- 1School of Earth Science, Yunnan University, Kunming, China
- 2Institute of Atmospheric Physics, Chinese Academy of Sciences, Beijing, China
- 3Natural Resources Institute, University of Greenwich, Greenwich, Medway, United Kingdom
- 4Department of Atmospheric Sciences, Texas A&M University, College Station, TX, United States
- 5Hefei Institutes of Physical Science, Chinese Academy of Sciences, Hefei, China
Anthropogenic heat release is the release of heat generated by anthropogenic energy consumption. The regional mean Anthropogenic heat release flux in Southwest China grew quickly from 0.06 Wm-2 in 1992 to a peak of 0.37 Wm-2 in 2019. This study examines the climatic effects and feedbacks of Anthropogenic heat release due to energy consumption in Southwest China during the boreal summer using simulations from the Community Earth System Model. The modeling results show that Anthropogenic heat release impacts on the lower-troposphere stability and affects large-scale atmospheric circulation in Southwest China, which transports more water vapor and consequently increases the humidity and low cloud cover in Southwest China. This effect impacts the energy balance at the surface by reducing the amount of incoming shortwave radiation that reaches the ground. Anthropogenic heat release decreases the average 2-m air temperature in Southwest China by 0.10 ± 0.01 K (1σ uncertainty) and also decreases the minimum and maximum air temperatures in Southwest China as well. Anthropogenic heat release contributes to cooler and wetter summers in Southwest China. The results show that Anthropogenic heat release is a non-negligible factor that impacts the climate of Southwest China. This study improves our understanding of the climate change resulting from human activities in Southwest China.
1 Introduction
Human activity has been considered as the predominant contributor to global warming over the past 100 years (IPCC, 2021). Large amounts of fossil fuels are consumed in human activities, which leads to more greenhouse gases and aerosols being released into the atmosphere. The greenhouse gas CO2 has been the main cause of global warming over the past 100 years (IPCC, 2021). Human activities have changed the composition of the atmosphere and the land use and coverage, further impacting on the energy balance of the Earth-atmosphere system. The energy balance of the Earth-atmosphere system is the predominant factor in global climate change (Hansen et al., 2005). Anthropogenic forcing, such as greenhouse gases and urbanization, increase the occurrence of extreme climate events (Peterson et al., 2013; Zhao et al., 2018). Anthropogenic heat release (AHR), which is an external energy source within the Earth-atmosphere system, directly impacts the energy balance of the Earth’s surface due to global energy consumption (Forster et al., 2007). With rapid global urbanization, more energy will be consumed as urban populations increase, and the effects of AHR on regional urban climates will be enhanced (IPCC, 2007). Previous research shows that AHR can impact regional and large-scale atmospheric circulations (Zhang et al., 2013; Chen et al., 2014; Chen et al., 2016) and further affect the global surface energy balance (Chen et al., 2019).
Southwest China (SWC) is a large region that includes Sichuan Province (SC), Guizhou Province (GZ), Yunnan Province (YN), and Chongqing City (CQ), and it occupies approximately 13% of the total area of China. The topography of SWC is very complex, including the Sichuan Basin and the Yunnan-Guizhou Plateau. SWC has a subtropical monsoon climate with hot and rainy summers and mild winters with little precipitation. Due to the geographical location and altitude variations, the climate in SWC varies, with significant differences in temperature and precipitation. SWC is a populated and agriculturally developed region, and urbanization has increased rapidly in recent years. Additionally, extreme climate events, such as extreme drought and rainfall appear more frequent in SWC under global climate warming (Zhai et al., 2005; Chen and Zhai, 2013; Ma et al., 2013; Chen and Sun, 2015). Previous research found an increasing risk of extreme wet events and dry events in SWC (Liu et al., 2014), which is strongly correlated with the abnormal transport of water vapor (Zhang et al., 2011; Li et al., 2014). Previous studies have focused on natural factors, such as sea surface temperature (SST) and atmospheric circulation (Chen et al., 2009; Liu et al., 2009; Lu et al., 2011), as well as anthropogenic factors, such as aerosols and urbanization (Fan et al., 2015; Chen and Frauenfeld, 2016). The rapid pace of urbanization in China in recent decades includes increased development in SWC. This intense urbanization has had an increasingly significant impact on the climate of SWC and is an important factor that cannot be ignored. Previous studies have found that the heating effect driven by AHR has an important influence on local urban climates and may impact local circulation, extreme high temperatures, and heavy rainfall (Zaksek and Ostir, 2012; Wang et al., 2015; Zhong et al., 2017). The uneven heating effects due to AHR will become more pronounced in the future due to increases in GHG emissions and urbanization related to future socioeconomic scenarios (Gridden et al., 2019; IPCC, 2021). AHR is an important factor for urban climate, and it is also an important aspect of urbanization. The possible climatic effects and influence mechanism of AHR due to global energy consumption in SWC have not yet attracted sufficient research attention. In this study, the climatic effects and physical feedbacks of AHR due to global energy consumption during the boreal summer in SWC are examined with the Community Earth System Model version 1.0 (CESM1).
2 Data, method and model experiments
2.1 The distribution of AHR in southwest China
Based on energy consumption statistics (http://www.stats.gov.cn/tjsj/ndsj/) from the National Bureau of Statistics of China (NBSC), the regional mean AHR flux in SWC from 1992 to 2020 and the annual mean distributions of the AHR flux in SWC from 1992 to 2013 are shown in Figure 1. According to the NBSC, the economy, population and energy consumption of SWC increased sharply between 1992 and 2020. As shown in Figure 1, the regional mean AHR flux in SWC grew quickly from 0.06 W m-2 in 1992 to a maximum of 0.37 Wm-2 in 2019 before decreasing in 2020 due to the COVID-19 pandemic.
