- 1Department of Internal Medicine, Geisinger Wyoming Valley Hospital, Wilkes Barre, PA, United States
- 2Department of CV Imaging, Courtois CMR Research Group at the Research Institute of the McGill University Health Centre, Montreal, Canada
- 3Division of Experimental Medicine, Departments of Medicine and Diagnostic Radiology, Universitaire de Santé McGill Site Glen, Montreal, QC, Canada
Introduction
Myocardial injury has been reported in patients with acute or previous COVID-19 and in relation to the SARS-CoV-2 vaccine (1). While infrequent, cardiac involvement has been associated with an impaired outcome (2). Several different mechanisms have been proposed in the literature for each entity. As the prime non-invasive diagnostic procedure for myocardial inflammation, cardiovascular magnetic resonance imaging (CMR) has played an essential role during the pandemic in diagnosing associated myocardial inflammation, having a similar diagnostic accuracy compared to invasive endomyocardial biopsy (3). There has been an extensive body of literature on CMR findings in all these syndromes that showed different patterns of myocardial pathology such as global myocardial edema, irreversible myocardial injury such as necrosis and scarring, as well as pericardial effusion. Several such studies have reported similarities of such patterns to those found in myocardial inflammation triggered by other viruses (4, 5), albeit with some difference in some studies (6, 7).
We aim to summarize the CMR findings and discuss areas of similarity.
Myocardial injury in acute COVID-19
Prevalence
Though the most virulent manifestation of COVID-19 is acute respiratory distress syndrome, cardiac injury reflected through elevated troponin concentrations has been increasingly reported (8, 9). Various studies have reported an overall prevalence of acute myocardial injury ranging 5%–38% (10). One study described myocardial injury prevalence of 36% with significant association with death and a higher troponin-I associated with a higher risk of death (11).
Clinical presentation
Myocardial injury is typically characterized by chest pain, dyspnea and palpitations, with or without elevated cardiac biomarkers such as high-sensitivity cardiac troponin (hsTn) and/or creatinine kinase MB (12). Notably, electrocardiographic and echocardiographic findings in patients with COVID-19-related myocardial injury can be normal (13).
Proposed mechanisms
Several etiologies have been proposed to cause myocardial injury in COVID-19, including direct viral injury, pre-existing chronic injury, supply-demand imbalance, multi-organ failure (14–16), stress-induced cardiomyopathy Takotsubo, or plaque rupture with ischemic events (16–18).
Infection with SARS-CoV-2 impairs endothelial function and hemostatic balance, increases thrombin activity, reduces plasminogen activator inhibitor-1 activity and accelerates the production of fibrin degradation products (19). Endothelial inflammation with vascular edema and disseminated intravascular coagulation may lead to microvascular dysfunction. These factors may exacerbate myocardial oxygen supply/demand imbalance due to hypoxia and tachycardia (20).
Diagnostic utility of CMR
CMR is unique in its capability to non-invasively characterize myocardial inflammation and injury. It is considered the gold standard imaging modality in diagnosing myocarditis (21). The CMR criteria for assessing myocardial inflammation (“Lake Louise Criteria”) include a high myocardial signal intensity in T2-weighted images indicating myocardial edema, increased early uptake of gadolinium with a high signal intensity in T1-weighted images (early gadolinium enhancement) as an indicator for hyperemia and capillary leakage, and a high signal intensity in late gadolinium enhancement (LGE) demonstrating necrosis or scar. Based on these criteria, CMR can identify myocardial damage with a sensitivity of 78% and specificity of 88% [AUC 0.83 (0.79–0.86)] (22). In 2018, T1 mapping and T2 mapping were included (3), leading to a similar or higher accuracy (75%–91.8%) as the original criteria (23, 24). The Lake Louise Criteria have significant value in guiding patient management (25) and are also part of the recommendations for using CMR in COVID-19 (26).
Pertinent CMR findings
Myocardial necrosis and scar assessed by LGE imaging are associated with an impaired outcome, while LGE-negative patients have an excellent prognosis, regardless of symptoms (27, 28). Myocarditis-induced injury is typically localized sub-epicardial and/or intramurally, frequently in the basal to mid-inferolateral segments (29). Myocardial edema as an invariable component of active inflammation can be visualized by native T2 mapping. This technique can verify active inflammation with a sensitivity of 89%. Native T1 mapping is also sensitive to myocardial injury and edema and thus can be used to visualize tissue inflammation (30).
Myocardial injury in post-COVID syndrome
Prevalence
While most patients with mild-moderate disease recover within 2 weeks, there is a percentage of the population which do not return to baseline even after 14–21 days (31). The prevalence of post-COVID-19 myocardial injury remains uncertain, with reported data ranging from 0.5% to 20% (32–35). Recent data indicate that as much as 7% of COVID-19-related mortalities may be attributable to myocarditis (36).
Clinical presentation
The Royal College of General Practitioners in the UK has divided COVID-19 infection into: (1) Acute COVID-19 (within 4 weeks after disease onset), (2) Ongoing Symptomatic COVID-19 (persisting for 4–12 weeks), and (3) Post-COVID-19 Syndrome (or Long COVID), if persistent after 12 weeks (32). Persistent chest pain has been reported in up to 20% of COVID-19 survivors over a 2-month follow-up and recurrent palpitations in up to 9% after 6 months, with shortness of breath reported in up to 8% (37).
