- 1School of Ecology and Environment, Beijing Technology and Business University, Beijing, China
- 2State Environmental Protection Key Laboratory of Food Chain Pollution Control, Beijing Technology and Business University, Beijing, China
- 3School of Environmental and Municipal Engineering, Lanzhou Jiaotong University, Lanzhou, China
- 4Chinese Research Academy of Environmental Sciences, Beijing, China
- 5School of Civil Engineer, Henan Polytechnic University, Jiaozuo, China
- 6School of Municipal and Environmental Engineering, Shandong Jianzhu University, Jinan, China
- 7CRRC Environmental Science and Technology Co, Ltd., Beijing, China
There is limited information on contaminations of per- and polyfluoroalkyl substances (PFASs) in the water source affected by agricultural activities. The contamination of PFASs was investigated in the sources of agricultural groundwater and nearby surface water from an important vegetable production base supply for Beijing and Tianjin, located in North China. Σ13PFAS concentrations ranged from 0.321 to 8.285 ng/L, with an average concentration of 2.022 ng/L in the groundwater and 19.673 ng/L in the surface water. With the increase in the carbon chain length, the mean concentrations of PFASs in groundwater generally showed a decreasing trend. The dominated congeners of short-chain perfluorobutanesulfonate and perfluorooctanoate acid (PFOA) were detected in all groundwater samples, with mean concentrations of 0.944 and 0.654 ng/L. The difference was that PFOA and perfluorooctanesulfonate (PFOS) were the dominant congeners in nearby surface water, with concentrations of 7.585 and 3.421 ng/L. Thus, the concentrations of PFOA and PFOS in the surface water were about 8.5 times higher than those in the groundwater, indicating that Σ13PFAS concentrations might decrease with the water migration from the overground to the underground. In addition, significant correlations were observed between PFASs and DOC/TN related to agricultural activities, suggesting a certain relationship existed between PFAS conger distributions and organic carbon/nutrients in water. Health risk assessment indicated that the PFAS exposure caused insignificantly immediate harm to residents in the studied area. This survey provided information on the sources, spatial distribution, and potential migration characteristics of PFASs in a typical agricultural area of North China.
Introduction
Per- and polyfluoroalkyl substances (PFASs) have occurred ubiquitously in the environment, causing global concerns due to their long-distance migration, bioaccumulation, and severe toxicity (Cao et al., 2019; Chen et al., 2022). Serious organic pollution in the water body leads to a decrease in the availability of usable fresh water. Additionally, they have some proprietary properties such as low surface energy and high surface-active effect as uniquely hydrophobic and lipophobic characteristics (Tang et al., 2022). However, limited information is available on PFAS contaminations in the sources of groundwater and surface water affected by agricultural activities. The Chinese government issued the Regulations on Groundwater Management (Order No. 748 of the State Council of the people’s Republic of China) at the end of 2021 to further strengthen the monitoring and protection of groundwater, especially in agriculture and rural areas. Besides, the limit values for PFOS and PFOA in drinking water are 40 and 80 ng/L, respectively, according to the Standards for Drinking Water Quality of China (GB5749-2022).
PFASs have been widely applied in various fields involving people’s daily consumer goods, business, and industry, such as surfactants, fire retardants, additives, lubricants, carpet cleaners, paper coatings, and cosmetics (Sims et al., 2022). PFASs have also been widely used in pesticides (herbicides, insecticides, etc.) as auxiliary agents and their packaging (Nascimento et al., 2018). As always, concerns about PFASs are increasing owing to their particular features and wide applications, as well as their global distributions and potential hazards. Therefore, PFOS, perfluorooctanoic acid (PFOA), and related compounds were listed as priority pollutants in the International Stockholm Convention (Chen et al., 2022). In the past decades, short-chain PFASs or no-fluorinated substances have been recommended by global manufacturers instead of short-chain PFASs (Sims et al., 2022; Tang et al., 2022).
A great amount of information has been available presently on the contaminations of PFASs in various environmental media such as soil (Gao et al., 2019; Sammut et al., 2019), water (Zhang et al., 2019; Wang et al., 2022), and air-dust (Liu et al., 2011; Zhang et al., 2020). PFASs have also been detected in biological tissue and blood (Valsecchi et al., 2013; Hu et al., 2023), human blood and urine (Zhang et al., 2013; Szabo et al., 2018), and remote polar regions (Pedersen et al., 2016). However, solving the problem of the exposure pathways of PFASs to the ecosystem is still the focus of all future work. Several studies indicated that drinking water sources could also be a potential exposure source (Gao et al., 2019; Barton et al., 2022). Evidently, there is a potential health risk of drinking water with PFAS contaminations for human bodies (Li et al., 2020; Lu et al., 2022). Notably, groundwater accounts for about one-third of the total water resources and twenty percent of the water supply in China. Groundwater, as one of the main global water resources, has been facing the pollution of various pollutants (i.e., PBDEs, PAHs, nitrobenzene, and chloroethylene). Nevertheless, information on groundwater as a water source slightly contaminated by PFASs, including irrigation and drinking water sources, remains limited. A few existing reports suggested that the globally detected PFASs in groundwater were at various levels ranging from the typically measured levels of ng/L to several μg/L, dominated by perfluorinated carboxylates (PFCAs) with less than or equal to eight carbons and PFOS (Murakami et al., 2009; Bao et al., 2011; Sammut et al., 2019; Wang et al., 2022).
