- College of Resources and Environment, Hunan Agricultural University, Changsha, China
Here, capacitive deionization technology (CDI) using modified activated carbon fiber felt (ACF) electrodes was proposed to provide a new strategy for the challenge of simultaneous phosphorus and fluoride wastewater treatment. The acid-modified iron-loaded ACF (A@Fe-ACF) was obtained by modifying ACF through a two-step impregnation method. After the modification, the oxygen-containing functional groups on ACF increased and provided more adsorption sites. The electron transfer efficiency on the A@Fe-ACF was increased by introducing Fe and synergistically promoted the adsorption of phosphorus and fluorine. Results showed that the removal efficiencies of total phosphorus (TP) and total fluorine (TF) in wastewater reached 89.4% and 85% under optimal conditions (voltage intensity 1.5 V, pH 7, plate spacing 1 cm), while the adsorption mechanism of phosphorus and fluorine was dominated by chemical adsorption. Meanwhile, A@Fe-ACF electrode has good recyclability and stability after five cycles.
1 Introduction
With the rapid development of the agricultural economy, water pollution caused by the discharge of phosphorus chemical sewage was increasingly severe (Xia et al., 2021). The phenomenon of excessive phosphorus and fluorine produced in the production process of phosphorus compound fertilizer has received significant attention. The characteristics of this type of wastewater are acidic, and the primary pollutants exist in various forms, such as F−, SiF62-, PO43-, H2PO4−, and HPO42-, posing serious harm to ecology and human health (Gouider et al., 2010). The neutralization precipitation process is the primary strategy to treat phosphorus and fluoride wastewater, while fluoride and phosphate ions can be precipitated and separated from the water body (Al-Harahsheh et al., 2014; Wang et al., 2020). However, there are problems with the high cost and the large amount of sludge caused by adding a large amount of neutralizing reagents. It is challenging to realize the requirements of water purification while considering the economy. Current treatment technology mainly focuses on phosphorus or fluorine removal alone, but the efficient process for simultaneous removal of phosphorus and fluoride still has a large exploration potential. In light of this, developing a clean, efficient, and sustainable technology for phosphorus and fluorine removal from wastewater is significant.
Capacitive deionization technology has been widely used as a promising electrochemical technology in the past decade due to low energy consumption, easy electrode regeneration, and no secondary pollution (Ayranci and Conway, 2001; Kalfa et al., 2020; Chai et al., 2021). The electric field is applied to the electrode to form an electric double layer at the electrode/solution interface, then the charged ions in the solution are enriched and concentrated at the electrode interface, thereby realizing the removal of pollutant targets. The electrode material is a crucial part of CDI which significantly influences this process. Among many reported electrode materials, activated carbon fiber felt (ACF), as a low-cost electrode material with high specific surface area, rich pore structure, and stable electrochemical performance, was widely used in the CDI treatment of wastewater (Foo and Hameed, 2009; Chen et al., 2015). The CDI process based on the ACF electrodes has achieved good results in treating phosphorus or fluorine-containing wastewater, and the effect of CDI could be further improved by modifying ACF (Zhang et al., 2020; Martinez-Vargas et al., 2021). In addition, CDI also played a vital role in composite wastewater containing phosphorus or fluorine (Gaikwad and Balomajumder, 2017). Optimized carbon-based electrodes will provide more adsorption sites, which creates opportunities to remove multiple contaminants (Kalfa et al., 2020). Although it has promising prospects in complex wastewater, the study of the simultaneous treatment of phosphorus and fluorine in wastewater through CDI still needs to be completed. Considering above these, treating phosphorus and fluorine in wastewater simultaneously based on CDI using the ACF electrode may be a meaningful and feasible solution.
To further improve the ACF electrode performance, it was modified by acid treatment and iron salt impregnation in our work for the simultaneous treatment of phosphorus and fluoride wastewater. Fe was introduced to enhance the binding with pollutants, reduce the resistance of ACF, and improve the electrochemical performance of electrode. After the electrodes were prepared, a series of characterizations were carried out. The influence of critical factors on the removal efficiency of phosphorus and fluoride wastewater was systematic explored to evaluate the potential in practical application. The mechanism of phosphorus and fluorine removal was also discussed. Finally, the stability and reusability of the modified ACF electrodes were investigated. This work aims to provide a new idea for the simultaneous treatment of phosphorus and fluoride wastewater.
2 Experiment section
2.1 Wastewater and regents
The phosphorus and fluoride wastewater used in this experiment was collected from the phosphate fertilizer factory (Zhuzhou, China), and the detailed information are: pH 3.53, 82.7 mg/L TF, 121.2 mg/L TP, 112.5 mg/L COD, 1800 mg/L SO42-, 45 mg/L NH3-N, and 3,320 μs/cm conductivity. All experiments were conducted using ultrapure water (UPH, 18.25 MΩ·cm). The pH of the wastewater was adjusted by 0.1 mM NaOH solution.
