- 1School of Resources Environment and Safety Engineering, Hunan University of Science and Technology, Xiangtan, China
- 2School of Energy and Electromechanical Engineering, Hunan University of Humanities, Science and Technology, Loudi, China
The air-lifting pump has the advantages of low cost, strong reliability, simple structure and convenient maintenance, especially in the fields of deep-sea mining, oilfield mining and mineral mining under complex geological conditions. The construction technology and air intake mode not only play a decisive role in the performance of the air-lifting pump, but also greatly hinder the engineering application of the air-lifting pump. Here, the air-lifting pump is taken as the research object, and the river sand is used as the experimental transport medium. The particle concentration-efficiency model is established based on the mechanical energy conservation theorem. The working performance characteristic parameters of the air-lifting pump under different construction technologies are obtained by a three-axis motion control system. On this basis, the working performance characteristic parameters of the air-lifting pump under different intake modes are obtained by changing the intake mode. The results show that the particle concentration-efficiency model can well describe the efficiency of lifting solid particles by the air-lifting pump. When the sand depth is less than 200 mm, the concentration and efficiency of solid particles transported by the air-lifting pump in the horizontal movement construction are significantly better than those in the fixed position construction. In the same construction technology, the solid particle concentration of the air-lifting pump in uniform intake mode is better than that in non-uniform intake mode, while the transportation efficiency of the air-lifting pump in non-uniform intake mode is better than that in uniform intake mode. The research results of this paper can provide an important theoretical reference value for the air-lifting pump in practical engineering applications.
Introduction
With the continuous development of the economy, the demand for mineral resources is increasing, and the available land resources are very limited. And a large number of mineral resources are affected by the complex hydrogeological conditions of rock mass structure (Liu et al., 2022), (Zhao et al., 2019), (Zhao et al., 2021), underground mineral resources with large reserves cannot be effectively mined and utilized, which is the high cost, poor safety and reliability of traditional mining methods. The best way to solve this problem is to use the air-lifting pump (also known as the air-lifting system or air-lifting) by borehole hydraulic mining (Tang et al., 2013), (Hu et al., 2012a), (Tang et al., 2012).
The air-lifting pump is a kind of fluid machinery that uses compressed air as a powerful medium to transport liquid phases such as oil and dangerous chemicals, or liquid-solid mixtures such as pulp and sediment. Compared with the traditional mining method, the air-lifting pump has the advantages of simple structure, low cost, high safety and reliability, and less environmental pollution, so it has become the focus of research. Enany (Enany et al., 2021) conducted an experimental study on the air-lifting pump under different air intake and submerged rates, and the results showed that the optimal value of the liquid phase efficiency of the air-lifting pump under the same intake air amount is between 0.6 and 0.75 for the submerged rate. Catrawedarma I. (Catrawedarma and DeendarliantoIndarto, 2021). experimented with identifying the gas-liquid two-phase flow structure in the air-lifting pump system by using the pressure difference signal. The pressure difference data in the lifting riser were analyzed by using the probability density function (PDF), power spectral density function (PSDF), Kolmogorov entropy and discrete wavelet transform (DWT). The results show that the liquid phase motion mechanism in the air-lifting riser can be divided into three regions with the change of the intake air volume. Then, Catrawedarma I (CatrawedarmaResnaraditya et al., 2021) used wavelet transform to analyze the pressure difference signal of the gas-liquid-solid three-phase flow structure in the air-lifting riser, so as to determine the wavelet energy distribution. The experimental results show that the wavelet analysis can well distinguish the flow pattern in the air-lifting riser. Shimizu (Shimizu and Shu, 2021) used water and two kinds of high viscosity shear slurry as the transport media to conduct experimental research on the air-lifting pump under different air intakes. The results show that the flow rate of the lift water is the same as the flow rate of the lift slurry when the air intake is high. Fayyadh (Fayyadh et al., 2020) performed an experimental study on the performance of the air-lifting pump under different air intake and submerged rates. The results show that both the air intake and submerged rate will have a significant impact on the performance of the air-lifting pump. Parviz E (Parviz et al., 2022) performed an experimental study on the optimal conditions of lifting solid particles by the air-lifting pump under different submerged rates. The results show that under the same air intake when the flow pattern is churn flow and the submerged rate is close to 0.89, the particle velocity reaches the maximum.
