- Aquatic Ecology Group, Department of Zoology, University of Cambridge Conservation Research Institute, University of Cambridge, Cambridge, United Kingdom
We face a food crisis. Suboptimal diet is the biggest cause of death worldwide, food production the biggest greenhouse gas emitting sector, and by 2050 an extra 2.5 billion people need affordable nutrition. Current farming systems will fail to tackle this crisis, and there is an urgent need to diversify global food production and find effective solutions in currently underexploited food sectors. Shipworms, or shell-less Teredo clams, could prove a highly valuable component of such solutions. Historically viewed as a marine pest, they have unique physiological characteristics which make them an ideal food source, including exceptionally fast growth rates, the ability to feed on waste wood or sustainable microalgae, and a high protein and omega 3 content. Today only a select few traditional cultures in the Philippines consume shipworms, but there is considerable opportunity to develop mechanisms to farm shipworms and provide a sustainable, nutrient rich, affordable food source. This will require significant challenges to be overcome, ranging from fundamental research to industry development to food processing and marketing. Leveraging new innovations in breeding, aquaculture feeds, growth systems, food processing methodologies and consumer engagement can however offer powerful solutions, and could help turn what was once a maritime villain into a nutritional savior.
Introduction
Our current food production system is failing us. Suboptimal diet is already the biggest cause of death worldwide and the global food system the single largest greenhouse-gas emitting sector. Yet by 2050 a further 2.5 billion people need access to affordable nutrition (Rockström et al., 2020). Farming of conventional crops including wheat, rice, and maize has received extensive investment over the last century, with productivity growing 2–3-fold between 1950 and 1990 (FAO, 2020a). However, yields are now beginning to stagnate, and the overreliance on this small variety of crops is a major explanation as to why 30% of humanity are now micronutrient deficient worldwide (Willett et al., 2019; FAO, 2020a). Other more nutrient rich food sources have received considerably lower research attention (Figure 1). In order to meet planetary health goals, there is an urgent need to diversify global food production and to seek productivity improvements in currently underexploited food sectors that can provide sustainable, nutrient rich, affordable food solutions (Willett et al., 2019; Rockström et al., 2020).
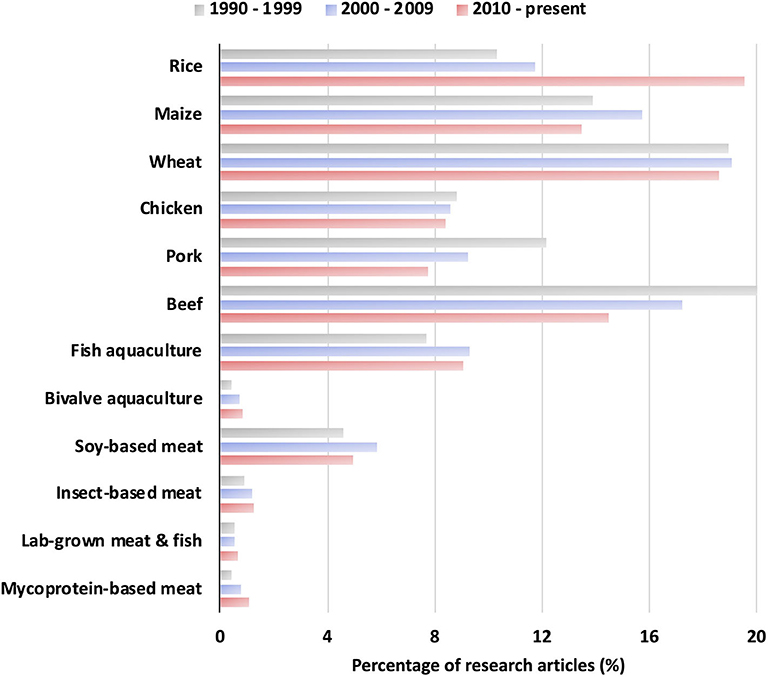
Figure 1. Percentage of all research articles published corresponding to key food types across each decade. Many food sources with a high sustainability and nutritional potential (bivalve aquaculture and below) continue to receive relatively low levels of research investment. Scopus (Elsevier, 2020) was used to count the total number of peer-reviewed publications corresponding to each food type between the dates 1990–1999, 2000–2009, and 2010–12/05/2020. Five most relevant search phrases were selected for each food type. To be counted articles had to include any one of the search phrases in the abstract, title or keywords. Search phrases were as follows. Rice: “rice farm,” “rice production,” “rice industry,” “rice food,” “rice field.” Maize: “maize farm,” “maize production,” “maize industry,” “maize food,” “maize field.” Wheat: “wheat farm,” “wheat production,” “wheat industry,” “wheat food,” “wheat field.” Chicken: “poultry farm,” “poultry production,” “chicken farm,” “chicken production,” “broiler farm.” Pork: “pork farm,” “pig farm,” “pork production,” “pig production,” “swine farm.” Beef: “beef farm,” “cattle farm,” “cow farm,” “cattle production,” “beef production.” Fish aquaculture: “fish farm,” “fish aquaculture,” “pisciculture,” “fish mariculture,” “fish hatchery.” Bivalve aquaculture: “bivalve aquaculture,” “bivalve farm,” “clam farm,” “mussel farm,” “oyster farm.” Soy-based meat: “tofu,” “tempeh,” “soy-based meat,” “soy protein,” “miso.” Insect-based meat: “insect meat,” “insect flour,” “insect food product,” “insect snack,” “insect burger.” Lab-grown meat and fish: “lab-grown meat,” “cultured artificial meat,” “lab-grown fish,” “in vitro meat,” “cell-based meat.” Mycoprotein-based meat: “mycoprotein,” “quorn,” “fusarium venenatum,” “fungiculture protein,” “fungal protein food.”