2.2 Preparation of modified electrode
The electrode preparation process was shown in Figure 1. The commercial ACF was cut into 5 × 10 cm squares, placed in a beaker, boiled with boiling water for 2 h, and soaked in absolute ethanol for 2 h to remove ash impurities in the ACF. Then, the ACF was soaked in 30% HNO3 solution for 6 h, washed with ultrapure water until the pH reached neutrality, and dried. Next, the acid-treated ACF was immersed in 200 ml of FeCl3 solution (0%–3%) for 12 h. After taking it out to dry, the ACF was put in a tube furnace for calcination at different temperatures under an N2 atmosphere for 4 h to obtain the A@Fe-ACF electrode.
2.3 Electrode characterization
The properties of the prepared ACF electrodes were characterized using different analytical techniques, including X-ray diffraction (XRD, Ultima IV, Japan), Scanning electron microscopy (SEM, Hitachi S4800, Japan), X-ray photoelectron spectroscopy (XPS, Thermo ESCALAB 250XI, United States), Fourier transform infrared absorption spectroscopy (FTIR, Niolet iN10, United States), surface area and pore size analyzer (BET, ASAP2460, United States), and electrochemical impedance spectroscopy measurements (EIS, CHI 660E, China).
2.4 Experiment procedure
The CDI process was performed in a special acrylic cuboid reactor (L = 10 cm, W = 5 cm, H = 12 cm), with A@Fe-ACF as the anode attached to a stainless-steel plate and graphite plate as the cathode (Figure 2; Supplementary Figure S1). The electrodes are connected to a direct current (DC) regulated power supply (MESTEK DP3030) and maintained at a constant distance. After applying a voltage to the electrodes to initiate the reaction, key parameters such as voltage intensity, initial pH, plate spacing, and phosphorus and fluorine (P/F) ratio on phosphorus and fluorine removal efficiency were investigated by a conventional parametric approach. At preselected time intervals, the extracted solutions were transferred to 5 ml sampling tubes and analyzed uniformly.
2.5 Analysis methods
The concentration changes of total phosphorus (TP) and total fluorine (TF) were used to represent the removal efficiency of phosphorus and fluorine. Molybdenum antimony anti-spectrophotometry was used to determine the concentration of total phosphorus. The ion-selective electrode method was used to determine the TF concentration (the indicator electrode was a fluoride ion-selective electrode, and the reference electrode was a saturated calomel electrode).
2.6 Statistics analysis
Data used in this study were entered in Excel, and all data were tested for normality (Shapiro-Wilk test) and homogeneity (Levene test) before analysis, and Duncan multiple comparisons were performed on the data means. TP and TF removal efficiency under different conditions were analyzed by one-way ANOVA. All data were statistically analyzed using IBM SPSS Statistics 23.0 software.
3 Results and discussion
3.1 Characteristics of A@Fe-ACF
The morphologies of ACF before and after modification are shown in Figure 3. The initial ACF surface was smooth and flat (Figure 3A), and it became rough after HNO3 modification (Figure 3B) due to the strong oxidizing property of HNO3 corroding the ACF surface. The surface morphology of ACF was changed after acid treatment, and the pore structure was increased, which significantly increased the specific surface area of ACF (Figure 4A). Furthermore, the acid-treated ACF could provide more loading sites for Fe, conducive to the tighter bonding between the loading metal and the carrier. It can be seen that the iron oxide was covered on the ACF in a sheet shape when the iron loading modification was carried out alone (Figure 3C). The smooth carbon fiber surface does not provide an excellent binding point for the Fe element and will lead to the iron oxide difficult to bonded on the ACF and is easy to fall off. When ACF was treated with acid and modified with Fe loading, it could be observed that Fe oxides were tightly bound on ACF in irregular granular form compared with the modification of Fe alone (Figures 3D–H). Although Fe loading leads to a slight decrease in the specific surface area (Figure 4A), the synergistic effect of Fe oxides has further improved the TP and TF removal. The calcination temperature is another key factor affecting the CDI performance of A@Fe-ACF, as the adsorption efficiency gradually increased with the temperature. However, the removal efficiency of TP and TF decreased when the temperature was higher than 400°C (Supplementary Figures S2A, 2B). It can be seen from SEM that the Fe oxide on the surface of ACF is evenly distributed at 400°C, while the Fe oxide begins to sinter, and the load area becomes smaller when the temperature increases to 500°C–600°C (Figures 3E, F), thus affecting the CDI efficiency of A@Fe-ACF. In addition, the load of Fe is also an essential factor. The introduction of iron improved the adsorption efficiency of phosphorus and fluorine in wastewater, while the adsorption capacity also increased significantly with the loading concentration (Supplementary Figure S2C). However, the higher loading concentration would lead to Fe oxide blocking pores and inhibit the effect of CDI process. Therefore, 2% Fe loading concentration and the 400°C calcination temperature were the optimal conditions for A@Fe-ACF. The electrochemical properties of the electrodes were compared using EIS (Supplementary Figure S3), and it can be seen that the transfer resistance of the ACF decreases significantly with Fe loading. The presence of Fe accelerated the electron transfer efficiency and improved the conductivity of the electrode, which facilitated the capacitive deionization process.
