby enhancing CH4 uptake (Savi et al., 2016; Yan et al., 2019; Wu et al., 2020a; Wu et al., 2021). As the temperature and precipitation vary across different grassland ecosystems, the effects of temperature rise and precipitation fluctuations on CH4 flux also differ among various grassland types. Furthermore, limited continuous observations of CH4 fluxes in different seasons in previous studies have resulted in gaps in understanding the influence of temperature and precipitation changes on CH4 dynamics.
Climate change also affects soil carbon and nitrogen pools (Zhang et al., 2018; Wei et al., 2020; Xie et al., 2020; Wang et al., 2022) leading changes in methane flux. Soil soluble carbon and nitrogen act as nutrient sources that influence the activity of methanotrophs and control soil CH4 oxidation processes (Shukla et al., 2013). For instance, changes in soil dissolved organic carbon content can affect soil CH4 flux by limiting the carbon mineralization process of methane-oxidizing bacteria, and indirectly impact the soil CH4 oxidation process by affecting other soil characteristics such as water content and soil inorganic nitrogen content (Tate, 2015). Inorganic nitrogen has long been considered an important factor in regulating soil CH4 oxidation capacity. The addition of NH4+ may inhibit, promote, or even have no significant effect on the soil CH4 oxidation process (Bodelier, 2011; Shukla et al., 2013; Tate, 2015). Additionally, variations in biomass, nutrient uptake, and photosynthetic characteristics among different plant species contribute to differences in ecosystem nitrogen and carbon cycles, as well as greenhouse gas fluxes (Han and Chen, 2020). Alterations in plant species composition significantly reduce CH4 flux, primarily due to a decrease in soil active carbon content (such as microbial biomass carbon) and soil enzyme activity (Luan et al., 2016). The effects of grassland species richness and functional group presence on CH4 production found that the emission flux of species mixtures can be significantly higher than that of the species monoculture because of the significant increase in biomass (Khalsa et al., 2014). Moreover, plant species richness can significantly increase CH4 flux (Zhang et al., 2012). However, few studies have examined how simulated climate change affects CH4 flux through vegetation. While abiotic factors are often considered when explaining the influencing factors of CH4, the response of biological factors, particularly vegetation community composition, to climate change is intuitively significant. Thus, understanding the impact of climate change on CH4 flux should not neglect the role of vegetation.
To obtain more accurate observations of the feedback on the CH4 flux and climate change, long-term monitoring is essential. Therefore, the aim of this study was to conduct warming and precipitation addition experiments in Inner Mongolia, China, in 2011, based on the climate change trend in temperate steppes. The study sought to elucidate (1) how warming and precipitation addition affect CH4 emission fluxes in temperate steppes, particularly during the growing and non-growing seasons, and (2) the main factors influencing CH4 fluxes. By monitoring vegetation and soil nutrient changes and analysing their relationship with greenhouse gas fluxes, we aimed to reveal the response of greenhouse gas fluxes to climate change in a typical steppe, providing further insights into the temporal dynamics and influencing factors of CH4 flux in grassland ecosystems.
2 Research method
2.1 Study site
The study site is located at the Grassland Ecology Research Base of Inner Mongolia University in Mauden Pasture (44°09’N, 116°29’” E, 1102 m above sea level), 40 km east of XilinHot (Figure 1). The region experiences a temperate continental climate characterised by cold and dry winters and hot and humid summers. The average annual temperature is 2.6°C, with the lowest temperature occurring in January (−23.8°C) and the highest temperature in July (24.9°C). The average annual precipitation is 271.42 mm, concentrated between May and September, which accounts for 87.3% of the total annual precipitation. The annual evaporation ranges from 1600 to 1800 mm. The soil in the study area is chestnut soil, and the vegetation type is typical steppe dominated by Stipa krylovii and Leymus chinensis.
2.2 Experimental design
The simulated warming and precipitation addition experiment commenced in July 2011 using open-top chamber (OTC). The OTC were fixed in the plots throughout the year to avoid disturbance. The warming treatment in this experiment was set at 2°C based on the IPCC Fourth Assessment Report. The OTC is a cone-shaped device made of polycarbonate (PC board) with a fan for temperature control. The upper bottom surface diameter of the OTC was 0.7 m, the lower bottom surface diameter was 1.2 m, and the height was 0.4 m. Precipitation addition was conducted twice each month during the growing season, equivalent to 20% of the average monthly precipitation from 1961 to 2010 (Wan et al., 2018). Precipitation was added after 18:00 to avoid damage to plants during high daytime temperatures. Four treatments were applied: control (CK), warming (T), warming and precipitation (TP), and precipitation (P). Each treatment had 4 replicates, resulting in a total of 16 experimental plots (Figure 1).
The greenhouse gas collection in this experiment used the platform of warming and precipitation addition experiment to collect and measure greenhouse gases in 2017–2018 after 6 years of continuous warming and precipitation addition and continued the warming and precipitation addition experiment.
2.3 Determination method
2.3.1 Determination of soil temperature and moisture
Temperature and moisture measurements were measured using a data collector with a (temperature probe model: DS18B20; humidity probe model: EC-5). Soil temperature and humidity were recorded at 10 cm intervals, and data were automatically recorded every 30 min.
2.3.2 Determination of greenhouse gas concentration
Static chamber gas chromatography was used to measure greenhouse gas concentration. The static chamber material was a PVC cylinder with a diameter of 0.4 m and a height of 0.3 m, were. The static chamber was placed at the base to collect the gas. Gas samples were collected at 0, 10, 20, and 30 minutes and injected into sealed glass gas cylinders. The sampling time was from May 2017 to February 2019, with measurements taken every 7 days during the growing season (May to early September) was measured every 7 days. During the spring freeze–thaw period (March to April), it was measured every 4 days, in late September to October every 15 days, and once a month from November to February of the following year. The collected samples were analyzed for CH4 concentration using gas chromatography (HP6890N, Agilent Company). The CH4 flux was calculated based on the difference in gas concentration within the time range of the static chamber (70).
2.3.3 Investigation of community characteristics
The community characteristics and biomass were determined using quadrat surveys. Monthly surveys were conducted during the growing season (May to August) in each plot, with a sampling area of 1×1m2. The surveys included assessments of community species composition, density, and height.
At the end of August, the growing season, the quadrat was selected outside the sample plot, and the plants in the quadrat were cut off on the ground and seeded into envelopes. They were then oven-dried at 65°C for 48 h to determine peak above-ground biomass (Xu et al., 2015b). A regression equation was used to calculate the biomass in the plot (Table S1).
2.3.4 Determination of soil carbon and nitrogen
Soil samples were collected at the end of August in 2017 and 2018, with the top 10 cm of soil collected using a 3 cm diameter soil drill. Fresh soil samples were partially air-dried and passed through a 0.15 mm sieve. The sieved soil was placed in tin cups for sample packaging. The total carbon and nitrogen content of the soil were determined using an elemental analyzer (Thermo Fisher, Thermo Fisher Scientific, USA). The microbial biomass, carbon, and nitrogen were determined using the chloroform fumigation method. After sieving the fresh soil through a 2 mm sieve, three portions of fresh soil (20 g each) were weighed. One portion was placed in an aluminum box and dried in an oven at 70°C to determine dry weight. The remaining two soil samples were placed in a 50 ml beaker. One of the beakers was placed into a vacuum pumping vessel and, at the same time, placed in a beaker containing 25 ml of chloroform. The chloroform was vacuum boiled for 5 min, and the piston and air pump were closed. The beaker was covered with black cloth, fumigated in the dark. for 24 h, removed, vacuumed three times for 5 min each time, and vacuum chloroform was added. Another beaker was used as a control without the fumigation treatment; the other treatments were consistent and placed under the same conditions. After 24 h, the treated soil samples were transferred to centrifuge tubes, and each bottle was injected with 50 ml 0.5 mol/L K2SO4 solution, shocked for 30 min, centrifuged, and filtered, and the filtrate was analyzed using a Multi N/C 3000 DOC analyzer (German Jena company). The extractable carbon and nitrogen were converted to MBC and MBN using conversion factors (Kec and Ken) of 0.45 both (Xu et al., 2010). NH4+-N and NO3−−N were determined by a continuous flow analyzer with 2 mol·L−1 KCL solution and water–soil ratio of 5:1. The soil samples (10 g) were weighed, and distilled water was added according to the ratio of water to soil (2:1). The soil samples were shaken at 25°C for 30 min and filtered with 0.45 μm filter membrane. The DOC and DON in the filtrate were determined using a Multi N/C 3000 DOC analyzer (Jena, Germany).
2.4 Data calculation and analysis
2.4.1 Greenhouse gas flux calculation
In the formula, F is the greenhouse gas flux, CH4 flux unit is (ug·m−2·h−1). A positive value represents emissions, and a negative value represents absorption. ρ is the density of a greenhouse gas under standard conditions, h is the height of the sampling static chamber (m), is the rate of change of a gas concentration with time, and T is the air temperature (°C) at the time of sampling.