The above research explored the working characteristics of the air-lifting pump based on experimental methods, which is beneficial to guide the structural design of the air-lifting pump to a certain extent. However, most of these studies focus on the performance of the air-lifting pump under different air intake and submerged rates, and rarely involve the influence of different construction technologies and intake methods on the transport performance of the air-lifting pump. Therefore, this paper takes the construction technology in the project as the research object, and intends to use river sand as the experimental transport medium. The transport characteristic parameters of the air-lifting pump under different construction technologies are expected to be obtained by the three-axis motion control system. On this basis, the transport characteristic parameters of the air-lifting pump under different intake modes are expected to be obtained by changing the intake mode.
Theoretical model
The gas-liquid-solid three-phase flow in the air-lifting pump is extremely complex, and efficiency is an important indicator to measure and evaluate the performance of the air-lifting pump. Here, it can be defined as the ratio of the solid particle’s energy contained at the outlet of the air-lifting riser to the input gas energy at the inlet hole. In order to obtain a more accurate gas-liquid-solid three-phase flow efficiency of the air-lifting pump, this paper modifies the gas-liquid two-phase flow efficiency model derived by Hu Dong et al. (Hu et al., 2012b), and the derivation process is as follows.
Figure 1 shows the schematic diagram of the air-lifting. The compressed air generated by the air compressor enters the air-lifting riser through the inlet hole to fully mix with the liquid phase, which forms a large volume of bullet-shaped bubbles under the action of the liquid phase, so as to promote the fluid upward movement in the front of the bubble, and then the gas-liquid mixed fluid in the air-lifting riser moves upward. When the drag force caused by the movement of the gas-liquid mixed fluid reaches the suspension critical point of the solid particles, the solid particles are forced to leave the deposit and migrate to the air-lifting riser. Therefore, it can be seen that the factors affecting the efficiency of the air-lifting pump include the gas phase velocity, the liquid phase velocity, the energy contained in the solid particles and the roughness of the riser wall. In order to facilitate the analysis, assuming that the riser wall is smooth, that is, ignoring the friction loss of the riser wall, and the gas temperature is constant, the energy of the solid particles at the outlet of the riser is the potential energy of the solid particles at the outlet. The kinetic energy and buoyancy work can be obtained from the following:
In the formula,
In the formula,
In the formula,
The energy of the input gas of the air lifting system can be obtained from the following formula:
In the formula,
Therefore, the lifting efficiency of the air lift pump is:
Experimental device and method
The experimental device
In order to facilitate experimental research, an experimental platform is built as shown in Figure 2. The experimental platform is mainly composed of the compressed air transport system, the air-lifting pump system, the sampling device and the transport pipeline system. Among them, the compressed air transport system mainly includes the air compressor, pressure gauge energy storage tank, gas flow meter and control valve switch. The air-lifting system mainly includes an air-lifting pump, the inner diameter of a 100 mm lifting pipe, the length, width and height of a 3,850 × 2,520 × 2,970 mm experimental pool respectively, river sand with a diameter of 2.5 mm and density of 2,534 kg/m3, and operating system controlling XYZ three-axis movement. The sampling device mainly includes a sampling tank, stopwatch, measuring cylinder and electronic scale; transport pipelines mainly include conveying compressed air pipes and water pipes to the experimental pool.
