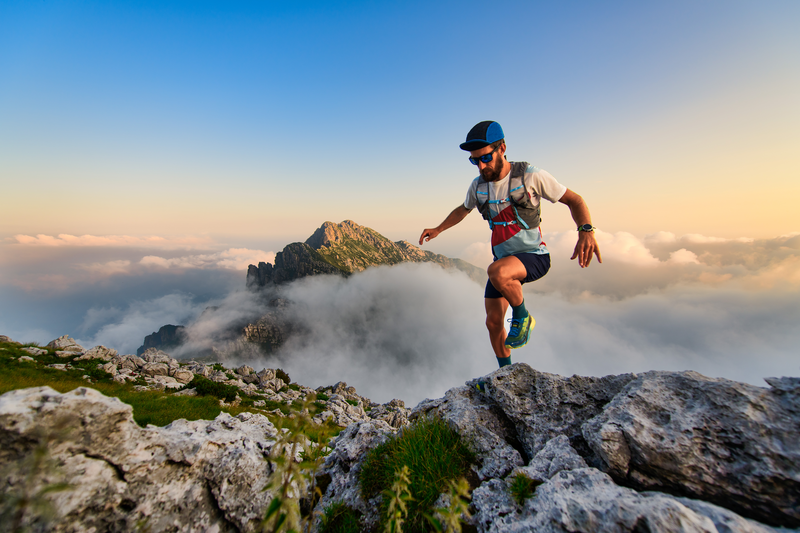
94% of researchers rate our articles as excellent or good
Learn more about the work of our research integrity team to safeguard the quality of each article we publish.
Find out more
ORIGINAL RESEARCH article
Front. Environ. Sci. , 20 January 2023
Sec. Water and Wastewater Management
Volume 10 - 2022 | https://doi.org/10.3389/fenvs.2022.918259
As a commonly used material in bioretention substrates, natural zeolite (NZ) provides decent adsorption capacity for cation pollutants and heavy metals, but limited ability to remove anion pollutants. Hexadecyltrimethylammonium bromide (HDTMA)-modified zeolite (MZ) was used as the bioretention substrate material. The performance of the media including runoff reduction, nitrate nitrogen (NO3−-N) removal, ammonium nitrogen (NH4+-N) removal, and total phosphorus (TP) removal was assessed by the column experiment. The effects of different levels of modification, ratio of zeolite in the substrate, and rainfall intensity on media performance were investigated. The results indicate that HDTMA-modified zeolite significantly improves the NO3−-N (up to 38.2 times of NZ) and TP (up to17.5 times of NZ) removal rate of media and slightly increases the NH4+-N (up to 1.5 times of NZ) purification performance of the substrate. Compared with the media with NZ, decline on both runoff volume reduction (maximum decline up to 32.9%) and flow rate reduction (maximum decline up to 29.9%) of the media with MZ were observed. Based on multiple regression analysis, quantitative relationship models between influencing factors and response variables were established (R2 > 0.793), the level of the effect of influencing factors on response variables was investigated, and the interactions between influencing factors were explored. The main effect analysis found that the degree of modification affects NO3−-N and TP removal rate of the substrate the most, and when the amount of HDTMA molecules loaded on the zeolite surface exceeds 0.09meq/g, the modification can no longer improve NO3−-N removal efficiency.
The increasing quantity and deteriorating quality of urban stormwater come with the rapid urbanization process (Fletcher et al., 2014; Chen et al., 2017), mainly because of the increasing impervious areas in the urban area (Li et al., 2018). Impervious areas such as roads and buildings not only block the infiltration process of rainwater but also accumulate various pollutants such as nutrients (nitrogen and phosphorus), organic matters, and heavy metal. The blocked infiltration process could increase flood risk, and accumulate pollutants would be washed into the receiving water bodies by stormwater runoff, which will cause water contamination (Walsh et al., 2012). Among these pollutants, nitrogen and phosphorus play significant roles in eutrophication, and relevant studies indicate that the majority of the nitrogen and phosphorus in stormwater runoff come from developed areas (Line and White, 2007; Davidson et al., 2010; Gao et al., 2019). A series of urban stormwater control measures have been adopted in response to these issues, such as bioretention, stormwater wetland, pervious pavement, and sedimentation ponds (Davis et al., 2009; Hatt et al., 2009; Baek et al., 2015).
Bioretention is an effective stormwater management technology that has been widely used to mitigate urban flooding risk and improve stormwater quality (Davis et al., 2009; DeBusk et al., 2011; Goh et al., 2019). The primary function component of bioretention is the substrate, which is commonly composed of topsoil (a general term for the upper or outmost layer of soil), compost, and aggregate amendments such as zeolite, biochar, and pumice (Jia et al., 2015; Liu and Fassman-Beck, 2016; Liu J. et al., 2017). The purpose of adding aggregate amendments in bioretention media is to adjust its saturated hydraulic conductivity (Ks) to address stormwater management objectives (Liu and Fassman-Beck, 2016). In addition to regulating Ks, different amendments were used in bioretention media to improve its water purification ability. Studies showed that amendments such as blast furnace slag, biochar, water treatment residuals (WTRs), and iron filings could improve the total phosphorus (TP) and total nitrogen (TN) removal ability of the bioretention media (Li et al., 2016; Chang et al., 2019; Poor et al., 2019; Zhou et al., 2022).
Zeolite is widely used in water treatment and environmental remediation as an adsorber or filler, due to its porous structure and cation exchange ability (Misaelides, 2011; Reddy et al., 2014). It is also a common material used for bioretention substrate (Jiang et al., 2019). Studies show that using zeolite as bioretention substrate media could achieve satisfying results in stormwater purification performance. Sari et al. (2019) investigated the treatment performance of bioretention media using quartz sand and zeolite, the results indicate that adding zeolite in substrate could significantly improve the removal efficiency of ammonium nitrogen (NH4+-N), Pb, and Zn. Wang et al. (2021) assessed three different mixtures of bioretention media, the media contain zeolite demonstrated better performance in the removal of the total nitrogen (TN) and NH4+-N than other medias without zeolite; however, results also pointed out that the media with zeolite has limited ability for the removal of total phosphorus (TP). Other researches also reported that substrate media using zeolite exhibited satisfactory effect on removing TN, NH4+-N, and heavy metals, but showed poor performance on TP and nitrate nitrogen (NO3−-N) removal (Jiang et al., 2019; N. Seelsaen et al., 2006; Wang et al., 2017).
Zeolite is a type of aluminosilicate mineral, due to its unique structure and chemical composition, zeolite possess negative charge and cation exchange properties, which is the reason it has limited effect on anionic pollutants (such as phosphate and NO3−-N) removal, but shows high adsorption capacity for cationic pollutants such as NH4+-N (Ejhieh and Masoudipour, 2010; Misaelides, 2011; Sharafzadeh and Nezamzadeh-Ejhieh, 2015). Because of the unique porous structure, high cation exchange capacity and high adsorption ability, zeolite has various applications, it has been used for the removal of Pb (II), the removal of Cu (II), and the modification of carbon paste electrode (Heidari-Chaleshtori and Nezamzadeh-Ejhieh, 2015; Alidusty and Nezamzadeh-Ejhieh, 2016; Tamiji and Nezamzadeh-Ejhieh, 2019; Nasiri-Ardali and Nezamzadeh-Ejhieh, 2020). Moreover, the cation exchange ability of zeolite also makes it available for surface modification by cationic surfactant, which could significantly enhance its capacity to adsorb anionic pollutants (Bowman, 2003; Shi et al., 2009; Nezamzadeh-Ejhieh and Mirzaeyan, 2013; Nezamzadeh-Ejhieh and Tavakoli-Ghinani, 2014). Studies indicate that when surfactant concentrations are higher than the critical micelle concentration (CMC), a bilayer of surfactant molecules will form on zeolite surface. The double layer of surfactant molecules reverses the charge of zeolite from negative to positive, which enable modified zeolite with the ability to adsorb anions, cations, and non-polar organic species simultaneously (Nezamzadeh-Ejhieh and Afshari, 2012; Nezamzadeh-Ejhieh and Shahanshahi, 2013; Saadat and Nezamzadeh-Ejhieh, 2016; Mahdavi and Nezamzadeh-Ejhieh, 2017). Naghash and Nezamzadeh-Ejhieh (2015) compared the removal efficiency of natural zeolite, hexadecyltrimethylammonium bromide (HDTMA)-modified zeolite, and hexadecylpridinium bromide (HDP)-modified zeolite, results show that under the same condition, phosphate adsorption capacity of zeolite was significantly improved by modifications, form 16 mmol/kg (natural zeolite) to 376 mmol/kg (HDP-modified zeolite) and 675 mmol/kg (HDTMA-modified zeolite). In the area of water treatment and environmental remediation, researches have provided convincing evidence and satisfactory results for using modified zeolite (MZ) to enhance the treatment performance of NO3−-N, phosphate, organic contaminants, and Pb (Yusof and Malek, 2009; Schick et al., 2011; Zhan et al., 2011; Anari-Anaraki and Nezamzadeh-Ejhieh, 2015; Tao et al., 2015; Liu et al., 2016). Additionally, surfactant-modified zeolite can be used to make nitrate selective electrode, and it shows satisfactory ability for quantification of nitrate in water and industrial products (Nezamzadeh-Ejhieh and Nematollahi, 2011; Nosuhi and Nezamzadeh-Ejhieh, 2018).