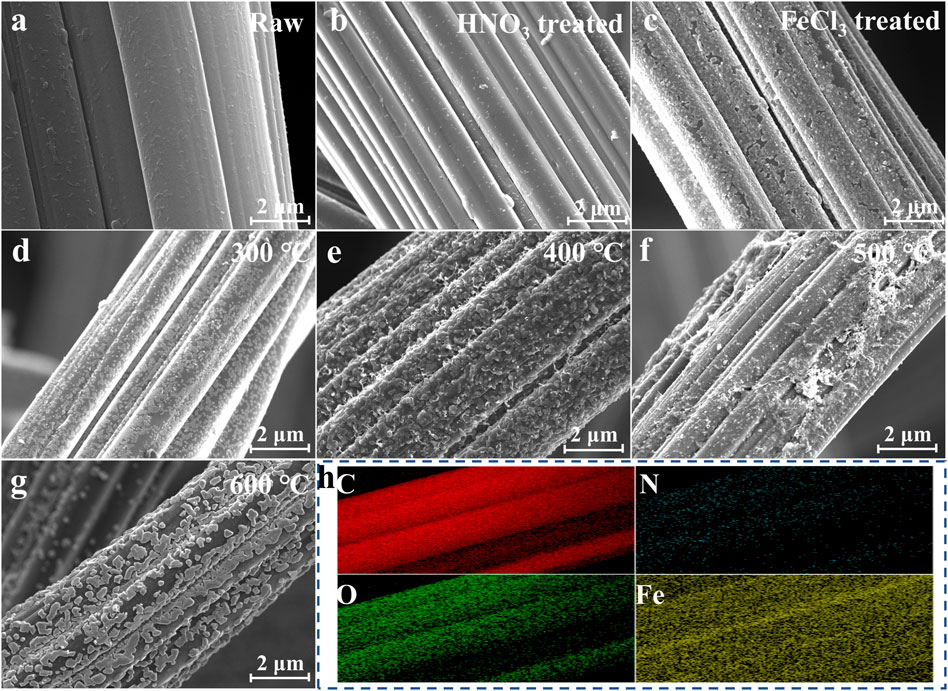
FIGURE 3. SEM of A@Fe-ACF under different calcination temperature: (A) Raw. (B) ACF treated by HNO3. (C) ACF treated by FeCl3. (D) 300°C. (E) 400°C. (F) 500°C. (G) 600 °C. (H) Element mapping of A@Fe-ACF.
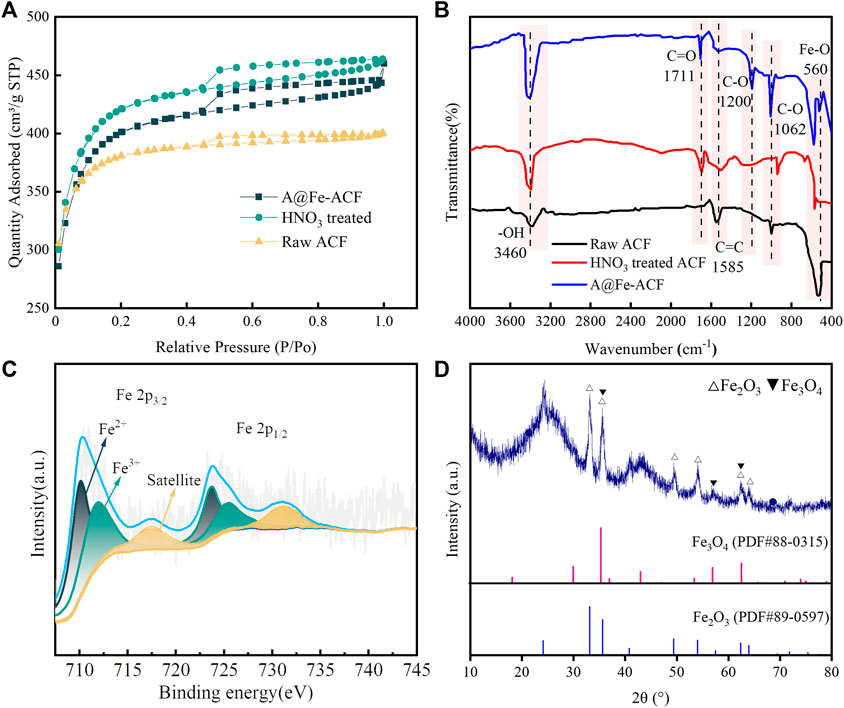
FIGURE 4. (A) N2-adsorption isotherms of electrodes with different modification. (B) FTIR of electrodes with different modifications. (C) XPS spectrum of A@Fe-ACF-400. (D) The XRD spectrum of A@Fe-ACF-400.
Figure 4B showed the FTIR images under different modification conditions. The characteristic peak at 3,460 cm−1 is the stretching vibration peak of -OH, and the absorption peak here is significantly enhanced after modification, indicating that the hydrophilicity of the material has been improved. The new peak at 1711 cm−1 may be the characteristic peak of C=O bond stretching vibration in the carboxylic acid group, representing the introduction of oxygen-containing functional groups after acid modification (Wu et al., 2015). The peak at 1,630 cm−1 represents the backbone vibration of C=C, where the intensity gradually decreased with ACF modification. This phenomenon might be caused by the high temperature brought by the heat treatment (Wang et al., 1994; Georgakopoulos, 2003). The enhancement of the C-O bond at 1,200 cm−1 and 1,062 cm−1 could be attributed to the production of more abundant oxygen-containing groups (Meng et al., 2018). Moreover, the metal oxide peak at 560 cm−1 is Fe-O (Verma et al., 2021), which further proved that Fe was efficiently loaded on the ACF surface.