2.4.2 Data analysis method
Microsoft Excel 2016 and IBM SPSS Statistics 24.0 (SPSS Inc., Chicago, IL, USA) were utilized for data processing and analysis. One-way analysis of variance (ANOVA) was employed to analyze the significance of differences between different treatments, and multiple comparisons were conducted using Duncan’s method. Correlation analysis was performed to analyze the relationship between greenhouse gas fluxes, environmental factors, and nutrients (results of soil carbon and nitrogen used in correlation heat map analysis in Table S2; results of community characteristics used in correlation heat map analysis in Tables S3 and S4). We used piecewise structural equation modelling (SEM) with AMOS 21.0 (Amos Development Co., Armonk, New York, USA) to evaluate the effects of temperature and moisture changes on CH4 emissions by affecting vegetation and soil carbon and nitrogen pools. Before establishing SEM, we conducted a stepwise regression analysis of CH4 flux and its influencing factors, and established formulas, CH4= −337.40 + 146.27 IV-L.c + 3.03 MBN (F = 10.92, P = 0.001). Finally, we verify the feasibility of the model through P > 0.05.
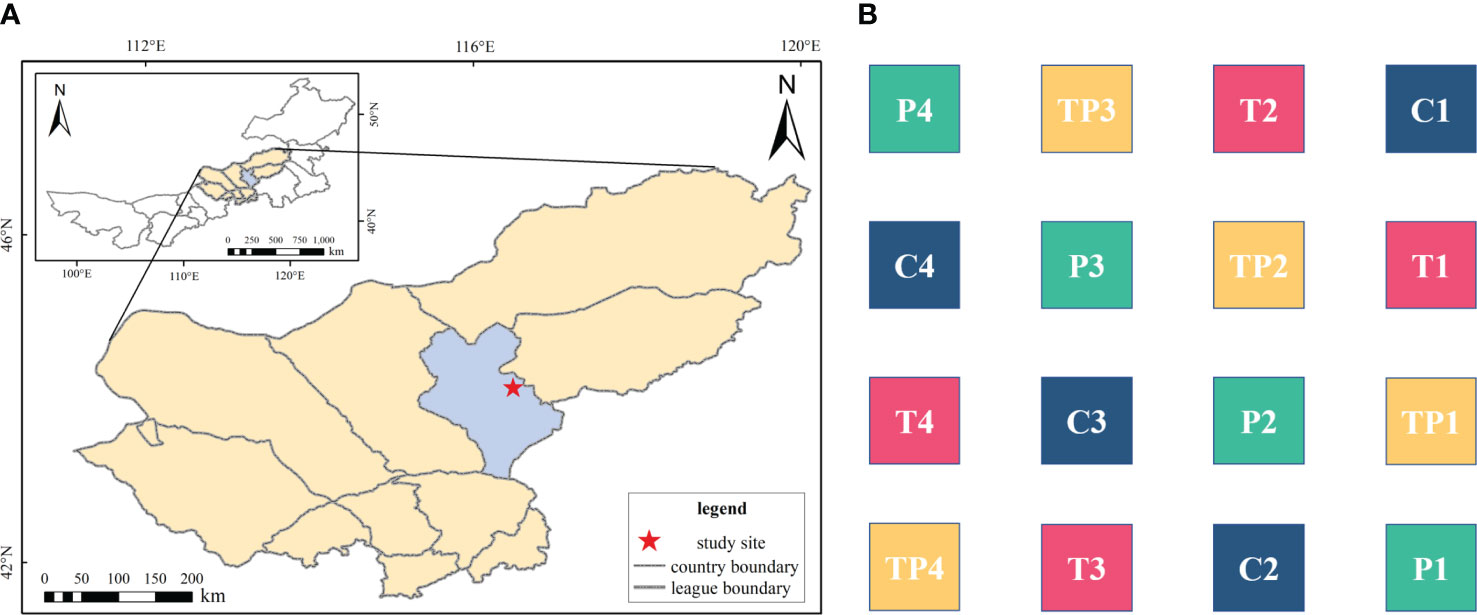
Figure 1 Study site (A) and the schematic diagram of experimental warming design (B). Control (C), warming (T, warming 2 °C), warming plus precipitation addition (TP, warming 2 °C plus precipitation addition 20%), and precipitation (P, precipitation addition 20%).
3 Results and analysis
3.1 Effects of warming and precipitation addition on CH4 flux in typical steppe
Typical steppe soil acts as a sink for CH4, with higher CH4 absorption during the growing season compared to the non-growing season (the percentage of CH4 absorption during the growing season to the non-growing season ranges from 1.27 to 2.47) (Figures 2, 3, 4B). The average CH4 flux during the growing season accounted for 55.80% to 69.71% of the entire year under different treatments (Figure 4A), while the non-growing season contributed 30.29% to 44.2% of the annual CH4 flux (Figure 4A). Warming and precipitation addition decreased the percentage of CH4 absorbed in growing season and increased that in non-growing season which account 67.66% and 32.34% under C, 59.81% and 40.19% under T, 62.71% and 37.29% under P, 62.03% and 37.97% under TP respectively. The addition of precipitation (P treatment) reduced the absorption of CH4 by the soil, likely due to decreased soil permeability caused by increased precipitation, which hinders the diffusion of CH4 into the soil. On the other hand, warming promoted the absorption of CH4 in typical steppe ecosystems.
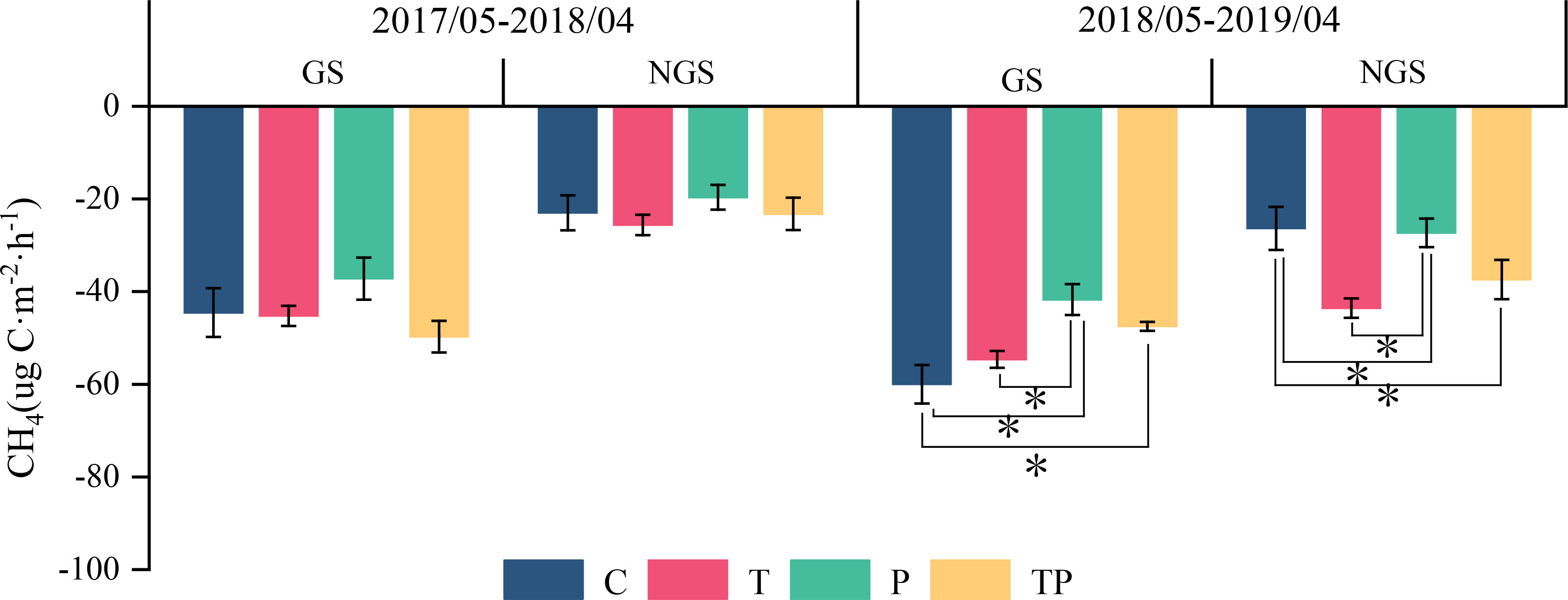
Figure 3 Effects of warming and precipitation addition on CH4 flux in growing and non-growing seasons.