In addition to enhanced pollutant removal efficiency, the surface modification process would also change hydrophilicity and hydrophobicity properties of zeolite (Nezamzadeh-Ejhieh and Raja, 2013). Hydrophilicity/Hydrophobicity of the material is the key factor which determines its interactions with water (Gray et al., 2014; Kameyama et al., 2019). Research suggests that hydrophobicity play fundamental roles in water movement and retention of material, and consequently affects its hydraulic properties (Edeh and Mašek, 2022). Hydraulic properties of substrate material were identified as important factors that affect the hydrological performance of bioretention (Liu and Fassman-Beck, 2016; Liu and Fassman-Beck, 2017a). Studies suggest that Ks and water retention characteristic (WRC), as the basic hydraulic properties of bioretention substrate, would affect overall hydrologic performance of the media (Lucas and Greenway, 2011; Paus et al., 2014; Fassman-Beck et al., 2015; Liu and Fassman-Beck, 2018, 2016). Moreover, different Ks indicate different hydraulic conductivity (K) of the media, and K can be predicted from measured data of the WRC and Ks (van Genuchten, 1980; Palla et al., 2009; Liu and Fassman-Beck, 2018). The previous research confirms that HDTMA modification would alter properties such as Ks and WRC of zeolite (Qin et al., 2022). Changes in WRC and Ks of the media will alter the hydrological performance of substrate, (Liu and Fassman-Beck, 2017a;Liu and Fassman-Beck, 2017b), therefore, more information regarding the hydrological performance of bioretention media with MZ is needed.
In the water treatment and environmental remediation area, HDTMA-modified zeolite is widespread used and has been considered to be safe for the environment, it also has been proved to be effective at TP, NO3−-N and NH4+-N removal (Li et al., 1998; Bowman, 2003; Schick et al., 2010). However, few researches explore the possibility of using HDTMA-modified zeolite as bioretention media. As mentioned earlier, HDTMA modification would not only improves pollutant adsorption capacity of zeolite, but also affect its hydraulic properties, which would impact hydrological performance of bioretention media with MZ. In this context, this paper investigated the runoff regulation ability and the NO3−N, NH4+-N, and TP removal performance of bioretention media with HDTMA-modified zeolite. The effect of different influencing factors (level of modification, zeolite ratio, and rainfall intensity) on hydrological and pollutant removal performance of substrate was studied. The level of modification and percentage of zeolite are substrate factors that directly affect media performance, rainfall intensity was included as environmental factor, to evaluate the substrate performance of stormwater management under different rainfall conditions. Through multiple regression analysis, the correlations between influencing factors and stormwater regulation performance (runoff reduction, NO3−-N, NH4+-N, and TP removal rate) were established, and the interaction between influencing factors was studied.
Natural zeolite (NZ), specifically, natural clinoptilolite (a type of zeolite) was used in this study. The clinoptilolites was sourced from Jinyun, Zhejiang, China, graded for 2–8 mm. The chemical compositions of NZ were 68.07% SiO2, 12.12% Al2O3, 2.37% CaO, 2.06% Na2O, and 1.01% Fe2O3. Peat soil and quartz sand were purchased from local horticulture company in Shanghai, China. Hexadecyltrimethylammonium bromide (HDTMA) was analytical grade reagents from Fisher Scientific, other chemical reagents involved in the experiments were analytical grade reagents purchased from Sinopharm.
Modified zeolite (MZ) in two levels of modification was prepared, 10% modified zeolite (ZHD10) and 50% modified zeolite (ZDH50). The level of modification was determined based on the percentage of the cation exchange capacity (CEC) of NZ, and the mass of HDTMA used for the modification was calculated by the equation suggested by Zohra et al. (2008):
where f is the portion of CEC satisfied by cation surfactant (dimensionless), Mcation is the mass of surfactant required for desired portion of CEC (g), CEC is the cation exchange capacity of zeolite (mol/g), MZ is the mass of NZ (g), GMWcation is the Gram molecular weight of HDTMA cation (g/mol), and X is the moles of charge per equivalent, which is 1 mol/equiv. for the HDTMA.
The CEC of NZ was calculated using the method introduced by Endo et al. (2013). The CEC value determined for the NZ used in the research is 164.75 cmol/kg. Based on the CEC value of NZ, required mass of HDTMA for the modification was calculated, which are 0.06g and 0.3 g for every 1 g of ZHD10% and ZHD50%.
NZ was washed by deionized water vigorously and dried in an oven at 105°C for 12 h. Washed NZ and deionized water in a solid-liquid ratio of 1:10 (w/w) were put into a chemical mixing tank, and the amount of HDTMA required for the modification was added, the blender of the mixing tank was set at 180 rpm. The mixing tank was placed in a water bath heater with a constant temperature of 40°C for 24 h. After 24 h, the zeolite was washed with deionized water until AgNO3 test indicate no bromide ions in the supernatant solution, then zeolite was dried in an oven at 105°C for 12 h.
After the modification, the Fourier transform infrared (FTIR) spectra of NZ and MZ were obtained in the region of 4,000 to 400 cm−1 by a Thermo Scientific Nicolet 6700 spectrophotometer. FTIR data were used to confirm whether the modification is successful. Thermogravimetric analysis (TGA) was conducted by a thermogravimetric analyzer, PerkinElmer, Pyris1. Based on the TGA data, the amount of surfactant loaded on zeolite surface were calculated using the thermogravimetric method (volatilization method), which are 0.05meq/g for ZHD10 and 0.09meq/g for ZHD50. Detailed discussion on the effect of HDTMA modification on zeolite characteristics can be found in the previous study (Qin et al., 2022).
Normally, a bioretention substrate is a mixture of various materials; the main component is soil (promotes infiltration and evapotranspiration of rainwater runoff), and other materials such as zeolite, coal slag, biochar, and perlite are added to improve the hydraulic and runoff treatment performance of the substrate (Davis et al., 2009; Brown and Hunt, 2011; Goh et al., 2019). In this research, the main purpose is to investigate the effect of HDTMA-modified zeolite on the runoff regulation performance of the substrate, to explore the possibility of using HDTMA-modified zeolite as bioretention substrate material, therefore, the substrate media used in this study were consist of only peat soil and zeolite. Peat soil is a general term for the soil formed by the natural accumulation of partially decayed biomass (Stracher et al., 2015). It is a dual-porosity material, makes it has relatively high Ks and high water retention ability (Hyväluoma et al., 2020).