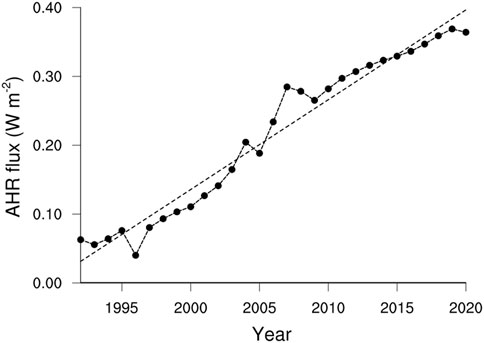
FIGURE 1. The mean flux of AHR in Southwest China from 1992 to 2020 with the trend fitting line (unit: W m-2), and the slope value k = 0.013.
The energy consumption statistics from the NBSC and National Oceanic and Atmospheric Administration (NOAA) Defense Meteorological Satellite Program (DMSP) Operational Linescan System (OLS) data (http://ngdc.noaa.gov/eog/dmsp/downloadV4composites.html) from 1992 to 2013 are applied in this study to derive the distribution of the AHR flux in SWC, as described in Chen et al. (2014). The AHR flux depends on the correlation between the DMSP/OLS data and energy consumption (Chen et al., 2012), and the errors in the results obtained using the DMSP/OLS data are generally within 12% (Chen et al., 2015). This approach has been proven to be useful for estimating the ARH distribution. The AHR distributions in SWC are shown in Figure 2, where the AHR distribution is closely related to the degree of regional development. Regions with high AHR fluxes are located in large cities, such as Chengdu, Chongqing, Kunming and Guiyang, where the AHR fluxes exceed 10 Wm-2. The changes in AHR from 1992 to 2013, shown in Figure 2, demonstrate that the climate effects of AHR are strongly correlated with economic and urban development in SWC. From Figure 2, we see that the effect of AHR becomes increasingly severe in conjunction with economic and urban development in SWC. This finding indicates that the climatic effects of AHR should not be ignored when considering climate change in SWC.
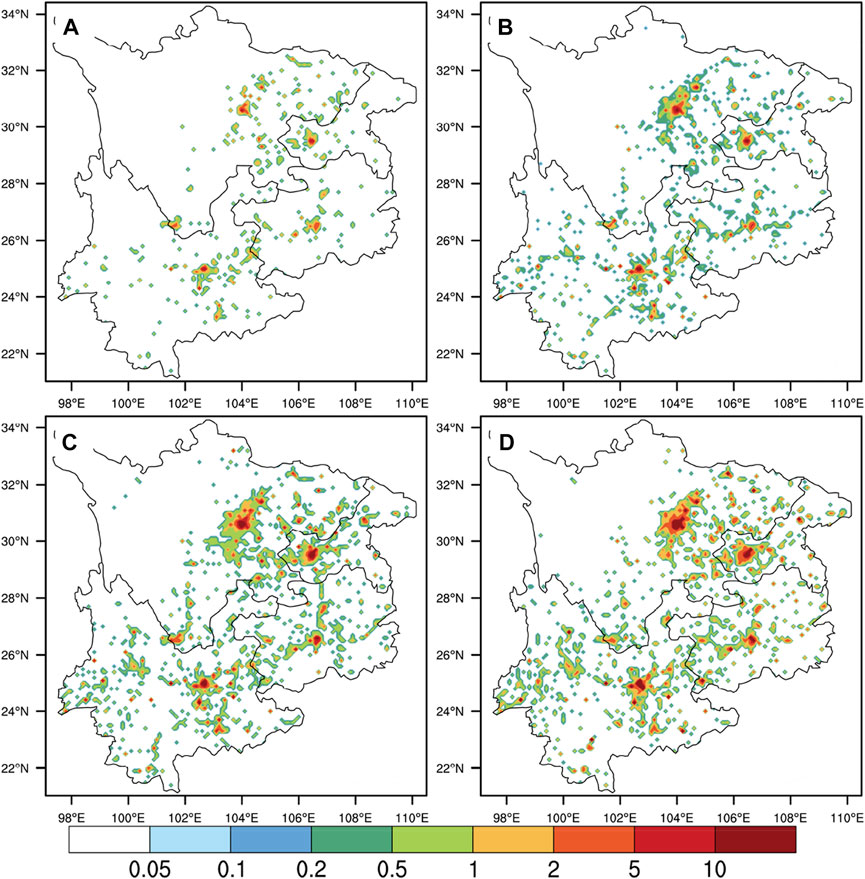
FIGURE 2. The distribution of AHR in southwest China (unit: W m-2, resolution: 0.1°× 0.1°): (A) in the year 1992; (B) in the year 2000; (C) in the year 2005; (D) in the year 2013.
2.2 Data and model experiments
AHR has non-negligible regional to global scale climatic effects (Zhang et al., 2013; Chen et al., 2016; Chen et al., 2019), which cannot be fully simulated in a regional model due to its boundary limitation. Therefore, a global climate model, the Community Earth System Model (CESM) version 1.0.6 (CESM1.0.6, http://www.cesm.ucar.edu/models/cesm1.0/), was adopted in this study. Here, global AHR was taken as the sensible heat by adding the excessive vertical flux convergence in the boundary layer near the surface (Zheng et al., 2013; Chen et al., 2019). The CESM1.0.6 model was run using the component set F_AMIP_CAM5 (http://www.cesm.ucar.edu/models/cesm1.0/cesm/cesm_doc_1 _0_4/x42. html#ccsm_ component_sets). A coupled land–atmosphere mode with prescribed monthly sea surface temperature and sea ice coverage (Hurrell et al., 2008) was configured following the Atmospheric Model Intercomparison Project (AMIP) protocols (Gates, 1992). The Community Land Model version 4 (CLM4) (Oleson et al., 2010) was coupled with CAM5 to represent the evolution of land surface boundary conditions, except that the anthropogenic heat module in CLM4 was turned off. Historical greenhouse gas concentrations, anthropogenic aerosol and precursor gas emissions were specified following Kay et al. (2015). Two sets of experiments are performed: one set of experiments considers AHR in the surface energy balance within the model, while the other set of experiments does not consider AHR. Each set of experiments includes five ensemble simulations to account for the internal variability by perturbing the initial air temperature as done by Kay et al. (2015). The horizontal resolution is 0.9° × 1.25 with 30 vertical levels, and the time step is 30 min.
