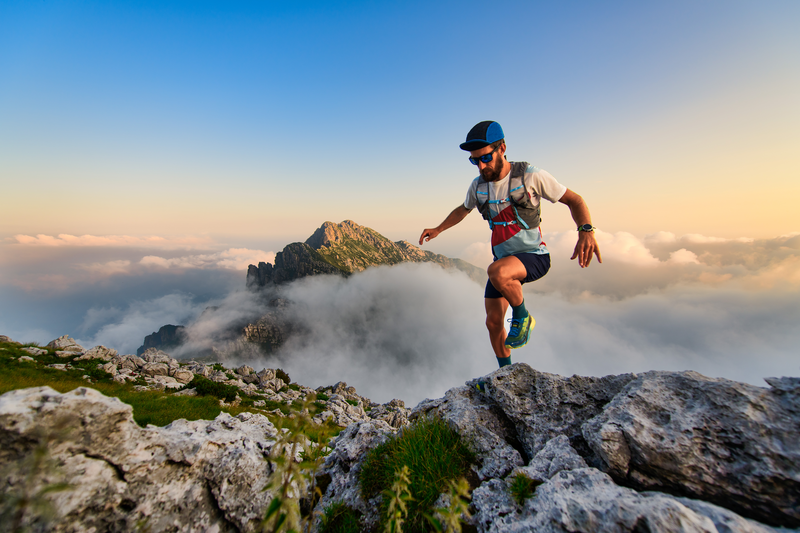
95% of researchers rate our articles as excellent or good
Learn more about the work of our research integrity team to safeguard the quality of each article we publish.
Find out more
HYPOTHESIS AND THEORY article
Front. Allergy , 21 November 2024
Sec. Infections and Microbiome
Volume 5 - 2024 | https://doi.org/10.3389/falgy.2024.1478279
This article is part of the Research Topic Hypotheses Explaining the Allergy Epidemic View all 11 articles
The Acari Hypothesis posits that acarians, i.e., mites and ticks, are causative agents of IgE-mediated conditions. This report further develops The Hypothesis, providing rationale for the childhood predilection of allergy. In short, Malassezia, a fungus native to human skin and utterly dependent on sebaceous lipids, prevents allergy by deterring acarians. Because sebum output is limited before puberty, children are more prone to allergy than are adults. Competition for sebaceous lipids by Staphylococcus aureus influences not only Malassezia number—and, consequently, allergic predisposition—but also lipid homeostasis. The latter, in turn, contributes to dyslipidemia and associated conditions, e.g., the metabolic syndrome.
Per the fourth installment of The Acari Hypothesis, modern hygienic practices disrupt human eccrine gland secretion, i.e., sweat, effectively increasing human—acarian interactions responsible for IgE-mediated allergic diseases (1). Although The Hypothesis and its corollaries provide rationales for why and how modern hygienic practices account for the ongoing allergy epidemic (2–5), the childhood predilection of some allergic diseases begs clarification. Indeed, the most remarkable epidemiologic finding shared by asthma, food allergy and atopic dermatitis is their increased incidence during childhood (6–8). Because IgE-mediated diseases are elicited by acarians acting on human epithelial surfaces, it is reasonable to assume epithelial surfaces of adults differ from those of children in a way that limits acarian activity. In this regard, the most conspicuous difference between the skin of pre- and post-pubertal humans is magnitude of sebum output by sebaceous glands (9, 10).
Sebum consists of a complex mixture of lipids that includes triglycerides, squalene, wax esters, cholesterol esters, free cholesterol, and fatty acids, Table 1 (11). Following puberty, sebum production increases 5-fold (9). Enhanced production continues through the seventh decade, after which the androgenic stimulation driving it decreases (9). Importantly, sebum output influences colonization of human skin by the lipid-dependent basidiomycete, Malassezia. Following puberty, Malassezia becomes the dominant eukaryote of the cutaneous microbiome (12), with malassezial colonization increasing by more than an order of magnitude (13, 14).
Table 1. Lipid composition of human sebum (11).
Because the behavior of Malassezia ranges from opportunism to commensalism to guardianship, the relationship between the fungus and humans has been difficult to characterize (12). The perception of Malassezia as opportunistic pathogen stems from its apparent etiologic involvement in many pathologies, most especially atopic dermatitis (AD) and seborrheic dermatitis (SD) (15–19). In the case of AD, afflicted persons synthesize IgE against an array of malassezial molecules (20, 21). According to The Hypothesis, the extensive targeting of such molecules by IgE indicates Malassezia is problematic for acarians (5). For this reason and because increased epithelial colonization by Malassezia aligns temporally with decreased incidence of allergic disease, the fungus participates in the anti-acarian defense of humans.
Although the hosting of fungi as a means of anti-acarian defense has never been described for mammals, mutualism of this sort has been described for plants. Indeed, some plants host fungal endophytes that protect them from bacterial and fungal pathogens and from phytophagous arthropods, including acarians (22). Fungal endophytes defend plants from acarians either via secretion of mycoacaricidal agents or via direct acarian inoculation (22).
Endophytic species of the basidiomycete, Meira, exemplify the acaropathogenic benefits fungal endophytes confer to host plants (23, 24). Meira geulakonigii protects citrus plants by killing the rust mite, Phyllocoptruta oleivora (25, 26). Culture medium from M. geulakonigii is acaricidal, indicating its anti-acarian activity is due to a toxin, not to fungal parasitism. As another example, Meira argove produces the mycoacaricide, argovin (4,5-dihydroxyindan-1-one), the anti-acarian effects of which have been well-characterized (27).
