- International Center of Insect Physiology and Ecology (icipe), Nairobi, Kenya
Arthropod pests and vectors constrain the livelihood opportunities of people in Africa by debilitating production of crops and livestock and through transmission of vector-borne diseases. In the absence of effective alternative management options to tackle these pests and vectors, there is extensive dependence on synthetic pesticides for their management on crop and livestock systems, with significant negative impacts on animal and human health, and the environment. Biopesticides are effective and environmentally sustainable alternatives to synthetic pesticides. At the International Centre of Insect Physiology and Ecology (icipe), the Arthropod Pathology Unit (APU) was established for effective biopesticide research-for-development (R4D), underpinned by a large repository of arthropod pathogens, protocols for lab bioassays and field efficacy testing, and an effective public-private partnership to generate new biopesticide products. The focus of icipe's APU has gradually transformed from basic to applied research leading to innovative, commercial products. Among the insect pathogens, greater focus has been placed on fungi, especially Metarhizium anisopliae, against key crop and livestock pests. Presently, three biopesticides based on M. anisopliae strains researched by icipe have been commercialized by Real IPM (Thika, Kenya) and are used on 132,994 ha in sub-Saharan Africa, with registration of additional products against animal ticks and the fall armyworm Spodoptera frugiperda pending. Our R4D activities on arthropod pathogens increasingly include bacteria, microsporidia, entomopathogenic nematodes and viruses. Recently, icipe is expanding R4D toward plant endophytes and rhizosphere inhabitants. The Centre also embarked on understanding the diversity, roles and possible exploitation of insect symbionts in key plant pest and disease vectors. In addition, key entomopathogens of reared insects for human food and animal feed need to be identified and controlled through high hygiene standards during rearing. Further, research is aimed at integrating biopesticides not only with other integrated pest management (IPM) technologies but also with pollination services.
Introduction
The Need for Biopesticides
In the absence of effective alternative management options to tackle pests, smallholder farmers rely extensively on indiscriminate application of synthetic pesticides. These synthetic pesticides are harmful to human health, detrimental to the environment and biodiversity, and lead to rapid build-up of resistance in the target pests while decimating natural enemies of pests, resulting in secondary pest outbreaks. In addition, presence of pesticide residues on export crops that are above the permissible maximum residue levels of importing nations results in informal trade barriers (Bailey et al., 2010).
Especially for smallholder farmers who rely on their crops as a primary dietary staple, it is essential to introduce management techniques of lower toxicity. Biopesticides may offer an essential alternative to the indiscriminate use of synthetic pesticides. Biopesticides are generally less toxic than conventional pesticides and therefore considered safer for human health. Biopesticides often only affect the target pest and therefore do not pose negative effects on the environment. Also, their use does not lead to resistance build-up in target pests.
The global market for biopesticides is valued at 3.0 billion USD, accounting for 5% of the global pesticide market (Marrone, 2014). With an annual compounded growth rate of more than 15%, it is anticipated that biopesticide market share will equal that of synthetic pesticides between 2040 and 2050 (Olson, 2015; Dalmas and Koutroubas, 2018).
According to the United States Environmental Protection Agency (EPA), biopesticides are pesticides derived from natural materials such as animals, plants, bacteria and certain minerals, and can be classified in three classes: (1) microbial pesticides, which consist of a microorganism as the active ingredient; (2) biochemical pesticides, which are naturally occurring substances that control pests by non-toxic mechanisms such as insect sex pheromones and plant extracts; and (3) plant-incorporated protectants, which are pesticidal substances that plants produce from genetic material that has been added to the plant. This review mainly focuses on icipe's R4D initiatives on microbial pesticides.
Biopesticide Use in Africa
In Africa, biopesticide use is still at its infancy and only accounts for 3% of the world biopesticide market, although little information is available on adoption rates of biopesticides on the continent (Olson, 2015). Although several biopesticides are registered in countries across Africa, few are developed on the continent itself (Grzywacz et al., 2019). However, changes are occurring, with South Africa and Kenya leading in biopesticide development and use. In South Africa, of the 31 products registered, seven are locally produced, mainly based on Beauveria bassiana (Hatting et al., 2019). A major supplier of biopesticides in Africa is Real IPM, which has marketed four strains from icipe (Metarhizium anisopliae strains ICIPE 69, 78, 62 and 7). Between 2015 and 2019, use of Real IPM's entomopathogenic fungi-based products has increased more than 3-fold from only 43,290 ha in 2015 to 132,980 ha in 2019 (Figure 1). Products based on M. anisopliae strain ICIPE 69, which target mealybugs, thrips, leafminers and fruit flies, constitute the largest African portfolio, the use of which increased the fastest, from 19,370 ha in 2015 to 80,420 ha in 2019, a more than 4-fold increase.
History of Biopesticide Research-for-Development at icipe
Figure 2 provides an overview of the history of biopesticide R4D at icipe. Research on insect pathogens at icipe started as early as in 1974, when virus infections were detected in field populations of the African armyworm Spodoptera exempta. In the 1970's, focus was on diagnosis of pathogens occurring on field-collected and reared insects, especially crop pests such as S. exempta and the sorghum shoot fly Atherigona soccata; and vectors such as mosquitoes and tsetse flies (Glossina spp.). Research also aimed to understand the mutualistic interaction between termites and symbiotic fungi belonging to Termitomyces sp. icipe formalized research into entomopathogens and its application for pest management with the establishment of the Insect Pathology and Pest Management Program in 1982, which subsequently transformed in the Pathology and Microbiology Department in 1994 and finally into the Arthropod Pathology Unit (APU) in 1999. Insect pathology research at icipe in the 1980's comprised systematic bioprospecting for entomopathogens using the Galleria mellonella bait method, establishment of a facility for bioassays, mass production and field testing. The efficacy of several strains of entomopathogenic fungi, microsporidia, Bacillus thuringiensis and other entomopathogenic bacteria was tested in the laboratory and the field against major staple food crop pests such as maize stemborers, the bean pod borer Maruca vitrata and the cassava green mite Mononychellus tanajoa. Research into management of malaria-vectoring mosquito species targeted evaluation of entomopathogenic fungi such as Coelomomyces sp. and microsporidia (mainly Nosema sp., Thelohania sp. and Duboscqia sp.). The identification of non-occluded viruses infecting salivary glands of tsetse flies triggered extensive basic research on the virus in the 1980's. Systematic bioprospecting for arthropod pathogens began in the 1980's leading to the establishment of an arthropod pathogen germplasm repository in 1992. In 1984, research on hildecarpin, a phytoalexin-like molecule elicited by cowpea after infection by non-pathogenic microbes, marked icipe's first foray into research on plant endophytes. During the 1990's, small-scale mass production of several insect pathogens was implemented: Hirsutella thompsoni for cassava green mite management; Nosema marucae for management of the Asian stemborer Chilo suppressalis and M. vitrata (Maniania, 1993); Metarhizium anisopliae and Beauveria bassiana for management of locusts, maize stemborers, ticks and tsetse flies; B. thuringiensis for management of the spotted stalk borer Chilo partellus, mosquitoes and filth flies; and baculoviruses for management of S. exempta. The 1990's also marked the beginning of non-target tests of entomopathogens such as N. marucae on parasitoids and predators of crop pests. In addition, research in the 1990's aimed to integrate entomopathogens with other sustainable IPM options such as intercropping, resistant host plants and semio-chemicals. During the 2000's, icipe's APU engaged in identification, screening and selection of entomopathogenic fungi; assessed the effect of biotic and abiotic factors on entomopathogens; and developed mass production techniques and formulations for some of the most effective strains. Targets included major invasive and indigenous pests of staples, legumes, vegetables and fruit crops, as well as livestock pests such as ticks and tsetse flies. The early 2000's also marked the beginning of icipe's research on insect endosymbionts, which is increasingly becoming relevant for deeper insights into insect ecology, behavior and management. During the 2010's, some of most effective M. anisopliae strains have been successfully commercialized with the private sector partner Real IPM. R4D against animal pests and disease vectors will soon yield registration of a first product based on M. anisopliae strain ICIPE 7. Concurrently, research focused on innovative formulations (oil-based, granular and dry spore formulations) and development of novel application strategies such as lure-and-infect, spot-sprays and autodissemination. Recently, extensive bioprospecting for plant endophytes has resulted in the identification of several strains against diverse pest constraints such as Liriomyza leafminers, thrips and the bean fly Ophiomyia phaseoli. Currently, biopesticide R4D is fully integrated into buy's 4H paradigm, comprising plant, animal, environmental and human health. Over the years, bioprospecting by icipe's APU has yielded a large repository of 485 arthropod pathogens including entomopathogenic fungi, bacteria, viruses, microsporida and, recently, entomopathogenic nematodes (Table 1). This repository is an invaluable resource for screening entomopathogens against key indigenous and invasive arthropod pests in sub-Saharan Africa. At present, icipe's APU is a reference point for biopesticide development in sub-Saharan Africa, with a unique facility, state-of-art equipment and excellent human resources to carry out biopesticide R4D.
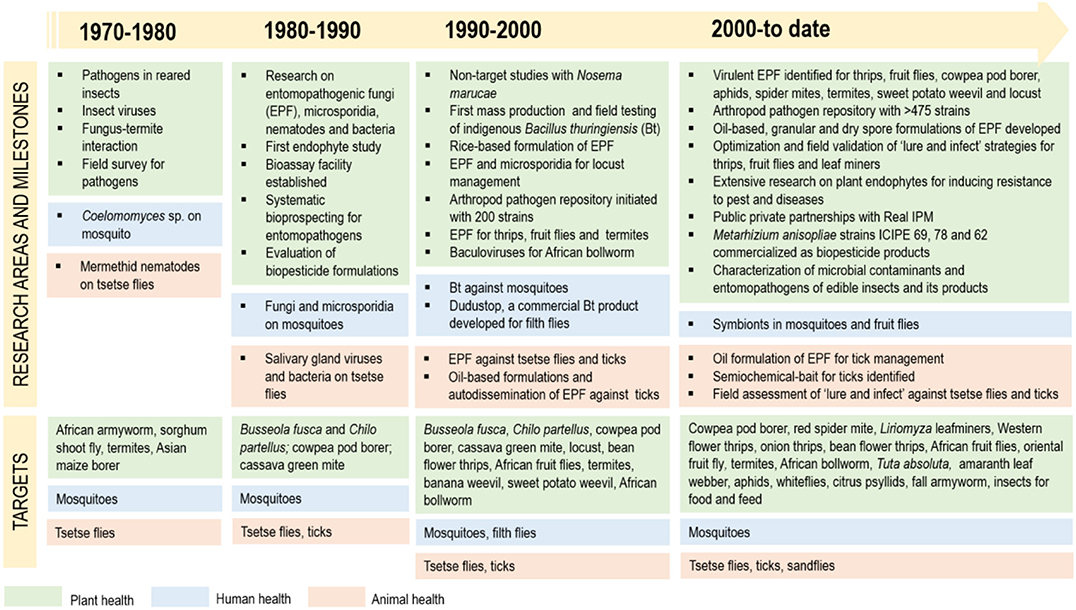
Figure 2. History of biopesticide R4D at the International Center of Insect Physiology and Ecology (icipe). Source: icipe.