A total of six substrate media were prepared by mixing NZ and MZ with peat soil in different ratio by percent volume. Detailed percentages of zeolite and peat soil were (1) 25% zeolite +75% peat soil (25% Z+75% S); (2) 50% zeolite +50% peat soil (50% Z+50% S); (3) 25% ZHD10 + 75% peat soil (25% ZHD10 + 75% S); (4) 50% ZHD10 + 50% peat soil (50% ZHD10 + 50% S); (5) 25% ZHD50 + 75% peat soil (25% ZHD50 + 75% S); and (6) 50% ZHD50 + 50% peat soil (50% ZHD50 + 50% S).
The hydrological and pollutants removal performance of different substrate was evaluated by laboratory column experiments. In filed application, bioretention system includes plants, but plants were not included in this study. Several studies indicate that plants have notable influence on bioretention media’s hydrological and pollutants removal performance (Lucas, 2010; Le Coustumer et al., 2012; Hua et al., 2017). This study aims to investigate the relationship between media factors and substrate performance under different rainfall conditions, therefore, no plants were included to limit the influence from other factors. As shown in Figure 1, a polymethyl methacrylate column with 20 cm inner diameters and 130 cm height was packed with three layers: (1) 10 cm of quartz sand (graded for 5–10 mm) as the drainage layer at the bottom; (2) 95 cm of the tested media at the middle; and (3) 2–3 cm layer of quartz sand (graded for 5–10 mm) at the top to prevent the media scoured by inflow. It should be noted that this is not a typical bioretention substrate structure. Such experimental setup is to explore the possibility of HDTMA-modified zeolite as bioretention media, not to evaluate its performance as the actual bioretention media.
NZ, MZ, and peat soil were dried in an oven for 24 h at 105°C before mixing, then peat soil and zeolite were blended in a drum mixer. While mixing, 100 g water was added for every 1 L media to prevent the possible hydrophobicity. Before packing the material into the column, a thin layer of Dow Corning high vacuum grease was applied in the interior of the column to reduce the water flow along the side of the column. The high vacuum grease is a silicone metalloid complex, which is insoluble in water, chemically resistant, and maintains its consistency over a wide temperature range (−40–204°C). Therefore, the application of high vacuum grease would not affect the chemical analysis results. When every 10 cm material in depth was filled, a 4.5 kg proctor hammer was dropped six times from 45 cm height to achieve compaction, which is a compaction procedure suggested by Liu and Fassman-Beck (2016).
Inflow was steadily supply by a peristaltic pump (Longer, BT600-2J) through silica gel tube, outlet of the tube was clamped by tubing clamp, from that end, about 30 cm of the tube was punctured with a needle (1.6 mm inner diameters) in every 1 cm, and put on the top layer of quartz sand in spiral shape to evenly distribute the inflow (Figure 2). The outflow from the column was recorded continuously by a tipping bucket rain gauge, and a glass beaker was placed under the rain gauge to collect runoff sample.
The main objective of the experiments was to investigate the effect of HDTMA modification on runoff reduction and NO3−-N, NH4+-N, and TP removal of media with zeolite on. The hydrological and pollutants treatment performance of all six substrates were tested under three rainfall intensities, which were 1-, 10-, and 100-year average recurrence interval (ARI) events of Shanghai, the corresponding rainfall depth are 36.5, 67.3, and 98.1 mm/h (SBQTS, 2017). The rainfall intensities were converted to inflow rates by rational method (Dooge, 1957), which were 19.17 ml/min (low inflow rate, 1-year ARI), 37.17 ml/L (medium inflow rate, 10-year ARI), and 51.33 ml/min (high inflow rate, 100-year ARI). Under each inflow rate condition, three replicas were conducted.
Synthetic stormwater was prepared by the mixture of municipal tap water with NO3−-N (KNO3), NH4+-N (NH4Cl), and TP (KH2PO4), and the concentrations of earlier pollutants were 5, 5, and 1 mg/L. Pollutants concentrations were set based on the average surface runoff quality of Shanghai (Han, 2015; Liu J. et. al., 2017). Synthetic stormwater was supplied by the peristaltic pump for 24 h under each rainfall intensity scenario. Outflow from the column was monitored by a tipping bucket rain gauge, which was connected with a computer that continuously works (1 min logging interval) since the pump was turned on. Water samples were collected by a borosilicate glass beaker in every 30 min since the outflow occurs, and stops when no outflow occurs from the column. Each sampling period last 15 min. The collected effluent sample was filtered with a 0.45 µm membrane filter.
Concentration of TP was determined using the ammonium molybdate spectrophotometric method, the Nessler’s reagent spectrophotometry method was used to test the NH4+-N concentration, and the ultraviolet spectrophotometer method was applied to analyze the NO3−-N concentration. The aforementioned methods are from Monitoring and Analysis Method of Water and Wastewater (Fourth Edition), and test procedures were conducted accordingly (MEEPRC, 2002). Event mean concentration (EMC) was used as the analytical parameter to evaluate the purification efficiency of the media. The average concentration of pollutants and the pollutant removal efficiency are calculated by the following equations:
where P is the efficiency of media pollutant removal; vi is the total inflow/outflow volume during the sampling period (L); ci is the pollutants concentration of inflow/outflow during the sampling period (mg/L); EMCin and EMCout are the mean concentrations of inflow and out flow pollutant.
During the experiment period, three rounds of experiments were conducted. In every round, each substrate was tested under different inflow rate conditions. Table 1 lists the test schedule of the first round. Schedules of round 2 (from 25th, Jan. 2021 to 12th, Mar. 2021) and round 3 (from 15th, Mar. 2021 to 13th, May. 2021) were arranged same as the first round, therefore, they were not listed here. One column was used for experiment. After one substrate was tested under one inflow rate condition, the column was emptied, and DOWSI Cleaner and Surface Prep Solvent was used to clean the high vacuum grease on the inner surface of the column, then high pressure water cleaner was used to wash the column with tap water. Finally, O.R. towel was used to wipe the column surface dry, and the column was ready to use for the next test.
The purpose of column experiment is characterizing the effect of different influencing factors on media’s stormwater regulation performance. The designed experiment has two factors with three levels (level of modification and rainfall intensity) and one factor with two levels (zeolite ratio), all possible combinations of different levels across these three factors for each response variable (runoff reduction, NO3−-N, NH4+-N, and TP removal rate) were tested, the effects of each factor on the response variables were investigate, and the quantitative models between factors and response variable were established. One-way analysis of variance (ANOVA) was performed to investigate statistical significance of factors on response variable. The multivariate regression analysis was used to establish quantitative relationship between factors and response variable. The data analysis was conducted by Minitab 19 Statistical Software.
Figure 3 shows the outflow rate of all media under the three tested inflow rate conditions. Table 2 lists the time of occurrence and flow rate for first flow, peak flow, and steady flow of tested media under the different inflow rate conditions. Three influencing factors examined in the experiments have significant effect on the runoff rate reduction of media with zeolite (p <0.05).
FIGURE 3. Outflow rate variation over time of different media under different inflow rate (A) 25% Z+75% S, (B) 50% Z+50% S, (C) 25% ZHD10 + 75% S, (D) 50% ZHD10 + 50% S, (E) 25% ZHD50 + 75% S, and (F) 50% ZHD50 + 50% S.
TABLE 2. Time of occurrence and flow rate for first flow, peak flow, and steady flow of different media under different inflow rates.