Given that other basidiomycetes protect their hosts from acarian parasitism, it is entirely reasonable to assume Malassezia protects humans from similar fate. Positioning Malassezia within sebaceous glands that constitutively secrete sebum provides a convenient means to deliver a Malassezia-derived anti-acarian agent to an epidermal surface. If humans provide to the fungus an essential nutrient whilst the fungus protects humans from acarian infestation, then the relationship between humans and Malessezia is most appropriately considered mutualistic. Such mutualism surely influences the physiology and pathophysiology of humans in unappreciated ways.
As for the involvement of Malassezia in human disease, firstly, not all Malassezia are constituents of normal skin flora, with some cross-colonization occurring due to cohabitation of humans and domestic mammals (28). Secondly, human sweat creates a microenvironmental ecosystem unfavorable not only to acarians, but also to a multitude of other microorganisms (29). Thus, even as disruption of the ecosystem by, for example, hygienic measures, enables problematic encounters between acarians and humans, it also enables problematic encounters between other invasive microorganisms and native Malassezia. As will be discussed next, Malassezia-associated diseases of humans are consequences of competition between native epidermal Malassezia and invasive organisms, e.g., Staphylococcus aureus.
Malassezia is a lipophilic basidiomycete that inhabits epithelia of warm-blooded animals (30). The fungal genus is the only one included in class Malasseziomycetes, subphylum Ustilaginomycotina, a taxon consisting primarily of plant pathogens (31). To date, 18 species of Malassezia have been identified (32).
Despite a requirement for long chain fatty acids, Malassezia lacks a gene for fatty acid synthase (33), rendering the fungus the only free-living one not able to synthesize fatty acids (34). Consequently, Malassezia must exploit exogenous lipid sources to survive. Human epidermis, upon which is constitutively secreted an abundance of fatty acids (35), is an ideal environment for malassezial colonization and propagation.
Although Malassezia subsists on the lipid-rich secretions of mammalian sebaceous glands, the relationship between mammals and Malassezia has, to date, not been considered mutualistic because: (1) colonization by the fungus has not been appreciated to confer substantial benefit to mammalian hosts, and (2) the fungus seems to play a role in some human diseases (12). As an important example of the latter, individuals with AD express anti-malassezial IgE (20, 21), which may influence the symptoms of the disorder (4).
Pathogen recognition receptors (PRRs) are utilized by invertebrates and vertebrates to identify and neutralize deleterious materials. As an example of invertebrate usage, ticks secrete into their saliva immunoglobulin-binding proteins that adsorb to and neutralize immunoglobulins ingested during a blood meal (36). Per The Hypothesis, mammals exploit acarian PRRs in the formation of IgE, to protect themselves from acarian vectorial activity (3). The anti-acarian specificity of IgE follows from how allergenicity is borne and conveyed. Namely, following complexation with an acarian PRR within the acarian digestive tract, substances become targeted by IgE when inoculated into a human, e.g., during an invasive encounter (3). IgE-targeted materials derive from either the acarian or its foodstuffs. The targeting of malassezial molecules by IgE indicates those molecules contributed to the diet of the operative acarian (5).
The ubiquity of acarians ensures frequent encounters with humans. Examples include well-defined ectoparasitism by Sarcoptes scabiei, Demodex spp. and a multitude of tick species. More subtle encounters involve synanthropic Pyroglyphidae, e.g., Dermatophagoides pteronyssinus and Dermatophagoides farinae. Indeed, these house dust mites may contribute most to the development of allergy: (1) they exist in increased number on the skin and in the homes of patients with IgE-mediated disease (37, 38), and (2) they are intimately associated with sources of common allergens. With regard to this last, note especially that house dust mites consume human and pet dander, fungi and wheat, and they use cockroaches as phoretic hosts (39–42).
Given that the primary foodstuffs of dust mites are human epidermal materials, the digestive tract of dust mites must be exposed to malassezial elements routinely (43). Furthermore, because most skin flora are not targeted by IgE, the specificity of the antibody for malassezial materials indicates a special affinity of the acarian PRR for Malassezia. For this reason, and because the increase in malassezial colonization that follows puberty coincides with the decrease in the incidence of allergy, it is reasonable to assume Malassezia is involved in the anti-acarian defense of humans.
Humans are not the only animals with sebaceous glands. Indeed, all extant mammalian lineages either now express sebaceous glands or did in the past (44–46). Like humans, many of the other mammals that have sebaceous glands host Malassezia (47–49). Because those mammals, too, are subject to acarian parasitism, sebaceous glands somehow effectively ward off acarians. Given that IgE and sebaceous glands are both defining features of mammalian species and cardinal to mammalian anti-acarian defense, it appears acarians very significantly influenced mammalian evolution. If that is the case, then other mammalian adaptations also arose in response to evolutionary pressure exerted by acarians.