There are major environmental and human health wins that could be made from research and industry investment in the farming of underexploited aquaculture species. Aquaculture is already the world's fastest growing food sector, growing 8.2% pa between 1970 and 2010 (FAO, 2020a,b). Growth has however been focussed on species such as salmon and shrimp, which are expensive to consumers and unsustainable to farm due to a reliance upon foods comprising predominantly of fish meal and oil from wild-caught fish (Willer and Aldridge, 2019a). The farming of bivalve shellfish (clams, mussels, oysters) is a highly attractive alternative. Bivalves have a higher protein content than beef, are a rich source of essential fatty acids and micronutrients, have a lower environmental footprint than all other meats and many plant crops, and are cheap to produce (Willer and Aldridge, 2019a). There is outstanding potential for growth of bivalve aquaculture; developing just 1% of the suitable coastline worldwide could fulfill the protein requirements of over one billion people (Gentry et al., 2017; United States Department of Agriculture Agricultural Research Service, 2020). Yet the bivalve industry remains small scale and artisanal. There are bivalve species with high farming potential such as shipworms which could play a key role in sustainable aquaculture, where further research and industry attention is required in order to realize food production potential (Waite et al., 2014).
Shipworms, or Teredo clams, are a saltwater bivalve species with unique life-history characteristics that could be leveraged to provide significant economic, sustainability, and nutritional benefits to human society. Shipworms grow exceptionally fast relative to other aquaculture species, reaching 30 cm (or ~250 g wet weight) within 6 months, compared to shelled bivalves which typically take 2–3 years to reach a harvestable size of around 7 cm (Helm and Bourne, 2004; Paalvast and van der Velde, 2011a), presenting a highly productive and efficient possibility for producing animal protein. Shipworms lack a shell for protection, instead burrowing into and feeding on submerged wood. This wood digesting ability has historically made shipworms a pest in the maritime industry, where they have damaged traditional wooden hulls and marine piling; an issue now reduced with modern engineering materials (Paalvast and van der Velde, 2011a). Whilst Teredo clams can survive on wood alone, obtaining additional nitrogen via symbiotic nitrogen fixing bacteria in their gills, they can also filter feed on marine detritus like other bivalves (Paalvast and van der Velde, 2013), thus presenting numerous options for low-environmental impact feeding in aquaculture production. Shipworms are rich in protein and essential omega-3 fatty acids, and like other bivalves can provide a highly valuable source of quality nutrition to humans (Paalvast and van der Velde, 2013; Sinyo et al., 2019; United States Department of Agriculture Agricultural Research Service, 2020).