Proposed mechanisms for post-COVID-19 syndromes
The underlying pathophysiology is poorly understood, including a systemic inflammatory response with cytokine storm, counter-balanced by a compensatory anti-inflammatory response syndrome to prevent widespread multiorgan dysfunction (38) as well as virus persistence and latent virus reactivation of SARS-COV-2. For long COVID, adrenal insufficiency and cerebral dysregulation have been discussed.
Diagnostic utility of CMR
CMR in COVID-19-related cardiac injury is highly mandated and rapidly growing, due to its ability not only to diagnose acute and chronic sequelae of myocardial inflammation but also to provide a more detailed understanding of the pathophysiological phenomenon behind cardiac involvement and differentiate them from other various pathological etiologies (39).
In patients that have recovered from COVID-19, the reported incidence of myocardial inflammation varies greatly, ranging from 2.4% to 30% (2), in one controversial paper even 78%. Non-ischemic scar patterns among participants suggest a non-ischemic cause of cardiac injury (40, 41). One study reported lower left ventricular ejection fraction, higher left ventricular volumes, higher native T1 values consistent with myocardial edema or interstitial fibrosis (42), and high T2 values suggesting myocardial edema (43) when compared with healthy control subjects and risk factor–matched control subjects. These findings correlated with higher levels of hsTn and active lymphocytic inflammation on endomyocardial biopsy specimens (41). Native T1 and T2 mapping provided the best discriminatory ability to detect COVID-19-associated myocardial disease (41).
CMR may play an important role not only during pandemics but also afterwards, as it can detect persistent scar tissue as well as right and left ventricular remodeling (44–46).
SARS-CoV-2 vaccine-related myocarditis
Prevalence and clinical presentation
There is significant epidemiological research and evidence on the reported adverse effects of myocarditis and pericarditis from these vaccines (47–53). The Center of Disease Control (CDC) in the US reported that cases of myocarditis were highest following the second dose of mRNA vaccination in young males (54). Patients usually present with chest pain, shortness of breath, fatigue, or palpitations, in order of prevalence (55, 56), with a temporal relationship to vaccine administration (52, 55, 57). The incidence remains low as seen in multiple studies and vaccine safety reports (28, 59), currently reported as between 0.5 and 2 per 100,000 people (54, 60).
Given the very high absolute number of vaccinations, however, this is and will remain a significant clinical problem.
Proposed mechanisms
Among several proposed mechanisms for this injury are a dysregulated immune response (61), and activation of the complement system via immune complex formation involving anti-spike protein antibodies (62). Another potentially important mechanism proposed is a direct effect of vaccine nanoparticles on the myocardium, with a subsequent complement activation (63).
Diagnostic utility of CMR
Most studies used the updated Lake Louise Criteria to diagnose myocarditis (3). CMR findings when summarized from multiple case series and original research articles report the severity of myocardial injury as mild (6, 52, 53, 57, 64–69). In several CMR studies on cardiac involvement in COVID-19, myocarditis was more prevalent than pericarditis. LGE was predominantly located in the inferior and inferolateral regions, subepicardial pattern and with co-located edema. Fronza et al. (70) and Groschel et al. (6), among others, described the regional distribution pattern of CMR findings, suggesting a basal/lateral predilection for irreversible injury. Fewer articles reported or mentioned pericarditis, which reported a lower prevalence of pericardial involvement with isolated pericarditis or co-located with myocardial LGE.
Discussion
CMR as the non-invasive diagnostic standard in patients with myocardial involvement in systemic disease has revealed evidence for myocardial inflammation and inflammatory injury in patients with acute COVID-19 or thereafter. This is of clinical importance as such injury may occur in less severe cases and still cause persisting symptoms and impair prognosis. In fact, patients with acute, chronic, or post-vaccination disease all appear to present with similar symptoms, most often non-typical chest pain, shortness of breath, palpitations, and fatigue. Of note, most studies have not found a significant relationship between symptoms and CMR findings (6, 71, 72).
In many post-COVID patients, regional or global myocardial edema can be found (32, 73–75). The typical distribution is a non-ischemic pattern with subepicardial scarring inferior and inferolateral, mostly basal and mid-ventricular. A few studies reported intra-myocardial injury in vaccine-related myocarditis compared to the other 2 groups with a more subepicardial pattern (6). Pericardial effusion and pericarditis were less common. In our experience, pericardial effusion, however, is often localized (mostly lateral-basal) and thus may have been missed in previous studies.
Groschel et al. (6) recently compared CMR findings between the three syndromes and found a higher global T1 in the post-COVID group compared to controls, and a higher basal T1 in the post-COVID and COVID vaccination group. The group with myocardial involvement after vaccination also had a higher segmental involvement rate. No statistical difference was found in myocardial T2 and ECV between the groups, but global T2 values between post-COVID and controls were significant. The most common regions were basal and midventricular, lateral, inferior, or inferolateral, with a similar distribution frequency among the groups. The authors speculated that the difference in higher T1 and lower T2 times could be confounded by age, BMI, or weight (76, 77).
Summarizing the literature, there is conflicting data from studies about inflammation and results for myocardial T1 and T2, likely because the duration between illness and CMR was variable, representing different stages of the disease. Furthermore, the composition of the studied patient populations was variable, some with in-patients or ICU admissions, others with out-patient settings. Moreover, symptom burden and proximity of CMR to the presence of symptoms were also variable. Finally, patient demographics and co-morbidities varied widely. The similarity in the scar pattern amongst the three groups (6, 67) as also seen in this case series at our center (67), with co-located edema and LGE, however, suggests a common pathophysiology (Figure 1).