The experimental method
Before the experiment, the simulated mineral particles were covered with river sand at the bottom of the whole experiment pool, and the horizontal instrument was used to keep the sand layer flat and the thickness uniform. Then, fill the experimental pool with water that can reach the preset submergence rate value. After that, turn on the air compressor and the control valve switch. When the indication value of the pressure gauge on the energy storage tank and the display value of the air flowmeter was kept stable, the submergence rate and air intake were kept unchanged. At the same time, operate the XYZ three-axis movement system to move the air-lifting pump to a predetermined position and start sampling in two ways: fixed position and horizontal movement. After that, the air intake mode is changed into the uniform distribution of air intake holes and the non-uniform distribution of air intake holes (As shown in Figure 3), repeat the above work, and dry and measure the solid particles after each sampling.
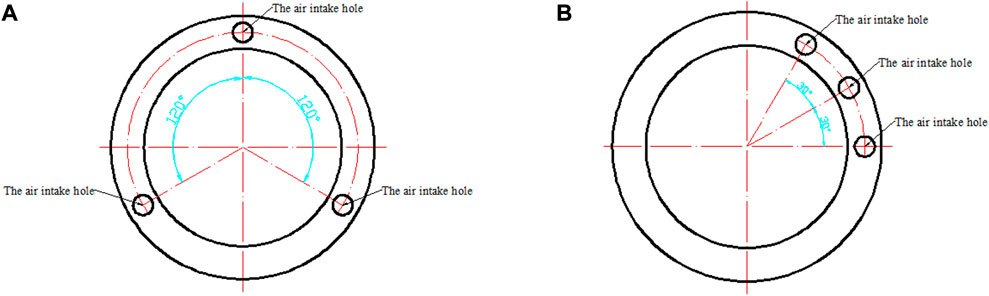
FIGURE 3. The air intake mode. (A) The uniform distribution of air intake holes (B) The non-uniform distribution of air intake holes.
Experimental results and analysis
Effect of different construction technology on the air-lifting pump transport characteristics
In order to analyze the influence of construction technology on the transport characteristics of the air-lifting pump in practical engineering, river sand is used as the experimental transport medium. The sampling is carried out by the three-axis movement operating system (moving along the horizontal direction and fixed position), combined with the construction technology in practical engineering. The influence of different construction technologies on the transport characteristics of the air-lifting pump under different sand depths of the same working conditions (the same air intake and the same submergence rate) is analyzed. The variation law shown in Figure 4 is obtained.
It can be seen from Figure 4 that under different construction methods, the particle concentration increases gradually with the increase of sand depth, and the concentration of the horizontal movement construction method is higher than that of the fixed position when the sand depth is less than 200 mm, while it is the opposite when the sand depth is higher than 200 mm. The reason may be that with the increase of sand depth, the depth of the air-lifting pump bottom suction mouth buried in the sand layer increases continuously, and the pressure gradient at the suction mouth edge increases. There is the liquid phase forms a vortex to entrain a large number of solid particles into the air-lifting pump, and they begin to move upward along the riser until the outlet end. In addition, in the upward movement process, the gas flow rate is affected by the resistance such as wall friction, which makes the flow rate in the central area of the riser much larger than that in the side wall area, causing the particles in the side wall area to slide along the side wall until they are mixed with the particles below. After the liquid phase is mixed with the liquid below, part of the liquid phase flow out of the pump body, then forms the particle plug in the riser. At this time, the gas energy in the riser is not enough to push the particle plug upward. Due to the gas phase being the continuous supplement, the rapid expansion of the gas phase at a moment pushes the particle plug to move rapidly upward to the riser outlet, showing a pulse phenomenon, which is more intense with the sand depth increases, so that the concentration increases with the sand depth increases.
In the fixed position construction method, when the depth is less than 200 mm, due to the effect of pressure gradient force, the range of liquid phase forming vortex entrainment solid particles is limited, and the solid particles at the bottom of the suction mouth are relatively unable to be supplemented in time. Only a conical pit is formed at the suction mouth. In the horizontal movement construction method, although the range of liquid phase entrainment solid particles is limited, the solid particles can be supplemented in time with the movement of the position. Therefore, in this depth range, the amount of solid particles is less than that in the horizontal movement construction method, and the concentration is lower. When the depth is greater than 200 mm, the depth range of liquid involving solid particles begins to move downward, which leads to the collapse of surrounding solid particles and the continuous inflow of solid particles into the suction port, so that the concentration increase of lifting solid particles. However, in the horizontal movement construction mode, the range of the liquid phase involving solid particles is shifted with the air-lifting pump, which does not cause the surrounding solid particles to collapse obviously. The amount of solid particles entering the air-lifting pump is reduced compared with the fixed position construction method, so the particle concentration is lower than the fixed position construction method.