Mammary glands may predate the origin of class Mammalia, but their modern-day expression is limited to extant mammalian lineages (50). Although the evolutionary pressure responsible for emergence of mammary glands is poorly understood, a leading theory holds that the glands evolved as a neomorphic mosaic, combining the properties of both sebaceous and apocrine glands (51). If a primary function of sebaceous glands is support of epidermal colonization by Malassezia, then mammary glands must somehow promote the growth and vertical transmission of the fungus. It should come as no surprise that Malassezia represents the dominant fungus present in the breastmilk of healthy mothers (52). Indeed, transmission of Malassezia between human mothers and their progeny has already been demonstrated: 89% of infants have detectable levels of dermal Malassezia on day 0, with 100% having detectable levels by day 1 (53). By day 30, malassezial diversity conforms to that of adults, with fungal genotypes being those of mothers (53). As will next be argued, not only does mutualism between humans and Malassezia provide rationale for mammary glands, but it also provides insight into human dermatopathologies attributed to Malassezia.
The Acari Hypothesis clarifies the role of some human adaptations in the anti-acarian defense of humans. According to The Hypothesis, both endogenous molecules, e.g., dermcidin and apolipoprotein D, and skin microbiota, e.g., Malassezia, protect humans from the vectorial threat posed by acarians (5). Although The Hypothesis was developed primarily to help ‘decipher’ allergy, it also addresses other matters pertinent to human pathophysiology, especially ones relating certain dermatopathologies to dyslipidemia (54, 55).
Multiple dermatopathologies are associated with dyslipidemia, including, most notably, SD, a chronic recurring skin condition characterized by greasy erythematous plaques and yellow-gray scale (56). Because anti-fungal therapy resolves the symptoms of SD, Malassezia is believed central to the pathophysiology of the disorder (57, 58). Relatedly, Malassezia influences sebum content via metabolism of triglycerides and liberation of free fatty acids, especially oleic acid (59). For reasons yet unknown, skin of persons with SD reacts strongly to oleic acid whilst skin of healthy individuals does not (60).
Although Malassezia is believed critical to the etiology of SD, the skin of persons with the disorder is also characterized by a striking bacterial dysbiosis, with S. aureus being the most abundant microorganism (61). S. aureus is a facultative, anaerobic, gram-positive bacterium that has historically been considered a constituent of the normal flora of human skin and nasal passages (62, 63). In addition to being an opportunistic pathogen, S. aureus contributes to the pathophysiology of IgE-mediated diseases, including AD, allergic rhinitis, and asthma (64–66). Like Malassezia, S. aureus is unusual in that persons with allergic conditions often produce IgE against proteins expressed by the organism. One study found that 27% of dust mite-sensitized patients who suffer from both asthma and allergic rhinitis express IgE against toxic shock syndrome toxin-1 (TSST-1) (66), a protein secreted by S. aureus. Another study found that 38% of patients with moderate AD express IgE against TSST-1 (67). As follows from The Hypothesis, the existence of IgE against TSST-1 confirms interaction between S. aureus and acarians and suggests TSST-1 has anti-acarian activity.
Numerous studies have shown oleic acid significantly impacts S. aureus physiology by decreasing bacterial adhesion (68), disrupting cell membranes (69) and/or limiting expression of bacterial virulence genes (70). Inasmuch as S. aureus is the most abundant organism on the skin of individuals with SD, it is entirely possible oleic acid reactivity is not a direct response to the lipid, rather it is a secondary response to materials expressed by S. aureus.
Importantly, molecular constituents of eccrine gland secretions have antimicrobial activity against S. aureus (29, 71). Just as hygienic removal of eccrine gland secretions fosters acarian infestation, it also fosters S. aureus colonization. Consequently, just as acarian dysbiosis can cause human disease, i.e., allergy, so, too, can bacterial dysbiosis cause human disease, e.g., SD. If the relationship between Malassezia and humans is mutualistic, then Malassezia must be native to the human epidermal ecosystem/microbiome. It follows that S. aureus should not be considered normal skin flora. Instead, the bacterium should be considered a strict pathogen; one that is invasive to the epidermal ecosystem and only present on contemporary humans because of modern hygiene.
Unlike Malassezia, S. aureus does not depend on exogenous fatty acids for its survival (72). Still, because de novo synthesis of fatty acids for bacterial membrane inclusion requires substantial energy expenditure, S. aureus scavenges fatty acids of its host (73, 74). Consistent with this operation, S. aureus expresses lipases that liberate fatty acids from triglycerides, the major component of sebaceous gland secretions (75). The importance of sebaceous lipids to S. aureus is further supported by the microanatomic distribution of the bacterium: its colonies preferentially cluster around pilosebaceous units (76, 77). Given both the lipid dependence of Malassezia and the co-localization of Malassezia and S. aureus, the two organisms undoubtedly compete for host lipids. The response of Malassezia toward S. aureus may be one of self-preservation. Alternatively, the anti-staphylococcal activity of Malassezia may benefit the host directly, a consequence of unappreciated evolutionary design.
Evidence indicates epidermal competition between Malassezia and S. aureus has systemic consequences. As one instructive example, severe SD is associated with development and progression of the dyslipidemia characteristic of the metabolic syndrome (MetS) (78, 79). If Malassezia contributes to the antimicrobial defense of humans, and sebum enables epidermal colonization by Malassezia, then the fungus influences lipid homeostasis. Further, because systemic lipids are undoubtedly trafficked to sebaceous glands during the epidermal inflammatory response, epidermal co-localization of pathogens that influence the well-being of either Malassezia or its human host may beget dyslipidemia.
