- 1PRC, INRAE, CNRS, IFCE, Université de Tours, Nouzilly, France
- 2U1195 INSERM Université Paris Saclay, Kremlin Bicêtre, France
- 3PAO, INRAE, Nouzilly, France
- 4Faculté de Médecine vétérinaire, Département des Sciences Cliniques-Clinique Equine, Université de Liège, Liège, Belgium
Precision livestock farming using omics approach to acquire precise and real-time data can help farmers in individual animal management and decision making. Since steroid hormones play a key role for the regulation of reproductive functions, reproduction management could be improved by characterizing the steroidome during reproductive stages. Moreover, saliva collection is a non-invasive, painless, inexpensive and easy sampling method. Thus, this prospective study proposes a steroidomic analysis in mare saliva during reproductive stages, that could help to identify potential biomarkers to accurately detect their reproductive stage in a welfare friendly production system, for real-time decision making at the individual animal level. Correlation between saliva and plasma steroidome was also investigated. Saliva and blood samples from 6 mares were collected in anestrus, in the follicular phase 3 days, 2 days and 1 day before ovulation and the day when ovulation was detected, in the luteal phase 6 days after ovulation and in gestation 18 days after ovulation and insemination. Steroidome analysis was performed by gas chromatography coupled to tandem mass spectrometry (GC-MS/MS). We characterized 25 and 36 steroids in saliva and plasma respectively. Pregnenolone concentrations in saliva during gestation were significantly higher than during anestrus or follicular phase and tended to be higher than during luteal phase. Most of the 5α-reduced metabolites of progesterone showed higher salivary concentrations during the luteal phase 6 days post-ovulation and during gestation 18 days post-ovulation compared to anestrus and follicular phase. These steroids could be potential salivary biomarkers of the reproductive stage of the mare. Further studies with a greater number of animals are in progress to confirm the reliability of these potential candidate biomarkers and to develop field-friendly assays.
Introduction
Precision livestock farming relies on several technological approaches to acquire precise and real-time data concerning production, reproduction, health and welfare of individual animals. Recently, omics approach has gained much attention to facilitate the ability for deep and precise phenotyping of livestock at the molecular level. Precision livestock farming using omics data can help farmers in individual animal management and decision making. Steroidomics, defined as the targeted analysis of the steroid content in a biological fluid, can help to improve precision of breeding and management of reproduction since steroids regulate essential biological functions including reproduction. Among biological fluids, saliva gains interest since it can be collected by non-invasive, painless, inexpensive and easy sampling methods, allowing the collection of repeated samples without the need for specialized staff. Thus, steroidomics analysis of saliva samples could help to improve precision livestock management of reproduction in a welfare friendly production system.
Horses are high value farm animals requiring careful management and single animal monitoring. They are seasonal breeders with a winter anestrus and a transitional phase bringing the mare back into cyclic activity in spring and summer with a cycle length of about 22 days (Aurich, 2011). Gestation length of 320 to 360 days does not leave much time for mating for farmers expecting one foal per year (Davies Morel et al., 2002). Moreover, racehorses born in the northern hemisphere are administratively considered born on January 1st, thus conception early in the year is advantageous to young horses competing in the same age category. For these reasons, reproduction management at the beginning of breeding season is critical for breeders.
Up to now, most studies in mares have focused solely on a few steroids instead of a whole steroidomic analysis, and steroids concentrations were generally measured in plasma. For example, progesterone (PROG) and 17β-estradiol (17β-E2) measurements in plasma of cyclic mares by enzyme immunoassay and radioimmunoassay, respectively, showed that concentrations of PROG begin to increase the day of ovulation, reach maximal concentrations at 6 to 8 days post-ovulation and decrease until day 14 post-ovulation whereas concentrations of 17β-E2 reach a peak 2 days before ovulation and then decrease (Ginther et al., 2005; Aurich, 2011). Gas chromatography coupled to mass spectrometry (GC-MS), liquid chromatography coupled to mass spectrometry (LC-MS) and tandem mass spectrometry (LC-MS/MS) analysis have been developed to measure some pregnanes (C21-steroids), androstanes (C19-steroids) and estranes (C18-steroids) in serum of male and female uncharacterized horses (Genangeli et al., 2017; Dufour et al., 2021) and in plasma throughout equine gestation (Scholtz et al., 2014; Legacki et al., 2016; Wynn et al., 2018a; Ledeck et al., 2022). Analysis in pregnant mares showed that plasmatic PROG concentrations were high during the first 12 weeks of pregnancy and declined progressively until undetectable levels by day 200. The plasmatic concentration of the 5α-reduced metabolite of PROG, 5α−dihydroprogesterone (5α-DHPROG), that is a biopotent progestin in horse (Scholtz et al., 2014), is present at 60% of PROG level until 12 weeks of pregnancy but, in contrast to PROG, continues to rise steadily and peaks by day 320 of gestation, sustaining equine pregnancies in the second half of gestation (Scholtz et al., 2014; Conley, 2016). On the contrary, salivary steroids concentrations have been poorly investigated in the equine. Only salivary analysis of testosterone by enzyme immunoassay (Munk et al., 2016) and cortisol by enzyme immunoassay (Nagel et al., 2012; Diego et al., 2016; Schwinn et al., 2018; Contreras-Aguilar et al., 2021) or LC-MS/MS (Sauer et al., 2020) have been investigated.
Analysis by immunoassay allows a high throughput, but this method has two major limitations. First, cross-reactivity reactions often overestimate steroid concentrations because of the presence of structurally very similar steroids. Secondly, only one or few steroids can be quantified. Instead, gas or liquid chromatography coupled to tandem mass spectrometry has emerged as the reference method for accurate and robust steroid analysis (Schumacher et al., 2015; Sauer et al., 2020). The combination of the high-resolution chromatography with the specificity of the detection of mass spectrometry allows a higher assay selectivity, sensitivity and precision. For example, (Sauer et al., 2020) showed that LC-MS/MS provided more precise results as compared to immunoassays, especially in the low concentration range (< 1ng/ml). Moreover, gas chromatography coupled to tandem mass spectrometry (GC-MS/MS) allows to separate and measure a large number of steroids characterized by similar physicochemical properties in a small volume and to analyse a whole steroidome (Liere and Schumacher, 2015). Thus, the high specificity of GC-MS/MS coupled with the use of isotopically labelled internal standards for steroids quantification permits to achieve reference values of concentrations without potential matrix effects.
Thus, the present study proposes an analysis of the steroidome in mares’ saliva during reproductive stages using GC-MS/MS. The steroidome in saliva is compared to plasma to investigate the relationship between the two fluids according to the physiological stage. This prospective study will help to identify potential salivary steroidal biomarkers to accurately detect the reproductive stage of the mare in a welfare friendly production system, for real-time decision making at the individual animal level.
Material and methods
Animals, housing and sample collection
This experiment involved six healthy Welsh type pony mares aged 5 to 9 years old, weighing 277 to 390 kg, that were fertile and had already given birth to a healthy foal. They were reared at the experimental farm from INRAE (Unité Expérimentale 1297 de Physiologie Animale de l’Orfrasière; https://doi.org/10.15454/1.5573896321728955E12) in France. From December to March, mares were penned in groups in an indoor stall on a straw bedding under natural daylight with free access to an outdoor paddock during the day. They were fed with 0.6 kg of concentrate (20% oats, 18% wheat straw, 16% wheat bran, 15% barley, 12% alfalfa; Eperon INRA, Axereal, Saint-Germain de Salles, France). Straw, salt licks (Sodical, Levalois-Perret, France) and water were available ad libitum. From April to November, they lived in groups in the pasture with salt licks and water available ad libitum.
All procedures on animals were conducted in accordance with the guidelines for the care and use of laboratory animals issued by the French Ministry of Agriculture and with the approval of the ethical review committee (Comité d’Ethique en Expérimentation Animale Val de Loire n°019) under number #18286.
Saliva and blood samples were collected at seven physiological stages from each mare:
-in anestrus,
-in the follicular phase before ovulation: 3 days, 2 days and 1 day before ovulation, and the day when ovulation was detected,
-in the luteal phase 6 days after ovulation,
-in gestation 18 days after ovulation and artificial insemination.
Saliva and blood samples collected during anestrus were recovered on February 7th. Anestrus was ascertained by plasmatic progesterone concentrations lower than 1 ng/ml every week and no increase over 1 ng/ml from December to the end of February, with PROG concentrations measured by enzyme-linked immunosorbent assay (Canepa et al., 2008) as previously described (Panzani et al., 2017).
Saliva and blood samples collected during the follicular and luteal phases were recovered between April 27th and June 20th. Saliva and blood samples collected during gestation were recovered between June 6th and August 3rd. Ovarian activity was assessed by routine transrectal ultrasound scanning using an EXAPad (IMV imaging, Angoulême, France) with a 7.5 Mhz liner probe. No saliva and blood samples were collected during the first estrus cycle of the season. Samples collection during the follicular phase started during the second estrus cycle when the largest follicle reached 28mm and was performed every day until detection of natural ovulation (mean ± SEM = 8.6 ± 0.6 days). Only samples collected 3 days, 2 days and 1 day before ovulation and the day when ovulation was detected were analysed. Samples collection during the luteal phase was performed 6 days after ovulation, at the time of maximal PROG concentration (Ginther et al., 2005; Aurich, 2011). On the subsequent estrus cycle, when the largest follicle reached 33mm, mares were inseminated with fresh equine semen from a Welsh pony stallion from our experimental stud (400 X 106 spermatozoa in 10 ml of extender (INRA96®, IMV Technologies, France)) (Pillet et al., 2008). A second insemination was performed the day after if the mare did not ovulate. The pregnancy was confirmed by visualization of the embryonic vesicle 14 days after ovulation using transrectal ultrasound scanning. If the pregnancy was confirmed, saliva and blood samples collection was performed 18 days after ovulation, to search for early salivary biomarkers of gestation, because concentrations of progesterone decrease 14 days following ovulation in non-pregnant mares and remain high in pregnant mares. The presence of the embryonic vesicle was ascertained again 18 days after ovulation using transrectal ultrasound scanning. If the pregnancy was not confirmed 14 days after ovulation, the mare was inseminated during the following estrus cycle.
Saliva and blood samples were collected from each mare in the morning between 9 am and 10 am to avoid circadian variations of steroids secretions. Mares were put in a stall without straw one hour before sampling, to avoid saliva contamination with food. Saliva was collected using a cotton swab (Sarstedt Salivette® ref. 51.1534.500; Sarstedt, Nümbrecht, Germany). The cotton swab was held with forceps and the mare was allowed to chew on it until it was soaked. Collection of saliva was well tolerated by the animals without any restraint of the horse needed. The cotton swab was immediately centrifuged at 3000 X g for 5 min at room temperature. The recovered saliva was aliquoted and stored at -80°C until analysis. Blood samples were collected from the jugular vein by experienced operators, using Vacutainer® heparinized tubes and 20G needles to ensure a smooth and animal welfare respectful procedure. They were then immediately centrifuged at 4000 X g for 10 min at room temperature. The recovered plasma was aliquoted and stored at -80°C until analysis.
