- 1School of Geo-Science and Technology, Zhengzhou University, Zhengzhou, China
- 2State Key Laboratory of Severe Weather, Chinese Academy of Meteorological Sciences, Beijing, China
- 3Joint Laboratory of Eco-Meteorology, Chinese Academy of Meteorological Sciences, Zhengzhou University, Zhengzhou, China
- 4College of Horticulture and Plant Protection, Henan University of Science and Technology, Luoyang, China
Understanding the effect of environmental factors on the net ecosystem CO2 exchange (NEE) and the response of NEE to rainfall events is of great significance for an accurate understanding of the carbon cycle for desert steppe ecosystems. Based on the long-term (2011–2018) eddy covariance flux data of a temperate desert steppe in Inner Mongolia, China, this study used path analysis to analyze the combined impact of the environmental factors on NEE. The results showed that during the growing season, vapor pressure deficit (VPD) and soil water content (SWC) was the most prominent environmental factor for the daytime NEE and nighttime NEE, respectively. NEE responds differently to individual environmental factors among multi-year climatic conditions. The size of rainfall event has significant impacts on NEE, it can effectively promote the CO2 uptake of the desert steppe ecosystem when rainfall event size is greater than 5 mm, and the NEE response increased with the rainfall event size. Moreover, NEE peaked approximately 1–3 days after a 5–10 mm rainfall event, while the rainfall event size >10 mm, it would take 3–5 days for NEE to reach a peak value; and yet, small rainfall events (< 5 mm) slightly increased CO2 emissions. During the growing season, carbon uptake increased with monthly rainfall, except in May. Our results are important for understanding the carbon cycle and its control mechanisms in the temperate desert steppe of Inner Mongolia.
1. Introduction
Over half of the carbon emitted by human activities is absorbed through nature’s carbon cycle, which has become one of the core problems of global change research (Gong et al., 2018; Zhou et al., 2020). Arid and semi-arid regions account for approximately 30% of the global carbon sequestration (Adams et al., 1990; Chen et al., 2021), and the ecosystems in these regions are highly sensitive to climate change because of low precipitation, strong sunlight, and high evaporation rates (Gu et al., 2018). Previous studies have revealed that climate change causes changes in meteorological and other environmental factors that affect both ecosystem photosynthesis and respiration and, as a result, the ecosystem carbon balance (Li et al., 2015; Nyantakyi-Frimpong and Bezner-Kerr, 2015). Grassland is the dominant ecosystem type in arid and semi-arid areas, covering approximately one-third of the global natural vegetation (Parton et al., 1995). Among terrestrial ecosystems, grasslands have a large carbon storage capacity, second only to forest ecosystems, and are critical to the global carbon balance (Yang and Zhou, 2013; Li et al., 2021).
Carbon flux is regulated by both environmental and biological factors, and the study of its driving factors has been a major research topic regarding the global carbon cycle, particularly under the premise of climate change (Fang et al., 2018). Water availability is the primary driver of plant growth (Zhang et al., 2020), and previous studies have shown that carbon flux over grassland ecosystems is closely related to the vapor pressure deficit (VPD) and soil moisture (Chen et al., 2018). In semi-arid grassland ecosystems, high carbon uptake usually occurs under humid conditions, whereas high carbon release is associated with warm and dry conditions (Ahlström et al., 2015). Meanwhile, it was proposed that the effects of environmental factors on NEE might differ among different climatic years, and the variation of NEE may even have different dominant factors for different climatic years (Aguilos et al., 2018; Wang et al., 2019). However, to date, few such studies have been conducted on the desert steppe.
Desert steppe is water-limited, and the carbon cycle in grassland ecosystems is sensitive to changes in rainfall (Zhang et al., 2020). Precipitation occurs in the form of discrete, intermittent, and unpredictable pulse events (Rey et al., 2017), the size of a single rainfall event can have a large impact on grassland carbon flux (Huxman et al., 2004). A previous study showed that small-pulse rainfall events (e.g., 2.1 mm) slightly increased CO2 emissions by stimulating microbial respiration, whereas large-pulse rainfall events (e.g., 19.7 mm) promoted CO2 uptake by affecting plant photosynthesis (Schwinning et al., 2004; Fa et al., 2015). A study of a Stipa grassland in Inner Mongolia, China showed that the CO2 uptake increased when the rainfall event size >10 mm (Peng et al., 2013), but another study of a Leymus chinensis grassland in Inner Mongolia showed that the CO2 uptake increased when the rainfall event size >5 mm (Hao et al., 2017). This suggests that the size of effective rainfall event may vary among different climate and vegetation types.
Herein, this study aims to investigate the impact of environmental factors on the net ecosystem CO2 exchange (NEE) and the response of NEE to rainfall events, based on eddy covariance flux data from 2011 to 2018 of a temperate desert steppe in Inner Mongolia, China. The long-term measurement campaign provided a broad range of conditions to thoroughly investigate the environmental regulations of NEE in the desert steppe ecosystem. The specific objectives are (1) to analyze the combined impact of the environmental factors on NEE, and compare the controlling factors of NEE between years with different climatic conditions; and (2) to determine the NEE response to rainfall events of different magnitudes.
2. Materials and methods
2.1. Site description
The research site is located in Damao Banner of Baotou city in Inner Mongolia (41°38.6′ N, 110°19.9′ E, 1409 m a.s.l.). Desert steppe vegetation covers the area including subregions with a temperate, continental, and semiarid climate. The Information and Research Institute of Meteorology, Hydrology, and Environment of Inner Mongolia reports that from 1990 to 2019, the site’s mean annual air temperature was 5.22°C and its mean annual precipitation was 247.4 mm. Most of the rainfall occurs during the growing season (May–September). The vegetation is dominated by a degraded Stipa klemenzii community. The soil was brown and calcified, with a calcified layer at 20–50 cm. The total K, total P, and organic carbon contents are 23.29 g kg−1, 0.31 g kg−1, and 12.67 g kg−1, respectively, and the average bulk density was 1.23 g cm−3, with a pH of 7.4.
2.2. Fluxes and meteorological measurements
From 2011 to 2018, the turbulence fluxes were monitored at a height of 4 m using an open-path eddy covariance system. An infrared gas analyzer (Li-7500, LICOR, Inc., Lincoln, NE, United States) and a 3D sonic anemometer (CSAT-3, Campbell Scientific, Inc., Logan, UT, United States) were employed to calculate the variations in the densities of gaseous CO2 and H2O at 10 Hz and three wind speed components, respectively. The original data were recorded and stored automatically at 10 Hz and 0.5 h online flux data were provided by a datalogger (CR5000, Campbell Scientific Inc., Logan, UT, United States).
During the research project (2011–2018), a shielded sensor (model HMP45C, Vaisala, Helsinki, Finland) was used to measure the air temperature (Ta) and relative humidity (RH) at levels of 1.5 and 3.5 m. From Ta and RH, the VPD was computed. Precipitation was measured at a height of 1.2 m using a tipping-bucket rain gauge (model 52203, RM Young Inc., Traverse City, MI, United States). Using a quantum sensor, photosynthetically active radiation (PAR) was recorded (4 m; LI190SB, LI-COR, Lincoln, United States). The soil temperature sensor comprised a thermistor (107 L, Campbell Scientific Inc., Logan, UT, United States) (5, 10, 15, 20, 40, and 80 cm) that was used to measure soil temperature (Ts). The soil temperature recorded at a depth of 10 cm was used in this study, defined as Ts. The volumetric water content of the soil at depths of 10 cm (SWC10), 20 cm (SWC20), 30 cm (SWC30), and 40 cm (SWC40) were monitored using time-domain reflectometry probes (Easy AG; Campbell Scientific Inc., Logan, UT, United States). Data loggers (CR23X, Campbell Scientific Inc., Logan, UT, United States) were used to collect all data, and 30 min mean values were automatically recorded and stored.
2.3. Flux calculations and data processing
Eddy covariance data were processed by EddyPro7.0.6 (LI-COR), including double rotation, Webb–Pearman–Leuning correction, potential temporal lags correction, frequency response correction etc. (Webb et al., 1980; Moncrieff et al., 1997; Reichstein et al., 2005). In this study, positive NEE values denoted a source of atmospheric CO2 at the location, whereas negative values denoted a carbon sink. During the observation period, the power supply may have been interrupted by abnormal weather or instruments. Half-hourly fluxes were filtered for: (1) incomplete measurements taken every half-hourly, (2) outliers, (3) low turbulence situations, and (4) rain events (Papale et al., 2006). A cutoff friction velocity (u*) was employed, and nocturnal fluxes below the threshold were eliminated (Zhu et al., 2006). Subsequently, the gaps in the flux data were filled using a marginal distribution sampling (MDS) technique (Reichstein et al., 2005). The flux data for 2013 were not obtained because of instrument failure.