The Climate Research Unit (CRU) gridded Time Series version 3.10 (TS3.10) dataset from monthly observations at meteorological stations is applied in this study to verify the reliability of the modeling results. Climate anomalies were interpolated into 0.5* 0.5 grid cells over all land areas worldwide (excluding Antarctica). The monthly climatological variables of the dataset include mean temperature, minimum and maximum temperatures, precipitation total, vapor pressure and cloud cover (Harris et al., 2014). Previous research has used the CRU grid dataset to study the climate and factors of temperature, precipitation and evaporation in different regions of China, and the results prove that the CRU grid dataset has high reliability in revealing the characteristics of climate change (Zhang et al., 2018). To evaluate the ability of CESM1 to simulate the mean horizontal wind, monthly variables from the fifth generation European Center for Medium Weather Forecasting (ECMWF) atmospheric reanalysis of the global climate (ERA5) are also utilized. They cover a span of from 1992 to 2013 with a 0.25* 0.25 horizontal resolution.
3 Modeling results analysis
The climatic effects and feedbacks of AHR due to energy consumption in the boreal summer (June-July-August, JJA) in SWC are analyzed with CESM1 in this study. The modeling results for the summer 2-m air mean temperature (T2m), average minimum 2-m air temperature (Tmin) and average maximum 2-m air temperature (Tmax) in SWC from 1992 to 2013 are reliable based on their comparison with Climatic Research Unit (CRU) data (as shown in Figures 3–5). The impacts of AHR on 2-m air temperatures in the summer in SWC are shown in Figure 6.
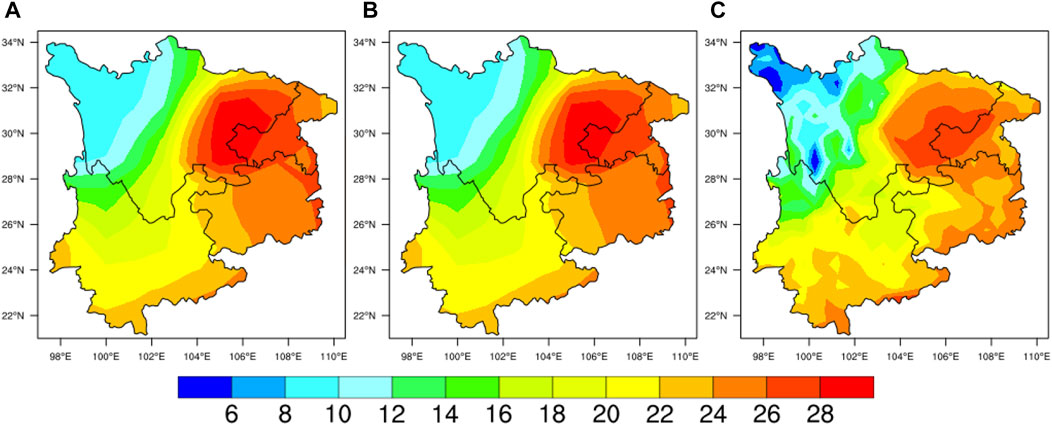
FIGURE 3. The 2-m air mean temperature (T2m) results for southwest China from 1992 to 2013 in the boreal summer (JJA): (A) control modeling results without AHR; (B) modeling results with AHR; (C) CRU data results (unit: °C).
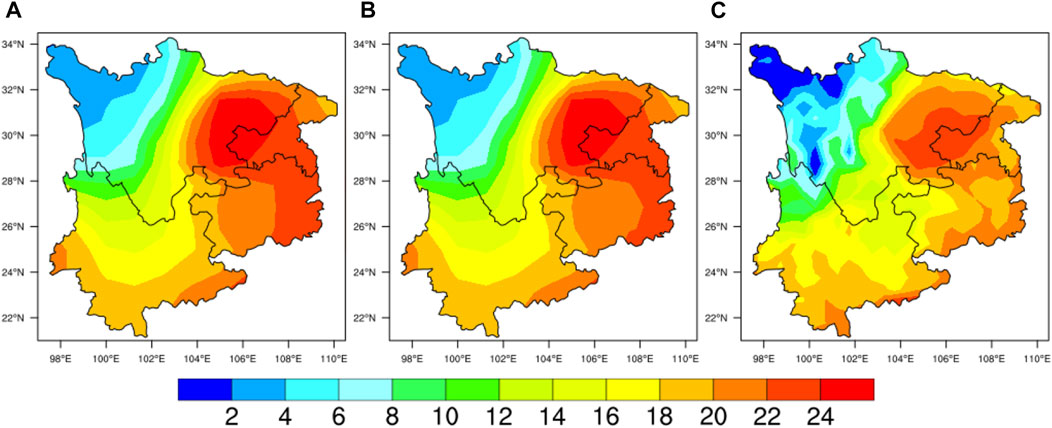
FIGURE 4. The 2-m air minimum temperature (Tmin) results for southwest China from 1992 to 2013 in the boreal summer (JJA): (A) control modeling results without AHR; (B) modeling results with AHR; (C) CRU data results (unit: °C).