Steroidomics analysis by gas chromatography coupled to tandem mass spectrometry (GC-MS/MS)
The protocol for the steroidome analysis in saliva and plasma by GC–MS/MS was previously described by Goudet and collaborators (Goudet et al., 2019; Goudet et al., 2021).
Steroids were extracted from saliva (797-1455 μl) and from plasma (1 ml) with methanol. The saliva and plasma were spiked with all the following internal standards for steroid quantification: 5 ng of 13C5-5α-dihydroprogesterone (DHPROG) for 5α/β-DHPROG and 5α/β-dihydroandrostenedione (5α/β-DHADIONE), 5 ng of 2H213C2-pregnenolone sulfate (PREGS) for PREGS, 5 ng of 13C3-dehydroepiandrosterone sulfate (DHEAS) for DHEAS, 1 ng of 13C3-testosterone for testosterone, 1 ng of 13C3-androstenedione (ADIONE) for ADIONE, 5 ng of 13C3-progesterone (PROG) for PROG, 5 ng of 13C5-20α-DHPROG for 20α/β-DHPROG, 2 ng of 13C3-deoxycorticosterone (DOC) for DOC, 2 ng of 19nor-PROG (Merck, Darmstadt, Germany) for 5α/β-dihydrodeoxycorticosterone (DHDOC), 2 ng of epietiocholanolone (Steraloids, Newport, USA) for androstenediol (ADIOL), etiocholanolone, epiandrosterone, 5α/β-dihydrotestosterone (DHT), 3α/β5α/β-tetrahydrotestosterone (THT), pregnenolone (PREG), 20α-dihydropregnenolone (DHPREG), 3α/β5α/β-tetrahydroprogesterone (THPROG), 5α20α-THPROG, 3α/β5α/β20α/β-hexahydroprogesterone (HHPROG) and 3α/β5α/β-tetrahydrodeoxycorticosterone (THDOC), 2 ng of 13C3-17α-hydroxyprogesterone (17α-OH PROG) for 17α-OH PROG, 17α-OH PREG and 16α-OH PROG, 2H8-corticosterone (B) for B, 5α/β-dihydrocorticosterone (DHB), 3α/β5α/β-tetrahydrocorticosterone (THB) and 11-dehydrocorticosterone (11-dehydroB), 50 ng of 13C3-cortisol (F) (Eurisotop, Saint Aubin, France) for F, 50 ng of 13C3-cortisone (E) for E, 10 ng of 2H7-aldosterone for aldosterone, 2 ng of 13C3-11-deoxycortisol (S) for S, 1 ng of 2H5-17β-estradiol (17β-E2) (Eurisotop) for 17β-E2 and 2-hydroxyestradiol (2-OH E2), 1 ng of 13C3-estrone (E1) for E1, 1 ng of 13C3-estriol (E3) for E3 and estetrol (E4) and 1 ng of 2H5-2-methoxyestradiol (2-ME2) (Eurisotop) for 2-ME2. All the internal standards were provided by Isosciences (PA, USA) except those that are specified.
The extracts were purified and fractionated by solid-phase extraction with the recycling procedure as previously described (Goudet et al., 2019). The unconjugated steroids-containing fraction was then filtered and further purified and fractionated by HPLC. The HPLC system was composed of a WPS-3000SL analytical autosampler and a LPG-3400SD quaternary pump gradient coupled with a SR-3000 fraction collector (Thermoscientific, USA). The HPLC separation was achieved with a Lichrosorb Diol column (25 cm, 4.6 mm, 5 μm) in a thermostated block at 30°C. The column was equilibrated in a solvent system of 90% heptane and 10% of a mixture composed of heptane/isopropanol (85/15). Elution was performed at a flow-rate of 1 ml/min, first with 90% heptane and 10% of heptane/isopropanol (85/15) for 15 min, then with a linear gradient to 100% acetone in 2 min. This mobile phase was kept constant for 13 min.
Two fractions were collected from the HPLC system: 5α/β-DHPROG and 5α/β-ADIONE were eluted in the first HPLC fraction (3-16 min) and were next silylated with 50 μl of a mixture N-methyl-N-trimethylsilyltrifluoroacetamide/ammonium iodide/dithioerythritol (1000:2:5) (vol/wt/wt) for 15 min at 70°C. The second fraction (16-29 min) containing all other steroids was derivatized with 25 μl heptafluorobutyric anhydride (HFBA) and 25 μl anhydrous acetone for 1 h at 80°C. Both fractions were dried under a stream of N2 and resuspended in heptane.
The sulfated steroids, previously isolated by solid-phase extraction, were derivatized with 20 µl HFBA and 100 µl anhydrous acetone for 30 min at 20°C.
The two HPLC fractions and the sulfated steroids were analyzed by GC-MS/MS in the same experimental conditions described by Goudet and collaborators (Goudet et al., 2019; Goudet et al., 2021). GC-MS/MS parameters for identification and quantification for steroids that were not targeted in our previous studies are specified in Supplementary Table S1.
The analytical protocol has been validated for all the targeted steroids by using 1 ml from a pool of male mice plasma (Téteau et al., 2022). The parameters of validation included the limit of detection, linearity, accuracy, intra- and inter-assay precisions and were all described by Teteau and collaborators (Téteau et al., 2022). The linearity was assessed by analyzing increasing amounts of mice plasma extracts (20, 50, 100 and 200 μl) in triplicate. The linearity was satisfactory for all the steroids with a coefficient of correlation ranging from 0.99 to 0.997. Five aliquots of 100 µl of mice plasma were used at two different times to determine the intra-assay and inter-assay coefficient of variation that was found at 7.5% and 10.5% for the targeted steroids, respectively. The accuracy of the assay was evaluated by determining the analytical recovery, which was defined as C/(C0+S) × 100(%). C is the concentration of the steroid in the spiked plasma extract (100 μl), C0 is the concentration of a steroid in the unspiked plasma extract (100 μl) and S is the spiked concentration. The accuracy was in the range of 95–106%.
Our analytical protocol used stable isotope dilution with highly specific GC-MS/MS analysis combined with upstream extensive sample purification/fractionation steps. Many groups (Schenck and Lehotay, 2000; Anastassiades et al., 2003; Mastovská et al., 2005; Chamkasem and Harmon, 2016) have reported that matrix effect is bypassed by introducing multiple extraction, purification, fractionation and derivatization steps combined by the use of stable isotope internal standards, especially 13C-steroids. Indeed, 13C-labelled steroids providing retention time matching to their unlabeled counterparts, this minimizes matrix effect at the ionization step (Olesti et al., 2021). Furthermore, GC-MS/MS, unlike to LC-MS/MS, by combining high resolution gas chromatography with electron impact ionization and the highly selective multiple reaction monitoring permits to eliminate the biological and chemical background, and is the analytical technology of choice to avoid any potential matrix effects for steroid measurements (Anastassiades et al., 2003; Wudy et al., 2018). Thus, the parameters of validation described above were effective for all types of biological fluids such as bovine oviductal fluid (Lamy et al., 2016), porcine saliva (Goudet et al., 2019; Goudet et al., 2021), ewes follicular and oviductal fluids (Téteau et al., 2022) or equine saliva (present paper).
Statistical analysis
Repeated measures one-way ANOVA followed by Tukey’s multiple comparison post-tests were used to identify potential differences in steroid concentrations between all the reproductive stages. The Shapiro-Wilks normality test was used to determine Gaussian distribution. Data are presented as mean and SEM. Pearson’s correlation was used to analyze associations between plasma and saliva steroid concentrations in mare. Statistical analyses were performed using GraphPad Prism version 6 (GraphPad Software, La Jolla California, USA). A significant difference was detected when P < 0.05.
Results
Among the 57 targeted steroids in plasma and saliva, GC-MS/MS analysis allowed the detection of 36 steroids in plasma and 25 in saliva (Table 1). All the 25 steroids detected in saliva were also detected in plasma, but 11 were detected in plasma only: 17α-OH PREG, 20β-DHPROG, 5α20α-THPROG, DOC, 3α5α-THDOC, 3α5β-THDOC, 3β5α-THDOC, aldosterone, cortisol, androstenedione and testosterone. The absolute concentrations of all the detected steroids are given in Table 2.
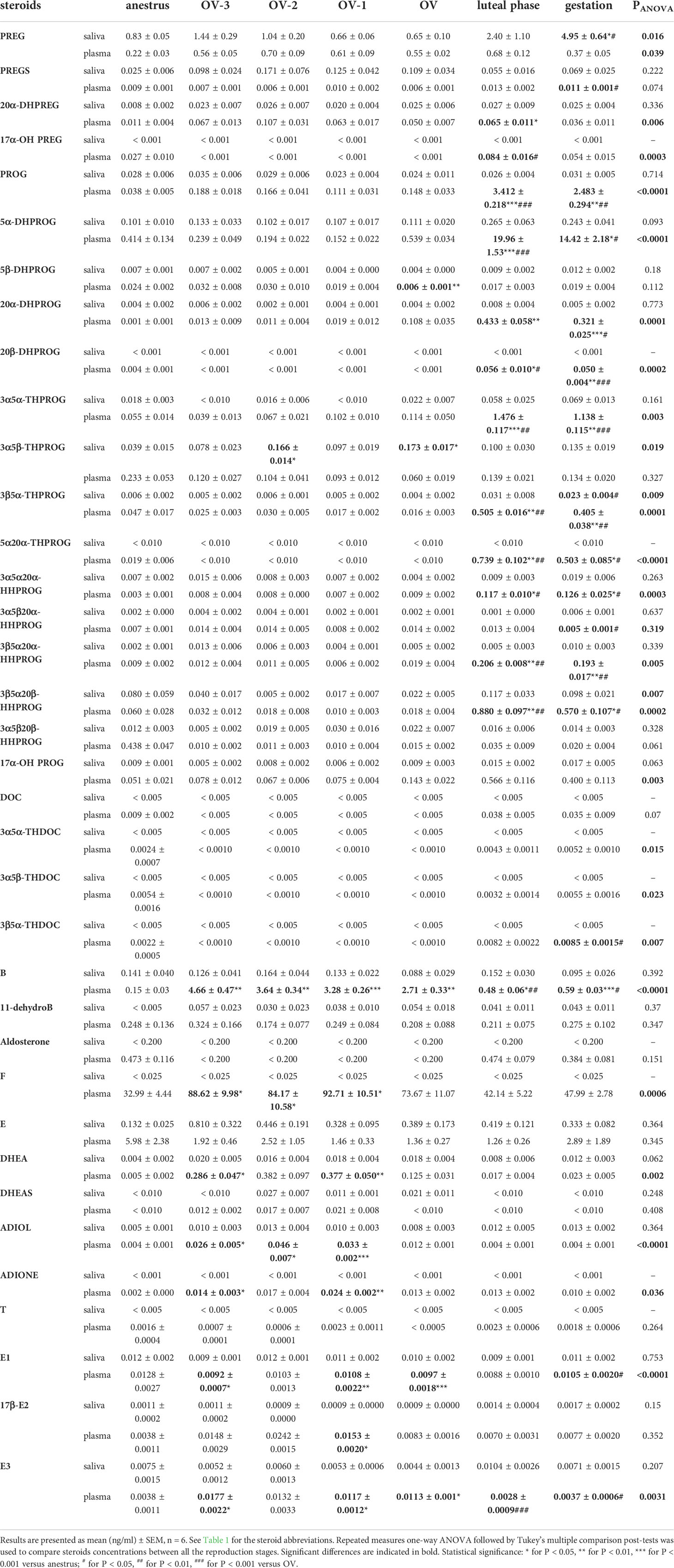
Table 2 Steroids profiling in saliva and plasma of mare in anestrus, 3 days before ovulation (OV-3), 2 days before ovulation (OV-2), 1 day before ovulation (OV-1), the day of ovulation (OV), in luteal phase (6 days after ovulation) and during gestation (18 days after ovulation).