From XPS analysis in Figure 4C, it could be observed that Fe in A@Fe-ACF-400 exists as Fe2+ and Fe3+, which could be attributed to the introduction of oxygen-containing functional groups promoted the redox on the electrode surface (Ren et al., 2020). The cycling of Fe2+/Fe3+ increased the current efficiency, thereby indirectly improving the effect of the CDI process (Wang et al., 2021). The iron element in A@Fe-ACF-400 mainly existed in Fe2O3 and Fe3O4 (shown in Figure 4D). The characteristics of Fe oxides could enhance the electron transfer in the electric field and improve the efficiency of electro adsorption to remove phosphorus and fluorine ions (Song et al., 2019).
3.2 Effect of key factors on CDI performance
Voltage intensity played a crucial role In the CDI process. It can be seen from Figures 5A, B that the removal of TP and TF showed a trend of first high and then low with the increase of voltage. When the voltage was 1.5 V, the removal of TP and TF reached 89.4% and 85%. The increase in voltage drives a higher current and establishes a steady-state faster, then the increase in electron mobility promotes the accumulation and adsorption of contaminant ions in the electric double-layer (Chai et al., 2020; Lin et al., 2022). However, the removal efficiency dropped to 83% and 78% when the voltage intensity was increased to 2 V. That is the excessively high voltage aggravating the hydrogen evolution side reaction Eq. 1, thus resulting in the desorption of PO43- and F− from the ACF and inhibiting the removal of phosphorus and fluorine (Ren et al., 2016). Therefore, 1.5 V was selected as the optimum voltage intensity. The solution pH is one of the essential factors to be considered in the removal of phosphorus and fluoride. Within a certain range, the removal efficiencies of TP and TF increased with the pH and reached the best at pH 7 (Figures 5C, D). The pH value significantly affects the existence of phosphate and fluoride ions in the solution Eqs. 2–4. When the pH is acidic, the uncharged H3PO4 occupies the dominant position, while when the pH is 7, the charged HPO42- increases (Huang et al, 2017a), then the hydration radius decreases, which is easier to be absorbed by the electric field (Gabelich et al., 2002; Huang et al., 2013). Moreover, fluorine mainly exists in the F− when the pH is more than 6, which benefits CDI treatment and stabilization, and a good electrostatic interaction under neutral conditions can positively influence the adsorption of F− (Martinez-Vargas et al., 2021). However, when the pH was higher than 7, the removal rate of TP and TF decreased instead. This trend may be attributed to the enhanced competition between phosphate ions and hydroxyl ions for electrode adsorption sites at higher pH (Huang et al., 2013). A Higher pH environment could cause electrostatic repulsion to re-release the adsorbed fluoride, thus reducing the removal efficiency (Yu et al., 2018). In conclusion, pH seven would be more suitable for A@Fe-ACF electrode CDI treatment of phosphorus-fluoride wastewater. It can be seen from Figures 5E, F that the removal efficiency of TP and TF was the highest when the plate spacing is 1 cm. The closer plate spacing improved the rate of ion migration between the plates, enhanced the ions enriched on the electric double layer and the thickness of the electric double layer, further reduced the migration distance of HPO42- and F− in the electric field, and accelerated the adsorption of target ions. An excessively close plate spacing would interfere with the reactions between cathode and anode, and the rapidly increasing current intensity may lead to side reactions (Chai et al., 2020). In contrast, the long distance in the CDI reaction could limit ion mobility and exacerbates energy consumption (Yang et al., 2020). Therefore, 1 cm was determined as the optimal plate spacing.