2.4. Characterization of rainfall events
Rainfall events can be described as discontinuous, highly varied, and usually comprise unpredictable pulses of rainfall (Li et al., 2013). The division of rainfall events is based on the absence of rainfall within 24 h after a certain rainfall time. Rainfall events were classified into six levels, according to their size (Table 1). Rainfall events of <10 mm were regarded as a small rainfall event, 10–25 mm as moderate rainfall event, and rainfall >25 mm as a heavy rainfall event.
Response of NEE to rainfall event (NEEresponse) was determined as the difference between mean daily NEE post-event (NEEafter) and mean daily NEE pre-event (NEEbefore) (Wang et al., 2017),
The relative response effect of NEE to rainfall event (NEEresponse, r) was calculated as the following equation:
2.5. Path analysis
Earlier studies suggested that the combined effects of the various environmental factors should receive more attention because the independent effect of any one factor alone is insufficient to explain the variations in NEE (Wang et al., 2016). To examine the combined impact of the environmental factors on NEE, and the relative importance of these factors, the path analysis method was used in this study. Path analysis is an extension of multiple regression and can be used to understand the causal structure of data (Kozak and Kang, 2006). The path analysis method reveals the relationships between dependent and independent variables using the direct and indirect path coefficients as well as the determinative coefficient (Yuan et al., 2001; Jiang et al., 2014). Based on the determinative coefficient, the sequence of the synthetic action of every independent variable to the response variable can be decided, and which variable is the principal decision variable can be identified (Yuan et al., 2001). Path analysis were applied using IBM SPSS Statistics 26.0.
3. Results
3.1. Environmental conditions
Seasonal fluctuations in air temperature (Ta), soil temperature (Ts), precipitation (Prec), photosynthetically active radiation (PAR), vapor pressure deficit (VPD), and volumetric soil water content (SWC) are shown in Figure 1. During the measurement campaign (2011–2018), Ta and Ts showed similar seasonal dynamics with a single-peak curve (Figures 1A,D). The highest monthly mean Ta was 22.39°C, which typically occurs in July, and the lowest monthly mean Ta was −13.92°C, which was observed in January. The monthly mean Ts ranged from −10.5 to −24.27°C, with January and July recording the lowest and highest values, respectively. During the study period (2011–2018), the annual precipitation ranged from 229.8 mm (in 2015) to 513.2 mm (in 2018), with the growth season (May–September) accounting for roughly 92.3% of the total. Seasonal variations in SWC matched well with the seasonal distribution of precipitation (Figures 1B,E), and the daily SWC ranged from 10% to 57%. PAR and VPD exhibited relatively large day-to-day fluctuations and their seasonal variations generally showed unimodal curves (Figures 1C,F). The peak PAR values usually occurred in May, and the average PAR in May was 44.62 mol m−2 day−1. The VPD typically peaks between May and July. The highest VPD (3.17 kPa) was observed in mid-July 2017.
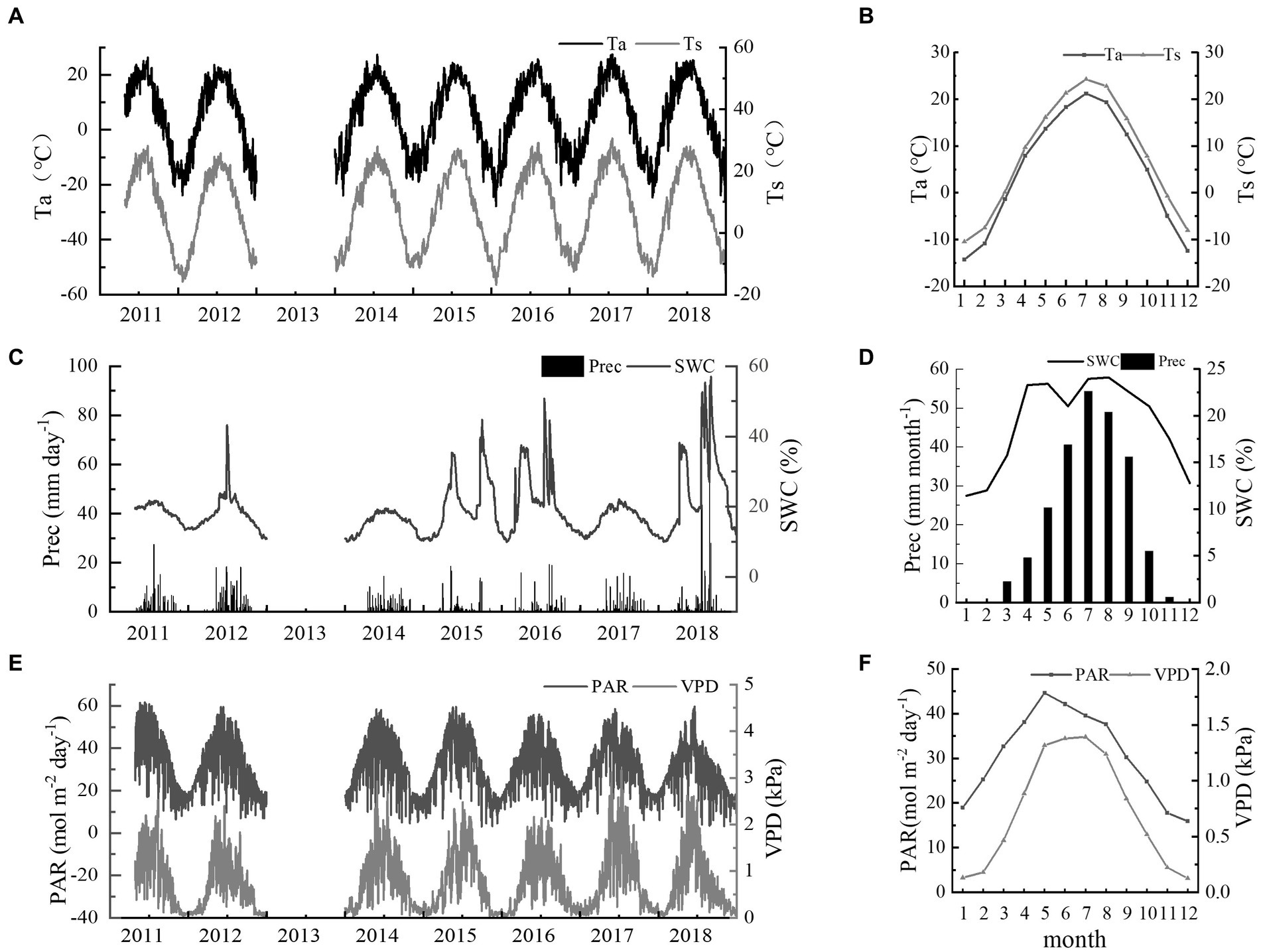
Figure 1. (A) Seasonal variations of daily mean air temperature (Ta) and soil temperature (Ts); (B) daily sum of precipitation (Prec) and daily average of volumetric soil water content (SWC) at 10 cm depth; (C) photosynthetically active radiation (PAR) and daily mean vapor pressure deficit (VPD). Seasonal variations in monthly mean values of (D) Ta and Ts; (E) Prec and SWC; and (F) PAR and VPD.
In this study, the differences between the growing season temperature and precipitation and the respective multi-year averages were used to define the annual climate types (Figure 2). Mean Ta for the growing season ranged from 17.42°C (2012) to 19.61°C (2017). Growing-season precipitation was highest in 2018 (474.9 mm, 112.1% higher than the multi-year (1990–2019) average of 223.9 mm) and lowest in 2015 (174.6 mm, 22.0% lower than the multi-year average). Accordingly, 2016 was selected to represent a normal year (normal climatic conditions), 2017 was classified as a warm year, and 2018 was classified as a warm and moist year (Figure 2).