PREG concentrations in saliva were significantly higher during gestation (4.95 ± 0.64 ng/ml) than during anestrus or follicular phase (0.65 ± 0.10 to 1.44 ± 0.29 ng/ml), whereas plasmatic concentrations were not significantly different between the physiological stages (0.22 ± 0.03 to 0.70 ± 0.09 ng/ml) (Figure 1; Table 2). Both direct metabolites of PREG, PREGS and 20α-DHPREG, were also detected in mare saliva but at much lower levels. Their concentrations in saliva were not significantly different between the physiological stages, whereas some differences were observed in plasma (Figure 1). 17α-OH PREG was not detected in saliva but plasmatic concentrations in the luteal phase were significantly higher than in the follicular phase (Figure 1).
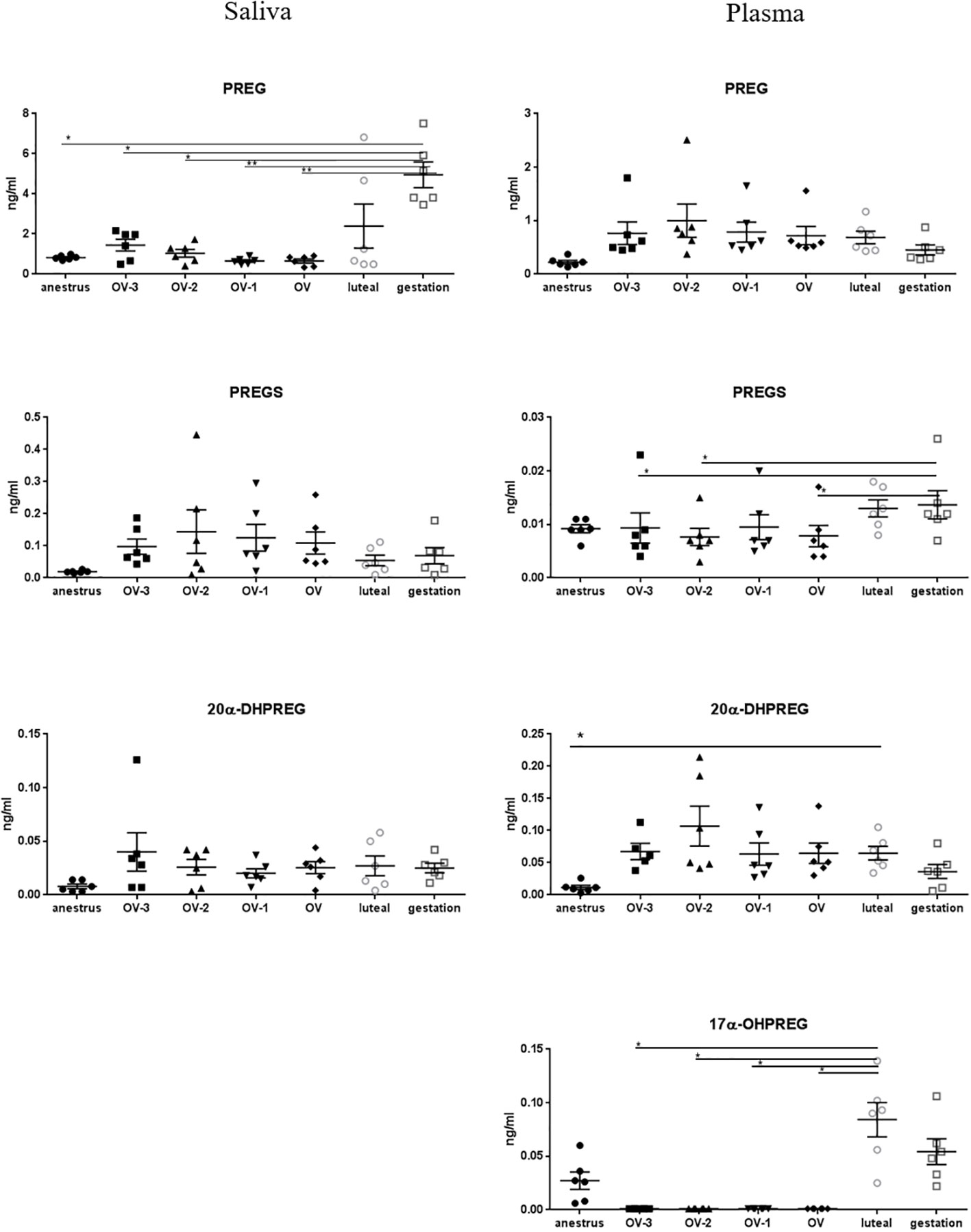
Figure 1 Gas chromatography coupled to tandem mass spectrometry (GC-MS/MS) measurements of the concentrations of pregnenolone (PREG), pregnenolone sulfate (PREGS), 20α-dihydropregnenolone (20α-DHPREG) and 17α-hydroxypregnenolone (17α-OHPREG) (ng/mL ± SEM) in mare saliva and plasma collected during anestrus, during follicular phase 3 days (OV-3), 2 days (OV-2) and 1 day (OV-1) before ovulation, the day when ovulation was detected (OV), during luteal phase 6 days post-ovulation (luteal), during gestation 18 days post-ovulation (gestation). For each steroid, the y-axis was standardized to the maximal concentration, so that the variations are easy-to-read. Statistical differences between physiological stages: * P < 0.05, ** P < 0.01.
PROG concentrations in saliva were low (from 0.023 ± 0.004 to 0.035 ± 0.006 ng/ml) and not significantly different between the physiological stages, whereas a marked significant increase was observed in plasma during the luteal phase (3.41 ± 0.22 ng/ml) and gestation (2.48 ± 0.29 ng/ml) compared to anestrus (0.038 ± 0.005 ng/ml) and all the follicular phases (0. 111 ± 0.031 to 0.188 ± 0.018 ng/ml) (Figure 2; Table 2). The reduced metabolites of PROG, 5α-, 20α- and 20β-DHPROG were characterized by a similar pattern in plasma. We found substantially high levels of the main metabolite of PROG, 5α-DHPROG, in the luteal phase (19.96 ± 1.53 ng/ml) and gestation (14.42 ± 2.18 ng/ml). Interestingly, the saliva levels of the progestagen 5α-DHPROG were also slightly increased in luteal phase (0.265 ± 0.063 ng/ml) and gestation (0.243 ± 0.041 ng/ml) compared to anestrus and follicular phases (0.101 ± 0.010 to 0.133 ± 0.033 ng/ml) but without reaching statistical difference (Figure 2; Table 2). Salivary concentrations of 5β-DHPROG and 20α-DHPROG were not significantly different between the physiological stages, whereas plasmatic concentrations of 5β-DHPROG were significantly lower on the day of ovulation compared to anestrus (Figure 2).
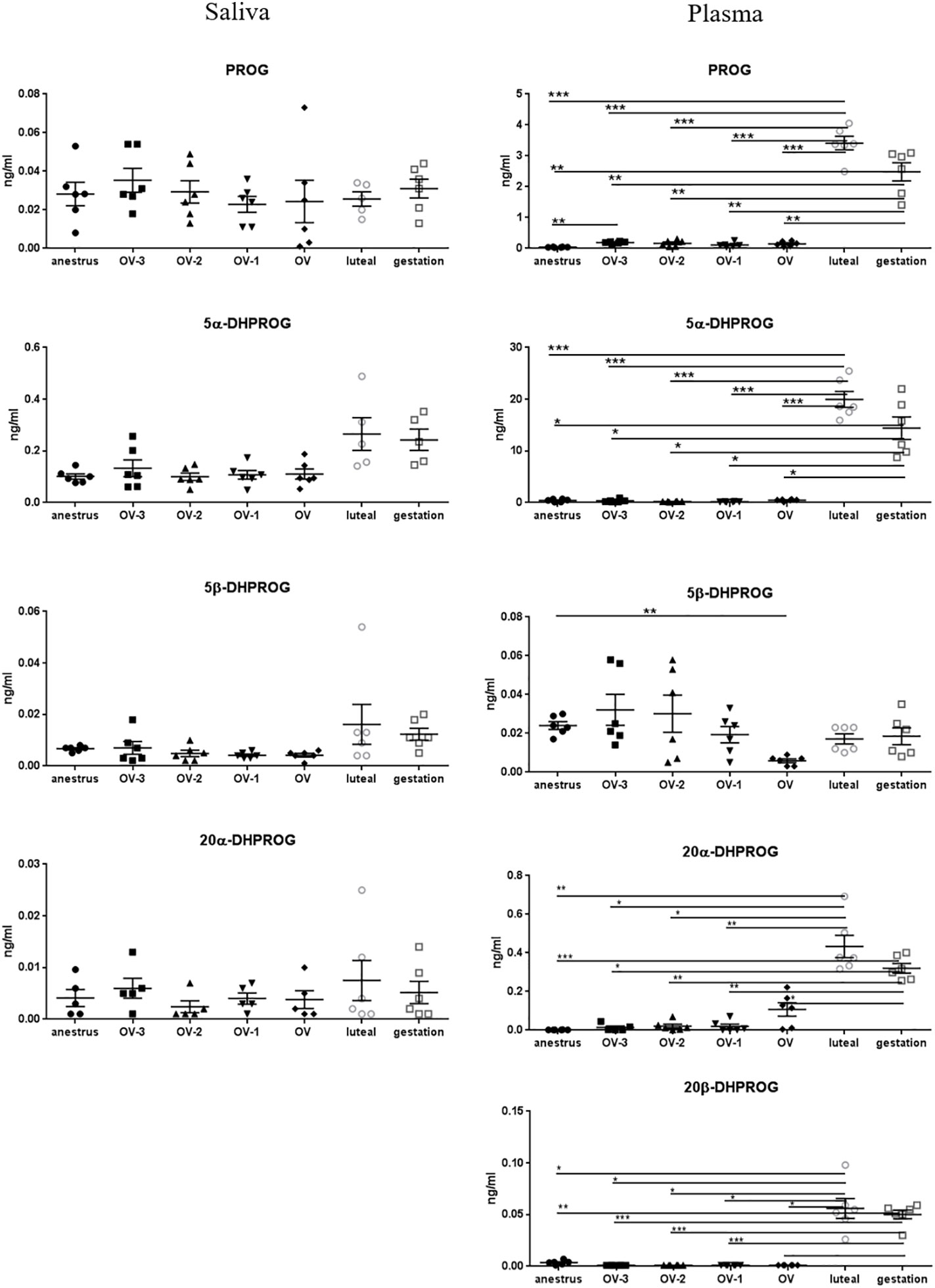
Figure 2 Gas chromatography coupled to tandem mass spectrometry (GC-MS/MS) measurements of the concentrations of progesterone (PROG), 5α-dihydroprogesterone (5α-DHPROG), 5β-DHPROG, 20α-DHPROG and 20β-DHPROG (ng/mL ± SEM) in mare saliva and plasma collected during anestrus, during follicular phase 3 days (OV-3), 2 days (OV-2) and 1 day (OV-1) before ovulation, the day when ovulation was detected (OV), during luteal phase 6 days post-ovulation (luteal), during gestation 18 days post-ovulation (gestation). For each steroid, the y-axis was standardized to the maximal concentration, so that the variations are easy-to-read. Statistical differences between physiological stages: * P < 0.05, ** P < 0.01, *** P < 0.001.