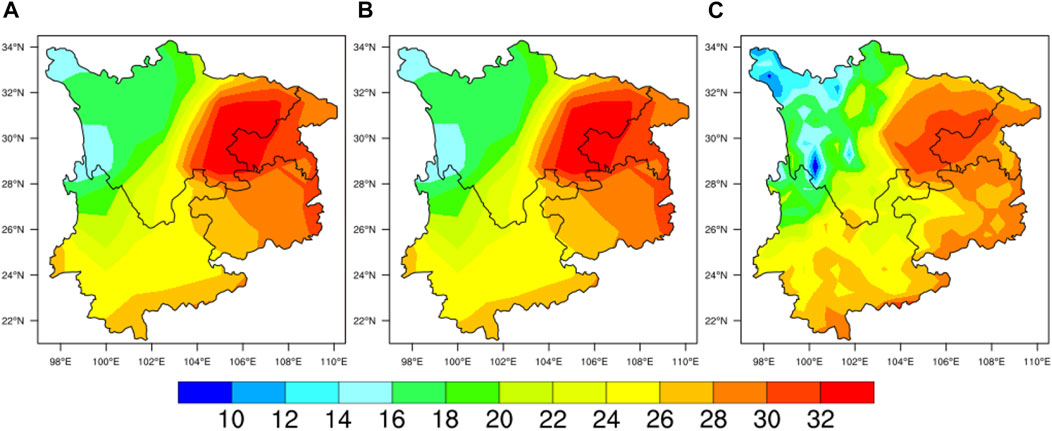
FIGURE 5. The 2-m air maximum temperature (Tmax) results for southwest China from 1992 to 2013 in the boreal summer (JJA): (A) control modeling results without AHR; (B) modeling results with AHR; (C) CRU data results (unit: °C).
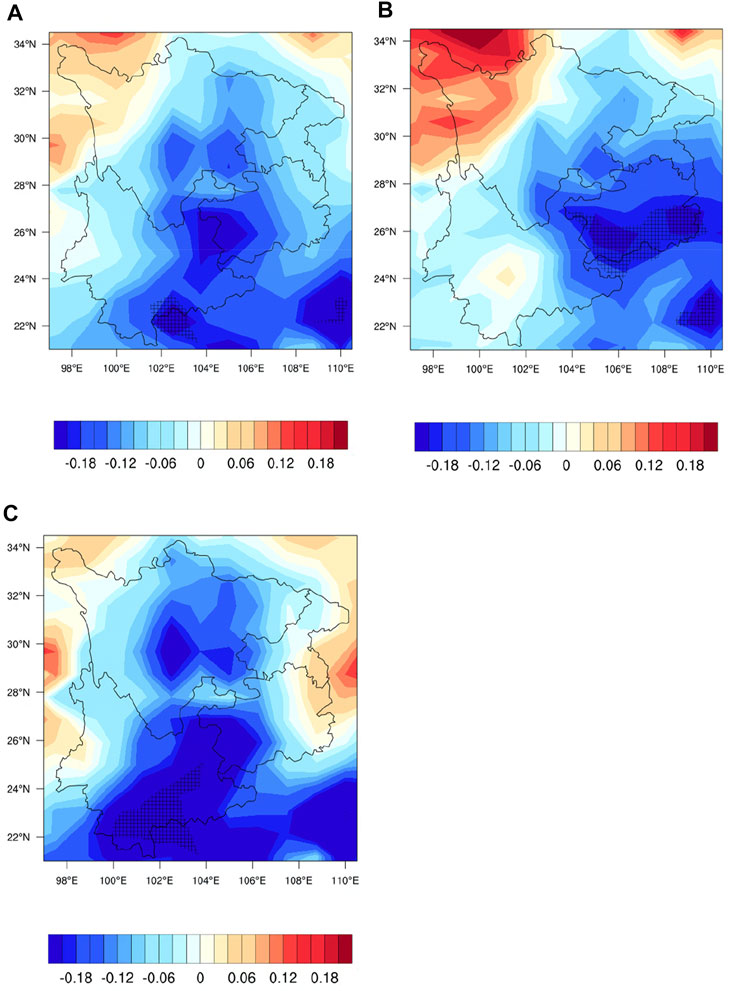
FIGURE 6. Effects of AHR on southwest China in the boreal summer (JJA) from 1992 to 2013: (A) 2-m air temperature (T2m, unit: °C); (B) minimum 2-m air temperature (Tmin, unit: °C); (C) maximum 2-m air temperature (Tmax, unit: °C). The plus signs in figure denote the change is statistically significant at the 0.05 level.
The modeling results show that AHR generally decreases the temperature in SWC during boreal summer. AHR decreases the average T2m in SWC by 0.10 ± 0.01 K (1σ uncertainty), average Tmax by 0.12 ± 0.02 K (1σ uncertainty), and average Tmin by 0.07 ± 0.01 K (1σ uncertainty). In Figure 6, the T2m obviously decreases in western GZ and eastern and southern YN. Overall, Tmin decreases in GZ, while Tmax decreases significantly in central SC, western GZ and southern YN. The possible physical mechanisms for the decreasing temperatures in SWC as a result of AHR are discussed below.
The modeling results for the mean horizontal wind (U, V) at 500 hPa from 1992 to 2013 are reliable based on their comparison with ERA5 data (as shown in Figure 7). The effects on the horizontal winds at 500 hPa due to AHR in the boreal summer (JJA) from 1992 to 2013 are shown in Figure 8. These results show that AHR can affect large-scale atmospheric circulation, as AHR can have a significant effect on the atmospheric vertical movement (Chen et al., 2019) due to its uneven heating effect, which can further impact large-scale atmospheric circulation. We examine the effects of AHR on the Asian summer monsoon (ASM). Webster and Yang (1992) defined an ASM index based on the vertical shear of the June–August (JJA) 850- and 200-hPa zonal winds averaged over 0°–20°N, 40–110°E, which is known as the Webster–Yang index (WYI) (Webster and Yang, 1992). The WYI depicts the thermally driven nature of the monsoon and has been widely used in both ASM research and operational prediction of the ASM. Figure 9 shows the effects of AHR on the WYI from 1992 to 2013. The results in Figure 9 show that the WYI is enhanced due to the heating effect of AHR. Previous research showed that AHR can impact on the thermal and dynamic feedback on atmospheric circulation and affect global surface temperature (Zhang et al., 2013; Chen et al., 2014; Chen et al., 2019). The possible mechanism for the impact of AHR on the ASM is that AHR, resulting from global energy consumption, can affect the land-sea surface temperature gradient, which can further impact the Asian summer monsoon.