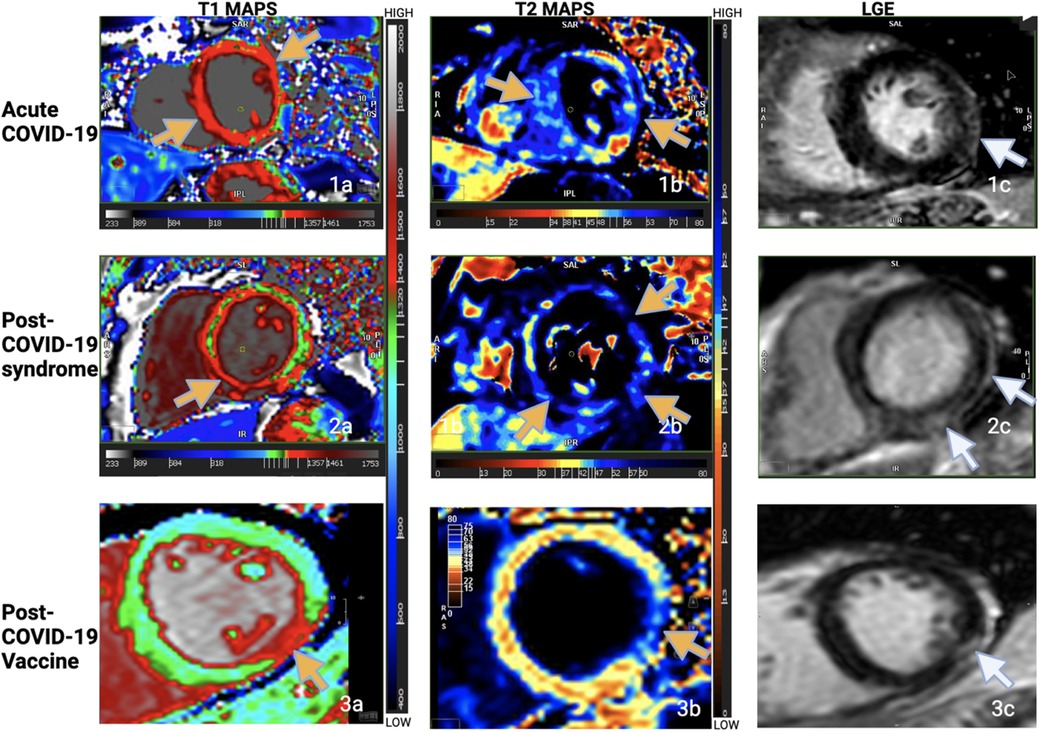
Figure 1. T1 maps, T2 maps, LGE imaging from one patient from each of the groups. Increased T1 and T2 times in the maps as shown by the yellow arrows. Subepicardial lateral or inferolateral scar in the basal or mid slices as shown by the white arrows. Note the pattern and location of myocardial injury in all three groups. (Figure adapted from the abstract poster by Garg et al. “Comparison of CMR Findings in Symptomatic Patients with Different COVID-Related Syndromes”, SCMR 25th annual scientific sessions, 2022. I am an author of this article; the conference grants authors the right to reuse their own figures without permission in future work.).
As explained in the subsections above, the underlying pathophysiology for cardiac injury in the three COVID-related conditions may reflect endotheliitis as direct injury and a systemic cytokine storm as indirect injury in the acute-COVID group, a compensatory anti-inflammatory response in post-COVID, and a dysregulated immune response in the COVID-vaccine group.
Endotheliitis, caused by either direct viral entry or activation of complement cascade may be a potential explanation. This endothelial injury may lead to microvascular dysfunction explaining the symptoms and CMR findings in these patients.
Several studies have studied microvascular dysfunction in acute COVID-19 (78, 79), post COVID (80–82), and post-COVID-vaccine (83) groups. This possibly common pathophysiology needs further exploration with methods such as stress CMR or novel non-invasive techniques like oxygenation-sensitive CMR (84).
The published evidence is still incomplete and subject to several limitations.
In most studies, only symptomatic patients were studied. Biopsy data are scarce, and while CMR is the de facto modality of choice and widely used for diagnosing myocarditis, endomyocardial biopsy (EMB) is still by many considered the gold standard. Most of the time, CMR findings are not corroborated with EMB because of the invasive nature of the latter and current guidelines that restrict it to specific, more severe cases. T1 and T2 values may vary between scanners and are therefore not generalizable between different centers or scanners. Furthermore, as mentioned above, reading CMR images requires expertise and are subject to inter-observer variability, likely explaining the varying prevalence of myocarditis between published cohorts. In athletes, regional fibrosis at the insertion points of the right ventricle, a non-specific finding, may be misinterpreted as inflammatory and lead to an overestimation of the incidence of myocardial involvement. Finally, abnormal LGE represents irreversible injury regardless of its stage. It could be acute, but also reflect such an insult years ago. Therefore, studies confined to LGE or T1 mapping lack information on acute or active inflammation (84).
In summary, CMR studies indicate a similarity of myocardial injury patterns between acute disease, post-COVID, or SARS-CoV-2 vaccination, suggesting a non-specific pathophysiology. It is therefore plausible that most instances of myocardial inflammation stem from generic inflammatory injury rather than direct viral injury. From a clinical standpoint, the exact underlying mechanism, however, is only partly significant. Barring rare cases of viral persistence (which is improbable in COVID), CMR-confirmed myocardial inflammatory injury (necrosis in a non-ischemic regional distribution) accompanied by edema as an active inflammation marker suffices for informing clinical decision-making.