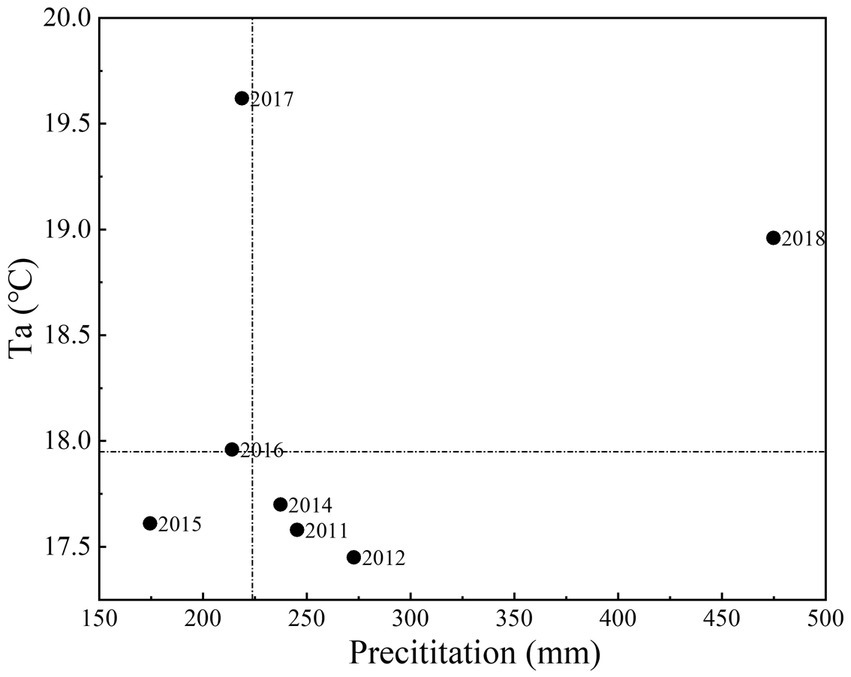
Figure 2. Average air temperature and cumulative precipitation during each growing season from 2011 to 2018. The growing season precipitation average over the long term (1990–2019) is shown by the horizontal dashed line (223.9 mm); the long-term average air temperature for the growing season is illustrated by the vertical dashed line (17.95°C).
3.2. Environmental controls on NEE
Path analysis method was used to examine the combined impact of the environmental factors on NEE (Figure 3), and the determinative coefficient generated by path analysis was used to determine the relative importance of environmental factors in NEE fluctuations (Table 2). As seen in Table 2, moisture-related environmental factors (e.g., VPD and SWC) had greater effects on NEE in the desert steppe ecosystems than heat-related environmental factors (e.g., Ta and Ts).
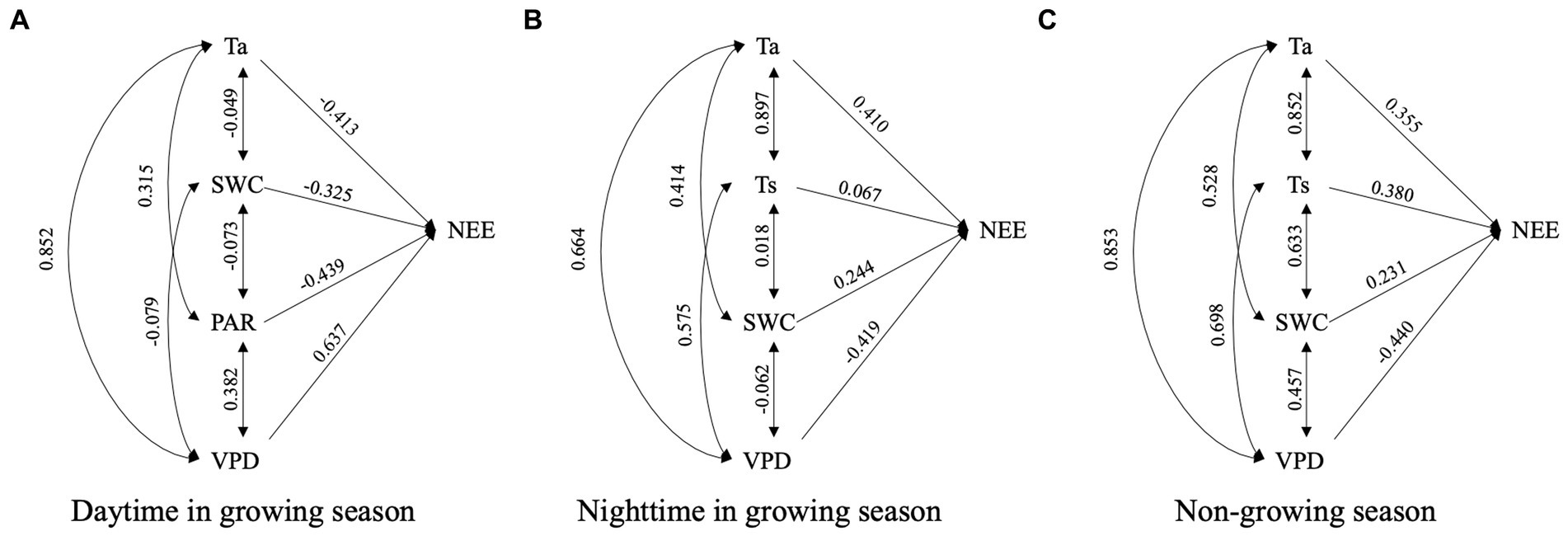
Figure 3. Path diagrams illustrating the effects of environmental factors on (A) daytime net ecosystem CO2 exchange (NEE) during the growing season, (B) nighttime NEE during the growing season, and (C) NEE during the non-growing season.
During the growing season, VPD was the most prominent environmental factor affecting the daytime NEE, followed by SWC, Ta, and PAR. Daytime NEE increased (i.e., carbon uptake reduced) with an increase in VPD and declined (i.e., carbon uptake increased) with an increase in Ta, SWC, and PAR. From the absolute values of path coefficient, there was little difference between the direct and indirect effects of VPD and Ta, whereas SWC and PAR mainly had direct effects on daytime NEE. For night-time NEE, SWC had the strongest effect, followed by VPD, Ts, and Ta. Among these, SWC, VPD, and Ta mainly had direct effects on nighttime NEE.
During the non-growing season, VPD was the most important factor controlling NEE, followed by Ts, SWC, and Ta. In contrast to the growing season, the influence of environmental factors on NEE during the non-growing season was mainly attributed to direct effects.
Moreover, the effects of environmental factors on NEE may vary under different climatic conditions (Aguilos et al., 2018; Wang et al., 2019). In this study, we considered three types of climatic conditions: normal, warm, warm and moist, which were classified according to the average air temperature and cumulative precipitation during the growing season (see Section 3.1 and Figure 2). Path diagrams illustrating the effects of environmental factors on daytime net ecosystem CO2 exchange for different climatic conditions (Figure 4). The results showed that in a normal year or warm year, moisture (SWC or VPD) was the primary factor controlling daytime NEE during the growing season, followed by Ta and PAR; in comparison, PAR proved to be the most important controlling factor for NEE in a warm and moist year (Table 3).
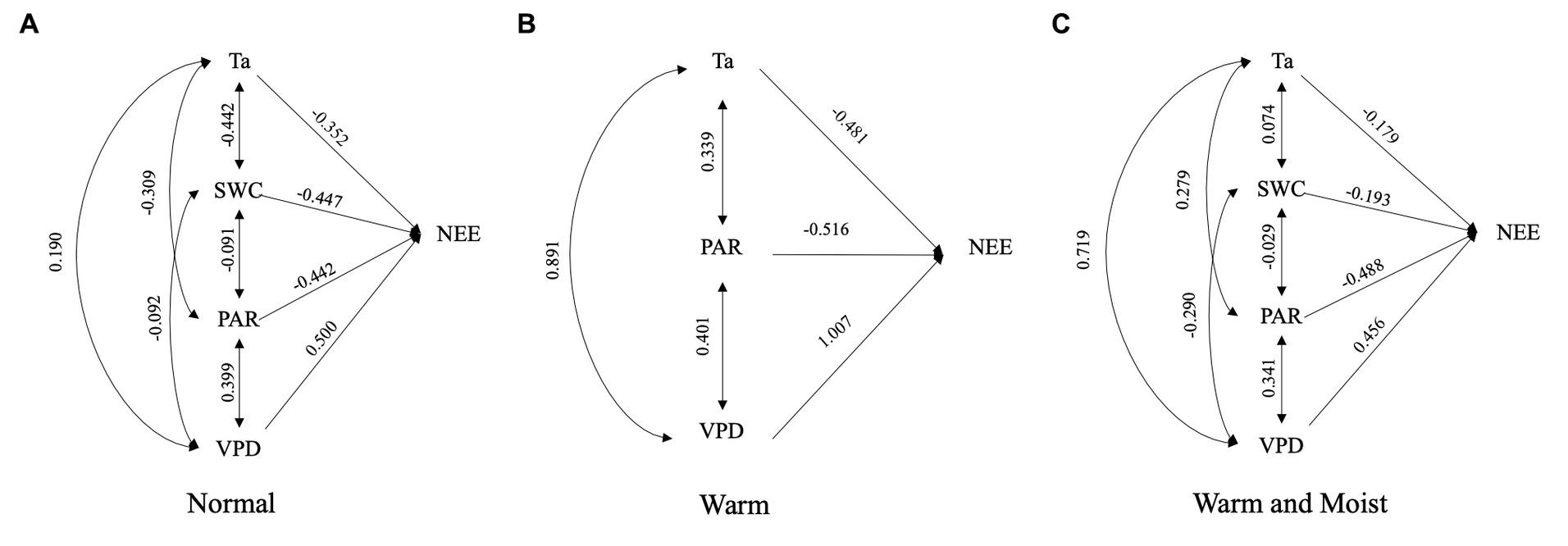
Figure 4. Path diagrams illustrating the effects of environmental factors on daytime net ecosystem CO2 exchange (NEE) for (A) normal year, (B) warm year, and (C) warm and moist year.