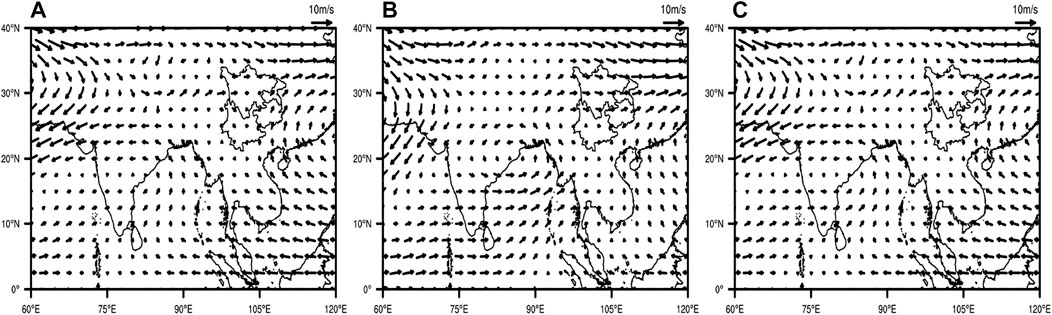
FIGURE 7. Mean horizontal wind (U, V) at 500 hPa (unit: m s−1) in the domain (0–40°N, 60°E−120°E) from 1992 to 2013 in the boreal summer (JJA): (A) CESM1 control modeling results without AHR; (B) ERA5 data results; (C) CESM1 modeling results with AHR.
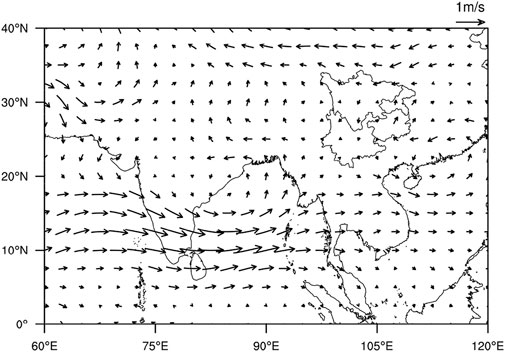
FIGURE 8. Effects of AHR on the horizontal wind (U, V) at 500 hPa in the boreal summer (JJA) from 1992 to 2013 (unit: m s−1).
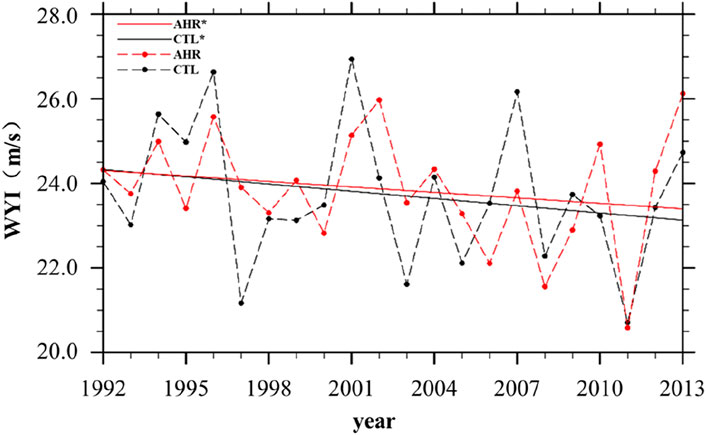
FIGURE 9. The Webster–Yang index (WYI) (unit: m s−1) changes from 1992 to 2013 in the boreal summer (JJA): the dashed line in black: CESM1 control modeling results (CTL) without AHR, the solid lines in black (CTL*) is the linear fitting results for control modeling results without AHR; the dashed line in red: CESM1 modeling results considering AHR (AHR), the solid lines in red (AHR*) is the linear fitting results for modeling results considering AHR.
The possible mechanism underlying the climate impact of AHR in SWC, as shown in Figure 10, is explored in this study. Figure 10A shows the impact of AHR on the relative humidity (RH) in SWC. RH increases in most regions of SWC, most obviously in northern Sichuan and southern Yunnan, while RH decreases in Guizhou and Chongqing. As shown by the results in Figures 8, 9, the large-scale atmospheric circulation is affected by AHR, which will bring more water vapor into SWC during boreal summer. The lower-troposphere stability (LTS) is defined as the difference between the potential temperature of the free troposphere (700 hPa) and the surface (LTS = θ700—θ0), which is strongly correlated with the low cloud fraction (Wood and Bretherton, 2006) and further affects the energy balance at the surface (Boucher et al., 2013). Figure 10B shows the impact of AHR on the LTS in SWC. According to the modeling results, LTS increases in most regions of SWC, with the greatest increase occurring in Sichuan and Yunnan. Based on the relationship between LTS and low clouds (Wood and Bretherton, 2006), the effect of AHR on the low cloud fraction is shown in Figure 10C. The low cloud fraction clearly increases in Sichuan, Yunnan and Guizhou. Low clouds are essential for energy balance in the Earth-atmosphere system (Boucher et al., 2013). The impact of AHR on the SWC summer surface energy balance is analyzed. The net surface shortwave (solar) radiation flux during summer in SWC changes due to the heating effect of AHR, as shown in Figure 10D. The regions where the net shortwave radiation fluxes at the surface clearly decrease are consistent with the regions where the low cloud fraction increases, as shown in Figure 10C. These results suggest that AHR exerts dynamic thermal impacts on the lower troposphere and affects the surface energy balance in SWC. The results suggest a possible AHR feedback mechanism in SWC during the summer. The AHR impacts the thermal and dynamic feedbacks within large-scale atmospheric circulation, which will transport more water vapor to SWC. AHR increases the LTS in SWC. Since the LTS strongly influences low clouds (Wood and Bretherton, 2006), an increased LTS causes an increase in the low cloud fraction, leading to less solar shortwave radiation reaching the surface in SWC. The decreased surface shortwave radiation will further decrease the surface air temperature. The results suggest a possible AHR feedback mechanism that contributes to cooler and wetter summers in SWC.