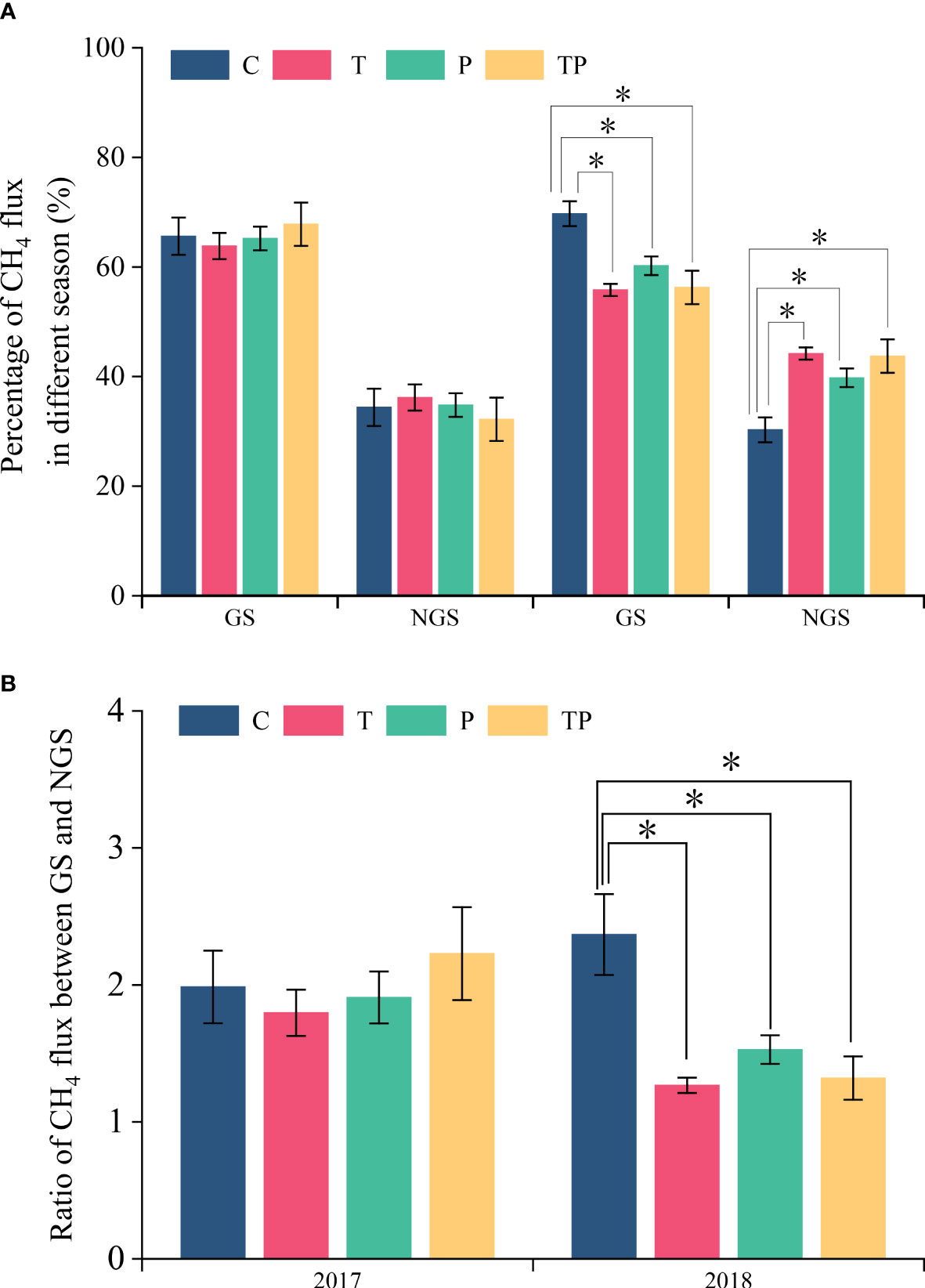
Figure 4 Percentage of CH4 flux in growing season and non-growing season in the whole year and CH4 emission of GS to NGS. C, Control, no warming and no precipitation addition; W, warming; P, precipitation addition; WP, warming plus precipitation addition; GS, growing season; NGS, non-growing season; * significant at P<0.05.
3.2 Relationships between CH4 and environmental factors
Warming and precipitation addition significantly affected soil temperature and moisture. Warming increased soil temperature and decreased soil moisture, while precipitation addition decreased soil temperature and increased soil moisture. Combined warming and precipitation addition can alleviate the adverse effects of individual warming on the soil environment (Wan et al., 2018). Soil temperature showed a significant positive correlation with CH4 flux (P<0.001). In this study, the variation in soil surface temperature explained the variation in CH4 flux well (R2=0.52) (Figure 5). With seasonal changes, the decrease in soil moisture caused by warming leaded to increased soil permeability (Juc´a and Maciel, 2006; Lu et al., 2023), promoting the diffusion of CH4 from the atmosphere into the deep soil and thereby enhancing CH4 absorption. In a typical steppe in semiarid areas, the increase in water may not reach the threshold level that affects methanotrophs, hence no significant correlation between CH4 flux and soil surface moisture was noted.
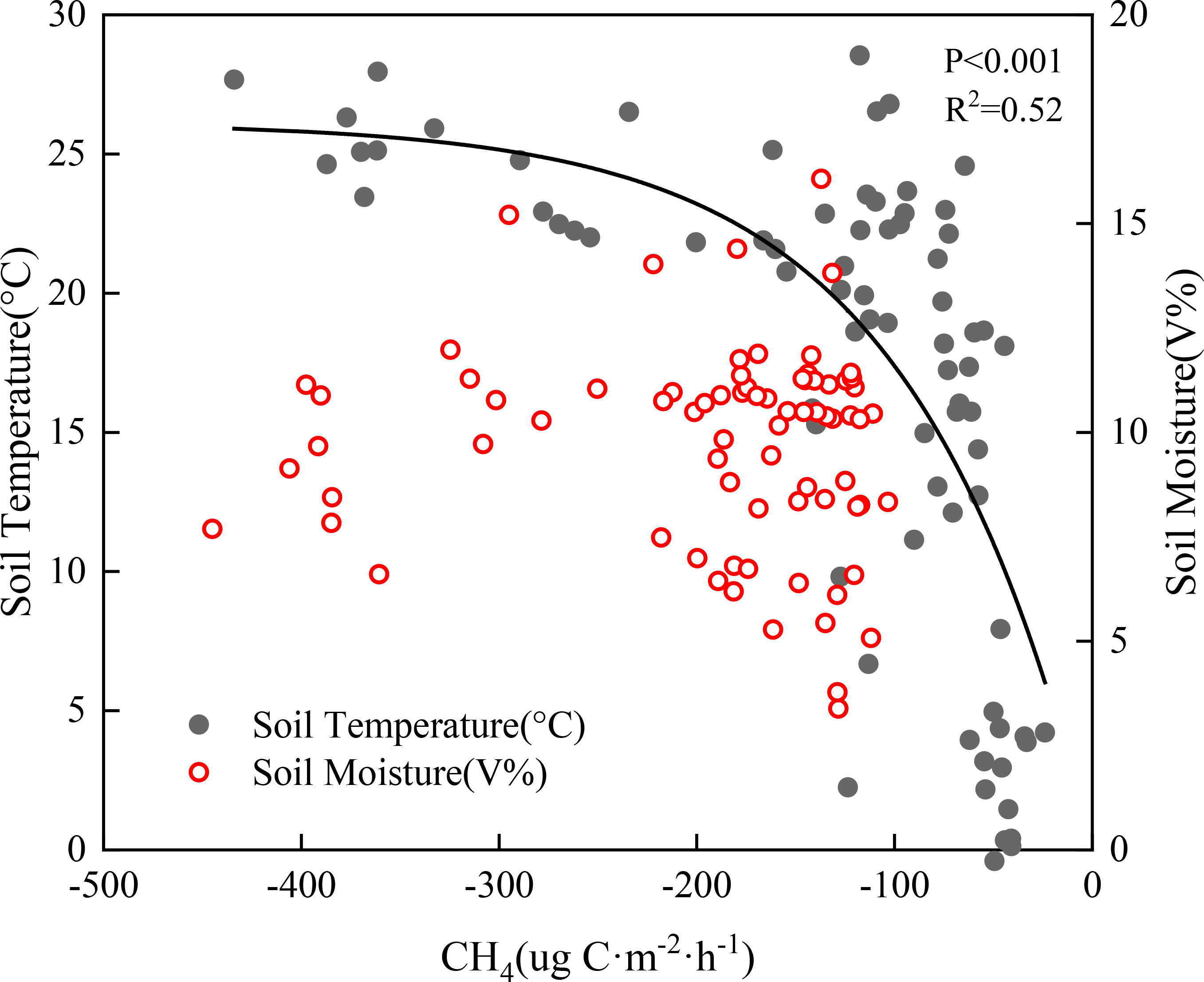
Figure 5 Correlation between soil temperature, soil moisture, and CH4 flux under warming and precipitation addition conditions.
3.3 Relationships between CH4 flux with soil factors and community factors
Community species diversity decreased, Leymus chinensis biomass increased, and Stipa krylovii biomass decreased due to warming and precipitation addition (community factors, soil carbon, and nitrogen under warming and precipitation are shown in Tables S2–S4).