This study was focused on the occurrence of PFASs in groundwater sources from an important vegetable production base supply for Beijing and Tianjin, located in North China. The spatial distribution, compositional profiles, migration and source identification, and human health assessment of PFASs were conducted. This study will help develop effective strategies to monitor PFAS pollution by providing systematic data on the PFASs in this area. To accomplish the above goals, a total of 13 PFASs, including 9 PFCAs (C6–C14) and 4 PFSAs (C4, C6, C8, and C10), were analyzed for groundwater samples collected from 16 private wells and one nearby surface water sample.
Materials and methods
Materials and chemicals
The standards, PFBS, perfluorohexanoic acid (PFHxA), PFHxS, perfluoroheptanoic acid (PFHpA), PFOA, perfluorononanoic acid (PFNA), perfluorodecanoic acid (PFDA), perfluoroundecanoic acid (PFUnDA), perfluorododecanoic acid (PFDoDA), perfluorotridecanoic acid (PFTrDA), and perfluorotetradecanoic acid (PFTeDA) were purchased from Sigma-Aldrich (Steinheim, Germany). PFOS was purchased from Dr. Ehrenstorfer GmbH (Germany). Perfluorodecanesulfonate (PFDS) and the internal standards of sodium perfluoro-1-[1,2,3,4–13C4]octanesulfonate (13C4-PFOS, MPFOS) and perfluoron-[1,2,3,4–13C4]octanoic acid (13C4-PFOA, MPFOA) were obtained from Wellington Laboratories (Guelph, Ontario, Canada).
Sample areas, collection, and preparation
Samples from groundwater and surface water were collected from a typic vegetable production area, an important vegetable production base supply for Beijing and Tianjin in North China in October 2021 (Figure 1). Groundwater samples from shallow aquifers were collected from 16 private wells at depths of 1.47–3.79 m (S1∼S16). The groundwater from these wells is used for vegetable irrigation and drinking water for residents. Meanwhile, one surface water sample was collected from the Beijing–Hangzhou Grand Canal nearby the groundwater wells (S17). Three trip blanks filled with ultrapure water were prepared. Meanwhile, six field spikes (three with target analytes of 50 ng/L, other three with target analytes of 100 ng/L) were also prepared to understand the recovery percentages of target analytes. All actual water samples were collected in duplicate. Formic acid (pH = 3–4) was added to prevent bacterial activity in water. Lastly, samples were stored at 4°C before analysis.
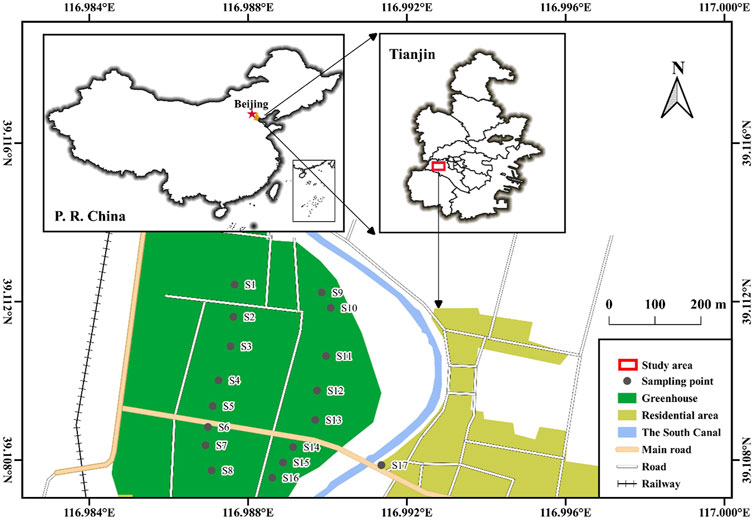
FIGURE 1. Sampling sites in the vegetable production base supply for Beijing and Tianjin, North China.