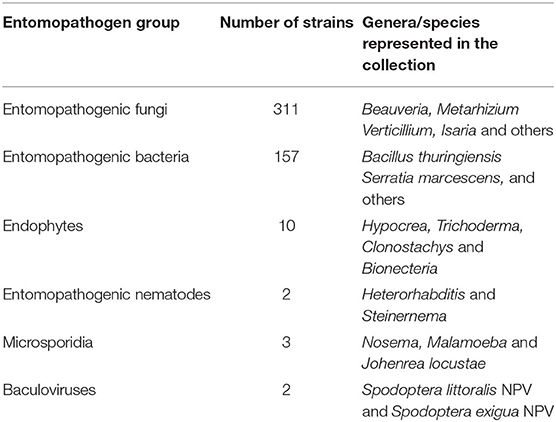
Table 1. Arthropod pathogen germplasm repository at the International Centre of Insect Physiology and Ecology (icipe) for biopesticides development.
Biopesticide Research as a Function of Targets
Plant Pests
A plethora of polyphagous indigenous and invasive pests, such as whiteflies, leafminers (Liriomyza sp.), cereal stemborers (C. partellus and Busseola fusca), the diamondback moth Plutella xylostella, the African bollworm Helicoverpa armigera, the red spider mite Tetranychus urticae, the tomato spider mite Tetranychus evansi, aphids, thrips, fruit flies, pod borers, pod suckers, storage beetles, the false codling moth Thaumatotibia leucotreta and, recently, the fall armyworm Spodoptera frugiperda and the tomato leafminer Tuta absoluta significantly reduce sustainable production of staple and horticultural crops, and consequently inflict enormous economic losses. In addition, some insects are vectors of serious plant diseases (e.g., thrips, whiteflies and the cowpea aphid Aphis craccivora). icipe has actively engaged in research to identify virulent entomopathogens of these pests, with a greater emphasis on entomopathogenic fungi belonging to the genera of Metarhizium sp. and Beauveria sp. Strains belonging to this genus are relatively easy to mass-produce, formulate and apply, and were found to be among the most virulent against Africa's pests. Longstanding research has yielded significant results, and currently, three fungal products based on M. anisopliae strains ICIPE 69, ICIPE 78 and ICIPE 62 are commercialized for management of key pests in several countries in sub-Saharan Africa as well as Canada (Table 2). Currently, screening efforts are expanding beyond M. anisopliae toward other entomopathogenic fungi such as B. bassiana and Isaria fumosorosea, bacteria, microsporidia, nematodes and viruses. A recent research thrust centers on endophytes such as Hypocrea lixii and Trichoderma sp.
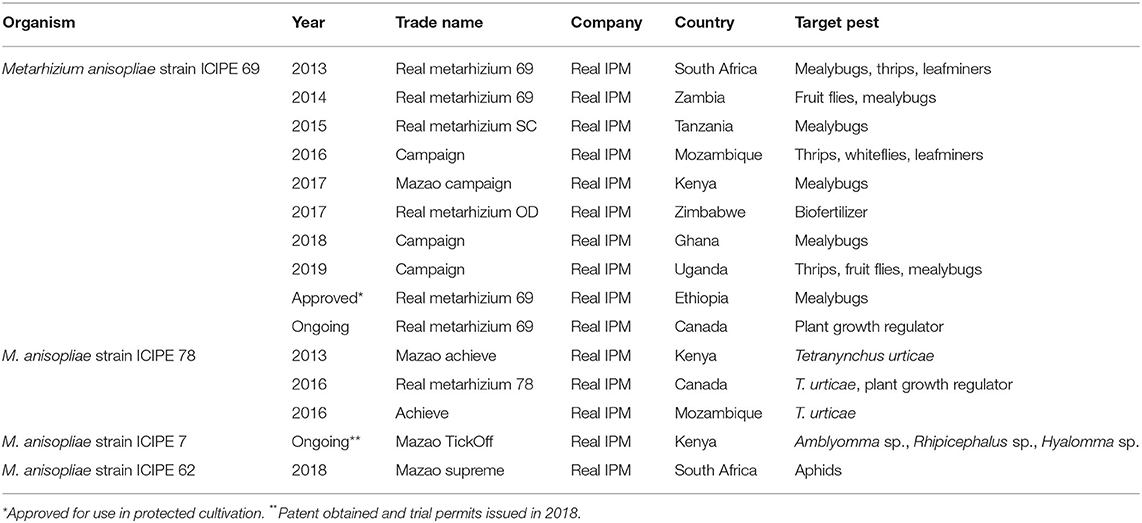
Table 2. Registered biopesticides based on research-for-development at the International Centre of Insect Physiology and Ecology (icipe).
Based on long-standing experience in developing M. anisopliae into biopesticides, the time taken from identification of virulent strains to commercialization as biopesticide products has considerably shortened. This is attributed largely to more efficient R4D related to product development, better interactions with regulatory authorities and policy makers, and stronger engagement with the private sector and partnership arrangements to identify market needs. For example, whereas it took >15 years from the first screening of M. anisopliae strain ICIPE 69 to development into the commercial product Real Metarhizium 69, we estimate that duration between first screening of M. anisopliae strain ICIPE 7 for S. frugiperda to commercialization is likely to take only 36 months. Faster biopesticide development is crucial to tackle biological control against emerging pests such as S. frugiperda, T. absoluta and the desert locust Schistocerca gregaria.
Animal Pests and Disease Vectors
Ticks (Rhipicephalus sp., Boophilus sp., Amblyomma sp.) and tsetse flies are two major constraints to livestock production through direct feeding/biting and as vectors of various vector-borne diseases. Management of ticks is largely undertaken with acaricides, which is increasingly becoming ineffective due to high levels of acaricide resistance. Management of tsetse flies involves sequential aerosol spraying technique (SAT), ground spraying, insecticide-treated targets such as odor-baited traps, repellent collars and sterile insect technique (Politzar and Cuisance, 1982; Gouteux and Lancien, 1986; Takken et al., 1986; Dransfield et al., 1990; Oladunmade et al., 1990; Bauer et al., 1995; Leak et al., 1995; Saini et al., 2017). These techniques have various limitations ranging from their efficacy, economically viability and environmental sustainability. Extensive bioprospecting has been conducted by icipe for virulent entomopathogens against both ticks and tsetse flies. Metarhizium anisopliae strain ICIPE 7 (Nchu et al., 2009, 2010a,b; Nana et al., 2012, 2016) and M. anisopliae strain ICIPE 30 (Maniania, 1998, 2002; Maniania et al., 2006, 2013) were identified as ideal biopesticide candidates against ticks and tsetse flies, respectively. Furthermore, M. anisopliae strain ICIPE 7 was found to be equally effective against both acaricide-resistant and -susceptible tick populations (Murigu et al., 2016). Research on the use of pathogens against animal pests and disease vectors has resulted in the development of the commercial product Mazao Tickoff (Real IPM), which is based on M. anisopliae strain ICIPE 7. icipe has also developed an innovative formulation of the product, with both tick repellent and entomopathogenic action patented and expected to be registered against the livestock ticks Amblyomma sp., Rhipicephalus sp. and Hyalomma sp. Metarhizium anisopliae strain ICIPE 30 is currently being registered against the tsetse flies Glossina morsitans morsitans, Glossina fuscipes fuscipes and Glossina pallidipes.
Developing biopesticides against animal pests and disease vectors included extensive research on novel formulations, such as oil-based formulations of M. anisopliae strain ICIPE 7. Trapping tsetse flies through use of odor baits holds promise as a viable and environmentally sustainable technology, and may be combined with biopesticides as a killing agent. Similarly, combination of baits with biopesticides is a novel avenue for tick control (Nana et al., 2016). Using a lure-and-infect approach, M. anisopliae strain ICIPE 30 has been integrated with odor-baited traps, and reduced Trypanosoma congolensis reproduction in G. fuscipes fuscipes and its ability to acquire or transmit the parasite. The impact of such an approach on vector reduction and parasite transmission capacity is currently being measured (Wamiti et al., 2018). The use of plant attractants equally constitutes a novel application strategy of entomopathogens for animal pests and disease vectors. Extracts from Calpurnea aurea, a Southern African fabaceous tree, were shown to be strong attractants of the zebra tick Rhipicephalus pulchellus and can be used to target biopesticide applications in pastures through spot-spraying (Nana et al., 2016).
Human Disease Vectors
Arthropod vector-borne diseases inflict more than 700,000 deaths annually, with mosquito-transmitted malaria alone causing 400,000 deaths globally. Most of these deaths occur in Africa and are exacerbated due to lack of capacity to effectively manage the vectors and the diseases they carry. Apart from mosquitoes, other important vectors of human diseases include tsetse flies, ticks, sand flies, fleas, black flies and triatomine bugs (WHO, 2020). icipe's biopesticide R4D has focused on management of mosquitoes, ticks, tsetse flies and sand flies. Screening for pathogenicity of M. anisopliae and B. bassiana strains against adult Phlebotomus duboscqi, an important vector of leishmaniasis, has resulted in the identification of six effective strains that could be further progressed for field testing and commercialization (Ngumbi et al., 2011). Research on biopesticides for mosquito (Anopheles gambiae and Aedes aegypti) management prior to the 2000's largely focused on bioprospecting for Bt, Coelomomyces sp. and microsporidia for management of larval stages, with large-scale field evaluation of commercial Bt israelensis formulations for mosquito management in Ethiopia and Kenya (ICIPE, 2012). Metarhizium anisopliae strain ICIPE 30, a strain from icipe's bioprospecting efforts, has been extensively researched for mosquito control by Ifakara Health Institute (Ifakara, Tanzania), Wageningen University (Wageningen, Netherlands) and the Liverpool School of Tropical Medicine of the University of Liverpool (Liverpool, UK). The strain proved to be highly virulent against A. gambiae under screenhouse conditions, with elevated virulence against pyrethroid-resistant populations (Howard et al., 2010; Mnyone et al., 2010, 2011).