The Acari Hypothesis is a construct with which to address unknowns relevant to IgE-mediated disease. As with any disease-related hypothesis, its utility depends upon its ability to facilitate mechanistic understanding. In this regard, not only does The Hypothesis provide rationale satisfying to allergy and its related issues, but it also helps to answer questions relevant both to human evolution and to pathophysiologies of other diseases. As one very salient example of the latter, MetS refers to co-occurrences of insulin resistance, obesity, dyslipidemia and hypertension (80). Persons who have MetS are at elevated risk of cardiovascular disease (81). As elaborated above, dysbiosis that results in S. aureus colonization and subsequent competition with Malassezia may yield the dyslipidemia of MetS. Indeed, in some animal models, S. aureus infection induces both insulin resistance and adipogenesis (82–84). Thus, S. aureus may be the causative agent of MetS. If that is so, then hygienic practices may drive heart disease in developed countries (ACR, submitted).
The original contributions presented in the study are included in the article/Supplementary Material, further inquiries can be directed to the corresponding author.
AR: Conceptualization, Investigation, Writing – original draft, Writing – review & editing. GR: Conceptualization, Funding acquisition, Resources, Writing – review & editing.
The author(s) declare financial support was received for the research, authorship, and/or publication of this article. This research was supported in part by funding to GR from the Department of Pathology, Feinberg School of Medicine, Northwestern University.
The authors declare that the research was conducted in the absence of any commercial or financial relationships that could be construed as a potential conflict of interest.
All claims expressed in this article are solely those of the authors and do not necessarily represent those of their affiliated organizations, or those of the publisher, the editors and the reviewers. Any product that may be evaluated in this article, or claim that may be made by its manufacturer, is not guaranteed or endorsed by the publisher.
1. Retzinger AC, Retzinger GS. The acari hypothesis, IV: revisiting the role of hygiene in allergy. Front Allergy. 2024;5:1415124. doi: 10.3389/falgy.2024.1415124
2. Retzinger AC, Retzinger GS. Mites, ticks, anaphylaxis and allergy: the Acari hypothesis. Med Hypotheses. 2020;144:110257. doi: 10.1016/j.mehy.2020.110257
3. Retzinger AC, Retzinger GS. The acari hypothesis, II: interspecies operability of pattern recognition receptors. Pathogens. 2021;10(9):1220. doi: 10.3390/pathogens10091220
4. Retzinger AC, Retzinger GS. The acari hypothesis, III: atopic dermatitis. Pathogens. 2022;11(10):1083. doi: 10.3390/pathogens11101083
5. Retzinger AC, Retzinger GS. The Acari Hypothesis, IV: revisiting the role of hygiene in allergy. Front Allergy. (2024) 5:1415124. doi: 10.3389/falgy.2024.1415124
6. Pakkasela J, Ilmarinen P, Honkamäki J, Tuomisto LE, Andersén H, Piirilä P, Hisinger-Mölkänen H, Sovijärvi A, Backman H, Lundbäck B, Rönmark E, Kankaanranta H, Lehtimäki L. Age-specific incidence of allergic and non-allergic asthma. BMC Pulm Med. 2020;20(1):9. doi: 10.1186/s12890-019-1040-2
7. Bylund S, Kobyletzki LB, Svalstedt M, Svensson Å. Prevalence and incidence of atopic dermatitis: a systematic review. Acta Derm Venereol. 2020;100(12):adv00160. doi: 10.2340/00015555-3510
8. Willits EK, Park MA, Hartz MF, Schleck CD, Weaver AL, Joshi AY. Food allergy: a comprehensive population-based cohort study. Mayo Clin Proc. 2018;93(10):1423–30. doi: 10.1016/j.mayocp.2018.05.031
9. Pochi PE, Strauss JS, Downing DT. Age-related changes in sebaceous gland activity. J Invest Dermatol. 1979;73(1):108–11. doi: 10.1111/1523-1747.ep12532792
10. Cotterill JA, Cunliffe WJ, Williamson B, Bulusu L. Age and sex variation in skin surface lipid composition and sebum excretion rate. Br J Dermatol. 1972;87(4):333–40. doi: 10.1111/j.1365-2133.1972.tb07419.x
11. Picardo M, Ottaviani M, Camera E, Mastrofrancesco A. Sebaceous gland lipids. Dermatoendocrinol. 2009;1(2):68–71. doi: 10.4161/derm.1.2.8472
12. Vijaya Chandra SH, Srinivas R, Dawson TL Jr, Common JE. Cutaneous malassezia: commensal, pathogen, or protector?. Front Cell Infect Microbiol. 2021;10:614446. doi: 10.3389/fcimb.2020.614446