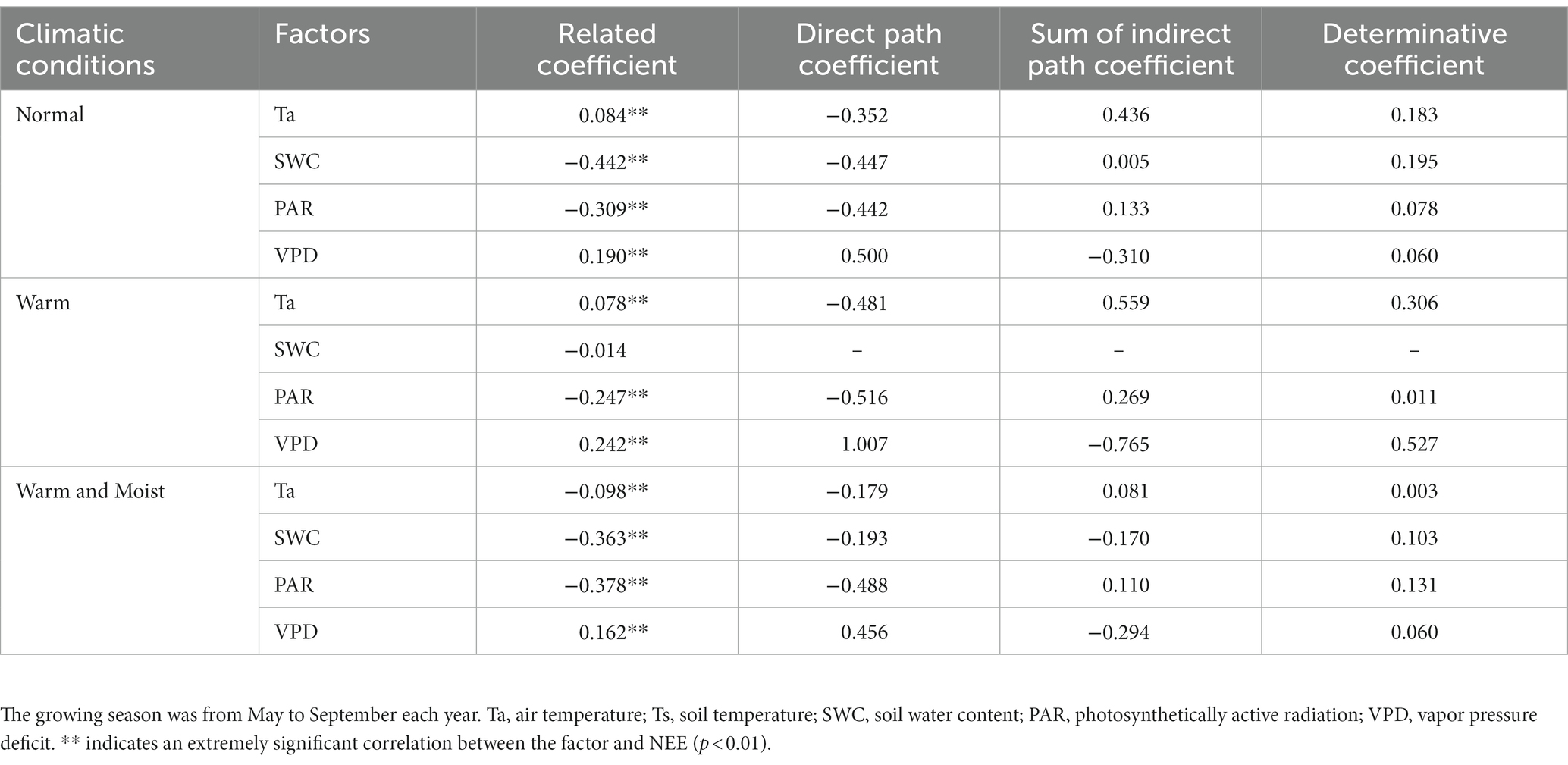
Table 3. Direct and indirect path coefficients of environmental factors on daytime NEE changes in the growing season under different climatic conditions.
3.3. Effects of rainfall events on NEE
The NEE at this site was primarily water-limited according to the results of the aforementioned environmental controls. The effects of rainfall events on NEE are further explored in this section. From 2011 to 2018 (excluding 2013), 258 rainfall events were recorded in the study area, of which 196 (75% of all rainfall events) occurred during the growing season. A total of 196 events from this seven-growing-season study were used for statistical analysis (Figure 5). Most rainfall events were small in the size, with the majority (61%) being <5 mm. However, despite the low proportion of large rainfall events (> 5 mm), the total rainfall from them was greater than that of small rainfall events (80% vs. 20%).
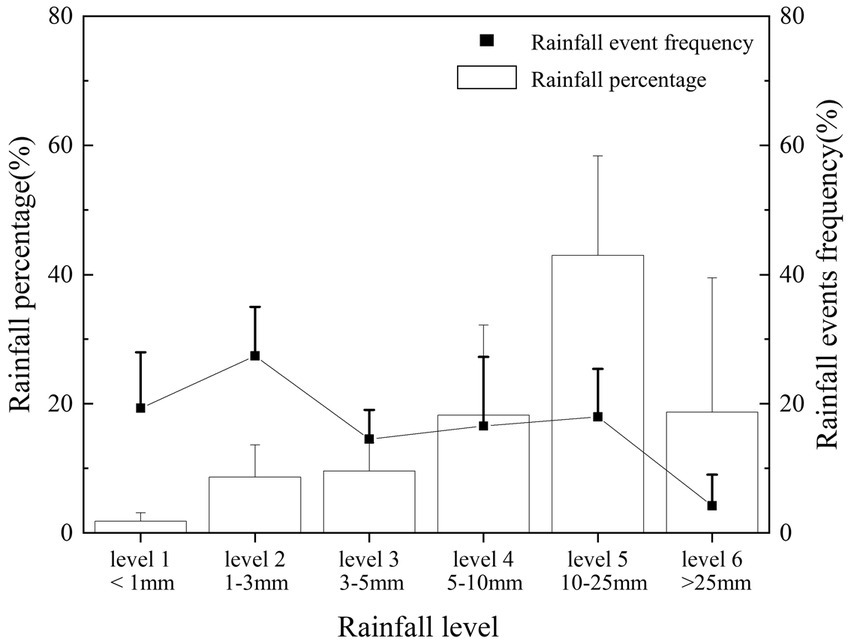
Figure 5. Frequency distributions of rainfall events and rainfall amounts from May to September between 2011 and 2018 (excluding 2013).
By comparing the daily average NEE in a sliding window of 3 days after rainfall with the daily average NEE including the 3 days before rainfall, the impact of the rainfall event on NEE was evaluated (Figure 6). The results showed that the CO2 uptake increased (negative NEEresponse in Figure 6) when the rainfall event was >5 mm, and the response increased with the size of the rainfall event. The relative response effect of NEE to rainfall event (NEEresponse, r) represented a different trend; that is, the CO2 uptake increased 10-, 8-, and 5-fold, respectively, compared to the uptake level before a rainfall event of 5–10 mm, 10–25 mm, and > 25 mm. Moreover, CO2 uptake peaked approximately 1–3 days after a 5–10 mm rainfall event. However, it took 3–5 days to reach the highest carbon uptake after a rainfall event of >10 mm; On the other hand, small rainfall events (< 5 mm) slightly increased CO2 emissions (positive NEEresponse in Figure 6).