When the percentage of zeolite in substrate is the same, under all inflow rate conditions, the time of the appearance of first flow, peak flow, and steady flow were reduced as the level of modification increased. For example, when zeolite ratio in substrate was 25%, under low inflow rate condition, first flow, peak flow, and steady flow of media with NZ were reached at 425 min, 731 min, and 1193 min, for media with ZHD10, these flow conditions occurred at 408 min, 606 min, and 1163 min, and for media with ZHD50, these flow conditions were reached at 361 min, 475 min, and 972 min. In terms of the outflow rate, when the same amount of zeolite was added in substrate, under all inflow rate conditions, the modification increases the outflow rate of media for first flow and steady flow, and the rates increased when the degree of modification increases. Except when the inflow rate was 37.17 ml/min, 25% zeolite was added in the media, the first flow rate of NZ, ZHD10, and ZHD50 were 8.5, 6.3, and 9.5 ml/min. This might cause by the preferential flow which was discussed later in this section. These patterns might be the results of decreased water retention ability of the media. In previous study, HDTMA modification was reported to alter the hydraulic properties of substrate media with zeolite, including WRC. Based on the effect of HDTMA modification on WRC of zeolite media, the water retention ability of media with zeolite decreases with the increased level of modification (Qin et al., 2022), lower the water retention ability, quicker the water flows through the media. In general, the HDTMA modification decreases the outflow rate reduction of the substrate with zeolite, but media with MZ could reach steady flow sooner than that of media with NZ.
The time of peak flow was reached and the peak flow rate of different media under different inflow rate did not exhibit obvious pattern. For instance, when inflow rate was 19.17 ml/min, the peak flow rate for media with 25% of NZ, ZHD10, and ZHD50 were 10.8, 13.5, and 13.5 ml/min. For substrate with 50% zeolite ratio, under the rainfall condition of 37.17 ml/min, the peak flow rate for NZ, ZHD10, and ZHD50 were 32.4, 31.3, and 34.3 ml/min. This might be attributed to the preferential flow, which is when water transport through soil or porous materials, it would travel through the material in the path with least resistance. Under saturated condition, it mainly associates with inflow volume and flow rate, under unsaturated status, it mostly associates with pore structure and hydrophobicity of the material (Flury, 1996; Uhlenbrook, 2006; Nimmo, 2012; Beven and Germann, 2013). Despite the same procedure was applied to prepare and pack the substrates, parameters such as interaggregate pores and soil hydrophobicity of the materials cannot be maintained as the same for each experiment. For the first flow, the WRC of substrate also plays an important role in water movement, thus most of experiments demonstrated the same pattern, and only one experiment condition did not fit in the pattern (25% zeolite ratio, medium inflow rate). As the experiment process continues, the water content in substrate had increased but yet saturated, interaggregate pores and material hydrophobicity have significant impact on water flows at this stage, due to the different pore structure and hydrophobicity in each column, preferential flow might causes the irregular peak flow rate for each set of experiment. Finally, when the media in the column reaches near saturated status, inflow volume and rate were steadily controlled, which limited the effects of preferential flow. Additionally, the preferential flow was suggested to be introduced by the bimodal pore structure of the media (Liu Y. et al., 2017a), substrate with zeolite was found to have such pore structure (Qin et al., 2022).
Under the condition of the same modification degree, with more zeolite added in the substrate, the earlier the time that first flow, peak flow, and steady flow appears, the higher the rate of these flow statuses. When material with different particle shapes and relatively larger particle size such as zeolite was mixed with soil, it would alter medias’ particle size distribution, increases its Ks and decreases water retention ability (Liu and Fassman-Beck, 2016; Qin et al., 2022), which makes the velocity of water flow through the substrate positively correlated with zeolite ratio in media.
When level of modification and percentage of zeolite were fixed, with the increased rainfall intensity, the time of production for first flow, peak flow, and steady flow were reduced and flow rates were increased. Except for 25% Z+75% S, the first flow rate of high inflow rate was the lowest (3.7 ml/min), which might be the result of preferential flow as well.
The runoff volume reduction rate is shown in Figure 4. It can be observed that three influencing factors examined in the experiments were all negatively correlated with the runoff volume reduction rate of media, and the effect of these factors on media’s runoff volume reduction rate were significant (p <0.05). This result also is in accordance with the effect of HDTMA modification on WRC of the media with zeolite. Previous study indicates that substrates with MZ have poor water retention capacity than that of media with NZ, because of the HDTMA molecules loaded on the zeolite surface (Qin et al., 2022). The result from Figure 4 is consistent with findings in the previous study. The most significant decreases occurred when percentage of zeolite was 50%, under high inflow rate condition, the runoff volume reduction rate drops from 53.2% of NZ to 20.3% of ZHD50. The quantitative relationship between influencing factors with stormwater volume reduction rate and the interaction between these factors were further discussed in multiple regression analysis section.
Figure 5 demonstrates the removal rate of different media under different inflow rare on NO3−-N, NH4+-N, and TP. Three influencing factors examined in the experiments have significant effect on media’s removal performance of aforementioned pollutants (p <0.05). The substrate with NZ demonstrates decent removal efficiency on NH4+-N because of the cation exchange capacity of NZ. The MZ slightly increases the NH4+-N removal ability of the substrate, because the HDTMA molecules can only load on the zeolite surface, the cation exchange property in the inner pore structure of zeolite remains intact (Nezamzadeh-Ejhieh and Raja, 2013). Additionally, during the modification process, some impurities inside the zeolite might be removed through the stirring and heating, so the removal ability of NH4+-N was enhanced. Moreover, loaded surfactant molecules on zeolite surface can interact with ammonium head groups (Sharafzadeh and Nezamzadeh-Ejhieh, 2015) and traps some of the NH4+-N molecules; therefore, the removal efficiency of NH4+-N increased as the modification degree increased.
FIGURE 5. Removal rate of different media on different pollutants (A) NH4+-N removal rate of substrate with 25% zeolite, (B) NH4+-N removal rate of substrate with 50% zeolite, (C) NO3−-N removal rate of substrate with 25% zeolite, (D) NO3−-N removal rate of substrate with 50% zeolite, (E) TP removal rate of substrate with 25% zeolite, and (F) TP removal rate of substrate with 50% zeolite.
Media with NZ barely shows removal ability for NO3−-N, due to the negative charges carried by zeolite that make NZ not able to adsorb anionic pollutants. After the modification, the surfactant molecules loaded on zeolite surface gives MZ anionic exchange property, combined with the cation exchange capacity reserved in inner pores of zeolite, MZ can adsorb anions and cations pollutants simultaneously (Bowman, 2003; Nezamzadeh-Ejhieh and Tavakoli-Ghinani, 2014; Anari-Anaraki and Nezamzadeh-Ejhieh, 2015; Hasheminejad and Nezamzadeh-Ejhieh, 2015). As the results indicate, under all inflow rates, the modification of zeolite significantly enhances the NO3−-N treatment performance of media. For instance, when percentage of zeolite was 25%, under low inflow rate condition, the removal rate for NO3−-N increases from 7.6% (NZ) to 48.91% (ZHD10) and 52.7% (ZHD50). Positive correlation was found between NO3−-N removal rate and level of modification. However, the degree of improvement is not high, given the fact that nearly twice of HDTMA molecules loaded on ZHD50 (0.09meq/g) than that of ZHD10 (0.05meq/g). This might attribute to some anions substances contained in the peat soil. When MZ and peat soil was mixed together and soaked in the water, some of the anions exchanged with cationic groups on the MZ surface, thus the improvement from the increased modification degree was relatively small.
As for TP removal, leaching of phosphorus was observed during the experiment when media with NZ was tested, which was up to 9.1% when percentage of zeolite was 25% under the low inflow rate condition. This phenomenon is common in the bioretention column study of non-vegetated substrate media (Hatt et al., 2009; Liu and Davis, 2014; Li et al., 2016), attribute to the phosphorus brought by the soil in the substrate. Without the plant uptake, phosphorus would leach from the column when other materials in the substrate have limited phosphorus removal efficiency. Phosphorus in the synthetic stormwater was dissolved phosphate, which is also anion pollutant like NO3−-N, therefore, positive correlation was found between level of modification and TP removal rate as well. However, the effect of MZ on TP adsorption improvement was not as notable as that of NO3−-N removal. For example, in substrate with 50% zeolite, under medium inflow rate, TP removal rate increased by 2.2% from ZHD10 to ZHD50, while NO3−-N removal efficiency raised by 7.9% from ZHD10 to ZHD50. This is because when phosphorus and NO3−-N were removed by MZ simultaneously, MZ would adsorb NO3−-N molecules preferentially (Li et al., 2017).