The correlation heat map of CH4 flux, which used negative values (higher absolute value indicating higher absorption), revealed that IV-L. c. is positively correlated with CH4 flux (P<0.01). The Simpson and Shannon indices were negatively correlated with CH4 flux (P<0.05). NH4+-N was negatively correlated with CH4 flux (P<0.05). The increase in the dominance of Leymus chinensis hampered CH4 absorption, whereas an increase in species diversity and NH4+ content promoted CH4 absorption. CH4 flux was not only affected by soil carbon and nitrogen but also by vegetation species diversity and dominant species dominance (Figure 6).
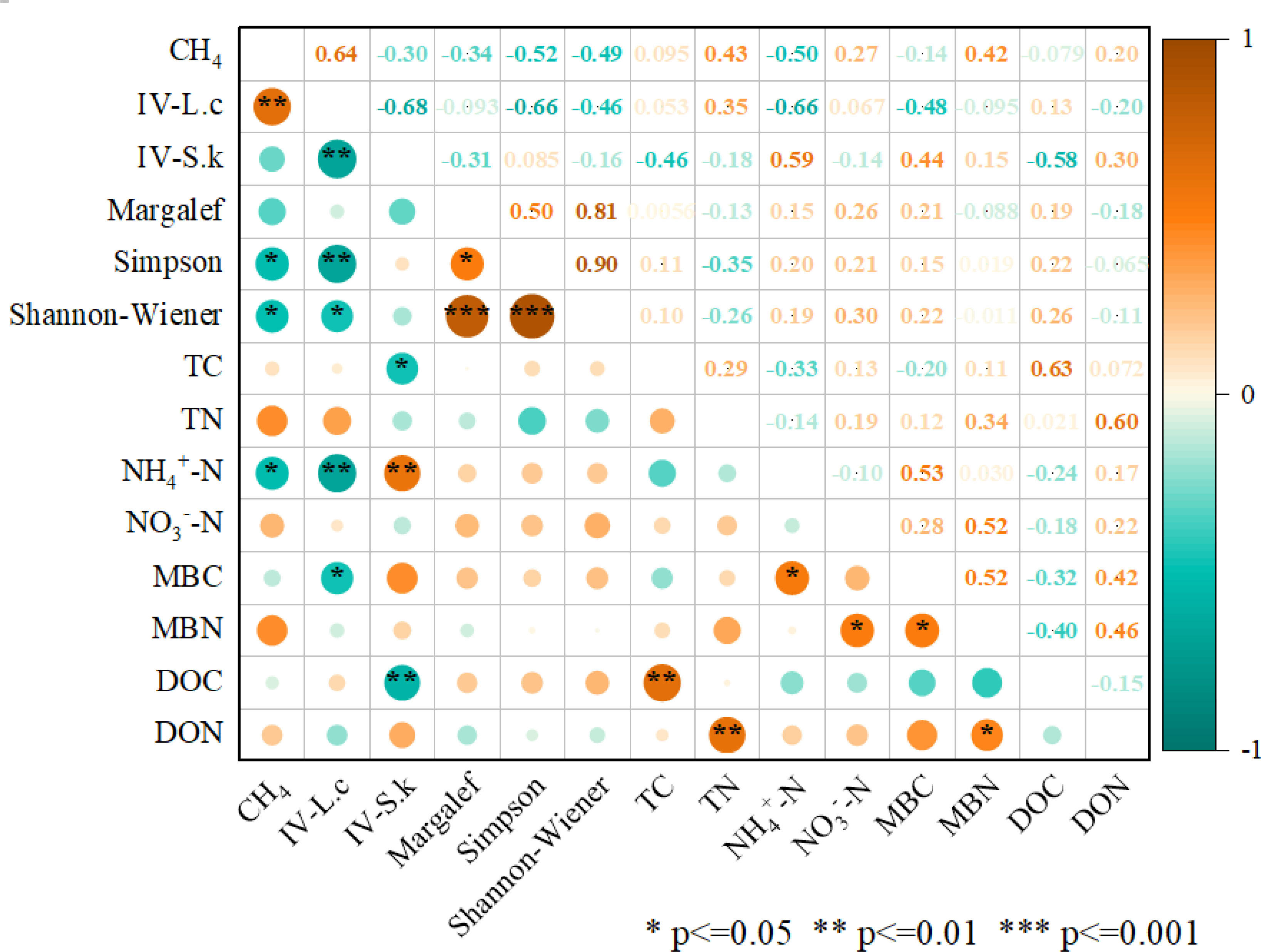
Figure 6 Correlation heat map of CH4 flux with soil factors and community factors. Significant differences shown at * P<0.05; ** P<=0.01; ***P<=0.001. IV-L.c, important value of Leymus chinensis; IV-S.k, important value of Stipa krylovii; Margalef, Margalef richness index; Simpson, Simpson’s diversity; TC, Total carbon; TN, Total nitrogen; NH4+-N, Ammonniacal nitrogen; NO3–N, Nitrate nitrogen; MBC, Microbial carbon; MBN, Microbial nitrogen; DOC, Dissolved organic carboon; DON, Soluble organic nitrogen.
Warming mainly affected CH4 flux by influencing IV-L. c. Precipitation addition precipitation addition primarily affected CH4 flux through its influence on soil nitrogen (Figure 7).
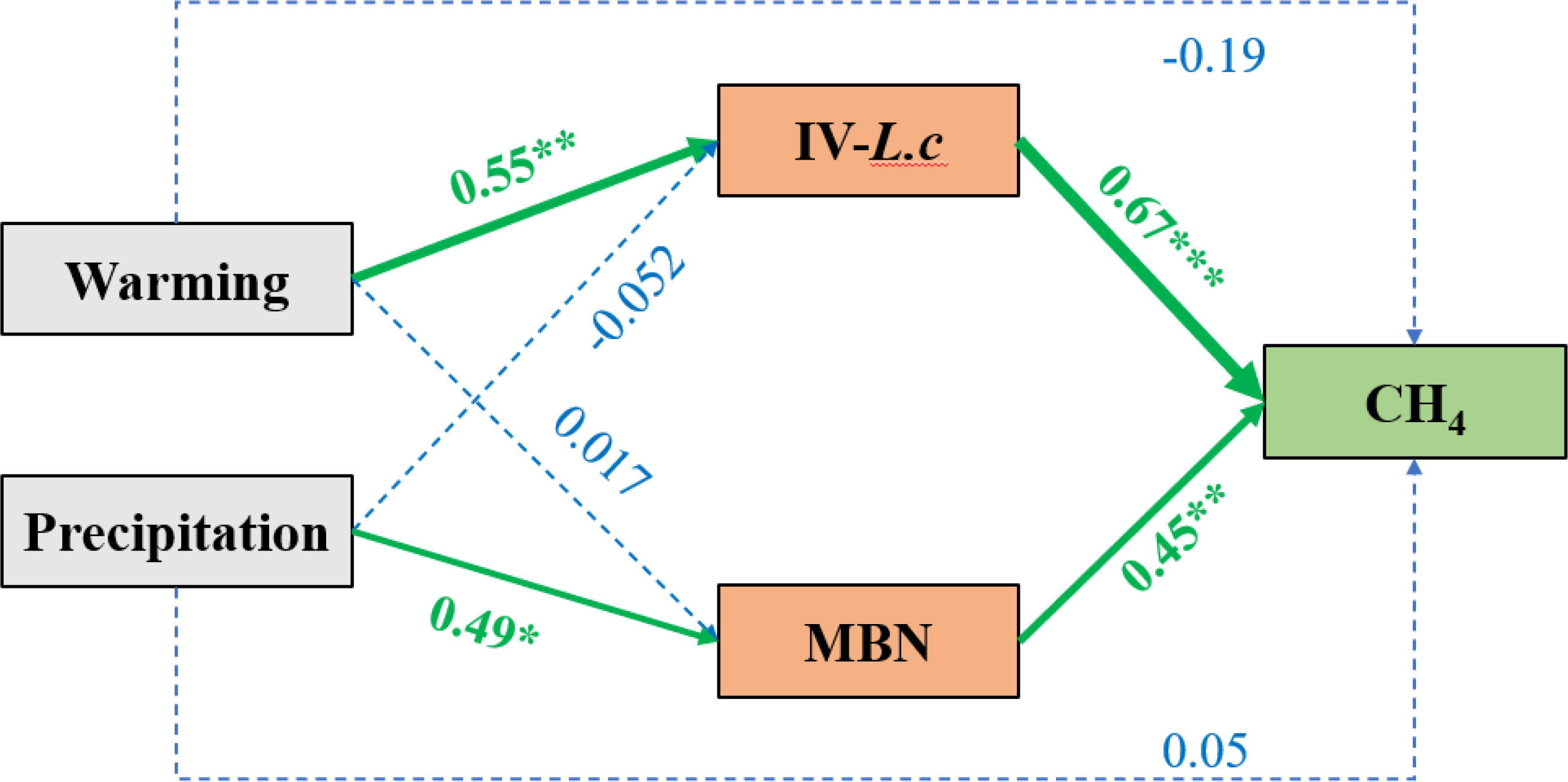
Figure 7 SEM model analysis of the influence mechanism of warming and precipitation on CH4 emission. Chi-square = 0.034; Probability level = 0.854. Significant differences shown at * P<0.05; ** P<=0.01; ***P<=0.001. IV-L.c, important value of Leyms chinensis; MBN, microbial biomass nitrogen. The thickness of the lines indicated the strength of the correlation. Green lines represent a significant positive correlation; red line represents a significant negative correlation; blue lines indicate that the correlation is not significant.