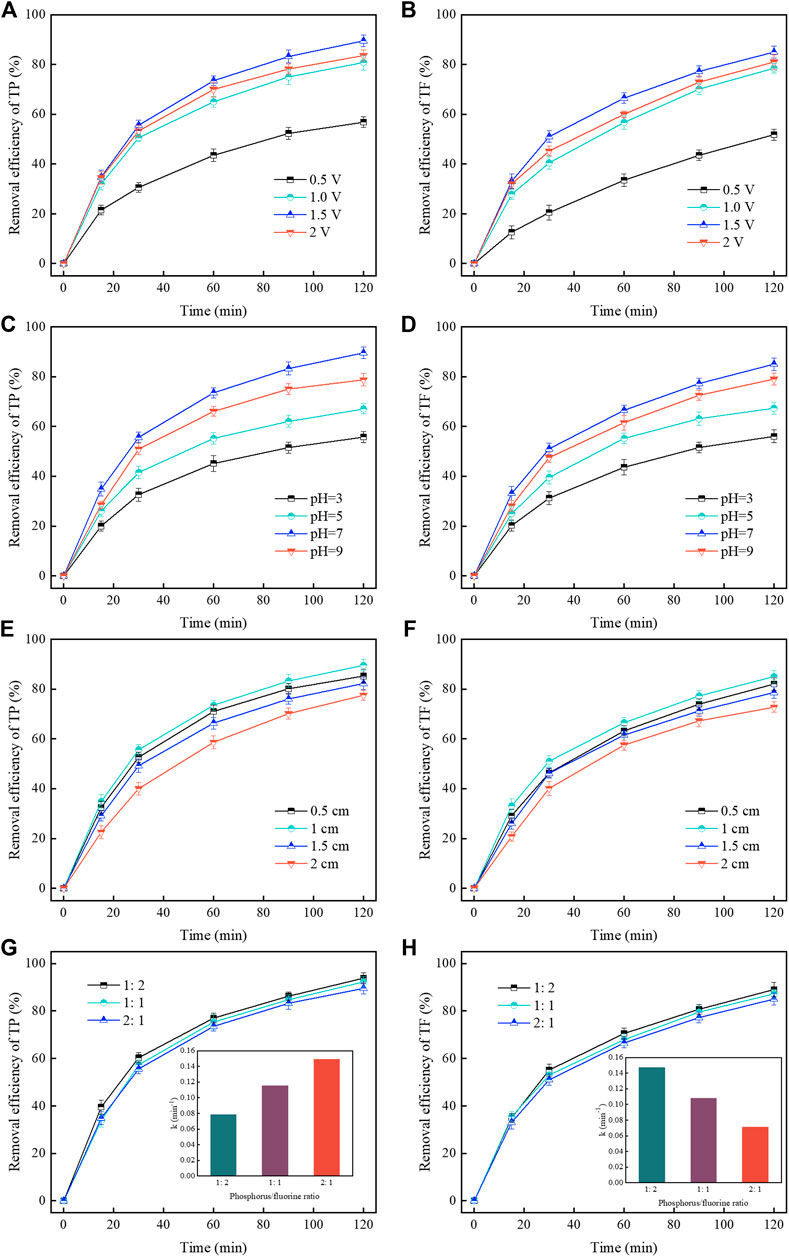
FIGURE 5. The effect of voltage intensity on removal efficiency: (A) TP. (B) TF. The effect of pH on removal efficiency: (C) TP. (D) TF. The effect of plate spacing on removal efficiency: (E) TP. (F) TF. The effect of phosphorus/fluorine ratio on removal efficiency: (G) TP (H) TF.
Exploring the effect of the P/F ratio in wastewater on the CDI is important. The removal of TF increased with decrease in the relative concentration of phosphorus (Figures 5G, H). The adsorption rate constant of TP decreased from 0.1492 to 0.0782, while that of TF increased from 0.071 to 0.147 when the P/F ratio changed from 2:1 to 1:2. For HPO42-, the value of Z/r (charge/radius) is higher than that of F−, which is beneficial to its adsorption and binding on ACF (Zuo et al., 2016). When the phosphorus concentration decreased, the binding competitiveness reduced, and the adsorption trend of F− increased. Therefore, the control of the P/F ratio in wastewater could be a pivotal step to further improving the simultaneous treatment efficiency.
By studying the adsorption process of the coexistence of NO3−, Cl−, SO42-, HCO3−, and SiO32-, it can be found that different anions concentrations have different effects on the TP and TF removal efficiency (Supplementary Figures S4A, 4B). The presence of NO3− and Cl− did not affect the removal of phosphorus and fluorine significantly, while the coexistence of SO42-, HCO3−, and SiO32- had certain effect on the adsorption capacity. That could be attributed to the adsorption competition between coexisting ions with F− and HPO42- (Yu et al., 2018). These anions will lead to the precipitation of Ca2+ in the wastewater, blocking the pores of the ACF and passivating the electrodes, thereby reducing the adsorption capacity of the reaction system (Yoosefian et al., 2017). Results indicated that A@Fe-ACF can still maintain high adsorption efficiency in the presence of different ion concentrations, showing relatively excellent anti-interference performance.
3.3 Adsorption mechanism
The removal efficiency of phosphorus and fluoride under different modified conditions is shown in Figures 6A, B, including the two cases of non-electric adsorption and CDI. It could be observed that under pure adsorption conditions, acid modification and iron impregnation slightly enhanced the adsorption of TP and TF. The removal efficiency of phosphorus and fluorine increased rapidly when the electric field was applied. The increase in the specific surface area caused by the etching of pores using HNO3 and the presence of Fe oxides provide more adsorption sites for ACF, so the acid modification assisted Fe loading significantly promoted the CDI efficiency. The adsorption kinetics of the A@Fe-ACF electrode CDI process was further investigated (Supplementary Figures S5A, 5B). The precision of the pseudo-second-order kinetic equation is better than that of the pseudo-first-order kinetics, indicating that chemical adsorption played a dominant role in this process.
The possible reaction mechanism of simultaneous phosphorus and fluoride wastewater treatment based on A@Fe-ACF electrode CDI is shown in Figure 7. Anions such as HPO42- and F− would move towards the anode under the action of the electric field and subsequently adsorbed on A@Fe-ACF superior. Meanwhile, the loading of Fe can promote the complexation of HPO42- and F−, and enhance the immobilization of pollutants on the electrodes.