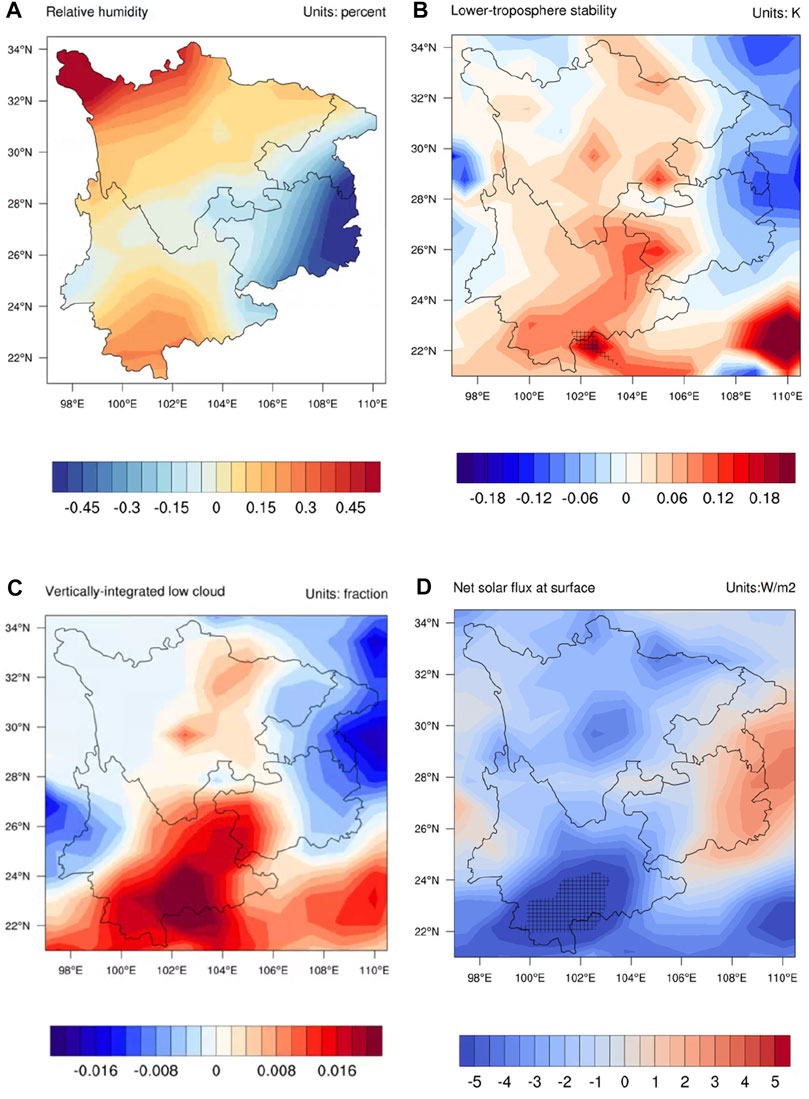
FIGURE 10. Effects of AHR on southwest China in the boreal summer (JJA) from 1992 to 2013: (A) relative humidity (unit: percent); (B) lower-troposphere stability (LTS) (unit: K); (C) low clouds (unit: fraction); (D) the net solar radiation flux at the surface (unit: W m-2). The plus signs in figure denote the change is statistically significant at the 0.05 level.
4 Discussion
Previous studies have found that the heating effect caused by AHR has an important impact on the local urban climate. Compared with the regional climatic effects on urban climate caused by AHR, the climate effect and physical feedback of AHR due to the energy consumption in SWC during the boreal summer, as addressed in this study, have not received adequate research attention. Possible physical mechanisms and climatic feedbacks are found in this study: AHR increases the sensible heat near the surface, increases the turbulence and affects the energy balance at the surface, which can impact the thermodynamic structure of the atmosphere and the stability of the lower troposphere. This will affect the low cloud fraction and solar shortwave radiation reaching the surface and the temperatures in SWC, which will impact on the large-scale atmospheric circulation further.
Considering the unique nature of the climatic effects caused by AHR, analysis of these effects should not be restricted to regional scales. Because of the boundary limitations of the regional models used in AHR research, we used global simulations from the CESM. We ran two sets of experiments with CESM1 to account for the internal variability. However, this research is not without limitations. The anthropogenic heat could be in the form of ground heat flux, sensible heat flux, or longwave radiation, and the proper proportion among them for the parameterization of AHR in climate models remains uncertain. In this research, we consider AHR to be sensible heat in the climate model, which is consistent with Zhang et al. (2013) and Chen et al. (2019). However, possible modeling errors may exist.
5 Conclusion
This study examined the climatic effects and feedbacks of AHR due to energy consumption in SWC during boreal summer using CESM1. AHR decreases the average 2-m air temperature, as well as the minimum and maximum air temperatures over SWC. The modeling results show that AHR can impact large-scale atmospheric circulation in SWC and the energy balance at the surface in SWC. The modeling results suggest a possible AHR feedback mechanism in the summer in SWC. AHR impacts the thermal and dynamic feedbacks within large-scale atmospheric circulation, which will transport more water vapor to SWC. AHR increases the LTS and the low cloud fraction, leading to less solar shortwave radiation reaching the surface in SWC. The decreased surface shortwave radiation further decreases the surface air temperature as a result. The results suggest a possible feedback mechanism that contributes to cooler and wetter summers in SWC due to AHR. AHR is a non-negligible factor that impacts summer temperatures in SWC.
The energy balance in the Earth-atmosphere system is an essential aspect of climate change. AHR is a direct and external energy source that acts on the Earth-atmosphere system and impacts the energy balance of the Earth’s surface. AHR impacts the large-scale atmospheric circulation and transport of water vapor to SWC. These results suggest that AHR is a non-negligible factor for climate change in SWC. AHR acts as a potential impact factor for the extreme weather and climate in SWC under future scenarios, which should receive more research focus. This study improves our understanding of the possible physical mechanisms by which human activities influence the climate in SWC, and they should not be ignored in future research.
Data availability statement
The original contributions presented in the study are included in the article/Supplementary Material, further inquiries can be directed to the corresponding author.
Author contributions
BC developed the idea. MZ and JZ contribute equally. BC, MZ, JZ, YZ, and ML performed most of the analysis. BC drafted the manuscript. All the authors discussed the concepts and edited the manuscript.