The percentage of zeolite in substrate was positively correlated with NO3−-N, NH4+-N, and TP removal rate, while negative correlation was found between rainfall intensities and the removal of these pollutants. In terms of pollutants removal, zeolite act as modifier in the substrate, it enhances the pollutants removal performance of substrate, therefore, with more zeolite used in the substrate, higher the pollutants removal rate of the media. Under the low inflow rate, highest NO3−-N, NH4+-N, and TP removal efficiency were reached when substrate contain 50% of ZHD50, which were 65.3%, 65.6%, and 18.7%, respectively.
As the inflow rate increases, the velocity of water flow through media increments, thus the time for zeolite to adsorb pollutants was shorten, and stability for the adsorption process was comprised, the removal efficiency of substrate on all pollutants decreases consequently. However, when NZ was used in substrate, more phosphorus leached from the substrate as the inflow rate decreased, possibly because more phosphorus from the peat soil could be dissolved in water when water move through media slows down.
A total of 54 set of experiments data from column studies were used to conduct multiple regression analysis between influencing factors and response variables, with the objective to establish quantitative relationship model between level of modification (represented by amount of HDTMA molecules loaded on zeolite surface), percentage of zeolite in substrate, rainfall intensity (represented by the inflow rate), and substrate stormwater regulation performance. The main effect plot and influencing factor interaction plot were generate based on fitted means using the least squares model. The quantitative relationships are shown in following formulas:
where Rr is the runoff volume reduction rate (%); Rp (NO3), Rp (NH4), and Rp (TP) are the removal rate of NO3−-N, NH4+-N, and TP (%); I is the inflow rate (ml/min); Z is zeolite ratio in substrate (%); M is the amount of HDTMA molecules loaded on the zeolite surface (meq/g); R2 is the coefficient of determination.
Figure 6 is the main effect plot of different response factors, which can be used to examine the levels of effect of influencing factors on response variables. The main effects plot graphs the response mean for each factor level connected by a line, levels of effect could be determined based on the slope of the line. Ratio of zeolite to the peat soil (Zratio) and level of modification of zeolite (Modification) were positively correlated with NO3−-N, NH4+-N, and TP removal rate, inflow rate (Inflow) was negatively correlated with the removal rate of these pollutants. All three factors were negatively correlated with the runoff volume reduction rate. The level of effect of different influencing factors on response variables are: (1) Zratio > Inflow > Modification for NH4+-N removal rate; (2) Modification > Inflow > Zratio for NO3−-N removal rate; (3) Modification > Zratio > Inflow for TP removal rate; and (4) Inflow > Modification > Zratio for the runoff volume reduction rate. HDTMA modification of zeolite has notable effect on all four response variables. When MZ is being considered as substrate material, its positive effect on water quality improvement and negative impact on water quantity control should both be taken into account. Additionally, the level of effect of Modification on NO3−-N removal rate reached the top when the amount of HDTMA molecules loaded on the zeolite surface was 0.09meq/g (ZHD50%), which means when the modification level of zeolite is beyond 50% of zeolite’s CEC value, increasing modification degree of zeolite would no longer improves the NO3−-N removal performance of substrate.
FIGURE 6. Main effect plot for different response variables (A) main effect plot for NH4+-N removal rate, (B) main effect plot for NO3− removal rate, (C) main effect plot for runoff volume reduction, and (D) main effect plot for TP removal rate.
Figure 7 shows the influencing factor interaction plot of different response variables. The effect of interaction between influencing factors exists on the runoff volume reduction rate, the TP removal rate, and the NO3−-N removal rate, no effect of interaction between influencing factors on the NH4+-N removal rate was found, which can also be observed from the relationship models. The interaction between Zratio and Modification suggests that when more zeolite with higher level of modification was added in the substrate, less runoff could be intercept by the substrate, but higher removal efficiency on NO3−-N could be achieved. The effect of interaction between Zratio and Modification was not found on the TP removal rate, probably due to the preferential adsorption of MZ on NO3−-N. When most of the adsorption capacity of MZ on anionic pollutants was occupied by NO3−-N, the interaction between substrate factors did not exhibit statistical impact on the TP removal rate. The interaction between Inflow and Modification appease to affect the TP and NO3−-N adsorption performance, increased level of modification could mitigate the decline in TP and NO3−-N removal rate of substrate as inflow rate raised, and with the increase of inflow, the improvement effect of increasing modification degree decreased.
FIGURE 7. Influencing factor interaction plot of (A) runoff volume reduction rate, (B) TP removal rate, and (C) NO3−-N removal rate.
This research has shown that HDTMA modification of zeolite could increases the NO3−-N, NH4+-N, and TP removal rate of bioretention media with zeolite. Under different conditions, removal performance of NO3−-N for media with MZ were 6.4–38.2 times than that of NZ, and NH4+-N removal rate increased by 1–1.5 times through the modification. The modification improves the phosphorus adsorption ability of media, but not as notable as that of NO3−-N adsorption. The highest TP removal rate was 18.7% ,while highest NO3−N removal rates was 65.3%. Percentage of zeolite and level of modification were found positively correlated with the removal rate beyond pollutants, while negative correlation was found between the inflow rate and pollutants removal efficiency. However, the modification was proved to reduce the reduction rate on runoff volume and outflow rate, because of the decreased water retention ability of media caused by the modification. Therefore, when hydrological regulation is the main control objective of the bioretention system, the negative effect of HDTMA-modified zeolite should be considered. In terms of outflow of the substrate, preferential flow was suggested to affect the time of peak flow was reached and peak flow rate, caused irregular fluctuation of peak flow status. Quantitative relationship model (R2>0.793) among influencing factors, hydrological and pollutants treatment performance was given, which can be useful for modeling and predicting the performance of the bioretention system, and for designing substrate media. Level of modification was found to have the most significant effect on NO3−-N and TP removal rate of substrate. The inflow rate was negatively correlated with removal efficiency of all three pollutants, but the increases of modification level was found to reduces the decrease of NO3−-N and TP adsorption efficiency while the inflow rate increases. Additionally, when loading amount of HDTMA molecules on zeolites exceeds 0.09meq/mg, increasing modification level cannot improve the removal efficiency of NO3−-N. The results from column experiment cannot represent the actual behavior of bioretention media with MZ in field application. Therefore, filed experiment for substrate with HDTMA-modified zeolite is needed, as well as long-term monitoring of its performance, for in-depth evaluation of zeolite substrate performance under service condition and model validation.
The raw data supporting the conclusions of this article will be made available by the authors, without undue reservation.
YQ developed the experiment procedures, collected and analyzed the data, and led the writing of the manuscript. MC and SL provided advice and background on zeolite modification and participated in the column experiments. YF contributed to the data analysis and modeling. XL and JQ reviewed and revised the manuscript and supervised the research project. All authors contributed to the design, implementation, and writing of the manuscript.
This work was supported by the Yunnan Erhai Lake Ecosystem Observation and Research Station (Grant No. 2020ZZ01) and the National Key Project of Water Pollution Control and Treatment (Grant No. 2014ZX07303-003-11).
The authors would like to acknowledge and appreciate the Instrumental Analysis Center of Shanghai Jiao Tong University for providing experimental equipment and performing thermogravimetric analysis.
The authors declare that the research was conducted in the absence of any commercial or financial relationships that could be construed as a potential conflict of interest.
All claims expressed in this article are solely those of the authors and do not necessarily represent those of their affiliated organizations, or those of the publisher, the editors, and the reviewers. Any product that may be evaluated in this article, or claim that may be made by its manufacturer, is not guaranteed or endorsed by the publisher.