3.4 Recycling and stable test
The recycling ability of the electrode is important for evaluating its stability during the CDI process. It can be seen from Figure 8 that after five cycles of A@Fe-ACF by adsorption-desorption, the removal efficiencies of TP and TF dropped to 83% and 77.6%, which still keep a good adsorption performance. The above results show that A@Fe-ACF has good stability in phosphorus-fluoride wastewater treatment.
3.5 Comparison with traditional strategies
Gradual removal of phosphate and fluoride from wastewater using precipitation was the most widely reported strategy (Table 1). After pH adjustment, phosphate and fluoride might precipitate in the form of CaHPO4, Ca3(PO4)2, and CaF2 (Grzmil and Wronkowski, 2006). However, the dosing of large amounts of neutralizing reagents required for higher pH and the adjustment of effluent pH can be costly and operationally burden (Xia et al., 2021). A large number of generated precipitates cannot be recovered entirely, and there is a risk of secondary pollution. Some new phosphorus and fluorine treatment technologies have been proposed and achieved certain results, but the longer treatment period and lower treatment efficiency are still enormous challenges (Wu et al., 2017; Yang et al., 2021). Efficient simultaneous removal of phosphate and fluoride from wastewater can be achieved by CDI using A@Fe-ACF. The presence of salt ions in wastewater could act as an electrolyte to improve the current efficiency, and the lower energy consumption and shorter reaction time will reduce the treatment cost. Based on the above advantages, the simultaneous removal of phosphorus and fluoride wastewater by CDI using an A@Fe-ACF electrode can be an innovative and feasible technology.
4 Conclusion
The A@Fe-ACF-400 electrode was prepared to simultaneously remove phosphorus and fluorine in wastewater by CDI. The removal of TP and TF could reach 89.4% and 85% with the optimal reaction conditions. The combined modification of acid treatment and iron loading enhanced the CDI performance using ACF while endow A@Fe-ACF-400 with good stability and recycling ability. In conclusion, A@Fe-ACF electrode CDI demonstrated promising prospects in the simultaneous treatment of phosphorus and fluoride wastewater.
Data availability statement
The original contributions presented in the study are included in the article/Supplementary Material, further inquiries can be directed to the corresponding author.
Author contributions
ZD wrote the first draft of the manuscript, organized the database, and performed the statistical analysis. TQ contributed to conception and design of the study. CB performed the statistical analysis. ZW checked the English grammar of the manuscript. YG checked the format of the manuscript. PQ contributed to conception and design of the study and provide funding supporting. All authors contributed to manuscript revision, read, and approved the submitted version.
Funding
This work was jointly supported by the Science and Technology Achievement Transformation and Industrialization Plan of Hunan Province (2020NK2001), funder: PQ, the National Natural Science Foundation of China (No. 51808215), funder: ZW, the Hunan Provincial Natural Science Foundation of China (2019JJ50253), funder: ZW.
Conflict of interest
The authors declare that the research was conducted in the absence of any commercial or financial relationships that could be construed as a potential conflict of interest.
Publisher’s note
All claims expressed in this article are solely those of the authors and do not necessarily represent those of their affiliated organizations, or those of the publisher, the editors and the reviewers. Any product that may be evaluated in this article, or claim that may be made by its manufacturer, is not guaranteed or endorsed by the publisher.
Supplementary material
The Supplementary Material for this article can be found online at: https://www.frontiersin.org/articles/10.3389/fenvs.2022.1087231/full#supplementary-material
References
Al-Harahsheh, M., Batiha, M., Kraishan, S., and Al-Zoubi, H. (2014). Precipitation treatment of effluent acidic wastewater from phosphate-containing fertilizer industry: Characterization of solid and liquid products. Sep. Purif. Technol. 123, 190–199. doi:10.1016/j.seppur.2013.12.027
Ayranci, E., and Conway, B. E. (2001). Adsorption and electrosorption at high-area carbon-felt electrodes for waste-water purification: Systems evaluation with inorganic, S-containing anions. J. Appl. Electrochem. 31, 257–266. doi:10.1023/A:1017528002713