4 Discussion
4.1 Effects of warming and precipitation addition on greenhouse gas flux in typical steppe
Grassland ecosystems act as sinks for CH4. Air temperature is an important factor that affects soil CH4 uptake, it can affect the activity of methanotrophs in the soil. Within a certain temperature range, the oxidation rate of CH4 in the soil increases with rising temperature. Additionally, warming leads to reduced soil water content through increased evaporation (Bokhorst et al., 2008), and soil water can exert physiological pressure on methanotrophs, which in turn affects the diffusion of CH4 in the soil (Luo et al., 2013; Li et al., 2015). In addition to soil temperature, soil moisture is also an important factor influencing CH4 flux. Decreased water content results in thinner plant roots, increased soil nitrogen cycling rates, enhanced methanotrophic community, reduced methanogen community, and increased CH4 uptake (Konda et al., 2010; Lawrence et al., 2015; Liu et al., 2015). Water addition, on the other hand, reduces soil permeability, hinders CH4 transmission from the atmosphere to the soil, and decreases CH4 absorption (Conrad, 2007). However, an increase in soil water content creates an anaerobic environment favorable for CH4-producing bacteria, resulting in reduced CH4 absorption and increased CH4 emissions (Dijkstra et al., 2013). In dry years, the increase in soil moisture caused by the addition of precipitation did not reach the threshold level to affect methanotrophs, thus having no significant impact on CH4 uptake (Zhang et al., 2017). However, other studies have suggested that net methane uptake increases with humidity under drought conditions (Von et al., 2009). The results differed in 2017, which was a more drought-prone year with higher temperatures and lower precipitation compared to the period of 2012–2022. Additionally, warming can promote CH4 absorption, while precipitation had an inhibitory effect on CH4 absorption, which is consistent with the results of Zhu et al. (2015) and Xu et al. (2015a). This indicates that temperature and water jointly regulate CH4 fluxes in typical steppes in arid and semi-arid regions, with increasing temperature strengthening CH4 absorption in the area. Warming increased CH4 absorption was closely correlated with soil temperature and soil moisture (Wang et al., 2021a). Warming-induced increase in soil temperature directly enhances methanotrophic and decreases methanogen abundance (Zheng et al., 2012; Peltoniemi et al., 2016), thus resulting in an enhancement of soil CH4 absorption. Furthermore, a lower soil water content was found under warming, which could have decreased anaerobic conditions by increasing air permeability and O2 diffusion in the soil (Chen et al., 2017), favoring microbial oxidation of CH4 (Dijkstra et al., 2013).
4.2 Effects of vegetation and soil carbon and nitrogen on greenhouse gas fluxes under warming and precipitation addition conditions
Simulated warming and precipitation have significant effects on soil carbon and nitrogen pools (Zhang et al., 2018; Wei et al., 2020; Wang et al., 2022), and greenhouse gas emissions are closely related to the stability of these pools (Wu et al., 2020b). Grasslands act as sinks for CH4, and therefore, increased soil nitrogen can inhibit CH4 oxidation by raising NH4+ concentration, resulting in reduced CH4 absorption. However, more soil nitrogen can alleviate the nitrogen limitation in grassland soil microorganisms, enhance microbial activity, and promote CH4 absorption. Thus, the effect of soil nitrogen on grassland CH4 flux is complex and depends on multiple factors. Previous studies have yielded different conclusions, with some reporting that in Inner Mongolian grasslands, nitrogen addition reduces soil CH4 uptake (Zhang et al., 2017). In the Tianshan grassland, nitrogen addition promotes CH4 uptake in (Li et al., 2012). Furthermore, the addition had no significant effect on CH4 emissions (Zhao et al., 2017). In the present study, soil NH4+ promoted CH4 absorption, while soil NO3− inhibited it. Soil NO3− increases soil redox potential, exerting osmotic pressure on methanotrophs and exhibiting toxic effects on them. Long-term nitrogen addition leads to changes in the methanotroph community and reduced abundance, ultimately inhibiting CH4 absorption (Treseder, 2008; Li et al., 2020). Thus, high concentrations of NO3− in soil can suppress methanotroph activity (Yue et al., 2016; Chen S. et al., 2019; Zhang et al., 2019; Pan et al., 2022), affecting their abundance and reducing CH4 absorption. Warming and precipitation affected CH4 flux by influencing soil nitrogen. Direct and indirect positive correlations were observed between precipitation enhancement and soil N and CH4 fluxes. However, increased temperature did not facilitate the effect of soil nitrogen or promote greenhouse gas emissions. Considering the warming and drying trends expected under future climate change, increasing CH4 absorption in the typical steppe area of Inner Mongolia would be beneficial.
Soil temperature and moisture explain most of the seasonal variations in CH4 fluxes, but the effects of environmental factors on greenhouse gas emissions and uptake are not only direct but also indirect through their influence on soil nutrients and vegetation growth (Shaver et al., 2000; Sampson et al., 2007; Aires et al., 2008; Xu and Wan, 2008; Kuzyakov and Gavrichkova, 2010; Nakano and Shinoda, 2010; Potthast et al., 2010; Phillips et al., 2012). Warming can impact species richness by altering soil nutrient content and plant interspecific relationships (Nogueira et al., 2017), while increased water availability improves soil water availability, alleviating the inhibitory effects of water deficit on plant growth (Xu et al., 2014), thereby affecting species diversity. Higher species diversity can promote biomass growth and significantly increase CH4 flux (Zhang et al., 2012). Plant phenology, which influences plant growth (Fu and Shen, 2022; Jiang et al., 2022; Han et al., 2023a), also indirectly affects CH4 flux. Although this study did not consider the impact of plant phenology on productivity and CH4 flux, it examined productivity and community structure under relatively stable plant growth conditions, which can still reflect community changes in response to environmental changes. Warming increased the Simpson, Shannon–Wiener, and Pielou indices (Table S3), and both the Simpson and Shannon indices were negatively correlated with CH4 flux (P<0.05). Community composition may also be related to plant production (Li et al., 2014; Wang et al., 2021b; Wang et al., 2021c; Han et al., 2023b), and changes in community composition and productivity have a significant impact on CH4 flux (Zhang et al., 2012; Khalsa et al., 2014). In this study, warming and precipitation addition significantly increased the dominance of the Leymus chinensis population and decreased the dominance of Stipa krylovii population (Table S4), and IV-L.c was significantly positively correlated with the CH4 flux (P<0.01). Warming primarily affected CH4 flux through changes in species dominance. As a dominant species, the increased biomass of Leymus chinensis in the community and its inhibitory effect on CH4 absorption was stronger. This showed that the dominance of Leymus chinensis increases and the species diversity index decreases, which aggravates the inhibition of CH4 absorption and weakens the function of the CH4 sink under a warmer future in typical grasslands.
It is well known that soil CH4 fluxes are determined by the balance between CH4 production from methanogens and CH4 oxidation from methanotrophs (Prabhu et al., 2013; Christiansen et al., 2015). Multiple studies have shown that soil microbial community structure was influenced by warming and precipitation changes (Yu et al., 2019; Zhang et al., 2021; Han et al., 2022; Zhong and Fu, 2022). Methanogenic bacteria typically exist in anaerobic environments, and long periods of soil water deficiency, increase contact with soil oxygen, making it difficult to establish low oxidation reduction potential for CH4 absorption through anaerobic respiration (Yang et al., 2017). The lower soil moisture under warming could lead to higher potential redox conditions, in which microbes degraded SOC by gradually using electron acceptors with higher redox potentials, leading to conditions less suitable for methanogenesis (Johnston et al., 2019; Tano et al., 2020). Meanwhile, soil oxygen content could increase with the decline of soil moisture, resulting in more favorable conditions for methane oxidation (Li et al., 2020). While this study mainly considered the impact of environmental factors and plant community characteristics on CH4, future research could improve our ability for projecting future CH4 absorption changes via a better understanding of microbial mechanisms in response to climate changes (Cavicchioli et al., 2019; Guo et al., 2020).
5 Conclusion
1) The typical grassland soil was sink of CH4. CH4 flux during non-growing seasons accounted for a large proportion of whole year, which account for 30.29% ~ 44.20% under different treatments and was an indispensable part of CH4 flux. Warming promoted the CH4 absorption flux during the non-growing seasons, while precipitation addition inhibited that during the growing seasons compared with C. Warming and precipitation addition both reduced the proportion of CH4 flux in the growing season to the whole year, and increased the proportion in the non-growing season, and the effect of warming was stronger than that of precipitation addition on that. Warming and precipitation addition increased the percentage of CH4 absorbed in non-growing season which account 32.34% under C, 40.19% under T, 37.29% under P, 37.97% under TP respectively.