Sample extraction, cleanup, and instrumental analysis
The extraction, cleanup, and analysis procedures for these water samples were similar to those described previously (Wei et al., 2018). One liter of water samples was filtered through prebaked GF/F glass fiber filters (pore size: 0.7 μm, Whatman, United States) and spiked with 2 ng of 13C4-PFOA and 13C4-PFOS as internal standards. Subsequently, the filtrates were loaded onto a single-use cartridge (Oasis® WAX), preconditioned with 4 ml of 0.1% ammonium hydroxide (in methanol), 4 ml of methanol, and 5 ml of water sequentially. Immediately, the interferences on the cartridge were washed off with 4 ml of 25 mmol/L acetate buffer solution (pH 4) and 10 ml of water. After the removal of the residual water by centrifugation for 30 min at 3,000 rpm, target analytes were eluted with 4 ml of methanol and 4 ml of 0.1% ammonium hydroxide (in methanol) successively. The flow rate was kept at 1 drop during the whole process. Then, eluate with 8 ml was concentrated to 200 µL with a gentle stream of high-purity nitrogen before injection.
Analyses were performed for 13 PFASs using a high-performance liquid chromatography (HPLC, P680 pump, Ultimate 3000 autosampler, Dionex, Sunnyvale, CA, United States) equipped with an electrospray ionization tandem mass spectrometer (ESI/MS/MS, API 3200, Applied Biosystems/MDS SCIEX, United States) operated in negative ion and MRM mode. PFASs were separated on a 120 C18 column (5 μm, 4.6 mm i.d. ×150 mm length; Dionex, Sunnyvale, CA, United States) with an aliquot of 10 µL injection. A 10 min dualistic gradient of 0.1% ammonium hydroxide (in methanol) (A) and 50 mM NH4OAc (B) delivered at a flow rate of 1 ml/min was started with 28% B, ramped to 5% B in 4 min, held for 3 min, and then increased to 28% B gradually until the 10th min.
Quality assurance, quality control, and statistical analysis
Quantification was performed using the internal standard method. The instrumental limits of detection (LODs) varied from 0.015 to 0.047 ng/ml for each kind of PFASs at a signal-to-noise ratio (S/N) of 3 or better than 3. No detectable concentrations of PFASs were detected in trip blanks, field blanks, procedural, as well as in solvent blank in this study. Recoveries of field spikes and spiked blanks ranged from 90.2% to 107.4%, with relative standard deviations (RSD) lower than 3.8%. Average matrix spike recoveries (n = 6) of PFAAs in samples ranged from 80.6% to 114.7%. RSD for each kind of PFAAs were all below 10%.
Statistical analysis was conducted using the SPSS 18.0 (SPSS International, Chicago, United States) and OriginPro 8.0 (OriginLab, Los Angeles, United States) software. Concentrations below LOD were assigned as zero during the calculations. The possible correlations among PFASs in water samples were examined by the Spearman rank correlation analysis (2-tailed). PFASs are considered significantly different if p < 0.05.
Other analyses
A TOC analyzer (MultiN/C2100, Germany) was used to determine DOC after filtrating through glass fiber filters with 0.7 µm (Whatman, United States). TN was measured by the alkali digestion method with potassium persulfate by a UV spectrophotometer.
Results and discussion
Levels and congener profiles
Levels and congener profiles of PFASs in the groundwater were summarized in Figure 1. It was found that Σ13PFASs in groundwater ranged from 0.321 to 8.285 ng/L (mean 2.022 ng/L). Table 1 shows that the PFAS levels in this studied area of Tianjin were close to the levels of the Yuqiao Reservoir in Tianjin found by Cao et al.(2019). However, the found levels were relatively lower than most of those in groundwater samples collected from other areas, such as Hubei, China (2.04 μg/L, nearby a PFSA manufacturing facility) (Gao et al., 2019), Shenzhen, China (dry) (135.48 ng/L) (Li et al., 2020), Melbourne, Australia (74 ng/L) (Szabo et al., 2018), Fuxin, China (485.21 ng/L) (Bao et al., 2011), and Tokyo (51.649 ng/L) (Murakami et al., 2009). This could be explanted that there were neither specific point pollution sources nor heavy industrial or commercial activities observed nearby the groundwater sampling sites. This suggested that the application of pesticides might not be a major pollution source for PFASs or a few PFAS residuals in the environment were brought back by soil, degraded by organisms in the soil, or transported elsewhere by atmosphere deposition. However, future research should be conducted on soil from the upper part, characteristics of water and soil, and other coexisting matters therein to get a comprehensive understanding of the distribution and transformation characteristics of PFASs.