Chai, Y., Qin, P., Wu, Z., Bai, M., Li, W., Pan, J., et al. (2021). A coupled system of flow-through electro-Fenton and electrosorption processes for the efficient treatment of high-salinity organic wastewater. Sep. Purif. Technol. 267, 118683. doi:10.1016/j.seppur.2021.118683
Chai, Y., Qin, P., Zhang, J., Li, T., Dai, Z., and Wu, Z. (2020). Simultaneous removal of Fe(II) and Mn(II) from acid mine wastewater by electro-Fenton process. Process Saf. Environ. Prot. 143, 76–90. doi:10.1016/j.psep.2020.06.026
Chen, Z., Zhang, H., Wu, C., Wang, Y., and Li, W. (2015). A study of electrosorption selectivity of anions by activated carbon electrodes in capacitive deionization. Desalination 369, 46–50. doi:10.1016/j.desal.2015.04.022
Foo, K. Y., and Hameed, B. H. (2009). A short review of activated carbon assisted electrosorption process: An overview, current stage and future prospects. J. Hazard. Mat. 170 (2–3), 552–559. doi:10.1016/j.jhazmat.2009.05.057
Gabelich, C. J., Tran, T. D., and Suffet, I. H. (2002). Electrosorption of inorganic salts from aqueous solution using carbon aerogels. Environ. Sci. Technol. 36, 3010–3019. doi:10.1021/es0112745
Gaikwad, M. S., and Balomajumder, C. (2017). Tea waste biomass activated carbon electrode for simultaneous removal of Cr(VI) and fluoride by capacitive deionization. Chemosphere 184, 1141–1149. doi:10.1016/j.chemosphere.2017.06.074
Georgakopoulos, A. (2003). Study of low rank Greek coals using FTIR spectroscopy. Energy sources. 25 (10), 995–1005. doi:10.1080/00908310390232442
Gouider, M., Feki, M., and Sayadi, S. (2010). Bioassay and use in irrigation of untreated and treated wastewaters from phosphate fertilizer industry. Ecotoxicol. Environ. Saf. 73 (5), 932–938. doi:10.1016/j.ecoenv.2009.12.021
Grzmil, B., and Wronkowski, J. (2006). Removal of phosphates and fluorides from industrial wastewater. Desalination 189 (1-3), 261–268. doi:10.1016/j.desal.2005.07.008
Huang, G. H., Chen, T. C., Hsu, S.-F., Huang, Y. H., and Chuang, S. H. (2013). Capacitive deionization (CDI) for removal of phosphate from aqueous solution. Desalination Water Treat. 52 (4-6), 759–765. doi:10.1080/19443994.2013.826331
Huang, H., Liu, J., Zhang, P., Zhang, D., and Gao, F. (2017a). Investigation on the simultaneous removal of fluoride, ammonia nitrogen and phosphate from semiconductor wastewater using chemical precipitation. Chem. Eng. J. 307, 696–706. doi:10.1016/j.cej.2016.08.134
Huang, X., He, D., Tang, W., Kovalsky, P., and Waite, T. D. (2017b). Investigation of pH-dependent phosphate removal from wastewaters by membrane capacitive deionization (MCDI). Environ. Sci. Water Res. Technol. 3 (5), 875–882. doi:10.1039/c7ew00138j
Kalfa, A., Shapira, B., Shopin, A., Cohen, I., Avraham, E., and Aurbach, D. (2020). Capacitive deionization for wastewater treatment: Opportunities and challenges. Chemosphere 241, 125003. doi:10.1016/j.chemosphere.2019.125003
Kong, L., Tian, Y., Pang, Z., Huang, X., Li, M., Yang, R., et al. (2019). Synchronous phosphate and fluoride removal from water by 3D rice-like lanthanum-doped La@MgAl nanocomposites. Chem. Eng. J. 371, 893–902. doi:10.1016/j.cej.2019.04.116
Lin, S., Yang, X., Liu, L., Li, A., and Qiu, G. (2022). Electrosorption of cadmium and arsenic from wastewaters using nitrogen-doped biochar: Mechanism and application. J. Environ. Manage. 301, 113921. doi:10.1016/j.jenvman.2021.113921
Martinez-Vargas, D. R., Larios-Durán, E. R., Chazaro-Ruiz, L. F., and Rangel-Mendez, J. R. (2021). Correlation between physicochemical and electrochemical properties of an activated carbon doped with lanthanum for fluoride electrosorption. Sep. Purif. Technol. 268, 118702. doi:10.1016/j.seppur.2021.118702
Meng, N., Ren, J., Liu, Y., Huang, Y., Petit, T., and Zhang, B. (2018). Engineering oxygen-containing and amino groups into two-dimensional atomically-thin porous polymeric carbon nitrogen for enhanced photocatalytic hydrogen production. Energy Environ. Sci. 11 (3), 566–571. doi:10.1039/c7ee03592f
Mohammadi, E., Daraei, H., Ghanbari, R., Dehestani Athar, S., Zandsalimi, Y., Ziaee, A., et al. (2019). Synthesis of carboxylated chitosan modified with ferromagnetic nanoparticles for adsorptive removal of fluoride, nitrate, and phosphate anions from aqueous solutions. J. Mol. Liq. 273, 116–124. doi:10.1016/j.molliq.2018.10.019
Ren, G., Zhou, M., Liu, M., Ma, L., and Yang, H. (2016). A novel vertical-flow electro-Fenton reactor for organic wastewater treatment. Chem. Eng. J. 298, 55–67. doi:10.1016/j.cej.2016.04.011
Ren, S., Usman, M., Tsang, D. C. W., S, O. T., Angelidaki, I., Zhu, X., et al. (2020). Hydrochar-facilitated anaerobic digestion: Evidence for direct interspecies electron transfer mediated through surface oxygen-containing functional groups. Environ. Sci. Technol. 54 (9), 5755–5766. doi:10.1021/acs.est.0c00112