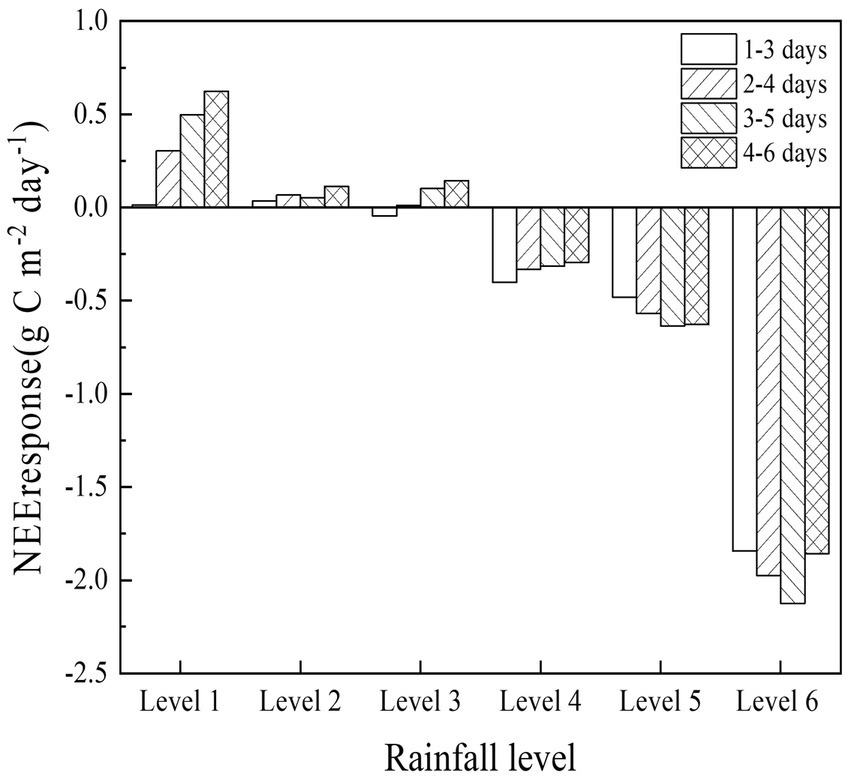
Figure 6. Variation of NEE before and after rainfall with different rainfall levels. The figure shows the difference between the daily average of the sliding window NEE for 3 days after a rainfall event and the daily average of the NEE for the 3 days before the rainfall event.
As there were differences in the size of rainfall events that occurred in different months. For example, May is dominated by small rainfall events (rainfall events of <5 mm account for 74.3% of the total), while July and August are dominated by large rainfall events. The frequency of rainfall events of >5 mm in July and August was 45.0% and 40.5%, respectively, and the rainfall percentage of rainfall events of >5 mm in July and August was 87.1% and 88.0%, respectively. Therefore, a correlation analysis was conducted between monthly precipitation and NEE during the growing season (Figure 7). The results showed that an increase in rainfall in May increased CO2 emissions from the desert steppe ecosystem, whereas an increase in rainfall from June–September increased the CO2 uptake. It supports the findings of previous related studies that seasonal distribution patterns of rainfall have important effects on the annual carbon balance (Dong, 2011). From the perspective of the entire growing season, NEE was greatly influenced by rainfall, and with an increase in rainfall, NEE decreased; that is, carbon uptake was enhanced.
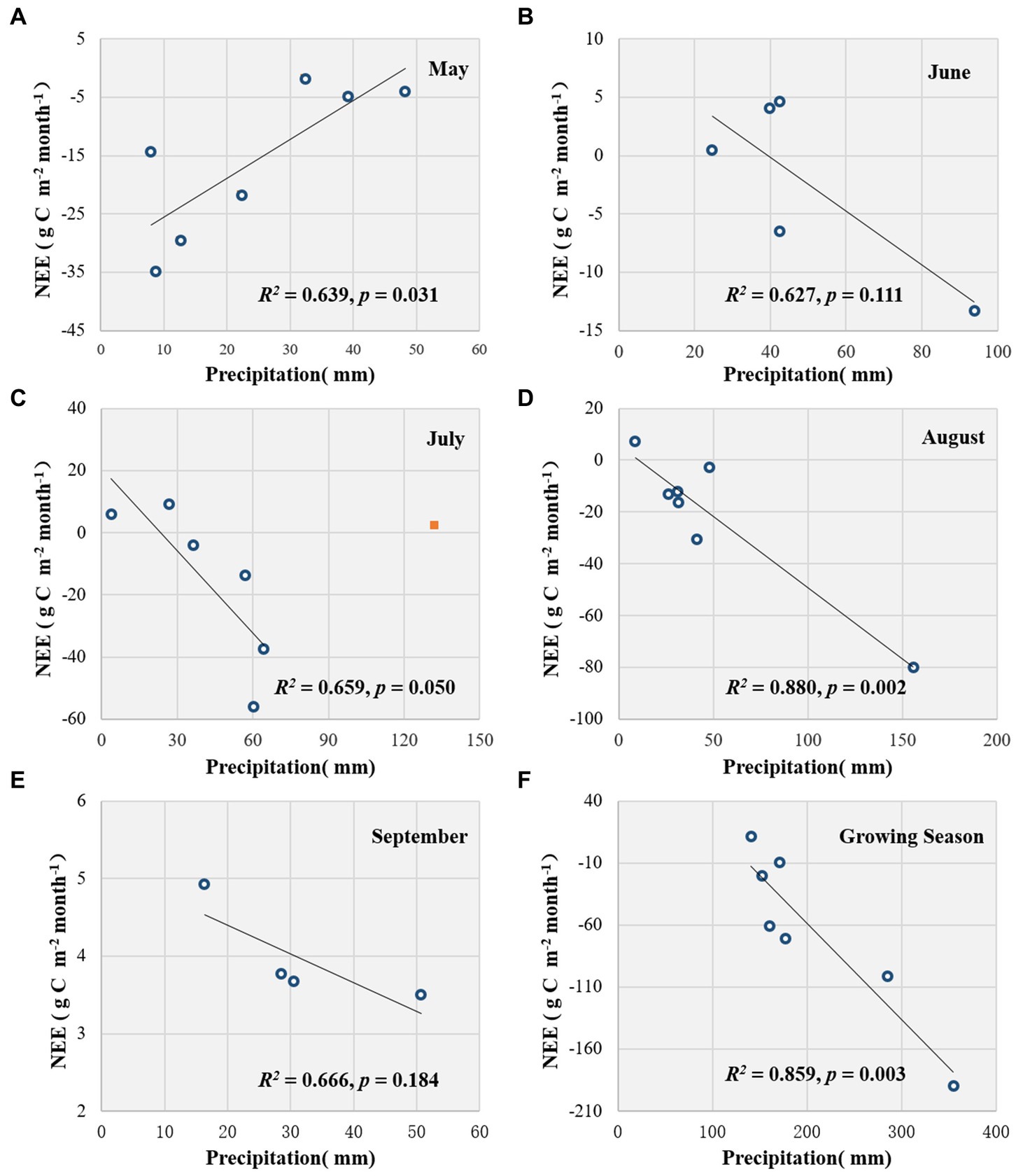
Figure 7. Relationship between NEE and corresponding rainfall. The relationship between NEE and rainfall in May (A), June (B), July (C), August (D), September (E), and the growing season (May–September) (F), respectively. The orange square in Plot C represented a singular point, and it was excluded from the regression; all the variables used in the analyses were monthly values, and if the effective observed data for a given month is less than 80%, that month’s value is excluded from the analysis.
4. Discussion
4.1. Constraints on NEE
It is generally agreed that in most ecosystems, radiation acts as the main regulating factor for NEE on a half-hourly scale (Baldocchi, 2014; Wang et al., 2019; Yan et al., 2023). However, certain studies have suggested that the two most important factors controlling carbon exchange in grassland ecosystems are temperature and water (Kim and Verma, 1990; Xue et al., 2014). This study showed that VPD (a function of temperature and humidity) had the largest effect on daytime NEE during the growing season, and that ecosystem carbon uptake decreased with increasing VPD, which is consistent with an earlier study in a desert steppe in Xilamuren (Li, 2020). VPD has a strong effect on stomatal conductance and can, therefore, modulate CO2 exchange between the canopy and the atmosphere (Novick et al., 2016; Umair et al., 2020; Xie et al., 2020). Lower VPD stimulates stomatal opening, which is thought to increase CO2 uptake (Wu et al., 2017). However, when VPD is higher than a certain threshold, plants may partially close their stomata to prevent excessive water loss; thus, ecosystem carbon uptake is constrained (Wang, 2015; Novick et al., 2016).