Ten congeners of PFASs were traced in all groundwater samples (Figure 2B), with a detection frequency of 76.9%. Meanwhile, concentrations of congener PFCAs were also detected at a comparable frequency in this study. These chemicals were dominated by PFBS and PFOA, accounting for 46% and 33%, with concentrations of 0–2.382 (mean 1.099) and 0.025–3.942 (mean 0.806) ng/L, respectively. In detail, PFBS concentrations were significantly two magnitudes higher than PFHxS and PFOS. Interestingly, a similar phenomenon was also found in groundwater collected from Fuxi, China (Bao et al., 2011). Log Koc and Kd values of PFASs were significantly correlated with the length of the carbon chain. Therefore, higher short-chain PFASs were detected in the aqueous phase compared to long chains (Chen et al., 2022). With the replacement of long-chain perfluorocarbons, the short carbon chain of PFBS is becoming the main type in environmental media.
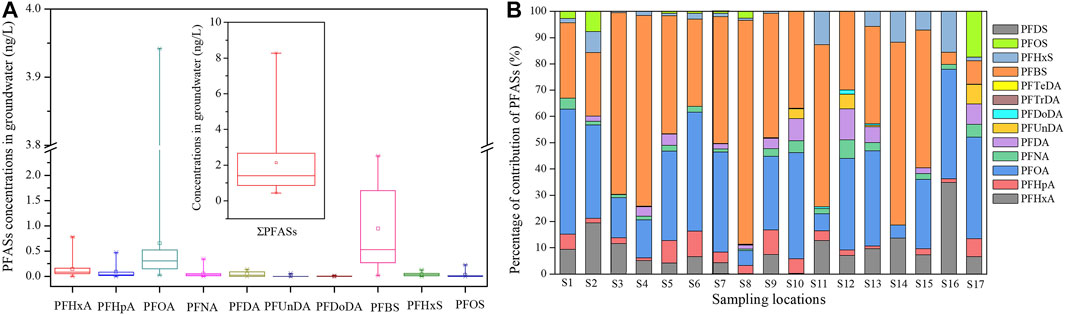
FIGURE 2. (A) Box plot distributions of PFAS congeners concentrations detected in groundwater samples. Within the box, sampling sites were represented by numbers ranging from 1 to 16. (B) Composition profiles of PFASs in groundwater samples (S1–S16) and surface water (S17).
PFAS migration and source identification
Figure 2 shows that mean concentrations of PFASs in groundwater decreased with the increase in carbon chain length, consistent with the conclusion that short-chain congeners were easier to reside in groundwater (Boiteux et al., 2012). Additionally, high water–soil ratios verified the relatively high possibility of transfer from soil to groundwater (Lindstrom et al., 2011). Similarly, our observation was also embodied indirectly by the limited removal of short-chain PFCAs by soils (Washington et al., 2010; Bao et al., 2011). It has also been noted that short-chain PFASs with a carboxylate head group presented a decreased sorption trend (Higgins and Luthy, 2006; Chen et al., 2022). Furthermore, it was also correlated with the solubility of PFASs, which increased with the decrease in carbon chain length, suggesting that the migration ability of short-chain PFASs as the superincumbent movement of the water body would be enhanced. Moreover, the point of precursor degradation also gave a reasonable explanation for the lack of detection of long-chain PFTrDA and PFTeDA in the present study.
Figure 3A shows that one corresponding surface water sample was collected from the Beijing–Hangzhou Grand Canal. It was no surprise that PFOA and PFOS in surface water were typical PFASs, up to 7.585 and 3.421 ng/L, respectively. Differently, concentrations of ΣPFASs in surface water samples were about 8.5 times higher than those in groundwater. Additionally, concentrations of every kind of PFASs detected in surface water were higher than those in the groundwater. PFAS contaminations in surface water might be influenced by industrial activities nearby.