13. Jo JH, Deming C, Kennedy EA, Conlan S, Polley EC, Ng WI. Diverse human skin fungal communities in children converge in adulthood. J Invest Dermatol. 2016;136(12):2356–63. doi: 10.1016/j.jid.2016.05.130
14. Sugita T, Suzuki M, Goto S, Nishikawa A, Hiruma M, Yamazaki T, Makimura K. Quantitative analysis of the cutaneous Malassezia microbiota in 770 healthy Japanese by age and gender using a real-time PCR assay. Med Mycol. 2009;48(2):229–33. doi: 10.1080/13693780902977976
15. Kim GK. Seborrheic dermatitis and Malassezia species: how are they related?. J Clin Aesthet Dermatol. 2009;2(11):14–7.20725575
16. Piérard GE, Ausma J, Henry F, Vroome V, Wouters L, Borgers M, Cauwenbergh G, Piérard-Franchimont C. A pilot study on seborrheic dermatitis using pramiconazole as a potent oral anti-Malassezia agent. Dermatology. 2007;214(2):162–9. doi: 10.1159/000098577
17. Tao R, Li R, Wang R. Skin microbiome alterations in seborrheic dermatitis and dandruff: a systematic review. Exp Dermatol. 2021;30(10):1546–53. doi: 10.1111/exd.14450
18. Glatz M, Bosshard PP, Hoetzenecker W, Schmid-Grendelmeier P. The role of Malassezia spp. In atopic dermatitis. J Clin Med. 2015;4(6):1217–28. doi: 10.3390/jcm4061217
19. Nowicka D, Nawrot U. Contribution of Malassezia spp. To the development of atopic dermatitis. Mycoses. 2019;62(7):588–96. doi: 10.1111/myc.12913
20. Mayser P, Gross A. Ige antibodies to Malassezia furfur, M. sympodialis and pityrosporum orbiculare in patients with atopic dermatitis, seborrheic eczema or pityriasis versicolor, and identification of respective allergens. Acta Derm Venereol. 2000;80(5):357–61. doi: 10.1080/000155500459303
21. Zhang E, Tanaka T, Tajima M, Tsuboi R, Kato H, Nishikawa A, Sugita T. Anti-Malassezia-Specific IgE antibodies production in Japanese patients with head and neck atopic dermatitis: relationship between the level of specific IgE antibody and the colonization frequency of cutaneous Malassezia species and clinical severity. J Allergy. 2011;2011:645670. doi: 10.1155/2011/645670
22. Grabka R, d'Entremont TW, Adams SJ, Walker AK, Tanney JB, Abbasi PA, Ali S. Fungal endophytes and their role in agricultural plant protection against pests and pathogens. Plants. 2022;11(3):384. doi: 10.3390/plants11030384
23. Gerson U, Gafni A, Paz Z, Sztejnberg A. A tale of three acaropathogenic fungi in Israel: Hirsutella, Meira and acaromyces. Exp Appl Acarol. 2008;46(1-4):183–94. doi: 10.1007/s10493-008-9202-6
24. Boekhout T, Theelen B, Houbraken J, Robert V, Scorzetti G, Gafni A, Gerson U, Sztejnberg A. Novel anamorphic mite-associated fungi belonging to the Ustilaginomycetes: Meira geulakonigii gen. Int J Syst Evol Microbiol. 2003;53(Pt 5):1655–64. doi: 10.1099/ijs.0.02434-0
25. Paz Z., Gerson U., Sztejnberg A. Assaying three new fungi against citrus mites in the laboratory, and a field trial. BioControl 52, 855–62 (2007). doi: 10.1007/s10526-006-9060-2
26. Paz Z, Burdman S, Gerson U, Sztejnberg A. Antagonistic effects of the endophytic fungus Meira geulakonigii on the citrus rust mite Phyllocoptruta oleivora. J Appl Microbiol. 2007;103(6):2570–9. doi: 10.1111/j.1365-2672.2007.03512.x
27. Paz Z., Bilkis I., Gerson U., Kerem Z., Sztejnberg A. Argovin, a novel natural product secreted by the fungus Meira argovae, is antagonistic to mites. Entomol Exp Appl. 2011, 140, 247–53. doi: 10.1111/j.1570-7458.2011.01155.x
28. Morris DO. Malassezia pachydermatis carriage in dog owners. Emerg Infect Dis. 2005;11(1):83–8. doi: 10.3201/eid1101.040882
29. Steffen H, Rieg S, Wiedemann I, Kalbacher H, Deeg M, Sahl HG, Peschel A, Götz F, Garbe C, Schittek B. Naturally processed dermcidin-derived peptides do not permeabilize bacterial membranes and kill microorganisms irrespective of their charge. Antimicrob Agents Chemother. 2006;50(8):2608–20. doi: 10.1128/AAC.00181-06
30. Gaitanis G, Magiatis P, Hantschke M, Bassukas ID, Velegraki A. The Malassezia genus in skin and systemic diseases. Clin Microbiol Rev. 2012;25(1):106–41. doi: 10.1128/CMR.00021-11
31. Wu G, Zhao H, Li C, Rajapakse MP, Wong WC, Xu J, Saunders CW, Reeder NL, Reilman RA, Scheynius A, Sun S, Billmyre BR, Li W, Averette AF, Mieczkowski P, Heitman J, Theelen B, Schröder MS, De Sessions PF, Butler G, Maurer-Stroh S, Boekhout T, Nagarajan N, Dawson TL. Genus-Wide comparative genomics of malassezia delineates its phylogeny, physiology, and niche adaptation on human skin. PLoS Genet. 2015;11(11):e1005614. doi: 10.1371/journal.pgen.1005614