Alas, therapeutic options for acute myocardial inflammation are limited. To combat COVID-related myocardial injury, we must develop better, personalized immune modulation strategies. The ball in the game against COVID-related myocardial injury is in the therapy court.
Author contributions
All authors listed have made a substantial, direct, and intellectual contribution to the work and approved it for publication.
Conflict of interest
The authors declare that the research was conducted in the absence of any commercial or financial relationships that could be construed as a potential conflict of interest.
Publisher's note
All claims expressed in this article are solely those of the authors and do not necessarily represent those of their affiliated organizations, or those of the publisher, the editors and the reviewers. Any product that may be evaluated in this article, or claim that may be made by its manufacturer, is not guaranteed or endorsed by the publisher.
References
1. Heymans S, Cooper LT. Myocarditis after COVID-19 mRNA vaccination: clinical observations and potential mechanisms. Nat Rev Cardiol. (2022) 19(2):75–7. doi: 10.1038/s41569-021-00662-w
2. Ammirati E, Lupi L, Palazzini M, Hendren NS, Grodin JL, Cannistraci CV, et al. Prevalence, characteristics, and outcomes of COVID-19–associated acute myocarditis. Circulation. (2022) 145(15):1123–39. doi: 10.1161/CIRCULATIONAHA.121.056817
3. Ferreira VM, Schulz-Menger J, Holmvang G, Kramer CM, Carbone I, Sechtem U, et al. Cardiovascular magnetic resonance in nonischemic myocardial inflammation: expert recommendations. J Am Coll Cardiol. (2018) 72(24):3158–76. doi: 10.1016/j.jacc.2018.09.072
4. Evertz R, Schulz A, Lange T, Backhaus SJ, Vollmann D, Kowallick JT, et al. Cardiovascular magnetic resonance imaging patterns of acute COVID-19 mRNA vaccine-associated myocarditis in young male patients: a first single-center experience. Front Cardiovasc Med. (2022) 9:4–7. doi: 10.3389/fcvm.2022.965512
5. Kim RJ, Kim HW, Jenista ER, Wendell DC, Azevedo CF, Campbell MJ, et al. Patients with acute myocarditis following mRNA COVID-19 vaccination. JAMA Cardiol. (2021) 6(10):1196–201. doi: 10.1001/jamacardio.2021.2828
6. Gröschel J, Bhoyroo Y, Blaszczyk E, Trauzeddel RF, Viezzer D, Saad H, et al. Different impacts on the heart after COVID-19 infection and vaccination: insights from cardiovascular magnetic resonance. Front Cardiovasc Med. (2022) 9:5–8. doi: 10.3389/fcvm.2022.916922
7. Artico J, Shiwani H, Moon JC, Gorecka M, McCann GP, Roditi G, et al. Myocardial involvement after hospitalization for COVID-19 complicated by troponin elevation: a prospective, multicenter, observational study. Circulation. (2023) 147(5):364–74. doi: 10.1161/CIRCULATIONAHA.122.060632
8. Inciardi RM, Lupi L, Zaccone G, Italia L, Raffo M, Tomasoni D, et al. Cardiac involvement in a patient with coronavirus disease 2019 (COVID-19). JAMA Cardiol. (2020) 5(7):819–24. doi: 10.1001/jamacardio.2020.1096
9. Shi S, Qin M, Shen B, Cai Y, Liu T, Yang F, et al. Association of cardiac injury with mortality in hospitalized patients with COVID-19 in Wuhan, China. JAMA Cardiol. (2020) 5(7):802–10. doi: 10.1001/jamacardio.2020.0950
10. Bavishi C, Bonow RO, Trivedi V, Abbott JD, Messerli FH, Bhatt DL. Special article—acute myocardial injury in patients hospitalized with COVID-19 infection: a review. Prog Cardiovasc Dis. (2020) 63(5):682–9. doi: 10.1016/j.pcad.2020.05.013
11. Lala A, Johnson KW, Januzzi JL, Russak AJ, Paranjpe I, Richter F, et al. Prevalence and impact of myocardial injury in patients hospitalized with COVID-19 infection. J Am Coll Cardiol. (2020) 76(5):533–46. doi: 10.1016/j.jacc.2020.06.007
12. Sandoval Y, Januzzi JL Jr, Jaffe AS. Cardiac troponin for assessment of myocardial injury in COVID-19. J Am Coll Cardiol. (2020) 76(10):1244–58. doi: 10.1016/j.jacc.2020.06.068
13. Deng Q, Hu B, Zhang Y, Wang H, Zhou X, Hu W, et al. Suspected myocardial injury in patients with COVID-19: evidence from front-line clinical observation in Wuhan, China. Int J Cardiol. (2020) 311:116–21. doi: 10.1016/j.ijcard.2020.03.087
14. Shi S, Qin M, Cai Y, Liu T, Shen B, Yang F, et al. Characteristics and clinical significance of myocardial injury in patients with severe coronavirus disease 2019. Eur Heart J. (2020) 41(22):2070–9. doi: 10.1093/eurheartj/ehaa408