Today shipworm harvest and consumption as a food is limited to a few select regions of southeast Asia, notably the Philippines and Thailand. Shipworms grow on decaying wood in the mangroves and are harvested by local populations. In some areas such as Bakhawan Eco Park (New Buswang, Panay, Philippines), the coastlines have been seeded with dead wood as part of mangrove restoration projects which in turn has increased the supply of shipworms for harvest, although there remain no formalized farming approaches (Aklan Government, 2020). Shipworms are sold raw in wet markets, and are particularly popular on Palawan island, Philippines where they are known as tamilok and in Trat Province, Thailand where they are known as priyang talay (Slow Food Foundation, 2020). Filipinos typically eat Tamilok raw and dipped in salt, chili and vinegar in a dish known as kinilaw, whilst the Thais eat priyang talay in curries or braised with fish paste and bananas in a stew (Sinyo et al., 2019; Aklan Government, 2020; Slow Food Foundation, 2020)). Over the last decade increasing popularity of shipworms as a food for tourists and increasing ecological threats to mangrove swamps has put pressure on shipworm supply, and socioeconomic benefits could be yielded from increased mangrove protection or improved shipworm culture practices (Sinyo et al., 2019; Aklan Government, 2020). Outside of southeast Asia, shipworms are still viewed as a pest. In Europe and the Americas climatic change is leading to increasing coastal salinity and temperature, making conditions more favorable to shipworms (Paalvast and van der Velde, 2011b). This could lead to the re-emergence of shipworms as a maritime nuisance, or alternatively with research and industry innovation and efforts to change consumer perception, could present a new opportunity to establish shipworm aquaculture.
Approach
Several approaches were leveraged to build a synthesis of the potential of shipworms as a food source and the key challenges and opportunities to establish aquaculture. Scopus was used (Elsevier, 2020) (Figure 1, legend) to count the percentage of all research articles published corresponding to key food types across each decade since 1990, and highlight the relatively low research investment in bivalve aquaculture. We spoke to stakeholders across the aquaculture and food industry to gain an insight on the potential obstacles in creating a new food type and methods which could be effective in providing a solution. This provided direction for a thorough literature review. We searched Scopus (Elsevier, 2020) and Google Scholar (Google, 2020) for studies on shipworms, aquaculture, and the development of unexploited food sectors. We used several key terms when covering the field: “shipworm,” “Teredo,” “bivalve,” “aquaculture,” “invasive species,” “sustainable nutrition,” “food safety,” “food processing,” “food marketing”; and included all relevant research up to and including 20th June 2020. Prior to our study, no studies had built a synthesis on the potential of shipworms for aquaculture.
Challenges and Opportunities
Production of shipworms could offer major advantages over other forms of aquaculture for food production. Farming would be significantly more sustainable than finfish aquaculture, with shipworms requiring waste wood or natural or sustainably grown algae rather than fish as a feed source, helping contribute toward circular economies in food production (Paalvast and van der Velde, 2013; Waite et al., 2014). The disadvantages of conventional bivalve aquaculture—notably the slow growth rates and energy investment in a shell which cannot be used as food—would be avoided. Shipworms would provide a valuable source of protein and essential fatty acids to replace less sustainable and more expensive meat and fish products. In order for this potential to be realized there are key challenges that must be overcome, ranging from fundamental research to industry development to food processing and marketing. Novel technologies and new application of innovations from other industries can however provide exciting opportunities for success (Figure 2).
Fundamental Research
Fundamental research is required in several key areas to allow the exploration and development of shipworm aquaculture. There are ~65 species of shipworm worldwide and we do not yet know which would be most appropriate for efficient farming and human nutrition (Horton et al., 2020). The only small-scale attempts at culture to date have involved floating logs in coastal waters or seeding mangroves with dead wood to encourage Teredo proliferation (Sinyo et al., 2019). When an appropriate species is identified, there will also be a need to develop scientific methodologies for trait selection and large-scale breeding of shipworm juveniles, which is challenging in bivalves due to high fecundity, self-fertilization, genetic load and segregation distortion (Hollenbeck and Johnston, 2018). The nutritional profile of shipworms also needs to be formally analyzed—whilst they are recognized to have a similar profile to other bivalves no quantitative data has been published in peer-reviewed literature (Paalvast and van der Velde, 2013; Sinyo et al., 2019). The most appropriate abiotic conditions for farming shipworms also need to be identified, including temperature, salinity, and saltwater mineral composition. We must also develop a greater understanding as to how climatic change may affect these values, as this may affect site selection for farms without facilities to control temperature and salinity (Filgueira et al., 2016). Crucial to successful shipworm aquaculture will be the selection and development of appropriate feeds. Research indicates that filter feeding is in fact the preferred source of dietary nutrition for shipworms, with wood drilling performed primarily to provide shelter (Paalvast and van der Velde, 2013), but we do not yet know what the most appropriate feed would be for shipworm farming. Infection control must also be considered. Disease is a major concern in aquaculture, costing the industry over US$6 billion annually, yet there is an urgent need to reduce the widespread use of antibiotics which is driving bacterial resistance and increasing threat to human health (Stentiford et al., 2017).