Biopesticide Development and Commercialization
Registered Biopesticides
icipe, in partnership with private sector partners, notably Real IPM, has developed and commercialized three entomopathogenic biopesticides based on the fungus M. anisopliae for the management of a variety of pests that attack crops (Table 2). All these biopesticides are based and marketed by Real IPM and its subsidiaries across sub-Saharan Africa and beyond. Metarhizium anisopliae strain ICIPE 69 is effective against thrips, fruit flies and mealybugs; M. anisopliae strain ICIPE 78 is effective against T. urticae; M. anisopliae strain ICIPE 62 is effective against aphids. In some countries, such as Canada and Zimbabwe, M. anisopliae strains ICIPE 69 and ICIPE 78 have been registered as biofertilizers or plant growth regulators. In addition, one biopesticide based on M. anisopliae strain ICIPE 7 is in the final stages of registration and subsequent commercialization for the management of livestock ticks and S. frugiperda.
In sub-Saharan Africa, biopesticides developed by icipe are registered and commercialized in nine countries: Ethiopia, Ghana, Kenya, Mozambique, South Africa, Tanzania, Uganda, Zambia and Zimbabwe. These biopesticides were applied on >89,000 ha and nearly 133,000 ha in 2019 and 2020, respectively, indicating an exponentially expanding coverage (Figure 1). We expect the number of countries and geographic coverage to increase significantly in the coming years. Real IPM, in collaboration with Elephant Vert (Rabat, Marocco), has begun registration of these icipe-based products in West African countries other than Ghana as well as Europe, Asia, the UK and the USA. Undoubtedly, growers, both small- and large-scale, are becoming increasingly aware of the benefits of using biopesticides and decide to apply them, backed by strong awareness campaigns and capacity building by icipe and its partners.
Biopesticides in the Pipeline
Several arthropod pathogens are being developed into biopesticides against plant and animal pests, and disease vectors (Table 3). The majority of arthropod pathogenic strains belong to M. anisopliae, and include M. anisopliae strain ICIPE 18 (against T. absoluta, Maruca vitrata, C. partellus and B. fusca) (Maniania, 1993), M. anisopliae strain ICIPE 20 (against T. absoluta and S. frugiperda and the pea leafminer Liriomyza huidobrensis) (Migiro et al., 2010; Mohamed et al., 2017; Akutse et al., 2019a), M. anisopliae strains ICIPE 40, ICIPE 41, ICIPE 315 and ICIPE 655 (against S. frugiperda) (Akutse et al., 2019a), M. anisopliae strain ICIPE 30 (against the amaranth leaf webber Spoladea recurvalis (Opisa et al., 2018, 2019); the stemborers C. partellus and B. fusca (Maniania, 1993); the mosquitoes A. gambiae and A. aegypti; the tsetse flies G. morsitans morsitans, G. fuscipes fuscipes and G. pallidipes; and the sand flies Phlebotomus martini and P. duboscqi) (Ngumbi et al., 2011; Ngure et al., 2015); and M. anisopliae strain ICIPE 51 (against the termite Macrotermes michaelseni) (Mburu et al., 2009, 2013). In addition, strains that already have been commercialized are being tested against others pests: M. anisopliae strains ICIPE 7 and ICIPE 78 against S. frugiperda (Akutse et al., 2019a); and M. anisopliae strain ICIPE 69 against T. leucotreta (Mkiga et al., 2020) and M. vitrata (Tumuhaise et al., 2015, 2018).
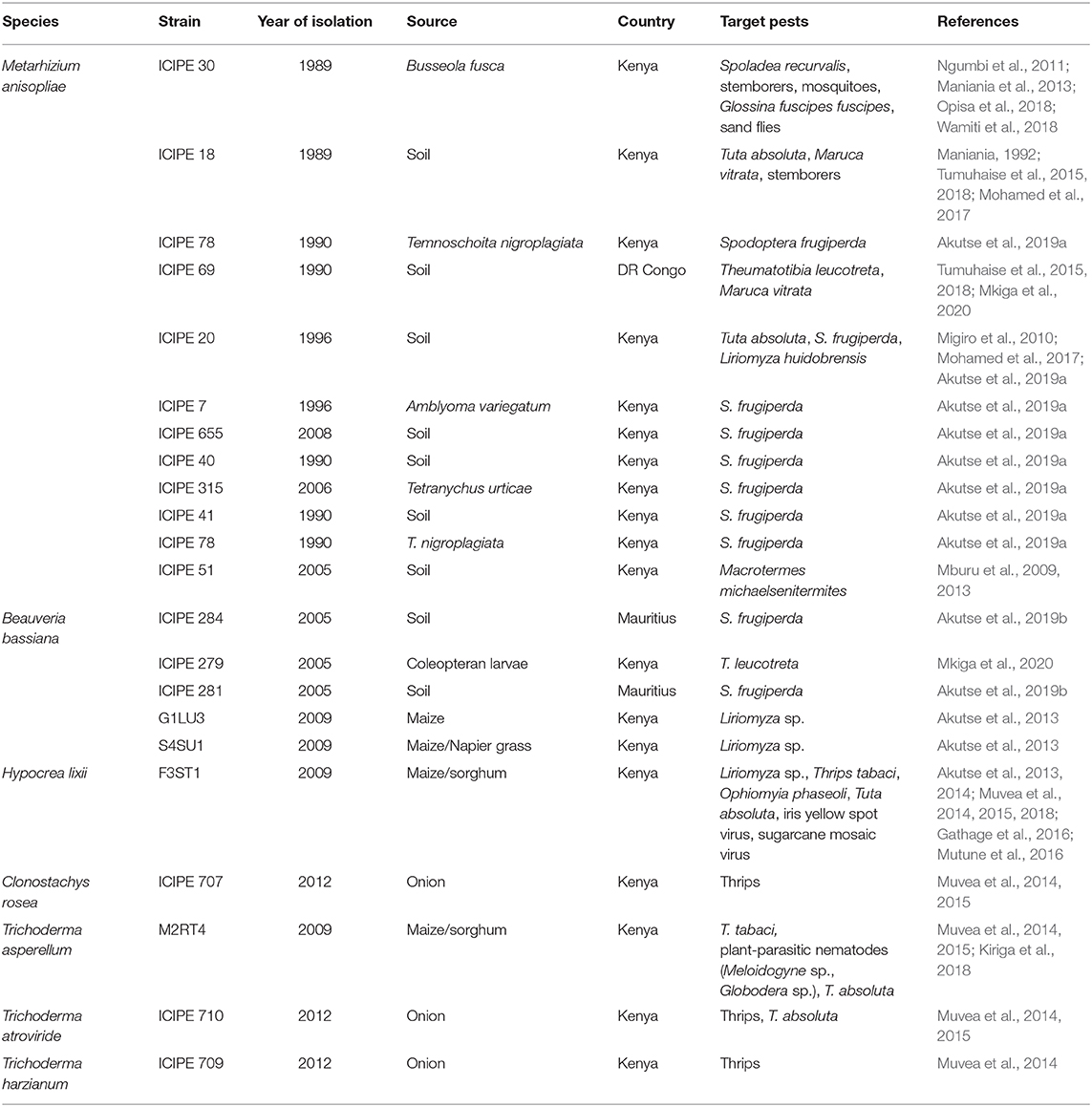
Table 3. Biopesticides in the pipeline based on research-for-development at the International Centre of Insect Physiology and Ecology (icipe).
Focus has equally shifted toward fungi other than M. anisopliae. Five B. bassiana strains (ICIPE 284, ICIPE 279, ICIPE 281, G1LU3 and S4SU1) are being tested against S. frugiperda, T. leucotreta and Liriomyza sp. (Migiro et al., 2010; Akutse et al., 2019b; Mkiga et al., 2020). Beauveria bassiana strain ICIPE 279, especially, is highly pathogenic to T. leucotreta (Mkiga et al., 2020), and we are confident that, in combination with M. anisopliae strain ICIPE 69, a biopesticide will soon be available against this devastating pest.
Following the detection of S. frugiperda in Africa, a great effort was placed on screening strains for control of this debilitating pest. Results from laboratory bioassay showed high virulence of M. anisopliae strains ICIPE 7, 20, 40, 41, 78, and 655, inflicting >90% egg and neonate mortality (Akutse et al., 2019a). Other strains were found to induce up to 100% mortality in adults, including B. bassiana strains ICIPE 621 and ICIPE 676, and M. anisopliae strains ICIPE 7, ICIPE 78 and ICIPE 315 (Akutse et al., 2019a). Since M. anisopliae strain ICIPE 78 is already commercialized, it is currently being fast-tracked through label extension for use against S. frugiperda, while M. anisopliae strain ICIPE 7 is being registered as a new formulation.
Research on endophytes offers novel opportunities to target cryptic pests and even diseases, largely due to systemic induced resistance as a mode of action. Endophytes such as Hypocrea lixii strain F3ST1, Trichoderma harzianum strain ICIPE 709, Clonostachys rosea strain ICIPE 707 and Trichoderma atroviride strain ICIPE 710 were found to be effective against Liriomyza sp., T. absoluta, the onion thrips Thrips tabaci and O. phaseoli, as well as the plant viruses iris yellow spot virus (IYSV) and sugarcane mosaic virus (SCMV) (Akutse et al., 2013, 2014; Muvea et al., 2014, 2015, 2018; Mutune et al., 2016). Trichoderma asperellum strain M2RT4 was found to be very effective against root knot nematodes (Meloidogyne sp.) and potato cyst nematodes (Globodera sp.) through systemic induced resistance (Kiriga et al., 2018).
Development of novel biopesticides against animal pests and disease vectors focuses on G. fuscipes fuscipes (Maniania, 1998, 2002; Maniania et al., 2006). Metarhizum anisopliae strain ICIPE 30 was found to negatively interfere with the multiplication of the parasite Trypanosoma congolense in the tsetse fly and reduced the capacity of G. fuscipes fuscipes to acquire or transmit the parasite (Wamiti et al., 2018).