15. Vestergaard KR, Jespersen CB, Arnadottir A, Sölétormos G, Schou M, Steffensen R, et al. Prevalence and significance of troponin elevations in patients without acute coronary disease. Int J Cardiol. (2016) 222:819–25. doi: 10.1016/j.ijcard.2016.07.166
16. Zhou F, Yu T, Du R, Fan G, Liu Y, Liu Z, et al. Clinical course and risk factors for mortality of adult inpatients with COVID-19 in Wuhan, China: a retrospective cohort study. Lancet. (2020) 395(10229):1054–62. Available at: https://www.sciencedirect.com/science/article/pii/S014067362030566332171076
17. Shi S, Qin M, Shen B, Cai Y, Liu T, Yang F, et al. Association of cardiac injury with mortality in hospitalized patients with COVID-19 in Wuhan, China. JAMA Cardiol. (2020) 5(7):802–10. doi: 10.1001/jamacardio.2020.0950
18. Meyer P, Degrauwe S, Van Delden C, Ghadri JR, Templin C. Typical takotsubo syndrome triggered by SARS-CoV-2 infection. Eur Heart J. (2020) 41(19):1860. doi: 10.1093/eurheartj/ehaa306
19. Tang N, Li D, Wang X, Sun Z. Abnormal coagulation parameters are associated with poor prognosis in patients with novel coronavirus pneumonia. J Thromb Haemostasis. (2020) 18(4):844–7. doi: 10.1111/jth.14768
20. Schultz MJ, Haitsma JJ, Zhang H, Slutsky AS. Pulmonary coagulopathy as a new target in therapeutic studies of acute lung injury or pneumonia—a review. Crit Care Med. (2006) 34(3):871–7. doi: 10.1097/01.CCM.0000201882.23917.B8
21. Abdel-Aty H, Boyé P, Zagrosek A, Wassmuth R, Kumar A, Messroghli D, et al. Diagnostic performance of cardiovascular magnetic resonance in patients with suspected acute myocarditis: comparison of different approaches. J Am Coll Cardiol. (2005) 45(11):1815–22. doi: 10.1016/j.jacc.2004.11.069
22. Kotanidis CP, Bazmpani MA, Haidich AB, Karvounis C, Antoniades C, Karamitsos TD. Diagnostic accuracy of cardiovascular magnetic resonance in acute myocarditis. JACC Cardiovasc Imaging. (2018) 11(11):1583–90. doi: 10.1016/j.jcmg.2017.12.008
23. Cundari G, Galea N, De Rubeis G, Frustaci A, Cilia F, Mancuso G, et al. Use of the new lake louise criteria improves CMR detection of atypical forms of acute myocarditis. Int J Cardiovasc Imaging. (2021) 37(4):1395–404. doi: 10.1007/s10554-020-02097-9
24. Li S, Duan X, Feng G, Sirajuddin A, Yin G, Zhuang B, et al. Multiparametric cardiovascular magnetic resonance in acute myocarditis: comparison of 2009 and 2018 lake louise criteria with endomyocardial biopsy confirmation. Front Cardiovasc Med. (2021) 8:739892. doi: 10.3389/fcvm.2021.739892
25. Lagan J, Fortune C, Hutchings D, Bradley J, Naish JH, Timoney R, et al. The diagnostic and prognostic utility of contemporary cardiac magnetic resonance in suspected acute myocarditis. Diagnostics (Basel). (2022) 12(1):8–10. Available at: https://pubmed.ncbi.nlm.nih.gov/35054323/
26. Petersen SE, Friedrich MG, Leiner T, Elias MD, Ferreira VM, Fenski M, et al. Cardiovascular magnetic resonance for patients with COVID-19. JACC Cardiovasc Imaging. (2022) 15(4):685–99. doi: 10.1016/j.jcmg.2021.08.021
27. Schumm J, Greulich S, Wagner A, Grün S, Ong P, Bentz K, et al. Cardiovascular magnetic resonance risk stratification in patients with clinically suspected myocarditis. J Cardiovasc Magn Reson. (2014) 16(1):14. doi: 10.1186/1532-429X-16-14
28. Stefan G, Julia S, Simon G, Anja W, Steffen S, Oliver B, et al. Long-term follow-up of biopsy-proven viral myocarditis. J Am Coll Cardiol. (2012) 59(18):1604–15. doi: 10.1016/j.jacc.2012.01.007
29. Friedrich MG, Sechtem U, Schulz-Menger J, Holmvang G, Alakija P, Cooper LT, White JA, Abdel-Aty H, Gutberlet M, Prasad S, Aletras Aet al.Cardiovascular magnetic resonance in myocarditis: a JACC white paper. J Am Coll Cardiol. (2009) 53(17):1475–87. doi: 10.1016/j.jacc.2009.02.007
30. Ferreira VM, Piechnik SK, Dall’Armellina E, Karamitsos TD, Francis JM, Ntusi N, et al. Native T1-mapping detects the location, extent and patterns of acute myocarditis without the need for gadolinium contrast agents. J Cardiovasc Magn Reson. (2014) 16(1):36. doi: 10.1186/1532-429X-16-36
31. Shah W, Hillman T, Playford ED, Hishmeh L. Managing the long term effects of COVID-19: summary of NICE SIGN, and RCGP rapid guideline. Br Med J. (2021) 372:n136. doi: 10.1136/bmj.n136
32. Kotecha T, Knight DS, Razvi Y, Kumar K, Vimalesvaran K, Thornton G, et al. Patterns of myocardial injury in recovered troponin-positive COVID-19 patients assessed by cardiovascular magnetic resonance. Eur Heart J. (2021) 42(19):1866–78. doi: 10.1093/eurheartj/ehab075