Alidusty, F., and Nezamzadeh-Ejhieh, A. (2016). Considerable decrease in overvoltage of electro-catalytic oxidation of methanol by modification of carbon paste electrode with Cobalt(II)-clinoptilolite nanoparticles. Int. J. Hydrogen Energy 41, 6288–6299. doi:10.1016/j.ijhydene.2016.02.149
Anari-Anaraki, M., and Nezamzadeh-Ejhieh, A. (2015). Modification of an Iranian clinoptilolite nano-particles by hexadecyltrimethyl ammonium cationic surfactant and dithizone for removal of Pb(II) from aqueous solution. J. Colloid Interface Sci. 440, 272–281. doi:10.1016/j.jcis.2014.11.017
Baek, S.-S., Choi, D.-H., Jung, J.-W., Lee, H.-J., Lee, H., Yoon, K.-S., et al. (2015). Optimizing low impact development (LID) for stormwater runoff treatment in urban area, Korea: Experimental and modeling approach. Water Res. 86, 122–131. doi:10.1016/j.watres.2015.08.038
Beven, K., and Germann, P. (2013). Macropores and water flow in soils revisited. Water Resour. Res. 49, 3071–3092. doi:10.1002/wrcr.20156
Bowman, R. S. (2003). Applications of surfactant-modified zeolites to environmental remediation. Microporous Mesoporous Mat. 61, 43–56. doi:10.1016/S1387-1811(03)00354-8
Brown, R. A., and Hunt, W. F. (2011). Impacts of media depth on effluent water quality and hydrologic performance of undersized bioretention cells. J. Irrig. Drain. Eng. 137, 132–143. doi:10.1061/(ASCE)IR.1943-4774.0000167
Chang, N., Wen, D., and Wanielista, M. P. (2019). Impact of changing environmental factors and species competition on iron filings-based green environmental media for nutrient removal in stormwater treatment. Environ. Prog. Sustain. Energy 38, 13087. doi:10.1002/ep.13087
Chen, J., Theller, L., Gitau, M. W., Engel, B. A., and Harbor, J. M. (2017). Urbanization impacts on surface runoff of the contiguous United States. J. Environ. Manage. 187, 470–481. doi:10.1016/j.jenvman.2016.11.017
Davidson, E. A., Savage, K. E., Bettez, N. D., Marino, R., and Howarth, R. W. (2010). Nitrogen in runoff from residential roads in a coastal area. Water Air Soil Pollut. 210, 3–13. doi:10.1007/s11270-009-0218-2
Davis, A. P., Hunt, W. F., Traver, R. G., and Clar, M. (2009). Bioretention technology: Overview of current practice and future needs. J. Environ. Eng. 135135, 1093–1117. doi:10.1061/(asce)0733-9372(2009)135:3(109)
DeBusk, K. M., Hunt, W. F., and Line, D. E. (2011). Bioretention outflow: Does it mimic nonurban watershed shallow interflow? J. Hydrol. Eng. 16, 274–279. doi:10.1061/(ASCE)HE.1943-5584.0000315
Dooge, J. C. I. (1957). The rational method for estimating flood peaks. Engineering 184 (1), 374–377.
Edeh, I. G., and Mašek, O. (2022). The role of biochar particle size and hydrophobicity in improving soil hydraulic properties. Eur. J. Soil Sci. 73, 13138. doi:10.1111/ejss.13138
Ejhieh, A. N., and Masoudipour, N. (2010). Application of a new potentiometric method for determination of phosphate based on a surfactant-modified zeolite carbon-paste electrode (SMZ-CPE). Anal. Chim. Acta X. 658, 68–74. doi:10.1016/j.aca.2009.10.064
Endo, M., Yoshikawa, E., Muramatsu, N., Takizawa, N., Kawai, T., Unuma, H., et al. (2013). The removal of cesium ion with natural Itaya zeolite and the ion exchange characteristics: Removal of cesium ion with natural Itaya zeolite. J. Chem. Technol. Biotechnol. 88, 1597–1602. doi:10.1002/jctb.4140
Fassman-Beck, E., Wang, S., Simcock, R., and Liu, R. (2015). Assessing the effects of bioretention’s engineered media composition and compaction on hydraulic conductivity and water holding capacity. J. Sustain. Water Built Environ. 1, 04015003. doi:10.1061/JSWBAY.0000799
Fletcher, T. D., Vietz, G., and Walsh, C. J. (2014). Protection of stream ecosystems from urban stormwater runoff: The multiple benefits of an ecohydrological approach. Prog. Phys. Geogr. Earth Environ. 38, 543–555. doi:10.1177/0309133314537671
Flury, M. (1996). Experimental evidence of transport of pesticides through field soils—a review. J. Environ. Qual. 25, 25–45. doi:10.2134/jeq1996.00472425002500010005x
Gao, Y., Jia, Y., Yu, G., He, N., Zhang, L., Zhu, B., et al. (2019). Anthropogenic reactive nitrogen deposition and associated nutrient limitation effect on gross primary productivity in inland water of China. J. Clean. Prod. 208, 530–540. doi:10.1016/j.jclepro.2018.10.137
Goh, H. W., Lem, K. S., Azizan, N. A., Chang, C. K., Talei, A., Leow, C. S., et al. (2019). A review of bioretention components and nutrient removal under different climates—Future directions for tropics. Environ. Sci. Pollut. Res. 26, 14904–14919. doi:10.1007/s11356-019-05041-0
Gray, M., Johnson, M. G., Dragila, M. I., and Kleber, M. (2014). Water uptake in biochars: The roles of porosity and hydrophobicity. Biomass Bioenergy 61, 196–205. doi:10.1016/j.biombioe.2013.12.010
Han, J. (2015). Characteristics of urban rainwater runoff pollution and simulation & optimization of discharge systems. PhD thesis. Shanghai: Fudan University.