Effect of different air intake modes on air-lifting pump transport characteristics
In order to investigate the influence of air intake mode on the transport characteristics of the air-lifting pump, the variation law of transport particle concentration characteristics of the air-lifting pump with sand depth under different air intake modes of the same working condition is obtained, as shown in the following Figure 5.
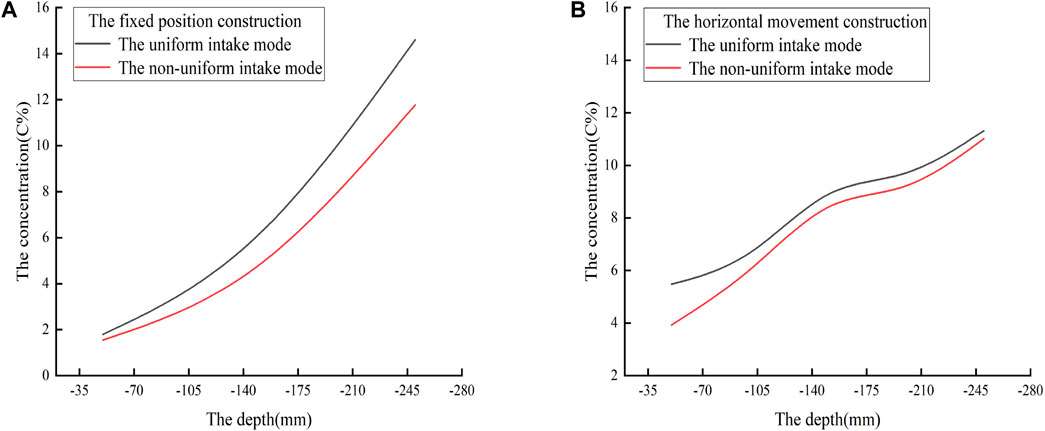
FIGURE 5. Variation of particle concentration with sand depth under different intake modes. (A) The fixed position construction method. (B) The horizontal movement construction method.
It can be seen from Figure 5 that the particle concentration under the two intake modes increases monotonically with the increase of depth, and no matter what kind of construction method, the concentration of particles transported by the air-lifting pump with a uniform arrangement of air holes is generally higher than that with the uneven arrangement of air holes. In the air intake method with A uniform arrangement of air holes, due to the 120-degree interval between the two air holes, the gas phase with the high-speed flow is sprayed out from the air hole, and the entrainment phenomenon is formed near the two sides of each air hole, which causes the liquid stirring particles and entraps the particles into the air-lifting pump. Subsequently, under the action of the air-lifting pump, the gas phase is wrapped around the solid particles in the form of small bubbles, so that a large density difference is formed in the air-lifting riser to achieve the purpose of lifting solid particles. At this time, the flow pattern of the air-lifting riser is the bubble flow, in which solid particles are most beneficial to the lifting. These results are similar to Grzegorz’s (Ligus et al., 2019), (Wang et al., 2021), (Barkai et al., 2019), (Alasadi and Habeeb, 2017) study on the air-lifting pump.