33. Rajpal S, Tong MS, Borchers J, Zareba KM, Obarski TP, Simonetti OP, et al. Cardiovascular magnetic resonance findings in competitive athletes recovering from COVID-19 infection. JAMA Cardiology. (2021) 6:116–8. Available at: https://jamanetwork.com/32915194
34. Martinez MW, Tucker AM, Bloom OJ, Green G, DiFiori JP, Solomon G, et al. Prevalence of inflammatory heart disease among professional athletes with prior COVID-19 infection who received systematic return-to-play cardiac screening. JAMA Cardiol. (2021) 10019:1–8. Available at: https://jamanetwork.com/journals/jamacardiology/fullarticle/2777308
35. Raisi-Estabragh Z, McCracken C, Cooper J, Fung K, Paiva JM, Khanji MY, et al. Adverse cardiovascular magnetic resonance phenotypes are associated with greater likelihood of incident coronavirus disease 2019: findings from the UK biobank. Aging Clin Exp Res. (2021) 33(4):1133–44. doi: 10.1007/s40520-021-01808-z
36. Ruan Q, Yang K, Wang W, Jiang L, Song J. Clinical predictors of mortality due to COVID-19 based on an analysis of data of 150 patients from Wuhan, China. Intensive Care Med. (2020) 46(5):846–8. doi: 10.1007/s00134-020-05991-x
37. Carvalho-Schneider C, Laurent E, Lemaignen A, Beaufils E, Bourbao-Tournois C, Laribi S, et al. Follow-up of adults with noncritical COVID-19 two months after symptom onset. Clin Microbiol Infect. (2021) 27(2):258–63. doi: 10.1016/j.cmi.2020.09.052
38. Siripanthong B, Asatryan B, Hanff TC, Chatha SR, Khanji MY, Ricci F, et al. The pathogenesis and long-term consequences of COVID-19 cardiac injury. JACC Basic Transl Sci. (2022) 7(3 Pt 1):294–308. doi: 10.1016/j.jacbts.2021.10.011
39. Elseidy SA, Awad AK, Vorla M, Fatima A, Elbadawy MA, Mandal D, et al. Cardiovascular complications in the post-acute COVID-19 syndrome (PACS). IJC Heart Vasc. (2022) 40:101012. doi: 10.1016/j.ijcha.2022.101012
40. Wang H, Li R, Zhou Z, Jiang H, Yan Z, Tao X, et al. Cardiac involvement in COVID-19 patients: mid-term follow up by cardiovascular magnetic resonance. J Cardiovasc Magn Reson. (2021) 23(1):14. doi: 10.1186/s12968-021-00710-x
41. Puntmann VO, Carerj ML, Wieters I, Fahim M, Arendt C, Hoffmann J, et al. Outcomes of cardiovascular magnetic resonance imaging in patients recently recovered from coronavirus disease 2019 (COVID-19). JAMA Cardiol. (2020) 5(11):1265–73. doi: 10.1001/jamacardio.2020.3557
42. Hinojar R, Varma N, Child N, Goodman B, Jabbour A, Yu CY, et al. T1 mapping in discrimination of hypertrophic phenotypes: hypertensive heart disease and hypertrophic cardiomyopathy. Circ Cardiovasc Imaging. (2015) 8(12):e003285. doi: 10.1161/CIRCIMAGING.115.003285
43. Li H, Zhu H, Yang Z, Tang D, Huang L, Xia L. Tissue characterization by mapping and strain cardiac MRI to evaluate myocardial inflammation in fulminant myocarditis. J Magn Reson Imaging. (2020) 52(3):930–8. doi: 10.1002/jmri.27094
44. Beitzke D, Salgado R, Francone M, Kreitner KF, Natale L, Bremerich J, et al. Cardiac imaging procedures and the COVID-19 pandemic: recommendations of the European society of cardiovascular radiology (ESCR). Int J Cardiovasc Imaging. (2020) 36(10):1801–10. doi: 10.1007/s10554-020-01892-8
45. Han Y, Chen T, Bryant J, Bucciarelli-Ducci C, Dyke C, Elliott MD, et al. Society for cardiovascular magnetic resonance (SCMR) guidance for the practice of cardiovascular magnetic resonance during the COVID-19 pandemic. J Cardiovasc Magn Reson. (2020) 22(1):26. doi: 10.1186/s12968-020-00628-w
46. Bernhard B, Schnyder A, Garachemani D, Fischer K, Tanner G, Safarkhanlo Y, et al. Prognostic value of right ventricular function in patients with suspected myocarditis undergoing cardiac magnetic resonance. JACC Cardiovasc Imaging. (2023) 16(1):28–41. doi: 10.1016/j.jcmg.2022.08.011
47. Non-FDA. Vaccines and related biological products advisory committee December 10, 2020 meeting briefing document-sponsor (2020).
48. Non-FDA. Vaccines and related biological products advisory committee December 17, 2020 meeting briefing document-sponsor (2020).
49. Surveillance of myocarditis (inflammation of the heart muscle) cases between December 2020 and May 2021 (including)|Ministry of Health. Available at: https://www.gov.il/en/departments/news/01062021-03 (Cited August 10, 2021).