Our findings also revealed that SWC played a significant role in explaining nighttime variation NEE (i.e., ecosystem respiration), as reported in other previous studies (Balogh et al., 2011; Vicca et al., 2014; Meena et al., 2020). This implies that soil moisture should be considered when simulating ecosystem respiration, especially in water-limited ecosystems. Ecosystem respiration is the sum of the plant (autotrophic) and heterotrophic microbial respiration. Water availability is one of the most significant constraints on plant and microbial growth and changes in soil moisture can significantly affect ecosystem respiration (Piao et al., 2019). Reduced SWC in water-limited ecosystems such as at our study site leads to restricted plant growth and decreased microbial decomposition; thus, ecosystem respiration also shows a downward trend.
Path analysis suggested that temperature played a subordinate role in influencing half-hourly NEE changes at this site, which is consistent with previous studies in semiarid grasslands; that is, soil moisture availability is more important than soil temperature in regulating respiration in water-limited ecosystems (Vargas et al., 2010; Thomey et al., 2011; Wang et al., 2015). In this desert steppe, during the daytime of the growing season, an increase in temperature promoted ecosystem CO2 uptake, while during the nighttime of the growing and non-growing seasons, an increase in temperature promoted ecosystem respiration and increased CO2 emissions.
The effects of environmental factors on NEE may vary under different climatic conditions (Aguilos et al., 2018; Wang et al., 2019). Take daytime NEE during the growing season, for example, in the normal year, SWC was the primary factor controlling NEE, and the significant impact of SWC on NEE is mainly attributed to its direct impact. A previous study also showed that SWC has a strong direct impact on the eco-physiological processes of desert steppe plants (Aguilos et al., 2018); in the warm year, VPD became the most prominent environmental factor influencing NEE since variations in VPD are closely correlated with changes in temperature, which in turn influences plant stomatal conductance and modifies NEE; In the warm and moist year, water restriction for carbon uptake was lessened along with increased rainfall, and the significant impact of PAR on NEE emerged, because PAR directly promotes plant photosynthesis and increases ecosystem carbon absorption (Yue et al., 2010). The significant effects of SWC and PAR were mainly attributed to their direct effects; regarding Ta, its effects on NEE were dominated by direct effects in a warm and moist year, whereas they were primarily indirect in a normal year or a warm year, which is consistent with previous research (Jia et al., 2014; Ouyang et al., 2014; Aguilos et al., 2018).
4.2. Responses of NEE to rainfall events
Studies have shown that in grassland ecosystems with limited water resources, the ecosystem carbon flux is closely related to annual precipitation at different locations and in different years (Huxman et al., 2004; Gemechu Legesse et al., 2021). Small rainfall events predominate in arid and semi-arid ecosystems, and the results showed that small-scale rainfall events can enhance the carbon emissions of the ecosystem (Tang et al., 2018); whereas rainfall events of >5 mm can effectively increase soil water content, which enhances the carbon uptake capacity of the ecosystem and leads to a longer duration of ecosystem changes (Chen and Wang, 2009; Wang et al., 2018). This is in line with our analysis results, rainfall events of <5 mm slightly increased CO2 emissions in the desert steppe, and rainfall events of >5 mm increased the CO2 uptake in the desert steppe. Hence it can be inferred that a rainfall event of 5 mm is considered ecologically significant for NEE responses in desert steppe ecosystems (Li et al., 2013).
The carbon uptake peak was delayed by 1–5 days after rainfall, which was due to the faster response of ecosystem respiration to rainfall than to photosynthesis (Delgado-Balbuena et al., 2022). In addition, this pattern may be influenced by the dry period after rainfall, which offsets the soil water deficit and decreases the photosynthetic rate (Harper et al., 2005). The relationship between rainfall, infiltration depth, and the response of ecosystem carbon exchange to rainfall patterns depends on the position of soil microflora and plant roots in the soil as well as temporal changes in microbial and plant responses to wetting episodes (Huxman et al., 2004). Larger rainfall events may penetrate deep into the soil, whereas smaller rainfall events are intercepted by the canopy or directly replenished to the top soil layer, where water evaporates and cannot reach the root system (Hao et al., 2010).
This study showed that seasonal distribution patterns of rainfall have a large impact on desert steppe NEE. The CO2 uptake increased with monthly rainfall from June to September, whereas it decreased with monthly rainfall in May. The main reason might be that small rainfall events (<5 mm) dominated in May, which are not expected to stimulate ecosystem productivity by altering the soil water content effectively, but instead increase ecosystem respiration and carbon emissions (Fay et al., 2008; Tang et al., 2018). It can be inferred that summer rainfall is more favorable for carbon sequestration than spring rainfall for desert steppe (Peng et al., 2013; Wang et al., 2018).
5. Conclusion
NEE of the studied desert steppe was primarily water-limited. Moisture-related environmental factors, VPD and SWC was the most prominent environmental factor for the daytime NEE and nighttime NEE during the growing season, respectively. But the interannual differences in climatic conditions can lead to changes in the controlling factor of NEE, e.g., PAR became the main controlling factor of NEE under climatic conditions of warm and moist.
The size of rainfall events has significant impacts on the NEE of the desert steppe. Small rainfall events (< 5 mm) slightly increased CO2 emissions; Rainfall events of >5 mm increased the CO2 uptake, and the NEE response increased with the rainfall event size. Moreover, NEE had a longer response time to rainfall events with a larger size, e.g., NEE peaked approximately 1–3 days after a 5–10 mm rainfall event, while the rainfall event size >10 mm, it would take 3–5 days for NEE to reach a peak value. During the growing season, carbon uptake increased with monthly rainfall, except in May. These findings are important for predicting the carbon balance trends in desert steppe ecosystems under various climate change scenarios.
Data availability statement
The original contributions presented in the study are included in the article/supplementary material, further inquiries can be directed to the corresponding authors.
Author contributions
LZ and GZ: conceptualization and funding acquisition. JS and LZ: methodology and writing—original draft preparation. JS, YY, and SZ: investigation. JS and YW: data curation. All authors contributed to the article and approved the submitted version.
Funding
This research is funded by the National Key Research and Development Program of China (grant number 2018YFA0606103), National Natural Science Foundation of China (grant number 42130514), National Science and Technology Basic Resources Survey Program of China (grant number 2019FY101302), and China Meteorological Administration Innovation Development Special Project, (grant number CXFZ2023P052).
Acknowledgments
The authors wish to acknowledge Feng Zhang and Deng Ao for the fieldwork.
Conflict of interest
The authors declare that the research was conducted in the absence of any commercial or financial relationships that could be construed as a potential conflict of interest.
Publisher’s note
All claims expressed in this article are solely those of the authors and do not necessarily represent those of their affiliated organizations, or those of the publisher, the editors and the reviewers. Any product that may be evaluated in this article, or claim that may be made by its manufacturer, is not guaranteed or endorsed by the publisher.
References
Adams, J. M., Faure, H., Faure-Denard, L., Mcglade, J. M., and Woodward, F. I. (1990). Increases in terrestrial carbon storage from the last glacial maximum to the present. Science 348, 711–714. doi: 10.1038/348711a0
Aguilos, M., Herault, B., Burban, B., Wagner, F., and Bonal, D. (2018). What drives long-term variations in carbon flux and balance in a tropical rainforest in French Guiana? Agric. For. Meteorol. 253-254, 114–123. doi: 10.1016/j.agrformet.2018.02.009
Ahlström, A., Raupach, M. R., Schurgers, G., Smith, B., Arneth, A., Jung, M., et al. (2015). The dominant role of semi-arid ecosystems in the trend and variability of the land CO2 sink. Science 348, 895–899. doi: 10.1126/science.aaa1668
Baldocchi, D. (2014). Measuring fluxes of trace gases and energy between ecosystems and the atmosphere - the state and future of the eddy covariance method. Glob. Chang. Biol. 20, 3600–3609. doi: 10.1111/gcb.12649
Balogh, J., Pinter, K., Foti, S., Cserhalmi, D., Papp, M., and Nagy, Z. (2011). Dependence of soil respiration on soil moisture, clay content, soil organic matter, and CO2 uptake in dry grasslands. Soil Biol. Biochem. 43, 1006–1013. doi: 10.1016/j.soilbio.2011.01.017
Chen, C., Jing, C. Q., Xing, W. Y., Deng, X. J., Fu, H. Y., and Guo, W. (2021). Desert grassland dynamics in the last 20 years and its response to climate change in Xinjiang. Acta Pratacul. Sin. 30, 1–14. doi: 10.11686/cyxb2020143
Chen, X. P., Liu, T. X., Wang, G. L., Duan, L. M., Lei, H. M., and Wang, D. (2018). Effects of temperature and moisture on net ecosystem CO2 exchange over a meadow wetland in the Horqin, China. Chin. J. Appl. Ecol. 29, 1523–1534. doi: 10.13287/j.1001-9332.201805.003
Chen, X. Q., and Wang, L. H. (2009). Progress in remote sensing Phenological research. Prog. Geogr. 28, 33–40. doi: 10.11820/dlkxjz.2009.01.005
Delgado-Balbuena, J., Loescher, H. W., Aguirre-Gutiérrez, C. A., Alfaro-Reyna, T., Pineda-Martínez, L. F., Vargaset, R., et al. (2022). Dynamics of short-term ecosystem carbon fluxes induced by precipitation events in a semiarid grassland. Biogeosci. Discuss. 1–29. doi: 10.5194/bg-2022-231
Dong, G. (2011). Carbon and water fluxes and water Ilse efficiency of the Svngnen meadow steppe in Northeast China. Master’s thesis. JL: Northeast Normal University.