In the air intake method with the non-uniform arrangement of air holes, that is, the air intake method of adjacent air holes, the interval between the air holes is small, and it is easy to form air film to prevent the liquid phase from entering the air riser, which does not have the conditions for the formation of entrainment phenomenon. Only the entrainment of the liquid phase is formed in the vicinity of the lateral air holes, which reduces the scope of entrainment and further weakens the stirring effect of the liquid phase on particles so that less amount of particles wrapped liquid phase entrapped into the air-lifting pump. In addition, in this air intake method with the non-uniform arrangement of air holes, the contact between gas and liquid-solid phase is not sufficient, and the phenomenon of small bubbles entrapping solid particles cannot be formed (bubble flow), which does not form a large density difference to promote the upward movement of the solid particles. Therefore, the concentration of the output solid particles of the air-lifting pump is the lowest in general by using the air intake method with the non-uniform arrangement of air holes.
Effect of different construction techniques on the air-lifting pump efficiency
In order to study the influence of different construction techniques on the efficiency of the air-lifting pump, the characteristic parameters of the air-lifting pump under different sand depths of construction techniques are inset into the efficiency Eq. 11, in which the efficiency curves of the air-lifting pump are obtained under different construction techniques methods of the same working condition as follows:
It can be seen from Figure 6 that under different construction methods, the lifting efficiency of the air-lifting pump increases gradually with the sand depth increase. When the sand depth is less than 200 mm, the air-lifting pump lifting efficiency of the horizontal movement construction method is significantly higher than that of the fixed position. When the sand depth is more than 200 mm, the opposite is true. The efficiency of the air-lifting pump in the two construction methods increases under the same working conditions (the same air intake and the same submergence rate). The reason may be that, on the one hand, the increase in the sand depth leads to the pressure gradient increase at the suction edge of the air-lifting pump bottom, which causes the solid particles around the suction to continuously influx into the suction and output through the air-lifting riser under the action of the air-lifting pump, which greatly increases the output (Zhao et al., 2019; Zhao et al., 2018; Zhao et al., 2020). On the other hand, the air intake remains unchanged, indicating that the entrainment caused by the gas phase remains unchanged, and the total energy input remains unchanged. Therefore, under different construction methods, the solid particles’ output increases (namely, the effective power increases) with the sand depth increase, and the total input energy remains unchanged, so that the efficiency is the increase of the air-lifting pump. This also shows that the depth from the air-lifting pump bottom suction mouth to the sand layer (namely, the pressure gradient) is one of the main factors affecting the efficiency of the air-lifting pump.
When the depth is less than 200 mm, the air intake remains unchanged during the operation of the two construction methods (namely, the total energy input remains unchanged), and the range of solid particles entrainment by the liquid phase forms a vortex is limited, which is not enough to cause the collapse of the solid particles around the suction mouth. This easily leads to a low concentration of solid particles under the fixed position construction method due to the failure to be supplemented in time. However, in the horizontal movement construction method, the range of liquid phase forming vortex entraining solid particles moves with the air-lifting pump movement, and the solid particles can be supplemented in time. Therefore, the air-lifting pump efficiency of the horizontal movement construction method is higher than that of the fixed position construction method. When the depth is greater than 200 mm, the total energy input remains unchanged. The fixed position construction method causes the range of liquid phase forming vortex to entrain solid particles to move downward. Under the pressure gradient force action, the solid particles around the suction mouth begin to collapse. There are continuous solid particles entering the air-lifting pump bottom suction mouth, and a large number of solid particles are transported out. However, the horizontal movement construction method is affected by the movement of the air-lifting pump, and the solid particles around the suction mouth are still not collapsed. The amount of solid particles entering the suction mouth is relatively small, and solid particles transported out are less. Therefore, the air-lifting pump efficiency of the fixed position construction method is higher than that of the horizontal movement construction method.
Effect of different intake modes on the air-lifting pump efficiency
In order to analyze the influence of different air intake modes on the air-lifting pump efficiency, under different air intake modes of the same working condition, the characteristic parameters of the air-lifting pump changing with the sand depth are obtained, and the air-lifting pump efficiency is obtained according to Eq. 11 as shown in the following Figure 7.