50. Polack FP, Thomas SJ, Kitchin N, Absalon J, Gurtman A, Lockhart S, et al. Safety and efficacy of the BNT162b2 mRNA COVID-19 vaccine. (2020) 383(27):2603–15. Available at: https://www.nejm.org/doi/full/10.1056/nejmoa203457733301246
51. Baden LR, el Sahly HM, Essink B, Kotloff K, Frey S, Novak R, et al. Efficacy and safety of the mRNA-1273 SARS-CoV-2 vaccine. (2020) 384(5):403–16. doi: 10.1056/NEJMoa2035389
52. Montgomery J, Ryan M, Engler R, Hoffman D, McClenathan B, Collins L, et al. Myocarditis following immunization with mRNA COVID-19 vaccines in members of the US military. JAMA Cardiol. (2021) 6(10):1202–6. doi: 10.1001/jamacardio.2021.2833
53. Diaz GA, Parsons GT, Gering SK, Meier AR, Hutchinson IV, Robicsek A. Myocarditis and pericarditis after vaccination for COVID-19. JAMA. (2021) 326(12):1210–2. doi: 10.1001/jama.2021.13443
54. CDC. Clinical considerations: myocarditis after mRNA COVID-19 vaccines. Available at: https://www.cdc.gov/vaccines/covid-19/clinical-considerations/myocarditis.html (Cited August 11, 2021).
55. Bozkurt B, Kamat I, Hotez PJ. Myocarditis with COVID-19 mRNA vaccines. Available at: http://ahajournals.org (Cited July 24, 2021).
56. Oster ME, Shay DK, Su JR, Gee J, Creech CB, Broder KR, et al. Myocarditis cases reported after mRNA-based COVID-19 vaccination in the US from December 2020 to August 2021. JAMA. (2022) 327(4):331–40. doi: 10.1001/jama.2021.24110
57. Shaw KE, Cavalcante JL, Han BK, Gössl M. Possible association between COVID-19 vaccine and myocarditis: clinical and CMR findings. JACC Cardiovasc Imaging. (2021) 14(9):1856. doi: 10.1016/j.jcmg.2021.06.002
58. Gargano JW. Use of mRNA COVID-19 vaccine after reports of myocarditis among vaccine recipients: update from the advisory committee on immunization practices—United States, June 2021. MMWR Morb Mortal Wkly Rep. (2021) 70(27):977–82. doi: 10.15585/mmwr.mm7027e2
59. CDC. Interim clinical considerations for use of COVID-19 vaccines. Available at: https://www.cdc.gov/vaccines/covid-19/clinical-considerations/covid-19-vaccines-us.html?CDC_AA_refVal=https%3A%2F%2Fwww.cdc.gov%2Fvaccines%2Fcovid-19%2Finfo-by-product%2Fclinical-considerations.html (Cited August 11, 2021).
60. Patone M, Mei XW, Handunnetthi L, Dixon S, Zaccardi F, Shankar-Hari M, et al. Risks of myocarditis, pericarditis, and cardiac arrhythmias associated with COVID-19 vaccination or SARS-CoV-2 infection. Nat Med. (2021) 28(2):410–22. doi: 10.1038/s41591-021-01630-0
61. Siripanthong B, Asatryan B, Hanff TC, Chatha SR, Khanji MY, Ricci F, et al. The pathogenesis and long-term consequences of COVID-19 cardiac injury. JACC Basic Transl Sci. (2022) 7(3):294–308. doi: 10.1016/j.jacbts.2021.10.011
62. Kadkhoda K. Post RNA-based COVID vaccines myocarditis: proposed mechanisms. Vaccine. (2022) 40(3):406–7. doi: 10.1016/j.vaccine.2021.11.093
63. Zuckerman JN. The importance of injecting vaccines into muscle. Br Med J. (2000) 321(7271):1237–8. doi: 10.1136/bmj.321.7271.1237
64. Abu Mouch S, Roguin A, Hellou E, Ishai A, Shoshan U, Mahamid L, et al. Myocarditis following COVID-19 mRNA vaccination. Vaccine. (2021) 39(29):3790–3. doi: 10.1016/j.vaccine.2021.05.087
65. Patel YR, Louis DW, Atalay M, Agarwal S, Shah NR. Cardiovascular magnetic resonance findings in young adult patients with acute myocarditis following mRNA COVID-19 vaccination: a case series. J Cardiovasc Magn Reson. (2021) 23(1):101. doi: 10.1186/s12968-021-00795-4
66. Ochs MM, Haass M, Hagstotz S, Giusca S, Korosoglou G. Case report: case series of isolated acute pericarditis after SARS-CoV-2 vaccinations. Front Cardiovasc Med. (2022) 9:2272. Available at: https://pubmed.ncbi.nlm.nih.gov/36061550/
67. Garg R, Chetrit M, Friedrich MG. Comparison of CMR findings in symptomatic patients with different COVID-related syndromes. SCMR Virtual scientific sessions 2022 (2022).