Hasheminejad, M., and Nezamzadeh-Ejhieh, A. (2015). A novel citrate selective electrode based on surfactant modified nano-clinoptilolite. Food Chem. x. 172, 794–801. doi:10.1016/j.foodchem.2014.09.057
Hatt, B. E., Fletcher, T. D., and Deletic, A. (2009). Hydrologic and pollutant removal performance of stormwater biofiltration systems at the field scale. J. Hydrology 365, 310–321. doi:10.1016/j.jhydrol.2008.12.001
Heidari-Chaleshtori, M., and Nezamzadeh-Ejhieh, A. (2015). Clinoptilolite nano-particles modified with aspartic acid for removal of Cu( ii ) from aqueous solutions: Isotherms and kinetic aspects. New J. Chem. 39, 9396–9406. doi:10.1039/C5NJ01631B
Hua, Y., Peng, L., Zhang, S., Heal, K. V., Zhao, J., and Zhu, D. (2017). Effects of plants and temperature on nitrogen removal and microbiology in pilot-scale horizontal subsurface flow constructed wetlands treating domestic wastewater. Ecol. Eng. 108, 70–77. doi:10.1016/j.ecoleng.2017.08.007
Hyväluoma, J., Räty, M., Kaseva, J., and Keskinen, R. (2020). Changes over time in near-saturated hydraulic conductivity of peat soil following reclamation for agriculture. Hydrol. Process. 34, 237–243. doi:10.1002/hyp.13578
Jia, H., Wang, X., Ti, C., Zhai, Y., Field, R., Tafuri, A. N., et al. (2015). Field monitoring of a LID-BMP treatment train system in China. Environ. Monit. Assess. 87 (6), 373–418. doi:10.1007/s10661-015-4595-2
Jiang, C., Li, J., Li, H., and Li, Y. (2019). An improved approach to design bioretention system media. Ecol. Eng. 136, 125–133. doi:10.1016/j.ecoleng.2019.06.014
Kameyama, K., Miyamoto, T., and Iwata, Y. (2019). The preliminary study of water-retention related properties of biochar produced from various feedstock at different pyrolysis temperatures. Materials 12, 1732. doi:10.3390/ma12111732
Le Coustumer, S., Fletcher, T. D., Deletic, A., Barraud, S., and Poelsma, P. (2012). The influence of design parameters on clogging of stormwater biofilters: A large-scale column study. Water Res. 46, 6743–6752. doi:10.1016/j.watres.2012.01.026
Li, C., Liu, M., Hu, Y., Shi, T., Qu, X., and Walter, M. T. (2018). Effects of urbanization on direct runoff characteristics in urban functional zones. Sci. Total Environ. 643, 301–311. doi:10.1016/j.scitotenv.2018.06.211
Li, C., Yao, J., Zhang, T., Xing, W., Liang, Y., and Xiang, M. (2017). Simultaneous removal of nitrogen and phosphorus by cetylpyridinium bromide modified zeolite. Water Sci. Technol. 76 (11-12), 2895–2906. doi:10.2166/wst.2017.459
Li, J., Jiang, C., Lei, T., and Li, Y. (2016). Experimental study and simulation of water quality purification of urban surface runoff using non-vegetated bioswales. Ecol. Eng. 95, 706–713. doi:10.1016/j.ecoleng.2016.06.060
Li, Z., Roy, S. J., Zou, Y., and Bowman, R. S. (1998). Long-term chemical and biological stability of surfactant-modified zeolite. Environ. Sci. Technol. 32, 2628–2632. doi:10.1021/es970841e
Line, D. E., and White, N. M. (2007). Effects of development on runoff and pollutant export. Water Environ. Res. 79, 185–190. doi:10.2175/106143006X111736
Liu, J., and Davis, A. P. (2014). Phosphorus speciation and treatment using enhanced phosphorus removal bioretention. Environ. Sci. Technol. 48, 607–614. doi:10.1021/es404022b
Liu, J., Huang, H., Huang, R., Zhang, J., Hao, S., Shen, Y., et al. (2016). Mechanisms of CPB modified zeolite on mercury adsorption in simulated wastewater. water Environ. Res. 88, 490–499. doi:10.2175/106143016X14504669767850
Liu, J., Zhang, F., Yang, Q., and Bi, D. (2017a). Characteristics of runoff pollution and rainwater resource utilization technology in Shanghai elevated road. J. Technol. 17 (4), 304–308. doi:10.3969/j.issn.2096-3424.2017.04.005
Liu, R., and Fassman-Beck, E. (2016). Effect of composition on basic properties of engineered media for living roofs and bioretention. J. Hydrol. Eng. 21 (6), 06016002. doi:10.1061/(ASCE)HE.1943-5584.0001373
Liu, R., and Fassman-Beck, E. (2017b). Hydrologic experiments and modeling of two laboratory bioretention systems under different boundary conditions. Front. Environ. Sci. Eng. 11 (4), 10–92. doi:10.1007/s11783-017-0951-5
Liu, R., and Fassman-Beck, E. (2017a). Hydrologic response of engineered media in living roofs and bioretention to large rainfalls: Experiments and modeling. Hydrol. Process. 31, 556–572. doi:10.1002/hyp.11044
Liu, R., and Fassman-Beck, E. (2018). Pore structure and unsaturated hydraulic conductivity of engineered media for living roofs and bioretention based on water retention data. J. Hydrol. Eng. 23, 04017065. doi:10.1061/(ASCE)HE.1943-5584.0001621
Liu, Y., Engel, B. A., Flanagan, D. C., Gitau, M. W., McMillan, S. K., and Chaubey, I. (2017b). A review on effectiveness of best management practices in improving hydrology and water quality: Needs and opportunities. Sci. Total Environ. 602, 580–593. doi:10.1016/j.scitotenv.2017.05.212
Lucas, W. C. (2010). Design of integrated bioinfiltration-detention urban retrofits with design storm and continuous simulation methods. J. Hydrol. Eng. 15, 486–498. doi:10.1061/(ASCE)HE.1943-5584.0000137
Lucas, W. C., and Greenway, M. (2011). Hydraulic response and nitrogen retention in bioretention mesocosms with regulated outlets: Part I-hydraulic response. Water Environ. Res. 83, 692–702. doi:10.2175/106143010X12780288628697
Mahdavi, M., and Nezamzadeh-Ejhieh, A. (2017). An aluminum selective electrode via modification of PVC membrane by modified clinoptilolite nanoparticles with hexadecyltrimethyl ammonium bromide (HDTMA-Br) surfactant containing Arsenazo III. J. Colloid Interface Sci. 494, 317–324. doi:10.1016/j.jcis.2017.01.102
Ministry of Ecology and Environment of People's Republic of China (Meeprc) (2002). “Chapter 3- Evaluation index and inorganic pollutants,” in Monitoring and analysis method of water and wastewater. Fourth Edition (Beijing, China: China Environmental Science Press), 45–90.
Misaelides, P. (2011). Application of natural zeolites in environmental remediation: A short review. Microporous Mesoporous Mat. 144, 15–18. doi:10.1016/j.micromeso.2011.03.024
Naghash, A., and Nezamzadeh-Ejhieh, A. (2015). Comparison of the efficiency of modified clinoptilolite with HDTMA and HDP surfactants for the removal of phosphate in aqueous solutions. J. Ind. Eng. Chem. 31, 185–191. doi:10.1016/j.jiec.2015.06.022
Nasiri-Ardali, M., and Nezamzadeh-Ejhieh, A. (2020). A comprehensive study on the kinetics and thermodynamic aspects of batch and column removal of Pb(II) by the clinoptilolite–glycine adsorbent. Mat. Chem. Phys. 240, 122142. doi:10.1016/j.matchemphys.2019.122142
Nezamzadeh-Ejhieh, A., and Afshari, E. (2012). Modification of a PVC-membrane electrode by surfactant modified clinoptilolite zeolite towards potentiometric determination of sulfide. Microporous Mesoporous Mat. 153, 267–274. doi:10.1016/j.micromeso.2011.12.054
Nezamzadeh-Ejhieh, A., and Mirzaeyan, E. (2013). Hexadecylpyridinium surfactant modified zeolite A as an active component of a polymeric membrane sulfite selective electrode. Mater. Sci. Eng. C 33, 4751–4758. doi:10.1016/j.msec.2013.07.040
Nezamzadeh-Ejhieh, A., and Nematollahi, Z. (2011). Surfactant modified zeolite carbon paste electrode (SMZ-CPE) as a nitrate selective electrode. Electrochimica Acta 56, 8334–8341. doi:10.1016/j.electacta.2011.07.013
Nezamzadeh-Ejhieh, A., and Raja, G. (2013). Modification of nanoclinoptilolite zeolite with hexadecyltrimethylammonium surfactant as an active ingredient of chromate-selective membrane electrode. J. Chem. 2013, 1–13. doi:10.1155/2013/685290
Nezamzadeh-Ejhieh, A., and Shahanshahi, M. (2013). Modification of clinoptilolite nano-particles with hexadecylpyridynium bromide surfactant as an active component of Cr(VI) selective electrode. J. Ind. Eng. Chem. 19, 2026–2033. doi:10.1016/j.jiec.2013.03.018