2) Increase of soil temperature, soil NH4+-N content and species diversity were conducive to CH4 absorption, but the increase of Leymus chinensis dominance was not conducive to that. Under future climate warming, the increase in Leymus chinensis population dominance and decrease in the species diversity index will further inhibit the absorption of CH4 and weaken the function of the CH4 sink in a typical steppe. However, there are many other ways in which warming affects CH4 absorption, and the results showed that warming promoted CH4 absorption. Therefore, we need to explore the mechanisms by which different factors affect CH4 flux in the future.
Data availability statement
The raw data supporting the conclusions of this article will be made available by the authors, without undue reservation.
Author contributions
HG and QG designed the research; ZW and RG performed the experiments; ZW and XMC analyzed the data and wrote the draft; ZW, XMC, HZ, XC, and CH revised the manuscript.
Funding
We gratefully acknowledge the financial support from the National Natural Science Foundation of China (32260278), Fundamental Research Funds for the Inner Mongolia Normal University (2022JBQN095), and Natural Science Foundation of Inner Mongolia (2021BS03024).
Conflict of interest
The authors declare that the research was conducted in the absence of any commercial or financial relationships that could be construed as a potential conflict of interest.
The reviewer YY declared a past co-authorship with the author ZW to the handling editor.
Publisher’s note
All claims expressed in this article are solely those of the authors and do not necessarily represent those of their affiliated organizations, or those of the publisher, the editors and the reviewers. Any product that may be evaluated in this article, or claim that may be made by its manufacturer, is not guaranteed or endorsed by the publisher.
Supplementary material
The Supplementary Material for this article can be found online at: https://www.frontiersin.org/articles/10.3389/fevo.2023.1193939/full#supplementary-material
References
Aires L. M. I., Pio C. A., Pereira J. S. (2008). Carbon dioxide exchange above a Mediterranean C3/C4 grassland during two climatologically contrasting years. Global Change Biol. 14 (3), 539–555. doi: 10.1111/j.1365-2486.2007.01507.x
Bodelier P. L. E. (2011). Interactions between nitrogenous fertilizers and methane cycling in wetland and upland soils. Curr. Opin. Environ. Sustainability 3 (5), 379–388. doi: 10.1016/j.cosust.2011.06.002
Bokhorst S., Huiskes A., Convey P., Bodegom P. V., Aerts R. (2008). Climate change effects on soil arthropod communities from the Falkland islands and the maritime Antarctic. Soil Biol. Biochem. 40 (7), 1547–1556. doi: 10.1016/j.soilbio.2008.01.017
Cavicchioli R., Ripple W. J., Timmis K. N., Azam F., Bakken L. R., Baylis M., et al. (2019). Scientists' warning to humanity: microorganisms and climate change. Nat. Rev. Microbiol. 17, 569–586. doi: 10.1038/s41579-019-0222-5
Chen S., Hao T., Goulding K., Misselbrook T. H., Liu X. J. (2019). Impact of 13-years of nitrogen addition on nitrous oxide and methane fluxes and ecosystem respiration in a temperate grassland. Environ. Pollut. 252, 675–681. doi: 10.1016/j.envpol.2019.03.069
Chen X. P., Wang G. X., Zhang T., Mao T. X., Wei D., Song C. L., et al. (2017). Effects of warming and nitrogen fertilization on GHG flux in an alpine swamp meadow of a permafrost region. Sci. Total Environment. 601–602, 1389–1399. doi: 10.1016/j.scitotenv.2017.06.028
Chen W., Wolf B., Yao Z., Brüggemann N., Butterbach-Bahl K., Liu C. Y., et al. (2010). Annual methane uptake by typical semiarid steppe in inner Mongolia. J. Geophysical Research-Atmospheres 115. doi: 10.1029/2009JD013783
Chen W., Zhang F., Wang B., Wang J. S., Tian D. S., Han G. X., et al. (2019). Diel and seasonal dynamics of ecosystem-scale methane flux and their determinants in an alpine meadow. J. Geophysical Research-Biogeosciences 124 (6), 1731–1745. doi: 10.1029/2019JG005011
Christiansen J. R., Romero A. J. B., Jørgensen N. O. G., Glaring M. A., Jørgensen C. J., Berg L. K., et al. (2015). Methane fluxes and the functional groups of methanotrophs and methanogens in a young Arctic landscape on disko island, West Greenland. Biogeochemistry 122, 15–33. doi: 10.1007/s10533-014-0026-7
Conrad R. (2007). Microbial ecology of methanogens and methanotrophs. Adv. Agron. 96, 1–63. doi: 10.1016/S0065-2113(07)96005-8
Dijkstra F. A., Morgan J. A., Follett R., Lecain D. (2013). Climate change reduces the net sink of CH4 and N2O in a semiarid grassland. Global Change Biol. 19 (6), 1816–1826. doi: 10.1111/gcb.12182
Dijkstra F. A., Morgan J. A., Von F. J. C., Follett R. (2011). Elevated CO2 and warming effects on CH4 uptake in a semiarid grassland below optimum soil moisture. J. Geophysical Research-Biogeosciences 116, G01007. doi: 10.1029/2010JG001288
Fu G., Shen Z. (2022). Asymmetrical warming of growing/non-growing season increases soil respiration during growing season in an alpine meadow. Sci. Total Environ. 812, G01007. doi: 10.1016/j.scitotenv.2021.152591
Guo X., Gao Q., Yuan M., Wang G., Zhou X., Feng J., et al. (2020). Gene-informed decomposition model predicts lower soil carbon loss due to persistent microbial adaptation to warming. Nat. Commun. 11, 4897. doi: 10.1038/s41467-020-18706-z
Han X., Chen B. M. (2020). Progress in the effects of warming on soil N2O and CH4emission and the underlying micro-bial mechanisms. Chin. J. Appl. Ecol. 31 (11), 3906–3914. doi: 10.13287/j.1001-9332.202011.026
Han F., Yu C., Fu G. (2022). Warming alters elevation distributions of soil bacterial and fungal communities in alpine grasslands. Global Ecol. Conserv. 39, e02306. doi: 10.1016/j.gecco.2022.e02306
Han F., Yu C., Fu G. (2023a). Asymmetric warming among elevations may homogenize plant α-diversity and aboveground net primary production of alpine grasslands. Front. Ecol. Evol. 11, 1126651. doi: 10.3389/fevo.2023.1126651
Han F., Yu C., Fu G. (2023b). Non-growing/growing season non-uniform-warming increases precipitation use efficiency but reduces its temporal stability in an alpine meadow. Front. Plant Sci. 14. doi: 10.3389/fpls.2023.1090204
IPCC. (2021). Climate change 2021–the physical science basis. Chem. Int. 43 (4), 22–23. doi: 10.1017/9781009157896
Jiang W. W., Meng L., Cheng Q. Y., Fu G. (2022). The change in environmental variables linked to climate change has a stronger effect on aboveground net primary productivity than does phenological change in alpine grasslands. Front. Plant Sci. 12. doi: 10.3389/fpls.2021.798633
Johnston E. R., Hatt J. K., He Z., Wu L., Guo X., Luo Y., et al. (2019). Responses of tundra soil microbial communities to half a decade of experimental warming at two critical depths. Proc. Natl. Acad. Sci. U. S. A. 116, 15096–15105. doi: 10.1073/pnas.1901307116
Juc´a J. F. T., Maciel F. J. (2006). Gas permeability of a compacted soil used in a landfill cover layer. Unsaturated Soils 2006, 1535–1546. doi: 10.1061/40802(189)128
Khalsa J., Fricke T., Weigelt A., Wachendorf M. (2014). Effects of species richness and functional groups on chemical constituents relevant for methane yields from anaerobic digestion: results from a grassland diversity experiment. Grass Forage Sci. 69 (1), 49–63. doi: 10.1111/gfs.12028
Konda R., Ohta S., Ishizuka S., Heriyanto J., Wicaksono A. (2010). Seasonal changes in the spatial structures of N2O, CO2, and CH4 fluxes from acacia mangium plantation soils in Indonesia. Soil Biol. Biochem. 42 (9), 1512–1522. doi: 10.1016/j.soilbio.2010.05.022