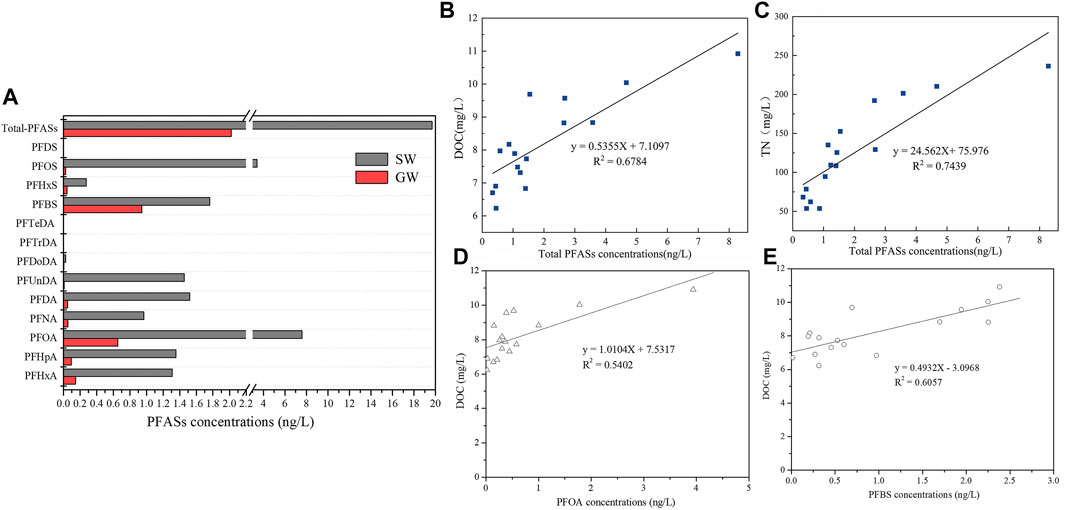
FIGURE 3. PFAS source identification. (A) Comparison of total PFAS concentrations and mean concentrations of each kind of PFASs in groundwater (GW) and surface water (SW). (B–E) Relationships between total PFAS concentrations and DOC/TN contents.
Correlations among various PFAS congers and total PFAS contents between groundwater and surface water were assessed, as shown in Supplementary Table S1 and Supplementary Figure S1. At different levels, the PFAS compounds exhibited significant correlations. In particular, there were strong correlations between PFHpA and PFOA, PFHpA and PFNA, PFHpA and PFBS, PFOA and PFNA, as well as PFDA and PFUnDA with correlation coefficients of 0.831, 0.828, 0.792, 0.917, and 0.806, respectively (p < 0.01). PFAS contents between groundwater and surface water showed correlation coefficients of 0.814 (p < 0.05). Statistically significant positive correlations suggested that these chemicals might be originated from the same or similar pollution sources.
Generally, environmental sources of PFASs are difficult to identify as PFASs used in a wide variety of fields are distributed throughout the globe and undergo complicated transformation pathways (Prevedouros et al., 2006; Chen et al., 2022). In particular, in the case that we do not know the specific activity locally, it will be hard to address source identification, except that the pollution sources are definite. Given this situation that no point pollution sources existed near the sampling sites in the present study, the highest PFBS concentrations detected may be attributed to the widespread daily usage of PFASs and then percolated through the soil after the degradation of other PFSAs, such as PFHxS and PFOS (Lindstrom et al., 2011; Feng et al., 2015).
To further trace the possible pollution sources, a correlation analysis was conducted between concentrations of Σ13PFASs and PFOA/PFBS and DOC/TN (Figures B∼E). Positive correlations were observed between Σ13PFASs/PFOA/PFBS and DOC/TN contents, conforming well to a linear equation with R2 = 0.5402–0.6784 (DOC) and R2 = 0.7439 (TN), respectively, by regression analysis. These results indicated that the distribution and levels of PFASs in water body might be related to dissolved organic carbon, nutrients, and inorganic salts, which came from the exudation of agricultural fertilizer contaminated by PFASs (Sinclair et al., 2006; Qi et al., 2016) and domestic sewage influenced by PFAS-containing products (Xie et al., 2013). The performance was affected by dissolved organic matter in groundwater (Li et al., 2022). Besides, it might be affected by human activities such as organic fertilizer, plastic film, household garbage, pesticides, and pesticides, which were used in this vegetable production base. However, the recognized interaction mechanism between organic matter/nitrogen and PFAS in water remains to be further studied.
Health risk assessment
The Standards for Drinking Water Quality of China (GB5749-2022) established for PFASs are limited to PFOS and PFOA, with 40 and 80 ng/L, respectively. Obviously, the concentration of PFASs was lower than the national standard in the studied groundwater and surface water. Notably, researchers had brought up a new approach by providing the provisional health-related indication values (HRIVs) to assess the health risks of lifelong exposure to short-chain PFASs in drinking water, based on the estimated half-lives for the elimination of PFASs from the human bodies (Bao et al., 2011). Accordingly, HRIVs of the short-chain PFASs detected in the present study were separately set as follows: PFBS 2.96, PFHxA 1.03, PFHpA 0.28, and PFHxS 0.31 μg/L. However, the general precautionary value (PVg) for all PFASs in drinking water was set to 0.1 μg/L in the long term (Wilhelm et al., 2010). The total PFAS concentrations, the total PFOA and PFOS concentrations, and each conger of PFAS concentration were all far lower than the lowest health-based guidance values reported accordingly, indicating that exposure to PFASs causes insignificantly immediate harm to residents in this area of North China.