Nezamzadeh-Ejhieh, A., and Tavakoli-Ghinani, S. (2014). Effect of a nano-sized natural clinoptilolite modified by the hexadecyltrimethyl ammonium surfactant on cephalexin drug delivery. C. R. Chim. 17, 49–61. doi:10.1016/j.crci.2013.07.009
Nimmo, J. R. (2012). Preferential flow occurs in unsaturated conditions. Hydrol. Process. 26, 786–789. doi:10.1002/hyp.8380
Nosuhi, M., and Nezamzadeh-Ejhieh, A. (2018). A sensitive and simple modified zeolitic carbon paste electrode for indirect voltammetric determination of nitrate. Ionics 24, 2135–2145. doi:10.1007/s11581-018-2456-z
Palla, A., Gnecco, I., and Lanza, L. G. (2009). Unsaturated 2D modelling of subsurface water flow in the coarse-grained porous matrix of a green roof. J. Hydrol. X. 379, 193–204. doi:10.1016/j.jhydrol.2009.10.008
Paus, K. H., Morgan, J., Gulliver, J. S., and Hozalski, R. M. (2014). Effects of bioretention media compost volume fraction on toxic metals removal, hydraulic conductivity, and phosphorous release. J. Environ. Eng. 140, 04014033. doi:10.1061/(ASCE)EE.1943-7870.0000846
Poor, C. J., Conkle, K., MacDonald, A., and Duncan, K. (2019). Water treatment residuals in bioretention planters to reduce phosphorus levels in stormwater. Environ. Eng. Sci. 36, 265–272. doi:10.1089/ees.2018.0254
Qin, Y., Chen, M., Yunqing, F., Li, X., Wang, J., and Qiu, J. (2022). Physical and hydraulic properties of bioretention substrate using hexadecyl trimethyl ammonium bromide (HDTMA) modified zeolite. Environ. Technol. 1, 13. doi:10.1080/09593330.2022.2056084
Reddy, K. R., Xie, T., and Dastgheibi, S. (2014). Removal of heavy metals from urban stormwater runoff using different filter materials. J. Environ. Chem. Eng. 2, 282–292. doi:10.1016/j.jece.2013.12.020
Saadat, M., and Nezamzadeh-Ejhieh, A. (2016). Clinoptilolite nanoparticles containing HDTMA and Arsenazo III as a sensitive carbon paste electrode modifier for indirect voltammetric measurement of Cesium ions. Electrochimica Acta 217, 163–170. doi:10.1016/j.electacta.2016.09.084
Sari, A. Y., Suwartha, N., Hartono, D. M., and Gusniani, I. (2019). Enhancing removal efficiency of heavy metals and ammonia in bioretention system using quartz sand and zeolite as filter media. IOP Conf. Ser. Mat. Sci. Eng. 536, 012071. doi:10.1088/1757-899X/536/1/012071
Schick, J., Caullet, P., Paillaud, J.-L., Patarin, J., and Mangold-Callarec, C. (2010). Batch-wise nitrate removal from water on a surfactant-modified zeolite. Microporous Mesoporous Mat. 132, 395–400. doi:10.1016/j.micromeso.2010.03.018
Schick, J., Caullet, P., Paillaud, J.-L., Patarin, J., and Mangold-Callarec, C. (2011). Nitrate sorption from water on a Surfactant-Modified Zeolite. Fixed-bed column experiments. Microporous Mesoporous Mat. 142, 549–556. doi:10.1016/j.micromeso.2010.12.039
Seelsaen, N., McLaughlan, R., Moore, S., Ball, J. E., and Stuetz, R. M. (2006). Pollutant removal efficiency of alternative filtration media in stormwater treatment. Water Sci. Technol. 54, 299–305. doi:10.2166/wst.2006.617
Shanghai Bureau of Quality and Technical Supervision (SBQTS) (2017). Standard of rainstorm intensity formula and design rainstorm distribution. DB31/T 1043-2017.
Sharafzadeh, S., and Nezamzadeh-Ejhieh, A. (2015). Using of anionic adsorption property of a surfactant modified clinoptilolite nano-particles in modification of carbon paste electrode as effective ingredient for determination of anionic ascorbic acid species in presence of cationic dopamine species. Electrochimica Acta 184, 371–380. doi:10.1016/j.electacta.2015.09.164
Shi, W., Shao, H., Li, H., Shao, M., and Du, S. (2009). Progress in the remediation of hazardous heavy metal-polluted soils by natural zeolite. J. Hazard. Mat. 170, 1–6. doi:10.1016/j.jhazmat.2009.04.097
Stracher, G. B., Prakash, A., and Rein, G. (2015). “Chapter 1 - smoldering-peat megafires: The largest fires on earth,” in Coal and peat fires: A global perspective (Boston: Elsevier), 1–11. doi:10.1016/B978-0-444-59510-2.00001-X
Tamiji, T., and Nezamzadeh-Ejhieh, A. (2019). Sensitive voltammetric determination of bromate by using ion-exchange property of a Sn(II)-clinoptilolite-modified carbon paste electrode. J. Solid State Electrochem. 23, 143–157. doi:10.1007/s10008-018-4119-4
Tao, Q., Hu, M., Ma, X., Xiang, M., Zhang, T. C., Li, C., et al. (2015). Simultaneous removal of ammonium and nitrate by HDTMA-modified zeolite. Water Sci. Technol. 72, 1931–1939. doi:10.2166/wst.2015.392
Uhlenbrook, S. (2006). Catchment hydrology—A science in which all processes are preferential. Hydrol. Process. 20, 3581–3585. doi:10.1002/hyp.6564
van Genuchten, M. Th. (1980). A closed-form equation for predicting the hydraulic conductivity of unsaturated soils. Soil Sci. Soc. Am. J. 44, 892–898. doi:10.2136/sssaj1980.03615995004400050002x
Walsh, C. J., Fletcher, T. D., and Burns, M. J. (2012). Urban stormwater runoff: A new class of environmental flow problem. PLoS ONE 7, e45814. doi:10.1371/journal.pone.0045814
Wang, H., Gan, H., Zhang, Z., Yu, Z., and Zhu, D. Z. (2021). Purification efficiency of bioretention with modified media under varied rain intensity and drying conditions. J. Environ. Eng. 147, 04021009. doi:10.1061/(ASCE)EE.1943-7870.0001868
Wang, X., Li, J., Li, S., and Zheng, X. (2017). A study on removing nitrogen from paddy field rainfall runoff by an ecological ditch–zeolite barrier system. Environ. Sci. Pollut. Res. 24, 27090–27103. doi:10.1007/s11356-017-0269-7
Yusof, A. M., and Malek, N. A. N. N. (2009). Removal of Cr(VI) and As(V) from aqueous solutions by HDTMA-modified zeolite Y. J. Hazard. Mat. 162, 1019–1024. doi:10.1016/j.jhazmat.2008.05.134
Zhan, Y., Lin, J., and Zhu, Z. (2011). Removal of nitrate from aqueous solution using cetylpyridinium bromide (CPB) modified zeolite as adsorbent. J. Hazard. Mat. 186, 1972–1978. doi:10.1016/j.jhazmat.2010.12.090
Zhou, J., Fan, X., Zhang, D., Tang, Y., Wang, X., Yuan, Z., et al. (2022). Potential exploration of Fe3O4/biochar from sludge as the media of bioretention system and its comparison with conventional media. Environ. Sci. Pollut. Res. 29, 37906–37918. doi:10.1007/s11356-021-17334-4
Keywords: bioretention substrate, zeolite, surface modification, stormwater pollutants, runoff regulation
Citation: Qin Y, Chen M, Liu S, Fang Y, Li X and Qiu J (2023) Runoff regulation and nitrogen and phosphorus removal performance of a bioretention substrate with HDTMA-modified zeolite. Front. Environ. Sci. 10:918259. doi: 10.3389/fenvs.2022.918259
Received: 12 April 2022; Accepted: 30 November 2022;
Published: 20 January 2023.
Edited by:
Ahmed El Nemr, National Institute of Oceanography and Fisheries (NIOF), EgyptReviewed by:
Cara Poor, University of Portland, United StatesCopyright © 2023 Qin, Chen, Liu, Fang, Li and Qiu. This is an open-access article distributed under the terms of the Creative Commons Attribution License (CC BY). The use, distribution or reproduction in other forums is permitted, provided the original author(s) and the copyright owner(s) are credited and that the original publication in this journal is cited, in accordance with accepted academic practice. No use, distribution or reproduction is permitted which does not comply with these terms.
*Correspondence: Jiangpin Qiu, anBxQHNqdHUuZWR1LmNu
Disclaimer: All claims expressed in this article are solely those of the authors and do not necessarily represent those of their affiliated organizations, or those of the publisher, the editors and the reviewers. Any product that may be evaluated in this article or claim that may be made by its manufacturer is not guaranteed or endorsed by the publisher.
Research integrity at Frontiers
Learn more about the work of our research integrity team to safeguard the quality of each article we publish.