Knowledge and methodologies gained from research in conventional agriculture and aquaculture can help tackle challenges in shipworm aquaculture. Current scientific literature suggests Teredo navalis may be a promising shipworm candidate for aquaculture, the same species as consumed by Philippine societies at present (Sinyo et al., 2019). The development of whole genome sequences and genetic linkage maps, as has been done in other bivalve aquaculture (Hollenbeck and Johnston, 2018) could be used to select for desirable traits such as faster growth, greater broodstock quality, and an improved profile regarding nutrition and palatability. Optimum temperatures and salinities for farming are likely to be between 10–25°C and 10–30%, respectively (Paalvast and van der Velde, 2011a); further research can refine these values, make predictions as to which coastal locations might become more ideal under climate change, and make use of new innovations in artificial salt formulations (e.g., Homarsel, produced by Zoutman, Belgium). Regarding feed for shipworm aquaculture, waste wood, live algae, and artificial or microencapsulated feeds are all viable options. The use of wood may allow the recycling of waste, and the use of microencapsulated feeds (Willer and Aldridge, 2017, 2019b) would avoid major challenges faced by conventional algal feed including quality inconsistencies, contamination, and poor shelf life (Willer and Aldridge, 2019a). Research would allow identification as to whether a combination of these feeds or a single feed is most optimal for growth. There are also emerging new opportunities for more sustainable disease control, including the use of probiotics and antimicrobial peptides (Destoumieux-Garzón et al., 2016; Hoseinifar et al., 2018), and it would be advisable to quantify the efficacy of their use in shipworms.
Industry Development
Industry will play the central role in the establishment of shipworm aquaculture, and will need to overcome challenges regarding facility design, safety and economics in order to develop and expand successfully. The practical aspects of setting up shipworm farms pose an initial hurdle. The invasive nature of shipworms means that they can represent a hazard if they escape into the natural marine environment (Paalvast and van der Velde, 2011a) and farming systems and infrastructure need to be designed to nullify this risk. Overzealous farming of other bivalves such as Magallana gigas has already been shown to displace important native species and modify marine ecosystems (Herbert et al., 2016). Food safety is also a major concern when farming filter feeding bivalves, and in polluted waters there is high potential for hazardous substances such as heavy metals, microplastics and toxic cyanobacteria to accumulate in bivalves—with oysters farmed in the South China Sea for example now containing ~11 microplastic particles per individual (Elston and Ford, 2011; Eriksen et al., 2014; Hossen et al., 2014; Willer and Aldridge, 2020a). Ocean water quality is likely to further decline as the human population expands and shipworm farms should be designed to cope with these changes (Häder et al., 2020). Coastal areas will undergo further urbanization and development, which could both restrict and provide new opportunities as to where shipworm farms could be located. The industry will also need to develop automated mechanisms to efficiently monitor shipworms and at harvest remove them from the woody substrates they burrow in. In conventional bivalve production the shucking (de-shelling) process in manufacturing packaged bivalve foods such as tinned clams is highly labor intensive and a major contributor to food costs (Hackney and Rippen, 2000), and while shipworms lack a shell it will be important to find cost-effective processing systems. Finally and crucially, a thorough economic assessment of the entire proposed production value chain will be required in order to ensure shipworm aquaculture is scalable and financially viable. Inadequate assessment could lead to expensive bottlenecks in production (Willer and Aldridge, 2019a), or at worst could result in complete failure of shipworm aquaculture (Shang, 1985).
Lessons learned from the commercial success of other forms of aquaculture could help ensure effective development of the shipworm industry. To mitigate risk of shipworms escaping into the open sea, farming of shipworms could involve the use of enclosed saltwater tanks, either semi-submerged on the coastline or onshore. The installation of flood protection structures and nearshore or offshore wind farms as a means to mitigate climatic change and its impacts could provide a new opportunity for mounting shipworm growth tanks. Larger enclosed areas could also be constructed within mangrove restoration projects, in parallel improving water quality and coastal protection (Aklan Government, 2020). There may also be an option to setup enclosed tanks in urban areas away from the sea as is seen in finfish aquaculture (Zohar et al., 2005), which would further reduce the risk of escape due to the lack of surrounding saltwater. Enclosed tanks would enable optimization of temperature and salinity to improve growth rates, and also the use of artificial seawater or clean depurated seawater which would dramatically increase food safety (Zohar et al., 2005; Willer and Aldridge, 2020b). Given that consumer fears regarding safety are one of the biggest barriers to increased bivalve consumption, the use of enclosed tanks could be the underpinning factor in the commercial success of shipworms as a food source (Birch and Lawley, 2012). Automation during growth and at the point of harvest through the use of optical sensors, machine vision systems and low-cost robotics could help avoid excessive labor costs in shipworm production, with this approach already yielding great benefits in finfish aquaculture (Saberioon et al., 2017). Identifying the minimal requirements and optimal arrangement of woody substrates for shipworm farming would further increase operating efficiency during growth and harvest. There is also the opportunity to use circular economies to divert side-streams of shipworm production back into the system for example for feed or wood production. To ensure commercial viability of shipworm farming, bioeconomic modeling methods that have recently been tried and tested across salmon, tilapia and shrimp aquaculture can be applied. Compared to using simple cost-benefit analyses alone, the use of scenario simulations and algorithm-based approaches can ensure that farming solutions identified are more resilient to changes in external economic and environmental factors (Llorente and Luna, 2016).