As for 5α-DHPROG, the salivary concentrations of both PROG metabolites, 3α5α- and 3β5α-THPROG, tended to be higher in the luteal phase and gestation compared to anestrus and follicular phases (Figure 3; Table 2). Significant differences were detected for the salivary concentration of 3β5α-THPROG between gestation (0.023 ± 0.004 ng/ml) and the OV-1 (0.005 ± 0.002 ng/ml) and OV (0.004 ± 0.002 ng/ml) stages. Salivary 3α5β-THPROG levels were higher at the OV-2 (0.166 ± 0.014 ng/ml) and OV (0.173 ± 0.017ng/ml) stages as compared to anestrus (0.039 ± 0.015 ng/ml), whereas no difference was detected in plasma (Figure 3). 5α20α-THPROG was not detected in saliva. Plasmatic 3α5α-, 3β5α- and 5α20α-THPROG levels were characterized by a significant increase in luteal phase and gestation relatively to anestrus and follicular phase (Figure 3, Table 2). 17α-OH PROG levels in plasma and saliva tend to increase in luteal phase and gestation relatively to anestrus and follicular phase without statistical significance (Table 2).
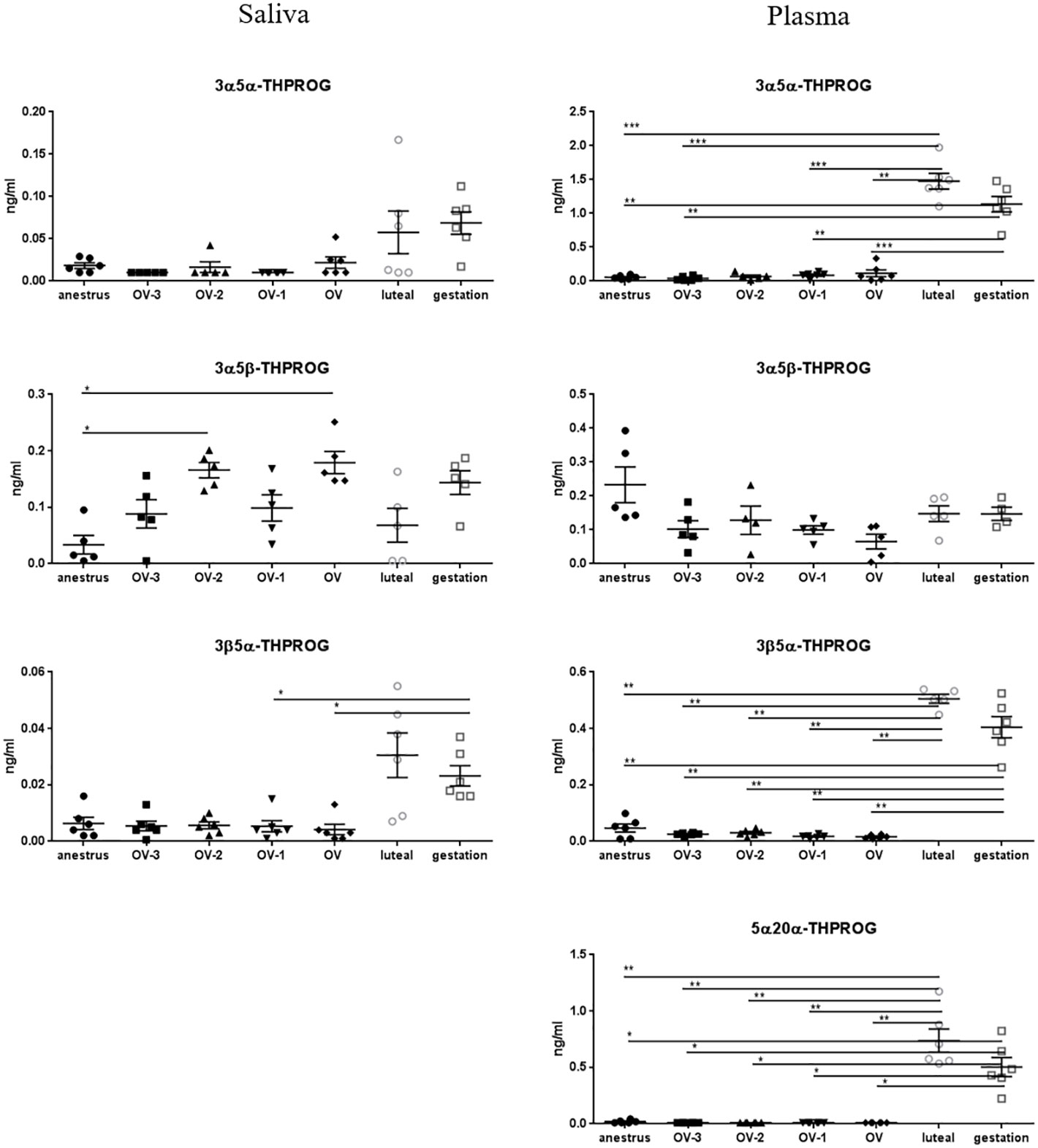
Figure 3 Gas chromatography coupled to tandem mass spectrometry (GC-MS/MS) measurements of the concentrations of 3α5α-tetrahydroprogesterone (3α5α-THPROG), 3α5β-THPROG, 3β5α-THPROG and 5α20α-THPROG (ng/mL ± SEM) in mare saliva and plasma collected during anestrus, during follicular phase 3 days (OV-3), 2 days (OV-2) and 1 day (OV-1) before ovulation, the day when ovulation was detected (OV), during luteal phase 6 days post-ovulation (luteal), during gestation 18 days post-ovulation (gestation). For each steroid, the y-axis was standardized to the maximal concentration, so that the variations are easy-to-read. Statistical differences between physiological stages: * P < 0.05, ** P < 0.01, *** P < 0.001.
Among the other PROG catabolites, the same tendency was observed in saliva for 3α5α20α- HHPROG with higher concentrations in gestation (0.019 ± 0.006 ng/ml) compared to the other stages (0.004 ± 0.002 to 0.015 ± 0.006 ng/ml) and for 3β5α20β-HHPROG in luteal phase (0.117 ± 0.033 ng/ml) and gestation (0.098 ± 0.021 ng/ml) compared to the other stages (0.005 ± 0.002 to 0.080 ± 0.059 ng/ml) but without statistical difference, except between gestation and the OV-2 stage for 3β5α20β-HHPROG (Figure 4; Table 2). Salivary concentrations of 3α5β20α- and 3β5α20α-HHPROG were not significantly different between the physiological stages. In plasma, 3α5α20α-, 3β5α20α- and 3β5α20β-HHPROG concentrations were significantly higher during luteal phase and gestation than during anestrus and the follicular phase (Figure 4). Plasmatic concentrations of 3α5β20α-HHPROG were significantly different between the day of ovulation and gestation. Salivary and plasmatic concentrations of 3α5β20β-HHPROG were not significantly different between the physiological stages (Table 2).
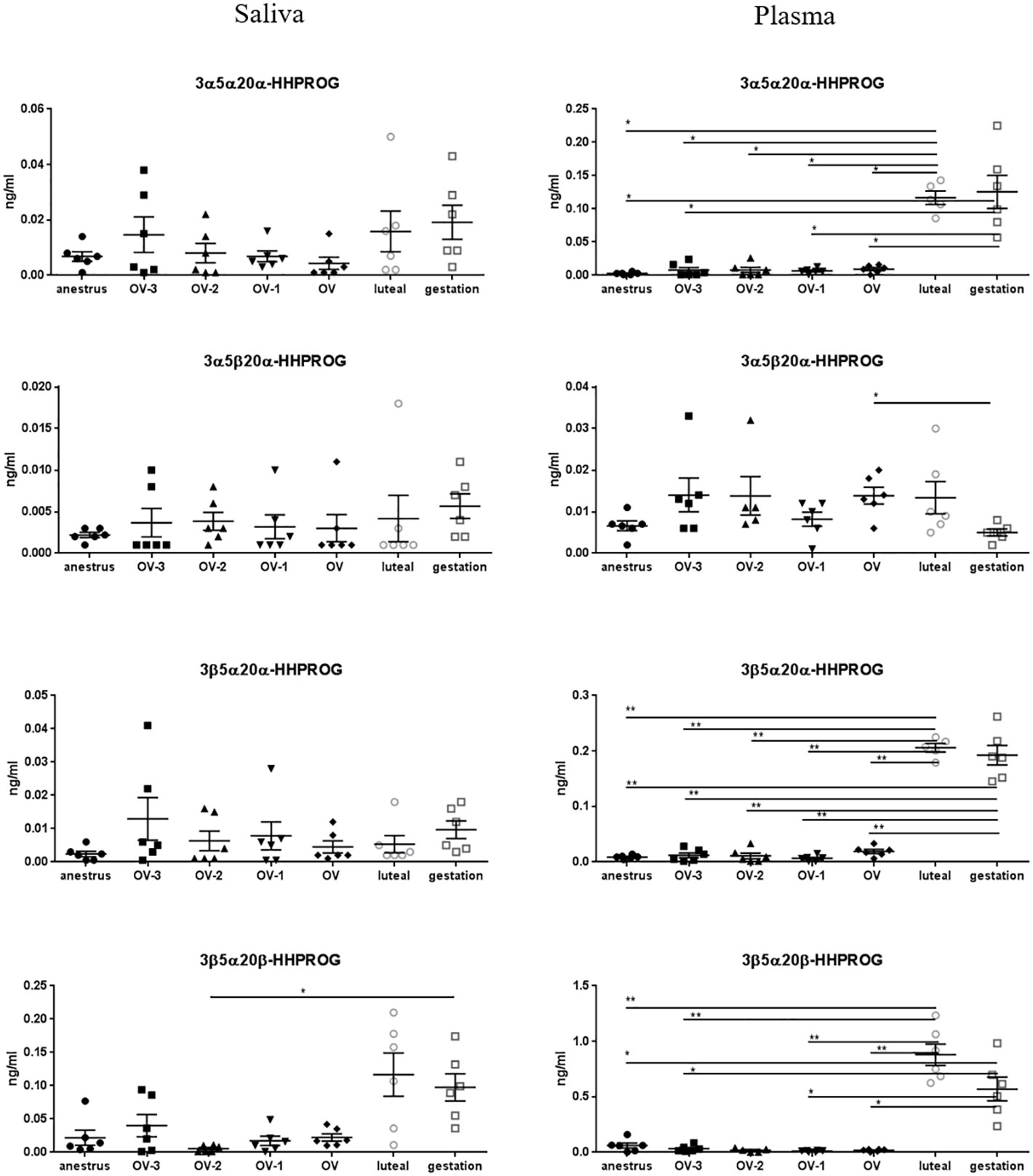
Figure 4 Gas chromatography coupled to tandem mass spectrometry (GC-MS/MS) measurements of the concentrations of 3α5α20α-hexahydroprogesterone (3α5α20α-HHPROG), 3α5β20α-HHPROG, 3β5α20α-HHPROG and 3β5α20β-HHPROG (ng/mL ± SEM) in mare saliva and plasma collected during anestrus, during follicular phase 3 days (OV-3), 2 days (OV-2) and 1 day (OV-1) before ovulation, the day when ovulation was detected (OV), during luteal phase 6 days post-ovulation (luteal), during gestation 18 days post-ovulation (gestation). For each steroid, the y-axis was standardized to the maximal concentration, so that the variations are easy-to-read. Statistical differences between physiological stages: * P < 0.05, ** P < 0.01.