32. Cho YJ, Kim T, Croll D, Park M, Kim D, Keum HL, Sul WJ, Jung WH. Genome of Malassezia arunalokei and its distribution on facial skin. Microbiol Spectr. 2022;10(3):e0050622. doi: 10.1128/spectrum.00506-22
33. Triana S, de Cock H, Ohm RA, Danies G, Wösten HAB, Restrepo S, González Barrios AF, Celis A. Lipid metabolic versatility in Malassezia spp. Yeasts studied through metabolic modeling. Front Microbiol. 2017;8:1772. doi: 10.3389/fmicb.2017.01772
34. Xu J, Saunders CW, Hu P, Grant RA, Boekhout T, Kuramae EE, Kronstad JW, Deangelis YM, Reeder NL, Johnstone KR, Leland M, Fieno AM, Begley WM, Sun Y, Lacey MP, Chaudhary T, Keough T, Chu L, Sears R, Yuan B, Dawson TL. Dandruff-associated Malassezia genomes reveal convergent and divergent virulence traits shared with plant and human fungal pathogens. Proc Natl Acad Sci. 2007;104(47):18730–5. doi: 10.1073/pnas.0706756104
35. Cao K, Liu Y, Liang N, Shen X, Li R, Yin H, Xiang L. Fatty acid profiling in facial sebum and erythrocytes from adult patients with moderate acne. Front Physiol. 2022;13:921866. doi: 10.3389/fphys.2022.921866
36. Wang H, Nuttall PA. Excretion of host immunoglobulin in tick saliva and detection of IgG-binding proteins in tick haemolymph and salivary glands. Parasitology. 1994;109 (Pt 4):525–30. doi: 10.1017/s0031182000080781
37. Beck HI, Korsgaard J. Atopic dermatitis and house dust mites. Br J Dermatol. 1989;120(2):245–51. doi: 10.1111/j.1365-2133.1989.tb07789.x
38. Teplitsky V, Mumcuoglu KY, Babai I, Dalal I, Cohen R, Tanay A. House dust mites on skin, clothes, and bedding of atopic dermatitis patients. Int J Dermatol. 2008;47(8):790–5. doi: 10.1111/j.1365-4632.2008.03657.x
39. Naegele A, Reboux G, Scherer E, Roussel S, Millon L. Fungal food choices of Dermatophagoides farinae affect indoor fungi selection and dispersal. Int J Environ Health Res. 2013;23(2):91–5. doi: 10.1080/09603123.2012.699029
40. Blanco C, Quiralte J, Castillo R, Delgado J, Arteaga C, Barber C, et al. Anaphylaxis after ingestion of wheat flour contaminated with mites. J Allergy Clin Immunol. 1997;99(3):308–13. doi: 10.1016/s0091-6749(97)70047-2
41. Yi FC, Chen JY, Chee KK, Chua KY, Lee BW. Dust mite infestation of flour samples. Allergy. 2009;64(12):1788–9. doi: 10.1111/j.1398-9995.2009.02116.x
42. Yusof AM. Cockroaches as potential mechanical vectors for mites infestation the first report in kuantan. Int J Zoo Res. 2018, 14, 71–6 doi: 10.3923/ijzr.2018.71.76
44. Lopes-Marques M, Machado AM, Alves LQ, Fonseca MM, Barbosa S, Sinding MS, Rasmussen MH, Iversen MR, Frost Bertelsen M, Campos PF, da Fonseca R, Ruivo R, Castro LFC. Complete inactivation of sebum-producing genes parallels the loss of sebaceous glands in cetacea. Mol Biol Evol. 2019;36(6):1270–80. doi: 10.1093/molbev/msz068
45. Huelsmann M, Hecker N, Springer MS, Gatesy J, Sharma V, Hiller M. Genes lost during the transition from land to water in cetaceans highlight genomic changes associated with aquatic adaptations. Sci Adv. 2019;5(9):eaaw6671. doi: 10.1126/sciadv.aaw6671
46. Springer MS, Gatesy J. Evolution of the MC5R gene in placental mammals with evidence for its inactivation in multiple lineages that lack sebaceous glands. Mol Phylogenet Evol. 2018;120:364–74. doi: 10.1016/j.ympev.2017.12.01040
47. Zia M., Mirhendi H., Toghyani M. Detection and identification of Malassezia species in domestic animals and aquatic birds by PCR-RFLP. Iran J Vet Res. 2015, 16(1), 36–41.27175148
48. Coutinho SD, Fedullo JD, Corrêa SH. Isolation of Malassezia spp. From cerumen of wild felids. Med Mycol. 2006;44(4):383–7. doi: 10.1080/13693780500411006
49. Hađina S, Bruvo Mađarić B, Kazazić S, Paradžik T, Reljić S, Pinter L, Huber Đ, Vujaklija D. Malassezia pachydermatis from brown bear: a comprehensive analysis reveals novel genotypes and distribution of all detected variants in domestic and wild animals. Front Microbiol. 2023;14:1151107. doi: 10.3389/fmicb.2023.1151107
50. Oftedal OT. The evolution of milk secretion and its ancient origins. Animal. 2012;6(3):355–68. doi: 10.1017/S1751731111001935
51. Blackburn D.G. Evolutionary origins of the mammary gland. Mammal Rev. 1991, 21, 81–96. doi: 10.1111/j.1365-2907.1991.tb00290.x
52. Boix-Amorós A, Martinez-Costa C, Querol A, Collado MC, Mira A. Multiple approaches detect the presence of fungi in human breastmilk samples from healthy mothers. Sci Rep. 2018;8(1):16829. doi: 10.1038/s41598-018-35165-1 Sci Rep. 2017;7(1):13016. Published 2017 October 12. doi: 10.1038/s41598-017-13270-x