Food Processing and Marketing
Research and industry development will provide the foundation for shipworm aquaculture, but unless challenges in food processing and marketing are tackled these efforts will be in vain. The first major challenge will be in turning shipworm meat into a product that is palatable for human consumption. Invertebrate-based foods such as bivalves and insects are viewed with fear and disgust by many western societies, and innovations in food manufacturing are being developed to overcome this hurdle (Dobermann et al., 2017). Molluscs also have a different muscle structure (obliquely striated, cross-striated, and smooth) to the striated muscles of fish and mammals, which may pose additional food processing challenges (Millman, 1967). To ensure widespread retail demand, it will also be essential to ensure that products developed fit a wide range of consumer tastes and cultural preferences (Apostolidis and McLeay, 2016), and this will be of equal importance in the restaurant and hospitality market alongside supermarkets. Products and dishes will also need to remain affordable to consumers. This requires measures to ensure production is efficient as well as approaches to avoid market monopolization which could drive up prices and reducing industry innovation, as has unfortunately been seen in the agricultural and mycoprotein industries (Howard, 2016). Paramount to the overall strategy will be for researchers and industry to work together to identify the most effective methods to market shipworm food products and promote consumer uptake.
There are a wide array of effective solutions that can be applied to the discussed challenges in the processing and marketing of shipworm-based foods. Innovations in food manufacturing made during the development of mycoprotein and insect-based foods can be applied to shipworm meat. These range from simple dehydration, powdering, and reconstitution, to thermoplastic extrusion and fiber spinning of meat proteins into a completely new form, which could help with the potential challenge of processing the different molluscan muscle types (Featherstone, 2015; Apostolidis and McLeay, 2016). This could enable the development of food products with a wide range of forms to fit a range of cultures, from burgers and fish fingers to meat-style pieces to use in traditional stews, and is an approach that has led to great commercial success for products including Quorn (USA), Beyond Meat (USA), and Eat Grub (UK) (Deroy et al., 2015). Working with high profile chefs and finding methods to explicitly present shipworms undisguised in a highly palatable form on a plate will also play a key role. Historically in Asia and increasingly in the West this strategy of including insects in premium dishes has been central to establishing a high level of cultural acceptance for insect-based foods (Deroy et al., 2015; Dobermann et al., 2017). Marinated shipworm kinilaw is already popular with both locals and tourists in the Philippines, and gaining further insight from Filipino culinary practices could help such dishes become a global delicacy (Sinyo et al., 2019). To ensure shipworm-based foods are easily affordable to consumers several mechanisms could be deployed. These include governmental and private investment to allow the industry to scale rapidly and achieve lower operating costs, subsidies to encourage purchase of nutritious shipworm-based food in place of less sustainable meat products (An, 2013), and enforcement of industry regulations to promote competition in shipworm production and food processing (Sodano and Verneau, 2014). Marketing will also play a pivotal role in encouraging consumer selection and purchase of bivalve based foods. Mass surveys, consumer co-creation activities, and domestic trials can help identify the most effective marketing levers to pull. A promotion strategy in which shipworms are double framed as an environmental solution to excessive meat consumption and as a protein packed superfood, an approach already being used in the seafood and insect industry (Schiemer et al., 2018), may prove one of the most effective mechanisms.