The data for the main glucocorticoids are shown in Figure 5. No differences in saliva were observed between all the physiological stages for corticosterone, 11-dehydrocorticosterone and cortisone with concentrations lower than 0.16ng/ml, 0.06ng/ml and 0.81ng/ml respectively (Table 2). The other glucocorticoids were not detected in saliva. In plasma, corticosterone concentrations were higher in follicular phase relatively to anestrus, luteal phase and gestation. The same pattern was observed for cortisol although less pronounced. Plasmatic levels of 3β5α-THDOC were significantly higher during gestation than during follicular phase (Figure 5). Plasmatic concentrations of DOC, 3α5α-THDOC, 3α5β-THDOC, 11-dehydrocorticosterone, aldosterone and cortisone were not significantly different between physiological stages (Table 2).
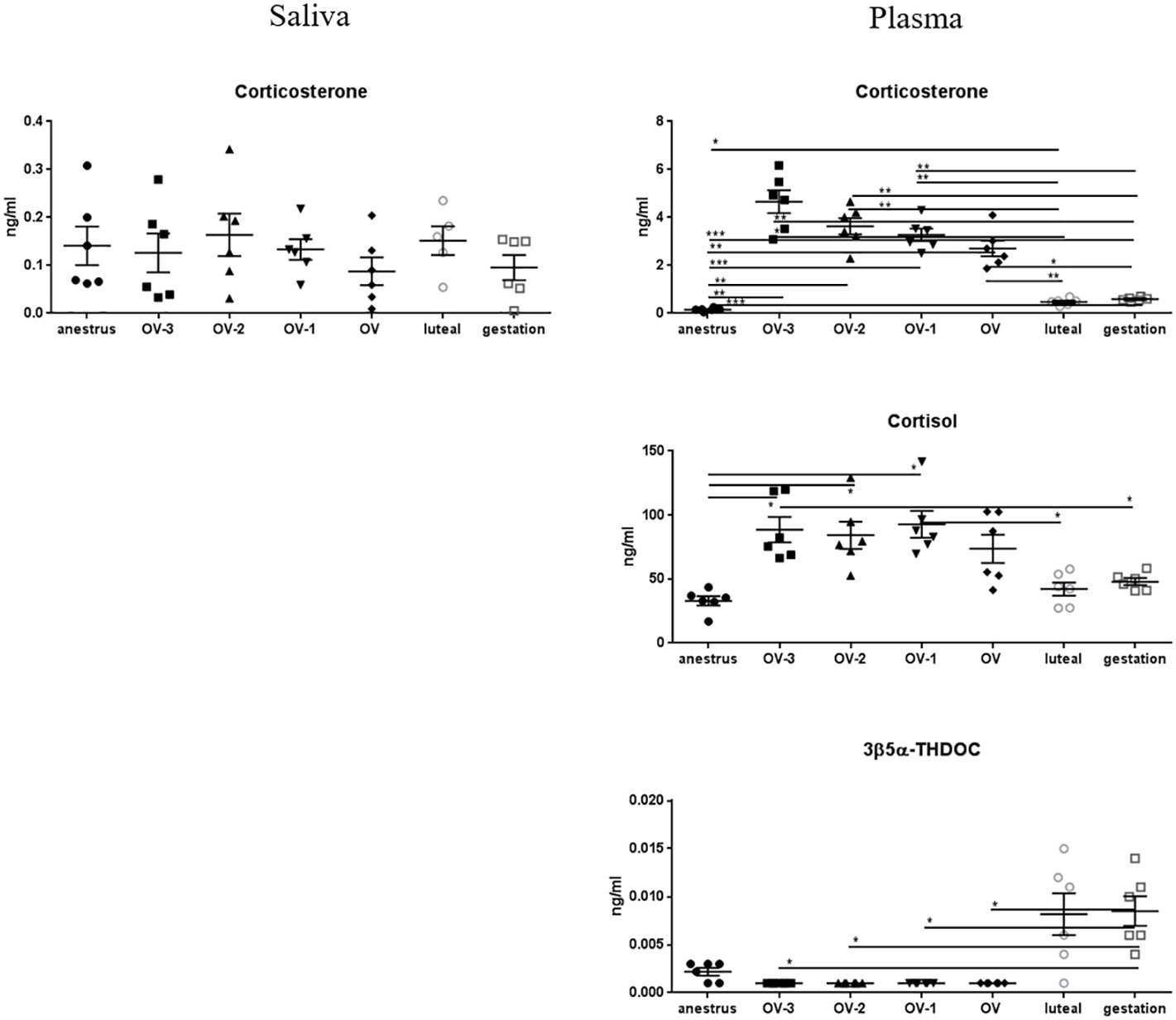
Figure 5 Gas chromatography coupled to tandem mass spectrometry (GC-MS/MS) measurements of the concentrations of corticosterone, cortisol and 3β5α-tetrahydrodeoxycorticosterone (3β5α-THDOC) (ng/mL ± SEM) in mare saliva and plasma collected during anestrus, during follicular phase 3 days (OV-3), 2 days (OV-2) and 1 day (OV-1) before ovulation, the day when ovulation was detected (OV), during luteal phase 6 days post-ovulation (luteal), during gestation 18 days post-ovulation (gestation). For each steroid, the y-axis was standardized to the maximal concentration, so that the variations are easy-to-read. Statistical differences between physiological stages: * P < 0.05, ** P < 0.01, *** P < 0.001.
Salivary concentrations of DHEA, ADIOL and DHEAS were lower than 0.03ng/ml and not significantly different between the physiological stages (Figure 6; Table 2). Plasmatic concentrations of DHEA, ADIOL and ADIONE were significantly higher during the follicular phase than during anestrus (for DHEA, ADIOL, ADIONE), luteal phase and gestation (for DHEA and ADIOL) (Figure 6; Table 2). No significant differences were observed in plasma for DHEAS and testosterone (Table 2). Estrone (E1), 17β-estradiol (17β-E2) and estriol (E3) concentrations in saliva were lower than 0.012ng/ml and not significantly different between the physiological stages, whereas plasmatic concentrations were significantly higher during the follicular phase than during anestrus (for E1, E2 and E3), luteal phase and gestation (for E1 and E3) (Figure 6; Table 2).
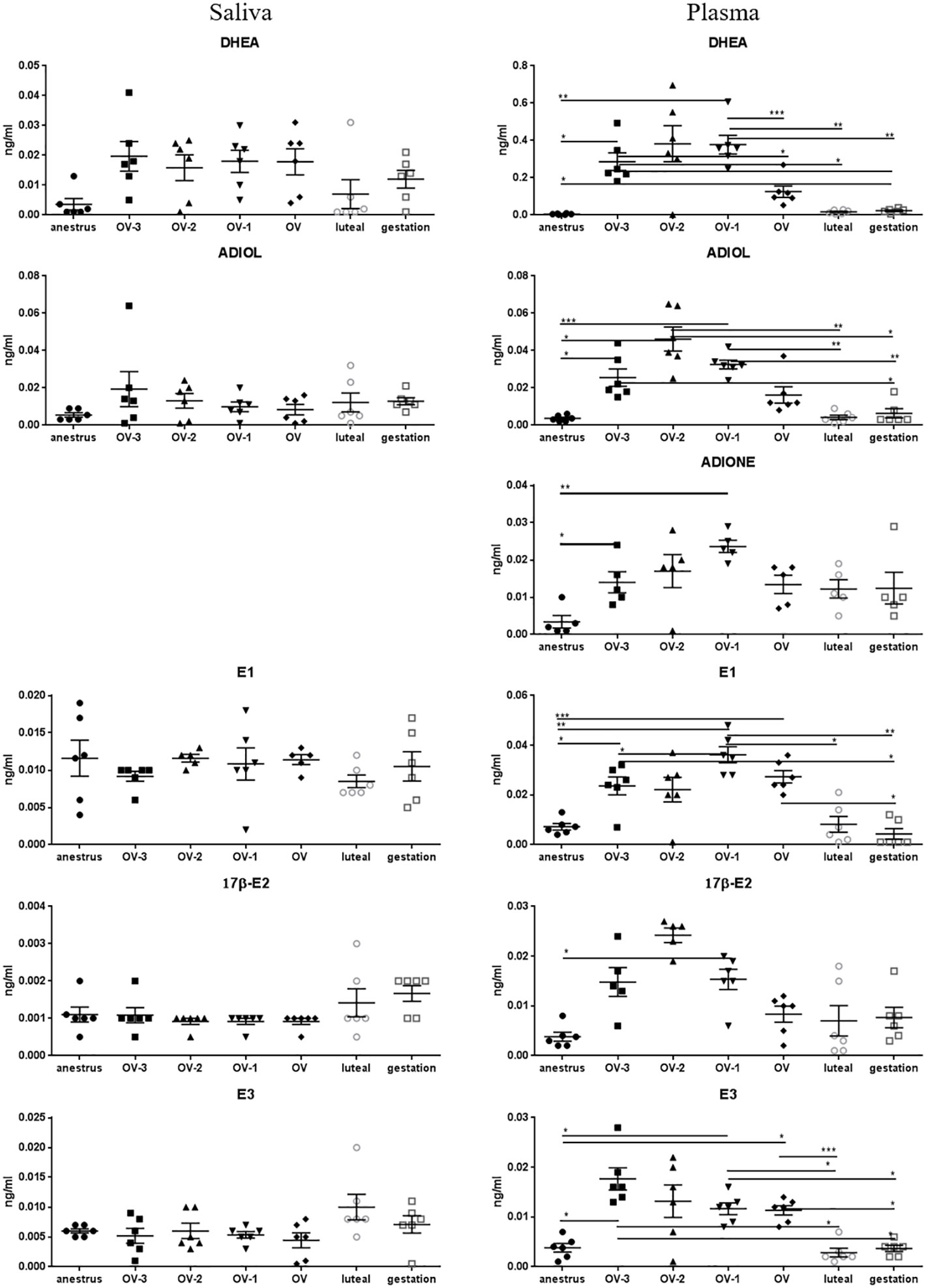
Figure 6 Gas chromatography coupled to tandem mass spectrometry (GC-MS/MS) measurements of the concentrations of dehydroepiandrosterone (DHEA), androstenediol (ADIOL), androstenedione (ADIONE), estrone (E1), 17β-estradiol (17β-E2) and estriol (E3) (ng/mL ± SEM) in mare saliva and plasma collected during anestrus, during follicular phase 3 days (OV-3), 2 days (OV-2) and 1 day (OV-1) before ovulation, the day when ovulation was detected (OV), during luteal phase 6 days post-ovulation (luteal), during gestation 18 days post-ovulation (gestation). For each steroid, the y-axis was standardized to the maximal concentration, so that the variations are easy-to-read. Statistical differences between physiological stages: * P < 0.05, ** P < 0.01, *** P < 0.001.