Fa, K. Y., Liu, J. B., Zhang, Y. Q., Wu, B., Qin, S. G., Feng, W., et al. (2015). CO2 absorption of sandy soil induced by rainfall pulses in a desert ecosystem. Hydrol. Process. 29, 2043–2051. doi: 10.1002/hyp.10350
Fang, Q. Q., Wang, G. Q., Xue, B. L., Liu, T. X., and Kiem, A. (2018). How and to what extent does precipitation on multi-temporal scales and soil moisture at different depths determine carbon flux responses in a water-limited grassland ecosystem? Sci. Total Environ. 635, 1255–1266. doi: 10.1016/j.scitotenv.2018.04.225
Fay, P. A., Kaufman, D. M., Nippert, J. B., Carlisle, J. D., and Harper, C. W. (2008). Changes in grassland ecosystem function due to extreme rainfall events: implications for responses to climate change. Glob. Chang. Biol. 14, 1600–1608. doi: 10.1111/j.1365-2486.2008.01605.x
Gemechu Legesse, T., Dong, G., Jiang, S. C., Chen, J. Y., Dong, X. B., Alemu Daba, N., et al. (2021). Small precipitation events enhance the Eurasian grassland carbon sink. Ecol. Indic. 131:108242. doi: 10.1016/j.ecolind.2021.108242
Gong, T. T., Lei, H. M., Yang, D. W., Yang, H. B., Liu, T. X., and Duan, L. M. (2018). Assessing impacts of extreme water and temperature conditions on carbon fluxes in two desert shrublands. J. Hydroelectr. Eng. 37, 32–46. doi: 10.11660/slfdxb.20180204
Gu, Q., Wei, J., Luo, S. C., Ma, M. G., and Tang, X. G. (2018). Potential and environmental control of carbon sequestration in major ecosystems across arid and semi-arid regions in China. Sci. Total Environ. 645, 796–805. doi: 10.1016/j.scitotenv.2018.07.139
Hao, Y., Wang, Y., Mei, X., and Cui, X. (2010). The response of ecosystem CO2 exchange to small precipitation pulses over a temperate steppe. Plant Ecol. 209, 335–347. doi: 10.1007/s11258-010-9766-1
Hao, Y. B., Zhou, C. T., Liu, W. J., Li, L. F., Kang, X. M., Jiang, L. L., et al. (2017). Aboveground net primary productivity and carbon balance remain stable under extreme precipitation events in a semiarid steppe ecosystem. Agric. For. Meteorol. 240-241, 1–9. doi: 10.1016/j.agrformet.2017.03.006
Harper, C. W., Blair, J. M., and Fay, P. A. (2005). Increased rainfall variability and reduced rainfall amount decreases soil CO2 flux in a grassland ecosystem. Glob. Chang. Biol. 11, 322–334. doi: 10.1111/j.1365-2486.2005.00899.x
Huxman, T. E., Snyder, K. A., Tissue, D., Leffler, A. J., Ogle, K., Pockman, W. T., et al. (2004). Precipitation pulses and carbon fluxes in semiarid and arid ecosystems. Oecologia 141, 254–268. doi: 10.1007/s00442-004-1682-4
Jia, X., Zha, T. S., Wu, B., Zhang, Y. Q, Gong, J. N., Qin, S. G., et al. (2014). Biophysical controls on net ecosystem CO2 exchange over a semiarid shrubland in northwest China. Biogeosci. 11, 4679–4693. doi: 10.5194/bg-11-4679-2014
Jiang, Y., Wang, P., Xu, X., and Zhang, J. (2014). Dynamics of carbon fluxes with responses to vegetation, meteorological and terrain factors in the south-eastern Tibetan plateau. Environ. Earth Sci. 72, 4551–4565. doi: 10.1007/s12524-0
Kim, J., and Verma, S. B. (1990). Carbon dioxide exchange in a temperate grassland ecosystem. Bound.-Layer Meteorol. 52, 135–149. doi: 10.1007/bf00123181
Kozak, M., and Kang, M. S. (2006). Note on modern path analysis in application to crop science. Commun. Biometry Crop Sci. 1, 32–34.
Li, Q. Q. (2020). Application of eddy covariance theory in carbon flux observation of Xilamuren Desert steppe. Master’s thesis. NM: Inner Mongolia Agricultural University.
Li, B. W., Wang, Q., Lu, W. W., Zhou, Y., Jiang, L. L., Liu, P. P., et al. (2021). The effects of warming and added water on key processes of grassland carbon cycle. Acta Ecol. Sin. 41, 1668–1679. doi: 10.5846/stxb201901010006
Li, C. F., Zhang, C., Luo, G. P., Chen, X., Maisupova, B., Madaminov, A. A., et al. (2015). Carbon stock and its responses to climate change in Central Asia. Glob. Chang. Biol. 21, 1951–1967. doi: 10.1111/gcb.12846
Li, F., Zhao, W. Z., and Liu, H. (2013). The response of aboveground net primary productivity of desert vegetation to rainfall pulse in the temperate desert region of Northwest China. PLoS One 8:e73003. doi: 10.1371/journal.pone.0073003
Meena, A., Hanief, M., Dinakaran, J., and Rao, K. S. (2020). Soil moisture controls the spatio-temporal pattern of soil respiration under different land use systems in a semi-arid ecosystem of Delhi, India. Ecol. Process. 9, 1–13. doi: 10.1186/s13717-020-0218-0
Moncrieff, J. B., Massheder, J. M., Debruin, H., Elbers, J., Friborg, T., Heusinkveld, B., et al. (1997). A system to measure surface fluxes of momentum, sensible heat, water vapour and carbon dioxide. J. Hydrol. 188-189, 589–611. doi: 10.1016/S0022-1694(96)03194-0
Novick, K. A., Ficklin, D. L., Stoy, P. C., Williams, C. A., Bohrer, G., Oishi, A. C., et al. (2016). The increasing importance of atmospheric demand for ecosystem water and carbon fluxes. Nat. Clim. Chang. 6, 1023–1027. doi: 10.1038/nclimate3114
Nyantakyi-Frimpong, H., and Bezner-Kerr, R. (2015). The relative importance of climate change in the context of multiple stressors in semi-arid Ghana. Glob. Environ. Change 32, 40–56. doi: 10.1016/j.gloenvcha.2015.03.003
Ouyang, Z., Chen, J., Becker, R., Chu, H., Xie, J., Shao, C., et al. (2014). Disentangling the confounding effects of PAR and air temperature on net ecosystem exchange at multiple time scales. Ecol. Complex. 19, 46–58. doi: 10.1016/j.ecocom.2014.04.005
Papale, D., Reichstein, M., Aubinet, M., Canfora, E., Bernhofer, C., Kutsch, W., et al. (2006). Towards a standardized processing of net ecosystem exchange measured with eddy covariance technique: algorithms and uncertainty estimation. Biogeosciences 3, 571–583. doi: 10.5194/bg-3-571-2006
Parton, W. J., Scurlock, J. M. O., Ojima, D. S., Schimel, D. S., Hall, D. O., Coughenour, M. B., et al. (1995). Impact of climate-change on grassland production and soil carbon worldwide. Glob. Chang. Biol. 1, 13–22. doi: 10.1111/j.1365-2486.1995.tb00002.x
Peng, S. S., Piao, S. L., Shen, Z. H., Ciais, P., Sun, Z. Z., Chen, S. P., et al. (2013). Precipitation amount, seasonality and frequency regulate carbon cycling of a semi-arid grassland ecosystem in Inner Mongolia, China: a modeling analysis. Agric. For. Meteorol. 178-179, 46–55. doi: 10.1016/j.agrformet.2013.02.002
Piao, S. L., Zhang, X. P., Chen, A. P., Liu, Q., Lian, X., Wang, X. H., et al. (2019). The impacts of climate extremes on the terrestrial carbon cycle: a review. Sci. China Earth Sci. 62, 1551–1563. doi: 10.1007/s11430-018-9363-5
Reichstein, M., Falge, E., Baldocchi, D., Papale, D., Aubinet, M., Berbigier, P., et al. (2005). On the separation of net ecosystem exchange into assimilation and ecosystem respiration: review and improved algorithm. Glob. Chang. Biol. 11, 1424–1439. doi: 10.1111/j.1365-2486.2005.001002.x