Conclusion
This study showed that the short carbon chain of PFBS was the main type of PFASs. The mean concentrations of PFASs in the groundwater decreased with the increase in the carbon chain length. There was an insignificant potential risk of PFAS exposure in the studied groundwater source for vegetable planting and resident drinking. However, the detection of the dominant PFASs at relatively higher levels should be especially focused on to prevent the local residents from health hazards due to their longtime exposure to PFASs in drinking water. Further work should be done to study the PFAS contaminations in groundwater on a large scale in China. Meanwhile, related surface water and soil should be considered to obtain a comprehensive understanding of PFAS levels, characteristics, and sources. Therefore, it is meaningful to connect the occurrence and distribution of PFASs with the site characterization parameters such as organic carbon, nutrients, inorganic constituents, and the coexisting organic contaminants.
Data availability statement
The raw data supporting the conclusions of this article will be made available by the authors without undue reservation.
Author contributions
XW: data curation, investigation, writing—original draft; HZ: investigation and data curation; XH: investigation; ZY: project administration; JL: investigation and writing—original draft; HZ: data curation; DY: investigation and writing—original draft; TL: investigation; BL and WZ: project administration and resources.
Funding
This study was supported by the State Key Laboratory for Free Exploration Project (2014-GOT-019-N-26).
Conflict of interest
TL was employed by CRRC Environmental Science and Technology Co, Ltd.
The remaining authors declare that the research was conducted in the absence of any commercial or financial relationships that could be construed as a potential conflict of interest.
Publisher’s note
All claims expressed in this article are solely those of the authors and do not necessarily represent those of their affiliated organizations or those of the publisher, the editors, and the reviewers. Any product that may be evaluated in this article, or claim that may be made by its manufacturer, is not guaranteed or endorsed by the publisher.
Supplementary material
The Supplementary Material for this article can be found online at: https://www.frontiersin.org/articles/10.3389/fenvs.2022.1071134/full#supplementary-material
References
Bao, J., Liu, W., Liu, L., Jin, Y., Dai, J., Ran, X., et al. (2011). Perfluorinated compounds in the environment and the blood of residents living near fluorochemical plants in Fuxin, China. Environ. Sci. Technol. 45, 8075–8080. doi:10.1021/es102610x
Barton, K.-E., Zell-Baran, L.-M., DeWitt, J.-C., Brindley, S., McDonough, C.-A., Higgins, C.-P., et al. (2022). Cross-sectional associations between serum PFASs and inflammatory biomarkers in a population exposed to AFFF-contaminated drinking water. Int. J. Hyg. Environ. Health 240, 113905. doi:10.1016/j.ijheh.2021.113905
Boiteux, V., Dauchy, X., Rosin, C., and Munoz, J.-F. (2012). National screening study on 10 perfluorinated compounds in raw and treated tap water in France. Arch. Environ. Contam. Toxicol. 63, 1–12. doi:10.1007/s00244-012-9754-7
Cao, X., Wang, C., Lu, Y., Zhang, M., Khan, K., Song, S., et al. (2019). Occurrence, sources and health risk of polyfluoroalkyl substances (PFASs) in soil, water and sediment from a drinking water source area. Ecotoxicol. Environ. Saf. 174, 208–217. doi:10.1016/j.ecoenv.2019.02.058
Chen, Y., Wei, L.-J., Luo, W., Jiang, N., Shi, Y.-L., Zhao, P., et al. (2022). Occurrence, spatial distribution, and sources of PFASs in the water and sediment from lakes in the Tibetan Plateau. J. Hazard. Mat. 13, 130170. doi:10.1016/j.jhazmat.2022.130170
Feng, X., Matt, F.-S., Thomas, R.-H., and John, S.-G. (2015). Perfluorooctane sulfonate (PFOS) and perfluorooctanoate (PFOA) in soils and groundwater of a U.S. metropolitan area: Migration and implications for human exposure. Water Res. 72, 64–74. doi:10.1016/j.watres.2014.09.052