Interestingly, we found correlations between plasma and saliva levels specifically for a steroid family, the 5α-reduced metabolites of progesterone, that are the most abundant in mare saliva. We found correlations between plasma and saliva for 5α-DHPROG (r = 0.58, P = 0.0001), 3α5α-THPROG (r = 0.44, P = 0.0043), 3β5α-THPROG (r = 0.60, P < 0.0001), 3α5α20α-HHPROG (r = 0.45, P = 0.0038) and 3β5α20β-HHPROG (r = 0.58, P < 0.0001). No significant correlations were found for all other steroids.
Discussion
The aim of this prospective study was to analyse the steroidome in mare saliva during reproductive stages, that could help to identify potential steroidal biomarkers to accurately detect their reproductive stage in a welfare friendly production system. The use of GC-MS/MS to analyse multiple steroids with very closed chemical structures with high specificity and sensitivity provided opportunities to characterize for the first time a whole panel of steroids and their precursors and metabolites in saliva and plasma. The present study allowed the identification of steroids whose salivary concentrations were different between the physiological stages. These steroids could be potential salivary biomarkers of the reproductive stage of the mares.
PREG concentrations in saliva during gestation, 18 days after ovulation, were 3 to 7 times higher than during anestrus or follicular phase, and tend to be higher than during luteal phase. Interestingly, salivary and plasma concentrations are similar during anestrus and in the follicular phase, whereas during gestation salivary concentrations are 13 times higher than plasmatic one. PREG concentrations have been measured previously in plasma from thoroughbred mares throughout gestation by LC-MS/MS, and concentrations lower than 1ng/ml were detected for the 20 first weeks of gestation (Legacki et al., 2016). Relatively high PREG levels were also observed in porcine saliva from young gilts and human saliva from female children with concentrations from 0.45 to 1.47ng/ml and 1.16 to 1.99ng/ml respectively (Majewska et al., 2014; Goudet et al., 2019; Goudet et al., 2021). The high concentration of PREG observed in equine saliva during gestation, 18 days after ovulation, may be related to a local production of PREG by salivary glands. PREG biosynthesis occurs in the rat salivary gland and the synthetase CYP11A1, the PREG synthesizing enzyme, is expressed in rat parotid, submandibular and sublingual gland (Miyashita et al., 2011). Furthermore, Spaan and collaborators (Spaan et al., 2009) have shown by immunohistochemistry a strong expression of steroid sulfatase enzyme in the basal part of acinar cells, suggesting that PREG could also be produced locally from the precursor pregnenolone sulfate. However, the absence of correlation between plasma pregnenolone sulfate and salivary pregnenolone and between pregnenolone sulfate and pregnenolone in saliva (to detect a potential local synthesis of pregnenolone from pregnenolone sulfate) cannot support the steroid sulfatase hypothesis. Thus, a local biosynthesis of PREG in the equine salivary gland could be hypothesized, with an upregulation related to the beginning of pregnancy. Our results allow us to expect that PREG concentration in saliva from mated mares, collected 18 days after ovulation, would allow to distinguish between pregnant and non-pregnant mares. Studies with a greater number of animals are in progress to evaluate the variations of the steroids concentrations among individual animals and to compare salivary concentrations of PREG in pregnant and non-pregnant mares collected 18 days after ovulation. Moreover, the high concentrations of PREG during early gestation are technically compatible with the future development of a commercial immunoassay kit at low cost. Thus, PREG may be a potential salivary biomarker of early gestation in the mare with a possible application in the field. The development of a salivary test of early gestation would allow to avoid painful blood sampling for classical pregnancy diagnosis. Transrectal ultrasound scanning of the female genital tract would still be necessary to detect twin pregnancy. However, the development of a salivary test of early gestation would avoid invasive transrectal ultrasound scanning for non-pregnant mares.
Up to now, PROG concentrations in plasma collected 17 to 18 days after ovulation have been used as a pregnancy diagnosis to distinguish the pregnant from the non-pregnant mares (Irvine et al., 1990). Our study confirms that plasmatic PROG concentrations are significantly higher during early gestation than during anestrus or follicular phase. PROG is produced by the primary corpus luteum after ovulation in mares up to the luteo-placental shift occurring around 105-110 days of gestation. Then, PROG concentrations decrease and are undetectable in the second half of gestation (Scholtz et al., 2014; Conley, 2016). PROG biosynthesis by the corpus luteum explains why its level is similar between the luteal phase 6 days post-ovulation in cyclic mares and at 18 days post-ovulation in pregnant mares. Our results showed that PROG concentrations in saliva are not significantly different between early gestation and the other physiological stages. Thus, the evaluation of PROG concentration in saliva from mated mares, collected 18 days after ovulation, will not allow distinguishing the pregnant from the non-pregnant mares, and will not be adequate for a salivary pregnancy diagnosis.
In our conditions, PROG concentrations in saliva were also not significantly different between follicular and luteal phase. In human and buffalo, salivary PROG levels in luteal phase were significantly higher compared to those in the follicular phase (Lee et al., 2015; Ravinder et al., 2016). However, in these studies, PROG concentrations were estimated using immunoassay kits and cross-reactions of structurally similar steroids occur (Wynn et al., 2018b) explaining why PROG concentrations may have been overestimated by PROG metabolites, some of them showing salivary concentrations higher during luteal phase compared to follicular phase in our study. We found here that 5α-DHPROG levels are 10-fold higher in saliva than PROG and this could explain these discrepancies between immunoassays and mass spectrometric studies.
Among the 15 targeted metabolites of PROG (dihydro-, tetrahydro-, hexahydro- and hydroxyprogesterone), 12 were detected by GC-MS/MS analysis in saliva and 14 in plasma. Plasmatic concentrations of several PROG metabolites have been previously evaluated in pregnant mare throughout gestation (Scholtz et al., 2014; Legacki et al., 2016), in the late pregnant mare (Wynn et al., 2018a) and in foals (Müller et al., 2019). However, our study is the first to perform a complete analysis of PROG metabolites in equine saliva and plasma. Few studies have been performed on saliva from domestic mammals: salivary concentrations of PROG metabolites have only been measured in porcine saliva from gilts around puberty (Goudet et al., 2019; Goudet et al., 2021), bovine saliva from neonatal calves (Aleman et al., 2020) and human saliva from pre-pubertal children (Majewska et al., 2014). In plasma, we found that 5α-DHPROG has a similar pattern than its precursor PROG with increasing concentrations in the luteal phase and in the early pregnancy. Several studies have shown that biopotencies of PROG and 5α-DHPROG were comparable in horse, as in zebra, elephants and rock hyrax (Conley, 2016). Moreover, Scholtz and collaborators showed that 5α-DHPROG, produced in placenta, replaces PROG in blood of pregnant mares in the second half of gestation (Scholtz et al., 2014). 5α-DHPROG is also present in cyclic mare and is rapidly synthesized by the 5α-reductase enzyme from the luteal PROG. However, our data show a discrepancy with the previous studies. Indeed, we found here that 5α-DHPROG concentrations are roughly 10-fold higher than PROG in plasma in the luteal phase 6 days post-ovulation and in gestation 18 days post-ovulation. By contrast, Scholtz and collaborators (Scholtz et al., 2014) and Conley and collaborators (Conley et al., 2018) showed by LC-MS/MS that 5α-DHPROG levels were 60% of PROG in both physiological conditions. This discrepancy could be explained by the use of different breeds of mare and/or by the different analytical methods using different internal standards for the absolute quantification.
Interestingly, by contrast to PROG, we found that the concentrations of 5α-DHPROG tend to be higher in luteal phase and in early pregnancy in saliva. The higher lipophilicity of 5α-DHPROG as compared to PROG could explain this result. Indeed, lipid-soluble steroids such as 5α-DHPROG can be transferred rapidly from the blood to saliva by passive diffusion (Riad-Fahmy et al., 1982). A second hypothesis could be the potential presence of the 5α-reductase in the salivary glands to produce locally 5α-DHPROG.
As for 5α-DHPROG, most of the other 5α-reduced metabolites detected in our study (3α5α- and 3β5α-THPROG, 3α5α20α- and 3β5α20β-HHPROG, except 3β5α20α-HHPROG) are characterized by salivary concentrations higher during the luteal phase and gestation compared to anestrus and the follicular phase, but without reaching statistical difference. This can be explained by the small number of mares incorporated in our study. For all these 5α-reduced metabolites, plasmatic concentrations also followed the same pattern than PROG and 5α-DHPROG with much higher levels during gestation and luteal phase compared to anestrus and follicular phase. Moreover, salivary concentrations are about 10 times lower than plasmatic ones. This suggests that the 5α-reduced metabolites of PROG could also be carried from blood to saliva. This is supported by the significant correlation found between plasma and saliva levels for the 5α-reduced metabolites of progesterone, 5α-DHPROG, 3α5α-THPROG, 3β5α-THPROG, 3α5α20α-HHPROG and 3β5α20β-HHPROG. Thus, the levels of 5α-reduced metabolites of progesterone in saliva reflect their plasma levels. Their higher salivary concentrations during luteal phase and early gestation allow us to hypothesize that the 5α-reduced metabolites of PROG could be potential salivary biomarkers of luteal phase and early gestation, compared to anestrus and follicular phase. Further studies with a greater number of animals are necessary to confirm the reliability of these candidate biomarkers. Moreover, commercial immunoassays of the 5α-reduced metabolites of PROG without cross-reactivity to other pregnanes have to be developed in order to consider a potential low-cost diagnostic test in the field. On the other hand, non-specific antisera should be taken into consideration as “group-specific” reagents in order to assess PROG and its structurally closely related reduced metabolites in saliva to control ovarian function or for pregnancy diagnosis (Schwarzenberger et al., 1995; Schwarzenberger et al., 1998).