Kuzyakov Y., Gavrichkova O. (2010). REVIEW: time lag between photosynthesis and carbon dioxide efflux from soil: a review of mechanisms and controls. Global Change Biol. 16 (12), 3386–3406. doi: 10.1111/j.1365-2486.2010.02179.x
Lawrence D. M., Koven C. D., Swenson S. C., Riley W., Slater A. G. (2015). Permafrost thaw and resulting soil moisture changes regulate projected high-latitude CO2 and CH4 emissions. Environ. Res. Lett. 10 (9), 094011. doi: 10.1088/1748-9326/10/9/094011
Li Y., Dong S., Liu S., Zhou H. K., Gao Q. Z., Cao G. M., et al. (2015). Seasonal changes of CO2, CH4 and N2O fluxes in different types of alpine grassland in the qinghai-Tibetan plateau of China. Soil Biol. Biochem. 80, 306–314. doi: 10.1016/j.soilbio.2014.10.026
Li K., Gong Y., Song W., He G. X., Hu Y. K., Tian C. Y., et al. (2012). Responses of CH4, CO2 and N2O fluxes to increasing nitrogen deposition in alpine grassland of the tianshan mountains. Chemosphere 88 (1), 140–143. doi: 10.1016/j.chemosphere.2012.02.077
Li Z., Lin J., Zhang T., Zhang N., Mu C., Wang J. (2014). Effects of summer nocturnal warming on biomass production of leymus chinensis in the songnen grassland of China: from bud bank and photosynthetic compensation. J. Agron. Crop Sci. 200 (1), 66–76. doi: 10.1111/jac.12041
Li F., Yang G., Peng Y., Wang G. Q., Qin S. Q., Song Y. T., et al. (2020). Warming effects on methane fluxes differ between two alpine grasslands with contrasting soil water status. Agric. For. Meteorology 290. doi: 10.1016/j.agrformet.2020.107988
Li H., Zhu J., Zhang F., Qin G., Li Y. K., Wang J. B., et al. (2022). The predominance of nongrowing season emissions to the annual methane budget of a semiarid alpine meadow on the northeastern qinghai-Tibetan plateau. Ecosystems 25 (3), 526–536. doi: 10.1007/s10021-021-00669-x
Lin X., Wang S., Hu Y., Luo C. Y., Zhang Z. H., Niu H. S., et al. (2015). Experimental warming increases seasonal methane uptake in an alpine meadow on the Tibetan plateau. Ecosystems 18 (2), 274–286. doi: 10.1007/s10021-014-9828-7
Liu M., Jin H., Luo D., Wang Q. F., Jin X. Y., Li X. Y., et al. (2015). Progress in studies of carbon emission from soil on the qinghai-Tibetan plateau. J. Glaciology Geocryology 37 (6), 1544–1554. doi: 10.7522/.issn.1000-0240.2015.0171
Lu S. F., Han Z. J., Lu L., Lan T. G., Wei X., Zhao T. Y. (2023). On measuring methods and influencing factors of air permeability of soils: an overview and a preliminary database. Geoderma 435, 116509. doi: 10.1016/j.geoderma.2023.116509
Luan J., Song H., Xiang C., Zhu D., Duoerji S. (2016). Soil moisture, species composition interact to regulate CO2 and CH4 fluxes in dry meadows on the Tibetan plateau. Ecol. Eng. 91, 101–112. doi: 10.1016/j.ecoleng.2016.02.012
Luo G. J., Kiese R., Wolf B., Butterbach-Bahl K. (2013). Effects of soil temperature and moisture on methane uptake and nitrous oxide emissions across three different ecosystem types. Biogeosciences 10 (5), 3205–3219. doi: 10.5194/bg-10-3205-2013
Ma T., Chen H., Kang X., Wang Y. F. (2016). Effects of enclosure time on the activity of methanotrophs in soils of the inner Mongolia grassland. Chin. J. Appl. Ecol. 22 (1), 0008–0012. doi: 10.3724/SP.J.1145.2015.05024
Nakano T., Shinoda M. (2010). Response of ecosystem respiration to soil water and plant biomass in a semiarid grassland. Soil Sci. Plant Nutr. 56 (5), 773–781. doi: 10.1111/j.1747-0765.2010.00502.x
Nogueira C., Bugalho M. N., Pereira J. S., Caldeira M. C. (2017). Extended autumn drought, but not nitrogen deposition, affects the diversity and productivity of a Mediterranean grassland. Environ. Exp. Bot. 138, 99–108. doi: 10.1016/j.envexpbot.2017.03.005
Pan H., Li Y., Meng C., Zheng Y., Liu X. M., Zhuge Y. P., et al. (2022). Effects of nitrogen levels on interactions between active methanotrophs and nitrifiers. Acta Pedologica Sin. 59 (2), 557–567. doi: 10.11766/trxb202101050577
Peltoniemi K., Laiho R., Juottonen H., Bodrossy L., Kell D. K., Minkkinen K., et al. (2016). Responses of methanogenic and methanotrophic communities to warming in varying moisture regimes of two boreal fens. Soil Biol. Biochem. 97, 144–156. doi: 10.1016/j.soilbio.2016.03.007
Phillips R. P., Meier I. C., Bernhardt E. S., Grandy S., Wickings K., Finzi A. C. (2012). Roots and fungi accelerate carbon and nitrogen cycling in forests exposed to elevated CO2. Ecol. Lett. 15 (9), 1042–1049. doi: 10.1111/j.1461-0248.2012.01827.x
Potthast K., Hamer U., Makeschin F. (2010). Impact of litter quality on mineralization processes in managed and abandoned pasture soils in southern Ecuador. Soil Biol. Biochem. 42 (1), 56–64. doi: 10.1016/j.soilbio.2009.09.025
Prabhu N. S., Kapil D. P., Virendra M. (2013). Environmental determinants of soil methane oxidation and methanotrophs. Crit. Rev. Environ. Sci. Technol. 43 (18), 1945–2011. doi: 10.1080/10643389.2012.672053
Sampson D. A., Janssens I. A., Yuste J. C., Ceulemans R. (2007). Basal rates of soil respiration are correlated with photosynthesis in a mixed temperate forest. Global Change Biol. 13 (9), 2008–2017. doi: 10.1111/j.1365-2486.2007.01414.x
Savi F., Di B. C., Canfora. L., Mondini C., Fares S. (2016). Environmental and biological controls on CH4 exchange over an evergreen Mediterranean forest. Agric. For. Meteorol. 226, 67–79. doi: 10.1016/j.agrformet.2016.05.014
Shaver G. R., Canadell J., Chapin F. S., Gurevitch J., Harte J. H. R., Henry G., et al. (2000). Global warming and terrestrial ecosystems: a conceptual framework for analysis. Bioscience 50 (10), 871–882. doi: 10.1641/0006-3568(2000)050[0871:GWATEA]2.0.CO;2
Shukla P. N., Pandey K. D., Mishra V. K. (2013). Environmental determinants of soil methane oxidation and methanotrophs. Crit. Rev. Environ. Sci. Technol. 43 (18), 1945–2011. doi: 10.1080/10643389.2012.672053
Tano B. F., Brou C. Y., Dossou-Yovo E. R., Saito K., Futakuchi K., Wopereis M. C. S., et al. (2020). Spatial and temporal variability of soil redox potential, pH and electrical conductivity across a toposequence in the savanna of West Africa. Agronomy 10, 1787. doi: 10.3390/agronomy10111787
Tate K. R. (2015). Soil methane oxidation and land-use change - from process to mitigation. Soil Biol. Biochem. 80, 260–272. doi: 10.1016/j.soilbio.2014.10.010
Treseder K. K. (2008). Nitrogen additions and microbial biomass: a meta-analysis of ecosystem studies. Ecol. Lett. 11 (10), 1111–1120. doi: 10.1111/j.1461-0248.2008.01230.x
Von F. J. C., Butters G., Duchateau P. C., Thelwell R. J., Siller R. (2009). In situ measures of methanotroph activity in upland soils: a reaction-diffusion model and field observation of water stress. J. Geophysical Research-Biogeosciences 114, G01015. doi: 10.1029/2008JG000731
Wan Z. Q., Hu G. Z., Chen Y. L., Chao L. M., Gao Q. Z. (2018). Ecological responses of stipa steppe in inner mongolia to experimentally increased temperature and precipitation 1: background and experimental design. Rangeland J. 40 (2), 143–146. doi: 10.1071/RJ16081
Wang Z., Hao X., Shan D., Han G. D., Zhao M. L., Willms W. D., et al. (2011). Influence of increasing temperature and nitrogen input on greenhouse gas emissions from a desert steppe soil in inner Mongolia. Soil Sci. Plant Nutr. 57 (4), 508–518. doi: 10.1080/00380768.2011.591283
Wang J. S., Quan Q., Chen W. N., Tian D. S., Ciais P., W. Crowther T., et al. (2021a). Increased CO2 emissions surpass reductions of non-CO2 emissions more under higher experimental warming in an alpine meadow. Sci. Total Environment. 769(11), 144559. doi: 10.1016/j.scitotenv.2020.144559
Wang J. W., Yu C., Fu G. (2021b). Warming reconstructs the elevation distributions of aboveground net primary production, plant species and phylogenetic diversity in alpine grasslands. Ecol. Indic. 133, 108355. doi: 10.1016/j.ecolind.2021.108355