Gao, Y., Liang, Y., Gao, K., Wang, Y., Wang, C., Fu, J., et al. (2019). Levels, spatial distribution and isomer profiles of perfluoroalkyl acids in soil, groundwater and tap water around a manufactory in China. Chemosphere 227, 305–314. doi:10.1016/j.chemosphere.2019.04.027
Higgins, C.-P., and Luthy, R.-G. (2006). Sorption of perfluorinated surfactants on sediments. Environ. Sci. Technol. 40, 7251–7256. doi:10.1021/es061000n
Hu, W., Zhang, M.-Y., Liu, L.-Y., Zhang, Z.-F., and Guo, Y. (2023). Perfluoroalkyl and polyfluoroalkyl substances (PFASs) crossing the blood-cerebrospinal fluid barrier: Their occurrence in human cerebrospinal fluid. J. Hazard. Mat. 442, 130003. doi:10.1016/j.jhazmat.2022.130003
Li, B.-B., Hu, L.-X., Yang, Y.-Y., Wang, T.-T., Liu, C., and Ying, G.-G. (2020). Contamination profiles and health risks of PFASs in groundwater of the Maozhou River basin. Environ. Pollut. 260, 113996. doi:10.1016/j.envpol.2020.113996
Li, J., Peng, G., Xu, X., Liang, E., Sun, W., Chen, Q., et al. (2022). Per- and polyfluoroalkyl substances (PFASs) in groundwater from a contaminated site in the North China Plain: Occurrence, source apportionment, and health risk assessment. Chemosphere 302, 134873. doi:10.1016/j.chemosphere.2022.134873
Lindstrom, A.-B., Strynar, M.-J., Delinsky, A.- D., Nakayama, S.-F., McMillan, L., Libelo, E.-L., et al. (2011). Application of WWTP biosolids and resulting perfluorinated compound contamination of surface and well water in Decatur, Alabama, USA. Environ. Sci. Technol. 45, 8015–8021. doi:10.1021/es1039425
Liu, W., Chen, S., Harada, K.-H., and Koizumi, A. (2011). Analysis of perfluoroalkyl carboxylates in vacuum cleaner dust samples in Japan. Chemosphere 85, 1734–1741. doi:10.1016/j.chemosphere.2011.09.024
Lu, G., Shao, P., Zheng, Y., Yang, Y., and Gai, N. (2022). Perfluoroalkyl substances (PFASs) in rivers and drinking waters from qingdao, China. Int. J. Environ. Res. Public Health 19, 5722. doi:10.3390/ijerph19095722
Murakami, M., Kuroda, K., Sato, N., Fukushi, T., Takizawa, S., and Takada, H. (2009). Groundwater pollution by perfluorinated surfactants in Tokyo. Environ. Sci. Technol. 43, 3480–3486. doi:10.1021/es803556w
Nascimento, R.-A., Nunoo, D.-B.-O., Bizkarguenaga, E., Schultes, L., Zabaleta, I., Benskin, J.-P., et al. (2018). Sulfluramid use in Brazilian agriculture: A source of per- and polyfluoroalkyl substances (PFASs) to the environment. Environ. Pollut. 242, 1436–1443. doi:10.1016/j.envpol.2018.07.122
Pedersen, K.-E., Letcher, R.-J., Sonne, C., Dietz, R., and Styrishave, B. (2016). Per- and polyfluoroalkyl substances (PFASs) – new endocrine disruptors in polar bears (Ursus maritimus)? Environ. Int. 96, 180–189. doi:10.1016/j.envint.2016.07.015
Prevedouros, K., Cousins, I.-T., Buck, R.-C., and Korzeniowski, S.-H. (2006). Sources, fate and transport of perfluorocarboxylates. Environ. Sci. Technol. 40, 32–44. doi:10.1021/es0512475
Qi, Y.-J., Huo, S.-L., Hu, S.-B., Xi, B.-D., Su, J., and Tang, Z.-W. (2016). Identification, characterization, and human health risk assessment of perfluorinated compounds in groundwater from a suburb of Tianjin, China. Environ. Earth Sci. 75, 432. doi:10.1007/s12665-016-5415-x
Sammut, G., Sinagra, E., Sapiano, M., Helmus, R., and de Voogt, P. (2019). Perfluoroalkyl substances in the Maltese environment – (II) sediments, soils and groundwater. Sci. Total Environ. 682, 180–189. doi:10.1016/j.scitotenv.2019.04.403
Sims, J.-L., Stroski, K.-M., Kim, S., Killeen, G., Ehalt, R., Simcik, M.-F., et al. (2022). Global occurrence and probabilistic environmental health hazard assessment of per- and polyfluoroalkyl substances (PFASs) in groundwater and surface waters. Sci. Total Environ. 816, 151535. doi:10.1016/j.scitotenv.2021.151535
Sinclair, E., Mayack, D.-T., Roblee, K., Yamashita, N., and Kannan, K. (2006). Occurrence of perfluoroalkyl surfactants in water, fish, and birds from New York State. Arch. Environ. Contam. Toxicol. 50, 398–410. doi:10.1007/s00244-005-1188-z