Partnerships, Scaling and Capacity Building
Throughout the years, and based on experience from developing four strains into commercial biopesticides, development of novel biopesticides has been streamlined, greatly reducing product development timeline between initial screening and registration, which is critical for tackling emerging invasive pests such as S. frugiperda. Based on icipe's large arthropod pathogen repository, diverse strains are mass-screened against key target pest in laboratory bioassays. Strains are mainly selected based on their previous screening history regarding virulence against respective orders or genera of arthropod pests, local availability, host range, ease for mass production and formulation by the private sector, and application by farmers. Those strains that have the lowest LT50 and/or LC50 are further evaluated under screenhouse conditions and, at a later stage, in controlled field experiments. In most cases, field efficacy trials and product formulation are conducted with private sector partners. Working with the private sector on the most promising strains creates buy-in for later commercialization into biopesticides. During the registration process, icipe, in collaboration with the private sector and accredited good laboratory practice (GLP) laboratories, facilitates the generation of required eco- and mammalian toxicity test data, and assures quality. Awareness among regulatory authorities and various stakeholders on potential products is equally created at this stage. Through collaborative R4D activities, icipe subsequently supports further enhancement of product effectiveness and use (e.g., development of autodissemination approaches, improvements in formulation and integration with other IPM options).
Partnerships are a critical component of icipe's R4D activities related to biopesticide development. The private sector is an essential partner, and collaboration has been and is strong with Real IPM, leading to the registration and development of several biopesticide products and application technologies. Real IPM was founded in 2003 and since 2019 is a member of the Biobest Group (Westerlo, Belgium). From small beginnings in Thika, the company grew quickly and currently employs 228 staff, making it a leader in the development of biological control products in Africa. icipe's public-private partnerships are expanding to include other commercial companies. Kenya Biologics (Thika, Kenya), HottiServe East Africa (Nairobi, Kenya), Russell IPM (Deeside, UK), Farm Track Consulting (Nairobi, Kenya) and, more recently, Koppert Biological Systems (Berkel en Rodenrijs, Netherlands), Provivi (Santa Monica, USA) and Dudutech (Nairobi, Kenya) have indicated interest to collaborate in development of biopesticides and associate products, such as pheromones and insect traps. To galvanize and streamline collaboration with private sector partners, icipe is establishing a Biocontrol Consortium, which links member biopesticide companies with a footprint or interest in Africa. The Biocontrol Consortium is a novel scaling strategy to spearhead biopesticide development and use in Africa by better rooting biopesticide research in actual demand from farmers and consumers. Mutual benefits will include (1) technical training and access to advanced research on biopesticide strains and technologies (e.g., modelling, formulations, application technologies); (2) access to aggregated data from member biopesticide companies related to sales and markets, allowing for better targeting of crops, pests and countries for the development of biopesticides, and more efficient and faster scaling and commercialization; and (3) a unified platform to inform policy and regulatory bodies on registration requirements and regulatory harmonization.
Beyond the private sector partners, icipe collaborates actively with national agricultural research and extension system (NARES) partners for research, field evaluation of biopesticides, scaling and dissemination; universities for research and capacity building; non-governmental organizations (NGOs) for scaling and dissemination; international research organizations such as the Center for Agriculture and Biosciences International (CABI) and the International Institute of Tropical Agriculture (IITA) for joint research and capacity building; local governments for dissemination; and regulatory authorities for registration of products. Partnerships with national policy and regulatory bodies are strong and essential for product registration. In Kenya, collaboration has been excellent with the Pest Control Products Board and the Directorate of Veterinary Services.
Together with NARES and NGOs, biopesticides are scaled with farmers as part of comprehensive IPM packages. Such scaling efforts, complementing existing extension efforts of Real IPM and other private companies, are focused on smallholder farms and often coincide with on-farm research of products prior to registration. For example, icipe's fruit fly IPM program, arguably the most advanced and comprising a strong biopesticide component, is currently being scaled in Kenya, Ethiopia, Mozambique, Tanzania, Uganda, Zambia, Malawi and Zimbabwe. These efforts are supported by intensive trainings, e.g., through the establishment of learning centers, training of extension officers and awareness creation on IPM strategies. So far, a total of 8,175,957 mango growers benefited, including 49,739 growers who directly benefited through hands-on training and received IPM starter packs, 627,200 growers who benefited through parasitoid releases and 6,499,018 growers who indirectly benefited through interaction with trained growers, extension officers, NGOs and communities service providers. In addition, one million growers were reached through airing of TV programs on fruit fly IPM via Shamba Shape Up (Nairobi, Kenya). Building youth and women entrepreneurship for small-scale biopesticide production are planned for the future.
Capacity building is an essential element of icipe's biopesticide R4D. On average 4–5 PhDs and >10 MScs are trained annually on various aspects of arthropod pathogens, including bioprospecting, isolation, molecular characterization, laboratory bioassays, formulations, application technologies, socio-economics and value chains. APU also has a strong postdoctoral program on basic and applied insect pathology research.
Integration of Biopesticide Research into IPM
The use of biopesticides needs to be fully integrated into an IPM approach. For tephritid fruit fly management, biopesticides can be used for soil application, as a killing agent in bait sprays or in a lure-and-infect approach with attractants in a package that includes monitoring, the use of parasitoids, orchard sanitation, postharvest technologies, bait sprays and male annihilation technology. For S. frugiperda, biopesticide development is targeted at all life stages of the pest in a package that includes effective monitoring, conservation of natural enemies and diversified cropping systems. For thrips, such as Frankliniella occidentalis, T. tabaci and Megalurothrips sjostedti, biopesticides can target both larval and adult stages as direct sprays, lure-and-infect application and as endophytes enhancing systemic resistance as part of a package comprised of resistant cultivars, habitat management, the use of healthy seeds and seedlings, and the use of kairamones and pheromones for effective monitoring. For management of T. absoluta, biopesticides are being developed that target adults as well as induce systemic resistance through endophytic activity against larvae. These biopesticides are integrated into a package that includes monitoring and mass trapping, resistant cultivars, protected cultivation, field sanitation and classical biological control.
Socio-economic studies are increasingly being conducted to estimate the impact of biopesticides as part of an IPM package on crop yield and income among smallholder farmers in sub-Saharan Africa. Studies in Kenya in 2015 have shown that application of two to three components of an IPM package, comprised of parasitoid release, biopesticides, orchard sanitation, food bait and male annihilation technique, reduced mango yield losses caused by tephritid fruit flies by 19–55% and resulted, on average, in a 22–48% increase in mango net income compared to the previous season (Kibira et al., 2015; Muriithi et al., 2016). Application of a biopesticide (M. anisopliae strain ICIPE 69) in combination with parasitoid release and orchard sanitation yielded the most impact, illustrating that biopesticides are preferentially combined within a well-tailored IPM package to ensure maximal benefit for smallholder farmers. A follow-up study in Kenya between 2016 and 2018 demonstrated that the use of such an IPM package increases mango farmers' net income by 9–137%, decreased insecticide use by 64–89% and impacted positively on food security (Midingoyi et al., 2019; Nyangau et al., 2020). Further, the use of IPM, including biopesticides, generates positive effects on human health and the environment by reducing the environmental impact quotient (EIQ) by 9–40% (Midingoyi et al., 2019; Mwungu et al., 2020). With an estimated economic value of 19 million USD per year in Kenya, the benefit-cost ratio of fruit fly IPM is estimated at 27:1 over 32 years, equating a 29% internal rate of return and with a potential to reduce the number of rural poor people by 72,642. Socio-economic studies on biopesticide use and impact are essential to inform and assist policy makers on the importance of biopesticides, and may facilitate registration and regional harmonization through enabling policies. Economic benefits of biopesticide use in comparison to synthetic pesticides are often considered only at farm level in terms of yield advantage and reduced input costs. However, a realistic comparison of biopesticides with synthetic pesticides should include cost associated with negative externalities of synthetic pesticide use, altered pricing of safer products from biopesticide use and social impacts related to employment generation. For subsequent socio-economic impact studies, icipe will strive to undertake holistic assessments of socio-economic impacts of biopesticides use.
A novel area of research is integration of IPM with pollination services. Globally, 35% of food crop production is dependent on insect pollination, with an economic value amounting to 153 billion EURO annually (Gallai et al., 2009). Avocado, cucurbits and papaya are economically important pollination-dependent crops in some sub-Saharan countries. IPM and pollination services can interact positively or negatively in multifarious ways to ensure healthier agricultural ecosystems. Especially for biopesticides, a concern is their negative impact on pollinators. Using the integrated pest and pollinator management (IPPM) paradigm, research is aimed at ensuring biopesticides are compatible with pollination services. An effective IPPM strategy relies on a deep understand of biopesticide-pollinator interactions. Current research at icipe on avocado focuses on selection of M. anisopliae strains against tephritid fruit flies and T. leucotreca that are not pathogenic to the Western honey bee Apis mellifera and the stingless bee Meliponula ferruginea. Even if some mortality among bees is experienced in the laboratory, negative interactions may not occur in the field due to, for example, grooming or other social behavior of bees. Furthermore, application technologies such as lure-and-infect using pheromones may better target pests and avoid contact with bees.
Future R4D Thrusts
Biopesticides Against Invasive and Migrant Pests in Sub-Saharan Africa
An immediate focus for icipe and partners will be development of biopesticides targeting Africa's invasive and migrant pest, particularly S. frugiperda and the desert locust Schistocerca gregaria. Entomopathogenic strains have been identified and are being tested against different life stages of S. frugiperda, including eggs, early instar larvae, pupae and adults, which will lead to availability of a broad arsenal of biopesticides against this debilitating pest. Currently, the most virulent strains are assessed for non-target effects on natural enemies and formulations are fine-tuned for optimal field applications as a function of life stage, with field efficacy evaluation and fast-tracking of registration or label extension envisaged during 2020. During 2019–2020, countries in Eastern Africa experienced unprecedented swarms of S. gregaria. The research program “Lutte biologique contre les locustes et sauteriaux” (LUBILOSA) was carried out in the late 1980's and early 1990's by CABI, IITA and the Department for Crop Protection Training of Niger, in partnership with icipe. LUBILOSA developed the biopesticide Green Muscle, based on M. anisopliae var. acridum strain IMI 330189, which is highly effective for controlling S. gregaria (Bateman et al., 2017). Green Muscle as well as the novel product Novacrid based on Metarhizium acridum strain EVCH 077 are commercialized and marketed by Elephant Vert. icipe is currently testing other entomopathogens to broaden the biopesticide product range against S. gregaria through its established partnership with the private sector and regulatory authorities for fast-tracking registration and commercialization. In addition, we will further explore the combination of biopesticides with an adult pheromone, discovered by icipe in the 90's, that renders hoppers solitary and leads to dispersal with increased mortality (Torto et al., 1994; Bashir and Hassanali, 2010). The adult pheromone is a blend of four commercially available compounds, including phenylacetonitrile (PAN) (80%), benzaldehyde, guaiacol and phenol, and has been extensively tested and registered in Sudan. The PAN-based pheromone blend is compatible with Green Muscle, and its integration may lower required biopesticide doses and enhance S. gregaria predation by natural enemies.