68. Kim RJ, Kim HW, Jenista ER, Wendell DC, Azevedo CF, Campbell MJ, et al. Patients with acute myocarditis following mRNA COVID-19 vaccination. JAMA Cardiol. (2021) 6(10):1196–201. doi: 10.1001/jamacardio.2021.2828
69. Shiyovich A, Witberg G, Aviv Y, Eisen A, Orvin K, Wiessman M, et al. Myocarditis following COVID-19 vaccination: magnetic resonance imaging study. Eur Heart J Cardiovasc Imaging. (2021) 9:990108. Available at: https://pubmed.ncbi.nlm.nih.gov/34739045/
70. Fronza M, Thavendiranathan P, Chan V, Karur GR, Udell JA, Wald RM, et al. Myocardial injury pattern at MRI in COVID-19 vaccine–associated myocarditis. Radiology. (2022) 304(3):553–62. doi: 10.1148/radiol.212559
71. Clark DE, Parikh A, Dendy JM, Diamond AB, George-Durrett K, Fish FA, et al. COVID-19 myocardial pathology evaluation in athletes with cardiac magnetic resonance (COMPETE CMR). Circulation. (2021) 143(6):609–12. doi: 10.1161/CIRCULATIONAHA.120.052573
72. Małek ŁA, Marczak M, Miłosz-Wieczorek B, Konopka M, Braksator W, Drygas W, et al. Cardiac involvement in consecutive elite athletes recovered from COVID-19: a magnetic resonance study. J Magn Reson Imaging. (2021) 53(6):1723–9. doi: 10.1002/jmri.27513
73. Esposito A, Palmisano A, Natale L, Ligabue G, Peretto G, Lovato L, et al. Cardiac magnetic resonance characterization of myocarditis-like acute cardiac syndrome in COVID-19. JACC Cardiovasc Imaging. (2020) 13:2462–5. doi: 10.1016/j.jcmg.2020.06.003
74. Huang L, Zhao P, Tang D, Zhu T, Han R, Zhan C, et al. Cardiac involvement in patients recovered from COVID-2019 identified using magnetic resonance imaging. (2020). doi: 10.1016/j.jcmg.2020.05.004
75. Ng MY, Ferreira VM, Leung ST, Yin Lee JC, Ho-Tung Fong A, To Liu RW, et al. Patients recovered from COVID-19 show ongoing subclinical myocarditis as revealed by cardiac magnetic resonance imaging. JACC Cardiovasc Imaging. (2020) 13(11):2476–8. doi: 10.1016/j.jcmg.2020.08.012
76. Homsi R, Yuecel S, Schlesinger-Irsch U, Meier-Schroers M, Kuetting D, Luetkens J, et al. Epicardial fat, left ventricular strain, and T1-relaxation times in obese individuals with a normal ejection fraction. Acta Radiol. (2019) 60(10):1251–7. doi: 10.1177/0284185119826549
77. Roy C, Slimani A, De Meester C, Amzulescu M, Pasquet A, Vancraeynest D, et al. Age and sex corrected normal reference values of T1, T2 T2* and ECV in healthy subjects at 3 T CMR. J Cardiovasc Magn Reson. (2017) 19(1):1–12. doi: 10.1186/s12968-016-0318-2
78. Lowenstein CJ, Solomon SD. Severe COVID-19 is a microvascular disease. Circulation. (2020) 142(17):1609–11. doi: 10.1161/CIRCULATIONAHA.120.050354
79. Nalugo M, Schulte LJ, Masood MF, Zayed MA. Microvascular angiopathic consequences of COVID-19. Front Cardiovasc Med. (2021) 8(636843):26.
80. Vallejo Camazón N, Teis A, Martínez Membrive MJ, Llibre C, Bayés-Genís A, Mateu L. Long COVID-19 and microvascular disease-related angina. Rev Esp Cardiol (English Edition). (2022) 75(5):444–6. doi: 10.1016/j.rec.2021.10.010
81. Vallejo N, Teis A, Mateu L, Bayés-Genís A. Persistent chest pain after recovery of COVID-19: microvascular disease-related angina? Eur Heart J Case Rep. (2021) 5(3):ytab105. Available at: https://academic.oup.com/ehjcr/article/5/3/ytab105/618457134113774
82. (12) (PDF) Participant(s) SUBMISSION PREVIEW: MICROVASCULAR DYSFUNCTION IN POST-COVID-19 PATIENTS-CASE SERIES Microvascular dysfunction in post-COVID-19 patients -Case series. Available at: https://www.researchgate.net/publication/368713807_Participants_SUBMISSION_PREVIEW_MICROVASCULAR_DYSFUNCTION_IN_POST-COVID-19_PATIENTS_-CASE_SERIES_Microvascular_dysfunction_in_post-COVID-19_patients_-Case_series (Cited March 10, 2023).
83. Aikawa T, Ogino J, Kita Y, Funayama N. Myocardial microthrombi after COVID-19 mRNA vaccination. Eur Heart J. (2021) 42(43):4501. doi: 10.1093/eurheartj/ehab727
84. Hillier E, Covone J, Friedrich MG. Oxygenation-sensitive cardiac MRI with vasoactive breathing maneuvers for the non-invasive assessment of coronary microvascular dysfunction. J Visualized Exp. (2022) (186):e64149. Available at: https://www.jove.com/v/64149/oxygenation-sensitive-cardiac-mri-with-vasoactive-breathing-maneuvers
Keywords: COVID-19, COVID vaccine, CMR (cardiovascular magnetic resonance), myocardial injury, phenotype
Citation: Garg R, Hussain M and Friedrich MG (2023) Phenotyping myocardial injury related to COVID and SARS-CoV-2 vaccination: insights from cardiovascular magnetic resonance. Front. Cardiovasc. Med. 10:1186556. doi: 10.3389/fcvm.2023.1186556
Received: 14 March 2023; Accepted: 16 May 2023;
Published: 15 June 2023.
Edited by:
Emanuele Bobbio, Sahlgrenska University Hospital, SwedenReviewed by:
Óscar M. Peiró, Joan XXIII University Hospital of Tarragona, Spain© 2023 Garg, Hussain and Friedrich. This is an open-access article distributed under the terms of the Creative Commons Attribution License (CC BY). The use, distribution or reproduction in other forums is permitted, provided the original author(s) and the copyright owner(s) are credited and that the original publication in this journal is cited, in accordance with accepted academic practice. No use, distribution or reproduction is permitted which does not comply with these terms.
*Correspondence: Ria Garg Z2FyZ3JpYTExMUBnbWFpbC5jb20=
†These authors share first authorship
‡This author shares senior authorship