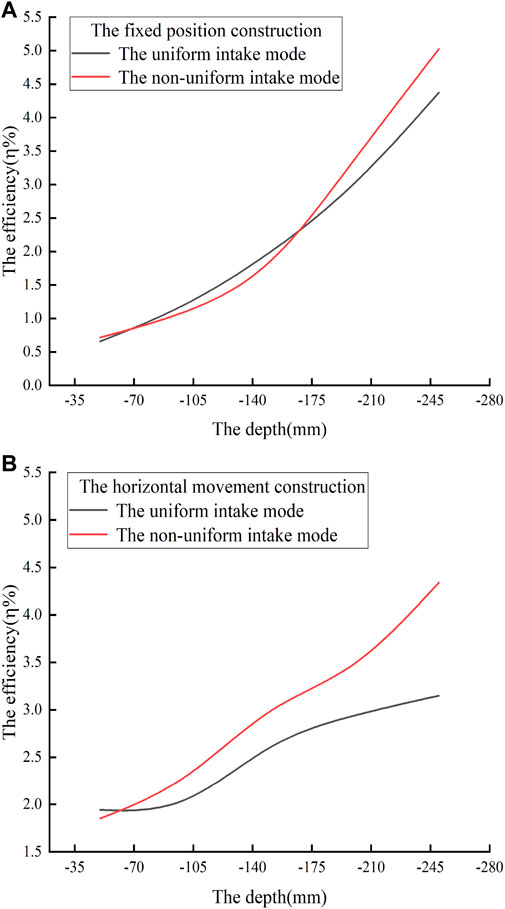
FIGURE 7. Variation of the air-lifting pump efficiency with sand depth under different intake modes. (A) The fixed position construction method. (B) The horizontal movement construction method.
It can be seen from the shape of the curve in Figure 7 that the air-lifting pump lifting efficiency under the two intake modes increases monotonically with the increase of sand depth. This indicates that the pressure gradient caused by the depth of the sand layer is the main factor affecting the air-lifting pump efficiency when the input of the gas phase energy is the same under the two intake modes (Wang et al., 2019; Wang et al., 2022). With the increase of sand depth, the pressure difference around the suction mouth of the air-lifting pump increases gradually, forcing the solid particles around the suction mouth of the air-lifting pump to continuously aggregate to the suction mouth, which the solid particles move upward along the air-lifting riser until the outlet end of the lifting riser under the action of the liquid phase. Due to the input of the gas phase energy remaining the same, this leads to a monotonically increasing trend in the lifting efficiency of the air-lifting pump with the increase of the depth under the two air intake modes.
It can also be seen from Figure 7 that the air-lifting pump efficiency growth rate of the non-uniform intake mode is faster than that of the uniform intake method under the two construction modes. This phenomenon may be related to resistance along the way. When the air intake mode is the uniform intake, there is a gap between airflow ejected from the adjacent air holes in the air-lifting pump, which makes contact between the gas phase and the liquid-solid two phases more fully, and the intense energy conversion occurs, forcing the liquid phase to carry solid particles to move upward along the riser wall. In the process of solid particles’ upward movement, the efficiency of the air-lifting pump is greatly reduced due to the influence of the resistance loss along the riser wall. When the air intake mode is non-uniform air intake, there is an overlap area between the airflow ejected from the adjacent air holes in the air-lifting pump, and it is not sufficient to contact with the liquid-solid two phases. And the energy conversion is usually completed in an instant, so that part of the energy is converted into the internal energy of the liquid-solid two phases, which reduces the amount of lifting solid particles. However, the non-uniform air intake mode is easy to form a gas film near the riser wall, which greatly reduces the resistance loss along the riser wall during the upward transportation of solid particles. Therefore, the lifting efficiency growth rate of the uniform intake mode is slower than that of the non-uniform intake mode.
Conclusion
1) Based on the mechanical energy conservation theorem, a particle concentration-efficiency model is established, which can well describe the efficiency of the air-lifting pump to lift solid particles.