53. Nagata R, Nagano H, Ogishima D, Nakamura Y, Hiruma M, Sugita T. Transmission of the major skin microbiota, Malassezia, from mother to neonate. Pediatr Int. 2012;54(3):350–5. doi: 10.1111/j.1442-200X.2012.03563.x
54. Shenoy C, Shenoy MM, Rao GK. Dyslipidemia in dermatological disorders. N Am J Med Sci. 2015;7(10):421–8. doi: 10.4103/1947-2714.168657
55. Nowowiejska J, Baran A, Flisiak I. Lipid alterations and metabolism disturbances in selected inflammatory skin diseases. Int J Mol Sci. 2023;24(8):7053. doi: 10.3390/ijms24087053
56. Borda LJ, Wikramanayake TC. Seborrheic dermatitis and dandruff: a comprehensive review. J Clin Investig Dermatol. (2015) 3(2):10. doi: 10.13188/2373-1044.1000019
57. Skinner RB Jr, Noah PW, Taylor RM, Zanolli MD, West S, Guin JD, Rosenberg EW. Double-blind treatment of seborrheic dermatitis with 2% ketoconazole cream. J Am Acad Dermatol. 1985;12(5 Pt 1):852–6. doi: 10.1016/s0190-9622(85)70107-7
58. Ford GP, Farr PM, Ive FA, Shuster S. The response of seborrhoeic dermatitis to ketoconazole. Br J Dermatol. 1984;111(5):603–7. doi: 10.1111/j.1365-2133.1984.tb06631.x
59. Ro BI, Dawson TL. The role of sebaceous gland activity and scalp microfloral metabolism in the etiology of seborrheic dermatitis and dandruff. J Investig Dermatol Symp Proc. 2005;10(3):194–7. doi: 10.1111/j.1087-0024.2005.10104.x
60. DeAngelis YM, Gemmer CM, Kaczvinsky JR, Kenneally DC, Schwartz JR, Dawson TL Jr. Three etiologic facets of dandruff and seborrheic dermatitis: Malassezia fungi, sebaceous lipids, and individual sensitivity. J Investig Dermatol Symp Proc. 2005;10(3):295–7. doi: 10.1111/j.1087-0024.2005.10119.x
61. Tamer F, Yuksel ME, Sarifakioglu E, Karabag Y. Staphylococcus aureus is the most common bacterial agent of the skin flora of patients with seborrheic dermatitis. Dermatol Pract Concept. 2018;8(2):80–4. doi: 10.5826/dpc.0802a04
62. Laux C, Peschel A, Krismer B. Staphylococcus aureus colonization of the human nose and interaction with other microbiome members. Microbiol Spectr. (2019) 7(2). doi: 10.1128/microbiolspec.GPP3-0029-2018
63. Reinoso EB, Ibañez F, Raspanti C, Bogni LO, Bogni CI. Characterization of Staphylococcus aureus strains isolated from humans in Argentina. J Basic Microbiol. 2006;46(4):286–93. doi: 10.1002/jobm.200510100
64. Kobayashi T, Glatz M, Horiuchi K, Kawasaki H, Akiyama H, Kaplan DH, Kong HH, Amagai M, Nagao K. Dysbiosis and staphylococcus aureus colonization drives inflammation in atopic dermatitis. Immunity. 2015;42:756–66. doi: 10.1016/j.immuni.2015.03.014
65. Jorde I, Schreiber J, Stegemann-Koniszewski S. The role of Staphylococcus aureus and its toxins in the pathogenesis of allergic asthma. Int J Mol Sci. 2023;24(1):654. doi: 10.3390/ijms24010654
66. Liu JN, Shin YS, Yoo HS, Nam YH, Jin HJ, Ye YM, Nahm DH, Park HS. The prevalence of serum specific IgE to superantigens in asthma and allergic rhinitis patients. Allergy Asthma Immunol Res. 2014;6(3):263–6. doi: 10.4168/aair.2014.6.3.263
67. Ong PY, Patel M, Ferdman RM, Dunaway T, Church JA. Association of staphylococcal superantigen-specific immunoglobulin e with mild and moderate atopic dermatitis. J Pediatr. 2008;153(6):803–6. doi: 10.1016/j.jpeds.2008.05.047
68. Stenz L, François P, Fischer A, Huyghe A, Tangomo M, Hernandez D, Cassat J, Linder P, Schrenzel J. Impact of oleic acid (cis-9-octadecenoic acid) on bacterial viability and biofilm production in staphylococcus aureus. FEMS Microbiol Lett. 2008;287(2):149–55. doi: 10.1111/j.1574-6968.2008.01316.x
69. Parsons JB, Yao J, Frank MW, Jackson P, Rock CO. Membrane disruption by antimicrobial fatty acids releases low-molecular-weight proteins from staphylococcus aureus. J Bacteriol. 2012;194(19):5294–304. doi: 10.1128/JB.00743-12
70. Lee JH, Kim YG, Lee J. Inhibition of staphylococcus aureus biofilm formation and virulence factor production by petroselinic acid and other unsaturated C18 fatty acids. Microbiol Spectr. 2022;10(3):e0133022. doi: 10.1128/spectrum.01330-22
71. Partridge SR, Baker MS, Walker MJ, Wilson MR. Clusterin, a putative complement regulator, binds to the cell surface of staphylococcus aureus clinical isolates. Infect Immun. 1996;64(10):4324–9. doi: 10.1128/iai.64.10.4324-4329.1996
72. Chen X, Teoh WP, Stock MR, Resko ZJ, Alonzo F 3rd. Branched chain fatty acid synthesis drives tissue-specific innate immune response and infection dynamics of staphylococcus aureus. PLoS Pathog. 2021;17(9):e1009930. doi: 10.1371/journal.ppat.1009930