Expansion Toward Animal and Human Diseases and Vectors
Another immediate research thrust is expansion of the biopesticide portfolio toward animal and human diseases and vectors. To enhance the tick biopesticide product based on M. anisopliae strain ICIPE 7, oil-based formulations for longer shelf-life will be optimized and integrated with tick repellents or attractants. Efficacy will also be tested on diverse groups of tick species in varied ecologies, while the effect of infection on vector transmission will be studied. For tsetse management, the lure-and-infect approach will be fine-tuned by combining biopesticides based on M. anisopliae strain ICIPE 30 with odor-baited traps in auto-dissemination devices and repellent collars. Human pests and disease vectors such as sand flies, biting flies, bed bugs and mosquitoes are a next frontier for biopesticide development. The common bed bug Cimex lectularius and the tropical bed bug Cimex hemipterus are found in tropical zones and temperate areas, respectively, but their populations overlap in Africa. Recent pest resurgence and persistence, partly caused by widespread resistance to synthetic pesticides, has raised major concerns in the region (Potter, 2004; Fourie and Crafford, 2018). Aprehend (Oldham Chemicals Company, Memphis, USA), a biopesticide based on B. bassiana, is currently registered for overseas markets, yet no product exists for Africa. At icipe, we aim to develop new biopesticides based on B. bassiana and other strains. Formulation and integration with pheromones or attractants will need to be a major focus to tackle these cryptic pests. Bed bugs, for example, produce large amounts of histamine as a component of their aggregation pheromone, which offers possibilities to include attractants into biopesticide products (Gries et al., 2015).
R4D into Insect Symbionts
There is a growing interest toward understanding the role of symbionts in modulating multitrophic interactions between plants, pests and their natural enemies, and exploring their role in biological control. For instance, presence of Wolbachia sp. and polydnavirus in the indigenous stemborer parasitoid Cotesia sesamiae influences its biological control effectiveness against maize stemborers (Ngi-Song and Mochiah, 2001; Dupas et al., 2008). Wolbachia sp. also plays a critical role in determining the genetic structure of C. sesamiae in sub-Saharan Africa (Branca et al., 2019) and can influence the diversity of the host. Close association of species-specific gut microbiomes can be useful to differentiate between sibling species as reported with Ceratitis rosa s. s and C. quilici (Khamis et al., 2020).
Considering these critical roles played by insect symbionts on the host and natural enemy biology, in recent years, icipe embarked on understanding the diversity, roles and possible exploitation of insect symbionts in key plant pest and disease vectors. Diverse Wolbachia sp. infections in invasive populations of Bactrocera dorsalis in Africa has been observed, which were previously unreported in Asia (Gichuhi et al., 2019). Critical insect stage- and population-dependent differences in gut bacterial communities have also been reported in S. frugiperda in Kenya (Gichuhi et al., 2020). Analysis of blood meals from wildlife in Masai Mara, Kenya has revealed the prevalence of the insect symbionts Sodalis glossinidius and Coxiella sp. in tsetse flies and ticks, respectively (Makhulu et al., 2020; Oundo et al., 2020). Distinct strains of endosymbiotic Spiroplasma sp. has been discovered in Anopheles arabiensis (Chepkemoi, 2016) and A. gambiae (Chepkemoi et al., 2017), major vectors of Plasmodium sp. in Africa.
Insect symbionts are known to influence the ability of vectors to transmit both human and animal diseases. Oundo et al. (2020) observed that Sodalis sp. endosymbionts can be associated with increased trypanosome infection rates in endemic ecologies. Sodalis glossinidius is known to influence susceptibility of Glossina sp. to trypanosomes of both humans and animals. These relationships can be utilized to develop novel vector and vector-borne disease management strategies, where symbionts can be disseminated into vector populations to limit their capacity to transmit human and animal disease. A possible malaria control approach involves the dissemination in mosquitoes of inherited symbiotic microbes to block Plasmodium sp. transmission. Recently, icipe has demonstrated that the vertically transmitted microsporidian symbiont Microsporidia MB in A. arabiensis can impair Plasmodium sp. transmission. As a microbe that is non-virulent and vertically transmitted, Microsporidia MB could be investigated as a strategy to limit malaria transmission (Herren et al., 2020).
R4D Toward Plant Endophytes and Rhizosphere Inhabitants
Systemic induced resistance is the elicitation of innate plant defense mechanisms through abiotic and biotic elicitors, including endophytes. Endophytes are promising candidates for biological control products: they reside within plants where they can tackle cryptic pests and are protected from adverse weather conditions, and allow for targeted application, requiring lower doses (Akello et al., 2007). Systemic induced resistance provides a new avenue of pest control, especially for cryptic pests such as nematodes and plant diseases (Bamisope et al., 2018a,b). We demonstrated the effectiveness of H. lixii strain F3ST1, T. harzianum strain ICIPE 709, C. rosea strain ICIPE 707 and T. atroviride strain ICIPE 710 against T. tabaci through systemic induced resistance after they were inoculated as endophytes in onion (Muvea et al., 2014, 2015). Hypocrea lixii strain F3ST1 was also effective in controlling Liriomyza sp. as an endophyte in beans in the laboratory and field (Akutse et al., 2013; Gathage et al., 2016), and T. asperellum strain M2RT4, H. lixii strain F3ST1, and B. bassiana strains ICIPE273 and G1LU3 controlled O. phaseoli in beans after seed inoculation (Mutune et al., 2016). Hypocrea lixii strain F3ST1 and T. asperellum strain M2RT4 reduce Meloidogyne sp. and Globodera sp. nematodes through systemic induced resistance (Kiriga et al., 2018). Interestingly, we found that H. lixii strain F3ST1 is effective against insect-vectored viral diseases (IYSV and SCMV) through systemic induced resistance, which may offer a new avenue of research. Virus infection and transmission was blocked in onion (Muvea et al., 2018) and secondary metabolites in such endophyte-thrips-virus mediated interactions are being elucidated.
Develop High-Throughput Screening and Bioassays for Rapid Selection of Potent Biopesticide Strains
Better understanding of the virulence factors of entomopathogens and identification of corresponding genes can provide gene targets to develop high-throughput screening procedures of icipe's entomopathogen repository, leading to more rapid and efficient selection of the optimal biopesticide strains. Several factors beyond the efficacy of entomopathogens determine success toward their development into biopesticide products, including environmental suitability, and amenability for mass production and horizontal transmission, among others. Current R4D efforts are already aimed at using genotyping to improve biopesticide development, such as studies toward M. anisopliae chitinase genes and virulence factors (Niassy et al., 2013). We found that the chitinase genes chi2 and chi4 of eight M. anisopliae strains (ICIPE 7, 20, 30, 41, 62, 63, 69 and 78) did not reveal major divergences as the predicted protein structure of chi2 was identical for all the selected strains. Despite the critical role of chitin digestion in fungal infection, chi2 and chi4 genes cannot serve as molecular markers to characterize observed variations in virulence among M. anisopliae strains. Further studies are warranted to explore the processes controlling upregulation of chitinase expression that could be responsible for different virulence characteristics. Using comparative in vitro chitin digestion techniques would be more appropriate to compare the quality and quantity of chitinase production among fungal strains.
Broaden Biopesticide Arsenal Toward Bacterial, and Nematode- and Virus-Based Biopesticides
Most emphasis of icipe's R4D has been placed on development of entomopathogenic fungi into biopesticide products. However, based on the nature of the target insects (e.g., virus-based biopesticides for lepidopteran pests) and their occurrence in specific microcosms (e.g., entomopathogenic nematodes for targeting life stages in the soil), we will broaden our arsenal of candidate entomopathogens. Already, icipe has proactively tested 19 Bt strains against second-instar larvae of S. frugiperda with the goal to develop Bt-based biopesticides from Africa (Cruz et al., 2018). Seven Bt strains were found highly effective, causing 100% mortality seven days post-treatment, with LT50 values ranging between 2.3 and 6.5 days. Further biological and molecular characterization of these strains are currently ongoing. We plan to mass-produce Bt-based biopesticides using liquid, semi-solid or solid-state fermentation for large-field testing (Fontana-Capalbo et al., 2001).
Research into Pathogens of Edible Insects
R4D into insects for food and feed is of growing interest and a new foray in which icipe has taken a lead for sub-Saharan Africa. Globally, >2,000 insect species form part of the traditional diets of at least 2 billion people. However, most edible insects are harvested from the wild, which may result in food safety issues for consumers. Ssepuuya et al. (2019) found high counts of Actinobacteria, Bacteroidetes, Firmicutes, Fusobacteria and Proteobacteria, making R. differens a potential source of food borne diseases. Hazard and critical control points need to be identified along the food supply chain to assess and mitigate microbiological risk, and taken into account for food safety frameworks.
Insects are also a cost-effective alternative protein source to substitute or replace expensive fish or soya bean meal in livestock and aquaculture feed. The black soldier fly Hermetia illucens, an organic waste decomposer, is the most researched candidate for feed with well-established mass-rearing protocols (Barragan-Fonseca et al., 2017). However, the wide range of organic waste substrate used for H. illucens rearing may also offer an excellent breeding ground for microbial communities that are horizontally transmitted, thereby negatively impacting food safety of larvae or larval meal. Our studies showed that Enterobactereaceae were the most abundant bacterial family among H. illucens larvae, followed by Dysgonomonadaceae, Wohlfahrtiimonadaceae and Enterococcaceae. Some members of the Enterobacteriaceae are significant human, animal and plant pathogens causing a range of infections. Therefore, food safety is an important aspect for the introduction of H. illucens and other insects as high-quality protein ingredients in feed. The choice of rearing substrate is crucial, coupled with pre-treatment or sterilization of organic waste substrates before usage as well as postharvest treatments of H. illucens larvae (Khamis et al., 2019).
Besides those harmful to the animal or human consuming the insect, a second category of microbes are those harmful to the insect itself. Only anecdotal evidence exists of entomopathogens infecting H. illucens or other insects during rearing. However, as the insect for food and feed industry takes hold, it is to be expected that entomopathogens will become a problem of large-scale rearing facilities. Key entomopathogens of reared insects need to be identified as they become more important, and controlled through high hygiene standards during rearing.