Wang J., Yu C., Fu G. (2021c). Asymmetrical warming between elevations may result in similar plant community composition between elevations in alpine grasslands. Front. Ecol Evol. 9, 757943. doi: 10.3389/fevo.2021.757943
Wang X., Zhong Z., Wang J. Y., Jian J. N., Yang G. H., Ren C. J., et al. (2022). Responses of soil carbon pool of abandoned grassland on the loess plateau to two-years warming and increased precipitation. Acta Pedologica Sinica. 43 (05), 2812–2821. doi: 10.13227/j.hjkx.202109219
Wei Y., Wu X., Zeng R., Cai C. F., Guo Z. L. (2020). Spatial variations of aggregate-associated humic substance in heavy-textured soils along a climatic gradient. Soil Tillage Res. 197 (1), 104497. doi: 10.1016/j.still.2019.104497
Wu H., Wang X., Ganjurjav H., Hu G. Z., Qin X. B., Gao Q. Z. (2020a). Effects of increased precipitation combined with nitrogen addition and increased temperature on methane fluxes in alpine meadows of the Tibetan plateau. Sci. Total Environ. 705 (2), 135818. doi: 10.1016/j.scitotenv.2019.135818
Wu X., Wang F., Li T., Fu B. J., Lv Y. H., Liu G. H. (2020b). Nitrogen additions increase N2O emissions but reduce soil respiration and CH4 uptake during freeze-thaw cycles in an alpine meadow. Geoderma 363, 114157. doi: 10.1016/j.geoderma.2019.114157
Wu J., Wang H., Li G., Wu J. H., Gong Y., Wei X. X., et al. (2021). Responses of CH4 flux and microbial diversity to changes in rainfall amount and frequencies in a wet meadow in the Tibetan plateau. Catena 202 (3), 105253. doi: 10.1016/j.catena.2021.105253
Xie M., Zhao L., Wu X., Tian L. M., Yue G. Y., Zhou H. Y., et al. (2020). Seasonal variations of nitrogen in permafrost-affected soils of the qinghai-Tibetan plateau. Catena 195. doi: 10.1016/j.catena.2020.104793
Xu X., Elias D. A., Graham D. E., Phelps T. J., Carroll S. L., Wullschleger S. D., et al. (2015a). A microbial functional group-based module for simulating methane production and consumption: application to an incubated permafrost soil. J. Geophysical Research-Biogeosciences 120 (7), 1315–1333. doi: 10.1002/2015JG002935
Xu Z. F., Hu R., Xiong P., Wan C. A., Cao G., Liu Q. (2010). Initial soil responses to experimental warming in two contrasting forest ecosystems, Eastern Tibetan plateau, China: nutrient availabilities, microbial properties and enzyme activities. Appl. Soil Ecol. 46, 291–299. doi: 10.1016/j.apsoil.2010.07.005
Xu Z. W., Ren H. Y., Cai J., Wang R. Z., Li M. H., Wan S. Q., et al. (2014). Effects of experimentally-enhanced precipitation and nitrogen on resistance, recovery and resilience of a semi-arid grassland after drought. Oecologia 176 (4), 1187–1197. doi: 10.1007/s00442-014-3081-9
Xu Z. W., Ren H. Y., Li M. H., Ruijven J. V., Han X. G., Wan S. Q., et al. (2015b). Environmental changes drive the temporal stability of semi-arid natural grasslands through altering species asynchrony. J. Ecol. 103 (5), 1308–1316. doi: 10.1111/1365-2745.12441
Xu W., Wan S. (2008). Water- and plant-mediated responses of soil respiration to topography, fire, and nitrogen fertilization in a semiarid grassland in northern China. Soil Biol. Biochem. 40 (3), 679–687. doi: 10.1016/j.soilbio.2007.10.003
Yan G., Xing Y., Lü X.-T., Xu L. J., Zhang J. H., Dai G. H., et al. (2019). Effects of artificial nitrogen addition and reduction in precipitation on soil CO2 and CH4 effluxes and composition of the microbial biomass in a temperate forest. Eur. J. Soil Sci. 70 (6), 1197–1211. doi: 10.1111/ejss.12812
Yang G., Peng Y., Olefeldt D., Chen Y. L., Wang G. Q., Li F., et al. (2017). Changes in methane flux along a permafrost thaw sequence on the Tibetan plateau. Environ. Sci. Technol. 52, 1244–1252. doi: 10.1021/acs.est.7b04979
Yu C., Han F., Fu G. (2019). Effects of 7 years experimental warming on soil bacterial and fungal community structure in the northern Tibet alpine meadow at three elevations. Sci. Total Environ. 655, 814–822. doi: 10.1016/j.scitotenv.2018.11.309
Yue P., Li K., Gong Y., Hu Y. K., Mohammat A., Christie P., et al. (2016). A five-year study of the impact of nitrogen addition on methane uptake in alpine grassland. Sci. Rep. 6. doi: 10.1038/srep32064
Zhang G.Y., Shen Z., Fu G. (2021). Function diversity of soil fungal community has little exclusive effects on the response of aboveground plant production to experimental warming in alpine grasslands. Appl. Soil Ecol. 168C. doi: 10.1016/j.apsoil.2021.104153
Zhang L., Hou L., Guo D., Li L. H., Xu X. F. (2017). Interactive impacts of nitrogen input and water amendment on growing season fluxes of CO2, CH4, and N2O in a semiarid grassland, northern China. Sci. Total Environ. 578 (feb.1), 523–534. doi: 10.1016/j.scitotenv.2016.10.219
Zhang Q., Shao M. A., Jia X., Zhang C. C. (2018). Understory vegetation and drought effects on soil aggregate stability and aggregate-associated carbon on the loess plateau in China. Soil Sci. Soc. America J. 82 (1), 106–114. doi: 10.2136/sssaj2017.05.0145
Zhang C.-B., Sun H.-Y., Ge Y., Gu B. J., Wang H., Chang J. (2012). Plant species richness enhanced the methane emission in experimental microcosms. Atmospheric Environ. 62, 180–183. doi: 10.1016/j.atmosenv.2012.08.034
Zhang J. Z., Zhou D., Guo X. D., Guo Y., Wang H., Cheng J. W., et al. (2019). Moderate grazing increases the abundance of soil methane-oxidizing bacteria and CH4 uptake rate in a typical steppe of inner Mongolia, China. J. Appl. Ecol. 06 (6), 1–11. doi: 10.13287/j.1001-9332.201906.035
Zhao Z., Dong S., Jiang X., Liu S. L., Ji H. Z., Li Y., et al. (2017). Effects of warming and nitrogen deposition on CH4, CO2 and N2O emissions in alpine grassland ecosystems of the qinghai-Tibetan plateau. Sci. Total Environ. 592, 565–572. doi: 10.1016/j.scitotenv.2017.03.082
Zheng Y., Yang W., Sun X., Wang S. P., Rui Y. C., Luo C. Y., et al. (2012). Methanotrophic community structure and activity under warming and grazing of alpine meadow on the Tibetan plateau. Appl. Microbiol. Biotechnol. 93, 2193–2203. doi: 10.1007/s00253-011-3535-5
Zhong Z., Fu G. (2022). Response of soil fungal species, phylogenetic and functional diversity to diurnal asymmetric warming in an alpine agricultural ecosystem. Agric. Ecosyst. Environ. 335. doi: 10.1016/j.agee.2022.107993
Zhu X., Luo C., Wang S., Zhang Z. H., Cui S. J., Bao X. Y., et al. (2015). Effects of warming, grazing/cutting and nitrogen fertilization on greenhouse gas fluxes during growing seasons in an alpine meadow on the Tibetan plateau. Agric. For. Meteorol. 214, 506–514. doi: 10.1016/j.agrformet.2015.09.008
Keywords: climate change, typical grassland, CH4, growing season, non-growing season
Citation: Chen X, Wan Z, Gu R, Ganjurjav H, Hu G, Gao Q, Chun X, Zhou H and Hai C (2023) Warming promoted CH4 absorption compared with precipitation addition in typical steppe in Inner Mongolia. Front. Ecol. Evol. 11:1193939. doi: 10.3389/fevo.2023.1193939
Received: 26 March 2023; Accepted: 21 June 2023;
Published: 07 July 2023.
Edited by:
Kerou Zhang, Chinese Academy of Forestry, ChinaReviewed by:
Yulong Yan, Inner Mongolia University, ChinaWeiwei Chen, Chinese Academy of Sciences (CAS), China
Xianglan Li, Beijing Normal University, China
Copyright © 2023 Chen, Wan, Gu, Ganjurjav, Hu, Gao, Chun, Zhou and Hai. This is an open-access article distributed under the terms of the Creative Commons Attribution License (CC BY). The use, distribution or reproduction in other forums is permitted, provided the original author(s) and the copyright owner(s) are credited and that the original publication in this journal is cited, in accordance with accepted academic practice. No use, distribution or reproduction is permitted which does not comply with these terms.
*Correspondence: Zhiqiang Wan, wanzhiqiang@imnu.edu.cn