Song, X., Liu, J., Jiang, Q., Zhang, P., Shao, Y., He, W., et al. (2019). Enhanced electron transfer and methane production from low-strength wastewater using a new granular activated carbon modified with nano-Fe3O4. Chem. Eng. J. 374, 1344–1352. doi:10.1016/j.cej.2019.05.216
Verma, R., Chauhan, A., NehaBatoo, K. M., Kumar, R., Hadhi, M., Raslan, E. H., et al. (2021). Effect of calcination temperature on structural and morphological properties of bismuth ferrite nanoparticles. Ceram. Int. 47 (3), 3680–3691. doi:10.1016/j.ceramint.2020.09.220
Wang, F. C., Feve, M., Lam, T. M., and Pascault, J.-P. (1994). FTIR analysis of hydrogen bonding in amorphous linear aromatic polyurethanes. I. Influence of temperature. J. Polym. Sci. B. Polym. Phys. 32 (8), 1305–1313. doi:10.1002/polb.1994.090320801
Wang, J., Dong, F., Wang, Z., Yang, F., Du, M., Fu, K., et al. (2020). A novel method for purification of phosphogypsum. Physicochem. Probl. Min. Process. 56 (5), 975–983. doi:10.37190/ppmp/127854
Wang, R., Liu, H., Zhang, K., Zhang, G., Lan, H., and Qu, J. (2021). Ni(II)/Ni(III) redox couple endows Ni foam-supported Ni2P with excellent capability for direct ammonia oxidation. Chem. Eng. J. 404, 126795. doi:10.1016/j.cej.2020.126795
Wu, T., Wang, G., Dong, Q., Qian, B., Meng, Y., and Qiu, J. (2015). Asymmetric capacitive deionization utilizing nitric acid treated activated carbon fiber as the cathode. Electrochimica Acta 176, 426–433. doi:10.1016/j.electacta.2015.07.037
Wu, Y., Han, R., Yang, X., Zhang, Y., and Zhang, R. (2017). Long-term performance of an integrated constructed wetland for advanced treatment of mixed wastewater. Ecol. Eng. 99, 91–98. doi:10.1016/j.ecoleng.2016.11.032
Xia, L., Zhang, W., Che, J., Chen, J., Wen, P., Ma, B., et al. (2021). Stepwise removal and recovery of phosphate and fluoride from wastewater via pH-dependent precipitation: Thermodynamics, experiment and mechanism investigation. J. Clean. Prod. 320, 128872. doi:10.1016/j.jclepro.2021.128872
Yang, H., Fu, P., Zhang, G., Zhang, Q., and Li, X. (2021). Adsorption removal of phosphate and fluoride from aqueous solution using oily sludge-based pyrolysis residue. Environ. Process. 8 (4), 1517–1531. doi:10.1007/s40710-021-00539-7
Yang, Z., Chen, H., Wang, J., Yuan, R., Wang, F., and Zhou, B. (2020). Efficient degradation of diisobutyl phthalate in aqueous solution through electro-Fenton process with sacrificial anode. J. Environ. Chem. Eng. 8 (5), 104057. doi:10.1016/j.jece.2020.104057
Yoosefian, M., Ahmadzadeh, S., Aghasi, M., and Dolatabadi, M. (2017). Optimization of electrocoagulation process for efficient removal of ciprofloxacin antibiotic using iron electrode; kinetic and isotherm studies of adsorption. J. Mol. Liq. 225, 544–553. doi:10.1016/j.molliq.2016.11.093
Yu, Z., Xu, C., Yuan, K., Gan, X., Feng, C., Wang, X., et al. (2018). Characterization and adsorption mechanism of ZrO2 mesoporous fibers for health-hazardous fluoride removal. J. Hazard. Mat. 346, 82–92. doi:10.1016/j.jhazmat.2017.12.024
Zhang, J., Tang, L., Tang, W., Zhong, Y., Luo, K., Duan, M., et al. (2020). Removal and recovery of phosphorus from low-strength wastewaters by flow-electrode capacitive deionization. Sep. Purif. Technol. 237, 116322. doi:10.1016/j.seppur.2019.116322
Keywords: capacitive deionization, acid-modified, iron-loaded, phosphorus and fluoride wastewater, simultaneous treatment
Citation: Dai Z, Qin T, Bai C, Wu Z, Gao Y and Qin P (2023) Simultaneous treatment of phosphorus and fluoride wastewater using acid-modified iron-loaded electrode capacitive deionization: Preparation and performance. Front. Environ. Sci. 10:1087231. doi: 10.3389/fenvs.2022.1087231
Received: 02 November 2022; Accepted: 05 December 2022;
Published: 23 January 2023.
Edited by:
Xinjiang Hu, Central South University Forestry and Technology, ChinaReviewed by:
Zhujian Huang, South China Agricultural University, ChinaLing Luo, Sichuan Agricultural University, China
Copyright © 2023 Dai, Qin, Bai, Wu, Gao and Qin. This is an open-access article distributed under the terms of the Creative Commons Attribution License (CC BY). The use, distribution or reproduction in other forums is permitted, provided the original author(s) and the copyright owner(s) are credited and that the original publication in this journal is cited, in accordance with accepted academic practice. No use, distribution or reproduction is permitted which does not comply with these terms.
*Correspondence: Pufeng Qin, qinpufeng@126.com