Conclusion
Research outcomes from icipe R4D on biopesticides have significantly contributed to control of arthropod pests and vectors that constrain the livelihood opportunities of people in Africa. Through public-private partnerships, icipe has developed and commercialized some biopesticides to tackle pests and vectors on crop and livestock systems that contributes to reduction in synthetic pesticides use and positively impact on animal, human and environmental health. Together with its partners, icipe continues to have a strong biopesticide research program built on solid research protocols to yield new bioproducts and based on its large repository and diverse strains of pathogens. icipe's future R4D thrusts include (1) developing high-throughput screening and bioassays models for rapid selection of potent biopesticide strains; (2) expanding public-private partnerships and develop biopesticides targeting emerging and invasive pests such as S. frugiperda and S gregaria; (3) expanding the portfolio of research on animal and human disease vectors, and broaden biopesticide arsenal toward bacterial, entomopathogenic nematode-, endophytes and virus-based biopesticides; (4) harnessing the benefit of IPM and pollination services; (4) build youth and women entrepreneurship for biopesticide production; and (5) assessing socioeconomic impacts of biopesticide use and inform policy makers for regional harmonization of policies enabling biopesticide registration and use. In sub-Saharan Africa, tackling current and emerging pests and disease vectors of plants, animals and humans will become more important due to agricultural intensification and climate change. Biopesticides provide a suitable alternative to chemical control and continued R4D efforts are required to yield solutions, especially for smallholder farmers.
Author Contributions
KA, SS, TD, and SE designed the review outline. The manuscript was written by KA, TD, and SS. KA, SS, TD, NM, and SE reviewed the manuscript. All authors listed have made a substantial, direct and intellectual contribution to the work, and approved it for publication.
Funding
The authors gratefully acknowledged the financial support for this research by the following organizations and agencies: UK's Foreign, Commonwealth & Development Office (FCDO), the Swedish International Development Cooperation Agency (Sida), the Swiss Agency for Development and Cooperation (SDC), the Federal Democratic Republic of Ethiopia, and the Government of the Republic of Kenya. The views expressed herein do not necessarily reflect the official opinion of the donors.
Conflict of Interest
The authors declare that the research was conducted in the absence of any commercial or financial relationships that could be construed as a potential conflict of interest.
Acknowledgments
Contributions from Beatrice Muriithi in providing input in the manuscript are acknowledged.
References
Akello, J., Dubois, T., Gold, C. S., Nakavuma, J, and Paparu, P. (2007). Beauveria bassiana Balsamo (Vuillemin) as a potential endophyte in tissue culture banana (Musa spp.). J. Invertebr. Pathol. 96, 34–42. doi: 10.1016/j.jip.2007.02.004
Akutse, K. S., Fiaboe, K. K., Van den Berg, J., Ekesi, S., and Maniania, N. K. (2014). Effects of endophyte colonization of Vicia faba (Fabaceae) plants on the life-history of leafminer parasitoids Phaedrotoma scabriventris (Hymenoptera: Braconidae) and Diglyphus isaea (Hymenoptera: Eulophidae). PLoS ONE 9:e109965. doi: 10.1371/journal.pone.0109965
Akutse, K. S., Kimemia, J. W., Ekesi, S., Khamis, F. M., Ombura, O. L., and Subramanian, S. (2019a). Ovicidal effects of entomopathogenic fungal isolates on the invasive fall armyworm Spodoptera frugiperda (Lepidoptera: Noctuidae). J. Appl. Entomol. 143, 626–634. doi: 10.1111/jen.12634
Akutse, K. S., Maniania, N. K., Fiaboe, K. K. M., Van den Berg, J., and Ekesi, S. (2013). Endophytic colonization of Vicia faba and Phaseolus vulgaris (Fabaceae) by fungal pathogens and their effects on the life-history parameters of Liriomyza huidobrensis (Diptera: Agromyzidae). Fungal Ecol. 6, 293–301. doi: 10.1016/j.funeco.2013.01.003
Akutse, K. S, Khamis, F. M., Ekesi, S., Kimemia, J. W., Opisa, S., Murunde, R., et al. (2019b). “Pathogenicity of entomopathogenic fungal isolates against fall armyworm moths Spodoptera frugiperda (Lepidoptera: Noctuidae),” in African Association of Insects Scientists (AAIS 2019). AAIS 23rd Biannual Meeting and Scientific Conference, Abidjan, Ivory Coast, 18–23 November 2019 (Nairobi, Kenya: AAIS).
Bailey, A., Chandler, D., Grant, W. P., Greaves, J., Prince, G., and Tatchell, M. (2010). Biopesticides: Pest Management and Regulation (Wallingford, UK: CABI). doi: 10.1079/9781845935597.0000
Bamisope, S. B., Dash, C. K., Akutse, K. S., Hussain, M., Qasim, M., and Wang, L. (2018a). Prospects of establishing fungal entomopathogens as endophytes for bio-control: an insight on how artificial inoculation methods affect endophytic colonization of host plants. Microbiol. Res. 217, 34–50. doi: 10.1016/j.micres.2018.08.016
Bamisope, S. B., Dash, C. K., Akutse, K. S., Keppanan, R., and Wang, L. (2018b). Fungal endophytes: beyond herbivore management. Front. Microbiol. 9:544. doi: 10.3389/fmicb.2018.00544
Barragan-Fonseca, K. B., Dicke, M., and van Loon, J. J. A. (2017). Nutritional value of the black soldier fly (Hermetia illucens L.) and its suitability as animal feed – a review. J. Insects Food Feed 3, 105–120. doi: 10.3920/JIFF2016.0055
Bashir, M. O., and Hassanali, A. (2010). Novel cross-stage solitarising effect of gregarious-phase adult desert locust [Schistocerca gregaria (Forskål)] pheromone on hoppers. J. Insect Physiol. 56, 640–645. doi: 10.1016/j.jinsphys.2010.01.012
Bateman, R., Jenkins, N., Kooyman, C., and Moore, D. (2017). “Chapter 23 - LUBILOSA: the development of an acridid-specific mycoinsecticide,” in Microbial Control of Insect and Mite Pests From Theory to Practice, ed L. A. Lacey (Amsterdam, Netherlands: Elsevier), 343–353. doi: 10.1016/B978-0-12-803527-6.00023-8
Bauer, B., Amsler-Delafosse, S., Clausen, P. H., Kabore, I., and Petrich-Bauer, J. (1995). Successful application of deltamethrin pour onto cattle in a campaign against tsetse flies (Glossina spp.) in the pastoral zone of Samorogouan, Burkina-Faso. Trop. Med. Parasitol. 46, 183–189.
Branca, A., Le Ru, B., Calatayud, P. A., Obonyo, J., Musyoka, B., Capdevielle-Dulac, C., et al. (2019). Relative influence of host, Wolbachia, geography and climate on the genetic structure of the Sub-Saharan parasitic wasp Cotesia sesamiae. Front. Ecol. Evol. 7:309. doi: 10.3389/fevo.2019.00309
Chepkemoi, S. T., Mararo, E., Butungi, H., Paredes, J., Masiga, D., Sinkins, S. P., et al. (2017). Identification of Spiroplasma insolitum symbionts in Anopheles gambiae. Wellcome Open Res. 2:90. doi: 10.12688/wellcomeopenres.12468.1
Chepkemoi, T. S. (2016). Molecular identification and characterization of Spiroplama in Anopheles arabiensis from Kenya. (M.Sc. thesis). University of Nairobi, Nairobi, Kenya.
Cruz, I., Bruce, A., Subramanian, S., Akutse, K. S., Mohamed, F. S., Niassy, N., et al. (2018). “Biological control and biorational pesticides for fall armyworm management,” in Fall Armyworm in Africa: A Guide for Integrated Pest Management, eds B. M. Prasanna, J. E. Huesing, R. Eddy, V. M. Peschke (Texcoco: CYMMIT), 63–88.
Dalmas, C. A., and Koutroubas, S. D. (2018). Current status and recent developments in biopesticide use. Agriculture 8:13. doi: 10.3390/agriculture8010013
Dransfield, R. D., Brightwell, R., Kyorku, C., and Williams, B. (1990). Control of tsetse fly (Diptera: Glossinidae) populations using traps at Nguruman, south-west Kenya. Bull. Entomol. Res. 80, 265–176. doi: 10.1017/S000748530005046X
Dupas, S., Gitau, C. W., Branca, A., Le Rü, B. P., and Silvain, J. F. (2008). Evolution of a polydnavirus gene in relation to parasitoid–host species immune resistance. J. Hered. 99, 491–499. doi: 10.1093/jhered/esn047
Fontana-Capalbo, D. M., Valicente, F. H., Oliveira Moraes, I. D., and Pelizer, L. H. (2001). Solid-state fermentation of Bacillus thuringiensis tolworthi to control fall armyworm in maize. Electr. J. Biotechnol. 4, 9–10. doi: 10.2225/vol4-issue2-fulltext-5
Fourie, J, and Crafford, D. (2018). “The bed bug resurgence in Africa,” in Advances in the Biology and Management of Modern Bed Bugs, eds S. L. Doggett, D. M. Miller, and C.Y. Lee (Hoboken, USA: Wiley), 87–94. doi: 10.1002/9781119171539.ch8
Gallai, N., Salles, J. M., Settele, J., and Vaissière, B. E. (2009). Economic valuation of the vulnerability of world agriculture confronted with pollinator decline. Ecol. Econom. 68, 810–821. doi: 10.1016/j.ecolecon.2008.06.014
Gathage, J. W., Lagat, Z. O., Fiaboe, K. K. M., Akutse, K. S., Ekesi, S., and Maniania, N. K. (2016). Prospects of fungal endophytes in the control of Liriomyza leafminer flies in common bean Phaseolus vulgaris under field conditions. Biocontrol 61, 741–753. doi: 10.1007/s10526-016-9761-0
Gichuhi, J., Subramanian, S., Khamis, F., Van den Berg, J., Du Plessis, H., Ekesi, S., et al. (2020). Diversity of fall armyworm, Spodoptera frugiperda and their gut bacterial community in Kenya. PeerJ 8:e8701. doi: 10.7717/peerj.8701
Gichuhi, J, Khamis, F. M., Van den Berg, J., Ekesi, S., and Herren, J. K. (2019). Unexpected diversity of Wolbachia associated with Bactrocera dorsalis (Diptera: Tephritidae) in Africa. Insects 10:155. doi: 10.3390/insects10060155
Gouteux, J. P., and Lancien, J. (1986). Le piège pyramidal à tsetse (Diptera, Glossinidae) pour la capture et la lutte. Essais comparatifs et description de nouveaux systèmes de capture. Trop. Med. Parasitol. 37, 61–66.