Funding
This work was supported by the National Natural Science Foundation of China (Nos. 42065009, 42175046, 42061052, and 42005023), the Natural Science Foundation of Yunnan Province (No. 201901BB050045), and the National Key Research and Development Program of China (2018YFA0606004).
Acknowledgments
The authors would like to give thanks to Tianjun Zhou at Institute of Atmospheric Physics, Chinese Academy of Sciences for his advice.
Conflict of interest
The authors declare that the research was conducted in the absence of any commercial or financial relationships that could be construed as a potential conflict of interest.
Publisher’s note
All claims expressed in this article are solely those of the authors and do not necessarily represent those of their affiliated organizations, or those of the publisher, the editors and the reviewers. Any product that may be evaluated in this article, or claim that may be made by its manufacturer, is not guaranteed or endorsed by the publisher.
Supplementary material
The Supplementary Material for this article can be found online at: https://www.frontiersin.org/articles/10.3389/feart.2023.1077939/full#supplementary-material
References
Boucher, O., Randall, D., and Artaxo, P. (2013). “Clouds and aerosols,” in Climate change 2013: The physical science basis. Contribution of working group I to the fifth assessment report of the intergovernmental panel on climate change. Editors T. F. Stocker, D. Qin, G.-K. Plattner, M. Tignor, S. K. Allen, J. Boschunget al. (Cambridge, England UK: Cambridge University Press).
Chen, B., Dong, L., Liu, X., Shi, G. Y., Nakajima, T., Chen, L., et al. (2016). Exploring the possible effect of anthropogenic heat release due to global energy consumption upon global climate: A climate model study. Int. J. Climatol. 36, 4790–4796. doi:10.1002/joc.4669
Chen, B., Dong, L., Shi, G. Y., Li, L., and Chen, L. (2014). Anthropogenic heat release: Estimation of global distribution and possible climate effect. J. Meteor. Soc. Jpn. 92A, 157–165. doi:10.2151/jmsj.2014-A10
Chen, B., Shi, G. Y., Wang, B., Tan, S., and Zhao, J. Q. (2012). Estimation of the anthropogenic heat release distribution in China from 1992 to 2009. J. Meteor Res. 26 (4), 507–515. doi:10.1007/s13351-012-0409-y
Chen, B., Wu, C., Liu, X., Chen, L., Wu, J., Yang, H., et al. (2019). Seasonal climatic effects and feedbacks of anthropogenic heat release due to global energy consumption with CAM5. Clim. Dyn. 52, 6377–6390. doi:10.1007/s00382-018-4528-1
Chen, B., Zhao, J. Q., Chen, L., and Shi, G. Y. (2015). Reply to the Comments of F. Fujibe on “Anthropogenic Heat Release: Estimation of Global Distribution and Possible Climate Effect” by Chen, B. et al. J. Meteorological Soc. Jpn. 93, 505–508. doi:10.2151/jmsj.2015-028
Chen, H., and Sun, J. (2015). Changes in climate extreme events in China associated with warming. Int. J. Climatol. 35, 2735–2751. doi:10.1002/joc.4168
Chen, L., and Frauenfeld, O. W. (2016). Impacts of urbanization on future climate in China. Clim. Dyn. 47, 345–357. doi:10.1007/s00382-015-2840-6
Chen, W., Wang, L., Xue, Y., and Sun, S. (2009). Variabilities of the spring river runoff system in East China and their relations to precipitation and sea surface temperature. Int. J. Climatol. 29, 1381–1394. doi:10.1002/joc.1785
Chen, Y., and Zhai, P. (2013). Persistent extreme precipitation events in China during 1951-2010. Clim. Res. 57, 143–155. doi:10.3354/cr01171
Fan, J., Rosenfeld, D., Yang, Y., Zhao, C., Leung, L. R., and Li, Z. (2015). Substantial contribution of anthropogenic air pollution to catastrophic floods in Southwest China. Geophys. Res. Lett. 42, 6066–6075. doi:10.1002/2015GL064479
Forster, P., Ramaswamy, V., Artaxo, P., Berntsen, T., Betts, R., Fahey, D. W., et al. (2007). “Changes in atmospheric constituents and in radiative forcing,” in Climate change 2007: The physical science basis. Contribution of working group I to the fourth assessment report of the intergovernmental panel on climate change. Editors S. Solomon, D. Qin, M. Manning, Z. Chen, M. Marquis, K. B. Averytet al. (Cambridge England UK: Cambridge University Press).
Gates, W. L. (1992). Amip: The atmospheric model Intercomparison Project. Bull. Amer. Meteor. Soc. 73, 1962–1970. doi:10.1175/1520-0477.(1992)073<1962:ATAMIP>2.0
Gridden, M. J., Smith, S., Fujimori, S., Luderer, G., and Kriegler, E. (2019). Global emissions pathways under different socioeconomic scenarios for use in CMIP6: A dataset of harmonized emissions trajectories through the end of the century. Geosci. Model Dev. 12, 1443–1475. doi:10.5194/gmd-12-1443-2019
Hansen, J., Nazarenko, L., Reudy, R., Sato, M., Willis, J., Del Genio, A., et al. (2005). Earth’s energy imbalance: Confirmation and implications. Science 39, 1431–1435. doi:10.1126/science.1110252
Harris, I., Jones, P. D., Osborn, T. J., and Lister, D. (2014). Updated high-resolution grids of monthly climatic observations–the CRU TS3. 10 Dataset. Int. J. Climatol. 34 (3), 623–642. doi:10.1002/joc.3711
Kay, J. E., Deser, C., Phillips, A., Mai, A., Hannay, C., Strand, G., et al. (2015). The community Earth system model (cesm) large ensemble Project: A community resource for studying climate change in the presence of internal climate variability. Bull. Amer. Meteor. Soc. 96, 1333–1349. doi:10.1175/BAMS-D-13-00255.1
Liu, X., Yang, Q., and Cheng, B. (2009). Study on anomalies of atmospheric circulation and water vapor field of the heavy drought in Sichuan-Chongqing region in midsummer 2006. Meteor. Mon. 35, 27–34. (in Chinese).