Conclusion
As the world's population grows by 200,000 people every day, we have a global responsibility to find new ways to feed everyone without further depleting the planet's already stretched natural resources. Finding viable, sustainable, affordable solutions will mean thinking outside-the-box and considering the previously inconsiderable. Shipworm aquaculture has outstanding potential to become a component of a broader global solution. Challenges need to be overcome, ranging from fundamental research and viability assessments to industry development to food processing and marketing. Yet there is an opportunity to provide our global community with a fast growing, sustainable, nutritious food source that could help remediate the catastrophic damage current food productions system are causing to environmental and human health. Policy changes will play a key role in stimulating research and industry efforts. Financial incentives that support sustainable shipworm research and aquaculture in place of less sustainable meat production, legislations that support mangrove restoration, coastal and urban aquaculture, and tax breaks on the production and retail of sustainable nutrient-dense foods are all options. Changing industry practice and consumer behavior will not be easy, but rewards are seldom reaped without new ways of thinking. Shifting our perception of shipworms as a pest and giving them a place on our plates is one change in our thinking that could yield great rewards.
Data Availability Statement
All datasets presented in this study are included in the article/supplementary material.
Author Contributions
DW led the investigation and wrote the manuscript. DA contributed to design, interpretation, and reviewed the manuscript. All authors gave final approval for publication.
Funding
DW was supported by the Biotechnology and Biological Sciences Research Council and DA was supported by a Dawson Fellowship at St Catharine's College, Cambridge.
Conflict of Interest
The authors declare that the research was conducted in the absence of any commercial or financial relationships that could be construed as a potential conflict of interest.
Acknowledgments
We thank the Biotechnology and Biological Sciences Research Council for supporting this investigation.
References
Aklan Government. (2020) Official Website of the Province of Aklan. Available online at: http://aklan.gov.ph (accessed August, 21 2020).
An, R. (2013). Effectiveness of subsidies in promoting healthy food purchases and consumption: a review of field experiments. Public Health Nutr.. 16, 1215–1228. doi: 10.1017/S1368980012004715
Apostolidis, C., and McLeay, F. (2016). It's not vegetarian, it's meat-free! Meat eaters, meat reducers and vegetarians and the case of Quorn in the UK. Soc. Bus. 6, 267–290. doi: 10.1362/204440816X14811339560938
Birch, D., and Lawley, M. (2012). Buying seafood: understanding barriers to purchase across consumption segments. Food Qual. Prefer.. 26, 12–21. doi: 10.1016/j.foodqual.2012.03.004
Deroy, O., Reade, B., and Spence, C. (2015). The insectivore's dilemma, and how to take the West out of it. Food Qual. Prefer.. 44, 44–55. doi: 10.1016/j.foodqual.2015.02.007
Destoumieux-Garzón, D., Rosa, R. D., Schmitt, P., Barreto, C., Vidal-Dupiol, J., Mitta, G., et al. (2016). Antimicrobial peptides in marine invertebrate health and disease. Philos. Trans. R. Soc. B Biol. Sci. 371:20150300. doi: 10.1098/rstb.2015.0300
Dobermann, D., Swift, J. A., and Field, L. M. (2017). Opportunities and hurdles of edible insects for food and feed. Nutr. Bull.. 42, 293–308. doi: 10.1111/nbu.12291
Elsevier (2020). Scopus. Available online at: https://www.scopus.com (accessed June 13, 2018).
Elston, R. A., and Ford, S. E. (2011). “Shellfish diseases and health management,” in Shellfish Aquaculture and the Environment, ed S. E. Shumway (Oxford, UK: Wiley-Blackwell), 359–394. doi: 10.1002/9780470960967.ch13
Eriksen, M., Lebreton, L. C. M., Carson, H. S., Thiel, M., Moore, C. J., Borerro, J. C., et al. (2014). Plastic pollution in the World's oceans: more than 5 trillion plastic pieces weighing over 250,000 tons afloat at sea. PLoS ONE. 9:e111913. doi: 10.1371/journal.pone.0111913
FAO (2020a). FAOSTAT Statistics Database. Available online at: http://www.fao.org/faostat/en/#data (accessed January 20, 2020).
FAO (2020b). Fishery and Aquaculture Statistics. Global Aquaculture Production 1950-2017 (FishstatJ). FAO Fisheries and Aquaculture Department. Rome. Available online at: www.fao.org/fishery/statistics/software/fishstatj/en (accessed January 20, 2020).
Featherstone, S. (ed.). (2015). “Ingredients used in the preparation of canned foods,” in A Complete Course in Canning and Related Processes (Oxford: Elsevier), 147–211. doi: 10.1016/B978-0-85709-678-4.00008-7
Filgueira, R., Guyondet, T., Comeau, L. A., and Tremblay, R. (2016). Bivalve aquaculture-environment interactions in the context of climate change. Glob. Chang. Biol.. 22, 3901–3913. doi: 10.1111/gcb.13346
Gentry, R. R., Froehlich, H. E., Grimm, D., Kareiva, P., Parke, M., Rust, M., et al. (2017). Mapping the global potential for marine aquaculture. Nat. Ecol. Evol. 1, 1317–1324. doi: 10.1038/s41559-017-0257-9
Google (2020). Google Scholar. Available online at: https://scholar.google.com (accessed August 20, 2020).