Gries, R., Britton, R., Holmes, M., Zhai, H., Draper, J., and Gries, G. (2015). Bed bug aggregation pheromone finally identified. Angew. Chem. Int. Ed Engl. 127, 1151–1154. doi: 10.1002/ange.201409890
Grzywacz, D., Cherry, A., and Maritime, C. (2019). Biological pesticides for Africa: why has so little research led to new products to help africa's poor? Outlooks Pest Manag. 2009, 1–5. doi: 10.1564/20feb00
Hatting, J. L., Moore, S. D., and Maland, A. P. (2019). Microbial control of phytophagous invertebrate pests in South Africa: current status and future prospects. J. Invertebr. Pathol. 165, 54–66. doi: 10.1016/j.jip.2018.02.004
Herren, J. K., Mbaisi, L. M., Mararo, E., Makhulu, E. E., Mobegi, V. A., Butungi, H., et al. (2020). A microsporidian impairs Plasmodium falciparum transmission in Anopheles arabiensis mosquitoes. Nat. Commun. 11:2187. doi: 10.1038/s41467-020-16121-y
Howard, A. F., Koenraadt, C. J., Farenhorst, M., Knols, B. G., and Takken, W. (2010). Pyrethroid resistance in Anopheles gambiae leads to increased susceptibility to the entomopathogenic fungi Metarhizium anisopliae and Beauveria bassiana. Malar. J. 9:168. doi: 10.1186/1475-2875-9-168
ICIPE (2012). External Evaluation of the Biovision – ICIPE Malaria IVM Projects in Kenya and Ethiopia (Nairobi: icipe).
Khamis, F. M., Mireji, P. O., Ombura, F. L., Malacrida, A. R., Awuoche, E. O., Rono, M., et al. (2019). Species-specific transcriptional profiles of the gut and gut microbiome of Ceratitis quilicii and Ceratitis rosa sensu stricto. Sci. Rep. 9:18355. doi: 10.1038/s41598-019-54989-z
Khamis, F. M., Ombura, F. L. O., Akutse, K. S., Subramanian, S., Mohamed, S. A., Fiaboe, K. K. M., et al. (2020). Insights in the global genetics and gut microbiome of black soldier fly, Hermetia illucens: implications for animal feed safety control. Front. Microbiol. 11:1538. doi: 10.3389/fmicb.2020.01538
Kibira, M., Affognon, H., Njehia, B., Muriithi, B., and Ekesi, S. (2015). Economic evaluation of integrated management of fruit fly in mango production in Embu County, Kenya. Afr. J. Agric. Res. 10, 343–335.
Kiriga, A. W., Haukeland, S., Kariuki, G. M., Coyne, D. L., and Beek, N. V. (2018). Effect of Trichoderma spp. and Purpureocillium lilacinum on Meloidogyne javanica in commercial pineapple production in Kenya. Biol. Control 119, 27–32. doi: 10.1016/j.biocontrol.2018.01.005
Leak, S. G. A., Mulatu, W., Rowlands, G. J., and D'Ieteren, G. D. M. (1995). A trial of a cypermethrin 'pour-on' insecticide to control Glossina pallipes, G. fuscipes and G. morsitans submorsitans (Diptera: Glossinidae) in south-west Ethiopia. Bull. Entomol. Res. 85, 241–251. doi: 10.1017/S0007485300034325
Makhulu, E. E., Villinger, J., Adunga, V. O., Jeneby, M. M., Kimathi, E. M., Mararo, E., et al. (2020). Tsetse blood-meal sources, endosymbionts, and trypanosome infections provide insight into African trypanosomiasis transmission in the Maasai Mara national reserve, a wildlife-human-livestock interface. bioRxiv [Preprint]. doi: 10.1101/2020.04.06.027367
Maniania, N. K. (1992). Pathogenicity of entomogenous fungi (Hyphomycetes) to larvae of the stem borers, Chilo partellus Swinhoe and Busseola fusca Fuller. Int. J. Trop. Insect Sci. 13, 691–696. doi: 10.1017/S1742758400007918
Maniania, N. K. (1993). Evaluation of three formulations of Beauveria bassiana (Bals.) Vuill. for control of the stem borer Chilo partellus (Swinhoe) (Lep. Pyralidae). J. Appl. Entomol. 115, 266–272. doi: 10.1111/j.1439-0418.1993.tb00389.x
Maniania, N. K. (1998). A device for infecting adult tsetse flies, Glossina spp., with an entomopathogenic fungus in the field. Biol. Control 11, 248–254. doi: 10.1006/bcon.1997.0580
Maniania, N. K. (2002). A low-cost contamination device for infecting adult tsetse flies, Glossina spp., with the entomopathogenic fungus Metarhizium anisopliae in the field. Biocontrol Sci. Technol. 12, 59–66. doi: 10.1080/09583150120110662
Maniania, N. K., Ekesi, S., Odulaja, A., Okech, M. A., and Nadel, D. J. (2006). Prospects of a fungus-contamination device for the control of tsetse fly Glossina fuscipes. Biocontrol Sci. Technol. 16, 129–139. doi: 10.1080/09583150500258503
Maniania, N. K., Okech, M. A., Adino, J. O., Opere, J. O., and Ekesi, S. (2013). Transfer of inoculum of Metarhizium anisopliae between adult Glossina morsitans morsitans and effects of fungal infection on blood feeding and mating behaviors. J. Pest Sci. 86, 285–292. doi: 10.1007/s10340-012-0473-7
Marrone, P. G. (2014). “The market and potential for biopesticides,” in Biopesticides: State of the Art and Future Opportunities, eds A. D. Gross, J. R. Coats, S. O. Duke, J. N. Seiber (Washington DC, USA: American Chemical Society), 245–258.
Mburu, D. M., Maniania, N. K., and Hassanali, A. (2013). Comparison of volatile blends and nucleotide sequences of two Beauveria bassiana isolates of different virulence and repellency towards the termite Macrotermes michealseni. J. Chem. Ecol. 39, 101–108. doi: 10.1007/s10886-012-0207-6
Mburu, D. M., Ochola, L., Maniania, N. K., Njagi, P. G., Gitonga, L. M., Ndung'u, M. W., et al. (2009). Relationship between virulence and repellency of entomopathogenic isolates of Metarhizium anisopliae and Beauveria bassiana to the termite Macrotermes michaelseni. J. Insect Physiol. 55, 774–780. doi: 10.1016/j.jinsphys.2009.04.015
Midingoyi, S. G., Kassie, M., Muriithi, B., Diiro, G., and Ekesi, S. (2019). Do farmers and the environment benefit from adopting integrated pest management practices? evidence from Kenya. J. Agric. Econom. 70, 452–470. doi: 10.1111/1477-9552.12306
Migiro, L. N., Maniania, N. K., Chabi-Olaye, A., and Vandenberg, J. (2010). Pathogenicity of entomopathogenic fungi Metarhizium anisopliae and Beauveria bassiana (Hypocreales: Clavicipitaceae) isolates to the adult pea leafminer (Diptera: Agromyzidae) and prospects of an autoinoculation device for infection in the field. Environ. Entomol. 39, 468–475. doi: 10.1603/EN09359
Mkiga, A. M., Mohamed, S. A., Du Plessis, H., Khamis, F. M., Akutse, K. S., and Ekesi, S. (2020). Metarhizium anisopliae and Beauveria bassiana: pathogenicity, horizontal transmission, and their effects on reproductive potential of Thaumatotibia leucotreta (Lepidoptera: Tortricidae). J. Econ. Entomol. 113, 660–668. doi: 10.1093/jee/toz342
Mnyone, L. L., Kirby, M. J., Mpingwa, M. W., Lwetoijera, D. W., Knols, B. G., Takken, W., et al. (2011). Infection of Anopheles gambiae mosquitoes with entomopathogenic fungi: effect of host age and blood-feeding status. Parasitol. Res. 108, 317–322. doi: 10.1007/s00436-010-2064-y
Mnyone, L. L., Koenraadt, C. J., Lyimo, I. N., Mpingwa, M. W., Takken, W., and Russell, T. L. (2010). Anopheline and culicine mosquitoes are not repelled by surfaces treated with the entomopathogenic fungi Metarhizium anisopliae and Beauveria bassiana. Parasit. Vectors 3:80. doi: 10.1186/1756-3305-3-80
Mohamed, S., Akutse, K. S., Khamis, F., Jehle, J., Tiba, S. B., Kroschel, J., et al. (2017). “Susceptibility of the tomato leafminer, Tuta absoluta (Lepidoptera: Gelechiidae), to some isolates of Metarhizium anisopliae and Phthorimaea operculella granulovirus (Phopgv) isolates,” in Proceeding of the African Association of Insects Scientists. AAIS 22nd Biannual Meeting and Scientific Conference, Wad Medani, Sudan (Nairobi: AAIS).
Murigu, M. M., Nana, P., Waruiru, R. M., Nga'nga, C. J., Ekesi, S., and Maniania, N. K. (2016). Laboratory and field evaluation of entomopathogenic fungi for the control of amitraz-resistant and susceptible strains of Rhipicephalus decoloratus. Vet. Parasitol. 225, 12–18. doi: 10.1016/j.vetpar.2016.05.026
Muriithi, B. W., Affognon, H. D., Diiro, G. M., Kingori, S. W., Tanga, C. M., Nderitu, P. W., et al. (2016). Impact assessment of integrated pest management (IPM) strategy for suppression of mango-infesting fruit flies in Kenya. Crop Protect. 81, 20–29. doi: 10.1016/j.cropro.2015.11.014
Mutune, B., Ekesi, S., Niassy, S., Matiru, V., Bii, C., and Maniania, N. K. (2016). Fungal endophytes as promising tools for the management of bean stem maggot Ophiomyia phaseoli on beans Phaseolus vulgaris. J. Pest Sci. 89, 993–1001. doi: 10.1007/s10340-015-0725-4
Muvea, A. M., Meyhöfer, R., Maniania, N. K., Poehling, H. M., Ekesi, S., and Subramanian, S. (2015). Behavioral responses of Thrips tabaci Lindeman to endophyte-inoculated onion plants. J. Pest Sci. 88, 555–562. doi: 10.1007/s10340-015-0645-3
Muvea, A. M., Meyhöfer, R., Subramanian, S., Poehling, H. M., Ekesi, S., and Maniania, N. K. (2014). Colonization of onions by endophytic fungi and their impacts on the biology of Thrips tabaci. PLoS ONE 9:e108242. doi: 10.1371/journal.pone.0108242
Muvea, A. M., Subramanian, S., Maniania, N. K., Poehling, H. M., Ekesi, S., and Meyhoefer, R. (2018). Endophytic colonization of onions induces resistance against viruliferous thrips and virus replication. Front. Plant Sci. 9:1785. doi: 10.3389/fpls.2018.01785
Mwungu, C. M., Muriithi, B., Ngeno, V., Affognon, H., Githiomi, C., Diiro, G., et al. (2020). Health and environmental effects of adopting an integrated fruit fly management strategy among mango farmers in Kenya. Afr. J. Agri. Resour. Econom. 15, 14–26.