Li, X., Zhou, W., Chen, D., and Song, J. (2014). Water vapor transport and moisture budget over eastern China: Remote forcing from the two types of El Niño. J. Clim. 27, 8778–8792. doi:10.1175/JCLI-D-14-00049.1
Liu, M., Xu, X., Sun, Y. A., Wang, K., Liu, W., and Zhang, X. (2014). Is southwestern China experiencing more frequent precipitation extremes? Environ. Res. Lett. 9, 064002. doi:10.1088/1748-9326/9/6/064002
Lu, E., Luo, Y., Zhang, R., Wu, Q., and Liu, L. (2011). Regional atmospheric anomalies responsible for the 2009–2010 severe drought in China. J. Geophys. Res. 116, D21114. doi:10.1029/2011JD015706
Ma, Z. F., Liu, J., Zhang, S. Q., Wen-Xiu, C., and Shu-Qun, Y. (2013). Observed climate changes in Southwest China during 1961–2010. Adv. Clim. Change Res. 4, 30–40. doi:10.3724/SP.J.1248.2013.030
Oleson, K. W., Lawrence, D. M., Gordon, B., Flanner, M. G., Kluzek, E., Peter, J., et al. (2010) Technical description of version 4.0 of the community land model (CLM), NCAR tech. Note NCAR/TN-461+STR.
Peterson, T. R., Richard, R. H., Robert, H., Kaiser, D. P., Brooks, H., Diffenbaugh, N. S., et al. (2013). Monitoring and understanding changes in heat waves, cold waves, floods, and droughts in the United States: State of knowledge. Bull. Amer. Meteor. Soc. 94, 821–834. doi:10.1175/BAMS-D-12-00066.1
IPCC (2007). “Climate change 2007: The physical science basis,” in Contribution of working group I to the fourth assessment report of the intergovernmental panel on climate change. Editors S. Solomon, D. Qin, M. Manning, Z. Chen, M. Marquis, K. B. Averytet al. (Cambridge, England UK: Cambridge University Press).
Wang, D., Jiang, P., Wang, G., and Wang, D. (2015). Urban extent enhances extreme precipitation over the Pearl River Delta, China. Atmos. Sci. Lett 16, 310–317. doi:10.1002/asl2.559
Webster, P. J., and Yang, S. (1992). Monsoon and ENSO: Selectively interactive systems. Quart. J. Roy. Meteor. Soc. 118, 877–926. doi:10.1002/qj.49711850705
Wood, R., and Bretherton, C. S. (2006). On the relationship between stratiform low cloud cover and lower-tropospheric stability. J. Clim. 19, 6425–6432. doi:10.1175/jcli3988.1
Zaksek, K., and Ostir, K. (2012). Downscaling land surface temperature for urban heat island diurnal cycle analysis. Remote Sens. Environ. 117, 114–124. doi:10.1016/j.rse.2011.05.027
IPCC (2021). “Climate change 2021: The physical science basis,” in Contribution of working group I to the sixth assessment report of the intergovernmental panel on climate change [Masson-Delmotte V. Editors P. Zhai, A. Pirani, S. L. Connors, C. Péan, S. Berger, N. Caudet al. (Cambridge, England UK: Cambridge University Press).
Zhai, P., Zhang, X., Wan, H., and Pan, X. (2005). Trends in total precipitation and frequency of daily precipitation extremes over China. J. Clim. 18, 1096–1108. doi:10.1175/JCLI-3318.1
Zhang, D., Song, X. F., Zhang, Y. H., Yang, L. H., and Yang, S. T. (2018). Variation characteristics of precipitation over weihe river basin based on CRU grid dataset during last 100 years. J. Arid. Land Res. Environ. (in Chin. 32, 142–148.
Zhang, G., Cai, M., and Hu, A. (2013). Energy consumption and the unexplained winter warming over northern Asia and North America. Nat. Clim. Change 3, 466–470. doi:10.1038/nclimate1803
Zhang, W., Wan, Y., Ren, J., and Fu, R. (2011). Analysis of water vapor transportation and its influence on rainfall anomaly in Yunnan during autumn and winter of 2009. Plateau Meteor 30, 1534–1542. (in Chinese).
Zhao, L., Michael, O., Zhu, Q., Baldwin, J. W., Ebi, K. L., Bou-Zeid, E., et al. (2018). Interactions between urban heat islands and heat waves. Environ. Res. Lett. 13, 034003. doi:10.1088/1748-9326/aa9f73
Keywords: anthropogenic heat release, atmospheric circulation, energy balance, cooler and wetter summer, Southwest China
Citation: Zhang M, Zhao J, Chen B, Zheng Y, Lu M, Wu X, Yang H, Liu X, Wu C, Zhang M, Zhao X, Jinlin Z, Luo T, Fan W and Shi G (2023) Anthropogenic heat due to energy consumption contributes to cooler and wetter summers in Southwest China. Front. Earth Sci. 11:1077939. doi: 10.3389/feart.2023.1077939
Received: 23 October 2022; Accepted: 24 February 2023;
Published: 10 March 2023.
Edited by:
Kondapalli Niranjan Kumar, National Centre for Medium Range Weather Forecasting, IndiaReviewed by:
Yuanjian Yang, Nanjing University of Information Science and Technology, ChinaAhmed Kenawy, Mansoura University, Egypt
Copyright © 2023 Zhang, Zhao, Chen, Zheng, Lu, Wu, Yang, Liu, Wu, Zhang, Zhao, Jinlin, Luo, Fan and Shi. This is an open-access article distributed under the terms of the Creative Commons Attribution License (CC BY). The use, distribution or reproduction in other forums is permitted, provided the original author(s) and the copyright owner(s) are credited and that the original publication in this journal is cited, in accordance with accepted academic practice. No use, distribution or reproduction is permitted which does not comply with these terms.
*Correspondence: Bing Chen, chenbing@ynu.edu.cn