2) Increasing the depth of the sand layer, the concentration and efficiency of the solid particles transported will increase accordingly by the air-lifting pump under different construction techniques, and when the depth of the sand layer is less than 200 mm, the concentration and efficiency of solid particles transported by the air-lifting pump of the horizontal movement construction are better than that of the fixed position construction.
3) In the same construction techniques, the concentration of solid particles transported by the air-lifting pump in uniform intake mode is better than that in non-uniform intake mode, while the transportation efficiency of the air-lifting pump in non-uniform intake mode is better than that in uniform intake mode.
Data availability statement
The original contributions presented in the study are included in the article/supplementary material, further inquiries can be directed to the corresponding author.
Author contributions
YZ was writing-review and editing of the whole manuscript; XW wrote the manuscript; CT, DH and PL designed and analyzed the experiments; MX assisted with structure and language of the manuscript; All authors read and approved the final manuscript.
Funding
This research is supported by the National Natural Science Foundation of China (No. 51774131), (No.52006061), and the Hunan Province Key Area R&D Program (2020NK 2063).
Conflict of interest
The authors declare that the research was conducted in the absence of any commercial or financial relationships that could be construed as a potential conflict of interest.
Publisher’s note
All claims expressed in this article are solely those of the authors and do not necessarily represent those of their affiliated organizations, or those of the publisher, the editors and the reviewers. Any product that may be evaluated in this article, or claim that may be made by its manufacturer, is not guaranteed or endorsed by the publisher.
References
Alasadi, A. A. M. H., and Habeeb, A. K. (2017). Experimental and numerical simulation of an airlift pump with conventional and modified air injection device[J]. Eng. J. 23 (2), 62–82.
Barkai, Allatchi Hassan, Hajem, M. E., Lacassagne, T., and Champagne, J-Y. (2019). Experimental study of a gas–liquid flow in vacuum air-lift column using an optical Bi-probe[J]. Fluids 4 (2), 80.
Catrawedarma, I. G. N. B., Deendarlianto , I., and Indarto, A. (2021). Statistical characterization of flow structure of air-water two-phase flow in airlift pump–bubble generator system[J]. Int. J. Multiph. Flow, 138. 103596. doi:10.1016/j.ijmultiphaseflow.2021.103596
CatrawedarmaResnaraditya, I. G. N. B., Aghid, Fadliqa, and Deendarlianto, Indarto (2021). Statistical characterization of the flow structure of air-water-solid particles three-phase flow in the airlift pump-bubble generator system[J]. Flow Meas. Instrum., 82. 102062. doi:10.1016/j.flowmeasinst.2021.102062
Enany, Parviz, Shevchenko, Oleksandr, and Drebenstedt, Carsten (2021). Experimental evaluation of airlift performance for vertical pumping of water in underground mines[J]. Mine Water Environ. 40 (4), 1–10.
Fayyadh, E. M., Mahdi, N., and Mohammed, A. F. (2020). The effect of air injection system on airlift pump performance. FME Trans. 48 (4), 800–807. doi:10.5937/fme2004800f
Hu, Dong, Tang, C. L., and Zhang, F. H. (2012). The effect of air injector on the performance of airlift. Adv. Mat. Res. 1791 (516-517), 1022–1027. doi:10.4028/www.scientific.net/amr.516-517.1022
Hu, Dong, Tang, C. L., Cai, S. P., and Zhang, F. H. (2012). The effect of air injection method on the airlift pump performance. J. Fluids Eng. 134 (11). doi:10.1115/1.4007592
Ligus, Grzegorz, Zajac, D., Masiukiewicz, M., and Anweiler, S. (2019). A new method of selecting the airlift pump optimum efficiency at low submergence ratios with the use of image analysis. Energies 12 (4), 735. doi:10.3390/en12040735
Liu, J., Zhao, Y., Tan, T., Zhang, L., Zhu, S., and Xu, F. (2022). Evolution and modeling of mine water inflow and hazard characteristics in southern coalfields of China: A case of meitanba mine. Int. J. Min. Sci. Technol. 32 (3), 513–524. doi:10.1016/j.ijmst.2022.04.001
Parviz, E., Oleksandr, S., and Carsten, D. (2022). Particle transport velocity in vertical transmission with an airlift pump[J]. Fluids 7 (3), 95.