The salivary concentrations of the targeted glucocorticoids were low and not significantly different between all the physiological stages. Cortisol concentrations had been previously measured in equine saliva and basal concentrations were between 0.15ng/ml and 3ng/ml using enzyme immunoassay (Nagel et al., 2012; Diego et al., 2016; Schwinn et al., 2018; Contreras-Aguilar et al., 2021) and lower than 1ng/ml using LC-MS/MS (Sauer et al., 2020). A method comparison showed that LC-MS/MS analysis, based on the physicochemical properties of the analyte, provides more accurate results than immunoassays, based on interactions between antigens and antibodies, due to cross-reactions of structurally similar steroids during immunoassay measurements (Sauer et al., 2020). In our conditions using GC-MS/MS, the salivary concentrations of glucocorticoids did not allow to differentiate the physiological stages. In particular, even if plasmatic cortisol concentrations were higher at the end of the follicular phase than in anestrus, luteal phase and gestation, GC-MS/MS analysis were not sufficiently sensitive to measure cortisol in saliva.
Among androstanes, DHEA, DHEAS and ADIOL were detected in equine saliva, but their salivary concentrations were not significantly different between physiological stages. Similarly, DHEA salivary concentrations in women were not significantly different throughout the menstrual cycle (Lee et al., 2015). However, some androstanes have been shown to be salivary biomarkers of some physiological stages. DHEA and ADIOL have been detected in porcine saliva and identified as biomarkers of the physiological status of prepubertal gilts (Goudet et al., 2019; Goudet et al., 2021). In human, salivary DHEAS concentrations are significantly increased during the second-third trimester of pregnancy compared to non-pregnant women (Sundararajan et al., 2021). Salivary DHEA and DHEAS levels are related to children pubertal development (Netherton et al., 2004; Whittle et al., 2020; Goldberg et al., 2021). Our data show higher plasma levels of DHEA and ADIOL in the follicular phase. We hypothesized that ovarian synthesis of these estrogenic precursors are upregulated and are the supply for increased 17β-E2 production and secretion at the end of the follicular phase. In mare pregnancy, the fetal gonadal androgens feed the estrogen secretion, highlighting the feto-placental unit (Conley, 2016). In our conditions, DHEA, DHEAS and ADIOL are not relevant salivary biomarkers of the targeted physiological stages in the mare.
E1, 17β-E2 and E3 were detected both in equine saliva and plasma. Salivary concentrations were not significantly different between the physiological stages, whereas plasmatic concentrations were higher in the follicular phase. Similarly, E1, 17β-E2 and E3 were detected in human saliva (Wingeier et al., 2017; Li et al., 2018). However, 17β-E2 salivary concentrations in women have been shown to be either high around ovulation (Munk et al., 2018) or not significantly different throughout the menstrual cycle (Lee et al., 2015). Few data are available on domestic mammals: E1, 17β-E2 and E3 were detected in saliva of prepubertal gilts (Goudet et al., 2019; Goudet et al., 2021), 17β-E2 was detected in saliva of pregnant sows (Jiang et al., 2019), male goats (Samir et al., 2020) and female buffalo with salivary 17β-E2 levels higher at the estrus stage than at the diestrus stage (Ravinder et al., 2016). To our knowledge, this is the first time that an estrogenic profile is determined in equine saliva. In our conditions, estranes are not relevant salivary biomarkers of the physiological stages in the mare.
Conclusion
Our aim was to analyse the steroidome of mare saliva during reproductive stages, that could help to identify potential steroidal biomarkers to accurately detect their reproductive stage in a welfare friendly production system. We identified steroids whose salivary concentrations were different between the targeted physiological stages. PREG concentrations in saliva were significantly higher during early gestation and the 5α-reduced metabolites of PROG showed higher concentrations during luteal phase and gestation. These steroids could be potential salivary biomarker of the reproductive stage of the mare. However, further studies with a greater number of animals are in progress to confirm the reliability of these candidate biomarkers and to develop field-friendly assays. This prospective study shows that steroidome analysis in saliva could help to improve the precision of livestock reproduction management in a welfare friendly production system.
Data availability statement
The original contributions presented in the study are included in the article/Supplementary Material. Further inquiries can be directed to the corresponding author.
Ethics statement
The animal study was reviewed and approved by Comité d’éthique en Expérimentation Animale Val de Loire n°019.
Author contributions
GG: conceptualization, methodology, validation, formal analysis, investigation, writing, visualization, supervision, funding acquisition. CD: methodology, validation, investigation, resources, writing. AP: methodology, validation, investigation, resources. LH: methodology, validation, investigation, resources. FR: conceptualization, methodology, investigation, resources SD: conceptualization, writing, funding acquisition. PL: conceptualization, methodology, validation, formal analysis, investigation, writing, visualization. All authors contributed to the article and approved the submitted version.
Funding
This work was supported by INRAE, IFCE and University of Liège.
Acknowledgments
We would like to thank the staff of the experimental stud, A. Gesbert, P. Barrière, T. Blard, Y. Gaude.
Conflict of interest
The authors declare that the research was conducted in the absence of any commercial or financial relationships that could be construed as a potential conflict of interest.
Publisher’s note
All claims expressed in this article are solely those of the authors and do not necessarily represent those of their affiliated organizations, or those of the publisher, the editors and the reviewers. Any product that may be evaluated in this article, or claim that may be made by its manufacturer, is not guaranteed or endorsed by the publisher.
Supplementary material
The Supplementary Material for this article can be found online at: https://www.frontiersin.org/articles/10.3389/fanim.2022.1055179/full#supplementary-material
References
Aleman M., Chigerwe M., Varga A., Madigan J. E. (2020). Steroid precursors, steroids, neuroactive steroids, and neurosteroids concentrations in serum and saliva of healthy neonatal heifer Holstein calves. J. Veterinary Internal Med. 34 (6), 2767–2775. doi: 10.1111/jvim.15957
Anastassiades M., Mastovská K., Lehotay S. J. (2003). Evaluation of analyte protectants to improve gas chromatographic analysis of pesticides. J. Chromatogr A 1015 (1-2), 163–184. doi: 10.1016/s0021-9673(03)01208-1
Aurich C. (2011). Reproductive cycles of horses. Anim. Reprod. Sci. 124 (3-4), 220–228. doi: 10.1016/j.anireprosci.2011.02.005
Canepa S., Laine A.-L., Bluteau A., Fagu C., Flon C., Monniaux D. (2008). Validation d'une méthode immunoenzymatique pour le dosage de la progestérone dans le plasma des ovins et des bovins. Cahier Des. Techniques l'INRA 64, 19–30. Available at: https://hal.inrae.fr/hal-02667281
Chamkasem N., Harmon T. (2016). Direct determination of glyphosate, glufosinate, and AMPA in soybean and corn by liquid chromatography/tandem mass spectrometry. Anal. Bioanal Chem. 408 (18), 4995–5004. doi: 10.1007/s00216-016-9597-6
Conley A. J. (2016). Review of the reproductive endocrinology of the pregnant and parturient mare. Theriogenology 86 (1), 355–365. doi: 10.1016/j.theriogenology.2016.04.049
Conley A. J., Scholtz E. L., Legacki E. L., Corbin C. J., Knych H. K., Dujovne G. D., et al. (2018). 5α-dihydroprogesterone concentrations and synthesis in non-pregnant mares. J. Endocrinol. 238 (1), 25–32. doi: 10.1530/joe-18-0215
Contreras-Aguilar M. D., Cerón J. J., Muñoz A., Ayala I. (2021). Changes in saliva biomarkers during a standardized increasing intensity field exercise test in endurance horses. Animal 15 (6), 100236. doi: 10.1016/j.animal.2021.100236
Davies Morel M. C. G., Newcombe J. R., Holland S. J. (2002). Factors affecting gestation length in the thoroughbred mare. Anim. Reprod. Sci. 74 (3), 175–185. doi: 10.1016/S0378-4320(02)00171-9
Diego R., Douet C., Reigner F., Blard T., Cognie J., Deleuze S., et al. (2016). Influence of transvaginal ultrasound-guided follicular punctures in the mare on heart rate, respiratory rate, facial expression changes, and salivary cortisol as pain scoring. Theriogenology 86 (7), 1757–1763. doi: 10.1016/j.theriogenology.2016.05.040
Dufour P., Courtois J., Seynaeve Y., Peeters S., Le Goff C., Cavalier E., et al. (2021). Development and validation of a liquid chromatography coupled to mass spectrometer (LC-MS) method for the simultaneous quantification of estrone-3-sulfate, progesterone, estrone and estradiol in serum of mares and American bisons. Res. Vet. Sci. 136, 343–350. doi: 10.1016/j.rvsc.2021.03.014
Genangeli M., Caprioli G., Cortese M., Laus F., Matteucci M., Petrelli R., et al. (2017). Development and application of a UHPLC-MS/MS method for the simultaneous determination of 17 steroidal hormones in equine serum. J. Mass Spectrom 52 (1), 22–29. doi: 10.1002/jms.3896
Ginther O. J., Gastal E. L., Gastal M. O., Beg M. A. (2005). Regulation of circulating gonadotropins by the negative effects of ovarian hormones in mares. Biol. Reprod. 73 (2), 315–323. doi: 10.1095/biolreprod.105.040253
Goldberg M., Ciesielski Jones A. J., McGrath J. A., Barker-Cummings C., Cousins D. S., Kipling L. M., et al. (2021). Urinary and salivary endocrine measurements to complement tanner staging in studies of pubertal development. PLoS One 16 (5), e0251598. doi: 10.1371/journal.pone.0251598
Goudet G., Liere P., Pianos A., Fernandez N., Cambourg A., Savoie J., et al. (2019). Evolution of steroid concentrations in saliva from immature to pubertal gilts for the identification of biomarkers of gilts receptivity to boar effect. Livestock Sci. 228, 5–17. doi: 10.1016/j.livsci.2019.07.018
Goudet G., Prunier A., Nadal-Desbarats L., Grivault D., Ferchaud S., Pianos A., et al. (2021). Steroidome and metabolome analysis in gilt saliva to identify potential biomarkers of boar effect receptivity. Animal 15 (2), 100095. doi: 10.1016/j.animal.2020.100095