Hackney, C. R., and Rippen, T. E. (2000). “The molluscan shellfish industry,” in Marine and Freshwater Products Handbook (Lancaster, PA: Technomic Publishing Co. Lancaster, PA, USA), 299–308. Available online at: https://www.routledge.com/Marine-and-Freshwater-Products-Handbook/Martin-Carter-Flick-Jr-Davis/p/book/9781566768894 (accessed June 23, 2020).
Häder, D.-P., Banaszak, A. T., Villafañe, V. E., Narvarte, M. A., González, R. A., and Helbling, E. W. (2020). Anthropogenic pollution of aquatic ecosystems: emerging problems with global implications. Sci. Total Environ.. 713:136586. doi: 10.1016/j.scitotenv.2020.136586
Helm, M., and Bourne, N. (2004). The Hatchery Culture of Bivalves: A Practical Manual. Rome. Available online at: http://www.fao.org/3/y5720e/y5720e00.htm (accessed June 23, 2020).
Herbert, R. J. H., Humphreys, J., Davies, C. J., Roberts, C., Fletcher, S., and Crowe, T. P. (2016). Ecological impacts of non-native Pacific oysters (Crassostrea gigas) and management measures for protected areas in Europe. Biodivers. Conserv.. 25, 2835–2865. doi: 10.1007/s10531-016-1209-4
Hollenbeck, C. M., and Johnston, I. A. (2018). Genomic tools and selective breeding in molluscs. Front. Genet.. 9:253. doi: 10.3389/fgene.2018.00253
Horton, T., Kroh, A., Ahyong, S., Bailly, N., Boyko, C. B., Brandão, S. N., et al. (2020). World Register of Marine Species (WoRMS). Available online at: http://www.marinespecies.org (accessed June 23, 2020).
Hoseinifar, S. H., Sun, Y.-Z., Wang, A., and Zhou, Z. (2018). Probiotics as means of diseases control in aquaculture, a review of current knowledge and future perspectives. Front. Microbiol.. 9:2429. doi: 10.3389/fmicb.2018.02429
Hossen, M. F., Hamdan, S., and Rahman, M. R. (2014). Cadmium and lead in blood cockle (Anadara granosa) from Asajaya, Sarawak, Malaysia. Sci. World J. 2014, 1–4. doi: 10.1155/2014/924360
Howard, P. H. (2016). Concentration and Power in the Food System: Who Controls What We Eat?. Bloomsbury Publishing. Available online at: https://books.google.co.uk/books?id=qyPYCgAAQBAJ (accessed June 23, 2020).
Llorente, I., and Luna, L. (2016). Bioeconomic modelling in aquaculture: an overview of the literature. Aquac. Int. 24, 931–948. doi: 10.1007/s10499-015-9962-z
Millman, B. M. (1967). Mechanisms of contraction in molluscan muscle. Am. Zool.. 7, 583–591. doi: 10.1093/icb/7.3.583
Paalvast, P., and van der Velde, G. (2011a). Distribution, settlement, and growth of first-year individuals of the shipworm Teredo navalis L. (Bivalvia: Teredinidae) in the port of Rotterdam area, the Netherlands. Int. Biodeterior. Biodegrad. 65, 379–388. doi: 10.1016/j.ibiod.2010.11.016
Paalvast, P., and van der Velde, G. (2011b). New threats of an old enemy: the distribution of the shipworm Teredo navalis L. (Bivalvia: Teredinidae) related to climate change in the port of Rotterdam area, the Netherlands. Mar. Pollut. Bull. 62, 1822–1829. doi: 10.1016/j.marpolbul.2011.05.009
Paalvast, P., and van der Velde, G. (2013). What is the main food source of the shipworm (Teredo navalis)? A stable isotope approach. J. Sea Res. 80, 58–60. doi: 10.1016/j.seares.2013.03.003
Rockström, J., Edenhofer, O., Gaertner, J., and DeClerck, F. (2020). Planet-proofing the global food system. Nat. Food 1, 3–5. doi: 10.1038/s43016-019-0010-4
Saberioon, M., Gholizadeh, A., Cisar, P., Pautsina, A., and Urban, J. (2017). Application of machine vision systems in aquaculture with emphasis on fish: state-of-the-art and key issues. Rev Aquac. 9, 369–387. doi: 10.1111/raq.12143
Schiemer, C., Halloran, A., Jespersen, K., and Kaukua, P. (2018). “Marketing insects: superfood or solution-food?,” in Edible Insects in Sustainable Food Systems, eds,. A., Halloran, R., and Flore, P., Vantomme, and N., Roos, (Cham: Springer International Publishing), 213–236. doi: 10.1007/978-3-319-74011-9_14