Szabo, D., Coggan, T.-L., Robson, T.-C., Currell, M., and Clarke, B.-O. (2018). Investigating recycled water use as a diffuse source of per- and polyfluoroalkyl substances (PFASs) to groundwater in Melbourne, Australia. Sci. Total Environ. 644, 1409–1417. doi:10.1016/j.scitotenv.2018.07.048
Tang, Z., Song, X., Xu, M., Yao, J., Ali, M., Wang, Q., et al. (2022). Effects of co-occurrence of PFASs and chlorinated aliphatic hydrocarbons on microbial communities in groundwater: A field study. J. Hazard. Mat. 435, 128969. doi:10.1016/j.jhazmat.2022.128969
Valsecchi, S., Rusconi, M., and Polesello, S. (2013). Determination of perfluorinated compounds in aquatic organisms: A review. Anal. Bioanal. Chem. 405, 143–157. doi:10.1007/s00216-012-6492-7
Wang, Q., Song, X., Wei, C., Ding, D., Tang, Z., Tu, X., et al. (2022). Distribution, source identification and health risk assessment of PFASs in groundwater from Jiangxi Province, China. Chemosphere 291, 132946. doi:10.1016/j.chemosphere.2021.132946
Washington, J.-W., Yoo, H., Ellington, J.-J., Jenkins, T.-M., and Libelo, E.-L. (2010). Concentrations, distribution, and persistence of perfluoroalkylates in sludge-applied soils near Decatur, Alabama, USA. Environ. Sci. Technol. 44, 8390–8396. doi:10.1021/es1003846
Wei, C., Wang, Q., Song, X., Chen, X., Fan, R., Ding, D., et al. (2018). Distribution, source identification and health risk assessment of PFASs and two PFOS alternatives in groundwater from non-industrial areas. Ecotoxicol. Environ. Saf. 152, 141–150. doi:10.1016/j.ecoenv.2018.01.039
Wilhelm, M., Bergmann, S., and Dieter, H.-H. (2010). Occurrence of perfluorinated compounds (PFCs) in drinking water of North Rhine-Westphalia, Germany and new approach to assess drinking water contamination by shorter-chained C4-C7 PFCs. Int. J. Hyg. Environ. Health 213, 224–232. doi:10.1016/j.ijheh.2010.05.004
Xie, S., Lu, Y., Wang, T., Liu, S., Jones, K., and Sweetman, A. (2013). Estimation of PFOS emission from domestic sources in the eastern coastal region of China. Environ. Int. 59, 336–343. doi:10.1016/j.envint.2013.06.015
Zafeiraki, E., Costopoulou, D., Vassiliadou, I., Leondiadis, L., Dassenakis, E., Traag, W., et al. (2015). Determination of perfluoroalkylated substances (PFASs) in drinking water from The Netherlands and Greece. Food Addit. Contam. Part A Chem. Anal. Control Expo. Risk Assess. 32, 2048–2057. doi:10.1080/19440049.2015.1086823
Zhang, B., He, Y., Huang, Y., Hong, D., Yao, Y., Wang, L., et al. (2020). Novel and legacy poly- and perfluoroalkyl substances (PFASs) in indoor dust from urban, industrial, and e-waste dismantling areas: The emergence of PFAS alternatives in China. Environ. Pollut. 263, 114461. doi:10.1016/j.envpol.2020.114461
Zhang, D.-Q., Zhang, W.-L., and Liang, Y.-N. (2019). Adsorption of perfluoroalkyl and polyfluoroalkyl substances (PFASs) from aqueous solution - a review. Sci. Total Environ. 694, 133606. doi:10.1016/j.scitotenv.2019.133606
Keywords: perfluoroalkyl and polyfluoroalkyl substances, water source, groundwater, agricultural activity, health risk assessment
Citation: Wang X, Zhang H, He X, Liu J, Yao Z, Zhao H, Yu D, Liu B, Liu T and Zhao W (2023) Contamination of per- and polyfluoroalkyl substances in the water source from a typical agricultural area in North China. Front. Environ. Sci. 10:1071134. doi: 10.3389/fenvs.2022.1071134
Received: 15 October 2022; Accepted: 01 December 2022;
Published: 23 January 2023.
Edited by:
Shihai Deng, Xi’an Jiaotong University, ChinaReviewed by:
Binghan Xie, Harbin Institute of Technology, Weihai, ChinaZhifeng Hu, Beijing Academy of Science and Technology, China
Copyright © 2023 Wang, Zhang, He, Liu, Yao, Zhao, Yu, Liu, Liu and Zhao. This is an open-access article distributed under the terms of the Creative Commons Attribution License (CC BY). The use, distribution or reproduction in other forums is permitted, provided the original author(s) and the copyright owner(s) are credited and that the original publication in this journal is cited, in accordance with accepted academic practice. No use, distribution or reproduction is permitted which does not comply with these terms.
*Correspondence: Zhiliang Yao, eWFvemhsQHRoLmJ0YnUuZWR1LmNu