Irvine C. H. G., Sutton P., Turner J. E., Mennick P. E. (1990). Changes in plasma progesterone concentrations from days 17 to 42 of gestation in mares maintaining or losing pregnancy. Equine Veterinary J. 22 (2), 104–106. doi: 10.1111/j.2042-3306.1990.tb04219.x
Jiang X., Lu N., Xue Y., Liu S., Lei H., Tu W., et al. (2019). Crude fiber modulates the fecal microbiome and steroid hormones in pregnant meishan sows. Gen. Comp. Endocrinol. 277, 141–147. doi: 10.1016/j.ygcen.2019.04.006
Lamy J., Liere P., Pianos A., Aprahamian F., Mermillod P., Saint-Dizier M. (2016). Steroid hormones in bovine oviductal fluid during the estrous cycle. Theriogenology 86 (6), 1409–1420. doi: 10.1016/j.theriogenology.2016.04.086
Ledeck J., Dufour P., Evrard É., Le Goff C., Peeters S., Brutinel F., et al. (2022). Evolution of 17-β-estradiol, estrone and estrone-sulfate concentrations in late pregnancy of different breeds of mares using liquid chromatography and mass spectrometry. Theriogenology 189, 86–91. doi: 10.1016/j.theriogenology.2022.06.004
Lee Y. H., Kim Y. Y., Chang J. Y., Kho H. S. (2015). Changes in oral mucosal MUC1 expression and salivary hormones throughout the menstrual cycle. Oral. Dis. 21 (8), 962–968. doi: 10.1111/odi.12367
Legacki E. L., Scholtz E. L., Ball B. A., Stanley S. D., Berger T., Conley A. J. (2016). The dynamic steroid landscape of equine pregnancy mapped by mass spectrometry. Reproduction 151 (4), 421–430. doi: 10.1530/rep-15-0547
Liere P., Schumacher M. (2015). Mass spectrometric analysis of steroids: all that glitters is not gold. Expert Rev. Endocrinol. Metab. 10 (5), 463–465. doi: 10.1586/17446651.2015.1063997
Li X. S., Li S., Kellermann G. (2018). Simultaneous determination of three estrogens in human saliva without derivatization or liquid-liquid extraction for routine testing via miniaturized solid phase extraction with LC-MS/MS detection. Talanta 178, 464–472. doi: 10.1016/j.talanta.2017.09.062
Majewska M. D., Hill M., Urbanowicz E., Rok-Bujko P., Bienkowski P., Namyslowska I., et al. (2014). Marked elevation of adrenal steroids, especially androgens, in saliva of prepubertal autistic children. Eur. Child Adolesc. Psychiatry 23 (6), 485–498. doi: 10.1007/s00787-013-0472-0
Mastovská K., Lehotay S. J., Anastassiades M. (2005). Combination of analyte protectants to overcome matrix effects in routine GC analysis of pesticide residues in food matrixes. Analytical Chem. 77 (24), 8129–8137. doi: 10.1021/ac0515576
Miyashita T., Okubo M., Shinomiya T., Nakagawa K., Kawaguchi M. (2011). Pregnenolone biosynthesis in the rat salivary gland and its inhibitory effect on secretion. J. Pharmacol. Sci. 115 (1), 56–62. doi: 10.1254/jphs.10267fp
Müller V., Curcio B. R., Toribio R. E., Feijó L. S., Borba L. A., Canisso I. F., et al. (2019). Cortisol, progesterone, 17αOHprogesterone, and pregnenolone in foals born from mare's hormone-treated for experimentally induced ascending placentitis. Theriogenology 123, 139–144. doi: 10.1016/j.theriogenology.2018.06.024
Munk R., Jensen R. B., Palme R., Christensen J. W. (2016). The effect of sex and time of day on testosterone concentrations in equine saliva and serum. Comp. Exercise Physiol. 12 (4), 177–182. doi: 10.3920/cep160020
Munk A. J. L., Zoeller A. C., Hennig J. (2018). Fluctuations of estradiol during women's menstrual cycle: Influences on reactivity towards erotic stimuli in the late positive potential. Psychoneuroendocrinology 91, 11–19. doi: 10.1016/j.psyneuen.2018.02.028
Nagel C., Erber R., Bergmaier C., Wulf M., Aurich J., Möstl E., et al. (2012). Cortisol and progestin release, heart rate and heart rate variability in the pregnant and postpartum mare, fetus and newborn foal. Theriogenology 78 (4), 759–767. doi: 10.1016/j.theriogenology.2012.03.023
Netherton C., Goodyer I., Tamplin A., Herbert J. (2004). Salivary cortisol and dehydroepiandrosterone in relation to puberty and gender. Psychoneuroendocrinology 29 (2), 125–140. doi: 10.1016/s0306-4530(02)00150-6
Olesti E., Boccard J., Visconti G., González-Ruiz V., Rudaz S. (2021). From a single steroid to the steroidome: Trends and analytical challenges. J. Steroid Biochem. Mol. Biol. 206, 105797. doi: 10.1016/j.jsbmb.2020.105797
Panzani D., Di Vita M., Lainé A.-L., Guillaume D., Rota A., Tesi M., et al. (2017). Corpus luteum vascularization and progesterone production in autumn and winter cycles of the mare: Relationship between ultrasonographic characteristics of corpora lutea and plasma progesterone concentration in the last cycles before anestrus. J. Equine Veterinary Sci. 56, 35–39. doi: 10.1016/j.jevs.2017.05.001
Pillet E., Batellier F., Duchamp G., Furstoss V., Vern Y. L. E., Kerboeuf D., et al. (2008). Freezing stallion semen in INRA96®-based extender improves fertility rates in comparison with INRA82. Dairy Sci. Technol. 88 (2), 257–265. doi: 10.1051/dst:2008002
Ravinder R., Kaipa O., Baddela V. S., Singhal Sinha E., Singh P., Nayan V., et al. (2016). Saliva ferning, an unorthodox estrus detection method in water buffaloes (Bubalus bubalis). Theriogenology 86 (5), 1147–1155. doi: 10.1016/j.theriogenology.2016.04.004
Riad-Fahmy D., Read G. F., Walker R. F., Griffiths K. (1982). Steroids in saliva for assessing endocrine function. Endocr. Rev. 3 (4), 367–395. doi: 10.1210/edrv-3-4-367
Samir H., Nyametease P., Elbadawy M., Fathi M., Mandour A. S., Radwan F., et al. (2020). Assessment of correlations and concentrations of salivary and plasma steroids, testicular morphometry, and semen quality in different climatic conditions in goats. Theriogenology 157, 238–244. doi: 10.1016/j.theriogenology.2020.08.002
Sauer F. J., Gerber V., Frei S., Bruckmaier R. M., Groessl M. (2020). Salivary cortisol measurement in horses: immunoassay or LC-MS/MS? Domest Anim. Endocrinol. 72, 106445. doi: 10.1016/j.domaniend.2020.106445
Schenck F. J., Lehotay S. J. (2000). Does further clean-up reduce the matrix enhancement effect in gas chromatographic analysis of pesticide residues in food? J. Chromatogr A 868 (1), 51–61. doi: 10.1016/s0021-9673(99)01137-1
Scholtz E. L., Krishnan S., Ball B. A., Corbin C. J., Moeller B. C., Stanley S. D., et al. (2014). Pregnancy without progesterone in horses defines a second endogenous biopotent progesterone receptor agonist, 5α-dihydroprogesterone. Proc. Natl. Acad. Sci. U.S.A. 111 (9), 3365–3370. doi: 10.1073/pnas.1318163111
Schumacher M., Guennoun R., Mattern C., Oudinet J. P., Labombarda F., De Nicola A. F., et al. (2015). Analytical challenges for measuring steroid responses to stress, neurodegeneration and injury in the central nervous system. Steroids 103, 42–57. doi: 10.1016/j.steroids.2015.08.013
Schwarzenberger F., Speckbacher G., Bamberg E. (1995). Plasma and fecal progestagen evaluations during and after the breeding season of the female vicuna (Vicuna vicuna ). Theriogenology 43 (3), 625–634. doi: 10.1016/0093-691x(94)00068-6
Schwarzenberger F., Walzer C., Tomasova K., Vahala J., Meister J., Goodrowe K. L., et al. (1998). Faecal progesterone metabolite analysis for non-invasive monitoring of reproductive function in the white rhinoceros (Ceratotherium simum). Anim. Reprod. Sci. 53 (1-4), 173–190. doi: 10.1016/s0378-4320(98)00112-2
Schwinn A. C., Sauer F. J., Gerber V., Bruckmaier R. M., Gross J. J. (2018). Free and bound cortisol in plasma and saliva during ACTH challenge in dairy cows and horses. J. Anim. Sci. 96 (1), 76–84. doi: 10.1093/jas/skx008
Spaan M., Porola P., Laine M., Rozman B., Azuma M., Konttinen Y. T. (2009). Healthy human salivary glands contain a DHEA-sulphate processing intracrine machinery, which is deranged in primary sjögren's syndrome. J. Cell Mol. Med. 13 (7), 1261–1270. doi: 10.1111/j.1582-4934.2009.00727.x
Sundararajan A., Vora K., Saiyed S., Natesan S. (2021). Comparative profiling of salivary cortisol and salivary DHEA-s among healthy pregnant and non-pregnant women. Horm. Metab. Res. 53 (9), 602–607. doi: 10.1055/a-1551-3722
Téteau O., Liere P., Pianos A., Desmarchais A., Lasserre O., Papillier P., et al. (2022). Bisphenol s alters the steroidome in the preovulatory follicle, oviduct fluid and plasma in ewes with contrasted metabolic status. Front. Endocrinol. (Lausanne) 13. doi: 10.3389/fendo.2022.892213
Whittle S., Barendse M., Pozzi E., Vijayakumar N., Simmons J. G. (2020). Pubertal hormones predict sex-specific trajectories of pituitary gland volume during the transition from childhood to adolescence. Neuroimage 204, 116256. doi: 10.1016/j.neuroimage.2019.116256
Wingeier M., La Marca-Ghaemmaghami P., Zimmermann R., Ehlert U. (2017). Is salivary estriol detectable in very early pregnancy? J. maternal-fetal neonatal Med. 30 (2), 228–232. doi: 10.3109/14767058.2016.1169522
Wudy S. A., Schuler G., Sánchez-Guijo A., Hartmann M. F. (2018). The art of measuring steroids: Principles and practice of current hormonal steroid analysis. J. Steroid Biochem. Mol. Biol. 179, 88–103. doi: 10.1016/j.jsbmb.2017.09.003
Wynn M. A. A., Ball B. A., Legacki E., Conley A., Loux S., May J., et al. (2018a). Inhibition of 5α-reductase alters pregnane metabolism in the late pregnant mare. Reproduction 155 (3), 251–258. doi: 10.1530/rep-17-0380
Keywords: steroids, saliva, plasma, equine, anestrus, follicular phase, luteal phase, pregnancy
Citation: Goudet G, Douet C, Pianos A, Haddad L, Reigner F, Deleuze S and Liere P (2022) Saliva and plasma steroidome in mare during reproductive stages: A GC-MS/MS study. Front. Anim. Sci. 3:1055179. doi: 10.3389/fanim.2022.1055179
Received: 27 September 2022; Accepted: 18 October 2022;
Published: 31 October 2022.
Edited by:
Suresh Neethirajan, Wageningen University and Research, NetherlandsReviewed by:
Dale Kelley, Oklahoma State University, United StatesAlan Conley, University of California, Davis, United States
Copyright © 2022 Goudet, Douet, Pianos, Haddad, Reigner, Deleuze and Liere. This is an open-access article distributed under the terms of the Creative Commons Attribution License (CC BY). The use, distribution or reproduction in other forums is permitted, provided the original author(s) and the copyright owner(s) are credited and that the original publication in this journal is cited, in accordance with accepted academic practice. No use, distribution or reproduction is permitted which does not comply with these terms.
*Correspondence: Ghylène Goudet, Z2h5bGVuZS5nb3VkZXRAaW5yYWUuZnI=