Shang, Y. C. (1985). Aquaculture economics: an overview. GeoJournal. 10, 299–304. doi: 10.1007/BF00462130
Sinyo, Y., Anggoro, S., and Soeprobawati, T. R. (2019). Morphologic characteristics and population density of Teredo Navalis L in mangrove forest area, Wailukum East Halmahera Regency. J. Phys. Conf. Ser. 1217:012141. doi: 10.1088/1742-6596/1217/1/012141
Sodano, V., and Verneau, F. (2014). Competition policy and food sector in the European union. J. Int. Food Agribus Mark. 26, 155–172. doi: 10.1080/08974438.2013.833576
Stentiford, G. D., Sritunyalucksana, K., Flegel, T. W., Williams, B. A. P., Withyachumnarnkul, B., Itsathitphaisarn, O., et al. (2017). New paradigms to help solve the global aquaculture disease crisis. PLoS Pathog. 13:e1006160. doi: 10.1371/journal.ppat.1006160
United States Department of Agriculture Agricultural Research Service (2020). USDA Food Composition Databases. Available online at: https://fdc.nal.usda.gov (accessed July 14, 2020).
Waite, R., Beveridge, M., Brummett, R., Castine, S., Chaiyawannakarn, N., Kaushik, S., et al. (2014). Improving productivity and environmental performance of aquaculture: creating a sustainable food future. World Resour. Inst. 6, 1–60. Available online at: https://www.wri.org/publication/improving-aquaculture
Willer, D., and Aldridge, D. C. (2017). Microencapsulated diets to improve bivalve shellfish aquaculture. R Soc Open Sci. 4:171142. doi: 10.1098/rsos.171142
Willer, D. F., and Aldridge, D. C. (2019a). Microencapsulated diets to improve bivalve shellfish aquaculture for global food security. Glob. Food Sec. 23, 64–73. doi: 10.1016/j.gfs.2019.04.007
Willer, D. F., and Aldridge, D. C. (2019b). Microencapsulated diets to improve growth and survivorship in juvenile European flat oysters (Ostrea edulis). Aquaculture. 505, 256–262. doi: 10.1016/j.aquaculture.2019.02.072
Willer, D. F., and Aldridge, D. C. (2020a). Sustainable bivalve farming can deliver food security in the tropics. Nat. Food. 1, 384–388. doi: 10.1038/s43016-020-0116-8
Willer, D. F., and Aldridge, D. C. (2020b). Vitamin bullets. microencapsulated feeds to fortify shellfish and tackle human nutrient deficiencies. Front Nutr. 7:102. doi: 10.3389/fnut.2020.00102
Willett, W., Rockström, J., Loken, B., Springmann, M., Lang, T., Vermeulen, S., et al. (2019). Food in the anthropocene: the EAT–lancet commission on healthy diets from sustainable food systems. Lancet 393, 447–492. doi: 10.1016/S0140-6736(18)31788-4
Keywords: aquaculture, shipworm, Teredo navalis, sustainable, nutrition, clam, food security, bivalve
Citation: Willer DF and Aldridge DC (2020) From Pest to Profit—The Potential of Shipworms for Sustainable Aquaculture. Front. Sustain. Food Syst. 4:575416. doi: 10.3389/fsufs.2020.575416
Received: 23 June 2020; Accepted: 25 August 2020;
Published: 15 September 2020.
Edited by:
Yasmina Sultanbawa, The University of Queensland, AustraliaReviewed by:
Ranjay K. Singh, Central Soil Salinity Research Institute (ICAR), IndiaLouwrens Hoffman, The University of Queensland, Australia
Copyright © 2020 Willer and Aldridge. This is an open-access article distributed under the terms of the Creative Commons Attribution License (CC BY). The use, distribution or reproduction in other forums is permitted, provided the original author(s) and the copyright owner(s) are credited and that the original publication in this journal is cited, in accordance with accepted academic practice. No use, distribution or reproduction is permitted which does not comply with these terms.
*Correspondence: David F. Willer, ZHc0NjAmI3gwMDA0MDtjYW0uYWMudWs=