Rey, A., Oyonarte, C., Moran-Lopez, T., Raimundo, J., and Pegoraro, E. (2017). Changes in soil moisture predict soil carbon losses upon rewetting in a perennial semiarid steppe in SE Spain. Geoderma 287, 135–146. doi: 10.1016/j.geoderma.2016.06.025
Schwinning, S., Sala, O. E., Loik, M. E., and Ehleringer, J. R. (2004). Thresholds, memory, and seasonality: understanding pulse dynamics in arid/semi-arid ecosystems. Oecologia 141, 191–193. doi: 10.1007/s00442-004-1683-3
Tang, Y. K., Jiang, J., Chen, C., Chen, Y. M., and Wu, X. (2018). Rainfall pulse response of carbon fluxes in a temperate grass ecosystem in the semiarid loess plateau. Ecol. Evol. 8, 11179–11189. doi: 10.1002/ece3.4587
Thomey, M. L., Collins, S. L., Vargas, R., Johnson, J. E., Brown, R. F., Natvig, D. O., et al. (2011). Effect of precipitation variability on net primary production and soil respiration in a Chihuahuan Desert grassland. Glob. Chang. Biol. 17, 1505–1515. doi: 10.1111/j.1365-2486.2010.02363.x
Umair, M., Kim, D., Ray, R. L., and Choi, M. (2020). Evaluation of atmospheric and terrestrial effects in the carbon cycle for forest and grassland ecosystems using a remote sensing and modeling approach. Agric. For. Meteorol. 295:108187. doi: 10.1016/j.agrformet.2020.108187
Vargas, R., Baldocchi, D. D., Allen, M. F., Bahn, M., Black, T. A., Collins, S. L., et al. (2010). Looking deeper into the soil: biophysical controls and seasonal lags of soil CO2 production and efflux. Ecol. Appl. 20, 1569–1582. doi: 10.1890/09-0693.1
Vicca, S., Bahn, M., Estiarte, M., Van Loon, E. E., Vargas, R., Alberti, G., et al. (2014). Can current moisture responses predict soil CO2 efflux under altered precipitation regimes? A synthesis of manipulation experiments (vol 11, pg 2991, 2014). Biogeosciences 11, 3307–3308. doi: 10.5194/bg-11-3307-2014
Wang, J. (2015). Study on flux observation and characteristic of cycle of carbon and water in Horqin dune-meadow landscape using Eddy covariance method. Master’s thesis. NM: Inner Mongolia Agricultural University.
Wang, Y., Chen, J. Q., Zhou, G. S., Shao, C. L., Chen, J., Wang, Y., et al. (2018). Predominance of precipitation event controls ecosystem CO2 exchange in an inner Mongolian desert grassland, China. J. Clean. Prod. 197, 781–793. doi: 10.1016/j.jclepro.2018.06.107
Wang, W. Y., Guo, J. X., Wang, Y. S., and Wu, K. (2015). Observing characteristics of CO2 flux and its influencing factors over Xilinhot grassland. J. Meteorol. Sci. 35, 100–107. doi: 10.3969/2013jms.0065
Wang, H., Tetzlaff, D., Dick, J. J., and Soulsby, C. (2017). Assessing the environmental controls on scots pine transpiration and the implications for water partitioning in a boreal headwater catchment. Agric. For. Meteorol. 240-241, 58–66. doi: 10.1016/j.agrformet.2017.04.002
Wang, S., Zhang, Y., Lü, S., Su, P., Shang, L., and Li, Z. (2016). Biophysical regulation of carbon fluxes over an alpine meadow ecosystem in the eastern Tibetan plateau. Int. J. Biometeorol. 60, 801–812. doi: 10.1007/s00484-015-1074-y
Wang, Y., Zhou, L., Jia, Q. Y., and Ping, X. Y. (2019). Direct and indirect effects of environmental factors on daily CO2 exchange in a rainfed maize cropland-a SEM analysis with 10 year observations. Field Crop Res. 242:107591. doi: 10.1016/j.fcr.2019.107591
Webb, E. K., Pearman, G. I., and Leuning, R. (1980). Correction of flux measurements for density effects due to heat and water vapour transfer. Q. J. R. Meteorol. Soc. 106, 85–100. doi: 10.1002/qj.49710644707
Wu, J., Guan, K., Hayek, M., Restrepo-Coupe, N., Wiedemann, K. T., Xu, X., et al. (2017). Partitioning controls on Amazon forest photosynthesis between environmental and biotic factors at hourly to interannual timescales. Glob. Chang. Biol. 23, 1240–1257. doi: 10.1111/gcb.13509
Xie, X. D., Wang, T. J., Yue, X., Li, S., Zhuang, B. L., and Wang, M. H. (2020). Effects of atmospheric aerosols on terrestrial carbon fluxes and CO2 concentrations in China. Atmos. Res. 237:104859. doi: 10.1016/j.atmosres.2020.104859
Xue, H. X., Li, Q., Huang, Y., Wang, Y. L., and Wu, D. L. (2014). The effect of soil environmental factors on the carbon flux over Stipa krylovii ecosystem. Sci. Geogr. Sin. 34, 1385–1392. doi: 10.13249/j.cnki.sgs.2014.011.1385
Yan, Y. J., Zhou, L., Zhou, G. S., Wang, Y., Song, J. X., Zhang, S., et al. (2023). Extreme temperature events reduced carbon uptake of a boreal forest ecosystem in Northeast China: evidence from an 11-year eddy covariance observation. Front. Plant Sci. 14:1119670. doi: 10.3389/fpls.2023.1119670
Yang, F. L., and Zhou, G. S. (2013). Sensitivity of temperate desert steppe carbon exchange to seasonal droughts and precipitation variations in Inner Mongolia. Plos One 8:e55418. doi: 10.1371/journal.pone.0055418
Yuan, Z. F., Zhou, J. Y., Guo, M. C., Lei, X. Q., and Xie, X. L. (2001). Decision coefficient-the decision index of path analysis. J. Northwest Sci. Tech. Univ. Agric. For 29, 133–135. doi: 10.13207/j.cnki.jnwafu.2001.05.035
Yue, G. Y., Zhao, L., Zhao, Y. H., and Li, Y. S. (2010). Research advances of grassland ecosystem CO2 flux on Qinghai-Tibetan plateau. J. Glaciol. Geocryol. 25, 1263–1267. doi: 10.3724/SP.J.1077.2010.01263
Zhang, R., Zhao, X. Y., Zuo, X. A., Degen, A. A., Li, Y. L., Liu, X. P., et al. (2020). Drought-induced shift from a carbon sink to a carbon source in the grasslands of Inner Mongolia. China. Catena 195:104845. doi: 10.1016/j.catena.2020.104845
Zhou, Y. Y., Li, X. R., Gao, Y. H., He, M. Z., Wang, M. M., Wang, Y. L., et al. (2020). Carbon fluxes response of an artificial sand-binding vegetation system to rainfall variation during the growing season in the Tengger Desert. J. Environ. Manag. 266:110556. doi: 10.1016/j.jenvman.2020.110556
Keywords: desert steppe, net ecosystem CO2 exchange, rainfall events, eddy covariance, environmental controls
Citation: Song J, Zhou L, Zhou G, Wang Y, Zhang S and Yan Y (2023) Hydrometeorological controls on net carbon dioxide exchange over a temperate desert steppe in Inner Mongolia, China. Front. Ecol. Evol. 11:1169297. doi: 10.3389/fevo.2023.1169297
Edited by:
Zhongqing Yan, Chinese Academy of Forestry, ChinaReviewed by:
Qinghai Song, Xishuangbanna Tropical Botanical Garden (CAS), ChinaBing Song, Ludong University, China
Xiaodong Zhang, Chinese Academy of Forestry, China
Copyright © 2023 Song, Zhou, Zhou, Wang, Zhang and Yan. This is an open-access article distributed under the terms of the Creative Commons Attribution License (CC BY). The use, distribution or reproduction in other forums is permitted, provided the original author(s) and the copyright owner(s) are credited and that the original publication in this journal is cited, in accordance with accepted academic practice. No use, distribution or reproduction is permitted which does not comply with these terms.
*Correspondence: Li Zhou, zhouli@cma.gov.cn; Guangsheng Zhou, zhougs@cma.gov.cn