73. Yao J, Rock CO. Exogenous fatty acid metabolism in bacteria. Biochimie. 2017;141:30–9. doi: 10.1016/j.biochi.2017.06.015
74. Sen S, Sirobhushanam S, Johnson SR, Song Y, Tefft R, Gatto C, Wilkinson BJ. Growth-environment dependent modulation of staphylococcus aureus branched-chain to straight-chain fatty acid ratio and incorporation of unsaturated fatty acids. PLoS One. 2016;11(10):e0165300. doi: 10.1371/journal.pone.0165300
75. Tanaka M, Kamitani S, Kitadokoro K. Staphylococcus aureus lipase: purification, kinetic characterization, crystallization and crystallographic study. Acta Crystallogr F Struct Biol Commun. 2018;74(Pt 9):567–70. doi: 10.1107/S2053230X18010506
76. Ten Broeke-Smits NJ, Kummer JA, Bleys RL, Fluit AC, Boel CH. Hair follicles as a niche of staphylococcus aureus in the nose; is a more effective decolonisation strategy needed?. J Hosp Infect. 2010;76(3):211–4. doi: 10.1016/j.jhin.2010.07.011
77. Lone AG, Atci E, Renslow R, Beyenal H, Noh S, Fransson B, Abu-Lail N, Park JJ, Gang DR, Call DR. Colonization of epidermal tissue by staphylococcus aureus produces localized hypoxia and stimulates secretion of antioxidant and caspase-14 proteins. Infect Immun. 2015;83(8):3026–34. doi: 10.1128/IAI.00175-15
78. Abdulrahman B.B., Elethawi A.M.D., Abdullah H.M.; assessment of lipid profile among patients with seborrheic dermatitis. Bali Med J. 2020, 9(3), 952–8. doi: 10.15562/bmj.v9i3.1959
79. Patil S., Thakare V., Dubey L., Menon A., Sharma H., Tetarbe T. A prospective observational study to analyze the association of lipid profile abnormality with disease severity in seborrheic dermatitis patients. Asian J Pharm Clin Res. 2022, 15, 100–2. doi: 10.22159/ajpcr.2022.v15i7.44673
80. Fahed G, Aoun L, Bou Zerdan M, Allam S, Bou Zerdan M, Bouferraa Y, Assi HI. Metabolic syndrome: updates on pathophysiology and management in 2021. Int J Mol Sci. 2022;23(2):786. doi: 10.3390/ijms23020786
81. Tune JD, Goodwill AG, Sassoon DJ, Mather KJ. Cardiovascular consequences of metabolic syndrome. Transl Res. 2017;183:57–70. doi: 10.1016/j.trsl.2017.01.001
82. Liu Y, Liu FJ, Guan ZC, Dong FT, Cheng JH, Gao YP, Li D, Yan J, Liu CH, Han DP, Ma CM, Feng JN, Shen BF, Yang G. The extracellular domain of Staphylococcus aureus LtaS binds insulin and induces insulin resistance during infection. Nat Microbiol. 2018;3(5):622–31. doi: 10.1038/s41564-018-0146-2
83. Vu BG, Stach CS, Kulhankova K, Salgado-Pabón W, Klingelhutz AJ, Schlievert PM. Chronic superantigen exposure induces systemic inflammation, elevated bloodstream endotoxin, and abnormal glucose tolerance in rabbits: possible role in diabetes. mBio. 2015;6(2):e02554. doi: 10.1128/mBio.02554-14
Keywords: The Acari Hypothesis, allergy, sebaceous glands, sebum, Malassezia, Staphylococcus aureus, dyslipidemia, metabolic syndrome
Citation: Retzinger AC and Retzinger GS (2024) The Acari Hypothesis, VI: human sebum and the cutaneous microbiome in allergy and in lipid homeostasis. Front. Allergy 5:1478279. doi: 10.3389/falgy.2024.1478279
Received: 9 August 2024; Accepted: 4 November 2024;
Published: 21 November 2024.
Edited by:
Maurizio Mennini, Sapienza University of Rome, ItalyReviewed by:
Shahid Karim, University of Southern Mississippi, United StatesCopyright: © 2024 Retzinger and Retzinger. This is an open-access article distributed under the terms of the Creative Commons Attribution License (CC BY). The use, distribution or reproduction in other forums is permitted, provided the original author(s) and the copyright owner(s) are credited and that the original publication in this journal is cited, in accordance with accepted academic practice. No use, distribution or reproduction is permitted which does not comply with these terms.
*Correspondence: Andrew C. Retzinger, YW5kcmV3LnJldHppbmdlckBnbWFpbC5jb20=
Disclaimer: All claims expressed in this article are solely those of the authors and do not necessarily represent those of their affiliated organizations, or those of the publisher, the editors and the reviewers. Any product that may be evaluated in this article or claim that may be made by its manufacturer is not guaranteed or endorsed by the publisher.
Research integrity at Frontiers
Learn more about the work of our research integrity team to safeguard the quality of each article we publish.