Shimizu, K., and Shu, T. (2021). Study on the performance of a 200 m airlift pump for water and highly-viscous shear-thinning slurry. Int. J. Multiph. Flow 142, 103726. doi:10.1016/j.ijmultiphaseflow.2021.103726
Tang, Chuanlin, Hu, Dong, and Yang, Lin (2012). Experimental study of performance characteristics of an air-lift for conveying river sand[J]. J. Basic Sci. Eng. 20 (3), 440–445.
Tang, Chuanlin, Hu, Dong, and Zhang, Fenghua (2013). Effect of air injector on the airlift performance in air-water-solid three-phase flow[J]. J. Energy Eng. 140 (1), 04013006.
Wang, Shu, Hu, D., Yang, F., Lin, P., and Tang, C. (2021). Pressure drop characteristics of airlift in transporting particles. Powder Technol. 394, 270–277. doi:10.1016/j.powtec.2021.08.021
Wang, S., Li, X., Yao, J., Gong, F., Li, X., Du, K., et al. (2019). Experimental investigation of rock breakage by a conical pick and its application to non-explosive mechanized mining in deep hard rock. Int. J. Rock Mech. Mining Sci. 122, 104063. doi:10.1016/j.ijrmms.2019.104063
Wang, S., Sun, L., Li, X., Zhou, J., Du, K., and Wang, S. (2022). Experimental investigation and theoretical analysis of indentations on cuboid hard rock using a conical pick under uniaxial lateral stress. Geomech. Geophys. Geo-Energy Geo-Resour. 8 (1), 34.
Zhao, Yanlin, Wang, Yixian, and Tang, Liming (2019). The compressive-shear fracture strength of rock containing water based on Druker-Prager failure criterion. Arab. J. Geosci. 12, 452. doi:10.1007/s12517-019-4628-1
Zhao, Y. L., Liu, Q., Zhang, C., Liao, J., Lin, H., and Wang, Y. (2021). Coupled seepage-damage effect in fractured rock masses: Model development and a case study. Int. J. Rock Mech. Min. Sci. 144, 104822. doi:10.1016/j.ijrmms.2021.104822
Zhao, Y., Wang, Y., Wang, W., Tang, L., and Liu, Q. (2019). Modeling of rheological fracture behavior of rock cracks subjected to hydraulic pressure and far field stresses. Theoret. App. Fract. Mech. 101, 59–66.
Zhao, Y., Zhang, L., Wang, W., Wan, W., and Ma, W. (2018). Separation of elastoviscoplastic strains of rock and a nonlinear creep model. Int. J. Geomech. 18 (1), 04017129.
Keywords: air-lifting pump, efficiency, three-phase flow, construction technology, air intake method
Citation: Zhao Y-L, Wang X-G, Tang C-L, Hu D, Lin P and Xia M-L (2023) Effect of construction technology on air-lifting pump mining. Front. Earth Sci. 10:1005600. doi: 10.3389/feart.2022.1005600
Received: 28 July 2022; Accepted: 21 September 2022;
Published: 10 January 2023.
Edited by:
Shaofeng Wang, Central South University, ChinaReviewed by:
Xianyang Qiu, Central South University, ChinaLan Cui, Institute of Rock and Soil Mechanics, Chinese Academy of Sciences (CAS), China
Copyright © 2023 Zhao, Wang, Tang, Hu, Lin and Xia. This is an open-access article distributed under the terms of the Creative Commons Attribution License (CC BY). The use, distribution or reproduction in other forums is permitted, provided the original author(s) and the copyright owner(s) are credited and that the original publication in this journal is cited, in accordance with accepted academic practice. No use, distribution or reproduction is permitted which does not comply with these terms.
*Correspondence: Xia-Guang Wang, happyapples8888@163.com