Nana, P., Ekesi, S., Nchu, F., and Maniania, N. K. (2016). Compatibility of Metarhizium anisopliae with Calpurnia aurea leaf extracts and virulence against Rhipicephalus pulchellus. J. Appl. Entomol. 140, 590–597. doi: 10.1111/jen.12289
Nana, P., Maniania, N. K., Maranga, R. O., Boga, H. I., Kutima, H. L., and Eloff, J. N. (2012). Compatibility between Calpurnia aurea leaf extract, attraction aggregation, and attachment pheromone and entomopathogenic fungus Metarhizium anisopliae on viability, growth, and virulence of the pathogen. J. Pest Sci. 85, 109–115. doi: 10.1007/s10340-011-0399-5
Nchu, F., Maniania, N. K., Hassanali, A., and Eloff, J. N. (2010a). Performance of a Metarhizium anisopliae-treated semiochemical-baited trap in reducing Amblyomma variegatum populations in the field. Vet. Parasitol. 169, 367–372. doi: 10.1016/j.vetpar.2010.01.020
Nchu, F., Maniania, N. K., Hassanali, A., and Eloff, J. N. (2010b). Optimizing modes of inoculation of Rhipicephalus ticks (Acari:Ixodidae) with a mitosporic entomopathogenic fungus in the laboratory. Exp. Appl. Acarol. 51, 373–382. doi: 10.1007/s10493-009-9330-7
Nchu, F., Maniania, N. K., Toure, A., Hassanali, A., and Eloff, J. N. (2009). The use of a semiochemical bait to enhance exposure of Amblyomma variegatum (Acari:Ixodidae) to Metarhizium anisopliae (Ascomycota: Hypocreales). Vet. Parasitol. 160, 279–284. doi: 10.1016/j.vetpar.2008.11.005
Ngi-Song, A. J., and Mochiah, M. B. (2001). Polymorphism for Wolbachia infections in eastern and southern African Cotesia sesamiae (Cameron) (Hymenoptera: Braconidae) populations. Int. J. Trop. Insect Sci. 21, 369–374. doi: 10.1017/S174275840000847X
Ngumbi, P. M., Irungu, L. W., Ndegwa, P. N., and Maniania, N. K. (2011). Pathogenicity of Metarhizium anisopliae (Metch) Sorok and Beauveria bassiana (Bals) Vuill to adult Phlebotomus duboscqi (Neveu-Lemaire) in the laboratory. J. Vector Borne Dis. 48, 37–40. doi: 10.1016/j.jip.2006.08.002
Ngure, P. K., Kasili, S., Anjili, C. O., Karanja, R. M., Kaburi, J., Mwangi, M., et al. (2015). Effects of Metarhizium anisopliae on sand fly populations in their natural habitats in Kenya. Afr. J. Health Sci. 29, 398–407. doi: 10.13140/RG.2.1.2469.0006
Niassy, S., Subramanian, S., Ekesi, S., Bargul, J. L., Villinger, J., and Maniania, N. K. (2013). Use of Metarhizium anisopliae chitinase genes for genotyping and virulence characterization. Biomed Res. Int. 2013:9. doi: 10.1155/2013/465213
Nyangau, P., Muriithi, B., Nzuma, J., Irungu, P., Gichungi, H., and Diiro, G. (2020). Impact of integrated fruit fly management strategy on food security among smallholder mango farmers in Kenya. Afr. J. Food Agric. Nutr. Dev. 20, 15431–15454. doi: 10.18697/ajfand.90.18455
Oladunmade, M. A., Feldmann, U., Takken, W., Tenabe, S. O., Hamann, H. J., Onah, J., et al. (1990). “Eradication of Glossina palpalis palpalis (Robineau Devoidsady) (Diptera: Glossinidae) from agropastoral land in central Nigeria by means of the sterile insect technique,” in Sterile Insect Technique for Tsetse Control and Eradication. Proceedings of the Final Research Coordination Meeting Held in Vom, Plateau State. (Vienna: International Atomic Energy Agency).
Olson, S. (2015). An analysis of the biopesticide market now and where is going. Outlook. Pest Manag. 26, 203–206. doi: 10.1564/v26_oct_04
Opisa, S., Du Plessis, H., Akutse, K. S., Fiaboe, K. K. M., and Ekesi, S. (2018). Effects of entomopathogenic fungi and Bacillus thuringiensis-based biopesticides on Spoladea recurvalis (Lepidoptera: Crambidae). J. Appl. Entomol. 142, 617–626. doi: 10.1111/jen.12512
Opisa, S., Du Plessis, H., Akutse, K. S., Fiaboe, K. K., and Ekesi, S. (2019). Horizontal transmission of Metarhizium anisopliae between Spoladea recurvalis (Lepidoptera: Crambidae) adults and compatibility of the fungus with the attractant phenylacetaldehyde. Microbial. Pathogenesis 131, 197–204. doi: 10.1016/j.micpath.2019.04.010
Oundo, J. W., Villinger, J., Jeneby, M., Ong'amo, G., Otiende, M. Y., Makhulu, E. E., et al. (2020). Pathogens, endosymbionts, and blood-meal sources of hostseeking ticks in the fast-changing Maasai Mara wildlife ecosystem. PLoS ONE 15:e0228366. doi: 10.1371/journal.pone.0228366
Politzar, H., and Cuisance, D. (1982). “SIT in the control and eradication of Glossina palpalis gambiensis,” in IAEA Proceedings of a Conference on Sterile Male Insect Release for Insect Pest Control, (Vienna: International Atomic Energy Agency), 101–109.
Saini, R. K., Orindi, B. O., Mbahin, N., Andoke, J. A., Muasa, P. N., Mbuvi, D. M., et al. (2017). Protecting cows in small holder farms in East Africa from tsetse flies by mimicking the odor profile of a non-host bovid. PLoS Negl. Trop. Dis. 11:e0005977. doi: 10.1371/journal.pntd.0005977
Ssepuuya, G., Wynants, E., Verreth, C., Crauwels, S., Lievens, B., Claes, J., et al. (2019). Microbial characterisation of the edible grasshopper Ruspolia differens in raw condition after wild-harvesting in Uganda. Food Microbiol. 77, 106–117. doi: 10.1016/j.fm.2018.09.005
Takken, W., Oladunmade, M. A., Dengwat, L., Feldmann, H. U., Onah, J., Tenabe, S. O., et al. (1986). The eradication of Glossina palpalis (Robineau- Desvoidy) (Diptera: Glossinidae) using traps, insecticide-impregnated targets and the sterile insect technique in central Nigeria. Bull. Entomol. Res. 76, 275–286. doi: 10.1017/S0007485300014747
Torto, B., Obeng-Ofori, D., Njagi, P. G. N., Hassanali, A., and Amiani, H. (1994). Aggregation pheromone system of adult gregarious desert locust Schistocerca gregaria (Forskål). J. Chem. Ecol. 20, 1749–1762. doi: 10.1007/BF02059896
Tumuhaise, V., Ekesi, S., Maniania, N. K., Tonnang, H. E. Z., Tanga, C. M., Ndegwa, P. N., et al. (2018). Temperature-dependent growth and virulence, and mass production potential of two candidate isolates of Metarhizium anisopliae (Metschnikoff) Sorokin for managing Maruca vitrata Fabricius (Lepidoptera: Crambidae) on cowpea. Afr. Entomol. 26, 73–84. doi: 10.4001/003.026.0073
Tumuhaise, V., Ekesi, S., Mohamed, S. A., Ndegwa, P. N., Irungu, L. W., Srinivasan, R., et al. (2015). Pathogenicity and performance of two candidate isolates of Metarhizium anisopliae and Beauveria bassiana (Hypocreales: Clavicipitaceae) in four liquid culture media for the management of the legume pod borer Maruca vitrata (Lepidoptera: Crambidae). Int. J. Trop. Insect Sci. 35, 34–47. doi: 10.1017/S1742758414000605
Wamiti, L. G., Khamis, F. M., Abd-alla, A. M. M., Ombura, F. L. O., Akutse, K. S., Subramanian, S., et al. (2018). Metarhizium anisopliae infection reduces Trypanosoma congolense reproduction in Glossina fuscipes fuscipes and its ability to acquire or transmit the parasite. BMC Microbiol. 18:142. doi: 10.1186/s12866-018-1277-6
WHO (2020). Vector-borne diseases. Geneva, Switzerland: World Health Organization. Available online at: https://www.who.int/news-room/fact-sheets/detail/vector-borne-diseases (accessed July 27, 2020).
Keywords: Africa, biological control, entomopathogenic fungus, Metarhizium anisopliae, public-private partnership
Citation: Akutse KS, Subramanian S, Maniania NK, Dubois T and Ekesi S (2020) Biopesticide Research and Product Development in Africa for Sustainable Agriculture and Food Security – Experiences From the International Centre of Insect Physiology and Ecology (icipe). Front. Sustain. Food Syst. 4:563016. doi: 10.3389/fsufs.2020.563016
Received: 17 May 2020; Accepted: 17 August 2020;
Published: 30 September 2020.
Edited by:
Surendra Dara, University of California System, United StatesReviewed by:
Tahira Fatima, Purdue University, United StatesMelissa Jean O'Neal, Marrone Bio Innovations, United States
Copyright © 2020 Akutse, Subramanian, Maniania, Dubois and Ekesi. This is an open-access article distributed under the terms of the Creative Commons Attribution License (CC BY). The use, distribution or reproduction in other forums is permitted, provided the original author(s) and the copyright owner(s) are credited and that the original publication in this journal is cited, in accordance with accepted academic practice. No use, distribution or reproduction is permitted which does not comply with these terms.
*Correspondence: Thomas Dubois, dGR1Ym9pc0BpY2lwZS5vcmc=